- 1Department of Anesthesiology, The Third Affiliated Hospital, Southern Medical University, Guangzhou, China
- 2Department of Anesthesiology, The First People’s Hospital of Kashgar, Xinjiang, China
- 3Department of Anesthesiology, The Second People’s Hospital of Panyu, Guangzhou, China
- 4Department of Anesthesiology, Guangzhou Eighth People’s Hospital, Guangzhou Medical University, Guangzhou, China
Sepsis is a systemic inflammatory response caused by a severe infection that leads to multiple organ damage, including acute kidney injury (AKI). In intensive care units (ICU), the morbidity and mortality associated with sepsis-associated AKI (SA-AKI) are gradually increasing due to lack of effective and early detection, as well as proper treatment. Non-coding RNAs (ncRNAs) exert a regulatory function in gene transcription, RNA processing, post-transcriptional translation, and epigenetic regulation of gene expression. Evidence indicated that miRNAs are involved in inflammation and programmed cell death during the development of sepsis-associated AKI (SA-AKI). Moreover, lncRNAs and circRNAs appear to be an essential regulatory mechanism in SA-AKI. In this review, we summarized the molecular mechanism of ncRNAs in SA-AKI and discussed their potential in clinical diagnosis and treatment.
Introduction
Sepsis is a common cause of acute conditions and death in patients with community-acquired and nosocomial infections (Wacker et al., 2013). The Third International Consensus (Sepsis-3) Definitions published in 2016 defines sepsis as life-threatening organ dysfunction caused by a dysregulated host response to infection (Singer et al., 2016). This condition can lead to various organ damage, such as cardiac insufficiency, acute respiratory distress syndrome, diffuse intravascular coagulation, and acute kidney injury (AKI).
AKI is a clinical syndrome caused by the rapid decline of renal function, associated with the risk of developing chronic kidney disease (CKD) and end-stage kidney disease (ESKD) (Bellomo et al., 2012). The incidence of death in AKI patients has been reported to be increased 5.5-fold compared with non-AKI patients (Chertow et al., 2005). The main causes of AKI are sepsis, obstruction, drugs, radiocontrast, and surgery; yet, sepsis is considered as one of the most common causes (Chertow et al., 2005; Uchino, 2005). In the intensive care unit (ICU), sepsis-associated AKI (SA-AKI) is diagnosed in up to 47% of cases and is a dominant public health concern associated with increased mortality and increased progression to CKD (Keir and Kellum, 2015). Effective and early detection, as well as proper treatment for patients with SA-AKI, are still missing. In addition, the molecular mechanisms of SA-AKI have not yet been fully explored.
The development of SA-AKI is a complex pathological process. Different from other types of AKI, renal blood perfusion in SA-AKI is not reduced, and even increased. And the culprit of SA-AKI is excessive inflammatory response (Kellum and Prowle, 2018). Over recent years, many studies have reported on the possible mechanism of SA-AKI, including the regulatory role of ncRNAs in the molecular mechanism of SA-AKI (Langenberg et al., 2014; Qin et al., 2020). The ncRNA family is transcribed from the genome without or with a small amount of protein-encoding potential that has multiple functions. ncRNAs can be divided into two main types: basic primary structure and regulatory ncRNAs. Basic structure ncRNAs exert a role similar to housekeeping genes in translation and splicing, including ribosomal RNA (rRNA), transfer RNA (tRNA), small nuclear RNA (snRNA), and so on. Regulatory ncRNAs mainly participate in the modification of other RNAs, including microRNA (miRNA), long non-coding RNA (lncRNA) and circular RNA (circRNA), piwi-interacting RNA (piRNA), small interfering RNA (siRNA), and so on. ncRNAs are involved in gene transcription, RNA processing, and post-transcriptional translation but also in the epigenetic regulation of gene expression (Cech and Steitz, 2014). Existing studies have demonstrated that ncRNAs might regulate gene expression by inhibiting target gene translation and inducing the degradation of target gene mRNA to regulate inflammation, programmed cell death, and oxidative stress in SA-AKI (Liu et al., 2020a; Luo et al., 2020a; Shi et al., 2020a). In the present review, we summarized the alteration, function, predictive value, and therapeutic potential of ncRNA (mainly miRNA, lncRNA, circRNA) in SA-AKI.
The Role of ncRNA in SA-AKI
ncRNAs exert a regulatory role in many diseases, including sepsis (Yong, 2020) and AKI (Yu et al., 2016). Numerous studies have indicated that ncRNAs regulate inflammation, programmed cell death, and oxidative stress in SA-AKI by regulating the expression of target genes or regulating various signaling pathways (Figure 1) (Chen et al., 2018a; Wang et al., 2020a). E.g., miRNAs can aggravate or alleviate SA-AKI by regulating the expression of forkhead box O3 (FOXO3), neuropilin 1 (NRP1) and thrombospondin 2 (THBS2) (Shen et al., 2019a; Luo et al., 2020a; Wang et al., 2020b). miR-155 can activate Janus activated kinase (JAK)-signal transducer and activator of transcription (STAT) signaling pathway via negatively regulating suppressor of cytokine signaling 1 (SOCS1) (Ren et al., 2017).
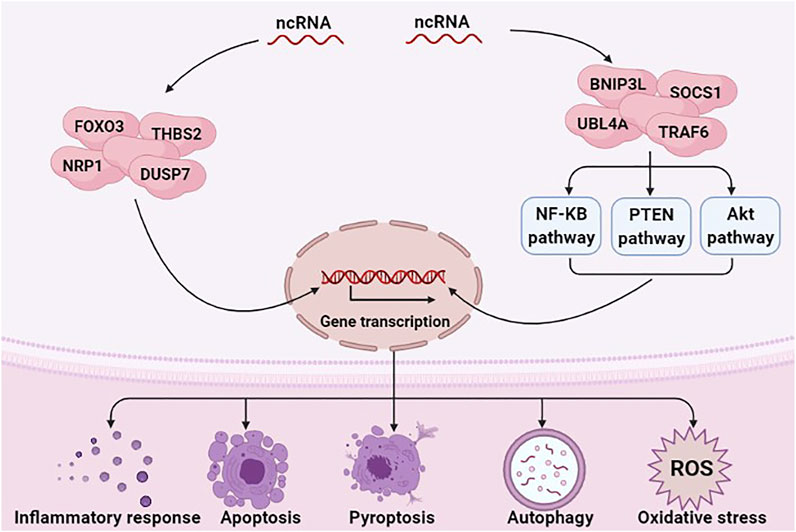
FIGURE 1. ncRNAs are involved in the pathological mechanism of SA-AKI. ncRNAs, including miRNAs, lncRNAs, and circRNAs, have a critical role in regulating inflammation response, programmed cell death, and oxidative stress in SA-AKI by regulating the expression of target genes or regulating various signaling pathways.
miR-21 is the most frequently studied miRNA in SA-AKI. It is ubiquitously expressed in many organs like the heart and kidney in mammals (Chuppa et al., 2018; Song et al., 2018). Previous studies have suggested that miR-21 is a critical regulator of inflammation, energy metabolism, and cancer biology (Gomez et al., 2015; Zheng et al., 2017). Moreover, miR-21 is believed to be a regulatory molecule of SA-AKI involved in the pathological process of kidney fibrosis and mediated podocyte apoptosis (Song et al., 2018; Chen et al., 2018b; Wei et al., 2020). Emerging evidence indicated that the expression of miR-21 is upregulated in patients with SA-AKI (Wei et al., 2020). miR-21 stably exists in blood and urine by binding to argonaute2 (ago2), an essential binding protein of miRNAs. Neuropilin-1 (Nrp-1), a receptor that can bind various ligands via its extracellular part that consists of several domains, can function as a cell “cargo” by transporting extracellular molecules, such as miR-21 into cells. miR-21a-3p is internalized via Nrp-1 and ago2 that are important mechanisms for the accumulation of miR-21a-3p in tubular epithelial cells (TECs) during SA-AKI (Figure 2) (Zou et al., 2020). It has been reported that overexpression of miR-21 aggravates renal dysfunction and promotes cell apoptosis during lipopolysaccharide (LPS)-stimulated AKI (Lin et al., 2019a; Wei et al., 2020). Lin et al found that miR-21 has a critical role in apoptosis and is involved in cellular metabolic processes such as lipid metabolism and cell cycle arrest via AKT/CDK2-FOXO1 pathway in SA-AKI (Lin et al., 2019a). However, some studies have reached different conclusions. Few studies revealed that miR-21 exerts an anti-apoptosis effect, while its overexpression can inhibit pro-apoptotic signaling pathways phosphatase and tension homolog (PTEN)/Akt and PDCD4/nuclear factor-κB (NF-κB) in the kidney, thereby alleviating kidney injury caused by sepsis (Jia et al., 2015; Fu et al., 2017; Yang et al., 2018; Pan et al., 2019). It has also been demonstrated that xenon and remote ischemic preconditioning can protect against SA-AKI by upregulating the expression of miR-21 (Jia et al., 2015; Pan et al., 2019). The reasons for these discrepant findings are unclear, and may be related to the usage of different models. Thus, more evidence is needed to clarify the role of miR-21 in SA-AKI.
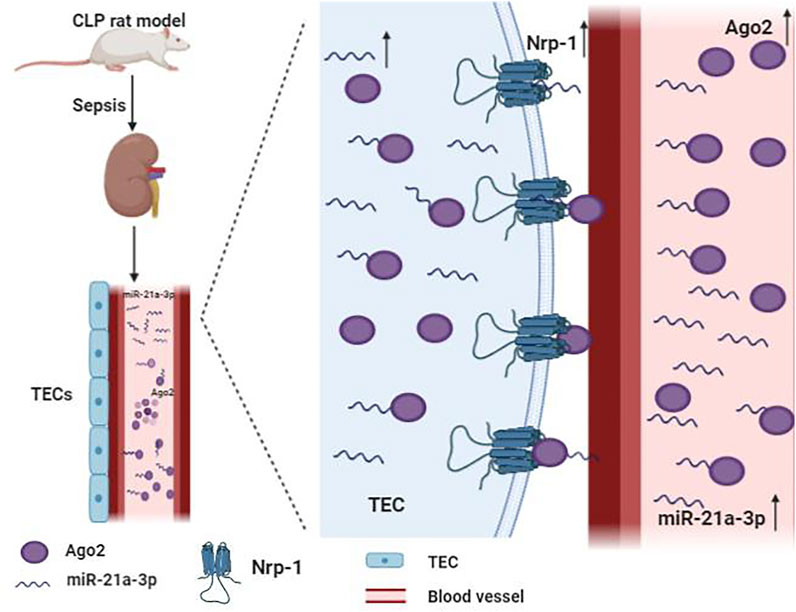
FIGURE 2. Mechanism of the accumulation of miR-21a-3p in tubular epithelial cells (TECs) during sepsis. miR-21a-3p stably exists in blood and urine by binding to argonaute2 (ago2). Neurocorin-1 (Nrp-1) acts as cell “cargo” transporting extracellular molecules, such as miR-21a-3p and miR-21a-3p-ago2, into cells.
miR-22 is a frequently analyzed miRNA in the context of SA-AKI. miR-22 has been confirmed to have a critical role in many kidney diseases (Fan et al., 2016; Zhang et al., 2018b). Studies have found that miR-22 is down-regulated in patients with SA-AKI (Ge et al., 2017); the same results were obtained in the animal models constructed by cecal ligation and puncture (CLP) and LPS-treated cells (Wang et al., 2020a). Furthermore, the overexpression of miR-22 can reduce the expression of inflammatory factors (IL-1β, IL-6, and TNF-α) and NO, as well as significantly reduce cell apoptosis via PTEN/high-mobility group Box 1 (HMGB1) and TLR4/NF-κB pathway, thereby alleviating the AKI caused by sepsis (Shen et al., 2018; Shen et al., 2019b; Wang et al., 2020a). Besides, miR-22 negatively regulates the expression of apoptosis-inducing factor mitochondrion-associated 1 (AIFM1), and AIFM1 knockdown significantly ameliorates LPS-induced renal cell apoptosis in vitro (Zhang et al., 2020a). These findings imply that upregulation of miR-22 acts as a defense mechanism to decrease inflammatory response and apoptosis. To sum up, miR-22 may be a potential target to treat SA-AKI.
The expression of lncRNA NEAT1 has been confirmed to be significantly higher in ischemia-induced AKI patients compared to the healthy control group (Jiang et al., 2019a). Meanwhile, the expression levels of NEAT1 were also obviously higher in the patients with sepsis than in healthy controls. Furthermore, NEAT1 has been shown to be positively correlated with the severity of AKI, the increased disease risk, and the unfavorable prognosis in sepsis patients (Chen et al., 2018a; Huang et al., 2018). Besides, the expression of NEAT1 was also upregulated in SA-AKI model rats constructed by CLP and LPS-stimulated cells (Wang et al., 2020c). These results suggest that NEAT1 could be used as a useful additive biomarker for the diagnosis of sepsis. Functional studies revealed that suppression of NEAT1 alleviates the inflammatory response and cell apoptosis in LPS-treated rat mesangial cells (RMCs) by targeting miR-204 and modulating the NF-κB pathway (Chen et al., 2018a). Another study suggested that the expression of NEAT1 could improve renal function and ameliorate LPS-induced inflammatory responses and apoptosis via miR-27a-3p/TAB3 axis (Wang et al., 2020c). In LPS-treated mouse RAW264.7 macrophages, knockdown of NEAT1 alleviated LPS-induced inflammation by promoting macrophage M2 polarization via miR-125a-5p/TRAF6/TAK1 axis (Wang and Guo, 2020). These studies indicated that NEAT1 might be a target for the treatment of patients with SA-AKI.
circRNA is also considered to be implicated in SA-AKI. circ_00114,428 were upregulated in SA-AKI serum specimens and LPS-induced HK2 cells, and circ_00114,428 knockdown attenuated SA-AKI by regulating cereblon (CRBN) expression via targeting miR-495-3p (He et al., 2021). circTLK1 contributed to SA-AKI by regulating inflammation and oxidative stress through the miR-106a-5p/HMGB1 axis. circTLK1 and miR-106a-5p may be employed as the potential targets for the treatment of SA-AKI (Xu et al., 2021). circ_0091702 was downregulated in SA-AKI patients and LPS-induced HK2 cells. It could attenuate SA-AKI via the miR-545-3p/THBS2 axis, indicating that circ_0091702 might be an important factor for relieving SA-AKI (Tan and Bei, 2021). Evidence indicated that ncRNAs remarkably change in SA-AKI, while upregulation or downregulation of the expression of ncRNAs could change the prognosis of SA-AKI.
ncRNAs Mediates Signaling Pathway in SA-AKI
The exact molecular mechanisms of SA-AKI are still unclear. However, existing studies have suggested that multiple signaling pathways contribute to the pathogenesis of SA-AKI, such as NF-κB, PTEN and Akt (Wang et al., 2020a; Zhong et al., 2020). Understanding the signaling pathways through which sepsis triggers AKI may promote new therapeutic approaches to prevent or reverse AKI.
NF-κB Signaling Pathway
NF-κB is a vital transcription factor in the cytoplasm composed of the dimeric p50/p65 and another inhibitory subunit IκB. When IκB is catalyzed by IκB kinase, it undergoes phosphorylation and degradation, causing NF-κB to activate rapidly. The activated NF-κB then enters the nucleus and binds to numerous gene promoters, regulating various pathological processes (Qin et al., 2020). NF-κB signaling pathway is an important signaling pathway in the process of inflammatory response and immune response, which can regulate apoptosis and stress response (Luo et al., 2020b). Growing evidence has indicated that ncRNAs are involved in the pathological process of SA-AKI by regulating the NF-κB signaling pathway (Figure 3). For example, miR-34b-3p can decrease the transcriptional activity of NF-κB by inhibiting ubiquitin-like protein 4A (UBL4A). The expression of miR-34b-3p is suppressed in the CLP mice model and the LPS-induced RMCs. Moreover, overexpression of miR-34b-3p could alleviate kidney tissue injury through downregulation of UBL4A/NF-κB (He et al., 2020a).
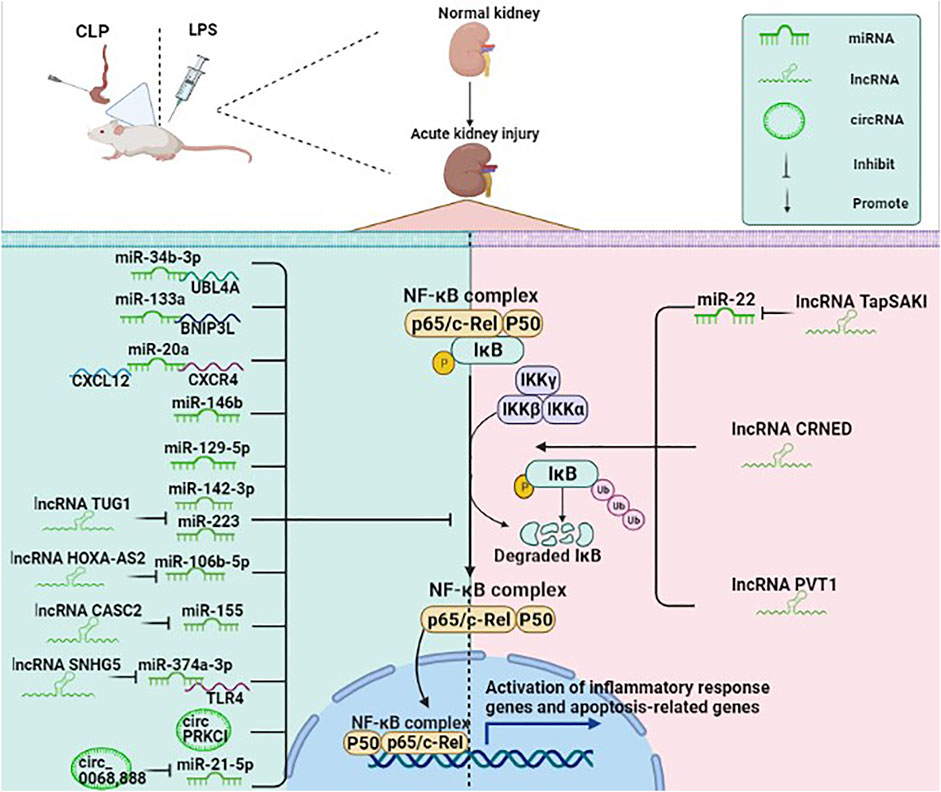
FIGURE 3. ncRNAs (mainly miRNAs, lncRNAs, circRNAs) participate in the NF-κB pathway in SA-AKI. NF-κB is a vital transcription factor in the cytoplasm in an inactive state, composed of the dimeric p50/p65 and another inhibitory subunit IκB. When IκB is catalyzed by IκB kinase, it undergoes phosphorylation and degradation, causing NF-κB to rapidly activate, while the activated NF-κB enters the nucleus and binds to numerous gene promoters, regulating various pathological processes. ncRNAs are involved in the pathological process of SA-AKI by regulating the NF-κB signaling pathway.
BCL2/adenovirus E1B interacting protein 3-like (BNIP3L) belongs to the Bcl-2 family and is a BH3-only pro-apoptotic factor. miR-133a can directly target the BNIP3L and then inhibit the NF-κB pathway, thereby inhibiting inflammation and apoptosis and ameliorating the renal function (Qin et al., 2020). Besides, previous studies have shown that the chemokine C-X-C motif ligand 12 (CXCL12)/C-X-C chemokine receptor type 4 (CXCR-4) axis could activate the NF-κB signaling pathway. miR-20a may exert anti-inflammatory and anti-apoptotic effects in LPS-stimulated HK-2 cells by inhibiting CXCL12/CXCR-4 and NF-κB signaling pathways (Zhang et al., 2020b). Human umbilical cord mesenchymal stem cell-derived exosomes (HucMSC-Ex) can decrease interleukin (IL)-1 receptor-associated kinase (IRAK1) level through the upregulation of miR-146b level, which suppress NF-κB activity, eventually alleviating SA-AKI and improving survival in mice with sepsis. HK-2 cells transfected with miR-146b inhibitor can significantly increase the translocation of NF-κB p-P65 to the nucleus, while HK-2 cells transfected with miR-146b mimic could lead to an opposite result, thus suggesting that miR-146b improves renal function by inhibiting NF-κB signaling pathway (Zhang et al., 2020c). miR-129-5p has been shown to reduce LPS-induced SA-AKI models in vitro and in vivo and exert a protective effect. miR-129-5p reduced the expression of nuclear NF-κB protein, thereby reducing podocyte apoptosis and inflammation (Huang et al., 2020a).
lncRNAs are involved in the NF-κB signaling pathway by regulating the miRNAs. A previous study suggested that lncRNA TrAnscript Predicting Survival in AKI (TapSAKI) was elevated in urine-derived sepsis (US)-induced kidney injury. It increased the expression of TLR4, p-p65, and caspase-3 by negatively regulating miR-22, promoting apoptosis and inflammatory response through the TLR4/NF-κB pathway (Shen et al., 2019b). Moreover, the expression of lncRNA taurine upregulated gene 1 (TUG1) is downregulated in patients with SA-AKI. The upregulation of TUG1 inactivates NF-κB signaling to mediate LPS-related cell damage by negatively regulating the miR-142-3p or miR-223 (Liu, 2019). A previous study suggested that lncRNA HOXA cluster antisense RNA 2 (HOXA-AS2) is downregulated in sepsis patients, CLP mouse models, and LPS-stimulated HK-2 cells while its overexpression alleviates SA-AKI by targeting miR-106b-5p and restrains the Wnt/β-catenin and NF-κB pathways (Wu et al., 2020). Another study has shown that the expression of lncRNA cancer susceptibility candidate 2 (CASC2) was significantly decreased in sepsis patients compared with healthy subjects, and the level of the CASC2 was negatively correlated with the severity of kidney damage. CASC2 inhibited inflammation, apoptosis, and oxidative stress by negatively regulating miR-155 and NF-κB pathways, thus suggesting that CASC2 could serve as a potential target for treating SA-AKI (Wang et al., 2020d). Evidence suggested that lncRNA small nuclear RNA host gene 5 (SNHG5) can combine with miR-374a-3p in SA-AKI, which inhibits NF-κB activity by targeting TLR4 (Wang et al., 2021a).
lncRNAs could also directly regulate the NF-κB pathway without regulating the expression of miRNA. lncRNA colorectal neoplasia differentially expressed gene (CRNDE) was significantly upregulated in mouse models constructed by LPS. Downregulation of CRNDE could effectively inhibit the activation of the TLR3/NF-κB pathway to alleviate SA-AKI (Sun et al., 2019). Huang et al found that increased expression of lncRNA plasmacytoma variant translocation 1 (PVT1) in LPS-induced inflammation injury in HK-2 cells promoted inflammatory factors and proteins p-IκBα and p-p65, while its inhibition reversed this process. This study indicated that PVT1 might regulate the NF-κB pathway, having a pivotal role in inflammation (Huang et al., 2017).
The studies on the role of circRNA in the NF-κB signaling pathway in SA-AKI are limited. circPRKCI has been shown to be significantly decreased in patients with urosepsis and LPS-treated HK2 cells. Moreover, the overexpression of circPRKCI could reduce the phosphorylation level of IκBα, and the expression of p50 and p65 in the nucleus, thus suppressing the NF-κB pathways and reducing inflammatory response (Shi et al., 2020a). Decreased hsa_circ_0068,888 expression was observed in LPS-stimulated HK-2 cells, while hsa_circ_0068,888 overexpression suppressed the activation of NF-κB pathway by negatively regulating miR-21-5p as shown by decreased p-p65 protein level and nuclear translocation of p65 (Wei et al., 2021).
All these studies demonstrated that ncRNAs participate in the activation of the NF-κB signaling pathway. Yet, the specific mechanism remains unclear.
Phosphatase and Tensin Homolog
PTEN, a lipid and protein phosphatase with widespread action, is a tumor suppressor gene that participates in the pathophysiological process of multiple organ injury (Ning, 2004; Lan et al., 2012; Bhatt et al., 2015). Recent evidence has shown that PTEN expression is increased in patients with sepsis and animal models of sepsis (Sisti et al., 2018).
PTEN can adjust the abundance of miRNAs by influencing enzymes involved in miRNAs maturation. For example, active PTEN can increase the expression of miR-125b and miR-21 and decrease the expression of miR-155 in the human embryonic kidney (HEK) 293 cells by regulating the nuclear localization of Drosha-Dgcr8 (part of the miRNA-processing complex) (Sisti et al., 2018). Conversely, miRNA can also regulate the expression of PTEN in SA-AKI, thereby mediating the pathological process of SA-AKI. Pan et al found an increased expression of PTEN in the CLP mouse models and LPS-treatment cells. Contrary, the upregulation of miR-21 induced by limb remote ischemic preconditioning (rIPC) reversed this process, exerting anti-apoptotic and anti-inflammatory effects and alleviating SA-AKI (Pan et al., 2019). Moreover, it has also been found that a protective role of miR-22-3p in SA-AKI may depend on the repression of PTEN (Wang et al., 2020a). Besides, a miR-205 agonist could improve the pathological morphology in the SA-AKI models by inhibiting the HMGB1-PTEN signaling pathway (Zhang et al., 2019a).
lncRNAs participate in the development of SA-AKI by regulating the PTEN signaling pathway. It has been found that lncRNA TCONS_00016406 (termed lncRNA 6406) was significantly downregulated in the SA-AKI models, and lncRNA 6406 could attenuate SA-AKI through modulating the miR-687/PTEN signaling pathway (Liu et al., 2020a). lncRNA TapSAKI positively regulates PTEN, and the knockdown of TapSAKI improves renal function (Shen et al., 2019b).
In the sepsis model, ncRNAs could improve AKI by inhibiting the expression of PTEN. In summary, PTEN may be a key factor in the pathological process of SA-AKI and may become a therapeutic target in the future.
Akt Signaling Pathway
Protein kinase B, also known as Akt, has been shown to be the encoding product of the retrovirus safety gene V-Akt, which plays an important role in cell survival and apoptosis. Growth and survival factors such as insulin can activate the Akt signaling pathway. The mammalian target of rapamycin (mTOR) is a downstream target of phosphatidylinositol 3 kinase (PI3K) and Akt pathways. Akt activates mTOR complex 1 (mTORC1) to inhibit autophagy. The Akt signaling pathway is involved in the course of SA-AKI (Zhao et al., 2020a).
Study have shown that during SA-AKI, miR-21-3p upregulates FOXO1 by directly targeting Akt and CDK2, and induces TECs cell cycle G1 arrest and apoptosis (Lin et al., 2019a). In CLP mice, miR-214 activates the Akt/mTOR pathway to inhibit autophagy in kidney tissues, suggested that miR-214 served a protective role against SA-AKI (Sang et al., 2021). In the SA-AKI model, the expression of miR-93 is significantly down-regulated, and miR-93 can reduce apoptosis and inflammatory response by activating Akt/mTOR signaling pathway, thus mitigate SA-AKI (Zhan et al., 2021). It is known from the above that ncRNA can reduce apoptosis or autophagy by activating the Akt signaling pathway, thus improving SA-AKI, which may be a new idea for the treatment of SA-AKI.
Role of ncRNAs in Cellular Mechanisms in SA-AKI
Accumulating evidence suggests that ncRNAs are involved in cellular mechanisms of SA-AKI, such as excessive inflammatory response, apoptosis, autophagy, and oxidative stress (Lee et al., 2012; Rousta et al., 2018; Zhang et al., 2019b).
Inflammatory Response
The inflammatory response is one of the crucial pathogeneses of SA-AKI. In sepsis, circulating toxins may act on endothelial cells, causing an overactivated state of the immune system. Endothelial cells, epithelial cells, neutrophils, macrophages, and lymphocytes produce large amounts of pro-inflammatory cytokines (such as TNF-α, IL-6, IL-1β, and IL-8), then intracellular pathways are activated, and more pro-inflammatory cytokines and chemokines are released (Verma and Molitoris, 2015). Excessive release of pro-inflammatory factors causes the damage of renal TECs, which in turn leads to AKI (Huang et al., 2015). The previous study has shown that the inhibition of inflammatory cytokine production could ameliorate the severity of LPS-induced kidney injury (Rousta et al., 2018). Moreover, relevant evidence showed that ncRNAs have an essential role in the inflammatory response of SA-AKI by regulating the production of inflammatory cytokines (Liu et al., 2020a).
Existing studies indicated that ncRNAs exert an anti-inflammatory effect in SA-AKI. For instance, when treating HK-2 cells with LPS, the expression of miR-20a and miR-942-5p was downregulated, and inflammatory cytokines were upregulated (Luo et al., 2020a; Zhang et al., 2020b). In septic rat models, increased expression of miR-22-3p and miR-191-5p could reduce the inflammatory response (Qin et al., 2019; Wang et al., 2020a). Some studies have shown that some miRNAs, lncRNAs, and circRNAs can suppress inflammation response by inhibiting the transcriptional activity of NF-κB, thereby alleviating SA-AKI (Liu, 2019; Ma et al., 2019; He et al., 2020a; Lu et al., 2020a; Shi et al., 2020a; Wang et al., 2020d; Qin et al., 2020). It has also been reported that propofol can upregulate the expression of miR-290-5p, thereby reducing the expression of inflammatory cytokines to attenuate SA-AKI (Zheng et al., 2018). lncRNA HOX transcript antisense RNA (HOTAIR) overexpression in sepsis rats could reduce the IL-1β and TNF-α to improve renal function (Jiang et al., 2019b). A novel lncRNA TCONS_00016406 significantly reduced inflammation by targeting the miR-687/PTEN axis to reduce the inflammatory cytokines (including IL-1β, TNF-α, and IL-18) in SA-AKI (Liu et al., 2020a). The differentiation level antagonizing nonprotein coding RNA (DANCR) was markedly decreased in AKI patients and LPS-treated HK-2 cells, and overexpression of DANCR could suppress inflammatory response and cell apoptosis by regulating miR-214 (Zhao et al., 2020b). Also, previous studies have shown that circ-Ttc3 and circVMA21 reduce inflammation caused by SA-AKI in CLP rat models (Shi et al., 2020b; Ma et al., 2021).
ncRNAs can aggravate kidney damage by increasing the inflammatory response. miR-152-3p is upregulated in patients with SA-AKI and positively correlated with serum creatinine (SCr), blood urea nitrogen (BUN), IL-1β, and TNF-α. Downregulation of miR-152-3p could alleviate inflammatory response (Ma et al., 2020). miR-106a is also upregulated in the serum of sepsis patients. The same results were observed in CLP mouse models and LPS-treated TCMK-1 cells (Shen et al., 2019a). Besides, miR-106a was negatively regulated by lncRNA HOXA cluster antisense RNA 2 (HOXA-AS2), while its suppression could decrease LPS-induced inflammation factor level in vitro (Shen et al., 2019a; Wu et al., 2020). The expression of miR-128-3p and miR-155 was also evidently upregulated in renal tissues in SA-AKI mouse models while their inhibition alleviated renal injury, markedly decreasing the inflammatory cytokines (Ren et al., 2017; Wang et al., 2020b). miR-107 was dramatically increased in the circulating endothelial cells (CECs) of septic AKI patients compared with non-septic AKI and septic non-AKI patients, and miR-107 increased TNF-α secretion by targeting dual-specificity phosphatase 7 (DUSP7) in endothelial cells (Wang et al., 2017). Moreover, a previous study suggested that lncRNA TapSAKI promotes the inflammatory response by negatively regulating miR-22 (Shen et al., 2019b). lncRNA CRNDE was upregulated in patients with sepsis and SA-AKI models, and CRNDE overexpression markedly boosted inflammatory cytokine levels, including TNF-α, IL-6, IL-8, and IL-1β. At the same time, interfering with the expression of CRNDE could reverse the result (Wang et al., 2020e; Wu, 2020). lncRNA PVT1 has been shown to be upregulated in LPS-induced SA-AKI models, and PVT1 could mediate the release of pro-inflammatory cytokines (Huang et al., 2017). circ-FANCA (hsa_circ_0040994) is highly expressed in SA-AKI, and circ-FANCA silence alleviated LPS-induced HK2 cell injury, including proliferation inhibition and inflammatory response (Li et al., 2021a).
The inflammatory response is a critical pathological process of SA-AKI. ncRNAs have a regulatory role in this process by increasing the inflammatory response.
Programmed Cell Death
Research suggested that SA-AKI is closely related to programmed cell death, a suicide protection measure initiated by gene regulation when cells are stimulated by internal and external environmental factors, including apoptosis, pyroptosis, and autophagy (Xue et al., 2019; Wang et al., 2020f; Ma et al., 2020).
Apoptosis
Apoptosis is a form of programmed cell death that depends on the activity of cysteine and cathepsin and can be triggered by exposure to stress stimuli as well as mitochondrial products. At present, it is thought that there is no significant apoptosis in the kidney during the progression of sepsis (Cao et al., 2019). However, the view that cell death caused by apoptosis during sepsis directly leads to microvascular dysfunction and organ failure, is still accepted by most people (Huang et al., 2020b). Some ncRNAs participate in the pathological process of SA-AKI via mediating cell apoptosis.
miRNAs have been confirmed to be involved in cell apoptosis. For example, miR-152-3p and miR-128-3p promote cell apoptosis to exacerbate renal dysfunction in sepsis (Wang et al., 2020b; Ma et al., 2020). miR-590-3p, miR-20a, miR-133a, and miR-942-5p exert an anti-apoptotic effect, while their overexpression can dramatically inhibit the expression of Bax (pro-apoptotic regulator) and increase the expression of Bcl-2 (anti-apoptotic regulator) in SA-AKI models (Ma et al., 2019; Luo et al., 2020a; Zhang et al., 2020b; Qin et al., 2020). miR-205 agonist has been shown to decrease apoptosis rate in the rat models of sepsis-induced renal injury, which suggested that miR-205 agonist could suppress cell apoptosis and alleviate renal injury (Zhang et al., 2019a).
lncRNA exerts anti-apoptotic or pro-apoptotic effects by regulating miRNA and signaling pathways. lncRNA TUG1 and lncRNA 6406 have an anti-apoptosis effect. They markedly mitigate LPS-induced cell damage by reducing cell apoptosis in SA-AKI, indicating that overexpressing TUG1 and 6406 may be an effective therapeutic strategy of SA-AKI (Liu et al., 2020a; Liu, 2019). lncRNA is also involved in the pro-apoptotic process of SA-AKI. It has been confirmed that interfering with the expression of lncRNA NEAT1 and lncRNA PVT1 could reduce apoptosis to facilitate the kidney cell injury caused by sepsis (Wang et al., 2020c; Huang et al., 2017). Moreover, lncRNA myocardial infarction-associated transcript (MIAT) may bind to miR-29a to promote cell apoptosis in LPS-stimulated cells (Zhang et al., 2019c). Through the miR-22/PTEN/TLR4/NF-κB pathway, lncRNA TapSAKI promotes cell apoptosis, thereby aggravating SA-AKI (Shen et al., 2019b). lncRNA ENST00000452391.1, also known as “sepsis-induced kidney injury associated transcript 1 (SIKIAT1)", acts as an apoptosis factor in SA-AKI and is upregulated in the peripheral blood samples of patients with SA-AKI. It could sponge miR-96-3p to facilitate Forkhead Box 1 (FOXA1) expression to promote cell apoptosis in LPS-stimulated HK-2 cells (Lu et al., 2020b).
lncRNA HOTAIR was upregulated in kidney tissues with sepsis and LPS-treated HK-2 cells. It was involved in cell apoptosis in SA-AKI. There are different conclusions regarding the role of HOTAIR in cell apoptosis. Shen et al suggested that HOTAIR promotes cell apoptosis by modulating miR-22/HMGB1 (Shen et al., 2018). Moreover, Jiang et al found that HOTAIR could inhibit the apoptosis of kidney cells in septic rats with AKI via inhibiting the miR-34a and increasing the Bcl-2 level. They also found that lncRNA HOTAIR overexpression can alleviate renal function (Jiang et al., 2019b). Yet, the exact role of HOTAIR in cell apoptosis in SA-AKI needs to be further confirmed.
The role of lncRNA CRNDE in regulating apoptosis in SA-AKI models is still controversial. Wang and others found that the down-regulation of lncRNA CRNDE promotes cell apoptosis and aggravates kidney injury by negatively regulating miR-181a-5p in sepsis (Wang et al., 2020g). In contrast, Wu et al suggested that the inhibition of lncRNA CRNDE could reduce cell apoptosis by inhibiting the NF-κB signaling pathway in SA-AKI (Sun et al., 2019; Wu, 2020).
Few studies in cell apoptosis in SA-AKI have focused on the circRNAs. circVMA21 has an anti-apoptotic role in SA-AKI, and overexpression of circVMA21 could inhibit apoptosis from ameliorating SA-AKI (Shi et al., 2020b). The expression of circRNA mitochondrial translation optimization one homolog (circMTO1) was significantly decreased in SA-AKI rat models, and LPS-stimulated RMCs and circMTO1 overexpression promoted cell viability, inhibited cell apoptosis. They also alleviated AKI by regulating miR-337 (Shi et al., 2020c). It has been reported that overexpression of circPRKCI increases cell viability and decreases cell apoptosis in LPS-treated HK-2 cells (Shi et al., 2020a). Knockdown of circ-FANCA could inhibit cell apoptosis and alleviate LPS-induced HK2 cell injury (Li et al., 2021a). The role of circRNAs in apoptosis and their specific mechanism in SA-AKI is still unclear. Additional studies are warranted to give a more profound insight into the role of circRNAs in apoptosis in SA-AKI.
Pyroptosis
Pyroptosis, also known as inflammatory cell necrosis, is manifested by the continuous expansion of cells until the cell membrane ruptures, which leads to the release of cell contents and activates a robust inflammatory response. Caspase-11, GSDMD, and inflammasomes can modulate pyrolysis and induce cell death under inflammatory and stress pathological conditions (Shi et al., 2017). It is key to study pyroptosis by detecting the expression of NLRP3, GSDMD and its related family members, as well as the cleavage of inflammatory caspases. Pyroptosis is a new type of programmed cell death that has been discovered and confirmed in the past decade, and its molecular mechanism of regulating the infectious diseases is a Frontier focus. However, how excessive pyroptotic signaling caused by infection triggers AKI remains to be understood. Evidence suggested that pyroptosis contributes to SA-AKI pathophysiology (Ye et al., 2019).
Although pyroptosis is a research hotspot related to sepsis, there is little evidence directly related to SA-AKI. For instance, lncRNA growth arrest specific transcript 5 (GAS5) has protective effects against SA-AKI via downregulating miR-579-3p to inhibit cell pyroptosis (Ling et al., 2021). lncRNA maternally expressed gene 3 (MEG3) promoted renal tubular epithelial pyroptosis by regulating the miR-18a-3p/GSDMD pathway in SA-AKI (Deng et al., 2021a). lncRNA PVT1 modulated nucleotide-binding oligomerization domain-like receptor protein 3 (NLRP3)-mediated pyroptosis in SA-AKI by targeting miR-20a-5p (Deng et al., 2021b). miR-21 is a critical positive regulator of the NF-κB pathway and NLRP3 inflammasomes in pyroptosis and septic shock (Xue et al., 2019). miR-30c-5p is down-regulated in patients with SA-AKI, septic mice, and HK-2 cells. miR-30c-5p inhibits the expression of pyroptosis-related marker caspase-11 and GSDMD by negatively regulating thioredoxin-interacting protein (TXNIP) (Li, 2020). Exosomal miR-93-5p also could regulate the TXNIP directly to influence the pyroptosis in renal epithelial cell (Juan et al., 2021). Studies suggested that miR-223-3p regulates endothelial cell pyroptosis by targeting NLRP3, and that miR-223-3p overexpression can partially reverse the cytotoxicity pyroptosis in HK-2 cells upon LPS stimulation by targeteing 3′UTR of NLRP3 and repressing its expression (Tan et al., 2020). Taken together, although the potential role of ncRNAs in pyroptosis has not been fully understood, emerging evidence shows the importance of ncRNAs in pyroptosis.
Autophagy
Autophagy is a process of engulfing one’s cytoplasmic proteins or organelles and coating them into vesicles, fusing with lysosomes to form autophagic lysosomes, and degrading the contents of the lysosomes, thereby fulfilling the metabolic needs of the cell itself and the renewal of specific organelles (Parzych and Klionsky, 2014). Studies have shown that ROS can trigger autophagy in mammalian cells and tissues in sepsis and AKI, and play a protective role by clearing pathogens, neutralizing microbial toxins, regulating cytokine release, reducing apoptosis oncotarget, and promoting antigen expression (Kaushal and Shah, 2016; Huang et al., 2019). However, related studies hold the opposite view that inhibition of autophagy can improve SA-AKI (Wang et al., 2020f), which may be related to the inhibition of autophagy can alleviate cell death when excessive autophagy. Currently, the role of autophagy in SA-AKI remains unclear and needs further study.
Autophagy is the pathological process of SA-AKI (Zhang et al., 2019b; Choi, 2020). ncRNAs participate in the pathological process of SA-AKI by regulating autophagy. It has been reported that miR-20a may promote AKI in septic rats by activating autophagy (Wang et al., 2020f). miR-526b could promote cell viability by inhibiting autophagy, and potentially targeting autophagy-associated gene 7 (ATG7) in SA-AKI (Liu et al., 2020b). miR-214 could alleviate AKI in septic mice by inhibiting the level of kidney autophagy through the regulation of the PTEN/AKT/mTOR pathway (Sang et al., 2021). Overexpression of TUG1 can reverse the expression of autophagy-related factors induced by LPS, like the increase of autophagy factors (LC3-II/I and beclin-1) and the decrease of p62 levels, thus inhibiting autophagy (Liu, 2019). In sepsis rats, lncRNA NKILA and the expression of autophagy-related proteins were significantly increased in kidney tissues, and lncRNA NKILA regulated the autophagy via PI3K/Akt pathway (Yang et al., 2020). At the same time, lncRNA NKILA knockdown represses cell autophagy in HK2 cells stimulated with LPS (Han et al., 2021). lncRNA SNHG14 was highly expressed in the plasma of sepsis patients with AKI and inhibited cell autophagy by negatively regulating miR-495-3p expression (Yang et al., 2021). ncRNA can affect the course of SA-AKI by affecting the expression of autophagy related factors, but the specific mechanism remains to be improved.
Oxidative Stress
Oxidative stress refers to the imbalance of the generation and clearance of oxygen free radicals in the body or cells. It results in the accumulation of reactive nitrogen species (RNS) and reactive oxygen species (ROS), including superoxide (-O₂-), hydrogen peroxide (H₂O₂) and hydroxyl free radical (HO), thus causing oxidative damage. The release of large amounts of ROS into the cytoplasm leads to oxidative modifications of proteins and lipids that severely affect cell function. Antioxidant enzymes such as superoxide dismutase (SOD), catalase (CAT), peroxidase (POD), glutathione S-transferase (GST), glutathione peroxidase (GSH-PX), glutathione reductase (GR), and thioredoxin peroxidase (TPX) can reflect antioxidant activity. The content of antioxidant glutathione (GSH) can reflect the antioxidant capacity of the body. And the content of malondialdehyde (MDA) can reflect the level of lipid peroxidation. The clinical significance of oxidative stress in SA-AKI has been confirmed by multiple studies. The stimulation of LPS leads to increased oxidative stress in the kidney tissues of mice, causing overproduction of reactive oxygen species (ROS) and MDA, reducing SOD, GSH, and CAT activities in kidney tissues, leading to SA-AKI (Zhang et al., 2017).
ncRNAs regulate oxidative stress in SA-AKI by changing the expression of oxidative stress-related factors. The overexpression of lncRNA 6406 can mitigate SA-AKI via oxidative stress suppression, as indicated by the sharp increase in SOD1, GSH, and HO-1 levels (Liu et al., 2020a). lncRNA small nucleolus RNA host gene 14 (SNHG14) was involved in the oxidative stress of LPS-induced HK-2 cell and SNHG14 overexpression can enhance SA-AKI through oxidative stress (Shi, 2021). circVMA21 and circ-Ttc3 mitigated the oxidative stress caused by SA-AKI. An increased expression of circ-Ttc3 and circVMA21 could markedly decrease the levels of ROS and MDA and increase GSH and the activities of SOD and CAT, attenuating the SA-AKI (Shi et al., 2020b; Li et al., 2021b; Ma et al., 2021). Moreover, a study suggested that circ-FANCA knockdown could increase the level of SOD and decrease the level of MDA to attenuate LPS-induced HK2 cell injury by regulating OXSR1 expression (Li et al., 2021a). Dexmedetomidine increased the expression of antioxidant markers SOD and CAT and decreased the levels of MDA and SOD on rats with SA-AKI by upregulating miR-146a expression (Ni et al., 2020). Oxidative stress is an essential pathological process of SA-AKI, while ncRNAs enhance the ability of cells to resist oxidative stress by reducing ROS production, thereby improving kidney function.
Clinical Applications of ncRNA in SA-AKI
ncRNAs have become a potential biomarker for SA-AKI detection. Moreover, regulation of their expression may provide new approaches for the treatment of SA-AKI.
Predictive Value
The expression of specific miRNAs is related to the severity and prognosis of the disease (Ge et al., 2017; Lin et al., 2019b). A clinical study showed that urinary miR-26b level is significantly increased in patients with SA-AKI and may be used for distinguishing between AKI sepsis from non-AKI sepsis with a sensitivity of 90.8% and specificity of 75.0%. Moreover, the urinary miR-26b level is closely related to the mortality of patients, which suggests that the urinary miR-26b may become the potential biomarker for SA-AKI (Zhang et al., 2018a). Through sample collection and statistical analysis, Huo et al found that miR-29a and miR-10a-5p were positively correlated with the expression of Scr, Cystatin C (Cys-C), and kidney injury molecule 1 (KIM-1), which resulted as independent risk factors for death in patients with sepsis. Furthermore, the auROC value of the combined detection of miR-29a and miR-10a-5p was more effective than that of the single detection of miR-29a, miR-10a-5p, Cys-C, Scr, and KIM-1, which indicated that miR-29a and miR-10a-5p have good predictive value in assessing the mortality of patients with sepsis (Huo et al., 2017). In septic mice following LPS or CLP treatment, serum and urinary miR-452 increased early before detectable renal dysfunction or tissue damage, and in septic patients, serum and urinary miR-452 levels were significantly higher in patients with AKI than in those without AKI. Moreover, the sensitivity of urinary miR-452 in detecting AKI in septic patients was significantly higher than that in urinary tissue inhibitor metalloproteinase-2 [TIMP2]* insulin-like growth factor binding protein-7 [IGFBP7], and urinary miR-452 was significantly positively correlated with SCr, suggested that urine miR-452 may be an effective biomarker for early detection of AKI in septic patients (Liu et al., 2020c). Septic patients with AKI also had significantly lower urinary miR-376b than septic patients without AKI, and urinary miR-376b was negatively correlated with Scr and BUN in septic patients, supporting its diagnostic value for septic AKI (Liu et al., 2020d).
The expression of lncRNA TCONS_00016233 is increased in the plasma of sepsis patients. The plasma TCONS_00016233 has been shown to be positively correlated with serum creatinine, TIMP2, IGFBP7, IL-1β, TNF-α, C-reactive protein (CRP), and urinary TCONS_00016233, thus indicating that TCONS_00016233 might serve as an early diagnosis marker for the septic AKI (Zhang et al., 2020a). As mentioned above, lncRNA TapSAKI have been confirmed to be differentially expressed in sepsis rats. Previous study has found that in patients with AKI, the circulating concentration of TapSAKI is an independent predictor of patients’ survival. Therefore, TapSAKI may become prognostic indicator of SA-AKI (Lorenzen et al., 2015).
These studies demonstrated that many ncRNAs are closely related to the occurrence and prognosis of SA-AKI and have potential predictive value (Table 1). However, whether ncRNAs could be used as clinical test index still requires extensive evaluation.
Therapeutic Potential
Correlation evidence revealed that kidney damage induced by sepsis could be improved by changing the expression of ncRNAs. Changing the expression of miR-93-5p, miR-126, miR-125b, miR-34a, and miR-155 can alleviate multiple organ damage, vascular leakage, inflammation, and cell apoptosis caused by sepsis, which indicates that changing the expression of miRNAs may become one of the crucial means to treat SA-AKI (Fan et al., 2014; He et al., 2020b). It has also been found that propofol, dexmedetomidine Ginkgolide A and dihydromyricetin reduce SA-AKI by regulating the miRNAs expression (Zheng et al., 2018; Ni et al., 2020; Li et al., 2021c; Tian et al., 2021). Moreover, another study found that curcumin alleviates renal function by suppressing lncRNA PVT1 in mice (Huang et al., 2020c). Resveratrol attenuated inflammation of SA-AKI by regulating lncRNA MALAT1 (Wang et al., 2021b). These studies have shown that changing the expression of ncRNAs can alleviate SA-AKI and can be used as a potential treatment for SA-AKI.
Gene therapy has become well accepted in clinical practice, while the related studies on SA-AKI are still limited. At present, gene therapy for SA-AKI is mainly used in animal model experiments by injecting the ncRNA’s siRNA or cDNA. However, this treatment method may become a major trend in the future and provide a new treatment strategy for SA-AKI.
Conclusion and Prospects
The current research on ncRNA-mediated SA-AKI has focused on miRNA. The role of ncRNA in the underlying mechanism of SA-AKI is being gradually discovered, and multitarget inhibition or overexpression has been shown to have significant beneficial results in animal models or cell models. Experiments have shown that SA-AKI can be improved by changing the expression of ncRNA with drugs. However, when translated into a clinical setting, these therapeutic methods face obstacles such as inadequate safety or efficiency, which should be addressed by future studies. Meanwhile, in SA-AKI patients and animal models, some ncRNAs expressions are associated with disease severity and prognosis, and serve as independent predictors of patient survival. Therefore, the ncRNAs have excellent prospects as disease biomarkers. However, ncRNA as a clinical trial indicator still needs to be widely evaluated.
Author Contributions
YC: Composing a first draft and proposal the research ideas; JZ: Review and revision; HJ, and ST: information retrieval; PL, and YC: Project Management; YF, and HC: supervise.
Funding
This work was supported by the grant from the Natural Science Foundation of Guangdong Province: 2019A1515011087 to JZ; Natural Science Foundation of Xinjiang Uygur Autonomous Region: 2018D01C016 to YF; National Natural Science Foundation of China: 82060130,81860130 to YF; Natural Science Foundation of Guangdong Province: 2021A1515012453 to HC; Guangzhou Science and Technology Innovation Commission: 202002030038 to HC.
Conflict of Interest
The authors declare that the research was conducted in the absence of any commercial or financial relationships that could be construed as a potential conflict of interest.
Publisher’s Note
All claims expressed in this article are solely those of the authors and do not necessarily represent those of their affiliated organizations, or those of the publisher, the editors and the reviewers. Any product that may be evaluated in this article, or claim that may be made by its manufacturer, is not guaranteed or endorsed by the publisher.
References
Bellomo R., Kellum J. A., Ronco C. (2012). Acute Kidney Injury. The Lancet 380 (9843), 756–766. doi:10.1016/s0140-6736(11)61454-2
Bhatt K., Wei Q., Pabla N., Dong G., Mi Q.-S., Liang M., et al. (2015). MicroRNA-687 Induced by Hypoxia-Inducible Factor-1 Targets Phosphatase and Tensin Homolog in Renal Ischemia-Reperfusion Injury. Jasn 26 (7), 1588–1596. doi:10.1681/asn.2014050463
Cao C., Yu M., Chai Y. (2019). Pathological Alteration and Therapeutic Implications of Sepsis-Induced Immune Cell Apoptosis. Cell Death Dis 10 (10), 782. doi:10.1038/s41419-019-2015-1
Cech T. R., Steitz J. A. (2014). The Noncoding RNA Revolution-Trashing Old Rules to Forge New Ones. Cell 157 (1), 77–94. doi:10.1016/j.cell.2014.03.008
Chen X., Zhao L., Xing Y., Lin B. (2018). Down-regulation of microRNA-21 Reduces Inflammation and Podocyte Apoptosis in Diabetic Nephropathy by Relieving the Repression of TIMP3 Expression. Biomed. Pharmacother. 108, 7–14. doi:10.1016/j.biopha.2018.09.007
Chen Y., Qiu J., Chen B., Lin Y., Chen Y., Xie G., et al. (2018). RETRACTED: Long Non-coding RNA NEAT1 Plays an Important Role in Sepsis-Induced Acute Kidney Injury by Targeting miR-204 and Modulating the NF-Κb Pathway. Int. immunopharmacology 59, 252–260. doi:10.1016/j.intimp.2018.03.023
Chertow G. M., Burdick E., Honour M., Bonventre J. V., Bates D. W. (2005). Acute Kidney Injury, Mortality, Length of Stay, and Costs in Hospitalized Patients. Jasn 16 (11), 3365–3370. doi:10.1681/asn.2004090740
Choi M. E. (2020). Autophagy in Kidney Disease. Annu. Rev. Physiol. 82, 297–322. doi:10.1146/annurev-physiol-021119-034658
Chuppa S., Liang M., Liu P., Liu Y., Casati M. C., Cowley A. W., et al. (2018). MicroRNA-21 Regulates Peroxisome Proliferator-Activated Receptor Alpha, a Molecular Mechanism of Cardiac Pathology in Cardiorenal Syndrome Type 4. Kidney Int. 93 (2), 375–389. doi:10.1016/j.kint.2017.05.014
Deng J., Tan W., Luo Q., Lin L., Zheng L., Yang J. (2021). Long Non-coding RNA MEG3 Promotes Renal Tubular Epithelial Cell Pyroptosis by Regulating the miR-18a-3p/GSDMD Pathway in Lipopolysaccharide-Induced Acute Kidney Injury. Front. Physiol. 12, 663216. doi:10.3389/fphys.2021.663216
Deng L. T., Wang Q. L., Yu C., Gao M. (2021). lncRNA PVT1 Modulates NLRP3-mediated P-yroptosis in S-eptic A-cute K-idney I-njury by T-argeting miR-20a-5p. Mol. Med. Rep. 23 (4). doi:10.3892/mmr.2021.11910
Elmore S. (2007). Apoptosis: a Review of Programmed Cell Death. Toxicol. Pathol. 35 (4), 495–516. doi:10.1080/01926230701320337
Fan H., Goodwin A. J., Chang E., Zingarelli B., Borg K., Guan S., et al. (2014). Endothelial Progenitor Cells and a Stromal Cell-Derived Factor-1α Analogue Synergistically Improve Survival in Sepsis. Am. J. Respir. Crit. Care Med. 189 (12), 1509–1519. doi:10.1164/rccm.201312-2163oc
Fan W., Huang J., Xiao H., Liang Z. (2016). MicroRNA-22 Is Downregulated in clear Cell Renal Cell Carcinoma, and Inhibits Cell Growth, Migration and Invasion by Targeting PTEN. Mol. Med. Rep. 13 (6), 4800–4806. doi:10.3892/mmr.2016.5101
Fu D., Dong J., Li P., Tang C., Cheng W., Xu Z., et al. (2017). MiRNA-21 Has Effects to Protect Kidney Injury Induced by Sepsis. Biomed. Pharmacother. 94, 1138–1144. doi:10.1016/j.biopha.2017.07.098
Ge Q.-M., Huang C.-M., Zhu X.-Y., Bian F., Pan S.-M. (2017). Differentially Expressed miRNAs in Sepsis-Induced Acute Kidney Injury Target Oxidative Stress and Mitochondrial Dysfunction Pathways. PLoS One 12 (3), e0173292. doi:10.1371/journal.pone.0173292
Gomez I. G., MacKenna D. A., Johnson B. G., Kaimal V., Roach A. M., Ren S., et al. (2015). Anti-microRNA-21 Oligonucleotides Prevent Alport Nephropathy Progression by Stimulating Metabolic Pathways. J. Clin. Invest. 125 (1), 141–156. doi:10.1172/jci75852
Han D., Fang R., Shi R., Jin Y., Wang Q. (2021). LncRNA NKILA Knockdown Promotes Cell Viability and Represses Cell Apoptosis, Autophagy and Inflammation in Lipopolysaccharide-Induced Sepsis Model by Regulating miR-140-5p/CLDN2 axis. Biochem. Biophys. Res. Commun. 559, 8–14. doi:10.1016/j.bbrc.2021.04.074
He J., Zhang B., Gan H. (2018). CIDEC Is Involved in LPS-Induced Inflammation and Apoptosis in Renal Tubular Epithelial Cells. Inflammation 41 (5), 1912–1921. doi:10.1007/s10753-018-0834-3
He S. Y., Wang G., Pei Y. H., Zhu H. P. (2020). miR ‐34b‐3p Protects against Acute Kidney Injury in Sepsis Mice via Targeting Ubiquitin‐like Protein 4A. Kaohsiung J. Med. Sci. 36 (10), 817–824. doi:10.1002/kjm2.12255
He Y., Sun Y., Peng J. (2021). Regulates Sepsis-Induced Kidney Injury by Targeting the miR-495-3p/CRBN Axis. Inflammation 44 (4), 1464–1477.
He Z., Wang H., Yue L. (2020). Endothelial Progenitor Cells-Secreted Extracellular Vesicles Containing microRNA-93-5p Confer protection against Sepsis-Induced Acute Kidney Injury via the KDM6B/H3K27me3/TNF-α axis. Exp. Cel. Res. 395 (2), 112173. doi:10.1016/j.yexcr.2020.112173
Huang G., Bao J., Shao X., Zhou W., Wu B., Ni Z., et al. (2020). Inhibiting Pannexin-1 Alleviates Sepsis-Induced Acute Kidney Injury via Decreasing NLRP3 Inflammasome Activation and Cell Apoptosis. Life Sci. 254, 117791. doi:10.1016/j.lfs.2020.117791
Huang M., Cai S., Su J. (2019). The Pathogenesis of Sepsis and Potential Therapeutic Targets. Int. J. Mol. Sci. 20 (21). doi:10.3390/ijms20215376
Huang Q., Huang C., Luo Y., He F., Zhang R. (2018). Circulating lncRNA NEAT1 Correlates with Increased Risk, Elevated Severity and Unfavorable Prognosis in Sepsis Patients. Am. J. Emerg. Med. 36 (9), 1659–1663. doi:10.1016/j.ajem.2018.06.008
Huang W., Lan X., Li X., Wang D., Sun Y., Wang Q., et al. (2017). RETRACTED: Long Non-coding RNA PVT1 Promote LPS-Induced Septic Acute Kidney Injury by Regulating TNFα and JNK/NF-κB Pathways in HK-2 Cells. Int. immunopharmacology 47, 134–140. doi:10.1016/j.intimp.2017.03.030
Huang W., Li X., Wang D., Sun Y., Wang Q., Bu Y., et al. (2020). Curcumin Reduces LPS-Induced Septic Acute Kidney Injury through Suppression of lncRNA PVT1 in Mice. Life Sci. 254, 117340. doi:10.1016/j.lfs.2020.117340
Huang X., Hou X., Chuan L., Wei S., Wang J., Yang X., et al. (2020). miR-129-5p Alleviates LPS-Induced Acute Kidney Injury via Targeting HMGB1/TLRs/NF-kappaB Pathway. Int. immunopharmacology 89, 107016. doi:10.1016/j.intimp.2020.107016
Huang Y., Zhou L.-s., Yan L., Ren J., Zhou D.-x., Li S.-S. (2015). Alpinetin Inhibits Lipopolysaccharide-Induced Acute Kidney Injury in Mice. Int. Immunopharmacology 28 (2), 1003–1008. doi:10.1016/j.intimp.2015.08.002
Huo R., Dai M., Fan Y., Zhou J. Z., Li L., Zu J. (2017). Predictive Value of miRNA-29a and miRNA-10a-5p for 28-day Mortality in Patients with Sepsis-Induced Acute Kidney Injury. Nan Fang Yi Ke Da Xue Xue Bao 37 (5), 646–651.
Jia P., Teng J., Zou J., Fang Y., Wu X., Liang M., et al. (2015). Xenon Protects against Septic Acute Kidney Injury via miR-21 Target Signaling Pathway. Crit. Care Med. 43 (7), e250–e259. doi:10.1097/ccm.0000000000001001
Jiang X., Li D., Shen W., Shen X., Liu Y. (2019). LncRNA NEAT1 Promotes Hypoxia‐induced Renal Tubular Epithelial Apoptosis through Downregulating miR‐27a‐3p. J. Cel Biochem 120 (9), 16273–16282. doi:10.1002/jcb.28909
Jiang Z. J., Zhang M. Y., Fan Z. W., Sun W. L., Tang Y. (2019). Influence of lncRNA HOTAIR on Acute Kidney Injury in Sepsis Rats through Regulating miR-34a/Bcl-2 Pathway. Eur. Rev. Med. Pharmacol. Sci. 23 (8), 3512–3519. doi:10.26355/eurrev_201904_17717
Juan C. X., Mao Y., Cao Q., Chen Y., Zhou L. B., Li S., et al. (2021). Exosome-mediated Pyroptosis of miR-93-TXNIP-NLRP3 Leads to Functional Difference between M1 and M2 Macrophages in Sepsis-Induced Acute Kidney Injury. J. Cel Mol Med 25 (10), 4786–4799. doi:10.1111/jcmm.16449
Kaushal G. P., Shah S. V. (2016). Autophagy in Acute Kidney Injury. Kidney Int. 89 (4), 779–791. doi:10.1016/j.kint.2015.11.021
Keir I., Kellum J. A. (2015). Acute Kidney Injury in Severe Sepsis: Pathophysiology, Diagnosis, and Treatment Recommendations. J. Vet. Emerg. Crit. Care 25 (2), 200–209. doi:10.1111/vec.12297
Kellum J. A., Prowle J. R. (2018). Paradigms of Acute Kidney Injury in the Intensive Care Setting. Nat. Rev. Nephrol. 14 (4), 217–230. doi:10.1038/nrneph.2017.184
Lan R., Geng H., Polichnowski A. J., Singha P. K., Saikumar P., McEwen D. G., et al. (2012). PTEN Loss Defines a TGF-β-Induced Tubule Phenotype of Failed Differentiation and JNK Signaling during Renal Fibrosis. Am. J. Physiology-Renal PhysiologyRenal Physiol. 302 (9), F1210–F1223. doi:10.1152/ajprenal.00660.2011
Langenberg C., Gobe G., Hood S., May C. N., Bellomo R. (2014). Renal Histopathology during Experimental Septic Acute Kidney Injury and Recovery*. Crit. Care Med. 42 (1), e58–e67. doi:10.1097/ccm.0b013e3182a639da
Lee S.-Y., Lee Y.-S., Choi H.-M., Ko Y.-S., Lee H.-Y., Jo S.-K., et al. (2012). Distinct Pathophysiologic Mechanisms of Septic Acute Kidney Injury. Crit. Care Med. 40 (11), 2997–3006. doi:10.1097/ccm.0b013e31825b912d
Leemans J. C., Butter L. M., Teske G. J. D., Stroo I., Pulskens W. P., Florquin S. (2012). The Toll Interleukin-1 Receptor (IL-1R) 8/single Ig Domain IL-1R-related Molecule Modulates the Renal Response to Bacterial Infection. Infect. Immun. 80 (11), 3812–3820. doi:10.1128/iai.00422-12
Li H., Zhang X., Wang P., Zhou X., Liang H., Li C. (2021). Knockdown of Circ-FANCA Alleviates LPS-Induced HK2 Cell Injury via Targeting miR-93-5p/OXSR1 axis in Septic Acute Kidney Injury. Diabetol. Metab. Syndr. 13 (1), 7. doi:10.1186/s13098-021-00625-8
Li J., Chen J., Yang Y., Ding R., Wang M., Gu Z. (2021). Ginkgolide A Attenuates Sepsis-Associated Kidney Damage via Upregulating microRNA-25 with NADPH Oxidase 4 as the Target. Int. immunopharmacology 95, 107514. doi:10.1016/j.intimp.2021.107514
Li X., Li R., Gong Q., Shi D., Song L., Song Y. (2021). Circular RNA circVMA21 Ameliorates Lipopolysaccharide (LPS)-induced Acute Kidney Injury by Targeting the miR-199a-5p/NRP1 axis in Sepsis. Biochem. biophysical Res. Commun. 548, 174–181. doi:10.1016/j.bbrc.2021.02.028
Li X. (2020). miR-30c-5p Alleviated Pyroptosis during Sepsis-Induced Acute Kidney Injury via Targeting TXNIP. Inflammation.
Lin Y., Ding Y., Song S., Li M., Wang T., Guo F. (2019). Expression Patterns and Prognostic Value of miR-210, miR-494, and miR-205 in Middle-Aged and Old Patients with Sepsis-Induced Acute Kidney Injury. Bosn J. Basic Med. Sci. 19 (3), 249–256. doi:10.17305/bjbms.2019.4131
Lin Z., Liu Z., Wang X., Qiu C., Zheng S. (2019). MiR-21-3p Plays a Crucial Role in Metabolism Alteration of Renal Tubular Epithelial Cells during Sepsis Associated Acute Kidney Injury via AKT/CDK2-FOXO1 Pathway. Biomed. Res. Int. 2019, 2821731. doi:10.1155/2019/2821731
Ling H., Li Q., Duan Z. P., Wang Y. J., Hu B. Q., Dai X. G. (2021). LncRNA GAS5 Inhibits miR-579-3p to Activate SIRT1/PGC-1α/Nrf2 Signaling Pathway to Reduce Cell Pyroptosis in Sepsis-Associated Renal Injury. Am. J. Physiol. Cel Physiol 321 (1), C117–C133. doi:10.1152/ajpcell.00394.2020
Liu X. (2019). Downregulation of lncRNA TUG1 Contributes to the Development of Sepsis-Associated Acute Kidney Injury via Regulating miR-142-3p/sirtuin 1 axis and Modulating NF-kappaB Pathway. J. Cel Biochem 120, 11331–11341. doi:10.1002/jcb.28409
Liu X., Zhu N., Zhang B., Xu S. B. (2020). Long Noncoding RNA TCONS_00016406 Attenuates Lipopolysaccharide-Induced Acute Kidney Injury by Regulating the miR-687/PTEN Pathway. Front. Physiol. 11, 622. doi:10.3389/fphys.2020.00622
Liu Y., Xiao J., Sun J., Chen W., Wang S., Fu R., et al. (2020). ATG7 Promotes Autophagy in Sepsis-induced A-cute K-idney I-njury and I-s I-nhibited by miR-526b. Mol. Med. Rep. 21 (5), 2193–2201. doi:10.3892/mmr.2020.11001
Liu Z., Tang C., He L., Yang D., Cai J., Zhu J., et al. (2020). The Negative Feedback Loop of NF-κB/miR-376b/NFKBIZ in Septic Acute Kidney Injury. JCI insight 5 (24). doi:10.1172/jci.insight.142272
Liu Z., Yang D., Gao J., Xiang X., Hu X., Li S., et al. (2020). Discovery and Validation of miR-452 as an Effective Biomarker for Acute Kidney Injury in Sepsis. Theranostics 10 (26), 11963–11975. doi:10.7150/thno.50093
Lorenzen J. M., Schauerte C., Kielstein J. T., Hübner A., Martino F., Fiedler J., et al. (2015). Circulating Long Noncoding RNA TapSAKI Is a Predictor of Mortality in Critically Ill Patients with Acute Kidney Injury. Clin. Chem. 61 (1), 191–201. doi:10.1373/clinchem.2014.230359
Lu S., Dong L., Jing X., Gen-Yang C., Zhan-Zheng Z. (2020). Abnormal lncRNA CCAT1/microRNA-155/SIRT1 axis Promoted Inflammatory Response and Apoptosis of Tubular Epithelial Cells in LPS Caused Acute Kidney Injury. Mitochondrion 53, 76–90. doi:10.1016/j.mito.2020.03.010
Lu S., Wu H., Xu J., He Z., Li H., Ning C. (2020). SIKIAT1/miR-96/FOXA1 axis Regulates Sepsis-Induced Kidney Injury through Induction of Apoptosis. Inflamm. Res. 69 (7), 645–656. doi:10.1007/s00011-020-01350-0
Luo M., Yan D., Sun Q., Tao J., Xu L., Sun H., et al. (2020). Ginsenoside Rg1 Attenuates Cardiomyocyte Apoptosis and Inflammation via the TLR4/NF‐kB/NLRP3 Pathway. J. Cel Biochem 121 (4), 2994–3004. doi:10.1002/jcb.29556
Luo N., Gao H. M., Wang Y. Q., Li H. J., Li Y. (2020). MiR-942-5p Alleviates Septic Acute Kidney Injury by Targeting FOXO3. Eur. Rev. Med. Pharmacol. Sci. 24 (11), 6237–6244. doi:10.26355/eurrev_202006_21521
Ma J., Li Y.-t., Zhang S.-x., Fu S.-z., Ye X.-z. (2019). MiR-590-3p Attenuates Acute Kidney Injury by Inhibiting Tumor Necrosis Factor Receptor-Associated Factor 6 in Septic Mice. Inflammation 42 (2), 637–649. doi:10.1007/s10753-018-0921-5
Ma P., Zhang C., Huo P., Li Y., Yang H. (2020). A Novel Role of the miR-152-3p/ERRFI1/STAT3 Pathway Modulates the Apoptosis and Inflammatory Response after Acute Kidney Injury. J. Biochem. Mol. Toxicol. 34, e22540. doi:10.1002/jbt.22540
Ma X., Zhu G., Jiao T., Shao F. (2021). Effects of Circular RNA Ttc3/miR-148a/Rcan2 axis on Inflammation and Oxidative Stress in Rats with Acute Kidney Injury Induced by Sepsis. Life Sci. 272, 119233. doi:10.1016/j.lfs.2021.119233
Meng X.-M., Ren G.-L., Gao L., Yang Q., Li H.-D., Wu W.-F., et al. (2018). NADPH Oxidase 4 Promotes Cisplatin-Induced Acute Kidney Injury via ROS-Mediated Programmed Cell Death and Inflammation. Lab. Invest. 98 (1), 63–78. doi:10.1038/labinvest.2017.120
Ni J., He J., Kang L., Zhong Z., Wang L., Yin S. (2020). Effects of Dexmedetomidine Pretreatment on Rats with Sepsis-Induced Acute Kidney Injury and miR-146a Expression. Cel Mol Biol (Noisy-le-grand) 66 (2), 93–98. doi:10.14715/cmb/2020.66.2.15
Ning K. (2004). Dual Neuroprotective Signaling Mediated by Downregulating Two Distinct Phosphatase Activities of PTEN. J. Neurosci. 24 (16), 4052–4060. doi:10.1523/jneurosci.5449-03.2004
Pan T., Jia P., Chen N., Fang Y., Liang Y., Guo M., et al. (2019). Delayed Remote Ischemic Preconditioning ConfersRenoprotection against Septic Acute Kidney Injury via Exosomal miR-21. Theranostics 9 (2), 405–423. doi:10.7150/thno.29832
Parzych K. R., Klionsky D. J. (2014). An Overview of Autophagy: Morphology, Mechanism, and Regulation. Antioxid. Redox signaling 20 (3), 460–473. doi:10.1089/ars.2013.5371
Qin L. Y., Wang M. X., Zhang H. (2020). MiR-133a Alleviates Renal Injury Caused by Sepsis by Targeting BNIP3L. Eur. Rev. Med. Pharmacol. Sci. 24 (5), 2632–2639. doi:10.26355/eurrev_202003_20532
Qin Y., Wang G., Peng Z. (2019). MicroRNA-191-5p Diminished Sepsis-Induced Acute Kidney Injury through Targeting Oxidative Stress Responsive 1 in Rat Models. Biosci. Rep. 39 (8). doi:10.1042/BSR20190548
Ren Y., Cui Y., Xiong X., Wang C., Zhang Y. (2017). Inhibition of microRNA-155 Alleviates Lipopolysaccharide-Induced Kidney Injury in Mice. Int. J. Clin. Exp. Pathol. 10 (9), 9362–9371.
Rousta A.-M., Mirahmadi S.-M. -S., Shahmohammadi A., Nourabadi D., Khajevand-Khazaei M.-R., Baluchnejadmojarad T., et al. (2018). Protective Effect of Sesamin in Lipopolysaccharide-Induced Mouse Model of Acute Kidney Injury via Attenuation of Oxidative Stress, Inflammation, and Apoptosis. Immunopharmacology and Immunotoxicology 40 (5), 423–429. doi:10.1080/08923973.2018.1523926
Sang Z., Dong S., Zhang P., Wei Y. (2021). miR-214 A-meliorates S-epsis-induced A-cute K-idney I-njury via PTEN/AKT/mTOR-regulated A-utophagy. Mol. Med. Rep. 24 (4). doi:10.3892/mmr.2021.12322
Shen J., Liu L., Zhang F., Gu J., Pan G. (2019). LncRNA TapSAKI Promotes Inflammation Injury in HK-2 Cells and Urine Derived Sepsis-Induced Kidney Injury. J. Pharm. Pharmacol. 71 (5), 839–848. doi:10.1111/jphp.13049
Shen J., Zhang J., Jiang X., Wang H., Pan G. (2018). LncRNA HOX Transcript Antisense RNA Accelerated Kidney Injury Induced by Urine-Derived Sepsis through the miR-22/high Mobility Group Box 1 Pathway. Life Sci. 210, 185–191. doi:10.1016/j.lfs.2018.08.041
Shen Y., Yu J., Jing Y., Zhang J. (2019). MiR-106a Aggravates Sepsis-Induced Acute Kidney Injury by Targeting THBS2 in Mice Model. Acta Cir Bras 34 (6), e201900602. doi:10.1590/s0102-865020190060000002
Shi C.-c., Pan L.-y., Peng Z.-y., Li J.-g. (2020). CircMTO1 Attenuated Acute Kidney Injury through Regulating miR-337. Inflammation 43 (4), 1304–1311. doi:10.1007/s10753-020-01209-w
Shi C. (2021). lncRNA SNHG14 Plays a Role in Sepsis-Induced Acute Kidney Injury by Regulating miR-93. Mediators Inflamm. 2021, 5318369. doi:10.1155/2021/5318369
Shi J., Gao W., Shao F. (2017). Pyroptosis: Gasdermin-Mediated Programmed Necrotic Cell Death. Trends Biochemical Sciences 42 (4), 245–254. doi:10.1016/j.tibs.2016.10.004
Shi X., Ma W., Li Y., Wang H., Pan S., Pan Y., et al. (2020). CircPRKCI Relieves Lipopolysaccharide‐induced HK2 Cell Injury by Upregulating the Expression of miR‐545 Target Gene ZEB2. Biofactors 46 (3), 475–486. doi:10.1002/biof.1620
Shi Y., Sun C. F., Ge W. H., Du Y. P., Hu N. B. (2020). Circular RNA VMA21 Ameliorates Sepsis‐associated Acute Kidney Injury by Regulating miR‐9‐3p/SMG1/inflammation axis and Oxidative Stress. J. Cel. Mol. Med. 24 (19), 11397–11408. doi:10.1111/jcmm.15741
Singer M., Deutschman C. S., Seymour C. W., Shankar-Hari M., Annane D., Bauer M., et al. (2016). The Third International Consensus Definitions for Sepsis and Septic Shock (Sepsis-3). JAMA 315 (8), 801–810. doi:10.1001/jama.2016.0287
Sisti F., Wang S., Brandt S. L., Glosson-Byers N., Mayo L. D., Son Y. M., et al. (2018). Nuclear PTEN Enhances the Maturation of a microRNA Regulon to Limit MyD88-dependent Susceptibility to Sepsis. Sci. Signal. 11 (528). doi:10.1126/scisignal.aai9085
Song N., Zhang T., Xu X., Lu Z., Yu X., Fang Y., et al. (2018). miR-21 Protects against Ischemia/Reperfusion-Induced Acute Kidney Injury by Preventing Epithelial Cell Apoptosis and Inhibiting Dendritic Cell Maturation. Front. Physiol. 9, 790. doi:10.3389/fphys.2018.00790
Sun B. Q., Sui Y. D., Huang H., Zou X. B., Chen S. C., Yu Z. K. (2019). Effect of lncRNA CRNDE on Sepsis-Related Kidney Injury through the TLR3/NF-Κb Pathway. Eur. Rev. Med. Pharmacol. Sci. 23 (23), 10489–10497. doi:10.26355/eurrev_201912_19688
Tan J., Fan J., He J., Zhao L., Tang H. (2020). Knockdown of LncRNA DLX6-AS1 Inhibits HK-2 Cell Pyroptosis via Regulating miR-223-3p/NLRP3 Pathway in Lipopolysaccharide-Induced Acute Kidney Injury. J. Bioenerg. Biomembr 52 (5), 367–376. doi:10.1007/s10863-020-09845-5
Tan M., Bei R. (2021). Circ_0091702 Serves as a Sponge of miR-545-3p to Attenuate Sepsis-Related Acute Kidney Injury by Upregulating THBS2. J. Mol. Histol. 52 (4), 717–728. doi:10.1007/s10735-021-09991-z
Tian X., Liu Y., Wang H., Zhang J., Xie L., Huo Y., et al. (2021). The Role of miR-199b-3p in Regulating Nrf2 Pathway by Dihydromyricetin to Alleviate Septic Acute Kidney Injury. Free Radic. Res. 55 (7), 842–852. doi:10.1080/10715762.2021.1962008
Uchino S. (2005). Acute Renal Failure in Critically Ill PatientsA Multinational, Multicenter Study. JAMA 294 (7), 813–818. doi:10.1001/jama.294.7.813
Verma S. K., Molitoris B. A. (2015). Renal Endothelial Injury and Microvascular Dysfunction in Acute Kidney Injury. Semin. Nephrol. 35 (1), 96–107. doi:10.1016/j.semnephrol.2015.01.010
Wacker C., Prkno A., Brunkhorst F. M., Schlattmann P. (2013). Procalcitonin as a Diagnostic Marker for Sepsis: a Systematic Review and Meta-Analysis. Lancet Infect. Dis. 13 (5), 426–435. doi:10.1016/s1473-3099(12)70323-7
Wang B., Wang Y., Xu K., Zeng Z., Xu Z., Yue D., et al. (2021). Resveratrol Alleviates Sepsis-Induced Acute Kidney Injury by Deactivating the lncRNA MALAT1/MiR-205 axis. cejoi 46 (3), 295–304. doi:10.5114/ceji.2021.109195
Wang J.-N., Yang Q., Yang C., Cai Y.-T., Xing T., Gao L., et al. (2020). Smad3 Promotes AKI Sensitivity in Diabetic Mice via Interaction with P53 and Induction of NOX4-dependent ROS Production. Redox Biol. 32, 101479. doi:10.1016/j.redox.2020.101479
Wang J., Chen Y., Tang Z., Hu D., Yao C., Yang L. (2020). LncRNA NEAT1 Regulated Inflammation and Apoptosis in a Rat Model of Sepsis-Induced Acute Kidney Injury via MiR-27a-3p/TAB3 axis. Biosci. Biotechnol. Biochem. 84 (11), 2215–2227. doi:10.1080/09168451.2020.1792760
Wang J., Song J., Li Y., Shao J., Xie Z., Sun K. (2020). Down-regulation of LncRNA CRNDE Aggravates Kidney Injury via Increasing MiR-181a-5p in Sepsis. Int. immunopharmacology 79, 105933. doi:10.1016/j.intimp.2019.105933
Wang J. X., Tao Y. L., Wang Z., Mao Q. J. (2020). MiR-20a Promotes Kidney Injury in Sepsis Rats through Autophagy. J. Biol. Regul. Homeost Agents 34 (4), 1277–1283. doi:10.23812/20-174-A
Wang L., Wang K., Tian Z. (2020). miR-128-3p Inhibits NRP1 Expression and Promotes Inflammatory Response to Acute Kidney Injury in Sepsis. Inflammation 43 (5), 1772–1779. doi:10.1007/s10753-020-01251-8
Wang M., Wei J., Shang F., Zang K., Ji T. (2020). Long Non-coding RNA CASC2 A-meliorates S-epsis-induced A-cute K-idney I-njury by R-egulating the miR-155 and NF-κB P-athway. Int. J. Mol. Med. 45 (5), 1554–1562. doi:10.3892/ijmm.2020.4518
Wang M., Wei J., Shang F., Zang K., Zhang P. (2021). Down-regulation of lncRNA SNHG5 Relieves Sepsis-Induced Acute Kidney Injury by Regulating the miR-374a-3p/TLR4/NF-Κb Pathway. J. Biochem. 169 (5), 575–583. doi:10.1093/jb/mvab008
Wang S., Zhang Z., Wang J., Miao H. (2017). MiR-107 Induces TNF-α Secretion in Endothelial Cells Causing Tubular Cell Injury in Patients with Septic Acute Kidney Injury. Biochem. Biophysical Res. Commun. 483 (1), 45–51. doi:10.1016/j.bbrc.2017.01.013
Wang W., Guo Z.-H. (2020). Downregulation of lncRNA NEAT1 Ameliorates LPS-Induced Inflammatory Responses by Promoting Macrophage M2 Polarization via miR-125a-5p/TRAF6/TAK1 Axis. Inflammation 43 (4), 1548–1560. doi:10.1007/s10753-020-01231-y
Wang X., Wang Y., Kong M., Yang J. (2020). MiR-22-3p Suppresses Sepsis-Induced Acute Kidney Injury by Targeting PTEN. Biosci. Rep. 40 (6). doi:10.1042/BSR20200527
Wang Y., Xu Z., Yue D., Zeng Z., Yuan W., Xu K. (2020). Linkage of lncRNA CRNDE Sponging miR-181a-5p with Aggravated Inflammation Underlying Sepsis. Innate Immun. 26 (2), 152–161. doi:10.1177/1753425919880946
Wei W., Yao Y.-Y., Bi H.-Y., Zhai Z., Gao Y. (2020). miR-21 Protects against Lipopolysaccharide-Stimulated Acute Kidney Injury and Apoptosis by Targeting CDK6. Ann. Transl Med. 8 (6), 303. doi:10.21037/atm.2020.03.01
Wei W., Yao Y., Bi H., Xu W., Gao Y. (2021). Circular RNA Circ_0068,888 Protects against Lipopolysaccharide-Induced HK-2 Cell Injury via Sponging microRNA-21-5p. Biochem. biophysical Res. Commun. 540, 1–7. doi:10.1016/j.bbrc.2020.12.018
Wu H., Wang J., Ma Z. (2020). Long Noncoding RNA HOXA-AS2 Mediates microRNA-106b-5p to Repress Sepsis-Engendered Acute Kidney Injury. J. Biochem. Mol. Toxicol. 34 (4), e22453. doi:10.1002/jbt.22453
Wu S. (2020). Effects and Mechanism of lncRNA CRNDE on Sepsis-Induced Acute Kidney Injury. Anal. Cel Pathol (Amst) 2020, 8576234. doi:10.1155/2020/8576234
Xu H.-P., Ma X.-Y., Yang C. (2021). Circular RNA TLK1 Promotes Sepsis-Associated Acute Kidney Injury by Regulating Inflammation and Oxidative Stress through miR-106a-5p/HMGB1 Axis. Front. Mol. Biosci. 8, 660269. doi:10.3389/fmolb.2021.660269
Xue Z., Xi Q., Liu H., Guo X., Zhang J., Zhang Z., et al. (2019). miR-21 Promotes NLRP3 Inflammasome Activation to Mediate Pyroptosis and Endotoxic Shock. Cel Death Dis 10 (6), 461. doi:10.1038/s41419-019-1713-z
Yang N., Wang H., Zhang L., Lv J., Niu Z., Liu J., et al. (2021). Long Non-coding RNA SNHG14 Aggravates LPS-Induced Acute Kidney Injury through Regulating miR-495-3p/HIPK1. Acta Biochim. Biophys. Sinica 53 (6), 719–728. doi:10.1093/abbs/gmab034
Yang R., Liu S., Wen J., Xue L., Zhang Y., Yan D., et al. (2018). Inhibition of Maternally Expressed Gene 3 Attenuated Lipopolysaccharide‐induced Apoptosis through Sponging miR‐21 in Renal Tubular Epithelial Cells. J. Cel Biochem 119 (9), 7800–7806. doi:10.1002/jcb.27163
Yang Y. M., Li Y. H., Ding L. L., Fu Y., Li N. (2020). Regulatory Effect of lncRNA NKILA on Autophagy Induced by Sepsis Kidney Injury. Eur. Rev. Med. Pharmacol. Sci. 24 (8), 4057. doi:10.26355/eurrev_202004_20974
Ye Z., Zhang L., Li R., Dong W., Liu S., Li Z., et al. (2019). Caspase-11 Mediates Pyroptosis of Tubular Epithelial Cells and Septic Acute Kidney Injury. Kidney Blood Press. Res. 44 (4), 465–478. doi:10.1159/000499685
Yong H. (2020). lncRNA MALAT1 Accelerates Skeletal Muscle Cell Apoptosis and Inflammatory Response in Sepsis by Decreasing BRCA1 Expression by Recruiting EZH2. Molecular Therapy. Nucleic acids 19, 28. doi:10.1016/j.omtn.2019.10.028
Yu T.-M., Palanisamy K., Sun K.-T., Day Y.-J., Shu K.-H., Wang I.-K., et al. (2016). RANTES Mediates Kidney Ischemia Reperfusion Injury through a Possible Role of HIF-1α and LncRNA PRINS. Sci. Rep. 6, 18424. doi:10.1038/srep18424
Zhan Y., Zhu M., Liu S., Lu J., Ni Z., Cai H., et al. (2021). MicroRNA-93 I-nhibits the A-poptosis and I-nflammatory R-esponse of T-ubular E-pithelial C-ells via the PTEN/AKT/mTOR P-athway in A-cute K-idney I-njury. Mol. Med. Rep. 24 (3). doi:10.3892/mmr.2021.12305
Zhang J., Wang C. J., Tang X. M., Wei Y. K. (2018). Urinary miR-26b as a Potential Biomarker for Patients with Sepsis-Associated Acute Kidney Injury: a Chinese Population-Based Study. Eur. Rev. Med. Pharmacol. Sci. 22 (14), 4604–4610. doi:10.26355/eurrev_201807_15518
Zhang L., He S., Wang Y., Zhu X., Shao W., Xu Q., et al. (2020). miRNA-20a Suppressed Lipopolysaccharide‐induced HK‐2 Cells Injury via NFκB and ERK1/2 Signaling by Targeting CXCL12. Mol. Immunol. 118, 117–123. doi:10.1016/j.molimm.2019.12.009
Zhang P., Yi L., Qu S., Dai J., Li X., Liu B., et al. (2020). The Biomarker TCONS_00016233 Drives Septic AKI by Targeting the miR-22-3p/AIFM1 Signaling Axis. Mol. Ther. - Nucleic Acids 19, 1027–1042. doi:10.1016/j.omtn.2019.12.037
Zhang R., Zhu Y., Li Y., Liu W., Yin L., Yin S., et al. (2020). Human Umbilical Cord Mesenchymal Stem Cell Exosomes Alleviate Sepsis-Associated Acute Kidney Injury via Regulating microRNA-146b Expression. Biotechnol. Lett. 42 (4), 669–679. doi:10.1007/s10529-020-02831-2
Zhang S., Ma J., Sheng L., Zhang D., Chen X., Yang J., et al. (2017). Total Coumarins from Hydrangea Paniculata Show Renal Protective Effects in Lipopolysaccharide-Induced Acute Kidney Injury via Anti-inflammatory and Antioxidant Activities. Front. Pharmacol. 8, 872. doi:10.3389/fphar.2017.00872
Zhang Y., Xia F., Wu J., Yang A. X., Zhang Y. Y., Zhao H., et al. (2019). MiR-205 Influences Renal Injury in Sepsis Rats through HMGB1-PTEN Signaling Pathway. Eur. Rev. Med. Pharmacol. Sci. 23 (24), 10950–10956. doi:10.26355/eurrev_201912_19798
Zhang Y., Zhang Y. Y., Xia F., Yang A. X., Qian J. X., Zhao H., et al. (2019). Effect of lncRNA-MIAT on Kidney Injury in Sepsis Rats via Regulating miR-29a Expression. Eur. Rev. Med. Pharmacol. Sci. 23 (24), 10942–10949. doi:10.26355/eurrev_201912_19797
Zhang Y., Zhao S., Wu D., Liu X., Shi M., Wang Y., et al. (2018). MicroRNA-22 Promotes Renal Tubulointerstitial Fibrosis by Targeting PTEN and Suppressing Autophagy in Diabetic Nephropathy. J. Diabetes Res. 2018, 4728645. doi:10.1155/2018/4728645
Zhang Y., Wang L., Meng L., Cao G., Wu Y. (2019). Sirtuin 6 Overexpression Relieves Sepsis-Induced Acute Kidney Injury by Promoting Autophagy. Cell Cycle 18 (4), 425–436. doi:10.1080/15384101.2019.1568746
Zhao H., Chen B., Li Z., Wang B., Li L. (2020). Long Noncoding RNA DANCR Suppressed Lipopolysaccharide-Induced Septic Acute Kidney Injury by Regulating miR-214 in HK-2 Cells. Med. Sci. Monit. 26, e921822. doi:10.12659/MSM.921822
Zhao Y., Feng X., Li B., Sha J., Wang C., Yang T., et al. (2020). Dexmedetomidine Protects against Lipopolysaccharide-Induced Acute Kidney Injury by Enhancing Autophagy through Inhibition of the PI3K/AKT/mTOR Pathway. Front. Pharmacol. 11, 128. doi:10.3389/fphar.2020.00128
Zheng G., Qu H., Li F., Ma W., Yang H. (2018). Propofol Attenuates Sepsis-Induced Acute Kidney Injury by Regulating miR-290-5p/CCL-2 Signaling Pathway. Braz. J. Med. Biol. Res. 51 (11), e7655. doi:10.1590/1414-431X20187655
Zheng P., Chen L., Yuan X., Luo Q., Liu Y., Xie G., et al. (2017). Exosomal Transfer of Tumor-Associated Macrophage-Derived miR-21 Confers Cisplatin Resistance in Gastric Cancer Cells. J. Exp. Clin. Cancer Res. 36 (1), 53. doi:10.1186/s13046-017-0528-y
Zhong Y., Wu S., Yang Y., Li G. q., Meng L., Zheng Q. y., et al. (2020). LIGHT Aggravates Sepsis‐associated Acute Kidney Injury via TLR4‐MyD88‐NF‐κB Pathway. J. Cel. Mol. Med. 24 (20), 11936–11948. doi:10.1111/jcmm.15815
Keywords: sepsis, acute kidney injury, miRNA, lncRNA, circRNA
Citation: Chen Y, Jing H, Tang S, Liu P, Cheng Y, Fan Y, Chen H and Zhou J (2022) Non-Coding RNAs in Sepsis-Associated Acute Kidney Injury. Front. Physiol. 13:830924. doi: 10.3389/fphys.2022.830924
Received: 08 December 2021; Accepted: 08 March 2022;
Published: 08 April 2022.
Edited by:
Denis Drygin, Regulus Therapeutics Inc., United StatesCopyright © 2022 Chen, Jing, Tang, Liu, Cheng, Fan, Chen and Zhou. This is an open-access article distributed under the terms of the Creative Commons Attribution License (CC BY). The use, distribution or reproduction in other forums is permitted, provided the original author(s) and the copyright owner(s) are credited and that the original publication in this journal is cited, in accordance with accepted academic practice. No use, distribution or reproduction is permitted which does not comply with these terms.
*Correspondence: Jun Zhou, emhvdWp1bjc4NDNAMTI2LmNvbQ==