- 1Institute of Plant Protection, Beijing Academy of Agriculture and Forestry Sciences, Beijing, China
- 2Department of Applied Chemistry, Innovation Center of Pesticide Research, China Agricultural University, Beijing, China
- 3College of Plant Protection, Shandong Agricultural University, Taian, China
Harmonia axyridis is an important natural enemy that consumes many agricultural and forestry pests. It relies on a sensitive olfactory system to find prey and mates. Odorant-binding proteins (OBPs) as the first-step of recognizing volatiles, transport odors through sensillum lymph to odorant receptors (ORs). However, little is known about the molecular mechanisms of H. axyridis olfaction. In this study, four H. axyridis antenna specific OBP genes, HaxyOBP3, 5, 12, and 15, were bacterially expressed and the binding features of the four recombinant proteins to 40 substances were investigated using fluorescence competitive binding assays. Three-dimensional structure modeling and molecular docking analysis predicted the binding sites between HaxyOBPs and candidate volatiles. Developmental expression analyses showed that the four HaxyOBP genes displayed a variety of expression patterns at different development stages. The expression levels of HaxyOBP3 and HaxyOBP15 were higher in the adult stage than in the other developmental stages, and HaxyOBP15 was significantly transcriptionally enriched in adult stage. Ligand-binding analysis demonstrated that HaxyOBP3 and HaxyOBP12 only combined with two compounds, β-ionone and p-anisaldehyde. HaxyOBP5 protein displayed binding affinities with methyl salicylate, β-ionone, and p-anisaldehyde (Ki = 18.15, 11.71, and 13.45 μM). HaxyOBP15 protein had a broad binding profile with (E)-β-farnesene, β-ionone, α-ionone, geranyl acetate, nonyl aldehyde, dihydro-β-ionone, and linalyl acetate (Ki = 4.33–31.01 μM), and hydrophobic interactions played a key role in the binding of HaxyOBP15 to these substances according to molecular docking. Taken together, HaxyOBP15 exhibited a broader ligand-binding spectrum and a higher expression in adult stage than HaxyOBP3, 5, and 12, indicating HaxyOBP15 may play a greater role in binding volatiles than other three HaxyOBPs. The results will increase our understanding of the molecular mechanism of H. axyridis olfaction and may also result in new management strategies (attractants/repellents) that increase the biological control efficacy of H. axyridis.
Introduction
Insects rely on sensitive olfactory systems to perceive chemical signals from the environment, which are important in locating mates, detecting food sources, and finding suitable oviposition sites (Sato et al., 2008; Brito et al., 2016). The interaction between odorant-binding proteins (OBPs) and odorants is the first-step to recognize chemicals, transporting external odors through sensillum lymph to odorant receptors (ORs; Laughlin et al., 2008; Glaser et al., 2015; Elfekih et al., 2016; Pelosi et al., 2018). In Antheraea polyphemus, the first OBP was identified showing the function of sex pheromone binding (Vogt and Riddiford, 1981). Since then, many OBPs have been identified in species from different insect orders, including Lepidoptera (Zhu et al., 2013; Yang et al., 2017; Zhang et al., 2017b), Diptera (Zhao et al., 2018; Chen et al., 2019), Hemiptera (Wang et al., 2017; Sun et al., 2020), Neuroptera (Li et al., 2015), and Coleoptera (Bin et al., 2017; Liu et al., 2018).
It is helpful for identifying the function to study the OBPs expression patterns (Gong et al., 2014; Tang et al., 2019). OBPs have a variety of functions depending on their distribution (Sun et al., 2012; Xue et al., 2016; Li et al., 2019b). Antennae-specific OBPs play important roles in detecting sex pheromones and plant volatiles (Sun et al., 2014; Liu et al., 2020).
The Asian multicolored ladybird beetle, H. axyridis (Coleoptera: Coccinellidae), as an important natural enemy, can prey on many pests, including aphids, whiteflies, and thrips. Since the early 21 century, this species has been successfully used to control pests of crops (Koch, 2003; Pervez and Omkar, 2006; Wang et al., 2015). Harmonia axyridis is an effective biological control agent, but it can also be a pest in some situations (Soares et al., 2007; Koch and Costamagna, 2016; Ovchinnikov et al., 2019). It may compete with native predators for common food resources, and bring pollution to wine production (Pickering et al., 2004; Katsanis et al., 2013; Grez et al., 2016).
Predators used aphid alarm pheromones and pest-induced volatiles to locate pest (Al Abassi et al., 2000; Hatt et al., 2019), which is an important communication way of pest-crop-natural enemy interactions in agricultural fields. It is necessary for enhancing natural enemies’ biological control efficacy to understand their olfactory systems. Therefore, the interaction of H. axyridis with plant volatiles and aphid pheromones may be important for enhancing the effectiveness of H. axyridis as a biological control agent.
We previously identified 19 putative OBPs and characterized their tissue expression patterns by quantitative real-time PCR (qRT-PCR) based on antennae and whole-body transcriptomes of H. axyridis (Qu et al., 2021). HaxyOBP3 (NCBI accession number MT150141), HaxyOBP5 (NCBI accession number MT150143), HaxyOBP12 (NCBI accession number MT150150), and HaxyOBP15 (NCBI accession number MT150153), specifically expressed in adult antennae, may play a more important role in the olfactory perception of H. axyridis. In the present study, these four antennae-specific OBPs were selected for detailed study. The development stage expression profiles of these genes were generated, and their binding characteristics to ligands were also conducted. In addition, protein structures were modeled in three dimensions, and their potential binding sites were studied by molecular docking. The results increase our comprehending of the molecular basis of olfaction of H. axyridis and may help to enhance their biological control effectiveness.
Materials and Methods
Insect Samples
Harmonia axyridis was obtained from Beijing Kuoye Tianyuan Biological Technology Co., Ltd., rearing in a growth chamber of the Beijing Academy of Agriculture and Forestry Sciences with the temperature of 23 ± 1°C, 16:8 h (L:D) photoperiod and 70% relative humidity. The adults and larvae were fed with aphid Aphis craccivora Koch (Qu et al., 2018). To determine the transcript levels of HaxyOBP3, 5, 12, and 15 under various developmental stages (eggs, first, second, third, and fourth instar, pupae, and male and female adults), samples were collected and stored at −80°C. Three biological replicates were conducted.
Specific Expression of OBP Genes
The TRIzol reagent (Invitrogen, Carlsbad, CA, United States) was used to extract total RNA samples based on the manufacturer’s instructions. The first-strand cDNA was synthesized using the PrimeScript™ RT reagent Kit (TAKARA, Japan) following the provided protocol. The development stage expression pattern of HaxyOBPs was assessed by qRT-PCR. qRT-PCR was performed on ABI PRISM 7500 (Applied Biosystems, United States). The reaction consisted of 10 μl SYBR Premix Ex TaqTM II (TaKaRa, Japan), 1 μl of each primer (10 μmol L−1), 2 μl cDNA, 0.4 μl Rox Reference Dye II (Takara, Japan), and 5.6 μl nuclease free water. The reaction conditions were 95°C for 30 s, followed by 40 cycles of 95°C for 5 s and 60°C for 34 s. Primers of HaxyOBPs were based on Qu et al. (2021). EF1A and RPS13 genes were used as housekeeping genes (Qu et al., 2018). All samples were tested in three biological replicates. The 2-△△CT method was used for relative quantification (Schmittgen and Livak, 2008). The differences in the transcript levels of HaxyOBPs in different developmental stages were compared by One-way ANOVA (SPSS 19.0, Chicago, IL, United States), followed by Tukey’s test. Heat map illustrating the log2 transformation of HaxyOBPs mRNA expression levels in different developmental stages.
Expression and Purification of Recombinant OBPs
The DNA sequences that encode the HaxyOBP3, 5, 12, and 15 proteins were chemically synthesized and cloned into pET30a (+) by GenScript (Nanjing, China; Wang et al., 2020b). The positive plasmid was then transformed into BL21 (DE3) cells for the expression of recombinant proteins, and proteins induced with 0.5 mmol/L isopropyl β-D-1-thiogalactopyranoside (IPTG) for 4 h at 37°C (HaxyOBP3) or 16 h at 15°C (HaxyOBP5, 12, and 15). HaxyOBP5 was expressed in the supernatant. HaxyOBP3, 12, and 15 were mainly found in inclusion bodies. Inclusion bodies were denatured by 8 M urea. Recombinant proteins of HaxyOBP3, 12, and 15 were dissolved and refolded based on the reported methods (Zhang et al., 2012).
Protein purification was performed with His-Tag Purification Resin column (Genscript Biology Company, Nanjing, Jiangsu, China) and purified by gradient imidazole buffer (20, 50, 100, 250, and 500 mmol·L−1). The purity and size of proteins were detected by SDS-PAGE, and concentrations of proteins were measured with bicinchoninic acid (BCA) Protein Assay Kit (ThermoFisher Scientific-Life Technologies, Carlsbad, CA, United States).
Competitive Fluorescence Binding Assay
A Cary Eclipse Fluorescence Spectrophotometer (Agilent Technologies, United States) was used to determine the results of the binding assay. N-phenyl-1-naphthylamine (1-NPN) for HaxyOBP15 and 4,4′-Dianilino-1,1′-binaphthyl-5,5′-disulfonic acid dipotassium salt (bis-ANS) for HaxyOBP3, 5, and 12 were chosen as the fluorescent probe. The excitation wavelength was 337 nm of 1-NPN and 295 nm of bis-ANS, and the emission spectrum was recorded between 350 and 500 nm for 1-NPN and between 300 and 550 nm for bis-ANS. The recombinant proteins prepared in Tris–HCl (50 mM, pH 7.4) was titrated with aliquots of 1 mM 1-NPN or bis-ANS to final concentrations ranging from 2 to 16 μM to measure the binding affinity. To further measure the binding affinity of ligands to HaxyOBPs, proteins and fluorescent probe at 2 μM were titrated with aliquots of 1 mM odorants. The binding constant (K1-NPN/bis-ANS) of 1-NPN or bis-ANS to HaxyOBPs was calculated by GraphPad Prism 5 software (GraphPad Software Inc.) with the equation Ki = [IC50]/(1 + [1-NPN]/K1-NPN) or [IC50]/(1 + [bis-ANS]/Kbis-ANS), where [1-NPN]/[bis-ANS] is the free concentration of 1-NPN/bis-ANS, and K1-NPN/Kbis-ANS is the dissociation constant of the protein/1-NPN (bis-ANS).
Three-Dimensional Modeling and Molecular Docking
Three-dimensional structure of HaxyOBP12 and HaxyOBP15, more than 30% homology with the OBP templates in the Protein Database,1 was modeled by Program MODELLER (Martí-Renom et al., 2000; Fiser et al., 2010), while HaxyOBP3 and HaxyOBP5 that had less than 30% homology were also generated using a deep residual neural network trRosetta (https://yanglab.nankai.edu.cn/trRosetta; Yang et al., 2020). Three methods, including Verify_3D, Procheck, and ERRAT were used to assess the final 3D model of HaxyOBPs protein (Laskowski et al., 1996; Webb and Sali, 2016). AutoDock Vina (version 1.1.2) was selected to analyze the binding mode between HaxyOBPs protein and compounds with the default parameters (Morris et al., 2009). The top ranked binding mode was evaluated according to the Vina docking score, and visually analyzed by PyMOL (version 1.9.0; http://www.pymol.org/).
Results
Developmental Stage Expression of HaxyOBPs
qRT-PCR was used to determine the expression levels of HaxyOBP3, 5, 12, and 15 in different developmental stages (Figure 1). HaxyOBP3 and HaxyOBP15 were both highly expressed in adults, and HaxyOBP15 had a significantly higher expression level in this stage. Transcripts of HaxyOBP5 were especially abundant in the first instar. In addition, HaxyOBP12 showed similar relative transcript levels in all developmental stages.
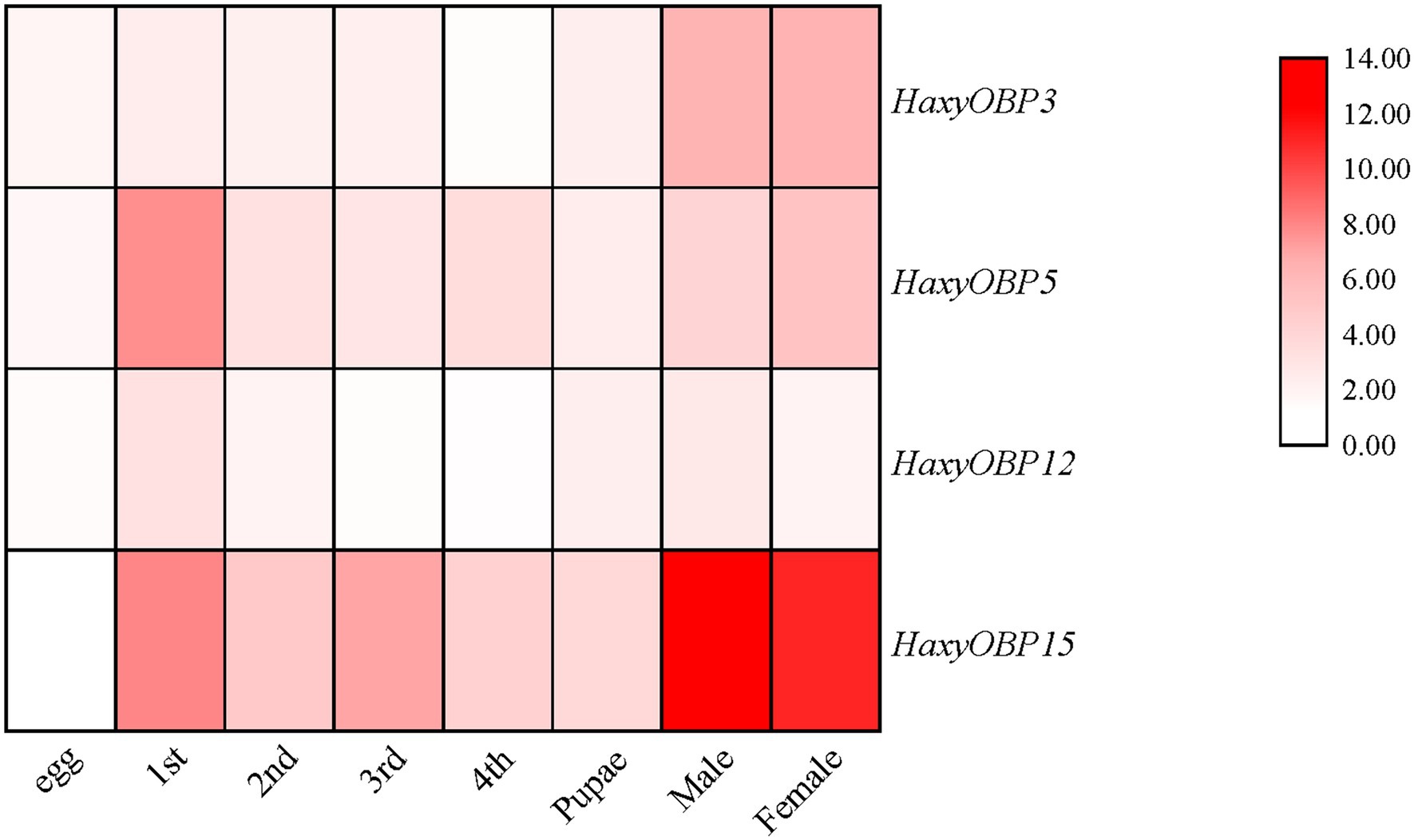
Figure 1. Relative expression levels of HaxyOBP3, 5, 12, and 15 genes in different developmental stages.
Expression and Purification of HaxyOBPs
The recombinant proteins of HaxyOBP3, 5, 12, and 15 were successfully expressed in the E. coli system induced by IPTG. HaxyOBP5 was mainly detected in the supernatant, while HaxyOBP3, 12, and 15 were present in inclusion bodies (Supplementary Figure S1). Therefore, 8 mol/L urea was used to extract the protein of HaxyOBP3, 12, and 15 before the purification. Renaturation, dialysis, and ultrafiltration were then used to obtain the purified target proteins of HaxyOBP3, 12, and 15. The OBPs were purified by nickel affinity chromatography. SDS-PAGE analysis revealed the final purified proteins as a single band, a molecular weight of about 15 kDa, consistent with the predicted molecular mass (Figure 2).
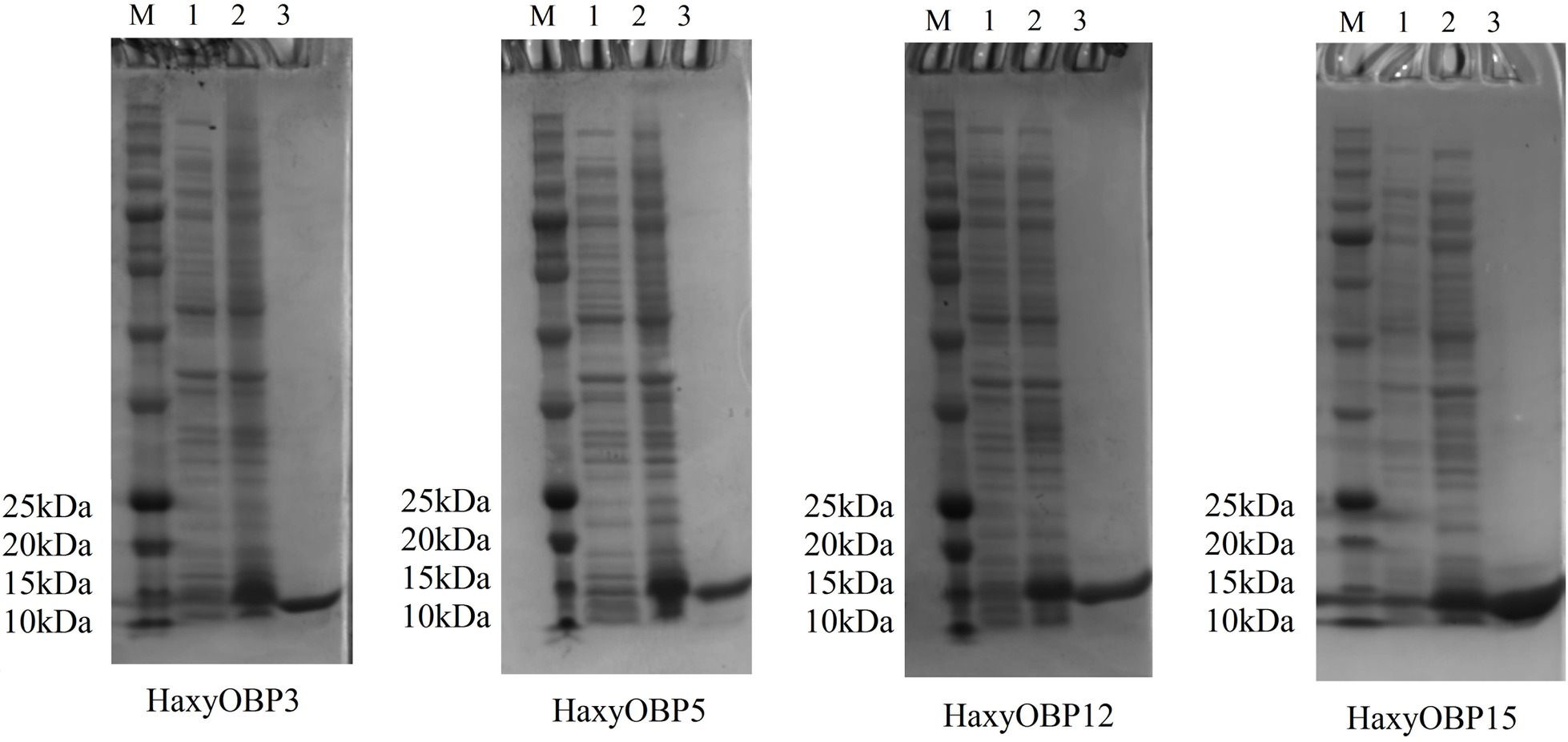
Figure 2. Expression and purification of HaxyOBP3, HaxyOBP5, HaxyOBP12, and HaxyOBP15. M: Marker; 1: Uninduced recombinant protein; 2: IPTG-induced recombinant protein; and 3: Purified proteins.
Binding Characteristics of HaxyOBPs
To determine the binding spectra of four HaxyOBPs recombinant proteins, fluorescence competitive binding assay was conducted. The binding characteristics of HaxyOBP3, 5, and 12 with fluorescent probe bis-ANS were detected by molecular fluorescence spectrometry, and the dissociation constants (Ki value) of HaxyOBP3, HaxyOBP5, and HaxyOBP12 were 3.07 ± 057, 2.23 ± 0.36, and 1.84 ± 0.10 μM, respectively. Using the same method, we detected the binding characteristics of HaxyOBP15 with 1-NPN, and the dissociation constant was 5.07 ± 0.31 μM (Figure 3).
Using 1-NPN or bis-ANS as a probe, 40 chemicals were used in competitive binding assay. HaxyOBP15 showed a broad binding profile with (E)-β-Farnesene, β-ionone, α-ionone, geranyl acetate, dihydro-β-ionone, nonyl aldehyde, and linalyl acetate, with the Ki values between 4.33 and 40.02 μM. HaxyOBP5 could bind methyl salicylate, β-ionone, and p-anisaldehyde, with the Ki values of 11.71, 13.45, and 18.15 μM, respectively. HaxyOBP3 and HaxyOBP12 showed narrow binding spectra and were able to only bind β-ionone and p-anisaldehyde, with the Ki values of 18.78 and 24.43 μM for HaxyOBP3 and 15.22 and 16.15 μM for HaxyOBP12, respectively (Figure 4; Table 1).
Homology Modeling and Molecular Docking
Sequence alignments showed that HaxyOBP12 and HaxyOBP15 share 44 and 31% amino acid identities with 6JPM and 4Z45, respectively. Sequence alignments showed that HaxyOBP3 and HaxyOBP5 share 29.27 and 28.93% amino acid identities, respectively, with the templates 1C3Y and 6QQ4, less than 30.00% (Table 2). The low identity may decrease the accuracy of the predicted model. So, we used the other method, trRosetta, to predict the model of HaxyOBP3 and HaxyOBP5. The trRosetta can predict the protein more accurately for the low identity sequence. The models predicted by Homology modeling were named Mod-HaxyOBP12 and Mod-HaxyOBP15. The models predicted by trRosetta were named trR-HaxyOBP3 and trR-HaxyOBP5.
For all of the predicted protein models, VERIFY3D, ERRAT, and Procheck were used to analyze the accuracy and reliability. The VERIFY3D (Supplementary Figure S2), ERRAT (Supplementary Figure S3), and Procheck (Supplementary Figure S4) showed that the models of Mod-HaxyOBP12, Mod-HaxyOBP15, trR-HaxyOBP3, and trR-HaxyOBP5 were reasonable.
The protein structures of HaxyOBP3, 5, 12, and 15 were composed of six typical α-helices, forming a hydrophobic binding cavity, which are the important features of insect OBPs (Figure 5).
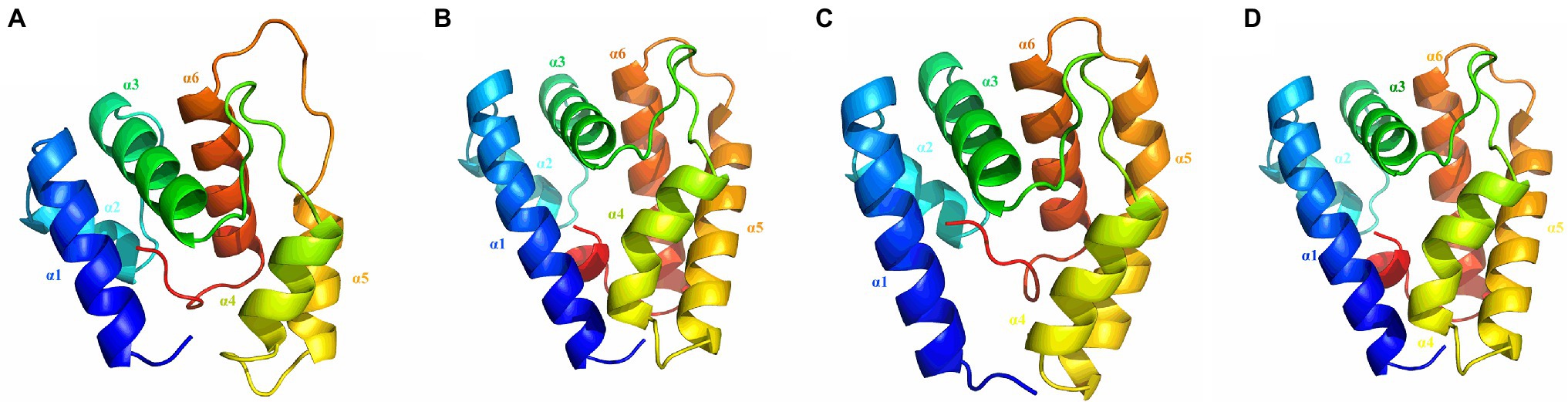
Figure 5. Three-dimensional structure mode of odorant binding proteins in Harmonia axyridis (A: HaxyOBP3; B: HaxyOBP5; C: HaxyOBP12; and D: HaxyOBP15).
According to the affinities between recombinant proteins and chemicals, we selected different numbers of ligands to study the docking conformation and binding energy with four HaxyOBPs proteins, including two ligands (β-ionone and p-anisaldehyde) for HaxyOBP3 and HaxyOBP12, three ligands (methyl salicylate, β-ionone, and p-anisaldehyde) for HaxyOBP5, and seven ligands [(E)-β-Farnesene, β-ionone, α-ionone, geranyl acetate, dihydro-β-ionone, nonyl aldehyde, and linalyl acetateone] for HaxyOBP15. The binding energy values were all negative and ranged from −5.13 to −7.31 kcal mol−1 (Table 3).
For HaxyOBP3, p-anisaldehyde bound the protein with Y46 and I115 and formed a “π-π” interaction with Y46. β-ionone bound the protein with F52, L68, V103, I113, and I115. The ligand formed hydrogen bond interactions with Y106. I115 is a common key residue for two ligands (Figure 6).
For HaxyOBP5, seven residues, including H73, I78, V85, A90, Y112, C115, and L131, were critical for binding affinity to β-ionone based on hydrophobic interactions. P-anisaldehyde formed a “π-π” interaction with Y112 of HaxyOBP5 and formed hydrophobic interactions with V85, A90, and L131. Methyl salicylate formed hydrogen bond interactions with L131 of HaxyOBP5 and hydrophobic interactions with V85, V87, A90, and Y112 (Figure 7).
Hydrophobic interactions were the important linkages between HaxyOBP12 and β-ionone and p-anisaldehyde. Three residues, including I91, A103, and A138, were critical for binding affinity to p-anisaldehyde. Four residues, including I91, L131, Y139, and L141, were critical for binding affinity to β-ionone (Figure 8).
For HaxyOBP15, hydrophobic interactions were the important linkages between HaxyOBP15 and β-ionone, dihydro-β-ionone, and α-ionone. Three residues, including H53, L58, and I130, appeared to be involved in the binding affinity to the three substances. (E)-β-Farnesene and HaxyOBP15 also have hydrophobic interactions, mediated by F9, L34, M48, I49, F52, H53, and L58. A hydrogen bonding interaction existed between HaxyOBP15 and geranyl acetate and linalyl acetate, with the key residue H53, and there were some hydrophobic residues involved in the interactions (Figure 9).
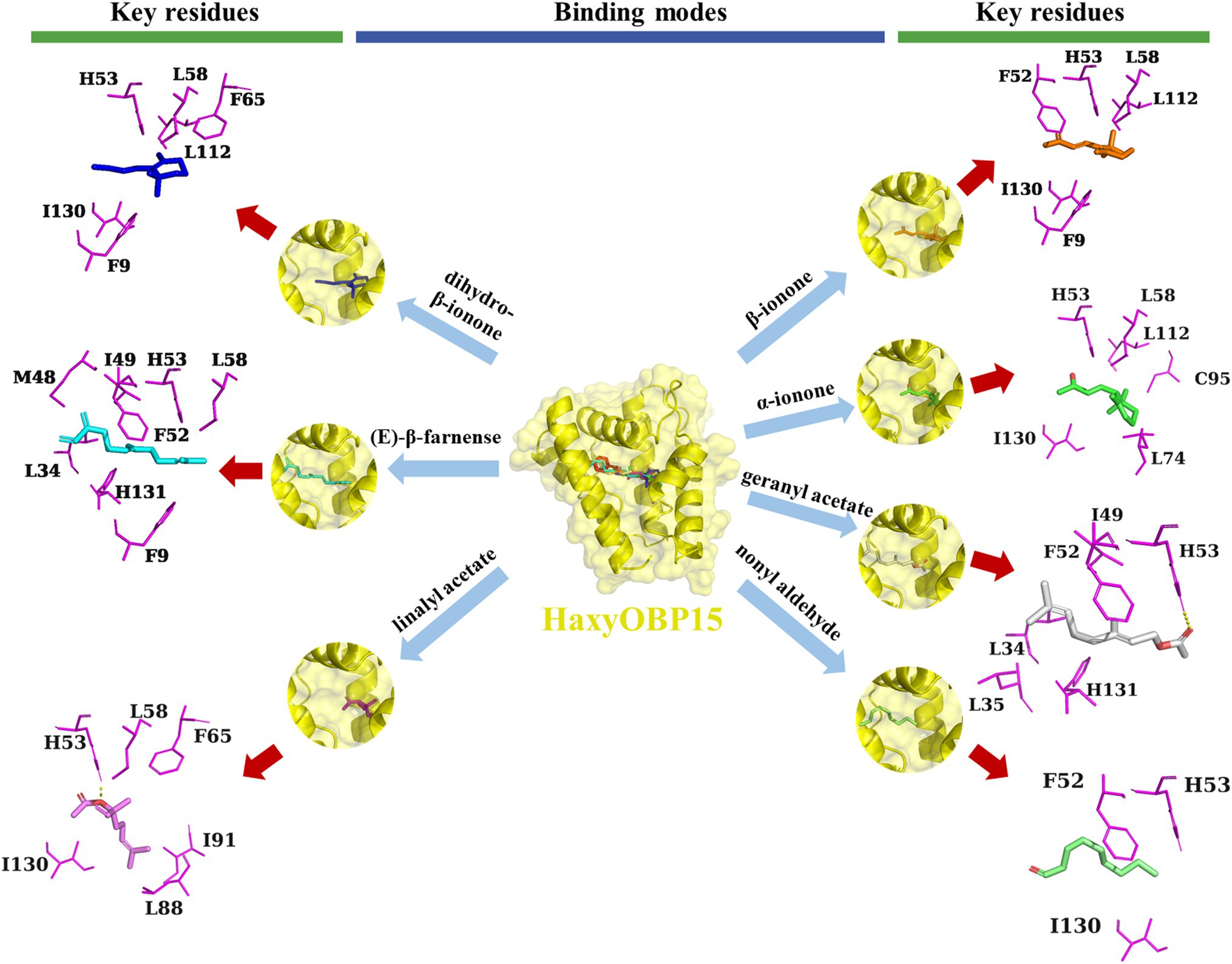
Figure 9. Binding pattern of HaxyOBP15 with β-ionone, dihydro-β-ionone, α-ionone, EBF, geranyl acetate, linalyl acetate, and nonyl aldehyde.
Discussion
Odorant-binding proteins are the front-line environmental odorant sensors, playing an essential role in insect behavior (Pelosi et al., 2006). Temporal and spatial expression patterns of OBPs in insects are interrelated with their specific physiological functions (Song et al., 2014; Sun et al., 2016; Zhang et al., 2017b). The transcripts of HaxyOBP3, 5, 12, and 15 were mainly restricted to adult antennae in our previous study (Qu et al., 2021), implying a role of these proteins in olfactory chemoreception. Clarifying the expression characteristics of insects’ OBPs at different developmental stages can also help to understand their functions in olfactory recognition (Ju et al., 2014). In this study, qRT-PCR indicated that HaxyOBP3, 5, 12, and 15 had different transcript levels during the different developmental stages of H. axyridis. HaxyOBP5 and HaxyOBP12 were abundant in the larval stage, indicating their connection to larval biological characteristics of H. axyridis. However, HaxyOBP3 and HaxyOBP15 were both highly expressed in adult stage, and the expression level of HaxyOBP15 was significantly higher in this stage, indicating they might be involved in adult-specific behaviors.
The results of the fluorescence binding assay also showed that HaxyOBP15 had a broader ligand-binding affinity, and it could bind seven substances including (E)-β-Farnesene, β-ionone, α-ionone, geranyl acetate, dihydro-β-ionone, nonyl aldehyde, and linalyl acetate, comparing with HaxyOBP3, 5, 12. These results were consistent with the fact that HaxyOBP15 gene showed significantly higher expression level in adult stage, indicating that HaxyOBP15 played a key role in olfactory communication of adult H. axyridis. Harmonia axyridis is an important natural enemy in many crops (Koch, 2003; Pervez and Omkar, 2006). Plant volatiles and sex pheromone are essential signal chemicals in pest-crop-natural enemy interactions (Li et al., 2018). Compared with larvae, adults of H. axyridis have a wider range of activities due to their ability to fly, and they need to recognize more odorants, so as to detect food sources or find suitable oviposition sites.
Homology modeling and molecular docking were used to further study the specific binding characteristics of OBPs. Classic OBPs usually has six α-helical domains, and fold together to form a compact pocket for combing odors (Pelosi et al., 2014). The present study showed that the predicted 3D structures of the four HaxyOBPs were consistent with those of classic OBPs, having six α-helical domains. Ligands are usually bound in a hydrophobic cavity of insect OBPs (Wogulis et al., 2006; Northey et al., 2016). In this study, molecular docking results showed that HaxyOBP15 broadly bound with more substances, suggesting that HaxyOBP15 may have adapted to binding to substances with different shapes and sizes. Some residues of HaxyOBP15 may specifically interact with functional groups of substances. For instance, HaxyOBP15 possess a key amino acid residue, H53, which appears to be involved in the recognition of a broad range of substances.
Among the 40 candidate compounds, nine compounds, including β-ionone, α-ionone, dihydro-β-ionone, geranyl acetate, nonanal, linalyl acetate, EBF, p-anisaldehyde, and methyl salicylate, bound to four HaxyOBPs according to fluorescence binding assay and molecular docking. β-ionone as a fragrance compound, existing in the flowers and fruits of many plants (Beekwilder et al., 2014; Fu et al., 2019; Guarino et al., 2021) and having a strong repellent effect on flea beetles, spider mites, and whiteflies (Caceres et al., 2016), could bind with the four HaxyOBPs proteins. Moreover, HaxyOBP15 could also bind with α-ionone and dihydro-β-ionone, the analogs of β-ionone. For HaxyOBP15, hydrophobic interactions played a key role in the binding of HaxyOBP15 to three substances based on molecular docking. Three substances have similar chemical structures, and also commonly exist in plant volatiles, playing an important role in interactions between plants and insects (Li et al., 2019a). For example, Dihydro-β-ionone is attractive to the crucifer flea beetle (Caceres et al., 2016). The bouquet of Philodendron adamantinum is mainly composed of dihydro-β-ionone, which can attract the beetle Erioscelis emarginata and promote the pollination process (Pereira et al., 2014). The α-ionone was widely used as a male attractant for Bractocera latifrons (Nishida et al., 2009).
HaxyOBP15 could also bind geranyl acetate, nonanal, and linalyl acetate, and H53 was the key residue between HaxyOBP15 and these three substances by molecular docking. Geranyl acetate is similar to (E)-ß-farnesene (EBF) in structure, but the polarity and hydrophilicity of two compounds are different. Geranyl acetate is an ester, and EBF is a hydrocarbon. Previous studies reported that geranyl acetate is a strong activator of OR5 of aphids and also has binding affinity to OBP3 and OBP7, demonstrating features shared by several other behaviorally active repellents (Zhang et al., 2017a). Linalyl acetate is a monoterpene ester, which can be isolated from essential oils of Chrysactinia mexicana, Lavandula angustifolia, and Thymus leptophyllus (Jan et al., 2016). In addition, the common volatile compound nonanal can attract female Grapholitha molesta in Y-tube experiment and is a critical volatile of tobacco for attracting female Helicoverpa assulta (Lu and Qiao, 2020; Wang et al., 2020a).
More importantly, fluorescence binding assay showed that HaxyOBP15 exhibited the strongest binding affinity with EBF. Molecular docking results also revealed that HaxyOBP15 and EBF displayed the strongest binding activity, having hydrophobic interactions mediated by F9, L34, M48, I49, F52, H53, and L58, with the lowest binding energy values. EBF, as main active component of aphid alarm pheromone (Bowers et al., 1977), can cause aphids to kick, stop feeding, and disperse from feeding site (Pickett et al., 1992), and mediates the winged morphs’s production (Kunert et al., 2005). Many aphid predators, such as hoverflies (Harmel et al., 2007), ground beetles (Kielty et al., 1996), and lady beetles (Nakamuta, 1991; Verheggen et al., 2007; Liu et al., 2014), utilize the aphid alarm pheromone EBF as a foraging cue. OBP3, 7, and 9 are associated with EBF perception in aphids (Qiao et al., 2009; Sun et al., 2011; Northey et al., 2016; Fan et al., 2017; Qin et al., 2020; Wang et al., 2021). The OBP1 of Chrysoperla sinica was able to bind EBF (Li et al., 2018), and the OBP10 of Chrysopa pallens mediated the perception of EBF (Li et al., 2017).
Although HaxyOBP3, 5, and 12 had a relatively narrow binding spectrum, comparing with HaxyOBP15, but they all bound to p-anisaldehyde. P-anisaldehyde is a naturally occurring fragrant phenolic compound that exists in anise, cumin, fennel, garlic, and other plant species (Boulogne et al., 2012). P-anisaldehyde is also a chemical communication substance of many insects (El-Sayed et al., 2008; Mainali and Lim, 2011; Thoming et al., 2020). For example, p-anisaldehyde can attract Frankliniella occidentalis and Thrips tabaci (Hollister et al., 1995; Koschier et al., 2000), and is an effective attractant for adults of Anthrenus verbasci (Imai et al., 2002). However, p-anisaldehyde has a repellent effect on some species, including Amblyomma americanum (Showler and Harlien, 2018) and Musca domestica (Showler and Harlien, 2019). In addition, HaxyOBP5 could bind with methyl salicylate (MeSA), a herbivore-induced plant volatile that is attractive to many predators such as ladybeetles (James, 2003a; Zhu and Park, 2005; Salamanca et al., 2017), lacewings (James, 2003b), hoverflies (Mallinger et al., 2011), mites (de Boer and Dicke, 2005), bugs (James, 2005), and aphid parasitoids (Gordon et al., 2013; Martini et al., 2014). In addition, MeSA has repellent effect on several aphid species (Ninkovic et al., 2003; Wang et al., 2019).
In summary, the expression levels of the four HaxyOBPs genes showed different in development stages, and HaxyOBP15 was significantly higher expressed in the adult of H. axyridis based on the results of qRT-PCR. Ligand binding assays and molecular docking demonstrated HaxyOBP15 exhibited high specificity for more substances, comparing with HaxyOBP3, 5, and 12, suggesting HaxyOBP15 may play the most prominent role in the olfactory chemoreception of H. axyridis. These results can provide insight into the mechanism of olfactory communication of H. axyridis and enhance the biological control effectiveness of H. axyridis.
Data Availability Statement
The original contributions presented in the study are included in the article/Supplementary Material, further inquiries can be directed to the corresponding authors.
Author Contributions
CQ and CL conceived and designed the experiments. CQ performed the experiments. CQ and F-qL analyzed the data. Z-kY and CQ provided the homology modeling and molecular docking and wrote the initial manuscript. CQ and H-pZ prepared the figures. CL, X-lY, and SW edited and reviewed the manuscript. All authors accepted the final version of the manuscript.
Funding
This work was supported by the China Agriculture Research System of MOF and MARA (CARS-24-C-03); the National Key Research and Development Program of China (2017YFD0200400); and the Shandong Province Modern Agricultural Technology System Peanut Innovation Team, China (SDAIT-04-08).
Conflict of Interest
The authors declare that the research was conducted in the absence of any commercial or financial relationships that could be construed as a potential conflict of interest.
Publisher’s Note
All claims expressed in this article are solely those of the authors and do not necessarily represent those of their affiliated organizations, or those of the publisher, the editors and the reviewers. Any product that may be evaluated in this article, or claim that may be made by its manufacturer, is not guaranteed or endorsed by the publisher.
Supplementary Material
The Supplementary Material for this article can be found online at: https://www.frontiersin.org/articles/10.3389/fphys.2022.829766/full#supplementary-material
Footnotes
References
Al Abassi, S., Birkett, M. A., Pettersson, J., Pickett, J. A., Wadhams, L. J., and Woodcock, C. M. (2000). Response of the seven-spot ladybird to an aphid alarm pheromone and an alarm pheromone inhibitor is mediated by paired olfactory cells. J. Chem. Ecol. 26, 1765–1771. doi: 10.1023/a:1005555300476
Beekwilder, J., van Rossum, H. M., Koopman, F., Sonntag, F., Buchhaupt, M., Schrader, J., et al. (2014). Polycistronic expression of a beta-carotene biosynthetic pathway in Saccharomyces cerevisiae coupled to beta-ionone production. J. Biotechnol. 192, 383–392. doi: 10.1016/j.jbiotec.2013.12.016
Bin, S. Y., Qu, M. Q., Li, K. M., Peng, Z. Q., Wu, Z. Z., and Lin, J. T. (2017). Antennal and abdominal transcriptomes reveal chemosensory gene families in the coconut hispine beetle, Brontispa longissima. Sci. Rep. 7:2809. doi: 10.1038/s41598-017-03263-1
Boulogne, I., Petit, P., Ozier-Lafontaine, H., Desfontaines, L., and Loranger-Merciris, G. (2012). Insecticidal and antifungal chemicals produced by plants: a review. Environ. Chem. Lett. 10, 325–347. doi: 10.1007/s10311-012-0359-1
Bowers, W. S., Nishino, C., Montgomery, M. E., and Nault, L. R. (1977). Structure-activity relationships of analogs of the aphid alarm pheromone, (E)-β-farnesene. J. Insect Physiol. 23, 697–701. doi: 10.1016/0022-1910(77)90086-5
Brito, N. F., Moreira, M. F., and Melo, A. C. A. (2016). A look inside odorant-binding proteins in insect chemoreception. J. Insect Physiol. 95, 51–65. doi: 10.1016/j.jinsphys.2016.09.008
Caceres, L. A., Lakshminarayan, S., Yeung, K. K. C., McGarvey, B. D., Hannoufa, A., Sumarah, M. W., et al. (2016). Repellent and attractive effects of alpha-, beta-, and Dihydro-beta- ionone to generalist and specialist herbivores. J. Chem. Ecol. 42, 107–117. doi: 10.1007/s10886-016-0669-z
Chen, J., Wang, F. L., Gui, L. Y., and Zhang, G. H. (2019). Identification and tissue distribution of odorant binding protein genes in the citrus fruit fly, Bactrocera minax (Enderlein) (Diptera: Tephritidae). J. Asia Pac. Entomol. 22, 256–262. doi: 10.1016/j.aspen.2019.01.011
de Boer, J. G., and Dicke, M. (2005). Information use by the predatory mite Phytoseiulus persimilis (Acari: Phytoseiidae), a specialised natural enemy of herbivorous spider mites. Appl. Entomol. Zool. 40, 1–12. doi: 10.1303/aez.2005.1
Elfekih, S., Chen, C. Y., Hsu, J. C., Belcaid, M., and Haymer, D. (2016). Identification and preliminary characterization of chemosensory perception-associated proteins in the melon fly Bactrocera cucurbitae using RNA-seq. Sci. Rep. 6:19112. doi: 10.1038/srep19112
El-Sayed, A. M., Byers, J. A., Manning, L. M., Juergens, A., Mitchell, V. J., and Suckling, D. M. (2008). Floral scent of Canada thistle and its potential as a generic insect attractant. J. Econ. Entomol. 101, 720–727. doi: 10.1603/0022-0493(2008)101[720:fsocta]2.0.co;2
Fan, J., Xue, W. X., Duan, H. X., Jiang, X., Zhang, Y., Yu, W. J., et al. (2017). Identification of an intraspecific alarm pheromone and two conserved odorant-binding proteins associated with (E)-beta-farnesene perception in aphid Rhopalosiphum padi. J. Insect Physiol. 101, 151–160. doi: 10.1016/j.jinsphys.2017.07.014
Fiser, A., Do, R. K. G., and Ali, A. (2010). Modeling of loops in protein structures. Protein Sci. 9, 1753–1773. doi: 10.1110/ps.9.9.1753
Fu, J. X., Hou, D., Wang, Y. G., Zhang, C., Bao, Z. Y., Zhao, H. B., et al. (2019). Identification of floral aromatic volatile compounds in 29 cultivars from four groups of Osmanthus fragrans by gas chromatography-mass spectrometry. Hortic. Environ. Biotechnol. 60, 611–623. doi: 10.1007/s13580-019-00153-5
Glaser, N., Gallot, A., Legeai, F., Harry, M., Kaiser, L., Le Ru, B., et al. (2015). Differential expression of the chemosensory transcriptome in two populations of the stemborer Sesamia nonagrioides. Inesct. Biochem. Molec. 65, 28–34. doi: 10.1016/j.ibmb.2015.07.008
Gong, Z. J., Miao, J., Duan, Y., Jiang, Y. L., Li, T., and Wu, Y. Q. (2014). Identification and expression profile analysis of putative odorant-binding proteins in Sitodiplosis mosellana (Gehin) (Diptera: Cecidomyiidae). Biochem. Biophys. Res. Commun. 444, 164–170. doi: 10.1016/j.bbrc.2014.01.036
Gordon, G. U. S. O., Wratten, S. D., Jonsson, M., Simpson, M., and Hale, R. (2013). Attract and reward: combining a herbivore-induced plant volatile with floral resource supplementation—multi-trophic level effects. Biol. Control 64, 106–115. doi: 10.1016/j.biocontrol.2012.10.003
Grez, A. A., Zaviezo, T., Roy, H. E., Brown, P. M. J., and Bizama, G. (2016). Rapid spread of Harmonia axyridis in Chile and its effects on local coccinellid biodiversity. Divers. Distrib. 22, 982–994. doi: 10.1111/ddi.12455
Guarino, S., Basile, S., Arif, M. A., Manachini, B., and Peri, E. (2021). Odorants of Capsicum spp. dried fruits as candidate attractants for Lasioderma serricorne F (Coleoptera: Anobiidae). Insects 12:9. doi: 10.3390/insects12010061
Harmel, N., Almohamad, R., Fauconnier, M.-L., Du Jardin, P., Verheggen, F., Marlier, M., et al. (2007). Role of terpenes from aphid-infested potato on searching and oviposition behavior of Episyrphus balteatus. Insect Sci. 14, 57–63. doi: 10.1111/j.1744-7917.2007.00126.x
Hatt, S., Xu, Q. X., Francis, F., and Osawa, N. (2019). Aromatic plants of East Asia to enhance natural enemies towards biological control of insect pests. A review. Entomol. Gen. 38, 275–315. doi: 10.1127/entomologia/2019/0625
Hollister, B., Ca Meron, E. A., and Teulon, D. (1995). Effect of p-Anisaldehyde and a yellow color on behavior and capture of Western flower Thrips. Thrips Biol. Manag. 276, 571–574. doi: 10.1007/978-1-4899-1409-5_85
Imai, T., Maekawa, M., and Tsuchiya, S. (2002). Attractiveness of p-anisaldehyde to the varied carpet beetle, Anthrenus verbasci (L.) (Coleoptera: Dermestidae). Appl. Entomol. Zool. 37, 505–508. doi: 10.1303/aez.2002.505
James, D. G. (2003a). Field evaluation of herbivore-induced plant volatiles as attractants for beneficial insects: methyl salicylate and the green lacewing, Chrysopa nigricornis. J. Chem. Ecol. 29, 1601–1609. doi: 10.1023/a:1024270713493
James, D. G. (2003b). Synthetic herbivore-induced plant volatiles as field attractants for beneficial insects. Environ. Entomol. 32, 977–982. doi: 10.1603/0046-225x-32.5.977
James, D. G. (2005). Further field evaluation of synthetic herbivore-induced plant volatiles as attractants for beneficial insects. J. Chem. Ecol. 31, 481–495. doi: 10.1007/s10886-005-2020-y
Jan, S., Kamili, A. N., Parray, J. A., and Bedi, Y. S. (2016). Differential response of terpenes and anthraquinones derivatives in Rumex dentatus and Lavandula officinalis to harsh winters across north-western Himalaya. Nat. Prod. Res. 30, 608–612. doi: 10.1080/14786419.2015.1030404
Ju, Q., Li, X., Jiang, X. J., Qu, M. J., Guo, X. Q., Han, Z. J., et al. (2014). Transcriptome and tissue-specific expression analysis of OBP and CSP genes in the dark black chafer. Arch. Insect. Biochem. 87, 177–200. doi: 10.1002/arch.21188
Katsanis, A., Babendreier, D., Nentwig, W., and Kenis, M. (2013). Intraguild predation between the invasive ladybird Harmonia axyridis and non-target European coccinellid species. BioControl 58, 73–83. doi: 10.1007/s10526-012-9470-2
Kielty, J. P., Allen-Williams, L. J., Underwood, N., and Eastwood, E. A. (1996). Behavioral responses of three species of ground beetle (Coleoptera: Carabidae) to olfactory cues associated with prey and habitat. J. Insect Behav. 9, 237–250. doi: 10.1007/BF02213868
Koch, R. L. (2003). The multicolored Asian lady beetle, Harmonia axyridis: a review of its biology, uses in biological control, and non-target impacts. J. Insect Sci. 3:32. doi: 10.1093/jis/3.1.32
Koch, R. L., and Costamagna, A. C. (2016). Reaping benefits from an invasive species: role of Harmonia axyridis in natural biological control of Aphis glycines in North America. BioControl 62, 331–340. doi: 10.1007/s10526-016-9749-9
Koschier, E. H., Kogel, W., and Visser, J. H. (2000). Assessing the attractiveness of volatile plant compounds to Western flower thrips Frankliniella occidentalis. J. Chem. Ecol. 26, 2643–2655. doi: 10.1023/A:1026470122171
Kunert, G., Otto, S., Rose, U. S. R., Gershenzon, J., and Weisser, W. W. (2005). Alarm pheromone mediates production of winged dispersal morphs in aphids. Ecol. Lett. 8, 596–603. doi: 10.1111/j.1461-0248.2005.00754.x
Laskowski, R. A., Rullmann, J., Macarthur, M. W., Kaptein, R., and Thornton, J. M. (1996). AQUA and PROCHECK-NMR: programs for checking the quality of protein structures solved by NMR. J. Biomol. NMR 8, 477–486. doi: 10.1007/BF00228148
Laughlin, J. D., Ha, T. S., Jones, D. N. M., and Smith, D. P. (2008). Activation of pheromone-sensitive neurons is mediated by conformational activation of pheromone-binding protein. Cell 133, 1255–1265. doi: 10.1016/j.cell.2008.04.046
Li, F., Li, D., Dewer, Y., Qu, C., Yang, Z., Tian, J. H., et al. (2019a). Discrimination of Oviposition deterrent volatile β-ionone by odorant-binding proteins 1 and 4 in the whitefly Bemisia tabaci. Biomol. Ther. 9:563. doi: 10.3390/biom9100563
Li, Z. B., Wei, Y., Sun, L., An, X. K., Dhiloo, K. H., Wang, Q., et al. (2019b). Mouthparts enriched odorant binding protein AfasOBP11 plays a role in the gustatory perception of Adelphocoris fasciaticollis. J. Insect Physiol. 117:103915. doi: 10.1016/j.jinsphys.2019.103915
Li, Z. Q., Zhang, S., Cai, X. M., Luo, J. Y., Dong, S. L., Cui, J. J., et al. (2017). Three odorant binding proteins may regulate the behavioural response of Chrysopa pallens to plant volatiles and the aphid alarm pheromone (E)-beta-farnesene. Insect Mol. Biol. 26, 255–265. doi: 10.1111/imb.12295
Li, Z. Q., Zhang, S., Cai, X. M., Luo, J. Y., Dong, S. L., Cui, J. J., et al. (2018). Distinct binding affinities of odorant-binding proteins from the natural predator Chrysoperla sinica suggest different strategies-to-hunt-prey. J. Insect Physiol. 111, 25–31. doi: 10.1016/j.jinsphys.2018.10.004
Li, Z. Q., Zhang, S., Luo, J. Y., Wang, S. B., Wang, C. Y., Lv, L. M., et al. (2015). Identification and expression pattern of candidate olfactory genes in Chrysoperla sinica by antennal transcriptome analysis. Comp. Biochem. Physiol. Part D Genom. Proteom. 15, 28–38. doi: 10.1016/j.cbd.2015.05.002
Liu, Y., Chi, B., Bo, Y., Zhu, Y., and Yong, L. (2014). Effects of E-β-farnesene release on the spatial distribution patterns of cabbage aphids and lady beetles. Acta Phytophy. Sin. 41, 754–760.
Liu, N. Y., Li, Z. B., Zhao, N., Song, Q. S., Zhu, J. Y., and Yang, B. (2018). Identification and characterization of chemosensory gene families in the bark beetle, Tomicus yunnanensis. Comp. Biochem. Physiol. Part D Genom. Proteom. 25, 73–85. doi: 10.1016/j.cbd.2017.11.003
Liu, Z., Liang, X. F., Xu, L., Keesey, I. W., Lei, Z. R., Smagghe, G., et al. (2020). An antennae-specific odorant-binding protein is involved in Bactrocera dorsalis olfaction. Front. Ecol. Evol. 8:63. doi: 10.3389/fevo.2020.00063
Lu, P. F., and Qiao, H. L. (2020). Peach volatile emission and attractiveness of different host plant volatiles blends to Cydia molesta in adjacent peach and pear orchards. Sci. Rep. 10:13658. doi: 10.1038/s41598-020-70685-9
Mainali, B. P., and Lim, U. T. (2011). Behavioral response of Western flower thrips to visual and olfactory cues. J. Insect Behav. 24, 436–446. doi: 10.1007/s10905-011-9267-7
Mallinger, R. E., Hogg, D. B., and Gratton, C. (2011). Methyl salicylate attracts natural enemies and reduces populations of soybean aphids (Hemiptera: Aphididae) in soybean Agroecosystems. J. Econ. Entomol. 104, 115–124. doi: 10.1603/ec10253
Martini, X., Pelz-Stelinski, K. S., and Stelinski, L. L. (2014). Plant pathogen-induced volatiles attract parasitoids to increase parasitism of an insect vector. Front. Ecol. Evol. 2:8. doi: 10.3389/fevo.2014.00008
Martí-Renom, M. A., Stuart, A. C., Fiser, A., Sánchez, R., and Sali, A. (2000). Comparative protein structure modeling of genes and genomes. Annu. Rev. Biophys. 29, 291–325. doi: 10.1146/annurev.biophys.29.1.291
Morris, G. M., Huey, R., Lindstrom, W., Sanner, M. F., Belew, R. K., Goodsell, D. S., et al. (2009). AutoDock4 and AutoDockTools4: automated docking with selective receptor flexibility. J. Comput. Chem. 30, 2785–2791. doi: 10.1002/jcc.21256
Nakamuta, K. (1991). Aphid alarm pheromone component, (E)-β-farnesene, and local search by a predatory lady beetle, Coccinella septempunctata bruckii MULSANT(Coleoptera:Coccinellidae). Appl. Entomol. Zool. 26, 1–7. doi: 10.1303/aez.26.1
Ninkovic, V., Ahmed, E., Glinwood, R., and Pettersson, J. (2003). Effects of two types of semiochemical on population development of the bird cherry oat aphid Rhopalosiphum padi in a barley crop. Agric. Forset. Entomol. 5, 27–34. doi: 10.1046/j.1461-9563.2003.00159.x
Nishida, R., Enomoto, H., Shelly, T. E., and Ishida, T. (2009). Sequestration of 3-oxygenated alpha-ionone derivatives in the male rectal gland of the solanaceous fruit fly, Bactrocera latifrons. Entomol. Exp. Appl. 131, 85–92. doi: 10.1111/j.1570-7458.2009.00835.x
Northey, T., Venthur, H., De Biasio, F., Chauviac, F.-X., Cole, A., Lisboa Ribeiro Junior, K. A., et al. (2016). Crystal structures and binding dynamics of odorant-binding protein 3 from two aphid species Megoura viciae and Nasonovia ribisnigri. Sci. Rep. 6:24739. doi: 10.1038/srep24739
Ovchinnikov, A. N., Belyakova, N. A., Ovchinnikova, A. A., and Reznik, S. Y. (2019). Factors determining larval cannibalistic behavior in invasive and native populations of the multicolored Asian ladybird, Harmonia axyridis. Entomol. Gen. 38, 243–254. doi: 10.1127/entomologia/2019/0702
Pelosi, P., Iovinella, I., Felicioli, A., and Dani, F. R. (2014). Soluble proteins of chemical communication: an overview across arthropods. Front. Physiol. 5:320. doi: 10.3389/fphys.2014.00320
Pelosi, P., Iovinella, I., Zhu, J., Wang, G., and Dani, F. R. (2018). Beyond chemoreception: diverse tasks of soluble olfactory proteins in insects. Biol. Rev. 93, 184–200. doi: 10.1111/brv.12339
Pelosi, P., Zhou, J. J., Ban, L. P., and Calvello, M. (2006). Soluble proteins in insect chemical communication. Cell. Mol. Life Sci. 63, 1658–1676. doi: 10.1007/s00018-005-5607-0
Pereira, J., Schlindwein, C., Antonini, Y., Dalia Maia, A. C., Doetterl, S., Martins, C., et al. (2014). Philodendron adamantinum (Araceae) lures its single cyclocephaline scarab pollinator with specific dominant floral scent volatiles. Biol. J. Linn. Soc. 111, 679–691. doi: 10.1111/bij.12232
Pervez, A., and Omkar, (2006). Ecology and biological control application of multicoloured Asian ladybird, Harmonia axyridis: a review. Biocontrol Sci. Tech. 16, 111–128. doi: 10.1080/09583150500335350
Pickering, G., Lin, J., Riesen, R., Reynolds, A., Brindle, I., and Soleas, G. (2004). Influence of Harmonia axyridis on the sensory properties of white and red wine. Am. J. Enol. Vitic. 55, 153–159.
Pickett, J. A., Wadhams, L. J., and Woodcock, C. M. (1992). The chemical ecology of aphids. Annu. Rev. Entomol. 37, 67–90. doi: 10.1146/annurev.en.37.010192.000435
Qiao, H. L., Tuccori, E., He, X. L., Gazzano, A., Field, L., Zhou, J. J., et al. (2009). Discrimination of alarm pheromone (E)-beta-farnesene by aphid odorant-binding proteins. Insect. Biochem. Molec. 39, 414–419. doi: 10.1016/j.ibmb.2009.03.004
Qin, Y. G., Yang, Z. K., Song, D. L., Wang, Q., Gu, S. H., Li, W. H., et al. (2020). Bioactivities of synthetic salicylate-substituted carboxyl (E)-β-Farnesene derivatives as ecofriendly agrochemicals and their binding mechanism with potential targets in aphid olfactory system. Pest Manag. Sci. 76, 2465–2472. doi: 10.1002/ps.5787
Qu, C., Wang, R., Che, W. N., Li, F. Q., Zhao, H. P., Wei, Y. Y., et al. (2021). Identification and tissue distribution of odorant binding protein genes in Harmonia axyridis (Coleoptera: Coccinellidae). J. Integr. Agric. 20, 2204–2213. doi: 10.1016/s2095-3119(20)63297-x
Qu, C., Wang, R., Che, W. N., Zhu, X. Q., Li, F. Q., and Luo, C. (2018). Selection and evaluation of reference genes for expression analysis using quantitative real-time PCR in the Asian ladybird Harmonia axyridis (Coleoptera: Coccinellidae). PLoS One 13:e0192521. doi: 10.1371/journal.pone.0192521
Salamanca, J., Souza, B., Lundgren, J. G., and Rodriguez-Saona, C. (2017). From laboratory to field: electro-antennographic and behavioral responsiveness of two insect predators to methyl salicylate. Chemoecology 27, 51–63. doi: 10.1007/s00049-017-0230-8
Sato, K., Pellegrino, M., Nakagawa, T., Nakagawa, T., Vosshall, L. B., and Touhara, K. (2008). Insect olfactory receptors are heteromeric liagnd-gated ion chanels. Nature 452, 1002–1006. doi: 10.1038/nature06850
Schmittgen, T. D., and Livak, K. J. (2008). Analyzing real-time PCR data by the comparative C-T method. Nat. Protoc. 3, 1101–1108. doi: 10.1038/nprot.2008.73
Showler, A. T., and Harlien, J. L. (2018). Botanical compound p-Anisaldehyde repels larval lone star tick (Acari: Ixodidae), and halts reproduction by gravid adults. J. Med. Entomol. 55, 200–209. doi: 10.1093/jme/tjx158
Showler, A. T., and Harlien, J. L. (2019). Lethal and repellent effects of the botanical p-Anisaldehyde on Musca domestica (Diptera: Muscidae). J. Econ. Entomol. 112, 485–493. doi: 10.1093/jee/toy351
Soares, A. O., Borges, I., Borges, P. A. V., Labrie, G., and Lucas, É. (2007). Harmonia axyridis: what will stop the invader? BioControl 53, 127–145. doi: 10.1007/s10526-007-9141-x
Song, Y. Q., Dong, J. F., Qiao, H. L., and Wu, J. X. (2014). Molecular characterization, expression patterns and binding properties of two pheromone-binding proteins from the oriental fruit moth, Grapholita molesta (Busck). J. Integr. Agric. 13, 2709–2720. doi: 10.1016/s2095-3119(13)60686-3
Sun, Y. L., Huang, L. Q., Pelosi, P., and Wang, C. Z. (2012). Expression in antennae and reproductive organs suggests a dual role of an odorant-binding protein in two sibling Helicoverpa species. PLoS One 7:e30040. doi: 10.1371/journal.pone.0030040
Sun, D. D., Huang, Y., Qin, Z. J., Zhan, H. X., Zhang, J. P., Liu, Y., et al. (2020). Identification of candidate olfactory genes in the antennal transcriptome of the stink bug Halyomorpha halys. Front. Physiol. 11:876. doi: 10.3389/fphys.2020.00876
Sun, Y. F., Qiao, H. L., Ling, Y., Yang, S. X., Rui, C. H., Pelosi, P., et al. (2011). New analogues of (E)-beta-Farnesene with insecticidal activity and binding affinity to aphid odorant-binding proteins. J. Agric. Food Chem. 59, 2456–2461. doi: 10.1021/jf104712c
Sun, L., Wei, Y., Zhang, D. D., Ma, X. Y., Xiao, Y., Zhang, Y. N., et al. (2016). The mouthparts enriched odorant binding protein 11 of the alfalfa plant bug Adelphocoris lineolatus displays a preferential binding behavior to host plant secondary metabolites. Front. Physiol. 7:201. doi: 10.3389/fphys.2016.00201
Sun, L., Xiao, H. J., Gu, S. H., Zhou, J. J., Guo, Y. Y., Liu, Z. W., et al. (2014). The antenna-specific odorant-binding protein AlinOBP13 of the alfalfa plant bug Adelphocoris lineolatusis expressed specifically in basiconic sensilla and has high binding affinity to terpenoids. Insect Mol. Biol. 23, 417–434. doi: 10.1111/imb.12089
Tang, Q. F., Shen, C., Zhang, Y., Yang, Z. P., Han, R. R., and Wang, J. (2019). Antennal transcriptome analysis of the maize weevil Sitophilus zeamais: identification and tissue expression profiling of candidate odorant-binding protein genes. Arch. Insect. Biochem. 101:e21542. doi: 10.1002/arch.21542
Thoming, G., Koczor, S., Szentkiralyi, F., Norli, H. R., Tasin, M., and Knudsen, G. K. (2020). Attraction of Chrysotropia ciliata(Neuroptera, Chrysopidae) males to P-Anisaldehyde, a compound with presumed pheromone function. J. Chem. Ecol. 46, 597–609. doi: 10.1007/s10886-020-01191-5
Verheggen, F. J., Fagel, Q., Heuskin, S., Lognay, G., Francis, F., and Haubruge, E. (2007). Electrophysiological and behavioral responses of the multicolored Asian lady beetle, Harmonia axyridis Pallas, to Sesquiterpene Semiochemicals. J. Chem. Ecol. 33, 2148–2155. doi: 10.1007/s10886-007-9370-6
Vogt, R. G., and Riddiford, L. M. (1981). Pheromone binding and inactivation by moth antennae. Nature 293, 161–163. doi: 10.1038/293161a0
Wang, C., Li, G. N., Miao, C. J., Zhao, M., Wang, B., and Guo, X. R. (2020a). Nonanal modulates oviposition preference in female Helicoverpa assulta (Lepidoptera: Noctuidae) via the activation of peripheral neurons. Pest Manag. Sci. 76, 3159–3167. doi: 10.1002/ps.5870
Wang, R., Li, F. Q., Zhang, W., Zhang, X. M., Qu, C., Tetreau, G., et al. (2017). Identification and expression profile analysis of odorant binding protein and chemosensory protein genes in Bemisia tabaci MED by head transcriptome. PLoS One 12:e0171739. doi: 10.1371/journal.pone.0171739
Wang, K., Liu, J. H., Zhan, Y. D., and Liu, Y. (2019). A new slow-release formulation of methyl salicylate optimizes the alternative control of Sitobion avenae (Fabricius; Hemiptera: Aphididae) in wheat fields. Pest Manag. Sci. 75, 676–682. doi: 10.1002/ps.5164
Wang, Q., Liu, J. T., Zhang, Y. J., Chen, J. L., Li, X. C., Liang, P., et al. (2021). Coordinative mediation of the response to alarm pheromones by three odorant binding proteins in the green peach aphid Myzus persicae. Insect Biochem. Mol. Biol. 130:103528. doi: 10.1016/j.ibmb.2021.103528
Wang, S. N., Shan, S., Yu, G. Y., Wang, H., Dhiloo, K. H., Khashaveh, A., et al. (2020b). Identification of odorant-binding proteins and functional analysis of antenna-specific AplaOBP1 in the emerald ash borer, Agrilus planipennis. J. Pest. Sci. 93, 853–865. doi: 10.1007/s10340-019-01188-4
Wang, S., Tan, X. L., Michaud, J. P., Shi, Z. K., and Zhang, F. (2015). Sexual selection drives the evolution of limb regeneration in Harmonia axyridis (Coleoptera: Coccinellidae). Bull. Entomol. Res. 105, 245–252. doi: 10.1017/s0007485315000036
Webb, B., and Sali, A. (2016). Comparative protein structure modeling using MODELLER. Curr. Protoc. Bioinformatics 47, 1–32. doi: 10.1002/0471250953.bi0506s47
Wogulis, M., Morgan, T., Ishida, Y., Leal, W. S., and Wilson, D. K. (2006). The crystal structure of an odorant binding protein from Anopheles gambiae: evidence for a common ligand release mechanism. Biochem. Biophys. Res. Commun. 339, 157–164. doi: 10.1016/j.bbrc.2005.10.191
Xue, W. X., Fan, J., Zhang, Y., Xu, Q. X., Han, Z. L., Sun, J. R., et al. (2016). Identification and expression analysis of candidate odorant-binding protein and chemosensory protein genes by antennal transcriptome of Sitobion avenae. PLoS One 11:e0161839. doi: 10.1371/journal.pone.0161839
Yang, J., Anishchenko, I., Park, H., Peng, Z., Ovchinnikov, S., and Baker, D. (2020). Improved protein structure prediction using predicted interresidue orientations. Proc. Natl. Acad. Sci. U.S.A. 117, 1496–1503. doi: 10.1073/pnas.1914677117
Yang, S. Y., Cao, D. P., Wang, G. R., and Liu, Y. (2017). Identification of genes involved in chemoreception in Plutella xyllostella by antennal transcriptome analysis. Sci. Rep. 7:11941. doi: 10.1038/s41598-017-11646-7
Zhang, T. T., Mei, X. D., Feng, J. N., Berg, B. G., Zhang, Y. J., and Guo, Y. Y. (2012). Characterization of three pheromone-binding proteins (PBPs) of Helicoverpa armigera (Hubner) and their binding properties. J. Insect Physiol. 58, 941–948. doi: 10.1016/j.jinsphys.2012.04.010
Zhang, R. B., Wang, B., Grossi, G., Falabella, P., Liu, Y., Yan, S. C., et al. (2017a). Molecular basis of alarm pheromone detection in aphids. Curr. Biol. 27, 55–61. doi: 10.1016/j.cub.2016.10.013
Zhang, Y. N., Zhu, X. Y., Ma, J. F., Dong, Z. P., Xu, J. W., Kang, K., et al. (2017b). Molecular identification and expression patterns of odorant binding protein and chemosensory protein genes in Athetis lepigone (Lepidoptera: Noctuidae). Peer J 5:e3157. doi: 10.7717/peerj.3157
Zhao, Y. H., Ding, J. F., Zhang, Z. Q., Liu, F., Zhou, C. G., and Mu, W. (2018). Sex- and tissue-specific expression profiles of odorant binding protein and chemosensory protein genes in Bradysia odoriphaga (Diptera: Sciaridae). Front. Physiol. 9:107. doi: 10.3389/fphys.2018.00107
Zhu, J. W., and Park, K. C. (2005). Methyl salicylate, a soybean aphid-induced plant volatile attractive to the predator Coccinella septempunctata. J. Chem. Ecol. 31, 1733–1746. doi: 10.1007/s10886-005-5923-8
Keywords: Harmonia axyridis, odorant-binding proteins, fluorescence competitive binding assays, molecular docking, volatile compounds
Citation: Qu C, Yang Z-k, Wang S, Zhao H-p, Li F-q, Yang X-l and Luo C (2022) Binding Affinity Characterization of Four Antennae-Enriched Odorant-Binding Proteins From Harmonia axyridis (Coleoptera: Coccinellidae). Front. Physiol. 13:829766. doi: 10.3389/fphys.2022.829766
Edited by:
Qingjun Wu, Insititute of Vegetables and Flowers (CAAS), ChinaReviewed by:
Na Yu, Nanjing Agricultural University, ChinaTiantao Zhang, State Key Laboratory for Biology of Plant Diseases and Insect Pests, Institute of Plant Protection (CAAS), China
Copyright © 2022 Qu, Yang, Wang, Zhao, Li, Yang and Luo. This is an open-access article distributed under the terms of the Creative Commons Attribution License (CC BY). The use, distribution or reproduction in other forums is permitted, provided the original author(s) and the copyright owner(s) are credited and that the original publication in this journal is cited, in accordance with accepted academic practice. No use, distribution or reproduction is permitted which does not comply with these terms.
*Correspondence: Chen Luo, bHVvY2hlbjEwMTBAMTI2LmNvbQ==; Xin-ling Yang, eWFuZ3hsQGNhdS5lZHUuY24=
†These authors have contributed equally to this work