- 1Key Laboratory of Animal Breeding Reproduction and Molecular Design for Jiangsu Province, College of Animal Science and Technology, Yangzhou University, Yangzhou, China
- 2Joint International Research Laboratory of Agriculture and Agri-Product Safety of Ministry of Education of China, Yangzhou University, Yangzhou, China
- 3College of Biotechnology, Jiangsu University of Science and Technology, Zhenjiang, China
Background: Sex differentiation is a complex and precisely regulated process by multiple genes in chicken. However, it is still unclear on the key genes of sex differentiation.
Objective: To explore the function of Tle4z1 screened by RNA-seq sequencing on sex differentiation during the development of chicken embryos.
Methods: Tle4z1 was differentially expressed from the RNA-seq of ESCs and PGCs in male and female chickens. Then, we established an effective method to overexpression or knocking down the expression of Tle4z1 in ovo and in vitro, respectively. Histomorphological observation, qRT-PCR and ELISA were applied to detect the function of Tle4z1 in the process of male sex differentiation by injecting vectors into embryos at day 0.
Results: It showed that Tle4z1 has significant male preference in embryonic day 4.5, such phenomenon persisted during the growth period of chicken embryos. Morphological observation results showed that the gonads on both sides of genetic male (ZZ) embryos with Tle4z1 knocking down developed asymmetrically, the gonadal cortex became thicker showing the typical characteristics of genetic female (ZW) gonads. Furthermore, the expression of Cyp19a1, which dominates female differentiation, was significantly increased, while the expression of male marker genes Dmrt1, Sox9, WT1 and AR was significantly downregulated. In addition, the concentration of testosterone also significantly decreased, which was positively correlated with the expression of Tle4z1 (P < 0.01). Conversely, the ZW embryo showed defeminized development when Tle4z1 was overexpressed.
Conclusion: We prove that the Tle4z1 is a novel gene through the male sexual differentiation via gene regulation process and synthesis of testosterone, which construct the basis for understanding the molecular mechanism of sex differentiation in chickens.
Introduction
Gender control technology is an important means to affect the economic development of livestock and poultry industry. The development of this technology can achieve single-sex directional breeding in the early sex differentiation of poultry to produce meat and eggs products with different gender needs. Existing studies have shown that the mode of sex determination is genotypic sex determination (GSD) in chicken (Wilhelm et al., 2007), but the molecular mechanism of sex differentiation is not clear, which has become an important factor restricting monosex culture of chicken. Therefore, exploring the key genes in the process of sex differentiation and investigating its biological functions are the basic premise to clarify the molecular mechanism of sex differentiation. Studies show that Dmrt1 located on Z chromosome can regulate gonadal differentiation in chicken. When knockdown Dmrt1 by RNA interference, it showed that the expression of DMRT1 decreased in vivo (Smith and Sinclair, 2004), hereditary male (ZZ) embryos showed feminized development, while the expression of Sox9 was downregulated, which confirmed that DMRT1 can regulate the expression of Sox9, and both jointly regulate testicular development (Hirst et al., 2018; Yamashita et al., 2019). Anti-Müllerian hormone was found to be expressed in both sexes of chicken embryos before Sox9. And the upregulation of sox9 expression will increase the secretion of anti-mullerian hormone expression (Oreal et al., 1998; Lan et al., 2013). Moreover, WT1 was found to promote the formation of sertoli cells, but all above genes were not the sex differentiation switching factors in chicken (Wen et al., 2016). With the in-depth study of sex determination and sex differentiation in poultry, the theories of cell-autonomous, hormone effect on sex determination, hypothesis of the dose effect of Z chromosome and the dominant effect of W chromosome have been put forward (Scheib, 1983; Lin et al., 1995; Graves, 2014; Zimmer et al., 2016). The essence of these hypotheses is to control the process of sex development by regulating the expression of downstream genes, so it is very important to screen the key genes in the process of sex determination and differentiation.
Based on the existing research, the research used ESCs and PGCs as materials for RNA-seq. To obtain the differentially expressed genes between the sexes during the ESCs and PGCs periods, the data is analyzed for grouping and comparison by Venn. According to its chromosome location, the key candidate gene Tle4z1, for sex differentiation on chicken Z chromosome was screened and its function in the process of sex differentiation was explored. Tle4z1 (transducin like enhancer of split4), a member of Tle/gro family, which acts as a co-factor, is specifically and highly expressed in embryonic stem cells and play an important role in early embryonic differentiation (Chen and Courey, 2000). Tle4 in mouse was discovered to promote embryonic stem cell differentiation (Laing et al., 2015). Gro is a transcriptional corepressor recruited to specific target promoters by hairy-related bHLH proteins to affect neurogenesis, segmentation and sex determination in Drosophila in vivo maternal requirements (Paroush et al., 1994). Meanwhile, Tle4, a member of the Tle/Gro family, has an effect on the asymmetric development of dorsal ventral patterns in vertebrates (Buscarlet and Stifani, 2007). However, the role of Tle4z1 on chicken Z chromosome in the process of sex determination and sex differentiation has not been reported.
The basis of the results in transcriptome sequencing, the key candidate gene Tle4z1 for male differentiation of chicken embryo was screened. To analyze the function of Tle4z1 in the process of chicken embryo sex differentiation, RNA interference and overexpression system was used to explore the direction of gonadal phenotypic differentiation, sex-related genes and testosterone expression changes after interference (ZZ embryo) and overexpression (ZW embryo) of Tle4z1 in chicken.
Materials and Methods
Ethics Statement and Experimental Animals
The procedures involving animals and their care conformed to the United States National Institute of Health guidelines (NIH Pub. No. 85–23, revised 1996). The experiments were conducted under the approval of the Ethics Committee of Yangzhou University for Laboratory and Experimental Animals. The fertilized eggs of Rugao yellow chicken were purchased from the Poultry Research Institute of Chinese Academy of Agricultural Sciences and hatched under the environment of 37°C with 70% humidity.
Sample Collection
The experimental eggs were hatched after 0 days of blunt-end injection. A total of three groups were divided into blank, sh-Tle4z1 and oe-Tle4z1 group, with 140 fertilized eggs in each group. Embryos at different stages were collected according to the chicken embryo development map (Hamburger Hamilton stage) and stored in −80°C for gonadal isolation and extraction.
The organs, such as heart, liver, spleen, lung, kidney, intestine, eye, ear, brain and gonad of chickens were collected from healthy roosters and hens, which growing in great feeding conditions, and were used to detect the expression profile of Tle4z1 organs. Blood samples were taken for sex identification.
Isolation of Chicken ESCs and PGCs
ESCs were derived from E0 chicken embryos and PGCs from the genital ridge of embryos at E4.5. All eggs were incubated under the environment of 37.5°C and 65% relative humidity. The separation and cultivation detailed steps of ESCs and PGCs are introduced in previous article (Zhang et al., 2015). Isolated ESCs and PGCs were used to detect the expression of Tle4z1 by qRT-PCR.
Construction of sh-Tle4z1 Lentivirus Interference Vector
According to the chicken Tle4z1 (NM_204237.1) gene sequence provided by Genebank, the RNA interference target sequence was designed (Supplementary Table 1), and the oligo sequence was synthesized(Supplementary Table 2). After annealing, the double-stranded DNA vector was formed. The ligated products were transformed into Escherichia coli supercells overnight culture, positive single bacteria were sequenced, and the correct sequences were compared. The plasmid was extracted and packaged with lentivirus (5 × 108TU/ml). The vector was transfected into DF-1 cells and the fluorescence expression was observed 72 h. The RNA was reverse transcribed into cDNA, and the interference efficiency was detected by qRT-PCR.
Construction of oe-Tle4z1 Lentivirus Overexpression Vector
The full-length cDNA sequence of Tle4z1 was obtained by using the cDNA of chicken PGCs as template. The amplification primer sequence is as follows: F: ATGATCCGCGACCTGAGCAAG; R: CTAATAAATAACTTCATAGACTGTAGCTTTCTTGTCCC CA. The target fragment was ligated with the enzyme-digested pCDH-CMV-MCS-EF1-TagRFP + Puro, and the competent cells were transformed and cultured overnight. The positive colonies were sequenced, and the plasmids were extracted for lentivirus packaging (1 × 108TU/ml). The vector was transfected into DF-1 cells, and the overexpression efficiency was detected.
Blunt Injection of Chicken Embryo
The MOI of lentiviral vector was optimized to be 10. The lentivirus concentrate of overexpression group (oe-Tle4z1) and interference group (sh-Tle4z1) was diluted with DMEM according to the ratio of 100 μL of each egg to the blunt end of E0. After being sealed with paraffin, the eggs were incubated normally at 38°C.
Sex Identification
Genomic DNA from blood was extracted to identify the sex of the embryo. Amplification was performed using CHD1 specific primers (CHD1-F: CTGCGAGAACGTGGCAACAGAGT; CHD1-R: ATTGAAATGATCCAGTGCTTG). The results showed that the hereditary female (ZW) had two strips at 580 and 423 bp, while the hereditary male (ZZ) had only one strip at 580 bp.
Paraffin Sections and Hematoxylin-Eosin Staining
The embryonic gonad of E18.5 was fixed with 4% paraformaldehyde for 24 h, and transferred to 70–100% ethanol was dehydrated. The tissue was embedded in paraffin after being immersed in xylene for 1 h. The paraffin tissue was continuously sliced with a thickness of 5–6 μm and baked at 55–60°C overnight. The slices were dewaxed with xylene and the ethanol concentration was permeated with water from high to low gradient. The treated slices were stained, soaked in hematoxylin dye for 2.5 min, rinsed under flowing water for 10 min, then soaked in eosin staining for 3–5 min, rinsed with flowing water for 5 min, dehydrated and sealed. The sections were observed under microscope.
Morphological Statistics
The intact fetal gonads were taken. The hereditary female and male gonads of the control group, 15 pairs of male gonads of the interference group and female gonads of the overexpression group were set. The morphology was taken under stereoscopic microscope, and the surface area of gonads was measured, and the ratio of surface area of bilateral gonads was calculated.
qRT-PCR
Total RNA was extracted from tissues and cells to synthesize cDNA by reverse transcription. The internal reference gene: β-actin, n = 3. And primers are shown in the Supplementary Table 3. The PCR reaction was carried out in the condition of 95°C 30 s, 95°C 10 s, 60°C 30 s, 40 cycles.
ELISA
The gonads at different developmental stages were weighed and crushed with PBS, and the supernatant was taken after 3,000 rpm centrifugation for 25–30 min at 4°C. The hormone of testosterone was detected with an ELISA kit for color development, and the absorbance value was detected at 450 nm. According to the concentration and OD value, the testosterone content of each group was analyzed.
Statistics
Relative gene expression was calculated using the 2-ΔΔCt method after qPCR. All experiments were performed in triplicate, and the data are presented as mean ± standard error. Significant differences between the groups were determined with two-sample t-tests in SPSS 17.0. (*P < 0.05, significant difference. **P < 0.01, extremely significant difference, ***P < 0.001, ****P < 0.0001). Prism7 software was used for mapping.
Results
Screening of Tle4z1, a Key Candidate Gene for Sex Differentiation
At different development stages of chicken embryos, germ cells were carried out by RNA-seq (Jin et al., 2020a). According to the result, 1021 differentially expressed genes (DEGs) were found in ESCs, including 517 DEGs in males and 504 DEGs in females, while 8,057 differentially expressed genes in PGCs, including 5,833 DEGs in males and 2,674 DEGs in females. In order to further study, the data of transcriptome sequencing between ESCs and PGCs between male and female were analyzed by Venn to find key candidate genes in the process of male sexual differentiation in chicken. 242 DEGs were screened. Map these genes on the chromosomes and watch out that there are six male highly expressed genes located on the Z chromosome (Figure 1A).
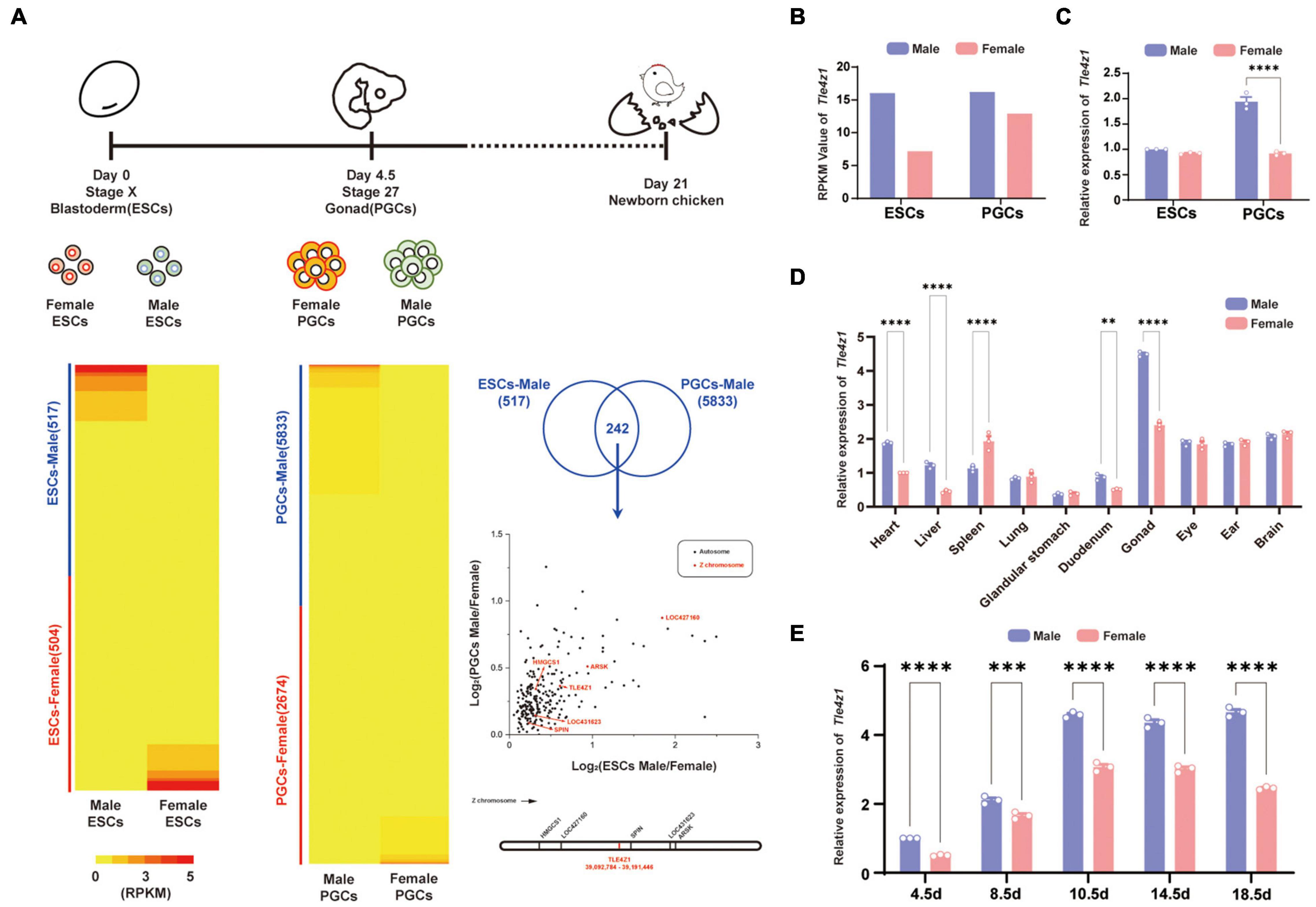
Figure 1. The sexually dimorphic expression of Tle4z1 in cells and tissues of chicken. (A) Experimental design of RNA-seq analysis (top). RPKM of differentially expressed genes(DEGs) in ESCs and PGCs of different genders (left bottom). Filter out male-specific high expression genes of Z chromosome (right). Venn analysis screened out 242 DEGs shared in male PGCs and ESCs. Volcano plot where the x-axis represents the level of differential expression of PGCs and the y-axis shows significant differences in expression of ESCs in both sexes. Genes in Z chromosome are indicated by red dots, and autosome chromosome genes are indicated by black dots. The diagram marks the locations of six male highly-expressed genes (right bottom). (B) RPKM of Tle4z1 in ESCs and PGCs of different genders. (C) Validation of mRNA expression of Tle4z1 by qRT-PCR. The expression of Tle4z1 in male ESCs was defined as 1 after normalization to β-actin. (D) Relative expression of Tle4z1 in adult chicken tissues as measured by qRT-PCR. (E) Relative expression of Tle4z1 during the development of chicken embryos by extraction of RNA from gonads at different stages (E4.5-E18.5). **P < 0.01, ***P < 0.001, ****P < 0.0001. All values are mean ± SEM. n = 3.
Among them, the expression of Tle4z1 appears male preference in ESCs and PGCs (Figure 1B), and we speculate that it may play a certain role in sex determination and differentiation. Further quantitative results showed that the expression of Tle4z1 in males was significantly higher than that in females in the stage of PGCs (P < 0.0001) (Figure 1C). Detection of adult chicken in different tissues also found that its specific high expression in testicular tissue, and the expression of Tle4z1 in male gonads was higher than that of female (P < 0.01) (Figure 1D). This phenomenon of sex preference expression also exists in the gonads of chicken embryos. From the development of chicken embryo E4.5, the level of Tle4z1 mRNA expression in male chicken embryo was significantly higher than that in female (P < 0.0001) (Figure 1E). These results suggest that Tle4z1, as a male-specific gene, may be involved in the process of male sex differentiation in chicken embryos.
Construction of Overexpression and Interference Lentiviral Vector of Tle4z1
In order to verify the function of Tle4z1 in the process of sex differentiation of chicken embryo, lentivirus interference and overexpression vector of Tle4z1 were designed and constructed (Figure 2A). The constructed vector with GFP fluorescent protein labeling was transfected into DF-1 cells. A large number of fluorescent expressions was observed 48 h later, indicating that the vector can be effectively expressed in vitro. The result showed that after interfering with Tle4z1, the level of Tle4z1 mRNA was downregulated. The interference activity of shRNA2-Tle4z1 was the strongest among the three shRNA targets (P < 0.05), so the target was selected to package lentivirus for follow-up experiments (Figure 2B). Meanwhile, the expression of Tle4z1 increased significantly after overexpression (P < 0.001) (Figure 2C). Further experiments showed that in the E4.5-E18.5 of chicken embryos, the mRNA level of Tle4z1 in female gonads of overexpression group was significantly higher than that of control group in vivo (P < 0.001) (Figure 2E), and Tle4z1 was inhibited after interference treatment in male gonads (P < 0.001) (Figure 2D), indicating that the recombinant vector could effectively interfere/overexpress Tle4z1 during chicken embryo development.
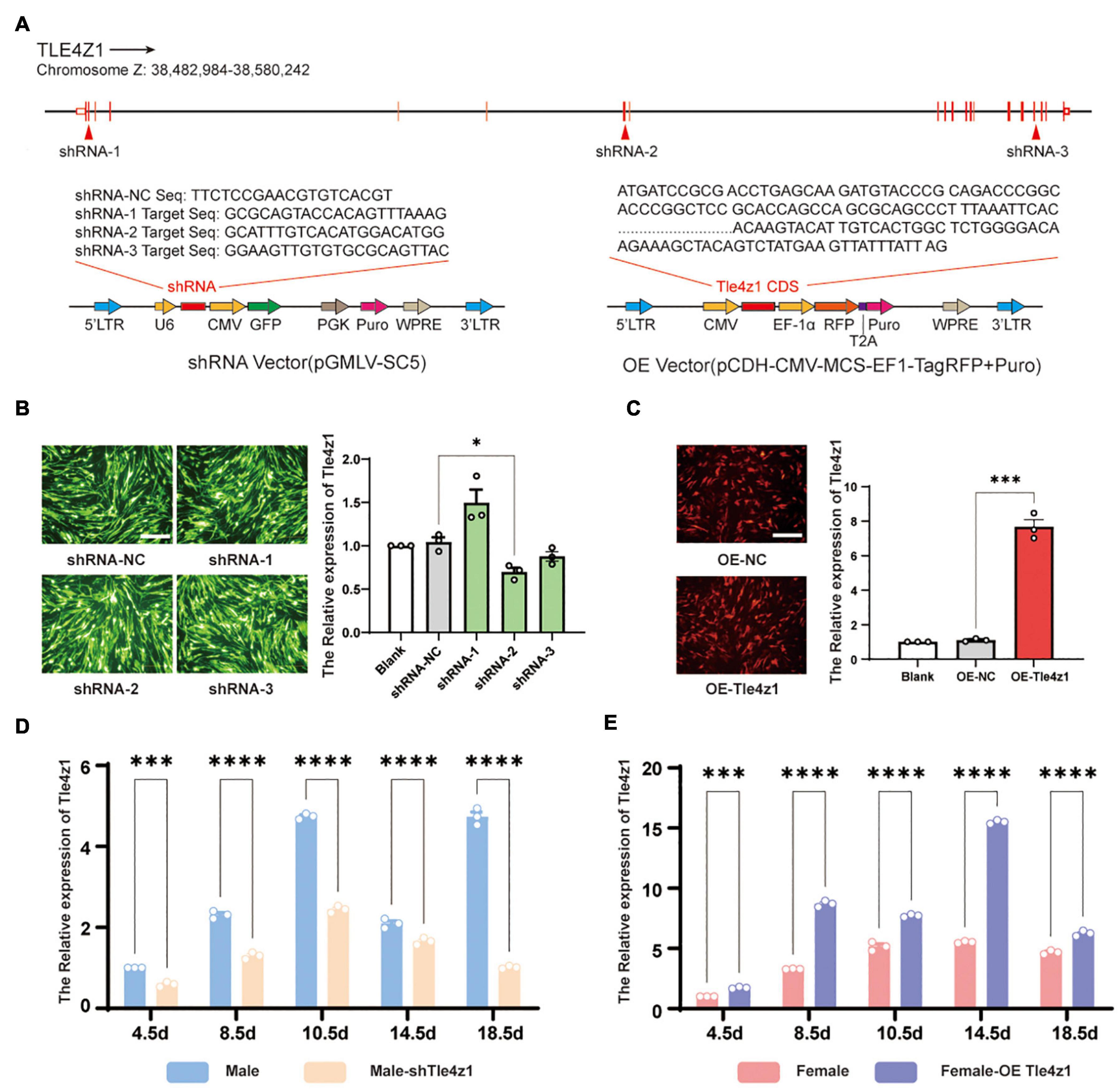
Figure 2. Construction and activity verification of Tle4z1 RNA interference/overexpression vector. (A) RNA interference and overexpression vector design of Tle4z1. (B) The GFP fluorescence was detected in DF-1 cells in vitro indicating effective lentivirus infection. Relative expression of Tle4z1 in DF1 cells transfected with lentiviral shRNA vectors for 48 h as measured by qRT-PCR. 2#shRNA vector has best effect on interfering the expression of Tle4z1. Scale bars = 100 μm. (C) The RFP fluorescence was detected in DF-1 cells in vitro indicating effective lentivirus infection. Relative expression of Tle4z1 in DF1 cells transfected with lentiviral overexpression vector for 48 h as measured by qRT-PCR. Scale bars = 100 μm. (D) Compared with normal male chicken embryos, the relative expression of Tle4z1 in male chicken embryos at different stages of development after blunt end injection of lentiviral interference vector in vivo. (E) Compared with normal female chicken embryos, the relative expression of Tle4z1 in female chicken embryos at different stages of development after blunt end injection of lentiviral overexpression vector in vivo. *P < 0.05, **P < 0.01, ***P < 0.001, ****P < 0.0001. All values are mean ± SEM. n = 3.
Tle4z1 Promotes Virilization of Chicken Embryonic Gonadal Phenotype
In order to explore the effect of Tle4z1 on the phenotypic differentiation of gonads, the gonads of chicken embryos hatched to 18.5 days after blunt injection of vectors were observed (Figure 3A). The statistical results showed that after interfering with the expression of Tle4z1, there was a significant asymmetric development of bilateral testes in the experimental group compared with the control group (P < 0.0001), while the atrophy of the right ovary in the experimental group was alleviated after overexpression of Tle4z1 (Figure 3B). HE staining of the gonadal sections showed that the female gonads overexpressing Tle4z1 were significantly thinner than normal female embryos, showing male characteristics, while the cortex of gonads after interference with Tle4z1 was significantly thicker, showing female-related characteristics (Figure 3C). These results indicate that Tle4z1 plays an important role in the development of gonads in chicken embryo.
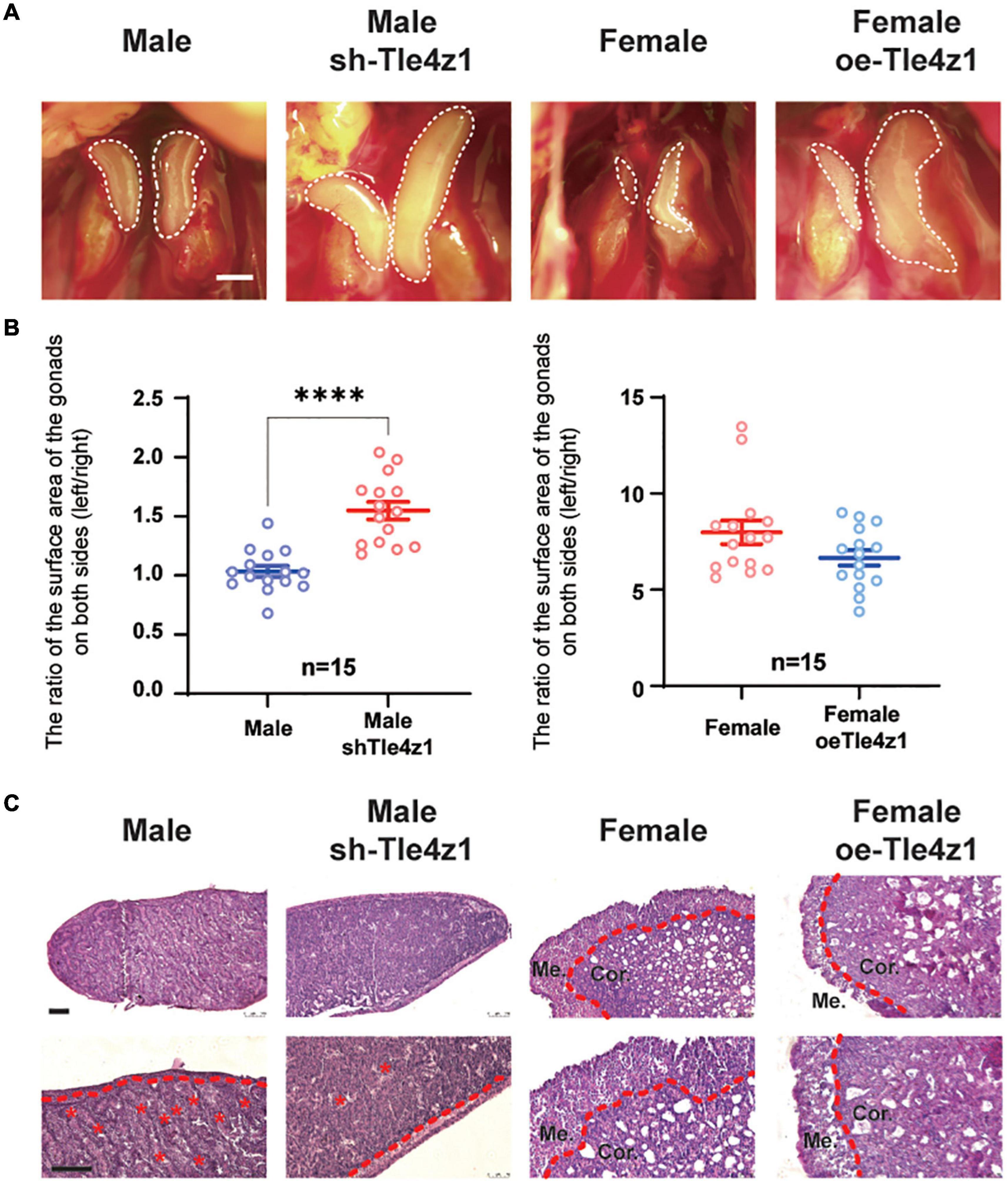
Figure 3. Tle4z1 promotes the differentiation of chicken embryo gonadal phenotype to male. (A) Phenotypic differentiation of gonadal morphology at E18.5. Left two pictures are the comparison of the morphological development difference of chicken embryo testis between control and interference group. Right two are ovarian morphology of the control and overexpression group. Scale bars = 2 mm. (B) Statistical analysis of the asymmetric development of the gonads on both sides of chicken embryos. The X-axis represents the group, and the Y-axis represents the ratio of the surface area of the gonads on both sides of the chicken embryo (left/right). ****P < 0.0001, values are means ± SEM. n = 15. (C) Photomicrographs of hematoxylin/eosin (H/E) staining of gonads cultured at E18.5. The dashed red line indicates the border between the medulla and cortex. Cor.: cortex layer; Me.: medulla layer; *tubuli seminiferi contorti; Scale bar = 100 μm.
Tle4z1—a Key Gene That Influences Sex Differentiation to Male
In this study, it has been found that Tle4z1 can affect the gonadal phenotypic differentiation of chicken embryos. We detected the sex differentiation genes by qRT-PCR. The results showed that at E18.5, the expression of genes related to male sex differentiation like Dmrt1, AR, WT1 and Sox9 in the gonads of ZZ embryos in treated group of interference decreased significantly, while the mRNA level of Cyp19a1, which dominate female differentiation, was significantly higher than that in normal ZZ embryos (P < 0.0001) (Figure 4A). After overexpressing Tle4z1, the expression of genes related to male differentiation, like Dmrt1, AR, WT1 and Sox9 increased significantly, while Cyp19a1 decreased significantly (P < 0.0001) (Figure 4C). The above results suggest that regulating the expression of Tle4z1 has an important effect on the sex differentiation of chicken embryos.
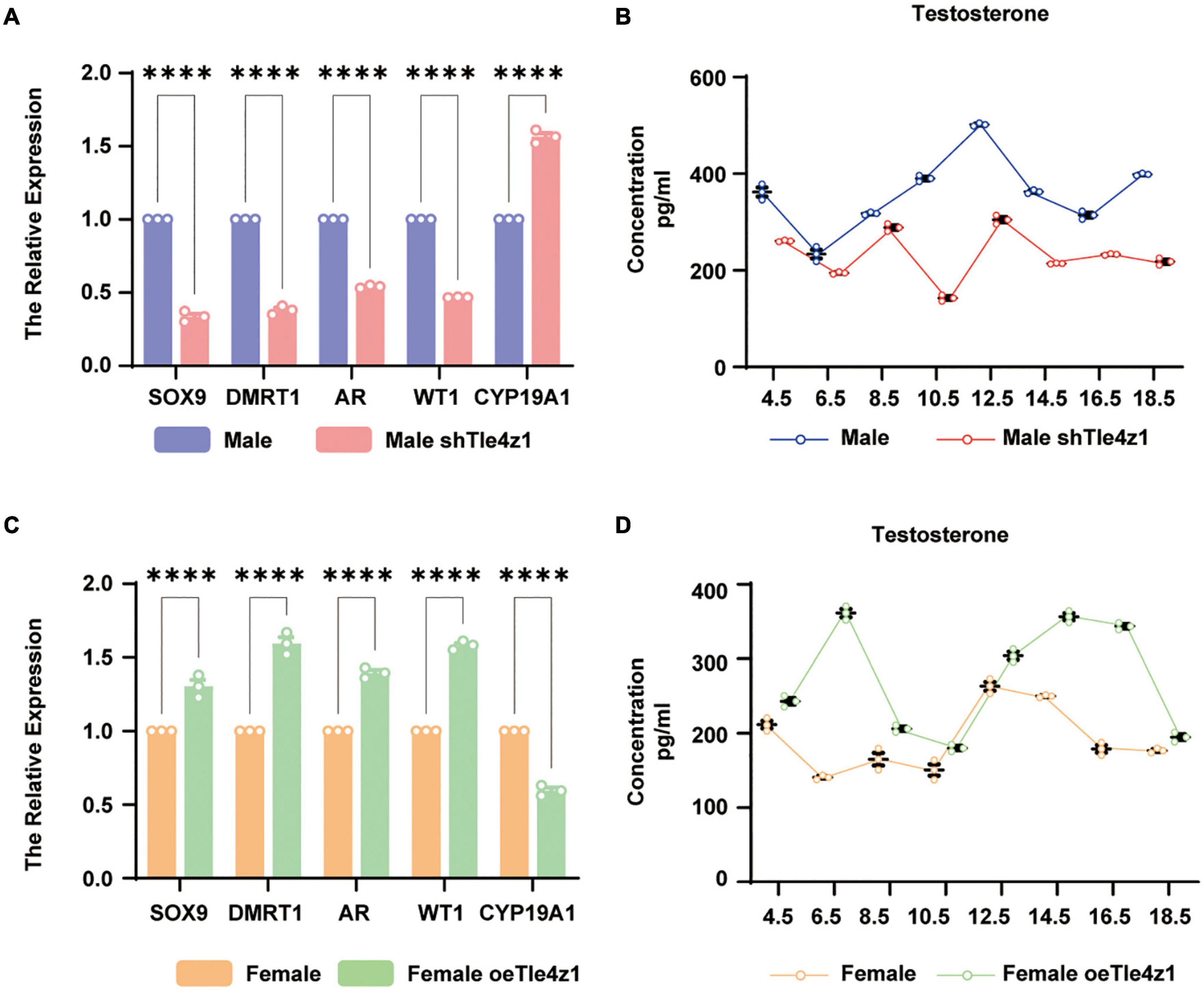
Figure 4. Tle4z1 promotes the sex differentiation of chicken embryos into males at mRNA and hormone levels. (A) Relative expression of sex-related genes (Sox9, DMRT1, AR, WT1 and CYP19A1) in male interference group at E18.5 by qRT-PCR. ****P < 0.0001, values are means ± SEM. n = 3. (B) Changes in the expression of testosterone in male interference group at E4.5, E6.5, E8.5, E10.5, E12.5, E14.5, E16.5, and E18.5 was detected by ELISA. (C) Relative expression of sex-related genes in female overexpression group at E18.5 by qRT-PCR. (D) Changes in the expression of testosterone in female overexpression group at E4.5, E6.5, E8.5, E10.5, E12.5, E14.5, E16.5, and E18.5 was detected by ELISA.
Recently, studies have shown that hormones are involved in the process of sex determination and differentiation of chicken embryos (Morris et al., 2018; Wang et al., 2019). For further research on the role of Tle4z1 in the process of sex differentiation of chicken embryos, this study also detected the changes of sex hormone levels during incubation E4.5-E18.5 after interfering or overexpressing Tle4z1 by ELISA. The results showed that testosterone in ZZ chicken embryos interfering with Tle4z1 expression decreased significantly and reached the lowest point at E18.5 (Figure 4B). On the contrary, the level of testosterone in gonad of ZW chicken embryo in overexpression treatment was significantly higher than that in normal female chicken embryo (P < 0.01) (Figure 4D). These results suggest that Tle4z1 can regulate the secretion of testosterone and play an important role in the male differentiation of chicken embryos.
Discussion
Scientists have never stopped exploring the topic of sex determination and sex differentiation in poultry. In mammals, sex determination and gonadal differentiation have been well-studied, but the unique egg-laying characteristics have hindered research in birds. In recent years, many key genes have also been discovered in sex differentiation. CRISPR/Cas9 system was used to find out that after knocking out DMRT1, the ZZ embryo testis had sex reversal, indicating that DMRT1 is crucial for testis development, and demonstrating the avian sex-determining mechanism is based on DMRT1 dosage (Ioannidis et al., 2021). Similarly, disruption of DMRT1 results in gonad feminization during the early stages of rooster development (Lee et al., 2021). Moreover, DMRT1 can directly or indirectly activate the expression of male factors HEMGN, SOX9 and AMH (Estermann et al., 2021). FOXD1 is an important marker of Sertoli cells upstream of SOX9 expression, and synergistically affects the differentiation and development of chicken embryo testis with Sox9 (Yu et al., 2019). Here, we successfully screened and identified a novel gene, Tle4z1, which plays an important role in male differentiation in chicken embryos. Functional validation results showed that interfering with Tle4z1 could inhibit normal sex differentiation in males, making them appear female, while overexpression of Tle4z1 could masculinize female embryos. At the same time, we found that Tle4z1 can regulate the expression of male marker genes such as Dmrt1, AR, WT1, Sox9 and testosterone.
Existing studies have shown that Tle4 generally affects the development of ear, eye and dorsal ventral nerve cells in the form of corepressor factor, but there are few studies on chickens (Zhu et al., 2002; Bajoghli et al., 2005; Tsuji and Hashimoto, 2005). We detected the expression of Tle4z1 in eyes, ears and brain of chickens, but it has no significant differential expression between male and female chickens in this study. We found that Tle4z1 was highly specifically expressed in male and female ESCs and PGCs. In combination with its expression of chicken embryo development stages, Tle4z1 was significantly more expressed in male than female chicken embryo gonads, suggesting that Tle4z1 may be involved in the differentiation process of male chicken embryos. Chicken is a typical model animal of asymmetric gonadal development. When chicken embryo develops to E8, the phenotypic changes of ovary can be distinguished, that is, the left ovary develops normally and the right ovary atrophies gradually. Injection of aromatase (Cyp19a1) into chicken embryos could reverse the embryonic phenotype of ZZ chicken (Hirst et al., 2018), but it had no effect on the gonadal morphology of ZW type. This phenomenon could only be temporarily affected by cell autonomy. In this study, we found that the asymmetric development of bilateral gonads of E18.5, ZW embryos was significantly improved after overexpression of Tle4z1, and the gonadal development of ZZ embryos showed a trend of asymmetric development in the interference group, which indicated that Tle4z1 could play a role in the gonadal development of both sexes and made the sexual phenotype of chicken embryos develop to male. The key role of this gene in the process of sex differentiation of chicken embryos was further verified by lentivirus interference and overexpression system. It was found that after interfering with the expression of Tle4z1 gene, the expression of male-specific genes Dmrt1, WT1, Sox9 was down-regulated, while the expression of female differentiation gene Cyp19a1 was up-regulated. The results of this study fully proved that the differentiation of Tle4z1 gene in the male gonad of chicken embryo is necessary.
In addition, sex differentiation and the maintenance of gonadal phenotype need to be regulated by hormone levels, and sex hormones play an indispensable role in maintaining the development of chicken embryos. The expression level of sex hormones in chicken embryos plays a key role in the sex differentiation of chicken embryos (Wang et al., 2019), so sex determination theory of TDF gene and the theory of cell autonomous sexual development are inseparable from the regulation of hormones, and the development of secondary sexual characteristics needs hormones to maintain. In our research, we detected the effect of Tle4z1 on testosterone level, and found that with the change of Tle4z1 gene expression, the testosterone level was always positively correlated with the expression level of Tle4z1. Androgen receptor gene AR is the target gene of androgen regulation, and testosterone can function through the targeted receptor gene AR (Wrobel et al., 2020). The expression of AR was detected at the transcriptional level, and it was found that it was also consistent with the change trend of Tle4z1 gene expression. All above shows that Tle4z1, as a key gene of male differentiation, participates in the process of sex differentiation of chicken embryos, which provides a theoretical basis for further exploration of the molecular mechanism of sex differentiation of chickens (Jin et al., 2020b; Jiang et al., 2021; Zhang et al., 2021).
In sum, we successfully screened and verified the key role of Tle4z1 in the process of sex differentiation from three aspects of morphology, mRNA and hormone, and revealed its function of promoting sex differentiation to male of chicken embryos, which provided new insights into the molecular mechanism of chicken sex differentiation.
Data Availability Statement
The data presented in the study are deposited in the Sequence Read Archive (SRA) database at National Center for Biotechnology Information (NCBI) repository, accession number PRJNA608148.
Ethics Statement
The animal study was reviewed and approved by Ethics Committee of Yangzhou University for Laboratory and Experimental Animals.
Author Contributions
BL, GC, and YZ contributed to conception and design of the study. KJ and QZ organized the database. XG, ZL, and CH performed the statistical analysis. JJ helped to perform the analysis with constructive discussions. CC performed the experiment and wrote the first draft of the article. CC and SZ wrote sections of the article. All authors contributed to article revision, read, and approved the submitted version.
Funding
This study was supported by the National Natural Science Foundation of China (31872341 and 31972547), the Belt and Road Innovation Cooperation Project of Jiangsu Science and Technology Program (BZ2019018), the Yangzhou Science and Technology Project (YZ2019146), the High-level talents support program of Yangzhou University, and the Project Funded by the Priority Academic Program Development of Jiangsu Higher Education Institutions.
Conflict of Interest
The authors declare that the research was conducted in the absence of any commercial or financial relationships that could be construed as a potential conflict of interest.
Publisher’s Note
All claims expressed in this article are solely those of the authors and do not necessarily represent those of their affiliated organizations, or those of the publisher, the editors and the reviewers. Any product that may be evaluated in this article, or claim that may be made by its manufacturer, is not guaranteed or endorsed by the publisher.
Acknowledgments
The authors would like to thank all of the participation of the family members in this study.
Supplementary Material
The Supplementary Material for this article can be found online at: https://www.frontiersin.org/articles/10.3389/fphys.2022.856980/full#supplementary-material
Abbreviations
AR, androgen receptor; Cyp19a1, cytochrome P450 family 19 subfamily A member 1; Dmrt1, doublesex and mab-3 related transcription factor 1; ESCs, Embryonic Stem Cells; E0, the 0 embryonic day; E4.5, the 4.5th embryonic day; E18.5, the 18.5th embryonic day; MOI, multiplicity of infection; PGCs, Primordial Germ Cells; qRT-PCR, Quantitative Real-time PCR; Sox9, SRY-box 9; Tle4z1, transducin like enhancer of split 4; WT1, Wilms tumor 1; sh- Tle4z1, shRNA- Tle4z1; oe- Tle4z1, overexpression- Tle4z1.
References
Bajoghli, B., Aghaallaei, N., and Czerny, T. (2005). Groucho corepressor proteins regulate otic vesicle outgrowth. Dev. Dynam. 233, 760–771. doi: 10.1002/dvdy.20398
Buscarlet, M., and Stifani, S. (2007). The ‘Marx’ of Groucho on development and disease. Trends Cell Biol. 17, 353–361. doi: 10.1016/j.tcb.2007.07.002
Chen, G., and Courey, A. J. (2000). Groucho/TLE family proteins and transcriptional repression. Gene 249, 1–16. doi: 10.1016/s0378-1119(00)00161-x
Estermann, M. A., Major, A. T., and Smith, C. A. (2021). Genetic Regulation of Avian Testis Development. Genes 12:1459. doi: 10.3390/genes12091459
Graves, J. A. (2014). Avian sex, sex chromosomes, and dosage compensation in the age of genomics. Chromos. Res. 22, 45–57. doi: 10.1007/s10577-014-9409-9
Hirst, C. E., Major, A. T., and Smith, C. A. (2018). Sex determination and gonadal sex differentiation in the chicken model. Int. J. Dev. Biol. 62, 153–166. doi: 10.1387/ijdb.170319cs
Ioannidis, J., Taylor, G., Zhao, D., Liu, L., Idoko-Akoh, A., Gong, D., et al. (2021). Primary sex determination in birds depends on DMRT1 dosage, but gonadal sex does not determine adult secondary sex characteristics. Proc. Natl. Acad. Sci. U S A. 118:e2020909118. doi: 10.1073/pnas.2020909118
Jiang, J., Zhang, C., Yuan, X., Li, J., Zhang, M., Shi, X., et al. (2021). Spin1z induces the male pathway in the chicken by down-regulating Tcf4. Gene 780:145521. doi: 10.1016/j.gene.2021.145521
Jin, K., Zhou, J., Zuo, Q., Song, J., Zhang, Y., Chang, G., et al. (2020a). Transcriptome Sequencing and Comparative Analysis of Amphoteric ESCs and PGCs in Chicken (Gallus gallus). Animals 10:2228. doi: 10.3390/ani10122228
Jin, K., Zuo, Q., Song, J., Zhang, Y., Chen, G., and Li, B. (2020b). CYP19A1 (aromatase) dominates female gonadal differentiation in chicken (Gallus gallus) embryos sexual differentiation. Biosci. Rep. 40:BSR20201576. doi: 10.1042/BSR20201576
Laing, A. F., Lowell, S., and Brickman, J. M. (2015). Gro/TLE enables embryonic stem cell differentiation by repressing pluripotent gene expression. Dev. Biol. 397, 56–66. doi: 10.1016/j.ydbio.2014.10.007
Lan, K. C., Chen, Y. T., Chang, C., Chang, Y. C., Lin, H. J., Huang, K. E., et al. (2013). Up-regulation of SOX9 in sertoli cells from testiculopathic patients accounts for increasing anti-mullerian hormone expression via impaired androgen receptor signaling. PLoS One 8:e76303. doi: 10.1371/journal.pone.0076303
Lee, H. J., Seo, M., Choi, H. J., Rengaraj, D., Jung, K. M., Park, J. S., et al. (2021). DMRT1 gene disruption alone induces incomplete gonad feminization in chicken. FASEB J. 35:e21876. doi: 10.1096/fj.202100902R
Lin, M., Thorne, M. H., Martin, I. C., Sheldon, B. L., and Jones, R. C. (1995). Development of the gonads in the triploid (ZZW and ZZZ) fowl, Gallus domesticus, and comparison with normal diploid males (ZZ) and females (ZW). Reprod. Fertil. Dev. 7, 1185–1197. doi: 10.1071/rd9951185
Morris, K. R., Hirst, C. E., Major, A. T., Ezaz, T., Ford, M., Bibby, S., et al. (2018). Gonadal and Endocrine Analysis of a Gynandromorphic Chicken. Endocrinology 159, 3492–3502. doi: 10.1210/en.2018-00553
Oreal, E., Pieau, C., Mattei, M. G., Josso, N., Picard, J. Y., Carré-Eusèbe, D., et al. (1998). Early expression of AMH in chicken embryonic gonads precedes testicular SOX9 expression. Dev. Dynam. 212, 522–532. doi: 10.1002/(SICI)1097-0177(199808)212:4<522::AID-AJA5>3.0.CO;2-J
Paroush, Z., Finley, R. L. Jr., Kidd, T., Wainwright, S. M., Ingham, P. W., Brent, R., et al. (1994). Groucho is required for Drosophila neurogenesis, segmentation, and sex determination and interacts directly with hairy-related bHLH proteins. Cell 79, 805–815. doi: 10.1016/0092-8674(94)90070-1
Scheib, D. (1983). Effects and role of estrogens in avian gonadal differentiation. Differentiat. Res. Biol. Divers. 23(Suppl.), S87–S92. doi: 10.1007/978-3-642-69150-8_15
Smith, C. A., and Sinclair, A. H. (2004). Sex determination: insights from the chicken. BioEssays News Rev. Mol. Cell. Dev. Biol. 26, 120–132. doi: 10.1002/bies.10400
Tsuji, S., and Hashimoto, C. (2005). Choice of either beta-catenin or Groucho/TLE as a co-factor for Xtcf-3 determines dorsal-ventral cell fate of diencephalon during Xenopus development. Dev. Genes Evolut. 215, 275–284. doi: 10.1007/s00427-005-0474-0
Wang, Y., Jin, G., Ma, M., and Xiang, X. (2019). Sex differences in serum steroid hormone levels during embryonic development in hen eggs. Poultry Sci. 98, 6053–6062. doi: 10.3382/ps/pez270
Wen, Q., Wang, Y., Tang, J., Cheng, C. Y., and Liu, Y. X. (2016). Sertoli Cell Wt1 Regulates Peritubular Myoid Cell and Fetal Leydig Cell Differentiation during Fetal Testis Development. PLoS One 11:e0167920. doi: 10.1371/journal.pone.0167920
Wilhelm, D., Palmer, S., and Koopman, P. (2007). Sex determination and gonadal development in mammals. Physiol. Rev. 87, 1–28. doi: 10.1152/physrev.00009.2006
Wrobel, E. R., Molina, E., Khan, N. Y., Akingbemi, B. T., Mendonca, M. T., and Navara, K. J. (2020). Androgen and mineralocorticoid receptors are present on the germinal disc region in laying hens: Potential mediators of sex ratio adjustment in birds? General Comparat. Endocrinol. 287:113353. doi: 10.1016/j.ygcen.2019.113353
Yamashita, S., Kataoka, K., Yamamoto, H., Kato, T., Hara, S., Yamaguchi, K., et al. (2019). Comparative analysis demonstrates cell type-specific conservation of SOX9 targets between mouse and chicken. Sci. Rep. 9:12560. doi: 10.1038/s41598-019-48979-4
Yu, X., Yuan, Y., Qiao, L., Gong, Y., and Feng, Y. (2019). The Sertoli cell marker FOXD1 regulates testis development and function in the chicken. Reprod. Fertil. Dev. 31, 867–874. doi: 10.1071/RD18214
Zhang, M., Xu, P., Sun, X., Zhang, C., Shi, X., Li, J., et al. (2021). JUN promotes chicken female differentiation by inhibiting Smad2. Cytotechnology 73, 101–113. doi: 10.1007/s10616-020-00447-y
Zhang, Z., Elsayed, A. K., Shi, Q., Zhang, Y., Zuo, Q., Li, D., et al. (2015). Crucial genes and pathways in chicken germ stem cell differentiation. J. Biol. Chem. 290, 13605–13621. doi: 10.1074/jbc.M114.601401
Zhu, C. C., Dyer, M. A., Uchikawa, M., Kondoh, H., Lagutin, O. V., and Oliver, G. (2002). Six3-mediated auto repression and eye development requires its interaction with members of the Groucho-related family of co-repressors. Development 129, 2835–2849. doi: 10.1242/dev.129.12.2835
Keywords: chicken, sex differentiation, Tle4z1, male, gene
Citation: Chen C, Zhou S, Lian Z, Jiang J, Gao X, Hu C, Zuo Q, Zhang Y, Chen G, Jin K and Li B (2022) Tle4z1 Facilitate the Male Sexual Differentiation of Chicken Embryos. Front. Physiol. 13:856980. doi: 10.3389/fphys.2022.856980
Received: 18 January 2022; Accepted: 28 February 2022;
Published: 07 April 2022.
Edited by:
Xiquan Zhang, South China Agricultural University, ChinaReviewed by:
Silvana Guioli, Francis Crick Institute, United KingdomPatricia Castillo-Briceno, Universidad Laica Eloy Alfaro de Manabi, Ecuador
Copyright © 2022 Chen, Zhou, Lian, Jiang, Gao, Hu, Zuo, Zhang, Chen, Jin and Li. This is an open-access article distributed under the terms of the Creative Commons Attribution License (CC BY). The use, distribution or reproduction in other forums is permitted, provided the original author(s) and the copyright owner(s) are credited and that the original publication in this journal is cited, in accordance with accepted academic practice. No use, distribution or reproduction is permitted which does not comply with these terms.
*Correspondence: Kai Jin, amluLmthaUB5enUuZWR1LmNu; Bichun Li, eXViY2xpQHl6dS5lZHUuY24=