- 1Laboratory of Comparative Physiology of Pigmentation, Department of Physiology, Institute of Biosciences, University of São Paulo, São Paulo, Brazil
- 2Center of Brain, Behavior and Metabolism, Institute of Neurobiology, Lübeck University, Lübeck, Germany
- 3Laboratory of Neurobiology, Department of Physiology and Biophysics, Institute of Biomedical Sciences, University of São Paulo, São Paulo, Brazil
- 4Department of Physiology, Institute of Biosciences, University of São Paulo, São Paulo, Brazil
- 5Department of Biology, University of Virginia, Charlottesville, United States
Environmental cues synchronize endogenous rhythms of many physiological processes such as hormone synthesis and secretion. Little is known about the diurnal pattern of hormones and gene expression of the Callinectes sapidus molt cycle. We aimed to investigate in the eyestalk and hepatopancreas of premolt and intermolt C. sapidus the following parameters: 1) the diurnal expression of the ecdysteroid receptor CasEcR isoforms, and the molt inhibiting hormone CasMIH; 2) the diurnal hemolymph ecdysteroid and melatonin levels; and 3) melatonin effects on the transcripts of the above-mentioned genes in intermolt C. sapidus. Ecdysteroid levels were higher in the premolt than the intermolt animals at all time points evaluated (ZTs). Premolt crabs displayed a variation of ecdysteroid concentration between time points, with a reduction at ZT17. No difference in the melatonin level was seen in either molt stage or between stages. In the eyestalk of intermolt animals, CasEcR expression oscillated, with a peak at ZT9, and premolt crabs have a reduction at ZT9; CasMIH transcripts did not vary along 24 h in either molt stage. Moreover, the evaluated eyestalk genes were more expressed at ZT9 in the intermolt than the premolt crabs. In the hepatopancreas, CasEcR expression showed a peak at ZT9 in premolt crabs. Exogenous melatonin (10−7 mol/animal) reduced the expression of both genes in the eyestalk at ZT17. In the hepatopancreas, melatonin markedly increased the expression of the CasEcR gene at ZT9. Taken altogether, our results are pioneer in demonstrating the daily oscillation of gene expression associated to molt cycle stages, as well as the daily ecdysteroid and melatonin levels and the remarkable influence of melatonin on the molt cycle of C. sapidus.
1 Introduction
The crustacean molt cycle is a complex process comprising behavioral, morphological, and physiological events that ultimately promote differentiation and growth (Lipcius and Herrnkind, 1982; Chang, 1995). The crustacean molt cycle may be staged in four phases: premolt (D), ecdysis (E), post-molt (A-B), and intermolt (C) (or anecdysis) (Spindler et al., 1974; Kuballa and Elizur, 2007). Every molt cycle stage is subdivided into several sub-stages. These sub-stages are comprised of morphological and physiological changes as a consequence of hormone fluctuations found in each stage (Loeb, 1993; Chang and Mykles, 2011).
The positive regulation of the cycle is mainly exerted by cholesterol-derived hormones, the ecdysteroids, which are synthesized and released by the Y-organ as inactive forms (Spaziani and Wang, 1993). The ecdysteroids are converted to molt-inducing factors in the target organs (Böcking et al., 1995; Spaziani et al., 1997), thus stimulating tissue-specific responses that culminate with ecdysis. The ecdysteroids bind to a nuclear receptor (EcR) that forms a heterodimer with the retinoic acid receptor (RXR) or ultraspiracle protein (USP), to evoke their responses (Chen et al., 2017). In C. sapidus CasEcR and CasRXR co-express in the eyestalks and Y-organ, but with different levels, suggesting response and sensitivity differences to ecdysteroids (Techa and Chung, 2013). In the insect Rhodnius prolixus, the ecdysteroid receptor oscillates in some of the hormone targets exhibiting a robust diurnal rhythm synchronized with the circulating levels of ecdysteroid, and daily migrating from the cytoplasm to the nucleus (Vafopoulou and Steel, 2006). In general, ecdysteroid concentration is low during the intermolt, peaks in the premolt, followed by a decrease just before the ecdysis (Chung, 2010).
Ecdysteroid release as well as its action in peripheral tissues are regulated by X-organ hormones localized in the crustacean eyestalk (Asazuma et al., 2009). The X-organ is responsible for producing and releasing many peptide hormones like the pigment dispersing and concentrating hormones (PDH and PCH, respectively), and the hyperglycemic hormone (CHH) superfamily (Hopkins, 2012; Webster et al., 2012; Katayama, 2016). The molt inhibiting hormone (MIH) is a representative peptide hormone of the CHH-type II family (Nakatsuji et al., 2009); its receptor is a membrane guanylyl cyclase, also identified in Callinectes sapidus (Zeng et al., 2008). MIH inhibits ecdysteroid production and secretion, through receptor binding on Y-organ (Spaziani et al., 1999). Hemolymph levels of the two hormones, ecdysteroids and MIH, vary in anti-phase throughout the cycle, i.e., in the premolt, the ecdysteroid concentration is high, whereas MIH remains high during intermolt and post-molt (Lee et al., 1998; Nakatsuji et al., 2000; Nakatsuji and Sonobe, 2004).
Another hormone, methyl farnesoate, is considered an accessory hormone of the molt induction, showing higher concentrations in the premolt stage (Nagaraju et al., 2006; Nagaraju, 2007; 2011); it is produced by the mandibular organ, increases ecdysteroid concentration in the Y-organ cells, and accelerates the molt cycle (Reddy et al., 2004).
In addition to the endocrine organs classically involved in the molt cycle, like the X-organ and the Y-organ, the hepatopancreas (midgut gland) also participates in the molt cycle. The hepatopancreas is an essential metabolic tissue responsible for the storage and distribution of nutrients, whose demands change throughout the cycle (Lipcius and Herrnkind, 1982; Huang et al., 2015). The proteomic analysis of Scylla paramamosain hepatopancreas demonstrated that 193 proteins responsible for exoskeleton and cuticle reconstruction, energy reserves, immune responses, and metabolism were altered between the molt stages (Liu et al., 2021). During the Eriocheir sinensis vitellogenesis, the hepatopancreas expresses four EcR isoforms with the same profile, which play a more important role during the earlier ovarian development stages (Su et al., 2020). Furthermore, the organ produces vitellogenin that is stimulated by MIH during the process of ovarian maturation in C. sapidus (Zmora et al., 2009; Luo et al., 2015).
Environmental cues, named zeitgebers (time givers in the German language), comprise physical factors such as light, temperature, salinity, and tides among others, which may have been imposed on the organisms since the species origin. These factors affect the physiological processes by synchronizing their endogenous rhythms with each other and with the environment (Stoner et al., 2013; Espinosa-Chaurand et al., 2017). This feature allows biological processes to occur in an appropriate order, thereby preventing concurrent activation of potentially incompatible mechanisms.
Among a variety of biological processes, endocrine signaling is one of the most integrative diurnal systems. In this sense, melatonin, the messenger of the darkness, emerges as an important hormone that contributes to the whole-body temporal regulation in vertebrates (Reiter et al., 2009; Markus and Ferreira, 2011; Dardente, 2012; Cipolla-Neto et al., 2014; Cipolla-Neto and Amaral, 2018). Melatonin is a ubiquitous and amphiphilic molecule derived from the amino acid tryptophan, which is converted to the bioactive hormone due to the action of the enzymes aryl-alkyl aminotransferase (AANAT) and hydroxymethyl transferase (ASMT) (Axelrod and Weissbach, 1960; Vivien-Roels and Pévet, 1993; Tan et al., 2016). It is widely accepted that melatonin oscillates in a circadian pattern in all vertebrates studied to date, peaking in the dark phase, because the biosynthesis limiting enzyme—AANAT—is inhibited by light through clock-regulated signaling (Wurtman et al., 1963; Reiter et al., 2014).
In Crustaceans, on the other hand, there is no consensus as the time of the day at which melatonin peaks in the various species as well as if this hormone displays an oscillatory pattern (Agapito et al., 1995; Tilden et al., 1997; Maciel et al., 2008; Markowska et al., 2009; Sainath et al., 2013; Han et al., 2018). As to melatonin actions in crustaceans, it has already been demonstrated that the indoleamine plays a role in limb regeneration (Tilden et al., 1997), ecdysteroid production (Sainath S. B. and Reddy P. S., 2010; Girish et al., 2015), antioxidant defense (Maciel et al., 2010; Geihs et al., 2016; She et al., 2019), color change (Nery et al., 1999), locomotor activity (Geihs et al., 2010), and hyperglycemia (Sainath SB. and Reddy PS., 2010; Maciel et al., 2014; Yang et al., 2018), among others. Many melatonin functions in crustaceans are related to the molt cycle, as shown by the increased levels of ecdysteroids in melatonin-treated Y-organ cells (Girish et al., 2015).
Little is known about the diurnal oscillation of molting hormones, and their role in the daily regulation of the molt cycle. In this study, we aimed to evaluate whether the gene expression of the ecdysteroid receptor (CasEcR) and the molt-inhibiting hormone (CasMIH) and the secretion of ecdysteroids and melatonin displayed a diurnal profile in intermolt and premolt animals. These molt stages were selected because they show the most striking hormone anti-phase peaks, which may be related to the parameters we chose to measure. Here we report a diurnal pattern of molt cycle-related genes and ecdysteroid and melatonin levels, suggesting melatonin as a positive modulator of the molt cycle in C. sapidus.
2 Material and Methods
2.1 Animals
Males and females (average weight: 55.64 ± 10.7 g, and average carapace width: 9.5 ± 0.58 cm) of Callinectes sapidus were purchased from fishermen in Iguape city, state of São Paulo, Brazil, between March and May of 2017. The animals were transported to the University of São Paulo and kept for at least 3 days in an open water system with constant aeration, 10 ppm salinity, 22°C, under light/dark cycles of 12:12 LD (white light, 420–750 nm, 400 lux, 58 μW/cm2), lights on 7 a.m. (ZT0) and off 7 p.m. (ZT12) (zeitgeber time - ZT). For all experiments, the animals’ molt cycle stages were determined according to the absence (intermolt) or presence (premolt) of the line on the 5th pereiopod (Figure 1) and the ecdysteroid levels (intermolt = <60 ng/ml; premolt = >60 ng/ml, according to Techa and Chung, 2013 and Roegner et al., 2019). Both sexes were used since there were no sex-related differences in the chosen outputs. Animal maintenance and experimentation were authorized by the Brazilian Ministry of the Environment (license SISBIO number 67295–3).
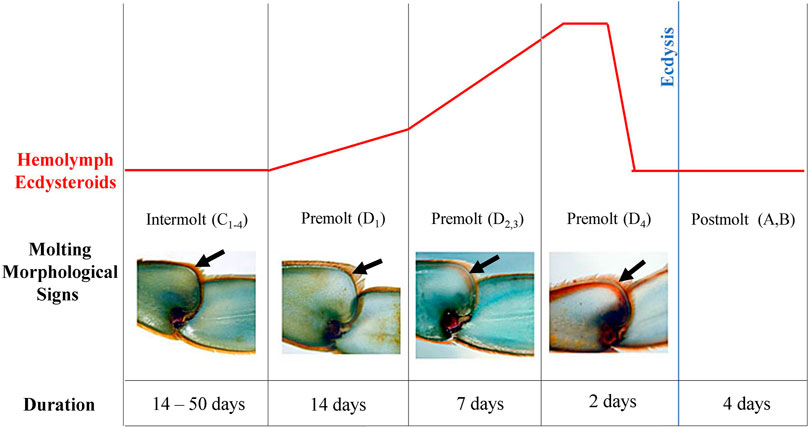
FIGURE 1. Illustration of molt cycle of the blue crab, Callinectes sapidus. The diagram shows the hemolymph ecdysteroid titer pattern (upper panel), morphological signals of the leg (black arrows), and duration of each stage during the molt cycle (bottom panel). Modified from Blue Crab. Info (https://www.bluecrab.info/redsign.htm).
2.2 Experimental Design
2.2.1 Hormone Concentrations and Gene Transcript Levels in Premolt and Intermolt C. sapidus
Premolt and intermolt animals previously identified and acclimated for at least 3 days under the conditions mentioned above were single housed in a closed circulation system for three more days. Samples were obtained every 8 h, at ZT1 (8 a.m.), ZT9 (4 p.m.), and ZT17 (midnight) (Figure 2A). Animals were cryo-anesthetized, the hemolymph (300 µL) was collected, centrifuged at 4,000 x g, at 4°C for 10 min, and the supernatant was stored at −80°C for later melatonin and ecdysteroid measurements; hepatopancreas and eyestalk were excised and stored at −80°C for qPCR analysis.
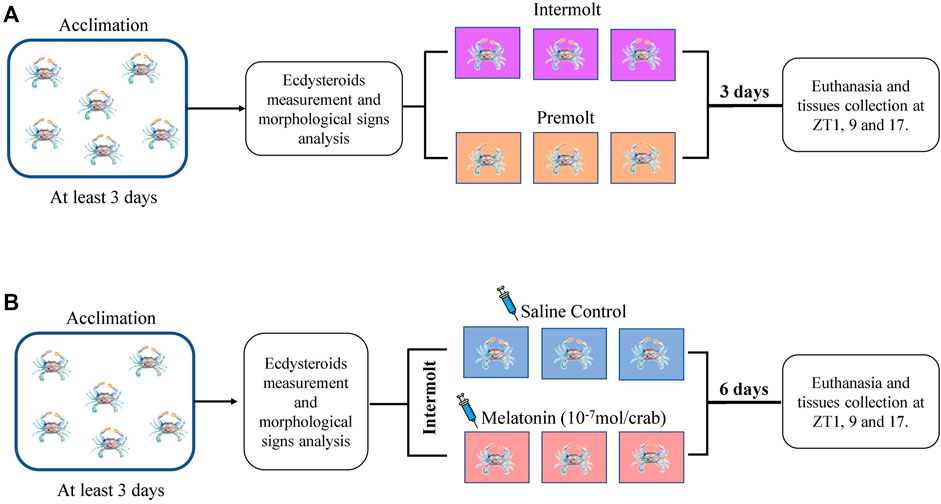
FIGURE 2. Experimental designs. (A) Timeline of the experiment to determine hemolymph ecdysteroids and gene expression of the intermolt and premolt crabs. (B) Timeline of the experiment to determine melatonin-injection effect on the hemolymph ecdysteroids and gene expression of the intermolt crabs.
2.2.2 Melatonin Effects on the Hormone Concentrations and Gene Transcript Levels in Intermolt C. sapidus
Melatonin (Sigma-Aldrich, St. Louis, MO, United States) was dissolved in 100% ethanol for a stock solution of 10−3 M, which was further diluted in 100 µL of Pantin solution (400 mM NaCl; 10 mM KCl; 27 mM anhydrous Na2SO4; 2.4 mM NaHCO3; 52.13 mM MgCl2.6H2O; 7.6 mM anhydrous CaCl2), pH 7.6, to obtain 10−7 mol/100 µL (Girish et al., 2015). This volume was daily injected at 1 p.m. (ZT6), into the arthrodial membrane of the 5th pereopod of intermolt crabs for 7 days. Control animals received the same volume of the eluent containing 1% ethanol. After that, the animals were processed as described above for hemolymph and organs collection (Figure 2B).
2.3 Measurement of Hormone Concentrations
Hemolymph ecdysteroid levels were determined by Enzyme-Linked Immunosorbent Assay (ELISA) adapted from McKinney et al. (2017). Melatonin quantification was also determined by ELISA according to the kit manufacturer’s instruction (IBL International, Flughafenstr, Hamburg, Germany).
2.4 Total Ribonucleic Acid Extraction and Reverse Transcriptase Reaction (Polymerase Chain Reaction)
The eyestalk and hepatopancreas were homogenized in Trizol (Ambion, Carlsbad, CA, United States), and after the extraction of total RNA, the samples were treated with DNAse I (turbo-DNase, Life Technologies, Carlsbad, CA, United States) following the manufacturer’s instructions. RNA concentration was determined in a Nanodrop spectrophotometer (Wilmington, DE, United States), and 1 μg of RNA was reverse transcribed in a reaction containing random hexamer primers, Superscript III (Life Technologies, Carlsbad, CA, United States), and other reagents, according to the manufacturer’s instructions.
2.5 Quantitative Polymerase Chain Reaction
Specific primers for the molt inhibiting hormone (CasMIH) and ecdysteroid receptor isoform (CasEcR) genes and ribosomal RPL12 (CasRPL12, utilized as normalizer), based on the GenBank sequences (http://www.ncbi.nlm.nih.gov/genbank), were designed with PrimerBlast (Table 1) and synthesized by IDT (Coralville, IA, United States). The single gene PCR reactions contained 300 nM of each primer, KAPA SYBR® Fast qPCR Mix 2x (KapaBiosystems, Wilmington, MA, United States), and DNase/RNase free water (Ambion, Carlsbad, CA, EUA). The assay was performed in the iQ5 thermocycler (Bio-Rad Laboratories, Hercules, CA, United States) as follows: 10 min at 95°C, followed by 45 cycles of 15 s at 95°C, 1 min at 60°C, and then 80 cycles of 10 s at 55°C, with a gradual increase of 0.5°C (melting curve to validate the specificity of the primers). For each gene, two replicates of each sample were used. Negative controls were routinely included with no template.
2.6 Statistical Analysis
The mRNA levels were calculated according to the 2-∆∆CT method (Livak and Schimittgen, 2001). The CT (amplification cycle) of each qPCR reaction was determined where the threshold crosses the geometrical portion of the amplification curves. ∆CT was found by subtracting CasRPL12 CT from each gene CT. The minimal mean value was then subtracted from each ∆CT sample value to obtain ∆∆CT, placed as the negative exponential in base 2.
The optical density (OD) values in the ELISA assays were interpolated in a 4 parameters standard curve for melatonin and semilog line standard curve for ecdysteroid, to obtain the concentration values which were expressed in pg/mL and ng/mL, respectively.
Outliers of the gene expression and hormone levels were identified using the ROUT method with Q = 10%, the Shapiro-Wilk test was used to check the normality, and the data were compared by Two-way ANOVA. To evaluate the influence of variables or the interaction between them, the F value was analyzed [F (DFn, DFd), p < 0.05], followed by Bonferroni’s post-test to compare the time points within a group, or the same time point among groups. Results are shown as median, quantiles, maximum, and minimum expression values of genes of interest, and p < 0.05 was established to reject the null hypothesis. All analyses were done in GraphPad Prism 8.0.
3 Results
3.1 Circulating Hormone Levels
Aiming to determine if ecdysteroid levels display a rhythm along the day, hemolymph of intermolt and premolt C. sapidus was collected and analyzed at three-time points (ZTs). We found a significant interaction between factors (molt stage and time) on the ecdysteroid levels (Table 2). As expected, ecdysteroids showed remarkably higher levels in premolt than intermolt animals, at all evaluated time points. Additionally, in the premolt crabs, the ecdysteroids showed a decrease at ZT17 in comparison to ZT1 (p = 0.0072) (Figure 3A).
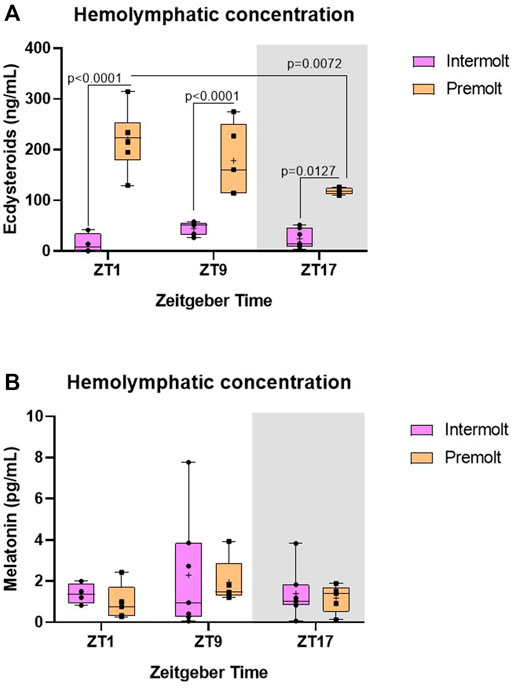
FIGURE 3. Concentration of ecdysteroids and melatonin in C. sapidus hemolymph. (A) Ecdysteroids (n = 4–7) and (B) melatonin (n = 4–7) were extracted 1, 9 and 17 h after lights on. The values are expressed as median, quantiles, maximum, and minimum concentration. p values refer to the differences between molt stages, or temporal points within a single molt group, as determined by Two-way ANOVA, followed by Bonferroni post-test. In this and the following figures, the gray rectangle represents the scotophase, and n is the animal number.
Melatonin shows a daily rhythm in most species. However, in C. sapidus our results showed no statistical differences between time points or molt stages (Table 2) where the circulating levels of melatonin were evaluated (Figure 3B).
3.2 Relative Gene Expression of CasEcR and CasMIH
Besides analyzing the daily temporal expression of the ecdysteroid receptor and MIH genes at three-time points over 24 h, the study enabled the comparison of two molt stages, intermolt and premolt.
The relative CasMIH expression in the eyestalk did not vary among time points in any molt stage. The F value from ANOVA indicates a significant impact of the molt stage (Table 2) and was confirmed by the post-test (p = 0.0288) at ZT9 (Figure 4A).
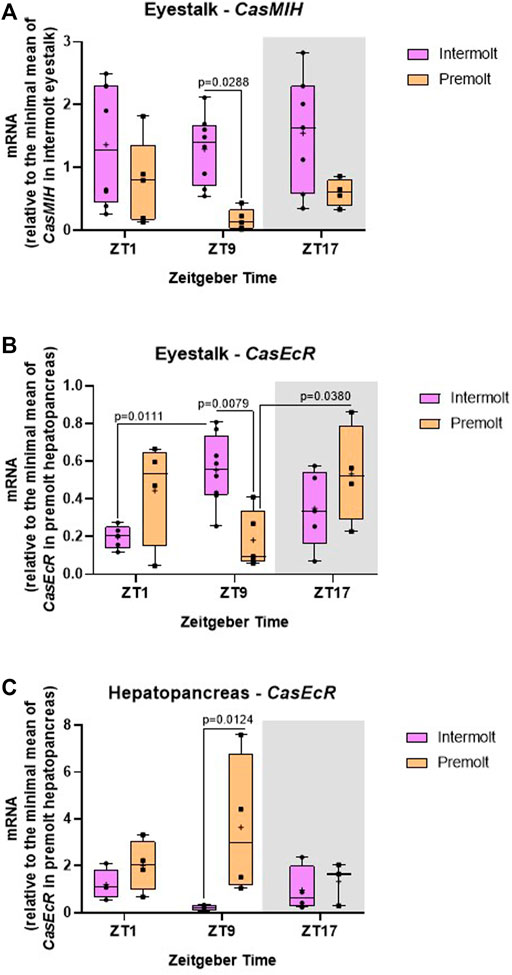
FIGURE 4. Temporal gene expression in intermolt and premolt C. sapidus. (A) CasMIH (n = 4–8) and (B) CasEcR (n = 4–8) in the eyestalks, and (C) CasEcR (n = 3–4) in the hepatopancreas. Total RNA was extracted 1, 9, and 17 h after lights on. Gene expression was normalized by CasRPL12 and the values of CasEcR and CasMIH were expressed relative to the minimal mean of CasEcR in premolt hepatopancreas and of CasMIH in intermolt eyestalk, respectively. p values refer to the differences between molt stages, or temporal points within a single molt group, as determined by Two-way ANOVA, followed by Bonferroni post-test.
We found a significant interaction between time and molt stage (Table 2) in CasEcR expression in the eyestalk. This gene exhibited a rhythm in both stages, with different patterns. Significant difference was observed at ZT9 between molt stages, with higher expression in the intermolt compared to premolt crabs (p = 0.079) (Figure 4B).
The hepatopancreas CasEcR showed a significant influence of the molt stage on its expression (Table 2). No variation among the time points was observed, however, a significant increase of CasEcR transcripts was seen at ZT9 in premolt compared to intermolt animals (p = 0.0124) (Figure 4C).
3.3 Effects of Exogenous Melatonin on the Gene Expression and Ecdysteroid Levels
Considering the possible effect of melatonin on the molt cycle, we analyzed the ecdysteroid levels and the relative expression of CasEcR and CasMIH genes in daily melatonin-injected intermolt crabs.
No statistical differences were observed in the ecdysteroids levels between control and melatonin-treated animals (Figure 5). At ZT17 the ecdysteroid levels tend to decrease in the hormone-treated crabs, however, this reduction was not statistically significant (Table 3).
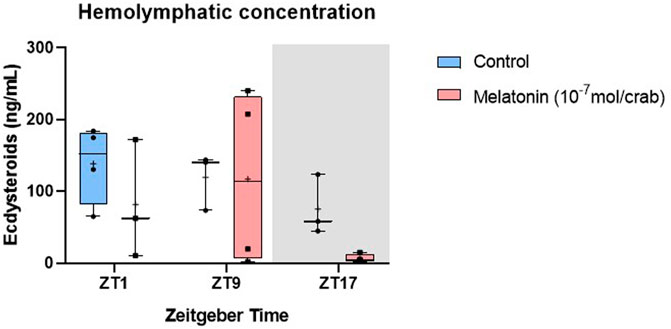
FIGURE 5. Concentration of ecdysteroids in intermolt C. sapidus hemolymph after daily injections of 10−7 mol melatonin/animal for 7 days. Ecdysteroids (n = 3–4) were extracted 1, 9 and 17 h after lights on. The values are expressed as median, quantiles, maximum, and minimum concentration.

TABLE 3. ANOVA parameters related to gene expression and hormone concentrations in melatonin-injected crabs.
In the eyestalk, the melatonin injection elicited a significant decrease in CasMIH expression (Table 3). This reduction was confirmed by the post-test at ZT17 (p = 0.0045) (Figure 6A). No oscillation in either molt group was found.
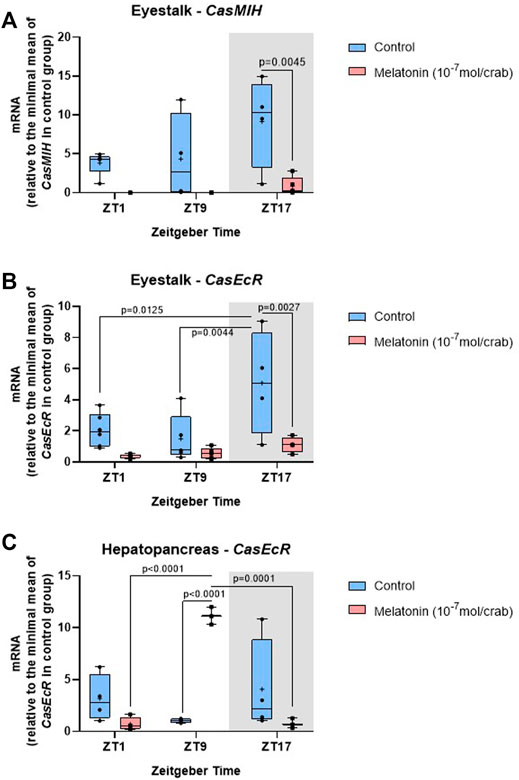
FIGURE 6. Temporal gene expression in intermolt C. sapidus after daily injections of 10−7 mol melatonin/animal for 7 days. (A) CasMIH (n = 3–5) and (B) CasEcR (n = 4–6) in the eyestalks, and (C) CasEcR (n = 3–4) in the hepatopancreas. Total RNA was extracted 1, 9, and 17 h after lights on. Gene expression was normalized by CasRPL12, and the values were expressed relative to the minimal mean of a control in each graph. p values refer to the differences between molt stages, or temporal points within a single molt group, as determined by Two-way ANOVA, followed by Bonferroni post-test.
The F value indicates a significant impact of both factors, time and treatment in the eyestalk CasEcR expression (Table 3). Control animals showed an oscillatory profile with a peak of expression at ZT17, which was abolished in the melatonin-injected animals. At the same time point, the gene expression was reduced in melatonin-treated animals compared to the control crabs (p = 0.0027) (Figure 6B).
An interaction between time and melatonin treatment was observed in the hepatopancreas showing a significant influence on the CasEcR transcripts (Table 3). The treated group displayed an oscillation with a peak at ZT9 in comparison to ZT1 and ZT17. In addition, a significantly higher CasEcR expression at ZT9 in melatonin-treated animals than in the control group was observed (p < 0.0001) (Figure 6C).
4 Discussion
Reports about diurnal oscillation of molt cycle-related genes are extremely rare in decapod crustaceans. Because this is an overly complex physiological process, which comprises several stages and phases with a variety of hormones (Lipcius and Herrnkind, 1982; Lachaise et al., 1993; Chang, 1995; Nakatsuji et al., 2009; Chang and Mykles, 2011; Mykles, 2011; Webster et al., 2012), a thorough comprehensive analysis becomes exceedingly difficult. In addition, many environmental factors affect hormone synthesis and secretion, and gene expression, thus strongly influencing the molt cycle (Guo et al., 2013; Stoner et al., 2013; Gong et al., 2015).
Ecdysteroids, the main hormones responsible for ecdysis, are synthesized and secreted by the Y-organ, under the molt-inhibiting hormone negative regulation. The first aim addressed in this study was to evaluate ecdysteroid levels, ecdysteroid receptors, and the molt-inhibiting hormone expression, in two molt stages and at three time-points of the day, events not yet reported for Callinectes sapidus.
Due to the ecdysteroid ecdysis-inducing action, higher hormone levels are expected in premolt animals. Our data confirm the literature studies, which report that premolt animals have a higher concentration in comparison to intermolt animals at all evaluated ZTs (Lee et al., 1998; Nakatsuji et al., 2000; Nakatsuji and Sonobe, 2004; Techa and Chung, 2013). Moreover, a temporal variation in premolt crabs was noted with a reduction of the ecdysteroid levels at ZT17.
For gene expression, molt stage (eyestalk CasMIH and hepatopancreas CasEcR) or their interaction (eyestalk CasEcR) showed significant results. Eyestalk holds the visual ganglia and is responsible for the synthesis of circadian molecules such as melatonin in many crustaceans, for example, Eriocheir sinensis and Palaemonetes sinensis (Han et al., 2018), and Neohelice granulata (Maciel et al., 2008). The X-organ-sinus gland complex seems to be the master of this rhythmicity (Aréchiga et al., 1985; 1993) and it is under the direct influence of light (Glantz et al., 1983), which would impact the daily variation of the hormones and gene expression evaluated in this work. In fact, our results showed that CasEcR oscillates along the time in the eyestalk of intermolt and premolt animals, although with different patterns. On the other hand, CasMIH did not display the same profile. Bearing that in mind, one may suggest that the synthesis and release of some hormones exhibit a diurnal profile in the eyestalk, but not necessarily the expression of CasMIH. Besides that, the variation among individuals may mask a possible oscillatory profile.
The relative expression of CasEcR and CasMIH in the eyestalk shows a similar profile: Both genes displayed higher expression at ZT9 in the intermolt than in premolt crabs. Previous studies showed that ecdysteroid receptors and CasMIH gene are related in the eyestalk since EcR is a nuclear receptor, that possesses a binding site in the MIH promoter and then influences its expression.
The hepatopancreas is one of the main targets of ecdysteroids, whose levels are high in the premolt stage, preceding the ecdysis. This organ plays a particularly important role in metabolic regulation, and it is responsible for calcium storage, which is fundamental for a successful molt cycle (Becker et al., 1974; Zanotto and Wheatly, 2002). As feeding behavior changes along the molt cycle, it is expected that the organ’s cell composition undergoes modifications to meet the nutritional demand during the cycle (Lipcius and Herrnkind, 1982; Ortega et al., 2011). Increased CasEcR expression in the hepatopancreas has been reported in response to ecdysis induction (Girish et al., 2015).
In our results, there was an increase of hepatopancreas CasEcR transcripts in the premolt animals, which may be related to the receptor function as ecdysis comes closer and ecdysteroid concentration rises in the hemolymph. However, this increase was statistically significantly only at ZT9, suggesting a possible rhythm of the CasEcR. In Rhodnius prolixus, ecdysone receptors exhibit a circadian rhythm in specific tissues in response or anticipation of the daily peaks of the hormone (Vafopoulou and Steel, 2006). In C. sapidus, we demonstrated that the ecdysteroid levels were higher at ZT1 and ZT9, and the increase of CasEcR transcripts at ZT9 may be in response to an increase of circulating ecdysteroids.
Ecdysteroids, MIH, methyl farnesoate, and its inhibitor are classic regulators of the molt cycle (Borst et al., 2001; Nagaraju, 2007; 2011). A strong candidate as a positive factor is melatonin, whose functions in the vertebrates are linked to its pattern of synthesis and release in the dark (Carrilo-Vico et al., 2005; Markus and Ferreira, 2011; Cipolla-Neto et al., 2014) since the synthesis key enzyme is inhibited by light, even in nocturnal species (Reiter et al., 2014).
One point to highlight is that, unlike the majority of vertebrates, melatonin concentration peak in crustaceans may vary according to the species: during the photophase, as in Procambarus clarkii (Agapito et al., 1995), Uca pugilator (Tilden et al., 2001) and Daphnia magna (Markowska et al., 2009), or in both the photophase and the scotophase as in N. granulata (Maciel et al., 2008). In C. sapidus, circulating melatonin did not exhibit a temporal oscillation in intermolt or premolt crabs, and no concentration difference was seen between stages. However, preliminary data with more time points evaluated every 4 h, along the 24 h (data not shown) suggest that both, ecdysteroids and melatonin, display an increase at ZT9.
In crustaceans, light does not seem to affect melatonin synthesis: C. sapidus and other species presented the highest concentrations during the photophase, suggesting that factors other than light regulate melatonin production in these animals. Unfortunately, most reports about melatonin concentration in crustacean hemolymph did not mention the stage of the molt cycle, temperature, and light conditions, which may be the reason for the inconsistencies seen in the literature. In our study, we focused on two stages of the molt cycle, and kept constant light-dark cycle, temperature, and water conditions, to clarify the controversial data about melatonin rhythmicity in crustaceans. Nevertheless, further experiments should be performed to confirm this finding.
To analyze a possible positive effect of melatonin on the molt cycle, the intermolt crabs were daily injected with melatonin for 7 days at ZT6 and the ecdysteroid levels, ecdysteroid receptor, and MIH expression were determined. The reason to use intermolt crabs was to verify whether melatonin treatment would induce the ecdysis; the injection time was based on the majority of the literature reports which claim that melatonin peaks in the photophase.
The ecdysteroid levels did not show variation, but a reduction at ZT17 in melatonin-treated animals was visible; but probably due to the individual variability the difference was not statistically significant. In our hypothesis, melatonin should evoke the same effect as methyl farnesoate on the molt cycle (Reddy et al., 2004); in other words, we expected a melatonin-induced increase in the production and secretion of ecdysteroids. A possibility is that the melatonin injections were sufficient to induce a later stage of premolt, where the ecdysteroid levels were reduced in C. sapidus.
Our results revealed a similar expression pattern of both genes in the eyestalk, where exogenous melatonin inhibited their transcription at ZT17, whereas, in the hepatopancreas, melatonin increased CasEcR expression at ZT9, suggesting organ-specific melatonin receptors.
The eyestalk X-organ/sinus gland complex synthesizes and stores MIH, respectively, a hormone required during the later stages, posterior to ecdysis, the postmolt and intermolt stages (Philippen et al., 2000; Nakatsuji et al., 2009). Nevertheless, it has been reported that in some species MIH is synthesized in the premolt stage, stored in the sinus gland until the releasing time, but never with higher levels than in the postmolt and intermolt periods (Nakatsuji et al., 2000). One has also to consider the interaction between ecdysteroid receptor and MIH in the eyestalk, where CasEcR may be acting in the later premolt stage before ecdysis. Techa and Chung (2015) suggested that ecdysteroids exert positive feedback on the MIH expression: after reaching certain levels, the ecdysteroids inhibit the molt cycle via EcR stimulation of MIH transcripts. Bearing this in mind, one may suggest a positive action of melatonin on the molt cycle, in which the MIH inhibition may emanate from CasEcR inhibition. We hypothesized that melatonin acts as a positive regulator of the molt cycle through an inhibitory action on the molt inhibitor hormone in the eyestalk as discussed above.
Ecdysteroid receptors in the target organs, such as the hepatopancreas, display distinct functions from those in the eyestalk since the signaling in the hepatopancreas could induce ecdysis (Shechter et al., 2007; Huang et al., 2015). In fact, as to the hepatopancreas, melatonin-treated crabs, which were in the intermolt stage, showed an increase in CasEcR levels at ZT9; in addition, this gene exhibited an oscillation in treated animals. It would be expected that the expression of the ecdysteroid receptors increases with the melatonin treatment. Interestingly, melatonin-injected intermolt crabs showed CasEcR expression similar to premolt crabs (Figure 4C), corroborating the hypothesis that melatonin has a positive effect on the molt cycle.
Taken altogether, our results demonstrated the lack of temporal variation in CasMIH gene expression in premolt and intermolt eyestalk animals. On the other hand, CasEcR displayed an oscillatory profile with a peak at ZT9 in the eyestalk of intermolt animals; in the hepatopancreas, this gene seems to oscillate in premolt animals. Importantly, our study highlights important limitations in the literature regarding melatonin secretion rhythm in crustaceans. In C. sapidus, we are the first to demonstrate melatonin presence and how this hormone behaves in premolt and intermolt stages.
We also demonstrated that exogenous melatonin has a positive effect on the molt cycle, leading to a gene expression in intermolt animals’ pattern similar to the one found in premolt crabs, probably affecting eyestalk and hepatopancreas physiology. In the eyestalk, we found an indirect activation of the molt cycle (through CasMIH and CasEcR inhibition) whereas in the hepatopancreas the indoleamine increased the expression of CasEcR. Ultimately, our results widened the knowledge about the influence of melatonin on molt-related genes and daily hormone variation, bringing an essential contribution to the field of comparative endocrinology.
Data Availability Statement
The original contributions presented in the study are included in the article/Supplementary Materials, further inquiries can be directed to the corresponding author.
Author Contributions
DDD, FPZ, and AMC conceptualized the study and the original hypothesis; DDD performed the experiments and, together with LDA and MNM, analyzed the data; AMC supervised the study, ensuring rigorous data quality control, and contributed to the discussion together with DDD, LDA, and MNM; DDD wrote the first draft of manuscript, which was critically revised by LDA, MNM, FPZ, and AMC. All authors have approved the definitive version of the manuscript and agreed to be accountable for all aspects of the study in ensuring the accuracy and integrity of any part of the study.
Funding
This work was supported by the Sao Paulo Research Foundation (FAPESP, grants 2012/50214-4, 2017/24615-5, and 2018/14728-0; the National Council of Technological and Scientific Development (CNPq, grants 303078/2019-7 and 428754/2018-0 and Coordination for the Improvement of Higher Education Personnel (CAPES)—Finance code—001. MNM is a Young Investigator of FAPESP (2017/26651-9 and 2019/19005-9), DDD is a fellow of FAPESP (2018/23043-0), and LDA was a fellow of FAPESP (2018/16511-8).
Conflict of Interest
The authors declare that the research was conducted in the absence of any commercial or financial relationships that could be construed as a potential conflict of interest.
Publisher’s Note
All claims expressed in this article are solely those of the authors and do not necessarily represent those of their affiliated organizations, or those of the publisher, the editors and the reviewers. Any product that may be evaluated in this article, or claim that may be made by its manufacturer, is not guaranteed or endorsed by the publisher.
Acknowledgments
We are thankful to Nelson Pereira and Denise Prete for providing the crabs whenever needed.
References
Agapito M. T., Herrero B., Pablos M. I., Miguel J. L., Recio J. M. (1995). Circadian Rhythms of Melatonin and Serotonin-N-Acetyltransferase Activity in Procambarus clarkii. Comp. Biochem. Physiology Part A Physiology 112 (1), 179–185. doi:10.1016/0300-9629(95)00071-e
Aréchiga H., Cortes J. L., Garcia U., Rodriguez-sosa L. (1985). Neuroendocrine Correlates of Circadian Rhythmicity in Crustaceans. Am. Zool. 25 (1), 265–274. doi:10.1093/icb/25.1.265
Aréchiga H., Fernéndez-Quiróz F., de Miguel F. F., Rodríguez-Sosa L. (1993). The Circadian System of Crustaceans. Chronobiology Int. 10 (1), 1–19. doi:10.3109/07420529309064477
Asazuma H., Nagata S., Nagasawa H. (2009). Inhibitory Effect of Molt-Inhibiting Hormone Onphantomexpression in the Y-Organ of the Kuruma Prawn,Marsupenaeus japonicus. Arch. Insect Biochem. Physiol. 72 (4), 220–233. doi:10.1002/arch.20335
Axelrod J., Weissbach H. (1960). Enzymatic O-Methylation of N-Acetylserotonin to Melatonin. Science 131 (3409), 1312. doi:10.1126/science.131.3409.1312
Becker G. L., Chen C.-H., Greenawalt J. W., Lehninger A. L. (1974). Calcium Phosphate Granules in the Hepatopancreas of the Blue Crab Callinectes sapidus. J. Cell. Biol. 61, 316–326. doi:10.1083/jcb.61.2.316
Böcking D., Dauphin-Villemant C., Lafont R. (1995). Metabolism of 3-dehydroecdysone in the Crayfish Orconectes Limosus (Crustacea: Decapoda). Eur. J. Entomol. 92 (1), 63–74.
Borst D. W., Ogan J., Tsukimura B., Claerhout T., Holford K. C. (2001). Regulation of the Crustacean Mandibular Organ. Am. Zool. 41 (3), 430–441. doi:10.1093/icb/41.3.430
Carrillo-Vico A., Guerrero J., Lardone P., Reiter R. (2005). A Review of the Multiple Actions of Melatonin on the Immune System. Endocrine 27 (2), 189–200. doi:10.1385/ENDO:27:2:189
Chang E. S., Mykles D. L. (2011). Regulation of Crustacean Molting: A Review and Our Perspectives. General Comp. Endocrinol. 172, 323–330. doi:10.1016/j.ygcen.2011.04.003
Chang E. S. (1995). Physiological and Biochemical Changes during the Molt Cycle in Decapod Crustaceans: an Overview. J. Exp. Mar. Biol. Ecol. 193, 1–14. doi:10.1016/0022-0981(95)00106-9
Chen X., Wang J., Yue W., Huang S., Chen J., Chen Y., et al. (2017). Structure and Function of the Alternatively Spliced Isoforms of the Ecdysone Receptor Gene in the Chinese Mitten Crab, Eriocheir Sinensis. Sci. Rep. 7 (1), 1–12. doi:10.1038/s41598-017-13474-1
Chung J. S. (2010). Hemolymph Ecdysteroids during the Last Three Molt Cycles of the Blue Crab, Callinectes sapidus: Quantitative and Qualitative Analyses and Regulation. Arch. Insect Biochem. Physiol. 73 (1), 1–13. doi:10.1002/arch.20327
Cipolla-Neto J., Amaral F. G., Afeche S. C., Tan D. X., Reiter R. J. (2014). Melatonin, Energy Metabolism, and Obesity: a Review. J. Pineal Res. 56, 371–381. doi:10.1111/jpi.12137
Cipolla-Neto J., Amaral F. G. d. (2018). Melatonin as a Hormone: New Physiological and Clinical Insights. Endocr. Rev. 39 (6), 990–1028. doi:10.1210/er.2018-00084
Dardente H. (2012). Melatonin-dependent Timing of Seasonal Reproduction by the Pars Tuberalis: Pivotal Roles for Long Daylengths and Thyroid Hormones. J. Neuroendocrinol. 24 (2), 249–266. doi:10.1111/j.1365-2826.2011.02250.x
Espinosa-Chaurand D., Vega-Villasante F., Carrillo-Farnés O., Nolasco-Soria H. (2017). Effect of Circadian Rhythm, Photoperiod, and Molt Cycle on Digestive Enzymatic Activity of Macrobrachium Tenellum Juveniles. Aquaculture 479, 225–232. doi:10.1016/j.aquaculture.2017.05.029
Geihs M. A., Vargas M. A., Maciel F. E., Caldas S. S., Cruz B. P., Primel E. G., et al. (2010). Effect of Melatonin in the Antioxidant Defense System in the Locomotor Muscles of the Estuarine Crab Neohelice Granulata (Decapoda, Brachyura). General Comp. Endocrinol. 166 (1), 72–82. doi:10.1016/j.ygcen.2009.09.018
Geihs M. A., Vargas M. A., Maciel F. E., Vakkuri O., Meyer-Rochow V. B., Allodi S., et al. (2016). Effects of Hypoxia and Reoxygenation on the Antioxidant Defense System of the Locomotor Muscle of the Crab Neohelice Granulata (Decapoda, Varunidae). J. Comp. Physiol. B 186 (5), 569–579. doi:10.1007/s00360-016-0976-2
Girish B. P., Swetha C., Reddy P. S. (2015). Induction of Ecdysteroidogenesis, Methyl Farnesoate Synthesis and Expression of Ecdysteroid Receptor and Retinoid X Receptor in the Hepatopancreas and Ovary of the Giant Mud Crab, Scylla serrata by Melatonin. General Comp. Endocrinol. 217-218, 37–42. doi:10.1016/j.ygcen.2015.05.007
Glantz R. M., Kirk M. D., Are´chiga H. (1983). Light Input to Crustacean Neurosecretory Cells. Brain Res. 265 (2), 307–311. doi:10.1016/0006-8993(83)90347-5
Gong J., Yu K., Shu L., Ye H., Li S., Zeng C. (2015). Evaluating the Effects of Temperature, Salinity, Starvation and Autotomy on Molting Success, Molting Interval and Expression of Ecdysone Receptor in Early Juvenile Mud Crabs, Scylla Paramamosain. J. Exp. Mar. Biol. Ecol. 464, 11–17. doi:10.1016/j.jembe.2014.12.008
Guo B., Wang F., Li Y., Dong S. (2013). Effect of Periodic Light Intensity Change on the Molting Frequency and Growth of Litopenaeus Vannamei. Aquaculture 396-399, 66–70. doi:10.1016/j.aquaculture.2013.02.033
Han Z., Li X., Li X., Xu W., Li Y. (2018). Circadian Rhythms of Melatonin in Haemolymph and Optic Lobes of Chinese Mitten Crab (Eriocheir Sinensis) and Chinese Grass Shrimp (Palaemonetes Sinensis). Biol. Rhythm Res. 50 (3), 400–407. doi:10.1080/09291016.2018.1452592
Hopkins P. M. (2012). The Eyes Have it: A Brief History of Crustacean Neuroendocrinology. General Comp. Endocrinol. 175, 357–366. doi:10.1016/j.ygcen.2011.12.002
Huang S., Wang J., Yue W., Chen J., Gaughan S., Lu W., et al. (2015). Transcriptomic Variation of Hepatopancreas Reveals the Energy Metabolism and Biological Processes Associated with Molting in Chinese Mitten Crab, Eriocheir Sinensis. Sci. Rep. 5 (1), 1–11. doi:10.1038/srep14015
Katayama H. (2016). Structure-activity Relationship of Crustacean Peptide Hormones. Biosci 80 (4), 633–641. doi:10.1080/09168451.2015.1116932
Kuballa A., Elizur A. (2007). Novel Molecular Approach to Study Moulting in Crustaceans. Bull. Fish. Res. Agen. 20, 53–57.
Lachaise F., Roux A. L., Hubert M., Lafont R. (1993). The Molting Gland of Crustaceans: Localization, Activity, and Endocrine Control (A Review). J. Crustacean Biol. 13 (2), 198–234. doi:10.1163/193724093X00020
Lee K. J., Watson R. D., Roer R. D. (1998). Molt-Inhibiting Hormone mRNA Levels and Ecdysteroid Titer during a Molt Cycle of the Blue Crab,Callinectes sapidus. Biochem. Biophysical Res. Commun. 249, 624–627. doi:10.1006/bbrc.1998.9215
Lipcius R. N., Herrnkind W. F. (1982). Molt Cycle Alterations in Behavior, Feeding and Diel Rhythms of a Decapod Crustacean, the Spiny Lobster Panulirus Argus. Mar. Biol. 68 (3), 241–252. doi:10.1007/bf00409591
Liu L., Fu Y., Xiao L., Liu X., Fang W., Wang C. (2021). iTRAQ-Based Quantitative Proteomic Analysis of the Hepatopancreas in Scylla Paramamosain during the Molting Cycle. Comp. Biochem. Physiology Part D Genomics Proteomics 40, 100870. doi:10.1016/j.cbd.2021.100870
Livak K. J., Schmittgen T. D. (2001). Analysis of Relative Gene Expression Data Using Real-Time Quantitative PCR and the 2−ΔΔCT Method. Methods 25 (4), 402–408. doi:10.1006/meth.2001.1262
Loeb M. J. (1993). Hormonal Control of Growth and Reproduction in the Arthropods: Introduction to the Symposium. Am. Zool. 33, 303–307. doi:10.1093/icb/33.3.303
Luo X., Chen T., Zhong M., Jiang X., Zhang L., Ren C., et al. (2015). Differential Regulation of Hepatopancreatic Vitellogenin (VTG) Gene Expression by Two Putative Molt-Inhibiting Hormones (MIH1/2) in Pacific White Shrimp (Litopenaeus Vannamei). Peptides 68, 58–63. doi:10.1016/j.peptides.2014.11.002
Maciel F. E., Geihs M. A., Vargas M. A., Cruz B. P., Ramos B. P., Vakkuri O., et al. (2008). Daily Variation of Melatonin Content in the Optic Lobes of the Crab Neohelice Granulata. Comp. Biochem. Physiology Part A Mol. Integr. Physiology 149 (2), 162–166. doi:10.1016/j.cbpa.2007.11.007
Maciel F. E., Ramos B. P., Geihs M. A., Vargas M. A., Cruz B. P., Meyer-Rochow V. B., et al. (2010). Effects of Melatonin in Connection with the Antioxidant Defense System in the Gills of the Estuarine Crab Neohelice Granulata. General Comp. Endocrinol. 165 (2), 229–236. doi:10.1016/j.ygcen.2009.07.009
Maciel F., Geihs M., Cruz B., Vargas M., Allodi S., Marins L., et al. (2014). Melatonin as a Signaling Molecule for Metabolism Regulation in Response to Hypoxia in the Crab Neohelice Granulata. Ijms 15 (12), 22405–22420. doi:10.3390/ijms151222405
Markowska M., Bentkowski P., Kloc M., Pijanowska J. (2009). Presence of Melatonin inDaphnia Magna. J. Pineal Res. 46 (2), 242–244. doi:10.1111/j.1600-079X.2008.00642.x
Markus R. P., Ferreira Z. S. (2011). The Immune-Pineal Axis: the Role of Pineal and Extra-Pineal Melatonin in Modulating Inflammation. Adv. Neuroimmune Biol. 1, 95–104. doi:10.3233/nib-2011-009
McKinney D. A., Strand M. R., Brown M. R. (2017). Evaluation of Ecdysteroid Antisera for a Competitive Enzyme Immunoassay and Extraction Procedures for the Measurement of Mosquito Ecdysteroids. General Comp. Endocrinol. 253, 60–69. doi:10.1016/j.ygcen.2017.08.028
Mykles D. L. (2011). Ecdysteroid Metabolism in Crustaceans. J. Steroid Biochem. Mol. Biol. 127 (3-5), 196–203. doi:10.1016/j.jsbmb.2010.09.001
Nagaraju G. P. C. (2007). Is Methyl Farnesoate a Crustacean Hormone? Aquaculture 272 (1-4), 39–54. doi:10.1016/j.aquaculture.2007.05.014
Nagaraju G. P. C., Reddy P. R., Reddy P. S. (2006). In Vitro methyl Farnesoate Secretion by Mandibular Organs Isolated from Different Molt and Reproductive Stages of the Crab Oziotelphusa Senex Senex. Fish. Sci. 72, 410–414. doi:10.1111/j.1444-2906.2006.01164.x
Nagaraju G. P. C. (2011). Reproductive Regulators in Decapod Crustaceans: An Overview. J. Exp. Biol. 214, 3–16. doi:10.1242/jeb.047183
Nakatsuji T., Keino H., Tamura K., Yoshimura S., Kawakami T., Aimoto S., et al. (2000). Changes in the Amounts of the Molt-Inhibiting Hormone in Sinus Glands during the Molt Cycle of the American Crayfish, Procambarus clarkii. Zoological Sci. 17 (8), 1129–1136. doi:10.2108/zsj.17.1129
Nakatsuji T., Lee C.-Y., Watson R. D. (2009). Crustacean Molt-Inhibiting Hormone: Structure, Function, and Cellular Mode of Action. Comp. Biochem. Physiology Part A Mol. Integr. Physiology 152 (2), 139–148. doi:10.1016/j.cbpa.2008.10.012
Nakatsuji T., Sonobe H. (2004). Regulation of Ecdysteroid Secretion from the Y-Organ by Molt-Inhibiting Hormone in the American Crayfish, Procambarus clarkii. General Comp. Endocrinol. 135, 358–364. doi:10.1016/j.ygcen.2003.11.001
Nery E. L. M., da Silva M. A., Castrucci A. M. L. (1999). Possible Role of Non-Classical Chromatophorotropins on the Regulation of the Crustacean Erythrophore. J. Exp. Zool. 284 (6), 711–716. doi:10.1002/(sici)1097-010x(19991101)284:6
Ortega P., e Sá M. G., Custódio M. R., Zanotto F. P. (2011). Separation and Viability of Gill and Hepatopancreatic Cells of a Mangrove Crab Ucides Cordatus. Vitro Cell.Dev.Biol.-Animal 47 (5-6), 346–349. doi:10.1007/s11626-011-9402-y
Philippen M. K., Webster S. G., Chung J. S., Dircksen H. (2000). Ecdysis of Decapod Crustaceans is Associated With a Dramatic Release of Crustacean Cardioactive Peptide into the Haemolymph. J. Exp. Biol. 203 (3), 521–536. doi:10.1242/jeb.203.3.521
Reddy P. R., Nagaraju G. P. C., Reddy P. S. (2004). Involvement of Methyl Farnesoate in the Regulation of Molting and Reproduction in the Freshwater Crab Oziotelphusa Senex Senex. J. Crust. Biol. 24 (3), 511–515. doi:10.1651/C-2478
Reiter R. J., Tan D.-X., Manchester L. C., Paredes S. D., Mayo J. C., Sainz R. M. (2009). Melatonin and Reproduction Revisited. Biol. Reprod. 81 (3), 445–456. doi:10.1095/biolreprod.108.075655
Reiter R. J., Tan D. X., Galano A. (2014). Melatonin: Exceeding Expectations. Physiology 29 (5), 325–333. doi:10.1152/physiol.00011.2014
Roegner M. E., Roer R. D., Watson R. D. (2019). Sarco/endoplasmic Reticulum Ca2+ ATPase (SERCA) Transcript Abundance in Y-Organs and Ecdysteroid Titer in Hemolymph during a Molting Cycle of the Blue Crab, Callinectes sapidus. Comp. Biochem. Physiology Part A Mol. Integr. Physiology 229, 76–80. doi:10.1016/j.cbpa.2018.12.006
Sainath S. B., Reddy P. S. (2010a). Melatonergic Regulation of Hemolymph Sugar Levels in the Freshwater Edible Crab, Oziotelphusa Senex Senex. J. Exp. Zool. A Ecol. Genet. Physiol. 313 (4), 201–208. doi:10.1002/jez.5910.1002/jez.594
Sainath S. B., Reddy P. S. (2010b). Evidence for the Involvement of Selected Biogenic Amines (Serotonin and Melatonin) in the Regulation of Molting of the Edible Crab, Oziotelphusa Senex Senex Fabricius. Aquaculture 302 (3-4), 261–264. doi:10.1016/j.aquaculture.2010.02.025
Sainath S. B., Swetha C., Reddy P. S. (2013). What Do We (Need to) Know about the Melatonin in Crustaceans? J. Exp. Zool. 319 (7), 365–377. doi:10.1002/jez.1800
She Q., Han Z., Liang S., Xu W., Li X., Zhao Y., et al. (2019). Impacts of Circadian Rhythm and Melatonin on the Specific Activities of Immune and Antioxidant Enzymes of the Chinese Mitten Crab (Eriocheir Sinensis). Fish Shellfish Immunol. 89, 345–353. doi:10.1016/j.fsi.2019.04.011
Shechter A., Tom M., Yudkovski Y., Weil S., Chang S. A., Chang E. S., et al. (2007). Search for Hepatopancreatic Ecdysteroid-Responsive Genes during the Crayfish Molt Cycle: from a Single Gene to Multigenicity. J. Exp. Biol. 210 (20), 3525–3537. doi:10.1242/jeb.006791
Spaziani E., DeSantis K., O’Rourke W. L., Wang J. D., O'Rourke B. D., Wang W. L., et al. (1997). The Clearance In Vivo and Metabolism of Ecdysone and 3-dehydroecdysone in Tissues of the crabCancer Antennarius. J. Exp. Zool. 279, 609–619. doi:10.1002/(sici)1097-010x(19971215)279:6<609::aid-jez9>3.0.co;2-r
Spaziani E., Mattson M. P., Wang W. L., McDougall H. E. (1999). Signaling Pathways for Ecdysteroid Hormone Synthesis in Crustacean Y-Organs. Am. Zool. 39 (3), 496–512. doi:10.1093/icb/39.3.496
Spaziani E., Wang W. L. (1993). Biosynthesis of Ecdysteroid Hormones by Crustacean Y-Organs: Conversion of Cholesterol to 7-dehydrocholesterol Is Suppressed by a Steroid 5α-Reductase Inhibitor. Mol. Cell. Endocrinol. 95, 111–114. doi:10.1016/0303-7207(93)90035-i
Spindler K.-D., Adelung D., Tchernigovtzeff C. (1974). A Comparison of the Methods of Molt Staging According to Drach and to Adelung in the Common Shore Crab, Carcinus maenas. Z. Naturforsch. C. Biosci. 29 (11-12), 754–756. doi:10.1515/znc-1974-11-1217
Stoner A. W., Copeman L. A., Ottmar M. L. (2013). Molting, Growth, and Energetics of Newly-Settled Blue King Crab: Effects of Temperature and Comparisons with Red King Crab. J. Exp. Mar. Biol. Ecol. 442, 10–21. doi:10.1016/j.jembe.2013.02.002
Su Y., Guo Q., Gong J., Cheng Y., Wu X. (2020). Functional Expression Patterns of Four Ecdysteroid Receptor Isoforms Indicate Their Different Functions during Vitellogenesis of Chinese Mitten Crab, Eriocheir Sinensis. Comp. Biochem. Physiology Part A Mol. Integr. Physiology 248, 110754. doi:10.1016/j.cbpa.2020.110754
Tan D.-X., Hardeland R., Back K., Manchester L. C., Alatorre-Jimenez M. A., Reiter R. J. (2016). On the Significance of an Alternate Pathway of Melatonin Synthesis via 5-methoxytryptamine: Comparisons across Species. J. Pineal Res. 61 (1), 27–40. doi:10.1111/jpi.12336
Techa S., Chung J. S. (2013). Ecdysone and Retinoid-X Receptors of the Blue Crab, Callinectes sapidus: Cloning and Their Expression Patterns in Eyestalks and Y-Organs during the Molt Cycle. Gene 527 (1), 139–153. doi:10.1016/j.gene.2013.05.035
Techa S., Chung J. S. (2015). Ecdysteroids Regulate the Levels of Molt-Inhibiting Hormone (MIH) Expression in the Blue Crab, Callinectes sapidus. Plos One 10 (4), e0117278. doi:10.1371/journal.pone.0117278
Tilden A. R., Alt J., Brummer K., Groth R., Herwig K., Wilson A., et al. (2001). Influence of Photoperiod on N-Acetyltransferase Activity and Melatonin in the Fiddler Crab Uca pugilator. General Comp. Endocrinol. 122 (3), 233–237. doi:10.1006/gcen.2001.7641
Tilden A. R., Rasmussen P., Awantang R. M., Furlan S., Goldstein J., Palsgrove M., et al. (1997). Melatonin Cycle in the Fiddler Crab Uca pugilator and Influence of Melatonin on Limb Regeneration. J. Pineal Res. 23, 142–147. doi:10.1111/j.1600-079X.1997.tb00347.x
Vafopoulou X., Steel C. G. H. (2006). Hormone Nuclear Receptor (EcR) Exhibits Circadian Cycling in Certain Tissues, but Not Others, during Development in Rhodnius prolixus (Hemiptera). Cell. Tissue Res. 323, 443–455. doi:10.1007/s00441-005-0076-1
Vivien-Roels B., Pévet P. (1993). Melatonin: Presence and Formation in Invertebrates. Experientia 49, 642–647. doi:10.1007/BF01923945
Webster S. G., Keller R., Dircksen H. (2012). The CHH-Superfamily of Multifunctional Peptide Hormones Controlling Crustacean Metabolism, Osmoregulation, Moulting, and Reproduction. General Comp. Endocrinol. 175 (2), 217–233. doi:10.1016/j.ygcen.2011.11.035
Wurtman R. J., Axelrod J., Phillips L. S. (1963). Melatonin Synthesis in the Pineal Gland: Control by Light. Science 142 (3595), 1071–1073. doi:10.1126/science.142.3595.1071
Yang X., Xu M., Huang G., Zhang C., Pang Y., Yang Z., et al. (2018). The Hyperglycemic Effect of Melatonin in the Chinese Mitten Crab, Eriocheir Sinensis. Front. Physiol. 9, 270. doi:10.3389/fphys.2018.00270
Zanotto F. P., Wheatly M. G. (2002). Calcium Balance in Crustaceans: Nutritional Aspects of Physiological Regulation. Comp. Biochem. Physiology Part A Mol. Integr. Physiology 133 (3), 645–660. doi:10.1016/s1095-6433(02)00202-7
Zheng J., Nakatsuji T., Roer R. D., Watson R. D. (2008). Studies of a Receptor Guanylyl Cyclase Cloned from Y-Organs of the Blue Crab (Callinectes sapidus), and its Possible Functional Link to Ecdysteroidogenesis. General Comp. Endocrinol. 155, 780–788. doi:10.1016/j.ygcen.2007.10.009
Zmora N., Sagi A., Zohar Y., Chung J. S. (2009). Molt-inhibiting Hormone Stimulates Vitellogenesis at Advanced Ovarian Developmental Stages in the Female Blue Crab, Callinectes sapidus 2: Novel Specific Binding Sites in Hepatopancreas and cAMP as a Second Messenger. Aquat. Biosyst. 5 (1), 1–11. doi:10.1186/1746-1448-5-6
Keywords: eyestalk, hepatopancreas, molt cycle, ecdysteroids, molt-inhibiting hormone
Citation: David DD, de Assis LVM, Moraes MN, Zanotto FP and Castrucci AMdL (2022) CasEcR and CasMIH Genes in the Blue Crab, Callinectes sapidus: A Temporal Evaluation and Melatonin Effects. Front. Physiol. 13:903060. doi: 10.3389/fphys.2022.903060
Received: 23 March 2022; Accepted: 26 May 2022;
Published: 21 June 2022.
Edited by:
Cristiano Bertolucci, University of Ferrara, ItalyReviewed by:
Jie Gong, Nantong University, ChinaIlie S. Racotta, Centro de Investigación Biológica del Noroeste (CIBNOR), Mexico
Copyright © 2022 David, de Assis, Moraes, Zanotto and Castrucci. This is an open-access article distributed under the terms of the Creative Commons Attribution License (CC BY). The use, distribution or reproduction in other forums is permitted, provided the original author(s) and the copyright owner(s) are credited and that the original publication in this journal is cited, in accordance with accepted academic practice. No use, distribution or reproduction is permitted which does not comply with these terms.
*Correspondence: Ana Maria de Lauro Castrucci, amdlcast@ib.usp.br