- 1State Key Laboratory for Biology of Plant Diseases and Insect Pests, Institute of Plant Protection, Chinese Academy of Agricultural Sciences, Beijing, China
- 2Agricultural Genome Institute at Shenzhen, Chinese Academy of Agricultural Sciences, Shenzhen, China
As an invasive species, Bemisia tabaci Mediterranean (MED) has notable potential to adapt to a wide range of environmental temperatures, which enables it to successfully spread after invasion and occupy habitats over a wide latitude range. It has been postulated that chromatin remodeling mechanisms are related to the rapid acquisition of adaptive traits and thermal resistance in invasive species; however, relevant experimental evidence is scarce. To identify the molecular characteristics and assess the role of chromatin remodelers in thermal stress within invasive MED and native Asia II 1 of the B. tabaci species complex, we identified 13 switching defective/sucrose non-fermenting (SWI/SNF) and 10 imitation switch (ISWI) family members in the B. tabaci genome, analyzed their molecular characteristics and structures, and identified key mutation sites between MED and Asia II 1, then cloned the catalytic subunits, and revealed the difference in thermal tolerance function. The results showed that the expression levels of Bt-BRM-1 and Bt-BRM-2 were significantly higher in MED than in Asia II 1 during heat stress, and Bt-BRM-2 expression was significantly higher during cold stress. In addition, RNA interference results indicated that the two target genes had similar temperature tolerance function in the both two cryptic species. This study is the first to identify and analyze the molecular characteristics of SWI/SNF and ISWI family members and reveal their potential key roles in temperature tolerance in poikilothermic ectotherms. The results will assist in understanding the underlying temperature adaptation mechanism of invasive insects and will enrich stress adaptation research systems from an epigenetic perspective.
Introduction
Epigenetics can induce phenotypic variation in numerous organisms via regulation of gene expression, by activating or silencing transposons, and by remodeling chromatin without altering the underlying nucleotide sequence (Bossdorf et al., 2008; Secco et al., 2015). Phenotypic variation caused by epigenetics is a reversible alteration that mediates the rapid and plastic responses of organisms to environmental perturbations, and it is considered to increase the capacity of organisms to adapt to environmental stresses (Johannes et al., 2009; Mirouze and Paszkowski 2011; Kumar 2018). Therefore, epigenetics has attracted unprecedented interest from researchers, particularly with respect to biological invasive species.
Chromatin remodeling is one of the most important components of epigenetics. In eukaryotes, packaging DNA into chromatin is essential for the organization and expression of the genome (Corona et al., 2007). However, the chromatin state determines the accessibility and transcriptional activity of specific genomic regions (Li et al., 2007). Intracellular evolution has produced a series of specific chromatin remodeling factors to ensure the dynamic binding of DNA and proteins in chromatin; these factors are multimeric proteins that utilize the energy generated from ATP hydrolysis to modulate the access of transcription factors and other regulatory proteins to genomic DNA by sliding nucleosomes and increasing chromatin fluidity (Corona and Tamkun 2004; Hopfner et al., 2012). Chromatin remodeling complexes are divided into four families according to the conserved domains of catalytic subunits: the switching defective/sucrose non-fermenting (SWI/SNF) family, the imitation switch (ISWI) family, the inositol requiring 80 (INO80) family, and the chromodomain helicase DNA-binding (CHD) family (Clapier and Cairns 2009).
The SWI/SNF family remodelers were initially purified from Saccharomyces cerevisiae and are composed of 8–15 subunits (Mohrmann and Verrijzer 2005). They have two forms in Drosophila (Clapier and Cairns 2009), namely brahma-associated protein (BAP) and polybromo-associated BAP (PBAP), both of which contain the same catalytic subunit, BRM. The catalytic enzyme BRM includes a helicase-SANT (HSA) domain and a C-terminal Bromo domain. The ISWI family of remodelers contain two to four subunits; they are characterized by ATPase ISWI as the catalytic core, and the catalytic enzyme ISWI is composed of an ATPase domain and a continuous HAND-SANT (SWI3, ADA2, N-CoR, TF III B)-SLIDE (SANT-like ISWI) domain (Clapier and Cairns 2009). The INO80 family of remodelers contain more than 10 subunits, and their ATPase contains a long insertion (Bao and Shen 2007a). Finally, the CHD family remodelers consist of one to ten subunits, and they were first purified from Xenopus laevis (Marfella and Imbalzano 2007).
Of these four families, SWI/SNF and ISWI remodeling factors have been widely studied for their biological responses to temperature stress. For instance, studies in Drosophila have shown that the nucleosome remodeling factor (NURF), which is a chromatin-remodeling complex that contains ISWI as its catalytic subunit, cooperates with the GAGA factor to mobilize nucleosomes on the promoter of heat shock genes, and facilitates the binding of heat shock transcription factors (HSFs) (Tsukiyama et al., 1994; Badenhorst et al., 2002). In Arabidopsis, BRM showed heat-induced transgenerational upregulation, and the binding of BRM to the HSFA2 region was enhanced by heat (Liu et al., 2019). Another study suggested that SWI/SNF remodelers and histone deacetylase interact and that both are involved in mediating the heat stress response of Arabidopsis (Buszewicz et al., 2016). Furthermore, the functional interplay between SWI/SNF and ISWI remodelers in the regulation of heat shock genes was determined in Saccharomyces cerevisiae, and inactivation of both remodelers could reduce the effective binding of HSF during the heat shock process (Erkina et al., 2010).
Insects are poikilothermic ectotherms, and subtle changes in ambient temperature can cause a series of physiological changes that affect their life-history parameters, such as their survival rate (Musolin 2007; Couret et al., 2014). Temperature is, therefore, one of the most important factors affecting the geographical distribution of ectotherms (Gutierrez and Ponti, 2014; Lancaster 2016). The thermal biology of insects is often investigated by assessing extreme thermal tolerance or by measuring the temperature performance of various traits across a permissive temperature range (Angilletta 2009; Sørensen et al., 2018). Using thermal tolerance or thermal performance as a proxy for thermal adaptation enables any association between a species and the thermal characteristics of their habitats to be detected (Andersen et al., 2015; MacLean et al., 2019).
The whitefly Bemisia tabaci (Gennadius) (Hemiptera: Aleyrodidae) is a notable pest in agriculture because it causes damage to more than 600 economic crops through phloem feeding, by transmitting plant viruses, and by depositing honeydew (Oliveira et al., 2001; Brown and Czosnek, 2002). It is considered to be a species complex, as it comprises at least 36 morphologically indistinguishable species (De Barro et al., 2011; Liu et al., 2012; Wang and Yang 2017). The Mediterranean (MED) cryptic species was first detected in China in 2003, and it has successfully colonized and rapidly invaded most provinces. In contrast, the native species Asia II 1 is mainly distributed in the small natural areas of southern China (e.g., Hainan, Guangxi, and Zhejiang provinces), and it appears to have no tendency to spread to other areas (Chu et al., 2005; Hu et al., 2011; Liu and Liu 2012). As an invasive species, MED has the immense potential to adapt to a wide range of environmental temperatures, which allows it to successfully spread after invasion and to occupy habitats over a wide latitudinal range (Lee 2002; Cui et al., 2008; Yu et al., 2012).
Previous studies have shown that exposure of invasive whiteflies to thermal stress is accompanied by rapid alterations in their gene expression (Lü and Wan, 2008; Mahadav et al., 2009; Lü et al., 2014a; Dai et al., 2018a). Lü et al. (2014b) conducted heat shock experiments with MED and found that its survival rate was significantly improved within two generations. The authors speculated that this rapid increase in viability might be related to epigenetics. Another study (unpublished) analyzed transcriptome data and found that the expression levels of SWI/SNF and ISWI remodelers were significantly different between invasive and native whiteflies under temperature stress. Collectively, these results indicate that a possible explanation for the species’ rapidly increased temperature resistance is the regulatory plasticity of genes mediated by epigenetic mechanisms, such as chromatin remodeling. Although it has often been postulated that chromatin remodeling mechanisms are related to the rapid acquisition of adaptive traits and thermal resistance in invasive species, relevant experimental evidence is scarce.
In this paper, we report a series of experiments aimed at revealing the molecular characteristics and roles of remodelers in invasive MED and native Asia II 1. First, SWI/SNF and ISWI family members were identified in the B. tabaci genome, and their molecular characteristics, including the gene structures and conserved motifs, were analyzed using bioinformatics methods. Second, the catalytic subunits of the remodelers were cloned from MED and Asia II 1, and their tertiary structures and evolutionary relationships were analyzed. Third, the expression patterns of the catalytic subunits were examined after exposure to temperature stress in the two cryptic species. Finally, the function of the catalytic subunits in thermal stress was determined using the RNA interference (RNAi) method. These data reveal the novel function of chromatin remodeling factors in invasive B. tabaci MED and will help us to understand the temperature adaptation mechanism of invasive insects.
Materials and Methods
Insect Rearing
The invasive MED and native Asia II 1 used in this study were reared separately in the laboratory, and species were confirmed every month by the mitochondrial cytochrome oxidase I gene sequence according to the primer sequences reported by Ji et al. (2020). Whiteflies were reared on healthy cotton plants, Gossypium hirsutum (L.) (Zhong No. 287), in a greenhouse maintained at 26 ± 1°C at 50–60% relative humidity, and with a 14:10 h light: dark cycle.
Identification and Characterization of SWI/SNF and ISWI Family Members in Whitefly Genome
Whole whitefly genome sequences were downloaded from the Whitefly Genome Project database (https://www.whiteflygenomics.org/cgi-bin/bta/index.cgi) (MEAM1, Chen et al., 2016). To identify the SWI/SNF and ISWI family members, blastp and tblastn were performed against the whitefly genome using the published remodelers from Drosophila melanogaster as queries with E-values ≤1e−15. All high-confidence sequences were submitted to the CDD database (https://www.ncbi.nlm.nih.gov/Structure/cdd/wrpsb.cgi), Pfam database (https://pfam.xfam.org/), and SMART (http://smart.embl.de/) to confirm the presence of conserved domains. Identified nucleotide and protein sequences of SWI/SNF and ISWI family members from the B. tabaci genome were submitted to EndMemo (http://www.endmemo.com/bio/gc.php), to calculate the GC content, and submitted to ExPASy (http://web.expasy.org/protparam/), to calculate the number of amino acids, molecular weights (MWs), and theoretical isoelectric points (PIs). The exon-intron structures of the remodelers were identified using TBtools (https://github.com/CJ-Chen/TBtools). The conserved motifs were predicted using the MEME program (https://meme-suite.org/meme/).
Validation and Analysis of the Catalytic Subunit of SWI/SNF Complexes
Because catalytic subunit plays a critical role in the function of the SWI/SNF complexes, we validated and analyzed the gene sequences encoding the catalytic subunit in both MED and Asia II 1. Based on the catalytic subunit sequences obtained from the whitefly genome, specific primers for BRM-1and BRM-2 were designed (listed in Supplementary Table S1) to validate the corresponding sequences in invasive MED and native Asia II 1 species. All polymerase chain reaction (PCR) amplifications were performed using FastPfu DNA Polymerase (TransGen, Beijing, China). The amplified fragments were purified using an AxyPrep DNA Gel Extraction Kit (Axygen, West Orange, NJ, United States), cloned into the pEASY-Blunt Vector (TransGen, Beijing, China), and subsequently sequenced.
The three-dimensional structure of the putative catalytic subunits was modelled using I-TASSER (https://zhanglab.ccmb.med.umich.edu/I-TASSER/). Conserved domains were predicted using SMART and CDD databases. Sequence alignments and similarity analyses were performed using DNAMAN version 7.0. Phylogenetic trees were constructed using the maximum likelihood method (based on the Whelan Goldman model) with 1000 bootstrap replications in MEGA 7.0 (Whelan and Goldman, 2001; Kumar et al., 2016). A color-coded pairwise identity matrix from amino acid sequences of remodelers belonging to different species was generated using SDT v1.2 software (Guo et al., 2021).
Short-Term Temperature Stress Treatments
Pre-experimental results showed that the survival rate of whiteflies began to decrease significantly at temperatures below 0°C or above 40°C, i.e., B. tabaci could survive normally at 0–40°C. Therefore, temperatures of 0°C, 12°C, 35°C, and 40°C were used in the short-term temperature stress treatments to determine whether temperature stress affected the mRNA expression of the catalytic subunits (Dai et al., 2018b). As adult age is associated with different responses to thermal tolerance (Bowler and Terblanche, 2008), we standardized the adult age using only newly emerged whitefly adults that were younger than 3 h old. One hundred females and 100 males were placed together in a 1.5-mL centrifuge tube, and the tubes were placed in a constant-temperature environment (0°C, 12°C, 35°C, and 40°C) for 1, 3, and 5 h. Whiteflies maintained at 26°C were used as control groups. After conducting the temperature stress treatment, the whitefly adults were frozen immediately with liquid nitrogen and stored at -80°C. Each treatment was replicated thrice.
RNA Isolation and Real-Time Quantitative PCR (qPCR)
Total RNA was extracted from whiteflies using TRIzol (Invitrogen, Carlsbad, CA, United States), and cDNA was synthesized using the cDNA Synthesis kit (TransGen, Beijing, China) following the manufacturer’s protocol. qPCR was performed using an ABI 7500 system (Applied Biosystems, Foster, CA). The amplification volume was 20 µL, comprising the following: 10.0 µL qPCR SYBR Green Master Mix (Yeasen, Shanghai, China), 0.8 µL primers (10 µM), 1.0 µL cDNA template, and 8.2 µL double distilled H2O. Three technical replicates were performed for each of the three biological replicates. The primers (including two reference genes, EF1-α and β-tubulin, to normalize the mRNA expression levels) are listed in Supplementary Table S1 (Miles 1965).
RNAi
dsRNA used in this study was synthesized using the MEGAscript T7 High Yield Transcription Kit (Ambion, Austin, TX, United States) with specific primers (Supplementary Table S1). Feeding was performed using the parafilm clip nutrient solution method, and the dsRNA was diluted to 0.3–0.5 µg/µL in a 10% sucrose solution for feeding assay (Miles 1965; Lü and Wan, 2011). Moreover, we set control groups, including untreated whiteflies, which were fed with EGFP-specific dsRNA or 10% sucrose. After feeding for 3 h, partial samples were immediately frozen in liquid nitrogen and stored at -80°C for analysis of mRNA expression levels. The remaining whiteflies were collected immediately for conducting the temperature resistance experiments or temperature preference behavioral assays.
Temperature Resistance After dsRNA Treatment
To analyze heat resistance, the knockdown times for adults exposed to high temperatures were measured (Bubli et al., 1998; Mitchell and Hoffmann 2010; Ma et al., 2014). Two whiteflies were randomly selected and placed in a 1.5-mL centrifuge tube, and the tube was maintained at a constant temperature of 45 ± 0.2°C in a water bath circulator (CC-106A, Huber Kältemaschinenbau GmbH). We measured the interval between the time the tube was placed in a water bath and the time when the whitefly lost control of its body and could not stand autonomously. For cold resistance, we measured the recovery time following a chill coma induced by cold shock (Gibert et al, 2001; Ma et al., 2014). Two newly emerged adults were randomly selected and placed in a 1.5-mL centrifuge tube, and the tube was exposed to -5 ± 0.2°C in a refrigeration bath circulator (K6-cc-NR, Huber Kältemaschinenbau GmbH) for 10 min; the recovery time was observed at 26 ± 0.2°C. Each treatment used 100 females and 100 males. Temperatures of 45°C and -5°C were selected based on pre-experiments that revealed them to be discrimination points for whitefly temperature tolerance (Ma et al., 2014).
Statistical Analyses
Relative expression levels were calculated using the 2-ΔΔCT method (Livak and Schmittgen 2001; Pfaffl 2001). Statistical analyses were conducted using the SAS 9.4 software package (SAS Institute, Cary, North Carolina), and a one-way analysis of variance followed by Duncan’s multiple range test was used to compare the differences between different treatments. Data are presented as mean ± standard error. Differences were considered statistically significant at p<0.05.
Results
Identification and Characterization of SWI/SNF and ISWI Family Members in Whitefly Genome
Remodelers are heteromeric proteins composed of several subunits(Clapier and Cairns 2009). In this study, 13 SWI/SNF family members were identified throughout the genome of B. tabaci, including three isoforms of subunit BRM; two isoforms of subunit BAP60 and BAP55; and one each of subunits OSA, BAP170, MOR, SNR1, BAP111, and Polybromo. For these subunits, the length ranged from 372 (Bt-SNR1) to 2079 amino acids (Bt-OSA), the MW was between 42.18 (Bt-SNR1) and 224.55 kDa (Bt-OSA), and the predicted PI ranged from 5.37 (Bt-moira) to 9.28 (Bt-BAP60-2). Moreover, 10 ISWI family members were identified from the whitefly genome, including four isoforms of subunit ISWI, as well as one each of subunits NURF301, ACF1, CHRAC16, CHRAC14, NURF55, and NURF38. For these subunits, the length ranged from 142 (Bt-CHRAC14) to 2691 amino acids (Bt-NURF301), the MW ranged from 15.45 (Bt-CHRAC14) to 300.48 kDa (Bt-NURF301), and the PI ranged from 4.61 (Bt-CHRAC14) to 9.32 (Bt-ISWI-3). Details of the SWI/SNF and ISWI remodelers obtained from the whitefly genome, including the gene length, GC%, and the predicted MW and PI, are presented in Table 1.
The exon-intron structures of the SWI/SNF and ISWI family members were determined by comparing the full-length cDNA sequences with the corresponding genomic DNA sequences. The results showed that remodelers from the different families had completely different exon-intron structures and numbers (Figure 1A). The conserved motifs of chromatin remodeling factors were identified using the MEME program, and the lengths of these motifs varied from 41 (motif 7) to 200 amino acids (motifs 4 and 10) (Figure 1B). The details of the putative motifs are presented in Table 2. Moreover, based on analyses of the Pfam and CDD databases, we found that motifs 1, 2, 3, 5, and 7 collectively formed a highly conserved ATPase domain, and the sequences of motifs 4, 6, and 8 corresponded to the HSA, HAND, and Bromo domains, respectively.
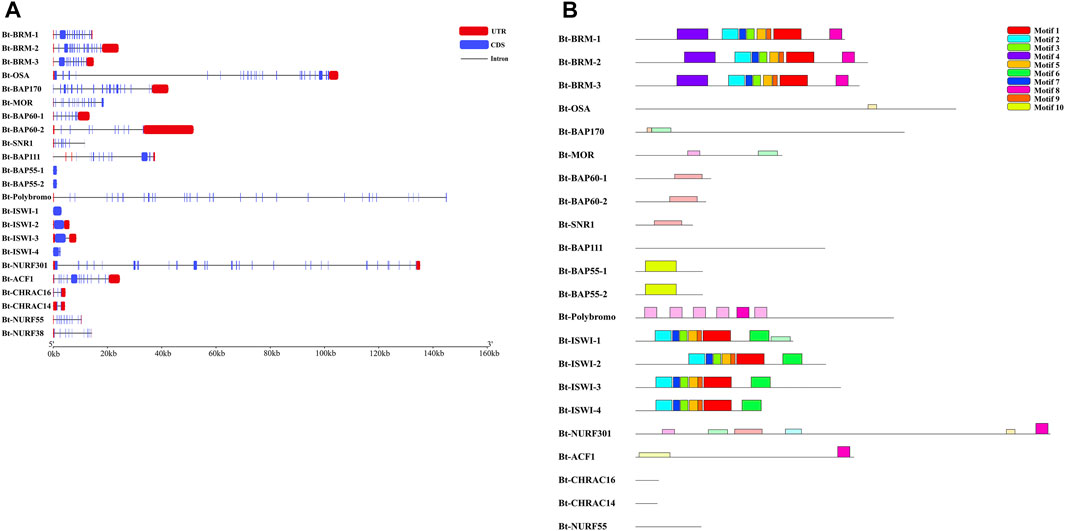
FIGURE 1. Gene structure and conserved motif analysis of remodelers in Bemisia tabaci. (A) Exon/intron organization of remodelers. Red boxes represent UTR, blue boxes represent exons and black lines represent introns. (B) Distribution of conserved motifs in remodelers. Ten putative motifs are indicated in different colored boxes. For details of motifs refer toTable 2.
Validation and Analysis of the Catalytic Subunit of SWI/SNF Complexes
Based on the sequences obtained from the whitefly genome, the catalytic subunits BRM-1 and BRM-2 were cloned from invasive MED and native Asia II 1. The open reading frame length and the deduced amino residue number of Bt-BRM-1 cloned from the two cryptic species were consistent with the corresponding sequence identified in the whitefly genome. However, the Bt-BRM-2 cloned from the two cryptic species had seven amino residues more than the corresponding sequence identified in the whitefly genome (206-212: PALNSTP). Moreover, various sites in the amino acid sequences were derived from Bt-BRM-1 and Bt-BRM-2 in MED and Asia II 1 (Table 3).
Conserved domain analysis indicated that both Bt-BRM-1 and Bt-BRM-2 had domain features unique to the SWI/SNF family: the HSA domain and the C-terminal bromodomain (Figures 2A, 3A). Moreover, I-TASSER was used to predict the three-dimensional structure of the proteins. The results showed that remodelers in B. tabaci had structures similar to those of yeast (Figures 2A, 3A)(Wagner et al., 2020). In addition, multiple sequence alignments of remodeler catalytic subunits from different species revealed that they were relatively conserved (Figures 2C, 3C). The similarity of Bt-BRM-1 and Bt-BRM-2 to other species exceeded 62.5 and 63.7%, respectively. Furthermore, the relationships between Bt-BRM-1 and Bt-BRM-2, and the homologous sequences from other species were analyzed (Figure 4), and remodelers of insects in the same order were clustered on the same branch of the phylogenetic tree.
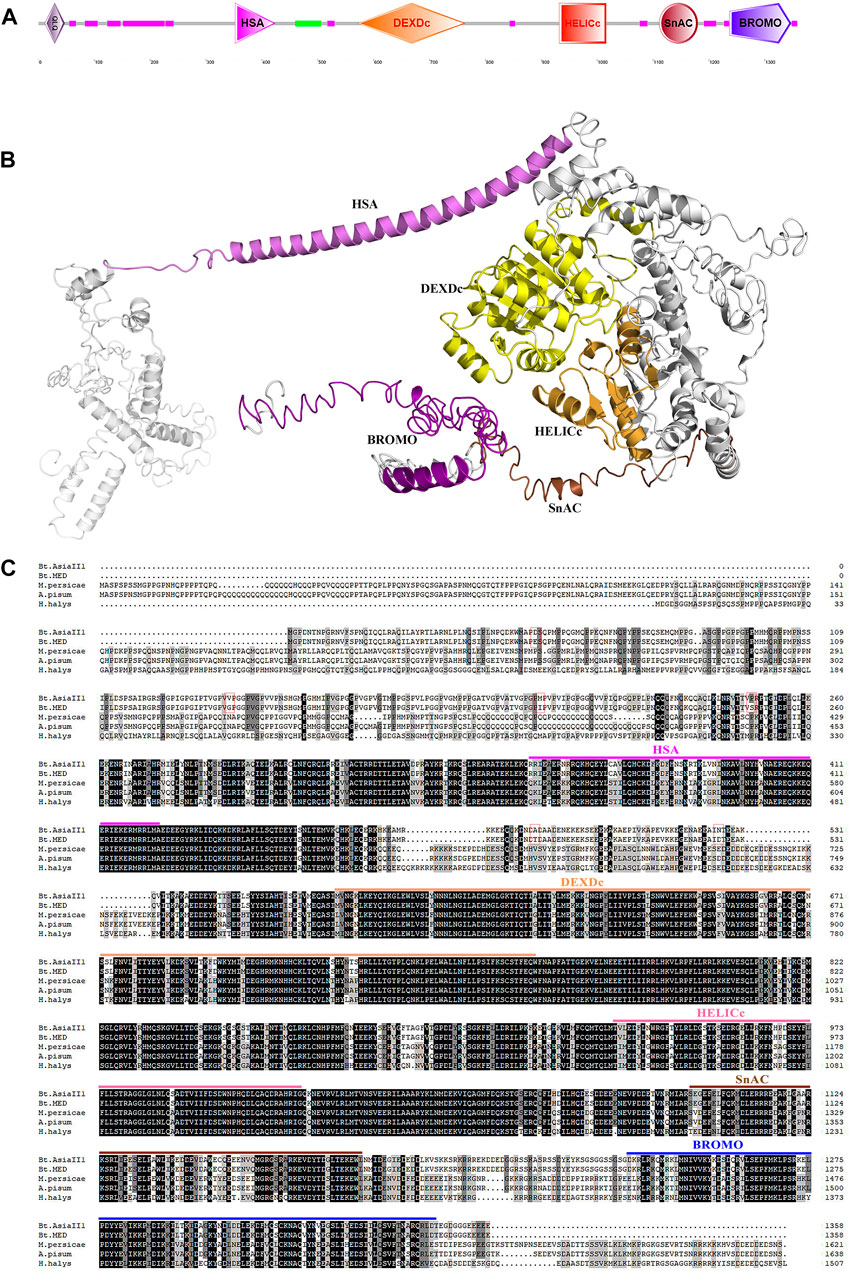
FIGURE 2. Conserved domains of Bt-BRM-1 in Bemisia tabaci. (A) Functional domains in Bt-BRM-1 protein. (B) The predicted tertiary structure of Bt-BRM-1. (C) Multiple alignments of the BRM protein from whiteflies and other insects. The conserved domains are highlighted in different colors and the amino acid sites that vary between MED and Asia II 1 are marked with red rectangles. Bt. Asia II 1: Bemisia tabaci Asia II 1; Bt. MED: Bemisia tabaci MED; M. persicae: Myzus persicae (XP_022166801.1); A. pisum: Acyrthosiphon pisum (XP_001947872.2); and H. halys: Halyomorpha halys (XP_014292008.1).
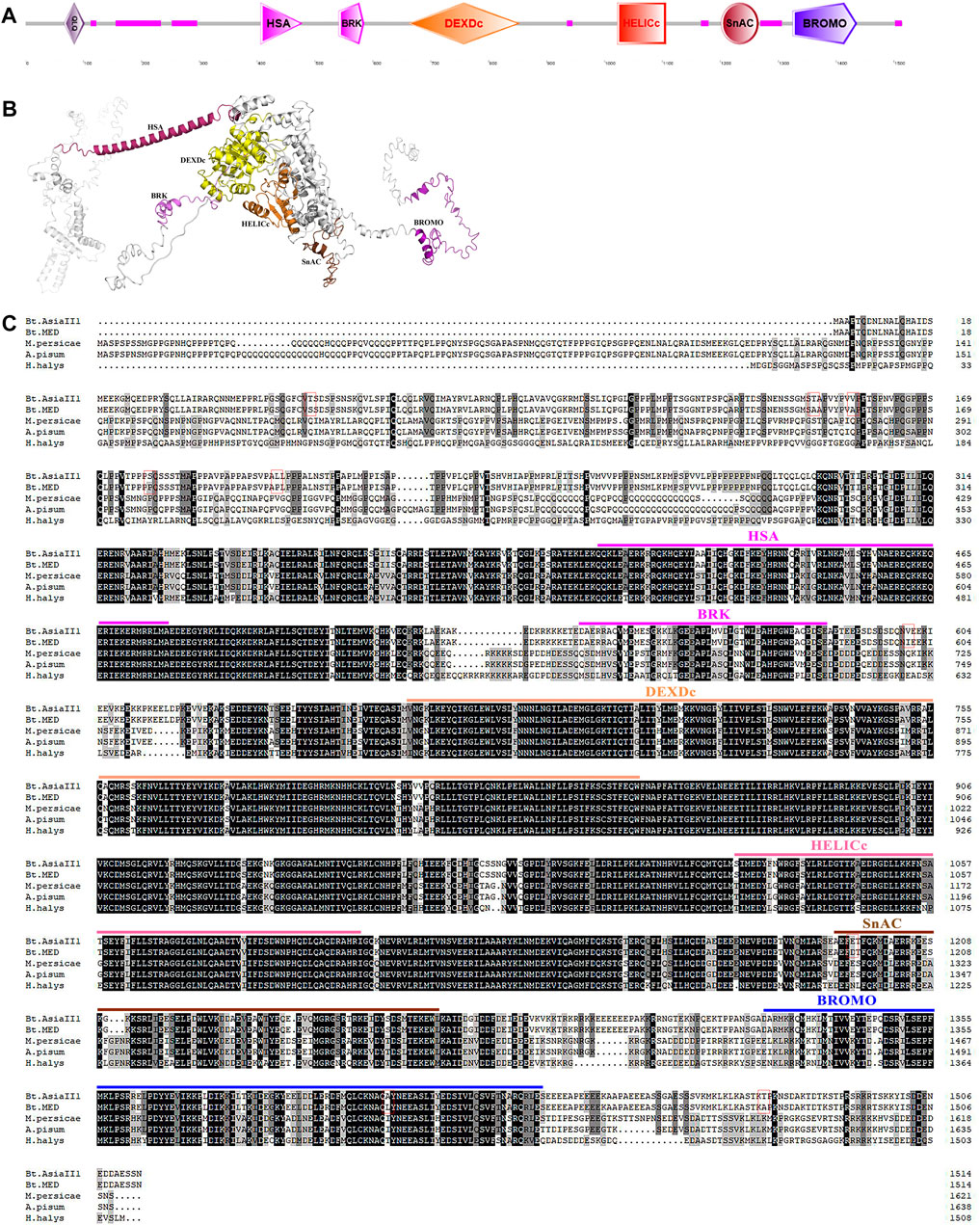
FIGURE 3. Conserved domains of Bt-BRM-2 in Bemisia tabaci. (A) Functional domains in Bt-BRM-2 protein. (B) The predicted tertiary structure of Bt-BRM-2. (C) Multiple alignments of the BRM protein from whiteflies and other insects. The conserved domains are highlighted in different colors and the amino acid sites that vary between MED and Asia II 1 are marked with red rectangles. Bt. Asia II 1: Bemisia tabaci Asia II 1; Bt. MED: Bemisia tabaci MED; M. persicae: Myzus persicae (XP_022166801.1); A. pisum: Acyrthosiphon pisum (XP_001947872.2); and H. halys: Halyomorpha halys (XP_014292008.1).
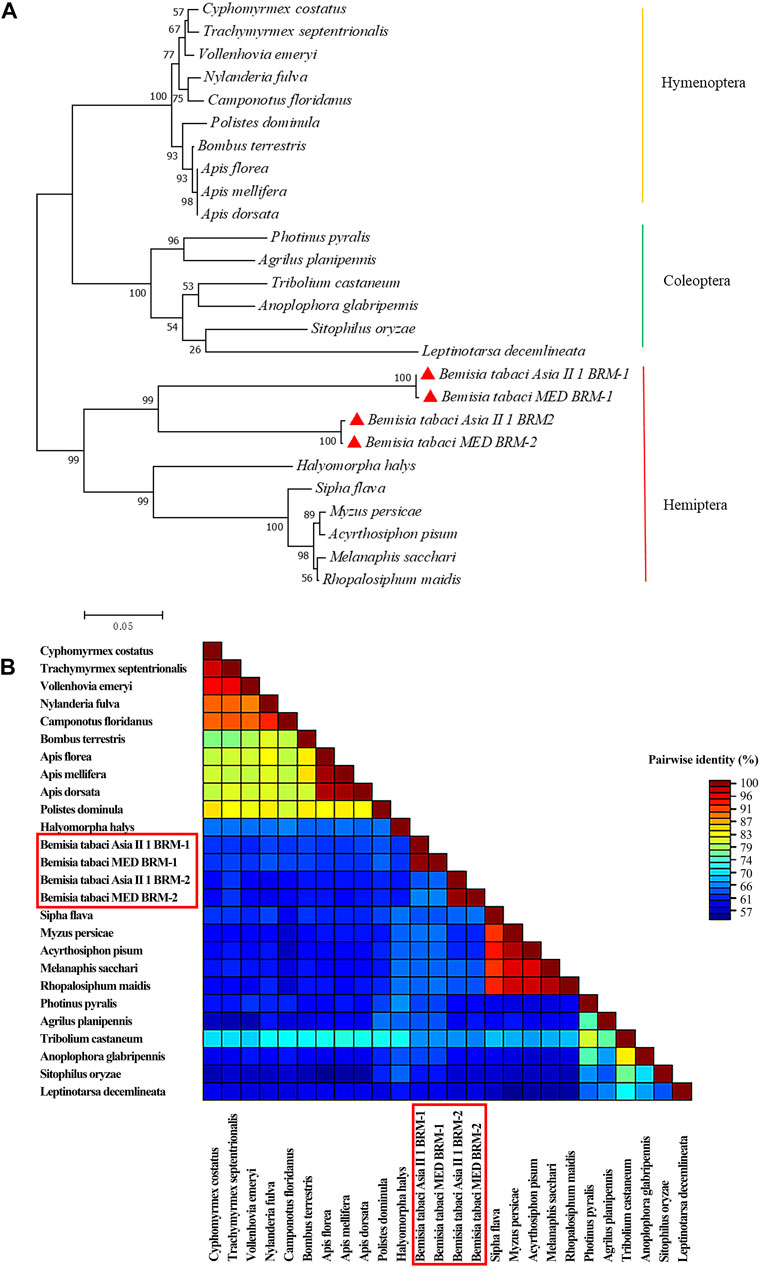
FIGURE 4. The relationships of Bt-BRM-1, Bt-BRM-2 and the homologous sequences from other species. (A) Phylogenetic analysis of the homologous sequences from various species performed using the maximum likelihood method based on the Whelan Goldman (WAG) model with 1,000 bootstrap replications. Bt-BRM-1 and Bt-BRM-2 are highlighted with red triangles, and the protein sequences which used for phylogenetic analysis are listed inSupplementary Table S2. (B) Color-coded pairwise identity matrix from amino acid sequences of remodelers from different species generated using SDT software. Bt-BRM-1 and Bt-BRM-2 are marked with red rectangles.
Expression Profiles of Catalytic Subunits Under Temperature Stress
To investigate whether temperature stress affects the mRNA expression of chromatin remodeling factors, whiteflies were exposed to hot and cold conditions for various time periods. Compared with individuals maintained at 26°C (the control temperature), the expression of Bt-BRM-1 gene in MED and Asia II 1 was downregulated under cold shock conditions. The expression pattern was opposite in the two cryptic species (upregulated in MED, but downregulated in Asia II 1) under heat shock conditions (Figure 5A). The expression level of Bt-BRM-2 in MED was significantly upregulated after exposure to short-term heat or cold conditions, whereas it was significantly downregulated in Asia II 1 (Figure 5B). Additionally, the mRNA levels of Bt-BRM-1 and Bt-BRM-2 in MED were significantly higher than those in Asia II 1 during heat stress, and the expression level of Bt-BRM-2 in MED was significantly higher than that in Asia II 1 during cold stress (Figure 6). These data indicated that remodelers in whiteflies were strongly induced by heat or cold stress, and the expression profiles differed significantly between the invasive MED and native Asia II 1 species.
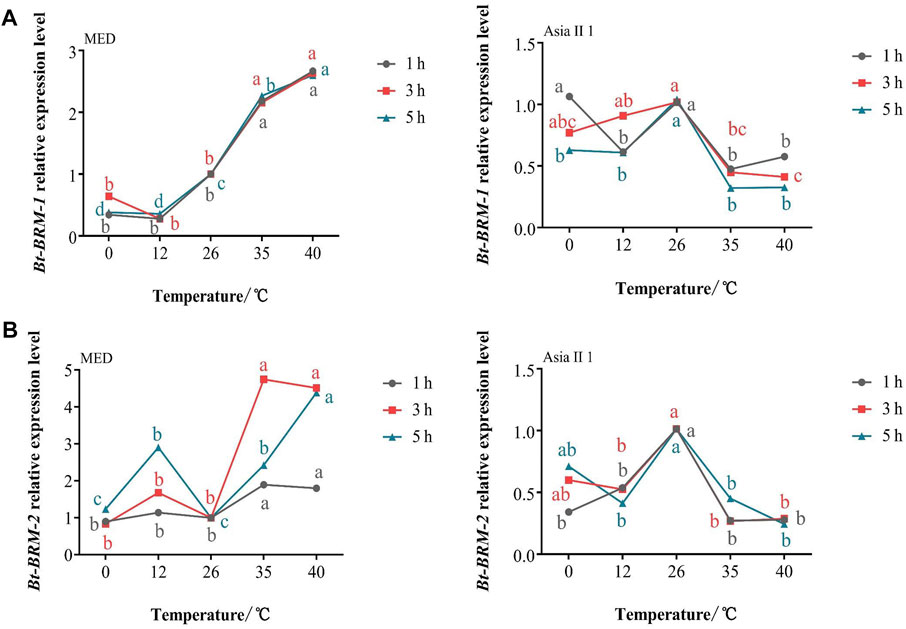
FIGURE 5. Variation trend of relative expression levels of Bt-BRM-1 (A) and Bt-BRM-2 (B) in MED and Asia II 1 after exposure to different temperatures. Different lowercase letters indicate significant difference at p < 0.05.
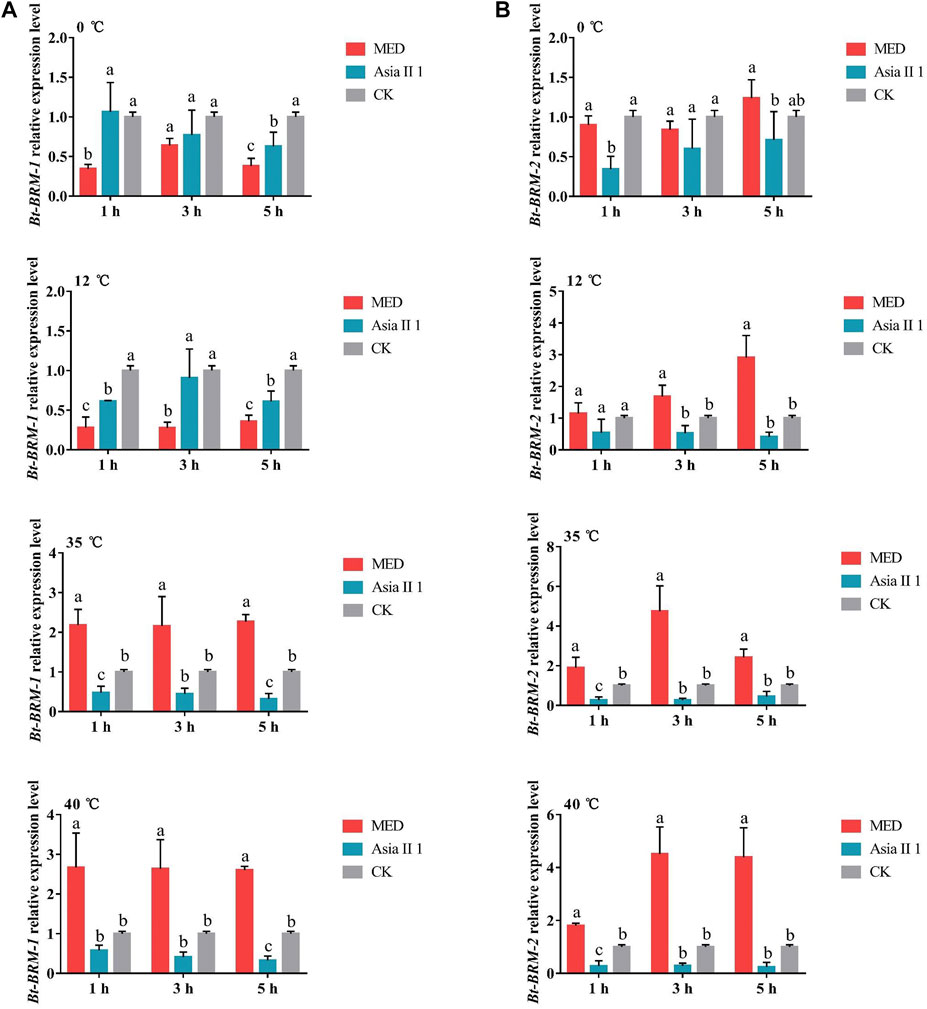
FIGURE 6. Relative expression levels of Bt-BRM-1 (A) and Bt-BRM-2 (B) in MED and Asia II 1 after exposure to different temperatures. Different lowercase letters indicate significant difference at p < 0.05.
Function of Chromatin Remodeling Factors During Thermal Stress
To explore the function of catalytic subunits, we fed newly emerged whitefly adults with dsRNA to silence the target genes. The expression levels of target genes in MED and Asia II 1 after feeding with dsRNA for 3 h were significantly lower than those in the control groups (Figure 7). The primer sequences used to detect mRNA expression are listed in Supplementary Table S1.
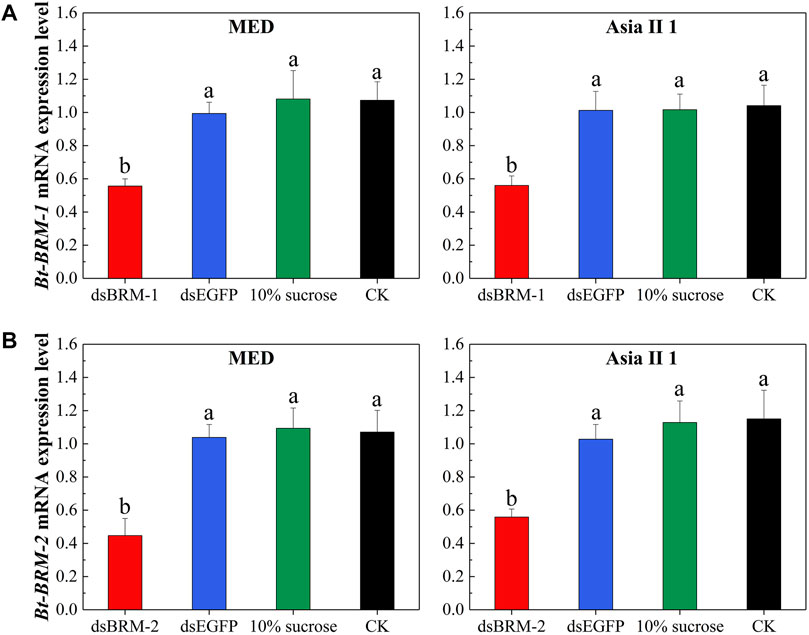
FIGURE 7. Effect of double-stranded RNA (dsRNA) treatment on expression levels of Bt-BRM-1 (A) and Bt-BRM-2 (B). The expression levels of target genes in MED and Asia II 1 after feeding with dsRNA for 3 h were significantly decreased compared with the controls: whiteflies untreated (CK), fed EGFP dsRNA (dsEGFP), or given 10% sucrose. The expression levels are expressed as the mean ± SE. Bars with different lowercase letters are significantly different at p < 0.05.
Compared with the control treatments, silencing Bt-BRM-1 and Bt-BRM-2 reduced the heat resistance of MED by 16.10 and 13.34%, respectively, while the reduction in heat resistance in Asia II 1 was 31.17 and 30.82%, respectively (Figure 8 and Supplementary Table S3). In addition, silencing Bt-BRM-2 reduced the cold resistance of MED by 59.40%, and the reduction of cold resistance in Asia II 1 was 32.89%, respectively (Figure 9 and Supplementary Table S4). However, there were no significant changes in the cold tolerance of the two cryptic species after silencing Bt-BRM-1.
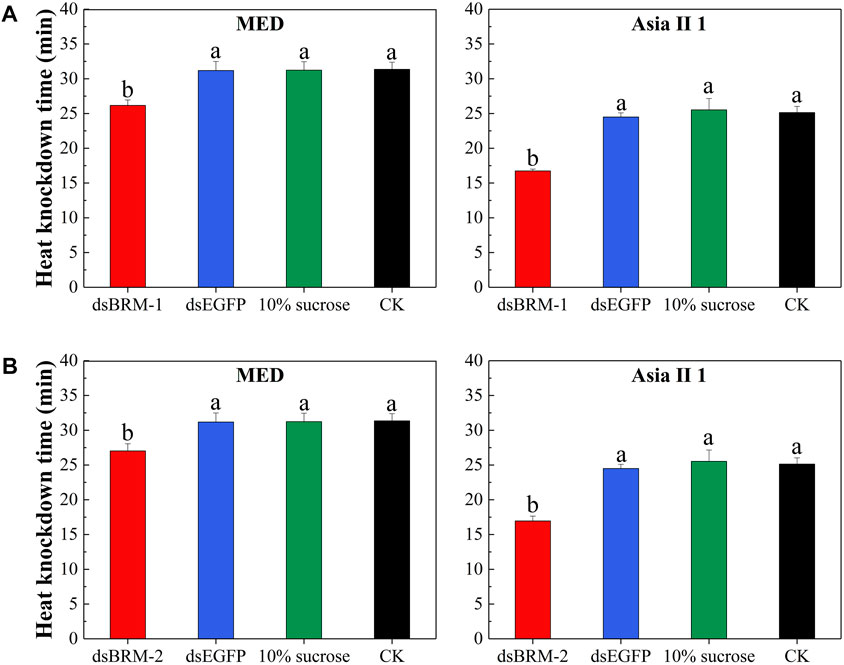
FIGURE 8. Mean heat knockdown time (TKD) after double-stranded RNA (dsRNA) feeding in whitefly adults. Compared with the control groups (whiteflies untreated (CK) or fed dsEGFP or 10% sucrose), TKD for MED and Asia II 1 after feeding with Bt-BRM-1 (A) or Bt-BRM-2 (B) dsRNA was significantly decreased. Moreover, heat resistance of the two cryptic species was reduced differently after interfering with the remodelers (details are listed inSupplementary Table S3). The data are presented as the mean ± SE; n = 200. Bars with different lowercase letters are significantly different at p < 0.05.
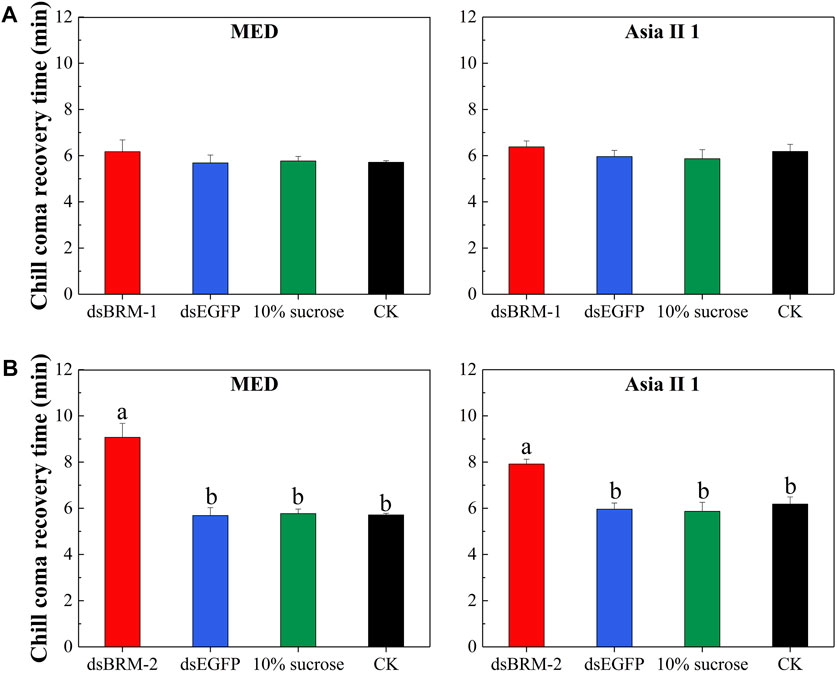
FIGURE 9. Mean chill coma recovery time (TRC) after double-stranded RNA (dsRNA) feeding in whiteflies. Compared with the controls (whiteflies untreated (CK), given dsEGFP, or 10% sucrose), TRC for adults feeding with dsBRM-2 (B) was significantly increased. Although the TRC of MED and Asia Ⅱ 1 increased after silencing Bt-BRM-1 (A), there was no statistically significant difference. Moreover, cold resistance of the two cryptic species was reduced differently after interfering with the remodelers (details are listed in Supplementary Table S4). The data are presented as the mean ± SE; n = 200. Bars with different lowercase letters are significantly different at p < 0.05.
Discussion
It is known that chromatin remodeling factors are related to biological responses to environmental stress, and they have been well characterized in several model organisms, such as Saccharomyces cerevisiae, Drosophila, X. laevis, and Arabidopsis thaliana (Bao and Shen 2007b; Yadon and Tsukiyama 2011). It is also known that the subunit numbers of SWI/SNF family remodelers vary in different organisms; for example, yeast has 11 to 15 subunits and Drosophila has 8. Analogously, the number of ISWI family remodeler subunits is two or three in yeast and four in Drosophila (Clapier and Cairns 2009). However, such knowledge is limited in non-model organisms (especially invasive insects). In this study, we identified, for the first time, 13 SWI/SNF family members and 10 ISWI family members in the whitefly genome, and we analyzed their exon-intron structures and conserved motifs using bioinformatics methods. Previous studies have found that catalytic subunits of chromatin remodeling factors share a conserved ATPase domain (Clapier and Cairns 2009). Similarly, motif analysis indicated that Bt-BRM-1 to Bt-BRM-3 (the catalytic subunit of SWI/SNF complexes) and Bt-ISWI-1 to Bt-ISWI-4 (the catalytic subunit of ISWI complexes) all have this special functional domain, which is required for remodeling and serves as a DNA-translocating motor to break histone-DNA contacts (Clapier and Cairns 2009).
To explore the function of chromatin remodeling factors in temperature adaptation of invasive and native B. tabaci species, we cloned two key catalytic subunits of SWI/SNF complexes. By analyzing the cloned catalytic subunit sequences from whiteflies, we found different amino residues between invasive MED and native Asia II 1; nevertheless, the sequences in MED and Middle East-Asia Minor 1 (MEAM1, another invasive species, with phenotypic plasticity similar to MED but higher than that of native species) were identical (predicted: XP_018897204.1). The distribution regions of invasive MED and MEAM1 in China are also approximately similar (Liu and Liu, 2012). These results indicate that nonsynonymous mutations in chromatin remodeling ATPase may be related to the plasticity and geographic distribution of invasive and native whiteflies. In addition, we found that the differential amino acid residues in Bt-BRM-2-D1194 E and L1408A between invasive and native B. tabaci were located in the SnAC and Bromo domains, respectively. These structures interact specifically with histones and play a crucial role in chromatin remodeling (Clapier and Cairns 2009; Sen et al., 2011). Jabba et al. (2014) demonstrated that minimal changes in the functional domain of the amino acid sequence were sufficient to generate a wide range of thermal sensitivities. Therefore, we hypothesize that the presence of these key mutation sites in remodelers may be one of the important factors influencing the temperature tolerance of invasive B. tabaci species, thus affecting their geographical distribution and dispersal.
Insects are poikilothermic ectotherms, and subtle changes in ambient temperature can cause a series of physiological changes and affect their life-history parameters, such as their survival rate (Couret et al., 2014; Musolin 2007). The rapid adaptation of invasive MED to various environmental conditions is likely the main reason for its successful colonization and rapid invasion (Cui et al., 2008; Yu et al., 2012; Munoz-Valencia et al., 2016). Studies have shown that the ability of organisms to cope with environmental stresses, such as heat or cold, relies on rapid and suitable mechanisms for reprogramming gene expression (Asensi-Fabado et al., 2017). ATP-dependent chromatin remodelers (e.g., SWI/SNF complexes) have been found to affect chromatin structure and play an essential role in regulating gene expression (Bartholomew 2014). Previous studies have demonstrated that the expression of chromatin remodeling factors can be induced by temperature stress. For example, CHR720 (SWI/SNF family) expression in Oryza sativa is clearly induced by cold stress (Hu et al., 2013). Similarly, in this study, the mRNA expression levels of catalytic subunits in whiteflies were found to be strongly altered under high or low temperatures. The expression patterns of Bt-BRM-1 and Bt-BRM-2 between MED and Asia II 1 were opposite under short-term heat stress. In addition, the mRNA levels of Bt-BRM-1 and Bt-BRM-2 in MED were significantly higher than those in Asia II 1 during heat treatment, and the expression level of Bt-BRM-2 in MED was significantly higher than that in Asia II 1 during cold treatment. Thus, we speculate that the difference between the expression patterns of remodelers between invasive MED and native Asia II 1 might be related to the former’s phenotypic plasticity and adaptability in response to environmental stresses.
ATP-dependent chromatin remodeling factors play multiple roles in the temperature stress response. For instance, Erkina et al. (2010) reported that combinatorial inactivation of SWI/SNF and ISWI eliminated the preloading of HSF promoters, thereby affecting the synthesis of heat shock proteins. Research in Drosophila has shown that the chromatin-remodeling complex NURF cooperates with the GAGA factor to mobilize nucleosomes on the promoter of the heat-shock genes, thereby creating a nucleosome-free domain over the promoter, which exposes suitable sites for HSFs (Tsukiyama et al., 1995; Badenhorst et al., 2002). In addition, research in Arabidopsis has demonstrated that the chromatin-remodeling complex BRM contributes to transgenerational thermo-memory (Badenhorst et al., 2002). These experiments indicate that chromatin remodeling factors are involved in biological responses to temperature. In this study, we used the RNAi method to identify the functions of the remodelers under temperature stress conditions. The results showed that the two chromatin remodeling ATPase genes affected the heat tolerance of the two cryptic species, and Bt-BRM-2 expression also affected the cold tolerance of the two cryptic species. Intriguingly, Bt-BRM-2 had a greater impact on cold resistance in invasive MED, whereas Bt-BRM-1 and Bt-BRM-2 had a greater impact on heat resistance in native Asia II 1. Therefore, we speculate that Bt-BRM-2 is an important regulatory factor for invasive MED diffusion to low temperature regions. However, the effects of the different expression patterns of the two chromatin remodeling elements on the specific regulatory mechanisms of temperature resistance between invasive MED and native Asia II 1 remains to be further studied.
To the best of our knowledge, this study is the first to identify and analyze the molecular characteristics of SWI/SNF and ISWI family members and reveal their possible key roles in temperature resistance in ectotherms. This study assists in understanding the adaptation mechanisms of invasive insects and enriches the body of literature focusing on stress from an epigenetic perspective.
Data Availability Statement
The original contributions presented in the study are included in the article/Supplementary Material, further inquiries can be directed to the corresponding author.
Ethics Statement
Ethical review and approval was not required for the animal study because insects.
Author Contributions
SJ, and XW did the experiments; SJ analyzed the results and wrote the paper; SJ and ZL did the bioinformatic analysis; WL helped to revise the introduction and results; FW helped to revised the discussion; ZL was responsible for the experiment design and the manuscripts’ revision.
Funding
This work was supported by the National Natural Science Foundation of China (32072494) and the National Key Research and Development Program (2021YFD1400200 and 2021YFC2600400).
Conflict of Interest
The authors declare that the research was conducted in the absence of any commercial or financial relationships that could be construed as a potential conflict of interest.
Publisher’s Note
All claims expressed in this article are solely those of the authors and do not necessarily represent those of their affiliated organizations, or those of the publisher, the editors and the reviewers. Any product that may be evaluated in this article, or claim that may be made by its manufacturer, is not guaranteed or endorsed by the publisher.
Supplementary Material
The Supplementary Material for this article can be found online at: https://www.frontiersin.org/articles/10.3389/fphys.2022.865172/full#supplementary-material
References
Andersen J. L., Manenti T., Sørensen J. G., MacMillan H. A., Loeschcke V., Overgaard J. (2015). How to assessDrosophilacold Tolerance: Chill Coma Temperature and Lower Lethal Temperature Are the Best Predictors of Cold Distribution Limits. Funct. Ecol. 29, 55–65. doi:10.1111/1365-2435.12310
Angilletta Jr. M. J. (2009). Thermal Adaptation: A Theoretical and Empirical Synthesis. Oxford: Oxford University Press. doi:10.1093/acprof:oso/9780198570875.001.1
Asensi-Fabado M.-A., Amtmann A., Perrella G. (2017). Plant Responses to Abiotic Stress: the Chromatin Context of Transcriptional Regulation. Biochim. Biophys. Acta (Bba) - Gene Regul. Mech. 1860, 106–122. doi:10.1016/j.bbagrm.2016.07.015
Badenhorst P., Voas M., Rebay I., Wu C. (2002). Biological Functions of the ISWI Chromatin Remodeling Complex NURF. Genes Dev. 16, 3186–3198. doi:10.1101/gad.1032202
Bao Y., Shen X. (2007a). SnapShot: Chromatin Remodeling Complexes. Cell 129, 632. doi:10.1016/j.cell.2007.04.018
Bao Y., Shen X. (2007b). INO80 Subfamily of Chromatin Remodeling Complexes. Mutat. Research/Fundamental Mol. Mech. Mutagenesis 618, 18–29. doi:10.1016/j.mrfmmm.2006.10.006
Bartholomew B. (2014). Regulating the Chromatin Landscape: Structural and Mechanistic Perspectives. Annu. Rev. Biochem. 83, 671–696. doi:10.1146/annurev-biochem-051810-093157
Bossdorf O., Richards C. L., Pigliucci M. (2008). Epigenetics for Ecologists. Ecol. Lett. 11, 106–115. doi:10.1111/j.1461-0248.2007.01130.x
Bowler K., Terblanche J. S. (2008). Insect thermal Tolerance: what Is the Role of Ontogeny, Ageing and Senescence? Biol. Rev. 83, 339–355. doi:10.1111/j.1469-185x.2008.00046.x
Brown J. K., Czosnek H. (2002). Whitefly Transmission of Plant Viruses. Adv. Bot. Res. 36, 65–100. doi:10.1016/s0065-2296(02)36059-2
Bubli O. A., Imasheva A. G., Loeschcke V. (1998). Selection for Knockdown Resistance to Heat in Drosophila melanogaster at High and Low Larval Densities. Evolution 52, 619–625. doi:10.1111/j.1558-5646.1998.tb01661.x
Buszewicz D., Archacki R., Palusiński A., Kotliński M., Fogtman A., Iwanicka-Nowicka R., et al. (2016). HD2C Histone Deacetylase and a SWI/SNF Chromatin Remodelling Complex Interact and Both Are Involved in Mediating the Heat Stress Response inArabidopsis. Plant Cel Environ. 39, 2108–2122. doi:10.1111/pce.12756
Chen W., Hasegawa D. K., Kaur N., Kliot A., Pinheiro P. V., Luan J., et al. (2016). The Draft Genome of Whitefly Bemisia Tabaci MEAM1, a Global Crop Pest, Provides Novel Insights into Virus Transmission, Host Adaptation, and Insecticide Resistance. BMC Biol. 14, 110. doi:10.1186/s12915-016-0321-y
Chu D., Zhang Y., Cong B., Xu B., Wu Q. (2005). Identification for Yunnan Q-Biotype Bemisia Tabaci Population. Chin. Bull Entomol 42, 54–56.
Clapier C. R., Cairns B. R. (2009). The Biology of Chromatin Remodeling Complexes. Annu. Rev. Biochem. 78, 273–304. doi:10.1146/annurev.biochem.77.062706.153223
Corona D. F., Siriaco G., Armstrong J. A., Snarskaya N., McClymont S. A., Scott M. P., et al. (2007). ISWI Regulates Higher-Order Chromatin Structure and Histone H1 Assembly In Vivo. Plos Biol. 5, e232–2021. doi:10.1371/journal.pbio.0050232
Corona D. F. V., Tamkun J. W. (2004). Multiple Roles for ISWI in Transcription, Chromosome Organization and DNA Replication. Biochim. Biophys. Acta (Bba) - Gene Struct. Expr. 1677, 113–119. doi:10.1016/j.bbaexp.2003.09.018
Couret J., Dotson E., Benedict M. Q. (2014). Temperature, Larval Diet, and Density Effects on Development Rate and Survival of Aedes aegypti (Diptera: Culicidae). Plos One 9, e87468. doi:10.1371/journal.pone.0087468
Cui X., Wan F., Xie M., Liu T. (2008). Effects of Heat Shock on Survival and Reproduction of Two Whitefly Species,Trialeurodes vaporariorumandBemisia tabaciBiotype B. J. Insect Sci. 8, 1–10. doi:10.1673/031.008.2401
Dai T.-M., Lü Z.-C., Liu W.-X., Wan F.-H. (2017). Selection and Validation of Reference Genes for qRT-PCR Analysis during Biological Invasions: the thermal Adaptability of Bemisia Tabaci MED. Plos One 12, e0173821. doi:10.1371/journal.pone.0173821
Dai T.-M., Lü Z.-C., Wang Y.-S., Liu W.-X., Hong X.-Y., Wan F.-H. (2018a). Molecular Characterizations ofDNA Methyltransferase 3and its Roles in Temperature Tolerance in the whitefly,Bemisia tabaciMediterranean. Insect Mol. Biol. 27, 123–132. doi:10.1111/imb.12354
Dai T.-M., Wang Y.-S., Liu W.-X., Lü Z.-C., Wan F.-H. (2018b). Thermal Discrimination and Transgenerational Temperature Response in Bemisia Tabaci Mediterranean (Hemiptera: Aleyrodidae): Putative Involvement of the Thermo-Sensitive Receptor BtTRPA. Environ. Entomol. 47, 204–209. doi:10.1093/ee/nvx202
De Barro P. J., Liu S.-S., Boykin L. M., Dinsdale A. B. (2011). Bemisia Tabaci: A Statement of Species Status. Annu. Rev. Entomol. 56, 1–19. doi:10.1146/annurev-ento-112408-085504
Dillon M. E., Liu R., Wang G., Huey R. B. (2012). Disentangling thermal Preference and the thermal Dependence of Movement in Ectotherms. J. Therm. Biol. 37, 631–639. doi:10.1016/j.jtherbio.2012.07.004
Erkina T. Y., Zou Y., Freeling S., Vorobyev V. I., Erkine A. M. (2010). Functional Interplay between Chromatin Remodeling Complexes RSC, SWI/SNF and ISWI in Regulation of Yeast Heat Shock Genes. Nucleic Acids Res. 38, 1441–1449. doi:10.1093/nar/gkp1130
Gibert P., Moreteau B., Pétavy G., Karan D., David J. R. (2001). Chill-coma Tolerance, a Major Climatic Adaptation Among Drosophila Species. Evol 55, 1063–1068. doi:10.1554/0014-3820(2001)055[1063:cctamc]2.0.co;2
Guo D., Zhao G., Li G., Wang C., Wang H., Liu Z., et al. (2021). Identification of a Mitogen‐activated Protein Kinase Kinase ( AccMKK4 ) from Apis cerana Cerana and its Involvement in Various Stress Responses. Insect Mol. Biol. 30, 325–339. doi:10.1111/imb.12698
Gutierrez A. P., Ponti L. (2014). Analysis of Invasive Insects: Links to Climate Change. Invasive Species Glo 4, 45–61. doi:10.1079/9781780641645.0045
Head L. M., Tang X., Hayley S. E., Goda T., Umezaki Y., Chang E. C., et al. (2015). The Influence of Light on Temperature Preference in Drosophila. Curr. Biol. 25, 1063–1068. doi:10.1016/j.cub.2015.02.038
Hopfner K.-P., Gerhold C.-B., Lakomek K., Wollmann P. (2012). Swi2/Snf2 Remodelers: Hybrid Views on Hybrid Molecular Machines. Curr. Opin. Struct. Biol. 22, 225–233. doi:10.1016/j.sbi.2012.02.007
Hu J., De Barro P., Zhao H., Wang J., Nardi F., Liu S.-S. (2011). An Extensive Field Survey Combined with a Phylogenetic Analysis Reveals Rapid and Widespread Invasion of Two Alien Whiteflies in China. Plos One 6, e16061. doi:10.1371/journal.pone.0016061
Hu Y., Zhu N., Wang X., Yi Q., Zhu D., Lai Y., et al. (2013). Analysis of rice Snf2 Family Proteins and Their Potential Roles in Epigenetic Regulation. Plant Physiol. Biochem. 70, 33–42. doi:10.1016/j.plaphy.2013.05.001
Jabba S., Goyal R., Sosa-Pagán J. O., Moldenhauer H., Wu J., Kalmeta B., et al. (2014). Directionality of Temperature Activation in Mouse TRPA1 Ion Channel Can Be Inverted by Single-point Mutations in Ankyrin Repeat Six. Neuron 82, 1017–1031. doi:10.1016/j.neuron.2014.04.016
Ji S.-x., Shen X.-n., Liang L., Wang X.-d., Liu W.-x., Wan F.-h., et al. (2020). Molecular Characteristics and Temperature Tolerance Function of the Transient Receptor Potential in the Native Bemisia Tabaci AsiaII3 Cryptic Species. J. Integr. Agric. 19, 2746–2757. doi:10.1016/s2095-3119(20)63226-9
Johannes F., Porcher E., Teixeira F. K., Saliba-Colombani V., Simon M., Agier N., et al. (2009). Assessing the Impact of Transgenerational Epigenetic Variation on Complex Traits. Plos Genet. 5, e1000530. doi:10.1371/journal.pgen.1000530
Kumar S. (2018). Epigenomics of Plant Responses to Environmental Stress. Epigenomes 2, 6. doi:10.3390/epigenomes2010006
Kumar S., Stecher G., Tamura K. (2016). MEGA7: Molecular Evolutionary Genetics Analysis Version 7.0 for Bigger Datasets. Mol. Biol. Evol. 33, 1870–1874. doi:10.1093/molbev/msw054
Kumar S. V., Wigge P. A. (2010). H2A.Z-containing Nucleosomes Mediate the Thermosensory Response in Arabidopsis. Cell 140 (1), 136–147. doi:10.1016/j.cell.2009.11.006
Lancaster L. T. (2016). Widespread Range Expansions Shape Latitudinal Variation in Insect thermal Limits. Nat. Clim Change 6, 618–621. doi:10.1038/nclimate2945
Lee C. E. (2002). Evolutionary Genetics of Invasive Species. Trends Ecol. Evol. 17, 386–391. doi:10.1016/s0169-5347(02)02554-5
Li B., Carey M., Workman J. L. (2007). The Role of Chromatin during Transcription. Cell 128, 707–719. doi:10.1016/j.cell.2007.01.015
Liu J., Feng L., Gu X., Deng X., Qiu Q., Li Q., et al. (2019). An H3K27me3 Demethylase-HSFA2 Regulatory Loop Orchestrates Transgenerational Thermomemory in Arabidopsis. Cell Res 29, 379–390. doi:10.1038/s41422-019-0145-8
Liu S.-s., Colvin J., De Barro P. J. (2012). Species Concepts as Applied to the Whitefly Bemisia Tabaci Systematics: How many Species Are There? J. Integr. Agric. 11, 176–186. doi:10.1016/s2095-3119(12)60002-1
Liu Y. Q., Liu S. S. (2012). Species Status of Bemisia Tabaci Complex and Their Distributions in China. J. Biosafety 21, 247–255. doi:10.3969/j.issn.2095-1787.2012.04.002
Livak K. J., Schmittgen T. D. (2001). Analysis of Relative Gene Expression Data Using Real-Time Quantitative PCR and the 2−ΔΔCT Method. Methods 25, 402–408. doi:10.1006/meth.2001.1262
Lü Z.-C., Li Q., Liu W.-X., Wan F.-H. (2014a). Transient Receptor Potential Is Essential for High Temperature Tolerance in Invasive Bemisia Tabaci Middle East Asia Minor 1 Cryptic Species. Plos One 9, e108428. doi:10.1371/journal.pone.0108428
Lü Z.-C., Wang Y.-M., Zhu S.-G., Yu H., Guo J.-Y., Wan F.-H. (2014b). Trade-offs between Survival, Longevity, and Reproduction, and Variation of Survival Tolerance in Mediterranean Bemisia Tabaci after Temperature Stress. J. Insect Sci. 14, 124. doi:10.1093/jis/14.1.124
Lü Z. C., Wan F. H. (2008). Differential Gene Expression in Whitefly (Bemisia Tabaci) B-Biotype Females and Males under Heat-Shock Condition. Comp. Biochem. Phys. D 3, 257–262. doi:10.1016/j.cbd.2008.06.003
Lü Z. C., Wan F. H. (2011). Using Double-Stranded RNA to Explore the Role of Heat Shock Protein Genes in Heat Tolerance in Bemisia Tabaci (Gennadius). J. Exp. Biol. 214, 764–769. doi:10.1242/jeb.047415
Ma F.-Z., Lü Z.-C., Wang R., Wan F.-H. (2014). Heritability and Evolutionary Potential in thermal Tolerance Traits in the Invasive Mediterranean Cryptic Species of Bemisia Tabaci (Hemiptera: Aleyrodidae). Plos One 9, e103279. doi:10.1371/journal.pone.0103279
MacLean H. J., Overgaard J., Kristensen T. N., Lyster C., Hessner L., Olsvig E., et al. (2019). Temperature Preference across Life Stages and Acclimation Temperatures Investigated in Four Species of Drosophila. J. Therm. Biol. 86, 102428. doi:10.1016/j.jtherbio.2019.102428
Mahadav A., Kontsedalov S., Czosnek H., Ghanim M. (2009). Thermotolerance and Gene Expression Following Heat Stress in the Whitefly Bemisia Tabaci B and Q Biotypes. Insect Biochem. Mol. Biol. 39, 668–676. doi:10.1016/j.ibmb.2009.08.002
Marfella C. G. A., Imbalzano A. N. (2007). The Chd Family of Chromatin Remodelers. Mutat. Research/Fundamental Mol. Mech. Mutagenesis 618, 30–40. doi:10.1016/j.mrfmmm.2006.07.012
Martin T. L., Huey R. B. (2008). Why “Suboptimal” Is Optimal: Jensen’s Inequality and Ectotherm thermal Preferences. Am. Nat. 171, 102–118. doi:10.1086/527502
Miles P. W. (1965). Studies on the Salivary Physiology of Plant-Bugs: The Salivary Secretions of Aphids. J. Insect Physiol. 11, 1261–1268. doi:10.1016/0022-1910(65)90119-8
Mirouze M., Paszkowski J. (2011). Epigenetic Contribution to Stress Adaptation in Plants. Curr. Opin. Plant Biol. 14, 267–274. doi:10.1016/j.pbi.2011.03.004
Mitchell K. A., Hoffmann A. A. (2010). Thermal Ramping Rate Influences Evolutionary Potential and Species Differences for Upper thermal Limits inDrosophila. Funct. Ecol. 24, 694–700. doi:10.1111/j.1365-2435.2009.01666.x
Mohrmann L., Verrijzer C. P. (2005). Composition and Functional Specificity of SWI2/SNF2 Class Chromatin Remodeling Complexes. Biochim. Biophys. Acta (Bba) - Gene Struct. Expr. 1681, 59–73. doi:10.1016/j.bbaexp.2004.10.005
Muñoz-Valencia V., González-Obando R., Díaz F. (2016). Rapid Responses to a strong Experimental Selection for Heat Hardening in the Invasive Whitefly Bemisia Tabaci MEAM 1. Entomol. Exp. Appl. 160, 147–155. doi:10.1111/eea.12466
Musolin D. L. (2007). Insects in a Warmer World: Ecological, Physiological and Life-History Responses of True Bugs (Heteroptera) to Climate Change. Glob. Change Biol 13, 1565–1585. doi:10.1111/j.1365-2486.2007.01395.x
Oliveira M. R. V., Henneberry T. J., Anderson P. (2001). History, Current Status, and Collaborative Research Projects for Bemisia Tabaci. Crop Prot. 20 (9), 709–723. doi:10.1016/s0261-2194(01)00108-9
Pfaffl M. W. (2001). A New Mathematical Model for Relative Quantification in Real-Time RT-PCR. Nucleic Acids Res. 29, 45e–45. doi:10.1093/nar/29.9.e45
Secco D., Wang C., Shou H., Schultz M. D., Chiarenza S., Nussaume L., et al. (2015). Stress Induced Gene Expression Drives Transient DNA Methylation Changes at Adjacent Repetitive Elements. Elife 4, e09343. doi:10.7554/eLife.09343
Sen P., Ghosh S., Pugh B. F., Bartholomew B. (2011). A New, Highly Conserved Domain in Swi2/Snf2 Is Required for SWI/SNF Remodeling. Nucleic Acids Res. 39, 9155–9166. doi:10.1093/nar/gkr622
Sørensen J. G., White C. R., Duffy G. A., Chown S. L. (2018). A Widespread Thermodynamic Effect, but Maintenance of Biological Rates through Space across Life's Major Domains. Proc. Biol. Sci. 285, 20181775. doi:10.1098/rspb.2018.1775
Sørensen J. G., Kristensen T. N., Overgaard J. (2016). Evolutionary and Ecological Patterns of thermal Acclimation Capacity in Drosophila: Is it Important for Keeping up with Climate Change? Curr. Opin. Insect Sci. 17, 98–104.
Tratter Kinzner M., Kinzner M.-C., Kaufmann R., Hoffmann A. A., Arthofer W., Schlick-Steiner B. C., et al. (2019). Is Temperature Preference in the Laboratory Ecologically Relevant for the Field? the Case of Drosophila Nigrosparsa. Glob. Ecol. Conservation 18, e00638. doi:10.1016/j.gecco.2019.e00638
Tsukiyama T., Becker P. B., Wu C. (1994). ATP-dependent Nucleosome Disruption at a Heat-Shock Promoter Mediated by Binding of GAGA Transcription Factor. Nature 367, 525–532. doi:10.1038/367525a0
Tsukiyama T., Daniel C., Tamkun J., Wu C. (1995). ISWI, a Member of the SWl2/SNF2 ATPase Family, Encodes the 140 kDa Subunit of the Nucleosome Remodeling Factor. Cell 83, 1021–1026. doi:10.1016/0092-8674(95)90217-1
Wagner F. R., Dienemann C., Wang H., Stützer A., Tegunov D., Urlaub H., et al. (2020). Structure of SWI/SNF Chromatin Remodeller RSC Bound to a Nucleosome. Nature 579, 448–451. doi:10.1038/s41586-020-2088-0
Wang X., Yang N. (2017). “The Whitefly Bemisia Tabaci (Gennadius),” in Biological Invasions and its Management in China. Editors F. H. Wan, M. X. Jiang, and A. B. Zhan (Dordrecht: Springer Netherlands), 159–182. doi:10.1007/978-94-024-0948-2_8
Whelan S., Goldman N. (2001). A General Empirical Model of Protein Evolution Derived from Multiple Protein Families Using a Maximum-Likelihood Approach. Mol. Biol. Evol. 18, 691–699. doi:10.1093/oxfordjournals.molbev.a003851
Yadon A. N., Tsukiyama T. (2011). SnapShot: Chromatin Remodeling: ISWI. Cell 144, 453. doi:10.1016/j.cell.2011.01.019
Keywords: Bemisia tabaci, BRM, chromatin remodeling factors, ISWI, SWI/SNF, thermal stress
Citation: Ji S-X, Wang X-D, Lin Z-K, Wan F-H, Lü Z-C and Liu W-X (2022) Characterization of Chromatin Remodeling Genes Involved in Thermal Tolerance of Biologically Invasive Bemisia tabaci. Front. Physiol. 13:865172. doi: 10.3389/fphys.2022.865172
Received: 06 February 2022; Accepted: 13 April 2022;
Published: 20 May 2022.
Edited by:
Zhijun Yu, Hebei Normal University, ChinaReviewed by:
Fernando Diaz, University of Arizona, United StatesXiudao Yu, Gannan Normal University, China
Copyright © 2022 Ji, Wang, Lin, Wan, Lü and Liu. This is an open-access article distributed under the terms of the Creative Commons Attribution License (CC BY). The use, distribution or reproduction in other forums is permitted, provided the original author(s) and the copyright owner(s) are credited and that the original publication in this journal is cited, in accordance with accepted academic practice. No use, distribution or reproduction is permitted which does not comply with these terms.
*Correspondence: Zhi-Chuang Lü, bHZ6aGljaHVhbmdAY2Fhcy5jbg==