- 1College of Plant Protection, Gansu Agricultural University/Biocontrol Engineering Laboratory of Crop Diseases and Pests of Gansu Province, Lanzhou, China
- 2CSIR—Savanna Agricultural Research Institute, Tamale, Ghana
- 3Forestry and Grassland Bureau of Lintao County, Dingxi, China
Botanical pesticide is highly recommended for integrated pest management (IPM), due to its merits such as environmental friendliness, safe to non-target organisms, operators, animals, and food consumers. The experiment was conducted to determine the lethal and sub-lethal effects of allyl isothiocyanate (AITC) on eggs, third instar larvae, pupae, and females and males of Bradysia impatiens Johannsen (B. impatiens). Different concentrations of AITC under ambient CO2 by the conical flask sealed fumigation method were used for the experiment. The results showed that there was a significant linear relationship between different concentrations of AITC and the toxicity regression equation of B. impatiens. The sub-lethal concentrations of AITC had significant effects on the larval stage, pupal stage, pupation rate, pupal weight, adult emergence rate, and oviposition. The pupation rate, pupal weight, and adult emergency rate were significantly (p < 0.05) affected by AITC fumigation. The pupation rate was the lowest after fumigation treatment of AITC at LC50 (36.67%), followed by LC25 (41.94%), compared with the CK (81.39%). Female longevity was significantly (p < 0.05) shortened by fumigation at LC25 (1.75 d) and LC50 (1.64 d), compared with that of CK (2.94 d). Male longevity was shorter at LC25 (1.56 d) than at LC50 (1.25 d) and had no significant difference between these two treatments. The fumigation efficiency of AITC was significantly increased under high CO2 condition. Furthermore, detoxification enzyme activities and antioxidant enzyme activities were accumulated under high CO2 condition. The fumigation method in the application of AITC can be useful in areas where B. impatiens is a major concern.
1 Introduction
Bradysia impatiens Johannsen is an economic important pest globally, which survives on mushrooms, chives, ornamental plants, and humus (Johannsen, 1912; Menzel et al., 2003; Santos et al., 2012; Gou et al., 2019). The larvae feed on the roots, stems, leaves, flowers, and even the whole seedling of host plants (Gou et al., 2020a; Gou et al., 2020b). B. impatiens was first reported on mushrooms in China (Shen et al., 2009). Other host plants of this pest include chive (Allium tuberosum) (Gou et al., 2015), onion (A. fistulosum) (Gou et al., 2015), lily (Lilium brownie) (Gou et al., 2015), carrot (Daucus carota) (Arimoto et al., 2018), poinsettia (Euphorbia pulcherrima) (Cheng et al., 2018), butterfly orchid (Phalaenopsis aphrodite) (Cheng et al., 2018), strawberry (Fragaria ananassa) (Sueyoshi and Yoshimatsu, 2019), and eucalyptus (Eucalyptus robusta). B. impatiens is an agricultural and forestry pest distributed in areas such as the United States, South Africa, Japan, the Netherlands, Brazil, Hawaiian Islands, the United Kingdom, Russia and other countries (Menzel et al., 2003; Gou et al., 2020b).
Botanical pesticide is highly recommended for integrated pest management (IPM) due to its merits of environmental friendliness, safe to non-target organisms, operators, and animal and food consumers. The development of the botanical control method has been proposed as one of the most important strategies for pest management on fruits, vegetables, and ornamental plants (Zhang et al., 2015; Cheng et al., 2018; Lu et al., 2020). AITC, commonly known as horseradish, is a volatile and aliphatic sulfur-containing compound naturally occurring in plants from the family of Cruciferae, such as: horseradish (Armoracia rusticana), mustard (Brassica nigra), cabbage (Brassica oleracea), and wasabi (Wasabia japonica) (Mayton et al., 1996; Wu et al., 2009; Williams et al., 2015; Li Y et al., 2018). AITC could be rapidly adsorbed and degraded in soil with a low risk of persistence (Ren et al., 2018); thus, it has been considered a potential botanical pesticide. It has been effectively used to control soil-borne fungi (Yim et al., 2016), plant pathogen (Smolinska et al., 2003; Troncoso et al., 2005; Ugolini et al., 2014), nematodes (Brolsma et al., 2014), weeds (Bangarwa and Norsworthy, 2014; Devkota and Norsworthy, 2014; Matteo et al., 2017), and pests (Wu H. et al., 2014; Deguenon et al., 2019; Zhang et al., 2020). Some storage pest species, including Sitophilus oryzae (Worfel et al., 1997), Sitophilus zeamais (Mansour et al., 2012), Callosobruchus maculatus (Zhang et al., 2016), Tribolium confusum (Li et al., 2011), and Plodia interpunctella (Liang et al., 2013), are particularly sensitive to AITC and have achieved remarkable fumigation effect. AITC also has good efficacy against adults of B. odoriphaga (Shi et al., 2017).
An increase in atmospheric carbon dioxide (CO2) concentration is predicted to continually increase from the current 400 ppm to between 750 and 1,300 ppm by the end of this century (Erda et al., 2005; Li et al., 2020). CO2 is an important regulator of respiration, and the spiracle of insects are kept open permanently under high concentrations of CO2 (higher than 10–20%) (Miller, 1974). Accordingly, they are likely to absorb more fumigants when their spiracle is open permanently. A mixture of fumigants and high concentrations of CO2 enhances toxicity to insects (Miller, 1974; Haritos et al., 2006). Several positive effects on various fumigants have been investigated, including methyl bromide, ethanedinitrile (Ramadan et al., 2021), ethyl formate (Haritos et al., 2006; Damcevski et al., 2010), and the essential oils from Perilla frutescens (L.) Britt. (Lamiaceae) (Ye et al., 2015), showing that the control efficacy against the targeted pests had been apparently strengthened under high concentrations of CO2. In general, there is a balance between the generation of reactive oxygen species (ROS) and their scavenging. However, when exposed to environmental stress, the balance is disrupted. Insecticidal stress causes an increase in the production of ROS, which causes oxidative damage (Lalouette et al., 2011). Excess ROS causes lipid peroxidation (LPO) and disrupts cell membrane fluidity, leading to cell lesions. The degree of membrane LPO can be determined indirectly by measuring the concentration of malondialdehyde (MDA) (Meng et al., 2009). Organisms have evolved complex adaptation-related mechanisms for eliminating ROS, such as molecular antioxidants and antioxidative enzymes (Joanisse and Storey, 1998), to maintain homeostasis and prevent ROS damage. Superoxide dismutase (SOD), catalase (CAT), peroxidase (POD), and glutathione-S-transferases (GSTs) are the most important components for protecting cells and maintaining homeostasis in various stress conditions by scavenging ROS (Felton and Summers, 1995). Numerous studies have used antioxidant responses to thermal stress as indicators of important physiological adaptation processes in insects (Felton and Summers, 1995). Detoxification enzymes in the insect body such as the GSTs, CarE, and cytochrome-b5 (Cyt-b5) are other important factors (Li et al., 2021; Liang et al., 2017) that decrease the effectiveness of insecticides by changing the metabolism (Xiao et al., 2009; Su et al., 2012).
Bradysia impatiens is considered an economic important pest due to its ability to inhibit the production of a wide range of agricultural crops. Consequently, wide ranges of synthetic insecticides are continuously used for its management in China. This type of strategy has been found to seriously increase environmental contamination and insecticide resistance and endangers the health of farm operators, animals, and food consumers. Botanical pesticides are considered safe in pest control because they have low or no toxic residue, making them safe to people and the environment. Thus, developing botanical control methods for the management of B. impatiens is paramount for crop production. This research is based on the hypothesis that the application of AITC and/or CO2 combination affects the performance of B. impatiens. The study is, therefore, conducted to determine the lethal and sub-lethal effects of allyl isothiocyanate (AITC) on eggs, third instar larvae, pupae, and females and males of B. impatiens Johannsen (B. impatiens).
2 Materials and Methods
2.1 Experimental Plant
The chive cultivar “pingjiu No. 2,” which is susceptible to B. impatiens (Gou et al., 2015), was planted in the experimental field of Gansu Agricultural University for 3 years. All necessary agronomic practices such as watering, stubble cutting, and farm manure application were carried out regularly, without chemical spraying.
2.2 Tested Insect
Larvae of B. impatiens were collected from chive plants in a greenhouse of the Pan’an town (34° 45′22′ N, 105° 7′2 ′E), Gansu County, Tianshui, China, in April 2019. The individuals of the laboratory population were reared on a self-developed artificial diet (Gou et al., 2020c). The eggs were collected and placed in transparent plastic rearing containers (upper diameter × lower diameter × height = 12 cm × 8 cm × 8 cm). The larvae were fed on chive rhizomes for three constant generations in a light growth chamber under (25 ± 1)°C temperature, 65–70% relative humidity (RH), and 16 L h: 8D h photoperiod. The eggs, third larvae, pupae, and newly emerged females and males were randomly selected for the analyses.
2.3. Chemicals
Technical grade AITC (active ingredient >98%) was purchased from Sigma, the United States of America GSTs, CarE, Cyt-b5, SOD, CAT, and POD assay kits were purchased from Shanghai Preferred Biotechnology, China.
2.4 Toxicity Process
2.4.1 Preparation of Allyl Isothiocyanate Solution
To achieve the mother liquor containing 9.5% AITC, technical grade AITC was dissolved in soybean oil with a volume ratio of 1: 9 V/V (Santos et al., 2011; Paes et al., 2012) and stored at 4°C in dark (maintain chemical activity). Soybean oil did not affect the toxicity tests on B. impatiens that was used as the solvent to minimize the volatilization rate of AITC, particularly when used at low quantities (Paes et al., 2012). Thus, preliminary experiments were conducted, and final concentrations of AITC including 0.0, 3.0, 6.0, 9.0, 12.0, and 15.0 μL/L were tested.
2.4.2 Fumigation Test
The experiment was carried out in the fume hood of the laboratory under ambient CO2 by the conical flask sealed fumigation method (Ren et al., 2018). A count of 100 eggs (1 day old), third larvae, pupae (1 day old), and unmated females and males were collected each and then placed in corresponding flasks (500 ml). Each flask contained fresh chive rhizomes (2-cm length) and had wet absorbent cotton with two filter papers (9-cm diameter) at the bottom. Aliquots of the AITC test solution (30 μL) were applied to the filter paper strips (1 cm × 6 cm) plugged at the top of the flask and then sealed with plug and parafilm. The untreated control flask had only soybean oil (30 μL). Three replicates were set up for each treatment. All flasks were incubated 24 h in the light growth chamber at (25 ± 1)°C, 65%–70% RH, and 16: 8 (L: D). The adults and larvae were considered dead with no observable motion when gently touched with a soft brush. The pupae were recorded for 5 d to observe the adult emergence number. The egg hatching numbers were recorded for 7d.
2.5 Effects of Sub-lethal Concentrations of Allyl Isothiocyanate on Growth Parameters of B. Impatiens
The AITC sub-lethal concentrations (LC25 and LC50) against third larvae obtained by fumigation were used to determine the growth parameters of B. impatiens. The solvent soybean oil without AITC was used as the control. Each treatment contained 100 third larvae with three replications. After 24 h treatment, 60 surviving larvae were transferred into three petri dishes (12-cm, 20 individuals in one dish) containing moistened filter papers and maintained in a growth chamber at 25°C, 65%–70% RH, and 16L: 8D. Water was added along the edge of the filter paper every day to maintain appropriate humidity and timely supplemented with fresh chive rhizomes without chemicals. The survivals and instars were recorded. The pupae were moved to petri dishes (35-mm, containing moistened filter paper) one by one and weighed within 48 h. We weighed the pupae with a single head using a millionth scale. Twenty heads were collected for each treatment and repeated three times. Meanwhile, the survival of pupae was monitored daily. Once an adult emerged, unmated males and females (1:1) were transferred into transparent plastic containers (as described earlier). Each container contained absorbent cottons and filter papers; the male and female longevity and the number of eggs laid by each female were recorded daily. Ten pairs were considered a group, one group was considered a replicate, and three replicates were used for the female oviposition and adult longevity monitoring.
The pupation rate (%) was calculated using the formula [(total number of collected pupae/total number of fourth instar larvae) × 100]. The emergence rate (%) was calculated using the formula [(total number of emerged adults/total number of collected pupae) × 100].
2.6 High CO2 and/or LC25 Allyl Isothiocyanate Fumigation Stress
To assess the effect of elevated CO2 on AITC fumigation efficacy against B. impatiens larvae, 100 heads third larvae of B. impatiens were placed in one conical flask (500 ml) with three replicates. The experiment was carried out with LC25 concentration of AITC as described in the previous paragraph. In addition to the AITC fumigation + high CO2 (AF + HC, under high CO2 concentration about 800 ppm with AF stress), the flasks containing the larvae were connected to a high-pressure CO2 cylinder via plastic tubing. CO2 was delivered to the flasks for 10 s at 50 kPa (about 800 ppm) (Wang et al., 2018). The flasks were immediately tightly sealed with plug and parafilm. Larvae without any treatment (CK, under ambient CO2 concentration about 400 ppm without AF stress), larvae treated only with AITC fumigation (AF, under ambient CO2 concentration about 400 ppm with AF stress), and larvae treated with only high CO2 (HC, under high CO2 concentration about 800 ppm without AF stress) were all placed at the same time. At 24, 48, and 72 h after treatment, the larvae were recovered under ambient air for 2 h and mortality was examined. The larvae were considered dead if they were immobile after being gently stimulated by a soft brush.
2.7 Enzyme Activity Assay
2.7.1 Preparation of Enzyme Sources and Determination and Calculation of Enzyme Activity
Third instar larvae of B. impatiens were collected from four treatments (CK, HC, AF, and AF + HC), washed with phosphate buffer solution (PBS, pH 7.0) containing 1.0 mmol/L ethylene diamide tetra acetic acid (EDTA), and then kept in a 1.5-ml centrifuge tube. Each tube contained 20 heads (about 0.05 g) with three replications and marked. Each sample was frozen in liquid nitrogen for 15 min and then homogenized in ice in 1.5 ml of 0.1 M PBS with a high-speed tissue grinder (TIANGEN). The supernatants were collected after the centrifugation of homogenates at 12,000 × g for 15 min at 4°C. The resultant supernatant was stored at −80°C and used as an enzyme source. We detected three detoxification enzyme activities, such as glutathione-S-transferase (GSTs), carboxylesterase (CarE), and cytochrome-b5 (Cyt-b5); three antioxidant enzyme activities, such as superoxide dismutase (SOD), catalase (CAT), and peroxidase (POD). The methods of determination and calculation for each enzyme were strictly referred to the instructions of the kit (Shanghai Preferred Biotechnology, China).
2.8 Statistical Analysis
The experimental data were statistically analyzed using SPSS23.0 software. The toxicity regression equation and the values of LC25 and LC50 were calculated by the Probit module (probability unit regression) (Wu H. H. et al., 2014). Differences among the treatments were subjected to Tukey’s test (p < 0.05). GraphPad Prism 6.0 software was used for plotting graphs.
3 Results
3.1 Toxicity of Allyl Isothiocyanate to B. Impatiens
The bioassay result indicated that AITC showed significant (p < 0.05) insecticidal effect on B. impatiens. After fumigation in the conical flask for 24 h, the values of LC50 against the female and male adults were 4.79 and 5.47 μL/L, respectively, which were significantly lower than those of the eggs (10.23 μL/L, χ2 = 1.30, p = 0.71), pupae (10.40 μL/L, χ2 = 1.92, p = 0.58), and third larvae (11.27 μL/L, χ2 = 1.55, p = 3.38) (Table 1).
3.2 Survival Rate
The bioassay results showed that increasing concentrations of AITC significantly (p < 0.05) decreased the survival rate of B. impatiens each stage (Figure 1), that is, significantly increased their mortality rates. Fumigation results showed that AITC had suppressive effects on each stage of B. impatien. For example, at 6 μL/L concentration of AITC, the survival rate of pupae (69%) was the least affected by AITC, with the highest survival rate and the strongest resistance, followed by eggs (60%) and larvae (58%). A concentration of 15 μL/L AITC was fatal to female and male adults, which caused 100% mortality.
3.3 Larval Stage and Pupal Stage
The developmental stage of B. impatiens was significantly (p < 0.05) affected by LC25 and LC50 fumigation treatment (Figure 2). The third larval stage was significantly (p < 0.05) prolonged after LC25 and LC50 of AITC stress; however, no obvious changes between those two sub-lethal concentrations were observed. AITC stress of LC25 prolonged third larval stage of 0.77 d, compared with LC50. After AITC treatment, LC50 prolonged the fourth larval stage by 0.05 d than LC25, compared with CK (3.13 d). The fumigation treatment prolonged the pupal stage of B. impatiens by 0.35 d at LC25 and 0.95 d at LC50. However, there was no significant difference between CK and LC25.
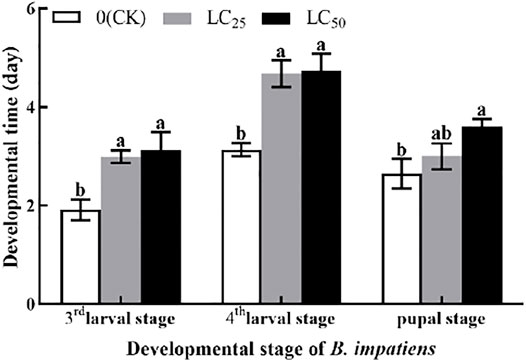
FIGURE 2. Changes in the third larval stage, fourth larval stage, and pupal stage of B. impatiens after sub-lethal concentration fumigation of AITC. Data represent mean ± SD of three replicates. Different lower-case letters indicate statistically significant difference between sub-lethal concentrations by Tukey’s test (p < 0.05).
3.4 Pupation Rate, Pupal Weight, and Adult Emergency Rate
The pupation rate, pupal weight, and adult emergency rate were significantly (p < 0.05) affected by AITC fumigation. The pupation rate was the lowest after fumigation treatment of AITC at LC50 (36.67%), followed by LC25 (41.94%), compared with the CK (81.39%) (Figure 3A). Pupal weight was reduced by 0.10 mg at LC25 and by 0.22 at LC50; however, there was no significant difference between the two sub-lethal concentrations (Figure 3B). The adult emergency rate in CK was significantly higher (65%; p < 0.05) than that of LC25 (50.17%) and LC50 (40%) treatments (Figure 3C).
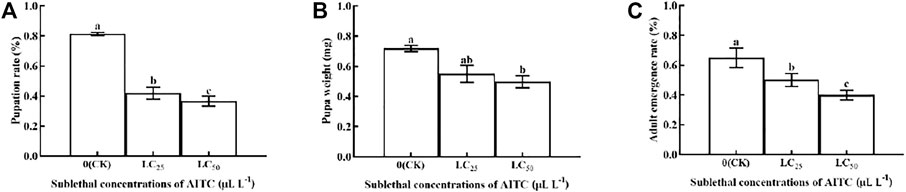
FIGURE 3. Changes in the pupation rate (A), pupa weight (B), and adult emergence rate (C) of B. impatiens after sub-lethal concentration fumigation of AITC. Data represent mean ± SD of three replicates. Different lower-case letters indicate statistically significant difference between sub-lethal concentrations by Tukey’s test (p < 0.05).
3.5 Adult Longevity and Oviposition
Female longevity was significantly (p < 0.05) shortened by fumigation at LC25 (1.75 d) and LC50 (1.64 d), compared with that in CK (2.94 d; Figure 4A). Male longevity was shorter at LC25 (1.56 d) than at LC50 (1.25 d) and had no significant difference between the two treatments (Figure 4B). Oviposition was significantly (p < 0.05) decreased at LC25 (22 eggs) and at LC50 (0 eggs) compared to the CK (75.10 eggs; Figure 4C). Obviously, AITC fumigation treatment was significantly inhibited by the oviposition of B. impatiens.
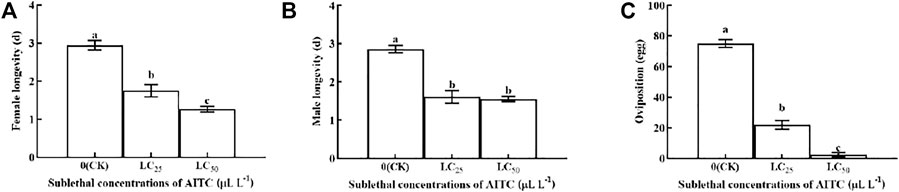
FIGURE 4. Changes in female longevity (A), male lingevity (B), and adult oviposition (C) of B. impatiens after sub-lethal concentration fumigation of AITC. Data represent mean ± SD of three replicates. Different lower-case letters indicate statistically significant difference between sub-lethal concentrations by Tukey’s test (p < 0.05).
3.6 Allyl Isothiocyanate Fumigation Efficiency Under High CO2
To determine the effect of high CO2 on AITC fumigation efficiency against B. impatiens, we incorporated high CO2 exposure of the third larvae during AITC LC25 treatment (Figure 5). Notably, high CO2 and/or AITC treatment apparently enhanced the mortality of B. impatiens (p < 0.05) with prolonged time. The larvae subjected to the AITC treatment alone displayed 28.67, 39, and 83.67% mortality at 24, 48, and 72 h time points, respectively. Exposure to high concentration of CO2 during AITC treatment substantially increased the mortality to 48, 59.33, and 99%.
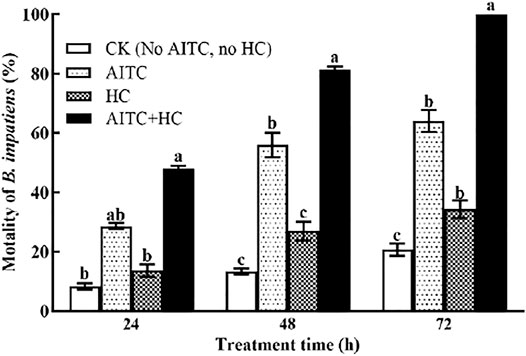
FIGURE 5. Changes in mortality of B. impatiens in three treatment hours under ambient CO2 or/and high CO2 conditions with and without AITC fumigation. CK: no AITC fumigation under ambient CO2 condition; AF: AITC fumigation; HC: high CO2 stress; AF + HC: AITC fumigation under high CO2 condition. Data represent mean ± SD of three replicates. Different lower-case letters indicate statistically significant difference between sub-lethal concentrations by Tukey’s test (p < 0.05).
3.7 Detoxification Enzyme Activity
Generally, AITC fumigation treatments had a significant (p < 0.05) effect on the detoxification enzyme activities. We measured GST, CraE, and Cyt-b5 activities in third larvae of B. impatiens after high CO2 and/or AITC fumigation for 24 h followed by recovery of normal environment for 2 h (Figure 6). GST activity was upregulated by either the AITC treatment alone or the combined treatment of AITC with high CO2, and the latter induced a higher response (Figure 6A). Similarly, CarE activity was also increased by these treatments (Figure 6B). Substantially higher CarE activity was obtained after the combined treatment than after individual treatment in third larvae. Enhanced Cyt-b5 activity was also detected under various treatments compared to that in the untreated group (Figure 6C), and high CO2 apparently stimulated these responses than being AITC used alone. Elevated detoxification enzymes may reduce the damage caused by AITC.
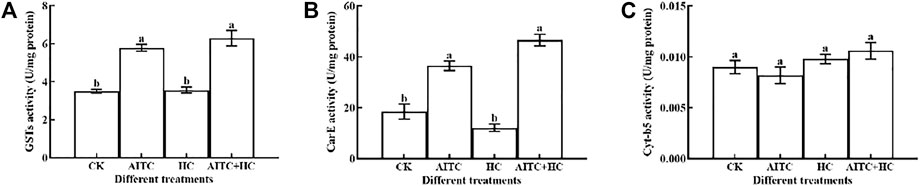
FIGURE 6. Changes in GST enzyme activity (A), CarE enzyme activity (B), and Cyt-b5 enzyme (C) activity of B. impatiens after AITC fumigation treatment under ambient CO2 or high CO2 conditions with and without AITC fumigation. CK: no AITC fumigation under ambient CO2 condition; AF: AITC fumigation; HC: high CO2 stress; AF + HC: AITC fumigation under high CO2 condition. Data represent mean ± SD of three replicates. Different lower-case letters indicate statistically significant difference between sub-lethal concentrations by Tukey’s test (p < 0.05).
3.8 Antioxidant Enzyme Activity
High concentration of CO2 often corresponds to low concentration of O2. This environment will stimulate the ROS of organisms and produce oxygen oxidative damage. After being fumigated by AITC and recovered indoors for 2 h, the activity of antioxidant enzymes in vivo was detected. The results showed that the activities of CAT and POD were inhibited, while the activities of SOD were significantly induced (p < 0.05; Figure 7). Under high CO2 concentration condition, the activities of SOD, CAT, and POD were significantly increased (p < 0.05). The activity of SOD significantly stimulated when treated by AITC under high CO2 compared with that of CK; however, the degree of activation was weaker than that of the two separate treatments (Figure 7A).
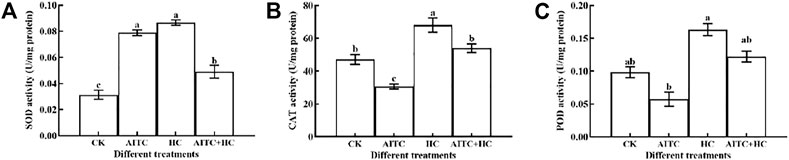
FIGURE 7. Changes in SOD enzyme activity (A), CAT enzyme activity (B), and POD enzyme activity (C) of B. impatiens after AITC fumigation treatment under ambient CO2 or high CO2 conditions with and without AITC fumigation. CK: no AITC fumigation under ambient CO2 condition; AF: AITC fumigation; HC: high CO2 stress; AF + HC: AITC fumigation under high CO2 condition. Data represent mean ± SD of three replicates. Different lower-case letters indicate statistically significant difference between sub-lethal concentrations by Tukey’s test (p < 0.05).
4 Discussion
Plant extracts are considered for pest management because they can be selective, biodegraded to non-toxic products, and have low toxicity to non-target organisms and environment (Ren et al., 2018). Allyl isothiocyanate (AITC) is a natural product formed from allyl glucosinolate hydrolysis and obtained from the damaged tissues of cruciferous plants (Clarke, 2010; Williams et al., 2015). Shi et al. (2017) reported that AITC has high fumigation bioactivity against eggs, larvae, pupae, and adults of B. odoriphaga (Shi et al., 2017). The application of AITC inhibits S. zeamai performance, particularly at the adult stage (Wu H. et al., 2014). In the present study, the AITC fumigation test showed greater lethal effects on female and male adults of B. impatiens than those on eggs, third larvae, and pupae. This is probably because the AITC fumigation contains high toxicity that might have interfered with their respiratory mechanism. We, therefore, speculate that AITC fumigation is more lethal to the adult B. impatiens and sub-lethal to its eggs, third larvae, and pupae stages. Consequently, the life cycle of B. impatiens should be considered when developing a pesticide application strategy, as its eggs, third larvae, and pupae stages are spent in the growing medium than on the host plant.
In indoor or outdoor field environment, insecticides produce lethal and sub-lethal effects on pests (Leviticus et al., 2020). Sub-lethal effects refer to ecological, physiological, or behavioral changes in surviving insects after being exposed to sub-lethal insecticide concentrations, which cannot kill them immediately but inhibits their growth, reproduction, and longevity (Li Z et al., 2018; Wang Q et al., 2019). The third larvae of Helicoverpa armigera when treated with LC10, LC25, and LC50 of chlorantraniliprole for 48 h showed significantly prolonged larval and pupal stages of the first generation (Ou et al., 2012). Liang et al. (2017) reported that the growth, development, and fecundity of Spodoptera litura third larvae were significantly inhibited by thiacloprid LC25 and LC50 (Liang et al., 2017). To understand toxicity impact for AITC on B. impatiens, its lethal and sub-lethal effects on third larvae were investigated. The results showed that larval and pupal stages were prolonged, pupae weight was lightened, rates of pupation and adult development were reduced, and oviposition was decreased after the third larvae were treated with LC25 and LC50 of AITC.
It is well-recognized that CO2, as a regulator of respiration, plays very important roles in the life activities of insects (Miller, 1974). However, when the CO2 concentration increased by 10–20%, insect spiracles remained open permanently (Miller, 1974), which provided a new idea for the efficient utilization of the fumigant. High CO2 combined with fumigation against many storage pests has been well-reported. Leelaja et al. (2007) found that elevated CO2, approximately 10–20%, could significantly enhance the fumigation effect of allyl acetate on S. oryzae, S. serrulata, T. ferrugineus, T. castaneum, and S. Dominica (Leelaja et al., 2007). Similarly, high CO2 increased the effectiveness of methyl benzoate against the larvae of Callosobruchus chinensis (Wang L et al., 2019) and significantly improved ethyl formate toxicity to T. castanea and Rhizopertha dominica (Haritos et al., 2006). Our findings illustrated that the exposed third larvae of B. impatiens to AITC under high CO2 conditions lead to a higher mortality than in normal atmosphere; moreover, these lethal effects were as high as 100% with prolonged time. This indicates that high CO2 concentration probably contributed greatly to the fumigant formation, which caused a high lethal effect on B. impatiens. Accordingly, the presence of CO2 concentration under green and mushroom houses must be examined when considering the management of B. impatiens.
Insects detoxify the different numerous exogenous and endogenous compounds due to the production of detoxification enzymes, including GSTs, CarE, and Cyt-b5, and their activities are considered to be an effective indicator monitoring the development of insect resistance to pesticides (Li et al., 2021; Bilal et al., 2020). The presence of insecticides is reported to stimulate the activities of detoxification enzymes due to the increased production of ROS (Jiang et al., 2020). The present results show that the enzyme activities of GSTs, CarE, and Cyt-b5 in B. impatiens third larvae were induced by the sub-lethal concentrations of AITC (LC25) after 24 h and significantly enhanced under high CO2. These results suggest that the detoxification enzymes may be adaptively activated in B. impatiens third larvae as a pro-survival mechanism in response to low concentration of AITC (LC25). Although B. impatiens developed resistance to some extent, it was not enough to defend them from the lethal effect of the AITC (LC25) under high CO2. Previous studies reported that increase in AITC exposure could influence the host plant physiology through accumulation of ROS. Although the present study speculates that AITC fumigation shows greater sub-lethal effects on the growth and reproduction of B. impatiens, AITC under high CO2 conditions may lead to host plant cell damage due to high accumulation of ROS. Thus, the level and frequency of AITC fumigation application must be considered in pest management.
5 Conclusion
The results of this study indicate that AITC is an effective plant extract which demonstrates greater lethal effects on the various stages of B. impatiens. The fumigation method showed effective insecticidal effect on the B. impatiens. AITC (LC25 and LC50) greatly showed sub-lethal effects on the growth and reproduction of B. impatiens. The AITC fumigation test showed greater lethal effects on female and male adults of B. impatiens than those on eggs, third larvae, and pupae. LC25 and LC50 of AITC prolonged larval and pupal stages. The rates of pupation and adult development were reduced, and oviposition was decreased after third larvae were under AITC stress. Moreover, high CO2 enhanced the insecticidal effects of AITC and caused changes in detoxification and antioxidant enzyme activities in B. impatiens. The fumigation method in the application of AITC can be useful in areas where B. impatiens is a major concern. Although the present study speculates that AITC fumigation shows greater sub-lethal effects on the growth and reproduction of the B. impatiens, AITC under high CO2 conditions may lead to host plant cell damage due to high accumulation of ROS. Thus, the level and frequency of AITC fumigation application must be considered in pest management.
Data Availability Statement
The original contributions presented in the study are included in the article/Supplementary Materials; further inquiries can be directed to the corresponding author.
Author Contributions
Y-PG and C-ZL studied the concept and designs. Y-PG and LM analyzed and interpreted the data. PQ, C-CL, and C-ZL investigated and collected the resources. Y-PG drafted the manuscript. PQ and J-JZ reviewed and edited the manuscript. J-JZ critically revised and proofread the manuscript. Y-PG, J-JZ, and C-ZL contributed to statistical analysis. C-ZL funded and supervised the study.
Funding
This research was funded by the Special Fund for Agro-scientific Research in the Public Interest (201303027), and the National Key Research and Development Program of China (2018YFD0200405).
Conflict of Interest
The authors declare that the research was conducted in the absence of any commercial or financial relationships that could be construed as a potential conflict of interest.
Publisher’s Note
All claims expressed in this article are solely those of the authors and do not necessarily represent those of their affiliated organizations, or those of the publisher, the editors, and the reviewers. Any product that may be evaluated in this article, or claim that may be made by its manufacturer, is not guaranteed or endorsed by the publisher.
Acknowledgments
The authors would like to acknowledge JJ Scientific Consultant Ltd., United Kingdom who provided valuable suggestions and contributed to the quality of this article.
References
Arimoto M., Uesugi R., Hinomoto N., Sueyoshi M., Yoshimatsu S.-i. (2018). Molecular Marker to Identify the Fungus Gnat, Bradysia Sp. (Diptera: Sciaridae), a New Pest of Welsh Onion and Carrot in Japan. Appl. Entomol. Zool 53, 419–424. doi:10.1007/s13355-018-0563-y
Bangarwa S. K., Norsworthy J. K. (2014). Purple Nutsedge Control with Allyl Isothiocyanate under Virtually Impermeable Film Mulch. Weed Technol. 28, 200–205. doi:10.1614/WT-D-13-00095.1
Bilal M., Xu C., Cao L., Zhao P., Cao C., Li F., et al. (2020). Indoxacarb‐loaded Fluorescent Mesoporous Silica Nanoparticles for Effective Control of Plutella Xylostella L. With Decreased Detoxification Enzymes Activities. Pest Manag. Sci. 76, 3749–3758. doi:10.1002/ps.5924
Brolsma K. M., van der Salm R. J., Hoffland E., de Goede R. G. M. (2014). Hatching of Globodera Pallida Is Inhibited by 2-propenyl Isothiocyanate In Vitro but Not by Incorporation of Brassica Juncea Tissue in Soil. Appl. Soil Ecol. 84, 6–11. doi:10.1016/j.apsoil.2014.05.011
Cheng D. M., Zhang R., Zhang Z. X. (2018). A Study on the Activity of Four Botanical Insecticides against the Larvae of Bradysia Difformis. Acta Agriculturae Universitatis Jiangxiensis 40, 1264. doi:10.13836/j.jjau.2018159
Clarke D. B. (2010). Glucosinolates, Structures and Analysis in Food. Anal. Methods 2 (4), 310–325. doi:10.1039/b9ay00280d
Damcevski K. A., Dojchinov G., Woodman J. D., Haritos V. S. (2010). Efficacy of Vaporised Ethyl Formate/carbon Dioxide Formulation against Stored-Grain Insects: Effect of Fumigant Concentration, Exposure Time and Two Grain Temperatures. Pest Manag. Sci. 66, 432–438. doi:10.1002/ps.1895
Deguenon J. M., Zhu J., Denning S., Reiskind M. H., Watson D. W., Roe R. M. (2019). Control of Filth Flies, Cochliomyia Macellaria (Diptera: Calliphoridae), Musca domestica (Diptera: Muscidae), and Sarcophaga bullata (Diptera: Sarcophagidae), Using Novel Plant-Derived Methyl Ketones. J. Med. Entomol. 56, 1704–1714. doi:10.1093/jme/tjz107
Devkota P., Norsworthy J. K. (2014). Allyl Isothiocyanate and Metham Sodium as Methyl Bromide Alternatives for weed Control in Plasticulture Tomato. Weed Technol. 28, 377–384. doi:10.1614/WT-D-13-00147.1
Erda L., Wei X., Hui J., Yinlong X., Yue L., Liping B., et al. (2005). Climate Change Impacts on Crop Yield and Quality with CO 2 Fertilization in China. Phil. Trans. R. Soc. B 360, 2149–2154. doi:10.1098/rstb.2005.1743
Felton G. W., Summers C. B. (1995). Antioxidant Systems in Insects. Arch. Insect Biochem. Physiol. 29, 187–197. doi:10.1002/arch.940290208
Gou Y., Guo S., Wang G., Liu C. (2020b). Effects of Short‐term Heat Stress on the Growth and Development of Bradysia Cellarum and Bradysia Impatiens. J. Appl. Entomol. 144, 315–321. doi:10.1111/jen.12733
Gou Y., Liu Q., Liu C. (2015). Effects of Different Host Plants on the Growth, Development and Reproduction of Bradysia Difformis. Plant Prot. 41, 28–32. doi:10.3969/j.issn.0529-1542.2015.01.005
Gou Y., Quandahor P., Zhang K., Guo S., Zhang Q., Liu C., et al. (2020c). Artificial Diet Influences Population Growth of the Root Maggot Bradysia Impatiens (Diptera: Sciaridae). J. Insect Sci. 20, 1–7. doi:10.1093/jisesa/ieaa123
Gou Y., Quandahor P., Zhang Y., Coulter J. A., Liu C. (2020a). Host Plant Nutrient Contents Influence Nutrient Contents in Bradysia Cellarum and Bradysia Impatiens. Plos One 15, e0226471. doi:10.1371/journal.pone.0226471
Gou Y., Wang G., Quandahor P., Liu Q., Liu C. (2019). Effects of Sex Ratio on Adult Fecundity, Longevity and Egg Hatchability of Bradysia Difformis Frey at Different Temperatures. Plos One 14, e0217867. doi:10.1371/journal.pone.0217867
Haritos V. S., Damcevski K. A., Dojchinov G. (2006). Improved Efficacy of Ethyl Formate against Stored Grain Insects by Combination with Carbon Dioxide in a 'dynamic' Application. Pest Manag. Sci. 62, 325–333. doi:10.1002/ps.1167
Jiang H., Chen Y., Ni J., Song J., Li L., Yu Z., et al. (2020). Biphasic Dose-Response of Components from Coptis Chinensis on Feeding and Detoxification Enzymes of Spodoptera Litura Larvae. Dose-Response 18, 155932582091634–155932582091638. doi:10.1177/1559325820916345
Joanisse D. R., Storey K. B. (1998). Oxidative Stress and Antioxidants in Stress and Recovery of Cold-hardy Insects. Insect Biochem. Mol. Biol. 28, 23–30. doi:10.1016/S0965-1748(97)00070-2
Johannsen O. A. (1912). The Fungus Gnats of north America, Part IV. Bull. Maine Agric. Exp. Station 200, 57–146.
Lalouette L., Williams C. M., Hervant F., Sinclair B. J., Renault D. (2011). Metabolic Rate and Oxidative Stress in Insects Exposed to Low Temperature thermal Fluctuations. Comp. Biochem. Physiol. A: Mol. Integr. Physiol. 158, 229–234. doi:10.1016/j.cbpa.2010.11.007
Leelaja B. C., Rajashekar Y., Vanitha Reddy P., Begum K., Rajendran S. (2007). Enhanced Fumigant Toxicity of Allyl Acetate to Stored-Product Beetles in the Presence of Carbon Dioxide. J. Stored Prod. Res. 43, 45–48. doi:10.1016/j.jspr.2005.09.004
Leviticus K., Cui L., Ling H., Jia Z. Q., Huang Q. T., Han Z. J., et al. (2020). Lethal and Sublethal Effects of Fluralaner on the Two‐spotted Spider Mites, Tetranychus Urticae Koch (Acari: Tetranychidae). Pest Manag. Sci. 76, 888–893. doi:10.1002/ps.5593
Li D. Y., Zhi J. R., Zhang T., Yue W. B. (2021). Effects of Multi-Generational Spinetoram Stress on the Detoxification Enzyme Activities, Development and Reproduction of Frankliniella occidentails (Thysanoptera: Thripidae). Acta Entomol. Sin. 64 (10), 1176–1186. doi:10.16380/j.kcxb.2021.10.007
Li J., Qiu G., Jin Y., Cai W., Hua H., Gao G., et al. (2011). Fumigation Toxicity of Allyl Isothiocyanate against Different Development Stages of Callosobruchus Chinensis L. Plant Quarantine 25, 25–27. doi:10.19662/j.cnki.issn1005-2755.2011.03.006
Li Y., Cao Y., Luo L., Zhang Z., Li J. (2018). Research Progresses on the Anti-microbial Activity and Application of Allyl Isothiocyanate in Agriculture. Plant Prot. 44, 108–119. doi:10.16688/j.zwbh.2018324
Li Y. S., Jin J., Liu X. B. (2020). Research Progress on Physiological Response of Crops to Elevated Atmospheric CO2 Concentration. Acta Agronomica Sinica 46, 1819–1830. doi:10.3724/sp.j.1006.2020.94121
Li Z., Guo Y., Liu Y., Wang X., Zhu Z. (2018). The Effects of Sublethal Levels of Insecticide on the wing Dimorphism, Development and Reproduction of Two Aphid Species. Chin. J. Appl. Entomol. 55, 896–903. doi:10.7679/j.issn.20951353.2018.109
Liang W., Xiang X., Wang X., Yang Q., Luo J. (2017). Effects of Sublethal Doses of Thiacloprid on the Detoxifying Enzyme Activities and the Growth and Reproduction of Spodoptera Litura (Lepidoptera: Noctuidae). Acta Entomologica Sinica 60, 1187–1197. doi:10.16380/j.kcxb.2017.10.009
Liang Y., Cheng Z., Cheng S., Qin H., Xiong H., Chen N., et al. (2013). Insecticidal Activities of Allyl Isothiocyanate against rice Brown Planthopper. Acta Agriculturae Jiangxi 25, 85–89. doi:10.19386/j.cnki.jxnyxb.2013.09.021
Lu X. P., Liu J. H., Weng H., Ma Z. Q., Zhang X. (2020). Efficacy of Binary Combinations between Methyl Salicylate and Carvacrol against Thrips Anaphothrips Obscurus : Laboratory and Field Trials. Pest Manag. Sci. 76, 589–596. doi:10.1002/ps.5551
Mansour E. E., Mi F., Zhang G., Jiugao X., Wang Y., Kargbo A. (2012). Effect of Allylisothiocyanate on Sitophilus Oryzae, Tribolium confusum and Plodia Interpunctella: Toxicity and Effect on Insect Mitochondria. Crop Prot. 33, 40–51. doi:10.1016/j.cropro.2011.11.010
Matteo R., Back M. A., Reade J. P. H., Ugolini L., Pagnotta E., Lazzeri L. (2018). Effectiveness of Defatted Seed Meals from Brassicaceae with or without Crude Glycerin against Black Grass (Alopecurus Myosuroides Huds.). Ind. Crops Prod. 111, 506–512. doi:10.1016/j.indcrop.2017.11.020
Mayton H., Olivier C., Vaughn S. F., Loria R. (1996). Correlation of Fungicidal Activity ofBrassicaSpecies with Allyl Isothiocyanate Production in Macerated Leaf Tissue. Phytopathology 86, 267–271. doi:10.1094/phyto-86-267
Meng J.-Y., Zhang C.-Y., Zhu F., Wang X.-P., Lei C.-L. (2009). Ultraviolet Light-Induced Oxidative Stress: Effects on Antioxidant Response of Helicoverpa Armigera Adults. J. Insect Physiol. 55, 588–592. doi:10.1016/j.jinsphys.2009.03.003
Menzel F., Smith J. E., Colauto N. B. (2003). Bradysia Difformis Frey and Bradysia Ocellaris (Comstock): Two Additional Neotropical Species of Black Fungus Gnats (Diptera: Sciaridae) of Economic Importance: A Redescription and Review. Ann. Entomol. Soc. America 96, 448–457. doi:10.1603/0013-8746(2003)096[0448:bdfabo]2.0.co;2
Miller P. L. (1974). Respiration-aerial Gas Transport. Physiol. Insecta 5, 345–402. doi:10.1016/b978-0-12-591606-6.50012-4
Ou S., Liang P., Song D., Shi X., Gao X. (2012). Effects of Sublethal Doses of Chlorpyrifos Benzamide on the Growth and Development and Detoxification Enzyme Activity of Helicoverpa Armigera. Plant Prot. 38, 1–8. doi:10.3969/j.issn.0529-1542.2012.04.001
Paes J. L., Faroni L. R. D. A., Dhingra O. D., Cecon P. R., Silva T. A. (2012). Insecticidal Fumigant Action of Mustard Essential Oil against Sitophilus Zeamais in maize Grains. Crop Prot. 34, 56–58. doi:10.1016/j.cropro.2011.11.021
Ramadan G. R. M., Abdelgaleil S. A. M., Shawir M. S., El-bakary A. S., Edde P. A., Phillips T. W. (2021). Carbon Dioxide Improves Ethanedinitrile Efficacy for Fumigating the Stored Product Pests Rhyzopertha dominica and Lasioderma Serricorne. J. Stored Prod. Res. 93, 1–7. doi:10.1016/j.jspr.2020.101736
Ren Z., Li Y., Fang W., Yan D., Huang B., Zhu J., et al. (2018). Evaluation of Allyl Isothiocyanate as a Soil Fumigant against Soil-Borne Diseases in Commercial Tomato (Lycopersicon esculentumMill.) Production in China. Pest Manag. Sci. 74, 2146–2155. doi:10.1002/ps.4911
Santos A., Zanetti R., Almado R. P., Serrão J. E., Zanuncio J. C. (2012). First Report and Population Changes ofBradysia difformis(Diptera: Sciaridae) onEucalyptusNurseries in Brazil. Fla. Entomologist 95, 569–572. doi:10.1653/024.095.0305
Santos J. C., Faroni L. R. A., Sousa A. H., Guedes R. N. C. (2011). Fumigant Toxicity of Allyl Isothiocyanate to Populations of the Red Flour Beetle Tribolium castaneum. J. Stored Prod. Res. 47, 238–243. doi:10.1016/j.jspr.2011.03.004
Shen D., Zhang H., Li Z., He S., Zhang T. (2009). Taxonomy and Dominace Analysis of Sciarid Fly Species (Diptera: Sciaridae) on Edible Fungi in Yunnan. Acta Entomologica Sinica 52, 934–940. doi:10.16380/j.kcxb.2009.08.014
Shi C.-h., Hu J.-r., Xie W., Yang Y.-t., Wang S.-l., Zhang Y.-j. (2017). Control of Bradysia Odoriphaga (Diptera: Sciaridae) with Allyl Isothiocyanate under Field and Greenhouse Conditions. J. Econ. Entomol. 110, 1127–1132. doi:10.1093/jee/tow303
Smolinska U., Morra M. J., Knudsen G. R., James R. L. (2003). Isothiocyanates Produced by Brassicaceae Species as Inhibitors of Fusarium Oxysporum. Plant Dis. 87, 407–412. doi:10.1094/PDIS.2003.87.4.407
Su J., Lai T., Li J. (2012). Susceptibility of Field Populations of Spodoptera Litura (Fabricius) (Lepidoptera: Noctuidae) in China to Chlorantraniliprole and the Activities of Detoxification Enzymes. Crop Prot. 42, 217–222. doi:10.1016/j.cropro.2012.06.012
Sueyoshi M., Yoshimatsu S. I. (2019). Pest Species of a Fungus Gnat Genus Bradysia Winnertz (Diptera: Sciaridae) Injuring Agricultural and Forestry Products in Japan, with a Review on Taxonomy of Allied Species. Entomol. Sci. 22, 317–333. doi:10.1111/ens.12373
Troncoso R., Espinoza C., Sánchez-Estrada A., Tiznado M. E., García H. S. (2005). Analysis of the Isothiocyanates Present in Cabbage Leaves Extract and Their Potential Application to Control Alternaria Rot in bell Peppers. Food Res. Int. 38, 701–708. doi:10.1016/j.foodres.2005.02.004
Ugolini L., Martini C., Lazzeri L., D’Avino L., Mari M. (2014). Control of Postharvest Grey Mould (Botrytis Cinerea Per.: Fr.) on Strawberries by Glucosinolate-Derived Allyl-Isothiocyanate Treatments. Postharvest Biol. Tech. 90, 34–39. doi:10.1016/j.postharvbio.2013.12.002
Wang L., Cheng W., Meng J., Speakmon M., Qiu J., Pillai S., et al. (2019). Hypoxic Environment Protects Cowpea Bruchid (Callosobruchus Maculatus) from Electron Beam Irradiation Damage. Pest Manag. Sci. 75, 726–735. doi:10.1002/ps.5172
Wang L., Cui S., Liu Z., Ping Y., Qiu J., Geng X. (2018). Inhibition of Mitochondrial Respiration under Hypoxia and Increased Antioxidant Activity after Reoxygenation of Tribolium castaneum. Plos One 13, e0199056. doi:10.1371/journal.pone.0199056
Wang Q., Cui L., Wang Q., Wang L., Xie N., Yuan H., et al. (2019). Effects of Sublethal Concentration of Indoxacarb on the Biological Parameters and Detoxifying Enzyme Activities in the Cotton Bollworm, Helicoverpa Armigera (Lepidoptera: Noctuidae) with Reduced Susceptibility to Indoxacarb. Acta Entomologica Sinica 62, 960–969. doi:10.16380/j.kcxb.2019.08.008
Williams J. R., Rayburn J. R., Cline G. R., Sauterer R., Friedman M. (2015). Effect of Allyl Isothiocyanate on Developmental Toxicity in Exposed Xenopus laevis Embryos. Toxicol. Rep. 2, 222–227. doi:10.1016/j.toxrep.2014.12.005
Worfel R. C., Schneider K. S., Yang T. C. S. (1997). Suppressive Effect of Allyl Isothiocyanate on Populations of Stored Grain Insect Pests. J. Food Process. Preservation 21, 9–19. doi:10.1111/j.1745-4549.1997.tb00764.x
Wu H. H., Wan P., Huang M. S. (2014). Introduction to the Calculation Method of Virulence Regression and the Use of Corresponding Software. Anhui Agric. Sci. 42, 9335. doi:10.13989/j.cnki.0517-6611.2014.27.033
Wu H., Liu X.-r., Yu D.-d., Zhang X., Feng J.-t. (2014). Effect of Allyl Isothiocyanate on Ultra-structure and the Activities of Four Enzymes in Adult Sitophilus Zeamais. Pestic. Biochem. Physiol. 109, 12–17. doi:10.1016/j.pestbp.2014.01.001
Wu H., Zhang G.-A., Zeng S., Lin K.-c. (2009). Extraction of Allyl Isothiocyanate from Horseradish (Armoracia Rusticana) and its Fumigant Insecticidal Activity on Four Stored-Product Pests of Paddy. Pest Manag. Sci. 65, 1003–1008. doi:10.1002/ps.1786
Xiao P., He J., Liu Y., Qiu X., Jiao Y. (2009). The Relationship of Resistance to Lambda-Cyhalothrin with Detoxification Enzyme Activity in Spodoptera Litura ( Fabricius) ( Lepidoptera: Noctuidae). Acta Entomol. Sinica 52, 1097–1102. doi:10.16380/j.kcxb.2009.10.011
Ye Y., Zhang B., Zhang R., Wang K. (2015). The Toxic Effects of Perilla Frutescens Essential Oils in Combination with CO2-enriched Modified Atmospheres on the Life Stages of Dermestes maculatus Degeer (Coleoptera: Dermestidae). Turkish J. Entomol. 39, 261–270. doi:10.16970/ted.47667
Yim B., Hanschen F. S., Wrede A., Nitt H., Schreiner M., Smalla K., et al. (2016). Effects of Biofumigation Using Brassica Juncea and Raphanus Sativus in Comparison to Disinfection Using Basamid on Apple Plant Growth and Soil Microbial Communities at Three Field Sites with Replant Disease. Plant Soil 406, 389–408. doi:10.1007/s11104-016-2876-3
Zhang C., Wu H., Zhao Y., Ma Z., Zhang X. (2016). Comparative Studies on Mitochondrial Electron Transport Chain Complexes of Sitophilus Zeamais Treated with Allyl Isothiocyanate and Calcium Phosphide. Pestic. Biochem. Physiol. 126, 70–75. doi:10.1016/j.pestbp.2015.07.009
Zhang M., Zhang D., Ren J., Pu S., Wu H., Ma Z. (2020). Target Verification of Allyl Isothiocyanate on the Core Subunits of Cytochrome C Oxidase in Sitophilus Zeamais by RNAi. Pest Manag. Sci. 77, 1292–1302. doi:10.1002/ps.6142
Keywords: Bradysia impatiens Johannsen, allyl isothiocyanate, sub-lethal effects, high CO2, detoxification enzyme activity, antioxidant enzyme activity
Citation: Gou Y-P, Quandahor P, Mao L, Li C-C, Zhou J-J and Liu C-Z (2022) Responses of Fungi Maggot (Bradysia impatiens Johannsen) to Allyl Isothiocyanate and High CO2. Front. Physiol. 13:879401. doi: 10.3389/fphys.2022.879401
Received: 19 February 2022; Accepted: 21 March 2022;
Published: 05 May 2022.
Edited by:
Bin Tang, Hangzhou Normal University, ChinaReviewed by:
Samira Kilani-Morakchi, University of Annaba, AlgeriaYujie Lu, Henan University of Technology, China
Muhammad Hafeez, Zhejiang University, China
Copyright © 2022 Gou, Quandahor, Mao, Li, Zhou and Liu. This is an open-access article distributed under the terms of the Creative Commons Attribution License (CC BY). The use, distribution or reproduction in other forums is permitted, provided the original author(s) and the copyright owner(s) are credited and that the original publication in this journal is cited, in accordance with accepted academic practice. No use, distribution or reproduction is permitted which does not comply with these terms.
*Correspondence: Chang-Zhong Liu, bGl1Y2h6aEBnc2F1LmVkdS5jbg==