- 1Centre of Marine Sciences (CCMAR), Universidade do Algarve, Faro, Portugal
- 2Universidade do Algarve, Faro, Portugal
- 3Department of Infection and Immunity, Luxembourg Institute of Health, Esch-sur-Alzette, Luxembourg
- 4Faculty of Science, Technology and Medicine, University of Luxembourg, Esch-sur-Alzette, Luxembourg
Food allergy is an abnormal immune response to specific proteins in a certain food. The chronicity, prevalence, and the potential fatality of food allergy, make it a serious socio-economic problem. Fish is considered the third most allergenic food in the world, affecting part of the world population with a higher incidence in children and adolescents. The main allergen in fish, responsible for the large majority of fish-allergic reactions in sensitized patients, is a small and stable calcium-binding muscle protein named beta-parvalbumin. Targeting the expression or/and the 3D conformation of this protein by adding specific molecules to fish diets has been the innovative strategy of some researchers in the fields of fish allergies and nutrition. This has shown promising results, namely when the apo-form of β-parvalbumin is induced, leading in the case of gilthead seabream to a 50% reduction of IgE-reactivity in fish allergic patients.
Introduction
The World Health Organization considers fish as one of the most frequent causes of food allergies. The prevalence of fish allergy is especially high in the young population, reaching up to 1% based on oral food challenge data and up to 7% based on questionnaires (Moonesinghe et al., 2016; Klueber et al., 2019). Allergy rates correlate also with a pronounced exposure to fish, either by consumption in local diets along coastal lines or by fish handling in the occupational field of fish-processing production lines (<36%) (Bonlokke et al., 2019). Allergic patients experience from mild and local over to severe and systemic reactions upon ingestion, inhalation, or skin contact with fish. The ingestion of fish in the low milligram range is sufficient to provoke clinical symptoms (e.g. 27.3 mg determined as ED10 value for cod) (Ballmer-Weber et al., 2015; Sørensen et al., 2017; Houben et al., 2020). Fish allergy often persists over a lifetime. Natural tolerance to fish might develop until adolescence, especially in patients with a disease onset during their first years of life (Xepapadaki et al., 2021). The diagnosis of fish allergy is established by combining the medical history with results from serological specific IgE-antibody determination and results from skin reactivity tests using authentic fish or fish extracts (Matricardi et al., 2016; Hilger et al., 2017; Klueber et al., 2019; Buyuktiryaki et al., 2021). Oral food challenges, i.e. medically supervised intakes of gradually increasing fish doses, are only performed in exceptional cases where the clinical reactivity is questioned (Matricardi et al., 2016). The clinical management of fish-allergic patients refers mainly to a strict avoidance of all kinds of fish (Figure 1). When accidental consumption occurs, allergic symptoms are treated with rescue medication, including antihistamines, β-2 sympathomimetic, inhaled corticosteroids and in severe cases, adrenaline (Tedner et al., 2022). Often, fish-allergic patients demand to consume this food because of its nutritional benefits on health, such as via minerals, vitamins, and polyunsaturated fatty acids (Kalic et al., 2021). Fish is an ideal source of omega-3 fatty acids, including EPA (eicosapentaenoic acid) and DHA (docosahexaenoic acid), which are associated with a reduced risk for chronic inflammatory diseases (Maulu et al., 2021; Chen et al., 2022). Dietary recommendations comprise the consumption of up to two servings of fish per week, a consumption level which is associated with a reduced risk for coronary heart disease (EFSA Scientific Committee, 2015; Chen et al., 2022). The diet-related benefit appears even larger than thought. Recent studies demonstrated that the human gut microbiome is influenced positively through fish consumption (Chen et al., 2022). Regular fish intake correlates with a healthy microbiota composition, meaning with an increased bacterial richness and alpha-diversity, which in turn is known to be associated with an improved immune response and a reduced inflammatory disease burden (Dale et al., 2019; Simione et al., 2020). There is a general notion that the traditional way of clinical management might be further advanced towards a more personalized dietary recommendation regarding (Klueber et al., 2019; Dijkema et al., 2020) which fish species the patient might need to avoid and which fish species the patient might consume safely (Figure 1). The benefit of including certain fishes into the nutrition plan of fish-allergic patients is two-fold. First, to balance the food intake via a high-quality and healthy protein source and second, to create opportunities to the immune system for potential tolerance development through targeted exposure (Maulu et al., 2021; Chen et al., 2022). Important for those approaches is the prior knowledge on fish species that are tolerated individually by the patient, a decision procedure that needs to be followed up specifically in the frame of the clinical diagnosis (Matricardi et al., 2016). The level of serum IgE-antibodies directed against fish proteins might be supportive in this decision (Kuehn et al., 2013; Hilger et al., 2017; Kalic et al., 2019; Rubin et al., 2020). A food challenge-based study demonstrated that patients with specific IgE to cod and salmon extract, IgE-levels >8.2 kUA/L and >5 kUA/L, respectively, shall be advised to avoid fish in general (Sørensen et al., 2017). This is, however, an approach that has the potential to be further advanced into a less stringent and patient-oriented praxis, based on clinical and molecular allergen knowledge (Klueber et al., 2019; Dijkema et al., 2020). Some fish species are better tolerated than others, which might be explained by low fish allergen contents as well as by specific properties of the allergenic fraction (Kuehn et al., 2010; Mourad and Bahna, 2015; Kobayashi et al., 2016b; Kalic et al., 2019; Pérez-Tavarez et al., 2021). Known low allergen content fishes are perch-like fishes, such as tuna and swordfish (Kuehn et al., 2010; Kobayashi Y. et al., 2016). Another fish with reduced molecular allergenicity is ray, a cartilaginous species (Kalic et al., 2019). Those species might be considered as naturally occurring hypoallergenic fish and thus, a valuable alternative to balance the avoidance diet of many fish-allergic patients. To identify, or even create, hypoallergenic fish is considered an important approach of high translational relevance in both therapy (Zuidmeer-Jongejan et al., 2015; Okamoto et al., 2021; Ugajin et al., 2021) and possibly, also prevention.
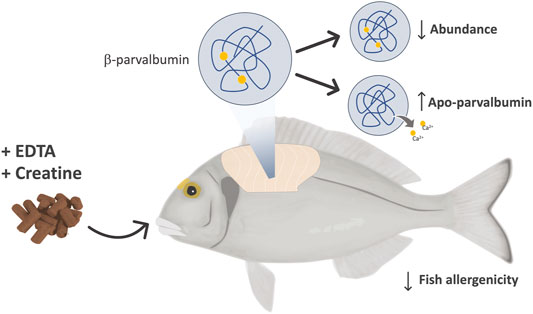
FIGURE 1. From diagnosis to traditional and advanced clinical approaches to manage fish-allergic patients. Under strict avoidance of any type of fish, patients risk maintaining their food allergy over lifetime. The consumption of single, individually tolerated fish species contributes to a balanced diet and most likely, promotes tolerance development. Hypoallergenic fish species, either naturally occurring or produced in targeted aquaculture, are key components to implement such a personalized approach in patient care.
Allergic reactions to fish are mainly based on type 2 hypersensitivity, thus involving allergen-specific type 2 helper (Th2) cells, which activate B cells to become plasma cells producing IgE-antibodies against fish allergens (Klueber et al., 2019). Fish allergens occur mostly in the fish muscle. The allergen database of the WHO/IUIS allergen nomenclature subcommittee comprises presently 40 fish allergens from 18 different fish species (Matricardi et al., 2016). Most allergens belong to the parvalbumin family, in addition to muscle enolases, aldolases, tropomyosins, triosephosphate isomerases and others. The most potent fish allergen is parvalbumin (Matricardi et al., 2016). This small muscle protein (10–12 kDa) is a calcium-binding protein of high stability towards effects of food processing (Costa et al., 2022). Parvalbumin does not only survive heat treatment, hence causing symptoms upon ingestion of prepared fish, it also can become aerosolized during fish handling, leading to respiratory symptoms upon inhalation. Muscle parvalbumin contents vary considerably in different fish species, which allows to explain the low allergenicity of tuna and swordfish, vs. the high allergenicity of cod and salmon (Kuehn et al., 2010; Mourad and Bahna, 2015; Kobayashi et al., 2016b; Kalic et al., 2019; Pérez-Tavarez et al., 2021). Parvalbumins seem to be also mainly implied in clinical cross-reactivity. Indeed, highly conserved IgE-epitopes occur across the fish species, representing an important reason why fish allergic patient mostly react to various fishes (Kuehn et al., 2014; Hilger et al., 2017). Multiple studies using different technology approaches focused on the identification of B cell epitopes of fish parvalbumins (Perez-Gordo et al., 2013; Carrera et al., 2019; Sharp et al., 2021), as they represent important targets motifs for developing vaccination strategies. Molecular changes can reduce the allergenicity of single fish molecules, which has been successfully shown in a pilot study on epitope-modified mutants of fish parvalbumins (Swoboda et al., 2013). A previous EU-project focused on the subcutaneous application of such a hypoallergenic, low IgE-binding fish allergen variant for the causative treatment of fish allergy (Zuidmeer-Jongejan et al., 2015). The drawback of such approaches is that, if translated into fish farming, genetically modified organisms are created, which cause controversy in human nutrition. Natural, low allergenic fish, such as tuna and ray, are of interest in this context, however they cannot serve the market. Other strategies to reduce the allergenicity of fish parvalbumin, through processing effects inducing protein glycosylation, aggregation or others, perform in the experimental setting (Pérez-Tavarez et al., 2019; Wu et al., 2022), though confirmatory in-vivo data are evasive.
A highly promising, innovative, and sustainable approach is to modify the allergenic properties of fish through targeted fish fed in aquaculture. Such hypoallergenic fish from aquaculture hold promise for valuable use in multiple domains. This fish might be used in the frame of fish allergy prevention, by early introduction instead of traditional fishes, to reduce the likelihood for allergic sensitization in high-risk families (Figure 1). Clearly, such hypoallergenic fish will be of relevance for fish-allergic patients, either as a safe alternative to balance their diets or even, as a potentially tolerance-inducing target in safe oral immunotherapy approaches.
Role of Nutrition is Fish Allergenicity
Fish is considered nowadays a healthier alternative to protein from other animals. This, together with capture restrictions resulting from wild fish exploitation translates in a worldwide increasing demand for fish and fish products, with aquaculture reaching the XXI century as the fastest-growing animal production sector industry (FAO, 2020). Aquaculture not only alleviates the pressure on marine fish stocks but also allows, in marked contrast to wild-caught fish, for a close monitorization of the entire fish production process, in a controlled environment, ensuring a safe and wholesome aquatic food product for human consumption. Fish nutrition is one of the main aquaculture research areas due to its essential role in promoting fish resilience, welfare, health, and growth, but also in improving the industry’s sustainability and the safety of the final product. Remarkable achievements have been made regarding dietary manipulation and feed supplementation towards enhancing the nutritional value and safety of fish products, e.g., diet enrichment with omega-3 fatty acids and other important nutrients, elimination of toxins, environmental contaminants, and veterinary drug residues (Tacon et al., 2020). Recently fish nutrition has also demonstrated to be a promisor approach to produce a hypoallergenic farmed fish, through the use of fish enriched diets with molecules capable of modulating fish allergenicity by tackling the concentration in muscle or inducing structural modifications in the main fish allergen protein parvalbumin. This was the approach reported in several key studies with gilthead seabream and European seabass (Schrama et al., 2018; De Magalhaes et al., 2020; Schrama et al., 2022), in which authors used creatine or ethylenediamine tetra-acetic acid (EDTA) enriched fish diets to either reduce parvalbumin concentration or induce apo-parvalbumin (a free calcium binding ion structural form of parvalbumin with reduced IgE-binding capacity), respectively (Figure 2). This approach can be considered a highly innovative strategy, given that forefront research is mostly focusing on treating food allergies using immunotherapies, while these studies target a hypoallergenic fish for food allergy prevention. A market study conducted in Portugal, evaluating the consumers’ willingness to pay for a hypoallergenic fish, concluded not only that half the consumers were willing to pay extra for this type of product, but also suggested that the willingness to pay for it was explained by the presence of the same family fish-allergy related pathologies and the fish unique characteristics and quality (Pereira et al., 2020).
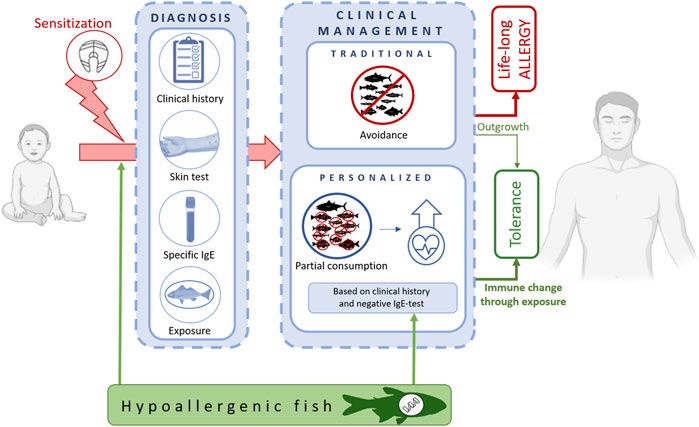
FIGURE 2. Fish diet supplementation strategy used in gilthead seabream (Sparus aurata) and European seabass (Dicentrarchus labrax) allergenicity modulation studies. Both species were fed for 3 months, in separate trials, using diets supplemented with different incorporation percentages of EDTA and creatine. EDTA-supplemented diets aimed at inducing the apo-form of the major fish allergen parvalbumin, a calcium-devoid conformation of this protein reported to be less immunoreactive, while the diets supplemented with creatine targeted parvalbumin’s abundance.
The experimental methodology used by authors involves the whole fish production process, from the formulation of diets, growth tests, animal welfare test, the quality and protein characterization of fish muscle, and finally testing in vitro the immunological reaction through an IgE-antibody binding test, using sera from patients with a proven fish allergy pathology. To monitor biological and metabolic modifications related to the use of both Creatine and EDTA enriched diets, authors used cutting-edge technologies like Proteomics and Metabolic Fingerprinting by Solid Phase Transmissive Fourier Transform Infrared (FT-IR) Spectroscopy (Schrama et al., 2018; De Magalhaes et al., 2020; Schrama et al., 2022). Proteomics has been the key technology used in many fish nutrition studies over the last decade with proven success in measuring biological effects associated with nutritional factors or a particular dietary treatment. The liver is the target organ in most of these studies due to its role in metabolism regulation and adaptation nutritional changes. Nevertheless, other organs/fluids like muscle, gut/intestine, plasma or mucus have also been successfully used in comparative proteomics studies (de Vareilles et al., 2012a; de Vareilles et al., 2012b; Matos et al., 2013; Schrama et al., 2017; Boonanuntanasarn et al., 2019; Cerqueira et al., 2020).
EDTA is a chelating agent widely present in industrial, cosmetic and food products. Its salt form, calcium disodium EDTA (CAS number: 62-33-9), is an authorized multifunctional food additive (E385) in the European Union (EU), according to Annex II and Annex III of the Regulation (EC) No 1333/2008 on food additives. The maximum levels allowed in several food categories range from 75 to 250 mg/kg, and the acceptable daily intake (ADI) is set to 1.9 mg/kg of body weight (Younes et al., 2018).
Several studies, on different fish species, have demonstrated that calcium-chelating is crucial to the structural preservation of parvalbumin and its IgE-binding capacity (Swoboda et al., 2007; Permyakov et al., 2008; Swoboda et al., 2013; Moraes et al., 2014; Kumeta et al., 2017). Thus, EDTA has been used in several fish allergens-related studies, to induce, in vitro, the apo-parvalbumin, i.e., the Ca2+-free form of parvalbumin, and explore its reduced IgE-affinity and potential decreased allergenicity. For example, in 1979, Apold & Elsayed used EDTA to induce the uncoupling of the calcium ions from the cod parvalbumin, Gad c 1. The authors reported that this allergen form, devoid of Ca2+, showed a IgE-reactivity reduction of around 30% (Apold and Elsayed, 1979). More recently, Mohammadi et al. (2017) showed a significantly decreased IgE-reactivity of the EDTA-treated wolf-herring parvalbumin, compared to the native protein. Additionally, other authors using a different chelating agent, the ethyleneglycoltetraacetic acid (EGTA), achieved similar results and conclusions regarding parvalbumin’s stability and immunoreactivity (Bugajska-Schretter et al., 1998; Tomura et al., 2008; Kobayashi et al., 2016a).
In the aquaculture field, EDTA has been used as fish preservative (Power et al., 1968; Chang et al., 2021) and feed supplement for different fish species, the later targeting heavy metal sequestration (Shalaby, 2007; Gopal et al., 2009; Iranshahi et al., 2011; Abdel-Tawwab et al., 2017) and fish allergenicity modulation (De Magalhaes et al., 2020; Schrama et al., 2022). In these recent studies, the in vivo capacity of EDTA to induce a calcium-depleted less reactive parvalbumin was assessed. The authors intended to change the ionic environment of the fish muscle, by using this chelating agent to scavenge for calcium ions, and therefore promote a potentially hypoallergenic fish (reduced content of Ca2+-bounded parvalbumin in the muscle). Two important Southern Europe aquaculture fish species, gilthead seabream and European seabass, were fed different diets, specifically formulated for this study, and supplemented with different concentrations of EDTA, for approximately 3 months. A concentration of 3% of EDTA showed a reduction of approximately 50% in gilthead seabream parvalbumin’s IgE-reactivity to cod-allergic patients’ sera, however no changes regarding European seabass were observed with supplementations of up to 4.5% of EDTA. Zootechnical, comparative gel-based proteomics and sensory analyses showed that EDTA supplementation did not affect fish growth, muscle metabolism and fish quality as edible food, respectively, in both species, unless higher concentrations of EDTA were used with negative effects on fish growth performance and feed efficiency. Moreover, to show that this potential hypoallergenic fish was safe for human consumption, the EDTA retained in the fish muscle was assessed by high-performance liquid chromatography (HPLC), and the concentration was below the aforementioned values established by the EU, for the two species (De Magalhaes et al., 2020; Schrama et al., 2022).
Follow-up IgE-reactivity studies using patients’ sera with a confirmed clinical reactivity to these species should be performed to improve and corroborate the reported results, since cases of monosensitivity to specific fish species or single parvalbumin isoforms are common (Kuehn et al., 2011). Enzyme-linked immunosorbent assays (ELISA) with antibodies specific for these species and the purified allergen should also be included in subsequent tests to confirm the reduction in the IgE-binding capacity upon EDTA presence. Lastly, it is important to note that to confirm a reduction in the allergenic potency of this protein more tests should be performed, such as in vitro digestibility tests, skin-prick tests, basophil histamine release assays and at the very end double-blind placebo-controlled food challenges (DBPCFC).
Creatine supplementation into fish diets has been investigated in several studies involving different fish species. Growth performance, sprint endurance and allergenicity were analysed in red drum (Sciaenops ocellatus) (Burns and Gatlin, 2019), rainbow trout (Onchorhynchus mykiss) (McFarlane et al., 2001) and Southern Europe species like gilthead seabream (Sparus aurata) (Schrama et al., 2018) and European seabass (Dicentrarchus labrax) (Schrama et al., 2022), respectively. In case of the Southern Europe species, creatine was used as a feed supplement aiming to target the expression of the major fish allergen and modulate these species’ allergenicity. Creatine is an important substrate for the energy homeostasis (Schrama et al., 2018), which is essential for all living organisms, including fish, and a well-known supplier for this energy is adenosine triphosphate (ATP) (Borchel et al., 2019). The reversible mechanism of creatine and ATP produce creatine phosphate and adenosine diphosphate (ADP), using the catalysing enzyme creatine kinase (Borchel et al., 2014). Also, a very low amount of creatine/creatine phosphate produces irreversibly creatinine, which is excreted, and therefore creatine needs to be synthesized or ingested through the diet (Borchel et al., 2019). Synthesis of creatine is regulated by the GATM (l-arginine:glycine amidinotransferase) and GAMT (guanidinoacetate-N-methyltransferase) enzymes (Schrama et al., 2022). Studies in rainbow trout confirmed the presence of GATM in muscle tissue (Borchel et al., 2019). Besides this, free and phosphorylated creatine has been found in the fish white muscle (Hunter, 1929), where it also contributes to the contraction/relaxation mechanism of this tissue (Schrama et al., 2018). Additionally, the expression of several proteins present in the muscle, with calcium (Ca2+) binding properties, influence the contractile properties of this tissue (Berchtold et al., 2000). Although the creatine metabolism in fish is not fully understood, it seems that the creatine mechanism also influences the calcium homeostasis (Kim et al., 2015). Ingestion of creatine seems to increase Ca2+-ATPase, resulting in less available free Ca2+ buffering (Kim et al., 2015). A well-known fish muscle Ca2+-binding protein is parvalbumin, which is described earlier. Thus, as creatine seems to reduce the calcium content, a decreased expression of calcium binding proteins, such as parvalbumin, might occur, and this was the rationale behind the allergenicity-modulation studies using creatine supplementation.
A control diet was supplemented with three different concentrations of creatine (2, 5 and 8%) and fed both species daily for 3 months, until satiety (Schrama et al., 2018; Schrama et al., 2022). Authors analysed the expression of the allergenic protein parvalbumin, in fish muscle samples, using comparative gel-based proteomics, besides the determinations of fish growth, welfare and quality. Muscle proteome showed a vast number of proteins present in this tissue, including the parvalbumin protein. However, no direct evidence was found that creatine supplementation, with these incorporation percentages, reduced the expression of parvalbumin and consequently allergenicity, neither in gilthead seabream nor in European seabass. Also, zootechnical results showed that Creatine supplementation did not affect fish growth, for both species. Although some differences were found in cortisol concentrations, authors could not link it directly with the experimental diets. Fish quality as edible food was preserved in gilthead seabream (no results were shown for European seabass).
Different studies should be conducted to understand the interaction between supplemented creatine and parvalbumin, including determination of calcium content in fish muscle (as this ion also regulates ATP (Berchtold et al., 2000)). Also, different concentrations of supplementation could be tested, as up to 8% of creatine did not show any negative effect in fish growth or creatine accumulation in muscle (Schrama et al., 2018; Schrama et al., 2022).
Conclusion
With the rise of food allergy incidence in the world and the absence of a cure, it is imperative to look at innovative strategies to fight this chronic disease. With fish being one of the top nine food allergens and increasingly seen as a source of healthy protein and high nutritional value, the new approach that relies on the modulation of the main fish allergen, with the perspective of allowing allergic patients to still include this nutritionally important food in their diets, is certainly seen as unique and promising, considering the recently published results. Nonetheless, we anticipate a few drawbacks in this new approach as there are limitations in the concentration of molecules like Creatine or EDTA in fish diets as they might negatively interfere with fish feed intake and may also accumulate in fish muscle in concentrations above those legally accepted. On the other hand, the reduction of approximately 50% in gilthead seabream parvalbumin’s IgE-reactivity to cod-allergic patients’ sera, when a concentration of 3% EDTA was added to fish diets, points to a promising strategy. We believe that supplementing fish diets with natural sources like microalgae reported to be rich in compounds with calcium chelating activity, can be a possible strategy. Hence, supplementation of fish feed with microalgal extracts could act both to supply calcium chelators to reduce the fish allergenicity and decrease the need for fish meal as a supply of polyunsaturated fatty acids (PUFA), thus increasing the economic and environmental sustainability of aquaculture.
Author Contributions
DS, RC, and CRM contributed equally to the paper and help with writing and figures. AK and PR share the last authorship and were responsible for writing, figures, paper structure, and content.
Funding
This work received Portuguese national funds from FCT - Foundation for Science and Technology through projects UIDB/04326/2020 and LA/P/0101/2020. RC is Supported by the Luxembourg National Research Fund on PRIDE program grant PRIDE17/11823097/MICROH. DS and CR acknowledge FCT PhD scholarships, Ref. SFRH/BD/136319/2018 and Ref. SFRH/BD/138884/2018, respectively.
Conflict of Interest
The authors declare that the research was conducted in the absence of any commercial or financial relationships that could be construed as a potential conflict of interest.
Publisher’s Note
All claims expressed in this article are solely those of the authors and do not necessarily represent those of their affiliated organizations, or those of the publisher, the editors and the reviewers. Any product that may be evaluated in this article, or claim that may be made by its manufacturer, is not guaranteed or endorsed by the publisher.
Acknowledgments
Figures were created in part with BioRender.com and the open-source graphics editor Inkscape (http://www.inkscape.org/).
References
Abdel-Tawwab M., El-Sayed G. O., Monier M. N., Shady S. H. (2017). Dietary EDTA Supplementation Improved Growth Performance, Biochemical Variables, Antioxidant Response, and Resistance of Nile tilapia, Oreochromis niloticus (L.) to Environmental Heavy Metals Exposure. Aquaculture 473, 478–486. doi:10.1016/j.aquaculture.2017.03.006
Apold J., Elsayed S. (1979). The Effect of Amino Acid Modification and Polymerization on the Immunochemical Reactivity of Cod Allergen M. Mol. Immunol. 16 (8), 559–564. doi:10.1016/0161-5890(79)90118-4
Ballmer-Weber B. K., Fernandez-Rivas M., Beyer K., Defernez M., Sperrin M., Mackie A. R., et al. (2015). How Much Is Too Much? Threshold Dose Distributions for 5 Food Allergens. J. Allergy Clin. Immunol. 135 (4), 964–971. doi:10.1016/j.jaci.2014.10.047
Berchtold M. W., Brinkmeier H., Müntener M. (2000). Calcium Ion in Skeletal Muscle: Its Crucial Role for Muscle Function, Plasticity, and Disease. Physiol. Rev. 80 (3), 1215–1265. doi:10.1152/physrev.2000.80.3.1215
Bonlokke J. H., Bang B., Aasmoe L., Rahman A. M. A., Syron L. N., Andersson E., et al. (2019). Exposures and Health Effects of Bioaerosols in Seafood Processing Workers - a Position Statement. J. Agromedicine 24 (4), 441–448. doi:10.1080/1059924X.2019.1646685
Boonanuntanasarn S., Nakharuthai C., Schrama D., Duangkaew R., Rodrigues P. M. (2019). Effects of Dietary Lipid Sources on Hepatic Nutritive Contents, Fatty Acid Composition and Proteome of Nile tilapia (Oreochromis niloticus). J. Proteomics 192, 208–222. doi:10.1016/j.jprot.2018.09.003
Borchel A., Verleih M., Kühn C., Rebl A., Goldammer T. (2019). Evolutionary Expression Differences of Creatine Synthesis-Related Genes: Implications for Skeletal Muscle Metabolism in Fish. Sci. Rep. 9 (1), 5429. doi:10.1038/s41598-019-41907-6
Borchel A., Verleih M., Rebl A., Kühn C., Goldammer T. (2014). Creatine Metabolism Differs between Mammals and Rainbow Trout (Oncorhynchus mykiss). Springerplus 3, 510. doi:10.1186/2193-1801-3-510
Bugajska-Schretter A., Elfman L., Fuchs T., Kapiotis S., Rumpold H., Valenta R., et al. (1998). Parvalbumin, a Cross-Reactive Fish Allergen, Contains IgE-Binding Epitopes Sensitive to Periodate Treatment and Ca2+ Depletion. J. Allergy Clin. Immunol. 101 (1), 67–74. doi:10.1016/S0091-6749(98)70195-2
Burns A. F., Gatlin D. M. (2019). Dietary Creatine Requirement of Red Drum (Sciaenops Ocellatus) and Effects of Water Salinity on Responses to Creatine Supplementation. Aquaculture 506, 320–324. doi:10.1016/j.aquaculture.2019.03.059
Buyuktiryaki B., Masini M., Mori F., Barni S., Liccioli G., Sarti L., et al. (2021). IgE-mediated Fish Allergy in Children. Medicina 57 (1), 76. doi:10.3390/medicina57010076
Carrera M., González-Fernández Á., Magadán S., Mateos J., Pedrós L., Medina I., et al. (2019). Molecular Characterization of B-Cell Epitopes for the Major Fish Allergen, Parvalbumin, by Shotgun Proteomics, Protein-Based Bioinformatics and IgE-Reactive Approaches. J. Proteomics 200, 123–133. doi:10.1016/j.jprot.2019.04.005
Cerqueira M., Schrama D., Silva T. S., Colen R., Engrola S. A. D., Conceição L. E. C., et al. (2020). How Tryptophan Levels in Plant-Based Aquafeeds Affect Fish Physiology, Metabolism and Proteome. J. Proteomics 221, 103782. doi:10.1016/j.jprot.2020.103782
Chang S.-H., Chen Y.-J., Tseng H.-J., Hsiao H.-I., Chai H.-J., Shang K.-C., et al. (2021). Applications of Nisin and EDTA in Food Packaging for Improving Fabricated Chitosan-Polylactate Plastic Film Performance and Fish Fillet Preservation. Membranes 11 (11), 852. doi:10.3390/membranes11110852
Chen J., Jayachandran M., Bai W., Xu B. (2022). A Critical Review on the Health Benefits of Fish Consumption and its Bioactive Constituents. Food Chem. 369, 130874. doi:10.1016/j.foodchem.2021.130874
Costa J., Villa C., Verhoeckx K., Cirkovic-Velickovic T., Schrama D., Roncada P., et al. (2022). Are Physicochemical Properties Shaping the Allergenic Potency of Animal Allergens? Clin. Rev. Allerg. Immunol. 62 (1), 1–36. doi:10.1007/s12016-020-08826-1
Dale H. F., Madsen L., Lied G. A. (2019). Fish-derived Proteins and Their Potential to Improve Human Health. Nutr. Rev. 77 (8), 572–583. doi:10.1093/nutrit/nuz016
De Magalhães C. R., Schrama D., Fonseca F., Kuehn A., Morisset M., Ferreira S. R., et al. (2020). Effect of EDTA Enriched Diets on Farmed Fish Allergenicity and Muscle Quality; a Proteomics Approach. Food Chem. 305, 125508. doi:10.1016/j.foodchem.2019.125508
de Vareilles M., Conceição L. E. C., Gómez-Requeni P., Kousoulaki K., Richard N., Rodrigues P. M., et al. (2012a). Dietary Lysine Imbalance Affects Muscle Proteome in Zebrafish (Danio rerio): A Comparative 2D-DIGE Study. Mar. Biotechnol. 14, 643–654. doi:10.1007/s10126-012-9462-3
de Vareilles M., Richard N., Gavaia P. J., Silva T. S., Cordeiro O., Guerreiro I., et al. (2012b). Impact of Dietary Protein Hydrolysates on Skeleton Quality and Proteome in Diplodus sargus Larvae. J. Appl. Ichthyology 28 (3), 477–487. doi:10.1111/j.1439-0426.2012.01986.x
Dijkema D., Emons J. A. M., Van de Ven A. A. J. M., Oude Elberink J. N. G. (2020). Fish Allergy: Fishing for Novel Diagnostic and Therapeutic Options. Clin. Rev. Allerg. Immunol. 62, 64–71. doi:10.1007/s12016-020-08806-5
EFSA Scientific Committee (2015). Statement on the Benefits of Fish/seafood Consumption Compared to the Risks of Methylmercury in Fish/seafood. EFSA J. 13 (1), 3982. doi:10.2903/j.efsa.2015.3982
FAO (2020). The State of World Fisheries and Aquaculture 2020. Sustain. action 35, 21–23. doi:10.4060/ca9229en
Gopal R., Narmada S., Vijayakumar R., Jaleel C. A. (2009). Chelating Efficacy of CaNa2 EDTA on Nickel-Induced Toxicity in Cirrhinus Mrigala (Ham.) through its Effects on Glutathione Peroxidase, Reduced Glutathione and Lipid Peroxidation. Comptes Rendus Biol. 332 (8), 685–696. doi:10.1016/j.crvi.2009.03.004
Hilger C., van Hage M., Kuehn A. (2017). Diagnosis of Allergy to Mammals and Fish: Cross-Reactive vs. Specific Markers. Curr. Allergy Asthma Rep. 17 (9), 1–12. doi:10.1007/s11882-017-0732-z
Houben G. F., Baumert J. L., Blom W. M., Kruizinga A. G., Meima M. Y., Remington B. C., et al. (2020). Full Range of Population Eliciting Dose Values for 14 Priority Allergenic Foods and Recommendations for Use in Risk Characterization. Food Chem. Toxicol. 146, 111831. doi:10.1016/j.fct.2020.111831
Hunter A. (1929). The Creatine Content of the Muscles and Some Other Tissues in Fishes. J. Biol. Chem. 81 (3), 513–523. doi:10.1016/S0021-9258(18)63706-8
Iranshahi F., Faramarzi M., Kiaalvandi S., Hosein M., Jalaee M., Dehghan M. (2011). Effectiveness of EDTA in Mobilizing of the Contaminant Metal Ions, Especially Cadmium from Tissue of Angel Fish (Pterophyllum scalare Schultze) Subjected to Chronic Poisoning with Cadmium Acetate. Am-Eur J. Agric. Environ. Sci. 11 (4), 519–527.
Kalic T., Morel-Codreanu F., Radauer C., Ruethers T., Taki A. C., Swoboda I., et al. (2019). Patients Allergic to Fish Tolerate Ray Based on the Low Allergenicity of its Parvalbumin. J. Allergy Clin. Immunol. Pract. 7 (2), 500–508. doi:10.1016/j.jaip.2018.11.011
Kalic T., Radauer C., Lopata A. L., Breiteneder H., Hafner C. (2021). Fish Allergy Around the World-Precise Diagnosis to Facilitate Patient Management. Front. Allergy 2, 732178. doi:10.3389/falgy.2021.732178
Kim J., Lee J., Kim S., Yoon D., Kim J., Sung D. J. (2015). Role of Creatine Supplementation in Exercise-Induced Muscle Damage: A Mini Review. J. Exerc Rehabil. 11 (5), 244–250. doi:10.12965/jer.150237
Klueber J., Schrama D., Rodrigues P., Dickel H., Kuehn A. (2019). Fish Allergy Management: from Component-Resolved Diagnosis to Unmet Diagnostic Needs. Curr. Treat. Options Allergy 6 (4), 322–337. doi:10.1007/s40521-019-00235-w
Kobayashi A., Ichimura A., Kobayashi Y., Shiomi K. (2016a). IgE-binding Epitopes of Various Fish Parvalbumins Exist in a Stereoscopic Conformation Maintained by Ca2+ Bindingfish Parvalbumins Exist in a Stereoscopic Conformation Maintained by Ca2+ Binding. Allergol. Int. 65 (3), 345–348. doi:10.1016/j.alit.2016.02.004
Kobayashi A., Kobayashi Y., Shiomi K. (2016b). Fish Allergy in Patients with Parvalbumin-specific Immunoglobulin E Depends on Parvalbumin Content rather Than Molecular Differences in the Protein Among Fish Species. Biosci. Biotechnol. Biochem. 80 (10), 2018–2021. doi:10.1080/09168451.2016.1189318
Kobayashi Y., Yang T., Yu C.-T., Ume C., Kubota H., Shimakura K., et al. (2016). Quantification of Major Allergen Parvalbumin in 22 Species of Fish by SDS-PAGE. Food Chem. 194, 345–353. doi:10.1016/j.foodchem.2015.08.037
Kuehn A., Hilger C., Lehners-Weber C., Codreanu-Morel F., Morisset M., Metz-Favre C., et al. (2013). Identification of Enolases and Aldolases as Important Fish Allergens in Cod, Salmon and Tuna: Component Resolved Diagnosis Using Parvalbumin and the New Allergens. Clin. Exp. Allergy 43 (7), 811–822. doi:10.1111/cea.12117
Kuehn A., Hutt-Kempf E., Hilger C., Hentges F. (2011). Clinical Monosensitivity to Salmonid Fish Linked to Specific IgE-Epitopes on Salmon and Trout Beta-Parvalbumins. Allergy 66 (2), 299–301. doi:10.1111/j.1398-9995.2010.02463.x
Kuehn A., Scheuermann T., Hilger C., Hentges F. (2010). Important Variations in Parvalbumin Content in Common Fish Species: A Factor Possibly Contributing to Variable Allergenicity. Int. Arch. Allergy Immunol. 153 (4), 359–366. doi:10.1159/000316346
Kuehn A., Swoboda I., Arumugam K., Hilger C., Hentges F. o. (2014). Fish Allergens at a Glance: Variable Allergenicity of Parvalbumins, the Major Fish Allergens. Front. Immunol. 5, 179. doi:10.3389/fimmu.2014.00179
Kumeta H., Nakayama H., Ogura K. (2017). Solution Structure of the Major Fish Allergen Parvalbumin Sco J 1 Derived from the Pacific Mackerel. Sci. Rep. 7 (1), 17160. doi:10.1038/s41598-017-17281-6
Matos E., Silva T. S., Wulff T., Valente L. M. P., Sousa V., Sampaio E., et al. (2013). Influence of Supplemental Maslinic Acid (Olive-derived Triterpene) on the Post-mortem Muscle Properties and Quality Traits of Gilthead Seabream. Aquaculture 396-399, 146–155. doi:10.1016/j.aquaculture.2013.02.044
Matricardi P. M., Kleine-Tebbe J., Hoffmann H. J., Valenta R., Hilger C., Hofmaier S., et al. (2016). EAACI Molecular Allergology User's Guide. Pediatr. Allergy Immunol. 27 (S23), 1–250. doi:10.1111/pai.12563
Maulu S., Nawanzi K., Abdel-Tawwab M., Khalil H. S. (2021). Fish Nutritional Value as an Approach to Children's Nutrition. Front. Nutr. 8, 780844. doi:10.3389/fnut.2021.780844
McFarlane W. J., Heigenhauser G. J. F., McDonald D. G. (2001). Creatine Supplementation Affects Sprint Endurance in Juvenile Rainbow Trout. Comp. Biochem. Physiology Part A Mol. Integr. Physiology 130 (4), 857–866. doi:10.1016/S1095-6433(01)00448-2
Mohammadi M., Mokhtarian K., Kardar G. A., Farrokhi S., Sadroddiny E., Khorramizadeh M. R., et al. (2017). Expression of Recombinant Parvalbumin from Wolf-Herring Fish and Determination of its IgE-Binding Capability. Food Agric. Immunol. 28 (4), 573–585. doi:10.1080/09540105.2017.1306493
Moonesinghe H., Mackenzie H., Venter C., Kilburn S., Turner P., Weir K., et al. (2016). Prevalence of Fish and Shellfish Allergy: a Systematic Review. Ann. Allergy, Asthma & Immunol. 117 (3), 264–272. doi:10.1016/j.anai.2016.07.015
Moraes A. H., Ackerbauer D., Kostadinova M., Bublin M., de Oliveira G. A., Ferreira F., et al. (2014). Solution and High-Pressure NMR Studies of the Structure, Dynamics, and Stability of the Cross-Reactive Allergenic Cod Parvalbumin Gad M 1. Proteins 82 (11), 3032–3042. doi:10.1002/prot.24664
Mourad A. A., Bahna S. L. (2015). Fish-allergic Patients May Be Able to Eat Fish. Expert Rev. Clin. Immunol. 11 (3), 419–430. doi:10.1586/1744666X.2015.1009896
Okamoto M., Takafuji S., Inoue S., Tanaka Y. (2021). Fish Allergy Tolerance 16 Months after Diagnosis. aei 49 (5), 25–27. doi:10.15586/aei.v49i5.313
Pereira L. N., Mendes J., Schrama D., Rodrigues P. M. L. (2020). Consumers' Willingness to Pay for Fish with a Low Allergenic Potential. J. Int. Food & Agribus. Mark. 34, 1–22. doi:10.1080/08974438.2020.1844108
Perez-Gordo M., Pastor-Vargas C., Lin J., Bardina L., Cases B., Ibáñez M. D., et al. (2013). Epitope Mapping of the Major Allergen from Atlantic Cod in Spanish Population Reveals Different IgE-Binding Patterns. Mol. Nutr. Food Res. 57 (7), 1283–1290. doi:10.1002/mnfr.201200332
Pérez-Tavarez R., Carrera M., Pedrosa M., Quirce S., Rodríguez-Pérez R., Gasset M. (2019). Reconstruction of Fish Allergenicity from the Content and Structural Traits of the Component β-parvalbumin Isoforms. Sci. Rep. 9 (1), 16298–16312. doi:10.1038/s41598-019-52801-6
Pérez-Tavarez R., Moreno H. M., Borderias J., Loli-Ausejo D., Pedrosa M., Hurtado J. L., et al. (2021). Fish Muscle Processing into Seafood Products Reduces β-parvalbumin Allergenicity. Food Chem. 364, 130308. doi:10.1016/j.foodchem.2021.130308
Permyakov S. E., Bakunts A. G., Denesyuk A. I., Knyazeva E. L., Uversky V. N., Permyakov E. A. (2008). Apo-parvalbumin as an Intrinsically Disordered Protein. Proteins 72 (3), 822–836. doi:10.1002/prot.21974
Power H. E., Sinclair R., Savagaon K. (1968). Use of EDTA Compounds for the Preservation of Haddock Fillets. J. Fish. Res. Bd. Can. 25 (10), 2071–2082. doi:10.1139/f68-184
Rubin Z. E., Gu H., Polk B. I. (2020). Seafood Graded Oral Food Challenge Outcomes in a Pediatric Tertiary Care Center. World Allergy Organ. J. 13 (5), 100121. doi:10.1016/j.waojou.2020.100121
Schrama D., Cerqueira M., Raposo C. S., Rosa da Costa A. M., Wulff T., Gonçalves A., et al. (2018). Dietary Creatine Supplementation in Gilthead Seabream (Sparus aurata): Comparative Proteomics Analysis on Fish Allergens, Muscle Quality, and Liver. Front. Physiol. 9, 1844. doi:10.3389/fphys.2018.01844
Schrama D., Raposo de Magalhães C., Cerqueira M., Carrilho R., Farinha A. P., Rosa da Costa A. M., et al. (2022). Effect of Creatine and EDTA Supplemented Diets on European Seabass (Dicentrarchus labrax) Allergenicity, Fish Muscle Quality and Omics Fingerprint. Comp. Biochem. Physiology Part D Genomics Proteomics 41, 100941. doi:10.1016/j.cbd.2021.100941
Schrama D., Richard N., Silva T. S., Figueiredo F. A., Conceição L. E. C., Burchmore R., et al. (2017). Enhanced Dietary Formulation to Mitigate Winter Thermal Stress in Gilthead Sea Bream (Sparus aurata): a 2D-DIGE Plasma Proteome Study. Fish. Physiol. Biochem. 43 (2), 603–617. doi:10.1007/s10695-016-0315-2
Shalaby A. M. E. (2007). Effect of EDTA on Toxicity Reduction of Cadmium in Relation to Growth, Some Haematological and Biochemical Profiles of Nile tilapia (Oreochromis niloticus). J. Fish. Aquatic Sci. 2 (2), 100–109. doi:10.3923/jfas.2007.100.109
Sharp M. F., Taki A. C., Ruethers T., Stephen J. N., Daly N. L., Lopata A. L., et al. (2021). IgE and IgG4 Epitopes Revealed on the Major Fish Allergen Lat C 1. Mol. Immunol. 131, 155–163. doi:10.1016/j.molimm.2020.12.033
Simione M., Harshman S. G., Castro I., Linnemann R., Roche B., Ajami N. J., et al. (2020). Maternal Fish Consumption in Pregnancy Is Associated with a Bifidobacterium-Dominant Microbiome Profile in Infants. Curr. Dev. Nutr. 4 (1), nzz133. doi:10.1093/cdn/nzz133
Sørensen M., Kuehn A., Mills E. N. C., Costello C. A., Ollert M., Småbrekke L., et al. (2017). Cross-reactivity in Fish Allergy: a Double-Blind, Placebo-Controlled Food-Challenge Trial. J. Allergy Clin. Immunol. 140 (4), 1170–1172. doi:10.1016/j.jaci.2017.03.043
Swoboda I., Balic N., Klug C., Focke M., Weber M., Spitzauer S., et al. (2013). A General Strategy for the Generation of Hypoallergenic Molecules for the Immunotherapy of Fish Allergy. J. Allergy Clin. Immunol. 132 (4), 979–981. doi:10.1016/j.jaci.2013.04.027
Swoboda I., Bugajska-Schretter A., Linhart B., Verdino P., Keller W., Schulmeister U., et al. (2007). A Recombinant Hypoallergenic Parvalbumin Mutant for Immunotherapy of IgE-Mediated Fish Allergy. J. Immunol. 178 (10), 6290–6296. doi:10.4049/jimmunol.178.10.6290
Tacon A. G. J., Lemos D., Metian M. (2020). Fish for Health: Improved Nutritional Quality of Cultured Fish for Human Consumption. Rev. Fish. Sci. Aquac. 28 (4), 449–458. doi:10.1080/23308249.2020.1762163
Tedner S. G., Asarnoj A., Thulin H., Westman M., Konradsen J. R., Nilsson C. (2022). Food Allergy and Hypersensitivity Reactions in Children and Adults-A Review. J. Intern Med. 291 (3), 283–302. doi:10.1111/joim.13422
Tomura S., Ishizaki S., Nagashima Y., Shiomi K. (2008). Reduction in the IgE Reactivity of Pacific Mackerel Parvalbumin by Mutations at Ca2+-Binding Sites. Fish. Sci. 74 (2), 411–417. doi:10.1111/j.1444-2906.2008.01538.x
Ugajin T., Kobayashi Y., Takayama K., Yokozeki H. (2021). A Parvalbumin Allergy Case Was Successfully Treated with Oral Immunotherapy Using Hypoallergenic Fish. Allergol. Int. 70 (4), 509–511. doi:10.1016/j.alit.2021.03.003
Wu Y., Lu Y., Huang Y., Lin H., Chen G., Chen Y., et al. (2022). Glycosylation Reduces the Allergenicity of Turbot (Scophthalmus maximus) Parvalbumin by Regulating Digestibility, Cellular Mediators Release and Th1/Th2 Immunobalance. Food Chem. 382, 132574. doi:10.1016/j.foodchem.2022.132574
Xepapadaki P., Christopoulou G., Stavroulakis G., Freidl R., Linhart B., Zuidmeer L., et al. (2021). Natural History of IgE-Mediated Fish Allergy in Children. J. Allergy Clin. Immunol. Pract. 9 (8), 3147–3156. doi:10.1016/j.jaip.2021.04.007
Younes M., Younes M., Aggett P., Aguilar F., Crebelli R., Dusemund B., et al. (2018). Scientific Opinion on the Evaluation of Authorised Ferric Sodium EDTA as an Ingredient in the Context of Regulation (EC) 258/97 on Novel Foods and Regulation (EU) 609/2013 on Food Intended for Infants and Young Children, Food for Special Medical Purposes and Total Diet Replacement for Weight Control. EFSA. J. 16 (8), e05369. doi:10.2903/j.efsa.2018.5369
Keywords: fish allergies, fish nutrition, parvalbumin, creatine, EDTA
Citation: Schrama D, Czolk R, Raposo de Magalhães C, Kuehn A and Rodrigues PM (2022) Fish Allergenicity Modulation Using Tailored Enriched Diets—Where Are We?. Front. Physiol. 13:897168. doi: 10.3389/fphys.2022.897168
Received: 16 March 2022; Accepted: 04 May 2022;
Published: 25 May 2022.
Edited by:
Carlo C. Lazado, Norwegian Institute of Food, Fisheries and Aquaculture Research (Nofima), NorwayReviewed by:
Monica Carrera, Spanish National Research Council (CSIC), SpainBimal Prasanna Mohanty, Indian Council of Agricultural Research (ICAR), India
Copyright © 2022 Schrama, Czolk, Raposo de Magalhães, Kuehn and Rodrigues. This is an open-access article distributed under the terms of the Creative Commons Attribution License (CC BY). The use, distribution or reproduction in other forums is permitted, provided the original author(s) and the copyright owner(s) are credited and that the original publication in this journal is cited, in accordance with accepted academic practice. No use, distribution or reproduction is permitted which does not comply with these terms.
*Correspondence: Pedro M. Rodrigues, pmrodrig@ualg.pt