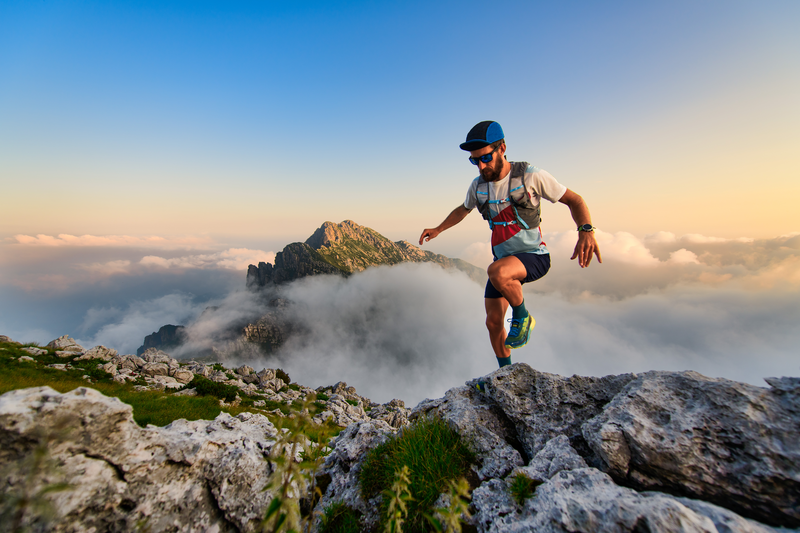
94% of researchers rate our articles as excellent or good
Learn more about the work of our research integrity team to safeguard the quality of each article we publish.
Find out more
ORIGINAL RESEARCH article
Front. Physiol. , 06 January 2023
Sec. Striated Muscle Physiology
Volume 13 - 2022 | https://doi.org/10.3389/fphys.2022.1048008
This article is part of the Research Topic Molecular Physiology of Skeletal Muscle Cells View all 4 articles
Cortisol is an essential regulator of neuroendocrine stress responses in teleost. Cortisol performs its effects through the modulation of glucocorticoid receptor (GR) and mineralocorticoid receptor (MR), activating gene expression. Until now the contribution of both receptors in the global transcriptional response in teleost skeletal muscle has not been explored. To understand in a comprehensive and global manner how GR and MR modulates the skeletal muscle transcriptomic response, we performed RNA-seq analysis. Juvenile rainbow trout (Oncorhynchus mykiss) pretreated with a suppressor of endogenous cortisol production were intraperitoneally injected with cortisol (10 mg/kg). We also included a treatment with mifepristone (GR antagonist) and eplerenone (MR antagonist) in the presence or absence of cortisol. cDNA libraries were constructed from the skeletal muscle of rainbow trout groups: vehicle, cortisol, mifepristone, eplerenone, mifepristone/cortisol and eplerenone/cortisol. RNA-seq analysis revealed that 135 transcripts were differentially expressed in cortisol vs. mifepristone/cortisol group, mainly associated to inflammatory response, ion transmembrane transport, and proteolysis. In the other hand, 68 transcripts were differentially expressed in cortisol vs. eplerenone/cortisol group, mainly associated to muscle contraction, and regulation of cell cycle. To validate these observations, we performed in vitro experiments using rainbow trout myotubes. In myotubes treated with cortisol, we found increased expression of cxcr2, c3, and clca3p mediated by GR, associated with inflammatory response, proteolysis, and ion transmembrane transport, respectively. Contrastingly, MR modulated the expression of myh2 and gadd45g mainly associated with muscle contraction and regulation of cell cycle, respectively. These results suggest that GR and MR have a differential participation in the physiological response to stress in teleost skeletal muscle.
Although the concept of stress has a bad reputation, physiological stress response helps to promote survival of an organism during threating situations (Balasch and Tort, 2019). The biochemical reactions that complements stress response plays an important role in the metabolic adjustments that are critical for meeting the increased energy requirement (Ellis et al., 2012). The stress response in teleost is a biphasic process. It starts with an initial release of catecholamines from the kidney chromaffin tissue, producing a short latency increase in plasma catecholamine levels (Suarez-Bregua et al., 2018). This is followed by the secretion of cortisol from the interrenal tissue, generating a long-latency and extended release of cortisol to the bloodstream (Wendelaar Bonga, 1997). Cortisol-mediated response in teleost plays a fundamental role in early metabolic adaptation, in which the increased secretion of cortisol allows long-term glucose maintenance through the enhancement of gluconeogenesis and amino acid catabolism (Mommsen et al., 1999).
Cortisol binds and activates specific corticoid receptors in target tissues. There are two types of corticoid steroid receptors in fish: mineralocorticoid receptor (MR) and glucocorticoid receptor (GR) (Greenwood et al., 2003). These receptors (MR and GR) evolved from an ancestral corticoid receptor (CR) in jawless fish through gene duplication and divergence (Baker and Katsu, 2019). The appearance of the MR in evolution occurred before the appearance of aldosterone synthase (Baker, 2003). Therefore, aldosterone, the main physiological mineralocorticoid in mammals, is not present in teleost fish (Fuller et al., 1976). MRs have a high affinity for cortisol, while GRs have a low affinity (Gilmour, 2005). Most fish possess one MR and two GR isoforms, GR1 and GR2 (Baker et al., 2013). In the rainbow trout, GR2 is more sensitive to reduced plasma cortisol levels, whereas GR1 is more sensitive to higher levels, a condition caused by acute stressors (Bury and Sturm, 2007). Once inside the cell, cortisol binds to cytoplasmic GR or MR and induces induce their dissociation from molecular chaperones. The hormone–receptor complex then translocate to the nucleus, where it dimerizes and binds to corticosteroid response elements of target genes (Aluru and Vijayan, 2009). Depending on the co-factors recruited, this binding leads to trans-activation or trans-repression of these genes. This mode of action is known as the genomic pathway because it involves gene expression (Bury and Sturm, 2007). In addition to its genomic pathway, cortisol also has effects characterized by rapid second messenger activation, which leads to acute cellular responses (Borski et al., 2001). This mode of action is known as the non-genomic pathway.
Although there are several studies that analyze the molecular response to cortisol in skeletal muscle, the contribution of GR and MR in these processes is unknown. Recently, it was determined that exogenous cortisol treatment is capable of emulating an acute stress condition in rainbow trout, promoting the differentially expression of transcripts associated to mRNA processing, protein ubiquitination, and transcription regulation (Aedo et al., 2019c). Additionally, it was determined the non-genomic action of cortisol associated with a membrane receptor complementary to the genomic actions (Aedo et al., 2019a). In the present work, we performed RNA-seq analysis to evaluate the early transcriptomic response of the skeletal muscle in juvenile rainbow trout treated with cortisol in the presence or absence of mifepristone or eplerenone. The use of mifepristone and eplerenone, both GR and MR antagonists, allowed us to discriminate between the participation of these receptors in cortisol-induced gene expression in rainbow trout skeletal muscle. Thus, we determined, for the first time, potential target genes, biological processes and signaling pathways modulated through these receptors.
The study adhered to animal welfare procedures and was approved by the bioethical committees of the Universidad Andres Bello and the National Commission for Scientific and Technological Research of the Chilean government.
Juvenile rainbow trout (9.70 g ± 26) were obtained from Pisciculture Rio Blanco (V region, Chile). Fish were maintained under natural temperatures and light:dark photoperiod conditions (14°C ± 1°C and L:D 12:12) and fed daily with commercial pellets, except for the day before the in vivo protocol. All fish were anesthetized with benzocaine in water (25 mg/L) and intraperitoneally treated with metyrapone (Sigma-Aldrich, St. Luis, MO, USA) (1 mg/kg of fish) for 1 h and then randomly divided into six groups. A group without treatment was maintained to quantify basal cortisol levels. Fish in the first and second groups were treated with vehicle solution (DMSO, PBS 1X) and cortisol (Sigma-Aldrich, St. Luis, MO, USA) at the concentration of 10 mg/kg. Fish in the third and fourth groups were treated with mifepristone (1 mg/kg) and mifepristone (1 mg/kg) plus cortisol (10 mg/kg). Finally, fish in the fifth and sixth groups were treated with eplerenone (1 mg/kg) and eplerenone (1 mg/kg) plus cortisol (10 mg/kg). After 3 hours of incubation with each compound, all the fish (n = 24) were euthanized by overdoses of benzocaine (300 mg/L). Blood was collected from the caudal vessel with a 1 mL heparinized (10 mg/mL) syringe. Plasma was obtained by centrifugation at 5,000 g for 10 min, immediately frozen in liquid nitrogen and stored at −80°C until analysis. Myotomal skeletal muscle was obtained from the epaxial area for all of the sampled fish, triturated after freezing with liquid nitrogen, placed in RNA later (Sigma-Aldrich, Sant Louis, MO, USA) for 24 h at 4°C and then stored at −80°C for subsequent analysis. Plasma cortisol and glucose levels were measured using the Cayman cortisol (Cayman Chemical, Ann Arbor, MI, USA) and glucose kits (Abcam, Cambridge, United Kingdom), respectively.
RNA was extracted from the skeletal muscle of the vehicle, cortisol, mifepristone, mifepristone/cortisol, eplerenone, and eplerenone/cortisol treated fish using the RNeasy Mini Kit (Qiagen, TX, United States) following the manufacturer’s recommendations. RNA integrity was confirmed by electrophoresis using a 1.2% agarose gel and the capillary electrophoresis Fragment Analyzer Automated CE System (Advanced Analytical Technologies, Inc., IA, United States). Only RNA samples with RQN ≥8.5 were used in further analyses. The amount of total RNA was measured by a fluorometer using the Qubit RNA BR assay kit (Invitrogen, CA, United States). Twenty-four cDNA libraries corresponding to 1 µg of an RNA of each condition, were generated using the TruSeq RNA Sample Preparation kit v2 (Illumina, United States). A total of 24 libraries were sequenced using a paired-end strategy (2 bp × 100 bp) with the HiSeq 2,500 (Illumina) platform of Macrogen (Seul, Korea). The raw data were deposited into the Sequence Read Archive (SRA) available on the NCBI database (PRJNA518130).
Raw reads were analyzed using CLC genomic workbench 9.0 software (CLC bio—Qiagen, TX, United States). Adapters were removed, and raw data were trimmed with low quality reads (Q < 20) and reads length >50 bp. Trimmed reads were mapped onto the reference rainbow trout genome using default mapping parameters: mismatches = 2, minimum fraction length = .9, minimum fraction similarity = .8, and maximum hits per read = 5.
Differential expression analysis in silico was based on reads that uniquely mapped to the reference and the proportional-based statistical K-test. Transcripts with absolute fold-change values ≥ 2.0 and an FDR corrected p-value < .05 were considered differentially expressed transcripts (DETs). A comparison between the vehicle vs. cortisol groups considered potential genes regulated by the whole spectrum of cortisol action. Comparison between cortisol vs. mifepristone/cortisol and cortisol vs. eplerenone/cortisol were considered to identify potential genes regulated by cortisol and mediated by the glucocorticoid and mineralocorticoid receptors, respectively. In addition, comparison between vehicle vs. mifepristone and vehicle vs. eplerenone were included to identify potential genes regulated by glucocorticoid and mineralocorticoid receptors under endogenous suppressed cortisol production, respectively.
The DETs were examined against the DAVID resource (https://david.ncifcrf.gov/) and then categorized based on GO terms for molecular functions, biological processes, cellular components, and KEGG pathways. The gene ID of DETs were extracted and used as input to the DAVID GO enrichment analysis. To obtain the GO ID of each rainbow trout transcript, we performed a BLASTx search against different fish databases, including rainbow trout, Atlantic salmon (Salmo salar), Coho salmon (Oncorhynchus kisutch), zebrafish (Danio rerio) and Atlantic cod (Gadus morhua). Custom IDs set were selected for DAVID analysis as the “Background” Standard settings for ease (1) and gene count (2).
Rainbow trout (Oncorhynchus mykiss) myotubes were prepared as previously reported (Espinoza et al., 2017). Briefly, skeletal muscle was obtained under sterile conditions and collected in Dulbecco’s Modified Eagle’s Medium (DMEM). After mechanical dissociation, the tissue was digested with a .2% solution of collagenase in DMEM for 1 h at 18°C. The suspension was centrifuged at 300 × g at 15°C for 5 min, and the resulting pellet was subjected to two series of enzymatic digestion with a .1% trypsin solution in DMEM for 20 min at 18°C. The cellular suspension was filtered through 40 μm nylon filters and seeded at a density of 2 × 106 cells per well in plates that were previously treated with poly-L-lysine and laminin. Myoblast were incubated at 15°C for 7 days under an air atmosphere and in a proliferating medium (DMEM, 9 mm NaHCO3, 20 mm HEPES, 10% fetal bovine serum, 100 U/L penicillin and 10 mg/mL streptomycin). Myoblast were incubated for an additional 7 days differentiating medium (DMEM, 9 mm NaHCO3, 20 mm HEPES, 100 U/mL penicillin and 10 mg/mL streptomycin). Then, myotubes were preincubated for 30 min with or without the GR antagonist mifepristone (50 nM) or MR antagonist eplerenone (50 nM) (Sigma, St. Luis, MO) and stimulated with cortisol (500 nM) or vehicle solution (DMSO- PBS 1X) for 1, 3, and 6 h. After each treatment, cells were collected for RNA isolation. All treatments were performed with an n = 4, in two independent experiments.
RNA from rainbow trout myotube were extracted using Trizol (Invitrogen, United States), according to the manufacturer’s instructions. RNA was quantified using Nanodrop equipment. A total of 1 μg of RNA from skeletal muscle was reverse transcribed into cDNA using the QuantiTect reverse transcription kit (Qiagen, TX, United States). Primers to amplify the candidate genes evaluated in this study were designed using Primer 3 software (http://frodo.wi.mit.edu/primer3/) and validated with NetPrimer (http://www.premierbiosoft.com/netprimer/). Quantitative PCR was performed using a Stratagene MX3000P qPCR system (Stratagene, La Jolla, CA, United States). Each qPCR reaction mixture contained 7.5 μL of 2× Brilliant® II SYBR® master mix (Stratagene, La Jolla, CA, USA), 6 µL of cDNA (40-fold diluted) and 250 nM of each primer in 20 µL of final volume. Control reactions included a no template control and a control without reverse transcriptase. The list of primers used in this study are found in Supplementary Table S1. Amplifications were performed in triplicate with the following thermal cycling conditions: initial activation at 95°C for 2 min, followed by 40 cycles of 30 s of denaturation at 95°C, 30 s of annealing at 54°C–60°C and 30 s of elongation at 72°C. The presence of a single PCR product was confirmed by performing a melting curve analysis of the PCR products. Relative expression analysis was conducted using geNorm software (https://genorm.cmgg.be/), and results were expressed as the fold induction compared with the vehicle group and using beta actin (actβ) and 40S ribosomal protein S30 (fau) as housekeeping genes.
All data were analyzed using two-way ANOVA and a Tukey’s honestly significant difference (HSD) as a post hoc test, using the Graph Prism 7.0 software (GraphPad Software, Inc., San Diego, CA). A probability level with a p-value < .05 was used as the minimum to indicate the statistical significance.
Juvenile rainbow trout were intraperitoneally injected with cortisol (10 mg/kg) in the presence or absence of a GR antagonist (mifepristone, 1 mg/kg) or a MR antagonist (eplerenone, 1 mg/kg). To inhibit the endogenous production of cortisol because of the fish manipulation, the individuals were pretreated with the endogenous cortisol inhibitor, metyrapone (1 mg/kg). Additionally, we quantified the basal levels of plasmatic cortisol in a non-manipulated group (33 ± 12 ng/mL), showing no significant differences with respect to the vehicle group (60 ± 53 ng/mL). As expected, plasma cortisol levels increased significantly in the cortisol (590 ± 89 ng/mL), mifepristone/cortisol (605 ± 120 ng/mL), eplerenone/cortisol (531 ± 101 ng/mL) groups compared to the vehicle (60 ± 53 ng/mL) (Figure 1A). There were no significant changes in the levels of cortisol in the plasma of fish treated only with mifepristone (71 ± 55 ng/mL) and eplerenone (68 ± 41 ng/mL) groups compared to vehicle. Similarly, there were a significant increases in plasma glucose levels in the cortisol (62 ± 7 mg/dL) and mifepristone/cortisol (57 ± 10 mg/dL) groups compared to the vehicle (20 ± 5 mg/dL) (Figure 1B). There were no significant changes in the levels of glucose in the plasma of fish treated only with mifepristone (20 ± 6 mg/dL) and eplerenone (31 ± 9 mg/dL) compared to vehicle. In addition, no significant differences were observed in glucose levels when comparing cortisol vs. mifepristone/cortisol and cortisol vs. eplerenone/cortisol treatments.
FIGURE 1. Plasma cortisol and glucose levels of rainbow trout. Cortisol (A) and glucose (B) levels were measured in the plasma of fish intraperitoneally treated with vehicle (VEH), cortisol (CORT), mifepristone (MIFE), eplerenone (EPLE), mifepristone plus cortisol (MIFE/CORT), eplerenone plus cortisol (EPLE/CORT). The results are expressed as a mean ± SEM, (n = 4). The “*” (p < .05) and “**” (p < .01) represent significant differences compared with the vehicle, mifepristone, eplerenone groups.
To determine the transcriptional response associated with cortisol and potentially modulated by GR and MR, we sequenced the RNA from the skeletal muscle of fish treated for 3 h with vehicle, cortisol, mifepristone, mifepristone/cortisol, eplerenone, and eplerenone/cortisol. A total of 660, 922, 848 million reads were obtained corresponding to 24 cDNA libraries. After discard adapters and low-quality reads were eliminated, we obtained 618, 153, 281 high-quality reads that were used for further RNA-seq analysis. A total of 433,592,411 high-quality reads (70.14%) were uniquely mapped against the 71,413 CDS of rainbow trout. Principal components analysis of the cDNA libraries of all sampled points are indicated in the Supplementary Figure S1.
To identify differentially expressed transcripts (DETs), samples were subjected to a series of paired comparisons against vehicle group. With cortisol treatment (vehicle vs. cortisol), 266 DETs were identified (255 up and 11 down). The complete list of the differentially expressed transcripts is included in Supplementary Table S2. The 266 differentially expressed transcripts (DETs) were analyzed using the DAVID database and categorized as biological process, molecular function, cellular component, and KEGG pathways. DETs were significantly enriched in a wide variety of biological processes, such as cell-cell adhesion, ion transport, and anion transmembrane transport. Among others relevant biological processes enriched include integrin-mediated signaling pathway, positive regulation of gene expression, regulation of cell proliferation, fatty acid metabolic process, glucose transmembrane transport, proteolysis, and muscle contraction (Table 1). For molecular function and cellular component, the most impacted GO terms were assigned to transmembrane transporter activity and apical plasma membrane, respectively (Supplementary Table S3). KEGG pathway analysis revealed that transcripts associated with pathogenic Escherichia coli infection, bile secretion, and metabolism of xenobiotics by cytochrome P450 were differentially expressed (Supplementary Table S3).
TABLE 1. Biological process GO terms of differentially expressed transcripts between the vehicle vs. cortisol groups.
We analyzed the participation of glucocorticoid receptor in gene expression under endogenous suppressed cortisol production through the in vivo administration of mifepristone. With mifepristone treatment (vehicle vs. mifepristone), 134 DETs were identifies (64 up and 70 down). The complete list of the differentially expressed transcripts is included in Supplementary Table S4. DETs were significantly enriched in biological processes, such as muscle filament sliding, muscle contraction, and ATP metabolic process. Among others relevant biological processes enriched include cardiac muscle contraction, cellular response to heat, intracellular receptor signaling pathway, steroid hormone mediated signaling pathway, positive regulation of transcription from RNA polymerase II promoter, cellular response to oxidative stress, and protein stabilization (Table 2). For molecular function and cellular component, the most impacted GO terms were assigned to microfilament motor activity and sarcomere, respectively (Supplementary Table S5). KEGG pathway analysis revealed that several transcripts associated with hypertrophic cardiomyopathy, dilated cardiomyopathy, PPAR signaling pathway were differentially expressed (Supplementary Table S5).
TABLE 2. Biological process GO terms of differentially expressed transcripts between the vehicle vs. mifepristone groups.
Similarly, we analyzed the participation of mineralocorticoid receptor in gene expression under endogenous suppressed cortisol production through the in vivo administration of eplerenone. With eplerenone treatment (vehicle vs. eplerenone), 111 DETs were identified (61 up and 50 down). The complete list of the differentially expressed transcripts is included in Supplementary Table S6. DETs were significantly enriched in biological processes, such as intermediate filament organization, circadian regulation of gene expression, and negative regulation of transcription from RNA polymerase II promoter. Among others relevant biological processes enriched include microtubule cytoskeleton organization, ion transport, extracellular matrix organization, sodium ion transport, fatty acid metabolic process, negative regulation of transcription DNA-templated, positive regulation of transcription from RNA polymerase II promoter (Table 3). For cellular component and molecular function, the most impacted GO terms for differentially expressed transcripts were assigned to neurofilament and structural constituent of cytoskeleton, respectively (Supplementary Table S7). KEGG pathway analysis revealed that several transcripts associated with protein digestion and absorption, ECM-receptor interaction, and amyotrophic lateral sclerosis were differentially expressed (Supplementary Table S7). A Venn diagram analysis revealed that 48 DETs were common between mifepristone and eplerenone treatments (Supplementary Figure S2), mainly associated to intermediate filament organization biological process.
TABLE 3. Biological process GO terms of differentially expressed transcripts in common between the vehicle vs. eplerenone groups.
To understand the effects of administration of a GR antagonist in cortisol-induced gene expression, we determined those DETs that showed a differential expression in the combined treatment of cortisol and mifepristone respect to cortisol administration (cortisol vs. mifepristone/cortisol). The comparative analysis identified 135 DETs (76 up and 59 down). The complete list of the differentially expressed transcripts is included in Supplementary Table S8. DETs were significantly enriched in biological processes, such as inflammatory response, metabolic process, and chloride transmembrane transport. Among others relevant biological processes enriched include oxidation-reduction process, proteolysis, ion transmembrane transport, adaptive immune response, cell proliferation, response to hypoxia, and protein folding (Table 4). In cellular components and molecular function, we found extracellular exosome and chaperone binding as the most impacted, respectively (Supplementary Table S9). KEGG pathway analysis revealed that several transcripts associated with leukocyte transendothelial migration, neutrophil extracellular trap formation, and leishmaniasis were differentially expressed (Supplementary Table S9).
TABLE 4. Biological process GO terms of differentially expressed transcripts in common between the cortisol vs. mifepristone/cortisol groups.
Similarly, the comparative analysis of cortisol vs. eplerenone/cortisol, identified 68 DETs (22 up and 46 down). The complete list of the differentially expressed transcripts is included in Supplementary Table S10. We found that the most enriched biological processes were muscle contraction, sarcomere organization, and cardiac muscle contraction. Among others relevant biological processes enriched include skeletal muscle contraction, transition between fast and slow fiber, muscle filament sliding, circadian regulation of gene expression, positive regulation of transcription DNA-templated, regulation of cell cycle, and regulation of neuron differentiation (Table 5). In cellular component and molecular function, sarcomere and actin binding were highly represented, respectively (Supplementary Table S11). In KEGG pathways, hypertrophic cardiomyopathy, dilated cardiomyopathy, and cardiac muscle contraction were overrepresented (Supplementary Table S11). A Venn diagram analysis revealed that only 2 DETs (zfand5, tnni2) were common between cortisol vs. mifepristone-cortisol and cortisol vs. eplerenone-cortisol (Supplementary Table S3), mainly associated to skeletal system morphogenesis. In all experimental groups no significant differences were detected in the expression of gr1, gr2, and mr (Supplementary Table S12).
TABLE 5. Biological process GO terms of differentially expressed transcripts in common between the cortisol vs. eplerenone/cortisol groups.
To validate the RNA-seq analysis and the differential participation of GR in contrasting biological processes, we selected a representative gene of inflammatory response (cxcr2), proteolysis (c3), and ion transmembrane transport (clca3p). Similarly, to determine the differential participation of MR, we selected a representative gene of muscle contraction (myh2), and regulation of cell cycle (gadd45g). The mRNA expression of cxcr2, c3, clca3p, myh2, gadd45g were monitored in myotube lysates 1, 3, and 6 h following cortisol treatment (500 nM) in the presence or absence of mifepristone (50 nM) or eplerenone (50 nM). At 1 h post-treatment, maximum increases in clca3p mRNA expressions were observed (Figure 2A). At 3 h post-treatment, maximum increases in cxcr2 mRNA expressions were observed (Figure 2B). At 6 h post-treatment, there was a maximum increase in c3 mRNA expression (Figure 2C). At 6 h post-treatment, there was a maximum decrease in myh2 mRNA expression (Figure 2D). No significant changes were observed in the expression of gadd45g under cortisol treatment (Figure 2E). Pretreatment of skeletal myotubes with the mifepristone significantly inhibited the cortisol-induced up-regulation of cxcr2, c3, and clca3p. Pretreatment of skeletal myotubes with the eplerenone significantly inhibited the cortisol-induced down-regulation of myh2. Significant changes in gene expression were observed for gadd45g under cortisol treatment in the presence of eplerenone.
FIGURE 2. qPCR validation of selected candidate transcripts in rainbow trout myotubes. (A–E) cxcr2, c3, clca3p, myh2, gadd45g expression in rainbow trout myotubes stimulated with cortisol (CORT) in the presence or absence of mifepristone (MIFE) or eplerenone (EPLE) for each indicated times. mRNA levels were analyzed by RT-qPCR and showed as a relative expression normalized against fau (40S ribosomal protein S30) as a housekeeping gene. The results are expressed as a mean ± SEM, (n = 6). The “*” (p < .05) and “**” (p < .01) represent significant differences compared with the vehicle, mifepristone, eplerenone groups. Abbreviations: cxcr2: C-X-C chemokine receptor type 2; c3: Complement C3; clca3p: Calcium-activated chloride channel regulator family member 3; myh2: Myosin-2; gadd45g: Growth arrest and DNA damage-inducible protein.
In the present study, we determined the potential contribution of glucocorticoid and mineralocorticoid receptors in global transcriptomic response of fish skeletal muscle induced by cortisol. It is important to mention that the control condition of our trials (vehicle group) corresponds to rainbow trout anesthetized with benzocaine and treated with metyrapone, registering a slight (non-significant) increase in plasma cortisol levels respect to non-manipulated individuals. In addition, the intraperitoneal administration of 10 mg/kg of plasmatic cortisol reached higher levels compared to a stressing condition. It has been described that typical cortisol elevations in response to acute stress in rainbow trout tend to range approximately ∼100 ng/mL (López-Patiño et al., 2014) but there are reported notable exceptions in other salmonid species (Maule et al., 1988; Mazik et al., 1991). The protocol implemented in this study has been previously validated by several publications (Aedo et al., 2019a; Aedo et al., 2019c; Aedo et al., 2019b; Aravena-Canales et al., 2021). Similarly, the co-administration of cortisol with mifepristone or eplerenone achieved similar plasma concentrations validating our experimental design. We determined that cortisol administration induced an increase in plasma glucose levels, which was not suppressed by the administration of mifepristone. Although this result is contradictory to that reported in higher vertebrates (Castinetti et al., 2010), we estimate that this difference may be due to the concentration of mifepristone used as well as its route of administration. However, additional experiments will be required to demonstrate this hypothesis. In addition, the administration of mifepristone or eplerenone alone did not induce changes in plasma glucose levels. These results agree with observations obtained in the same species (Vijayan et al., 1994).
To better identify the molecular mechanism mediated by GR or MR in skeletal muscle, we analyzed gene expression through RNA-seq. In general terms, we determined in cortisol vs. mifepristone/cortisol analysis that GR has a potential participation in the expression of transcripts associated with inflammatory response, ATP metabolic process, chloride transmembrane transport, oxidation-reduction process, proteolysis, ion transmembrane transport, adaptive immune response, among others. On the other hand, in cortisol vs. eplerenone/cortisol analysis, MR has a potential participation in the expression of transcripts associated with muscle contraction, sarcomere organization, transition between fast and slow fiber, muscle filament sliding, circadian regulation of gene expression, positive regulation of transcription, regulation of cell cycle. It is important to emphasize that the results obtained indicate potential targets mediated by GR and MR. This is mainly due to the limitation in determining the in vivo specificity of mifepristone and eplerenone as GR and MR antagonists, and also because the analyzes of differential expression were circumscribed to paired comparisons (cortisol vs. antagonist/cortisol). However, for future experiments it will be important to consider the incorporation of controls to determine the specificity of both antagonists, as well as to include other differential expression comparative analyzes such as antagonist vs. antagonist/cortisol. Interestingly, the results obtained by this in silico approach were validated using primary culture of rainbow trout muscle cells, revealing the regulatory role of GR in inflammatory response, proteolysis, and ion transmembrane transport. MR has a regulatory role in muscle contraction and regulation of cell cycle. These results suggest the differential participation of GR and MR in skeletal muscle biological processes in lower vertebrates, as has been described in mammals (Howard et al., 2022).
As expected, exogenous cortisol modulated 3 hours post administration the differential expression of a reduced number of transcripts. These transcripts were associated with biological processes such as cell-cell adhesion, ion transport, proteolysis, among other processes. These categories of biological processes modulated by cortisol are consistent, with recent studies describing the genomic and non-genomic effects of cortisol on the regulation of gene expression in rainbow trout (Oncorhynchus mykiss) and Gilthead Sea Bream (Sparus aurata) skeletal muscle (Aedo et al., 2019a; Aedo et al., 2019c; Aedo et al., 2019b; Aedo et al., 2021; Aravena-Canales et al., 2021). Interestingly, in the present study, glucose transport appears as a category of biological processes overrepresented under cortisol treatment. Although increases in plasma glucose after stress events have been widely described in species like Coho salmon (Oncorhynchus kisutch) or European eel (Anguilla) (Wendelaar Bonga, 1997; Mommsen et al., 1999; Kuo et al., 2013), reports associated with modulation of genes associated to glucose transport mediated by cortisol in vertebrate skeletal muscle are limited (Gray et al., 2006). Among the differentially expressed transcripts associated with glucose transport, we identified in the present study slc2a11 and slc5a1. slc2a11 encodes for a class II sugar transport facilitator (GLUT11), and slc5a1 encodes for sodium/glucose cotransporter 1 (SGLT1). Although both transporters have shown increases in their expression under glucocorticoid treatment in mammalian models, there are no antecedents reported in lower vertebrates (Zhao and Keating, 2007). We hypothesize that slc2a11 and slc5a1 differential expression contribute to the increase in plasma glucose in fish under stress. These transcripts did not show changes in their differential expression in the presence of mifepristone or eplerenone, suggesting an alternative mechanism in their regulation. Interestingly, it has been described in mammalian myotubes that glucocorticoids inhibit glucose uptake via a non-genomic mechanisms (Gong et al., 2016), which suggests a potential non-genomic mechanism in their expression. Taken together, these results confirm the fundamental role of cortisol in glucose homeostasis during the early response to stress. It has also been described that prolonged cortisol secretion induces catabolic-related process, mainly mediated by ubiquitin proteasome (UPS) and autophagy lysosomal (ALS) systems (Aedo et al., 2015; Valenzuela et al., 2018). Consistently, we observed in the present work changes in the expression of transcripts associated with proteolysis.
Among the biological processes modulated by GR, we identified proteolysis and inflammatory response. It is well known that stressful conditions can differentially affect the immune response depending on stressor duration (Rebl and Goldammer, 2018). An acute stressor stimulates the immune response, particularly innate responses, while a chronic stressor suppresses the immune response, enhancing infection susceptibility (Nardocci et al., 2014). We determined that GR modulated the expression of c3 and cxcr2, results that were consistent with in vitro validation in rainbow trout myotubes. C3 protein plays a central role in the activation of the complement system. The complement is a part of the immune system and consists of multiple components with biological functions such as defense against pathogens and immunomodulation (Zheng et al., 2022). The complement system is an ancient defense mechanism present in invertebrates and vertebrate (Bavia et al., 2022). Concordantly, it has been reported in mammals that glucocorticoid receptor antagonist mifepristone inhibited C3 synthesis induced by dexamethasone or hydrocortisone in human alveolar epithelial cell line (Zach et al., 1993). In fish, it has been determined that Eurasian perch (Perca fluviatilis) under repeated emersions stress has a reduced c3 expression (Douxfils et al., 2014). More recently, in rainbow trout it was determined that the acute stress associated with air exposure stress induces the upregulation of c3 expression (Khansari et al., 2019). cxcr2 encodes for receptor for interleukin-8 which is a powerful neutrophil chemotactic factor (Matsushima et al., 2022). In mammals, has been observed that blocking of GR with mifepristone significantly diminished the expression of cxcr2 in an in vitro murine allogeneic skin transplantation model induced by dexamethasone (Liao et al., 2014). In fish, the modulation of cxcr2 expression is unclear, although there are reports linking the expression of its ligand with GR (Philip et al., 2012).
Other biological processes overrepresented under cortisol treatment mediated by GR is ion transmembrane transport. clca3p encodes for a calcium-activated chloride channel regulator family member 3 (Altamura et al., 2020). Although there are no previous reports linking stress or cortisol with changes in the expression of this gene in fish skeletal muscle, the role of cortisol in ion and chloride transport is well-documented. Cortisol has a fundamental participation in the adaptation of teleost to a seawater environment through the upregulation of the expression levels of nkaa1 (Sodium/potassium-transporting ATPase subunit alpha), nkcc (Sodium chloride cotransporter), and cftr (Cystic fibrosis transmembrane conductance regulator) in gills (Kiilerich et al., 2007; Kiilerich et al., 2011). Cortisol is also involved in adaptation to fresh water. In the gills of euryhaline teleost, cortisol increases the expression of nkaa1a, which participates in ion uptake by chloride cells in fresh water (McCormick et al., 2008). In agreement with our observations, it has been determined that ion transmembrane transport associated to the osmoregulatory role of cortisol is mediated by GR, but not by MR. In Atlantic salmon the administration of mifepristone completely block cortisol-induced expression of nkaa1a and nkaa1b (McCormick et al., 2008; Tipsmark et al., 2009). Furthermore, it has been determined that translational knockdown of GR inhibited sodium uptake in zebrafish (Kumai et al., 2012).
Among the biological processes modulated by MR, we identified regulation of cell cycle. gadd45g encodes for growth arrest and DNA damage-inducible protein gamma (Patel et al., 2022). It was found that aldosterone treatment of human renal epithelial cells resulted in significant up-regulation of gadd45g. This up-regulation was inhibited by spironolactone, a MR antagonist (Kellner et al., 2003). Interestingly, it has been determined that in vertebrates gadd45g is essential for primary sex determination and testis development (Johnen et al., 2013). Consistently, it has been reported on the role of the MR in gonadal differentiation and fish reproduction. In vitro cortisol treatment in testis primary culture of Japanese eels (Anguilla japonica) induced DNA replication and improves MR-induced spermatogonia proliferation (Ozaki et al., 2006). It was found that MR is expressed in the testicular tissue of rainbow trout, and MR expression increased close to spermiation (Milla et al., 2008). The relationship between the mineralocorticoid receptor and cell cycle regulation has recently been addressed in a murine myotubes. It has been determined that aldosterone inhibited the process of muscle cell differentiation through increased intracellular oxidative stress, a phenomenon that was blocked in the presence of eplerenone (Lee et al., 2021), suggesting a fundamental role of MR in the modulation of muscle cell proliferation and differentiation.
In the present work, one of the most impacted biological processes under treatment with eplerenone corresponds to skeletal muscle contraction. myh2 encodes for myosin 2, a fundamental protein for skeletal muscle cytoskeleton organization. Our observations are consistent with results published in human skeletal muscle (Howard et al., 2022). Similar to teleost skeletal muscle, MR is present in various types of mammals muscle groups, as well as in differentiated myoblasts and myotubes (Chadwick et al., 2015). The treatment of human myotubes combined with aldosterone and eplerenone modulated the expression of genes associated with muscle contraction, in particular myh2 expression (Chadwick et al., 2017a). These findings also agree with recent observations in zebrafish. It has been described that during early development, exogenous glucocorticoid (GC) administration reduces body mass in vertebrates, and this is associated mainly with the glucocorticoid receptor (GR) activation. However, a recently developed work using ubiquitous GR and MR knockout zebrafish (Danio rerio) in combination with exogenous cortisol treatment revealed that MR activation favors protein deposition and GR activation stimulates proteolysis, while their combined activation is involved in cortisol-mediated growth suppression (Faught and Vijayan, 2020). The same group described that postnatal triglyceride accumulation is regulated by mineralocorticoid receptor activation under basal and stress conditions. Using MR and GR knockout zebrafish, it was demonstrated that MR activation, is involved in triglyceride accumulation during the larval development post feeding (Faught and Vijayan, 2019). However, lipid and triglyceride metabolism were not categories represented under the eplerenone treatments. In addition, we also determined that the administration of mifepristone and eplerenone alone induce changes in gene expression in rainbow trout skeletal muscle. Among the biological processes modulated by both antagonists are cell adhesion, muscle contraction, and transcription. Interestingly, in a study carried out in human myoblasts, the administration of both antagonists modulated the differential expression of a small number of genes in categories similar to those reported in our analysis (Chadwick et al., 2017b), suggesting conserved functionalities of both receptors between higher and lower vertebrates.
In the present study, we identified for the first time a set of DETs and biological processes, differentially induced by cortisol and the potentially modulated by GR and MR in rainbow trout skeletal muscle. In cortisol vs. mifepristone/cortisol group, biological processes such as inflammatory response, ion transmembrane transport, proteolysis were overrepresented. In cortisol vs. eplerenone/cortisol group, BP were significantly enriched in muscle contraction, and regulation of cell cycle. These results suggest that both receptors have a differential participation to stress response in teleost skeletal muscle.
The datasets presented in this study can be found in online repositories. The names of the repository/repositories and accession number(s) can be found below: https://www.ncbi.nlm.nih.gov/bioproject/PRJNA518130.
The animal study was reviewed and approved by Bioethical committees of the Universidad Andres Bello.
JA: Methodology, Data curation, Writing–original draft, Writing–review and editing. DA-C: Methodology, Formal analysis, Writing–original draft, Writing–review, and editing. RZ: Methodology, Data curation, Writing–review, and editing. AM: Investigation, Supervision, Funding acquisition, Writing–review, and editing. JV: Conceptualization, Investigation, Supervision, Funding acquisition, Writing–review, and editing.
This work was supported by: Fondo Nacional de Desarrollo Científico y Tecnológico (FONDECYT) Grant 1201498 (to JV) and 3210050 (to JA); Fondo de Financiamiento de Centros de Investigación en Áreas Prioritarias (FONDAP) Grant INCAR 15110027, Concurso de Apoyo a Centros de Excelencia en Investigación FONDAP 2022 1522A0004. DA-C also acknowledges support received by ANID, Ph.D. Scholarship 21220218.
The authors declare that the research was conducted in the absence of any commercial or financial relationships that could be construed as a potential conflict of interest.
All claims expressed in this article are solely those of the authors and do not necessarily represent those of their affiliated organizations, or those of the publisher, the editors and the reviewers. Any product that may be evaluated in this article, or claim that may be made by its manufacturer, is not guaranteed or endorsed by the publisher.
The Supplementary Material for this article can be found online at: https://www.frontiersin.org/articles/10.3389/fphys.2022.1048008/full#supplementary-material
Aedo J., Aravena-Canales D., Ruiz-Jarabo I., Oyarzún R., Molina A., Martínez-Rodríguez G., et al. (2021). Differential metabolic and transcriptional responses of gilthead seabream (sparus aurata) administered with cortisol or cortisol-BSA. Animals 11, 3310. doi:10.3390/ani11113310
Aedo J. E., Fuentes-Valenzuela M., Molina A., Valdés J. A. (2019a). Quantitative proteomics analysis of membrane glucocorticoid receptor activation in rainbow trout skeletal muscle. Comp. Biochem. Physiology Part D Genomics Proteomics 32, 100627. doi:10.1016/j.cbd.2019.100627
Aedo J. E., Maldonado J., Aballai V., Estrada J. M., Bastias-Molina M., Meneses C., et al. (2015). mRNA-seq reveals skeletal muscle atrophy in response to handling stress in a marine teleost, the red cusk-eel (Genypterus chilensis). BMC Genomics 16, 1024. doi:10.1186/s12864-015-2232-7
Aedo J. E., Ruiz-Jarabo I., Martínez-Rodríguez G., Boltaña S., Molina A., Valdés J. A., et al. (2019b). Contribution of non-canonical cortisol actions in the early modulation of glucose metabolism of Gilthead Sea Bream (sparus aurata). Front. Endocrinol. 10, 779. doi:10.3389/fendo.2019.00779
Aedo J. E., Zuloaga R., Bastias-Molina M., Meneses C., Boltaña S., Molina A., et al. (2019c). Early transcriptomic responses associated with the membrane-initiated action of cortisol in the skeletal muscle of rainbow trout (Oncorhynchus mykiss). Physiol. Genomics 51, 596–606. doi:10.1152/physiolgenomics.00042.2019
Altamura C., Desaphy J.-F., Conte D., De Luca A., Imbrici P. (2020). Skeletal muscle ClC-1 chloride channels in health and diseases. Pflugers Arch. - Eur. J. Physiol. 472, 961–975. doi:10.1007/s00424-020-02376-3
Aluru N., Vijayan M. M. (2009). Stress transcriptomics in fish: A role for genomic cortisol signaling. General Comp. Endocrinol. 164, 142–150. doi:10.1016/j.ygcen.2009.03.020
Aravena-Canales D., Aedo J. E., Molina A., Valdés J. A. (2021). Regulation of the early expression of MAFbx/atrogin-1 and MuRF1 through membrane-initiated cortisol action in the skeletal muscle of rainbow trout. Comp. Biochem. Physiology Part B Biochem. Mol. Biol. 253, 110565. doi:10.1016/j.cbpb.2021.110565
Baker M. E. (2003). Evolution of glucocorticoid and mineralocorticoid responses: Go fish. Endocrinology 144, 4223–4225. doi:10.1210/en.2003-0843
Baker M. E., Funder J. W., Kattoula S. R. (2013). Evolution of hormone selectivity in glucocorticoid and mineralocorticoid receptors. J. Steroid Biochem. Mol. Biol. 137, 57–70. doi:10.1016/j.jsbmb.2013.07.009
Baker M. E., Katsu Y. (2019). “Evolution of the mineralocorticoid receptor,” in Vitamins and hormones (Elsevier), 17–36. doi:10.1016/bs.vh.2018.10.009
Balasch J. C., Tort L. (2019). Netting the stress responses in fish. Front. Endocrinol. 10, 62. doi:10.3389/fendo.2019.00062
Bavia L., Santiesteban-Lores L. E., Carneiro M. C., Prodocimo M. M. (2022). Advances in the complement system of a teleost fish, Oreochromis niloticus. Fish Shellfish Immunol. 123, 61–74. doi:10.1016/j.fsi.2022.02.013
Borski R. J., Hyde G. N., Fruchtman S., Tsai W. S. (2001). Cortisol suppresses prolactin release through a non-genomic mechanism involving interactions with the plasma membrane. Comp. Biochem. Physiol. B, Biochem. Mol. Biol. 129, 533–541. doi:10.1016/s1096-4959(01)00358-x
Bury N. R., Sturm A. (2007). Evolution of the corticosteroid receptor signalling pathway in fish. Gen. Comp. Endocrinol. 153, 47–56. doi:10.1016/j.ygcen.2007.03.009
Castinetti F., Conte-Devolx B., Brue T. (2010). Medical treatment of cushing’s syndrome: Glucocorticoid receptor antagonists and mifepristone. Neuroendocrinology 92, 125–130. doi:10.1159/000314224
Chadwick J. A., Bhattacharya S., Lowe J., Weisleder N., Rafael-Fortney J. A. (2017a). Renin-angiotensin-aldosterone system inhibitors improve membrane stability and change gene-expression profiles in dystrophic skeletal muscles. Am. J. Physiology-Cell Physiology 312, C155–C168. doi:10.1152/ajpcell.00269.2016
Chadwick J. A., Hauck J. S., Gomez-Sanchez C. E., Gomez-Sanchez E. P., Rafael-Fortney J. A. (2017b). Gene expression effects of glucocorticoid and mineralocorticoid receptor agonists and antagonists on normal human skeletal muscle. Physiol. Genomics 49, 277–286. doi:10.1152/physiolgenomics.00128.2016
Chadwick J. A., Hauck J. S., Lowe J., Shaw J. J., Guttridge D. C., Gomez-Sanchez C. E., et al. (2015). Mineralocorticoid receptors are present in skeletal muscle and represent a potential therapeutic target. FASEB J. 29, 4544–4554. doi:10.1096/fj.15-276782
Douxfils J., Lambert S., Mathieu C., Milla S., Mandiki S. N. M., Henrotte E., et al. (2014). Influence of domestication process on immune response to repeated emersion stressors in Eurasian perch (Perca fluviatilis, L.). Comp. Biochem. Physiology Part A Mol. Integr. Physiology 173, 52–60. doi:10.1016/j.cbpa.2014.03.012
Ellis T., Yildiz H. Y., López-Olmeda J., Spedicato M. T., Tort L., Øverli Ø., et al. (2012). Cortisol and finfish welfare. Fish Physiology Biochem. 38, 163–188. doi:10.1007/s10695-011-9568-y
Espinoza M. B., Aedo J. E., Zuloaga R., Valenzuela C., Molina A., Valdés J. A. (2017). Cortisol induces reactive oxygen species through a membrane glucocorticoid receptor in rainbow trout myotubes: C ortisol- M ediated N on- G enomic S ignaling in F ISH. J. Cell. Biochem. 118, 718–725. doi:10.1002/jcb.25676
Faught E., Vijayan M. M. (2020). Glucocorticoid and mineralocorticoid receptor activation modulates postnatal growth. J. Endocrinol. 244, 261–271. doi:10.1530/JOE-19-0358
Faught E., Vijayan M. M. (2019). Postnatal triglyceride accumulation is regulated by mineralocorticoid receptor activation under basal and stress conditions. J. Physiol. 597, 4927–4941. doi:10.1113/JP278088
Fuller J. D., Mason P. A., Fraser R. (1976). Gas-liquid chromatography of corticosteroids in plasma of salmonidae. J. Endocrinol. 71, 163–164. doi:10.1677/joe.0.0710163
Gilmour K. M. (2005). Mineralocorticoid receptors and hormones: Fishing for answers. Endocrinology 146, 44–46. doi:10.1210/en.2004-1390
Gong H., Liu L., Ni C.-X., Zhang Y., Su W.-J., Lian Y.-J., et al. (2016). Dexamethasone rapidly inhibits glucose uptake via non-genomic mechanisms in contracting myotubes. Archives Biochem. Biophysics 603, 102–109. doi:10.1016/j.abb.2016.05.020
Gray S., Stonestreet B. S., Thamotharan S., Sadowska G. B., Daood M., Watchko J., et al. (2006). Skeletal muscle glucose transporter protein responses to antenatal glucocorticoids in the ovine fetus. J. Endocrinol. 189, 219–229. doi:10.1677/joe.1.06589
Greenwood A. K., Butler P. C., White R. B., DeMarco U., Pearce D., Fernald R. D. (2003). Multiple corticosteroid receptors in a teleost fish: Distinct sequences, expression patterns, and transcriptional activities. Endocrinology 144, 4226–4236. doi:10.1210/en.2003-0566
Howard Z. M., Gomatam C. K., Piepho A. B., Rafael-Fortney J. A. (2022). Mineralocorticoid receptor signaling in the inflammatory skeletal muscle microenvironments of muscular dystrophy and acute injury. Front. Pharmacol. 13, 942660. doi:10.3389/fphar.2022.942660
Johnen H., González-Silva L., Carramolino L., Flores J. M., Torres M., Salvador J. M. (2013). Gadd45g is essential for primary sex determination, male fertility and testis development. PLoS ONE 8, e58751. doi:10.1371/journal.pone.0058751
Kellner M., Peiter A., Hafner M., Feuring M., Christ M., Wehling M., et al. (2003). Early aldosterone up-regulated genes: New pathways for renal disease? Kidney Int. 64, 1199–1207. doi:10.1046/j.1523-1755.2003.00216.x
Khansari A. R., Balasch J. C., Vallejos-Vidal E., Teles M., Fierro-Castro C., Tort L., et al. (2019). Comparative study of stress and immune-related transcript outcomes triggered by Vibrio anguillarum bacterin and air exposure stress in liver and spleen of gilthead seabream (Sparus aurata), zebrafish (Danio rerio) and rainbow trout (Oncorhynchus mykiss). Fish Shellfish Immunol. 86, 436–448. doi:10.1016/j.fsi.2018.11.063
Kiilerich P., Kristiansen K., Madsen S. S. (2007). Cortisol regulation of ion transporter mRNA in Atlantic salmon gill and the effect of salinity on the signaling pathway. J. Endocrinol. 194, 417–427. doi:10.1677/JOE-07-0185
Kiilerich P., Tipsmark C. K., Borski R. J., Madsen S. S. (2011). Differential effects of cortisol and 11-deoxycorticosterone on ion transport protein mRNA levels in gills of two euryhaline teleosts, Mozambique tilapia (Oreochromis mossambicus) and striped bass (Morone saxatilis). J. Endocrinol. 209, 115–126. doi:10.1530/JOE-10-0326
Kumai Y., Nesan D., Vijayan M. M., Perry S. F. (2012). Cortisol regulates Na+ uptake in zebrafish, Danio rerio, larvae via the glucocorticoid receptor. Mol. Cell. Endocrinol. 364, 113–125. doi:10.1016/j.mce.2012.08.017
Kuo T., Harris C. A., Wang J.-C. (2013). Metabolic functions of glucocorticoid receptor in skeletal muscle. Mol. Cell. Endocrinol. 380, 79–88. doi:10.1016/j.mce.2013.03.003
Lee J. Y., Kim D. A., Choi E., Lee Y. S., Park S. J., Kim B.-J. (2021). Aldosterone inhibits in vitro myogenesis by increasing intracellular oxidative stress via mineralocorticoid receptor. Endocrinol. Metab. 36, 865–874. doi:10.3803/EnM.2021.1108
Liao J., Wang X., Bi Y., Shen B., Shao K., Yang H., et al. (2014). Dexamethasone potentiates myeloid-derived suppressor cell function in prolonging allograft survival through nitric oxide. J. Leukoc. Biol. 96, 675–684. doi:10.1189/jlb.2HI1113-611RR
López-Patiño M. A., Hernández-Pérez J., Gesto M., Librán-Pérez M., Míguez J. M., Soengas J. L. (2014). Short-term time course of liver metabolic response to acute handling stress in rainbow trout, Oncorhynchus mykiss. Comp. Biochem. Physiology Part A Mol. Integr. Physiology 168, 40–49. doi:10.1016/j.cbpa.2013.10.027
Matsushima K., Yang D., Oppenheim J. J. (2022). Interleukin 8 and MCAF: Novel inflammatory cytokines inducible by IL 1 and TNF. Cytokine 153, 2–13. doi:10.1016/1043-4666(89)91043-0
Maule A. G., Schreck C. B., Bradford C. S., Barton B. A. (1988). Physiological effects of collecting and transporting emigrating juvenile chinook salmon past dams on the columbia river. Trans. Am. Fish. Soc. 117, 245–261. doi:10.1577/1548-8659(1988)117<0245:peocat>2.3.co;2
Mazik P. M., Simco B. A., Parker N. C. (1991). Influence of water hardness and salts on survival and physiological characteristics of striped bass during and after transport. Trans. Am. Fish. Soc. 120, 121–126. doi:10.1577/1548-8659(1991)120<0121:iowhas>2.3.co;2
McCormick S. D., Regish A., O’Dea M. F., Shrimpton J. M. (2008). Are we missing a mineralocorticoid in teleost fish? Effects of cortisol, deoxycorticosterone and aldosterone on osmoregulation, gill Na+, K+-ATPase activity and isoform mRNA levels in atlantic salmon. General Comp. Endocrinol. 157, 35–40. doi:10.1016/j.ygcen.2008.03.024
Milla S., Terrien X., Sturm A., Ibrahim F., Giton F., Fiet J., et al. (2008). Plasma 11-deoxycorticosterone (DOC) and mineralocorticoid receptor testicular expression during rainbow trout Oncorhynchus mykiss spermiation: Implication with 17alpha, 20beta-dihydroxyprogesterone on the milt fluidity? Reproductive Biol. Endocrinol. 6, 19. doi:10.1186/1477-7827-6-19
Mommsen T. P., Vijayan M. M., Moon T. W. (1999). Cortisol in teleosts: Dynamics, mechanisms of action, and metabolic regulation. Rev. Fish Biol. Fish. 9, 211–268. doi:10.1023/A:1008924418720
Nardocci G., Navarro C., Cortés P. P., Imarai M., Montoya M., Valenzuela B., et al. (2014). Neuroendocrine mechanisms for immune system regulation during stress in fish. Fish Shellfish Immunol. 40, 531–538. doi:10.1016/j.fsi.2014.08.001
Ozaki Y., Higuchi M., Miura C., Yamaguchi S., Tozawa Y., Miura T. (2006). Roles of 11beta-hydroxysteroid dehydrogenase in fish spermatogenesis. Endocrinology 147, 5139–5146. doi:10.1210/en.2006-0391
Patel K., Murray M. G., Whelan K. A. (2022). “Roles for GADD45 in development and cancer,” in Gadd45 stress sensor genes advances in experimental medicine and biology. Editors M. R. Zaidi, and D. A. Liebermann (Cham: Springer International Publishing), 23–39. doi:10.1007/978-3-030-94804-7_2
Philip A. M., Daniel Kim S., Vijayan M. M. (2012). Cortisol modulates the expression of cytokines and suppressors of cytokine signaling (SOCS) in rainbow trout hepatocytes. Dev. Comp. Immunol. 38, 360–367. doi:10.1016/j.dci.2012.07.005
Rebl A., Goldammer T. (2018). Under control: The innate immunity of fish from the inhibitors’ perspective. Fish Shellfish Immunol. 77, 328–349. doi:10.1016/j.fsi.2018.04.016
Suarez-Bregua P., Guerreiro P. M., Rotllant J. (2018). Stress, glucocorticoids and bone: A review from mammals and fish. Front. Endocrinol. 9, 526. doi:10.3389/fendo.2018.00526
Tipsmark C. K., Jørgensen C., Brande-Lavridsen N., Engelund M., Olesen J. H., Madsen S. S. (2009). Effects of cortisol, growth hormone and prolactin on gill claudin expression in Atlantic salmon. General Comp. Endocrinol. 163, 270–277. doi:10.1016/j.ygcen.2009.04.020
Valenzuela C. A., Zuloaga R., Mercado L., Einarsdottir I. E., Björnsson B. T., Valdés J. A., et al. (2018). Chronic stress inhibits growth and induces proteolytic mechanisms through two different nonoverlapping pathways in the skeletal muscle of a teleost fish. Am. J. Physiology-Regulatory, Integr. Comp. Physiology 314, R102–R113. doi:10.1152/ajpregu.00009.2017
Vijayan M. M., Reddy P. K., Leatherland J. F., Moon T. W. (1994). The effects of cortisol on hepatocyte metabolism in rainbow trout: A study using the steroid analogue RU486. General Comp. Endocrinol. 96, 75–84. doi:10.1006/gcen.1994.1160
Wendelaar Bonga S. E. (1997). The stress response in fish. Physiol. Rev. 77, 591–625. doi:10.1152/physrev.1997.77.3.591
Zach T. L., Herrman V. A., Hill L. D., Leuschen M. P. (1993). Effect of steroids on the synthesis of complement C3 in a human alveolar epithelial cell line. Exp. Lung Res. 19, 603–616. doi:10.3109/01902149309031731
Zhao F.-Q., Keating A. (2007). Functional properties and genomics of glucose transporters. CG 8, 113–128. doi:10.2174/138920207780368187
Keywords: cortisol, glucocorticoid receptor, mineralocorticoid receptor, RNA-seq, skeletal muscle
Citation: Aedo JE, Zuloaga R, Aravena-Canales D, Molina A and Valdés JA (2023) Role of glucocorticoid and mineralocorticoid receptors in rainbow trout (Oncorhynchus mykiss) skeletal muscle: A transcriptomic perspective of cortisol action. Front. Physiol. 13:1048008. doi: 10.3389/fphys.2022.1048008
Received: 19 September 2022; Accepted: 22 December 2022;
Published: 06 January 2023.
Edited by:
Jonas Roland Knudsen, University of Copenhagen, DenmarkReviewed by:
Danilo Lustrino, Federal University of Sergipe, BrazilCopyright © 2023 Aedo, Zuloaga, Aravena-Canales, Molina and Valdés. This is an open-access article distributed under the terms of the Creative Commons Attribution License (CC BY). The use, distribution or reproduction in other forums is permitted, provided the original author(s) and the copyright owner(s) are credited and that the original publication in this journal is cited, in accordance with accepted academic practice. No use, distribution or reproduction is permitted which does not comply with these terms.
*Correspondence: Juan Antonio Valdés, anZhbGRlc0B1bmFiLmNs
†These authors have contributed equally to this work
Disclaimer: All claims expressed in this article are solely those of the authors and do not necessarily represent those of their affiliated organizations, or those of the publisher, the editors and the reviewers. Any product that may be evaluated in this article or claim that may be made by its manufacturer is not guaranteed or endorsed by the publisher.
Research integrity at Frontiers
Learn more about the work of our research integrity team to safeguard the quality of each article we publish.