- 1Libin Cardiovascular Institute of Alberta, University of Calgary, Calgary, AB, Canada
- 2Faculty of Kinesiology, University of Calgary, Calgary, AB, Canada
- 3Cumming School of Medicine, Calgary, AB, Canada
Whether average sex differences in cardiorespiratory fitness can be mainly explained by blood inequalities in the healthy circulatory system remains unresolved. This study evaluated the contribution of blood volume (BV) and oxygen (O2) carrying capacity to the sex gap in cardiac and aerobic capacities in healthy young individuals. Healthy young women and men (n = 28, age range = 20–43 years) were matched by age and physical activity. Echocardiography, blood pressures, and O2 uptake were measured during incremental exercise. Left ventricular end-diastolic volume (LVEDV), stroke volume (SV), cardiac output (Q), peak O2 uptake (VO2peak), and BV were assessed with precise methods. The test was repeated in men after blood withdrawal and reduction of O2 carrying capacity, reaching women’s levels. Before blood normalization, exercise cardiac volumes and output (LVEDV, SV, Q) adjusted by body size and VO2peak (42 ± 9 vs. 50 ± 11 ml⋅min–1⋅kg–1, P < 0.05) were lower in women relative to men. Blood normalization abolished sex differences in cardiac volumes and output during exercise (P ≥ 0.100). Likewise, VO2peak was similar between women and men after blood normalization (42 ± 9 vs. 40 ± 8 ml⋅min–1⋅kg–1, P = 0.416). In conclusion, sex differences in cardiac output and aerobic capacity are not present in experimental conditions matching BV and O2 carrying capacity between healthy young women and men.
Introduction
Peak oxygen uptake (VO2peak) per kg of body weight, a hallmark of aerobic exercise capacity, is on average ∼15–25% lower in women relative to men with similar training status, although substantial overlap exists (Rusko, 1992; Lundby and Robach, 2015). In theory, multiple variables along the O2 transport and utilization chain might potentially explain such a sex dimorphism in VO2peak (Lundby et al., 2017). Indeed, inherent sex differences can be found in the structure and function of the lungs, blood, heart, arteries, skeletal muscle fibers and mitochondria, where O2 is finally consumed (Pfaffenberger et al., 2013; Lundby and Robach, 2015; Joyner et al., 2016; Sheel et al., 2016; Regitz-Zagrosek and Kararigas, 2017; Montero et al., 2018). The step down the O2 cascade that ultimately limits VO2peak in women and thus primarily explain this sex dimorphism has long remained speculative (Charkoudian and Joyner, 2004; Diaz-Canestro and Montero, 2019, 2020).
On the basis of cumulative evidence since the inception of modern exercise physiology (Kjellberg et al., 1949; Dill et al., 1974; Brotherhood et al., 1975; Heinicke et al., 2001; Levine, 2008; Lundby and Robach, 2015; Montero et al., 2015, 2016a,2016b,2017; Lundby et al., 2017; Montero and Lundby, 2017a,b, 2018), we recently tested the contribution of two definite blood variables in an older cohort (mean age = 64 ± 8 years, range = 42–88 years) (Diaz-Canestro et al., 2021). Blood volume (BV) and O2 carrying capacity were experimentally manipulated in men to match women’s levels (Diaz-Canestro et al., 2021). As a result, the large sex difference in VO2peak was precisely abolished (Diaz-Canestro et al., 2021). Yet, key central determinants of O2 delivery, i.e., cardiac filling, stroke volume (SV) and output (Q), remained elevated during exercise in men (relative to women) after blood normalization (Diaz-Canestro et al., 2021). In parallel, cardiac afterload was reduced in men following blood normalization, plausibly facilitating venous return, cardiac filling, and Q at high exercise intensities due to additional peripheral vasodilation induced by lowered blood O2 content, which in turn may affect blood flow distribution and O2 extraction (Calbet et al., 2006; Lundby et al., 2008; Casey and Joyner, 2012; Diaz-Canestro et al., 2021). Taken together, the sex gap in VO2peak in the older cohort seems to be explained by the interaction of BV and O2 carrying capacity with hemodynamic and molecular regulatory mechanisms that determine optimal delivery of O2 via the circulatory system. Whether these findings can be extrapolated to healthy young individuals with a circulatory system not affected by the inexorable effects of aging remains unknown. Specifically, could BV and O2 carrying capacity explain sex differences in cardiorespiratory fitness at the period of life in that feats of endurance performance are achieved? The answer to such question will provide relevant and plausibly sensitive insight since the aforementioned blood characteristics are amenable to lifestyle as well as pharmacological modification (Jelkmann and Lundby, 2011; Lundby and Robach, 2015; Montero et al., 2015, 2017; Montero and Lundby, 2017a).
This study aimed to experimentally assess the impact of sex differences in BV and O2 carrying capacity on cardiac function, central hemodynamics, and O2 uptake during incremental exercise in healthy young individuals. It was hypothesized that the match of BV and O2 carrying capacity in young men and women would eliminate the sex gap in cardiac as well as aerobic capacities.
Materials and Methods
Participants
Twenty-eight healthy young adult women and men (< 44 years) were recruited via electronic/printed advertisements on community notice boards in the city of Calgary. Moderate-to-vigorous physical activity (MVPA) levels were determined from established questionnaires as previously described (Montero et al., 2016c). Inclusion criteria comprised healthy status, absence of current medical symptoms or medication limiting incremental exercise testing, and no history of cardiac, pulmonary, or neuromuscular diseases. Individuals fulfilling the above criteria but having donated blood within 3 months prior to the study were excluded. The study was approved by the Conjoint Health Research Ethics Board (REB18-1654) of the University of Calgary and conducted in accordance with the declaration of Helsinki. Prior to the start of the experiments, informed oral and written consents were obtained from all participants.
Experimental Design
Participants were required to report to our laboratory at least once, depending on sex and a voluntary familiarization visit. Each man was assessed twice, prior to and after blood normalization relative to a previously assessed woman with similar age and physical activity level (one-to-one matching). Time of day of testing sessions was kept consistent for each men and women–men matched pair with a minimum of 48 h and a maximum of 7 days between the first (baseline) and second (blood normalization) sessions. All individuals were instructed to avoid strenuous exercise, alcohol and caffeine from 24 h prior to testing, as well as to maintain their usual baseline activity and daily dietary habits throughout the study. All measurements were performed after a 5-h fasting period in a quiet room with controlled temperature between 22 and 23°C. Prior to testing the participants completed demographic and clinical questionnaires and rested in supine position for 20 min in order to stabilize cardiovascular, hemodynamic and hematological variables.
Experimental Measures
Hemoglobin Mass (Hbmass) and Blood Volumes
Hemoglobin mass (Hbmass) was determined using the classic carbon monoxide (CO) rebreathing technique integrated in a semi-automated system with a very low typical error of measurement (TE ≤ 1.2%), as previously described (Montero et al., 2015, 2017; Siebenmann et al., 2017). In brief, following 20 min of supine rest, 2 ml of blood (baseline) was sampled from the median cubital vein via a 20-G venflon (BD, United States) and analyzed immediately in duplicate for percent carboxyhemoglobin (%HbCO), hemoglobin (Hb) concentration and hematocrit (Hct) (ABL80, Radiometer, Denmark). Individuals performed the aerobic capacity test with unaltered hematological variables in the first testing session. After the aerobic capacity test was completed and following 20 min of supine recovery, they breathed 100% O2 for 4 min to flush the nitrogen from the airways. After closing the O2 input, a bolus of 1.5 ml/kg of 99.5% chemically pure CO (Air Liquide, Canada) was administrated into the breathing circuit. Individuals rebreathed this gas mixture for 10 min. Then, an additional 2 ml blood sample was obtained and analyzed in duplicate as aforementioned. The change in%HbCO is used to calculate Hbmass, taking into account the small amount of CO that remains in the rebreathing circuit at the end of the procedure. Total red blood cell volume (RBCV), plasma volume (PV), and blood volume (BV) were determined from Hbmass, baseline Hb concentration and baseline Hct (Montero et al., 2015, 2017; Siebenmann et al., 2017).
Transthoracic Echocardiography and Central Hemodynamics
Apical four-chamber and two-chamber cine-loops were continuously recorded via high-resolution ultrasound (Mindray Medical M9, United States) and analyzed offline (TOMTEC Imaging Systems, Royal Philips, Netherlands) at rest and during predetermined levels of incremental exercise relative to peak heart rate (HRpeak) (60, 70, 80, 90, and 100% HRpeak) as well at a given submaximal workload (100 W). Following the American Society of Echocardiography and the European Association of Cardiovascular Imaging recommendations, two-dimensional (2D) cardiac chamber quantification was performed using the modified Simpson method (biplane method of disks) by tracing the endocardial border in both apical four-chamber and two-chamber views at end-diastole and end-systole (Pellikka et al., 2007; Lang et al., 2015). Systolic blood pressure (SBP), diastolic blood pressure (DBP), and mean arterial pressure (MAP) at the heart level were continuously assessed non-invasively via Finometer PRO (Finapres Medical Systems, Netherlands) (Schutte et al., 2004), with data exported into a pre-established acquisition software (Labchart 7, AD Instruments, United Kingdom). Stroke volume (SV) was determined as left ventricular end-diastolic volume (LVEDV) minus left ventricular end-systolic volume (LVESV), while the product of SV and HR provided cardiac output (Q). It should be noted that 2D echocardiography intrinsically underestimates SV and Q due to the geometry of the heart (Patel et al., 2021), yet its high temporal resolution and reliability is required to obtain precise images in a given cardiac cycle during exercise. The Fick equation was used to assess the effect of blood normalization on peak arteriovenous O2 difference, a variable that was not directly measured but estimated by the ratio of VO2peak and Qpeak. Systemic vascular resistance (SVR) was calculated as the ratio of MAP and Q. Total arterial compliance was determined by the pulse pressure (PP) method (SV/PP) (Chemla et al., 1998). Echochardiographic variables are commonly normalized by body surface area (BSA = 0.007184⋅weight0.425⋅height0.725) (Du Bois and Du Bois, 1989). The reproducibility of key echocardiographic and hemodynamic measurements [within-subject coefficient of variation (CV)] during incremental exercise in our laboratory is ≤ 6% for left ventricular (LV) volumes, ≤ 3% for blood pressures and ≤ 7% for SVR.
Aerobic Capacity
An established incremental exercise protocol (Montero et al., 2015, 2017; Montero and Lundby, 2017b) was performed using an electromagnetic cycle ergometer (KICKR Core trainer, Wahoo, United States) integrated within a large lower body negative pressure chamber (LBNP) (165 × 82 × 108 cm) designed for exercise echocardiography (Tymko SB, Canada). The LBNP comprises an electric hydraulic jack that enables to select any degree from 0 to 45° of left lateral tilting (Supplementary Figure 1). The combination of left semilateral supine body position (17° relative to the horizontal) with lower body negative pressure allows for the simultaneous assessment of cardiac function—which requires a left lateral body position for high-quality and reproducible imaging—and aerobic capacity via the regulation of negative pressure inside the chamber (–50 mmHg) to induce hemodynamic loads characteristic of the upright position, a physiological requirement to achieve VO2peak (Murthy et al., 1994; Boda et al., 2000). The test started with a warm-up period of 3 min at 20–30 W workloads. Thereafter, the workload was increased by 15–30 W every 60 s until exhaustion was reached in a total duration of 7–10 min. O2 uptake and CO2 output were continuously measured (CardioCoach VO2, KORR Medical, United States). Calibration of the gas analyzers and the flowmeter was performed prior to each test. Breath-by-breath values were averaged over 15s (Martin-Rincon and Calbet, 2020). The highest breath-by-breath average value was taken as VO2peak provided that two of the following established criteria were fulfilled: Plateau in O2 uptake despite increased workload, age-predicted HRpeak ± 10 bpm (HRpeak = 211–0.64 × age) (Nes et al., 2013), respiratory exchange ratio ≥ 1.1 (Montero et al., 2015; Kaminsky et al., 2017).
Blood Normalization
BV and O2 carrying capacity were reduced in men to the same levels of women with similar age and physical activity level (one-to-one matching) in the second testing session. The opposite approach, i.e., to increase these variables in women to the level of men was not implemented due the convoluted and uncertain methodology related to autologous blood transfusion. In order to match BV in the second testing session, a 20 G venflon (BD, United States) was placed in the median cubital vein and a certain amount of blood (8.1 ± 2.3 ml⋅kg–1, ranging from 0 to 11.3 ml⋅kg–1) was withdrawn immediately before starting the measurements, which resulted in identical BV per kg between men and women. Hemoconcentration was not influenced by this procedure, Hb concentration and Hct were unaltered (P ≥ 0.816) O2 carrying capacity was defined as the concentration in blood of Hb able to carry O2 [i.e., effective Hb (g⋅dl–1) = total Hb (g⋅dl–1)–(HbCO (g⋅dl–1) + methemoglobin (g⋅dl–1)]. Accordingly, a small quantity of CO, determined by the difference in effective Hb between men and women, was introduced in the rebreathing system in which men breathed for 10 min in order to reduce their O2 carrying capacity to women’s level. The level of effective Hb was monitored prior to as well as right after exercise testing in each men via venous blood sampling to control and corroborate the reduction of blood O2 carrying capacity to the desired levels (Christensen et al., 1993; Gonzalez-Alonso et al., 2001; Montero and Lundby, 2019). During the blood normalization procedure, men rested in supine position with their lower body inside the LBNP-exercise chamber.
Statistical Analysis
Statistical analyses were performed using SPSS 22.0 (SPSS, Chicago, IL). Data were tested for normal distribution with the Kolmogorov-Smirnov test and for homogeneity of variances with Levene’s test. Two-way ANOVA with repeated measures was performed to assess echocardiographic, hemodynamic, and pulmonary variables in women and men prior to and after blood normalization, with group (women, men prior to/after blood normalization) and time (60, 70, 80, 90, and 100% HRpeak) as between- and within-subject factors, respectively. When F was significant in the ANOVA, pair-wise specific comparisons were carried out via independent sample t-tests. Pair-wise specific comparisons were secondary and explorative in nature, thus they were not corrected for alpha inflation. A two-tailed P-value less than 0.05 was considered significant. All data are reported as mean (± SD) unless otherwise stated.
Results
General Characteristics
Table 1 present main baseline characteristics of the study subjects. Women had smaller anthropometric indices (height, weight, BSA) than men (P ≤ 0.006). Physical activity levels, determined by MVPA, did not differ between women and men (P ≥ 0.140). Key LV volumetric variables (LVEDV, LVESV, SV indexed by BSA) were lower in women (P ≤ 0.039), whereas HR was similar between sexes. Blood pressures at rest did not differ between sexes, whereas women presented with higher SVR and decreased total arterial compliance (P ≤ 0.028). Cardiorespiratory fitness, as represented by VO2peak, was lower in women relative to men (P = 0.049).
Blood O2 Carrying Capacity and Blood Volume
Hematological parameters in women and men before and after blood normalization are shown in Table 2. Blood O2 carrying capacity, characterized by effective Hb concentration, and Hct were lower in women compared with men (P < 0.001). Blood normalization matched effective Hb (11.9 ± 0.9 vs. 12.0 ± 0.8 g⋅dl–1, P = 0.696) and BV (86.2 ± 11.5 vs. 86.2 ± 10.2 ml⋅kg–1, P = 0.987) in women and men. The BV withdrawn from men was 8.1 ± 2.8 ml⋅kg–1, slightly superior to a standard blood donation (Rios et al., 2010).
Exercise Echocardiography and Hemodynamics During Exercise
LV volumes and function during incremental exercise are displayed in Figure 1. Prior to blood normalization, LV volumes (LVEDV, LVESV, SV) were lower in women compared with men (P ≤ 0.022). Consequently, women showed reduced Q at any given relative exercise intensity (P = 0.004). Blood normalization abolished sex differences in LV volumes and output (P ≥ 0.100). Women and men presented similar LVEF, an overall index of systolic function, irrespective of blood normalization (P ≥ 0.086). Likewise, sex differences were not observed in central blood pressures during incremental exercise prior to or after blood normalization (P ≥ 0.185). In contrast, SVR was higher in women relative to men before blood normalization (P = 0.007), a sex difference that was vanished after blood normalization (P = 0.810) (Figure 2). HRpeak was lower in women relative to men before blood normalization (170.1 ± 12.9 vs. 179.9 ± 9.4 bpm, P = 0.024) and was not altered after blood normalization in men (179.5 ± 9.2 bpm). Blood normalization induced a –13.0% reduction in estimated peak arteriovenous O2 difference in men. At a given absolute submaximal exercise workload (100 W) (Figure 3), women presented reduced LVEDV and SV (P ≤ 0.019) but higher HR (P = 0.013) compared with men prior to blood normalization, which resulted in similar Q (P = 0.656) between sexes. Blood normalization abolished sex differences in submaximal LV volumes and HR (P ≥ 0.109).
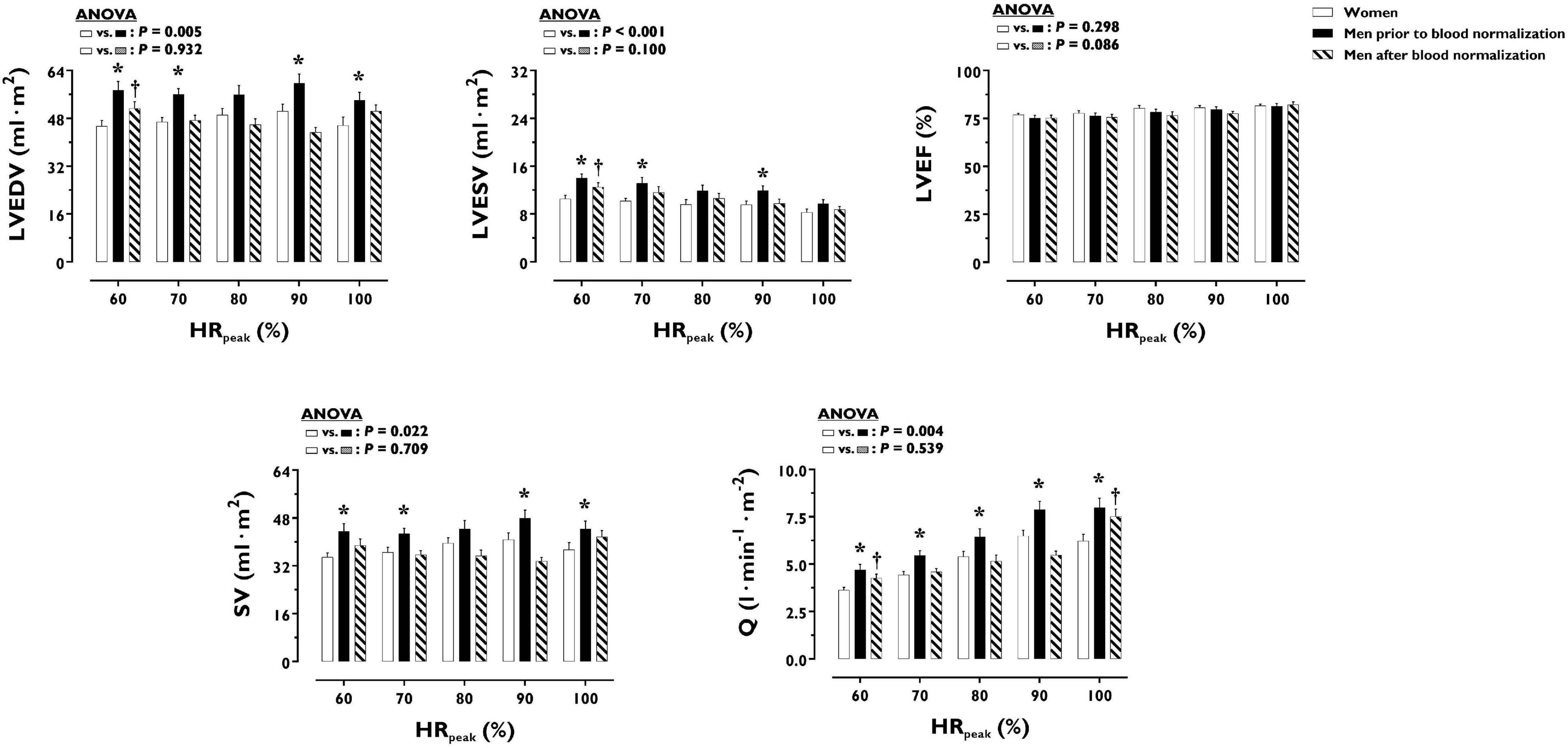
Figure 1. Left ventricular volumes and function during incremental exercise in women and men prior to and after blood normalization. *P < 0.05 between women and men prior to blood normalization. †P < 0.05 between women and men after blood normalization. Data are expressed as mean ± SEM. Number of biological observations for each graph: LVEDV (n = 195), LVESV (n = 195), LVEF (n = 195), SV (n = 195), Q (n = 195). Echocardiographic data were analyzed within ± 5 bmp of specific percentages of HRpeak. HRpeak, peak heart rate; LVEDV, left ventricular end-diastolic volume; LVEF, left ventricular ejection fraction; LVESV, left ventricular end-systolic volume; Q, cardiac output; SV, stroke volume.
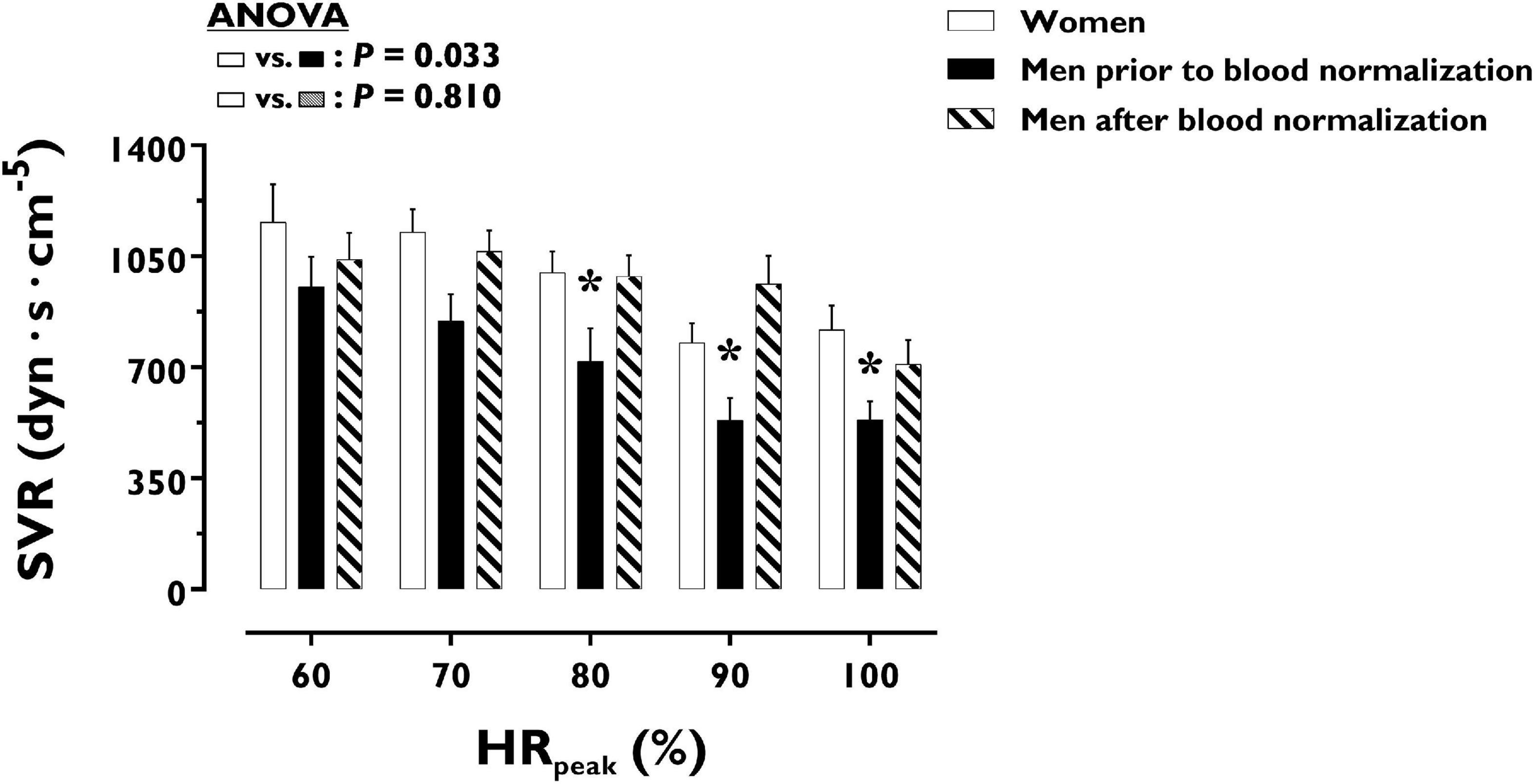
Figure 2. Systemic vascular resistance during incremental exercise in women and men prior to and after blood normalization. *P < 0.05 between women and men prior to blood normalization. †P < 0.05 between women and men after blood normalization. Data are expressed as mean ± SEM. Number of biological observations in the graph: n = 145. Echocardiographic data were analyzed within ± 5 bmp of specific percentages of HRpeak. HRpeak, peak heart rate; SVR, systemic vascular resistance.
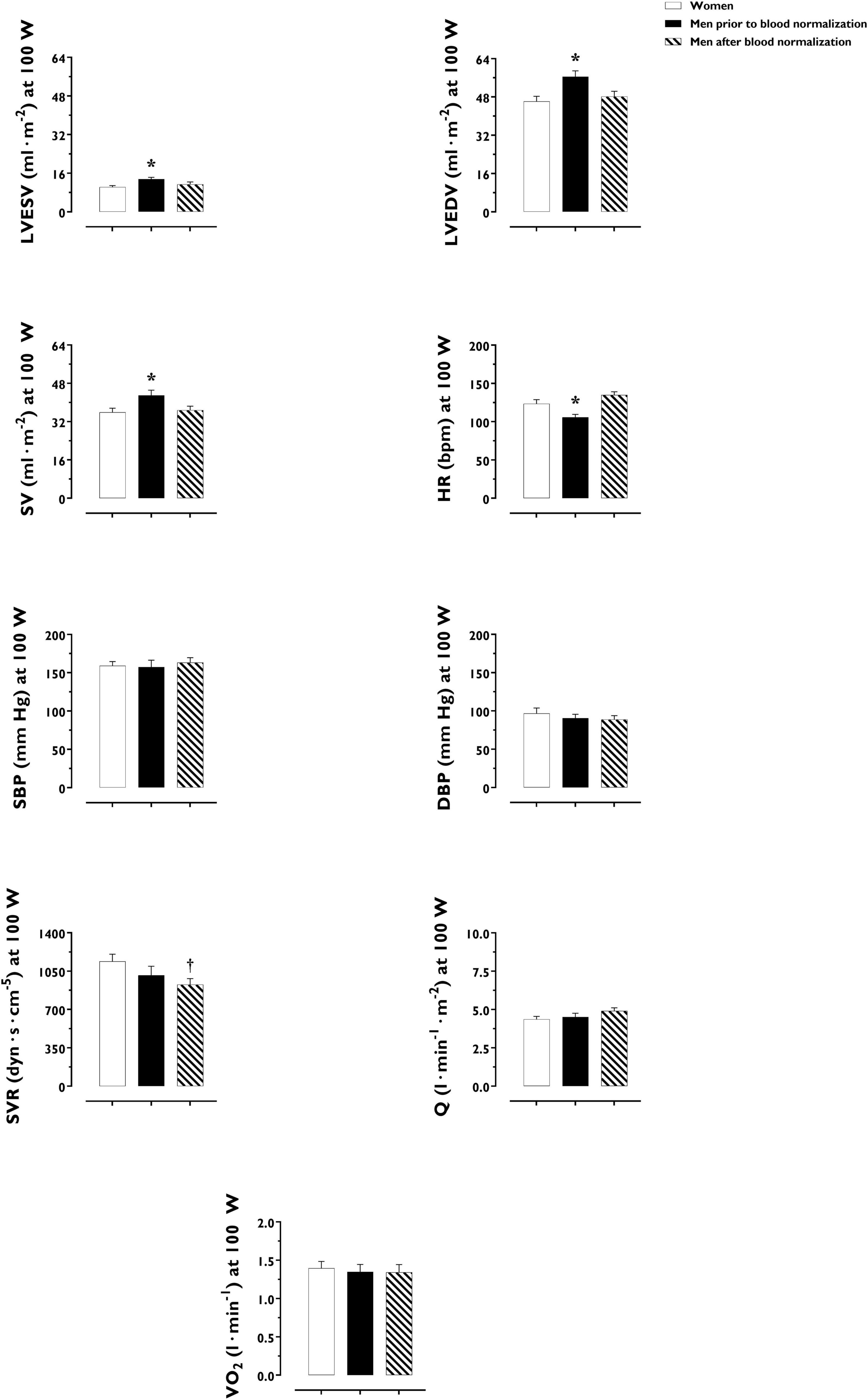
Figure 3. Cardiac, hemodynamic and pulmonary variables at a given fixed submaximal exercise workload (100 W) in women and men prior to and after blood normalization. *P < 0.05 between women and men prior to blood normalization. †P < 0.05 between women and men after blood normalization. Data are expressed as mean ± SEM. Number of biological observations for each graph: LVEDV (n = 42), LVESV (n = 42), SV (n = 42), HR (n = 42), SBP (n = 38), SVR (n = 38), Q (n = 42), VO2 (n = 35). HR, heart rate; LVEDV, left ventricular end-diastolic volume; LVEF, left ventricular ejection fraction; Q, cardiac output; SBP, systolic blood pressure; SV, stroke volume; SVR, systemic vascular resistance; VO2, oxygen uptake (per kg of body weight).
Aerobic Capacity
O2 uptake throughout incremental exercise is displayed in Figure 4. At any specific relative exercise intensity, O2 uptake was reduced in women compared with men before blood normalization (P = 0.010). After blood normalization, there was no sex difference in O2 uptake during exercise (P = 0.159). VO2peak was similar in women and men after blood normalization (42 ± 9 vs. 40 ± 8 ml⋅min–1⋅kg–1, P = 0.416). At an absolute submaximal exercise workload (100 W) (Figure 3), O2 uptake (l⋅min–1) did not differ in women and men before (P = 0.760) and after (P = 0.721) blood normalization.
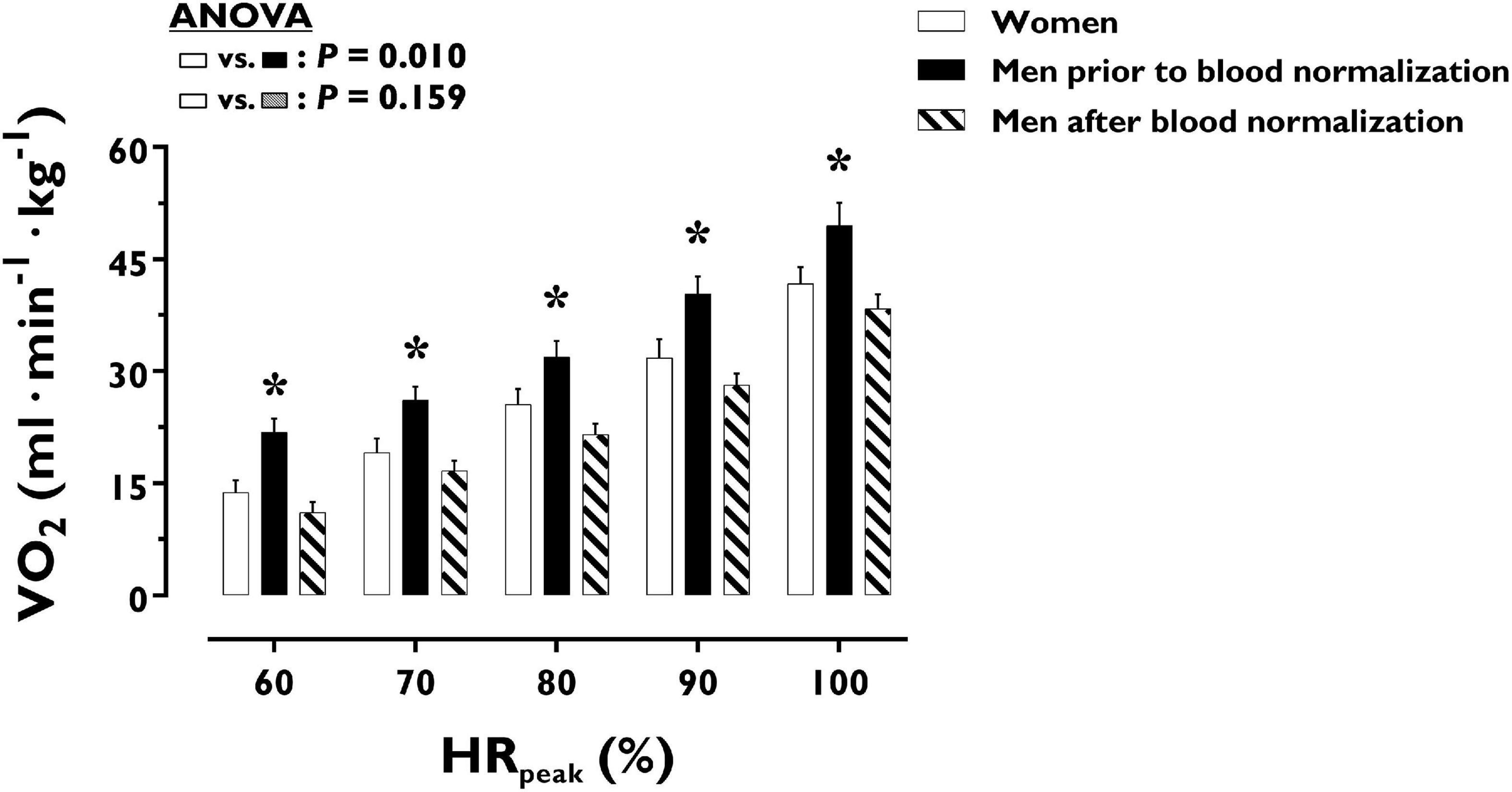
Figure 4. Oxygen uptake during incremental exercise in women and men prior to and after blood normalization. *P < 0.05 between women and men prior to blood normalization. †P < 0.05 between women and men after blood normalization. Data are expressed as mean ± SEM. Number of biological observations in the graph: n = 210. Echocardiographic data were analyzed within ± 5 bmp of specific percentages of HRpeak. HRpeak, peak heart rate; VO2, oxygen uptake (per kg of body weight).
Discussion
The main purpose of this study was to experimentally determine the contribution of key hematological variables on sex differences in cardiorespiratory fitness in healthy young individuals. According to the initial hypothesis, blood normalization between women and men virtually eliminated sex differences in cardiac function, including key LV volumetric determinants of cardiac pumping capacity. Similarly, large sex differences in aerobic capacity were abolished after blood normalization. The present findings support the fundamental contribution of blood variables determining O2 delivery to cardiorespiratory fitness in healthy young individuals.
The connection between the most precious fluid in the human body and aerobic exercise capacity was first acknowledged in the aftermath of World War II (Kjellberg et al., 1949). Likewise, the weakening effects of blood donation on endurance athletes were already noticed in the 1940s when the Springfield College ruled that “no man may place his name on the blood donors list while actively engaged in a varsity sport” (Karpovich and Millman, 1941). Eight decades later, thanks to the progressive development of accurate methods to assess hematological, cardiovascular and metabolic systems at work, the underlying physiology is fundamentally understood. In the presence of normal lung function, cardiorespiratory fitness, as commonly represented by VO2peak, is essentially, albeit not exclusively, determined by the circulatory capacity to deliver O2 (Levine, 2008; Lundby et al., 2017). Such a cardinal variable is not only a function of blood O2 carrying capacity (Lundby et al., 2017; Montero et al., 2019). Indeed, blood plays a fundamental role as a primary hemodynamic “driver” of the human circulatory system. The more blood fills the system, particularly the heart, the greater the output (Q = SV × HR), until a plateau is reached, conforming to the Frank-Starling mechanism or “Law of the heart,” as plainly formulated at the dawn of twentieth century (Maestrini, 1915; Pezza, 1974). The primary role of BV in determining peak cardiac and aerobic capacities, i.e., Qpeak and VO2peak, has been unequivocally demonstrated by phlebotomy studies showing proportional reduction in BV, Qpeak and VO2peak, which do not recover until BV is reestablished (Panebianco et al., 1995; Meurrens et al., 2016; Van Remoortel et al., 2017). Likewise, typical increments in Qmax and VO2max (7–10%) induced by endurance training are reverted to pre training levels after negating training-induced gains in BV (Bonne et al., 2014; Montero et al., 2015), despite the presence of marked skeletal muscle adaptations in healthy young individuals (Montero et al., 2015). Yet, we cannot obviate the fact that the vast majority of available evidence supporting our current understanding arises from studies comprised of men (Levine, 2008; Lundby et al., 2017; Montero and Lundby, 2018). Would the average sex differences in cardiorespiratory fitness be explained by BV and effective Hb concentration? The current study experimentally demonstrates the complete elimination of a 19% sex gap in VO2peak, which is by no means negligible, following blood normalization by means of blood withdrawal and O2 carrying capacity reduction via CO rebreathing in men. Although CO might increase the affinity of Hb for O2, it should be noted that the decrease in estimated peak arteriovenous O2 difference following blood normalization in men (–13.0%) coincided with the change in effective Hb (–13.0%) (Table 2), thus supporting the reduction in blood O2 content as the main experimental effect. While these results concur with the initial hypothesis, the striking contribution of blood variables to the sex dimorphism in VO2peak might not be generally expected. Certainly, blood is not the only variable along the O2 transport and utilization chain known to possess sex-specific characteristics (Sheel et al., 2016; Montero et al., 2018; Diaz-Canestro and Montero, 2020). In this respect, the present findings underline that not every sex difference along the O2 cascade, from the lungs to the mitochondria in skeletal muscle, primarily determines the gap in VO2peak between young women and men.
Notwithstanding the straightforward effects of blood normalization on sex differences in aerobic capacity, the impact on certain cardiovascular outcomes merits further attention. As illustrated in Figure 1, LV volumetric determinants of cardiac pumping capacity during incremental exercise did not differ between women and men after blood normalization. Yet, a trend is noted for smaller effects on LVEDV and SV at the highest exercise intensity. Indeed, Qpeak was reduced in men after blood normalization but remained increased compared with women. Similar, but of greater magnitude, effects of blood normalization on cardiac filling and pumping capacity were noticed in a previous older cohort (Diaz-Canestro et al., 2021). In this older cohort, sex differences in LVEDV, SV, and Q were mostly unaltered by the experimental matching of BV and O2 carrying capacity throughout the incremental exercise (Diaz-Canestro et al., 2021). Thus, additional factors besides decreased systemic blood flow must contribute to eliminating sex differences in VO2peak after blood normalization in both young and older individuals. In the latter, men exhibited a marked and stable decrease in cardiac afterload during exercise, as represented by lower SBP, after blood normalization (Diaz-Canestro et al., 2021). Such hemodynamic alteration might be attributed to the peripheral (compensatory) vasodilation induced by the relative state of anemic hypoxia that follows the reduction of blood O2 carrying capacity and arterial O2 content (Malo et al., 1984; Gonzalez-Alonso et al., 2001). Higher histamine production during hypoxic exercise may additionally contribute to increased vasodilation (Ely et al., 2020). In this respect, extra peripheral vasodilation via the infusion of vasodilators in the femoral artery is known to facilitate SV and thereby enhance cardiac pumping capacity during cycling exercise (Calbet et al., 2006; Gonzalez-Alonso et al., 2008; Bada et al., 2012). Accordingly, when the blood of older men is manipulated to have the O2 carrying capacity of age-matched women, the concomitant decrease in peripheral vascular tone and cardiac afterload may contribute to preserving Q throughout the incremental exercise (Diaz-Canestro et al., 2021). While seemingly paradoxical, such an O2-dependent peripheral vasodilation blunts O2 extraction in exercising limbs (Calbet et al., 2006). This could be the collateral consequence of altered blood flow distribution induced by the vasodilation of arterioles irrigating inactive muscle fibers and non-muscular tissues, which under normoxic exercise are mainly influenced by sympathetic-mediated vasoconstriction—an indispensable adjustment in order to optimize the limited capacity to deliver blood in the systemic circulation during exercise, notably with aging (Calbet et al., 2006; Lundby et al., 2008; Hearon and Dinenno, 2016). Whether the above physiological construct can be extrapolated to the present cohort including young individuals remains speculative. Indeed, the reduction in SVR during incremental exercise was attenuated in young men after blood normalization (matching with women’s levels), indicating that increased peripheral vasoconstriction plausibly induced by hypovolemia prevailed over compensatory vasodilation. Likewise, the herein substantially lesser magnitude of cardiac function preservation after blood normalization implies the influence of aging. At a young age, the intrinsically higher compliance of the heart could entail greater sensibility to changes in filling pressures, resulting in larger effects of blood withdrawal on LVEDV and thereby SV (Fujimoto et al., 2012). Additionally or alternatively, the firmly established increase in baseline vasoconstrictor signaling with aging may leave more “room” for vasodilatory responses in young individuals exposed to the combination of reduced arterial O2 content and blood withdrawal (Hearon and Dinenno, 2016). Further complementary research is needed to ascertain the role of peripheral mechanisms potentially modulating convective and diffusive components of O2 transport and therefore contributing to sex differences in cardiorespiratory fitness in young and older populations.
The final goal of any domain of physiology is to elucidate how a given function works, thus empowering to control outcomes as desired. The comprehension of the mechanisms that explain the generally lower exercise capacity of women relative to similarly trained men might open new avenues for the discovery of effective interventions, with the aim to improve performance and health outcomes in female and male populations (Lundby and Robach, 2015). In this respect, VO2peak is both a crucial determinant of endurance performance as well as a strong prognostic marker of cardiovascular and all-cause mortality (Kodama et al., 2009; Lundby and Robach, 2015). With respect to the major impact of VO2peak on exercise capacity, the question arises as to whether women and men might have similar potential to perform in endurance events if only BV and O2 carrying capacity were matched (Diaz-Canestro and Montero, 2020). Of note, recent studies have demonstrated higher mitochondrial content and oxidative capacity in skeletal muscle of healthy young women compared with men matched by age, VO2peak and running performance (Cardinale et al., 2018; Montero et al., 2018; Diaz-Canestro and Montero, 2020). Hence, women may indeed possess certain metabolic advantages at the lower end of the O2 transport and utilization chain (Diaz-Canestro and Montero, 2020). Notwithstanding, whether women could outperform men with a similar capacity to deliver O2 has been suggested in long-distance races but remains to be ascertained (Speechly et al., 1996; Montero et al., 2018; Diaz-Canestro and Montero, 2020). What can be certainly inferred thus far is that the fundamental determinants of endurance performance in men are readily amenable to modification, this being through specific training, hemodynamic or pharmacological stimuli targeting BV and O2 carrying capacity (Montero et al., 2016d; Montero and Lundby, 2018; Lundby and Montero, 2019). The present findings may have broader implications for health outcomes. In this regard, women generally present a ∼10% lower blood O2 carrying capacity than men, virtually throughout the adult lifespan (Murphy et al., 2010; Murphy, 2014). While blood Hb concentration is a strongly regulated variable mainly unresponsive to typical lifestyle interventions (Lundby and Montero, 2019), the key pathways regulating erythropoiesis are well established and thus potentially targeted by novel lifestyle and/or pharmacological strategies (Montero et al., 2016d; Montero and Lundby, 2018, 2019). Nonetheless, experimental studies have first to confirm the potential acute and long-term benefits of increasing the capacity to deliver O2 in women (Lundby and Montero, 2019). Ultimately, physiology, via the progressive disclosure of how the human body works, may contribute to keep questioning ingrained notions about women and men’s physical capacities.
Limitations
Healthy individuals were selected in order to exclude the potential influence of disease-specific confounding factors. The potential influence of the menstrual phase on blood flow distribution during exercise, venous compliance, and body composition (in particular muscle mass) remains to be elucidated. In addition, potential unsought effects of –50 mmHg LBNP on central hemodynamics during acute leg cycling exercise, if any, remain to be assessed with invasive measures of ventricular filling pressures. Furthermore, the second testing (blood normalization) session was not performed in women. Consequently, the potential influence of ordering effects was not controlled. Finally, the selected experimental approach takes female BV and O2 carrying capacity as the “control” variables. Further studies might attempt to reassess the present findings using the inverse approach, i.e., expanding female BV and increasing O2 carrying capacity to match with male counterparts, if possible using a randomized double-blind cross-over design.
Conclusion
In conclusion, blood normalization in healthy young men and women abolishes sex differences in cardiac and aerobic capacities. The fundamental role of definite blood variables such as BV and O2 carrying capacity in determining sex differences in cardiorespiratory fitness is herein experimentally demonstrated, in a circulatory system not altered by aging or disease.
Data Availability Statement
The original contributions presented in the study are included in the article/Supplementary Material; further inquiries can be directed to the corresponding author/s.
Ethics Statement
The studies involving human participants were reviewed and approved by the Conjoint Health Research Ethics Board (REB18-1654) of the University of Calgary. The patients/participants provided their written informed consent to participate in this study.
Author Contributions
DM: conception and design of the experiments. CD-C, BP, AS, and DM: data collection, analysis, interpretation, and drafting the article or revising it critically for important intellectual content. All authors contributed to the article and approved the submitted version.
Funding
This work was funded by the Swiss National Science Foundation (P2ZHP3-184211 to CD-C) and the Natural Sciences and Engineering Research Council of Canada (Discovery Grant, RGPIN-2019-04833 to DM).
Conflict of Interest
The authors declare that the research was conducted in the absence of any commercial or financial relationships that could be construed as a potential conflict of interest.
Publisher’s Note
All claims expressed in this article are solely those of the authors and do not necessarily represent those of their affiliated organizations, or those of the publisher, the editors and the reviewers. Any product that may be evaluated in this article, or claim that may be made by its manufacturer, is not guaranteed or endorsed by the publisher.
Acknowledgments
We thank the study participants for their willingness, time, and effort devoted to this study.
Supplementary Material
The Supplementary Material for this article can be found online at: https://www.frontiersin.org/articles/10.3389/fphys.2022.747903/full#supplementary-material
References
Bada, A. A., Svendsen, J. H., Secher, N. H., Saltin, B., and Mortensen, S. P. (2012). Peripheral vasodilatation determines cardiac output in exercising humans: insight from atrial pacing. J. Physiol. 590, 2051–2060. doi: 10.1113/jphysiol.2011.225334
Boda, W. L., Watenpaugh, D. E., Ballard, R. E., and Hargens, A. R. (2000). Supine lower body negative pressure exercise simulates metabolic and kinetic features of upright exercise. J. Appl. Physiol. 89, 649–654. doi: 10.1152/jappl.2000.89.2.649
Bonne, T. C., Doucende, G., Fluck, D., Jacobs, R. A., Nordsborg, N. B., Robach, P., et al. (2014). Phlebotomy eliminates the maximal cardiac output response to six weeks of exercise training. Am. J. Physiol. Regul. Integr. Comp. Physiol. 306, R752–R760. doi: 10.1152/ajpregu.00028.2014
Brotherhood, J., Brozovic, B., and Pugh, L. G. (1975). Haematological status of middle- and long-distance runners. Clin. Sci. Mol. Med. 48, 139–145. doi: 10.1042/cs0480139
Calbet, J. A., Lundby, C., Sander, M., Robach, P., Saltin, B., and Boushel, R. (2006). Effects of ATP-induced leg vasodilation on VO2 peak and leg O2 extraction during maximal exercise in humans. Am. J. Physiol. Regul. Integr. Comp. Physiol. 291, R447–R453. doi: 10.1152/ajpregu.00746.2005
Cardinale, D. A., Larsen, F. J., Schiffer, T. A., Morales-Alamo, D., Ekblom, B., Calbet, J. A. L., et al. (2018). Superior intrinsic mitochondrial respiration in women than in men. Front. Physiol. 9:1133. doi: 10.3389/fphys.2018.01133
Casey, D. P., and Joyner, M. J. (2012). Compensatory vasodilatation during hypoxic exercise: mechanisms responsible for matching oxygen supply to demand. J. Physiol. 590, 6321–6326. doi: 10.1113/jphysiol.2012.242396
Charkoudian, N., and Joyner, M. J. (2004). Physiologic considerations for exercise performance in women. Clin. Chest Med. 25, 247–255. doi: 10.1016/j.ccm.2004.01.001
Chemla, D., Hebert, J. L., Coirault, C., Zamani, K., Suard, I., Colin, P., et al. (1998). Total arterial compliance estimated by stroke volume-to-aortic pulse pressure ratio in humans. Am. J. Physiol. 274, H500–H505. doi: 10.1152/ajpheart.1998.274.2.H500
Christensen, P., Waever Rasmussen, J., and Winther Henneberg, S. (1993). Accuracy of a new bedside method for estimation of circulating blood volume. Crit. Care Med. 21, 1535–1540. doi: 10.1097/00003246-199310000-00023
Diaz-Canestro, C., and Montero, D. (2019). Sex dimorphism of VO2max trainability: a systematic review and meta-analysis. Sports Med. 49, 1949–1956. doi: 10.1007/s40279-019-01180-z
Diaz-Canestro, C., and Montero, D. (2020). Unveiling women’s powerhouse. Exp. Physiol. 105, 1060–1062. doi: 10.1113/EP088395
Diaz-Canestro, C., Pentz, B., Sehgal, A., and Montero, D. (2021). Sex differences in cardiorespiratory fitness are explained by blood volume and oxygen carrying capacity. Cardiovasc. Res. 118, 334–343. doi: 10.1093/cvr/cvab028
Dill, D. B., Braithwaite, K., Adams, W. C., and Bernauer, E. M. (1974). Blood volume of middle-distance runners: effect of 2,300-m altitude and comparison with non-athletes. Med. Sci. Sports 6, 1–7. doi: 10.15373/22778179/may2014/193
Du Bois, D., and Du Bois, E. F. (1989). A formula to estimate the approximate surface area if height and weight be known. Nutrition 5, 303–311; discussion 312–303.
Ely, M. R., Ratchford, S. M., La Salle, D. T., Trinity, J. D., Wray, D. W., and Halliwill, J. R. (2020). Effect of histamine-receptor antagonism on leg blood flow during exercise. J. Appl. Physiol. 128, 1626–1634. doi: 10.1152/japplphysiol.00689.2019
Fujimoto, N., Hastings, J. L., Bhella, P. S., Shibata, S., Gandhi, N. K., Carrick-Ranson, G., et al. (2012). Effect of ageing on left ventricular compliance and distensibility in healthy sedentary humans. J. Physiol. 590, 1871–1880. doi: 10.1113/jphysiol.2011.218271
Gonzalez-Alonso, J., Mortensen, S. P., Jeppesen, T. D., Ali, L., Barker, H., Damsgaard, R., et al. (2008). Haemodynamic responses to exercise, ATP infusion and thigh compression in humans: insight into the role of muscle mechanisms on cardiovascular function. J. Physiol. 586, 2405–2417. doi: 10.1113/jphysiol.2008.152058
Gonzalez-Alonso, J., Richardson, R. S., and Saltin, B. (2001). Exercising skeletal muscle blood flow in humans responds to reduction in arterial oxyhaemoglobin, but not to altered free oxygen. J. Physiol. 530, 331–341. doi: 10.1111/j.1469-7793.2001.0331l.x
Hearon, C. M. Jr., and Dinenno, F. A. (2016). Regulation of skeletal muscle blood flow during exercise in ageing humans. J. Physiol. 594, 2261–2273. doi: 10.1113/JP270593
Heinicke, K., Wolfarth, B., Winchenbach, P., Biermann, B., Schmid, A., Huber, G., et al. (2001). Blood volume and hemoglobin mass in elite athletes of different disciplines. Int. J. Sports Med. 22, 504–512. doi: 10.1055/s-2001-17613
Jelkmann, W., and Lundby, C. (2011). Blood doping and its detection. Blood 118, 2395–2404. doi: 10.1182/blood-2011-02-303271
Joyner, M. J., Wallin, B. G., and Charkoudian, N. (2016). Sex differences and blood pressure regulation in humans. Exp. Physiol. 101, 349–355. doi: 10.1113/EP085146
Kaminsky, L. A., Imboden, M. T., Arena, R., and Myers, J. (2017). Reference standards for cardiorespiratory fitness measured with cardiopulmonary exercise testing using cycle ergometry: data from the fitness registry and the importance of exercise national database (FRIEND) registry. Mayo Clin. Proc. 92, 228–233. doi: 10.1016/j.mayocp.2016.10.003
Karpovich, P. V., and Millman, N. (1941). Athletes as blood donors. Res. Q. Am. Assoc. Health Phys. Educ. Recreat. 13, 166–168. doi: 10.1080/10671188.1942.10624742
Kjellberg, S. R., Rudhe, U., and Sjöstrand, T. (1949). Increase of the amount of hemoglobin and blood volume in connection with physical training. Acta Physiol. Scand. 19, 146–151. doi: 10.1111/j.1748-1716.1949.tb00146.x
Kodama, S., Saito, K., Tanaka, S., Maki, M., Yachi, Y., Asumi, M., et al. (2009). Cardiorespiratory fitness as a quantitative predictor of all-cause mortality and cardiovascular events in healthy men and women: a meta-analysis. JAMA 301, 2024–2035. doi: 10.1001/jama.2009.681
Lang, R. M., Badano, L. P., Mor-Avi, V., Afilalo, J., Armstrong, A., Ernande, L., et al. (2015). Recommendations for cardiac chamber quantification by echocardiography in adults: an update from the American Society of Echocardiography and the European Association of Cardiovascular Imaging. Eur. Heart J. Cardiovasc. Imaging 16, 233–270. doi: 10.1093/ehjci/jev014
Levine, B. D. (2008). VO2max: what do we know, and what do we still need to know? J. Physiol. 586, 25–34. doi: 10.1113/jphysiol.2007.147629
Lundby, C., Boushel, R., Robach, P., Moller, K., Saltin, B., and Calbet, J. A. (2008). During hypoxic exercise some vasoconstriction is needed to match O2 delivery with O2 demand at the microcirculatory level. J. Physiol. 586, 123–130. doi: 10.1113/jphysiol.2007.146035
Lundby, C., and Montero, D. (2019). Did you know-why does maximal oxygen uptake increase in humans following endurance exercise training? Acta Physiol. 227:e13371. doi: 10.1111/apha.13371
Lundby, C., Montero, D., and Joyner, M. (2017). Biology of VO2 max: looking under the physiology lamp. Acta Physiol. 220, 218–228. doi: 10.1111/apha.12827
Lundby, C., and Robach, P. (2015). Performance enhancement: What are the physiological limits? Physiology 30, 282–292. doi: 10.1152/physiol.00052.2014
Maestrini, D. (1915). Sulla genesi dell’automatismo cardiaco. Arch. Farmacol. Sper. Sci. Affin. 467–480.
Malo, J., Goldberg, H., Graham, R., Unruh, H., and Skoog, C. (1984). Effect of hypoxic hypoxia on systemic vasculature. J Appl. Physiol. Respir. Environ. Exerc. Physiol. 56, 1403–1410. doi: 10.1152/jappl.1984.56.5.1403
Martin-Rincon, M., and Calbet, J. A. L. (2020). Progress update and challenges on VO2max testing and interpretation. Front. Physiol. 11:1070. doi: 10.3389/fphys.2020.01070
Meurrens, J., Steiner, T., Ponette, J., Janssen, H. A., Ramaekers, M., Wehrlin, J. P., et al. (2016). Effect of repeated whole blood donations on aerobic capacity and hemoglobin mass in moderately trained male subjects: a randomized controlled trial. Sports Med. Open 2:43. doi: 10.1186/s40798-016-0067-7
Montero, D., Breenfeldt-Andersen, A., Oberholzer, L., Haider, T., Goetze, J. P., Meinild-Lundby, A. K., et al. (2017). Erythropoiesis with endurance training: dynamics and mechanisms. Am. J. Physiol. Regul. Integr. Comp. Physiol. 312, R894–R902. doi: 10.1152/ajpregu.00012.2017
Montero, D., Cathomen, A., Jacobs, R. A., Fluck, D., De Leur, J., Keiser, S., et al. (2015). Haematological rather than skeletal muscle adaptations contribute to the increase in peak oxygen uptake induced by moderate endurance training. J. Physiol. 593, 4677–4688. doi: 10.1113/JP270250
Montero, D., Diaz-Canestro, C., Flammer, A., and Lundby, C. (2016a). Unexplained anemia in the elderly: potential role of arterial stiffness. Front. Physiol. 7:485. doi: 10.3389/fphys.2016.00485
Montero, D., Diaz-Canestro, C., Keiser, S., and Lundby, C. (2016b). Arterial stiffness is strongly and negatively associated with the total volume of red blood cells. Int. J. Cardiol. 221, 77–80. doi: 10.1016/j.ijcard.2016.06.265
Montero, D., Houben, A. J., Koster, A., Muris, D. M., Schram, M. T., Gronenschild, E. H., et al. (2016c). Physical activity is associated with glucose tolerance independent of microvascular function: the maastricht study. J. Clin. Endocrinol. Metab. 101, 3324–3332. doi: 10.1210/jc.2016-1526
Montero, D., Rauber, S., Goetze, J. P., and Lundby, C. (2016d). Reduction in central venous pressure enhances erythropoietin synthesis: role of volume-regulating hormones. Acta Physiol. 218, 89–97. doi: 10.1111/apha.12708
Montero, D., Diaz-Canestro, C., Oberholzer, L., and Lundby, C. (2019). The role of blood volume in cardiac dysfunction and reduced exercise tolerance in patients with diabetes. Lancet Diabetes Endocrinol. 7, 807–816. doi: 10.1016/S2213-8587(19)30119-6
Montero, D., and Lundby, C. (2017a). Red cell volume response to exercise training: association with aging. Scand. J. Med. Sci. Sports 27, 674–683. doi: 10.1111/sms.12798
Montero, D., and Lundby, C. (2017b). Refuting the myth of non-response to exercise training: ‘non-responders’ do respond to higher dose of training. J. Physiol. 595, 3377–3387. doi: 10.1113/JP273480
Montero, D., and Lundby, C. (2018). Regulation of red blood cell volume with exercise training. Compr. Physiol. 9, 149–164. doi: 10.1002/cphy.c180004
Montero, D., and Lundby, C. (2019). Arterial oxygen content regulates plasma erythropoietin independent of arterial oxygen tension: a blinded crossover study. Kidney Int. 95, 173–177. doi: 10.1016/j.kint.2018.09.015
Montero, D., Madsen, K., Meinild-Lundby, A. K., Edin, F., and Lundby, C. (2018). Sexual dimorphism of substrate utilization: differences in skeletal muscle mitochondrial volume density and function. Exp. Physiol. 103, 851–859. doi: 10.1113/EP087007
Murphy, W. G. (2014). The sex difference in haemoglobin levels in adults - mechanisms, causes, and consequences. Blood Rev. 28, 41–47. doi: 10.1016/j.blre.2013.12.003
Murphy, W. G., Tong, E., and Murphy, C. (2010). Why do women have similar erythropoietin levels to men but lower hemoglobin levels? Blood 116, 2861–2862. doi: 10.1182/blood-2010-07-294595
Murthy, G., Watenpaugh, D. E., Ballard, R. E., and Hargens, A. R. (1994). Supine exercise during lower body negative pressure effectively simulates upright exercise in normal gravity. J. Appl. Physiol. 76, 2742–2748. doi: 10.1152/jappl.1994.76.6.2742
Nes, B. M., Janszky, I., Wisloff, U., Stoylen, A., and Karlsen, T. (2013). Age-predicted maximal heart rate in healthy subjects: the HUNT fitness study. Scand. J. Med. Sci. Sports 23, 697–704. doi: 10.1111/j.1600-0838.2012.01445.x
Panebianco, R. A., Stachenfeld, N., Coplan, N. L., and Gleim, G. W. (1995). Effects of blood donation on exercise performance in competitive cyclists. Am. Heart J. 130, 838–840. doi: 10.1016/0002-8703(95)90085-3
Patel, H. N., Miyoshi, T., Addetia, K., Henry, M. P., Citro, R., Daimon, M., et al. (2021). Normal values of cardiac output and stroke volume according to measurement technique, age, sex, and ethnicity: results of the world alliance of societies of echocardiography study. J. Am. Soc. Echocardiogr. 34, 1077–1085.e1. doi: 10.1016/j.echo.2021.05.012
Pellikka, P. A., Nagueh, S. F., Elhendy, A. A., Kuehl, C. A., Sawada, S. G., and American Society Of Echocardiography. (2007). American society of echocardiography recommendations for performance, interpretation, and application of stress echocardiography. J. Am. Soc. Echocardiogr. 20, 1021–1041. doi: 10.1016/j.echo.2007.07.003
Pfaffenberger, S., Bartko, P., Graf, A., Pernicka, E., Babayev, J., Lolic, E., et al. (2013). Size matters! Impact of age, sex, height, and weight on the normal heart size. Circ. Cardiovasc. Imaging 6, 1073–1079. doi: 10.1161/CIRCIMAGING.113.000690
Regitz-Zagrosek, V., and Kararigas, G. (2017). Mechanistic pathways of sex differences in cardiovascular disease. Physiol. Rev. 97, 1–37. doi: 10.1152/physrev.00021.2015
Rios, J. A., Fang, J., Tu, Y., Wright, D. J., Spencer, B., Hillyer, C. D., et al. (2010). The potential impact of selective donor deferrals based on estimated blood volume on vasovagal reactions and donor deferral rates. Transfusion 50, 1265–1275. doi: 10.1111/j.1537-2995.2009.02578.x
Rusko, H. K. (1992). Development of aerobic power in relation to age and training in cross-country skiers. Med. Sci. Sports Exerc. 24, 1040–1047.
Schutte, A. E., Huisman, H. W., Van Rooyen, J. M., Malan, N. T., and Schutte, R. (2004). Validation of the Finometer device for measurement of blood pressure in black women. J. Hum. Hypertens. 18, 79–84. doi: 10.1038/sj.jhh.1001639
Sheel, A. W., Dominelli, P. B., and Molgat-Seon, Y. (2016). Revisiting dysanapsis: sex-based differences in airways and the mechanics of breathing during exercise. Exp. Physiol. 101, 213–218. doi: 10.1113/EP085366
Siebenmann, C., Keiser, S., Robach, P., and Lundby, C. (2017). CORP: The assessment of total hemoglobin mass by carbon monoxide rebreathing. J. Appl. Physiol. 123, 645–654. doi: 10.1152/japplphysiol.00185.2017
Speechly, D. P., Taylor, S. R., and Rogers, G. G. (1996). Differences in ultra-endurance exercise in performance-matched male and female runners. Med. Sci. Sports Exerc. 28, 359–365. doi: 10.1097/00005768-199603000-00011
Keywords: blood volume, hemoglobin mass, cardiac function, aerobic capacity, sex
Citation: Diaz-Canestro C, Pentz B, Sehgal A and Montero D (2022) Differences in Cardiac Output and Aerobic Capacity Between Sexes Are Explained by Blood Volume and Oxygen Carrying Capacity. Front. Physiol. 13:747903. doi: 10.3389/fphys.2022.747903
Received: 27 July 2021; Accepted: 02 February 2022;
Published: 17 March 2022.
Edited by:
Preeti H. Jethwa, University of Nottingham, United KingdomReviewed by:
Alexander Hansen, University of Innsbruck, AustriaIain Parsons, Ministry of Defence, United Kingdom
Copyright © 2022 Diaz-Canestro, Pentz, Sehgal and Montero. This is an open-access article distributed under the terms of the Creative Commons Attribution License (CC BY). The use, distribution or reproduction in other forums is permitted, provided the original author(s) and the copyright owner(s) are credited and that the original publication in this journal is cited, in accordance with accepted academic practice. No use, distribution or reproduction is permitted which does not comply with these terms.
*Correspondence: David Montero, ZGF2aWQubW9udGVyb2JhcnJpbEB1Y2FsZ2FyeS5jYQ==; ZGF2aWQubW9udGVyby5iYXJyaWxAZ21haWwuY29t