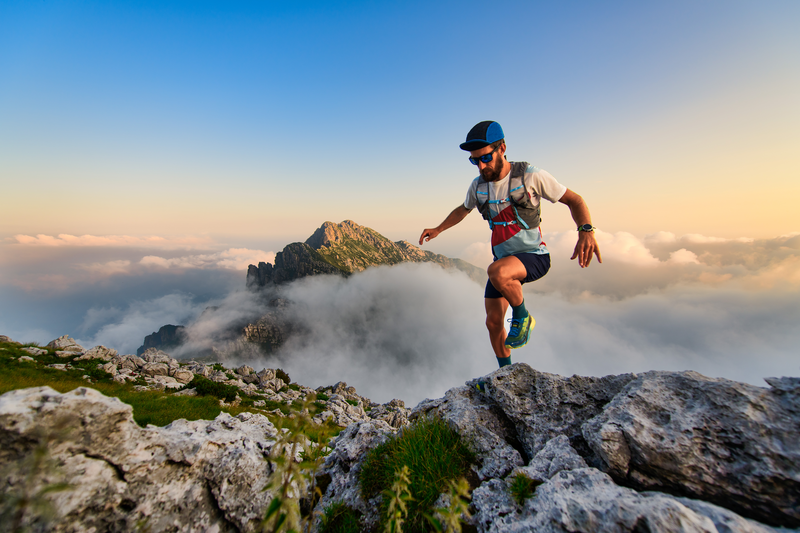
95% of researchers rate our articles as excellent or good
Learn more about the work of our research integrity team to safeguard the quality of each article we publish.
Find out more
ORIGINAL RESEARCH article
Front. Physiol. , 18 February 2022
Sec. Aquatic Physiology
Volume 13 - 2022 | https://doi.org/10.3389/fphys.2022.805861
This article is part of the Research Topic Crustacean Reproductive Physiology and its Applications View all 11 articles
Ferritin plays an essential role in organismic and cellular iron homeostasis in Macrobrachium nipponense. In this study, we aimed to investigate the role of ferritin in the sexual development of male M. nipponense. According to the qPCR analysis of different tissues and developmental stages, ferritin exhibited high expression levels in the testis and androgenic gland, from post-larval developmental stage 5 (PL5) to PL15, indicating that it may be involved in gonad differentiation and development, especially in male sexual development. In situ hybridization and qPCR analysis in various reproductive cycles of the testis indicated that ferritin may play an essential role in spermatogonia development in M. nipponense. RNAi analysis revealed that ferritin positively affected mRNA expression of the insulin-like androgenic gland (Mn-IAG) and the secretion of testosterone, and thus positively affected testis development in M. nipponense. This study highlighted the functions of ferritin in the sexual development of male M. nipponense and provided important information for the establishment of a technique to regulate the process of testis development in M. nipponense.
The oriental river prawn, Macrobrachium nipponense (Crustacea; Decapoda; and Palaemonidae), is an important commercial species in China (Cai and Shokita, 2006; Salman et al., 2006; Ma et al., 2011), with an annual production of 225,321 tons in 2019 (Zhang et al., 2020). There are significant differences in growth performance of male and female M. nipponense in aquaculture systems. Compared to their female counterparts, male M. nipponense exhibit better growth performance (Ma et al., 2011). Rapid gonad development has negative effects on the sustainable development of the M. nipponense aquaculture industry. A previous study reported that the both testis and ovaries of prawns are mature at 40 days after hatching (Jin et al., 2016). This results in inbreeding between the newborn prawns. Inbreeding leads to multiple generations in the same pond and the degradation of germplasm qualities, resulting in the prawns with smaller market size, and decreased ability to resist diseases (Fu et al., 2012; Jin et al., 2021a,b). Therefore, studies on the sexual development of male M. nipponense have received increased attention in recent years, with the aim of establishing an artificial technique to produce all male progeny on a commercial scale and regulate the process of testis development.
The androgenic gland has become a target tissue for studying male sexual differentiation and development in crustacean species in recent years. The hormones secreted by the androgenic gland have been reported to play positive regulatory roles in the processes driving male sexual differentiation and characteristics, in particular, the promotion of testes development in crustacean species (Sagi et al., 1986, 1990). Insulin-like androgenic gland hormone (IAG) is the main gene expressed and analyzed in the androgenic gland (Ventura et al., 2009, 2011; Rosen et al., 2010). It has been shown to play essential roles in promoting male sex determination and sex differentiation in most crustacean species, including Fenneropenaeus chinensis (Li et al., 2012), Scylla paramamosain (Huang et al., 2014), Lysmata vittata (Liu et al., 2021), Fenneropenaeus merguiensis (Zhou et al., 2021), and M. nipponense (Li et al., 2015a). Silencing of IAG in male M. rosenbergii by RNA interference (RNAi) may also lead to a complete sex reversal (Ventura et al., 2012). A previous study reported that IAG was expressed in the androgenic gland in M. nipponense (Ma et al., 2016). Thus, the genes in the androgenic gland have received a lot of attention in recent years and the transcriptome and miRNA library of the androgenic gland have been constructed for M. nipponense (Jin et al., 2013, 2015). A series of genes identified in the androgenic gland transcriptome have been analyzed and proven to be involved in the sexual development mechanisms of male M. nipponense (Jin et al., 2014, 2018b; Li et al., 2015a; Ma et al., 2016).
Previous studies have found that ferritin regulates cellular and organism-wide iron homeostasis, and thus protects cells from damage by excess iron (Picard et al., 1996; Konijn et al., 1999; Kakhlon et al., 2001; Torti and Torti, 2002). In addition, ferritin has multiple other functions, e.g., in development, cell activation, and angiogenesis (Parthasarathy et al., 2002; Coffman et al., 2008; Alkhateeb and Connor, 2010; Wang et al., 2010). A total of three ferritin subunits were identified in M. nipponense, including ferritin, ferritin light-chain subunit, and ferritin heavy-chain subunit. A previous study revealed that ferritin with accession no. KC825355 in GenBank plays an essential role in organismic and cellular iron homeostasis in M. nipponense (Sun et al., 2014). However, ferritin was also highly expressed in the androgenic gland, which is predicted to have additional roles in the mechanisms of sexual development in male M. nipponense (Jin et al., 2018a).
In this study, we aimed to further analyze the function of ferritin in M. nipponense, especially in relation to its role in male sexual development, using Quantitative real-time PCR (qPCR), in situ hybridization, and RNAi, combined with histological observations and testosterone measurements. The results of this study highlighted the functions of ferritin in M. nipponense, providing a basis for further studies on the mechanism of male sexual development in other crustacean species.
Permission for all experiments involving M. nipponense was obtained from the Institutional Animal Care and Use Ethics Committee of the Freshwater Fisheries Research Center, Chinese Academy of Fishery Sciences (Wuxi, China).
The relative mRNA expression of Mn-ferritin was measured using qPCR. Different mature tissues included the testis, androgenic gland, ovary, intestine, hepatopancreas, and heart. The full-sibs population was hatched and cultured, and specimens at different larval and post-larval developmental stages were collected every 5 days during their maturation process. Testes were collected during the reproductive season in July, when the water temperature was ≥28°C and the illumination time was ≥16 h. Testes were also collected during the non-reproductive season in January, when the water temperature was ≤15°C and illumination time was ≤12 h. Samples of each tissue or stage were collected from fifty individual prawns. Ten prawns were pooled together to form one biological replicate, in order to minimize the effects of individual differences and five biological replicates were conducted. The procedures of RNA isolation and synthesizing cDNA have been described in detail in previous studies (Jin et al., 2014, 2018b). Briefly, total RNA was extracted from each tissue, using the UNlQ-10 Column TRIzol Total RNA Isolation Kit (Sangon, Shanghai, China) following the manufacturer’s protocol. A total of 1 μg total RNA from each tissue was used to synthesize the cDNA template by using the PrimeScript RT reagent Kit (Takara Bio Inc., Japan). The expression level of each tissue was determined using the UltraSYBR Mixture (CWBIO, Beijing, China). All of the qPCR analyses in this study were performed on the Bio-Rad iCycler iQ5 Real-Time PCR System (Bio-Rad, Hercules, CA, United States), which was used to carry out the SYBR Green RT-qPCR assay. All qPCR reactions were run in three technical replicates. Table 1 lists the primers used for qPCR analysis, including the eukaryotic translation initiation factor 5A (EIF 5A), which was identified as a suitable reference gene for PCR analysis in M. nipponense (Hu et al., 2018). The relative mRNA expressions of Mn-ferritin were calculated, based on the 2–ΔΔCT comparative CT method (Livak and Schmittgen, 2001).
The mRNA locations of Mn-ferritin were analyzed by in situ hybridization. Paraformaldehyde (4%) (Sangon, Shanghai, China) was used to fix the tissue samples until the experiment was carried out. The androgenic gland and hepatopancreas were sampled during the reproductive season (28°C), while testes were collected from both of the reproductive season (28°C) and non-reproductive season (15°C). Procedures of primer design and in situ hybridization have been described in detail in previous studies (Jin et al., 2018b; Li et al., 2018). Briefly, Primer 5 software was used to design the anti-sense and sense probes with a DIG tag for the in-situ hybridization study, based on the cDNA sequence of Mn-ferritin. Table 1 lists the primers used for in situ hybridization analysis, and the primers with DIG signals were synthesized by Shanghai Sangon Biotech Company. The anti-sense probe and sense probe were prepared for the experimental group and the control group, respectively. All of the collected testes (15 and 28°C), androgenic glands (28°C) and hepatopancreas (28°C) were embedded by paraffin. The in situ hybridization study was performed on 4 μm thick sections of paraffin-embedded tissues using the ZytoFast PLUS CISH implementation kit (ZytoVision GmBH, Bremerhaven, Germany), following the manufacturer’s protocol. The sections were incubated in 3% H2O2 for 10 min. After rinsing in deionized water (DW), target retrieval was achieved using 0.5 mg/ml pepsin digestion in a humidity chamber for 10 min. The slides were incubated in EDTA solution at 95°C for 15 min after being washed in DW. The slides were then washed in DW, drained off, and 20 μL of CISH anti-sense probe and sense probe were poured over each slide. Denaturation was carried out at 75°C for 5 min, followed by hybridization at 37°C for 60 min in the ThermoBrite TM hybridization chamber (Vysis Inc, Downers Grove, IL, United States). Tris-buffered-saline (TBS) washing was carried out at 55°C for 5 min, and then at room temperature for five min. Mouse-anti-DIG (ZytoVision GmBH, Bremerhaven, Germany) was dropped over each slide, and incubated in a humidity chamber at 37°C for 30 min. Three washings were carried out with TBS, each lasting for 1 min, before and after incubating slides in anti-mouse-HRP-polymer for 30 min at room temperature. The 3,3′-diaminobenzidine (DAB) solution was prepared as per guidelines (ZytoFast PLUS CISH; ZytoVision GmbH) and 50 μL was poured over each slide for 10 min at room temperature. After washing, hematoxylin–eosin was used for counterstaining (see below for details). Slides were dehydrated in graded ethanol solutions, air dried and mounted with a mixture of distyrene, plasticizer, and xylene (DPX). Slides were examined under a light microscope.
RNA interference was performed to analyze the novel regulatory roles of ferritin in the mechanism of sexual development in male M. nipponense. A specific RNAi primer with a T7 promoter site was designed in the open reading frame of Mn-ferritin, using Snap Dragon tools1 (Table 1). The Mn-ferritin dsRNA was synthesized using the Transcript Aid™ T7 High Yield Transcription kit (Fermentas Inc., Burlington, ON, Canada), based on the manufacturer’s protocol. Six hundred healthy, mature, male M. nipponense with a bodyweight of 2.11–2.78 g were collected approximately 5 months after hatching from Tai Lake in Wuxi, China (120°13′44″E, 31°28′22″N). These male prawns were randomly divided into the RNAi group and 0.9% saline group with each group containing 300 prawns; 0.9% saline group was considered as the negative control. As described in a previous study (Jiang et al., 2014; Li et al., 2018), prawns from the RNAi group were injected with 4 μg/g Mn-ferritin dsRNA. Prawns from the control group were injected with an equal volume of 0.9% saline, according to the prawn’s body weight. Androgenic gland samples were collected from both the control group and the RNAi group on days 1, 7, and 14, after 0.9% saline and Mn-ferritin dsRNA injection, and the Mn-ferritin mRNA expression was measured by qPCR, permitting confirmation of silencing efficiency. The androgenic gland samples were collected from five individual prawns and pooled together to form a biological replicate, and five biological replicates were performed. The mRNA expression of Mn-IAG was measured using the same cDNA templates in order to analyze the regulatory relationship between Mn-ferritin and Mn-IAG.
Testes were collected from the both control group and the RNAi group 1, 7, and 14 days after the injection of 0.9% saline and Mn-ferritin dsRNA, and stored at −20°C. Testes were collected from forty individual prawns at each time point in both control and RNAi groups. Eight prawns were pooled together to form one biological replicate, in order to produce a total tissue sample weight of 0.2 g, and five biological replicates were performed. The testosterone was extracted from the testes by adding 5 mL 100% methyl alcohol and fully grinding the sample. The ground samples were kept at 4°C for 4 h and centrifuged at 3,000 rpm for 5 min. The supernatant was collected and directly used to measure the content of testosterone. The testosterone concentration in triplicate samples was measured using an Access 2 Immunoassay System (Beckman Coulter, Inc., Brea, CA, United States) (Jin et al., 2019).
Hematoxylin and eosin staining was used to measure the morphological differences of the testis between the control group and RNAi group. The procedure of HE staining has been well described in previous studies (ShangGuan et al., 1991; Ma et al., 2006). Briefly, the tissues were first dehydrated by using 50, 70, 80, 95, and 100% ethanol. The tissues were then made transparent and embedded using alcohol: xylene (1:1), xylene, xylene: wax (1:1), and wax. The embedded tissues were sectioned to a thickness of 5 μm using a slicer (Leica, Wetzlar, Germany), and placed on a slide. The slides were then stained with HE for 3–8 min. The slides were observed under an Olympus SZX16 microscope (Olympus Corporation, Tokyo, Japan).
SPSS Statistics 23.0 (IBM, Armonk, NY, United States) was used to conduct all statistical analyses. The test of homogeneity of variances was performed prior to ANOVA analysis and t-test (Sig. >0.05). Meanwhile, a linear regression analysis was performed on each set of data. The mean residual of each group data is close to 0, and the residual deviation is close to 1 for the linear regression analysis, indicating that the residuals of the data are normally distributed and can be analyzed. Statistical differences were identified by ANOVA analysis of variance followed by the least significant difference and Duncan’s multiple range tests. The statistical difference between the control group and the RNAi group on the same day was assessed using the paired t-test. Quantitative data were expressed as mean ± standard deviation. A P-value < 0.05 was considered to be statistically significant.
The physiological function of Mn-ferritin was reflected by tissue distribution in M. nipponense, verified by qPCR (Figure 1A). According to qPCR analysis, Mn-ferritin showed the highest expression level in the hepatopancreas (P < 0.05), followed by the testis and androgenic gland. The intestine had the lowest expression level. The mRNA expressions of Mn-ferritin in the testis and androgenic gland were over five times higher than that of the intestine (P < 0.05).
Figure 1. qPCR analysis of Mn-ferritin in different tissues and developmental stages. The amount of Mn-ferritin mRNA was normalized to the EIF transcript level. Data are shown as mean ± SD (standard deviation) of tissues from five biological replicates. Letters indicate expression differences between different samples. Highest expression level of Mn-ferritin was observed in the hepatopancreas, followed by the testis and androgenic gland. Mn-ferritin expression was generally higher during the post-larval developmental stages than the larval developmental stages, and the expressions remained at a high level from PL5 to PL15. Mn-ferritin expression level in the testis of the non-reproductive season was significantly higher than that in the testis of the reproductive season. (A) Expression characterization in different tissues. (B) Expression characterization in different developmental stages. (C) Expression characterization in different reproductive seasons of testis.
During the different developmental stages, Mn-ferritin expression was generally higher during the post-larval developmental stages than the larval developmental stages. Mn-ferritin expression remained stable during the larval developmental stages, with no significant difference in expression levels (P > 0.05). Mn-ferritin expression peaked during the post-larval developmental stage (PL) at PL5 (P < 0.05) and remained at a high level until PL15 (Figure 1B). Mn-ferritin expression level in the testis of the non-reproductive season was 2.43-fold higher than that in the testis of the reproductive season (P < 0.05) (Figure 1C).
In situ hybridization was used to determine the mRNA locations of Mn-Ferritin in different tissues. According to the HE staining, the cell types in hepatopancreas were secretory cells, basement membrane, lumen, storage cells and transferred vacuoles, and strong DIG signals were also observed in these cells (Figure 2). Sperm was the dominant cell type in the testis of the reproductive season, as well as a small number of spermatogonia and spermatocytes. However, the dominant cell types in the testis of the non-reproductive season were spermatogonia and spermatocytes. The DIG signals in the testis of the non-reproductive season were stronger than that in the reproductive season. Androgenic gland cells and funicular structures were observed in the androgenic gland (Figure 2). Strong DIG signals for Mn-ferritin were only observed in spermatogonia in the testis of M. nipponense, revealed by in situ hybridization analysis. No DIG signals were observed in spermatocytes and sperm. In the androgenic gland, no DIG signal was directly observed in the androgenic gland cells. In addition, strong DIG signals were observed in the funicular structure surrounding the androgenic gland cells (Figure 2). No DIG signals were observed when the sense RNA probe was used.
Figure 2. In situ hybridization analysis of ferritin in M. nipponense. In situ hybridization analyses of ferritin were performed in hepatopancreas, testis, and androgenic gland. AG, androgenic gland; AGC, androgenic gland cells; B, secretory cells of type B; BM, basement membrane; CT, connective tissue; FS, funicular structure; He, hepatopancreas; L, lumen; M, muscle; R, storage cells of type R; T (NRS), testis of the non-reproductive season; T (RS), testis of the reproductive season; TV, transferred vacuoles; S, sperm; SC, spermatocyte; SG, spermatogonia. Scale bars = 20 μm. The positively stained cells with DIG signals in antisense labels are characterized by “brown color”. Strong DIG signals were observed in all cell types of the hepatopancreas. Strong DIG signal was only observed in the spermatogonia in the testis of the non-reproductive season and reproductive season. Strong DIG signal was also observed in the funicular structure, surrounding the androgenic gland cells.
This study aimed to analyze the potentially novel functions of Mn-ferritin during sexual development in male M. nipponense using RNAi. Male prawns were used for RNAi analysis. qPCR analysis revealed that Mn-ferritin expression remained at a stable level on different days after the treatment of 0.9% saline (P > 0.05). However, Mn-ferritin expressions were dramatically decreased after the treatment with Mn-ferritin dsRNA. Mn-ferritin expressions decreased over 90% at day 7 and day 14 after the Mn-ferritin dsRNA injection, compared with that of the 0.9% saline injection on the same day (P < 0.01) (Figure 3A).
Figure 3. Expression characterization of Mn-ferritin and Mn-IAG, and measurement of the contents of testosterone at different days after Mn-ferritin dsRNA injection. The amount of Mn-ferritin and Mn-IAG mRNA was normalized to the EIF transcript level. Data are shown as mean ± SD (standard deviation) of tissues from five biological replicates. Lowercases indicated significant expression difference among different time points in the same treated group and capital letters indicated the significant difference between the RNAi group and control group on the same day after treatment (P < 0.05). The Mn-ferritin expressions, Mn-IAG expressions, and the contents of testosterone showed slight differences on different days after the injection of 0.9% saline (P > 0.05). However, the Mn-ferritin expressions, Mn-IAG expressions, and the contents of testosterone were significantly decreased on different days after the injection of Mn-ferritin dsRNA, and showed significant differences on days 7 and 14 (P < 0.01), compared to those of 0.9% saline injection. (A) Expression characterization of Mn-ferritin after Mn-ferritin dsRNA injection. (B) Expression characterization of Mn-IAG after Mn- ferritin dsRNA injection. (C) Measurement of the contents of testosterone after Mn-ferritin dsRNA injection.
Mn-IAG expression was measured using the same cDNA template after the injection of Mn-ferritin dsRNA. The qPCR analysis revealed that there were no significant differences in Mn-IAG expression on different days after the injection of 0.9% saline (P > 0.05). However, the Mn-IAG expressions in the RNAi group decreased with decreasing Mn-Ferritin. The Mn-IAG expression on day 1 in the RNAi group only decreased by 25%, compared with the injection of 0.9% saline on the same day. However, expression levels decreased by over 60% on days 7 and 14 and showed significant differences with the injection of 0.9% saline on the same day (P < 0.01) (Figure 3B).
Testosterone levels were measured on days 1, 7, and 14 after Mn-ferritin dsRNA injection. Testosterone levels significantly decreased (up to 60%) on days 7 and 14 in the RNAi group, compared with that of 0.9% saline injection on the same day (P < 0.01) (Figure 3C).
There were no significant differences in the testis on different days in the control group. Sperm cells were the dominant cell type in the testis of the 0.9% saline injection. However, the number of sperm gradually decreased from day 1 to 14 after the Mn-ferritin dsRNA injection. The numbers of spermatogonia and spermatocytes were much higher than the number of sperm on day 7 in the RNAi group, and sperm was rarely recorded on day 14 in the RNAi group (Figure 4).
Figure 4. Histological observations of testis between 0.9% saline and Mn-ferritin dsRNA treated group by HE staining. Sperm was the dominant cell type in the testis of 0.9% saline group, while the number of sperm gradually decreased from day 1 to 14 after the Mn-ferritin dsRNA injection. CT, connective tissue; S, sperm; SC, spermatocyte; SG, Spermatogonia. Scale bars = 20 μm.
Ferritin has been confirmed to be involved in multiple physiological function regulation, such as development, cell activation, and angiogenesis (Parthasarathy et al., 2002; Coffman et al., 2008; Alkhateeb and Connor, 2010; Wang et al., 2010). Recent studies showed that it was differentially expressed in the proteomic profiling analysis of the androgenic gland between M. nipponense in reproductive season and non-reproductive season (Jin et al., 2018a). In addition, ferritin also showed a higher expression level in the androgenic gland than that of the testis and ovaries, verified by qPCR (Jin et al., 2018a). Thus, ferritin was predicted to be involved in the mechanism of male sexual development in M. nipponense, due to the positive regulatory role of the androgenic gland in male sexual development in crustacean species (Jin et al., 2018a). In the present study, we aimed to further investigate the potentially novel functions of ferritin in the mechanism of male sexual development of M. nipponense.
The tissue distributions of ferritin showed different expression patterns in various species. Ferritin exhibited the highest expression in the hemocytes of L. vannamei (Hsieh et al., 2006), while Pacifastacus leniusculus had the highest expression levels of ferritin in the hepatopancreas (Huang et al., 1996). In the current study, ferritin also showed the highest expression level in the hepatopancreas of M. nipponense, followed by the testis and the androgenic gland, whereas expression in the ovary was relatively low. Similar results were also obtained in M. rosenbergii (Qiu et al., 2008). However, the prawn hepatopancreas was not responsive to iron injections. Thus, the high expression of ferritin in the hepatopancreas could be regulated at the post-transcriptional level (Huang et al., 1999). Previous studies revealed that ferritin also showed the highest expression levels in the hepatopancreas, and the levels were upregulated after iron and pathogen challenge in M. nipponense, indicating that ferritin played essential roles in organismic and cellular iron homeostasis of M. nipponense, protecting the cells from damage by excess iron (Sun et al., 2014). In addition, the expressions of Mn-ferritin were higher in the testis and the androgenic gland than the other tested tissues (P < 0.05), except hepatopancreas in this study. This indicates that ferritin may have additional functions in the mechanism of male sexual development in M. nipponense, based on the essential roles of the testis and the androgenic gland on male sexual development in crustacean species. Furthermore, this indicates that iron homeostasis may mediate gonad development in M. nipponense. The Mn-ferritin expression in the testis was slightly higher than that in the androgenic gland in this study (P > 0.05), which is different from the previous study. A reasonable explanation for the difference is that the testis development is continuous. Thus, the cell numbers of spermatogonium, spermatocyte, and sperm in the testis of this study may be different from those in the previous study.
The ferritin expression has shown dramatic differences during different developmental stages of aquatic species. During continuous developmental stages, ferritin was expressed in the zoea stage, as well as the megalopa and juvenile crab I stage in Scylla paramamosain (Zhang et al., 2011). The ferritin of Exopalaemon carinicauda was rarely expressed at the gastrula and zoea stage, whereas it was significantly upregulated from the egg protozoea stage, and showed the highest expression level at the post-larvae stages (Zhang et al., 2015). In the present study, the expression level of ferritin remained stable and low during the larval developmental stages of M. nipponense, compared to those of the post-larval developmental stages. During the post-larval developmental stages, Mn-ferritin expression increased dramatically, peaked at PL5, and remained at a high level until PL15. Mn-ferritin exhibited higher expression levels during the post-larval developmental stages than the larval developmental stages. Histological observations during the larval and post-larval developmental stages of M. nipponense have proven that the sex-differentiation sensitive period of M. nipponense is from PL7 to PL22 (Jin et al., 2016), during which time the androgenic gland, testis, and ovary differentiated and matured. The dramatically high expression levels from PL5 to PL15 indicated that ferritin may play vital roles in gonad differentiation and development in M. nipponense.
The in situ hybridization analysis of ferritin has been reported for several species. A ferritin homolog is ubiquitously expressed in Branchiostoma belcheri (Li et al., 2008). Ferritin mRNA is highly expressed in the mantle fold of Pinctada fucata (Zhang et al., 2003). L-ferritin mRNA was observed to be upregulated in iron-loaded rats. L-ferritin mRNA was localized in the colonic crypt, villus epithelial cells, small intestinal crypt, and surface epithelial cells (Jeffrey et al., 1996). In the present study, strong DIG signals for ferritin were observed in all cell types in the hepatopancreas, indicating that the hepatopancreas was the main organ for iron homeostasis in M. nipponense. Interestingly, strong DIG signals were only detected in spermatogonia in the testis of both reproductive season and non-reproductive season, indicating that ferritin may promote spermatogonia development or activate testis development. DIG signals in the testes from the non-reproductive season were stronger than those from the reproductive season. qPCR analysis revealed that Mn-ferritin in the testes of the non-reproductive season was higher than in those of the reproductive season, which is consistent with the in situ hybridization analysis. Histological observations revealed that the main cell type in the testis of the reproductive season was sperm, while spermatogonia and spermatocytes were the dominant cell type in the testis of the non-reproductive season (Jin et al., 2020). This result further confirmed that ferritin promoted spermatogonia development. Our data also showed that strong DIG signals were detected in the funicular structure surrounding the androgenic gland cells, while no signal was directly detected in the androgenic gland cells. Previous research indicated that the androgenic gland development began with the formation of the funicular structure, then androgenic gland cells were formed and filled the funicular structure (Jin et al., 2016). The in situ hybridization of ferritin in the androgenic gland of M. nipponense suggested ferritin played essential roles in the development of the funicular structure, most likely supporting the formation of androgenic gland cells.
RNA interference was efficiently used to analyze the gene functions through knockdown of the gene expression of target genes, which has been widely used in M. nipponense (Jiang et al., 2014; Li et al., 2015a,2018). In this study, Mn-ferritin expression levels on days 7 and 14 in the RNAi group were significantly lower than the control group on the same day, indicating the dsRNA can efficiently inhibit Mn-ferritin expression. We also found that, after the injection of Mn-ferritin dsRNA, the Mn-IAG expressions decreased with decreasing Mn-ferritin expression, indicating that ferritin positively regulated IAG expression in M. nipponense. IAG has been reported to positively promote the male differentiation and development in crustacean species (Ventura et al., 2009, 2011). RNAi of IAG showed a significant inhibitory effect on male sexual differentiation and development of secondary sexual characteristics, including the formation of spermatogenesis in M. rosenbergii (Ventura et al., 2012). IAG has been proven to be expressed in the androgenic gland of M. nipponense (Ma et al., 2016). Furthermore, the expressions of IAG were regulated by the expressions of crustacean hyperglycemic hormone, gonad inhibiting hormone, and molting inhibiting hormone in M. nipponense (Li et al., 2015b). Our data also revealed that the testosterone levels in the RNAi group were lower than that of control group on the same day. Testosterone has been proven to result in sex reversal in Oreochromis mossambicus (Cao et al., 1994), allogynogenetic crucian carp (Carassius auratus) (Lou et al., 1994; Zhang et al., 2000), Xiphophorus hellerii (Han et al., 2010) and the grouper Epinephelus akaara (Li et al., 2006). In addition, testosterone promotes the development of testes and sperm in Eriocheir sinensis, and is involved in early gonad differentiation and development in M. nipponense (Jin et al., 2016). The positive regulatory roles between the expression of ferritin and IAG, and the secretion of testosterone indicated that ferritin was involved in the mechanism of male sexual development in M. nipponense. Histological observations of testis indicated that the number of sperm decreased dramatically after injection of Mn-ferritin dsRNA. Sperm was rarely found on day 14 after the injection of Mn-ferritin dsRNA. This indicates that ferritin was involved in the regulation of testis development in M. nipponense by inhibiting the expression of Mn-IAG and the secretion of testosterone, indicating iron homeostasis was involved in the process of testis development in M. nipponense.
We proved that ferritin played an essential role in the sexual development of male M. nipponense. Ferritin exhibited a positive regulation in M. nipponense testis development by regulating Mn-IAG expression and the secretion of testosterone, indicating that iron homeostasis was also involved in the testis development of M. nipponense. The present study provided an essential basis for the future establishment of novel techniques to regulate testis development in M. nipponense.
The original contributions presented in the study are included in the article/supplementary material, further inquiries can be directed to the corresponding author/s.
The animal study was reviewed and approved by the Institutional Animal Care and Use Ethics Committee of the Freshwater Fisheries Research Center, Chinese Academy of Fishery Sciences (Wuxi, China). Written informed consent was obtained from the owners for the participation of their animals in this study.
SBJ designed and wrote the manuscript. HF supervised the experiment. SFJ provided the experimental prawns. YX performed the qPCR analysis. HQ performed the in situ hybridization analysis. WZ performed the RNAi analysis. YG performed the histological observations. YW revised the manuscript. All authors contributed to the article and approved the submitted version.
This research was supported by grants from the Central Public-Interest Scientific Institution Basal Research Fund, CAFS (2021JBFM02 and 2020TD36), National Key R&D Program of China (2018YFD0900201), Jiangsu Agricultural Industry Technology System (JATS[2020]461), China Agriculture Research System-48 (CARS-48), and New Cultivar Breeding Major Project of Jiangsu Province (PZCZ2 01745).
The authors declare that the research was conducted in the absence of any commercial or financial relationships that could be construed as a potential conflict of interest.
All claims expressed in this article are solely those of the authors and do not necessarily represent those of their affiliated organizations, or those of the publisher, the editors and the reviewers. Any product that may be evaluated in this article, or claim that may be made by its manufacturer, is not guaranteed or endorsed by the publisher.
Alkhateeb, A. A., and Connor, J. R. (2010). Nuclear ferritin: a new role for ferritin in cell biology. Biochim. Biophys. Acta 1800, 793–797. doi: 10.1016/j.bbagen.2010.03.017
Cai, Y., and Shokita, S. (2006). Report on a collection of freshwater shrimps (Crustacea: Decapoda: Caridea) from the Philippines, with descriptions of four new species. Raffles Bull. Zool. 54, 245–270.
Cao, L. Q., Tao, Y. G., and Tan, H. Z. (1994). Studies on unisexualization in Red Tilapia. J. Fish. China 18, 75–80.
Coffman, L. G., Brown, J. C., Johnson, D. A., Parthasarathy, N., D’Agostino, R. B. Jr., Lively, M. O., et al. (2008). Cleavage of high-molecular-weight kininogen by elastase and tryptase is inhibited by ferritin. Am. J. Physiol. Lung Cell. Mol. Physiol. 294, L505–L515. doi: 10.1152/ajplung.00347.2007
Fu, H. T., Jiang, S. F., and Xiong, Y. W. (2012). Current status and prospects of farming the giant river prawn (Macrobrachium rosenbergii) and the oriental river prawn (Macrobrachium nipponense) in china. Aquac. Res. 43, 993–998. doi: 10.1111/j.1365-2109.2011.03085.x
Han, J., Xu, Y., Yu, Y. M., Xu, L. N., and Fang, Z. Q. (2010). Effects of 17 alpha-methyltestosterone on the reversal of male resistance in Viviparous Swordtail Fish (Xiphophorus helleri). Acta Lab. Anim. Sci. Sin. 18, 484–488.
Hsieh, S. L., Chiu, Y. C., and Kuo, C. M. (2006). Molecular cloning and tissue distribution of ferritin in Pacific white shrimp (Litopenaeus vannamei). Fish Shellfish Immunl. 21, 279–283. doi: 10.1016/j.fsi.2005.12.003
Hu, Y. N., Fu, H. T., Qiao, H., Sun, S. M., Zhang, W. Y., Jin, S. B., et al. (2018). Validation and evaluation of reference genes for Quantitative real-time PCR in Macrobrachium nipponense. Int. J. Mol. Sci. 19:2258. doi: 10.3390/ijms19082258
Huang, T. S., Law, J. H., and Soderhall, K. (1996). Purification and cDNA cloning of ferritin from the hepatopancreas of the freshwater crayfish Pacifastacus leniusculus. Eur. J. Endocrinol. 236, 450–456. doi: 10.1111/j.1432-1033.1996.00450.x
Huang, T. S., Melefors, O., Lind, M. I., and Soderhall, K. (1999). An atypical iron-responsive element (IRE) within crayfish ferritin mRNA and an iron regulatory protein 1 (IRP1)-like protein from crayfish hepatopancreas. Insect Biochem. Mol. Biol. 29, 1–9. doi: 10.1016/s0965-1748(98)00097-6
Huang, X. S., Ye, H. H., Huang, H. Y., Yang, Y. N., and Gong, J. (2014). An insulin-like androgenic gland hormone gene in the mud crab, Scylla paramamosain, extensively expressed and involved in the processes of growth and female reproduction. Gen. Comp. Endocrinol. 204, 229–238. doi: 10.1016/j.ygcen.2014.06.002
Jeffrey, G. P., Basclain, K. A., and Allen, T. L. (1996). Molecular regulation of transferrin receptor and ferritin expression in the rat gastrointestinal tract. Gastroenterology. 110, 790–800. doi: 10.1053/gast.1996.v110.pm8608889
Jiang, F. W., Fu, H. T., Qiao, H., Zhang, W. Y., Jiang, S. F., Xiong, Y. W., et al. (2014). The RNA interference regularity of transformer-2 gene of oriental river Prawn Macrobrachium nipponense. Chin. Agric. Sci. Bul. 30, 32–37.
Jin, S. B., Fu, H. T., Jiang, S. F., Xiong, Y. W., Qiao, H., Zhang, W. Y., et al. (2015). Identification of androgenic gland microRNAs and their targeted genes for discovery of sex-related microRNAs in oriental river prawn, Macrobrachium nipponense. Genet. Mol. Res. 14, 18396–18406. doi: 10.4238/2015.December.23.27
Jin, S. B., Fu, H. T., Zhou, Q., Sun, S. M., Jiang, S. F., Xiong, Y. W., et al. (2013). Transcriptome analysis of androgenic gland for discovery of novel genes from the Oriental River Prawn, Macrobrachium nipponense, using Illumina Hiseq 2000. PLoS One 8:e76840. doi: 10.1371/journal.pone.0076840
Jin, S. B., Hu, Y. N., Fu, H. T., Jiang, S. F., Xiong, Y. W., Qiao, H., et al. (2019). Potential functions of gem-associated protein 2-like isoform X1 in the Oriental River Prawn Macrobrachium nipponense: cloning, qPCR, In Situ Hybridization, and RNAi Analysis. Int. J. Mol. Sci. 20:3995. doi: 10.3390/ijms20163995
Jin, S. B., Hu, Y. N., Fu, H. T., Sun, S. M., Jiang, S. F., Xiong, Y. W., et al. (2020). Analysis of testis metabolome and transcriptome from the oriental river prawn (Macrobrachium nipponense) in response to different temperatures and illumination times. Comp. Biochem. Phys. D 34:100662. doi: 10.1016/j.cbd.2020.100662
Jin, S. B., Jiang, S. F., Xiong, Y. W., Qiao, H., Sun, S. M., Zhang, W. Y., et al. (2014). Molecular cloning of two tropomyosin family genes and expression analysis during development in oriental river prawn, Macrobrachium nipponense. Gene 546, 390–397. doi: 10.1016/j.gene.2014.05.014
Jin, S. B., Zhang, Y., Guan, H. H., Fu, H. T., Jiang, S. F., Xiong, Y. W., et al. (2016). Histological observation of gonadal development during post-larva in oriental river prawn, Macrobrachium nipponense. Chin. J. Fish. 29, 11–16.
Jin, S. B., Hu, Y. N., Fu, H. T., Jiang, S. F., Xiong, Y. W., Qiao, H., et al. (2021a). Identification and characterization of the succinate dehydrogenase complex iron sulfur subunit B gene in the Oriental River Prawn, Macrobrachium nipponense. Front. Genet. 12:698318. doi: 10.3389/fgene.2021.698318
Jin, S. B., Fu, H. T., Sun, S. M., Jiang, S. F., Xiong, Y. W., Gong, Y. S., et al. (2018b). iTRAQ-based quantitative proteomic analysis of the androgenic glands of the oriental river prawn, Macrobrachium nipponense, during nonreproductive and reproductive seasons. Comp. Biochem. Phys. D 26, 50–57. doi: 10.1016/j.cbd.2018.03.002
Jin, S. B., Fu, H. T., Jiang, S. F., Xiong, Y. W., Sun, S. M., Qiao, H., et al. (2018a). Molecular cloning, expression, and in Situ Hybridization analysis of forkhead box protein L2 during development in Macrobrachium nipponense. J. World Aquacul. Soc. 49, 429–440.
Jin, S. B., Hu, Y. N., Fu, H. T., Jiang, S. F., Xiong, Y. W., Qiao, H., et al. (2021b). Identification and Characterization Of The Pyruvate Dehydrogenase E1 gene in the Oriental River Prawn, Macrobrachium nipponense. Front. Endocrinol. 12:752501. doi: 10.3389/fendo.2021.752501
Kakhlon, O., Gruenbaum, Y., and Cabantchik, Z. I. (2001). Repression of the heavy ferritin chain increases the labile iron pool of human K562 cells. Biochem. J. 356, 311–316. doi: 10.1042/0264-6021:3560311
Konijn, A. M., Glickstein, H., Vaisman, B., Meyron-Holtz, E. G., Slotki, I. N., and Cabantchik, Z. I. (1999). The cellular labile iron pool and intracellular ferritin in K562 cells. Blood 94, 2128–2134.
Li, F., Qiao, H., Fu, H. T., Sun, S. M., Zhang, W. Y., Jin, S. B., et al. (2018). Identification and characterization of opsin gene and its role in ovarian maturation in the oriental river prawn Macrobrachium nipponense. Comp. Biochem. Phys. B 218, 1–12. doi: 10.1016/j.cbpb.2017.12.016
Li, G. L., Liu, X. C., and Lin, H. R. (2006). Effects of 17α-methyltestosterone on sex reversal in red-spotted grouper, Epinephelus akaara. J Fish China 30, 145–150.
Li, M., Saren, G. W., and Zhang, S. C. (2008). Identification and expression of a ferritin homolog in amphioxus Branchiostoma belcheri: evidence for its dual role in immune response and iron metabolism. Comp. Biochem. Phys. B 150, 263–270. doi: 10.1016/j.cbpb.2008.03.014
Li, S. H., Li, F. H., Sun, Z., and Xiang, J. H. (2012). Two spliced variants of insulin-like androgenic gland hormone gene in the Chinese shrimp, Fenneropenaeus chinensis. Gen. Comp. Endocrinol. 177, 246–255. doi: 10.1016/j.ygcen.2012.04.010
Li, F. J., Bai, H. K., Xiong, Y. W., Fu, H. T., Jiang, F. W., Jiang, S. F., et al. (2015a). Molecular characterization of insulin-like androgenic gland hormone-binding protein gene from the oriental river prawn Macrobrachium nipponense and investigation of its transcriptional relationship with the insulin-like androgenic gland hormone gene. Gen. Comp. Endocr. 216, 152–160. doi: 10.1016/j.ygcen.2014.12.007
Li, F. J., Bai, H. K., Zhang, W. Y., Fu, H. T., Jiang, F. W., Liang, G. X., et al. (2015b). Cloning of genomic sequences of three crustacean hyperglycemic hormone superfamily genes and elucidation of their roles of regulating insulin-like androgenic gland hormone gene. Gene. 561, 68–75. doi: 10.1016/j.gene.2015.02.012
Liu, F., Shi, W., Ye, H., Liu, A., and Zhu, Z. (2021). RNAi reveals role of insulin-like androgenic gland hormone 2 (IAG2) in sexual differentiation and growth in hermaphrodite shrimp. Front. Mar. Sci. 8:666763.
Livak, K. J., and Schmittgen, T. D. (2001). Analysis of relative gene expression data using real-time quantitative PCR and the 2-ΔΔCT method. Methods 25, 402–408.
Lou, Y. D., Song, T. F., Wang, Y. M., Wei, H., Wu, M. C., Xu, H. M., et al. (1994). Studies on sex control in allogynogenetic crucian carp. J Fish China 3, 169–176.
Ma, K. Y., Feng, J. B., Lin, J. Y., and Li, J. L. (2011). The complete mitochondrial genome of Macrobrachium nipponense. Gene 487, 160–165. doi: 10.1016/j.gene.2011.07.017
Ma, K. Y., Li, J. L., and Qiu, G. F. (2016). Identification of putative regulatory region of insulin-like androgenic gland hormone gene (IAG) in the prawn Macrobrachium nipponense and proteins that interact with IAG by using yeast two-hybrid system. Gen. Comp. Endocr. 229, 112–118. doi: 10.1016/j.ygcen.2016.03.019
Ma, X. K., Liu, X. Z., Wen, H. S., Xu, Y. J., and Zhang, L. J. (2006). Histological observation on gonadal sex differentiation in Cynoglossus semilaevis Günther. Mar. Fish. Res. 27, 55–61.
Parthasarathy, N., Torti, S. V., and Torti, F. M. (2002). Ferritin binds to light chain of human Hkininogen and inhibits kallikrein-mediated bradykinin release. Biochem. J. 365, 279–286. doi: 10.1042/BJ20011637
Picard, V., Renaudie, F., Porcher, C., Hentze, M. W., Grandchamp, B., and Beaumont, C. (1996). Overexpression of the ferritin H subunit in cultured erythroid cells changes the intracellular iron distribution. Blood 87, 2057–2064. doi: 10.1182/blood.v87.5.2057.bloodjournal8752057
Qiu, G. F., Zheng, L., and Liu, P. (2008). Transcriptional regulation of ferritin mRNA levels by iron in the freshwater giant Prawn, Macrobrachium rosenbergii. Comp. Biochem. Phys. B 150, 320–325. doi: 10.1016/j.cbpb.2008.03.016
Rosen, O., Manor, R., Weil, S., Gafni, O., Linial, A., Aflalo, E. D., et al. (2010). A sexual shift induced by silencing of a single insulin-like gene in crayfish: ovarian upregulation and testicular degeneration. PLoS One 5:e15281. doi: 10.1371/journal.pone.0015281
Sagi, A., Cohen, D., and Milner, Y. (1990). Effect of androgenic gland ablation on morphotypic differentiation and sexual characteristics of male freshwater prawns, Macrobrachium rosenbergii. Gen. Comp. Endocr. 77, 15–22. doi: 10.1016/0016-6480(90)90201-v
Sagi, A., Cohen, D., and Wax, Y. (1986). Production of Macrobrachium rosenbetgii in momosex population: yield characteristes under intensive monoculture conditions in cages. Aquaculture. 51, 265–275.
Salman, S. D., Page, T. J., Naser, M. D., and Yasser, A. G. (2006). The invasion of Macrobrachium nipponense (De Haan, 1849) (Caridea: Palaemonidae) into the southern Iraqi marshes. Aquat. Invasions 1, 109–115.
ShangGuan, B. M., Liu, Z. Z., and Li, S. Q. (1991). Histological Studies on Ovarian Development in Scylla serrata. J. Fish. Sci. China 15, 96–103.
Sun, S. M., Gu, Z. M., Fu, H. T., Zhu, J., Xuan, F. J., and Ge, X. P. (2014). Identification and characterization of a Macrobrachium nipponense ferritin subunit regulated by iron ion and pathogen challenge. Fish Shellfish Immunol. 40, 288–295. doi: 10.1016/j.fsi.2014.07.002
Torti, F. M., and Torti, S. V. (2002). Regulation of ferritin genes and protein. Blood 99, 3505–3516. doi: 10.1182/blood.v99.10.3505
Ventura, T., Manor, R., Aflalo, E. D., Weil, S., Khalaila, I., Rosen, O., et al. (2011). Expression of an androgenic gland-specific insulin-like peptide during the course of prawn sexual and morphotypic differentiation. ISRN Endocrinol. 2011:476283. doi: 10.5402/2011/476283
Ventura, T., Manor, R., Aflalo, E. D., Weil, S., Raviv, S., Glazer, L., et al. (2009). Temporal silencing of an androgenic gland-specific insulin-like gene affecting phenotypical gender differences and spermatogenesis. Endocrinology 150, 1278–1286. doi: 10.1210/en.2008-0906
Ventura, T., Manor, R., Aflalo, E. D., Weil, S., Rosen, O., and Sagi, A. (2012). Timing sexual differentiation: full functional sex reversal achieved through silencing of a single insulin-like gene in the prawn, Macrobrachium rosenbergii. Biol. Reprod. 86:90. doi: 10.1095/biolreprod.111.097261
Wang, W., Knovich, M. A., Coffman, L. G., Torti, F. M., and Torti, S. V. (2010). Serum ferritin: past, present and future. BBA Gen. Subjects 1800, 760–769. doi: 10.1016/j.bbagen.2010.03.011
Zhang, D., Jiang, K. J., Zhang, F. Y., Ma, C. Y., Shi, Y. H., Qiao, Z. G., et al. (2011). Isolation and characterization of a ferritin cDNA from the Mud Crab Scylla paramamosain. J. Crustacean Biol. 31, 345–351.
Zhang, J. Q., Gui, T. S., Wang, J., and Xiang, J. H. (2015). The ferritin gene in ridgetail white prawn Exopalaemon carinicauda: cloning, expression and function. Int. J. Mol. Sci. 72, 320–325. doi: 10.1016/j.ijbiomac.2014.08.036
Zhang, P. Y., Hu, W., Wang, Y. P., and Zhu, Z. Y. (2000). Methyltestosterone induction of sex reversal in allogynogenetic crucian carp. Hereditas 22, 25–27.
Zhang, X. L., Cui, L. F., Li, S. M., Liu, X. Z., Han, X., Jiang, K. Y., et al. (2020). “Bureau of fisheries, Ministry of Agriculture, P.R.C. Fisheries Economic Statistics,” in China Fishery Yearbook, eds X. J. Yu, L. J. Xu, F. X. Wu, D. D. Song, and H. Q. Gao (Beijing: China Agricultural Press), 24.
Zhang, Y., Meng, Q. X., Jiang, T. M., Wang, H. Z., Xie, L. P., and Zhang, R. Q. (2003). A novel ferritin subunit involved in shell formation from the pearl oyster (Pinctada fucata). Comp. Biochem. Phys. B 135, 43–54. doi: 10.1016/s1096-4959(03)00050-2
Keywords: Macrobrachium nipponense, ferritin peptide, male sexual development, RNAi, testosterone
Citation: Jin S, Fu H, Jiang S, Xiong Y, Qiao H, Zhang W, Gong Y and Wu Y (2022) RNA Interference Analysis Reveals the Positive Regulatory Role of Ferritin in Testis Development in the Oriental River Prawn, Macrobrachium nipponense. Front. Physiol. 13:805861. doi: 10.3389/fphys.2022.805861
Received: 31 October 2021; Accepted: 25 January 2022;
Published: 18 February 2022.
Edited by:
Heinrich Dircksen, Stockholm University, SwedenReviewed by:
Gloria Yepiz-Plascencia, Centro de Investigación en Alimentación y Desarrollo, Consejo Nacional de Ciencia y Tecnología (CONACYT), MexicoCopyright © 2022 Jin, Fu, Jiang, Xiong, Qiao, Zhang, Gong and Wu. This is an open-access article distributed under the terms of the Creative Commons Attribution License (CC BY). The use, distribution or reproduction in other forums is permitted, provided the original author(s) and the copyright owner(s) are credited and that the original publication in this journal is cited, in accordance with accepted academic practice. No use, distribution or reproduction is permitted which does not comply with these terms.
*Correspondence: Hongtuo Fu, ZnVodEBmZnJjLmNu, orcid.org/000-0003-2974-9464
Disclaimer: All claims expressed in this article are solely those of the authors and do not necessarily represent those of their affiliated organizations, or those of the publisher, the editors and the reviewers. Any product that may be evaluated in this article or claim that may be made by its manufacturer is not guaranteed or endorsed by the publisher.
Research integrity at Frontiers
Learn more about the work of our research integrity team to safeguard the quality of each article we publish.