- Department of Anatomy, Histology, and Embryology, Faculty of Medicine, Semmelweis University, Budapest, Hungary
The current review is an update on experimental approaches in which birds serve as model species for the investigation of typical failure symptoms associated with autism spectrum disorder (ASD). The discussion is focused on deficiencies of social behavior, from social interactions of domestic chicks, based on visual and auditory cues, to vocal communication in songbirds. Two groups of pathogenetic/risk factors are discussed: 1) non-genetic (environmental/epigenetic) factors, exemplified by embryonic exposure to valproic acid (VPA), and 2) genetic factors, represented by a list of candidate genes and signaling pathways of diagnostic or predictive value in ASD patients. Given the similarities of birds as experimental models to humans (visual orientation, vocal learning, social cohesions), avian models usefully contribute toward the elucidation of the neural systems and developmental factors underlying ASD, improving the applicability of preclinical results obtained on laboratory rodents. Furthermore, they may predict potential susceptibility factors worthy of investigation (both by animal studies and by monitoring human babies at risk), with potential therapeutic consequence.
Introduction
With many problems in biomedicine, the key to success is finding a suitable model system/species. Experimental models are essential for evidence-based studies, especially those of the interventional type (which would be impractical or ethically unacceptable to carry out in humans). They also help finding the appropriate level of explanation, blissfully avoiding the traps of Scylla and Charybdis, extreme reductionism (cf. the problem described as “the janitor’s dream” by Calvin, 1998) or undue generalization. Model systems, however, may fail at times. Ever since, there has been an increasing demand for alternative model species (Bolker, 2012; Yartsev, 2017) and phylogenetic comparisons to improve the poor applicability of preclinical results obtained mostly on laboratory rodents (Perrin, 2014).
Autism Spectrum Disorder (ASD) is one of the most common neurodevelopmental disorders associated with altered social behavior. The variable and multifaceted character of the disease necessitates reliable animal models and multilateral approaches. Birds as evolutionary alternatives to mammals, with a wide behavioral repertoire, may well represent a special window of observation. Animal models may help discern genetic vs. non-genetic (epigenetic, environmental) factors in the etiology of ASD (Ergaz et al., 2016). Experimental intervention may help reveal the pathogenetic causes during gestation, and whether such risk factors can be antagonized or reversed.
The current summary is not intended to give a comprehensive account of available animal models for different autism related syndromes (cf. previous thorough reviews, Ergaz et al., 2016; Nicolini and Fahnestock, 2018). Here, we are focusing on those applied on avian species, elaborating on the valproic acid model (an example for non-genetic interventions), and on a set of genetic modifications, in which birds have been used as experimental subjects. Avian models have helped in the past to solve problems such as neural plasticity in early learning (McCabe and Horn, 1994; Rose, 2000), adult neurogenesis (Goldman and Nottebohm, 1983; Nottebohm, 2005), thanks to the existence of common patterns in evolution. In many ways, birds are better models of human behavior than mammals. They are highly visual beings, display vocal communication, even vocal learning, and often live in pairs or flocks, exposed to multiple social signals.
Newly hatched domestic chicks have often been used as models in studies of behavioral neuroscience (Bolhuis and Honey, 1998; Rose, 2000; Zachar et al., 2008), because they can display complex behaviors, not confounded by earlier experience (Rose, 2000). Just like newborn humans, chicks have a predisposition to prefer the proximity of conspecifics (for review see Di Giorgio et al., 2017). Whether such predispositions are affected by autism is a matter of debate (Elsabbagh et al., 2013; Jones and Klin, 2013; Sgadò et al., 2018; Zachar et al., 2019), however, the social bonds based on innate stimulus preferences are certainly impaired by ASD. The social interactions of domestic chicks are based on visual and auditory cues (Koshiba et al., 2013), i.e., traits that are more human-like than the olfactory-biased sociability of most mammals (Brennan and Kendrick, 2006).
There are striking behavioral similarities between children with ASD and domestic chicks with socio-sensory deprivation (Koshiba et al., 2016), further supporting feasibility of the avian model.
Chicks react to social isolation by displaying behaviors aimed at reuniting with conspecifics (Gallup and Suarez, 1980), and they prefer larger groups of siblings over smaller ones (Zachar et al., 2017). The drive to reinstatement can be evaluated by measurement of distress vocalization (Marx et al., 2001; Takeuchi et al., 1996; Yazaki et al., 1999; Montevecchi et al., 1973; Zsedényi et al., 2014). Such innate gregariousness of naïve domestic chicks likely relies on the social brain network (SBN, Goodson, 2005), and affiliation to siblings is likely processed similarly to other social behaviors (Mayer et al., 2017). The mesolimbic dopaminergic reward system is amply interconnected and overlapping with SBN forming the phylogenetically conservative social decision-making network (O'Connell and Hofmann, 2011) (Figure 1). Therefore, the separation-reinstatement paradigm of the young domestic chick can be an appropriate laboratory model of sociability [e.g., the test for group preference (Zachar et al., 2017) and other tests of belongingness or aggregation, see Nishigori et al., 2013].
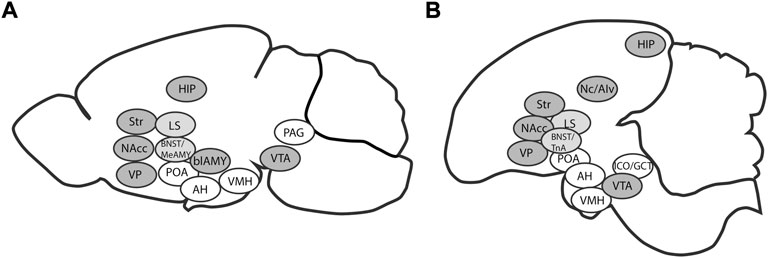
FIGURE 1. Brain regions constituting the social brain network (white), the mesolimbic dopaminergic reward system (dark grey) and their overlap (light grey) in mammals (A) and in birds (B). The two interconnected networks are collectively designated as the social decision-making network. AH: anterior hypothalamus, blAMY: basolateral amygdala (avian homologue Nc/AIv: caudal nidopallium/ventral intermediate arcopallium), BNST: bed nucleus of the stria terminalis, HIP: hippocampus, LS: lateral septum, MeAMY: medial extended amygdala (avian homologue TnA: nucleus taeniae), NAcc: nucleus accumbens, PAG: periaqueductal gray (avian homologue ICO/GCT: intercollicular nucleus/midbrain central gray), POA: preoptic area, Str: striatum, VMH: ventromedial hypothalamus, VP: ventral pallidum, VTA: ventral tegmental area. Based on O'Connell and Hofmann, 2011.
Song learning and singing in oscine birds is often paralleled with the human language (affected by ASD). Remarkably, however, no studies known to us have been reported on effect of embryonic VPA treatment on the vocal behavior of songbirds (either learned singing or innate calls).
Relevant VPA based (or other environmental intervention-based) models should also consider the importance of innate calls. Parental care requires intense cooperation through coordination and synchronization of behavior, and an intense communication between the parents. Zebra finches change their vocal communication over pair formation and during nest building and incubation (Gill et al., 2015). They reduce the amount of distance calls and courtship singing, while increasing the frequency of the low amplitude calls specifically used for short distance communication between the pairs (D’Amelio et al., 2017). Furthermore, the acoustic interactions between the two members of the pair become more synchronized (Gill et al., 2015). Coordinated duetting between the parents may function as negotiations over the parental effort, at least during the egg incubation phase (Boucaud et al., 2016, 2017). Compared to widely studied courtship songs, little is known about the short distance calls (Ter Maat et al., 2014). The neural substrate responsible for these calls overlaps, at least partially, with the brain’s song system (Gobes et al., 2009; Giret et al., 2015), however the different social function implies the involvement of different regions and/or genes. The elaborate male song is a learned behavior, and, in this sense, it is more like human speech than are innate calls. The shorter calls are suitable for individual recognition (Elie and Theunissen, 2018) and for promoting cooperation between individuals, another facet of similarity with human language. The latter is often neglected in the scientific literature on birdsong. Moreover, female zebra finches are also capable of cooperative vocalization. They possess a less developed (but otherwise homologous) neural network for processing song than that of males (Shaughnessy et al., 2019). Such, more specific, songbird models might provide novel insight into cooperative vocalization in normal or pathological states.
Embryonic treatment with VPA
A well-established ASD model for laboratory rodents is prenatal exposure to valproic acid (VPA), a known antiepileptic substance (Rodier et al., 1996, for comprehensive reviews see Roullet et al., 2013; Nicolini and Fahnestock, 2018) and mood stabilizing agent (Cipriani et al., 2013). The key factor in the action of VPA seems to be inhibition of histone deacetylase (Göttlicher et al., 2001), affecting gene expression and transcription during CNS development.
VPA has been used in birds first as a teratogenic agent. Domestic chicken eggs were injected with VPA at critical times of development to study malformations (Barnes et al., 1996; Whitsel et al., 2002; Hsieh et al., 2013), or modifications of gene expression (Akhtar et al., 2015), e.g., reduction of PAX6 (Zosen et al., 2022). The reported distinct and dose-dependent effects of VPA on brain development potentially reflect on behavioral measures (Barnes et al., 1996; Zosen et al., 2022).
In relation to autism-related social deficits, administration of VPA in ovo caused impairment of social behavior (but not imprinting) in chicks (Nishigori et al., 2013). VPA alters the approach response to visual cues resembling to conspecifics such as simulated biological motion (Lorenzi et al., 2019; Matsushima et al., 2022) or face like stimuli (Adiletta et al., 2021), suggesting an early effect on social stimulus processing similar to autistic children. VPA abolished the innate visual predispositions of chicks to hen features, while imprinting remained unaffected (Sgadò et al., 2018). Similarly, in a study by Zachar et al. (2019), early learning (passive avoidance training) and color discrimination were not impaired by VPA treatment. At variance with the results of Sgadò et al. (2018), albeit at a later phase of post-embryonic development, VPA exposure did not affect the innate approach preference of birds for the larger over smaller group of conspecifics, or for companion birds with natural facial features over those with blurred features (Zachar et al., 2019). However, VPA did attenuate social exploration and the recognition of familiar conspecifics, by the end of the third week post-hatch, drawing attention to the importance of early social exploration in human ASD (Zachar et al., 2019). The corollary from these studies is that subtle alterations in innate predispositions and social exploration might well predict the future manifestation of ASD. Therefore, a standardized recording and monitoring of human babies at risk during the early postnatal period would be highly recommended practice.
The valproate model exemplifies the potential role of epigenetic/environmental factors in the pathogenesis of ASD. Other animal models, including those applied mainly in songbirds, are based upon genomic alterations.
Genomic alterations
Deficits in the acquisition of culturally transmitted social skills, including speech and language are important early indicators of ASD (Tager-Flusberg et al., 2005; Mody and Belliveau, 2013; Sperdin and Schaer, 2016). The elaboration of birdsong is often compared to the complexity of human speech (Aamodt et al., 2019). Songbirds may represent useful models for certain aspects of ASD both in terms of vocal communication and sociability. Several candidate genes, common to songbirds and humans, have been described to participate in the production and socially meaningful perception of song/speech (for an overview of zebra finch studies see Panaitof, 2012). Genomic interventions in altricial songbirds may help understand the etiology of some of the failure symptoms in ASD. By contrast, very few studies have tackled the genetic basis of social behavior of precocial birds. Of five candidate genes, TTRAP showed a correlation with social behavior of domestic chicks (Johnsson et al., 2018). Though none of those five genes were confirmed candidates in human autism (Satterstrom et al., 2020), TTRAP is associated with language-related regions (Pinel et al., 2012).
The following account is not intended to cover the ever-expanding plethora of genomic factors that are potentially linked to the pathogenesis of ASD. We merely attempted here to summarize the most promising lines of investigation, in which songbirds played an important part as experimental subjects.
FOXP1, FOXP2
The Forkhead Box transcription factors FOXP1 and FOXP2 were found to be linked to speech and language disorders (Lai et al., 2001), and are among the risk genes for autism (Satterstrom et al., 2020). Similarly distributed in the developing human and songbird language-related centers, they proved to be promising candidates for cross-species studies (Teramitsu et al., 2004). Knockdown of FoxP2 in the basal ganglia song nucleus, Area X, was found to impair singing in zebra finches (Haesler et al., 2007). The importance of FoxP2 in the regulation of singing has been supported by other suppression or overexpression studies (Murugan et al., 2013; Heston and White, 2015). In a more recent zebra finch study, FoxP1 was found to be expressed mainly in striatal-projecting HVC neurons (forebrain mirror neurons). Knockdown of FoxP1 expression in juvenile birds led to a selective learning deficit, affecting the ability to form memories essential for the cultural transmission of behavior (adult model song) (Garcia-Oscos et al., 2021).
Cntnap2
An important target of FOXP2, Contactin-associated protein-like 2 (Cntnap2) (Spiteri et al., 2007) has been identified as an autism susceptibility gene (Alarcón et al., 2008). This gene is considered a risk factor for language-related disorders, including ASD, language impairment, and stuttering (Arking et al., 2008; Li et al., 2010). A specific enrichment of the CNTNAP2 protein was found in the song nuclei of male zebra finches (Condro and White, 2014), pointing to a generalized role in vocal learning across vertebrate species.
FXS, FMRP
Fragile X syndrome (FXS) is the most common inherited form of ASD, characterized by hyperactivity, impulsivity, and anxiety, as well as by defective language development. Many FXS symptoms appear early in life, together with emerging autistic features (Hagerman et al., 2017). A trinucleotide repeat disorder, silencing of the gene leads to the loss of its product, Fragile X mental retardation 1 protein (FMRP). FMRP is an RNA-binding protein regulating the translation of numerous mRNAs instrumental in the development and maintenance of synapses. FXS animal models are based on the loss of neural plasticity and an imbalance between inhibitory and excitatory neuronal circuits, also mimicking certain clinical symptoms of ASD. FMRP is a promising target for therapeutic intervention. The gene and its product have been identified in the vocal control system of the zebra finch, recommended as a model for FXS-associated language disorders (Winograd et al., 2008). Curiously, however, despite obvious therapeutic advantages, experimental interventions on FXS or FMRP, in relation to ASD, have not yet been reported in avian species.
In addition to songbirds, FMRP has been located also in the brainstem auditory nuclei of domestic chicks, with a specific role in dendritic dynamics (Wang et al., 2014) and axonal growth (Wang et al., 2020). In this capacity, FMRP is just one of many genomic factors to regulate axonal pathfinding, some of which have been demonstrated in avian vocal learning-relevant regions, e.g., the SLIT-ROBO system (Wang et al., 2015).
ADNP
ADNP is an essential protein instrumental in brain development and neural plasticity, thereby determining a host of social and cognitive functions potentially malfunctioning in autism. Mutations in ADNP system have been found in human ASD cases (Helsmoortel et al., 2014; Satterstrom et al., 2020). An established experimental model, Adnp± mice develop impairments of cognitive and social behaviors (Vulih-Shultzman et al., 2007), resulting in Alzheimer’s disease related symptoms, as well as autistic features (Malishkevich et al., 2015). Notably, male Adnp± mice are more seriously affected, mimicking a similar prevalence of failure symptoms in human subjects with ASD. The sex- and age-related expression of ADNP mRNA was reported in different areas (cerebellum, cerebrum, brainstem) of the zebra finch (Hacohen-Kleiman et al., 2015), with a distinct sexual dimorphism (young males expressing higher levels of ADNP than females, in agreement with the notion that only males perform courtship singing). The gene expression profile was largely confirmed in the domesticated canary, and ADNP mRNA was found to be enriched mainly in the mesopallium, harboring centers for sensory integration and higher auditory processing (Hacohen-Kleiman et al., 2020).
mTOR
Owing to its role in experience-dependent synaptic plasticity (Garza-Lombó and Gonsebatt, 2016), the Mechanistic Target of Rapamycin (mTOR) signaling cascade has been implicated as a factor in the etiology of ASD, based chiefly on mouse models (Chen et al., 2014; Kazdoba et al., 2016). In an elegant study on zebra finch (Ahmadiantehrani and London, 2017) mTOR signaling was activated in the auditory forebrain by memorization of tutor song in adult males but not in younger males (not old enough to copy song) or in females (who cannot sing). Both the inhibition and constitutive activation of mTOR during tutor experiences diminished copying of tutor song. Remarkably, constitutive mTOR activation lowered the ‘social engagement’ of juvenile zebra finches during tutor experiences, somewhat similarly to the situation found in humans with autism. The findings bear relevance for the role of the onset of mTOR cascade in the encoding of early life experience to determine future behavior.
Glycogen synthase kinase-3 (GSK-3)
GSK-3 is a highly conserved serine/threonine protein kinase that plays a central role in a wide variety of cellular processes associated with cognition and behavior (Beurel et al., 2015). In a recent study on zebra finch, inhibition of the splice variant GSK-3β was found to attenuate social recognition and decision making (Moaraf et al., 2022). Interestingly, birds are “natural knockouts” for the GSK-3α gene (Alon et al., 2011), enabling selective investigation of the effects of GSK-3β. Although GSK-3β is not among the 102 key risk genes recently defined for autism, being the 150th among 18,000 observed genes (Satterstrom et al., 2020), the findings related to social recognition in songbirds may potentially indicate a future involvement and predict a novel line of investigation in this direction.
Novel aspects of convergent genomic regions
Based on a meta-analysis of convergence between avian and human accelerated genomic regions (AR), the important study by Cahill et al. (2021) casts light on the regulation of vocal learning in different clades of birds (“rediscovered” two to three times during avian evolution) and that of human speech. In addition to known AR such as FOXP2 (already discussed above), further novel candidate genes were ‘mined’ in this study. For example, NR2F1, a neurodevelopment regulating transcription factor with predicted function in vocalization behavior, proved to be the highest density AR hotspot specific to vocal learning birds, and it is also a SFARI class S gene for ASD (Abrahams et al., 2013).
In most of the cases described above the genomic risk factors had been identified first in humans, then confirmed in mammalian model systems, and avian experiments “followed suit” as logical sequels. Notably, however, in the last two paragraphs examples were given for a reverse order of events: avian studies taking the lead to predict potential susceptibility factors worthy of investigation.
Dual subject studies
The past decade witnessed a tendency for coupled/comparative studies, in which the results obtained from avian and human subjects were jointly analyzed. Most of these studies tackled different behavioral features of diagnostic or therapeutic significance of ASD (Koshiba et al., 2016; Kelley et al., 2017; Galizio et al., 2020; Shvarts et al., 2020). In addition, molecular neuroanatomical studies, carried out on multiple species, including man, have also been reported, e.g., for the comparative localization of FMRP (see above) in the auditory system (Wang et al., 2014). Dual subject reports further highlight the translational importance of investigation into mechanisms across species, in which birds have a fair and growing share.
Notion from comprehensive animal experiments will likely be extrapolated to normal and impaired regulation of social behaviors in humans. By model building of causal and therapeutic significance, avian experiments continue to contribute toward the elucidation of anatomically traceable neural systems and developmental factors underlying human autism spectrum disorder.
Author contributions
All authors listed have made a substantial, direct, and intellectual contribution to the work and approved it for publication.
Funding
Grant sponsors: National Research, Development and Innovation Office, FK 131966; Semmelweis University Program of Excellence; Hungarian Academy of Sciences, Bolyai Scholarship to GZ; UNKP Bolyai + Scholarship to GZ.
Conflict of interest
The authors declare that the research was conducted in the absence of any commercial or financial relationships that could be construed as a potential conflict of interest.
Publisher’s note
All claims expressed in this article are solely those of the authors and do not necessarily represent those of their affiliated organizations, or those of the publisher, the editors and the reviewers. Any product that may be evaluated in this article, or claim that may be made by its manufacturer, is not guaranteed or endorsed by the publisher.
References
Aamodt C. M., Farias-Virgens M., White S. A. (2019). Birdsong as a window into language origins and evolutionary neuroscience. Philos. Trans. R. Soc. Lond. B Biol. Sci. 375, 20190060–20191789. doi:10.1098/RSTB.2019.0060
Abrahams B. S., Arking D. E., Campbell D. B., Mefford H. C., Morrow E. M., Weiss L. A., et al. (2013). SFARI gene 2.0: A community-driven knowledgebase for the autism spectrum disorders (ASDs). Mol. Autism 4, 36. doi:10.1186/2040-2392-4-36
Adiletta A., Pedrana S., Rosa-Salva O., Sgadò P. (2021). Spontaneous visual preference for face-like stimuli is impaired in newly-hatched domestic chicks exposed to valproic acid during embryogenesis. Front. Behav. Neurosci. 15, 733140. doi:10.3389/fnbeh.2021.733140
Ahmadiantehrani S., London S. E. (2017). Bidirectional manipulation of mTOR signaling disrupts socially mediated vocal learning in juvenile songbirds. Proc. Natl. Acad. Sci. U. S. A. 114, 9463–9468. doi:10.1073/pnas.1701829114
Akhtar L., Khan M. Y., Minhas L. A. (2015). The effect of prenatal administration of valproic acid on the survivability and day of hatching of chick embryo. J. Pak. Med. Assoc. 65 (2), 175–178.
Alarcón M., Abrahams B. S., Stone J. L., Duvall J. A., Perederiy J. V., Bomar J. M., et al. (2008). Linkage, association, and gene-expression analyses identify CNTNAP2 as an autism-susceptibility gene. Am. J. Hum. Genet. 82, 150–159. doi:10.1016/j.ajhg.2007.09.005
Alon L. T., Pietrokovski S., Barkan S., Avrahami L., Kaidanovich-Beilin O., Woodgett J. R., et al. (2011). Selective loss of glycogen synthase kinase-3α in birds reveals distinct roles for GSK-3 isozymes in tau phosphorylation. FEBS Lett. 585 (8), 1158–1162. doi:10.1016/j.febslet.2011.03.025
Arking D. E., Cutler D. J., Brune C. W., Teslovich T. M., West K., Ikeda M., et al. (2008). A common genetic variant in the neurexin superfamily member CNTNAP2 increases familial risk of autism. Am. J. Hum. Genet. 82, 160–164. doi:10.1016/j.ajhg.2007.09.015
Barnes G. L., Marian B. D., Tuan R. T. (1996). Valproic acid-induced somite teratogenesis in the chick embryo: Relationship with pax-I gene expression. Teratology 54, 93–102. doi:10.1002/(SICI)1096-9926(199606)54:2<93:AID-TERA5>3.0.CO;2-5
Beurel E., Grieco S. F., Jope R. S. (2015). Glycogen synthase kinase-3 (GSK3): Regulation, actions, and diseases. Pharmacol. Ther. 148, 114–131. doi:10.1016/j.pharmthera.2014.11.016
Bolhuis J. J., Honey R. C. (1998). Imprinting, learning and development: From behaviour to brain and back. Trends Neurosci. 21, 306–311. doi:10.1016/S0166-2236(98)01258-2
Bolker J. (2012). Model organisms: There's more to life than rats and flies. Nature 491 (7422), 31–33. doi:10.1038/491031a
Boucaud I. C. A., Mariette M. M., Villain A. S., Vignal C. (2016). Vocal negotiation over parental care? Acoustic communication at the nest predicts partners' incubation share. Biol. J. Linn. Soc. 117, 322–336. doi:10.1111/bij.12705
Boucaud I. C. A., Perez E. C., Ramos L. S., Griffith S. C., Vignal C. (2017). Acoustic communication in zebra finches signals when mates will take turns with parental duties. Behav. Ecol. 28, 645–656. doi:10.1093/beheco/arw189
Brennan P. A., Kendrick K. M. (2006). Mammalian social odours: Attraction and individual recognition. Philos. Trans. R. Soc. Lond. B Biol. Sci. 361, 2061–2078. doi:10.1098/rstb.2006.1931
Cahill J. A., Armstrong J., Deran A., Khoury C. J., Paten B., Haussler D., et al. (2021). Positive selection in noncoding genomic regions of vocal learning birds is associated with genes implicated in vocal learning and speech functions in humans. Genome Res. 31, 2035–2049. doi:10.1101/gr.275989.121
Calvin W. H. (1998). Competing for consciousness: A darwinian mechanism at an appropriate level of explanation. J. Conscious. Stud. 5, 389–404.
Chen J., Alberts I., Li X. (2014). Dysregulation of the IGF-I/PI3K/AKT/mTOR signaling pathway in autism spectrum disorders. Int. J. Dev. Neurosci. 35, 35–41. doi:10.1016/j.ijdevneu.2014.03.006
Cipriani A., Reid K., Young A. H., Macritchie K., Geddes J. (2013). Valproic acid, valproate and divalproex in the maintenance treatment of bipolar disorder. Cochrane Database Syst. Rev. 10, CD003196. doi:10.1002/14651858.CD003196.pub2
Condro M. C., White S. A. (2014). Distribution of language-related Cntnap2 protein in neural circuits critical for vocal learning. J. Comp. Neurol. 522, 169–185. doi:10.1002/cne.23394
D'Amelio P. B., Trost L., Ter Maat A. (2017). Vocal exchanges during pair formation and maintenance in the zebra finch (Taeniopygia guttata). Front. Zool. 14, 13. doi:10.1186/s12983-017-0197-x
Di Giorgio E., Loveland J. L., Mayer U., Rosa-Salva O., Versace E., Vallortigara G. (2017). Filial responses as predisposed and learned preferences: Early attachment in chicks and babies. Behav. Brain Res. 325, 90–104. doi:10.1016/j.bbr.2016.09.018
Elie J. E., Theunissen F. E. (2018). Zebra finches identify individuals using vocal signatures unique to each call type. Nat. Commun. 9, 4026. doi:10.1038/s41467-018-06394-9
Elsabbagh M., Gliga T., Pickles A., Hudry K., Charman T., Johnson M. H., the BASIS Team (2013). The development of face orienting mechanisms in infants at-risk for autism. Behav. Brain Res. 251, 147–154. doi:10.1016/j.bbr.2012.07.030
Ergaz Z., Weinstein-Fudim L., Ornoy A. (2016). Genetic and non-genetic animal models for autism spectrum disorders (ASD). Reprod. Toxicol. 64, 116–140. doi:10.1016/j.reprotox.2016.04.024
Galizio A., Higbee T. S., Odum A. L. (2020). Choice for reinforced behavioral variability in children with autism spectrum disorder. J. Exp. Anal. Behav. 113, 495–514. doi:10.1002/jeab.591
Gallup G. G., Suarez S. D. (1980). An ethological analysis of open-field behaviour in chickens. Anim. Behav. 28, 368–378. doi:10.1016/s0003-3472(80)80045-5
Garcia-Oscos F., Koch T. M. I., Pancholi H., Trusel M., Daliparthi V., Co M., et al. (2021). Autism-linked gene FoxP1 selectively regulates the cultural transmission of learned vocalizations. Sci. Adv. 7, eabd2827. doi:10.1126/sciadv.abd2827
Garza-Lombó C., Gonsebatt M. E. (2016). Mammalian target of rapamycin: Its role in early neural development and in adult and aged brain function. Front. Cell. Neurosci. 10, 157. doi:10.3389/fncel.2016.00157
Gill L. F., Goymann W., Ter Maat A., Gahr M. (2015). Patterns of call communication between group-housed zebra finches change during the breeding cycle. Elife 4, e07770. doi:10.7554/eLife.07770
Giret N., Menardy F., Del Negro C. (2015). Sex differences in the representation of call stimuli in a songbird secondary auditory area. Front. Behav. Neurosci. 9, 290. doi:10.3389/fnbeh.2015.00290
Gobes S. M., Ter Haar S. M., Vignal C., Vergne A. L., Mathevon N., Bolhuis J. J. (2009). Differential responsiveness in brain and behavior to sexually dimorphic long calls in male and female zebra finches. J. Comp. Neurol. 516, 312–320. doi:10.1002/cne.22113
Goldman S. A., Nottebohm F. (1983). Neuronal production, migration, and differentiation in a vocal control nucleus of the adult female canary brain. Proc. Natl. Acad. Sci. U. S. A. 80, 2390–2394. doi:10.1073/pnas.80.8.2390
Goodson J. L. (2005). The vertebrate social behavior network: Evolutionary themes and variations. Horm. Behav. 48, 11–22. doi:10.1016/j.yhbeh.2005.02.003
Göttlicher M., Minucci S., Zhu P., Krämer O. H., Schimpf A., Giavara S., et al. (2001). Valproic acid defines a novel class of HDAC inhibitors inducing differentiation of transformed cells. EMBO J. 20, 6969–6978. doi:10.1093/emboj/20.24.6969
Hacohen-Kleiman G., Barnea A., Gozes I. (2015). Adnp: A major autism mutated gene is differentially distributed (age and gender) in the songbird brain. Peptides 72, 75–79. doi:10.1016/j.peptides.2015.04.008
Hacohen-Kleiman G., Moaraf S., Kapitansky O., Gozes I. (2020). Sex-and region-dependent expression of the autism-linked ADNP correlates with social- and speech-related genes in the canary brain. J. Mol. Neurosci. 70, 1671–1683. doi:10.1007/s12031-020-01700-x
Haesler S., Rochefort C., Georgi B., Licznerski P., Osten P., Scharff C. (2007). Incomplete and inaccurate vocal imitation after knockdown of FoxP2 in songbird basal ganglia nucleus Area X. PLoS Biol. 5, e321. doi:10.1371/journal.pbio.0050321
Hagerman R., Berry-Kravis E., Hazlett H. C., Bailey D. B., Moine H., Kooy R. F., et al. (2017). Fragile X syndrome. Nat. Rev. Dis. Prim. 3, 17065. doi:10.1038/nrdp.2017.65
Helsmoortel C., Vulto-van Silfhout A., Coe B., Vandeweyer G., Rooms L., van den Ende J., et al. (2014). A SWI/SNF-related autism syndrome caused by de novo mutations in ADNP. Nat. Genet. 46, 380–384. doi:10.1038/ng.2899
Heston J. B., White S. A. (2015). Behavior-linked FoxP2 regulation enables zebra finch vocal learning. J. Neurosci. 35, 2885–2894. doi:10.1523/JNEUROSCI.3715-14.2015
Hsieh C. L., Chen K. C., Ding C. Y., Tsai W. J., Wu J. F., Peng C. C. (2013). Valproic acid substantially downregulated genes folr1, IGF2R, RGS2, COL6A3, EDNRB, KLF6, and pax-3, N-acetylcysteine alleviated most of the induced gene alterations in chicken embryo model. Rom. J. Morphol. Embryol. 54 (4), 993–1004.
Johnsson M., Henriksen R., Fogelholm J., Höglund A., Jensen P., Wright D. (2018). Genetics and genomics of social behavior in a chicken model. Genetics 209, 209–221. doi:10.1534/genetics.118.300810
Jones W., Klin A. (2013). Attention to eyes is present but in decline in 2-6-month-old infants later diagnosed with autism. Nature 504 (7480), 427–431. doi:10.1038/nature12715
Kazdoba T. M., Leach P. T., Crawley J. N. (2016). Behavioral phenotypes of genetic mouse models of autism. Genes. Brain Behav. 15, 7–26. doi:10.1111/gbb.12256
Kelley M. E., Nadler C. B., Rey C., Cowie S., Podlesnik C. A. (2017). Noncontingent reinforcement competes with response performance. J. Exp. Anal. Behav. 107, 343–353. doi:10.1002/jeab.255
Koshiba M., Karino G., Mimura K., Nakamura S., Yui K., Kunikata T., et al. (2016). Psycho-cognitive intervention for ASD from cross-species behavioral analyses of infants, chicks and common marmosets. CNS Neurol. Disord. Drug Targets 15 (5), 578–586. doi:10.2174/1871527315666160413121613
Koshiba M., Shirakawa Y., Mimura K., Senoo A., Karino G., Nakamura S. (2013). Familiarity perception call elicited under restricted sensory cues in peer-social interactions of the domestic chick. PLoS ONE 8, e58847. doi:10.1371/journal.pone.0058847
Lai C. S., Fisher S. E., Hurst J. A., Vargha-Khadem F., Monaco A. P. (2001). A forkhead-domain gene is mutated in a severe speech and language disorder. Nature 413, 519–523. doi:10.1038/35097076
Li X., Hu Z., He Y., Xiong Z., Long Z., Peng Y., et al. (2010). Association analysis of CNTNAP2 polymorphisms with autism in the Chinese Han population. Psychiatr. Genet. 20, 113–117. doi:10.1097/YPG.0b013e32833a216f
Lorenzi E., Pross A., Rosa-Salva O., Versace E., Sgadò P., Vallortigara G. (2019). Embryonic exposure to valproic acid affects social predispositions for dynamic cues of animate motion in newly-hatched chicks. Front. Physiol. 10, 501. doi:10.3389/fphys.2019.00501
Malishkevich A., Amram N., Hacohen-Kleiman G., Magen I., Giladi E., Gozes I. (2015). Activity-dependent neuroprotective protein (ADNP) exhibits striking sexual dichotomy impacting on autistic and Alzheimer’s pathologies. Transl. Psychiatry 5, e501. doi:10.1038/tp.2014.138
Marx G., Leppelt J., Ellendorff F. (2001). Vocalisation in chicks (Gallus gallus dom.) during stepwise social isolation. Appl. Anim. Behav. Sci. 75, 61–74. doi:10.1016/S0168-1591(01)00180-0
Matsushima T., Miura M., Patzke N., Toji N., Wada K., Ogura Y., et al. (2022). Impaired epigenesis of imprinting predispositions causes autism-like behavioral phenotypes in domestic chicks. bioRxiv 19, 492744. doi:10.1101/2022.05.19.492744
Mayer U., Rosa-Salva O., Vallortigara G. (2017). First exposure to an alive conspecific activates septal and amygdaloid nuclei in visually naïve domestic chicks (Gallus gallus). Behav. Brain Res. 317, 71–81. doi:10.1016/j.bbr.2016.09.031
McCabe B. J., Horn G. (1994). Learning-related changes in Fos-like immunoreactivity in the chick forebrain after imprinting. Proc. Natl. Acad. Sci. U. S. A. 91, 11417–11421. doi:10.1073/pnas.91.24.11417
Moaraf S., Rippin I., Terkel J., Eldar-Finkelman H., Barnea A. (2022). GSK-3β inhibition in birds affects social behavior and increases motor activity. Front. Physiol. 13, 881174. doi:10.3389/fphys.2022.881174
Mody M., Belliveau J. W. (2013). Speech and language impairments in autism: Insights from behavior and neuroimaging. N. Am. J. Med. Sci. 5, 157–161. doi:10.7156/v5i3p157
Montevecchi W. A., Gallup G. G., Dunlap W. P. (1973). The peep vocalization in group reared chicks (Gallus domesticus): Its relation to fear. Anim. Behav. 21 (1), 116–123. doi:10.1016/s0003-3472(73)80049-1
Murugan M., Harward S., Scharff C., Mooney R. (2013). Diminished FoxP2 levels affect dopaminergic modulation of corticostriatal signaling important to song variability. Neuron 80 (6), 1464–1476. doi:10.1016/j.neuron.2013.09.021
Nicolini C., Fahnestock M. (2018). The valproic acid-induced rodent model of autism. Exp. Neurol. 299, 217–227. doi:10.1016/j.expneurol.2017.04.017
Nishigori H., Kagami K., Takahashi A., Tezuka Y., Sanbe A., Nishigori H. (2013). Impaired social behavior in chicks exposed to sodium valproate during the last week of embryogenesis. Psychopharmacol. Berl. 227, 393–402. doi:10.1007/s00213-013-2979-y
Nottebohm F. (2005). The neural basis of birdsong. PLoS Biol. 3 (5), e164. doi:10.1371/journal.pbio.0030164
O'Connell L. A., Hofmann H. A. (2011). The vertebrate mesolimbic reward system and social behavior network: A comparative synthesis. J. Comp. Neurol. 519, 3599–3639. doi:10.1002/cne.22735
Panaitof S. C. (2012). A songbird animal model for dissecting the genetic bases of autism spectrum disorder. Dis. Markers 33, 241–249. doi:10.3233/DMA-2012-0918
Perrin S. (2014). Preclinical research: Make mouse studies work. Nature 507 (7493), 423–425. doi:10.1038/507423a
Pinel P., Fauchereau F., Moreno A., Barbot A., Lathrop M., Zelenika D., et al. (2012). Genetic variants of FOXP2 and KIAA0319/TTRAP/THEM2 locus are associated with altered brain activation in distinct language-related regions. J. Neurosci. 32, 817–825. doi:10.1523/JNEUROSCI.5996-10.2012
Rodier P. M., Ingram J. L., Tisdale B., Nelson S., Romano J. (1996). Embryological origin for autism: Developmental anomalies of the cranial nerve motor nuclei. J. Comp. Neurol. 370, 247–261. doi:10.1002/(SICI)1096-9861(19960624)370:2<247:AID-CNE8>3.0.CO;2-2
Rose S. P. (2000). God's organism? The chick as a model system for memory studies. Learn. Mem. 7, 1–17. doi:10.1101/lm.7.1.1
Roullet F. I., Lai J. K., Foster J. A. (2013). In utero exposure to valproic acid and autism--a current review of clinical and animal studies. Neurotoxicol. Teratol. 36, 47–56. doi:10.1016/j.ntt.2013.01.004
Satterstrom F. K., Kosmicki J. A., Wang J., Breen M. S., De Rubeis S., An J. Y., et al. (2020). Large-scale exome sequencing study implicates both developmental and functional changes in the neurobiology of autism. Cell. 180, 568–584. doi:10.1016/j.cell.2019.12.036
Sgadò P., Rosa-Salva O., Versace E., Vallortigara G. (2018). Embryonic exposure to valproic acid impairs social predispositions of newly hatched chicks. Sci. Rep. 8, 5919. doi:10.1038/s41598-018-24202-8
Shaughnessy D. W., Hyson R. L., Bertram R., Wu W., Johnson F. (2019). Female zebra finches do not sing yet share neural pathways necessary for singing in males. J. Comp. Neurol. 527, 843–855. doi:10.1002/cne.24569
Shvarts S., Jimenez-Gomez C., Bai J. Y. H., Thomas R. R., Oskam J. J., Podlesnik C. A. (2020). Examining stimuli paired with alternative reinforcement to mitigate resurgence in children diagnosed with autism spectrum disorder and pigeons. J. Exp. Anal. Behav. 113, 214–231. doi:10.1002/jeab.575
Sperdin H. F., Schaer M. (2016). Aberrant development of speech processing in young children with autism: New insights from neuroimaging biomarkers. Front. Neurosci. 10, 393. doi:10.3389/fnins.2016.00393
Spiteri E., Konopka G., Coppola G., Bomar J., Oldham M., Ou J., et al. (2007). Identification of the transcriptional targets of FOXP2, a gene linked to speech and language, in developing human brain. Am. J. Hum. Genet. 81, 1144–1157. doi:10.1086/522237
Tager-Flusberg T., Paul R., Lord C. E. (2005). “Language and communication in autism,” in Handbook of autism and pervasive developmental disorders, and behavior. Editors R. P. F. Volkmar, A. Klin, and D. J. Cohen (New Jersey, United States: Wiley), 335–364.
Takeuchi H., Yazaki Y., Matsushima T., Aoki K. (1996). Expression of Fos-like immunoreactivity in the brain of quail chick emitting the isolation-induced distress calls. Neurosci. Lett. 220 (3), 191–194. doi:10.1016/s0304-3940(96)13256-0
Ter Maat A., Trost L., Sagunsky H., Seltmann S., Gahr M. (2014). Zebra finch mates use their forebrain song system in unlearned call communication. PLoS One 9, e109334. doi:10.1371/journal.pone.0109334
Teramitsu I., Kudo L. C., London S. E., Geschwind D. H., White S. A. (2004). Parallel FoxP1 and FoxP2 expression in songbird and human brain predicts functional interaction. J. Neurosci. 24, 3152–3163. doi:10.1523/JNEUROSCI.5589-03.2004
Vulih-Shultzman I., Pinhasov A., Mandel S., Grigoriadis N., Touloumi O., Pittel Z., et al. (2007). Activity-dependent neuroprotective protein snippet NAP reduces tau hyperphosphorylation and enhances learning in a novel transgenic mouse model. J. Pharmacol. Exp. Ther. 323, 438–449. doi:10.1124/jpet.107.129551
Wang R., Chen C. C., Hara E., Rivas M. V., Roulhac P. L., Howard J. T., et al. (2015). Convergent differential regulation of SLIT-ROBO axon guidance genes in the brains of vocal learners. J. Comp. Neurol. 523, 892–906. doi:10.1002/cne.23719
Wang X., Kohl A., Yu X., Zorio D. A. R., Klar A., Sela-Donenfeld D., et al. (2020). Temporal-specific roles of fragile X mental retardation protein in the development of the hindbrain auditory circuit. Development 147, dev188797. doi:10.1242/dev.188797
Wang Y., Sakano H., Beebe K., Brown M. R., de Laat R., Bothwell M., et al. (2014). Intense and specialized dendritic localization of the fragile X mental retardation protein in binaural brainstem neurons: A comparative study in the alligator, chicken, gerbil, and human. J. Comp. Neurol. 522, 2107–2128. doi:10.1002/cne.23520
Whitsel A. I., Johnson C. B., Forehand C. J. (2002). An in ovo chicken model to study the systemic and localized teratogenic effects of valproic acid. Teratology 66, 153–163. doi:10.1002/tera.10093
Winograd C., Clayton D., Ceman S. (2008). Expression of fragile X mental retardation protein within the vocal control system of developing and adult male zebra finches. Neuroscience 157, 132–142. doi:10.1016/j.neuroscience.2008.09.005
Yartsev M. M. (2017). The emperor's new wardrobe: Rebalancing diversity of animal models in neuroscience research. Science 358, 466–469. doi:10.1126/science.aan8865
Yazaki Y., Matsushima T., Aoki K. (1999). Testosterone modulates stimulation-induced calling behavior in Japanese quails. J. Comp. Physiol. A 184, 13–19. doi:10.1007/s003590050302
Zachar G., Schrott A., Kabai P. (2008). Context-dependent prey avoidance in chicks persists following complete telencephalectomy. Brain Res. Bull. 76 (3), 289–292. doi:10.1016/j.brainresbull.2008.02.017
Zachar G., Tóth A. S., Balogh M., Csillag A. (2017). Effect of nucleus accumbens lesions on socially motivated behaviour of young domestic chicks. Eur. J. Neurosci. 45, 1606–1612. doi:10.1111/ejn.13402
Zachar G., Tóth A. S., Gerecsei L. I., Zsebők S., Ádám Á., Csillag A. (2019). Valproate exposure in ovo attenuates the acquisition of social preferences of young post-hatch domestic chicks. Front. Physiol. 10, 881. doi:10.3389/fphys.2019.00881
Zosen D., Austdal L. P. E., Bjørnstad S., Lumor J. S., Paulsen R. E. (2022). Antiepileptic drugs lamotrigine and valproate differentially affect neuronal maturation in the developing chick embryo, yet with PAX6 as a potential common mediator. Neurotoxicol. Teratol. 90, 107057. doi:10.1016/j.ntt.2021.107057
Zsedényi C. K., Zachar G., Csillag A., Ádám Á. (2014). Effect of synthetic cathinones: Mephedrone, butylone and 3, 4 methylene-dioxypyrovalerone (MDPV) on social separation induced distress vocalization, vigilance and postural control of young domestic chicks. Neurosci. Lett. 580, 88–93. doi:10.1016/j.neulet.2014.07.027
Keywords: social behavior, songbird, ASD, dopamin, valproic acid, genes
Citation: Csillag A, Ádám Á and Zachar G (2022) Avian models for brain mechanisms underlying altered social behavior in autism. Front. Physiol. 13:1032046. doi: 10.3389/fphys.2022.1032046
Received: 30 August 2022; Accepted: 17 October 2022;
Published: 28 October 2022.
Edited by:
Sandra G. Velleman, The Ohio State University, United StatesCopyright © 2022 Csillag, Ádám and Zachar. This is an open-access article distributed under the terms of the Creative Commons Attribution License (CC BY). The use, distribution or reproduction in other forums is permitted, provided the original author(s) and the copyright owner(s) are credited and that the original publication in this journal is cited, in accordance with accepted academic practice. No use, distribution or reproduction is permitted which does not comply with these terms.
*Correspondence: András Csillag, Y3NpbGxhZy5hbmRyYXNAbWVkLnNlbW1lbHdlaXMtdW5pdi5odQ==