- 1Yancheng Institute of Technology, Yancheng, China
- 2School of Biotechnology, Jiangsu University of Science and Technology, Zhenjiang, China
- 3Department of Biological Sciences, Biomolecular Sciences Institute, Florida International University, Miami, FL, United States
- 4Heilongjiang Provincial Key Laboratory of Cold Water Fish Germplasm Resources and Aquaculture, Heilongjiang River Fisheries Research Institute, Chinese Academy of Fishery Sciences, Harbin, China
- 5Yantai Marine Economic Research Institute, Yantai, China
- 6Laboratory of Fish Immunology and Nutrigenomics, Applied Animal and Aquatic Sciences Research Unit, Division of Fisheries, Faculty of Technology, Mahasarakham University, Maha Sarakham, Thailand
The aquaculture industry is vital in providing a valuable protein food source for humans, but generates a huge amount of solid and dissolved wastes that pose great risks to the environment and aquaculture sustainability. Suspended solids (in short SS), one of the aquaculture wastes, are very difficult to be treated due to their high organic contents. The bioconversion from wastewater, food effluents, and activated sludge into poly-β-hydroxybutyrate (PHB) is a sustainable alternative to generate an additional income and could be highly attractive to the agricultural and environmental management firms. However, little is known about its potential application in aquaculture wastes. In the present study, we first determined that 7.2% of SS was PHB. Then, the production of PHB was increased two-fold by the optimal fermentation conditions of wheat bran and microbial cocktails at a C/N ratio of 12. Also, the PHB-enriched SS showed a higher total ammonia nitrogen removal rate. Importantly, we further demonstrated that the PHB-enriched SS as a feed could promote fish growth and up-regulate the expression of the immune-related genes. Our study developed an eco-friendly and simple approach to transforming problematic SS wastes into PHB-enriched high-quality food for omnivorous fish, which will increase the usage efficiency of SS and provide a cheaper diet for aquatic animals.
1 Introduction
The aquaculture industry provides human society with a nutritional food source and high proteins, vitamins, and macro minerals (Henriksson et al., 2018; Han et al., 2019; Heiderscheidt et al., 2020). During the rapid development of the aquaculture industry, two problems have become increasingly apparent: 1) feeding is expensive and 2) a large amount of waste and discharge is generated. The aquaculture waste, containing high nitrogen and phosphorus, poses enormous environmental risks. In Japan, for example, one ton of pond-raised aqua-fish can generate 0.1 kg phosphorus and 0.8 kg nitrogen which equals to the daily waste produced by 73 people (Bangar et al., 2017). Therefore, aquaculture waste has raised the concerns of environmental scientists and challenges the sustainable development of the aquaculture (Ahmed et al., 2019; Han et al., 2019). Many countries have established standards for wastewater discharge, and strictly require that aquaculture wastes can be emitted into the environment only within the allowable range.
The byproducts of feeding and excretion, such as unconsumed food, undigested components, feces, chemicals, and untapped inputs, are the sources of aquaculture wastes (Kokou & Fountoulaki, 2018; Becke et al., 2020; Gibson et al., 2020). The wastes from aquaculture can be divided into solid wastes and suspended wastes, both contain high organic contents (Olusegun et al., 2016; Gao et al., 2020; Hesni et al., 2020; Naughton et al., 2020; Rosa et al., 2020; Sun et al., 2020). The solid wastes include the settled solids sunk to the bottom of the pond and the suspended solids (in short SS) floating in the culture water. In the traditional aquaculture system, the SS are the majority of waste products, and are challenging to be removed due to their high organic contents (Badiola et al., 2012; Becke et al., 2020). However, the SS must be properly managed to avoid the pollution to the surrounding environment and reduce the harm to aquatic animals (Holm-Nielsen et al., 2009; Kiani et al., 2020). Notably, SS can be fermented by bacteria to remove toxic organic contents for resource recycling (Ge and Champagne, 2017; Das et al., 2018; Amadu et al., 2021). These fast-growing microbes will change the toxic ammonia (NH3) and nitrite (NO2−) into non-toxic nitrate (NO3−) (Lananan et al., 2014; Rommozzi et al., 2020). These ammonium ions and NH3 are produced during aquaculture (Karri et al., 2018; Yun et al., 2019).
The treatments about promoting microbial growth have been successfully applied in wastewater treatment (Ge et al., 2014; Qiu et al., 2020a; Xu M. et al., 2020; Xu X. et al., 2020). It can remove nitrogen and phosphorus from the aquatic environment (Qiu et al., 2020b; Xu M. et al., 2020; Xu X. et al., 2020). It also promotes microbial growth to produce valuable energy alternatives such as bio-fuel, natural antioxidants, food additives, or biopolymers (Meixner et al., 2017; Ge et al., 2018; Jochum et al., 2018; Qiu et al., 2020b). Among these alternatives, popular food additives and biopolymers such as poly-β-hydroxybutyrate (PHB) or polyhydroxyalkanoates (PHAs) are the most significant compounds that have been studied (Amadu et al., 2021). PHB or PHAs are not soluble in water. As intracellular energy storage, they form “inclusion bodies” within the cytoplasm in prokaryotic organisms. The chain of PHB is shorter than that of PHAs (Carpine et al., 2018). PHB is biodegradable, biocompatible, and nontoxic (Hrabak, 1992; Arun et al., 2006; Ben Rebah et al., 2007). A considerable effort has been made to produce PHB or PHAs using various wastes, such as municipal wastewater (Chua et al., 2003), sugar cane molasses (Albuquerque et al., 2010), paper mill wastewater (Bengtsson et al., 2008), and food waste (Venkateswar Reddy et al., 2015). However, transforming aquaculture wastes into PHAs or PHB has not been well-investigated (Krasaesueb et al., 2019).
The biofloc technology is a new strategy to reduce the total ammonia nitrogen (TAN) effectively through heterotrophic microbiota by adjusting the C/N ratio in the culture water via external carbon addition (Avnimelech, 1999; Zhang et al., 2018). It was reported that SS in the biofloc system contains 15–20% PHB (Schryver & Verstraete, 2009; Ruan et al., 2011). The commercial PHB has been used as a dietary supplement to improve the growth, immunity, and disease resistance of aquatic animals (Deng et al., 2014; Defoirdt et al., 2018; Meirong et al., 2018; Qiao et al., 2018, 2020). However, the high prices for commercial PHB and water-insolubility greatly hinder its application in aquaculture.
In this study, we analyzed the PHB content in SS waste from a traditional aquaculture system, and tried to increase the PHB production by adjusting the carbon source, various C/N ratios, and bacterial strains. Furthermore, we evaluated the TAN removal during the PHB-enrichment process, analyzed the effects of PHB-enriched SS on the growth, and innate immunity of an omnivorous fish—gibel carp (Carassius auratus gibelio). This study will provide a good low-cost method to treat and recycle aquaculture solid wastes, and present a potential high-quality fish food and convenient method for PHB application in aquaculture.
2 Materials and Methods
2.1 Suspended Solid Collection and Analysis
Three indoor concrete tanks cultured gibel carps (40.33 ± 5.12 g) at the Yancheng Institute of Technology were randomly selected to acquire SS. Gibel carp was fed with commercial diets (Tongwei Feeding Company, China) to satiation three times (6:30 to 7:00 a.m., 1:30 p.m. to 2:00 p.m., and 7:00 p.m. to 7:30 p.m.) each day under a 12D/12L cycle. The diets contained 32.25% crude protein, 5.90% crude lipid, 1.18% calcium, and 1.23% total phosphorus. Water temperature was maintained at 25.0 ± 2.0°C and dissolved oxygen (DO) kept higher than 5 mg L−1. SS were sampled in situ using the Imhoff cone, that is, culture water from three tanks was withdrawn from the middle of tank into the Imhoff cone. Then, SS were collected from the bottom of the Imhoff cone (Zhang et al., 2018). The obtained SS were used to analyze the basic characterization according to the standard method (APHA, 2012; Krasaesueb et al., 2019), including pH, temperature, concentration of nitrate (NO3−–N), nitrite (NO2−–N), ammonium (NH4+–N), phosphate (PO43-–P), and total phosphorus. Finally, we removed water- and acetone-soluble materials with the following steps: 1) collecting pellets by centrifugation of the SS at 8,000 rpm for 10 min, 2) resuspending the pellet in acetone (2V), 3) collecting pellets again by centrifugation of the SS at 8,000 rpm for 10 min, 4) washing with sterile distilled water, and 5) freeze-drying the pellets to check the PHB content as described below.
2.2 Poly-β-Hydroxybutyrate Content and Characterization in Suspended Solids
2.2.1 Crude Poly-β-Hydroxybutyrate Extraction
The PHB was extracted according to the procedure described by Ruan et al. (2011) with some modifications. In brief, the freeze-dried SS were dissolved in 10% NaClO and disrupted by ultrasonic for 8 min (intermittent 5 s, working 5 s, 4°C), and the precipitate was obtained by centrifugation at 8,000 rpm for 15 min at room temperature. Then, the pellet was washed twice with acetone and sterile distilled water to remove lipids by centrifugation at 8,000 rpm for 10 min. Finally, the crude PHB was obtained after the pellet dried at 50°C for 8 h in an oven.
2.2.2 Poly-β-Hydroxybutyrate Content Analysis
The crude PHB was diluted with chloroform at 1:40 (dry weight of SS:volume of chloroform, g:mL) at 40°C for 11 h, and the pellet was collected by centrifugation, dried at 70°C for 8 h in the oven. Then, the PHB extract was diluted with chloroform at 80°C using a rotary evaporator (Eyela N-1000), and dried to a constant mass. A thin film of pure PHB was obtained and quantified using spectrometric analysis based on the standard curve (Law & Slepecky, 1961). The purity of PHB was analyzed by gas chromatography (GC–MS, Agilent 7890B/5977A) (Albuquerque et al., 2010; Alsafadi & Al-Mashaqbeh, 2017).
2.2.3 Poly-β-Hydroxybutyrate Characterization
The structural and material properties of the pure PHB were compared to a commercial PHB (Aldrich, Sigma). The surface morphology and elements of the pure PHB were observed by the scanning electron microscopy (SEM–EDS). The Fourier-transform infrared (FT-IR) spectra of the pure PHB extract were detected in the range of 400–4,000 cm−1 using a spectrophotometer (FT-IR Thermo is 10) (Naumann et al., 1991; Venkateswar Reddy et al., 2015).
2.3 Bacterial Community Analysis With Poly-β-Hydroxybutyrate Accumulation in Suspended Solids
2.3.1 DNA Extraction and PCR Amplification
Since PHB is synthesized by bacteria and the yield correlates with bacterial diversity closely (Crab et al., 2012; Qiao et al., 2018; Amadu et al., 2021), the bacterial community in SS was investigated by high-throughput sequencing using a MiSeq sequencing platform. In brief, the quadruple SS from each tank were sampled, immediately frozen in liquid nitrogen, and then transferred to −80°C for DNA extraction. Microbial DNA from the SS was extracted using the E.Z.N.A.® soil DNA kit (OMEGA, United States) following the manufacturer’s protocol. PCR was used to amplify the V4–V5 region through primers 907R (5′-CCGTCAATTCMTTTRAGTT T-3′) and 515F (5′-barcode-GTGCCAGCMGCCGCGG-3′) (Qiao et al., 2019b). PCR products were purified using the AxyPrep DNA gel extraction kit (Axygen Biosciences, Union City, CA, United States).
2.3.2 Illumina MiSeq Sequencing
The purified PCR products were first quantified using Qubit®3.0 (Life Invitrogen). About 24 amplicons with different barcodes were mixed equally, and the pooled products were chosen to construct an Illumina Paired–End library according to the Illumina’s genomic DNA library preparation procedure. Finally, the amplicon library was paired-end sequenced (2 × 250) on the MiSeq sequencing platform (Illumina, United States) according to the standard protocols (Sangon Biotech (Shanghai) Co., Ltd.).
2.3.3 Bioinformatics Analysis
The bioinformatics analysis from Illumina sequencing was constructed using the methods described previously (Qiao et al., 2019b). In brief, QIIME (version 1.17) was used to de-multiplex and quality-filter the raw FASTQ files, and any unassembled reads were discarded. Bacterial operational taxonomic units (OTUs) were generated using the uclust function in QIIME (http://qiime.org/scripts/pick.outs.html). The Shannon index calculated by mothur (version v.1.30.1) was analyzed to illustrate α-diversity. The OTUs were mapped to a gg13.5 database by QIIME’s command “pick_ closed_otus” at 97% similarity. The OTU abundance was automatically normalized using 16S rRNA gene copy numbers from known bacterial genomes in Integrated Microbial Genomes.
2.4 Accumulation Optimization of Poly-β-Hydroxybutyrate in Suspended Solids
PHB as the carbon and energy reserves is stored in bacterial cells at the appropriate C/N ratio. PHB production is related to the C/N ratio, carbon sources, and bacterial community (Crab et al., 2012; Qiao et al., 2018; Amadu et al., 2021). Based on the most practical usage of carbon sources and probiotics, and the C/N ratio in aquaculture (Laranja et al., 2018), we designed various C/N ratios, carbon sources, and additional bacteria to study parameters that affect the yield of PHB. The detailed protocols are: 1) SS were collected, 2) then diluted into sterilized freshwater, 3) different C/N ratios (12, 16, and 20) using molasses as the sole carbon resource were set to illustrate the effect of the C/N ratio on PHB accumulation, 4) different carbon sources (molasses, starch, wheat bran, and corn meal) and bacteria (Bacillus subtilis SY01, Pseudomonas putida CGMCC15104 and Lactobacillus, and a cocktail of Pse. putida and Lactobacillus) were separately added based on the total ammonia concentration by adapting the C/N ratio of 12 each day for 7 days, and 5) PHB accumulation was evaluated. Pse. putida CGMCC15104 and Lactobacillus were obtained as described previously (Xu, 2019), and B. subtilis SY01 and Pse. putida were isolated from SS in our laboratory (Xu, 2019). During the SS treatment, the usage dose of carbon sources was calculated according to the TAN concentration and kept the C/N ratio of 12. The carbon sources were diluted with water and sprinkled in the water twice every 12 h within 1 day. The final bacterial concentration of addition was 1.0 × 107 CFU mL−1. All the experimental groups were conducted in triplicates.
The relative production rate of PHB was calculated according to Eq. 1.
2.5 Benefit Evaluation of Poly-β-Hydroxybutyrate-Enriched Suspended Solid Treatment in the Aquaculture System
2.5.1 Poly-β-Hydroxybutyrate-Enriched Suspended Solid Treatment
Based on the results from part 2.4 (Figure 6), PHB-enriched SS were obtained by adjusting the C/N ratio, carbon source, and probiotics addition. In brief, SS were randomly collected from three indoor concrete tanks and three outdoor ponds, which cultured fish and shrimp (Table 2). Then, the collected SS were diluted in sterilized freshwater at 1:10 (dry weight of SS:volume of freshwater). These SS were treated and kept at a C/N ratio of 12 by the addition of wheat bran twice every 12 h within 1 day under 24 h of continuous aeration for 7 days at pH 8.0 and temperature 25°C. Meanwhile, the cocktail of Pseudomonas and Lactobacillus was added at day 1 and day 3.
2.5.2 Effects of Suspended Solid Treatment on the Removal Rate of Total Ammonia Nitrogen
At day 0 and day 7, the TAN concentration in the supernatant was measured according to the indophenol method of Koroleff (1976). The removal percentage of TAN from SS solutions was calculated according to Eq. 2.
where Ic is the initial concentration (mg L−1) of TAN (day 0), and Fc is the final concentration (mg L−1) (day 7).
2.5.3 The Nutritional Composition of Suspended Solids and Their Effects on the Growth Performance of Gibel Carp
2.5.3.1 Nutritional Composition Analysis of Suspended Solids
In order to test whether SS could be up-taken by fish, and determine the composition of SS that affects fish production, we first detected the nutritional composition of SS. The nutritional composition was analyzed as our previous description (Zhang et al., 2018). In brief, basal SS were collected using the Imhoff cone and dried in an oven at 105°C to constant weight or freeze-dried to analyze the proximate composition, including the crude protein, lipid, ash, and total amino acid content (AOAC, 1995). The PHB content in the PHB-enriched SS and untreated SS was analyzed as described in Section 2.2.2.
2.5.3.2 Effects of Suspended Solids on the Growth Performance of Gibel Carp
Gibel carp is a main ornamental freshwater culture species, and it can uptake SS in water as food sources (Zhang et al., 2018). Thus, in this study, gibel carp was chosen as a representative ornamental species to evaluate the potential usage of treated SS in aquaculture. Gibel carp (mean body weight of 12.03 g) was bought from a fish farm at Dafeng, Jiangsu province. Prior to the experiments, fish were acclimated for 2 weeks in a tank with continuous aeration at water pH of 7.4–8.2, and temperature of 25–27°C under a 12D/12L cycle. Fish were fed with a commercial diet (Tongwei, China) three times daily at 3% of their body weight, and 30% of water was exchanged. After acclimation, 30 individuals were sampled and separately weighed. Other fish were then randomly distributed into the experimental tanks.
Three groups were set, including the PHB-enriched SS addition (SSA), untreated SS addition (USSA), and no SS addition (NSSA) groups (Figure 1). The PHB content in PHB-enriched SS and untreated SS was 17.34 ± 4.76% and 7.06 ± 1.76%, respectively (Supplementary Table S2). Each group was housed in quadruplicate plastic tanks (size: 60 cm × 50 cm × 40 cm) for 30 days, and each tank included 20 fish. None of the fish in these three groups were fed with a commercial diet in order to test whether SS could be up-taken by fish, and further ensure positive effects of PHB in SS, that is, treated SS could potentially be used as feed. Total suspended solid (TSS) concentration is a good indicator for evaluating suspended solids. Based on the previous study (Zhang et al., 2018), the optimal TSS concentration for gibel carp was 600–800 mg L−1. Thus, TSS concentration in PHB-enriched SSA and USSA groups of the present study was kept at 600 mg L−1 through mixing aerated freshwater and stock SS water as previously described by Zhang et al. (2018), and TSS concentration was set at 10 mg L−1 (close to TSS concentration in natural freshwater) in the NSSA group by daily water-exchange. The TSS concentration was determined according to the Standard Methods for Examination of Water and Wastewater (APHA, 2012). During 30 days of feeding experiment, all fish were cultured at pH of 7.4–8.2, and a temperature of 25 ± 2°C under a 12D/12L cycle. The dead individuals would be cleaned up immediately.
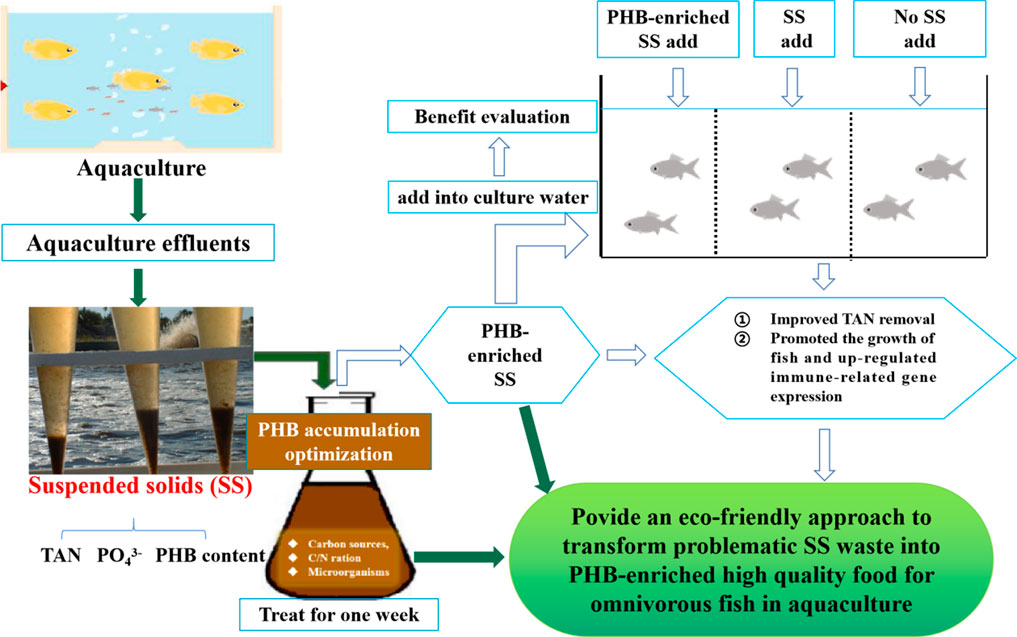
FIGURE 1. Graphical representation of the aquaculture suspended solid (SS) treatment process by adding carbons considering PHB accumulation.
At 30 days, all the fish from each group were weighed to calculate the growth parameters, including weight gain (WGR), specific growth rate (SGR), and thermal growth coefficient (TGC) according to Eqs 3–5.
where FW and IW are the final and initial body weight, respectively, T is duration of the experiment in days, and t is the mean daily water temperature.
2.5.3.3 Effects of Suspended Solid Treatment on the Immune-Related Gene Expression of Gibel Carp
At day 30, five fish were sampled from each group, and anesthetized with tricaine methane sulfonate (MS-222) at 200 mg L−1 for immune-related gene transcription analysis. The spleen and gill (50–100 mg each tissue) were sampled, immediately immersed in 500 μL RNAiso Plus (Sigma), and stored at −80°C until total RNA extraction. RNeasy mini kit (Qiagen, Valencia, CA, United States) was used to extract total RNA (Zhang et al., 2017; Qiao et al., 2019a), and the quantity and purity of RNA were analyzed using a Nanodrop ND-1000 spectrophotometer. First-strand cDNA synthesis was conducted using the PrimeScript™ first-strand cDNA Synthesis kit (Takara Bio, Dalian, China) and the OligodTPrimer. Six immune-related genes, including heat shock protein 70 (hsp70), tyrosine-protein kinase (JAK), interleukin-11 (IL-11), serine/threonine-protein kinase mTOR (mTOR), phosphatidylinositol 3-kinase regulatory subunit alpha (PIK3R1), and intelectin (ITLN), were selected to evaluate the different treatment of SS on the immune-related gene expression of gibel carp. The gene-specific primer list is shown in Supplementary Table S1, and quantitative real time PCR (qRT-PCR) performance was conducted as described in our previous publications (Zhang et al., 2018; Qiao et al., 2020). The relative transcriptional levels of different genes were determined using the following formula: ΔCt = Ct (target) - Ct (internal). The relative fold changes of a specific gene in fish from the PHB-enriched SSA and USSA groups were compared to those from the NSSA group using the 2−ΔΔCt method (Livak & Schmittgen, 2001).
2.6 Data Processing and Statistical Analysis
All data are represented as mean ± standard deviation from triplicated or quadruple samples. Statistical analysis was conducted by Duncan’s multiple range test using one-way ANOVA with SPSS software (version 24.0). The significant difference was set at p value less than 0.05.
3 Results
3.1 Characterization of Suspended Solids
The pH of the collected SS was 8.12 ± 0.05, and the temperature was 27.20 ± 0.26°C. The concentrations of NO3−–N, NO2−–N, NH4+–N, TAN, PO43-–P, and TP in SS were 14.57 ± 0.91 mg L−1, 1.88 ± 0.03 mg L−1, 3.15 ± 0.48 mg L−1, 19.6 ± 1.37 mg L−1, 9.45 ± 1.04 mg L−1, and 11.10 ± 0.40 mg L−1, respectively. The content of PHB in SS was 7.27 ± 0.91% (w/w dry weight) (Table 1). Apparently, nitrogen and phosphorus are the dominant elements in SS.
3.2 Polymer Structure and Material Properties of Poly-β-Hydroxybutyrate in Suspended Solids
The FT-IR spectra of the extracted PHB were measured to confirm the practical structure (Figure 2). The band at 1724 cm−1 corresponded to the stretching of the C=O bond, whereas a series of intense bands located at 980–1,457 cm−1 corresponded to the stretching of the C–O bond of the ester group. The methylene C–H stretching vibration near 2,933 cm−1 was also observed. The presence of absorption bands at 1724 cm−1 and 1,281 cm−1 in the extracted PHB sample were characteristics of C=O and C–O stretching groups, and identical to standard PHB. GC–MS analysis showed that the retention time of the extracted PHB sample was 2.214 min (Figure 3). SEM–EDS analysis showed that the content of elements C and O in the PHB extract from SS was 63.56% of carbon and 35.93% of oxygen, respectively (Figure 4). These data confirmed that our extract PHB is the same as the standard PHB.
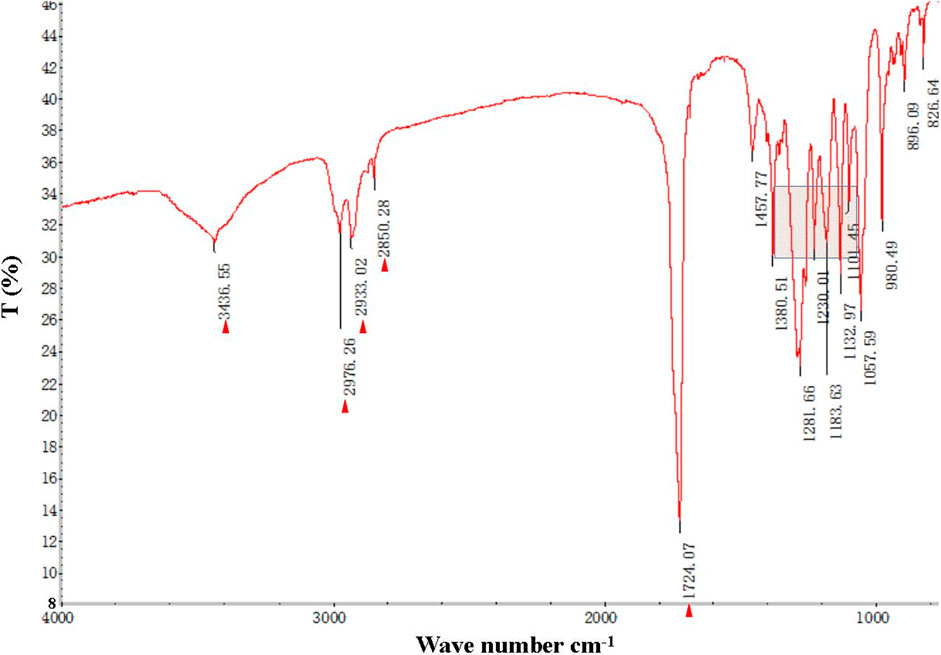
FIGURE 2. Fourier transform infrared (FT-IR) spectra of PHB extract from SS. Red triangle and box with blue line mean bands of PHB.
3.3 Bacterial Diversity Related to Poly-β-Hydroxybutyrate Accumulation in Suspended Solids
We analyzed the bacterial diversity in SS (Supplementary Figure S1). The plateau OTU rarefaction curve after the similarity cutoff of 97% indicated that the sequencing data were reasonable. Furthermore, the Shannon index and relative abundance suggested that the sequences obtained can represent most bacteria in each sample.
The results of OTU analysis using 16S rRNA gene copy numbers from known bacterial genomes showed that the relative abundance of ɤ-Proteobacteria, β-Proteobacteria, α-Proteobacteria, Saprospirae, Flavobacteria, Deltaproteobacteria, Cytophagia, and Fusobacteria was 24%, 21%, 19%, 7%, 7%, 2%, 2%, and 1%, respectively, at the class level (Figure 5A). At the family and genus levels, the relative abundance of Comamonas, Xanthomonadaceae_unclassified, Acinetobacter, Saprospiraceae_ unclassified, Xanthobacteraceae_unclassified, Wautersiella, Pseudomonas, and Myxococcales_unclassified were 9%, 6%, 5%, 5%, 5%, 4%, 3%, and 3%, respectively (Figure 5B). Thus, Comamonas, Acinetobacter, and Pseudomonas are the most dominant bacteria related to PHB production in SS.
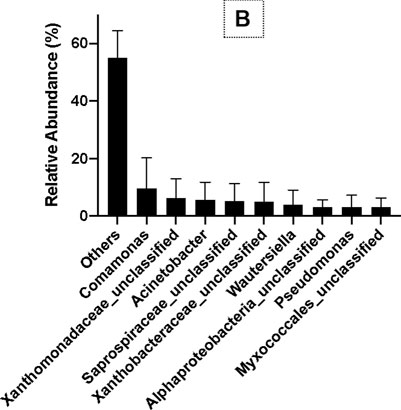
FIGURE 5. Bacterial diversity at the class level (A) and the genus level (B) in SS by MiSeq sequencing.
3.4 Poly-β-Hydroxybutyrate Accumulation Optimization
To improve PHB production, the effects of cultivation conditions (C/N ratio, carbon sources, and probiotic addition) during the treatment process were studied. The results showed that more PHB could be produced in SS at a C/N ratio of 12 with wheat bran as the carbon source, and the bacterial addition of a cocktail of Pseudomonas and Lactobacillus, through which the relative production rate could be improved by ∼200% (Figure 6).
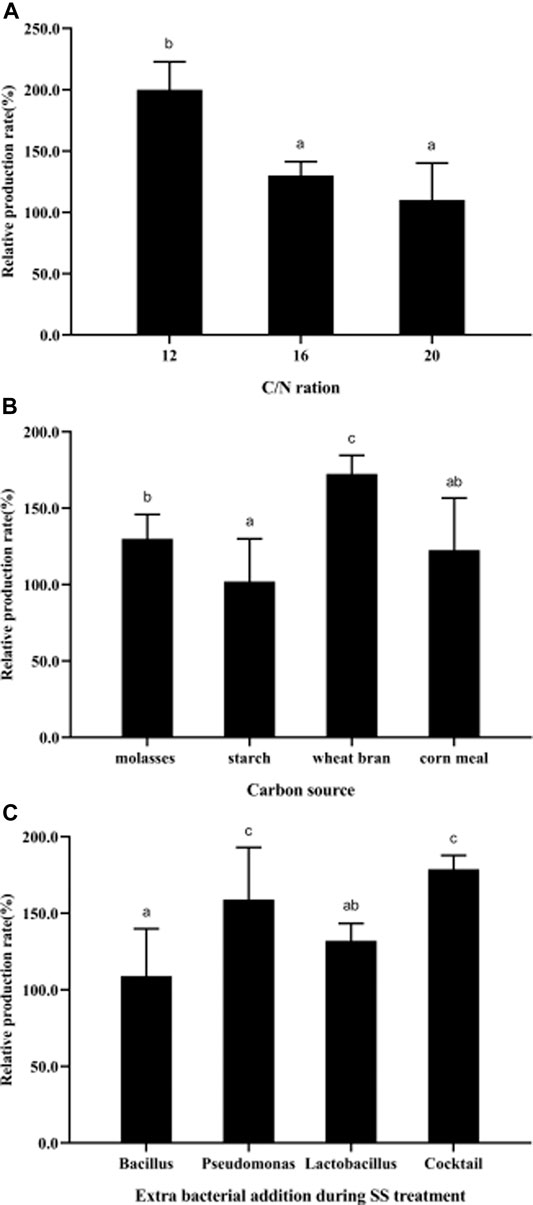
FIGURE 6. Parametric optimization for high-yield PHB production during SS treatment. (A), C/N ration; (B), carbon sources; (C), probiotics widely used in practical aquaculture. All the data are presented as mean ± SE. Values marked with different small letters indicates significant differences among groups (p < 0.05). The strain information used in this study is: Bacillus, Bacillus subtilis; Pseudomonas, Pseudomonas putida; Cocktail, cocktail of Pseudomonas and Lactobacillus.
3.5 Benefit Evaluation of Poly-β-Hydroxybutyrate-Enriched Suspended Solids in Aquaculture System
3.5.1 Poly-β-Hydroxybutyrate-Enriched Suspended Solids Improved TAN Removal From Suspended Solids
The remediation rate in the aquaculture effluents for TAN was more than 87%. The remediation rates in SS collected from the indoor concrete tank 1 (cultured gibel carp), indoor concrete tank 2 (cultured gibel carp), indoor concrete tank 3 (cultured shrimp), outdoor pond 1 (cultured gibel carp), outdoor pond 2 (polyculture of freshwater fish), and outdoor pond 3 (cultured shrimp), were 87.19 ± 5.73%, 91.11 ± 8.94%, 87.76 ± 6.27%, 88.55 ± 3.86%, 90.17 ± 7.49%, and 80.06 ± 6.31%, respectively (Table 2). The final TAN concentration after treatment was below the tolerance range of fish or shrimp.

TABLE 2. Effect of the PHB-enriched treatment on total ammonia nitrogen (TAN) removal rate from suspended solids (SS).
3.5.2 Poly-β-Hydroxybutyrate-Enriched Suspended Soilds Promoted Fish Growth
The content of crude protein, crude lipid, ash, and total amino acids in basal SS was 29.84% (percentage of dry matter), 3.16%, 19.09%, and 14.96%, respectively. The PHB contents in the untreated SS and PHB-enriched SS were 7.06 ± 1.76% and 17.34 ± 4.76%, respectively (Supplementary Table S2).
The WG and SGR of gibel carp in the PHB-enriched SSA and USSA groups were significantly higher than those of the NSSA group. SS could be up-taken by gibel carp as a food source from water, and PHB-enriched SS had a greater effect on the growth performance. The WG, SGR, and survival rate in the PHB-enriched SSA group were 39.15%, 1.66% d−1, and 100%, while those in the NSSA group were -50.38%,-1.36% d−1 and 50%, respectively (Table 3). In the USSA group, the survival rate (23.3 ± 0.03%) was lower, possibly owing to the higher concentration of NH4+–N and NO2−–N, and non-feeding in the untreated SS.
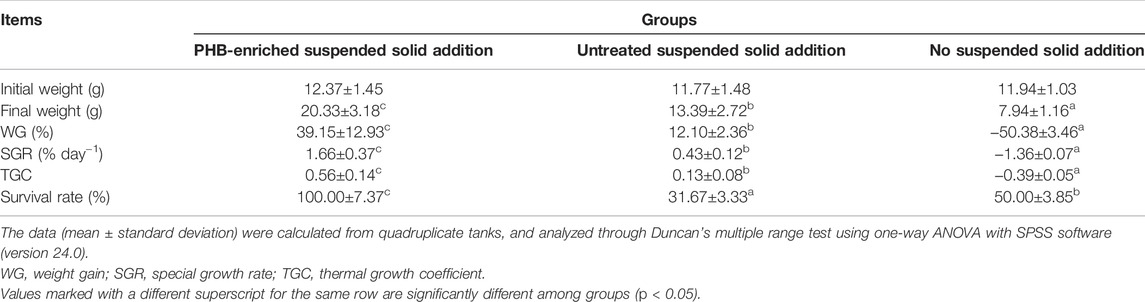
TABLE 3. Growth performance of gibel carp (Carassius auratus gibelio) at day 30 after fed with PHB-enriched suspended solids.
3.5.3 Poly-β-Hydroxybutyrate-Enriched SS Up-Regulated the Immune-Related Gene Expression in Fish
We fed gibel carp with the PHB-enriched SS and examined the immune-related gene expression. The untreated SS were used as the control. The data showed that SS supplementation could up-regulate mRNA expression of the six immune-related genes. In particular, the expression levels of genes hsp70, JAK, and mTOR in both the gills and spleen were up-regulated in the PHB-enriched SSA group. Compared to the negative control NSSA group, the expressions of hsp70 and JAK in the spleen from the PHB-enriched SSA group were significantly up-regulated by 105.36-fold and 53.79-fold, respectively. The mRNA expressions in the gills of the experimental group were 69.45-fold and 81.74-fold of that in the control group. The transcriptional levels of the genes in the survival fish of the USSA group were higher than those in the NSSA group, but lower than those in the PHB-enriched SSA group (Figure 7).
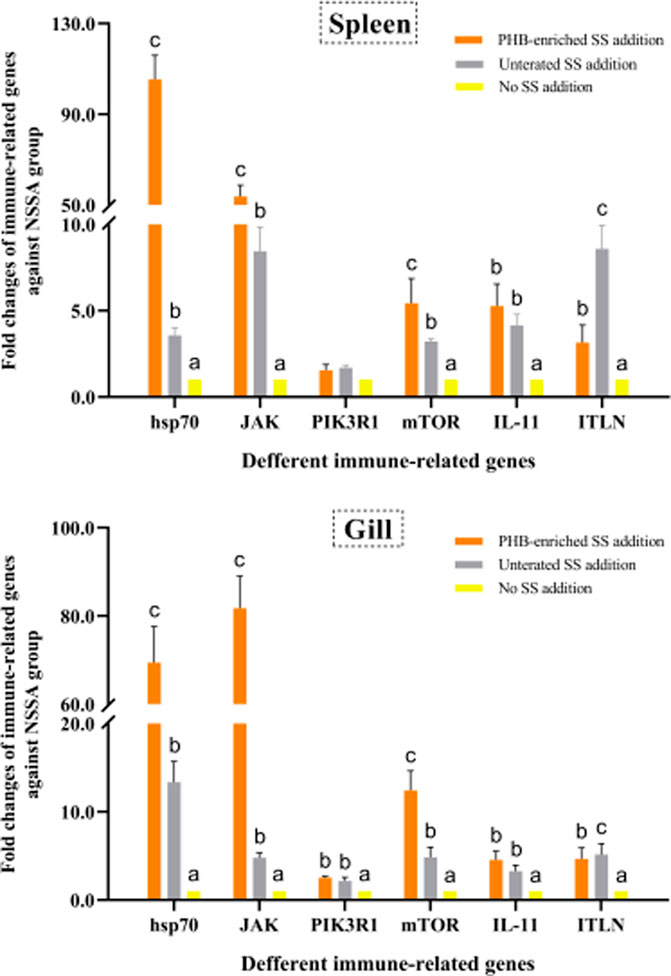
FIGURE 7. Effects of PHB-IRenriched and untreated SS addition in culture water on the immune-related gene expression in the spleen and gills of gibel carp (Carassius auratus gibelio).
4 Discussion
PHB exists in SS from both the traditional aquaculture system and zero-water exchange system modulated by the carbon source addition. In the traditional aquaculture system, the content of pure PHB in the untreated basal SS was lower than the content of crude PHB from SS with carbon source addition during the aquaculture process (Ruan et al., 2011). GC–MS analysis showed that the retention time of our purified PHB was 2.214 min, while the PHB standard (Sigma) was 2.189 min and PHB from Achromobacter marplatensis was 2.21 min (Pol et al., 2017). In the infrared spectrum, the purified PHB from SS had an obvious band at 1724 cm−1 which was the absorption peak of hydroxyl in carboxylic acid. A series of bands at 2,850 cm−1, 3,436 cm−1, 2,976 cm−1, and 2,933 cm−1, were observed, corresponding to the stretching vibration of the -CH, -CO, -CH3, and -CH2, which were the typical characteristics of PHB. We also observed a large amount of C–O vibration between 1,057 cm−1 and 1,380 cm−1, which is consistent with the results reported by Rodríguez-Contreras et al. (2017). These data confirmed that SS from the traditional aquaculture system contained PHB and SS has the potential for PHB production.
PHB, as an intracellular energy store, is synthesized by microbes, and PHB yield is closely related to the C/N ratio, carbon sources, and bacterial diversity (Crab et al., 2012; Qiao et al., 2018; Amadu et al., 2021). Thus, the primary bacterial diversity in SS associated with PHB accumulation was analyzed, and then the accumulation condition optimized. The microbial community in SS, activated sludge, and aquaculture wastes is more complex than a single bacterial medium. The predominant phyla in SS from this study were Proteobacteria, Saprospirae, and Flavobacteriia. The predominant genera were Comamonas, Xanthomonadaceae_unclassified, Acinetobacter, Saprospiraceae_unclassified, Xanthobacteraceae_unclassified, Wautersiella, Pseudomonas and Myxococcales_unclassified. Similarly, in the activated sludge, the main functional microorganisms are phosphorus accumulating organisms (0.43%–5.34%) and glycogen accumulating organisms (0.16%–10.08%). Eight microorganisms were predicted as major PHB accumulators (Ju et al., 2008; Wang et al., 2013). Proteobacteria, Acidobacteria, and Burkholderiales were the dominant bacterial population associated with PHB production in the activated sludge (Wang et al., 2013; Miao et al., 2016), and the genera related to denitrification were identified as Paracoccus, Ottowia, Theresa, and Comamonadaceae_unclassified, accounting for 46.21% of total bacteria. Thus, Proteobacteria, Comamonas, Acinetobacter, and Pseudomonas in SS are potentially related to PHB production. Similar results were also reported in wastewater and activated sludge (Ju et al., 2008; Wang et al., 2013; Miao et al., 2016). Based on the bacteria isolated from SS using the Sudan Black B staining method (data not shown), and probiotics used in the aquaculture practice, the effects of bacterial strains including Bacillus sp., Pseudomonas sp., and Lactobacillus sp. on PHB accumulation in SS were studied. The results showed that Pseudomonas sp. and Lactobacillus sp. had more effective improvement in PHB production.
The C/N ratio and carbon sources are the two key factors in stimulating SS formation and PHB accumulation (Avnimelech, 1999; Fontenot et al., 2007; Albuquerque et al., 2010; Amadu et al., 2021). In the present study, we recommended the optimal C/N ratio is 12:1, which is slightly higher than that in shrimp cultured wastewater (C/N ratio of 10:1) (Fontenot et al., 2007), but much lower than that of previous studies which did not add additional bacteria supplementation in water (Wu et al., 2016; Alsafadi & Al-Mashaqbeh, 2017; Pol et al., 2017; Rodríguez-Contreras et al., 2017). Also, the cost of organic carbon can directly affect the economic profitability of culture industry (Kiani et al., 2020; Iber et al., 2021). Wheat bran as a carbon source promoted more PHB production than molasses, starch, and corn meal. These four kinds of carbon resources can significantly reduce the cultural costs (Solaiman et al., 2006). Previous studies showed that olive mill wastewater could be used as the sole carbon source to produce PHAs by Haloferax mediterranei (Alsafadi & Al-Mashaqbeh, 2017). In solid-state fermentation, Cupriavidus necator cultured in the medium with soy cake or soy cake with sugarcane molasses could produce PHB (Oliveira et al., 2007; Sharma et al., 2016). In pure bacterial culture, the carbon source significantly affects PHB production by Pseudomonas sp. (Matsusaki et al., 1998), Bacillus aryabhattai (Balakrishna Pillai et al., 2017), and C. necator (Sharma et al., 2016). The maximum PHB production by B. aryabhattai was obtained when glucose was used as the sole carbon source, rather than fructose, maltose, starch, or glycerol (Balakrishna Pillai et al., 2017). Taken together, we recommended the C/N ratio of 12, wheat bran as carbon source, and mixed bacterial addition of Pseudomonas sp. and Lactobacillus sp., which could improve the PHB accumulation during SS treatment.
PHB enrichment in SS could eliminate TAN efficiently. The nitrogen in SS is one of detrimental elements in aquaculture systems and the main components of fish fecal droppings (Lazzari & Baldisserotto, 2008; Lananan et al., 2014; Dauda et al., 2019; Rommozzi et al., 2020). In this study, the TAN removal rate of PHB enrichment was more than 87%, which is higher than bioremediation treatment in shrimp culture (68.53%) (Tsukuda et al., 2015), but lower than microalga treatment (100%) (Viegas et al., 2021). As reported previously, for hydroponic bio-filtration, TAN removal was 0.18–0.22 g m−2 d, TN removal was 2.64–4.32 g m−2 d; for sand biofiltration, TN in a rainbow trout (Oncorhynchus mykiss) culture system was reduced from 17.52 mg L−1–14.11 mg L−1. For biofloc technology, the removal of TAN in shrimp and mullet culture systems was 0.12–0.17 mg L−1, which was safe for the aquaculture species. TAN can be determined by measuring the ammonia and ammonium ions (Dauda et al., 2019; Kır et al., 2019).
Although PHB is an eco-friendly material and can be degraded into CO2 and H2O, whether the treated SS (PHB-enriched SS) has the potential to be recycled and reused in the culture system still remains unclear. Previous reports demonstrated that PHB supplementation in diet and culture water can improve the growth, immunity, and disease resistance of aquatic animals, such as tilapia (Oreochromis mossambicus), soiny mullet (Liza haematocheila), Pacific white shrimp (Litopenaeus vannamei), and giant river prawn (Macrobrachium rosenbergii) (Nhan et al., 2010; Schryver et al., 2010; Deng et al., 2014; Xu et al., 2014; Meirong et al., 2018; Qiao et al., 2019a, 2019b, 2020). Dietary PHB supplementation can up-regulate the mRNA expression of some immune-related genes such as Hsp70, MHCs, TLRs, IL-8, hepcidin, pbpA, and AOX in fish (Qiao et al., 2019a, 2020). Additionally, PHB can enhance the disease resistance of aquatic animals against both bacterial and viral infections (Deng et al., 2014; Defoirdt et al., 2018; Van Hung et al., 2019; Qiao et al., 2020). In the present study, PHB-enriched SS can be up-taken by gibel carp, which is in accordance with the previous studies which confirmed that SS can be up-taken by omnivorous fish and shrimp (Nhan et al., 2010; Meirong et al., 2018; Wu et al., 2018; Zhang et al., 2018). The nutritional composition of SS was similar to that composition of SS obtained from the tilapia and shrimp BFT system (Luo et al., 2014; Rajkumar et al., 2016), which can meet the demands of fish and shrimp (Luo et al., 2014; Rajkumar et al., 2016; Zhang et al., 2018). Compared with the untreated SS, the PHB-enriched SS (more than 2-fold) showed positive effects on fish growth and immunity. The WG, SGR, and survival rate of fish cultured in water that contained PHB-enriched SS were higher than those of untreated SS and no SS supplementation groups without feeding, which might be more related to higher PHB accumulation, rather than the probiotics stimulation. Accordingly, it was reported that PHB-accumulating bacteria can improve the survival and growth of shrimp, artemia, and fish more effectively than single probiotics (Gao et al., 2018; Laranja et al., 2018; Krasaesueb et al., 2019). The WG (39.15%) and SGR (1.66% day−1) in the PHB-enriched SS group were higher than those in the bacterial single treatment group, which were 20.46% and 0.40% day−1, respectively (Xu, 2019). It suggested that ‘PHB + probiotics’ treatment is much better than single probiotic usage in fish culture. The lowest survival rate of gibel carp in the untreated SS group without feeding was observed, which might be caused by higher NO2− concentration and non-feeding. NO2− is an intermediate toxic compound, which affects the hemoglobin in carrying oxygen and threatens to destabilize electrolytes (Jiang et al., 2014). As a result, the higher concentration of NO2− in water causes stress, weakens growth, damages the internal organs, and reduces disease tolerance of aquatic animals, and thus harms aquaculture farming (Hurtado et al., 2016). A lower survival rate (50.00 ± 3.85%) in the no SS addition group was also observed, which is considered to relate to the 1 month non-feeding as reported by Zhang et al. (2018). The 1 month survival rate of gibel carp (41.02 ± 3.2 g) in the non-feeding freshwater culture group was 50.00 ± 7.37% (Zhang et al., 2018). PHB-enriched SS could up-regulate the expressions of the immune-related genes, such as hsp70, ITLN, and JAK in the spleen and gills of gibel carp. The spleen is an immune organ of fish (Li et al., 2014), and the gills are one of the first organs directly exposed to the water environment, and are considered as a major organ related to the mucosal immunity (Murray et al., 2007; Andrews et al., 2010). The hsp70, ITLN, and JAK play important roles in the immune response of gibel carp against both viral and bacterial infections (Podok et al., 2014a, 2014b; Xu et al., 2014). Hsp70, as a molecular chaperone, plays very important roles in anti-oxidation, anti-apoptosis, cellular immunity, and innate immune response (Wachstein et al., 2012). The expression of ITLN in gibel carp fed only with bioflocs at a TSS concentration of 600 mg L−1 for 30 days was up-regulated by 78.1-fold (Zhang et al., 2018). The present study would provide a better food source to aquatic animals, and greatly simplify the addition process of PHB in diets due to its water insolubility and reduction of the costs of commercial products.
Thus, from an ecological point of view, PHB can be enriched in SS to reduce nitrogen emission and be non-toxic to the environment, since polymer PHB is naturally degraded into CO2 and H2O. Notedly, this study provides a novel method for using SS in aquaculture systems to reduce waste emission. From an economic point of view, PHB acclimation is derived from low-cost raw materials, and PHB-enriched SS can be reused as a food source to promote fish growth and immunity. It provides cheaper food, and a simple and feasible approach to apply PHB in aquaculture.
5 Conclusion
PHB exists in SS from aquaculture system. PHB accumulation in SS is related to Proteobacteria, Comamonas, Acinetobacter, and Pseudomonas. Optimizing the carbon source and microbial addition increased PHB production in SS. The PHB-enrichment SS reduced TAN, improved fish growth, and boosted fish immunity. We studied SS waste in the aquaculture system and developed an approach to transforming SS waste into high-quality fish food.
Data Availability Statement
The original contributions presented in the study are included in the article/Supplementary Material, further inquiries can be directed to the corresponding authors.
Ethics Statement
This study was conducted in accordance with the regulations for the administration of laboratory animals in Jiangsu province, China.
Author Contributions
GQ: supervision, conceptualization, review and editing, and funding acquisition. XL: methodology, software, analysis, and writing—original draft. MZ: project administration, reviewing and editing, and funding acquisition. YS, YZ, PC, YS, and PC: resources, methodology, software, analysis. ZZ and PL: sampling from outdoor ponds and indoor tanks and funding acquisition. JL, ZQ, and ZW: supervision, resources, writing—review and editing, and validation. EW: English editing.
Funding
This research was financially supported by the National Nature Science Foundation of China (Grant no. 32071265, 31602179), and partially by the National Key R&D Program of China (2019YFD0900302), and by the Projects on Social Science of Philosophy in Colleges and Universities of Jiangsu Province (2020SJA 1923), and by the Projects on High-Quality Development of Fisheries of Yancheng (YCSCY2021020, YCSCY2021014).
Conflict of Interest
The authors declare that the research was conducted in the absence of any commercial or financial relationships that could be construed as a potential conflict of interest.
Publisher’s Note
All claims expressed in this article are solely those of the authors and do not necessarily represent those of their affiliated organizations, or those of the publisher, the editors, and the reviewers. Any product that may be evaluated in this article, or claim that may be made by its manufacturer, is not guaranteed or endorsed by the publisher.
Acknowledgments
We thank JL, Julian Ramelow, and EW for English editing. We also thank the eight reviewers for very constructive feedback and suggestions for article structure organization, data analysis, and literature.
Supplementary Material
The Supplementary Material for this article can be found online at: https://www.frontiersin.org/articles/10.3389/fphys.2022.797625/full#supplementary-material
References
Ahmed N., Thompson S., Glaser M. (2019). Global Aquaculture Productivity, Environmental Sustainability, and Climate Change Adaptability. Environ. Manage. 63, 159–172. doi:10.1007/s00267-018-1117-3
Albuquerque M. G. E., Torres C. A. V., Reis M. A. M. (2010). Polyhydroxyalkanoate (PHA) Production by a Mixed Microbial Culture Using Sugar Molasses: Effect of the Influent Substrate Concentration on Culture Selection. Water Res. 44 (11), 3419–3433. doi:10.1016/j.watres.2010.03.021
Alsafadi D., Al-Mashaqbeh O. (2017). A One-Stage Cultivation Process for the Production of Poly-3-(hydroxybutyrate-Co-Hydroxyvalerate) from Olive Mill Wastewater by Haloferax Mediterranei. New Biotechnol. 34, 47–53. doi:10.1016/j.nbt.2016.05.003
Amadu A. A., Qiu S., Ge S., Addico G. N. D., Ameka G. K., Yu Z., et al. (2021). A Review of Biopolymer (Poly-β-Hydroxybutyrate) Synthesis in Microbes Cultivated on Wastewater. Sci. Total Environ. 756, 143729. doi:10.1016/j.scitotenv.2020.143729
Andrews M., Battaglene S., Cobcroft J., Adams M., Noga E., Nowak B. (2010). Host Response to the Chondracanthid copepod Chondracanthus goldsmidi, a Gill Parasite of the Striped trumpeter, Latris lineata (Forster), in Tasmania. J. Fish. Dis. 33, 211–220. doi:10.1111/j.1365-2761.2009.01107.x
AOAC, Association of Official Analytical Chemists (1995). Official Methods of Analysis. 16th ed. Arlington, VA: AOAC International.
APHA (2012). Standard Methods for the Examination of Water and Wastewater. 22nd. Washington DC: American Public Health Association.
Arun A., Murugappan R. M., David Ravindran A. D., Veeramanikandan V., Balaji S. (2006). Utilization of Various Industrial Wastes for the Production of Poly-β-hydroxybutyrate (PHB) by Alcaligenes Eutrophus. Afr. J. Biotechnol. 5 (17), 1524–1527. doi:10.4314/ajb.v5i17.43150
Avnimelech Y. (1999). Carbon/nitrogen Ratio as a Control Element in Aquaculture Systems. Aquaculture 176 (3–4), 0–235. doi:10.1016/S0044-8486(99)00085-X
Badiola M., Mendiola D., Bostock J. (2012). Recirculating Aquaculture Systems (RAS) Analysis: Main Issues on Management and Future Challenges. Aquacultural Eng. 51, 26–35. doi:10.1016/j.aquaeng.2012.07.004
Balakrishna Pillai A., Jaya Kumar A., Thulasi K., Kumarapillai H. (2017). Evaluation of Short-Chain-Length Polyhydroxyalkanoate Accumulation in Bacillus Aryabhattai. Braz. J. Microbiol. 48 (3), 451–460. doi:10.1016/j.bjm.2017.01.005
Bangar C., Mhaske P., Parasur V., Pawar S. (2017). “Comparative Study of Removal of Dairy Waste Characteristics by Using Various Natural and Chemical Coagulants,” in Special Issue: National Conference "MOMENTUM-17.Int. J. Res. Adv. Technol.Vol. 14
Becke C., Schumann M., Geist J., Brinker A. (2020). Shape Characteristics of Suspended Solids and Implications in Different Salmonid Aquaculture Production Systems. Aquaculture 516, 734631. doi:10.1016/j.aquaculture.2019.734631
Bengtsson S., Werker A., Christensson M., Welander T. (2008). Production of Polyhydroxyalkanoates by Activated Sludge Treating a Paper Mill Wastewater. Bioresour. Technol. 99 (3), 509–516. doi:10.1016/j.biortech.2007.01.020
Benrebah F., Prevost D., Yezza A., Tyagi R. (2007). Agro-industrial Waste Materials and Wastewater Sludge for Rhizobial Inoculant Production: a Review. Bioresour. Technol. 98, 3535–3546. doi:10.1016/j.biortech.2006.11.066
Carpine R., Raganati F., Olivieri G., Hellingwerf K. J., Pollio A., Salatino P., et al. (2018). Poly-β-hydroxybutyrate (PHB) Production by Synechocystis PCC6803 from CO2: Model Development. Algal Res. 29, 49–60. doi:10.1016/j.algal.2017.11.011
Chua A. S. M., Takabatake H., Satoh H., Mino T. (2003). Production of Polyhydroxyalkanoates (PHA) by Activated Sludge Treating Municipal Wastewater: Effect of pH, Sludge Retention Time (SRT), and Acetate Concentration in Influent. Water Res. 37 (15), 3602–3611. doi:10.1016/S0043-1354(03)00252-5
Crab R., Defoirdt T., Bossier P., Verstraete W. (2012). Biofloc Technology in Aquaculture: Beneficial Effects and Future Challenges. Aquaculture 356-357, 351–356. doi:10.1016/j.aquaculture.2012.04.046
Das S. K., Sathish A., Stanley J. (2018). Production of Biofuel and Bioplastic from Chlorella Pyrenoidosa. Mater. Today Proc. 5 (83), 16774–16781. doi:10.1016/j.matpr.2018.06.020
Dauda A. B., Ajadi A., Tola-Fabunmi A. S., Akinwole A. O. (2019). Waste Production in Aquaculture: Sources, Components and Managements in Different Culture Systems. Aquacult. Fish. 4 (3), 81–88. doi:10.1016/j.aaf.2018.10.002
De Schryver P., Sinha A. K., Kunwar P. S., Baruah K., Verstraete W., Boon N., et al. (2010). Poly-β-hydroxybutyrate (PHB) Increases Growth Performance and Intestinal Bacterial Range-Weighted Richness in Juvenile European Sea Bass (Dicentrarchus labrax). Appl. Microbiol. Biotechnol. 86 (5), 1535–1541. doi:10.1007/s00253-009-2414-9
De Schryver P., Verstraete W. (2009). Nitrogen Removal from Aquaculture Pond Water by Heterotrophic Nitrogen Assimilation in Lab-Scale Sequencing Batch Reactors. Bioresour. Technol. 100 (3), 1162–1167. doi:10.1016/j.biortech.2008.08.043
Defoirdt T., Mai Anh N. T., De Schryver P. (2018). Virulence-inhibitory Activity of the Degradation Product 3-hydroxybutyrate Explains the Protective Effect of Poly-β-Hydroxybutyrate against the Major Aquaculture Pathogen Vibrio campbellii. Sci. Repsci. Rep. 8 (1), 7245. doi:10.1038/s41598-018-25385-w
Deng K. Y., Kong J., Meng X. H., Luo K., Cao B. Y., Liu N., et al. (2014). Effects of Poly-β-Hydroxybutyrate on WSSV Resistance in Fenneropenaeus chinensis. J. Fish. Sci. China 8, 1167–1174. doi:10.1074/jbc.M113.510743
Fontenot Q., Bonvillain C., Kilgen M., Boopathy R. (2007). Effects of Temperature, Salinity, and Carbon: Nitrogen Ratio on Sequencing Batch Reactor Treating Shrimp Aquaculture Wastewater. Bioresour. Technol. 98 (9), 1700–1703. doi:10.1016/j.biortech.2006.07.031
Gao M., Du D., Bo Z., Sui L. (2019). Poly-β-hydroxybutyrate (PHB)-accumulating Halomonas Improves the Survival, Growth, Robustness and Modifies the Gut Microbial Composition of Litopenaeus Vannamei postlarvae. Aquaculture 500, 607–612. doi:10.1016/j.aquaculture.2018.10.032
Gao Y., Guo L., Shao M., Hu F., Wang G., Zhao Y., et al. (2020). Denitrification Performance Evaluation and Kinetics Analysis with Mariculture Solid Wastes (MSW) Derived Carbon Source in marine Recirculating Aquaculture Systems (RAS). Bioresour. Technol. 313, 123649. doi:10.1016/j.biortech.2020.123649
Ge S., Champagne P. (2017). Cultivation of the marine Macroalgae Chaetomorpha Linum in Municipal Wastewater for Nutrient Recovery and Biomass Production. Environ. Sci. Technol. 51 (6), 3558–3566. doi:10.1021/acs.est.6b06039
Ge S., Peng Y., Qiu S., Zhu A., Ren N. (2014). Complete Nitrogen Removal from Municipal Wastewater via Partial Nitrification by Appropriately Alternating Anoxic/aerobic Conditions in a Continuous Plug-Flow Step Feed Process. Water Res. 55, 95–105. doi:10.1016/j.watres.2014.01.058
Ge S., Qiu S., Tremblay D., Viner K., Champagne P., Jessop P. G. (2018). Centrate Wastewater Treatment with Chlorella vulgaris: Simultaneous Enhancement of Nutrient Removal, Biomass and Lipid Production. Chem. Eng. J. 342, 310–320. doi:10.1016/j.cej.2018.02.058
Gibson T. F., Watanabe W. O., Losordo T. M., Whitehead R. F., Carroll P. M. (2020). Evaluation of Chemical Polymers as Coagulation Aids to Remove Suspended Solids from marine Finfish Recirculating Aquaculture System Discharge Using a Geotextile Bag. Aquacultural Eng. 90, 102065. doi:10.1016/j.aquaeng.2020.102065
Han P., Lu Q., Fan L., Zhou W. (2019). A Review on the Use of Microalgae for Sustainable Aquaculture. Appl. Sci. 9 (11), 2377. doi:10.3390/app9112377
Heiderscheidt E., Tesfamariam A., Pulkkinen J., Vielma J., Ronkanen A.-k. (2020). Solids Management in Freshwater-Recirculating Aquaculture Systems: Effectivity of Inorganic and Organic Coagulants and the Impact of Operating Parameters. Sci. Total Environ. 742, 140398. doi:10.1016/j.scitotenv.2020.140398
Henriksson P. J. G., Belton B., Jahan K. M.-e., Rico A. (2018). Measuring the Potential for Sustainable Intensification of Aquaculture in Bangladesh Using Life Cycle Assessment. Proc. Natl. Acad. Sci. U.S.A. 115 (12), 2958–2963. doi:10.1073/pnas.1716530115
Hesni M. A., Hedayati A., Qadermarzi A., Pouladi M., Zangiabadi S., Naqshbandi N. (2020). Using Chlorella Vulgaris and Iron Oxide Nanoparticles in a Designed Bioreactor for Aquaculture Effluents Purification. Aquacultural Eng. 90, 102069. doi:10.1016/j.aquaeng.2020.102069
Holm-Nielsen J. B., Al Seadi T., Oleskowicz-Popiel P. (2009). The Future of Anaerobic Digestion and Biogas Utilization. Bioresour. Technol. 100, 5478–5484. doi:10.1016/j.biortech.2008.12.046
Hrabak O. (1992). Industrial Production of Poly-β-Hydroxybutyrate. FEMS Microbiol. Lett. 103, 251–255. doi:10.1016/0378-1097(92)90317-h
Hurtado C. F., Cancino-Madariaga B., Torrejón C., Villegas P. P. (2016). Separation of Nitrite and Nitrate from Water in Aquaculture by Nanofiltration Membrane. Desalination Water Treat. 57, 26050–26062. doi:10.1080/19443994.2016.1160440
Iber B. T., Okomoda V. T., Rozaimah S. A., Kasan N. A. (2021). Eco-friendly Approaches to Aquaculture Wastewater Treatment: Assessment of Natural Coagulants Vis-A-Vis Chitosan. J. Bioresour. Technol. 15, 100702. doi:10.1016/j.biteb.2021.100702
Jiang Q., Dilixiati A., Zhang W., Li W., Wang Q., Zhao Y., et al. (2014). Effect of Nitrite Exposure on Metabolic Response in the Freshwater Prawn Macrobrachium nipponense. Cent. Eur. J. Biol. 9, 86–91. doi:10.2478/s11535-013-0167-4
Jochum M., Moncayo L. P., Jo Y.-K. (2018). Microalgal Cultivation for Biofertilization in rice Plants Using a Vertical Semi-closed Airlift Photobioreactor. PLoS One 13 (9), e0203456. doi:10.1371/journal.pone.0203456
Ju Z. Y., Forster I., Conquest L., Dominy W., Kuo W. C., David Horgen F. (2008). Determination of Microbial Community Structures of Shrimp Floc Cultures by Biomarkers and Analysis of Floc Amino Acid Profiles. Aquac. Res. 39 (2), 118–133. doi:10.1111/j.1365-2109.2007.01856.x
Karri R. R., Sahu J. N., Chimmiri V. (2018). Critical Review of Abatement of Ammonia from Wastewater. J. Mol. Liquids 261, 21–31. doi:10.1016/j.molliq.2018.03.120
Kiani S., Kujala K., T. Pulkkinen J., Aalto S. L., Suurnäkki S., Kiuru T., et al. (2020). Enhanced Nitrogen Removal of Low Carbon Wastewater in Denitrification Bioreactors by Utilizing Industrial Waste toward Circular Economy. J. Clean. Prod. 254, 119973. doi:10.1016/j.jclepro.2020.119973
Kır M., Sunar M. C., Gök M. G. (2019). Acute Ammonia Toxicity and the Interactive Effects of Ammonia and Salinity on the Standard Metabolism of European Sea Bass (Dicentrarchus labrax). Aquaculture 511, 734273. doi:10.1016/j.aquaculture.2019.734273
Kokou F., Fountoulaki E. (2018). Aquaculture Waste Production Associated with Antinutrient Presence in Common Fish Feed Plant Ingredients. Aquaculture 495, 295–310. doi:10.1016/j.aquaculture.2018.06.003
Koroleff F. (1976). Determination of Nutrients: 8. Total and Organic Nitrogen,” in Methods of seawater analysis. Editor K. A. Grasshoff, 161–181.
Krasaesueb N., Incharoensakdi A., Khetkorn W. (2019). Utilization of Shrimp Wastewater for Poly-β-Hydroxybutyrate Production by Synechocystis Sp. PCC 6803 Strain ΔSphU Cultivated in Photobioreactor. Biotechnol. Rep. 23, e00345. doi:10.1016/j.btre.2019.e00345
Lananan F., Abdul Hamid S. H., Din W. N. S., Ali N. a., Khatoon H., Jusoh A., et al. (2014). Symbiotic Bioremediation of Aquaculture Wastewater in Reducing Ammonia and Phosphorus Utilizing Effective Microorganism (EM-1) and Microalgae (Chlorella sp.). Int. Biodeterioration Biodegradation 95, 127–134. doi:10.1016/j.ibiod.2014.06.013
Laranja J. L. Q., De Schryver P., Ludevese-Pascual G. L., Amar E. C., Aerts M., Vandamme P., et al. (2018). High Amorphous Poly-Beta-Hydroxybutyrate (PHB) Content in a Probiotic bacillus Strain Displays Better Protective Effects In Vibrio -challenged Gnotobiotic Artemia. Aquaculture 487, 15–21. doi:10.1016/j.aquaculture.2018.01.005
Law J. H., Slepecky R. A. (1961). Assay of Poly-Β-Hydroxybutyric Acid. J. Bacteriol. 82 (1), 33–36. doi:10.1128/jb.82.1.33-36.1961
Lazzari R., Baldisserotto B. (2008). Nitrogen and Phosphorus Waste in Fish Farming. Bol. Inst. Pesca 34 (4), 591–600.
Li G., Zhao Y., Wen L., Liu Z., Yan F., Gao C. (2014). Identification and Characterization of microRNAs in the Spleen of Common Carp Immune Organ. J. Cel. Biochem. 115 (10), 1768–1778. doi:10.1002/jcb.24843
Livak K. J., Schmittgen T. D. (2001). Analysis of Relative Gene Expression Data Using Real-Time Quantitative PCR and the 2−ΔΔCT Method. Methods 25 (4), 402–408. doi:10.1006/meth.2001.1262
Luo G., Gao Q., Wang C., Liu W., Sun D., Li L., et al. (2014). Growth, Digestive Activity, Welfare, and Partial Cost-Effectiveness of Genetically Improved Farmed tilapia (Oreochromis niloticus) Cultured in a Recirculating Aquaculture System and an Indoor Biofloc System. Aquaculture 422-423 (423), 1–7. doi:10.1016/j.aquaculture.2013.11.023
Matsusaki H., Manji S., Taguchi K., Kato M., Fukui T., Doi Y. (1998). Cloning and Molecular Analysis of the Poly(3-Hydroxybutyrate) and Poly(3-Hydroxybutyrate- Co -3-hydroxyalkanoate) Biosynthesis Genes in Pseudomonas Sp. Strain 61-3. J. Bacteriol. 180 (24), 6459–6467. doi:10.1128/JB.180.24.6459-6467.1998
Meixner K., Troschl C., Fritz I., Drosg B. (2017). Cyanobacteria for Bioplastic Production. Univ. Nat. Resour. Life Sci. Vienna Dep. Agrobiotechnology. IFA-Tulln Inst. Environ. Biotechnol., 27049. doi:10.13140/RG.2.2.32919.27049
Miao L., Wang S., Li B., Cao T., Zhang F., Wang Z., et al. (2016). Effect of Carbon Source Type on Intracellular Stored Polymers during Endogenous Denitritation (ED) Treating Landfill Leachate. Water Res. 100, 405–412. doi:10.1016/j.watres.2016.05.010
Murray H. M., Leggiadro C. T., Douglas S. E. (2007). Immunocytochemical Localization of Pleurocidin to the Cytoplasmic Granules of Eosinophilic Granular Cells from the winter Flounder Gill. J. Fish. Biol. 70, 336–345. doi:10.1111/j.1095-8649.2007.01452.x
Naughton S., Kavanagh S., Lynch M., Rowan N. J. (2020). Synchronizing Use of Sophisticated Wet-Laboratory and In-Field Handheld Technologies for Real-Time Monitoring of Key Microalgae, Bacteria and Physicochemical Parameters Influencing Efficacy of Water Quality in a Freshwater Aquaculture Recirculation System: A Case Study from the Republic of Ireland. Aquaculture 526, 735377. doi:10.1016/j.aquaculture.2020.735377
Naumann D., Helm D., Labischinski H. (1991). Microbiological Characterizations by FT-IR Spectroscopy. Nature 351 (6321), 81–82. doi:10.1038/351081a0
Nhan D. T., Wille M., De Schryver P., Defoirdt T., Bossier P., Sorgeloos P. (2010). The Effect of Poly β-hydroxybutyrate on Larviculture of the Giant Freshwater Prawn (Macrobrachium rosenbergii). Aquaculture 302 (1–2), 76–81. doi:10.1016/j.aquaculture.2010.02.011
Oliveira F. C., Dias M. L., Castilho L. R., Freire D. M. G. (2007). Characterization of Poly(3-Hydroxybutyrate) Produced by Cupriavidus Necator in Solid-State Fermentation. Bioresour. Technol. 98 (3), 633–638. doi:10.1016/j.biortech.2006.02.022
Olusegun A., Babatunde D., Abiodun O. (2016). Haematological Response of Clarias gariepinus Juveniles Reared in Treated Wastewater after Waste Solids Removal Using Alum or Moringa Oleifera Seed Powder. Int. J. Aquacult. 6. doi:10.5376/ija.2016.06.0011
Podok P., Wang H., Xu L., Xu D., Lu L. (2014b). Characterization of Myeloid-specific Peroxidase, Keratin 8, and Dual Specificity Phosphatase 1 as Innate Immune Genes Involved in the Resistance of Crucian Carp (Carassius auratus Gibelio) to Cyprinid Herpesvirus 2 Infection. Fish Shellfish Immunol. 41 (2), 531–540. doi:10.1016/j.fsi.2014.10.001
Podok P., Xu L., Xu D., Lu L. (2014a). Different Expression Profiles of Interleukin 11 (IL-11), Intelectin (ITLN) and Purine Nucleoside Phosphorylase 5a (PNP 5a) in Crucian Carp (Carassius auratus Gibelio) in Response to Cyprinid Herpesvirus 2 and Aeromonas Hydrophila. Fish Shellfish Immunol. 38 (1), 65–73. doi:10.1016/j.fsi.2014.03.001
Pol A. P., Erika A. C., Alexey N., Christina E., Rybak Jürgen., Martin K., et al. (2017). Bacterial Community and PHB-Accumulating Bacteria Associated with the wall and Specialized Niches of the Hindgut of the forest Cockchafer (Melolontha Hippocastani). Front. Microbiol. 8 (e51557), 291. doi:10.3389/fmicb.2017.00291
Qiao G., Chen P., Sun Q., Zhang M., Zhang J., Li Z., et al. (2020). Poly-β-hydroxybutyrate (PHB) in Bioflocs Alters Intestinal Microbial Community Structure, Immune-Related Gene Expression and Early Cyprinid Herpesvirus 2 Replication in Gibel Carp (Carassius auratus Gibelio). Fish Shellfish Immunol. 97, 72–82. doi:10.1016/j.fsi.2019.12.045
Qiao G., Sun Q., Zhang M., Xu C., Lv T., Qi Z., et al. (2019a). Antioxidant System of Soiny Mullet (Liza haematocheila) Is Responsive to Dietary Poly-β-Hydroxybutyrate (PHB) Supplementation Based on Immune-Related Enzyme Activity and De Novo Transcriptome Analysis. Fish Shellfish Immunol. 95, 314–327. doi:10.1016/j.fsi.2019.10.042
Qiao G., Xu C., Sun Q., Xu D.-H., Zhang M., Chen P., et al. (2019b). Effects of Dietary Poly-β-Hydroxybutyrate Supplementation on the Growth, Immune Response and Intestinal Microbiota of Soiny Mullet (Liza haematocheila). Fish Shellfish Immunol. 91, 251–263. doi:10.1016/j.fsi.2019.05.038
Qiao G., Zhang M., Li Y., Xu C., Xu D.-H., Zhao Z., et al. (2018). Biofloc Technology (BFT): An Alternative Aquaculture System for Prevention of Cyprinid Herpesvirus 2 Infection in Gibel Carp (Carassius auratus Gibelio). Fish Shellfish Immunol. 83, 140–147. doi:10.1016/j.fsi.2018.09.015
Qiu S., Shen Y., Zhang L., Ma B., Amadu A. A., Ge S. (2020a). Antioxidant Assessment of Wastewater-Cultivated Chlorella Sorokiniana in Drosophila melanogaster. Algal Res. 46, 101795. doi:10.1016/j.algal.2020.101795
Qiu S., Wang L., Chen Z., Yang M., Yu Z., Ge S. (2021b). An Integrated Mainstream and Sidestream Strategy for Overcoming Nitrite Oxidizing Bacteria Adaptation in a Continuous Plug-Flow Nutrient Removal Process. Bioresour. Technol. 319, 124133. doi:10.1016/j.biortech.2020.124133
Rajkumar M., Pandey P. K., Aravind R., Vennila A., Bharti V., Purushothaman C. S. (2016). Effect of Different Biofloc System on Water Quality, Biofloc Composition and Growth Performance in Litopenaeus vannamei (Boone, 1931). Aquac. Res. 47 (11), 3432–3444. doi:10.1111/are.12792
Rodríguez-Contreras A., García Y., Manero J. M., Rupérez E. (2017). Antibacterial PHAs Coating for Titanium Implants. Eur. Polym. J. 90, 66–78. doi:10.1016/j.eurpolymj.2017.03.004
Rommozzi E., Giannakis S., Giovannetti R., Vione D., Pulgarin C. (2020). Detrimental vs. Beneficial Influence of Ions during Solar (SODIS) and Photo-Fenton Disinfection of E. coli in Water: (Bi)carbonate, Chloride, Nitrate and Nitrite Effects. Appl. Catal. B: Environ. 270, 118877. doi:10.1016/j.apcatb.2020.118877
Rosa J., Lemos M. F. L., Crespo D., Nunes M., Freitas A., Ramos F., et al. (2020). Integrated Multitrophic Aquaculture Systems - Potential Risks for Food Safety. Trends Food Sci. Technol. 96, 79–90. doi:10.1016/j.tifs.2019.12.008
Ruan Y.-J., Zhu L., Xu X.-Y. (2011). Study on the Flocs Poly-β-Hydroxybutyrate Production and Process Optimization in the Bio-Flocs Technology System. Bioresour. Technol. 102 (16), 7599–7602. doi:10.1016/j.biortech.2011.05.028
Sharma P. K., Fu J., Spicer V., Krokhin O. V., Cicek N., Sparling R., et al. (2016). Global Changes in the Proteome of Cupriavidus Necator H16 during Poly-(3-Hydroxybutyrate) Synthesis from Various Biodiesel By-Product Substrates. AMB Expr. 6 (1). doi:10.1186/s13568-016-0206-z
Solaiman D. K. Y., Ashby R. D., Foglia T. A., Marmer W. N. (2006). Conversion of Agricultural Feedstock and Coproducts into Poly(hydroxyalkanoates). Appl. Microbiol. Biotechnol. 71 (6), 783–789. doi:10.1007/s00253-006-0451-1
Sun H., He Z., Zhang M., Yen L., Cao Y., Hu Z., et al. (2020). Spatial Variation of Soil Properties Impacted by Aquaculture Effluent in a Small-Scale Mangrove. Mar. Pollut. Bull. 160, 111511. doi:10.1016/j.marpolbul.2020.111511
Tsukuda S., Christianson L., Kolb A., Saito K., Summerfelt S. (2015). Heterotrophic Denitrification of Aquaculture Effluent Using Fluidized Sand Biofilters. Aquacultural Eng. 64, 49–59. doi:10.1016/j.aquaeng.2014.10.010
Van Hung N., Bossier P., Hong N. T. X., Ludeseve C., Garcia‐Gonzalez L., Nevejan N., et al. (2019). Does Ralstonia Eutropha , Rich in Poly‐β Hydroxybutyrate (PHB), Protect Blue Mussel Larvae against Pathogenic Vibrios ? J. Fish Dis. 42 (6), 777–787. doi:10.1111/jfd.12981
Venkateswar Reddy M., Mawatari Y., Yajima Y., Seki C., Hoshino T., Chang Y.-C. (2015). Poly-3-hydroxybutyrate (PHB) Production from Alkylphenols, Mono and Poly-Aromatic Hydrocarbons Using Bacillus Sp. CYR1: A New Strategy for Wealth from Waste. Bioresour. Technol. 192, 711–717. doi:10.1016/j.biortech.2015.06.043
Viegas C., Gouveia L., Gonçalves M. (2021). Aquaculture Wastewater Treatment through Microalgal. Biomass Potential Applications on Animal Feed, Agriculture, and Energy. J. Environ. Manage. 286, 112187. doi:10.1016/j.jenvman.2021.112187
Wachstein J., Tischer S., Figueiredo C., Limbourg A., Falk C., Immenschuh S., et al. (2012). HSP70 Enhances Immunosuppressive Function of CD4+CD25+FoxP3+ T Regulatory Cells and Cytotoxicity in CD4+CD25− T Cells. PLoS ONE 7 (12), e51747. doi:10.1371/journal.pone.0051747
Wang Y., Cai J., Lan J., Liu Z., He N., Shen L., et al. (2013). Biosynthesis of Poly(hydroxybutyrate-Hydroxyvalerate) from the Acclimated Activated Sludge and Microbial Characterization in This Process. Bioresour. Technol. 148, 61–69. doi:10.1016/j.biortech.2013.08.102
Wu H., Fan Z., Jiang X., Chen J., Chen G.-Q. (2016). Enhanced Production of Polyhydroxybutyrate by Multiple Dividing E. coli. Microb. Cel Fact. 15 (1), 128–140. doi:10.1186/s12934-016-0531-6
Wu Z. B., Gatesoupe F.-J., Li T. T., Wang X. H., Zhang Q. Q., Feng D. Y., et al. (2018). Significant Improvement of Intestinal Microbiota of Gibel Carp (Carassius auratus Gibelio ) after Traditional Chinese Medicine Feeding. J. Appl. Microbiol. 124 (3), 829–841. doi:10.1111/jam.13674
Xu L., Podok P., Xie J., Lu L. (2014). Comparative Analysis of Differential Gene Expression in Kidney Tissues of Moribund and Surviving Crucian Carp (Carassius auratus Gibelio) in Response to Cyprinid Herpesvirus 2 Infection. Arch. Virol. 159 (8), 1961–1974. doi:10.1007/s00705-014-2011-9
Xu M., Tremblay P. L., Ding R., Xiao J., Wang J., Kang Y., et al. (2020). Photo-augmented PHB Production from CO2 or Fructose by Cupriavidus Necator and Shape-Optimized CdS Nanorods. Sci. Total Environ. 753, 142050. doi:10.1016/j.scitotenv.2020.142050
Xu X., Ma B., Lu W., Feng D., Wei Y., Ge C., et al. (2020). Effective Nitrogen Removal in a Granule-Based Partial-Denitrification/anammox Reactor Treating Low C/N Sewage. Bioresour. Technol. 297, 122467. doi:10.1016/j.biortech.2019.122467
Xu Y. (2019). Isolation, Identification and Efficacy Analysis of Probiotics from the Intestinal Tract of Gibel Carp (Carassius auratus Gibelio). Master thesis (Dalian: Dalian Ocean University), 23–41. (in Chinese with English Abstract).
Yun L., Yu Z., Li Y., Luo P., Jiang X., Tian Y., et al. (2019). Ammonia Nitrogen and Nitrite Removal by a Heterotrophic Sphingomonas Sp. Strain LPN080 and its Potential Application in Aquaculture. Aquaculture 500, 477–484. doi:10.1016/j.aquaculture.2018.10.054
Zhang M., Li Y., Xu D.-H., Qiao G., Zhang J., Qi Z., et al. (2018). Effect of Different Water Biofloc Contents on the Growth and Immune Response of Gibel Carp Cultured in Zero Water Exchange and No Feed Addition System. Aquac. Res. 49 (4), 1647–1656. doi:10.1111/are.13620
Keywords: aquaculture solid wastes, nitrogenous compounds, biopolymer, poly-β-hydroxybutyrate (PHB), accumulation optimization, fish food
Citation: Qiao G, Li X, Li J, Zhang M, Shen Y, Zhao Z, Zhang Y, Qi Z, Chen P, Sun Y, Cang P, Liu P, Wangkahart E and Wang Z (2022) An Eco-Friendly Conversion of Aquaculture Suspended Solid Wastes Into High-Quality Fish Food by Improving Poly-β-Hydroxybutyrate Production. Front. Physiol. 13:797625. doi: 10.3389/fphys.2022.797625
Received: 19 October 2021; Accepted: 15 April 2022;
Published: 26 May 2022.
Edited by:
Jin Niu, Sun Yat-sen University, ChinaReviewed by:
Burcu Omuzbuken, Dokuz Eylül University, TurkeyOmid Safari, Ferdowsi University of Mashhad, Iran
Jitendra Kumar Sundaray, Indian Council of Agricultural Research, India
Copyright © 2022 Qiao, Li, Li, Zhang, Shen, Zhao, Zhang, Qi, Chen, Sun, Cang, Liu, Wangkahart and Wang. This is an open-access article distributed under the terms of the Creative Commons Attribution License (CC BY). The use, distribution or reproduction in other forums is permitted, provided the original author(s) and the copyright owner(s) are credited and that the original publication in this journal is cited, in accordance with accepted academic practice. No use, distribution or reproduction is permitted which does not comply with these terms.
*Correspondence: Zhitao Qi, cWl6aGl0YW9AeWNpdC5lZHUuY24=; Zisheng Wang, d3pzMzk5QDEyNi5jb20=
†These authors have contributed equally to this work