- 1Department of Pediatrics, Johns Hopkins University School of Medicine, Baltimore, MD, United States
- 2Department of Pediatrics, Affiliated Hospital of Jiangnan University, Wuxi, China
- 3Department of Cellular and Molecular Physiology, Johns Hopkins University School of Medicine, Baltimore, MD, United States
- 4Department of Pediatrics, Rutgers University Medical School, New Brunswick, NJ, United States
- 5Department of Cardiovascular Sciences, Center for Metabolic Disease Research, Temple University School of Medicine, Philadelphia, PA, United States
- 6Department of Pediatrics, University of Washington, Seattle’s Children’s Hospital, Seattle, United States
The kisspeptin receptor, crucial for hypothalamic control of puberty and reproduction, is also present in the pituitary gland. Its role in the pituitary gland is not defined. Kisspeptin signaling via the Kiss1r could potentially regulate reproductive function at the level of pituitary gonadotrope. Using Cre/Lox technology, we deleted the Kiss1r gene in pituitary gonadotropes (PKiRKO). PKiRKO males have normal genital development (anogenital distance WT: 19.1 ± 0.4 vs. PKiRKO: 18.5 ± 0.4 mm), puberty onset, testes cell structure on gross histology, normal testes size, and fertility. PKiRKO males showed significantly decreased serum FSH levels compared to WT males (5.6 ± 1.9 vs. 10.2 ± 1.8 ng/ml) with comparable LH (1.1 ± 0.2 vs. 1.8 ± 0.4 ng/ml) and testosterone levels (351.8 ± 213.0 vs. 342.2 ± 183.0 ng/dl). PKiRKO females have normal puberty onset, cyclicity, LH and FSH levels and fertility. Overall, these findings indicate that absence of pituitary Kiss1r reduces FSH levels in male mice without affecting testis function. PKiRKO mice have normal reproductive function in both males and females.
Introduction
Kisspeptin and its G-protein coupled receptor (KISS1R/GPR54) have a critical role in regulating the hypothalamic control of puberty and reproduction in all mammalian species tested to date (de Roux et al., 2003; Funes et al., 2003; Gottsch et al., 2004; Messager et al., 2005; Teles et al., 2008; Dror et al., 2013; Witham et al., 2013; Martins Trevisan et al., 2020; Padda et al., 2021). In rodents, kisspeptin is highly expressed in hypothalamic anteroventral periventricular nucleus (AVPV) and arcuate nucleaus regions (ARC) (Seminara, 2005; Clarkson and Herbison, 2006; Martins Trevisan et al., 2020). Kiss1r is expressed in hippocampus, septum, rostral preoptic area (rPOA), anteroventral nucleus of the thalamus, and throughout the arcuate nucleus (Kauffman et al., 2007; Herbison et al., 2010; Higo et al., 2016; Ozaki et al., 2019). Conditional knock-out studies defined the crucial role of Kiss1r receptor signaling in the GnRH neuron for normal reproductive development and fertility (Clarkson et al., 2009; Novaira et al., 2014). Kiss1r is also expressed in much smaller amounts in the pituitary (Gutiérrez-Pascual et al., 2007; Witham et al., 2013) as well as in the placenta, liver, pancreas and intestine (Brown et al., 2008; Song et al., 2014; de Pedro et al., 2015).
The pituitary incorporates diverse cues and signals to affect gonadotropin (luteinizing hormone and follicle stimulating hormone) release including estradiol (Singh et al., 2009), testosterone (Ramaswamy et al., 2007), progesterone (Girmus and Wise, 1992), metabolic hormones such as insulin (Williams et al., 1987; Brothers et al., 2010). Hypothalamus derived factors including GnRH travel from the hypothalamic median eminence to the pituitary using the portal venous system. Kisspeptin is released to the circulation and has potential to activate KISS1R signaling cascades of pituitary cells.
Kisspeptin increases gonadotropin gene expression in mouse primary pituitary cells in culture (Witham et al., 2013). Kiss1r expression is enhanced in the pituitary of female mice during the estradiol-induced LH surge (Clarkson et al., 2008), suggesting a possible role for kisspeptin as part of the constellation of regulatory inputs to the pituitary gonadotroph required for the preovulatory release of gonadotropins. We sought to examine the physiological role of pituitary gonadotroph kisspeptin receptor in vivo using a novel kisspeptin receptor knockout mouse (PKiRKO mouse). In male mice with absence of the gonadotroph kisspeptin receptor, FSH levels are lower than wild type mice, gonads have normal size and hormone production, and fertility is normal.
Materials and Methods
Generation of Gonadotrope-Specific Kiss1R Knockout Mice (PKiRKO)
To generate pituitary Kiss1 receptor (Kiss1R) knockout (PKiRKO) mice, we crossed Kiss1r heterozygous (fl/wt) female mice (Novaira et al., 2014) with αGSU transgenic (αCre+/-) male (Naik et al., 2006; Brothers et al., 2010) mice. The αGSU transgenic (αCre+/-) effectively deletes floxed genes specifically in pituitary gonadotrophs and thyrotropes (Brothers et al., 2010). F1: female mice (Kiss1R fl/wt; αCre+) and male mice (Kiss1R fl/fl; αCre-) were crossed to produce PKiRKO mice (Kiss1R fl/fl; αCre+). Litter mates (Kiss1r fl/wt; αCre- and Kiss1r fl/fl; αCre-) were used as controls (referred as WT). DNA was extracted as described previously (Wolfe et al., 2008). Genotyping primers were designed to detect the presence of αCre and the floxed allele, WT allele, or knockout allele of Kiss1r: P1 sense (located in exon 1) and P3 antisense (located in exon 3). PCR of tail genomic DNA using primers P1 and P3 will amplify a 2096-bp amplicon to indicate the floxed Kiss1r allele and 1882-bp amplicon to indicate the WT allele. Genomic DNA obtained from the pituitary using primers P1 and P3 will amplify a 1120-bp amplicon if the sequence between the LoxP sites is excised indicating a KO allele. Primer set for αCre is αCre F: GCCACCACCGCCCTGCTTAAGTAA; R: GCCACCACCGCCCTGCTTAAGTAA); for Kiss1r is P1: CTGGTCGGAAACTCATTGGT; P3: AGAGTGGCACATGTGGCTTG.
Animals
Adult male and female mice (>2 months old) were used in this study. All animal studies were carried out in accordance with National Institutes of Health guidelines on animal care regulations and were approved by the Animal Care and Use Committee of the Johns Hopkins University. Mice were maintained under constant conditions of light and temperature (14: 10 h light⁄dark cycle; 22 C) and were fed a normal chow and water ad libitum.
Quantitative Real-Time PCR (qPCR)
RNA was extracted from the pituitary, liver, ovaries, uterus, testes, epididymis, adipose tissue and from two hypothalamic fragments encompassing the arcuate and the anteroventral periventricular nucleus (AVPV) (Quennell et al., 2011), using TRIzol reagent (Ambion life technologies, Carlsbad CA, United States ), according to the protocol provided by the supplier. 1µg of RNA was reverse transcribed to cDNA using an iScript cDNA kit (Bio-Rad Laboratories). Real-time qPCR was performed to determine the presence and relative expression levels of Kiss1r mRNA in the various tissues. Real-time qPCR was performed in duplicate using SYBR Green Master Mix (Bio-Rad Laboratories) and the CFX Connect qPCR machine (Bio-Rad Laboratories). Kiss1r primer sequences are Sense: CTGCCACAGACGTCACTTTC; antisense, 5-ACATACCAGCGGTCCACACT. For each primer, PCR efficiency was determined by measuring a 10-fold serial dilutions of cDNA and reactions having 95 and 105% PCR efficiency were included in subsequent analyses. Relative differences in cDNA concentration between WT and PKiRKO mice were then calculated using the comparative threshold cycle (Ct) method. To compare the difference of Kiss1r expression in the same tissue between WT and PKiRKO, a △Ct was calculated to normalize for internal control using the equation: Ct (Kiss1R)—Ct (18S). △△Ct was calculated: △Ct (PKiRKO) -△Ct (WT). Relative Kiss1R mRNA levels were then calculated using the equation fold difference = 2△△Ct.
Pubertal Onset and Assessment
Preputial separation (PPS) in males was assessed daily beginning after postnatal day 21 by determining whether the prepuce could be manually retracted with gentle pressure. PPS is testosterone dependent and thus is an indicator of activation of the reproductive axis in males. Puberty in rodents is dependent on body weight (Baker, 1985) hence, Body weight (BW) of PKiRKO and wild type were assessed once a week in prepuberty (day 21) through adulthood (day 49). Anogenital distance is testosterone dependent and was determined at 8 weeks of age. Wet testicular weights were determined in freshly dissected mice at 2 months of age. In females, age at vaginal opening and first estrus are two markers of puberty onset and were assessed for daily in mice after 21 days of life until achieved. Vaginal smears were obtained daily over a period of 14 consecutive days in 6- to 11-week-old mice and cellular morphology examined under microscope. BW was also recorded at the age of PPS and first estrus.
Hormone Assays
Blood samples were collected from submandibular vein (Wu et al., 2014; Wang et al., 2019) between 9:00 and 10:00 a.m. and basal levels of serum LH and FSH were measured. LH and FSH were measured using a Milliplex MAP immunoassay (Mouse Pituitary panel; Millipore, Massachusetts) on a Luminex 200IS platform (Luminex Corporation). The assay detection limit for LH was 0.012 ng/ml and for FSH was 0.061 ng/ml. The intra-assay and interassay coefficients of variation (CV) for LH and FSH were between 5 and 9%. Testosterone levels were measured by radioimmunoassay by the University of Virginia Ligand Assay Core (Charlottesville, Virginia) and the intra-assay CV was 5% and inter-assay CV was 9%.
Fertility Assessment
To determine whether male PKiRKO mice were fertile relative to controls, fertility was examined in WT and PKiRKO mice using a rotating mating protocol. 3 WT and 4 PKiRKO male mice were housed individually with proven fertile WT female mice for 14 consecutive days and then were separated. If a pregnancy ensued, 1 week after female gave birth, a new male was inserted. Males were rotated among the proven females. To assess fertility in females, a similar strategy was used. Females were housed individually with proven fertile WT male mice for 14 consecutive days and then separated. If a pregnancy ensued, 1 week after female gave birth, a new male was inserted, rotating among the seven proven males. The duration of the fertility study was 4 months with four rotations for each mouse.
Data Analysis
Data were analyzed by two tailed unpaired student t tests (parametric) with normality of residuals test Shapiro-Wilk (W)) using Prism software (GraphPad Software, Inc., La Jolla, CA). All results are expressed as mean ± SEM (standard error of the mean). p < 0.05 was defined as statistically significant.
Results
Generation of Pituitary Specific Kiss1r Knockout Mice
PKiRKO (Kiss1Rfl/fl; αCre+) mice were generated by Cre recombinase mediated excision of exon 2 of the Kiss1r, resulting in an attenuated Kiss1r gene in pituitary, as shown in the schematic diagram of Figure 1A. The PCR product of genotyping indicates the homozygous floxed-Kiss1r alleles (2096 bp) and WT alleles (1882 bp) (Figure 1B); both bands are present in the heterozygous floxed-Kiss1r mouse schematized by Novaira HJ et al. (Novaira et al., 2014). Shown in Figure 1C Lane 1 is a WT αCre-male mice, followed by two αCre + male mice in lanes 2 and 3 showed by PCR of genotyping.
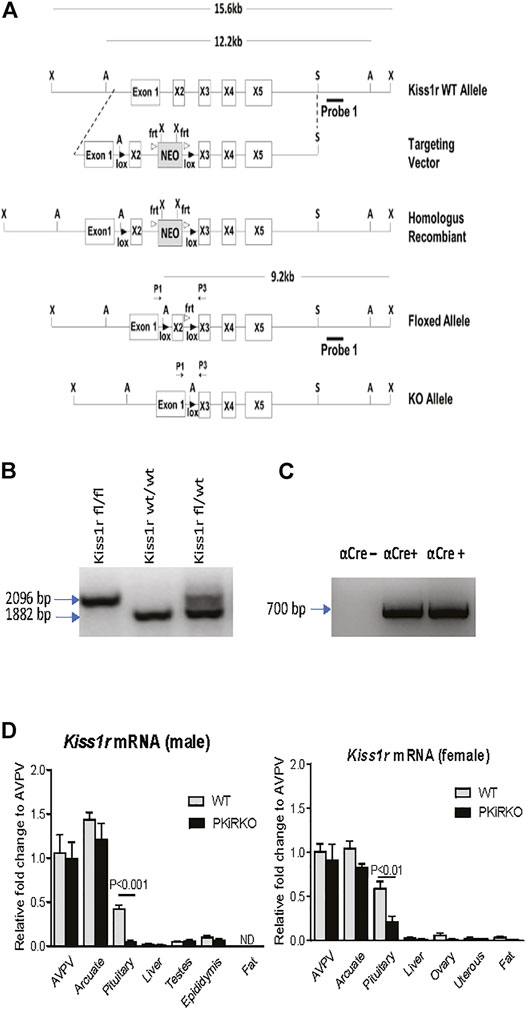
FIGURE 1. Development of the PKiRKO mouse. (A) schematic diagrams of constructs used to generate PKiRKO mice. Mice bearing LoxP sites flanking exon 2 of the Kiss1r were crossed with transgenic mice expressing Cre recombinase specifically in Gonadotrophs (αGSU). (B) Genotyping by PCR analysis of the genomic DNA produced a 2096 bp amplicon in mice with a floxed allele and an 1882 bp amplicon in kisspeptin fl/fl mice, also shown are both bands present in the heterozygous floxed-Kiss1r. (C) Lane 1-αCre+ transgene negative. Lane 2 & 3- αCre+ transgene positive mice. Amplicon size is 700 bp. (D) Quantitative RT-PCR analysis of Kiss1r mRNA extracted from male and female mouse tissues. Kiss1r was significantly reduced (87.6% for male, 63.7% for female) in the pituitary of PKiRKO (KO) mice compared with that in wild type (WT) mice, but no difference in Kiss1r expression was observed in other tissues. ** = p < 0.01. *** = p < 0.001. Data are means ± SEM (n = 8 for pituitary, n = 4 for other tissues).
Pituitary and Tissue Kiss1r mRNA Expression in PKiRKO Mice
qPCR demonstrated a reduction in Kiss1r mRNA by 88% (Figure 1D) and 64% (Figure 1D) in the pituitary of male and female PKiRKO mice (n = 8), respectively, compared with WT mice (n = 8). In contrast, no difference in Kiss1r mRNA was observed between PKiRKO and control animals in other tissues including the hypothalamus, adipose tissue, liver, or gonads (p > 0.05, n = 4) (Figure 1D). There was more Kiss1r mRNA detected in the pituitaries of female PKiRKO mice.
Pubertal Onset and Reproductive Function Are Normal in PKiRKO Mice
Body weight (BW) was not different between male or female PKiRKO and WT mice (Figure 2A). In males, PPS (preputial separation) is an androgen dependent process that serves as an external sign of male puberty onset (Marty et al., 2001). No significant difference was observed age of PPS between the WT and PKiRKO groups ((28.1 ± 0.6 vs. 27.8 ± 0.6 days of life), Figure 2B), or achieved anogenital distance (Figure 2C). The age of vaginal opening and first estrus (Figure 2D), and anogenital distance (Figure 2E) was not different between female WT and PKiRKO groups. The BW at age of pubertal achievement (PPS at day 28 in males (BW: 14 ± 1 vs. 15 ± 1 g) and first estrous at day 35 in females (BW: 18 ± 1 vs. 16 ± 1 g) was not significantly different between WT and PKiRKO animals. In sexually mature mice, gonad weight is a marker of reproductive tissue function. Gonad weights were comparable between PKiRKO and WT male and female mice (Figure 3 A and B). Estrous cycling pattern was similar between PKiRKO and WT mice (Figure 3C); percent time spent at each cycle stage was not significantly different. Testes (Figure 3D) or ovary (Figure 3E) histology was not different between PKiRKO and WT mice. The morphology of seminiferous tubules were comparable between WT and PKiRKO male mice (Figure 3D). The number of sperm in the seminiferous tubules was not statistically different between WT and PKiRKO mice (data not shown).
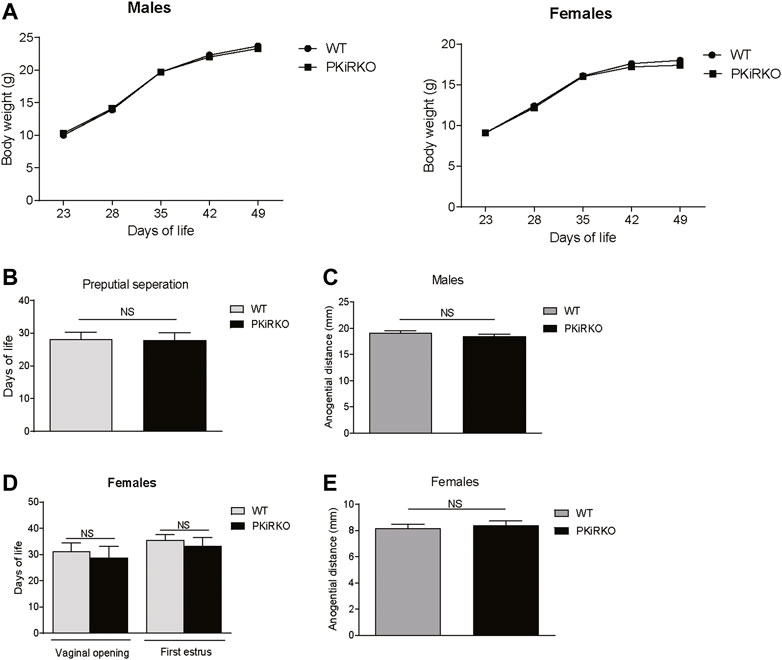
FIGURE 2. PKiIRKO mice have normal puberty onset. (A) Body weight change over time was not different between PKiRKO (n = 15) and WT (n = 15) male mice or female PKiRKO (n = 15) and WT (n = 15) mice. (B) No significant difference was observed in age of preputial separation (PPS) in males. WT (n = 15) PKiRKO (n = 15). (C) Anogenital distance was not different between WT and PKiRKO male mice (n = 6 each group). (D) No difference was observed in age at vaginal opening or first estrus. (E) Anogenital distance was not different between WT and PKiRKO female mice.
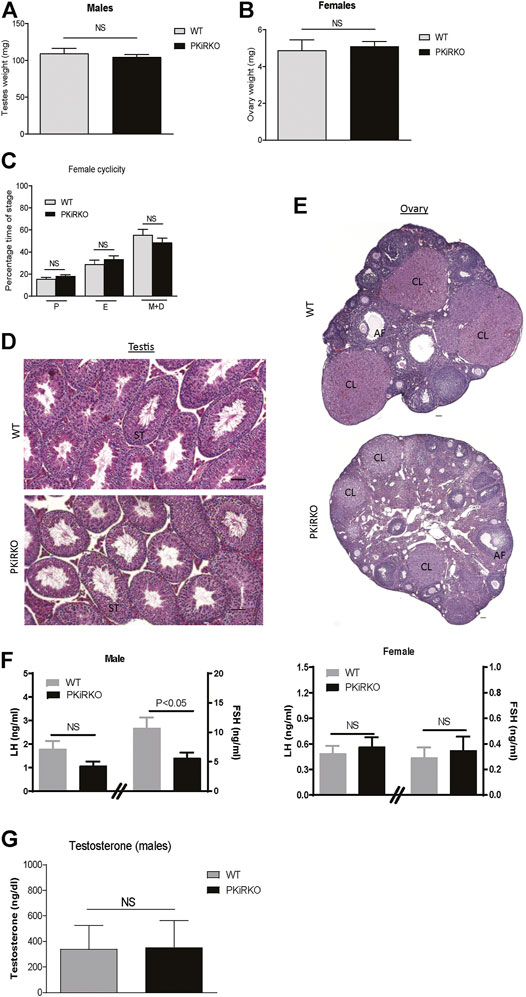
FIGURE 3. PKiRKO mice have normal reproductive function. (A). Testes weight was not significantly different between PKiRKO and WT male mice (n = 6). (B) Ovary weight was not significantly different between PKiRKO and WT female mice (n = 6). (C) Vaginal cytology was examined daily over a period of 14 consecutive days in 6- to 11-week-old mice. WT and PKiRKO groups both exhibited regular estrous cycles the percentage time spent at each cycle stage did not differ between groups. N = 6 each group. (D) Testis structure between WT and PKiRKO by H&E staining. ST: seminiferous tubule. (E) ovary structure between WT and PKiRKO. CL: copora lutea; AF: antral follicles. There was comparable testis and ovary structure. (F) Morning LH (left Y axis and FSH (right Y axis) in male (left panel) and female (right panel) mice. n = 6, p > 0.05) (G) Testosterone levels are not different between PKiRKO and WT mice (n = 6 each group). NS = No significant difference.
Basal serum LH levels in PKiRKO males (1.8 ± 0.4 vs. 1.1 ± 0.2 ng/ml) or females (0.5 ± 0.1 vs. 0.6 ± 0.1 ng/ml) were not significantly different relative to WT mice, while FSH levels (10.2 ± 1.8 vs. 6.0 ± 1.9 ng/ml) were significantly lower in PKiRKO males (Figure 3F). Testosterone levels were not significantly different between WT and PKiRKO mice (Figure 3G).
Normal Fertility in PKiRKO Mice
PKiRKO male and female mice demonstrated a normal ability to produce offspring, as shown in Figure 4 A-C. Female WT mice bore their first litter with a similar latency after introduction to PKiRKO males or WT males ((22.0 ± 0.8days, n = 4 (PKiRKO) vs. 22.3 ± 0.6 days, n = 3(WT); p > 0.05)) (Figure 4A). Female WT mice had the same number of litters with PKiRKO and WT sires ((3.8 ± 0.5, n = 4 (PKiRKO) vs. 3.7 ± 0.6, n = 3 (WT); p > 0.05)) (Figure 4B) and a similar number of pups per litter ((7.4 ± 1.4, n = 4 (PKiRKO) vs. 9.3 ± 1.1, n = 3 (WT); p > 0.05)) (Figure 4C). Similar results were found between PKiRKO females and WT females. Female WT and PKiRKO mice bore their first litter with a similar latency after introduction to WT males ((22.4 ± 0.1 day, n = 6 (PKiRKO) vs. 22.6 ± 0.1 day, n = 7(WT); p > 0.05)) (Figure 4A, right panel). Female WT and PKiRKO mice had the same number of litters with WT males ((3.3 ± 0.2, n = 6 (PKiRKO) vs. 3.7 ± 0.2, n = 6 (WT); p > 0.05)) (Figure 4B, right panel) and a similar number of pups per litter ((8.3 ± 0.6, n = 6 (PKiRKO) vs. 9.7 ± 1.0, n = 6 (WT); p > 0.05)) (Figure 4C, right panel).
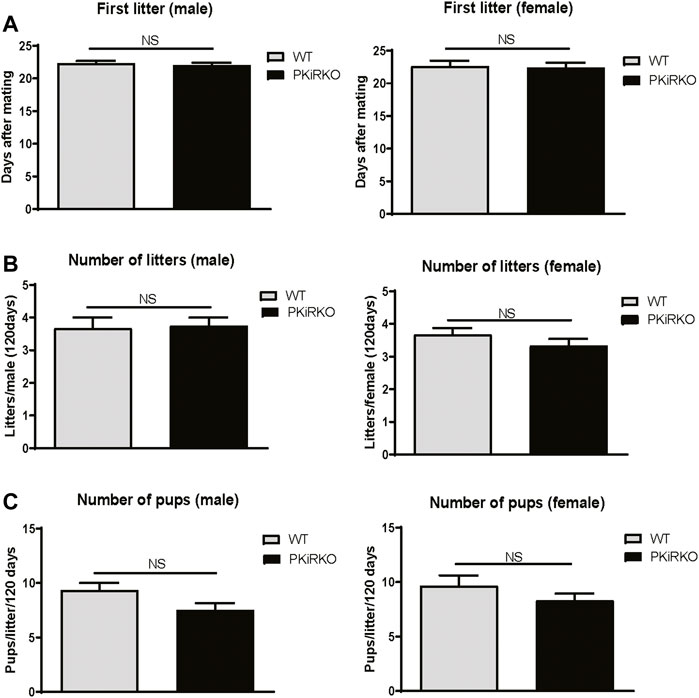
FIGURE 4. PKiRKO Mice Have Normal Fertility. (A) After introduction with WT female (left panel) or male (right panel) respectively, the day of first litter was recorded. Values are mean ± S.E.M. NS, no significant. (B) Total numbers of litters per male (left panel) or female (right panel) was not significantly different between WT and PKiRKO mice. (C) Number of pups per litter was also not significantly different between WT and PKiRKO mice (male, left panel; female right panel).
Discussion
Expression of KISS1R in the pituitary gonadotroph (Gutiérrez-Pascual et al., 2007) implicates the pituitary gonadotroph as a possible target for kisspeptin action, in addition to its well described role in GnRH neuron function. The functional significance of pituitary and gonadotroph KISS1R is not well defined and may be the target of either centrally or peripherally derived KISS1. We sought to assess the role of KISS1 signaling at the level of the pituitary gonadotroph in mice by the development of a gonadotroph Kiss1 knockout model (PKiRKO). We demonstrate that near complete reduction of pituitary Kiss1r expression in males is associated with lower FSH levels in males with normal gonad function and reproductive function. Similar fertility results were also found between PKiRKO females and WT females. The pituitary Kiss1r signaling is not essential for reproduction. This is indirectly supported by recently published paper which showed Kiss1 rescue in the KNDy neurons restored LH pulses and follicular development in female global Kiss1 knockout rats. (Nagae et al., 2021).
Qualitative PCR analysis indicated that the pituitary gland had the most amount of Kiss1r expression outside of the hypothalamus (Figure 1D). Kiss1r mRNA expression is detectable at low levels in tissues such as ovary, liver and brown adipose tissues with defined physiological roles for Kiss1r (Song et al., 2014; Tolson et al., 2020; Ruohonen et al., 2022). Kiss1r is expressed on mouse pituitary gonadotrophs in rat (Richard et al., 2008) and sheep (Backholer et al., 2009). The PKiRKO mice had an 88% reduction in Kiss1r gene expression in the pituitaries of male mice and 64% in female mice. The gonadotroph comprises a minority of the pituitary cell population so kisspeptin receptor expression in other pituitary cells may account for the observed residual expression in the gonadotrope specific KO mice. Indeed, the kisspeptin receptor has been colocalized to ovine and goldfish lactotrophs and somatotrophs (Smith et al., 2008; Yang et al., 2010). The αGSU transgenic Cre mouse effectively deletes floxed genes in pituitary gonadotrophs (Brothers et al., 2010) indicating that the residual expression of Kiss1r in the pituitary of PKirKO may be not be in the gonadotroph.
The PKiRKO male mice had a significantly lower morning FSH level (60% of WT, Figure 3F). This lower FSH did not seem to affect reproductive function, as the PKiRKO mice also had normal testosterone, testes cell structure on gross histology, and normal testes size (Figure 3). Global null Kiss1r male mice exhibit infertility, undetectable LH and testosterone with rescue of, spermatogenesis upon testosterone supplementation. FSH levels were 10% of WT males (Goto et al., 2020). The lack of LH and testosterone together with extremely low FSH levels accounted for the differences in spermatogenesis and testicular development in these males. Mice with complete absence of FSH exhibit decreased testes size and sperm numbers and motility (Kumar et al., 1997); Therefore, there may be a threshold FSH needed for normal spermatogenesis and fertility. FSH levels in PKiRKO male mice were 60% of WT so possibly above a threshold and thus accounted for the lack of dramatic phenotype in the PKiRKO mice. However, long term effects of lower FSH on testis maybe very subtle and need to be carefully examined in the future.
Investigators have observed KISS1 induction of LH secretion in cultured primary rat and primate pituitary cells (Navarro et al., 2005; Luque et al., 2011) derived from females and up-regulation of gonadotropin gene β-subunits, LHβ and FSHβ gene expression in LβT2 cells (Witham et al., 2013; Goto et al., 2020), although others have seen no direct effect of KISS1 on LH or FSH secretion (Matsui et al., 2004; Thompson et al., 2004). Studies suggest that KISS1 may act directly on pituitary gonadotropes to stimulate LH release (Castellano et al., 2006; Gutiérrez-Pascual et al., 2007; Luque et al., 2011) or to increase gonadotropin or Gnrhr gene expression (Naor et al., 1980) were observed in vitro using cell lines or primary culture. The complex hormonal milieu of the in vivo pituitary may account for the lack of difference in LH and fertility between the control and PKiRKO animals in this study.
The LH and FSH response to peripheral (Matsui et al., 2004) and central (Messager et al., 2005) administration of kisspeptin is used to assess GnRH neuron activity. The LH and FSH of humans and mice with disorders of GnRH neuron causing hypogonadism do not rise after peripheral kisspeptin administration (Chan et al., 2014). These data agree with our results that there does not appear to be a critical role for kisspeptin receptor on gonadotrope regulation of LH secretion, and gonadal function.
Overall this study suggests that absence of kisspeptin signaling in pituitary gonadotrophs affects FSH levels in males without affecting testes function or fertility in both male and female mice.
Data Availability Statement
The original contributions presented in the study are included in the article/Supplementary Materials, further inquiries can be directed to the corresponding author.
Ethics Statement
The animal study was reviewed and approved by Animal Care and Use Committee of the Johns Hopkins University.
Author Contributions
YM and OA equally contribute the manuscript. YM, OA, SR, XY, SD, AW and SW contributed to the conceptual design, performance of experiments, interpretation, and analysis of data, and writing and editing the manuscript.
Funding
This work was supported by the National Institutes of Health (Grants R00-HD068130 04 and R01-HD09551201A1 to SW; U01-HD066432-05, R01-HD068777-01A1 and R01-DK101591-04 to AW).
Conflict of Interest
The authors declare that the research was conducted in the absence of any commercial or financial relationships that could be construed as a potential conflict of interest.
Publisher’s Note
All claims expressed in this article are solely those of the authors and do not necessarily represent those of their affiliated organizations, or those of the publisher, the editors, and the reviewers. Any product that may be evaluated in this article, or claim that may be made by its manufacturer, is not guaranteed or endorsed by the publisher.
References
Backholer K., Smith J., Clarke I. J. (2009). Melanocortins May Stimulate Reproduction by Activating Orexin Neurons in the Dorsomedial Hypothalamus and Kisspeptin Neurons in the Preoptic Area of the Ewe. Endocrinology 150, 5488–5497. doi:10.1210/en.2009-0604
Baker E. R. (1985). Body Weight and the Initiation of Puberty. Clin. Obstet. Gynecol. 28, 573–579. doi:10.1097/00003081-198528030-00013
Brothers K. J., Wu S., DiVall S. A., Messmer M. R., Kahn C. R., Miller R. S., et al. (2010). Rescue of Obesity-Induced Infertility in Female Mice Due to a Pituitary-specific Knockout of the Insulin Receptor. Cel Metab. 12, 295–305. doi:10.1016/j.cmet.2010.06.010
Brown R. E., Imran S. A., Ur E., Wilkinson M. (2008). KiSS-1 mRNA in Adipose Tissue Is Regulated by Sex Hormones and Food Intake. Mol. Cell Endocrinol. 281, 64–72. doi:10.1016/j.mce.2007.10.011
Castellano J. M., Navarro V. M., Fernández-Fernández R., Castaño J. P., Malagón M. M., Aguilar E., et al. (2006). Ontogeny and Mechanisms of Action for the Stimulatory Effect of Kisspeptin on Gonadotropin-Releasing Hormone System of the Rat. Mol. Cell Endocrinol. 257-258, 75–83. doi:10.1016/j.mce.2006.07.002
Chan Y.-M., Lippincott M. F., Butler J. P., Sidhoum V. F., Li C. X., Plummer L., et al. (2014). Exogenous Kisspeptin Administration as a Probe of GnRH Neuronal Function in Patients with Idiopathic Hypogonadotropic Hypogonadism. J. Clin. Endocrinol. Metab. 99, E2762–E2771. doi:10.1210/jc.2014-2233
Clarkson J., d'Anglemont de Tassigny X., Moreno A. S., Colledge W. H., Herbison A. E. (2008). Kisspeptin-GPR54 Signaling Is Essential for Preovulatory Gonadotropin-Releasing Hormone Neuron Activation and the Luteinizing Hormone Surge. J. Neurosci. 28, 8691–8697. doi:10.1523/JNEUROSCI.1775-08.2008
Clarkson J., d’Anglemont de Tassigny X., Colledge W. H., Caraty A., Herbison A. E. (2009). Distribution of Kisspeptin Neurones in the Adult Female Mouse Brain. J. Neuroendocrinol 21, 673–682. doi:10.1111/j.1365-2826.2009.01892.x
Clarkson J., Herbison A. E. (2006). Postnatal Development of Kisspeptin Neurons in Mouse Hypothalamus; Sexual Dimorphism and Projections to Gonadotropin-Releasing Hormone Neurons. Endocrinology 147, 5817–5825. doi:10.1210/en.2006-0787
de Pedro M. A., Morán J., Díaz I., Murias L., Fernández- Plaza C., González C., et al. (2015). Circadian Kisspeptin Expression in Human Term Placenta. Placenta 36, 1337–1339. doi:10.1016/j.placenta.2015.09.009
de Roux N., Genin E., Carel J.-C., Matsuda F., Chaussain J.-L., Milgrom E. (2003). Hypogonadotropic Hypogonadism Due to Loss of Function of the KiSS1-Derived Peptide Receptor GPR54. Proc. Natl. Acad. Sci. U.S.A. 100, 10972–10976. doi:10.1073/pnas.1834399100
Dror T., Franks J., Kauffman A. S. (2013). Analysis of Multiple Positive Feedback Paradigms Demonstrates a Complete Absence of LH Surges and GnRH Activation in Mice Lacking Kisspeptin Signaling. Biol. Reprod. 88, 146. doi:10.1095/biolreprod.113.108555
Funes S., Hedrick J. A., Vassileva G., Markowitz L., Abbondanzo S., Golovko A., et al. (2003). The KiSS-1 Receptor GPR54 Is Essential for the Development of the Murine Reproductive System. Biochem. Biophysical Res. Commun. 312, 1357–1363. doi:10.1016/j.bbrc.2003.11.066
Girmus R. L., Wise M. E. (1992). Progesterone Directly Inhibits Pituitary Luteinizing Hormone Secretion in an Estradiol-dependent Manner1. Biol. Reprod. 46, 710–714. doi:10.1095/biolreprod46.4.710
Goto T., Hirabayashi M., Watanabe Y., Sanbo M., Tomita K., Inoue N., et al. (2020). Testosterone Supplementation Rescues Spermatogenesis and In Vitro Fertilizing Ability of Sperm in Kiss1 Knockout Mice. Endocrinology 161, bqaa092. doi:10.1210/endocr/bqaa092
Gottsch M. L., Cunningham M. J., Smith J. T., Popa S. M., Acohido B. V., Crowley W. F., et al. (2004). A Role for Kisspeptins in the Regulation of Gonadotropin Secretion in the Mouse. Endocrinology 145, 4073–4077. doi:10.1210/en.2004-0431
Gutiérrez-Pascual E., Martínez-Fuentes A. J., Pinilla L., Tena-Sempere M., Malagón M. M., Castaño J. P. (2007). Direct Pituitary Effects of Kisspeptin: Activation of Gonadotrophs and Somatotrophs and Stimulation of Luteinising Hormone and Growth Hormone Secretion. J. Neuroendocrinol 19, 521–530. doi:10.1111/j.1365-2826.2007.01558.x
Herbison A. E., d'Anglemont de Tassigny X., Doran J., Colledge W. H. (2010). Distribution and Postnatal Development of Gpr54 Gene Expression in Mouse Brain and Gonadotropin-Releasing Hormone Neurons. Endocrinology 151, 312–321. doi:10.1210/en.2009-0552
Higo S., Honda S., Iijima N., Ozawa H. (2016). Mapping of Kisspeptin Receptor mRNA in the Whole Rat Brain and its Co-localisation with Oxytocin in the Paraventricular Nucleus. J. Neuroendocrinol 28, 1–8. doi:10.1111/jne.12356
Kauffman A. S., Park J. H., McPhie-Lalmansingh A. A., Gottsch M. L., Bodo C., Hohmann J. G., et al. (2007). The Kisspeptin Receptor GPR54 Is Required for Sexual Differentiation of the Brain and Behavior. J. Neurosci. 27, 8826–8835. doi:10.1523/JNEUROSCI.2099-07.2007
Kumar T. R., Wang Y., Lu N., Matzuk M. M. (1997). Follicle Stimulating Hormone Is Required for Ovarian Follicle Maturation but Not Male Fertility. Nat. Genet. 15, 201–204. doi:10.1038/ng0297-201
Luque R. M., Córdoba-Chacón J., Gahete M. D., Navarro V. M., Tena-Sempere M., Kineman R. D., et al. (2011). Kisspeptin Regulates Gonadotroph and Somatotroph Function in Nonhuman Primate Pituitary via Common and Distinct Signaling Mechanisms. Endocrinology 152, 957–966. doi:10.1210/en.2010-1142
Martins Trevisan C., Naslavsky M. S., Monfardini F., Wang J., Zatz M., Peluso C., et al. (2020). Variants in the Kisspeptin-GnRH Pathway Modulate the Hormonal Profile and Reproductive Outcomes. DNA Cel Biol. 39, 1012–1022. doi:10.1089/dna.2019.5165
Marty M. S., Crissman J. W., Carney E. W. (2001). Evaluation of the Male Pubertal Onset Assay to Detect Testosterone and Steroid Biosynthesis Inhibitors in CD Rats. Toxicol. Sci. 60, 285–295. doi:10.1093/toxsci/60.2.285
Matsui H., Takatsu Y., Kumano S., Matsumoto H., Ohtaki T. (2004). Peripheral Administration of Metastin Induces Marked Gonadotropin Release and Ovulation in the Rat. Biochem. Biophysical Res. Commun. 320, 383–388. doi:10.1016/j.bbrc.2004.05.185
Messager S., Chatzidaki E. E., Ma D., Hendrick A. G., Zahn D., Dixon J., et al. (2005). Kisspeptin Directly Stimulates Gonadotropin-Releasing Hormone Release via G Protein-Coupled Receptor 54. Proc. Natl. Acad. Sci. U.S.A. 102, 1761–1766. doi:10.1073/pnas.0409330102
Nagae M., Uenoyama Y., Okamoto S., Tsuchida H., Ikegami K., Goto T., et al. (2021). Direct Evidence that KNDy Neurons Maintain Gonadotropin Pulses and Folliculogenesis as the GnRH Pulse Generator. Proc. Natl. Acad. Sci. U.S.A. 118, e2009156118. doi:10.1073/pnas.2009156118
Naik K., Pittman I., Wolfe A., Miller R. S., Radovick S., Wondisford F. E. (2006). A Novel Technique for Temporally Regulated Cell Type-specific Cre Expression and Recombination in the Pituitary Gonadotroph. J. Mol. Endocrinol. 37, 63–69. doi:10.1677/jme.1.02053
Naor Z., Clayton R. N., Catt K. J. (1980). Characterization of Gonadotropin-Releasing Hormone Receptors in Cultured Rat Pituitary Cells. Endocrinology 107, 1144–1152. doi:10.1210/endo-107-4-1144
Navarro V. M., Castellano J. M., Fernández-Fernández R., Tovar S., Roa J., Mayen A., et al. (2005). Characterization of the Potent Luteinizing Hormone-Releasing Activity of KiSS-1 Peptide, the Natural Ligand of GPR54. Endocrinology 146, 156–163. doi:10.1210/en.2004-0836
Novaira H. J., Sonko M. L., Hoffman G., Koo Y., Ko C., Wolfe A., et al. (2014). Disrupted Kisspeptin Signaling in GnRH Neurons Leads to Hypogonadotrophic Hypogonadism. Mol. Endocrinol. 28, 225–238. doi:10.1210/me.2013-1319
Ozaki S., Higo S., Iwata K., Saeki H., Ozawa H. (2019). Region-specific Changes in Brain Kisspeptin Receptor Expression during Estrogen Depletion and the Estrous Cycle. Histochem. Cel Biol 152, 25–34. doi:10.1007/s00418-018-01767-z
Padda J., Khalid K., Moosa A., Syam M., Kakani V., Imdad U., et al. (2021). Role of Kisspeptin on Hypothalamic-Pituitary-Gonadal Pathology and its Effect on Reproduction. Cureus 13, e17600. doi:10.7759/cureus.17600
Quennell J. H., Howell C. S., Roa J., Augustine R. A., Grattan D. R., Anderson G. M. (2011). Leptin Deficiency and Diet-Induced Obesity Reduce Hypothalamic Kisspeptin Expression in Mice. Endocrinology 152, 1541–1550. doi:10.1210/en.2010-1100
Ramaswamy S., Seminara S. B., Pohl C. R., DiPietro M. J., Crowley W. F., Plant T. M. (2007). Effect of Continuous Intravenous Administration of Human Metastin 45-54 on the Neuroendocrine Activity of the Hypothalamic-Pituitary-Testicular Axis in the Adult Male Rhesus Monkey (Macaca mulatta). Endocrinology 148, 3364–3370. doi:10.1210/en.2007-0207
Richard N., Galmiche G., Corvaisier S., Caraty A., Kottler M. L. (2008). KiSS‐1 and GPR54 Genes Are Co‐Expressed in Rat Gonadotrophs and Differentially Regulated In Vivo by Oestradiol and Gonadotrophin‐Releasing Hormone. J. Neuroendocrinology 20, 381–393. doi:10.1111/j.1365-2826.2008.01653.x
Ruohonen S. T., Gaytan F., Usseglio Gaudi A., Velasco I., Kukoricza K., Perdices-Lopez C., et al. (2022). Selective Loss of Kisspeptin Signaling in Oocytes Causes Progressive Premature Ovulatory Failure. Hum. Reprod. 37, 806–821. doi:10.1093/humrep/deab287
Seminara S. B. (2005). Metastin and its G Protein-Coupled Receptor, GPR54: Critical Pathway Modulating GnRH Secretion. Front. Neuroendocrinology 26, 131–138. doi:10.1016/j.yfrne.2005.10.001
Singh S. P., Wolfe A., Ng Y., DiVall S. A., Buggs C., Levine J. E., et al. (2009). Impaired Estrogen Feedback and Infertility in Female Mice with Pituitary-specific Deletion of Estrogen Receptor Alpha (ESR1). Biol. Reprod. 81, 488–496. doi:10.1095/biolreprod.108.075259
Smith J. T., Rao A., Pereira A., Caraty A., Millar R. P., Clarke I. J. (2008). Kisspeptin Is Present in Ovine Hypophysial portal Blood but Does Not Increase during the Preovulatory Luteinizing Hormone Surge: Evidence that Gonadotropes Are Not Direct Targets of Kisspeptin In Vivo. Endocrinology 149, 1951–1959. doi:10.1210/en.2007-1425
Song W.-J., Mondal P., Wolfe A., Alonso L. C., Stamateris R., Ong B. W. T., et al. (2014). Glucagon Regulates Hepatic Kisspeptin to Impair Insulin Secretion. Cel Metab. 19, 667–681. doi:10.1016/j.cmet.2014.03.005
Teles M. G., Bianco S. D. C., Brito V. N., Trarbach E. B., Kuohung W., Xu S., et al. (2008). AGPR54-Activating Mutation in a Patient with Central Precocious Puberty. N. Engl. J. Med. 358, 709–715. doi:10.1056/NEJMoa073443
Thompson E. L., Patterson M., Murphy K. G., Smith K. L., Dhillo W. S., Todd J. F., et al. (2004). Central and Peripheral Administration of Kisspeptin-10 Stimulates the Hypothalamic-Pituitary-Gonadal axis. J. Neuroendocrinol 16, 850–858. doi:10.1111/j.1365-2826.2004.01240.x
Tolson K. P., Marooki N., De Bond J. A. P., Walenta E., Stephens S. B. Z., Liaw R. B., et al. (2020). Conditional Knockout of Kisspeptin Signaling in Brown Adipose Tissue Increases Metabolic Rate and Body Temperature and Lowers Body Weight. FASEB j. 34, 107–121. doi:10.1096/fj.201901600R
Wang Z., Feng M., Awe O., Ma Y., Shen M., Xue P., et al. (2019). Gonadotrope Androgen Receptor Mediates Pituitary Responsiveness to Hormones and Androgen-Induced Subfertility. JCI Insight 4, 127817. doi:10.1172/jci.insight.127817
Williams T. C., Berelowitz M., Berk M. A., Frohman L. A. (1987). Differential Effects of Insulin- and Proinsulin-Induced Hypoglycemia on Pituitary Hormone and Catecholamine Secretion. Diabetes Care 10, 278–285. doi:10.2337/diacare.10.3.278
Witham E. A., Meadows J. D., Hoffmann H. M., Shojaei S., Coss D., Kauffman A. S., et al. (2013). Kisspeptin Regulates Gonadotropin Genes via Immediate Early Gene Induction in Pituitary Gonadotropes. Mol. Endocrinol. 27, 1283–1294. doi:10.1210/me.2012-1405
Wolfe A., Divall S., Singh S. P., Nikrodhanond A. A., Baria A. T., Le W. W., et al. (2008). Temporal and Spatial Regulation of CRE Recombinase Expression in Gonadotrophin-Releasing Hormone Neurones in the Mouse. J. Neuroendocrinol 20, 909–916. doi:10.1111/j.1365-2826.2008.01746.x
Wu S., Chen Y., Fajobi T., DiVall S. A., Chang C., Yeh S., et al. (2014). Conditional Knockout of the Androgen Receptor in Gonadotropes Reveals Crucial Roles for Androgen in Gonadotropin Synthesis and Surge in Female Mice. Mol. Endocrinol. 28, 1670–1681. doi:10.1210/me.2014-1154
Yang B., Jiang Q., Chan T., Ko W. K. W., Wong A. O. L. (2010). Goldfish Kisspeptin: Molecular Cloning, Tissue Distribution of Transcript Expression, and Stimulatory Effects on Prolactin, Growth Hormone and Luteinizing Hormone Secretion and Gene Expression via Direct Actions at the Pituitary Level. Gen. Comp. Endocrinol. 165, 60–71. doi:10.1016/j.ygcen.2009.06.001
Keywords: kisspeptin receptor, GnRH, pituitary, reproduction, LH, FSH, puberty
Citation: Ma Y, Awe O, Radovick S, Yang X, Divall S, Wolfe A and Wu S (2022) Lower FSH With Normal Fertility in Male Mice Lacking Gonadotroph Kisspeptin Receptor. Front. Physiol. 13:868593. doi: 10.3389/fphys.2022.868593
Received: 02 February 2022; Accepted: 31 March 2022;
Published: 26 April 2022.
Edited by:
Vikas Kumar Roy, Mizoram University, IndiaReviewed by:
Yoshihisa Uenoyama, Nagoya University, JapanRadha Chaube, Banaras Hindu University, India
Copyright © 2022 Ma, Awe, Radovick, Yang, Divall, Wolfe and Wu. This is an open-access article distributed under the terms of the Creative Commons Attribution License (CC BY). The use, distribution or reproduction in other forums is permitted, provided the original author(s) and the copyright owner(s) are credited and that the original publication in this journal is cited, in accordance with accepted academic practice. No use, distribution or reproduction is permitted which does not comply with these terms.
*Correspondence: Sheng Wu, sheng.wu@temple.edu
†Present Address: Andrew Wolfe, Division of Physiological and Pathological Sciences, NationalInstitutes of Health, Bethesda, MD, United States
‡These authors share first authorship