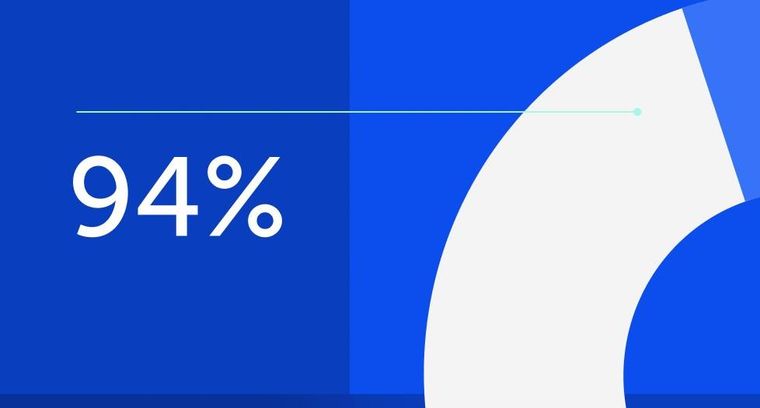
94% of researchers rate our articles as excellent or good
Learn more about the work of our research integrity team to safeguard the quality of each article we publish.
Find out more
REVIEW article
Front. Physiol., 08 August 2022
Sec. Exercise Physiology
Volume 13 - 2022 | https://doi.org/10.3389/fphys.2022.903713
This article is part of the Research TopicExercise Physiology and its Role in Chronic Disease Prevention and Treatment - Mechanisms and InsightsView all 17 articles
Numerous studies demonstrated the strong link between dyslipidemia and the cardiovascular risk. Physical activity and exercise represent effective prevention and therapy strategies for dyslipidemia and at the same time counteract numerous comorbidities that often accompany the disease. The physiological mechanisms are manifold, and primary mechanisms might be an increased energy consumption and associated adaptations of the substrate metabolism. Recent studies showed that there are bidirectional interactions between dyslipidemia and the immune system. Thus, abnormal blood lipids may favor pro-inflammatory processes, and at the same time inflammatory processes may also promote dyslipidemia. Physical activity has been shown to affect numerous immunological processes and has primarily anti-inflammatory effects. These are manifested by altered leukocyte subtypes, cytokine patterns, stress protein expression, and by reducing hallmarks of immunosenescence. The aim of this review is to describe the effects of exercise on the treatment dyslipidemia and to discuss possible immunological mechanisms against the background of the current literature.
One of the key risk factors for the development of cardiovascular disease is hyper- and dyslipidemia. Both terms denote lipid metabolism disorders which are not quite uniformly defined, as in some cases the transported blood lipids, individual lipoprotein fractions or a combination of blood lipids and lipoproteins are used for clinical diagnosis. Hyperlipidemia mainly describes hypercholesterolemia (cholesterol >200 mg/dl or 5.2 mmol/L), hypertriglyceridemia (elevated triglycerides >150 mg/dl or 1.7 mmol/L) and combined hyperlipidemia (elevated cholesterol and elevated triglycerides). Altered lipoproteins mean hyperlipoproteinemia (mostly increased LDL), hypolipoproteinemia (mostly decreased HDL), and dyslipoproteinemia (high LDL and low HDL levels). Hyperlipidemia is suggested to be a major risk factor for the development of atherosclerosis. In particular, an increase in LDL is associated with an increased risk of cardiovascular disease, including coronary heart disease (CHD) and stroke (Pressler, 2017).
Cholesterol-rich LDL and other apolipoprotein B (ApoB)-containing lipoproteins, including very low-density lipoproteins (VLDL), intermediate density lipoproteins (IDL) and lipoprotein(a), m[Lp(a)], have a direct impact on the development of atherosclerosis and its cardiovascular consequences. The increased concentrations of these lipid fractions in the blood lead to functional changes in endothelial barrier function because lipids travel freely between the vessel lumen and the vessel wall. Hence, LDL and other ApoB-containing lipoproteins enter and leave the arterial intima especially at sites vulnerable for plaque formation. This subsequently leads to a retention and accumulation of cholesterol-rich lipoproteins within the intima. It is suggested that the higher the LDL-C concentrations, the higher the probability of retention, what creates the basis for the development of an arteriosclerotic plaques (Goldstein and Brown, 2015). In endothelial cells, enzymatic systems are activated, which induce an overproduction of reactive oxygen species (ROS). The increased oxidative stress in turn exhibits pro-atherogenic effects and induces pro-inflammatory signal cascades. The activated endothelium starts to express various inflammatory cytokines, chemokines and to express adhesion molecules, causing leukocytes adherence to the vascular endothelium. Depending on the degree of activation, immune cells transmigrate and infiltrate the vessel wall. Increased ROS formation and inflammation inhibits the production and bioavailability of nitric oxide (NO), which further exacerbates endothelial dysfunction (van Diepen et al., 2013).
Migrating monocytes play a special role in these processes, because these cells differentiate into macrophages and transform into foam cells after taking up modified LDL via scavenger receptors (Sorci-Thomas and Thomas, 2016). Macrophage foam cells (MFCs) play a crucial role in the initiation and progression of atherosclerosis. At the same time, LDL cholesterol directly contributes to activation of the NLRP3 inflammasome, a cytosolic multiprotein complex of the innate immune system, which enhances the endothelial inflammatory response (Grebe and Latz, 2013). The multiple local inflammatory activities lead to an increased systemic production of C-reactive protein (CRP), tumour necrosis factor-alpha (TNF-α), interleukin (IL)-6 and IL-1, which are also thought to play a causal role in the development and progression of atherosclerosis. Conversely, systemic inflammatory processes, which are promoted by obesity, nicotine abuse, psychological stress or various autoimmune diseases, can promote local endothelial dysfunctions (Alack et al., 2019).
The subclinical inflammatory process is bi-directionally linked to lipid metabolism. On the one hand, inflammatory activities lead to increased cholesterol reverse transport and thus to increased formation of VLDLs (Khovidhunkit et al., 2004). On the other hand, circulating LDL has an increased tendency to oxidize, which explains the increased plasma levels of oxidized LDL (oxLDL) in patients with chronic inflammatory diseases (García-Gómez et al., 2014). OxLDL has a strong atherogenic effect, can promote inflammatory processes at the endothelium and even have a toxic effect on endothelial cells (Roma et al., 1992). Conversely, many immune cells interact with different classes of lipids and thus control their differentiation. In particular, the development of inflammatory leukocyte subtypes is favored (Hubler and Kennedy, 2016).
Physiological aging is frequently accompanied by a progressive increase in the concentration of circulating inflammatory cytokines. In particular, T cell senescence appears to have a bidirectional relationship with the development of chronic low-grade inflammation (Franceschi, 2007). On the one hand, chronic inflammation is a driver of cellular senescence by constantly activating immune cells. On the other hand, senescent cell types often present a more pro-inflammatory phenotype and thus become a potent source for the secretion of pro-inflammatory cytokines (Davalos et al., 2010).
While the described immune aging processes are part of the physiological remodeling of the immune system in old age, they are accelerated by lifestyle factors, such as overnutrition, inactivity and the resulting overweight. Dyslipidemia may also accelerate the immune aging process. Data suggests that the metabolism of immune cells, especially T cells, is influenced by the altered lipid concentration in the environment. In particular, cholesterol metabolism of T cells is disturbed which was demonstrated to inhibit their proliferation and favor the development of pro-inflammatory phenotypes (Boßlau et al., 2021; Kim et al., 2021). Progressive “inflammaging”, which is bi-directionally accelerated by lipid changes, leads to a vicious circle of dyslipidemia and maladaptive immune-aging. Inflammatory signaling pathways are continuously activated and initiate atypical cytotoxic activity toward endogenous structures such as the endothelium, which may ultimately favor the development of cardiovascular diseases (CVDs) (Nakajima et al., 2002).
Typical inflammatory markers, produced by many types of senescent cell in atherogenesis, are IL-1a, IL-1β, IL- 6, IL-8, IL-18 and TNF-a (Coppé et al., 2010; Freund et al., 2010; Prattichizzo et al., 2016). These cytokines promote inflammation locally in a paracrine manner and perhaps at a systemic level (Stojanović et al., 2020). The chronic inflamed endothelium tends to become dysfunctional and allow deposits to build up, which also recruit adaptive immune cells by way of their inflammatory process (Libby et al., 2002). Besides MFCs, T cells play a curricular role as major regulators of atherogenesis. They can either act as positive or negative modulators of plaques. In particular, CD4+ cells are considered to be an important cell type (Daugherty and Rateri, 2002; Hansson, 2005). Activated effector memory (EM) and central memory (CM) subtypes accumulate and thus stimulate the progression of atherogenesis. Inside the atherosclerotic plaques the cells release pro-inflammatory cytokines and bind to antigens of cholesterol-rich lipoproteins (Ammirati et al., 2012). After feeding a hypercholesterolemia-inducing diet, antigen-specific T cell clones actively expand in the plaque (Centa et al., 2018). CD4+ cells in plaque are specific for oxLDL since LDL is a relevant autoantigen that could drive the autoimmune response against intrinsic proteins in the atherosclerotic plaque (Stemme et al., 1995) (Wolf and Ley, 2019).
These findings are in agreement with our own data demonstrating that T effector memory re-expressing CD45RA (T-EMRA cells) are highly associated with body fat (Boßlau et al., 2021). T-EMRA cells are also strong producers of inflammatory cytokines and exhibit cytotoxic activity toward the endothelium, probably contributing to plaque erosion. Therefore, they tend to be associated with unstable plaques and with severe CVD, and are considered a predictor of mortality in the elderly (Stojanović et al., 2020). In addition to the pro-atherogenic properties of highly differentiated CD4+ T cells, also CD8+ cells are involved in atherosclerotic remodeling, although little is known about their role in pathogenesis (Carrasco et al., 2022). It is suggested that CD8+ cells contribute to inflammatory processes in the plaque, which might favor its instability. However, the antigen specificity of these CD8 lymphocytes is poorly understood (Kolbus et al., 2010; Kyaw et al., 2013).
Heat shock proteins (HSPs) are expressed when cells are exposed to cellular stress factors such as hypoxia or infection. In addition, there are data showing that HSPs are also increased intracellularly and extracellularly in endothelial cells during atherosclerosis, and increased extracellular levels are associated with systemic inflammation (Xu et al., 2012). It is well known that proteins like HSP 70 can modulate the inflammatory response in the context of cellular stress reactions (Noble and Shen, 2012). Already in early stages of atherosclerosis, an increased expression and release of HSPs was shown. It is suggested that the HSP-induction results from one or a combination of factors, such as hyperlipidaemia, diabetes, smoking, and hypertension (Zhu et al., 2003). Increased oxidative stress might be the primary trigger which leads to the induction of HSP expression in vascular smooth muscle cells and in serum (Liao et al., 2000). It was further demonstrated that HSP60 serum levels correlate with the total cholesterol, LDL, and ApoB and negatively with adiponectin, and the intensity of HSP expression also correlates positively with the severity of atherosclerosis (Pockley, 2002). Another source of HSP60 are endothelial cells which are stressed by dyslipidemia. HSP60 synthesis and release modifies endothelial cells to targets of HSP60 specific T cells, as they express increased adhesion molecules. This favors the formation of macrophage-derived foam cells (Hashikawa et al., 2021). Mechanistically, HSP60 has been shown to contribute directly to the development of arteriosclerosis due to an increased synthesis of E-selectin andVCAM-1 within the endothelial cell (Kol et al., 1999). In response of chronic upregulation, these adhesion molecules participate in monocyte accumulation in the arterial intima. These results are supported by studies indicating that HSP 60 is selectively located in atherosclerotic lesions rather than non-atherosclerotic areas of the arterial wall (Guisasola et al., 2009).
The physiological processes triggered by increased HSP expression inside and outside the cell are divergent. While HSPs exhibit protective and anti-inflammatory activity intracellularly, increased extracellular levels results in pro-inflammatory signals (De et al., 2000). This divergence appears to be based in the fact that increased extracellular appearance of HSPs is often the result of a chronic and marked HSP expression, which is a consequence of cardiovascular stress, such as chronic exposure to oxidative stress. Then, the protective function is partially lost, and HSPs may promote atherosclerosis (Krüger et al., 2019).
The clear links between lipid metabolism disorders and cardiovascular morbidity and mortality already make it clear that there is, conversely, also a causal relationship between a therapeutically reduced LDL level, increased HDL and a reduction in the cardiovascular risk profile. Scientifically, this is undisputed, so that the treatment of elevated lipid levels aimed at individual targets represents a key component of risk modification in the primary and secondary prevention of cardiovascular disease (Pressler, 2017). The steadily increasing prevalence of dyslipidemia requires the progressive use of lifestyle measures in addition to drug therapy. Many randomized controlled trials and meta-analyses have convincingly demonstrated that regular physical activity is effective in both the prevention and treatment of hyperlipidemia and dyslipidemia. Conversely, this is underpinned by the fact that a sedentary lifestyle is a major cause of dyslipidemia and cardiovascular disease (Albarrati et al., 2018).
Epidemiological studies and prospective intervention studies provide clear evidence that physical activity (in the sense of total activity) and also exercise training can reduce cardiovascular morbidity and mortality by improving lipid profiles (Rhee et al., 2019). The best relationship can be established between the activity level and increased HDL values as well as reduced triglyceride values. Regarding HDL levels, a meta-analysis with 19 included studies showed that HDL2-C levels increased significantly through regular endurance training. It was interesting to note that this effect also occurred independently of changes in body weight and BMI (Kelley and Kelley, 2006). There are contradictory data regarding the effect of physical activity on LDL and its subfractions. However, with regard to the small LDL particles, which are classified as particularly atherogenic, there are indications that these are reduced by physical training (Varady et al., 2005).
The mechanisms of these effects are manifold. The primary one is certainly the metabolic effect of exercise, as the increased energy metabolism of active people increases both the metabolism due to more muscular activity and, in the long term, the resting metabolism. Accordingly, an increased calorie intake can be at least partially compensated for by exercise. Due to the increased energy turnover and the addressed substrate utilization pathways, different systems of the body adapt functionally and structurally to the corresponding metabolic challenge. For example, the reduction in plasma triglyceride concentration is primarily due to the upregulation of lipoprotein lipase (LPL) activity and quantity in skeletal muscle. Moderate endurance exercise in particular uses intramuscular triglycerides as a primary source of energy, depending on the duration (Watt et al., 2002). In addition to enzyme systems of the musculature, other processes of lipid metabolism are also promoted by physical activity. For example, exercise increases the activity of hormone-sensitive lipases (HSLs) in adipose tissue and muscle, allowing triglycerides to be more efficiently converted to free fatty acids and mobilized from the tissues. The expression of plasma membrane fatty acid binding proteins, such as FABPPM, also increases with regular activity, allowing fatty acids to enter the muscle cell more efficiently. The intramuscular capacity to bind free fatty acids in the cytosol and transport them to the mitochondria for β-oxidation is also improved by regular activity (Ringseis et al., 2011). The mechanism of HDL increase through exercise is only partially understood. One cause seems to be the increase in adiponectin secretion through exercise. Adiponectin is a protein hormone that is predominantly produced by adipocytes and is involved in the regulation of glucose levels and fatty acid breakdown. Adiponectin concentrations could be positively correlated with HDL-C levels in men and women in several studies. Thus, as an anti-inflammatory adipokine, adiponectin could also have a metabolic mediator role, since an influence of adipokine on HDL synthesis in the liver has been shown (Greene et al., 2012). Furthermore, regular activity reduces hepatic triglyceride secretion. Since peripheral HDL levels increase at the same time, both processes could be related in the context of activity (Couillard et al., 2001).
In addition to affecting blood lipids, physical exercise has a positive immune-regulating effect, which can foster anti-inflammation and thus can promote metabolic and cardiovascular health. Two mechanisms are important here. Exercise seems to positively influence cellular immune senescence, in addition to the release of pro- and anti-inflammatory cytokines. Thus, exercise affects both systems, which, as described above, are in a bidirectional relationship with chronic low-grade inflammation (Rosa-Neto et al., 2022).
With regard to T cell differentiation and expression of pro-inflammatory subtypes, research shows that training status and the starting of exercise training are related to senescent hallmarks (Duggal et al., 2019; Weyh et al., 2020). Cross-sectional data show that trained individuals or those with a long history of exercise have fewer senescent CD4+ as well as CD8+ CD28−CD57+ cells. Additionally, they had fewer differentiated CD4+ CM cells, CD8+ CM and EM cells and fewer highly differentiated CD4+ and CD8+ T-EMRA cells (Spielmann et al., 2011; Minuzzi et al., 2018). As described above, these cell types also play an important role in the development of atherosclerosis and plaque formation, in addition to being associated with antigens of cholesterol-rich lipoproteins. In parallel, a higher proportion of naïve T cells is observed. The beginning of regular exercise in previously inactive subjects can also affect hallmarks of T-cell aging. After only 3 weeks of endurance training in prediabetic subjects, a proportional increase in naïve and central memory T cells was found, while at the same time the proportion of senescent CD8+ T-EMRA cells decreased (Philippe et al., 2019). Six weeks of a combined strength and endurance training increased the CD4+/CD8+ cell ratio in the elderly (Despeghel et al., 2021). However, further studies need to confirm these results as well as recommendations for the type, intensity, and duration of exercise.
Regular exercise also affects components of the innate immune system. Accordingly, it was shown in animal experiments that regular treadmill exercise induced the conversion of M1 to M2 macrophages, which corresponds to a change from a classical, more pro-inflammatory type to an alternative, more immunoregulatory type. This altered polarization is associated with a change in the expression profile of cytokines towards more anti-inflammatory messengers (Gleeson et al., 2011; Oliveira et al., 2013). An important mechanism shows that anti-inflammatory signals occur from the active skeletal muscle itself. Thus, during muscle contraction, increased IL-6 is released, described as myokine. IL-6 has an anti-inflammatory effect through exercise by stimulating the production of immune regulatory mediators such as IL-10 and the IL-1 receptor antagonist (Steensberg et al., 2003) as well as the downregulation of TNF-α by monocytes and macrophages (Starkie et al., 2003). IL-7 (Haugen et al., 2010) and IL-15 (Rinnov et al., 2014) are also myokines that may stimulate lymphocyte proliferation. IL-7 is assumed to exert a protective effect on the thymus. IL-15 appears to have effects on the induction of better survival of naïve T cells. Both cytokines were increased in subjects who were physically active throughout their lives, compared to their inactive controls (Duggal et al., 2018). In contrast to endurance training, which can reduce systemic IL-6 and TNF-α levels (Zheng et al., 2019), resistance training seems to have no or marginal effect on chronic inflammation (Rose et al., 2021). A combination of endurance and resistance exercise seems to affect basal levels of pro- or anti-inflammatory cytokines like IL-6, -8 and -10, even in the presence of inflammatory comorbidities (Despeghel et al., 2021). Similarly, it was also shown that exercise in postmenopausal obese women with dyslipidemia reduces systemic levels of TNF-α, which in turn has positive effects on metabolic health (Biteli et al., 2021).
Long-term exercise training improves the intracellular HSP response, which was demonstrated in muscle tissue and leukocytes (Fehrenbach et al., 2001; Yamada et al., 2008). Specifically, the expression of intracellular Hsp72 is suggested to be important to stabilize intracellular processes during conditions of increased oxidative stress which occurs in the context of systemic inflammation and dyslipidemia. (Morimoto, 1993; Febbraio and Koukoulas, 2000). Accordingly, we suggest that physical activity can strengthen cellular resilience by means of increased intracellular HSP induction, but at the same time prevent excessive release of HSPs into the extracellular space (Figure 1).
FIGURE 1. Interaction of blood lipids, cellular and molecular components of the immune system and stress proteins in an inactive lifestyle and an active lifestyle. The lower left half of the vessel visualizes the consequences of an active lifestyle, where reactive oxygen species (ROS) are effectively reduced, a more anti-inflammatory environment prevails, and blood lipids are present with a rather low LDL and higher HDL. The contrast is the upper right half of the vessel, where a more proinflammatory environment prevails, with higher LDL levels and accumulated senescent cells. An arteriosclerotic plaque has also already formed here, containing foam cells and infiltrated by more inflammatory leukocyte subpopulations.
According to the S1 Guideline “Screening in sports”, a sports medical screening should be carried out in the sense of a health examination to detect latent or pre-existing diseases that may pose a risk. Previous data indicate that targeted sporting activities have greater effects on changes in the lipid profile than a non-sport-based increase in everyday activity. Nevertheless, even more active daily life, which includes walking and strolling, climbing stairs and various physical activities of daily living, can contribute to the positive modification of plasma lipids. If we look at controlled exercise training, greater effects can be expected, especially through regular moderate and endurance activities. Current data recommend exercising for at least 30 min (or more) on as many days as possible. These amounts can also be started at a reduced level (e.g., shorter units of 10 min duration) and increased over time.
Practically, such activities can be achieved as walking and Nordic walking, jogging runs or cycling rides, by swimming or by participating in “cardio courses” in fitness facilities (Pressler, 2017). Intensities in the basic endurance range, i.e., below the individual anaerobic threshold, are recommended for such training units. Patients can be told as a rule of thumb for moderate-intensity aerobic training that you are active at a level that increases breathing and heart rate but still allows you to maintain yourself. In the area of endurance training, numerous health effects of High-Intensity Interval Training (HIIT) have also been demonstrated in recent years. This involves short-term intensive interval units that are usually performed on an ergometer or in running. At present, there is not enough data to assess the long-term effects of this type of training on a given lipometabolic disorder. Nevertheless, the studies show that HIIT also has significant effects on fat metabolism. Strong adrenergic innervation can improve the sensitivity of HSLs in adipose tissue, reduce metabolic stress in visceral fat and induce anti-inflammatory effects via stimulation of steroid hormone biosynthesis pathways (Sun et al., 2020). Accordingly, so-called “polarized training”, which is a combination of basic and HIIT training, can certainly be an effective training for dyslipidemia when individually designed. The effectiveness of pure strength training on the lipid profile is contradictory in the literature. Nevertheless, strength training is always considered useful in the context of general health promotion in order to provide repeated stimuli to maintain muscle mass and thus functionally maintain a certain “everyday athleticism”, prevent sarcopenia and promote the resilience of the musculoskeletal system (Barajas-Galindo et al., 2021). Here, the recommendations are 8–15 repetitions per exercise, which should be performed at least twice a week. This includes a 5–10 min warm-up with light aerobic activity. Each strengthening exercise should also be technically prepared so that it is performed correctly.
If statin therapy is indicated, it can be combined with activity and sports programs without any problems. If patients follow the activity recommendations in the long term, effects on blood lipids, especially on HDL and triglycerides, can already be expected after a few weeks. Finally, it should be mentioned that most patients with lipometabolic disorders have multiple morbidities that are influenced by physical activity. Here, it is particularly important to clarify the patients’ fitness for sports by means of a sports medicine stress examination, depending on the indication.
KK did the literature reseach, developed the idea, written and edited the manuscript. PT did the literature reseach, written and edited the manuscript. CW the literature reseach, developed the idea, written and edited the manuscript.
The authors declare that the research was conducted in the absence of any commercial or financial relationships that could be construed as a potential conflict of interest.
All claims expressed in this article are solely those of the authors and do not necessarily represent those of their affiliated organizations, or those of the publisher, the editors and the reviewers. Any product that may be evaluated in this article, or claim that may be made by its manufacturer, is not guaranteed or endorsed by the publisher.
Alack K., Pilat C., Krüger K. (2019). Current knowledge and new challenges in exercise immunology. Dtsch. Z. Sportmed. 70, 250–260. doi:10.5960/dzsm.2019.391
Albarrati A. M., Alghamdi M. S. M., Nazer R. I., Alkorashy M. M., Alshowier N., Gale N., et al. (2018). Effectiveness of low to moderate physical exercise training on the level of low-density lipoproteins: A systematic review. Biomed. Res. Int. 2018, 5982980. doi:10.1155/2018/5982980
Ammirati E., Cianflone D., Vecchio V., Banfi M., Vermi A. C., Metrio M. de, et al. (2012). Effector memory T cells are associated with atherosclerosis in humans and animal models. J. Am. Heart Assoc. 1, 27–41. doi:10.1161/JAHA.111.000125
Barajas-Galindo D. E., González Arnáiz E., Ferrero Vicente P., Ballesteros-Pomar M. D. (2021). Effects of physical exercise in sarcopenia. A systematic review. Endocrinol. Diabetes Nutr. 68, 159–169. doi:10.1016/j.endien.2020.02.007
Biteli P., Barbalho S. M., Detregiachi C. R. P., Dos Santos Haber J. F., Chagas E. F. B. (2021). Dyslipidemia influences the effect of physical exercise on inflammatory markers on obese women in post-menopause: A randomized clinical trial. Exp. Gerontol. 150, 111355. doi:10.1016/j.exger.2021.111355
Boßlau T. K., Wasserfurth P., Krüger B., Reichel T., Palmowski J., Nebl J., et al. (2021). Abdominal obesity-related disturbance of insulin sensitivity is associated with CD8+ EMRA cells in the elderly. Cells 10. doi:10.3390/cells10050998
Carrasco E., Gómez de Las Heras M. M., Gabandé-Rodríguez E., Desdín-Micó G., Aranda J. F., Mittelbrunn M., et al. (2022). The role of T cells in age-related diseases. Nat. Rev. Immunol. 22, 97–111. doi:10.1038/s41577-021-00557-4
Centa M., Prokopec K. E., Garimella M. G., Habir K., Hofste L., Stark J. M., et al. (2018). Acute loss of apolipoprotein E triggers an autoimmune response that accelerates atherosclerosis. Arterioscler. Thromb. Vasc. Biol. 38, e145–e158. doi:10.1161/ATVBAHA.118.310802
Coppé J.-P., Desprez P.-Y., Krtolica A., Campisi J. (2010). The senescence-associated secretory phenotype: The dark side of tumor suppression. Annu. Rev. Pathol. 5, 99–118. doi:10.1146/annurev-pathol-121808-102144
Couillard C., Després J. P., Lamarche B., Bergeron J., Gagnon J., Leon A. S., et al. (2001). Effects of endurance exercise training on plasma HDL cholesterol levels depend on levels of triglycerides: Evidence from men of the health, risk factors, exercise training and genetics (HERITAGE) family study. Arterioscler. Thromb. Vasc. Biol. 21, 1226–1232. doi:10.1161/hq0701.092137
Daugherty A., Rateri D. L. (2002). T lymphocytes in atherosclerosis: The yin-yang of Th1 and Th2 influence on lesion formation. Circ. Res. 90, 1039–1040. doi:10.1161/01.RES.0000021397.28936.F9
Davalos A. R., Coppe J.-P., Campisi J., Desprez P.-Y. (2010). Senescent cells as a source of inflammatory factors for tumor progression. Cancer Metastasis Rev. 29, 273–283. doi:10.1007/s10555-010-9220-9
De A. K., Kodys K. M., Yeh B. S., Miller-Graziano C. (2000). Exaggerated human monocyte IL-10 concomitant to minimal TNF-alpha induction by heat-shock protein 27 (Hsp27) suggests Hsp27 is primarily an antiinflammatory stimulus baltimore, Md(1950). J. Immunol. 165, 3951–3958. doi:10.4049/jimmunol.165.7.3951
Despeghel M., Reichel T., Zander J., Krüger K., Weyh C. (2021). Effects of a 6 Week low-dose combined resistance and endurance training on T cells and systemic inflammation in the elderly. Cells 10. doi:10.3390/cells10040843
Duggal N. A., Niemiro G., Harridge S. D. R., Simpson R. J., Lord J. M. (2019). Can physical activity ameliorate immunosenescence and thereby reduce age-related multi-morbidity? Nat. Rev. Immunol. 19, 563–572. doi:10.1038/s41577-019-0177-9
Duggal N. A., Pollock R. D., Lazarus N. R., Harridge S., Lord J. M. (2018). Major features of immunesenescence, including reduced thymic output, are ameliorated by high levels of physical activity in adulthood. Aging Cell 17, e12750. doi:10.1111/acel.12750
Febbraio M. A., Koukoulas I. (2000). HSP72 gene expression progressively increases in human skeletal muscle during prolonged, exhaustive exercise bethesda, Md(1985). J. Appl. Physiol., 89, 1055–1060. doi:10.1152/jappl.2000.89.3.1055
Fehrenbach E., Niess A. M., Veith R., Dickhuth H. H., Northoff H. (2001). Changes of HSP72-expression in leukocytes are associated with adaptation to exercise under conditions of high environmental temperature. J. Leukoc. Biol. 69, 747–754.
Franceschi C. (2007). Inflammaging as a major characteristic of old people: Can it be prevented or cured? Nutr. Rev. 65, S173–S176. doi:10.1111/j.1753-4887.2007.tb00358.x
Freund A., Orjalo A. V., Desprez P.-Y., Campisi J. (2010). Inflammatory networks during cellular senescence: Causes and consequences. Trends Mol. Med. 16, 238–246. doi:10.1016/j.molmed.2010.03.003
García-Gómez C., Bianchi M., La Fuente D. d., Badimon L., Padró T., Corbella E., et al. (2014). Inflammation, lipid metabolism and cardiovascular risk in rheumatoid arthritis: A qualitative relationship? World J. Orthop. 5, 304–311. doi:10.5312/wjo.v5.i3.304
Gleeson M., Bishop N. C., Stensel D. J., Lindley M. R., Mastana S. S., Nimmo M. A., et al. (2011). The anti-inflammatory effects of exercise: Mechanisms and implications for the prevention and treatment of disease. Nat. Rev. Immunol. 11, 607–615. doi:10.1038/nri3041
Goldstein J. L., Brown M. S. (2015). A century of cholesterol and coronaries: From plaques to genes to statins. Cell 161, 161–172. doi:10.1016/j.cell.2015.01.036
Grebe A., Latz E. (2013). Cholesterol crystals and inflammation. Curr. Rheumatol. Rep. 15, 313. doi:10.1007/s11926-012-0313-z
Greene N. P., Fluckey J. D., Lambert B. S., Greene E. S., Riechman S. E., Crouse S. F., et al. (2012). Regulators of blood lipids and lipoproteins? PPARδ and AMPK, induced by exercise, are correlated with lipids and lipoproteins in overweight/obese men and women. Am. J. Physiol. Endocrinol. Metab. 303, E1212–E1221. doi:10.1152/ajpendo.00309.2012
Guisasola M. C., Dulín E., Almendral J., García-Barreno P. (2009). Reduction of heat shock protein antibody levels by statin therapy. Lipids 44, 317–324. doi:10.1007/s11745-008-3265-3
Hansson G. K. (2005). Inflammation, atherosclerosis, and coronary artery disease. N. Engl. J. Med. 352, 1685–1695. doi:10.1056/NEJMra043430
Hashikawa N., Ido M., Morita Y., Hashikawa-Hobara N. (2021). Effects from the induction of heat shock proteins in a murine model due to progression of aortic atherosclerosis. Sci. Rep. 11, 7025. doi:10.1038/s41598-021-86601-8
Haugen F., Norheim F., Lian H., Wensaas A. J., Dueland S., Berg O., et al. (2010). IL-7 is expressed and secreted by human skeletal muscle cells. Am. J. Physiol. Cell Physiol. 298, C807–C816. doi:10.1152/ajpcell.00094.2009
Hubler M. J., Kennedy A. J. (2016). Role of lipids in the metabolism and activation of immune cells. J. Nutr. Biochem. 34, 1–7. doi:10.1016/j.jnutbio.2015.11.002
Kelley G. A., Kelley K. S. (2006). Aerobic exercise and HDL2-C: A meta-analysis of randomized controlled trials. Atherosclerosis 184, 207–215. doi:10.1016/j.atherosclerosis.2005.04.005
Khovidhunkit W., Kim M.-S., Memon R. A., Shigenaga J. K., Moser A. H., Feingold K. R., et al. (2004). Effects of infection and inflammation on lipid and lipoprotein metabolism: Mechanisms and consequences to the host. J. Lipid Res. 45, 1169–1196. doi:10.1194/jlr.R300019-JLR200
Kim D., Chung H., Lee J.-E., Kim J., Hwang J., Chung Y., et al. (2021). Immunologic aspects of dyslipidemia: A critical regulator of adaptive immunity and immune disorders. J. Lipid Atheroscler. 10, 184–201. doi:10.12997/jla.2021.10.2.184
Kol A., Bourcier T., Lichtman A. H., Libby P. (1999). Chlamydial and human heat shock protein 60s activate human vascular endothelium, smooth muscle cells, and macrophages. J. Clin. Invest. 103, 571–577. doi:10.1172/JCI5310
Kolbus D., Ramos O. H., Berg K. E., Persson J., Wigren M., Björkbacka H., et al. (2010). CD8+ T cell activation predominate early immune responses to hypercholesterolemia in Apoe⁻(/)⁻ mice. BMC Immunol. 11, 58. doi:10.1186/1471-2172-11-58
Krüger K., Reichel T., Zeilinger C. (2019). Role of heat shock proteins 70/90 in exercise physiology and exercise immunology and their diagnostic potential in sports bethesda, Md(1985). J. Appl. Physiol. 126, 916–927. doi:10.1152/japplphysiol.01052.2018
Kyaw T., Winship A., Tay C., Kanellakis P., Hosseini H., Cao A., et al. (2013). Cytotoxic and proinflammatory CD8+ T lymphocytes promote development of vulnerable atherosclerotic plaques in apoE-deficient mice. Circulation 127, 1028–1039. doi:10.1161/CIRCULATIONAHA.112.001347
Liao D. F., Jin Z. G., Baas A. S., Daum G., Gygi S. P., Aebersold R., et al. (2000). Purification and identification of secreted oxidative stress-induced factors from vascular smooth muscle cells. J. Biol. Chem. 275, 189–196. doi:10.1074/jbc.275.1.189
Libby P., Ridker P. M., Maseri A. (2002). Inflammation and atherosclerosis. Circulation 105, 1135–1143. doi:10.1161/hc0902.104353
Minuzzi G., Luis R., Chupel M. U., Rosado F., dos Santos J. V., Simpson R. J., et al. (2018). Effects of lifelong training on senescence and mobilization of T lymphocytes in response to acute exercise. Exerc. Immunol. Rev. 24, 72–84.
Morimoto R. I. (1993). Cells in stress: Transcriptional activation of heat shock genes. Sci. (New York, N.Y.) 259, 1409–1410. doi:10.1126/science.8451637
Nakajima T., Schulte S., Warrington K. J., Kopecky S. L., Frye R. L., Goronzy J. J., et al. (2002). T-cell-mediated lysis of endothelial cells in acute coronary syndromes. Circulation 105, 570–575. doi:10.1161/hc0502.103348
Noble E. G., Shen G. X. (2012). Impact of exercise and metabolic disorders on heat shock proteins and vascular inflammation. Autoimmune Dis. 2012, 836519. doi:10.1155/2012/836519
Oliveira A. G., Araujo T. G., Carvalho B. M., Guadagnini D., Rocha G. Z., Bagarolli R. A., et al. (2013). Acute exercise induces a phenotypic switch in adipose tissue macrophage polarization in diet-induced obese rats. Obesity 21, 2545–2556. doi:10.1002/oby.20402
Philippe M., Gatterer H., Burtscher M., Weinberger B., Keller M., Grubeck-Loebenstein B., et al. (2019). Concentric and eccentric endurance exercise reverse hallmarks of T-cell senescence in pre-diabetic subjects. Front. Physiol. 10, 684. doi:10.3389/fphys.2019.00684
Pockley A. G. (2002). Heat shock proteins, inflammation, and cardiovascular disease. Circulation 105, 1012–1017. doi:10.1161/hc0802.103729
Prattichizzo F., Nigris V. de, La Sala L., Procopio A. D., Olivieri F., Ceriello A. (2016). Inflammaging" as a druggable target: A senescence-associated secretory phenotype-centered view of type 2 diabetes. Oxid. Med. Cell. Longev. 2016, 1810327. doi:10.1155/2016/1810327
Pressler A. (2017). A run a day keeps lipids at bay? Regular exercise as a treatment of dyslipidaemias. Dtsch. Z. Sportmed. 2017, 253–260. doi:10.5960/dzsm.2017.304
Rhee E.-J., Kim H. C., Kim J. H., Lee E. Y., Kim B. J., Kim E. M., et al. (2019). 2018 Guidelines for the management of dyslipidemia. Korean J. Intern. Med. 34, 723–771. doi:10.3904/kjim.2019.188
Ringseis R., Mooren F.-C., Keller J., Couturier A., Wen G., Hirche F., et al. (2011). Regular endurance exercise improves the diminished hepatic carnitine status in mice fed a high-fat diet. Mol. Nutr. Food Res. 55, S193–S202. doi:10.1002/mnfr.201100040
Rinnov A., Yfanti C., Nielsen S., Akerström T. C. A., Peijs L., Zankari A., et al. (2014). Endurance training enhances skeletal muscle interleukin-15 in human male subjects. Endocrine 45, 271–278. doi:10.1007/s12020-013-9969-z
Roma P., Bernini F., Fogliatto R., Bertulli S. M., Negri S., Fumagalli R., et al. (1992). Defective catabolism of oxidized LDL by J774 murine macrophages. J. Lipid Res. 33, 819–829. doi:10.1016/S0022-2275(20)41507-X
Rosa-Neto J. C., Lira F. S., Little J. P., Landells G., Islam H., Chazaud B., et al. (2022). Immunometabolism-fit: How exercise and training can modify T cell and macrophage metabolism in health and disease. Exerc. Immunol. Rev. 28, 29–46.
Rose G. L., Mielke G. I., Durr M., Schaumberg M. A. (2021). Effect of resistance training on chronic inflammation: A systematic review and meta‐analysis. Transl. Sports Med. 4, 900–913. doi:10.1002/tsm2.294
Sorci-Thomas M. G., Thomas M. J. (2016). Microdomains, inflammation, and atherosclerosis. Circ. Res. 118, 679–691. doi:10.1161/CIRCRESAHA.115.306246
Spielmann G., McFarlin B. K., O'Connor D. P., Smith P. J. W., Pircher H., Simpson R. J., et al. (2011). Aerobic fitness is associated with lower proportions of senescent blood T-cells in man. Brain Behav. Immun. 25, 1521–1529. doi:10.1016/j.bbi.2011.07.226
Starkie R., Ostrowski S. R., Jauffred S., Febbraio M., Pedersen B. K. (2003). Exercise and IL-6 infusion inhibit endotoxin-induced TNF-alpha production in humans. FASEB J. official Publ. Fed. Am. Soc. Exp. Biol. 17, 884–886. doi:10.1096/fj.02-0670fje
Steensberg A., Fischer C. P., Keller C., Møller K., Pedersen B. K. (2003). IL-6 enhances plasma IL-1ra, IL-10, and cortisol in humans. Am. J. Physiol. Endocrinol. Metab. 285, E433–E437. doi:10.1152/ajpendo.00074.2003
Stemme S., Faber B., Holm J., Wiklund O., Witztum J. L., Hansson G. K., et al. (1995). T lymphocytes from human atherosclerotic plaques recognize oxidized low density lipoprotein. Proc. Natl. Acad. Sci. U. S. A. 92, 3893–3897. doi:10.1073/pnas.92.9.3893
Stojanović S. D., Fiedler J., Bauersachs J., Thum T., Sedding D. G. (2020). Senescence-induced inflammation: An important player and key therapeutic target in atherosclerosis. Eur. Heart J. 41, 2983–2996. doi:10.1093/eurheartj/ehz919
Sun L., Li F.-H., Li T., Min Z., Yang L.-D., Gao H.-E., et al. (2020). Effects of high-intensity interval training on adipose tissue lipolysis, inflammation, and metabolomics in aged rats. Pflugers Arch. 472, 245–258. doi:10.1007/s00424-020-02351-y
van Diepen J. A., Berbée J. F. P., Havekes L. M., Rensen P. C. N. (2013). Interactions between inflammation and lipid metabolism: Relevance for efficacy of anti-inflammatory drugs in the treatment of atherosclerosis. Atherosclerosis 228, 306–315. doi:10.1016/j.atherosclerosis.2013.02.028
Varady K. A., St-Pierre A. C., Lamarche B., Jones P. J. H. (2005). Effect of plant sterols and endurance training on LDL particle size and distribution in previously sedentary hypercholesterolemic adults. Eur. J. Clin. Nutr. 59, 518–525. doi:10.1038/sj.ejcn.1602106
Watt M. J., Heigenhauser G. J. F., Dyck D. J., Spriet L. L. (2002). Intramuscular triacylglycerol, glycogen and acetyl group metabolism during 4 h of moderate exercise in man. J. Physiol. 541, 969–978. doi:10.1113/jphysiol.2002.018820
Weyh C., Krüger K., Strasser B. (2020). Physical activity and diet shape the immune system during aging. Nutrients 12, E622. doi:10.3390/nu12030622
Wolf D., Ley K. (2019). Immunity and inflammation in atherosclerosis. Circ. Res. 124, 315–327. doi:10.1161/CIRCRESAHA.118.313591
Xu Q., Metzler B., Jahangiri M., Mandal K. (2012). Molecular chaperones and heat shock proteins in atherosclerosis. Am. J. Physiol. Heart Circ. Physiol. 302, H506–H514. doi:10.1152/ajpheart.00646.2011
Yamada P., Amorim F., Moseley P., Schneider S. (2008). Heat shock protein 72 response to exercise in humans. Sports Med. 38, 715–733. doi:10.2165/00007256-200838090-00002
Zheng G., Qiu P., Xia R., Lin H., Ye B., Tao J., et al. (2019). Effect of aerobic exercise on inflammatory markers in healthy middle-aged and older adults: A systematic review and meta-analysis of randomized controlled trials. Front. Aging Neurosci. 11, 98. doi:10.3389/fnagi.2019.00098
Keywords: inflammation, immunosenescence, sports therapy, metabolism, cardiovascular disease
Citation: Krüger K, Tirekoglou P and Weyh C (2022) Immunological mechanisms of exercise therapy in dyslipidemia. Front. Physiol. 13:903713. doi: 10.3389/fphys.2022.903713
Received: 24 March 2022; Accepted: 07 July 2022;
Published: 08 August 2022.
Edited by:
Ismail Laher, University of British Columbia, CanadaReviewed by:
David B. Bartlett, Duke University, United StatesCopyright © 2022 Krüger, Tirekoglou and Weyh. This is an open-access article distributed under the terms of the Creative Commons Attribution License (CC BY). The use, distribution or reproduction in other forums is permitted, provided the original author(s) and the copyright owner(s) are credited and that the original publication in this journal is cited, in accordance with accepted academic practice. No use, distribution or reproduction is permitted which does not comply with these terms.
*Correspondence: Karsten Krüger, S2Fyc3Rlbi5rcnVlZ2VyQHNwb3J0LnVuaS1naWVzc2VuLmRl
Disclaimer: All claims expressed in this article are solely those of the authors and do not necessarily represent those of their affiliated organizations, or those of the publisher, the editors and the reviewers. Any product that may be evaluated in this article or claim that may be made by its manufacturer is not guaranteed or endorsed by the publisher.
Research integrity at Frontiers
Learn more about the work of our research integrity team to safeguard the quality of each article we publish.