- 1Department of Anesthesiology and Intensive Care, Gregorio Marañón Hospital, Madrid, Spain
- 2Department of Pharmacology and Toxicology, Faculty of Medicine, Universidad Complutense, Madrid, Spain
- 3Department of Cardiovascular Surgery, Gregorio Marañón Hospital, Madrid, Spain
- 4Department of Surgery, Faculty of Medicine, Universidad Complutense, Madrid, Spain
Cardiac output (CO) measurement is mandatory in patients with left ventricular assist devices (LVADs). Thermodilution with pulmonary artery catheter (PAC) remains the clinical gold standard to measure CO in these patients, however it is associated with several complications. Therefore, the agreement between PAC and new, minimally invasive monitoring methods in LVAD needs to be further investigated. The aim of this study was to assess the accuracy and reliability of transpulmonary thermodilution with a PiCCO2 monitor compared with pulmonary artery thermodilution with PAC in a LVAD. Continuous-flow LVADs were implanted in six mini-pigs to assist the left ventricle. We studied two methods of measuring CO—intermittent transpulmonary thermodilution (COTPTD) by PiCCO2 and intermittent pulmonary artery thermodilution by CAP, standard technique (COPTD)—obtained in four consecutive moments of the study: before starting the LVAD (basal moment), and with the LVAD started in normovolemia, hypervolemia (fluid overloading) and hypovolemia (shock hemorrhage). A total of 72 paired measurements were analysed. At the basal moment, COTPTD and COPTD were closely correlated (r2 = 0.89), with a mean bias of −0.085 ± 0.245 L/min and percentage error of 16%. After 15 min of partial support LVAD, COTPTD and COPTD were closely correlated (r2 = 0.79), with a mean bias of −0.040 ± 0.417 L/min and percentage error of 26%. After inducing hypervolemia, COTPTD and COPTD were closely correlated (r2 = 0.78), with a mean bias of −0.093 ± 0.339 L/min and percentage error of 13%. After inducing hypovolemia, COTPTD and COPTD were closely correlated (r2 = 0.76), with a mean bias of −0.045 ± 0.281 L/min and percentage error of 28%. This study demonstrates a good agreement between transpulmonary thermodilution by PiCCO monitor and pulmonary thermodilution by PAC in the intermittent measurement of CO in a porcine model with a continuous-flow LVAD.
Introduction
In the last decades, mechanical circulatory support has emerged as an option for patients with heart failure resistant to pharmacotherapy (Tickoo and Bardia, 2020). However, this therapy is associated with mortality, especially during the first 30 days postimplantation (Nepomuceno et al., 2020). Thus, cardiac output (CO) monitoring is essential in patients with left ventricular assist devices (LVADs) (Birati and Rame, 2014; Uriel et al., 2016). Pulmonary artery catheter (PAC) by pulmonary thermodilution is the standard technique for monitoring CO in patients with LVADs (Saxena et al., 2020). However, severe complications have been associated with this invasive form of hemodynamic monitoring in critically ill patients (Bossert et al., 2006).
New and less invasive hemodynamic monitoring techniques have emerged in recent years (Mateu Campos et al., 2012). The PiCCO (pulse index continuous cardiac output) system is currently the only monitor that uses transpulmonary thermodilution to measure CO. It is a minimally invasive form of monitoring (it requires only an arterial and a venous access) that provides information on blood flows and intravascular volumes (Mateu Campos et al., 2012. A PiCCO monitor can measure CO by pulse-contour analysis (continuously) or transpulmonary thermodilution (intermittently) (Halvorsen et al., 2006). Transpulmonary thermodilution is increasingly used for critically ill patients (Teboul et al., 2016). However, it has not yet been validated for hemodynamic monitoring in circulatory support devices.
Thus, in this study, we assess the agreement of the measured values of the PiCCO transpulmonary thermodilution CO (COTPTD) with the pulmonary artery thermodilution CO (COPTD) in a porcine model with a continuous-flow LVAD with normovolemia, hypervolemia (fluid overload), and hypovolemia (bleeding).
Materials and methods
The study was carried out in the Experimental Medicine and Surgery Unit of the Gregorio Marañón University General Hospital (ES280790000-087). The study was performed in accordance with European Union guidelines on the protection of animals used for experimental and other scientific purposes (Directive 2010/63/EU and Spanish Royal Decree RD 53/2013 BOE) and was approved by the Ethics Committee on animal studies at our institution.
Anaesthesia and surgical protocol
We use six healthy mini-pigs with a mean weight of 42.8 ± 9.9 kg. The previously described anesthetic protocol was applied (Morillas-Sendín et al., 2015; Quintana-Villamandos et al., 2021). After premedication with 20 mg/kg intramuscular ketamine (Ketolar, Parke-Davis, Madrid, Spain) and 0.04 mg/kg atropine (Atropina Braun, Serra Pamies, Reus, Spain), anesthesia was induced with 2.5 μg/kg intravenous fentanyl (Fentanest, Kern Pharma, Barcelona, Spain) and 4 mg/kg propofol (Diprivan 1%, AstraZeneca, Madrid, Spain). After tracheal intubation, each animal was connected to a volume-controlled ventilator (Dräger SA1, Dräger Medical AG, Lübeck, Germany) with an FIO2 of 1, an inspiratory-to-expiratory ratio of 1:2, a tidal volume of 12–15 ml/kg and a respiratory rate adjusted to maintain normocapnia. Anesthesia was maintained with intravenous fentanyl (2.5 μg/kg for 30 min) and propofol in continuous infusion (11–12 mg/kg/h). All animals received an infusion of saline solution (8 ml/kg/h). The PAC (7.5 F Swan-Ganz CCOmbo catheter, Edwards Lifesciences, Irvine, CA, United States) was inserted into the right internal jugular vein for intermittent CO measurement by pulmonary thermodilution and connected to a monitor (Vigilance, Edwards Critical Care Division, Irvine, CA, United States). We used a PiCCO2 set (Pulsion Medical Systems, Munich, Germany) for intermittent CO measurement by transpulmonary thermodilution that consisted of three components: a catheter (5F, 20 cm) inserted into the right femoral artery with a solid-state thermistor 5 mm from its tip, an injection device that connects to the distal lumen of a standard central venous catheter (inserted into the left internal jugular vein) and the PiCCO2 monitor (connected to the right femoral catheter). Finally, an epicardial echocardiography was performed using the Vivid S5 system (GE Healthcare, Germany) equipped with a 4 MHz probe (3Sc-RS, GE).
The established surgical protocol was applied (Morillas-Sendín et al., 2015; Quintana-Villamandos et al., 2021). A Biomedicus 540 centrifugal pump was implanted in the mini-pigs undergoing continuous-flow support. After median sternotomy, the animal was heparinised (4 mg/kg), a partial aortic cross-clamp was applied (just for anastomosing the output cannula of the LVAD to the aorta), and at 2 cm an aortotomy was performed. The output cannula of the LVAD was anastomosed to the ascending aorta, and the input cannula was placed through the apex of the left ventricle. Finally, both cannulas were connected to the device. Input flow was measured using an ultrasound transducer attached to the input cannula of the device.
Experimental protocol and measurements
The experimental protocol is summarised in Figure 1. After sternotomy, an epicardial echocardiography was performed, and we excluded pigs with aortic insufficiency, tricuspid regurgitation, atrial septal defects or ventricular septal defects from the study. After implanting the LVAD, console parameters were adjusted to obtain a pump flow of 50% (partial support) of the baseline CO (before the LVAD is initiated) using the PAC. Measurements of CO using both methods—COPTD and COTPTD—were obtained before starting the LVAD, and then with partial support LVAD during three periods (after 15 min of LVAD, after inducing hypervolemia, and after inducing hypovolemia). We induced hypervolemia with fluid overloading (75% serum saline + 25% gelatine) to increase the mean arterial pressure to 130 mmHg and/or the central venous pressure up to 20 mmHg, and we induced hypovolemia by controlled hemorrhage to decrease the mean arterial pressure to around 50 mmHg (Bendjelid et al., 2010).
Data analysis
The results are expressed as mean + standard deviation (SD). CO determinations using the two methods, COPTD (standard technique) and COTPTD, were compared at different moments using paired Student’s t-test. Statistical significance was set at p < 0.05. Bias was defined as the mean difference between the two methods. A comparison of the bias between the two methods was assessed by Bland–Altman analysis (Odor et al., 2017). The percentage error was calculated as two SDs of the bias divided by the mean of the CO obtained by the standard technique. A percentage error of less than ±30% is recommended as clinically acceptable when comparing a new method to the current reference method (Odor et al., 2017). Linear regression analysis was used to assess the relationship between the CO values obtained by the two methods. The statistical analysis was performed using IBM SPSS Statistics for Windows, version 20.0 (IBM Corp, Armonk, NY, United States) and GraphPad Prism 6.0 (GraphPad Software, CA, United States).
Results
In the present study, a mini-pig presented with atrial septal defects (detected by echocardiography) and was therefore excluded from the study (this is crucial to prevent end-organ damage due to hypoxemia by a right–left atrial shunt) (Quintana-Villamandos et al., 2012). A total of 72 paired measurements (three measurements from each stage per animal) were obtained from six consecutive mini-pigs. The reproducibility of the CO values over the study period is reported in Table 1.
Before starting LVAD, there were no significant differences between the measured COTPTD and COPTD values (3.15 ± 0.72 vs. 3.06 ± 0.64 L/min, respectively, p = 0.16). COTPTD and COPTD were closely correlated (r2 = 0.89), with a mean bias (± SD) of −0.085 ± 0.245 L/min and percentage error of 16% (Figures 2A, 3A).
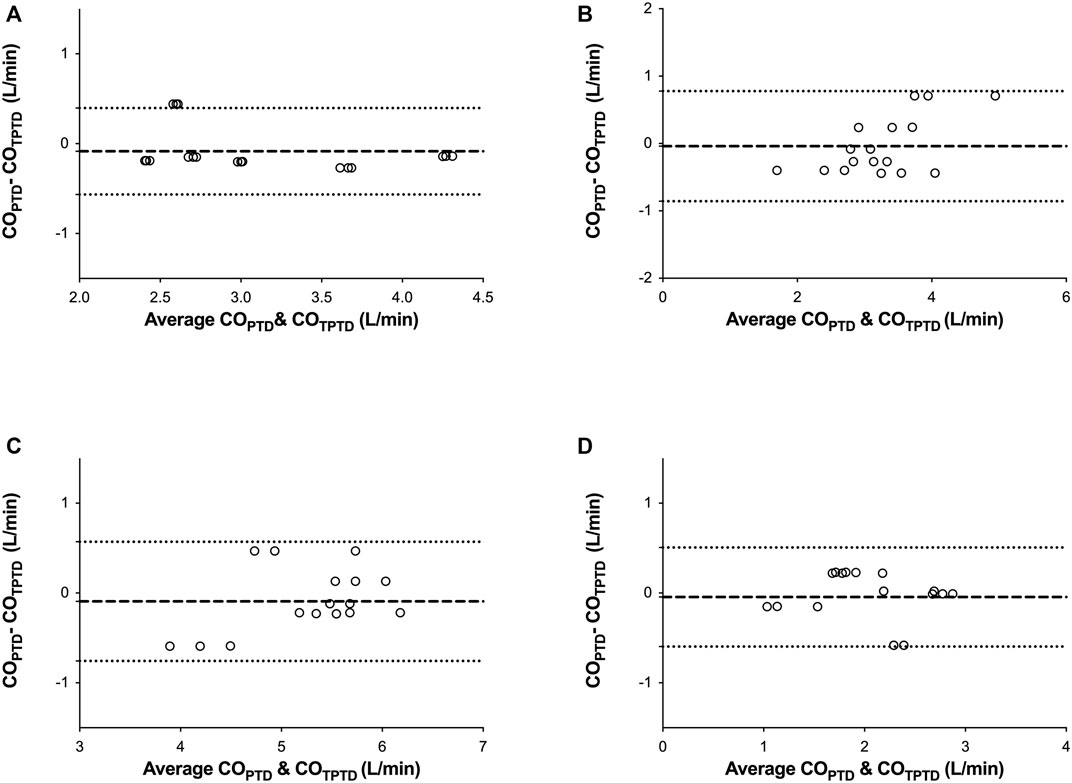
FIGURE 2. Correlation between the cardiac output (CO) measurements obtained by transpulmonary thermodilution (COTPTD) and intermittent pulmonary artery thermodilution (COPTD) in four consecutive moments of the study: before starting the left ventricular assist devices (LVADs) (A), with the LVAD started in normovolemia (B), hypervolemia (fluid overloading) (C), and hypovolemia (shock hemorrhage) (D).
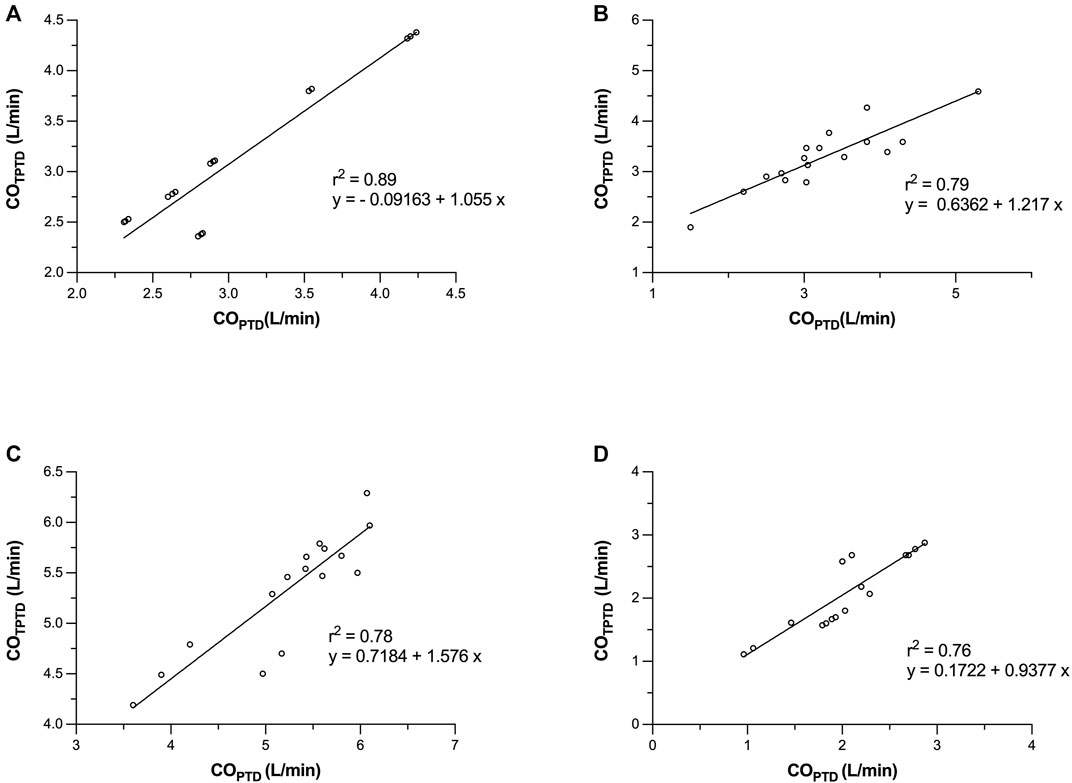
FIGURE 3. Bland–Altman representation depicting the agreement between the cardiac output (CO) measurements obtained by transpulmonary thermodilution (COTPTD) and intermittent pulmonary artery thermodilution (COPTD) in four consecutive moments of the study: before starting the left ventricular assist devices (LVADs) (A), with the LVAD started in normovolemia (B), hypervolemia (fluid overloading) (C), and hypovolemia (shock hemorrhage) (D).
After 15 min of partial support, there were no significant differences between COTPTD and COPTD (3.27 ± 0.60 vs. 3.23 ± 0.85 L/min, respectively, p = 0.68). COTPTD and COPTD were closely correlated (r2 = 0.79), with a mean bias (± SD) of −0.040 ± 0.417 L/min and a percentage error of 26% (Figures 2B, 3B).
After hypervolemia was induced, there were no significant differences between COTPTD and COPTD (5.35 ± 0.57 vs. 5.26 ± 0.71 L/min, respectively, p = 0.26). COTPTD and COPTD were closely correlated (r2 = 0.78), with a mean bias (± SD) of −0.093 ± 0.339 L/min and a percentage error of 13% (Figures 2C, 3C).
After hypovolemia was induced, there were no significant differences between COTPTD and COPTD (2.09 ± 0.57 vs. 2.04 ± 0.53 L/min, respectively, p = 0.51). COTPTD and COPTD were closely correlated (r2 = 0.76), with a mean bias (± SD) of −0.045 ± 0.281 L/min and a percentage error of 28% (Figures 2D, 3D).
Discussion
The PiCCO system was compared to PAC in LVAD, and the results showed satisfactory agreement of CO values in a porcine model of normovolemia, hypervolemia and hypovolemia.
PAC is the clinical reference standard for CO measurement in critically ill patients (Vincent, 2012; Garan et al., 2020). PAC is still recommended by the interdisciplinary S3 guidelines in a cardiac surgical setting, and this indication includes patients with LVADs (Kanchi, 2011; Habicher et al., 2018). Continuous thermodilution by PAC has been validated in both continuous-flow (Quintana-Villamandos et al., 2021) and pulsatile-flow (Mets et al., 2002) LVADs, because it is crucial to know the status of end-organ perfusion in these patients (Birati and Rame, 2014). In a previous study, our group has validated continuous thermodilution by PAC using intermittent thermodilution by PAC (standard technique), in six minipigs with continuous-flow LVADs (Quintana-Villamandos et al., 2021). The study demosntrated that continuous thermodilution by PAC could be an alternative method of measuring CO in a continuous-flow LVAD in a porcine model of normovolemia, fluid overload, and bleeding. However, thermodilution by PAC is an invasive technique that has been associated with several complications (i.e. arrhythmia, coiling, knotting, carotid puncture, pulmonary infarction, intrapulmonary bleeding, pulmonary embolism, infection, pericardial effusion, pulmonary artery rupture and cardiac valvular damage) (Bossert et al., 2006; Evans et al., 2009; Sangkum et al., 2016; Benito-Saz et al., 2019). Thus, in the present work, our group studies a new CO monitoring (the PiCCO system) in a continuous-flow LVAD in a porcine model of normovolemia, fluid overload, and bleeding. We used another group of six minipigs to validate the PiCCO system (transpulmonary thermodilution) using intermittent thermodilution by PAC (standard technique). This is the first study to show the PiCCO system as an alternative to PAC in the measurement of CO in a porcine model with a continuous-flow LVAD.
Technological advances are necessary for minimally invasive or noninvasive CO measurement. Alternatives to the PAC have been developed, including transoesophageal echocardiography, arterial wave contour analysis and transpulmonary thermodilution (De Backer and Vincent, 2018). The PiCCO device uses a combination of transpulmonary thermodilution and pulse contour analysis. It is comparable with PAC thermodilution (Chakravarthy et al., 2007; Monnet and Teboul, 2017). CO measurement using the PiCCO can be influenced by valve pathology (i.e. tricuspid regurgitation and aortic insufficiency), intracardiac shunts, extracorporeal circulations and changes in body temperature. These potential sources of error are common to both PiCCO and PAC (Heerdt et al., 2001; Sami et al., 2007).
The PiCCO system is the most widely used monitor that employs transpulmonary thermodilution to measure CO. Intermittent bolus transpulmonary thermodilution is based on the Stewart–Hamilton equation; a cold saline bolus is introduced into the circulation through a central venous catheter, in which the external temperature sensor is located. Once in circulation, the thermistor located at the tip of the arterial catheter detects variations in the temperature, generating a thermodilution curve (Litton and Morgan, 2012). Transpulmonary thermodilution by PiCCO shows advantages relative to pulmonary thermodilution by PAC. PiCCO is less invasive (while it requires a central venous catheter and an arterial catheter, these are already required for all critical patients), and therefore it has few associated complications (e.g. inflammation and catheter-related infection (Sangkum et al., 2016). It is independent of respiratory cycles and able to measure parameters other than CO, including the extravascular lung water (to measure subclinical pulmonary edema), the global end-diastolic volume (volume in the heart at end-diastole) and the intrathoracic blood volume (volume in the heart and pulmonary circulation), both of which are used to estimate the cardiac preload (Litton and Morgan, 2012).
COTPTD does not provide a continuous measurement and needs calibration after acute haemodynamic changes (Monnet and teboul, 2017; Rozental et al., 2021). However, the reproducibility of COTPTD is higher (7% precision) than that of COPTD (15% precision) (Giraud el al., 2017; Rozental et al., 2021). The good reproducibility of transpulmonary thermodilution is related to the longer transit time of the thermal bolus (20 s), which reduces the artefacts produced by respiration, compared to pulmonary thermodilution (3–4 s). PAC may offer some advantage in patients with right heart dysfunction (De Backer and Vincent, 2018). However, in the present study we use a porcine model with a continuous-flow LVAD without right heart failure. PAC provides a series of measurements that are important for the management of patients with cardiogenic shock, especially right heart failure or adult respiratory distress syndrome. In these cases, the measurement of right cardiac output or the evaluation of pulmonary haemodynamics (in order to diagnose the presence of pulmonary hypertension and guide treatment) remain an indispensable part of proper monitoring (Monnet and Teboul, 2017). Furthermore, it also allows to determine the filling pressures of the left ventricle by occluding the pulmonary artery with the distal balloon and measuring the pulmonary artery occluded pressure. This parameter represents the filling pressures of the left atrium, and its morphology could contribute a lot of information to diagnose, for example, a significant mitral regurgitation. An infrared detector at the end of the pulmonary artery catheter allows continuous measurement of mixed venous saturation. It offers a real-time determination of the oxygen supply - demand ratio with good accuracy in real time (Bootsma et al., 2022a; Bootsma et al., 2022b). Transpulmonary thermodilution (by PiCCO device) is an alternative to PAC as a measure of CO (Litton and Morgan, 2012; Beurton et al., 2019). However, the use of PiCCO in patients with LVAD requires further validation. In the present study, we show that transpulmonary thermodilution could be an alternative method of monitoring CO in an LVAD in a porcine model.
We recognise some limitations of this study that should be noted. First, in the present work we study the COTPTD by PiCCO in a porcine model with a continuous-flow LVAD. However, the pulsatile-flow and continuous-flow LVADs show differences in hemodynamic response and ventricular unloading, and thus it is necessary to investigate monitoring systems on both LVADs (Cheng et al., 2014). Second, the CO measurements obtained from PAC are influenced more by the respiratory cycle than the transpulmonary thermodilution; however, in the present study, all measurements were made at the end of expiration when the variations are minimal. Finally, the LVAD is designed to be used in patients with heart failure; however, it is possible to study the validity of transpulmonary thermodilution for the determination of CO in LVAD in a healthy porcine model because the native ventricle can adjust to LVAD unloading by reducing flow through the aortic valve. Further investigations are necessary to reproduce these results in patients with heart failure.
Conclusion
In conclusion, this study shows a good agreement between transpulmonary thermodilution by PiCCO monitor and pulmonary thermodilution by PAC in the intermittent measurement of CO in a porcine model with a continuous-flow LVAD.
Data availability statement
The original contributions presented in the study are included in the article/Supplementary Material; further inquiries can be directed to the corresponding author.
Ethics statement
The animal study was reviewed and approved by Ethics Committee of Hospital General Universitario Gregorio Marañón, Madrid, Spain.
Author contributions
BQ-V and JC conceived the project, analysed the data, interpreted the results of experiments and edited the manuscript. MB, IF, and MR performed the experiments, discussed the results, and approved the submitted version.
Funding
This work was supported by a grant from Spanish Health Ministry (number FIS PI08/1480) and Fondos Feder.
Conflict of interest
The authors declare that the research was conducted in the absence of any commercial or financial relationships that could be construed as a potential conflict of interest.
Publisher’s note
All claims expressed in this article are solely those of the authors and do not necessarily represent those of their affiliated organizations, or those of the publisher, the editors and the reviewers. Any product that may be evaluated in this article, or claim that may be made by its manufacturer, is not guaranteed or endorsed by the publisher.
Reference
Bendjelid K., Giraud R., Siegenthaler N., Michard F. (2010). Validation of a new transpulmonary thermodilution system to assess global end-diastolic volume and extravascular lung water. Crit. Care 14, R209. doi:10.1186/cc9332
Benito-Saz P., Garrido A., Quintana-Villamandos B. (2019). Perforation of the left ventricle wall due to the insertion of a pulmonary artery catheter. A case report. Rev. Esp. Anestesiol. Reanim. Engl. Ed. 66, 528–532. doi:10.1016/j.redar.2019.06.001
Beurton A., Teboul J. L., Monnet X. (2019). Transpulmonary thermodilution techniques in the haemodynamically unstable patient. Curr. Opin. Crit. Care 25, 273–279. doi:10.1097/MCC.0000000000000608
Birati E. Y., Rame J. E. (2014). Left ventricular assist device management and complications. Crit. Care Clin. 30, 607–627. doi:10.1016/j.ccc.2014.04.001
Bootsma I. T., Boerma E. C., de Lange F., Scheeren T. W. L. (2022a). The contemporary pulmonary artery catheter. Part 1: Placement and waveform analysis. J. Clin. Monit. Comput. 36, 5–15. doi:10.1007/s10877-021-00662-8
Bootsma I. T., Boerma E. C., Scheeren T. W. L., de Lange F. (2022b). The contemporary pulmonary artery catheter. Part 2: Measurements, limitations, and clinical applications. J. Clin. Monit. Comput. 36, 17–31. doi:10.1007/s10877-021-00673-5
Bossert T., Gummert J. F., Bittner H. B., Barten M., Walther T., Falk V., et al. (2006). Swan-ganz catheter-induced severe complications in cardiac surgery: Right ventricular perforation, knotting, and rupture of a pulmonary artery. J. Card. Surg. 21, 292–295. doi:10.1111/j.1540-8191.2006.00235.x
Chakravarthy M., Patil T. A., Jayaprakash K., Kalligudd P., Prabhakumar D., Jawali V. (2007). Comparison of simultaneous estimation of cardiac output by four techniques in patients undergoing off-pump coronary artery bypass surgery--a prospective observational study. Ann. Card. Anaesth. 10, 121–126. doi:10.4103/0971-9784.37937
Cheng A., Williamitis C. A., Slaughter M. S. (2014). Comparison of continuous-flow and pulsatile-flow left ventricular assist devices: Is there an advantage to pulsatility? Ann. Cardiothorac. Surg. 3, 573–581. doi:10.3978/j.issn.2225-319X.2014.08.24
De Backer D., Vincent J. L. (2018). The pulmonary artery catheter: Is it still alive? Curr. Opin. Crit. Care 24, 204–208. doi:10.1097/MCC.0000000000000502
Evans D. C., Doraiswamy V. A., Prosciak M. P., Silviera M., Seamon M. J., Rodriguez Funes V., et al. (2009). Complications associated with pulmonary artery catheters: A comprehensive clinical review. Scand. J. Surg. 98, 199–208. doi:10.1177/145749690909800402
Garan A. R., Kanwar M., Thayer K. L., Zweck E., et al. (2020). Complete hemodynamic profiling with pulmonary artery catheters in cardiogenic shock is associated with lower in-hospital mortality. JACC. Heart Fail. 8, 903–913. doi:10.1016/j.jchf.2020.08.012
Giraud R., Siegenthaler N., Merlani P. (2017). Reproducibility of transpulmonary thermodilution cardiac output measurements in clinical practice: A systematic review. J. Clin. Monit. Comput. 31, 43–51. doi:10.1007/s10877-016-9823-y
Habicher M., Zajonz T., Heringlake M., Boning A., TreSkatSch S., et al. (2018). S3 guidelines on intensive medical care of cardiac surgery patients: Hemodynamic monitoring and cardiovascular system-an update. Anaesthesist 67, 375–379. doi:10.1007/s00101-018-0433-6
Halvorsen P. S., Espinoza A., Lundblad R., Cvancarova M., Hol P. K., Fosse E. (2006). Agreement between PiCCO pulse-contour analysis, pulmonal artery thermodilution and transthoracic thermodilution during off-pump coronary artery by-pass surgery. Acta Anaesthesiol. Scand. 50, 1050. doi:10.1111/j.1399-6576.2006.01118.x
Heerdt P. M., Blessios G. A., Beach M. L. (2001). Flow dependency of error in thermodilution measurement of cardiac output during acute tricuspid regurgitation. J. Cardiothorac. Vasc. Anesth. 15, 183–187. doi:10.1053/jcan.2001.21947
Kanchi M. (2011). Do we need a pulmonary artery catheter in cardiac anesthesia? - an Indian perspective. Ann. Card. Anaesth. 14, 25–29. doi:10.4103/0971-9784.74396
Litton E., Morgan M. (2012). The PiCCO monitor: A review. Anaesth. Intensive Care 40, 393–409. doi:10.1177/0310057X1204000304
Mateu Campos M. L., Ferrándiz Sellés A., Gruartmoner de Vera G., Mesquida Febrer J., Sabatier Cloarec C., Poveda Hernández Y., et al. (2012). Techniques available for hemodynamic monitoring. Advantages and limitations. Med. Intensiva 36, 434–444. doi:10.1016/j.medin.2012.05.003
Mets B., Frumento R. J., Bennett-Guerrero E., Naka Y. (2002). Validation of continuous thermodilution cardiac output in patients implanted with a left ventricular assist device. J. Cardiothorac. Vasc. Anesth. 16, 727–730. doi:10.1053/jcan.2002.128411
Monnet X., Teboul J. L. (2017). Transpulmonary thermodilution: Advantages and limits. Crit. Care 21, 147. doi:10.1186/s13054-017-1739-5
Morillas-Sendín P., Delgado-Baeza E., Delgado-Martos M. J., Barranco M., del Cañizo J. F., Ruíz M., et al. (2015). Effects of sevoflurane and propofol on organ blood flow in left ventricular assist devices in pigs. Biomed. Res. Int. 2015, 898373. doi:10.1155/2015/898373
Nepomuceno R. G., Goldraich L. A., De S., Hegazy A. F. (2020). Critical care management of the acute postimplant LVAD patient. Can. J. Cardiol. 36, 313–316. doi:10.1016/j.cjca.2019.11.034 (
Odor P. M., Bampoe S., Cecconi M. (2017). Cardiac output monitoring: Validation studies-how results should be presented. Curr. Anesthesiol. Rep. 7, 410–415. doi:10.1007/s40140-017-0239-0
Quintana-Villamandos B., Barranco M., Fernández I. (2021). New advances in monitoring cardiac output in circulatory mechanical assistance devices. A validation study in a porcine model. Front. Physiol. 12, 634779. doi:10.3389/fphys.2021.634779
Quintana-Villamandos B., Rodríguez-Bernal G. J., Pérez-Caballero R. (2012). Severe hypoxaemia with a left ventricular assist device in a minipig model with an undiagnosed congenital cardiac disease. Lab. Anim. 46, 77–80. doi:10.1258/la.2011.011067
Rozental O., Thalappillil R., White R. S., Rozental O. (2021). To swan or not to swan: Indications, alternatives, and future directions. J. Cardiothorac. Vasc. Anesth. 35, 600–615. doi:10.1053/j.jvca.2020.07.067
Sami A., Sami A., Rochdil N. (2007). PiCCO monitoring accuracy in low body temperature. Am. J. Emerg. Med. 25, 845–846. doi:10.1016/j.ajem.2006.11.046
Sangkum L., Liu G. L., Yu L. (2016). Minimally invasive or noninvasive cardiac output measurement: An update. J. Anesth. 30, 461–480. doi:10.1007/s00540-016-2154-9
Saxena A., Garan A. R., Kapur N. K. (2020). Value of hemodynamic monitoring in patients with cardiogenic shock undergoing mechanical circulatory support. Circulation 141, 1184–1197. doi:10.1161/CIRCULATIONAHA.119.043080
Teboul J. L., Saugel B., Cecconi M., De Backer D., Hofer C. K., Monnet X., et al. (2016). Less invasive hemodynamic monitoring in critically ill patients. Intensive Care Med. 42, 1350–1359. doi:10.1007/s00134-016-4375-7
Tickoo M., Bardia A. (2020). Anesthesia at the edge of life: Mechanical circulatory support. Anesthesiol. Clin. 38, 19–33. doi:10.1016/j.anclin.2019.11.002
Uriel N., Sayer G., Addetia K., Fedson S., Kim G. H., Rodgers D., et al. (2016). Hemodynamic ramp tests in patients with left ventricular assist devices. JACC. Heart Fail. 4, 208–217. doi:10.1016/j.jchf.2015.10.001
Keywords: cardiac output, continuous-flow LVAD, minipig, pulmonary thermodilution, transpulmonary thermodilution
Citation: Quintana-Villamandos B, Barranco M, Fernández I, Ruiz M and Del Cañizo JF (2022) Cardiac output monitoring with pulmonary versus trans-cardiopulmonary thermodilution in left ventricular assist devices: Interchangeable methods?. Front. Physiol. 13:889190. doi: 10.3389/fphys.2022.889190
Received: 03 March 2022; Accepted: 05 August 2022;
Published: 02 September 2022.
Edited by:
Eun Bo Shim, Kangwon National University, South KoreaReviewed by:
Jean-Louis Vincent, Université libre de Bruxelles, BelgiumGrace M. Arteaga, Mayo Clinic, United States
Copyright © 2022 Quintana-Villamandos, Barranco, Fernández, Ruiz and Del Cañizo. This is an open-access article distributed under the terms of the Creative Commons Attribution License (CC BY). The use, distribution or reproduction in other forums is permitted, provided the original author(s) and the copyright owner(s) are credited and that the original publication in this journal is cited, in accordance with accepted academic practice. No use, distribution or reproduction is permitted which does not comply with these terms.
*Correspondence: Begoña Quintana-Villamandos, YmVnb3F1aW50aUBnbWFpbC5jb20=
†Present Address: Begoña Quintana-Villamandos, Department of Anaesthesiology Hospital Gregorio Marañón, Madrid, Spain