- National Brain Research Centre, Manesar, India
The opioid system in the brain is responsible for processing affective states such as pain, pleasure, and reward. It consists of three main receptors, mu- (μ-ORs), delta- (δ-ORs), and kappa- (κ-ORs), and their ligands – the endogenous opioid peptides. Despite their involvement in the reward pathway, and a signaling mechanism operating in synergy with the dopaminergic system, fewer reports focus on the role of these receptors in higher cognitive processes. Whereas research on opioids is predominated by studies on their addictive properties and role in pain pathways, recent studies suggest that these receptors may be involved in learning. Rodents deficient in δ-ORs were poor at recognizing the location of novel objects in their surroundings. Furthermore, in chicken, learning to avoid beads coated with a bitter chemical from those without the coating was modulated by δ-ORs. Similarly, μ-ORs facilitate long term potentiation in hippocampal CA3 neurons in mammals, thereby having a positive impact on spatial learning. Whereas these studies have explored the role of opioid receptors on learning using reward/punishment-based paradigms, the role of these receptors in natural learning processes, such as vocal learning, are yet unexplored. In this review, we explore studies that have established the expression pattern of these receptors in different brain regions of birds, with an emphasis on songbirds which are model systems for vocal learning. We also review the role of opioid receptors in modulating the cognitive processes associated with vocalizations in birds. Finally, we discuss the role of these receptors in regulating the motivation to vocalize, and a possible role in modulating vocal learning.
Introduction
Acoustic communication is important for the survival of animals living in large social groups. Many species of animals utilize innate vocalizations to relay information to others. The context for such innate vocalizations may be acquired from the environment (DeVries et al., 2015; Wegdell et al., 2019) but is not affected by changes in auditory input during early development (Cheney et al., 1992; Hammerschmidt et al., 2012). In contrast, some animals have elaborate vocal repertoires which are learnt during the course of development and even in adulthood (Figure 1; Harcus, 1977; Eens, 1997; Hardy and Parker, 1997; Dowsett-Lemaire, 2008; Gammon and Altizer, 2011; Balsby et al., 2012; Janik, 2014; Mello, 2014; Reichmuth and Casey, 2014; Stoeger and Manger, 2014; Favaro et al., 2016; Mori et al., 2018; Johnson and Clark, 2020; Vernes and Wilkinson, 2020; Dalziell et al., 2021). Neuroethologists study these vocal learners to understand the intricacies and origins of human speech acquisition.
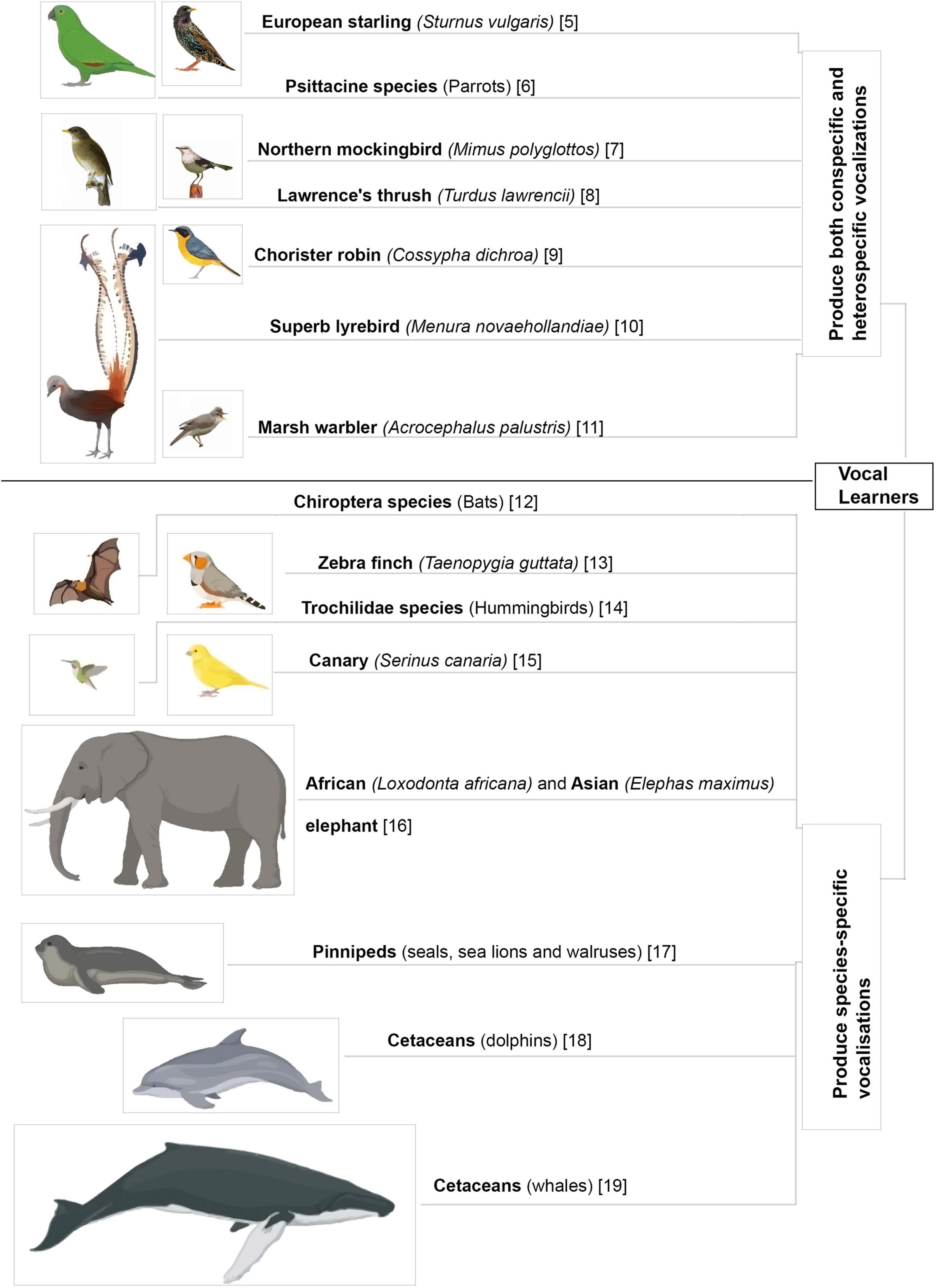
Figure 1. Different animal species that learn to vocalize. Vocal mimics further enhance their vocal repertoires by including heterospecific and environmental sounds in their vocalizations (Harcus, 1977; Eens, 1997; Hardy and Parker, 1997; Dowsett-Lemaire, 2008; Gammon and Altizer, 2011; Balsby et al., 2012; Janik, 2014; Mello, 2014; Reichmuth and Casey, 2014; Stoeger and Manger, 2014; Favaro et al., 2016; Mori et al., 2018; Johnson and Clark, 2020; Vernes and Wilkinson, 2020; Dalziell et al., 2021).
One such avian species which learns to produce complex vocal signals are songbirds. Like humans, these birds live in intricate social groups wherein vocal communication is essential (Zann, 1996; Fernandez et al., 2017; Tchernichovski et al., 2017). Birdsong is used to attract mates, mark territories and is the defining feature of songbirds, refined across generations by sexual selection (Zann, 1996). One of the first studies that hinted at song learning in passerine birds was performed by Barrington (1773). In a letter to the Royal Society of London, he wrote about the stages of song learning in young birds, differentiating begging calls for food, distance calls for communication and learnt vocalizations. He stated that the initial phase of song production in young birds was very similar to babbling in human babies, which was strongly influenced by the social environment (Barrington, 1773). Furthermore, active tutors were found to be essential for both young songbirds and human babies. Earlier studies had demonstrated that human babies did not learn language from audio/video recordings (Kuhl et al., 2003). Similarly, Baptista and Petrinovich (1984, 1986) showed that white crowned sparrows (Zonotrichia leucophrys) tutored with taped songs of conspecific adult males until 50 days post hatch chose to learn the songs of a live heterospecific tutor (strawberry finches, Amandava amandava) presented to them after the sensitive period for learning ended. These findings suggested that vocal learners chose to learn from a live heterospecific tutor rather than from the taped vocalizations of conspecifics, showing that social interactions were important for vocal learning.
The presence of a strong social influence and an internal reward guiding vocal learning [reviewed in Riters (2011) and Riters et al. (2019)] hints at the involvement of neuromodulators in vocal learning during the sensitive period. One of the most potent neuromodulator groups that are associated with social reward and motivation is the endogenous opioid system. It is composed of opioid receptors (ORs) and their ligands, the endogenous opioid peptides. The four primary subtypes of opioid receptors include μ (mu)-ORs encoded by the OPRM1 gene (Chen et al., 1993a; Fukuda et al., 1993; Wang et al., 1993), δ (delta)-ORs encoded by the OPRD1 gene (Evans et al., 1992; Kieffer et al., 1992), κ (kappa)-ORs encoded by OPRK1 gene (Chen et al., 1993b; Meng et al., 1993; Minami et al., 1993; Yasuda et al., 1993) and Nociceptin/Orphanin FQ receptors encoded by the OPRL1 gene (Fukuda et al., 1994; Mollereau et al., 1994; Wang et al., 1994; Table 1). The present review focuses mainly on the two most studied subtypes, that is, the μ- and δ-ORs. Besides being activated by their respective endogenous ligands (Pleuvry, 1991), endorphins and enkephalins, respectively, the ORs also bind to a lesser degree to the other opioid ligands (Jordan et al., 2000). The endorphins are synthesized after post-translational modification of the precursor prohormone preproopiomelanocortin (POMC; Smyth, 1983), whereas δ-ORs are activated by enkephalins derived from the peptide precursor preproenkephalin (PENK; Hughes et al., 1975). A third type of opioid ligand, the dynorphins, synthesized from prodynorphin bind to κ-ORs (Goldstein et al., 1979, 1981). By binding to these receptors, opioids influence a variety of physiological activities including analgesia, hunger, motivation, anxiety and even learning (Bodnar, 2004; Wilson and Junor, 2008; Kibaly et al., 2019). Whereas research on opioid addiction has largely overshadowed the role of these receptors in higher cognition, recent studies have shown their involvement in learning (Meilandt et al., 2004; Bertran-Gonzalez et al., 2013).
Both μ- and δ-ORs can act to modulate different kinds of learning, with some researchers hypothesizing that these receptors help in learning the association between drug and reward in addiction (Klenowski et al., 2015). In the present review, we will discuss the anatomical distribution of opioid ligands and receptors in the brain of songbirds and how these receptors may modulate vocalization and vocal learning.
Vocal Learning and the Underlying Neural Circuitry
The process of vocal learning begins with the perception of adult vocalizations. Human babies are exposed to language in utero and newborns respond more to their mother’s voice and language (DeCasper and Fifer, 1980; Moon et al., 1993). This suggests that before learning semantics and grammar, babies learn to identify phonetic arrangements specific to their native language. Songbirds too begin to learn parental vocal signals early in development. The superb fairy wren (Malurus splendens) learns its mother’s incubation calls in ovo and uses a similar vocal structure in its own begging calls for food (Colombelli-Negrel et al., 2014). This has been tested by showing that the embryos of this species of birds show an increased heart rate in response to a playback of tutor songs in ovo (Colombelli-Negrel and Kleindorfer, 2017).
After hatching, the young birds begin an early phase of learning is called the sensory phase, during which they memorize their tutor’s songs. An increased response to the playback of a known song in white-crowned sparrows, trained using songs taped from the tutor, suggests the presence of a memory of the imitated song (Nelson, 1997). Once the song template is learnt, young birds begin to sing a soft and immature subsong (Immelmann, 1969). This is the sensorimotor phase during which the bird tries to match its own song to the “mental template” it had acquired during the sensory phase. Auditory feedback helps in matching the bird’s own vocalizations to that of their fathers/tutors (Konishi, 1965; Brainard and Doupe, 2000). With practice, the vocalizations of the young bird become more structured, but still possess the ability to undergo change. These vocalizations are called plastic songs, which finally develop into a fully structured unchangeable vocal pattern in adulthood (Eales, 1985; Slater and Jones, 1998). For closed-ended learners such as zebra finches (Taenopygia guttata) and white crowned sparrows (Zonotrichia leucophrys), the adult song does not undergo further change and is aptly referred to as “crystallized song” (Immelmann, 1969; Marler and Peters, 1982; Böhner, 1990; Zann, 1990).
For songbirds, the process of vocal learning and production is controlled by specific brain areas called song control nuclei. Nottebohm et al. (1982) showed the presence of five such nuclei in the songbird brain that were associated with vocal control. These nuclei included the pallial sensorimotor nucleus HVC (used as a proper name) which projects to a pallial motor nucleus RA (robust nucleus of the arcopallium) in the caudal part of the brain and forms the vocal motor pathway (VMP), which is important for vocalization since it controls the movements of the syrinx (Nottebohm and Arnold, 1976; Nottebohm et al., 1982). A nucleus in the avian basal ganglia, Area X, also receives projections from HVC and projects to the thalamic nucleus DLM (dorsolateral nucleus of the medial thalamus), which in turn projects to LMAN (lateral magnocellular nucleus of the anterior nidopallium, LMAN). The pathway connecting Area X, DLM and LMAN forms a thalamocortical basal ganglia loop called the anterior forebrain pathway (AFP; Nottebohm et al., 1976; Bottjer et al., 1989), responsible for vocal learning (Bottjer et al., 1984; Scharff and Nottebohm, 1991). The two pathways are interconnected via projections from LMAN to RA (Figure 2; Herrmann and Arnold, 1991).
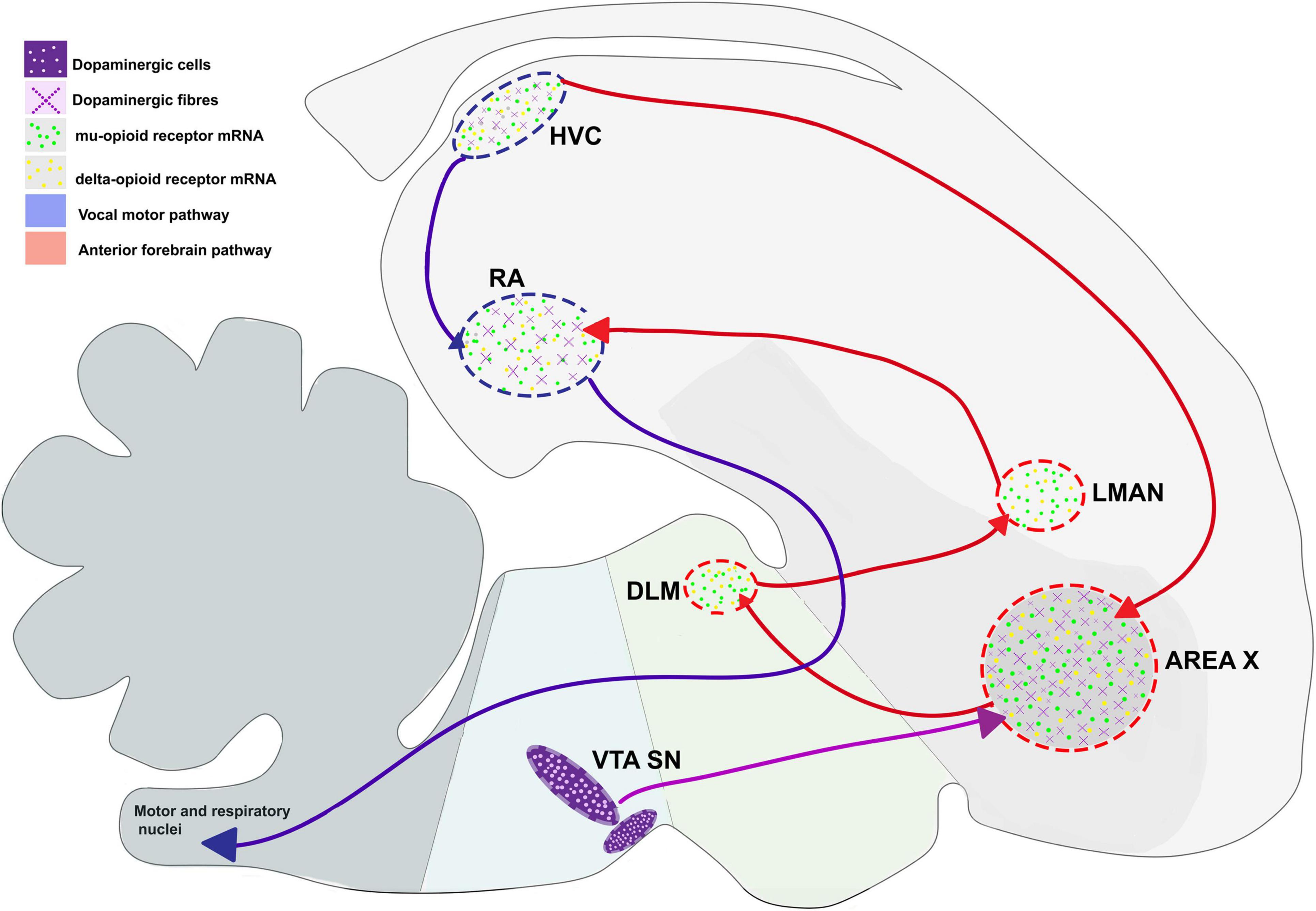
Figure 2. Expression pattern of μ- and δ-opioid receptors and the dopaminergic system in the vocal control nuclei in the songbird brain, based on data from Bottjer (1993) and Khurshid et al. (2009). Blue arrows represent connections of HVC connections, red arrows represent connections of AFP, and violet arrows represent dopaminergic input. DLM, dorsolateral nucleus of the medial thalamus; LMAN, lateral magnocellular nucleus of the anterior nidopallium; RA, robust nucleus of the arcopallium; SN, substantia nigra; VTA, ventral tegmental area.
Signaling and Neural Expression of the Endogenous Opioid System
The opioid receptors are members of the G-protein coupled receptor (GPCR) family. They are composed of seven hydrophobic transmembrane domains connected via intra and extra-cellular loops and possess N- or amino and C or carboxylic groups at the end terminals. Structurally, the μ- and δ-ORs have a binding pocket that interacts with the respective ligand and specifically recognizes the morphinan group (Table 1; Mansour et al., 1997; Granier et al., 2012). Following activation, μ-ORs inhibit adenyl cyclase and voltage-gated Ca2+ channels, and stimulate G protein-activated inwardly rectifying K+ channels (GIRKs) and phospholipase Cβ by the activation of the Gαi/o and Gβγ subunits (Childers, 1991). Similarly, for δ-ORs, the activation of the Gαi/o and Gβγ subunits results in modulation of the activity of calcium channels (P/Q-, N-, and L-type), GIRKs, and inhibition of adenylyl cyclase which reduces the level of intracellular calcium via inhibition of cAMP-dependent calcium channels. Together, these events result in the inhibition of neural activity (Kieffer and Evans, 2009; Al-Hasani and Bruchas, 2011; Gendron et al., 2016). Once activated, both μ- and δ-ORs are internalized and δ-ORs are specifically degraded via the lysosomal pathway (Whistler et al., 2002). In contrast, μ-ORs may continue to be involved in signal transduction even after internalization and only unbound μ-ORs are recycled (Al-Hasani and Bruchas, 2011).
μ-ORs and Their Ligands
In mammals, μ-ORs and their ligands (enkephalins and endorphins) are mostly concentrated in the hippocampus, thalamic nuclei, amygdala, locus coeruleus, parabrachial nucleus, and the nucleus of the solitary tract (Bloom et al., 1978; Di Giulio et al., 1979; Gall et al., 1981). Within the striatum, these receptors are concentrated in patches, which are embedded in a matrix intensely stained for acetylcholine and rich in Substance P (Pert et al., 1976; Brimblecombe and Cragg, 2017). Although the patch and matrix organization of the mammalian striatum is absent in birds, levels of μ-ORs are uniformly high across the striatum in birds including pigeons (Columba livia) (Reiner et al., 1989), chick (Gallus gallus) (Csillag et al., 1990), juncos (Junco hyemalis) (Gulledge and DeViche, 1995; Gulledge and Deviche, 1999) and zebra finches. Furthermore, μ-ORs are present across development in some of the song control regions of juncos HVC, RA, LMAN, and Area X (Gulledge and DeViche, 1995; Gulledge and Deviche, 1999), especially in RA, and in all song control nuclei including HVC, RA, LMAN, Area X, and DLM in adult male zebra finches (Figure 2; Khurshid et al., 2009).
Comparatively fewer studies have been conducted to detect opioid ligands in songbirds. Initial studies used specific antibodies to detect the presence of leu-enkephalin (Ryan et al., 1981) and met-enkephalin (Ryan et al., 1981; Bottjer and Alexander, 1995) in different song control nuclei. Both reports demonstrated the presence of enkephalinergic fibers and somata in components of the VMP and AFP in adult male zebra finches. Additionally, Carrillo and Doupe (2004) found that medium spiny neurons as well as large DLM-projecting neurons in Area X were immunoreactive for leu-enkephalin. These findings were confirmed by Xie et al. (2010), who used mass spectrometry and MALDI-TOF to demonstrate that both proopiomelanocortin (POMC), the precursor peptide of β-endorphin, and preproenkephalin (PENK, the precursor of enkephalin) were present in Area X, LMAN, HVC, and RA.
The presence of both POMC and PENK as well as μ-ORs in components of the VMP (HVC→RA) and AFP (the neural circuit connecting LMAN, Area X, and DLM) in songbirds suggests that they may be able to modulate both vocalization and vocal learning and/or singing in different social contexts. In particular, the localization of μ-ORs in the avian striatum suggests that these receptors may be important for the control of reward-guided behavior, since undirected singing and song learning (cf., Scharff and Nottebohm, 1991) during practice is thought to be internally rewarding (Riters et al., 2019).
δ-ORs and Their Ligands
The neural expression of δ-ORs has been well-documented in rodents. Developmentally, δ-OR expression begins in the pons and the hypothalamus at embryonic day 13 (E 13.5), whereas μ- and κ-ORs are detected in the basal ganglia and midbrain at E 11.5. The prenatal expression of δ-ORs at day E 17.5 and E 19.5 is very low and restricted to the caudate putamen, parabrachial nucleus and olfactory tubercle (Zhu et al., 1998). In contrast, in adult rodents, δ-ORs are distributed in the olfactory tubercle, cerebral cortex, amygdala, nucleus accumbens, and striatum (Mansour et al., 1987, 1994) and have a low, yet detectable presence in the hippocampus and VTA (Erbs et al., 2015). Since this pattern of expression is absent in the prenatal stages, it is possible that they may influence the development of neural circuits developing after birth and modulate the associated cognitive processes. Furthermore, the expression of δ-ORs in areas regulating reward, motivation, learning, memory, and emotional processing (Jutkiewicz, 2018) suggests that these receptors may be involved in cognitive processes such as vocal learning.
In songbirds (zebra finches), the expression of enkephalin is similar to that of δ-ORs. Neuronal fibers immunoreactive for met-enkephalin are distributed across the pallium and are concentrated in song control areas including HVC, RA, LMAN, Area X, and DLM (Ryan et al., 1981; Bottjer and Alexander, 1995). Patterns of δ-OR expression mirrored these results, with δ-OR mRNA being localized to the song control nuclei (LMAN, HVC, RA), all parts of the pallium and hippocampus, and Area X expressing the highest levels of these receptors in adult male zebra finches (Khurshid et al., 2009; Parishar et al., 2021).
As in rodents (see above), it is possible that the expression of δ-ORs and their ligands (enkephalins) may be developmentally regulated in songbirds as well. An earlier study has found higher levels of δ-OR expression in Area X and RA of juvenile juncos (Gulledge and DeViche, 1995), whereas Carrillo and Doupe (2004) have shown that higher levels of leu-enkephalin are present in Area X in juvenile zebra finches, compared to those in adult birds of both species. Another study by Wada et al. (2006) demonstrated that singing for a 30-min duration led to the expression of preproenkephalin (PENK, the precursor of enkephalin) in the song control regions HVC and Area X in adult and juvenile male zebra finches. Furthermore, the expression of PENK (which primarily binds δ-ORs) as well as mRNA for μ-ORs was upregulated in the mPOA (medial preoptic nucleus) in male European starlings (Sturnus vulgaris) during fall, a season marked by increased undirected singing (Riters et al., 2014). The mPOA is connected to the ventral tegmental area (VTA) in male starlings, and lesions of this nucleus lead to deficits in the motivation to sing to females and other courtship-associated behaviors (Riters and Ball, 1999; Alger et al., 2009).
Taken together, these findings suggest that the endogenous opioid system may be involved in modulating song-induced reward associated with positive affect [reviewed in Riters (2011) and Riters et al. (2019)] and may also be involved in guiding vocal learning during development.
The Role of the Endogenous Opioid System in Vocalization and the Motivation to Sing
Besides their involvement in reward and motivation in mammals, μ-ORs are also important for socialization and singing in birds. Female-directed (FD) singing is a highly motivated behavior in which both the mesolimbic dopaminergic systems as well as the opioid system are involved. Recent studies have shown that blocking dopamine receptors using antagonists leads to a decrease in courtship singing in zebra finches (Schroeder and Riters, 2006; Rauceo et al., 2008). The expression of endogenous opioids has been demonstrated in song control nuclei and areas important for motivation and reward in zebra finches (Bottjer and Alexander, 1995; Carrillo and Doupe, 2004). Furthermore, systemic administration of high doses of naloxone (a general opioid receptor agonist with higher affinity for μ- compared to δ-ORs) led to small increases in the number of FD songs in male starlings (Riters et al., 2005), whereas there was a significant decrease in their number following injections of the μ-OR agonist, fentanyl (Schroeder and Riters, 2006). In addition to the endogenous opioids, μ-ORs are expressed in the song control nuclei as well as the VTA-SNc complex in adult male zebra finches (Khurshid et al., 2009, 2010). In contrast to their effects on male starlings, systemic administration of low doses of the opioid antagonist naloxone leads to a decrease in both FD and undirected (UD) singing in adult male zebra finches. Despite different results in the two species, both sets of findings suggest that ORs are involved in the motivation to sing. In naloxone-treated birds, the decrease in the motivation to sing was accompanied by changes in the quality of song: spectral features (including goodness of pitch, frequency, and amplitude modulation) decreased, whereas the duration of songs and intersyllable intervals (temporal features) increased in length, compared to vehicle-treated controls (Khurshid et al., 2010).
Studies on starlings by Kelm-Nelson et al. (2013) and Riters et al. (2014) have demonstrated that mPOA is involved in the motivation to sing. Kelm-Nelson and Riters (2013) have demonstrated that high levels of μ-ORs and enkephalin are present in mPOA in birds which are poor singers (Kelm-Nelson et al., 2013). As mentioned above, Riters et al. (2014) have reported an increase in the expression of PENK and μ-OR mRNA in the mPOA of male starlings during undirected singing in fall. More recently, research on male European starlings suggests that there is a correlation between reward associated with singing behavior and opioid-related gene expression in mPOA. These findings have been confirmed by Stevenson et al. (2020), wherein blocking μ-ORs in mPOA leads to a significant decrease in undirected song and hinders the association between singing and a positive affective state.
Recent studies have shown that blocking ORs with naloxone specifically in components of the AFP, as opposed to systemic injections, led to changes in the motivation to sing as well as those in the acoustic features of FD songs in adult male zebra finches (Kumar et al., 2019, 2020). Infusions of naloxone into LMAN (Kumar et al., 2019) resulted in a significant decrease in the number of FD songs (Kumar et al., 2020). Blocking ORs in both LMAN and Area X led to significant decreases in the length of motifs produced during FD song. Whereas blocking ORs in LMAN led to significant decreases in the amplitude modulation of motifs at a specific dose (100 ng/ml) of naloxone (Kumar et al., 2019), the same manipulation in Area X led to significant decreases in frequency and amplitude modulation and pitch goodness as well as a significant increase in pitch (Kumar et al., 2020). Additionally, blocking ORs in LMAN and Area X led to changes in the spectral quality of individual syllables in directed songs. Furthermore, naloxone infusion into Area X resulted in a local increase in dopamine. These results suggest that altering opioid modulation in LMAN and Area X may lead to changes downstream at the level of the ventral tegmental area (VTA) which sends dopaminergic projections to Area X, among other targets (Gale et al., 2008).
The Role of Opioid Receptors in Learning
μ-ORs and Learning
Besides playing a role in modulating the motivation to sing as well as the spectro-temporal features of song, both μ- and δ-ORs may play a role in modulating song learning since they are present in components of the AFP, including Area X, LMAN, and DLM (Khurshid et al., 2009; Parishar et al., 2021). These findings are supported by earlier reports demonstrating that these receptors are involved in different kinds of learning.
Studies in the Rodent Model System
Aloyo et al. (1993) showed that μ-ORs could modulate associative learning. Using Pavlovian conditioning in rabbits, they conditioned the nictitating membrane reflex to an audio tone using an air puff. Whereas intraventricular administration of saline did not interfere with the learning process, intraventricular injections of D-Ala, Me- Phe-, Gly-ol enkephalin (DAMGO), a μ-OR agonist, impaired conditional learning in experimental animals. This effect was blocked by μ-OR antagonist naloxone (Aloyo et al., 1993). Furthermore, Loh and Galvez (2014) demonstrated that opioid modulation regulated associative learning in a rodent model system. They used a trace paradigm – whisker–trace–eye blink (WTEB) conditioning, wherein an eye blink, elicited using a periorbital electric shock, was conditioned to whisker stimulation. They observed that if naloxone was administered before the conditioning, it was capable of significantly impairing associative learning. However, administration of naloxone after learning did not have any effect on the conditional association in this paradigm (Loh and Galvez, 2014).
To further establish that μ-ORs are involved in motor learning, Lawhorn et al. (2009) used the dermorphin-saporin toxin which specifically targets and destroys neurons in the striosomes which express μ-OR in the basal ganglia. When these mice were tested on motor tasks, they showed specific impairments only on the rotarod test. Striosomes project to substantia nigra pars compacta, a major source of dopaminergic input to the striatum, and ablating μ-OR-positive neurons in this region would lead to a decrease in dopamine release. Since dopaminergic feedback from the midbrain to the striatal and cortical circuits may provide the necessary reinforcement needed to learn and perform on the rotarod, its absence would lead to deficits in motor learning (Lawhorn et al., 2009). In contrast to this study, Cominski et al. (2014) has shown that the loss of μ-ORs leads to an increase in hippocampal neurogenesis which in turn facilitates spatial learning. Furthermore, the μ-OR antagonist naltrexone is known to facilitate spatial learning and memory formation in mice by increasing AMPA receptor phosphorylation and membrane insertion (Kibaly et al., 2016). Yet another study (Laurent et al., 2015) has shown that μ- and δ-ORs play important roles in incentive learning and in value-based and stimulus-based decision-making in mice.
Although these learning paradigms cannot be compared directly to vocal learning in birds, striatal-based learning involves different aspects of social association (Carouso-Peck and Goldstein, 2019), timing (Gobes et al., 2019) and cued dopaminergic input (Gadagkar et al., 2016), each of which are important factors for vocal learning.
Studies on δ-ORs and Learning in the Chicken Model System
Initial studies on the involvement of δ-ORs in learning were performed on chicks (Gallus gallus) by training them on a passive avoidance task. In this experiment, birds were provided with steel beads coated with a bitter tasting chemical called methyl anthranilate (MEA), which they are averse to. A different set of birds, used as controls, were presented with steel beads coated with water. Both experimental and control birds were presented with a single steel bead and their latency to peck and aversive behavior after pecking at the bead was measured to estimate their behavioral response. Experimental birds learned to associate the steel bead with the bitter taste and avoided pecking at the other bead whereas control birds did not avoid the bead. Administration of leu-enkephalin and D-Pen–2, L-Pen–5 enkephalin (DPLPE), a δ-OR selective agonist, into the intermediate medial hyperstriatum ventrale 5 min before training resulted in poor performance on this task. This study also demonstrated that the amnesia caused by δ-OR agonists was reversed by administration of δ-OR antagonists (Patterson et al., 1989). Csillag et al. (1993) also used a passive avoidance task similar to that used by Patterson et al. (1989) to establish the role of these receptors in learning. In their paradigm, 1-day old chicks were trained to peck at a chrome bead coated with methyl anthranilate or water (control). Neural tissue from the trained birds was tested for binding with radio-labeled ligands specific for δ-, μ-, and κ-ORs. Interestingly, there was higher binding for the δ-OR ligand 3H-DPDPE in the striatal areas medial striatum (MSt) and lateral striatum (LSt) (Csillag et al., 1993) in birds which performed well on the avoidance task. In another study, site-specific injections of δ-OR antagonist ICI-174,864 in MSt abolished the avoidance learning for the bitter-tasting bead in 1-day old chicks (Freeman and Young, 2000).
Studies on δ-ORs and Learning in the Rodent Model System
Recent studies on δ-ORs and learning have provided further evidence that these receptors show changes in expression patterns based on the learning experience. Bertran-Gonzalez et al. (2013) trained mice on a Pavlovian instrumental transfer protocol (PIT) in which associations learnt following the delivery of a reward influences the behavior toward two external cues. In this study, a sound was linked to a food reward, followed by training on a second task where a lever press delivered the food reward. A successful test session of the instrumental transfer comprised of the mouse pressing the lever when the sound stimulus was presented. The researchers observed an increase in the expression of δ-ORs in cholinergic interneurons within the shell of nucleus accumbens in mice that had learnt the reward association as well as the instrumental transfer (Bertran-Gonzalez et al., 2013). Interestingly, the level of learning determined the extent of δ-OR expression. These results were similar to those shown in the earlier study by Csillag et al. (1993), which demonstrated an increased binding for δ-ORs in the striatum of chicks trained on an avoidance task.
Another study explored the function of δ-ORs in the hippocampus and their role in learning by using δ-OR gene knockout mice (Oprd1–/–). These gene-deficient mice were poor in place recognition (hippocampal-based learning). Interestingly, they performed better on tasks involving the striatum, such as balancing on an accelerating rotarod, compared to wild type mice (controls). Peripheral injections of the δ-OR antagonist naltrindole in normal mice were able to produce learning deficits similar to the Oprd1–/– mice. This study concluded that δ-ORs are involved in hippocampal-based learning and possibly modulate parvalbumin interneurons that regulate long term potentiation (Le Merrer et al., 2013). Recently, Leroy et al. (2017) has demonstrated a more specific role for hippocampal δ-ORs. They injected naltrindole into the CA2 region of the hippocampus of young mice interacting with their mates. The study revealed that blocking δ-ORs impairs social memory formation by a failure to induce plasticity in the CA2 region (Leroy et al., 2017).
Despite these findings, learning and memory can never be separated from internally reinforcing reward signals, making it difficult to isolate the role of opioid receptors in learning and memory formation from reward related processes in the brain. It is therefore possible that opioid receptors might modulate learning and memory not just via inhibition of local circuits but also by regulating dopaminergic signaling.
Modulation of Dopaminergic Signaling Via μ-ORs: Effects of the Opioid System on the Reward Pathway
The interaction between μ-ORs and dopaminergic signaling has been extensively studied in association with addiction and pain pathways (Van Vliet et al., 1990; Li et al., 2016; Burns et al., 2019). Recently, Galaj et al. (2020) have shown that μ-ORs are highly expressed by the GABAergic neurons of the substantia nigra pars reticulata (SNr), that sends dense innervations to the dopamine rich substantia nigra pars compacta (SNc) and ventral tegmental area (VTA) in mice. They observed that optogenetic activation of SNr GABAergic neurons increased heroin intake and reduced heroin-primed drug seeking, whereas inhibition of these neurons induced optical cranial self-activation and place-preference. These results hint at the opioid modulation of reward via inhibitory projections of the SNr to SNc and VTA (Galaj et al., 2020).
Earlier research shows that δ-OR signaling also influences dopaminergic circuitry and vice versa. Dopaminergic afferents acting via D2 receptors are known to inhibit the production of enkephalin which preferentially binds δ-ORs in striatal neurons (Normand et al., 1988; Jiang et al., 1990; Llorens-Cortes et al., 1991). Conversely, blocking D2 receptors increases the production of enkephalin in the striatum (Steiner and Gerfen, 1999). This coupling between δ-OR and dopaminergic signaling may be responsible for memory retrieval and increased retention of information in mice with induced amnesia (Dubrovina and Ilyutchenok, 1996). Dopaminergic release in the striatum also regulates predictive learning by signaling for error in performance (Iordanova, 2009).
Interestingly, the pharmacological activation of μ-ORs in the ventral striatum induces the activation of δ-OR subtypes, which further enhances dopamine release within the area (Hirose et al., 2005). Opioids can exert control over dopaminergic signaling via different mechanisms. The ORs expressed by GABAergic neurons in VTA or on medium spiny neurons in the striatum lead to the disinhibition of dopaminergic neurons thereby causing an increased release of dopamine. Furthermore, ORs present on dopaminergic neurons in the VTA can inhibit the release of dopamine in the striatum [reviewed in Xi and Stein (2002)]. Taken together, a fine balance between dopaminergic and opioid signaling is required for behavioral reinforcement.
The error prediction and behavioral reinforcement through dopaminergic signaling from the midbrain is essential for vocal learning as well. Evaluative signals in the form of bursts of dopamine released in Area X in zebra finches shape the spectral features of song according to the desired template (Gadagkar et al., 2016; Xiao et al., 2018). Since μ- and δ-ORs are present in components of the AFP (such as LMAN and Area X) which are linked to the VTA-SN complex in birds (Ding and Perkel, 2002; Kumar et al., 2019, 2020), it is possible that the complex interplay between OR activation and dopamine release plays a role in the structuring of vocal patterns during song learning, as discussed below.
Opioid Regulation of Socially Rewarding Behavior and Vocalizations May Also Be Involved in the Regulation of Vocal Learning
Vocal learning is a social process (Tchernichovski et al., 2017) which is rewarding in itself (Riters and Stevenson, 2012). Whereas rodents do not display socially guided vocal learning, certain vocalizations are associated with socially rewarding behavior (Humphreys and Einon, 1981). One such behavior is social play, wherein rodents emit short bursts of high frequency vocalizations (<0.5 s; ∼ 50 Hz) while playing. A play-associated place preference can be established in rodents (Normansell and Panksepp, 1990), suggesting that it is intrinsically rewarding. Similarly, singing-induced place preference can be established in European starlings and zebra finches singing undirected songs (Riters and Stevenson, 2012), also indicating that undirected song, which is produced during learning and for song maintenance, is intrinsically rewarding [reviewed in Riters et al. (2019)]. This play-associated vocal behavior is also demonstrated when young rodents are placed in a spatial location associated with play behavior (Knutson et al., 1998). Opioid agonists such as morphine increase these play-associated vocalizations when administered chronically (Hamed and Boguszewski, 2018). Furthermore, response to play vocalizations is enhanced by opioid agonists and reduced by antagonists (Wohr and Schwarting, 2009). These findings suggest that opioids enable behaviors associated with social activity. In case of a social learning process, like vocal learning, this could help in shaping vocal structure by directing attention toward adult vocalizations produced by tutors and/or other members of the flock (Chen et al., 2016).
Earlier studies (Khurshid et al., 2010; Kumar et al., 2019, 2020) have demonstrated that the opioid system can modulate different aspects of singing. Based on the neuroanatomical localization of ORs in the song control areas of oscines, it is possible that these receptors may be involved in vocal learning (Gulledge and Deviche, 1999). As mentioned above, findings from Wada et al. (2006) have demonstrated that there is an increase in the level of the opioid ligand pre-proenkephalin in Area X after singing in juvenile male birds. An increase in the activation of ORs in Area X could potentially lead to a decrease in the activity of MSNs and a disinhibition of pallidal neurons. This would ultimately lead to the disinhibition of the VTA-SN complex and an increase in DA release in Area X, which acts as an evaluatory signal, shown to play an important role in vocal learning (Figure 3; Gadagkar et al., 2016; Xiao et al., 2018). Additionally, ORs are involved in associative learning, modulate dopaminergic signaling, and are differentially expressed in the brain in the developmental phase rather than in the adulthood in mammals and songbirds, which suggests that ORs may influence cognitive processes such as vocal learning.
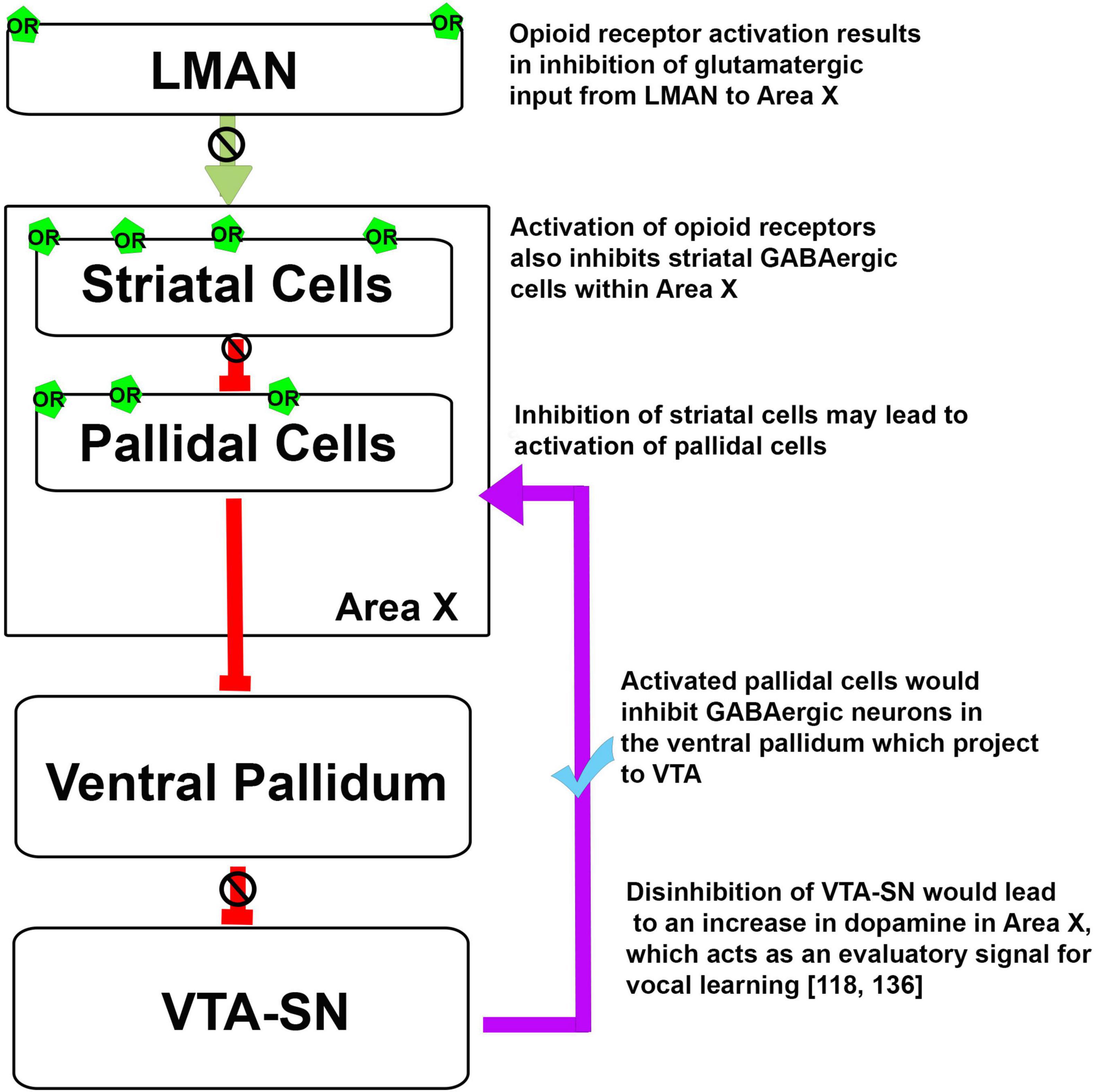
Figure 3. Possible mechanism for the opioid modulation of dopaminergic input to Area X and the regulation of vocal learning. Green arrow, Glutamatergic projection; red arrows, GABAergic projections; violet arrow, dopaminergic projection, OR, opioid receptors.
Conclusion
A number of studies on different species of mammals and birds have demonstrated that the endogenous opioid system is involved in higher cognitive functions including learning. Whereas the endogenous opioid system has been shown to modulate the motivation to vocalize and also effects the acoustic properties of song in different species of songbirds, recent anatomical findings demonstrate that it is present in components of the AFP. Given that the AFP is involved in learning, is connected to the VTA-SN complex and can influence dopamine release, the endogenous opioid system may potentially modulate vocal learning during the sensitive period. Experiments wherein the ORs are blocked or activated in young songbirds at different time points during the sensitive period would provide further insights into the role of the endogenous opioid system in vocal learning.
Author Contributions
UAS and SI contributed to scientific discussions and writing leading to this review. SI approved the final manuscript. Both authors contributed to the article and approved the submitted version.
Funding
This work was supported by a grant (EMR/2015/001422) from Department of Science and Technology (DST), India awarded to SI and core funds from NBRC, Manesar.
Conflict of Interest
The authors declare that the research was conducted in the absence of any commercial or financial relationships that could be construed as a potential conflict of interest.
Publisher’s Note
All claims expressed in this article are solely those of the authors and do not necessarily represent those of their affiliated organizations, or those of the publisher, the editors and the reviewers. Any product that may be evaluated in this article, or claim that may be made by its manufacturer, is not guaranteed or endorsed by the publisher.
References
Adapa, I. D., and Toll, L. (1997). Relationship between binding affinity and functional activity of nociceptin/orphanin FQ. Neuropeptides 31, 403–408. doi: 10.1016/s0143-4179(97)90032-9
Alger, S. J., Maasch, S. N., and Riters, L. V. (2009). Lesions to the medial preoptic nucleus affect immediate early gene immunolabeling in brain regions involved in song control and social behavior in male European starlings. Eur. J. Neurosci. 29, 970–982. doi: 10.1111/j.1460-9568.2009.06637.x
Al-Hasani, R., and Bruchas, M. R. (2011). Molecular mechanisms of opioid receptor-dependent signaling and behavior. Anesthesiology 115, 1363–1381. doi: 10.1097/ALN.0b013e318238bba6
Aloyo, V. J., Romano, A. G., and Harvey, J. A. (1993). Evidence for an involvement of the mu-type of opioid receptor in the modulation of learning. Neuroscience 55, 511–519. doi: 10.1016/0306-4522(93)90520-p
Balsby, T. J., Momberg, J. V., and Dabelsteen, T. (2012). Vocal imitation in parrots allows addressing of specific individuals in a dynamic communication network. PLoS One 7:e49747. doi: 10.1371/journal.pone.0049747
Baptista, L. F., and Petrinovich, L. (1984). Social interaction, sensitive phases and the song template hypothesis in the white-crowned sparrow. Anim. Behav. 32, 172–181. doi: 10.1016/s0003-3472(84)80335-8
Baptista, L. F., and Petrinovich, L. (1986). Song development in the white-crowned sparrow: social factors and sex differences. Ani. Behav. 34, 1359–1371. doi: 10.1016/s0003-3472(86)80207-x
Barrington, D. (1773). XXXI. Experiments and observations on the singing of birds, by the Hon. Daines Barrington, Vice Pres. R. S. In a letter to Mathew Maty, M. D. Sec. R. S. Philos. Trans. R. Soc. Lond. 63, 249–291. doi: 10.1098/rstl.1773.0031
Bertran-Gonzalez, J., Laurent, V., Chieng, B. C., Christie, M. J., and Balleine, B. W. (2013). Learning-related translocation of delta-opioid receptors on ventral striatal cholinergic interneurons mediates choice between goal-directed actions. J. Neurosci. 33, 16060–16071. doi: 10.1523/JNEUROSCI.1927-13.2013
Bigoni, R., Rizzi, D., Rizzi, A., Camarda, V., Guerrini, R., Lambert, D. G., et al. (2002). Pharmacological characterisation of [(pX)Phe4]nociceptin(1-13)amide analogues. 1. In vitro studies. Naunyn Schmiedebergs Arch. Pharmacol. 365, 442–449. doi: 10.1007/s00210-002-0548-8
Bloom, F., Battenberg, E., Rossier, J., Ling, N., and Guillemin, R. (1978). Neurons containing beta-endorphin in rat brain exist separately from those containing enkephalin: immunocytochemical studies. Proc. Natl. Acad. Sci. U. S. A. 75, 1591–1595. doi: 10.1073/pnas.75.3.1591
Bodnar, R. J. (2004). Endogenous opioids and feeding behavior: a 30-year historical perspective. Peptides 25, 697–725. doi: 10.1016/j.peptides.2004.01.006
Böhner, J. (1990). Early acquisition of song in the zebra finch, Taeniopygia guttata. Anim. Behav. 39, 369–374. doi: 10.1016/s0003-3472(05)80883-8
Bottjer, S. W. (1993). The distribution of tyrosine hydroxylase immunoreactivity in the brains of male and female zebra finches. J. Neurobiol. 24, 51–69. doi: 10.1002/neu.480240105
Bottjer, S. W., and Alexander, G. (1995). Localization of met-enkephalin and vasoactive intestinal polypeptide in the brains of male zebra finches. Brain Behav. Evol. 45, 153–177. doi: 10.1159/000113547
Bottjer, S. W., Halsema, K. A., Brown, S. A., and Miesner, E. A. (1989). Axonal connections of a forebrain nucleus involved with vocal learning in zebra finches. J. Comp. Neurol. 279, 312–326. doi: 10.1002/cne.902790211
Bottjer, S. W., Miesner, E. A., and Arnold, A. P. (1984). Forebrain lesions disrupt development but not maintenance of song in passerine birds. Science 224, 901–903. doi: 10.1126/science.6719123
Brainard, M. S., and Doupe, A. J. (2000). Auditory feedback in learning and maintenance of vocal behaviour. Nat. Rev. Neurosci. 1, 31–40. doi: 10.1038/35036205
Brimblecombe, K. R., and Cragg, S. J. (2017). The striosome and matrix compartments of the striatum: a path through the labyrinth from neurochemistry toward function. ACS Chem. Neurosci. 8, 235–242. doi: 10.1021/acschemneuro.6b00333
Burns, J. A., Kroll, D. S., Feldman, D. E., Kure Liu, C., Manza, P., Wiers, C. E., et al. (2019). Molecular imaging of opioid and dopamine systems: insights into the pharmacogenetics of opioid use disorders. Front. Psychiatry 10:626. doi: 10.3389/fpsyt.2019.00626
Carouso-Peck, S., and Goldstein, M. H. (2019). Female social feedback reveals non-imitative mechanisms of vocal learning in zebra finches. Curr. Biol. 29, 631–636.e3. doi: 10.1016/j.cub.2018.12.026
Carrillo, G. D., and Doupe, A. J. (2004). Is the songbird Area X striatal, pallidal, or both? An anatomical study. J. Comp. Neurol. 473, 415–437. doi: 10.1002/cne.20099
Chen, Y., Matheson, L. E., and Sakata, J. T. (2016). Mechanisms underlying the social enhancement of vocal learning in songbirds. Proc. Natl. Acad. Sci. U. S. A. 113, 6641–6646. doi: 10.1073/pnas.1522306113
Chen, Y., Mestek, A., Liu, J., Hurley, J. A., and Yu, L. (1993a). Molecular cloning and functional expression of a mu-opioid receptor from rat brain. Mol. Pharmacol. 44, 8–12.
Chen, Y., Mestek, A., Liu, J., and Yu, L. (1993b). Molecular cloning of a rat kappa opioid receptor reveals sequence similarities to the mu and delta opioid receptors. Biochem. J. 295(Pt 3), 625–628. doi: 10.1042/bj2950625
Cheney, D. L., Owren, M. J., Dieter, J. A., and Seyfarth, R. M. (1992). ‘Food’ calls produced by adult female rhesus (Macaca Mulatta) and Japanese (M. Fuscata) macaques, their normally-raised offspring, and offspring cross-fostered between species. Behaviour 120, 218–231. doi: 10.1163/156853992x00615
Childers, S. R. (1991). Opioid receptor-coupled second messenger systems. Life Sci. 48, 1991–2003. doi: 10.1016/0024-3205(91)90154-4
Colombelli-Negrel, D., Hauber, M. E., and Kleindorfer, S. (2014). Prenatal learning in an Australian songbird: habituation and individual discrimination in superb fairy-wren embryos. Proc. Biol. Sci. 281:20141154. doi: 10.1098/rspb.2014.1154
Colombelli-Negrel, D., and Kleindorfer, S. (2017). Prenatal environment affects embryonic response to song. Biol. Lett. 13:20170302. doi: 10.1098/rsbl.2017.0302
Cominski, T. P., Jiao, X., Catuzzi, J. E., Stewart, A. L., and Pang, K. C. (2014). The role of the hippocampus in avoidance learning and anxiety vulnerability. Front. Behav. Neurosci. 8:273. doi: 10.3389/fnbeh.2014.00273
Csillag, A., Bourne, R. C., and Stewart, M. G. (1990). Distribution of mu, delta, and kappa opioid receptor binding sites in the brain of the one-day-old domestic chick (Gallus domesticus): an in vitro quantitative autoradiographic study. J. Comp. Neurol. 302, 543–551. doi: 10.1002/cne.903020310
Csillag, A., Stewart, M. G., Szekely, A. D., Magloczky, Z., Bourne, R. C., and Steele, R. J. (1993). Quantitative autoradiographic demonstration of changes in binding to delta opioid, but not mu or kappa receptors, in chick forebrain 30 minutes after passive avoidance training. Brain Res. 613, 96–105. doi: 10.1016/0006-8993(93)90459-z
Dalziell, A. H., Maisey, A. C., Magrath, R. D., and Welbergen, J. A. (2021). Male lyrebirds create a complex acoustic illusion of a mobbing flock during courtship and copulation. Curr. Biol. 31 1970–1976.e4. doi: 10.1016/j.cub.2021.02.003
DeCasper, A. J., and Fifer, W. P. (1980). Of human bonding: newborns prefer their mothers’ voices. Science 208, 1174–1176. doi: 10.1126/science.7375928
DeVries, M. S., Cordes, M. A., Stevenson, S. A., and Riters, L. V. (2015). Differential relationships between D1 and D2 dopamine receptor expression in the medial preoptic nucleus and sexually-motivated song in male European starlings (Sturnus vulgaris). Neuroscience 301, 289–297. doi: 10.1016/j.neuroscience.2015.06.011
Di Giulio, A. M., Majane, E. M., and Yang, H. Y. (1979). On the distribution of [met5]- and [leu5]-enkephalins in the brain of the rat, guinea-pig and calf. Br. J. Pharmacol. 66, 297–301. doi: 10.1111/j.1476-5381.1979.tb13679.x
Ding, L., and Perkel, D. J. (2002). Dopamine modulates excitability of spiny neurons in the avian basal ganglia. J. Neurosci. 22, 5210–5218. doi: 10.1523/jneurosci.22-12-05210.2002
Dooley, C. T., Spaeth, C. G., Berzetei-Gurske, I. P., Craymer, K., Adapa, I. D., Brandt, S. R., et al. (1997). Binding and in vitro activities of peptides with high affinity for the nociceptin/orphanin FQ receptor, ORL1. J. Pharmacol. Exp. Ther. 283, 735–741.
Dowsett-Lemaire, F. (2008). The imitative range of the song of the marsh warbler acrocephalus palustris, with special reference to imitations of african birds. Ibis 121, 453–468. doi: 10.1111/j.1474-919x.1979.tb06685.x
Dubrovina, N. I., and Ilyutchenok, R. (1996). Dopamine and opioid regulation of the memory retrieval recovery in mice. Behav. Brain Res. 79, 23–29. doi: 10.1016/0166-4328(95)00258-8
Eales, L. A. (1985). Song learning in zebra finches: some effects of song model availability on what is learnt and when. Anim. Behav. 33, 1293–1300. doi: 10.1016/s0003-3472(85)80189-5
Eens, M. (1997). Understanding the complex song of the European starling: an integrated ethological approach. Adv. Study Behav. 26, 355–434. doi: 10.1016/s0065-3454(08)60384-8
Erbs, E., Faget, L., Scherrer, G., Matifas, A., Filliol, D., Vonesch, J. L., et al. (2015). A mu-delta opioid receptor brain atlas reveals neuronal co-occurrence in subcortical networks. Brain Struct. Funct. 220, 677–702. doi: 10.1007/s00429-014-0717-9
Evans, C. J., Keith, D. E. Jr., Morrison, H., Magendzo, K., and Edwards, R. H. (1992). Cloning of a delta opioid receptor by functional expression. Science 258, 1952–1955. doi: 10.1126/science.1335167
Favaro, L., Neves, S., Furlati, S., Pessani, D., Martin, V., and Janik, V. M. (2016). Evidence suggests vocal production learning in a cross-fostered Risso’s dolphin (Grampus griseus). Anim. Cognit. 19, 847–853. doi: 10.1007/s10071-016-0961-x
Fernandez, M. S. A., Vignal, C., and Soula, H. A. (2017). Impact of group size and social composition on group vocal activity and acoustic network in a social songbird. Anim. Behav. 127, 163–178. doi: 10.1016/j.anbehav.2017.03.013
Freeman, F. M., and Young, I. G. (2000). Identification of the opioid receptors involved in passive-avoidance learning in the day-old chick during the second wave of neuronal activity. Brain Res. 864, 230–239. doi: 10.1016/s0006-8993(00)02181-8
Fukuda, K., Kato, S., Mori, K., Nishi, M., and Takeshima, H. (1993). Primary structures and expression from cDNAs of rat opioid receptor delta- and mu-subtypes. FEBS Lett. 327, 311–314. doi: 10.1016/0014-5793(93)81011-n
Fukuda, K., Kato, S., Mori, K., Nishi, M., Takeshima, H., Iwabe, N., et al. (1994). cDNA cloning and regional distribution of a novel member of the opioid receptor family. FEBS Lett. 343, 42–46. doi: 10.1016/0014-5793(94)80603-9
Gadagkar, V., Puzerey, P. A., Chen, R., Baird-Daniel, E., Farhang, A. R., and Goldberg, J. H. (2016). Dopamine neurons encode performance error in singing birds. Science 354, 1278–1282. doi: 10.1126/science.aah6837
Galaj, E., Han, X., Shen, H., Jordan, C. J., He, Y., Humburg, B., et al. (2020). Dissecting the role of GABA neurons in the VTA versus SNr in opioid reward. J. Neurosci. 40, 8853–8869. doi: 10.1523/JNEUROSCI.0988-20.2020
Gale, S. D., Person, A. L., and Perkel, D. J. (2008). A novel basal ganglia pathway forms a loop linking a vocal learning circuit with its dopaminergic input. J. Comp. Neurol. 508, 824–839. doi: 10.1002/cne.21700
Gall, C., Brecha, N., Karten, H. J., and Chang, K. J. (1981). Localization of enkephalin-like immunoreactivity to identified axonal and neuronal populations of the rat hippocampus. J. Comp. Neurol. 198, 335–350. doi: 10.1002/cne.901980211
Gammon, D. E., and Altizer, C. E. (2011). Northern Mockingbirds produce syntactical patterns of vocal mimicry that reflect taxonomy of imitated species. J. Field Ornithol. 82, 158–164. doi: 10.1111/j.1557-9263.2011.00318.x
Gendron, L., Cahill, C. M., von Zastrow, M., Schiller, P. W., and Pineyro, G. (2016). Molecular pharmacology of delta-opioid receptors. Pharmacol. Rev. 68, 631–700. doi: 10.1124/pr.114.008979
Gobes, S. M. H., Jennings, R. B., and Maeda, R. K. (2019). The sensitive period for auditory-vocal learning in the zebra finch: consequences of limited-model availability and multiple-tutor paradigms on song imitation. Behav. Processes 163, 5–12. doi: 10.1016/j.beproc.2017.07.007
Goldstein, A., Fischli, W., Lowney, L. I., Hunkapiller, M., and Hood, L. (1981). Porcine pituitary dynorphin: complete amino acid sequence of the biologically active heptadecapeptide. Proc. Natl. Acad. Sci. U. S. A. 78, 7219–7223. doi: 10.1073/pnas.78.11.7219
Goldstein, A., Tachibana, S., Lowney, L. I., Hunkapiller, M., and Hood, L. (1979). Dynorphin-(1-13), an extraordinarily potent opioid peptide. Proc. Natl. Acad. Sci. U. S. A. 76, 6666–6670. doi: 10.1073/pnas.76.12.6666
Gong, J., Strong, J. A., Zhang, S., Yue, X., DeHaven, R. N., Daubert, J. D., et al. (1998). Endomorphins fully activate a cloned human mu opioid receptor. FEBS Lett. 439, 152–156. doi: 10.1016/s0014-5793(98)01362-3
Granier, S., Manglik, A., Kruse, A. C., Kobilka, T. S., Thian, F. S., Weis, W. I., et al. (2012). Structure of the delta-opioid receptor bound to naltrindole. Nature 485, 400–404. doi: 10.1038/nature11111
Gulledge, C. C., and DeViche, P. (1995). Autoradiographic localization of opioid receptors in vocal control regions of a male passerine bird (Junco hyemalis). J. Comp. Neurol. 356, 408–417. doi: 10.1002/cne.903560308
Gulledge, C. C., and Deviche, P. (1999). Age- and sex-related differences in opioid receptor densities in the songbird vocal control system. J. Comp. Neurol. 404, 505–514.
Hamed, A., and Boguszewski, P. M. (2018). “Chapter 31 - effects of morphine and other opioid ligands on emission of ultrasonic vocalizations in rats,” in Handbook of Behavioral Neuroscience, ed. S. M. Brudzynski (Amsterdam: Elsevier), 327–334. doi: 10.1016/b978-0-12-809600-0.00031-7
Hammerschmidt, K., Reisinger, E., Westekemper, K., Ehrenreich, L., Strenzke, N., and Fischer, J. (2012). Mice do not require auditory input for the normal development of their ultrasonic vocalizations. BMC Neurosci. 13:40. doi: 10.1186/1471-2202-13-40
Harcus, J. L. (1977). The functions of mimicry in the vocal behaviour of the Chorister Robin. Z. Tierpsychol. 44, 178–193. doi: 10.1111/j.1439-0310.1977.tb00992.x
Hardy, J. W., and Parker, T. A. (1997). The nature and probable function of vocal copying in lawrence’s thrush, Turdus lawrencii. Ornithol. Monogr. 48, 307–320. doi: 10.2307/40157540
Herrmann, K., and Arnold, A. P. (1991). The development of afferent projections to the robust archistriatal nucleus in male zebra finches: a quantitative electron microscopic study. J. Neurosci. 11, 2063–2074. doi: 10.1523/JNEUROSCI.11-07-02063.1991
Hirose, N., Murakawa, K., Takada, K., Oi, Y., Suzuki, T., Nagase, H., et al. (2005). Interactions among mu- and delta-opioid receptors, especially putative delta1- and delta2-opioid receptors, promote dopamine release in the nucleus accumbens. Neuroscience 135, 213–225. doi: 10.1016/j.neuroscience.2005.03.065
Hughes, J., Smith, T. W., Kosterlitz, H. W., Fothergill, L. A., Morgan, B. A., and Morris, H. R. (1975). Identification of two related pentapeptides from the brain with potent opiate agonist activity. Nature 258, 577–580. doi: 10.1038/258577a0
Humphreys, A. P., and Einon, D. F. (1981). Play as a reinforcer for maze-learning in juvenile rats. Anim. Behav. 29, 259–270. doi: 10.1016/s0003-3472(81)80173-x
Immelmann, K. (1969). “Song development in the zebra finch and other estrildid finches,” in Bird Voealizations, ed. R. A. Hinde (Cambridge: Cambridge University Press), 61–74.
Iordanova, M. D. (2009). Dopaminergic modulation of appetitive and aversive predictive learning. Rev. Neurosci. 20, 383–404. doi: 10.1515/revneuro.2009.20.5-6.383
Janik, V. M. (2014). Cetacean vocal learning and communication. Curr. Opin. Neurobiol. 28, 60–65. doi: 10.1016/j.conb.2014.06.010
Jiang, H. K., McGinty, J. F., and Hong, J. S. (1990). Differential modulation of striatonigral dynorphin and enkephalin by dopamine receptor subtypes. Brain Res. 507, 57–64. doi: 10.1016/0006-8993(90)90522-d
Johnson, K. E., and Clark, C. J. (2020). Ontogeny of vocal learning in a hummingbird. Anim. Behav. 167, 139–150. doi: 10.1016/j.anbehav.2020.07.010
Jordan, B. A., Cvejic, S., and Devi, L. A. (2000). Opioids and their complicated receptor complexes. Neuropsychopharmacology 23, S5–S18. doi: 10.1016/S0893-133X(00)00143-3
Jutkiewicz, E. M. (2018). Handbook of Experimental Pharmacology. Cham: Springer International Publishing AG.
Kelm-Nelson, C. A., and Riters, L. V. (2013). Curvilinear relationships between mu-opioid receptor labeling and undirected song in male European starlings (Sturnus vulgaris). Brain Res. 1527, 29–39. doi: 10.1016/J.Brainres.2013.06.010
Kelm-Nelson, C. A., Stevenson, S. A., Cordes, M. A., and Riters, L. V. (2013). Modulation of male song by naloxone in the medial preoptic nucleus. Behav. Neurosci. 127, 451–457. doi: 10.1037/a0032329
Khurshid, N., Agarwal, V., and Iyengar, S. (2009). Expression of mu- and delta-opioid receptors in song control regions of adult male zebra finches (Taenopygia guttata). J. Chem. Neuroanat. 37, 158–169. doi: 10.1016/j.jchemneu.2008.12.001
Khurshid, N., Hameed, L. S., Mohanasundaram, S., and Iyengar, S. (2010). Opioid modulation of cell proliferation in the ventricular zone of adult zebra finches (Taenopygia guttata). FASEB J. 24, 3681–3695. doi: 10.1096/fj.09-146746
Kibaly, C., Kam, A. Y., Loh, H. H., and Law, P. Y. (2016). Naltrexone facilitates learning and delays extinction by increasing AMPA receptor phosphorylation and membrane insertion. Biol. Psychiatry 79, 906–916. doi: 10.1016/j.biopsych.2015.04.019
Kibaly, C., Xu, C., Cahill, C. M., Evans, C. J., and Law, P. Y. (2019). Non-nociceptive roles of opioids in the CNS: opioids’ effects on neurogenesis, learning, memory and affect. Nat. Rev. Neurosci. 20, 5–18. doi: 10.1038/s41583-018-0092-2
Kieffer, B. L., Befort, K., Gaveriaux-Ruff, C., and Hirth, C. G. (1992). The delta-opioid receptor: isolation of a cDNA by expression cloning and pharmacological characterization. Proc. Natl Acad. Sci. U. S. A. 89, 12048–12052. doi: 10.1073/pnas.89.24.12048
Kieffer, B. L., and Evans, C. J. (2009). Opioid receptors: from binding sites to visible molecules in vivo. Neuropharmacology 56, (Suppl. 1), 205–212. doi: 10.1016/j.neuropharm.2008.07.033
Klenowski, P., Morgan, M., and Bartlett, S. E. (2015). The role of delta-opioid receptors in learning and memory underlying the development of addiction. Br. J. Pharmacol. 172, 297–310. doi: 10.1111/bph.12618
Knutson, B., Burgdorf, J., and Panksepp, J. (1998). Anticipation of play elicits high-frequency ultrasonic vocalizations in young rats. J. Comp. Psychol. 112, 65–73. doi: 10.1037/0735-7036.112.1.65
Konishi, M. (1965). The role of auditory feedback in the control of vocalization in the White-crowned Sparrow. Z. Tierpsychol. 22, 770–783. doi: 10.1111/j.1439-0310.1965.tb01688.x
Kuhl, P. K., Tsao, F. M., and Liu, H. M. (2003). Foreign-language experience in infancy: effects of short-term exposure and social interaction on phonetic learning. Proc. Natl. Acad. Sci. U. S. A. 100, 9096–9101. doi: 10.1073/pnas.1532872100
Kumar, S., Mohapatra, A. N., Pundir, A. S., Kumari, M., Din, U., Sharma, S., et al. (2020). Blocking opioid receptors in a songbird cortical region modulates the acoustic features and levels of female-directed singing. Front. Neurosci. 14:554094. doi: 10.3389/fnins.2020.554094
Kumar, S., Mohapatra, A. N., Sharma, H. P., Singh, U. A., Kambi, N. A., Velpandian, T., et al. (2019). Altering opioid neuromodulation in the songbird basal ganglia modulates vocalizations. Front. Neurosci. 13:671. doi: 10.3389/fnins.2019.00671
Laurent, V., Morse, A. K., and Balleine, B. W. (2015). The role of opioid processes in reward and decision-making. Br. J. Pharmacol. 172, 449–459. doi: 10.1111/bph.12818
Lawhorn, C., Smith, D. M., and Brown, L. L. (2009). Partial ablation of mu-opioid receptor rich striosomes produces deficits on a motor-skill learning task. Neuroscience 163, 109–119. doi: 10.1016/j.neuroscience.2009.05.021
Le Merrer, J., Rezai, X., Scherrer, G., Becker, J. A., and Kieffer, B. L. (2013). Impaired hippocampus-dependent and facilitated striatum-dependent behaviors in mice lacking the delta opioid receptor. Neuropsychopharmacology 38, 1050–1059. doi: 10.1038/npp.2013.1
Leroy, F., Brann, D. H., Meira, T., and Siegelbaum, S. A. (2017). Input-timing-dependent plasticity in the hippocampal CA2 region and its potential role in social memory. Neuron 95, 1089–1102.e5.
Li, C., Sugam, J. A., Lowery-Gionta, E. G., McElligott, Z. A., McCall, N. M., Lopez, A. J., et al. (2016). Mu opioid receptor modulation of dopamine neurons in the periaqueductal gray/dorsal raphe: a role in regulation of pain. Neuropsychopharmacology 41, 2122–2132. doi: 10.1038/npp.2016.12
Li, S., Zhu, J., Chen, C., Chen, Y. W., Deriel, J. K., Ashby, B., et al. (1993). Molecular cloning and expression of a rat kappa opioid receptor. Biochem. J. 295(Pt 3), 629–633. doi: 10.1042/bj2950629
Llorens-Cortes, C., Zini, S., Gros, C., and Schwartz, J. C. (1991). Dopaminergic regulation of enkephalin release. J. Neurochem. 56, 1368–1375. doi: 10.1111/j.1471-4159.1991.tb11434.x
Loh, R. M., and Galvez, R. (2014). Opioid antagonism impairs acquisition of forebrain-dependent trace-associative learning: an eyeblink conditioning analysis. Pharmacol. Biochem. Behav. 118, 46–50. doi: 10.1016/j.pbb.2014.01.005
Mansour, A., Fox, C. A., Burke, S., Meng, F., Thompson, R. C., Akil, H., et al. (1994). Mu, delta, and kappa opioid receptor mRNA expression in the rat CNS: an in situ hybridization study. J. Comp. Neurol. 350, 412–438. doi: 10.1002/cne.903500307
Mansour, A., Khachaturian, H., Lewis, M. E., Akil, H., and Watson, S. J. (1987). Autoradiographic differentiation of mu, delta, and kappa opioid receptors in the rat forebrain and midbrain. J. Neurosci. 7, 2445–2464.
Mansour, A., Taylor, L. P., Fine, J. L., Thompson, R. C., Hoversten, M. T., Mosberg, H. I., et al. (1997). Key residues defining the mu-opioid receptor binding pocket: a site-directed mutagenesis study. J. Neurochem. 68, 344–353. doi: 10.1046/j.1471-4159.1997.68010344.x
Marler, P., and Peters, S. (1982). Developmental overproduction and selective attrition: new processes in the epigenesis of birdsong. Dev. Psychobiol. 15, 369–378. doi: 10.1002/dev.420150409
Meilandt, W. J., Barea-Rodriguez, E., Harvey, S. A., and Martinez, J. L. Jr. (2004). Role of hippocampal CA3 mu-opioid receptors in spatial learning and memory. J. Neurosci. 24, 2953–2962. doi: 10.1523/JNEUROSCI.5569-03.2004
Mello, C. V. (2014). The zebra finch, Taeniopygia guttata: an avian model for investigating the neurobiological basis of vocal learning. Cold Spring Harb. Protoc. 2014, 1237–1242. doi: 10.1101/pdb.emo084574
Meng, F., Xie, G. X., Thompson, R. C., Mansour, A., Goldstein, A., Watson, S. J., et al. (1993). Cloning and pharmacological characterization of a rat kappa opioid receptor. Proc. Natl. Acad. Sci. U. S. A. 90, 9954–9958. doi: 10.1073/pnas.90.21.9954
Minami, M., Toya, T., Katao, Y., Maekawa, K., Nakamura, S., Onogi, T., et al. (1993). Cloning and expression of a cDNA for the rat kappa-opioid receptor. FEBS Lett. 329, 291–295. doi: 10.1016/0014-5793(93)80240-u
Mollereau, C., Parmentier, M., Mailleux, P., Butour, J. L., Moisand, C., Chalon, P., et al. (1994). ORL1, a novel member of the opioid receptor family. Cloning, functional expression and localization. FEBS Lett. 341, 33–38. doi: 10.1016/0014-5793(94)80235-1
Moon, C., Cooper, R. P., and Fifer, W. P. (1993). Two-day-olds prefer their native language. Infant Behav. Dev. 16, 495–500. doi: 10.1016/0163-6383(93)80007-u
Mori, C., Liu, W. C., and Wada, K. (2018). Recurrent development of song idiosyncrasy without auditory inputs in the canary, an open-ended vocal learner. Sci. Rep. 8:8732. doi: 10.1038/s41598-018-27046-4
Nelson, D. A. (1997). Social Interaction and Sensitive Phases for Song Learning: A Critical Review, Social Influences on Vocal Development. New York, NY: Cambridge University Press, 7–22.
Normand, E., Popovici, T., Onteniente, B., Fellmann, D., Piatier-Tonneau, D., Auffray, C., et al. (1988). Dopaminergic neurons of the substantia nigra modulate preproenkephalin A gene expression in rat striatal neurons. Brain Res. 439, 39–46. doi: 10.1016/0006-8993(88)91459-x
Normansell, L., and Panksepp, J. (1990). Effects of morphine and naloxone on play-rewarded spatial discrimination in juvenile rats. Dev. Psychobiol. 23, 75–83. doi: 10.1002/dev.420230108
Nottebohm, F., and Arnold, A. (1976). Sexual dimorphism in vocal control areas of the songbird brain. Science 194, 211–213. doi: 10.1126/science.959852
Nottebohm, F., Kelley, D. B., and Paton, J. A. (1982). Connections of vocal control nuclei in the canary telencephalon. J. Comp. Neurol. 207, 344–357. doi: 10.1002/cne.902070406
Nottebohm, F., Stokes, T. M., and Leonard, C. M. (1976). Central control of song in the canary, Serinus canarius. J. Comp. Neurol. 165, 457–486. doi: 10.1002/cne.901650405
Parishar, P., Sehgal, N., and Iyengar, S. (2021). The expression of delta opioid receptor mRNA in adult male zebra finches (Taenopygia guttata). PLoS One 16:e0256599. doi: 10.1371/journal.pone.0256599
Patterson, T. A., Schulteis, G., Alvarado, M. C., Martinez, J. L. Jr., Bennett, E. L., Rosenzweig, M. R., et al. (1989). Influence of opioid peptides on learning and memory processes in the chick. Behav. Neurosci. 103, 429–437. doi: 10.1037//0735-7044.103.2.429
Pert, C. B., Kuhar, M. J., and Snyder, S. H. (1976). Opiate receptor: autoradiographic localization in rat brain. Proc. Natl. Acad. Sci. U. S. A. 73, 3729–3733. doi: 10.1073/pnas.73.10.3729
Pleuvry, B. J. (1991). Opioid receptors and their ligands: natural and unnatural. Br. J. Anaesth. 66, 370–380. doi: 10.1093/bja/66.3.370
Rauceo, S., Harding, C. F., Maldonado, A., Gaysinkaya, L., Tulloch, I., and Rodriguez, E. (2008). Dopaminergic modulation of reproductive behavior and activity in male zebra finches. Behav. Brain Res. 187, 133–139. doi: 10.1016/j.bbr.2007.09.003
Raynor, K., Kong, H., Chen, Y., Yasuda, K., Yu, L., Bell, G. I., et al. (1994). Pharmacological characterization of the cloned kappa-, delta-, and mu-opioid receptors. Mol. Pharmacol. 45, 330–334.
Reichmuth, C., and Casey, C. (2014). Vocal learning in seals, sea lions, and walruses. Curr. Opin. Neurobiol. 28, 66–71. doi: 10.1016/j.conb.2014.06.011
Reiner, A., Brauth, S. E., Kitt, C. A., and Quirion, R. (1989). Distribution of mu, delta, and kappa opiate receptor types in the forebrain and midbrain of pigeons. J. Comp. Neurol. 280, 359–382. doi: 10.1002/cne.902800304
Riters, L. V. (2011). Pleasure seeking and birdsong. Neurosci. Biobehav. Rev. 35, 1837–1845. doi: 10.1016/j.neubiorev.2010.12.017
Riters, L. V., and Ball, G. F. (1999). Lesions to the medial preoptic area affect singing in the male European starling (Sturnus vulgaris). Horm. Behav. 36, 276–286. doi: 10.1006/hbeh.1999.1549
Riters, L. V., Schroeder, M. B., Auger, C. J., Eens, M., Pinxten, R., and Ball, G. F. (2005). Evidence for opioid involvement in the regulation of song production in male European starlings (Sturnus vulgaris). Behav. Neurosci. 119, 245–255. doi: 10.1037/0735-7044.119.1.245
Riters, L. V., Spool, J. A., Merullo, D. P., and Hahn, A. H. (2019). Song practice as a rewarding form of play in songbirds. Behav Processes 163, 91–98. doi: 10.1016/j.beproc.2017.10.002
Riters, L. V., and Stevenson, S. A. (2012). Reward and vocal production: song-associated place preference in songbirds. Physiol. Behav. 106, 87–94. doi: 10.1016/j.physbeh.2012.01.010
Riters, L. V., Stevenson, S. A., DeVries, M. S., and Cordes, M. A. (2014). Reward associated with singing behavior correlates with opioid-related gene expression in the medial preoptic nucleus in male European starlings. PLoS One 9:e115285. doi: 10.1371/journal.pone.0115285
Ryan, S. M., Arnold, A. P., and Elde, R. P. (1981). Enkephalin-like immunoreactivity in vocal control regions of the zebra finch brain. Brain Res. 229, 236–240. doi: 10.1016/0006-8993(81)90763-0
Scharff, C., and Nottebohm, F. (1991). A comparative study of the behavioral deficits following lesions of various parts of the zebra finch song system: implications for vocal learning. J. Neurosci. 11, 2896–2913. doi: 10.1523/JNEUROSCI.11-09-02896.1991
Schroeder, M. B., and Riters, L. V. (2006). Pharmacological manipulations of dopamine and opioids have differential effects on sexually motivated song in male European starlings. Physiol. Behav. 88, 575–584. doi: 10.1016/j.physbeh.2006.05.011
Simonin, F., Gaveriaux-Ruff, C., Befort, K., Matthes, H., Lannes, B., Micheletti, G., et al. (1995). kappa-Opioid receptor in humans: cDNA and genomic cloning, chromosomal assignment, functional expression, pharmacology, and expression pattern in the central nervous system. Proc. Natl. Acad. Sci. U. S. A. 92, 7006–7010. doi: 10.1073/pnas.92.15.7006
Slater, P. J. B., and Jones, A. E. (1998). Practice and song development in zebra finches. Behaviour 135, 1125–1136.
Smyth, D. G. (1983). beta-Endorphin and related peptides in pituitary, brain, pancreas and antrum. Br. Med. Bull. 39, 25–30. doi: 10.1093/oxfordjournals.bmb.a071786
Steiner, H., and Gerfen, C. R. (1999). Enkephalin regulates acute D2 dopamine receptor antagonist-induced immediate-early gene expression in striatal neurons. Neuroscience 88, 795–810. doi: 10.1016/s0306-4522(98)00241-3
Stevenson, S. A., Piepenburg, A., Spool, J. A., Angyal, C. S., Hahn, A. H., Zhao, C., et al. (2020). Endogenous opioids facilitate intrinsically-rewarded birdsong. Sci. Rep. 10:11083. doi: 10.1038/s41598-020-67684-1
Stoeger, A. S., and Manger, P. (2014). Vocal learning in elephants: neural bases and adaptive context. Curr. Opin. Neurobiol. 28, 101–107. doi: 10.1016/j.conb.2014.07.001
Tchernichovski, O., Feher, O., Fimiarz, D., and Conley, D. (2017). How social learning adds up to a culture: from birdsong to human public opinion. J. Exp. Biol. 220, 124–132. doi: 10.1242/jeb.142786
Toll, L., Berzetei-Gurske, I. P., Polgar, W. E., Brandt, S. R., Adapa, I. D., Rodriguez, L., et al. (1998). Standard binding and functional assays related to medications development division testing for potential cocaine and opiate narcotic treatment medications. NIDA Res. Monogr. 178, 440–466.
Van Vliet, B. J., Mulder, A. H., and Schoffelmeer, A. N. (1990). Mu-opioid receptors mediate the inhibitory effect of opioids on dopamine-sensitive adenylate cyclase in primary cultures of rat neostriatal neurons. J. Neurochem. 55, 1274–1280. doi: 10.1111/j.1471-4159.1990.tb03135.x
Vernes, S. C., and Wilkinson, G. S. (2020). Behaviour, biology and evolution of vocal learning in bats. Philos. Trans. R. Soc. Lond. B Biol. Sci. 375:20190061. doi: 10.1098/rstb.2019.0061
Wada, K., Howard, J. T., McConnell, P., Whitney, O., Lints, T., Rivas, M. V., et al. (2006). A molecular neuroethological approach for identifying and characterizing a cascade of behaviorally regulated genes. Proc. Natl. Acad. Sci. U. S. A. 103, 15212–15217. doi: 10.1073/pnas.0607098103
Wang, J. B., Imai, Y., Eppler, C. M., Gregor, P., Spivak, C. E., and Uhl, G. R. (1993). mu opiate receptor: cDNA cloning and expression. Proc. Natl. Acad. Sci. U. S. A. 90, 10230–10234. doi: 10.1073/pnas.90.21.10230
Wang, J. B., Johnson, P. S., Imai, Y., Persico, A. M., Ozenberger, B. A., Eppler, C. M., et al. (1994). cDNA cloning of an orphan opiate receptor gene family member and its splice variant. FEBS Lett. 348, 75–79. doi: 10.1016/0014-5793(94)00557-5
Wegdell, F., Hammerschmidt, K., and Fischer, J. (2019). Conserved alarm calls but rapid auditory learning in monkey responses to novel flying objects. Nat. Ecol. Evol. 3, 1039–1042. doi: 10.1038/s41559-019-0903-5
Whistler, J. L., Enquist, J., Marley, A., Fong, J., Gladher, F., Tsuruda, P., et al. (2002). Modulation of postendocytic sorting of G protein-coupled receptors. Science 297, 615–620. doi: 10.1126/science.1073308
Wilson, M. A., and Junor, L. (2008). The role of amygdalar mu-opioid receptors in anxiety-related responses in two rat models. Neuropsychopharmacology 33, 2957–2968. doi: 10.1038/sj.npp.1301675
Wohr, M., and Schwarting, R. K. (2009). Ultrasonic communication in rats: effects of morphine and naloxone on vocal and behavioral responses to playback of 50-kHz vocalizations. Pharmacol. Biochem. Behav. 94, 285–295. doi: 10.1016/j.pbb.2009.09.008
Xi, Z. X., and Stein, E. A. (2002). GABAergic mechanisms of opiate reinforcement. Alcohol Alcohol. 37, 485–494. doi: 10.1093/alcalc/37.5.485
Xiao, L., Chattree, G., Oscos, F. G., Cao, M., Wanat, M. J., Roberts, T. F., et al. (2018). Circuit sufficient to guide birdsong learning. Neuron 98, 208–221 e5. doi: 10.1016/j.neuron.2018.02.020
Xie, F., London, S. E., Southey, B. R., Annangudi, S. P., Amare, A., Rodriguez-Zas, S. L., et al. (2010). The zebra finch neuropeptidome: prediction, detection and expression. BMC Biol. 8:28. doi: 10.1186/1741-7007-8-28
Yasuda, K., Raynor, K., Kong, H., Breder, C. D., Takeda, J., Reisine, T., et al. (1993). Cloning and functional comparison of kappa and delta opioid receptors from mouse brain. Proc. Natl. Acad. Sci. U. S. A. 90, 6736–6740. doi: 10.1073/pnas.90.14.6736
Zadina, J. E., Hackler, L., Ge, L. J., and Kastin, A. J. (1997). A potent and selective endogenous agonist for the mu-opiate receptor. Nature 386, 499–502.
Zann, R. (1990). Song and call learning in wild zebra finches in south-east Australia. Anim. Behav. 40, 811–828.
Zann, R. A. (1996). The Zebra Finch: A Synthesis of Field and Laboratory Studies. Oxford: Oxford University Press.
Zhu, J., Chen, C., Xue, J. C., Kunapuli, S., DeRiel, J. K., and Liu-Chen, L. Y. (1995). Cloning of a human kappa opioid receptor from the brain. Life Sci. 56, L201–L207. doi: 10.1016/0024-3205(94)00507-o
Zhu, J., Luo, L. Y., Li, J. G., Chen, C., and Liu-Chen, L. Y. (1997). Activation of the cloned human kappa opioid receptor by agonists enhances [35S]GTPgammaS binding to membranes: determination of potencies and efficacies of ligands. J. Pharmacol. Exp. Ther. 282, 676–684.
Keywords: songbirds, endogenous opioids, opioid receptors, learning, reward, basal ganglia
Citation: Singh UA and Iyengar S (2022) The Role of the Endogenous Opioid System in the Vocal Behavior of Songbirds and Its Possible Role in Vocal Learning. Front. Physiol. 13:823152. doi: 10.3389/fphys.2022.823152
Received: 26 November 2021; Accepted: 31 January 2022;
Published: 22 February 2022.
Edited by:
Toshiya Matsushima, Hokkaido University, JapanCopyright © 2022 Singh and Iyengar. This is an open-access article distributed under the terms of the Creative Commons Attribution License (CC BY). The use, distribution or reproduction in other forums is permitted, provided the original author(s) and the copyright owner(s) are credited and that the original publication in this journal is cited, in accordance with accepted academic practice. No use, distribution or reproduction is permitted which does not comply with these terms.
*Correspondence: Soumya Iyengar, c291bXlhLmdvdkBuYnJjLmFjLmlu; c291bXlhQG5icmMuYWMuaW4=