- 1Molecular Imaging and Theranostics Laboratory, Baker Heart and Diabetes Institute, Melbourne, VIC, Australia
- 2Baker Department of Cardiometabolic Health, University of Melbourne VIC, Melbourne, VIC, Australia
- 3Department of Microbiology, Anatomy, Physiology and Pharmacology, La Trobe University, Bundoora, VIC, Australia
- 4Department of Anatomy and Physiology, University of Melbourne, Parkville, VIC, Australia
- 5Cardiovascular Endocrinology Laboratory, Baker Heart and Diabetes Institute, Melbourne, VIC, Australia
The global burden of ischemic heart disease is burgeoning for both men and women. Although advances have been made, the need for new sex-specific therapies targeting key differences in cardiovascular disease outcomes in men and women remains. Mineralocorticoid receptor directed treatments have been successfully used for blood pressure control and heart failure management and represent a potentially valuable therapeutic option for ischemic cardiac events. Clinical and experimental data indicate that mineralocorticoid excess or inappropriate mineralocorticoid receptor (MR) activation exacerbates ischemic damage, and many of the intracellular response pathways activated in ischemia and subsequent reperfusion are regulated by MR. In experimental contexts, where MR are abrogated genetically or mineralocorticoid signaling is suppressed pharmacologically, ischemic injury is alleviated, and reperfusion recovery is enhanced. In the chronic setting, mineralocorticoid signaling induces fibrosis, oxidative stress, and inflammation, which can predispose to ischemic events and exacerbate post-myocardial infarct pathologies. Whilst a range of cardiac cell types are involved in mineralocorticoid-mediated regulation of cardiac function, cardiomyocyte-specific MR signaling pathways are key. Selective inhibition of cardiomyocyte MR signaling improves electromechanical resilience during ischemia and enhances contractile recovery in reperfusion. Emerging evidence suggests that the MR also contribute to sex-specific aspects of ischemic vulnerability. Indeed, MR interactions with sex steroid receptors may differentially regulate myocardial nitric oxide bioavailability in males and females, potentially determining sex-specific post-ischemic outcomes. There is hence considerable impetus for exploration of MR directed, cell specific therapies for both women and men in order to improve ischemic heart disease outcomes.
Introduction
Ischemic heart disease is a leading cause of mortality and morbidity for both men and women (Murray et al., 2012; Wong, 2014). Although the burden of ischemic heart disease has steadily decreased in higher-income countries over the past 25 years, the combination of population growth and aging has led to a 35% increase in deaths from ischemic heart disease globally since 1990 (Moran et al., 2014). Ischemic heart disease is most commonly associated with major coronary vessel atherosclerotic occlusion eventuating in myocardial infarction (MI), though impaired microvascular function is increasingly implicated as an underlying mechanism, especially in women (Maric-Bilkan et al., 2016; Mehta et al., 2016). While survival following a sudden cardiac event has improved, the impact of chronic cardiovascular disease post-MI is increasing. Despite new therapeutic interventions significantly improving acute MI survival, the probability of mortality within 5 years after a first MI remains at approximately 50% (Mozaffarian et al., 2015). Women are especially vulnerable to premature death within 1 and 5 years post-MI regardless of age (Mehta et al., 2016), and are more likely to develop heart failure (Leening et al., 2014). A new generation of novel molecular candidate targets for optimal therapeutic interventions in men and women with ischemic heart disease is urgently required. Mineralocorticoid receptor (MR) directed treatments have emerged as a valuable therapeutic approach in this setting (Buonafine et al., 2018). This review focuses on the clinical/pre-clinical evidence relating to MR influence in the myocardium, and the therapeutic potential for MR antagonists in treating or preventing cardiac ischemia and reperfusion injury in both men and women.
Mineralocorticoid Receptor Activation and Cardiac Ischemic Injury
The MR is a steroid hormone receptor present in many cell types within the myocardium (Young and Clyne, 2021). MR activation by endogenous ligands aldosterone and cortisol (corticosterone in rodents) is conveyed by a combination of rapid (within minutes) “non-genomic” MR signaling pathways and “genomic” gene transcription and protein synthesis over hours-days (Alzamora et al., 2000; Hayashi et al., 2008; Nolly et al., 2014; Hermidorff et al., 2017; Ong and Young, 2017). The MR was initially recognized for its role in sodium and water homeostasis which occurs via direct regulation of sodium, potassium, and other electrolyte handling proteins in the distal nephron. Extensive experimental and clinical studies have highlighted the direct detrimental impact inappropriate MR activation has on the cardiovascular system (Young and Rickard, 2015).
Increased activation of MR via inappropriately elevated mineralocorticoid levels or tissue injury are associated with cardiovascular comorbidity and structural remodeling, including fibrosis and myocardial hypertrophy, thereby predisposing the ischemic heart to poor outcomes (Young and Clyne, 2021). MR antagonists have proven benefit for patients with all cause heart failure, with heart failure post-MI and in patients with moderate heart failure, and show potential for patients with heart failure with preserved ejection fraction (HFpEF) (Pitt et al., 1999; Pitt et al., 2003; Markowitz et al., 2012; Miller and Howlett, 2015). Clinical studies (Table 1) indicate that MR antagonists are underutilized and can provide specific benefit for patients with acute MI and high aldosterone levels (Beygui et al., 2009; Rao et al., 2013; Wong et al., 2021). Serum biomarkers for collagen turnover within the RALES and EPHESUS trials indicated MR suppression limits structural remodeling of the extracellular matrix in all cause heart failure and post-MI. However, clinical trials of MR antagonists also suggest that MI classification, heart failure status and timing of MR antagonist administration remain important factors in determining post-MI cardiovascular outcomes (Bulluck et al., 2019; Chen et al., 2021; Mares et al., 2022). The ALBATROSS trial concluded that early MR antagonist administration initiated within 72 h post-MI and prior to the onset of heart failure did not improve patient outcomes and survival six months post-MI (Beygui et al., 2016). In contrast, data from larger cohort populations in combination with results of the REMINDER trial indicated that MR antagonist use reduces the rate of cardiovascular related death irrespective of heart failure status (Beygui et al., 2018). Similarly, the MINIMISE trial reported mixed outcomes in acute ST-segment elevation myocardial infarction (STEMI) patients when MR antagonist was administered immediately prior to reperfusion (Bulluck et al., 2019). In this study infarct size at 3 months was unaffected, while left ventricular remodeling was reduced by MR antagonist therapy. This relative protection from structural remodeling prior to the onset of heart failure post-MI may be key to the benefits observed with MR antagonist administration. With the ongoing development of non-steroidal MR antagonists, additional trials will be required to determine their efficacy in different patient cohorts over an extended period post-MI to improve outcomes.
Ischemia, Reperfusion, and the Mineralocorticoid Receptor
The mechanisms that underlie the tissue response to injury during ischemia/reperfusion and the progression to cardiac dysfunction have been studied extensively (Davidson et al., 2019). Interruption of coronary flow to the myocardium impairs cardiomyocyte steady-state metabolism, ultimately leading to dysfunction, arrhythmias, and cell death. The cellular response involves a complex series of events during ischemia can lead to cross-sarcolemmal ion imbalance, activation of stress-responsive intracellular signaling pathways and disruption of critical metabolic processes that contribute to the cardiac pathology. Reperfusion of the myocardium is hence essential to salvage viable myocardium, though this in itself can exacerbate the demise of “at risk” cardiomyocytes (Heusch, 2020). The loss of myocardium culminates in fibrosis and scarring, disrupting normal conduction pathways, which can promote vulnerability to arrhythmia and increase myocardial stiffness. Compensatory hypertrophic growth of the surviving myocardium post-MI maintains functional capacity in the short term, but ultimately the myocardium is unable to compensate for increased wall pressures leading to failure of myocardial pump function and death.
Preclinical and clinical studies further indicate aldosterone excess is a damage provocateur in the ischemic context (Tables 1 and 2). Stimulation of MR by cortisol or aldosterone increase infarct size in ex vivo rat hearts even at low doses (Mihailidou et al., 2009), via mechanisms that are at least partly attributable to greater cardiomyocyte apoptotic vulnerability. Cardiomyocytes demonstrate aldosterone-induced apoptosis via rapid activation of calcineurin and NADPH oxidase/apoptosis signal-regulating kinase one signaling complexes (ASK1) (Mano et al., 2004; Hayashi et al., 2008). The activated MR also mediates upregulation of Ca2+ influx that augments cardiomyocyte apoptosis (Ferron et al., 2011). These detrimental actions are exacerbated by MR potentiation of reactive fibrotic remodeling (Brilla and Weber, 1992; Brilla, 2000; Rickard et al., 2009). Exposure of rodents to exogenous mineralocorticoids upregulates transcription of genes underlying extracellular matrix turnover and cardiac remodeling signaling cascades (Rude et al., 2005; Tsai et al., 2013). Together, these findings highlight the potential for MR inhibition to minimize myocardial remodeling following ischemia/reperfusion injury via both genomic and potentially non-genomic pathways.
Pre-Ischemic Regulation of Mineralocorticoid Receptor Activity
Many studies have assessed the conditioning capacity of pharmacological agents to minimize injury when administered prior to the ischemic insult. Both long-term and acute inhibition of MR prior to an ischemic event has been shown to be beneficial. Spironolactone administered to rats for 1 month minimized ischemic contracture in isolated hearts subjected to 25 min low-flow ischemia (Rochetaing et al., 2003), indicating a Ca2+-dependent mechanism underlying greater myocardial tolerance to the ischemic challenge. During reperfusion, hearts from rats receiving spironolactone exhibited greater functional recovery and less ventricular arrhythmias. Similarly, hearts perfused with 1 μM eplerenone immediately prior ischemia exhibited improved functional recovery in reperfusion and reduced infarct size (Chai et al., 2005). Paradoxically, aldosterone administered prior to ischemia has also been shown to significantly improve contractile function in reperfusion (Yoshino et al., 2014). This observation appears to be mediated by an MR-independent mechanism, for example via rapid activation of p38-MAPK which is an important mediator of ischemic preconditioning (Bassi et al., 2008; Bell et al., 2008).
Targeting Reperfusion Injury—A Role for Pharmacological Regulation of the Mineralocorticoid Receptor
Studies administering pharmacological agents prior to an ischemic insult have both provided considerable insight into the mechanisms of ischemia/reperfusion and identified numerous conditioning agents that could benefit patients undergoing cardiac surgery (Venugopal et al., 2009). However, the practical application of such agents in the clinical setting of a sudden, major ischemic event is very limited and the development of cardioprotective agents that can be administered at the time of reperfusion is a top priority.
Early studies showed that treatment with MR antagonists post-MI had little or no effect on the progression of infarct-healing but can prevent development of reactive fibrosis in the viable rodent myocardium (Delyani et al., 2001; Mill et al., 2003). However, in subsequent studies MR antagonists were found to be functionally beneficial following in vivo-MI in rodents for reducing fibrosis of viable myocardium, abrogating increases in left ventricular end diastolic pressure and left ventricular end diastolic volume, and maintaining left ventricular function (Cittadini et al., 2003; Fraccarollo et al., 2003; Fraccarollo et al., 2005). Eplerenone administered post-MI also reduces the onset and progress of cardiac tissue fibrosis (myocardial and aortic), enhances left ventricle ejection fraction and cardiac output, and limits left ventricle systolic area and weight independently of blood pressure (Masson et al., 2004; Wang et al., 2004). Thus, the timing of MR antagonist therapy is key to the functional and gene expression outcome in vivo. In addition to fibrotic and functional outcomes, MR blockers improve neovascular formation and reduce thinning and dilation of infarcted myocardial walls at early (3 days) and late (7 weeks) time points in experimental rodents. This action underpins the improvement in ventricular wall function and is associated with transient up-regulation of monocyte chemoattractant protein 1 (MCP-1), early monocyte and macrophage infiltration and expression of tumour necrosis factor alpha (TNFα) (Fraccarollo et al., 2008). These beneficial outcomes are mirrored in transgenic mice lacking the MR in cardiomyocytes, underscoring the central role of the receptor for the cardiomyocyte response to ischemia/reperfusion and are discussed further below (Fraccarollo et al., 2011).
Investigation of the effects of MR suppression on global remodeling of the left ventricular chamber have reported variable findings. In a rat model of MI, spironolactone showed no benefit for reducing left ventricle cardiac chamber mass index and wall thickness despite reduced cardiomyocyte cross sectional area and less fibrosis (Enomoto et al., 2005). Whereas other studies show reversal of left ventricle dilation and dysfunction with MR blockade post-MI via mechanisms that include suppression of NADPH oxidase and mitochondrial superoxide production (Matsumoto et al., 2004).
Genetic Manipulation of Mineralocorticoid Receptor Signaling Reveals Ischemic Vulnerability
To more precisely understand the specific myocardial cell types involved in mediating MR-dependent adverse and beneficial outcomes, targeted genetic manipulation studies have proved to be particularly informative (Table 2). Initial MR deletion mouse models were homologous MR knockout, which displayed neonatal lethality due to sodium wasting (Berger et al., 1998). Subsequent studies focused on genetic manipulation of MR in specific cell types, including macrophages, vascular smooth muscle, endothelial and cardiomyocytes (Young and Rickard, 2015). The cardiomyocyte MR knockout mouse (myo-MRKO) has demonstrated a range of novel and important actions of the MR in the regulation of the tissue response to cardiac ischemia including promoting an appropriate wound healing response in the infarct zone, enhanced neovascularization of the scar and maintenance of the microvascular capillary network, which together support cardiac functional recovery and long term viability (Fraccarollo et al., 2011).
Our studies subjecting ex vivo myo-MRKO hearts to an acute IR challenge demonstrated improved contractile functional recovery and lower vulnerability to arrhythmias compared with wild-type controls (Bienvenu et al., 2015). This was associated with reduced expression of the sodium-hydrogen exchanger (NHE-1) and reduced CaMKII autophosphorylation, both of which are predicted to minimize cardiomyocyte Na+ and Ca2+ loading and suppress cardiac dysfunction in IR (Bell et al., 2014; Mattiazzi et al., 2015; Shattock et al., 2015). Genetic inhibition of CaMKII combined with MR antagonist treatment improved functional recovery and reduced diffuse fibrosis, suggesting that targeting both pathways can potentially improve contractile performance and reduce arrhythmic activity (Driessen et al., 2020). In a chronic model of MI, suppressing cardiomyocyte MR signaling was also beneficial. Targeted ablation of cardiomyocyte MR did not affect infarct size in vivo, yet morphological changes were minimized, and ventricular function better maintained in the subsequent 8 weeks post-MI (Fraccarollo et al., 2011). Expression of genes associated with hypertrophy, stiffness and fibrosis was lower in surviving myocardium from myo-MRKO mice and myocardial/mitochondrial superoxide production was diminished (Fraccarollo et al., 2011). The authors concluded that a suppressed NFκB-mediated inflammatory response was key to minimizing apoptosis and enhancing healing in these mice.
Deletion of MR in other specific cell types of the myocardium (vascular smooth muscle, endothelial, macrophage cells) demonstrate cell-specific regulation of myocardial injury and repair pathways in a manner that would be predicted to confer protection in the ischemic setting (Bienvenu et al., 2012; Gueret et al., 2016; Fraccarollo et al., 2019). This demonstrates the importance of MR signaling across numerous cardiac cell types in the ischemic context. This may be critical to determining how targeting MR signaling may be optimized post-MI. Further studies are hence required to explore in greater detail how MR-mediated regulation of different cell populations within the heart interact to determine the functional and morphological responses to IR (Figure 1).
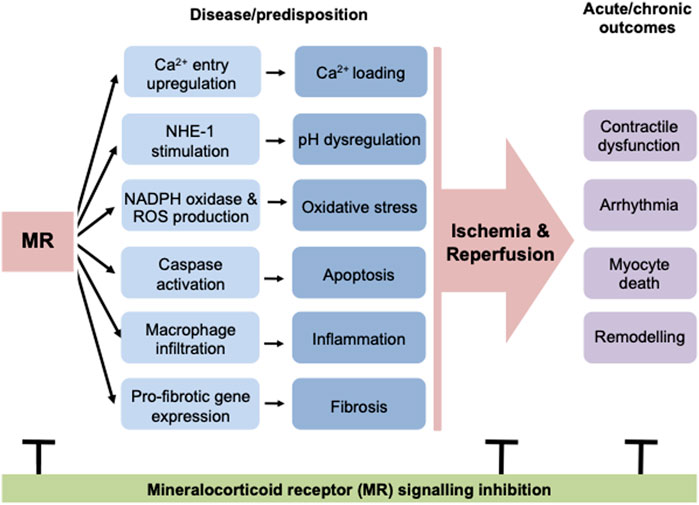
FIGURE 1. MR activation leads to many detrimental changes in the heart, including cardiomyocyte cell death, inflammation and fibrosis. In addition to these structural changes, MR signaling leads to stimulation of calcium and sodium flux in cardiomyocytes, predisposing these cells to calcium loading and pH dysregulation. Overall these modulations of basal cardiac structure and function predispose the heart to ischemic events and result in worse outcomes after ischemia/reperfusion, increasing the incidence of contractile dysfunction and arrhythmia and also increasing cell death and consequently reactive fibrosis. The negative impacts of MR activation at a structural and functional level can be abrogated at early and late timepoints, both before and after an ischemic event. MR, mineralocorticoid receptor; NHE-1, sodium hydrogen exchanger-1; ROS, reactive oxygen species.
Mineralocorticoid Receptor Signaling, Sex Specificity and Cardioprotection
The incidence of cardiovascular disease (and more specifically ischemic heart disease) differs between the sexes, with earlier onset in men and increasing incidence in women post-menopause (Leening et al., 2014). Men and women exhibit differences in symptom presentation, efficacy of diagnosis and response to interventions (McSweeney et al., 2016). More specifically, in relation to myocardial infarction, sex-specific differences in pathophysiological mechanisms and outcomes is now being emphasized (Mehta et al., 2016). Women are more likely to develop heart failure after an acute MI, underscoring the need for new strategies and therapies to adequately address long term MI recovery in both sexes (Westerman and Wenger, 2016).
Most clinical studies assessing suppression of MR signaling have involved predominantly male cohorts, and even though data are adjusted to consider sex as a covariate, differences in clinical outcomes between men and women may not be observed (Pitt et al., 2003; Kanashiro-Takeuchi et al., 2009; Nicolaou, 2021). Moreover, serum aldosterone levels have been strongly correlated with left ventricular mass in females, but not males even when serum aldosterone levels are not elevated and the MR inhibitory effect of progesterone is taken into consideration (Vasan et al., 2004). Given the importance of cardiac hypertrophy and serum aldosterone levels as independent cardiovascular disease risk factors, this observation indicates that some of the differences observed for males and females in the clinical setting may also have an MR-dependent mechanism. Endothelial MR expression has been shown to be higher in female vessel wall, when compared with males (Faulkner et al., 2019). This observation reflects the fact that females have higher circulating levels of progesterone which is a natural antagonist of the MR and estrogen acting via the ERα inhibits MR activation. Higher aldosterone levels and potentially tissue expression of MR in females is likely to be compensatory in premenopausal women.
Steroid hormone ligands for both receptors derive from the same cholesterol-derived progestogen pre-cursors. Relative levels of corticosteroids and sex steroids are hence closely linked. It is well established that estrogen receptors (ER) and MR are co-expressed in cardiomyocytes, fibroblasts and vascular cells (Lombès et al., 1995; Grohe et al., 1997), and that these can modulate cardiac structure and function in settings of IR. Transgenic animal models have shown that female hearts overexpressing ERα receptor subtype are relatively protected from MI-induced fibrosis and exhibit improved neovascularization (Mahmoodzadeh et al., 2014). Overexpression of the ERβ receptor subtype improves survival and cardiac function post-MI in both sexes, due in part to reduced disturbance of cardiomyocyte intracellular Ca2+ store management (Bell et al., 2013). In male hearts specifically, functional improvement was related to a lower level of cardiac fibrosis highlighting the role of ERβ in post-infarct remodeling (Schuster et al., 2016). In rodent models activation of both ERα and ERβ protects against the detrimental blood pressure, fibrotic and hypertrophic effects of mineralocorticoid excess (Arias-Loza et al., 2007). For example, deoxycorticosterone/salt-induced cardiovascular damage is reduced in females with intact ER signaling. Whereas genetic ablation of ERβ signaling produces a differential response to mineralocorticoid excess-induced cardiac fibrosis associated with mTOR (mammalian target of rapamycin) activation in both male and female mice (Gurgen et al., 2011; Gurgen et al., 2013). Moreover, the transcriptional activity of vascular MR can be inhibited by ERα, suggesting that MR/ER interactions contribute to the mechanisms of sex differences in cardiac MR activity (Barrett Mueller et al., 2014; Biwer et al., 2021). Rapid aldosterone effects may also be medicated by the G-protein coupled ER, though evidence of direct aldosterone binding remains contentious (Rossol-Haseroth et al., 2004; Michea et al., 2005; Gros et al., 2011; Gros et al., 2013). An important consideration moving forward will be developing a more detailed understanding of the nature of the interaction of MR with all ER subtypes in influencing genomic and rapid activation signaling pathways (Funder, 2011).
Experimental studies that specifically address sex-difference in MR involvement in cardiac ischemia are limited but some important leads have been reported. Suppression of MR signaling in rodents by eplerenone administered after MI has been shown to achieve more effective attenuation of left ventricular end diastolic volume enlargement in female compared to male hearts (Kanashiro-Takeuchi et al., 2009). Additionally, LV ejection fraction was increased in female hearts. Transcriptome analysis revealed that for female hearts, eplerenone reversed transcriptional responses for 19% of down regulated genes and for 44% of up-regulated genes, whereas only 4% of genes up-regulated in male hearts responded to eplerenone. These data indicate that MR blockade may preferentially reduce structural and functional changes in female hearts through initiation of specific transcriptional responses (Kanashiro-Takeuchi et al., 2009).
Nitric oxide (NO) signaling is known to be important in mediating cardioprotection (Griffiths et al., 2021)—and there is clear evidence of MR involvement in NO modulation in endothelial and other cell types. Heart failure is associated with low levels of bioavailable NO, and clinical evidence indicates sex differences in NO mediated responses (Zamani et al., 2015). In addition, an imbalance of NO levels has been shown to be a key component in the development of heart failure with preserved ejection fraction in males, but not necessarily females (Schiattarella et al., 2019; Tong et al., 2019). There is extensive experimental evidence to indicate that in female cardiac disease states, NO production and involvement in ROS modulation is important (Murphy et al., 2011; Casin et al., 2018). Targeting NO bioavailability is therefore considered an attractive target for sex-specific therapies in heart failure, but less is known about possible benefits in ischemic disease states. Given that there is evidence for an MR-NO signaling link in endothelial cells, regulating the MR has the potential to optimize NO conditions and support cardiomyocyte protection during cardiac ischemia/reperfusion (Jia et al., 2016; Victorio et al., 2016). Our experimental findings have shown that after chronic in vivo treatment with an NO-synthase inhibitor, male and female cardiomyocyte specific MR-KO mice exhibit different cardiac ex vivo responses. While female hearts showed MR-dependent abrogation of NO-deficiency induced ischemic injury (reperfusion arrhythmia, diastolic dysfunction and impaired contractile recovery), male animals did not exhibit a similar MR-NO deficiency response interaction (Bienvenu et al., 2017). More recently, it has been shown that ERα mediated NO production can abrogate the detrimental impact of MR activation in the microvasculature of obese female rodents, highlighting the interplay between MR and ER in the endothelium (Biwer et al., 2021). Further work is required to define the nature of the signaling relationship between MR, ER, and NO in order to effectively exploit this signaling axis for therapeutic outcomes in tissue injury due to ischemia and reperfusion.
Conclusion
In this review, the accumulating clinical and preclinical data indicating important involvement of MR signaling in mediating both acute and longer term cardiac ischemic damage have been considered. Whilst a range of cardiac cell types are involved (macrophages, endothelial, and vascular smooth muscle), the cardiomyocyte-specific mineralocorticoid signaling pathways appear to be key. Evidence supports a role for increased aldosterone levels and MR activation in mediating sex-specific aspects of ischemic vulnerability through MR-ER receptor interactions providing important insights into ischemic heart disease in women. While early clinical trials of MRA showed equal protection for heart failure in females as well as males, discrepancies remain between translation of experimental outcomes and observed clinical sex-differences in the etiology and diagnosis of heart failure. Thus, there is considerable impetus for exploration of mineralocorticoid-directed, cell-specific therapies for both women and men in order to improve ischemic heart disease outcomes. Specific, ongoing challenges involve dissecting the integrative nature of the MR-ER-NO signaling axis so that sex-specific therapies can be identified to address both acute and chronic phases of ischemic injury. In this setting, preclinical mechanistic investigations of cell specific MR interactions with pathways regulating ischemia injury have considerable capacity to inform ongoing clinical studies.
Author Contributions
LB and JB contributed equally to this manuscript and share first authorship. LD and MY contributed equally to this manuscript and share senior authorship. All authors (LB, JB, KW, LD, MY) contributed to the drafting of the manuscript and approved the submitted version.
Conflict of Interest
The authors declare that the research was conducted in the absence of any commercial or financial relationships that could be construed as a potential conflict of interest.
Publisher’s Note
All claims expressed in this article are solely those of the authors and do not necessarily represent those of their affiliated organizations, or those of the publisher, the editors and the reviewers. Any product that may be evaluated in this article, or claim that may be made by its manufacturer, is not guaranteed or endorsed by the publisher.
References
Alzamora R., Michea L., Marusic E. T. (2000). Role of 11β-Hydroxysteroid Dehydrogenase in Nongenomic Aldosterone Effects in Human Arteries. Hypertension 35 (5), 1099–1104. doi:10.1161/01.hyp.35.5.1099
Arias-Loza P.-A., Hu K., Dienesch C., Mehlich A. M., König S., Jazbutyte V., et al. (2007). Both Estrogen Receptor Subtypes, α and β, Attenuate Cardiovascular Remodeling in Aldosterone Salt-Treated Rats. Hypertension 50 (2), 432–438. doi:10.1161/HYPERTENSIONAHA.106.084798
Barrett Mueller K., Lu Q., Mohammad N. N., Luu V., McCurley A., Williams G. H., et al. (2014). Estrogen Receptor Inhibits Mineralocorticoid Receptor Transcriptional Regulatory Function. Endocrinology 155 (11), 4461–4472. doi:10.1210/en.2014-1270
Bassi R., Heads R., Marber M., Clark J. (2008). Targeting P38-MAPK in the Ischaemic Heart: Kill or Cure? Curr. Opin. Pharmacol. 8 (2), 141–146. doi:10.1016/j.coph.2008.01.002
Bell J. R., Bernasochi G. B., Varma U., Raaijmakers A. J. A., Delbridge L. M. D. (2013). Sex and Sex Hormones in Cardiac Stress-Mechanistic Insights. J. Steroid Biochem. Mol. Biol. 137, 124–135. doi:10.1016/j.jsbmb.2013.05.015
Bell J. R., Eaton P., Shattock M. J. (2008). Role of P38-Mitogen-Activated Protein Kinase in Ischaemic Preconditioning in Rat Heart. Clin. Exp. Pharmacol. Physiol. 35 (2), 126–134. doi:10.1111/j.1440-1681.2007.04794
Bell J. R., Vila-Petroff M., Delbridge L. M. D. (2014). CaMKII-dependent Responses to Ischemia and Reperfusion Challenges in the Heart. Front. Pharmacol. 5, 96. doi:10.3389/fphar.2014.00096
Berger S., Bleich M., Schmid W., Cole T. J., Peters J., Watanabe H., et al. (1998). Mineralocorticoid Receptor Knockout Mice: Pathophysiology of Na + Metabolism. Proc. Natl. Acad. Sci. U.S.A. 95 (16), 9424–9429. doi:10.1073/pnas.95.16.9424
Beygui F., Cayla G., Roule V., Roubille F., Delarche N., Silvain J., et al. (2016). Early Aldosterone Blockade in Acute Myocardial Infarction. J. Am. Coll. Cardiol. 67 (16), 1917–1927. doi:10.1016/j.jacc.2016.02.033
Beygui F., Montalescot G., Vicaut E., Rouanet S., Van Belle E., Baulac C., et al. (2009). Aldosterone and long-term outcome after myocardial infarction: A substudy of the French nationwide Observatoire sur la Prise en charge hospitalière, l'Evolution à un an et les caRactéristiques de patients présentant un infArctus du myocarde avec ou sans onde Q (OPERA) study. Am. Heart J. 157 (4), 680–687. doi:10.1016/j.ahj.2008.12.013
Beygui F., Van Belle E., Ecollan P., Machecourt J., Hamm C. W., Lopez De Sa E., et al. (2018). Individual Participant Data Analysis of Two Trials on Aldosterone Blockade in Myocardial Infarction. Heart 104 (22), 1843–1849. doi:10.1136/heartjnl-2018-312950
Bienvenu L. A., Morgan J., Reichelt M. E., Delbridge L. M. D., Young M. J. (2017). Chronic In Vivo Nitric Oxide Deficiency Impairs Cardiac Functional Recovery after Ischemia in Female (But Not Male) Mice. J. Mol. Cell. Cardiol. 112, 8–15. doi:10.1016/j.yjmcc.2017.08.012
Bienvenu L. A., Morgan J., Rickard A. J., Tesch G. H., Cranston G. A., Fletcher E. K., et al. (2012). Macrophage Mineralocorticoid Receptor Signaling Plays a Key Role in Aldosterone-independent Cardiac Fibrosis. Endocrinology 153 (7), 3416–3425. doi:10.1210/en.2011-2098
Bienvenu L. A., Reichelt M. E., Morgan J., Fletcher E. K., Bell J. R., Rickard A. J., et al. (2015). Cardiomyocyte Mineralocorticoid Receptor Activation Impairs Acute Cardiac Functional Recovery after Ischemic Insult. Hypertension 66 (5), 970–977. doi:10.1161/HYPERTENSIONAHA.115.05981
Biwer L. A., Carvajal B. V., Lu Q., Man J. J., Jaffe I. Z. (2021). Mineralocorticoid and Estrogen Receptors in Endothelial Cells Coordinately Regulate Microvascular Function in Obese Female Mice. Hypertension 77 (6), 2117–2126. doi:10.1161/hypertensionaha.120.16911
Brilla C. G., Weber K. T. (1992). Mineralocorticoid Excess, Dietary Sodium, and Myocardial Fibrosis. J. Lab. Clin. Med. 120 (6), 893–901.
Brilla C. G. (2000). Aldosterone and Myocardial Fibrosis in Heart Failure. Herz 25 (3), 299–306. doi:10.1007/s000590050024
Bulluck H., Fröhlich G. M., Nicholas J. M., Mohdnazri S., Gamma R., Davies J., et al. (2019). Mineralocorticoid Receptor Antagonist Pre-treatment and Early Post-treatment to Minimize Reperfusion Injury after ST-Elevation Myocardial Infarction: The MINIMIZE STEMI Trial. Am. Heart J. 211, 60–67. doi:10.1016/j.ahj.2019.02.005
Buonafine M., Bonnard B., Jaisser F. (2018). Mineralocorticoid Receptor and Cardiovascular Disease. Am. J. Hypertens. 31 (11), 1165–1174. doi:10.1093/ajh/hpy120
Casin K. M., Fallica J., Mackowski N., Veenema R. J., Chan A., St. Paul A., et al. (2018). S -Nitrosoglutathione Reductase Is Essential for Protecting the Female Heart from Ischemia-Reperfusion Injury. Circ. Res. 123 (11), 1232–1243. doi:10.1161/circresaha.118.313956
Chai W., Garrelds I. M., Arulmani U., Schoemaker R. G., Lamers J. M. J., Danser A. H. J. (2005). Genomic and Nongenomic Effects of Aldosterone in the Rat Heart: Why Is Spironolactone Cardioprotective? Br. J. Pharmacol. 145 (5), 664–671. doi:10.1038/sj.bjp.0706220
Chen Q., Zhao D., Sun J., Lu C. (2021). Aldosterone Blockade in Acute Myocardial Infarction: A Systematic Review and Meta-Analysis. Cardiovasc. Ther. 2021, 1–18. doi:10.1155/2021/1710731
Cittadini A., Monti M. G., Isgaard J., Casaburi C., Stromer H., Di Gianni A., et al. (2003). Aldosterone Receptor Blockade Improves Left Ventricular Remodeling and Increases Ventricular Fibrillation Threshold in Experimental Heart Failure. Cardiovasc. Res. 58 (3), 555–564. doi:10.1016/s0008-6363(03)00251-7
Davidson S. M., Ferdinandy P., Andreadou I., Bøtker H. E., Heusch G., Ibáñez B., et al. (2019). Multitarget Strategies to Reduce Myocardial Ischemia/Reperfusion Injury. J. Am. Coll. Cardiol. 73 (1), 89–99. doi:10.1016/j.jacc.2018.09.086
Delyani J. A., Robinson E. L., Rudolph A. E. (2001). Effect of a Selective Aldosterone Receptor Antagonist in Myocardial Infarction. Am. J. Physiology-Heart Circulatory Physiology 281 (2), H647–H654. doi:10.1152/ajpheart.2001.281.2.h647
Driessen H. E., Fontes M. S., Stuijvenberg L., Brans M. A., Goumans M. J., Vos M. A., et al. (2020). A Combined CaMKII Inhibition and Mineralocorticoid Receptor Antagonism via Eplerenone Inhibits Functional Deterioration in Chronic Pressure Overloaded Mice. J. Cell. Mol. Med. 24 (15), 8417–8429. doi:10.1111/jcmm.15355
Enomoto S., Yoshiyama M., Omura T., Matsumoto R., Kusuyama T., Kim S., et al. (2005). Effects of Eplerenone on Transcriptional Factors and mRNA Expression Related to Cardiac Remodelling after Myocardial Infarction. Heart 91 (12), 1595–1600. doi:10.1136/hrt.2004.046540
Faulkner J. L., Kennard S., Huby A.-C., Antonova G., Lu Q., Jaffe I. Z., et al. (2019). Progesterone Predisposes Females to Obesity-Associated Leptin-Mediated Endothelial Dysfunction via Upregulating Endothelial MR (Mineralocorticoid Receptor) Expression. Hypertension 74 (3), 678–686. doi:10.1161/hypertensionaha.119.12802
Ferron L., Ruchon Y., Renaud J.-F., Capuano V. (2011). T-type Ca2+ Signalling Regulates Aldosterone-Induced CREB Activation and Cell Death through PP2A Activation in Neonatal Cardiomyocytes. Cardiovasc Res. 90 (1), 105–112. doi:10.1093/cvr/cvq379
Fraccarollo D., Berger S., Galuppo P., Kneitz S., Hein L., Schütz G., et al. (2011). Deletion of Cardiomyocyte Mineralocorticoid Receptor Ameliorates Adverse Remodeling after Myocardial Infarction. Circulation 123 (4), 400–408. doi:10.1161/CIRCULATIONAHA.110.983023
Fraccarollo D., Galuppo P., Hildemann S., Christ M., Ertl G., Bauersachs J. (2003). Additive Improvement of Left Ventricular Remodeling and Neurohormonal Activation by Aldosterone Receptor Blockade with Eplerenone and ACE Inhibition in Rats with Myocardial Infarction. J. Am. Coll. Cardiol. 42 (9), 1666–1673. doi:10.1016/j.jacc.2003.05.003
Fraccarollo D., Galuppo P., Schmidt I., Ertl G., Bauersachs J. (2005). Additive Amelioration of Left Ventricular Remodeling and Molecular Alterations by Combined Aldosterone and Angiotensin Receptor Blockade after Myocardial Infarction. Cardiovasc. Res. 67 (1), 97–105. doi:10.1016/j.cardiores.2005.03.001
Fraccarollo D., Galuppo P., Schraut S., Kneitz S., van Rooijen N., Ertl G., et al. (2008). Immediate Mineralocorticoid Receptor Blockade Improves Myocardial Infarct Healing by Modulation of the Inflammatory Response. Hypertension 51 (4), 905–914. doi:10.1161/HYPERTENSIONAHA.107.100941
Fraccarollo D., Thomas S., Scholz C.-J., Hilfiker-Kleiner D., Galuppo P., Bauersachs J. (2019). Macrophage Mineralocorticoid Receptor Is a Pleiotropic Modulator of Myocardial Infarct Healing. Hypertension 73 (1), 102–111. doi:10.1161/HYPERTENSIONAHA.118.12162
Funder J. W. (2011). GPR30, Mineralocorticoid Receptors, and the Rapid Vascular Effects of Aldosterone. Hypertension 57 (3), 370–372. doi:10.1161/HYPERTENSIONAHA.110.165076
Griffiths K., Lee J. J., Frenneaux M. P., Feelisch M., Madhani M. (2021). Nitrite and Myocardial Ischaemia Reperfusion Injury. Where Are We Now? Pharmacol. Ther. 223, 107819. doi:10.1016/j.pharmthera.2021.107819
Grohé C., Kahlert S., Löbbert K., Stimpel M., Karas R. H., Vetter H., et al. (1997). Cardiac Myocytes and Fibroblasts Contain Functional Estrogen Receptors 1. FEBS Lett. 416 (1), 107–112. doi:10.1016/s0014-5793(97)01179-4
Gros R., Ding Q., Liu B., Chorazyczewski J., Feldman R. D. (2013). Aldosterone Mediates its Rapid Effects in Vascular Endothelial Cells through GPER Activation. Am. J. Physiology-Cell Physiology 304 (6), C532–C540. doi:10.1152/ajpcell.00203.2012
Gros R., Ding Q., Sklar L. A., Prossnitz E. E., Arterburn J. B., Chorazyczewski J., et al. (2011). GPR30 Expression Is Required for the Mineralocorticoid Receptor-independent Rapid Vascular Effects of Aldosterone. Hypertension 57 (3), 442–451. doi:10.1161/HYPERTENSIONAHA.110.161653
Gueret A., Harouki N., Favre J., Galmiche G., Nicol L., Henry J.-P., et al. (2016). Vascular Smooth Muscle Mineralocorticoid Receptor Contributes to Coronary and Left Ventricular Dysfunction after Myocardial Infarction. Hypertension 67 (4), 717–723. doi:10.1161/HYPERTENSIONAHA.115.06709
Gürgen D., Hegner B., Kusch A., Catar R., Chaykovska L., Hoff U., et al. (2011). Estrogen Receptor-β Signals Left Ventricular Hypertrophy Sex Differences in Normotensive Deoxycorticosterone Acetate-Salt Mice. Hypertension 57 (3), 648–654. doi:10.1161/HYPERTENSIONAHA.110.166157
Gürgen D., Kusch A., Klewitz R., Hoff U., Catar R., Hegner B., et al. (2013). Sex-specific mTOR Signaling Determines Sexual Dimorphism in Myocardial Adaptation in Normotensive DOCA-Salt Model. Hypertension 61 (3), 730–736. doi:10.1161/HYPERTENSIONAHA.111.00276
Hayashi H., Kobara M., Abe M., Tanaka N., Gouda E., Toba H., et al. (2008). Aldosterone Nongenomically Produces NADPH Oxidase−Dependent Reactive Oxygen Species and Induces Myocyte Apoptosis. Hypertens. Res. 31 (2), 363–375. doi:10.1291/hypres.31.363
Hermidorff M. M., de Assis L. V. M., Isoldi M. C. (2017). Genomic and Rapid Effects of Aldosterone: what We Know and Do Not Know Thus Far. Heart Fail Rev. 22 (1), 65–89. doi:10.1007/s10741-016-9591-2
Heusch G. (2020). Myocardial Ischaemia-Reperfusion Injury and Cardioprotection in Perspective. Nat. Rev. Cardiol. 17 (12), 773–789. doi:10.1038/s41569-020-0403-y
Jia G., Habibi J., Aroor A. R., Martinez-Lemus L. A., DeMarco V. G., Ramirez-Perez F. I., et al. (2016). Endothelial Mineralocorticoid Receptor Mediates Diet-Induced Aortic Stiffness in Females. Circ. Res. 118 (6), 935–943. doi:10.1161/CIRCRESAHA.115.308269
Kanashiro-Takeuchi R. M., Heidecker B., Lamirault G., Dharamsi J. W., Hare J. M. (2009). Sex-specific Impact of Aldosterone Receptor Antagonism on Ventricular Remodeling and Gene Expression after Myocardial Infarction. Clin. Transl. Sci. 2 (2), 134–142. doi:10.1111/j.1752-8062.2009.00094.x
Leening M. J. G., Ferket B. S., Steyerberg E. W., Kavousi M., Deckers J. W., Nieboer D., et al. (2014). Sex Differences in Lifetime Risk and First Manifestation of Cardiovascular Disease: Prospective Population Based Cohort Study. BMJ 349, g5992. doi:10.1136/bmj.g5992
Lombès M., Alfaidy N., Eugene E., Lessana A., Farman N., Bonvalet J. P. (1995). Prerequisite for Cardiac Aldosterone Action. Mineralocorticoid Receptor and 11 Beta-Hydroxysteroid Dehydrogenase in the Human Heart. Circulation 92 (2), 175–182. doi:10.1161/01.cir.92.2.175
Mahmoodzadeh S., Leber J., Zhang X., Jaisser F., Messaoudi S., Morano I., et al. (2014). Cardiomyocyte-specific Estrogen Receptor Alpha Increases Angiogenesis,Lymphangiogenesis and Reduces Fibrosis in the Female Mouse Heart Post-Myocardial Infarction. J. Cell. Sci. Ther. 05 (1), 153. doi:10.4172/2157-7013.1000153
Mano A., Tatsumi T., Shiraishi J., Keira N., Nomura T., Takeda M., et al. (2004). Aldosterone Directly Induces Myocyte Apoptosis through Calcineurin-dependent Pathways. Circulation 110 (3), 317–323. doi:10.1161/01.CIR.0000135599.33787.CA
Mares A., Rodriguez T., Deoker A., Lehker A., Mukherjee D. (2022). Effect of Mineralocorticoid Receptor Antagonists in Heart Failure with Preserved Ejection Fraction and with Reduced Ejection Fraction - A Narrative Review. Cvp 20 (1), 46–51. doi:10.2174/1570161119666210720120439
Maric-Bilkan C., Arnold A. P., Taylor D. A., Dwinell M., Howlett S. E., Wenger N., et al. (2016). Report of the National Heart, Lung, and Blood Institute Working Group on Sex Differences Research in Cardiovascular Disease. Hypertension 67 (5), 802–807. doi:10.1161/hypertensionaha.115.06967
Markowitz M., Messineo F., Coplan N. L. (2012). Aldosterone Receptor Antagonists in Cardiovascular Disease: a Review of the Recent Literature and Insight into Potential Future Indications. Clin. Cardiol. 35 (10), 605–609. doi:10.1002/clc.22025
Masson S., Staszewsky L., Annoni G., Carlo E., Arosio B., Bai A., et al. (2004). Eplerenone, a Selective Aldosterone Blocker, Improves Diastolic Function in Aged Rats with Small-To-Moderate Myocardial Infarction. J. Cardiac Fail. 10 (5), 433–441. doi:10.1016/j.cardfail.2004.02.010
Matsumoto R., Yoshiyama M., Omura T., Kim S., Nakamura Y., Izumi Y., et al. (2004). Effects of Aldosterone Receptor Antagonist and Angiotensin II Type I Receptor Blocker on Cardiac Transcriptional Factors and mRNA Expression in Rats with Myocardial Infarction. Circ. J. 68 (4), 376–382. doi:10.1253/circj.68.376
Mattiazzi A., Bassani R. A., Escobar A. L., Palomeque J., Valverde C. A., Vila Petroff M., et al. (2015). Chasing Cardiac Physiology and Pathology Down the CaMKII Cascade. Am. J. Physiology-Heart Circulatory Physiology 308 (10), H1177–H1191. doi:10.1152/ajpheart.00007.2015
McSweeney J. C., Rosenfeld A. G., Abel W. M., Braun L. T., Burke L. E., Daugherty S. L., et al. (2016). Preventing and Experiencing Ischemic Heart Disease as a Woman: State of the Science. Circulation 133 (13), 1302–1331. doi:10.1161/CIR.0000000000000381
Mehta L. S., Beckie T. M., DeVon H. A., Grines C. L., Krumholz H. M., Johnson M. N., et al. (2016). Acute Myocardial Infarction in Women. Circulation 133 (9), 916–947. doi:10.1161/CIR.0000000000000351
Michea L., Delpiano A. M., Hitschfeld C., Lobos L., Lavandero S., Marusic E. T. (2005). Eplerenone Blocks Nongenomic Effects of Aldosterone on the Na+/H+ Exchanger, Intracellular Ca2+ Levels, and Vasoconstriction in Mesenteric Resistance Vessels. Endocrinology 146 (3), 973–980. doi:10.1210/en.2004-1130
Mihailidou A. S., Loan Le T. Y., Mardini M., Funder J. W. (2009). Glucocorticoids Activate Cardiac Mineralocorticoid Receptors during Experimental Myocardial Infarction. Hypertension 54 (6), 1306–1312. doi:10.1161/HYPERTENSIONAHA.109.136242
Mill J. G., Milanez M. d. C., Resende M. M. d., Gomes M. d. G. S., Leite C. M. (2003). Spironolactone Prevents Cardiac Collagen Proliferation after Myocardial Infarction in Rats. Clin. Exp. Pharmacol. Physiol. 30 (10), 739–744. doi:10.1046/j.1440-1681.2003.03906.x
Miller R. J. H., Howlett J. G. (2015). Evolving Role for Mineralocorticoid Receptor Antagonists in Heart Failure with Preserved Ejection Fraction. Curr. Opin. Cardiol. 30 (2), 168–172. doi:10.1097/HCO.0000000000000147
Moran A. E., Forouzanfar M. H., Roth G. A., Mensah G. A., Ezzati M., Flaxman A., et al. (2014). The Global Burden of Ischemic Heart Disease in 1990 and 2010. Circulation 129 (14), 1493–1501. doi:10.1161/CIRCULATIONAHA.113.004046
Mozaffarian D., Benjamin E. J., Go A. S., Arnett D. K., Blaha M. J., Cushman M., et al. (2015). Heart Disease and Stroke Statistics-2015 Update. Circulation 131 (4), e29–322. doi:10.1161/CIR.0000000000000152
Murphy E., Lagranha C., Deschamps A., Kohr M., Nguyen T., Wong R., et al. (2011). Mechanism of Cardioprotection: what Can We Learn from Females? Pediatr. Cardiol. 32 (3), 354–359. doi:10.1007/s00246-010-9877-4
Murray C. J., Vos T., Lozano R., Naghavi M., Flaxman A. D., Michaud C., et al. (2012). Disability-adjusted Life Years (DALYs) for 291 Diseases and Injuries in 21 Regions, 1990-2010: a Systematic Analysis for the Global Burden of Disease Study 2010. Lancet 380 (9859), 2197–2223. doi:10.1016/S0140-6736(12)61689-4
Nicolaou P. A. (2021). Sex Differences in Heart Failure Medications Targeting the Renin-Angiotensin-Aldosterone System. Eur. J. Pharmacol. 897, 173961. doi:10.1016/j.ejphar.2021.173961
Nolly M. B., Caldiz C. I., Yeves A. M., Villa-Abrille M. C., Morgan P. E., Amado Mondaca N., et al. (2014). The Signaling Pathway for Aldosterone-Induced Mitochondrial Production of Superoxide Anion in the Myocardium. J. Mol. Cell. Cardiol. 67, 60–68. doi:10.1016/j.yjmcc.2013.12.004
Ong G. S. Y., Young M. J. (2017). Mineralocorticoid Regulation of Cell Function: the Role of Rapid Signalling and Gene Transcription Pathways. J. Mol. Endocrinol. 58 (1), R33–r57. doi:10.1530/jme-15-0318
Pitt B., Remme W., Zannad F., Neaton J., Martinez F., Roniker B., et al. (2003). Eplerenone, a Selective Aldosterone Blocker, in Patients with Left Ventricular Dysfunction after Myocardial Infarction. N. Engl. J. Med. 348 (14), 1309–1321. doi:10.1056/NEJMoa030207
Pitt B., Zannad F., Remme W. J., Cody R., Castaigne A., Perez A., et al. (1999). The Effect of Spironolactone on Morbidity and Mortality in Patients with Severe Heart Failure. N. Engl. J. Med. 341 (10), 709–717. doi:10.1056/NEJM199909023411001
Rao K. K., Enriquez J. R., de Lemos J. A., Alexander K. P., Chen A. Y., McGuire D. K., et al. (2013). Use of Aldosterone Antagonists at Discharge after Myocardial Infarction: Results from the National Cardiovascular Data Registry Acute Coronary Treatment and Intervention Outcomes Network (ACTION) Registry-Get with the Guidelines (GWTG). Am. Heart J. 166 (4), 709–715. doi:10.1016/j.ahj.2013.06.020
Rickard A. J., Morgan J., Tesch G., Funder J. W., Fuller P. J., Young M. J. (2009). Deletion of Mineralocorticoid Receptors from Macrophages Protects against Deoxycorticosterone/salt-Induced Cardiac Fibrosis and Increased Blood Pressure. Hypertension 54 (3), 537–543.doi:10.1161/hypertensionaha.109.131110
Rochetaing A., Chapon C., Marescaux L., Bouil A. L., Furber A., Kreher P. (2003). Potential Beneficial as Well as Detrimental Effects of Chronic Treatment with Lisinopril and (Or) Spironolactone on Isolated Hearts Following Low-Flow Ischemia in Normal and Infarcted Rats. Can. J. Physiol. Pharmacol. 81 (9), 864–872. doi:10.1139/y03-081
Rossol-Haseroth K., Zhou Q., Braun S., Boldyreff B., Falkenstein E., Wehling M., et al. (2004). Mineralocorticoid Receptor Antagonists Do Not Block Rapid ERK Activation by Aldosterone. Biochem. Biophysical Res. Commun. 318 (1), 281–288. doi:10.1016/j.bbrc.2004.04.026
Rude M. K., Duhaney T.-A. S., Kuster G. M., Judge S., Heo J., Colucci W. S., et al. (2005). Aldosterone Stimulates Matrix Metalloproteinases and Reactive Oxygen Species in Adult Rat Ventricular Cardiomyocytes. Hypertension 46 (3), 555–561. doi:10.1161/01.HYP.0000176236.55322.18
Schiattarella G. G., Altamirano F., Tong D., French K. M., Villalobos E., Kim S. Y., et al. (2019). Nitrosative Stress Drives Heart Failure with Preserved Ejection Fraction. Nature 568 (7752), 351–356. doi:10.1038/s41586-019-1100-z
Schuster I., Mahmoodzadeh S., Dworatzek E., Jaisser F., Messaoudi S., Morano I., et al. (2016). Cardiomyocyte-specific Overexpression of Oestrogen Receptor β Improves Survival and Cardiac Function after Myocardial Infarction in Female and Male Mice. Clin. Sci. (Lond) 130 (5), 365–376. doi:10.1042/CS20150609
Shattock M. J., Ottolia M., Bers D. M., Blaustein M. P., Boguslavskyi A., Bossuyt J., et al. (2015). Na+/Ca2+exchange and Na+/K+-ATPase in the Heart. J. Physiol. 593 (6), 1361–1382. doi:10.1113/jphysiol.2014.282319
Tong D., Schiattarella G. G., Jiang N., May H. I., Lavandero S., Gillette T. G., et al. (2019). Female Sex Is Protective in a Preclinical Model of Heart Failure with Preserved Ejection Fraction. Circulation 140 (21), 1769–1771. doi:10.1161/circulationaha.119.042267
Tsai C.-F., Yang S.-F., Chu H.-J., Ueng K.-C. (2013). Cross-talk between Mineralocorticoid Receptor/angiotensin II Type 1 Receptor and Mitogen-Activated Protein Kinase Pathways Underlies Aldosterone-Induced Atrial Fibrotic Responses in HL-1 Cardiomyocytes. Int. J. Cardiol. 169 (1), 17–28. doi:10.1016/j.ijcard.2013.06.046
Vasan R. S., Evans J. C., Benjamin E. J., Levy D., Larson M. G., Sundstrom J., et al. (2004). Relations of Serum Aldosterone to Cardiac Structure. Hypertension 43 (5), 957–962. doi:10.1161/01.HYP.0000124251.06056.8e
Venugopal V., Ludman A., Yellon D. M., Hausenloy D. J. (2009). 'Conditioning' the Heart during Surgery. Eur. J. Cardio-Thoracic Surg. 35 (6), 977–987. doi:10.1016/j.ejcts.2009.02.014
Victorio J. A., Clerici S. P., Palacios R., Alonso M. J., Vassallo D. V., Jaffe I. Z., et al. (2016). Spironolactone Prevents Endothelial Nitric Oxide Synthase Uncoupling and Vascular Dysfunction Induced by β-Adrenergic Overstimulation. Hypertension 68 (3), 726–735. doi:10.1161/HYPERTENSIONAHA.116.07911
Wang D., Liu Y.-H., Yang X.-P., Rhaleb N.-E., Xu J., Peterson E., et al. (2004). Role of a Selective Aldosterone Blocker in Mice with Chronic Heart Failure. J. Cardiac Fail. 10 (1), 67–73. doi:10.1016/s1071-9164(03)00578-5
Westerman S., Wenger N. K. (2016). Women and Heart Disease, the Underrecognized Burden: Sex Differences, Biases, and Unmet Clinical and Research Challenges. Clin. Sci. (Lond) 130 (8), 551–563. doi:10.1042/CS20150586
Wong E. C., Fordyce C. B., Wong G., Lee T., Perry‐Arnesen M., Mackay M., et al. (2021). Predictors of the Use of Mineralocorticoid Receptor Antagonists in Patients with Left Ventricular Dysfunction Post‐ST‐Segment-Elevation Myocardial Infarction. Jaha 10 (14), e019167. doi:10.1161/jaha.120.019167
Wong N. D. (2014). Epidemiological Studies of CHD and the Evolution of Preventive Cardiology. Nat. Rev. Cardiol. 11 (5), 276–289. doi:10.1038/nrcardio.2014.26
Yoshino T., Nagoshi T., Anzawa R., Kashiwagi Y., Ito K., Katoh D., et al. (2014). Preconditioning Actions of Aldosterone through P38 Signaling Modulation in Isolated Rat Hearts. J. Endocrinol. 222 (2), 289–299. doi:10.1530/JOE-14-0067
Young M. J., Clyne C. D. (2021). Mineralocorticoid Receptor Actions in Cardiovascular Development and Disease. Essays Biochem. 65 (6), 901–911. doi:10.1042/ebc20210006
Young M. J., Rickard A. J. (2015). Mineralocorticoid Receptors in the Heart: Lessons from Cell-Selective Transgenic Animals. J. Endocrinol. 224 (1), R1–R13. doi:10.1530/JOE-14-0471
Keywords: cardiomyocyte, ischemia-reperfusion, mineralocorticoid, sex differences, nitric oxide
Citation: Bienvenu LA, Bell JR, Weeks KL, Delbridge LMD and Young MJ (2022) New Perspectives on Sex Steroid and Mineralocorticoid Receptor Signaling in Cardiac Ischemic Injury. Front. Physiol. 13:896425. doi: 10.3389/fphys.2022.896425
Received: 15 March 2022; Accepted: 27 May 2022;
Published: 29 June 2022.
Edited by:
James Todd Pearson, National Cerebral and Cardiovascular Center, JapanReviewed by:
Rosemeire Kanashiro-Takeuchi, University of Miami, United StatesCopyright © 2022 Bienvenu, Bell, Weeks, Delbridge and Young. This is an open-access article distributed under the terms of the Creative Commons Attribution License (CC BY). The use, distribution or reproduction in other forums is permitted, provided the original author(s) and the copyright owner(s) are credited and that the original publication in this journal is cited, in accordance with accepted academic practice. No use, distribution or reproduction is permitted which does not comply with these terms.
*Correspondence: James R. Bell, ai5iZWxsQGxhdHJvYmUuZWR1LmF1
†These authors have contributed equally to this work and share first authorship
‡These authors have contributed equally to this work and share senior authorship