- 1Department of Biological Sciences, Faculty of Pharmaceutical Sciences, Teikyo University, Tokyo, Japan
- 2Department of Molecular Biology, Faculty of Pharmaceutical Sciences, Teikyo University, Tokyo, Japan
- 3Department of Biology, Faculty of Science, Hokkaido University, Sapporo, Japan
Hippocampal formation (HF) plays a key role in cognitive and emotional processing in mammals. In HF neural circuits, serotonin receptors (5-HTRs) modulate functions related to cognition and emotion. To understand the phylogenetic continuity of the neural basis for cognition and emotion, it is important to identify the neural circuits that regulate cognitive and emotional processing in animals. In birds, HF has been shown to be related to cognitive functions and emotion-related behaviors. However, details regarding the distribution of 5-HTRs in the avian brain are very sparse, and 5-HTRs, which are potentially involved in cognitive functions and emotion-related behaviors, are poorly understood. Previously, we showed that 5-HTR1B and 5-HTR3A were expressed in chick HF. To identify additional 5-HTRs that are potentially involved in cognitive and emotional functions in avian HF, we selected the chick orthologs of 5-HTR1D, 5-HTR1E, 5-HTR1F, 5-HTR2B, 5-HTR5A, and 5-HTR7 and performed in situ hybridization in the chick telencephalon. We found that 5-HTR1D, 5-HTR1E, 5-HTR5A, and 5-HTR7 were expressed in the chick HF, especially 5-HTR1D and 5-HTR1E, which showed subdivision- and layer-selective expression patterns, suggesting that the characteristic 5-HT regulation is involved in cognitive functions and emotion-related behaviors in these HF regions. These findings can facilitate the understanding of serotonin regulation in avian HF and the correspondence between the HF subdivisions of birds and mammals.
Introduction
The modulation of various neural functions by serotonin (5-hydroxytryptamine, 5-HT) is phylogenetically conserved (Marin et al., 2020). In particular, the association of the 5-HT system with cognition, behavior, and emotion is evolutionarily conserved in the animal kingdom (Kandel et al., 2000; Bacque-Cazenave et al., 2020). In mammals, processing of both cognition and emotion have been shown to involve the hippocampal formation (HF) (Fanselow and Dong, 2010; Anacker and Hen, 2017). The macrohistological features of HF are well-conserved in all mammals. More specifically, HF consists of similarly convoluted and interlocked 3-layered subdivisions: the dentate gyrus (DG), Ammon’s horns or Cornu ammonis (CA) fields 1 to 3 (CA1 to CA3), and the subiculum (Hevner, 2016; Medina et al., 2017b). Information flow in the mammalian HF was described as a “trisynaptic circuit”, in which the entorhinal cortex (EC) projects to the DG, DG provides the projection to CA3 known as “mossy fiber”, and CA3 relays it to CA1 (Sloviter and Lomo, 2012). To understand the phylogenetic continuity of the neural basis for cognition and emotion, it is essential to reveal the neural mechanisms in HF that process cognitive and emotional behaviors in nonmammalian animals. Birds are well-fitted model animals for understanding the evolutionary continuity of the neural basis of cognition and emotion (Rosa Salva et al., 2015; Papini et al., 2019). For instance, the polymorphism of the 5-HT transporter gene, which affects the levels of gene expression, has been suggested to modulate fear-related behavior in chickens (Krause et al., 2017; Phi Van et al., 2018; Krause et al., 2019). In addition, hippocampal formation in birds (HF, hippocampus proper (Hp), and area parahippocampalis (APH)) has been shown to be related to cognitive functions, such as spatial navigation (Colombo and Broadbent, 2000; Matsushima et al., 2003; Bingman, et al., 2005; Mayer, et al., 2016; Sherry, et al., 2017; Payne, et al., 2021), and controlling the stress response (Smulders, 2017; Smulders, 2021) and emotions, such as anxiety-like behavior (Mayer, et al., 2018; Morandi-Raikov and Mayer, 2020; Parada, et al., 2021). However, the neural circuits that control these behaviors and 5-HT regulation in the avian HF are largely unknown.
The avian HF is homologous to that of mammals (Reiner, et al., 2004; Herold, et al., 2015; Striedter, 2016). The ancient origin of HF in the amniote was supported by both recent large-scale and single-cell transcriptome studies (Belgard, et al., 2013; Tosches, et al., 2018). However, the avian HF is composed of a layered arrangement of densely packed neurons with poorly defined boundaries, whereas the mammalian HF has a clear laminar organization (Atoji and Wild, 2006; Herold, et al., 2015; Striedter, 2016). The existence of many subdivisions in the avian HF has been proposed to arise from multiple aspects, such as developmental origin, connectivity, histochemistry, and immunohistochemistry (Kuenzel and Masson, 1988; Atoji and Wild, 2004; Atoji and Wild, 2006; Suarez, et al., 2006; Puelles, et al., 2007; Gupta, et al., 2012; Herold, et al., 2014; Abellan, et al., 2014; Atoji, et al., 2016; Medinia, et al., 2017b). However, to date, a one-to-one correspondence of subdivisions between the avian and mammalian HF has not been established, especially owing to the controversy regarding its homology with the mammalian Hp (dentate gyrus and Ammon’s horn) (Atoji and Wild, 2004; Atoji and Wild, 2006; Kempermann, 2012; Herold, et al., 2014; Abellan, et al., 2014; Streidter, 2016; Hevner, 2016; Atoji et al., 2016; Medina, et al., 2017b).
In mammals, various types of the 5-HT receptor (5-HTR) subfamily genes are expressed in the HF and are thought to play important roles in cognitive and emotional functions (Tanaka, et al., 2012; Strac et al., 2016; Zmudzka, et al., 2018; O’Leary, et al., 2020; Vilaro, et al., 2020). In our previous study, we pointed out that 5-HTR1B and 5-HTR3A were expressed in chick HF in a clear and characteristic manner (Fujita, et al., 2020). In the present study, we performed a detailed analysis and determined the subdivision of HF in which 5-HTR1B and 5-HTR3A are expressed. To comprehensively identify more 5-HTRs that are potentially involved in cognitive and emotional functions in avian HF, we investigated the expression of 5-HTR subfamily genes that were not analyzed in our previous study (Fujita, et al., 2020). We selected the chick orthologues of 5-HTR1D, 5-HTR1E, 5-HTR1F, 5-HTR2B, 5-HTR5A, and 5-HTR7 and found that 5-HTR1D, 5-HTR1E, 5-HTR5A, and 5-HTR7 were expressed in the chick HF. Among them, 5-HTR1D and 5-HTR1E showed subdivision- and layer-selective expression patterns, suggesting a characteristic 5-HT regulation in these regions. Our findings can be used as a basis for understanding 5-HT regulation in avian HF and the correspondence between the HF subdivisions of birds and mammals.
Materials and Methods
Animals
Fertilized eggs of domestic chicks (Gallus domesticus, Cobb strain) were purchased from a local dealer (3-M, Aichi, Japan) and incubated at Teikyo University (Kaga, Itabashi-ku, Tokyo, Japan). Animal experiments were performed as previously described (Yamaguchi et al., 2008a; 2008b). Newly hatched chicks (P0) were transferred to dark plastic enclosures in a dark, warm cage at 30°C for 1 day (P1). We used seven chicks for 5-HTR1D probes, nine for 5-HTR1E, eight for 5-HTR1F, seven for 5-HTR2B, six for 5-HTR5A, six for 5-HTR7, three for 5-HTR3A, five for 5-HTR1B, and six for lymphoid enhancer factor 1 (LEF1) (Supplementary Table S1). As for 5-HTR3A and 5-HTR1B, we have already investigated the expression of 5-HTR3A and 5-HTR1B throughout the entire brain in our previous study. In this study we focused on the expression of 5-HTR3A and 5-HTR1B in the HF. All procedures were reviewed and approved by the Committee on Animal Experiments of Teikyo University and were conducted in accordance with the guidelines of the national regulations for animal welfare in Japan.
Tissue Preparation
P1 chicks were anesthetized by intraperitoneal injection (0.40 ml/individual) of a 1:1 solution of ketamine (10 mg/ml, Ketalar-10, Sankyo Co., Tokyo, Japan) and xylazine (2 mg/ml, Sigma, St. Louis, MO, United States). For brain fixation, the anesthetized chicks were transcardially perfused with 4% paraformaldehyde in 0.1 M phosphate buffered saline (pH 7.5, PFA-PBS). Whole brain specimens were dissected and immediately immersed in PFA-PBS for 1 or 2 days at 4°C. For cryoprotection, fixed brain samples were placed in an 18% sucrose/PFA-PBS solution for 2 days at 4°C. Subsequently, brains with sucrose substitution were embedded in Tissue-Tek OCT compound (Sakura Finetechnical, Tokyo, Japan), frozen immediately on dry ice, and stored at −80°C until sectioning. Frozen brain blocks were cut into 18 µm-thick sections using a cryostat (Leica CM3050S or Leica CM 1850, Leica Biosystems, Nußloch, Germany). Serial coronal sections were prepared at the level of A14.4–A4.4, corresponding to those of the atlas by Kuenzel and Masson (1988).
cDNA Cloning
Total RNA was extracted from chick brains using the TRIzol reagent (Invitrogen, Carlsbad, CA, United States) and reverse-transcribed using the SuperScript III kit (Invitrogen) with an oligo (dT) primer, according to the manufacturer’s protocol. Reverse transcription polymerase chain reaction (RT-PCR) for the amplification of LEF1 was performed using gene-specific primers: forward, 5′-GATCCCCTTCAAGGACGAAG-3′; and reverse, 5′-GCCAAGAGGTGGTGTTATCTG-3′. PCR products were subcloned into the pGEM-T easy vector (Promega, Madison, WI, United States), the sequence of which was validated using Sanger sequencing. For 5-HTR1B, 5-HTR1D, 5-HTR1E, 5-HTR1F, 5-HTR2B, 5-HTR3A, 5-HTR5A, and 5-HTR7 probes, we used previously generated plasmids (Fujita et al., 2020; Fujita et al., 2022).
RNA Probe Preparations
Plasmids containing cDNA fragments for 5-HTR1B, 5-HTR1D, 5-HTR1E, 5-HTR1F, 5-HTR2B, 5-HTR3A, 5-HTR5A, 5-HTR7, and LEF1 were amplified by PCR using the M13 primer pair. Amplicons containing T7 and SP6 promoter sites were purified using a PCR purification kit (Qiagen, Valencia, CA, United States). Digoxigenin (DIG)-labelled sense and antisense RNA probes were prepared by in vitro transcription using a DIG RNA labelling kit (Roche, Basel, Switzerland) according to the manufacturer’s protocol.
In situ Hybridization
ISH experiments were performed as previously described in Fujita et al. (2019), with some modifications. Brain section specimens were refixed in 4% PFA-PBS, pretreated, and hybridized with DIG-labelled RNA probes at 70°C. After stringent washes with a series of saline-sodium citrate (SSC) buffers, hybridized probes were detected via immunohistochemical examination using an alkaline phosphatase-conjugated anti-DIG antibody (1:1,000; Roche). For signal visualization, a chromogenic reaction with a nitro blue tetrazolium/5-bromo-4-chloro-3-indolyl phosphate (NBT/BCIP) was performed at 25°C for the following durations: 5-HTR1D, 5-HTR1E, 5-HTR1F, and 5-HTR2B, 18–42.5 h; 5-HTR5A, 18.3–39.5 h; 5-HTR7, 19.5–39.8 h; 5-HTR1B, 18.2–39 h; 5-HTR3A, 19.5 h; and LEF1, 18–38.8 h. Sense probes were used as negative controls in every experiment.
Image Acquisition and Data Processing
Bright-field images of whole sections on each slide glass were semiautomatically taken using the NanoZoomer 2.0 HT or NanoZoomer XR systems (Hamamatsu Photonics, Shizuoka, Japan). Microscopic fields of interest were cropped using the NDP.view2 software (ver. 2.7.25; Hamamatsu Photonics, Shizuoka, Japan). Cropped images were converted to 8-bit images and their brightness and contrast were adjusted using ImageJ (ver. 1.52a, National Institute of Health, Bethesda, MD, United States).
Terminology of Avian Hippocampal Formation
All histological terminologies used in this study were based on the atlas of Kuenzel and Masson (1988) and the descriptions of the avian brain nomenclature consortium (Reiner et al., 2004), except for HF and its subdivisions. The subdivision schemes of avian HF were based on various aspects and species, such as tract tracing and Nissl staining in pigeons (Atoji and Wild, 2004; Atoji and Wild, 2006), histochemistry of neurotransmitter radioligands in pigeons (Herold, et al., 2014), immunohistochemistry of several neurochemical markers in developing chickens (Suarez, et al., 2006), and combinatorial expression patterns of morphogenetic genes in developing chickens (Abellan, et al., 2014). No uniform nomenclature has been applied across species (Herold, et al., 2015). In this study, the histological position of HF in chicks was based on the nomenclature set by Puelles et al. (2007). Based on previous studies (Kuenzel and Masson, 1988; Atoji and Wild, 2004; Atoji and Wild, 2006; Suarez, et al., 2006; Puelles, et al., 2007; Abellan, et al., 2014; Herold, et al., 2014), we used the following subdivision terms: V-shaped complex (V), dorsal medial region (DM), ectopic part of the rostral APH (APHre), and dorsal lateral region (DL) (Figure 1). The relationships between the terminologies of avian HF used in this study and those in previous studies are summarized in Table 1.
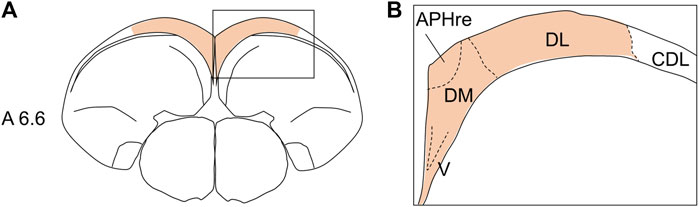
FIGURE 1. Position of chick HF and its subdivisions (A) diagram of the coronal section of the chick telencephalon showing the HF position (A). Subdivisions of the chick HF (B). See Table 1 for chick HF terminology. Regarding the HF range, to this day, no consensus or view for the inclusion of CDL exists (Puelles et al., 2007; Medina et al., 2017b). APHre, ectopic part of the rostral area parahippocampalis; CDL, corticoidea dorsolateralis; DL, dorsal lateral region of HF; DM, dorsal medial region of HF; V, V-shaped complex. Levels of sections were in accordance with those mentioned in Kuenzel and Masson’s atlas (Kuenzel and Masson, 1988).
Results
Selection of Chick Orthologues of Mammalian 5-HTR Genes and LEF1 as APHre Marker Gene
We initially selected the chick orthologs of mammalian 5-HTR genes, namely 5-HTR1B, 5-HTR1D, 5-HTR1E, 5-HTR1F, 5-HTR2B, 5-HTR3A, 5-HTR5A, and 5-HTR7. Among these, the expression patterns of 5-HTR1B and 5-HTR3A in the chick telencephalon have been previously reported (Fujita, et al., 2020). The chicken genome also contains 5-HTR6 (International Chicken Genome Sequencing Consortium, 2004); yet we could not obtain a subclone in our study. LEF1 is an important transcription factor for granule cell production in the dentate gyrus (Galceran, et al., 2000). We found that the chick LEF1 ortholog exhibited sequence similarities with that of humans: 95% (protein) and 85.2% (DNA). In our previous studies, we searched for sequence similarities between chick 5-HTR1B, 5-HTR1D, 5-HTR1E, 5-HTR1F, 5- HTR2B, 5-HTR3A, 5-HTR5A, and 5-HTR7 with those from other animals (Fujita et al., 2020; Fujita et al., 2022). The accession numbers and molecular characteristics of ortholog gene probes are summarized in Supplementary Table S2. We accordingly designed probes to detect multiple transcript variants of orthologs registered in the database. Consecutively, we performed in situ hybridization (ISH) and analyzed the expression patterns of these orthologs in the telencephalon of chicks.
Expression of 5-HTR1D in Chick Telencephalon
To comprehensively examine the expression pattern of 5-HTR1D in the chick telencephalon, we performed ISH using the 5-HTR1D probe on coronal sections at approximately A14.4 to A 4.6 of naive chicks on posthatch day 1 (P1). We detected signals in a large part of the mesopallium (Figure 2A–E, A′-E′), entopallium (Figure 2B, B′), field L (Figures 2D,E, D′-E′), a part of the hyperpallium (Figure 2C, C′), a large part of the arcopallium (Figure 2C,D, C′-D′), and the lateral part of the nidopallium (Figure 2C–F, C′-F′). In addition, we detected signals in a part of the DM (Figure 2C–F, C′-F′), DL in a characteristic layered manner (Figure 2C–F, C′-F′), and the corticoidea dorsolateralis area (CDL) (Figure 2D–F, D′-F′).
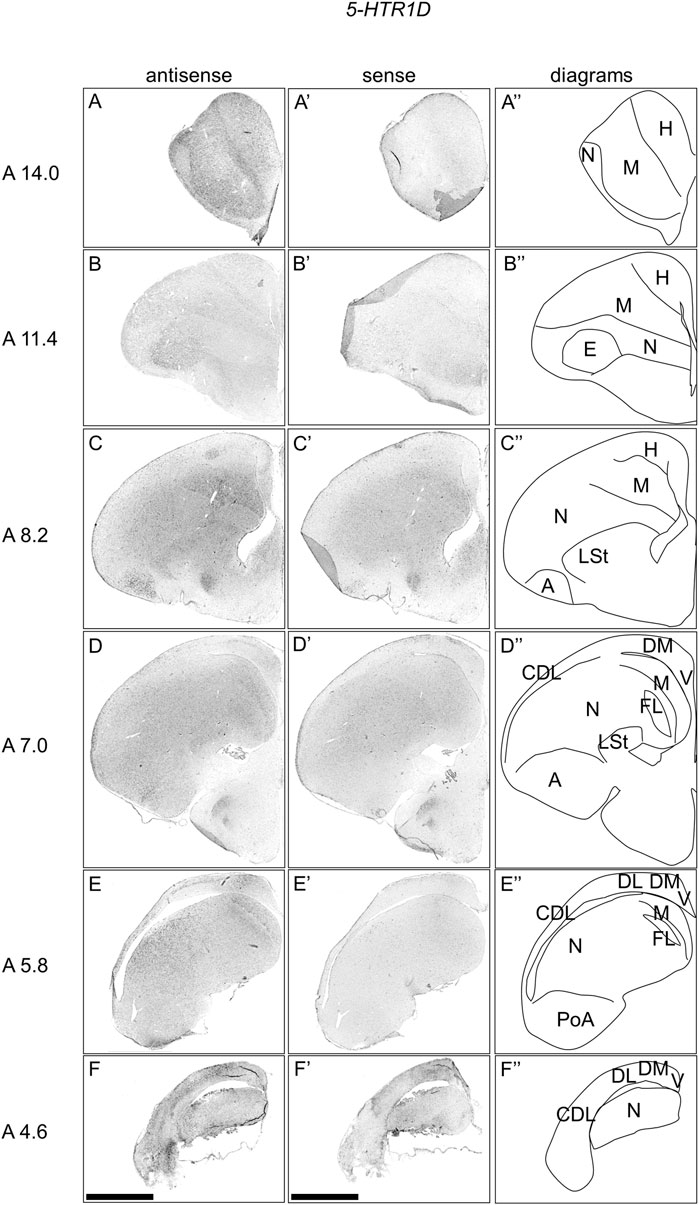
FIGURE 2. In situ hybridization of 5-HTR1D in P1 chick telencephalons. Digoxigenin-labelled RNA antisense (A–F) and sense (A′–F′) 5-HTR1D probes were used for in situ hybridization in coronal sections of the P1 chick telencephalon. To evaluate the expression patterns of 5-HTR1D, sections from seven chicks were analyzed, and representative images from four chick brain sections are shown. Diagrams of coronal sections are shown in the rightmost panels (A–F″). Levels of sections (A 14.0 to A 4.6) were in accordance with those mentioned in Kuenzel and Masson’s chick atlas (Kuenzel and Masson, 1988). A, arcopallium; CDL, area corticoidea dorsolateralis; DL, dorsal lateral region of HF; DM, dorsal medial region of HF; E, entopallium; FL, field L; H, hyperpallium; LSt, lateral striatum; M, mesopallium; N, nidopallium; PoA, posterior pallial amygdala; V, V-shaped complex; P1: posthatch day 1. Scale bars = 2.5 mm.
Expression of 5-HTR1E in Chick Telencephalon
We examined the expression pattern of 5-HTR1E in sections A14.2 to A6.0 of P1 chick telencephalons (Figure 3). We detected strong signals in the nucleus taeniae of the amygdala (TnA) (Figure 3E, E′), and a part of the DM, in a cluster manner (Figure 3C–F, C′-F′). We also detected signals in a part of the mesopallium (Figure 3A–F, A′-F′), hyperpallium (Figure 3D, D′), DL in a layered manner (Figure 3D–F, D′-F′), and CDL (Figure 3F, F′).
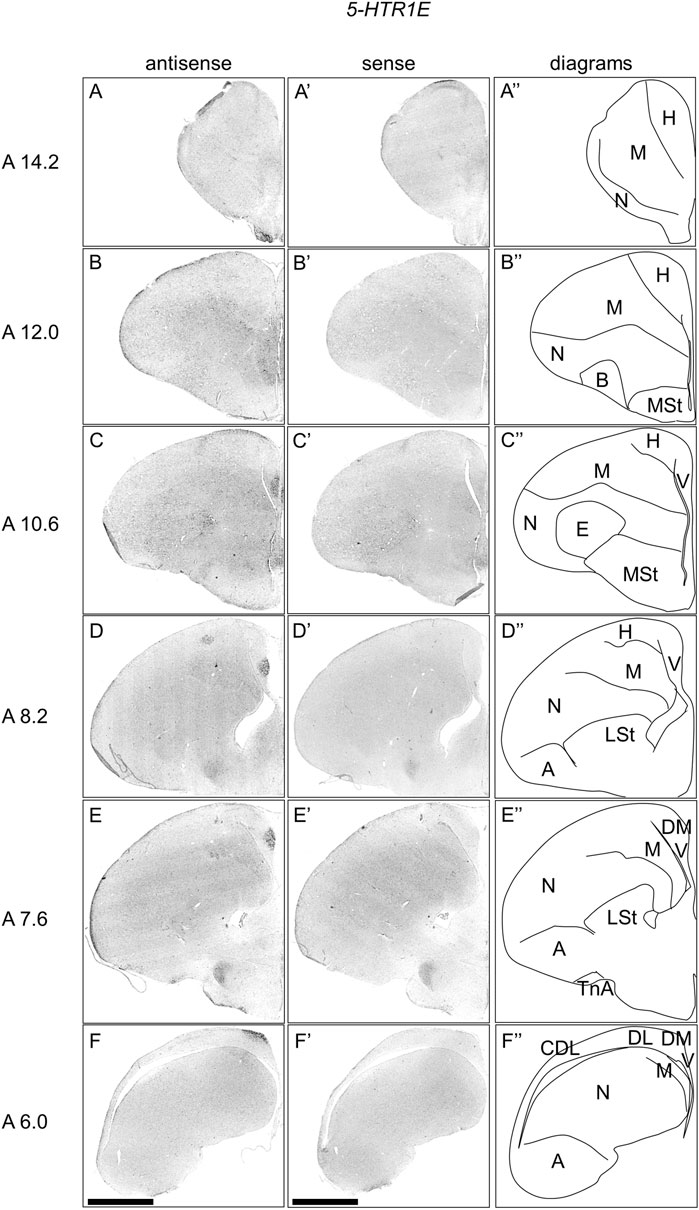
FIGURE 3. In situ hybridization of 5-HTR1E in P1 chick telencephalons. Digoxigenin-labelled RNA antisense (A–F) and sense (A′–F′) 5-HTR1E probes were used for in situ hybridization in coronal sections of the P1 chick telencephalon. To evaluate the expression patterns of 5-HTR1E, sections from nine chicks were analyzed, and representative images from three chick brain sections are shown. Diagrams of coronal sections are shown in the rightmost panels (A–F″). Levels of sections (A 14.2 to A 6.0) were in accordance with those mentioned in Kuenzel and Masson’s chick atlas (Kuenzel and Masson, 1988). A: arcopallium; B: basorostralis; CDL: the area corticoidea dorsolateralis; DL: the dorsal lateral region of HF; DM: the dorsal medial region of HF; E: entopallium; H: hyperpallium; LSt lateral striatum; M, mesopallium; MSt, medial striatum; N, nidopallium; TnA, nucleus taeniae of the amygdala; V, V-shaped complex; P1: posthatch day 1. Scale bars = 2.5 mm.
Comparison of 5-HTR1E and LEF1 Expression Patterns in Chick APH
To compare the expression patterns between 5-HTR1E and LEF1, which is the APHre region marker (Abellan et al., 2014), we performed ISH using 5-HTR1E and LEF1 probes in neighboring sections of A8.8 and A6.6, respectively (Figure 4). Interestingly, we found that the regions of expression of 5-HTR1E and LEF1 in the APHre matched well at both sections (Figure 4D,E, J-K, D′-E′, J′-K′).
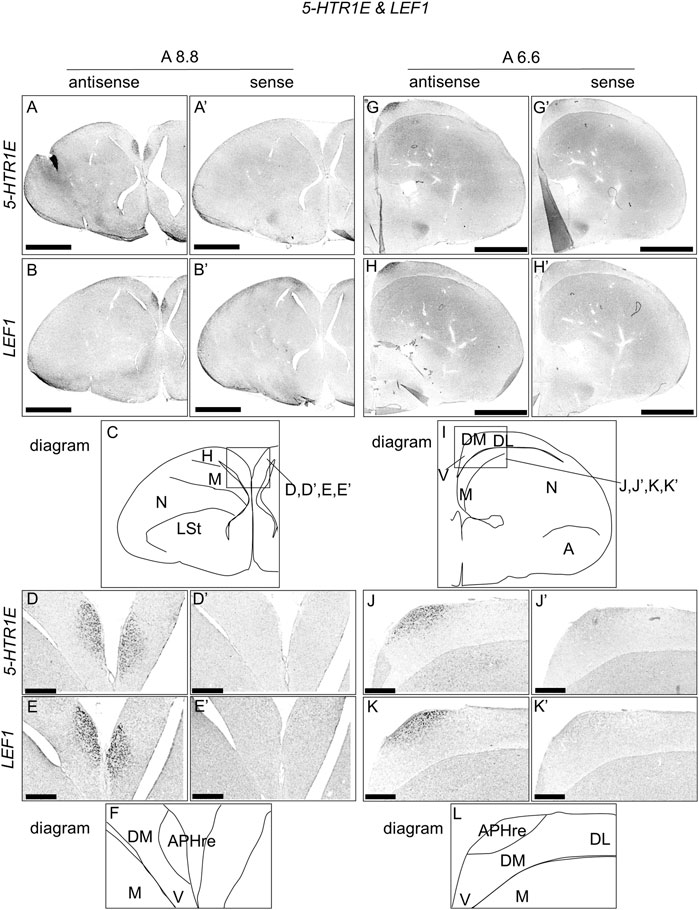
FIGURE 4. Comparison of 5-HTR1E and LEF1 expression patterns in P1 chick telencephalon sections. In situ hybridization using DIG-labelled RNA antisense and sense 5-HTR1E (A,D,G,J, and A′,D′,G′,J′), respectively, and LEF1 (B,E,H,K, and B′,E′,H′,K′), respectively probes in P1 chick brain coronal sections are shown. Panels (C) (F) (I), and (L) show diagrams of (A) (D) (G), and (J), respectively. Levels of sections (A8.8 and A6.6) were in accordance with those mentioned in Kuenzel and Masson’s chick atlas (Kuenzel and Masson, 1988). A: arcopallium; APHre: ectopic part of the rostral area hippocampalis; DL, dorsal lateral region of HF; DM, dorsal medial region of HF; H, hyperpallium; LSt, lateral striatum; M, mesopallium; N, nidopallium; V, V-shaped complex; P1: posthatch day 1. Scale bars = 2.5 mm (A,B,G,H,A′,B′,G′,H′) and 500 µm (D,E,J,K,D′,E′,J′,K′).
Expression of 5-HTR1F in Chick Telencephalon
We then examined the expression patterns of 5-HTR1F in sections A14.4 to A5.0 of P1 chick telencephalons (Figure 5). Our analysis revealed the presence of signals in the interstitial part of the hyperpallium (Figure 5A,B, A′-B′).
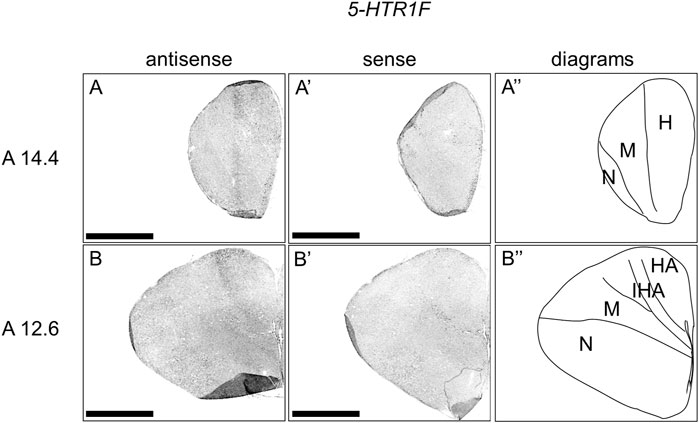
FIGURE 5. In situ hybridization of 5-HTR1F in P1 chick telencephalon. Digoxigenin-labelled RNA antisense (A–B) and sense (A′–B′) 5-HTR1F probes were used for in situ hybridization in coronal sections of the P1 chick telencephalon. To evaluate the expression patterns of 5-HTR1F, sections from eight chicks were analyzed, and representative images from two chick brain sections are shown (A″–B″) Diagrams of coronal sections are shown in the rightmost panels. Levels of sections (A14.4 and A12.6) were in accordance with those mentioned in Kuenzel and Masson’s chick atlas (Kuenzel and Masson, 1988). H, hyperpallium; HA, hyperpallium apicale; IHA, interstitial part of the hyperpallium; M, mesopallium; N, nidopallium; P1: posthatch day 1. Scale bars = 2.5 mm.
Expression of 5-HTR5A in Chick Telencephalon
We also examined the expression patterns of 5-HTR5A in sections A13.8 to A4.4 of P1 chick telencephalons (Figure 6). We accordingly detected the expression of 5-HTR5A in the dorsal arcopallium, lateral nidopallium, DL, and CDL (Figure 6A,B, A′-B′).
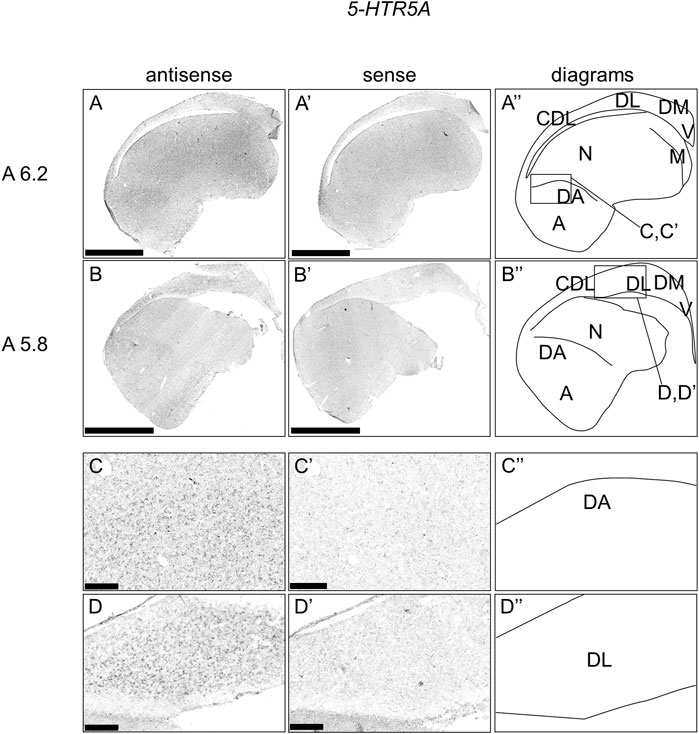
FIGURE 6. In situ hybridization of 5-HTR5A in P1 chick telencephalon. Digoxigenin-labelled RNA antisense (A–D) and sense (A′–D′) 5-HTR5A probes were used for in situ hybridization in coronal sections of the P1 chick telencephalon. To evaluate the expression patterns of 5-HTR5A, sections from six chicks were analyzed, and representative images from two chick brain sections are shown (A″–D″) Diagrams of coronal sections are shown in the rightmost panels. Levels of sections (A 6.2 and A 5.8) are in accordance with those mentioned in Kuenzel and Masson’s chick atlas (Kuenzel and Masson, 1988) (C–D and C′–D′) Magnified views of brain areas shown in the boxes in (A″ and B″). A: arcopallium; CDL, area corticoidea dorsolateralis; DA, dorsal arcopallium; DL, dorsal lateral region of HF; DM, dorsal medial region of HF; M, mesopallium; N, nidopallium; V, V-shaped complex; P1: posthatch day 1. Scale bars = 2.5 mm (A–B and A′–B′) and 250 µm (C–D and C′–D′).
Expression of 5-HTR7 in Chick Telencephalon
We further examined the expression patterns of 5-HTR7 in sections A13.6 to A4.4 of P1 chick telencephalons (Figure 7) and found that 5-HTR7 was mainly expressed in a large part of the arcopallium, lateral nidopallium, DM, DL (Figure 7A–C, A′-C′), and CDL (Figure 7B,C, B′-C′).
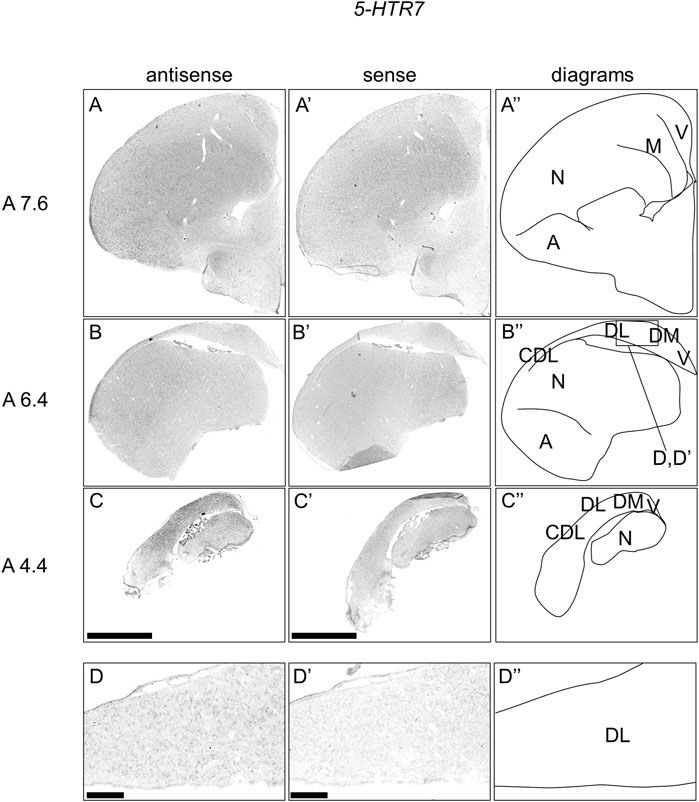
FIGURE 7. In situ hybridization of 5-HTR7 in P1 chick telencephalons. Digoxigenin-labelled RNA antisense (A–D) and sense (A′–D′) 5-HTR7 probes were used for in situ hybridization in coronal sections of the P1 chick telencephalon. To evaluate the expression patterns of 5-HTR7, sections from six chicks were analyzed, and representative images from three chick brain sections are shown (A″–D″) Diagrams of coronal sections are shown in the rightmost panels. Levels of sections (A 7.6, A 6.4, and A 4.4) were in accordance with those mentioned in Kuenzel and Masson’s chick atlas (Kuenzel and Masson, 1988) (D and D′) Magnified views of brain areas shown in the boxes in (B″). A, arcopallium; CDL, area corticoidea dorsolateralis; DL, dorsal lateral region of HF; DM, dorsal medial region of HF; M, mesopallium; N, nidopallium; V, V-shaped complex; P1: posthatch day 1. Scale bars = 2.5 mm (A–C and A′–C′) and 250 µm (D and D′).
Expression of 5-HTR2B in Chick Telencephalon
Finally, we examined the expression patterns of 5-HTR2B in sections A13.2 to A5.0, but did not detect any signal (data not shown), suggesting that the levels of expression of 5-HTR2B were either very low or cells expressing 5-HTR2B were very rare.
Comparison of 5-HTR1B, 5-HTR1D, 5-HTR1E, and 5-HTR3A Expression Patterns in Chick Hippocampal Formation
We found that 5-HTR1D and 5-HTR1E were clearly expressed in chick HF. In our previous study, we revealed the characteristic expression patterns of 5-HTR1B and 5-HTR3A (Fujita, et al., 2020). Subsequently, to understand the relationship between the regions of expression of these 5-HTRs in chick HF, we performed ISH using 5-HTR1B, 5-HTR1D, 5-HTR1E, and 5-HTR3A probes on neighboring sections of A8.8 to A8.6 (Figures 8, 9A6.6 to A6.4 (Figures 10–13). We found that 5-HTR1B was expressed in the whole DL (Figure 8A, A′, Figure 9, Figure 10A, A′, Figure 13), whereas sparsely in the DM (Figure 8A, A′, Figure 9, Figure 10A, A′, Figure 12). We also detected the expression of 5-HTR1D in a part of DM (Figure 8B, B′, Figure 9, Figure 10B, B′, Figure 12), and in DL in a layered manner (Figure 8B, B′, Figure 9, Figure 10B, B′, Figure 13). We found that 5-HTR1E was expressed in APHre (Figure 8C, C′, Figure 9, Figure 10C, C′, Figure 12), and in DL in a layered manner (Figure 8C, C′, Figure 9, Figure 10C, C′, Figure 13). We detected sparse signals of expression of 5-HTR3A in both the DM and DL (Figure 8D, D′, Figure 9, Figure 10D, D’, Figure 12, Figure 13). Finally, we observed that 5-HTR1B and 5-HTR3A were sparsely expressed in V, whereas we detected a faint expression of 5-HTR1E and no expression of 5-HTR1D in this region (Figure 11).
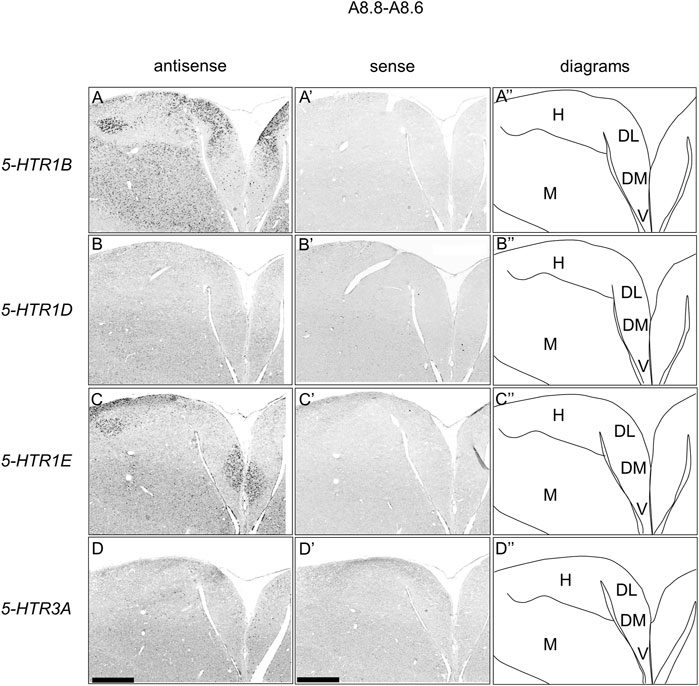
FIGURE 8. Comparison of 5-HTRs expression patterns in HF in P1 chick telencephalons using neighboring sections at approximately A 8.8 level. In situ hybridization using DIG-labelled RNA antisense and sense 5-HTR1B (A and A′), 5-HTR1D (B and B′), 5-HTR1E (C and C′), and 5-HTR3A (D and D′) probes in coronal sections of P1 chick telencephalons (A″–D″) Diagrams of coronal sections are shown in the rightmost panels. DL, dorsal lateral region of HF; DM, dorsal medial region of HF; H, hyperpallium; M, mesopallium; V, V-shaped complex; P1: posthatch day 1. Scale bars = 1 mm.
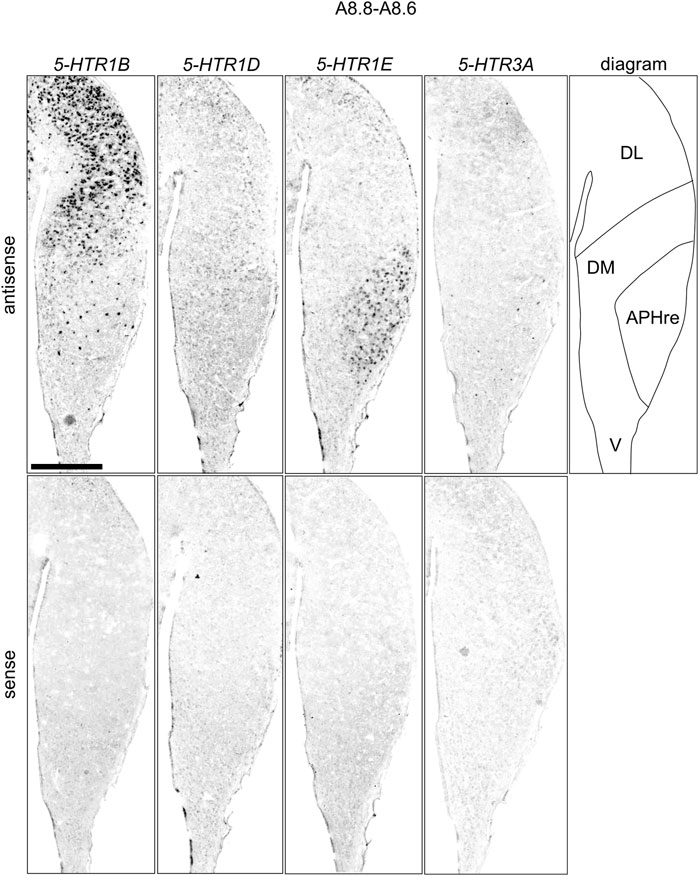
FIGURE 9. Comparison of 5-HTRs expression patterns in HF in P1 chick telencephalons using neighboring sections at approximately A 8.8 level focused on HF. Magnified views of HF surrounding regions are shown in Figure 8. APHre: ectopic part of the rostral area hippocampalis; DL: dorsal lateral region of HF; DM: dorsal medial region of HF; V: V-shaped complex; P1: posthatch day 1. Scale bars = 1 mm.
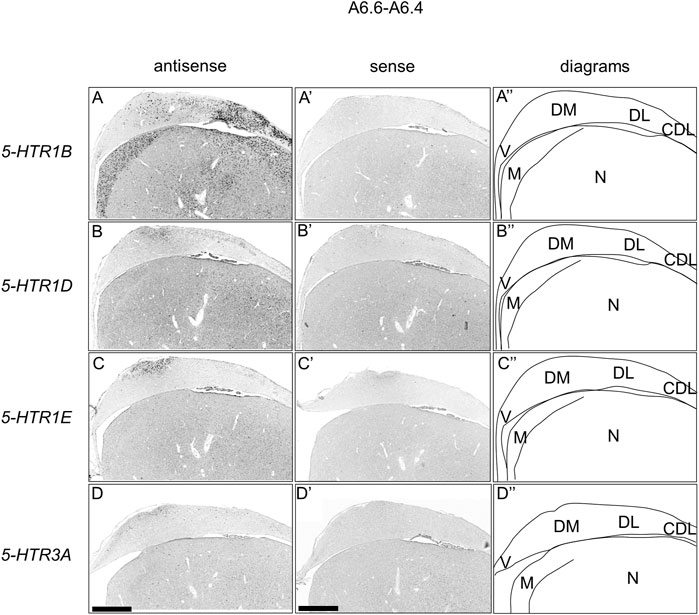
FIGURE 10. Comparison of 5-HTRs expression patterns in HF in P1 chick telencephalons using neighboring sections at approximately A 6.6. In situ hybridization using DIG-labelled RNA antisense and sense 5-HTR1B (A and A′), 5-HTR1D (B and B′), 5-HTR1E (C and C′), and 5-HTR3A (D and D′) probes in coronal sections of P1 chick telencephalons (A″–D″) Diagrams of coronal sections are shown in the rightmost panels. CDL: area corticoidea dorsolateralis; DL: dorsal lateral region of HF; DM: dorsal medial region of HF; M: mesopallium; N: nidopallium; V: V-shaped complex; P1: posthatch day 1. Scale bars = 1 mm.
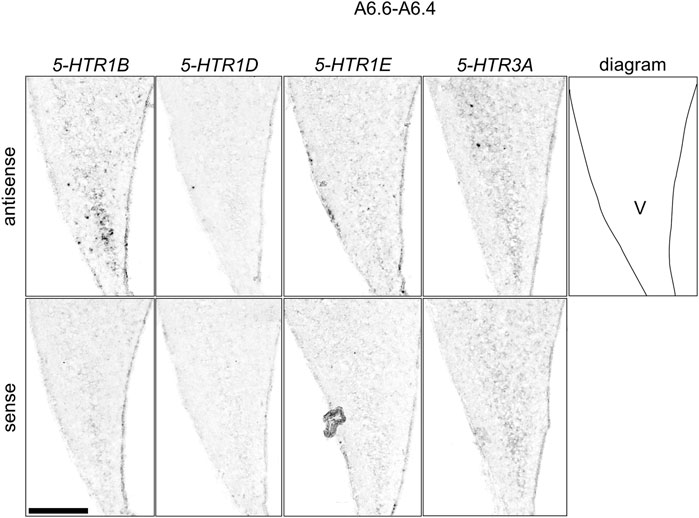
FIGURE 11. Comparison of 5-HTRs expression patterns in the HF in the P1 chick telencephalons using neighboring sections around A 6.6 focused on V. Magnified views of HF surrounding regions shown in Figure 10. V: V-shaped complex; P1: posthatch day 1. Scale bars = 250 µm.
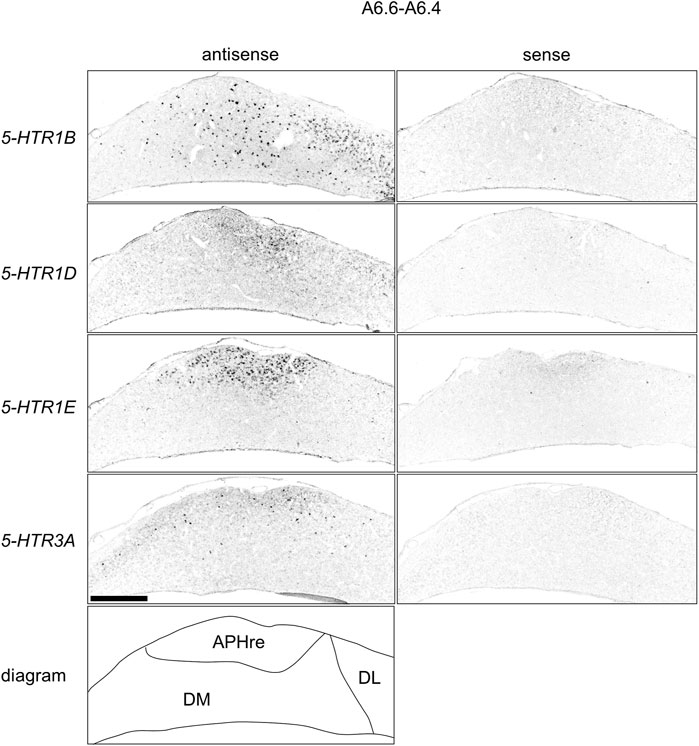
FIGURE 12. Comparison of 5-HTRs expression patterns in HF in P1 chick telencephalons using neighboring sections at approximately A 6.6 focused on DM. Magnified views of HF surrounding regions shown in Figure 10. APHre, ectopic part of the rostral area parahippocampalis; DL, dorsal lateral region of HF; DM, dorsal medial region of HF; P1: posthatch day 1. Scale bars = 500 µm.
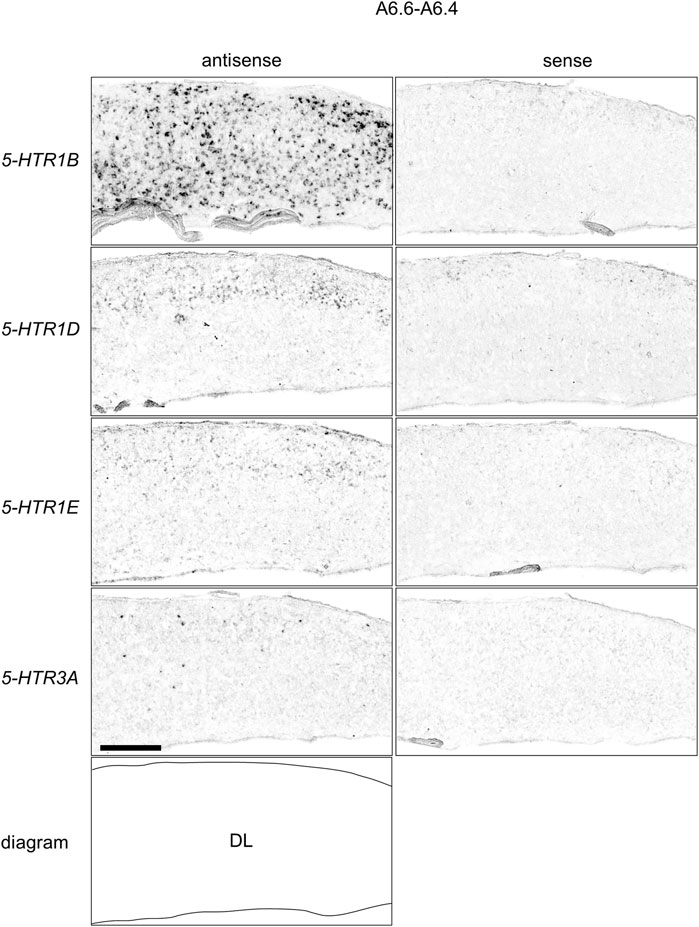
FIGURE 13. Comparison of 5-HTRs expression patterns in HF in P1 chick telencephalons using neighboring sections at approximately A 6.6 focused on DL. Magnified views of HF surrounding regions shown in Figure 10. DL: dorsal lateral region of HF; P1: posthatch day 1. Scale bars = 250 µm.
Discussion
Expression Patterns of 5-HTR Subfamily Genes in Chick HF
In the present study, we revealed that 4 5-HTRs, 5-HTR1B, 5-HTR1D, 5-HTR1E, and 5-HTR3A, were expressed in a subdivision- and layer-selective manner in chick HF (Figure 14). We also found that 5-HTR5A and 5-HTR7, which were faintly expressed, did not clearly show subdivision- or layer-selective expression patterns in the chick HF.
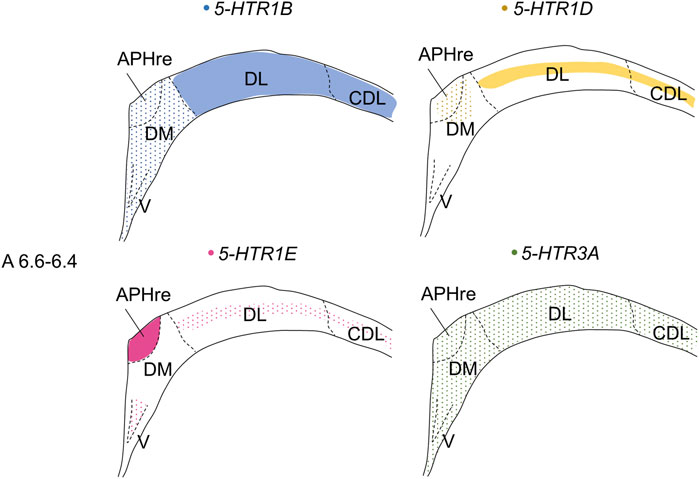
FIGURE 14. Schematic summary of expression patterns of 5-HTR1B, 5-HTR1D, 5-HTR1E, and 5-HTR3A in subdivisions in the P1 chick HF. Representative expression patterns in sections at approximately A 6.6 to A 6.4 are shown in colored areas (blue, 5-HTR1B; yellow, 5-HTR1D; magenta, 5-HTR1E; green, 5-HTR3A). A dotted pattern indicates the sparse distribution of expressing cells. Levels of sections were in accordance with those mentioned in Kuenzel and Masson’s chick atlas (Kuenzel and Masson, 1988). APHre: ectopic part of the rostral area parahippocampalis; CDL, corticoidea dorsolateralis; DL, dorsal lateral region of HF; DM, dorsal medial region of HF; V, V-shaped complex; P1: posthatch day 1.
We found that 5-HTR1B was highly expressed in the DL, whereas sparsely in V, DM, APHre, while 5-HTR3A was expressed in all the V, DM, DL, APHre, sparsely. In comparison, in mammals, 5-Htr1b is expressed in Cornu Ammonis 1 (CA1) and CA3 pyramidal cells, GABAergic interneurons of the hilus, granule cells of dentate gyrus (DG), the subgranular zone, the layer II pyramidal cells in the entorhinal cortex (EC) (Tanaka, et al., 2012), while 5-Htr3A is expressed in GABAergic interneurons of the hilus, in the subgranular zone, in scattered EC cells, CA1, CA3, and DG (Tecott, et al., 1993; Fonseca et al., 2001; Morales and Wang 2002; Tanaka, et al., 2012; Koyama, et al., 2017). Considering the expression patterns of 5-HTR1B and 5-HTR3A, our results support the correspondence of V and DM in the avian HF to the mammalian hippocampal proper (DG and CA subfields) (Montagnese et al., 1996; Szekely, 1999; Atoji and Wild, 2004; Atoji and Wild, 2006; Suarez, et al., 2006; Puelles et al., 2007; Herold et al., 2014; Abellan et al., 2014; Atoji et al., 2016; Medina et al., 2017b). To date, a one-to-one correspondence of subdivisions between the avian and mammalian HF has not been established, while various studies have postulated on possible areas in avian HF corresponding to mammalian DG. One suggestion is that the mammalian DG corresponds to V in avian HF (Atoji and Wild, 2004; Suarez, et al., 2006; Puelles et al., 2007; Gupta, et al., 2012; Herold et al., 2014; Atoji et al., 2016). Another theory is that the mammalian DG corresponds to DM in avian HF (Montagnese et al., 1996; Szekely, 1999). However, another possibility is that DG might be a novel acquisition in mammals, which would imply that there is no homolog in birds. The avian HF has undergone divergence over hundreds of millions of years of evolution, thus making it difficult to compare the subdivisions of HF between birds and mammals (Hevner, 2016; Striedter, 2016). In fact, although no macrostructure corresponding to mossy fibers in the mammalian DG has been observed in avian HF (Faber et al., 1989; Montagnese et al., 1993, 1996; Tombol et al., 2000; Herold, et al., 2014), this does not exclude the existence of a neuron population in the avian HF corresponding to granule cells in the mammalian DG. This candidate neuronal cell population corresponding to granule cells might be unevenly distributed in the avian HF. We assumed that using chick orthologs of another marker gene to distinguish pyramidal from granule cells in mammalian HF could provide new clues for the correspondence between avian and mammalian HF.
In chicks, 5-HTR1E was highly expressed in APHre, and weakly in the V and DL in a layer-selective manner, suggesting that the APHre cell population that preferentially expresses 5-HTR1E has novel characteristics. In contrast, in mammals, 5-Htr1e was shown to be expressed in the CA fields and DG (Bruinvels et al., 1994a; Mengod, et al., 2006; Vilaro et al., 2020). However, because the relationship between APHre in avian HF and other HF subdivisions and the function of APHre are completely unknown (Abellan et al., 2014), it was difficult to determine the correspondence of APHre to the mammalian subdivision of HF. In the future, a better understanding of the features of APHre in chicks with respect to multiple aspects, such as connectivity, electrophysiological properties, and behavioral function, is expected to help determine the correspondence of APHre subdivisions.
Patch RNA-sequencing analysis in mammals showed that 5-Htr1d was expressed in the GABAergic interneurons of CA1 (Luo, et al., 2019). We here found that 5-HTR1D was expressed in DM, APHre, and DL in a layer-selective manner. Based on this, we assumed that 5-HTR1D might also be expressed in GABAergic interneurons in chick HF.
In the past, the cytoarchitecture of avian HF was considered quite different from that of the mammalian 3-layered HF, as it appeared to have completely lost its layered structure. Three layers are clearly present in lizards but less visible in crocodiles (closest to birds) as for HF homologue. (Hevner, 2016; Striedter, 2016). However, detailed immunohistochemical analysis and combinatorial expression analysis using developmental regulatory genes revealed the existence of a layered cytoarchitecture orthogonal to radial glial fibers in the chick HF during its developmental stages (Redies et al., 2001; Abellan et al., 2014). Such a layered structure has also been confirmed in the HF of adult pigeons (Suarez et al., 2006; Herold et al., 2019). In our study, we showed that the expression patterns of 5-HTR1D and 5-HTR1E in DL were layered, suggesting a selective regulation of the DL layer by these receptors. Our data indicated that the neuronal population in this layer might have functional roles in cognition and emotion.
We also examined the expression of 5-HTR2B and failed to detect it in chick telencephalons, suggesting either a low level of expression of 5-HTR2B or the rarity of expressing cells. Interestingly, the expression level of 5-Htr2b in the telencephalon of mammals was found to be low (Bonaventure et al., 2002; Vilaro, et al., 2020). This finding was consistent with our obtained results for the expression of 5-HTR2B.
Possible Functions of Chick 5-HTRs in Chick Telencephalon Other Than HF
Regarding its the expression pattern, we noticed that 5-HTR1D was expressed in a large part of the mesopallium, arcopallium, and a part of the hyperpallium, nidopallium, HF, CDL, and major part of the intercalated nidopallium (entopallium and field L (Jarvis et al., 2013)). Of note, the intercalated nidopallium receives sensory projections from the thalamus (Reiner et al., 2004; Jarvis et al., 2013). We previously showed that 5-HTR2C was preferentially expressed in intercalated nidopallium in chicks (Fujita et al., 2020). Considering the expression combination of 5-HTR1D and 5-HTR2C, it is possible that these 2 5-HTRs might work together in sensory input information processing in the intercalated nidopallium in birds. In mammals, 5-HTR1D was demonstrated to be distributed in the frontoparietal cortex, primary olfactory cortex, accumbens nucleus, caudate-putamen, and lateral mammillary nucleus (Bruinvels, et al., 1994a, 1994b; Vilaro et al., 2020). However, the regional expression of 5-HTR1D in the chick hyperpallium appeared to be limited (Figure 2C, C’), suggesting a serotonergic modulation in the neuronal population of the hyperpallium via 5-HTR1D.
In the case of the expression pattern of 5-HTR1E, we found that the major regions of expression were the APHre, TnA, and a part of the hyperpallium. We previously showed that 5-HTR2C and 5-HTR4 were preferentially expressed in the TnA in chicks (Fujita, et al., 2020), whereas in mammals, 5-HTR2C and 5-HTR4 are expressed in the amygdala (Huang and Kandel, 2007; Bombardi 2014; Bocchio et al., 2016). TnA is considered to be the counterpart of the mammalian medial amygdala (Reiner et al., 2004; Yamamoto et al., 2005; Yamamoto and Reiner, 2005; Hanics et al., 2017), which is functionally associated with social behaviors, including sexual behavior and social interactions (Ikebuchi, et al., 2009; Mayer et al., 2017; Mayer, et al., 2019). These findings indicated that 5-HT might play a key role in shaping social responses in birds and mammals. In this study, we found another 5-HTR, 5-HTR1E, which was preferentially expressed in TnA. Whereas, in mammals, 5-Htr1e is expressed in the amygdala (Lowther et al., 1992; Bruinvels et al., 1994a; Mengod et al., 2006). Our findings regarding 5-HTR1E also support the potentially conserved roles of 5-HTRs in the mammalian medial amygdala and avian TnA. Furthermore, the expression patterns of 5-HTR1E and 5-HTR1D in the hyperpallium, appeared to be similar (Figure 8A,C, A′, C’), suggesting a serotonergic modulation in the neuronal population of the hyperpallium via these receptors.
Regarding the expression pattern of 5-HTR1F, we observed that it was selectively expressed in the interstitial part of the hyperpallium (IHA). According to developmental studies, the hyperpallium of birds is a region homologous to the mammalian neocortex (Fernandez, et al., 1998; Puelles et al., 2000), and is composed of four pseudolayers: the apical part of the hyperpallium, IHA, the intercalated part of the hyperpallium, and the densocellular part of the hyperpallium (Medina and Reiner 2000; Reiner et al., 2004). Among them, the IHA has projection terminals of sensory information from the thalamus, which is considered equivalent to layer IV of the mammalian neocortex (Bangnoli and Burkhalter, 1983; Miceli and Reperant, 1985; Miceli et al., 1990; Atoji et al., 2018; Atoji and Wild, 2019). Despite some species differences, 5-Htr1F is expressed in the intermediate cortical layers (layers IV and V) in mammals (Waeber and Moskowitz 1995; Mengod et al., 1996; Pascual et al., 1996; Lucaites et al., 2005). Taken together, both 5-HTR1F-expressing neurons in chick IHA and 5-Htr1F-expressing neurons in layer IV of the mammalian neocortex might have conserved functions in processing sensory input under serotonergic modulation.
We also detected that 5-HTR5A was expressed in the dorsal arcopallium, lateral nidopallium, HF, and CDL. In mammalian brains, 5-HTR5A is distributed in the piriform cortex, habenula, and HF, suggesting its involvement in the regulation of cognition, anxiety, and sensory perception (Plassat et al., 1992; Erlander et al., 1993; Matthes et al., 1993; Kinsey et al., 2001; Mengod et al., 2006; Vilaro et al., 2020). The dorsal arcopallium is the proposed region homologous to the basolateral amygdala in terms of embryonic origin and expression combinations of conserved morphogenetic genes (Martinez-Garcia et al., 2009; Medina et al., 2017a; Martinez-Garcia and Lanuza, 2018). The similarity in the regional expression of 5-HTR5A between mammalian and chick telencephalons suggested its conserved function in the serotonergic modulation in telencephalons.
Finally, we observed that 5-HTR7 was expressed in the arcopallium, lateral nidopallium, HF, and CDL. In the mammalian telencephalon, 5-Htr7 is distributed in some brain regions, including the HF and amygdala (Gustafson et al., 1996; Mengod et al., 1996; Martin-Cora and Pazos, 2004; Mengod et al., 2006; Tanaka et al., 2012). This finding suggested the conserved 5-HTR7-mediated serotonergic modulation in the amygdala and HF between birds and mammals.
Conclusion
We comprehensively revealed the expression patterns of 5-HTR subfamily genes in the chick telencephalon and specifically found that 5-HTR1D, 5-HTR1E, 5-HTR5A, and 5-HTR7 were expressed in chick HF. These receptors might be involved in the regulation of HF neural circuits that control cognitive and emotion-related functions in birds. In addition, we found that 5-HTR1B, 5-HTR1D, 5-HTR1E, and 5-HTR3A were expressed in HF in a subdivision- and layer-selective manner. Our findings can facilitate the improved understanding of the correspondence between the avian and mammalian HF.
Data Availability Statement
The original contributions presented in the study are included in the article/Supplementary Material, further inquiries can be directed to the corresponding author.
Ethics Statement
The animal study was reviewed and approved by Teikyo University.
Author Contributions
TF and SY designed the study and performed the experiments; TF, NA, CM, EF, KH and SY analyzed the data; TF, NA, CM, TM, KH and SY wrote the paper. All authors have reviewed the manuscript.
Funding
This work was supported by the Fund for the Promotion of Joint International Research (Fostering Joint International Research [B]) (TF, TM, KH. 19KK0211), Grants-in-Aid for Scientific Research from the Japan Society for the Promotion of Science (SY, 24590096, 15K07945, 18K06667, 21K06535; NA, 24790089, 20K06915; TM, 25291071, 18K07351; C.M, 20K16472 and KH, 26440182, 17K07492, 20K06747), the Uehara Memorial Foundation (SY), the Sagawa Foundation for Promotion of Cancer Research (SY), a Grant-in-Aid for Scientific Research on Innovative Areas “Memory dynamism” (26115522), “Adaptive circuit shift” (15H01449) and “Evolinguistics” (20H05012) from the Ministry of Education, Culture, Sports, Science and Technology (KH), the Naito Foundation (KH.), and the Japan Foundation for Applied Enzymology (KH).
Conflict of Interest
The authors declare that the research was conducted in the absence of any commercial or financial relationships that could be construed as a potential conflict of interest.
Publisher’s Note
All claims expressed in this article are solely those of the authors and do not necessarily represent those of their affiliated organizations, or those of the publisher, the editors and the reviewers. Any product that may be evaluated in this article, or claim that may be made by its manufacturer, is not guaranteed or endorsed by the publisher.
Acknowledgments
We thank Y. Nishiwaki, T. Sato, A. Suzuki, and M. Takagi (Teikyo University, Faculty of Pharmaceutical Sciences) for their technical assistance. We would like to thank Editage (www.editage.com) for English language editing.
Supplementary Material
The Supplementary Material for this article can be found online at: https://www.frontiersin.org/articles/10.3389/fphys.2022.882633/full#supplementary-material
References
Abellán A., Desfilis E., Medina L. (2014). Combinatorial Expression of Lef1, Lhx2, Lhx5, Lhx9, Lmo3, Lmo4, and Prox1 Helps to Identify Comparable Subdivisions in the Developing Hippocampal Formation of Mouse and Chicken. Front. Neuroanat. 8, 59. doi:10.3389/fnana.2014.00059
Anacker C., Hen R. (2017). Adult Hippocampal Neurogenesis and Cognitive Flexibility - Linking Memory and Mood. Nat. Rev. Neurosci. 18, 335–346. doi:10.1038/nrn.2017.45
Atoji Y., Sarkar S., Wild J. M. (2018). Differential Projections of the Densocellular and Intermediate Parts of the Hyperpallium in the pigeon (Columba livia). J. Comp. Neurol. 526, 146–165. doi:10.1002/cne.24328
Atoji Y., Sarkar S., Wild J. M. (2016). Proposed Homology of the Dorsomedial Subdivision and V-Shaped Layer of the Avian hippocampus to Ammon's Horn and Dentate Gyrus, Respectively. Hippocampus 26, 1608–1617. doi:10.1002/hipo.22660
Atoji Y., Wild J. M. (2006). Anatomy of the Avian Hippocampal Formation. Rev. Neurosciences 17, 3–15. doi:10.1515/revneuro.2006.17.1-2.3
Atoji Y., Wild J. M. (2004). Fiber Connections of the Hippocampal Formation and Septum and Subdivisions of the Hippocampal Formation in the pigeon as Revealed by Tract Tracing and Kainic Acid Lesions. J. Comp. Neurol. 475, 426–461. doi:10.1002/cne.20186
Atoji Y., Wild J. M. (2019). Projections of the Densocellular Part of the Hyperpallium in the Rostral Wulst of Pigeons (Columba livia). Brain Res. 1711, 130–139. doi:10.1016/j.brainres.2019.01.001
Bacqué-Cazenave J., Bharatiya R., Barrière G., Delbecque J. P., Bouguiyoud N., Di Giovanni G., et al. (2020). Serotonin in Animal Cognition and Behavior. Int. J. Mol. Sci. 21, 1649. doi:10.3390/ijms21051649
Bagnoli P., Burkhalter A. (1983). Organization of the Afferent Projections to the Wulst in the pigeon. J. Comp. Neurol. 214, 103–113. doi:10.1002/cne.902140111
Belgard T. G., Montiel J. F., Wang W. Z., García-Moreno F., Margulies E. H., Ponting C. P., et al. (2013). Adult Pallium Transcriptomes surprise in Not Reflecting Predicted Homologies across Diverse Chicken and Mouse Pallial Sectors. Proc. Natl. Acad. Sci. U.S.A. 110, 13150–13155. doi:10.1073/pnas.1307444110
Bingman V. P., Gagliardo A., Hough G. E., Ioale P., Kahn M. C., Siegel J. J. (2005). The Avian hippocampus, Homing in Pigeons and the Memory Representation of Large-Scale Space. Integr. Comp. Biol. 45, 555–564. doi:10.1093/icb/45.3.555
Bocchio M., Mchugh S. B., Bannerman D. M., Sharp T., Capogna M. (2016). Serotonin, Amygdala and Fear: Assembling the Puzzle. Front. Neural Circuits 10, 24. doi:10.3389/fncir.2016.00024
Bombardi C. (2014). Neuronal Localization of the 5-HT2 Receptor Family in the Amygdaloid Complex. Front. Pharmacol. 5, 68. doi:10.3389/fphar.2014.00068
Bonaventure P., Guo H., Tian B., Liu X., Bittner A., Roland B., et al. (2002). Nuclei and Subnuclei Gene Expression Profiling in Mammalian Brain. Brain Res. 943, 38–47. doi:10.1016/s0006-8993(02)02504-0
Bruinvels A. T., Landwehrmeyer B., Gustafson E. L., Durkin M. M., Mengod G., Branchek T. A., et al. (1994a). Localization of 5-HT1B, 5-HT1Dα, 5-HT1E and 5-HT1F Receptor Messenger RNA in Rodent and Primate Brain. Neuropharmacology 33, 367–386. doi:10.1016/0028-3908(94)90067-1
Bruinvels A. T., Landwehrmeyer B., Probst A., Palacios J., Hoyer D. (1994b). A Comparative Autoradiographic Study of 5-HT1D Binding Sites in Human and guinea-pig Brain Using Different Radioligands. Mol. Brain Res. 21, 19–29. doi:10.1016/0169-328x(94)90374-3
Colombo M., Broadbent N. (2000). Is the Avian hippocampus a Functional Homologue of the Mammalian hippocampus? Neurosci. Biobehavioral Rev. 24, 465–484. doi:10.1016/s0149-7634(00)00016-6
Corrales Parada C. D., Morandi-Raikova A., Rosa-Salva O., Mayer U. (2021). Neural Basis of Unfamiliar Conspecific Recognition in Domestic Chicks (Gallus Gallus domesticus). Behav. Brain Res. 397, 112927. doi:10.1016/j.bbr.2020.112927
D. Székely A. (1999). The Avian Hippocampal Formation: Subdivisions and Connectivity. Behav. Brain Res. 98, 219–225. doi:10.1016/s0166-4328(98)00087-4
Erlander M. G., Lovenberg T. W., Baron B. M., De Lecea L., Danielson P. E., Racke M., et al. (1993). Two Members of a Distinct Subfamily of 5-hydroxytryptamine Receptors Differentially Expressed in Rat Brain. Proc. Natl. Acad. Sci. U.S.A. 90, 3452–3456. doi:10.1073/pnas.90.8.3452
Faber H., Braun K., Zuschratter W., Scheich H. (1989). System-specific Distribution of Zinc in the Chick Brain. A Light- and Electron-Microscopic Study Using the Timm Method. Cell Tissue Res 258, 247–257. doi:10.1007/BF00239445
Fanselow M. S., Dong H.-W. (2010). Are the Dorsal and Ventral Hippocampus Functionally Distinct Structures? Neuron 65, 7–19. doi:10.1016/j.neuron.2009.11.031
Fernandez A. S., Pieau C., Reperant J., Boncinelli E., Wassef M. (1998). Expression of the Emx-1 and Dlx-1 Homeobox Genes Define Three Molecularly Distinct Domains in the Telencephalon of Mouse, Chick, Turtle and Frog Embryos: Implications for the Evolution of Telencephalic Subdivisions in Amniotes. Development 125, 2099–2111. doi:10.1242/dev.125.11.2099
Fonseca M. I., Ni Y. G., Dunning D. D., Miledi R. (2001). Distribution of Serotonin 2A, 2C and 3 Receptor mRNA in Spinal Cord and Medulla Oblongata. Mol. Brain Res. 89, 11–19. doi:10.1016/s0169-328x(01)00049-3
Fujita T., Aoki N., Fujita E., Matsushima T., Homma K. J., Yamaguchi S. (2019). The Chick Pallium Displays Divergent Expression Patterns of Chick Orthologues of Mammalian Neocortical Deep Layer-specific Genes. Sci. Rep. 9, 20400. doi:10.1038/s41598-019-56960-4
Fujita T., Aoki N., Mori C., Fujita E., Matsushima T., Homma K. J., et al. (2020). The Dorsal Arcopallium of Chicks Displays the Expression of Orthologs of Mammalian Fear Related Serotonin Receptor Subfamily Genes. Sci. Rep. 10, 21183. doi:10.1038/s41598-020-78247-9
Fujita T., Aoki N., Mori C., Fujita E., Matsushima T., Homma K. J., et al. (2022). Serotonergic Neurons in the Chick Brainstem Express Various Serotonin Receptor Subfamily Genes. Front. Physiol. 2548, 815997. doi:10.3389/fphys.2021.815997
Galceran J., Miyashita-Lin E. M., Devaney E., Rubenstein J. L., Grosschedl R. (2000). Hippocampus Development and Generation of Dentate Gyrus Granule Cells Is Regulated by LEF1. Development 127, 469–482. doi:10.1242/dev.127.3.469
Gupta S., Maurya R., Saxena M., Sen J. (2012). Defining Structural Homology between the Mammalian and Avian hippocampus through Conserved Gene Expression Patterns Observed in the Chick Embryo. Dev. Biol. 366, 125–141. doi:10.1016/j.ydbio.2012.03.027
Gustafson E. L., Durkin M. M., Bard J. A., Zgombick J., Branchek T. A. (1996). A Receptor Autoradiographic and In Situ Hybridization Analysis of the Distribution of the 5-ht7 Receptor in Rat Brain. Br. J. Pharmacol. 117, 657–666. doi:10.1111/j.1476-5381.1996.tb15241.x
Hanics J., Teleki G., Alpár A., Székely A. D., Csillag A. (2017). Multiple Amygdaloid Divisions of Arcopallium Send Convergent Projections to the Nucleus Accumbens and Neighboring Subpallial Amygdala Regions in the Domestic Chicken: a Selective Pathway Tracing and Reconstruction Study. Brain Struct. Funct. 222, 301–315. doi:10.1007/s00429-016-1219-8
Herold C., Bingman V. P., Ströckens F., Letzner S., Sauvage M., Palomero-Gallagher N., et al. (2014). Distribution of Neurotransmitter Receptors and Zinc in the Pigeon (Columba livia) Hippocampal Formation: A Basis for Further Comparison with the Mammalian Hippocampus. J. Comp. Neurol. 522, 2553–2575. doi:10.1002/cne.23549
Herold C., Coppola V. J., Bingman V. P. (2015). The Maturation of Research into the Avian Hippocampal Formation: Recent Discoveries from One of the Nature's Foremost Navigators. Hippocampus 25, 1193–1211. doi:10.1002/hipo.22463
Herold C., Schlömer P., Mafoppa-Fomat I., Mehlhorn J., Amunts K., Axer M. (2019). The hippocampus of Birds in a View of Evolutionary Connectomics. Cortex 118, 165–187. doi:10.1016/j.cortex.2018.09.025
Hevner R. F. (2016). Evolution of the Mammalian Dentate Gyrus. J. Comp. Neurol. 524, 578–594. doi:10.1002/cne.23851
Huang Y.-Y., Kandel E. R. (2007). 5-hydroxytryptamine Induces a Protein Kinase A/mitogen-activated Protein Kinase-Mediated and Macromolecular Synthesis-dependent Late Phase of Long-Term Potentiation in the Amygdala. J. Neurosci. 27, 3111–3119. doi:10.1523/jneurosci.3908-06.2007
Ikebuchi M., Hasegawa T., Bischof H.-J. (2009). Amygdala and Socio-Sexual Behavior in Male Zebra Finches. Brain Behav. Evol. 74, 250–257. doi:10.1159/000264660
International Chicken Genome Sequencing Consortium (2004). Sequence and Comparative Analysis of the Chicken Genome Provide Unique Perspectives on Vertebrate Evolution. Nature 432, 695–716. doi:10.1038/nature03154
Jarvis E. D., Yu J., Rivas M. V., Horita H., Feenders G., Whitney O., et al. (2013). Global View of the Functional Molecular Organization of the Avian Cerebrum: Mirror Images and Functional Columns. J. Comp. Neurol. 521, 3614–3665. doi:10.1002/cne.23404
Kandel E. R., Schwartz J. H., Jessell T. M., Siegelbaum S., Hudspeth A. J., Mack S. (2000). Principles of Neural Science. New York: McGraw-Hill.
Kempermann G. (2012). New Neurons for 'survival of the Fittest'. Nat. Rev. Neurosci. 13, 727–736. doi:10.1038/nrn3319
Kinsey A. M., Wainwright A., Heavens R., Sirinathsinghji D. J. S., Oliver K. R. (2001). Distribution of 5-HT5A, 5-HT5B, 5-HT6 and 5-HT7 Receptor mRNAs in the Rat Brain. Mol. Brain Res. 88, 194–198. doi:10.1016/s0169-328x(01)00034-1
Koyama Y., Kondo M., Shimada S. (2017). Building a 5-HT3A Receptor Expression Map in the Mouse Brain. Sci. Rep. 7, 42884. doi:10.1038/srep42884
Krause E. T., Kjaer J. B., Dudde A., Schrader L., Phi-Van L. (2019). Fear but Not Social Behaviour Is Affected by a Polymorphism in the 5'-flanking Region of the Serotonin Transporter (5-HTT) Gene in Adult Hens. Behav. Brain Res. 361, 50–53. doi:10.1016/j.bbr.2018.12.029
Krause E. T., Kjaer J. B., Lüders C., Van L. P. (2017). A Polymorphism in the 5′-flanking Region of the Serotonin Transporter (5-HTT) Gene Affects Fear-Related Behaviors of Adult Domestic Chickens. Behav. Brain Res. 330, 92–96. doi:10.1016/j.bbr.2017.04.051
Kuenzel W. J., Masson M. (1988). A Stereotaxic Atlas of the Brain of the Chick (Gallus domesticus). Baltimore: Johns Hopkins University Press.
Lowther S., Paermentier F. D., Crompton M. R., Horton R. W. (1992). The Distribution of 5-HT1D and 5-HT1E Binding Sites in Human Brain. Eur. J. Pharmacol. 222, 137–142. doi:10.1016/0014-2999(92)90473-h
Lucaites V. L., Krushinski J. H., Schaus J. M., Audia J. E., Nelson D. L. (2005). [3H]LY334370, a Novel Radioligand for the 5-HT1F Receptor. II. Autoradiographic Localization in Rat, guinea Pig, Monkey and Human Brain. Naunyn-schmiedeberg's Arch. Pharmacol. 371, 178–184. doi:10.1007/s00210-005-1036-8
Luo X., Muñoz-Pino E., Francavilla R., Vallée M., Droit A., Topolnik L. (2019). Transcriptomic Profile of the Subiculum-Projecting VIP GABAergic Neurons in the Mouse CA1 hippocampus. Brain Struct. Funct. 224, 2269–2280. doi:10.1007/s00429-019-01883-z
Marin P., Bécamel C., Chaumont-Dubel S., Vandermoere F., Bockaert J., Claeysen S. (2020). “Classification and Signaling Characteristics of 5-HT Receptors: toward the Concept of 5-HT Receptosomes,” in Handbook of Behavioral Neuroscience (Elsevier), 91–120. doi:10.1016/b978-0-444-64125-0.00005-0
Martín‐Cora F. J., Pazos A. (2004). Autoradiographic Distribution of 5‐HT7 Receptors in the Human Brain Using [3H] Mesulergine: Comparison to Other Mammalian Species. Br. J. Pharmacol. 141, 92–104. doi:10.1038/sj.bjp.0705576
Martínez-García F., Lanuza E. (2018). Evolution of Vertebrate Survival Circuits. Curr. Opin. Behav. Sci. 24, 113–123. doi:10.1016/j.cobeha.2018.06.012
Martınez-Garcıa F., Novejarque A., Lanuza E. (2009). 15 the Evolution of the Amygdala in Vertebrates. Evol. Neurosci. 313, 392. doi:10.1016/B0-12-370878-8/00139-7
Matsushima T., Izawa E.-I., Aoki N., Yanagihara S. (2003). The Mind through Chick Eyes : Memory, Cognition and Anticipation. Zoolog. Sci. 20, 395–408. doi:10.2108/zsj.20.395
Matthes H., Boschert U., Amlaiky N., Grailhe R., Plassat J. L., Muscatelli F., et al. (1993). Mouse 5-hydroxytryptamine5A and 5-hydroxytryptamine5B Receptors Define a New Family of Serotonin Receptors: Cloning, Functional Expression, and Chromosomal Localization. Mol. Pharmacol. 43, 313–319.
Mayer U., Rosa-Salva O., Loveland J. L., Vallortigara G. (2019). Selective Response of the Nucleus Taeniae of the Amygdala to a Naturalistic Social Stimulus in Visually Naive Domestic Chicks. Sci. Rep. 9, 9849. doi:10.1038/s41598-019-46322-5
Mayer U., Bhushan R., Vallortigara G., Lee S. A. (2018). Representation of Environmental Shape in the hippocampus of Domestic Chicks (Gallus gallus). Brain Struct. Funct. 223, 941–953. doi:10.1007/s00429-017-1537-5
Mayer U., Pecchia T., Bingman V. P., Flore M., Vallortigara G. (2016). Hippocampus and Medial Striatum Dissociation during Goal Navigation by Geometry or Features in the Domestic Chick: An Immediate Early Gene Study. Hippocampus 26, 27–40. doi:10.1002/hipo.22486
Mayer U., Rosa-Salva O., Vallortigara G. (2017). First Exposure to an Alive Conspecific Activates Septal and Amygdaloid Nuclei in Visually-Naïve Domestic Chicks (Gallus gallus). Behav. Brain Res. 317, 71–81. doi:10.1016/j.bbr.2016.09.031
Medina L., Abellán A., Desfilis E. (2017b). Contribution of Genoarchitecture to Understanding Hippocampal Evolution and Development. Brain Behav. Evol. 90, 25–40. doi:10.1159/000477558
Medina L., Abellán A., Vicario A., Castro-Robles B., Desfilis E. (2017a). “The Amygdala,” in Evolution of Nervous Systems (Second Edition). 1, 427–478. doi:10.1016/B978-0-12-804042-3.00019-1
Medina L., Reiner A. (2000). Do birds Possess Homologues of Mammalian Primary Visual, Somatosensory and Motor Cortices? Trends Neurosciences 23, 1–12. doi:10.1016/s0166-2236(99)01486-1
Mengod G., Vilaró M. T., Cortés R., López-Giménez J. F., Raurich A., Palacios J. M. (2006). “Chemical Neuroanatomy of 5-HT Receptor Subtypes in the Mammalian Brain,” in The serotonin receptors (Humana Press). Editors Roth B. L., 319–364. doi:10.1007/978-1-59745-080-5_10
Mengod G., Vilaró M. T., Raurich A., López-Giménez J. F., Cortés R., Palacios J. M. (1996). 5-HT Receptors in Mammalian Brain: Receptor Autoradiography Andin Situ Hybridization Studies of New Ligands and Newly Identified Receptors. Histochem. J. 28, 747–758. doi:10.1007/bf02272148
Miceli D., Repérant J. (1985). Telencephalic Afferent Projections from the Diencephalon and Brainstem in the pigeon. A Retrograde Multiple-Label Fluorescent Study. Exp. Biol. 44, 71–99.
Miceli D., Marchand L., Repérant J., Rio J.-P. (1990). Projections of the Dorsolateral Anterior Complex and Adjacent Thalamic Nuclei upon the Visual Wulst in the pigeon. Brain Res. 518, 317–323. doi:10.1016/0006-8993(90)90990-s
Montagnese C. M., Geneser F. A., Krebs J. R. (1993). Histochemical Distribution of Zinc in the Brain of the Zebra Finch (Taenopygia Guttata). Anat. Embryol. (Berl) 188, 173–187. doi:10.1007/BF00186251
Montagnese C. M., Krebs J. R., Meyer G. (1996). The Dorsomedial and Dorsolateral Forebrain of the Zebra Finch, Taeniopygia guttata: ;A Golgi Study. Cel Tissue Res. 283, 263–282. doi:10.1007/s004410050537
Morales M., Wang S.-D. (2002). Differential Composition of 5-Hydroxytryptamine3Receptors Synthesized in the Rat CNS and Peripheral Nervous System. J. Neurosci. 22, 6732–6741. doi:10.1523/jneurosci.22-15-06732.2002
Morandi-Raikova A., Mayer U. (2020). The Effect of Monocular Occlusion on Hippocampal C-Fos Expression in Domestic Chicks (Gallus gallus). Sci. Rep. 10, 7205. doi:10.1038/s41598-020-64224-9
O'leary O. F., Codagnone M. G., Cryan J. F. (2020). “Revisiting the Behavioral Genetics of Serotonin: Relevance to Anxiety and Depression,” in Handbook of Behavioral Neuroscience (Elsevier), 665–709. doi:10.1016/b978-0-444-64125-0.00038-4
Papini M. R., Penagos-Corzo J. C., Pérez-Acosta A. M. (2019). Avian Emotions: Comparative Perspectives on Fear and Frustration. Front. Psychol. 9, 2707. doi:10.3389/fpsyg.2018.02707
Pascual J., Del Arco C., Romón T., Del Olmo E., Pazos A. (1996). [3H]Sumatriptan Binding Sites in Human Brain: Regional-dependent Labelling of 5-HT1D and 5-HT1F Receptors. Eur. J. Pharmacol. 295, 271–274. doi:10.1016/0014-2999(95)00748-2
Payne H. L., Lynch G. F., Aronov D. (2021). Neural Representations of Space in the hippocampus of a Food-Caching Bird. Science 373, 343–348. doi:10.1126/science.abg2009
Phi Van V. D., Krause E. T., Phi-Van L. (2018). Modulation of Fear and Arousal Behavior by Serotonin Transporter (5-HTT) Genotypes in Newly Hatched Chickens. Front. Behav. Neurosci. 12, 284. doi:10.3389/fnbeh.2018.00284
Plassat J. L., Boschert U., Amlaiky N., Hen R. (1992). The Mouse 5HT5 Receptor Reveals a Remarkable Heterogeneity within the 5HT1D Receptor Family. EMBO J. 11, 4779–4786. doi:10.1002/j.1460-2075.1992.tb05583.x
Puelles L., Kuwana E., Puelles E., Bulfone A., Shimamura K., Keleher J., et al. (2000). Pallial and Subpallial Derivatives in the Embryonic Chick and Mouse Telencephalon, Traced by the Expression of the Genes Dlx-2, Emx-1, Nkx-2.1, Pax-6, and Tbr-1. J. Comp. Neurol. 424, 409–438. doi:10.1002/1096-9861(20000828)424:3<409::aid-cne3>3.0.co;2-7
Puelles L., Martinez-de-la-Torre M., Watson C., Martinez S., Paxinos G. (2007). The Chick Brain in Stereotaxic Coordinates and Alternate Stains. San Diego: Academic Press, Elsevier.
Redies C., Medina L., Puelles L. (2001). Cadherin Expression by Embryonic Divisions and Derived gray Matter Structures in the Telencephalon of the Chicken. J. Comp. Neurol. 438, 253–285. doi:10.1002/cne.1315
Reiner A., Perkel D. J., Bruce L. L., Butler A. B., Csillag A., Kuenzel W., et al. (2004). Revised Nomenclature for Avian Telencephalon and Some Related Brainstem Nuclei. J. Comp. Neurol. 473, 377–414. doi:10.1002/cne.20118
Rosa Salva O., Mayer U., Vallortigara G. (2015). Roots of a Social Brain: Developmental Models of Emerging Animacy-Detection Mechanisms. Neurosci. Biobehavioral Rev. 50, 150–168. doi:10.1016/j.neubiorev.2014.12.015
Sherry D. F., Grella S. L., Guigueno M. F., White D. J., Marrone D. F. (2017). Are There Place Cells in the Avian Hippocampus? Brain Behav. Evol. 90, 73–80. doi:10.1159/000477085
Sloviter R. S., Lømo T. (2012). Updating the Lamellar Hypothesis of Hippocampal Organization. Front. Neural Circuits 6, 102. doi:10.3389/fncir.2012.00102
Smulders T. V. (2021). Telencephalic Regulation of the HPA axis in Birds. Neurobiol. Stress 15, 100351. doi:10.1016/j.ynstr.2021.100351
Smulders T. V. (2017). The Avian Hippocampal Formation and the Stress Response. Brain Behav. Evol. 90, 81–91. doi:10.1159/000477654
Strac D. S., Pivac N., Muck-Seler D. (2016). The Serotonergic System and Cognitive Function. Translational Neurosci. 7, 35–49. doi:10.1515/tnsci-2016-0007
Striedter G. F. (2016). Evolution of the Hippocampus in Reptiles and Birds. J. Comp. Neurol. 524, 496–517. doi:10.1002/cne.23803
Suárez J., Dávila J. C., Real M. á., Guirado S., Medina L. (2006). Calcium-binding Proteins, Neuronal Nitric Oxide Synthase, and GABA Help to Distinguish Different Pallial Areas in the Developing and Adult Chicken. I. Hippocampal Formation and Hyperpallium. J. Comp. Neurol. 497, 751–771. doi:10.1002/cne.21004
Tanaka K. F., Samuels B. A., Hen R. (2012). Serotonin Receptor Expression along the Dorsal-Ventral axis of Mouse hippocampus. Phil. Trans. R. Soc. B 367, 2395–2401. doi:10.1098/rstb.2012.0038
Tecott L. H., Maricq A. V., Julius D. (1993). Nervous System Distribution of the Serotonin 5-HT3 Receptor mRNA. Proc. Natl. Acad. Sci. U.S.A. 90, 1430–1434. doi:10.1073/pnas.90.4.1430
Tömböl T., Davies D. C., Németh A., Sebestény T., Alpár A. (2000). A Comparative Golgi Study of Chicken (Gallus domesticus) and Homing pigeon (Columba livia) hippocampus. Anat. Embryol. 201, 85–101. doi:10.1007/pl00008235
Tosches M. A., Yamawaki T. M., Naumann R. K., Jacobi A. A., Tushev G., Laurent G. (2018). Evolution of Pallium, hippocampus, and Cortical Cell Types Revealed by Single-Cell Transcriptomics in Reptiles. Science 360, 881–888. doi:10.1126/science.aar4237
Vilaró M., Cortés R., Mengod G., Hoyer D. (2020). “Distribution of 5-HT Receptors in the central Nervous System: an Update,” in Handbook of Behavioral Neuroscience (Elsevier), 121–146.
Waeber C., Moskowitz M. A. (1995). [3H]sumatriptan Labels Both 5-HT1D and 5-HT1F Receptor Binding Sites in the guinea Pig Brain: an Autoradiographic Study. Naunyn Schmiedebergs Arch. Pharmacol. 352, 263–275. doi:10.1007/BF00168556
Yamaguchi S., Fujii-Taira I., Katagiri S., Izawa E.-I., Fujimoto Y., Takeuchi H., et al. (2008a). Gene Expression Profile in Cerebrum in the Filial Imprinting of Domestic Chicks (Gallus gallus Domesticus). Brain Res. Bull. 76, 275–281. doi:10.1016/j.brainresbull.2008.02.002
Yamaguchi S., Fujii-Taira I., Murakami A., Hirose N., Aoki N., Izawa E.-I., et al. (2008b). Up-regulation of Microtubule-Associated Protein 2 Accompanying the Filial Imprinting of Domestic Chicks (Gallus gallus Domesticus). Brain Res. Bull. 76, 282–288. doi:10.1016/j.brainresbull.2008.02.010
Yamamoto K., Reiner A. (2005). Distribution of the Limbic System-Associated Membrane Protein (LAMP) in pigeon Forebrain and Midbrain. J. Comp. Neurol. 486, 221–242. doi:10.1002/cne.20562
Yamamoto K., Sun Z., Wang H. B., Reiner A. (2005). Subpallial Amygdala and Nucleus Taeniae in Birds Resemble Extended Amygdala and Medial Amygdala in Mammals in Their Expression of Markers of Regional Identity. Brain Res. Bull. 66, 341–347. doi:10.1016/j.brainresbull.2005.02.016
Keywords: chick, hippocampal formation, serotonin receptor, subdivision, layer
Citation: Fujita T, Aoki N, Mori C, Fujita E, Matsushima T, Homma KJ and Yamaguchi S (2022) Chick Hippocampal Formation Displays Subdivision- and Layer-Selective Expression Patterns of Serotonin Receptor Subfamily Genes. Front. Physiol. 13:882633. doi: 10.3389/fphys.2022.882633
Received: 24 February 2022; Accepted: 24 March 2022;
Published: 08 April 2022.
Edited by:
Krystyna Pierzchala-Koziec, University of Agriculture in Krakow, PolandReviewed by:
Matthew J. Robson, University of Cincinnati, United StatesUwe Mayer, University of Trento, Italy
Copyright © 2022 Fujita, Aoki, Mori, Fujita, Matsushima, Homma and Yamaguchi. This is an open-access article distributed under the terms of the Creative Commons Attribution License (CC BY). The use, distribution or reproduction in other forums is permitted, provided the original author(s) and the copyright owner(s) are credited and that the original publication in this journal is cited, in accordance with accepted academic practice. No use, distribution or reproduction is permitted which does not comply with these terms.
*Correspondence: Shinji Yamaguchi, c2hpbmppLXlAcGhhcm0udGVpa3lvLXUuYWMuanA=