- Guizhou Provincial Key Laboratory for Agricultural Pest Management of the Mountainous Region, Institute of Entomology, Guizhou University, Guiyang, China
Aspongopus chinensis is a Chinese traditional edible and medicinal insect, which is in great demand in the society. This insect reproduces once a year which is caused by reproductive diapause resulting in insufficient production in wild resources. However, the mechanism of diapause in A. chinensis is still unclear. In this study, we focus on the relationship between juvenile hormones (JHs) and A. chinensis diapause. The results showed that JHIII concentration in diapause adult individuals was significantly lower than that in diapause termination adult individuals. When exogenous JHⅢ was injected into diapause adults, the rate of mating was increased significantly, development of the reproductive systems was accelerated, consumption of fat intensified, the expression of juvenile hormone acid o-methyl-transferase (JHAMT) was upregulated, and juvenile hormone epoxide hydrolase (JHEH) and fatty acid synthase (FAS) gene expressions were downregulated. In addition, RNAi of JHAMT decreased JH concentration, delayed the development of reproductive systems, slowed down fat consumption, and delayed the mean mating occurrence time significantly. Conversely, RNAi of JHEH resulted in an increased concentration of JH, development of reproductive systems was accelerated, consumption of fat was intensified, and mean mating occurrence time advanced significantly. Taken together, these findings uncovered that JH plays an important role in regulating reproductive diapause in A. chinensis and, thus, could provide a theoretical basis for further research on the diapause of A. chinensis.
Introduction
Aspongopus chinensis Dallas, 1851, is a Chinese traditional edible and medicinal insect (Guo et al., 2019). The previous studies showed that hemolytic lymph extracted from A. chinensis inhibits the activity of cancer cells (Yang et al., 2017; Tan et al., 2019a, Tan et al., 2019b). Therefore, A. chinensis is in great demand in the society. However, despite its medicinal value and information about its bioecology being discovered (Wei et al., 2015; Gu et al., 2017), it has a diapause for up to 7 months and reproduces once a year (Wei et al., 2015) which results in insufficient production in the wild resources. To improve the utilization of A. chinensis resources, we focused our attention on A. chinensis diapause.
Studies have shown that diapause is a developmental arrest that is induced by photoperiod and temperature signals before the unfavorable environmental arrival (Tauber et al., 1986). Insects enter diapause to avoid adverse environments and ensure population continuity (Denlinger 2002). Diapause occurs at the species-specific developmental stage including eggs, larvae, pupas, and adults in insects (Bale and Hayward 2010). Diapause in the adult phase is a reproductive diapause, such as A. chinensis, and the main performance is arrested ovarian development and lipid accumulation (Denlinger et al., 2012; Denlinger and Armbruster 2014).
Juvenile hormones (JHs) are considered to be the primary hormones that regulate reproduction in many insects (Jindra et al., 2013; Smykal and Raikhel 2015). The change of juvenile hormone is one of the important factors for the maintenance and termination of diapause in insects. JH was lower during diapause but increased gradually after the diapause termination that was demonstrated in Drosophila melanogaster, Riptortus clavatus, and Poecilocoris lewisi (Herman 1981; Chinzei et al., 1992; Miyawaki et al., 2006). In the absence of JH during diapause, insects mainly exhibit arrested reproductive development, increased lipid accumulation, and higher stress tolerance (Denlinger and Armbruster 2014). In the adult diapause insects, such as Coleoptera, Hemiptera, and Lepidoptera, an injection of exogenous JH or JH analogs (JHAs) promotes reproductive behavior (De Kort 1981; Kort 1990; Kopper et al., 2001; Evenden et al., 2007; Hoffmann et al., 2007; Toyomi et al., 2010; Jindra et al., 2013). However, it remains unknown whether and how does JH terminate diapause in A. chinensis.
JH is biosynthesized through the mevalonate pathway. Insects are unable to biosynthesize sterols due to the lack of squalene synthase and lanolin sterol synthase. After farnesyl diphosphate was biosynthesized, JH follows a unique route to biosynthesized (Beenakers et al., 1985). Juvenile hormone acid O-methyl-transferase (JHAMT) is a key enzyme regulated in the JH synthesis pathway and is essential for the normal biosynthesis of JH (Minakuchi et al., 2008). Studies have shown that the use of RNAi to knock down Schistocerca gregaria JHAMT will lead to a decrease in the concentration of juvenile hormones and delay the development of the reproductive system (Marchal et al., 2011). In Blattella germanica, knockdown with JHAMT resulted in a significant decrease in the JH concentration accompanied by a decrease in the expression of the vitellogenin gene in the fat body (Dominguez and Maestro 2018). Juvenile hormone epoxide hydrolase (JHEH) has been proved to play an important role in JH degradation (Share et al., 1988; Lassiter et al., 1994; Halarankar et al., 2016). For example, the use of RNAi technology to knockdown with the JHEH in Leptinotarsa decemlineata larvae will significantly reduce the pupation and molting. In the study of Coccinella septempunctata L., it was found that JHEH RNAi in diapause adults will increase the concentration of JH, resulting in increasing expression of vitellogenin and promoting the development of the reproductive system. In our preliminary study, we conducted a high-throughput transcriptome sequencing analysis of diapause and diapause termination of A. chinensis (Wu et al., 2019). The data showed that JHEH and JHAMT genes have a significant difference in gene expression between diapause and diapause termination. Compared with diapause, JHAMT gene expression was significantly upregulated, JHEH gene expression was significantly downregulated in diapause termination. Taken together, JH biosynthesis genes JHAMT and degradation genes JHEH may regulate the concentration of JH together that causes A. chinensis to be in diapause state or diapause terminations. However, it remains unknown whether JH participates in reproductive diapause in A. chinensis.
In the present study, JH concentration in A. chinensis was detected, and results showed that JHIII concentration in diapause individuals was significantly lower than that in diapause termination individuals. Subsequently, the exogenous JHIII was injected into diapause adults of A. chinensis. Overall, after JHⅢ injection, the rate of mating increased significantly, development of the reproductive systems accelerated, consumption of fat intensified, the expression of JHAMT was upregulated, and JHEH and fatty acid synthase (FAS) gene expressions were downregulated. Furthermore, RNAi of JHAMT resulted in decreased JH concentration, development of reproductive systems was delayed, the consumption of fat slowed down, and mean mating occurrence time was delayed significantly. Conversely, RNAi of JHEH resulted in an increased concentration of JH, development of reproductive systems was accelerated, consumption of fat intensified, and mean mating occurrence time advanced significantly. These findings uncovered that JH plays an important role in regulating reproductive diapause in A. chinensis and, thus, could provide a theoretical basis for further research on the diapause of A. chinensis.
Materials and Methods
Insect Gathering and Rearing
Adults of A. chinensis in diapause were collected from Bijie City, Guizhou Province, southwest China. Individuals were kept at 28 ± 1°C with 85 ± 5% humidity under a 16/8 h (light/dark) photoperiod condition (Guo et al., 2019). Mating was used as the standard of diapause termination.
Juvenile Hormone Ⅲ Determination
The method of extracting juvenile hormone Ⅲ through high-performance liquid chromatography (HPLC) was referenced Hao et al. (2012). The samples of two A. chinensis (diapause or diapause termination) were placed in a glass homogenizer, 1 ml methanol and 1 ml ethyl ether was added to the homogenate. After homogenization, 4 ml n-hexane was added. It was then centrifuged at 12,000 r/min for 10 min and supernatant was collected. The precipitation was repeatedly extracted with n-hexane 3 times. The collected liquid samples were dried with a nitrogen blower before placing them into the instrument. The volume was made to 1 ml with the mobile phase. The chromatographic conditions include mobile phase methanol: ether (80: 20), column temperature is 25°C, the flow rate is 0.5 ml/min, sample injected 10 μL, ultraviolet detection wavelength is 218 nm; JHⅢ used isocratic elution, the sample was compared with the prototype of the peak area of quantitative. Each treatment had 3 biological replicates.
Juvenile Hormone Ⅲ Injecting
The under-wing injection method was used. Exogenous JHIII was diluted with acetone to 5, 10, 50, 100, and 200 ng/μL, acetone as a control. For each adult individual, 2 μL acetone was injected by using a 5 μL microsyringe (Shanghai gaoge industrial and trading co., LTD.). We set five repetitions, each with 10 A. chinensis (5 males and 5 females). After injecting, the treated diapause adults were placed in labeled milk tea cups, respectively, in an environment at a temperature of 28 ± 1°C, relative humidity of 85 ± 5%, photoperiod (16 L: 8 D), and the pumpkin (Cucurbita moschata Duch) seedlings were used for rearing (Wu et al., 2019). The number of deaths and the termination of diapause were monitored daily.
dsRNA Treatment
Primers (Table 1) used for dsRNA were designed by Primer 5 program. JHEH or JHAMT double-stranded RNA (dsRNA) was prepared using the T7 RNA polymerases containing the appropriate gene and injected into A. chinensis adult. Adults received 1 μL of dsRNA at a concentration of 400 ng/μL in solution; control insects were injected with GFP dsRNA derived from Aequorea victoria genes.
Anatomy of the Reproductive System
Randomly selected A. chinensis was treated by dsRNA. The reproductive system was dissected in the precooled 75% alcohol from three females and three males every 7 days until mating to investigate tissue-specific expression profiles under a stereomicroscope (SMZ250, Nikon). Day 0 was the control group. We set three repetitions and carefully measured and recorded the size of the reproductive organs.
Nile Red Staining of Aspongopus chinensis Fat Bodies
Fat bodies were dissected in the precooled phosphate buffer saline, and the adherent fat bodies were carefully removed with forceps as thoroughly as possible under a stereomicroscope (SZM-7045, Nikon). The fat bodies from different time periods (every 7 days) were washed twice with 1× PBS buffer fixed with 4% paraformaldehyde for 30 min at room temperature. It was then washed twice with 1× PBS buffer. The fat bodies were then incubated in Nile Red solution (1 mg/ml Nile Red was diluted to 1 ug/mL by 0.1% Triton X-100) for 90 min at room temperature to stain the lipid droplets. Then it was washed twice with 1× PBS buffer. The cell nuclei were stained with 1 μg/ml DAPI (Sigma, D8417, United States) for an additional 15 min. Finally, it was washed twice with 1× PBS buffer. Fat body cell images were captured using a Nikon bright-field inverted light microscope (Tokyo, Japan). Day 0 was the control group. We set three repetitions, each with six A. chinensis (three males and three females).
Detection of Triglyceride Content
The total TG levels were determined by the liquid triglycerides (GPO-PAP) method (Liu et al., 2016) using the Triglycerides Assay Kit (Nanjing Jiancheng Institute, China) according to the manufacturer’s instructions. After accurately weighing the fat body, we added anhydrous ethanol at a weight (g) to volume (ml) ratio of 1: 9. Mechanical homogenization was conducted under ice bath condition. Then, after centrifugation at 2,500 rpm for 10 min, the supernatant was taken; 2.5 μL of the supernatant and 250 μL reaction solution were added to a 96-well plate and incubated at 37°C for 10 min. The absorbance was measured at 510 nm using an automated microplate reader (Bio-Rad, Hercules, CA, United States). Three independent biological replicates and five technical replicates were performed for every treatment. The formula used for calculation was as follows.
Quantitative Real-Time PCR
Total RNAs from whole bodies were isolated using RNAeasy™ Animal RNA isolation Kit with Spin Column (Shanghai, Beyotime) according to the manufacturer’s instructions. RNA concentration was determined using a spectrophotometer (ND-2000, United States, Thermo Scientific). To avoid genomic DNA contamination, total RNA was treated with RNase-free DNase water (Pekin, Solarbio). The first-strand cDNA was synthesized using a SuperScript double-stranded cDNA synthesis kit according to the manufacturer’s instructions. Primers (Table 2) used for qPCR were designed by the Primer 5 program. qPCR was performed with a T100TM PCR instrument (T100TM, United States, Bio-Rad) using the iTaqTM Universal SYBR®Green Supermix under the following reaction conditions: 95°C for 10 min; 40 cycles of 94°C for 30 s, 53°C for 30 s, and 72°C for 20 s. The stable reference gene beta-actin of A. chinensis was used for normalization (Wu 2019). All qPCR reactions were carried out in three biological replicates, and three technical replicates were performed for each sample. The relative expression levels were calculated using the 2−ΔΔCT method (Livak and Schmittgen 2001).
Data Analysis
Results were presented as mean ± SE (standard error) based on at least three independent biological replications. Differences between the two groups were analyzed by an independent t-test. One-way ANOVA followed by Duncan’s multiple comparison was used for the comparison among more than two different conditions. p values less than 0.05 (*), 0.01 (**), or 0.001 (***) were considered to be statistically significant. All data were subjected to statistical analysis using the SPSS software (version 22; SPSS Inc., Chicago, IL, United States). Graphical representations were performed using Excel 2007 software.
Results
JHIII in Diapause Was Lower Than That in Diapause Termination
High-performance liquid chromatography was used to detect juvenile hormones. The result indicated that the UV absorption peak of standard JHIII was at 218 nm, and the retention time was 8 min approximately (Figure 1A). Subsequently, the JHⅢ of A. chinensis in diapause and diapause termination were detected. A small peak appeared at the time close to the standard sample both in diapause (Figure 1B) and diapause termination samples (Figure 1C). However, the peak in the chromatogram of diapause termination was higher than that in diapause. The JHIII content was quantified according to the peak areas of samples and standards, and the data showed that JHIII concentration in diapause (4.3016 ± 0.0449 ng/μL) was significantly lower than that in diapause termination (9.6873 ± 1.6157 ng/μL) (Figure 1D). It implies that the increase in JHIII content in A. chinensis was involved in terminating diapause.
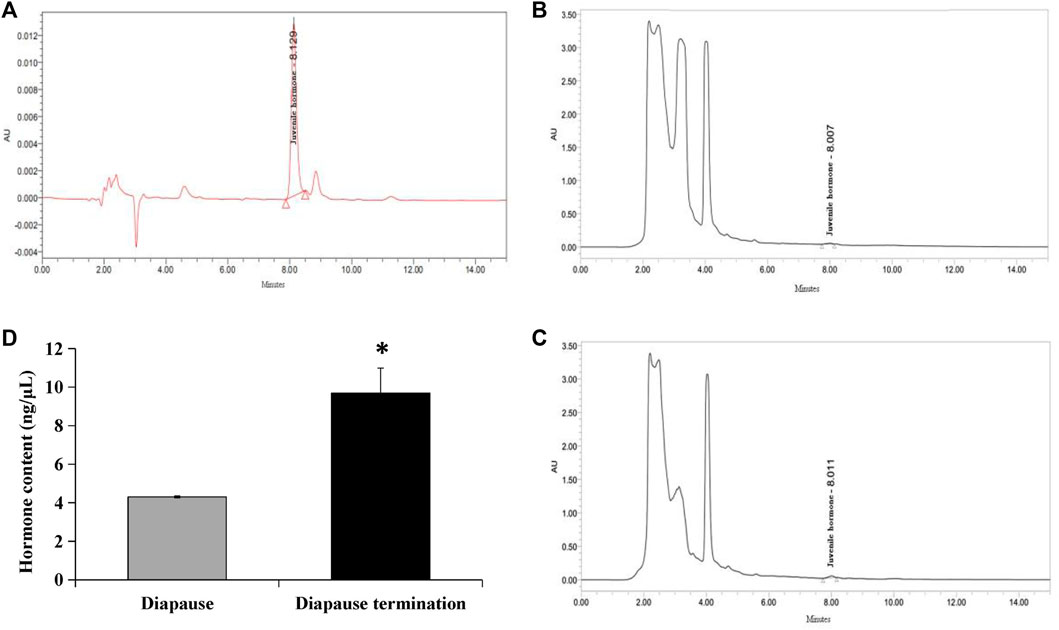
FIGURE 1. Determination of JHⅢ in diapause and diapause termination adult individuals. (A) Chromatogram of JHIII standard sample. (B) Chromatogram of JHIII in diapause of A. chinensis. (C) Chromatogram of JHIII in diapause termination of A. chinensis. (D) Quantification of JHIII content in diapause and diapause termination. *, p < 0.05 (independent t-test).
Exogenous JHIII Injection Affects Reproductive Diapause
To further explore the relationship between JH and A. chinensis diapause, the exogenous JHIII injecting was employed. The survival rate of A. chinensis was above 90% after injecting with different JHIII concentrations (Supplementary Table S1). In addition, the rate of mating was counted at day 30. The results showed that JHⅢ injection could increase the rate of mating significantly, and 50 ng/μL JHIII injection had the highest mating rate (91.600% ± 0.038%) (Table 3).
In the male adult, the reproductive system is mainly composed of six parts: a pair of testis (which is divided into seven testicular follicles), a pair of vas deferens, a seminal vesicle, an ejaculatory bulb, an ejaculatory duct, and a pair of accessory glands (Supplementary Figure S1). Correspondingly, in the female adult of A. Chinensis, the reproductive system is mainly composed of a pair of ovaries (which divided into seven ovarioles and with a terminal filament at the apex of each ovariole), a pair of the lateral oviduct, a common oviduct, and a pear-shaped spermatheca, with no accessory glands (Supplementary Figure S2). However, after injection of exogenous JHIII, the male (Figure 2) and female (Figure 3) reproductive organs of A. Chinensis developed rapidly to maturity in about 30 days, and the length and width of reproductive system structures (testis, vas deferens, seminal vesicle, ejaculatory bulb in male; ovarioles, lateral oviducts, common oviduct, spermatheca in female) increased significantly.
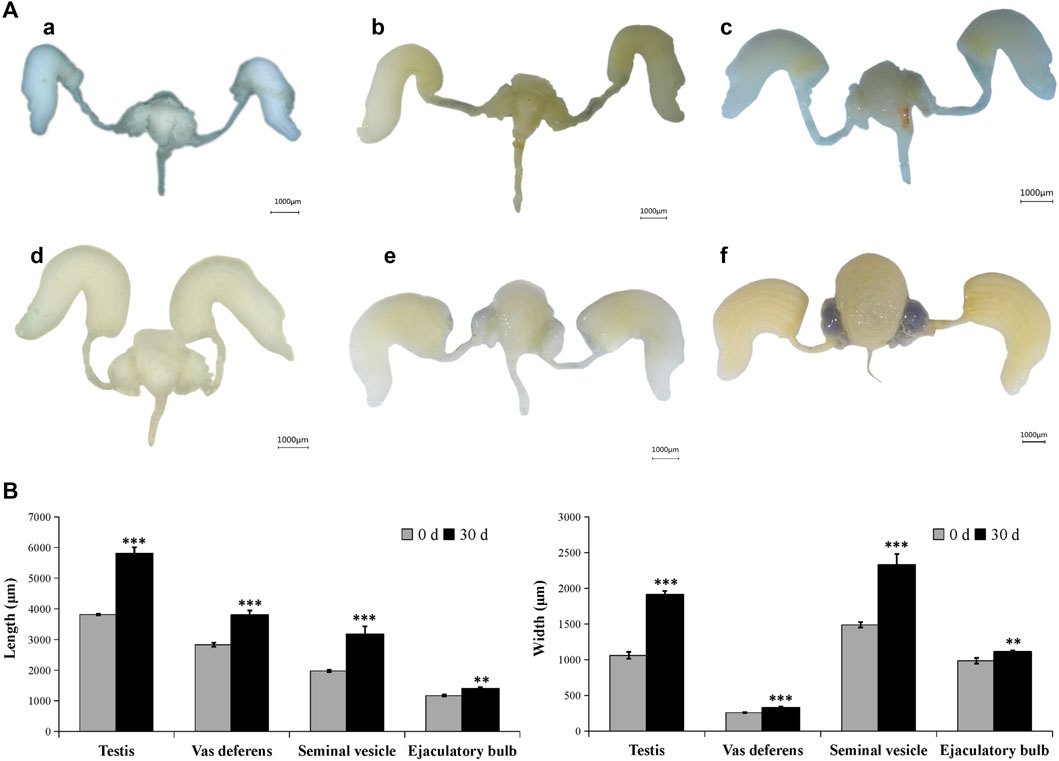
FIGURE 2. Development pattern of male reproductive system after JHIII injection. (A) Photograph of male reproductive system at different day after JHIII injection. a, 0th day; b, 7th day; c, 14th day; d, 21st day; e, 28th day; f, 30th day. (B) Length and width of male reproductive structures (testis, vas deferens, seminal vesicle, ejaculatory bulb). **, p < 0.01; ***, p < 0.001 (independent t-test).
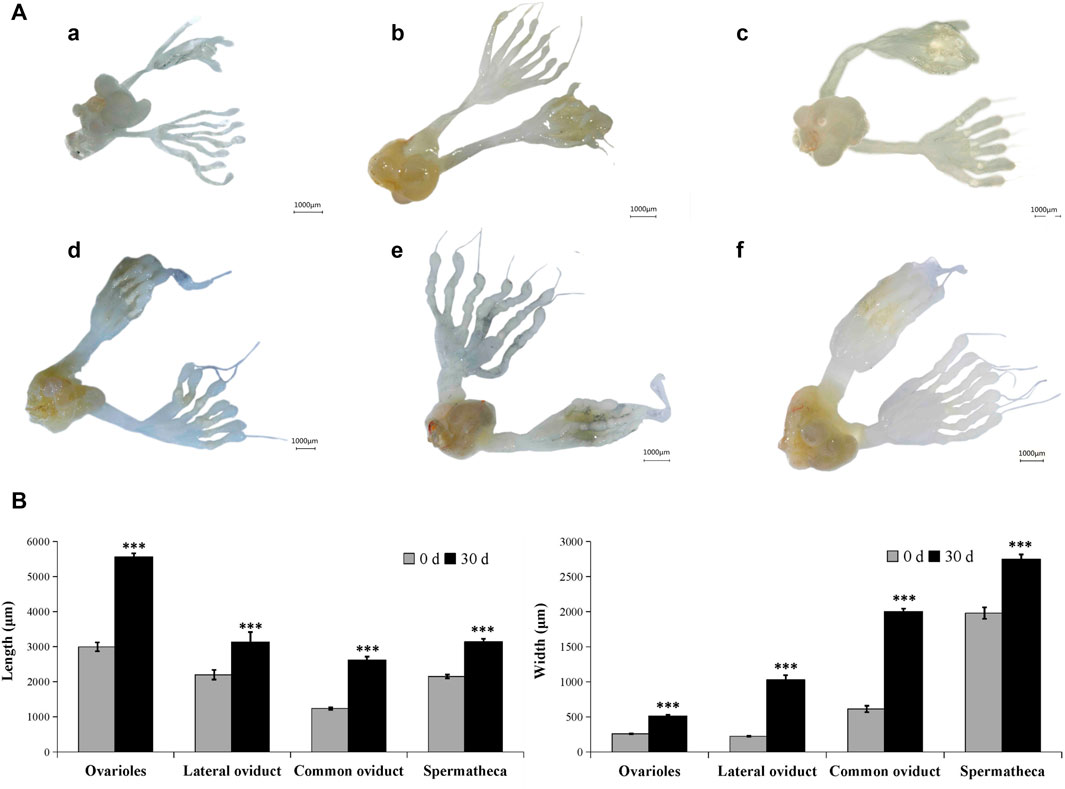
FIGURE 3. Development pattern of female reproductive system after JHIII injection. (A) Photograph of female reproductive system at different day after JHIII injection. a, 0th day; b, 7th day; c, 14th day; d, 21st day; e, 28th day; f, 30th day. (B) Length and width of female reproductive structures (ovarioles, lateral oviducts, common oviduct, spermatheca). ***, p < 0.001 (independent t-test).
The lipid droplets were consumed as energy for reproductive development after diapause termination. Thus, Nile Red staining was used to detect the change of lipid droplets after JHIII injection. In the beginning, the lipid droplets filled the whole fat body. After JHIII injection, the stored lipid droplets in A. chinensis were reduced over time (Figure 4A). In addition, the triglycerides levels were reduced after JHIII injection which is consistent with the results of Nile Red staining (Figure 4B).
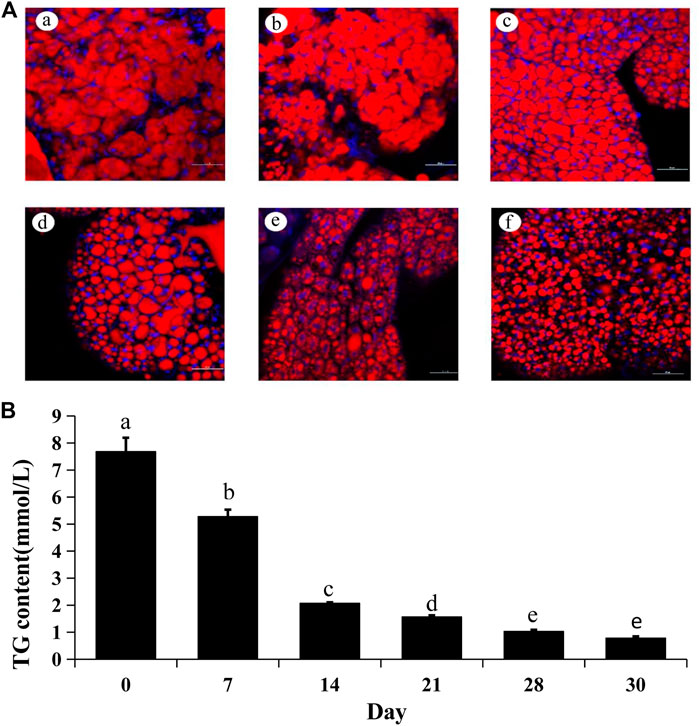
FIGURE 4. Lipid changes after JHIII injection in the fat body of A. chinensis. (A) Nile Red staining of fat body after JHIII injection. a, 0th day; b, 7th day; c, 14th day; d, 21st day; e, 28th day; f, 30th day. Red signal indicates the lipid droplets stained by Nile Red, and blue signal indicates the nucleus stained by DAPI. (B) Triglyceride (TG) contents. The different lowercase letters above bar are significant difference at p < 0.05 (one-way ANOVA followed by Duncan’s multiple comparison).
After the exogenous JHIII was injected into diapause adults of A. chinensis, the relative expressions of JHEH, JHAMT, and FAS were measured by qPCR. The data showed that the expression of the JHAMT was upregulated significantly. Whereas the expressions of JHEH and FAS were downregulated significantly (Figure 5).
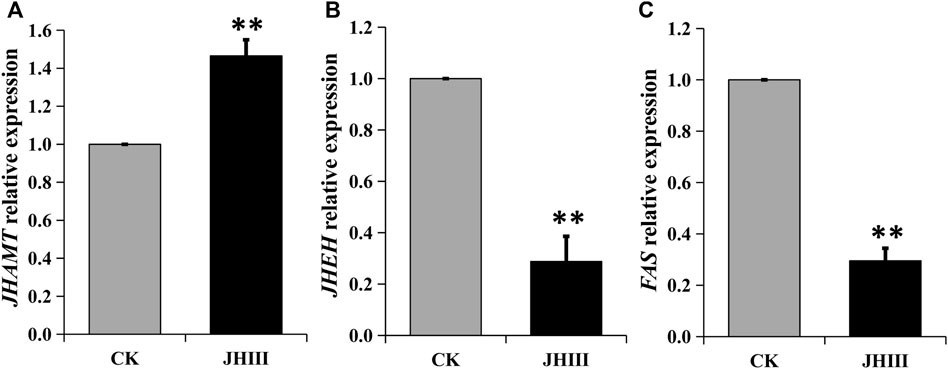
FIGURE 5. Relative expression of JHAMT (A), JHEH (B), FAS (C) genes after JHIII injection. **, p < 0.01 (independent t-test).
The results given above demonstrated that JH could promote reproductive development but reduce lipid storage suggesting that JH has opposite effects on reproductive diapause.
Effects of JHEH and JHAMT RNAi on Reproductive Development
Firstly, we synthesized the dsRNA of JHEH and JHAMT genes, and the expressions of JHEH and JHAMT in A. chinensis were significantly inhibited after injection of dsRNA (Supplementary Figure S3). The inhibition rate was more than 80%, indicating that the interference effect is better. Next, the anatomy of the reproductive system of the A. chinensis after dsRNA injection is shown in Figures 6, 7. Compared with the control group (CK or dsGFP), the male or female reproductive system of the dsJHAMT experimental group developed significantly slower, while the dsJHEH experimental group developed faster. With the passage of time, the testis, vas deferens, seminal vesicles and ejaculatory bulb gradually developed in the dsJHEH group developed and matured earlier, while the dsJHAMT group developed more slowly (Supplementary Figure S4). The ovarioles, lateral oviduct, spermatheca, and common oviduct in each experimental group developed gradually over time, and the dsJHAMT group developed slowly at 0, 7, 14, 21, 28, 35, and 42 days, while the dsJHEH group developed on the 42nd day (Supplementary Figure S5).
The results of Nile Red staining showed that the fat body cells in the early stage of diapause were full of lipids, and the fat was gradually consumed over time. Compared with the CK and dsGFP group, the fat consumption of dsJHEH was significantly higher, while the fat consumption of dsJHAMT was not obvious (Figure 8). Compared with the control group (dsGFP) and the blank group (CK), the consumption rate of triglycerides in the dsJHAMT group was relatively slow, while the consumption of triglycerides in the dsJHEH group was intensified (Figure 9A), which was consistent with the results of Nile Red staining.
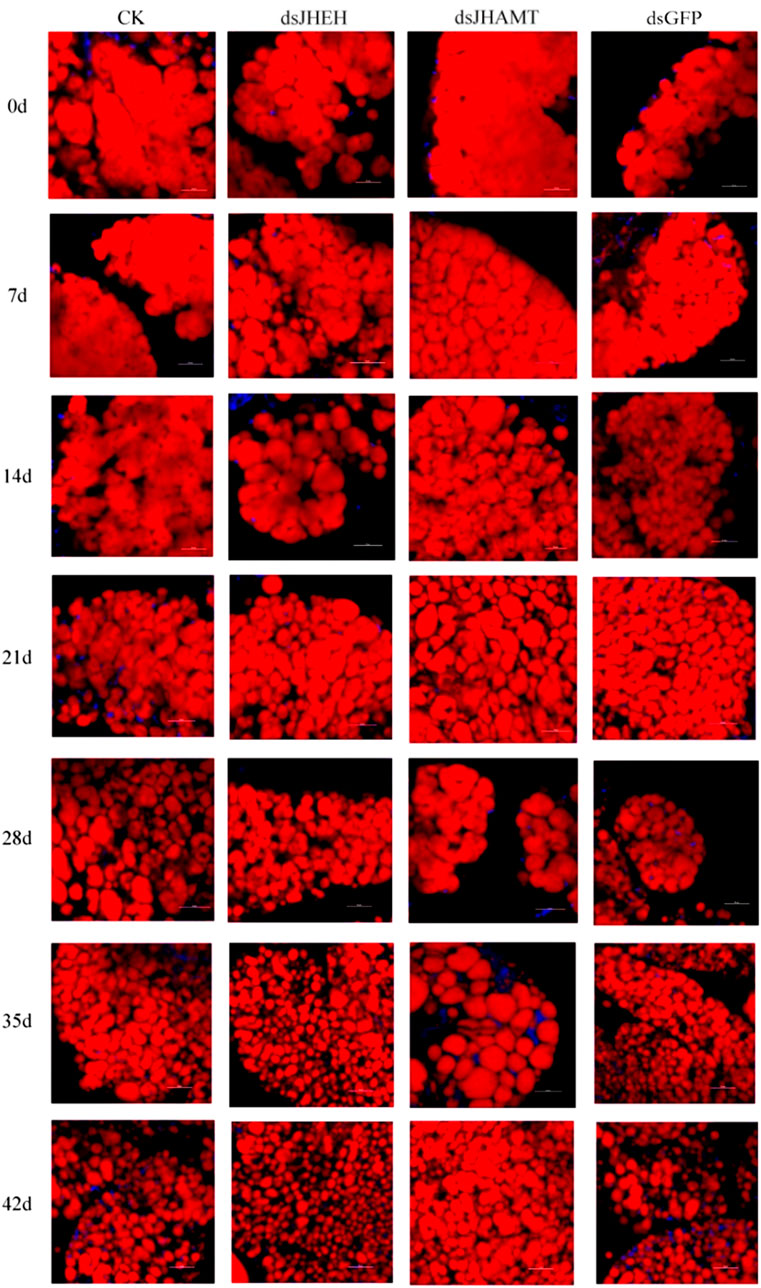
FIGURE 8. Lipid change in the fat body after injection of dsRNA in A. chinensis. Red signal indicates the lipid droplets stained by Nile Red, and blue signal indicates the nucleus stained by DAPI.
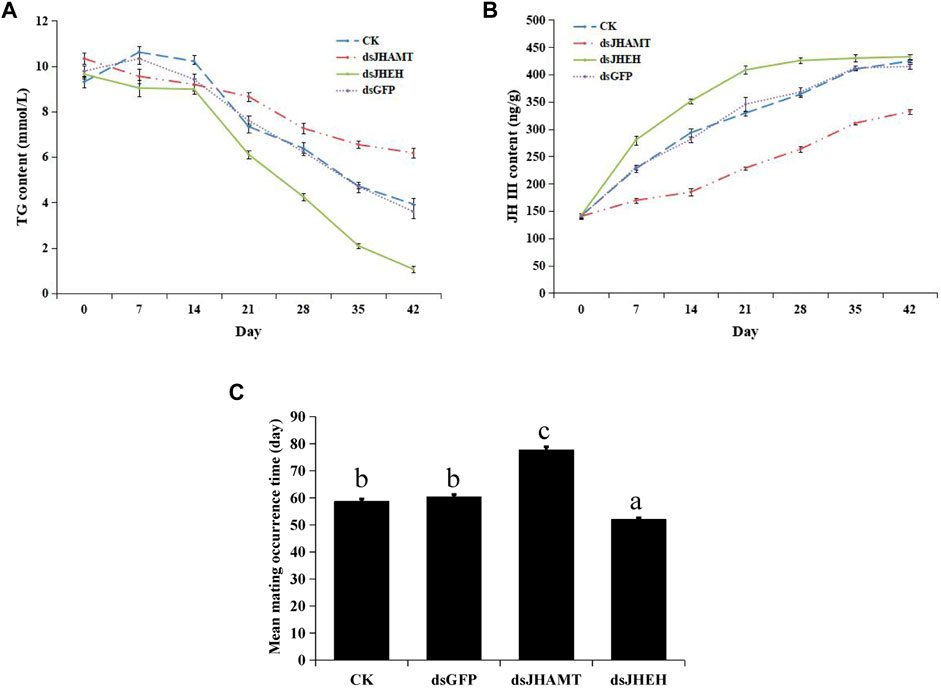
FIGURE 9. Triglyceride content (A), juvenile hormone concentration (B) and mean mating occurrence time (C) after dsRNA injection. The different lowercase letters above bar are significant difference at p < 0.05 (one-way ANOVA followed by Duncan’s multiple comparison).
We calculated the concentration of juvenile hormone according to the standard curve (Supplementary Figure S6). Juvenile hormones in diapause A. chinensis are a process of accumulation (CK group in Figure 9B). In the dsGFP group, the concentration of juvenile hormone increased from 140.04 ng/g to 414.38 ng/g with time. After injection of dsJHEH, the degradation of juvenile hormone was inhibited, and the growth rate of juvenile hormone concentration was significantly higher than that of other groups. Compared with dsGFP, after injection of dsJHAMT, the synthesis of juvenile hormone was significantly inhibited, and the growth rate of juvenile hormone was slowed down (Figure 9B and Supplementary Table S2).
At last, the mean mating occurrence time after dsRNA injection was calculated. The results showed that the mean mating occurrence times of the CK and dsGFP groups were at 58.67 ± 0.88 and 60.33 ± 0.88 days, respectively. The injection of dsJHAMT was at 77.67 ± 1.20 days, which was delayed significantly compared with other groups, while the dsJHEH injection group was at 52.00 ± 0.58 days, and their mating occurrence time was significantly earlier (Figure 9C).
Discussion
Diapause can occur in any period in insects including eggs, larvae, pupae, and adults. It is well known that hormones, such as diapause hormone, ecdysteroids, and JH, control insect diapause (Denlinger 2002). The obligate diapause of A. chinensis occurred in adults complied by reproductive arrested. Most of the previous works on adult diapause focused on JH, and the results supported that JH plays a major role in regulating adult diapause in insects (Denlinger et al., 2012). In the present study, we found JH concentration was lower in diapause adults than in diapause determination adults. JH application can stimulate reproductive maturation and terminated diapause. These results suggested JH is involved in A. chinensis diapause which is consistent with the adult diapause study in other insects. However, JH is not the exclusive cause of diapause induction and maintenance. For example, in D. melanogaster, the ovaries produce a low level of ecdysteroids during diapause but a high level in terminating diapause (Richard et al., 1998), and 20-hydroxyecdysone (20E) injection can terminate diapause in a dose-dependent manner (Richard et al., 2001). Similar results were found in Colaphellus bowringi (Guo et al., 2021). In addition, a study in L. decemlineata showed that injection of a combination of 20E and JH are much more effective in breaking diapause than only JH injection (Lefevere 1989). The evidences above imply that both JH and ecdysteroid have been implicated in the regulation of diapause. But in A. chinensis, other hormones, such as ecdysteroid, are involved in diapause regulation should be further studied.
JHAMT and JHEH are key enzymes in JH synthesis and degradation, respectively. RNAi of JHAMT decreased the JH concentration, and JHEH knockdown increased the concentration of JH. Especially, when exogenous JH injection resulted in increasing JHAMT gene expression and downregulating JHEH gene expression. This performance uncovers that JHAMT or JHEH with juvenile hormone exits feedback regulation. Nevertheless, there are many genes in the decision of JH concentration except for JHAMT and JHEH, and other genes involved in diapause of A. chinensis need further study. On the other side, despite we found JH to play an important role in the regulation of A. chinensis adult diapause, the downstream response mechanism remains a mystery. However, some cell signaling pathways, such as insulin signaling, have been confirmed to regulate insect diapause (Hahn and Denlinger 2011). In the study of Culex pipens, the addition of JH to diapausing individuals reduces FoxO (a key intracellular effector in insulin signaling) levels in the fat body (Sim and Denlinger 2013). Moreover, in A. chinensis, the transcriptome analysis discovered that the FoxO gene expression was a significant difference between diapause adults and diapause termination adults (Wu et al., 2019). Therefore, insulin signaling may be downstream of JH for regulating adult diapause in A. chinensis.
It is known that lipids, especially triacylglyceride, are the most important energy reserve in most diapausing insects. Diapause individuals have greater triacylglyceride accumulation than development individuals in some species, and increased triacylglyceride storage is thought to be an important factor for mitigating the metabolic demands of diapause (Hahn and Denlinger 2007). Further, when lipid metabolic rate is high, the duration of diapause is shorter, and when the metabolic rate is low, the diapause duration is longer. Presumably, these supported that the amount of stores or rate of utilization of lipid adjusted the duration of diapause (Hahn and Denlinger 2011). In A. chinensis, we found the lipid droplets fill the whole fat body in the earlier diapause, and the consumption of lipid was accomplished with diapause maintenance. When exogenous JH application was done in diapause A. chinensis, lipid consumption was increased. The results suggest lipid or triacylglyceride may play an important role in diapause maintenance, and JH targeted the downstream lipid for elevating the metabolic rate resulting in a shorter diapause. However, another previous study has proposed that lipid accumulation in Polistes wasps produces JH suppression which is a prerequisite for diapause (Hunt et al., 2007). From this, JH and lipid regulated adults’ diapause may play a role in reciprocation and intricacy, which will be an open question.
The central feature of adult diapause is reproductive arrest. Most previous studies were focused on females, and oocyte development arrest was characterized for the diapause. However, there are few studies conducted on reproductive diapause in male insects (Denlinger et al., 2012). Here, we focus on the reproductive diapause in female adults and male adults equally, and the reproductive system characteristics of diapause males were observed in detail. Furthermore, the previous view states that the status of the testes may not be a consistent reliable indicator of diapause in males. Thus, in males, the most conspicuous feature was their failure to mate with receptive females which were used to determine diapause in a few studies (Pener 1992). Whereas, in our findings, similar to females, the developmental stages of the male reproductive organ are stable and observable either in JH application or RNAi injection. These results indicated that male reproductive organs (including testes) can be used for diapause monitoring in A. chinensis.
In summary, our study found that JH was lower in diapause adults than that in diapause termination adults in A. chinensis. Exogenous JH application can terminate diapause, promoted the development of the reproductive system both in males and females, and accelerated lipid consumption. For another, the disruption of JHEH and JHAMT modulated the concentration of JH, promoted or inhibited the reproductive system development and lipid consumption. This funding uncovered that the JH is an important factor in regulating adult diapause in A. chinensis and, thus, could provide a basis for further research.
Data Availability Statement
The original contributions presented in the study are included in the article/Supplementary Material, further inquiries can be directed to the corresponding authors.
Author Contributions
J-JG and W-ZZ participated in the study design; W-ZZ, Y-FW, and Z-YY participated in experimental work; W-ZZ wrote the manuscript; and J-JG and H-YL critically revised the manuscript.
Funding
This work was supported by the National Natural Science Foundation of China (82160743), Lancang-Mekong Cooperation Special Fund Projects [JiaoWaiSiYa (2020)619], and Youth Talents of Science and Technology Projects from the Guizhou Educational Department [Qian Jiao He KY Zi (2021) 078].
Conflict of Interest
The authors declare that the research was conducted in the absence of any commercial or financial relationships that could be construed as a potential conflict of interest.
Publisher’s Note
All claims expressed in this article are solely those of the authors and do not necessarily represent those of their affiliated organizations, or those of the publisher, the editors, and the reviewers. Any product that may be evaluated in this article, or claim that may be made by its manufacturer, is not guaranteed or endorsed by the publisher.
Supplementary Material
The Supplementary Material for this article can be found online at: https://www.frontiersin.org/articles/10.3389/fphys.2022.873580/full#supplementary-material
Supplementary Figure S1 | Internal reproductive organs of a sexually mature male of A. chinensis, dorsal view (A) and ventral view (B). AG, accessory gland; ED, ejaculatory duct; EB, ejaculatory bulb; SV, seminal vesicle; T, testis; VD, vas deferens.
Supplementary Figure S2 | Reproductive organs of a sexually mature female of A. chinensis. CO, common oviduct; LO, lateral oviduct; Ov, ovary; Ovl, ovariole; S, spermatheca; TF, terminal filament.
Supplementary Figure S3 | Relative expression of JHEH (A) and JHAMT (B) in A. chinensis after RNAi. **, p < 0.01 (independent t-test).
Supplementary Figure S4 | Males reproductive system development after RNAi in A. chinensis. Average length and width measurements of testis (A, B), vas deferens (C, D), seminal vesicle (E, F) and ejaculatory bulb (G, H). The different lowercase letters above bar are significant difference at p < 0.05 (one-way ANOVA followed by Duncan’s multiple comparison).
Supplementary Figure S5 | Female reproductive system development after RNAi in A. chinensis. Average length and width measurements of ovarioles (A, B), lateral oviduct (C, D), spermatheca (E, F) and common oviduct (G, H). The different lowercase letters above bar are significant difference at p < 0.05 (one-way ANOVA followed by Duncan’s multiple comparison).
Supplementary Figure S6 | Standard curve of juvenile hormone.
Supplementary Table S1 | Survival of diapause A. chinensis injected with JHIII for 48 h.
Supplementary Table S2 | Juvenile hormone concentration after dsRNA injection.
References
Bale J. S., Hayward S. A. L. (2010). Insect Overwintering in a Changing Climate. J. Exp. Biol. 213, 980–994. doi:10.1242/jeb.037911
Beenakkers A. M. T., Horst D. J. V. D., Marrewijk W. J. A. V. (1985) Insect Lipids and Lipoproteins, and Their Role in Physiological Processes. Prog. Lipid Res., 24, 19–67. dio: doi:10.1016/0163-7827(85)90007-4
Chinzei Y., Miura K., Kobayashi L., Shinoda T., Numata H. (1992). Cyanoprotein: Developmental Stage, Sex and Diapause-dependent Expression, and Synthesis Regulation by Juvenile Hormone in the Bean bug,Riptortus Clavatus. Arch. Insect Biochem. Physiol.Archives Insect Biochem. Physiol. 20, 61–73. doi:10.1002/arch.940200107
De Kort C. A. D. (1981). Hormonal and Metabolic Regulation of Adult Diapause in the colorado Beetle, Leptinotarsa decemlineata (Coleoptera: Chrysomelidae). entomologia 7, 261–271. doi:10.1111/j.1365-2311.1981.tb00637.x10.1127/entom.gen/7/1981/261
Denlinger D. L., Armbruster P. A. (2014). Mosquito Diapause. Annu. Rev. Entomol. 59, 73–93. doi:10.1146/annurev-ento-011613-162023
Denlinger D. L. (2002). Regulation of Diapause. Annu. Rev. Entomol. 47, 93–122. doi:10.1146/annurev.ento.47.091201.145137
Denlinger D. L., Yocum G. D., Rinehart J. P. (2012). Hormonal Control of Diapause. Insect Endocrinol. 8, 430–463. doi:10.1016/B978-0-12-384749-2.10010-X
Dominguez C. V., Maestro J. L. (2018). Expression of Juvenile Hormone acidO-Methyltransferase and Juvenile Hormone Synthesis inBlattella Germanica. Insect Sci. 25, 787–796. doi:10.1111/1744-7917.12467
Evenden M. L., Armitage G., Lau R. (2007). Effects of Nutrition and Methoprene Treatment upon Reproductive Diapause in Caloptilia Fraxinella (Lepidoptera: Gracillariidae). Physiol. Entomol. 32, 275–282. doi:10.1111/j.1365-3032.2007.00581.x
Gu X. Y., Wei C., Feng Y., Guo J. J. (2017). Mating and Oviposition Rhythms of Aspongopus Chinensis Dallas. Chin. J. Appl. Entomol. 54, 121–125. doi:10.7679/j.issn.2095-1353.2017.014
Guo J. J., Tan J., Wei C., Feng Y., Jin D. J. (2019). Overwintering Conditions Optimization of Aspongopus Chinensis. J. Mountain Agric. Biol. 38, 71–74. doi:10.15958/j.cnki.sdnyswxb.2019.02.012
Guo S., Tian Z., Wu Q.-W., King-Jones K., Liu W., Zhu F., et al. (2021). Steroid Hormone Ecdysone Deficiency Stimulates Preparation for Photoperiodic Reproductive Diapause. Plos Genet. 17 (2), e1009352–28. doi:10.1371/journal.pgen.1009352
Hahn D. A., Denlinger D. L. (2011). Energetics of Insect Diapause. Annu. Rev. Entomol. 56, 103–121. doi:10.1146/annurev-ento-112408-085436
Hahn D. A., Denlinger D. L. (2007). Meeting the Energetic Demands of Insect Diapause: Nutrient Storage and Utilization. J. Insect Physiol. 53, 760–773. doi:10.1016/j.jinsphys.2007.03.018
Halarnkar P. P., Jackson G. P., Straub K. M., Schooley D. A. (1993). Juvenile Hormone Catabolism inManduca Sexta: Homologue Selectivity of Catabolism and Identification of a Diol-Phosphate Conjugate as a Major End Product. Experientia 49 (12), 988–994. doi:10.1007/BF02125646
Hao Z. P., Yuan Z. Q., Zhang J., Zhao J. R., Shi Z. H. (2012). Comparison of Hydrogen Peroxide, Antioxidant Enzymes and Hormones between Diapause and Non-diapause Prepupae of Cotesia Vestalis. Chin. J. Biol. Control. 28, 165–169. doi:10.3969/j.issn.2095-039X.2012.02.002
Herman W. S. (1981). Studies on the Adult Reproductive Diapause of the Monarch Butterfly,danaus Plexippus. Biol. Bull. 160 (1), 89–106. doi:10.2307/1540903
Hoffmann E. J., VanderJagt J., Whalon M. E. (2007). Pyriproxyfen Activates Reproduction in Prediapause Northern Strain Plum Curculio (Conotrachelus Nenuphar Herbst). Pest Manag. Sci. 63, 835–840. doi:10.1002/ps.1397
Hunt J. H., Kensinger B. J., Kossuth J. A., Henshaw M. T., Norberg K., Wolschin F., et al. (2007). A Diapause Pathway Underlies the Gyne Phenotype in Polistes Wasps, Revealing an Evolutionary Route to Caste-Containing Insect Societies. Proceedings of the National Academy of Sciences of the United States of America 104, 14020-25. doi:10.1073/pnas.0705660104
Jindra M., Palli S. R., Riddiford L. M. (2013). The Juvenile Hormone Signaling Pathway in Insect Development. Annu. Rev. Entomol. 58, 181–204. doi:10.1146/annurev-ento-120811-153700
Kopper B. J., Shu S., Charlton R. E., Ramaswamy S. B. (2001). Evidence for Reproductive Diapause in the Fritillary Speyeria Idalia (Lepidoptera: Nymphalidae). Physiology, Biochemistry And Toxicology 94, 427–432. doi:10.1603/0013-8746(2001)094
Kort C. A. D. (1990). Thirty-five Years of Diapause Research with the Colorado Potato Beetle. Entomologia Experimentalis et Applicata 56, 1–13. doi:10.1007/BF0057961010.1111/j.1570-7458.1990.tb01376.x
Lassiter M. T., Apperson C. S., Crawford C. L., Roe R. M. (1994). Juvenile Hormone Metabolism during Adult Development of Culex quinquefasciatus (Diptera: Culicidae). J. Med. Entomol. 31 (4), 586–593. doi:10.1093/jmedent/31.4.586
Lefevere K. S. (1989). Endocrine Control of Diapause Termination in the Adult Female Colorado Potato Beetle, Leptinotarsa decemlineata. J. Insect Physiol. 35, 197–203. doi:10.1016/0022-1910(89)90005-X
Liu W., Li Y., Zhu L., Zhu F., Lei C.-L., Wang X.-P. (2016). Juvenile Hormone Facilitates the Antagonism between Adult Reproduction and Diapause through the Methoprene-Tolerant Gene in the Female Colaphellus Bowringi. Insect Biochem. Mol. Biol. 74, 50–60. doi:10.1016/j.ibmb.2016.05.004
Livak K. J., Schmittgen T. D. (2001). Analysis of Relative Gene Expression Data Using Real-Time Quantitative PCR and the 2−ΔΔCT Method. Methods 25 (4), 402–408. doi:10.1006/meth.2001.1262
Marchal E., Zhang J., Badisco L., Verlinden H., Hult E. F., Van Wielendaele P., et al. (2011). Final Steps in Juvenile Hormone Biosynthesis in the Desert Locust, Schistocerca gregaria. Insect Biochem. Mol. Biol. 41, 219–227. doi:10.1016/j.ibmb.2010.12.007
Minakuchi C., Namiki T., Yoshiyama M., Shinoda T. (2008) RNAi-Mediated Knockdown of Juvenile Hormone Acid O-Methyltransferase Gene Causes Precocious Metamorphosis in the Red Flour Beetle Tribolium castaneum. FEBS J., 275(11), 2919–2931. dio: doi:10.1111/j.1742-4658.2008.06428.x
Miyawaki R., Tanaka S. I., Numata H. (2006) Role of Juvenile Hormone in the Control of Summer Diapause in Adult Poecilocoris Lewisi (Heteroptera: Scutelleridae). Formos. Entomologist, 26(1), 1–10. dio:doi:10.6661/TESFE.2006001
M.Th. Beenakkers A., Van der Horst D. J., Van Marrewijk W. J. A. (1985). Insect Lipids and Lipoproteins, and Their Role in Physiological Processes,and Their Role in Physiological Processes. Prog. lipid Res. 24, 19–67. doi:10.1016/0163-7827(85)90007-4
Pener M. P. (1992). Environmental Cues, Endocrine Factors, and Reproductive Diapause in Male Insects. Chronobiology Int. 9, 102–113. doi:10.3109/07420529209064521
Richard D. S., Jones J. M., Barbarito M. R., Stacy Cerula S., Detweiler J. P., Fisher S. J., et al. (2001). Vitellogenesis in Diapausing and Mutant Drosophila melanogaster: Further Evidence for the Relative Roles of Ecdysteroids and Juvenile Hormones. J. Insect Physiol. 47, 905–913. doi:10.1016/S0022-1910(01)00063-4
Richard D. S., Watkins N. L., Serafin R. B., Gilbert L. I. (1998). Ecdysteroids Regulate Yolk Protein Uptake by Drosophila melanogaster Oocytes. J. Insect Physiol. 44, 637–644. doi:10.1016/S0022-1910(98)00020-1
Share M. R., Roe R. M. (1988). A Partition Assay for the Simultaneous Determination of Insect Juvenile Hormone Esterase and Epoxide Hydrolase Activity. Anal. Biochem. 169 (1), 81–88. doi:10.1016/0003-2697(88)90257-6
Sim C., Denlinger D. L. (2013). Juvenile Hormone III Suppresses Forkhead of Transcription Factor in the Fat Body and Reduces Fat Accumulation in the Diapausing Mosquito,Culex pipiens. Insect Mol. Biol. 22 (1), 1–11. doi:10.1111/j.1365-2583.2012.01166.x
Smykal V., Raikhel A. S. (2015). Science Direct. Curr. Opin. Insect Sci. 37, 33. doi:10.1016/j.clinmicnews.2015.01.008
Tan J., Tian Y., Cai R., Luo R., Guo J. (2019a). Chemical Composition and Antiproliferative Effects of a Methanol Extract of Aspongopus Chinensis Dallas. Evidence-Based Complement. Altern. Med. 2019, 1–9. doi:10.1155/2019/2607086
Tan J., Tian Y., Cai R., Yi T., Jin D., Guo J. (2019b). Antiproliferative and Proapoptotic Effects of a Protein Component Purified from Aspongopus Chinensis Dallas on Cancer Cells In Vitro and In Vivo. Evidence-Based Complement. Altern. Med. 2019, 1–12. doi:10.1155/2019/8934794
Taylor L. R., Tauber M. J., Tauber C. A., Masaki S. (1987). Seasonal Adaptations of Insects. J. Anim. Ecol. 56, 374. doi:10.2307/4831
Toyomi K., Tetsuro S., Kanako K., Yasufumi O., Hideharu N. (2010). Biological Activities of Juvenile Hormone III Skipped Bisepoxide in Last Instar Nymphs and Adults of a Stink Bug, Plautia Stali. J. Insect Physiol. 57, 147–152. doi:10.1016/j.jinsphys.10.003
Wei C., Shu G. Z., Luo H. S., Guo J. J. (2015). Morphological and Biological Characteristics of Aspongonpus Chinensis. J. Mountain Agric. Biol. 34, 26–30. doi:10.15958/j.cnki.sdnyswxb.04.006
Wu Y., Tian Y., Tan J., Zhao S., Zhou W., Luo R., et al. (2021). Differential Metabolism of Juvenile Hormone III between Diapause and Non-diapause of Aspongopus Chinensis Dallas (Hemiptera: Dinidoridae) Revealed by Transcriptome Sequencing. J. Asia-Pacific Entomol. 24, 199–204. doi:10.1016/j.aspen.2021.02.009
Keywords: Aspongopus chinensis, JH, reproduction system, JHEH, JHAMT, diapause
Citation: Zhou W-Z, Wu Y-F, Yin Z-Y, Guo J-J and Li H-Y (2022) Juvenile Hormone Is an Important Factor in Regulating Aspongopus chinensis Dallas Diapause. Front. Physiol. 13:873580. doi: 10.3389/fphys.2022.873580
Received: 11 February 2022; Accepted: 01 April 2022;
Published: 09 May 2022.
Edited by:
Wen Liu, Huazhong Agricultural University, ChinaReviewed by:
Yifan Zhai, Shandong Academy of Agricultural Sciences, ChinaYong-Cheng Dong, Anhui Agricultural University, China
Copyright © 2022 Zhou, Wu, Yin, Guo and Li. This is an open-access article distributed under the terms of the Creative Commons Attribution License (CC BY). The use, distribution or reproduction in other forums is permitted, provided the original author(s) and the copyright owner(s) are credited and that the original publication in this journal is cited, in accordance with accepted academic practice. No use, distribution or reproduction is permitted which does not comply with these terms.
*Correspondence: Jian-Jun Guo, ampndW9AZ3p1LmVkdS5jbg==; Hai-Yin Li, aHlsaTNAZ3p1LmVkdS5jbg==
†These authors share first authorship