- Insect Pest and Vector Laboratory, Department of Zoology, Acharya Narendra Dev College, University of Delhi, New Delhi, India
Aedes aegypti is responsible for the global spread of several ailments such as chikungunya, dengue, yellow fever, and Zika. The use of synthetic chemicals is the primary intervention in mosquito management. However, their excessive utilization resulting in the spread of toxic ingredients in the environment and posing threats to beneficial organisms has prompted the recommendation for the use of biologically synthesized nanocomposites as a promising approach for vector control. Silver nanocomposites were synthesized using leaf (AL-AgNCs) and stem (AS-AgNCs) extracts of Achyranthes aspera. The early fourth instars of A. aegypti were exposed to lethal doses of these nanocomposites to evaluate their effects on larval development, behavior, morphology, and mid-gut histoarchitecture. The cellular damage and deposition of nanocomposite residues in the mid-gut were studied using light and transmission electron microscopy. The A. aspera silver nanocomposite (AA-AgNC)-exposed larvae exhibited dose-dependent extended duration of development and diminished adult emergence, but did not exhibit modified behavior. Intense damage to the cuticle membrane and slight contraction in the internal membrane of anal papillae were noticed. Morphologically, the mid-gut appeared disorganized, darkly pigmented, and shrunk. Histological investigations of the mid-gut revealed significantly disordered internal architecture with lysed cells, damaged peritrophic membrane and microvilli, disintegrated epithelial layer, and a ruptured and displaced basement membrane. Visualization of the larval mid-gut through TEM showed severe cellular damage and aggregation of black spots, indicating the deposition of silver particles released by AA-AgNCs. The investigations revealed the bio-efficacy of A. aspera-mediated AgNCs against A. aegypti inducing stomach and contact toxicity in the larvae. The utilization of AA-AgNCs is recommended for A. aegypti management as a safe and effective intervention.
Introduction
Aedes aegypti L., the prime and highly widespread mosquito vector, is responsible for the transmission of viruses causing various ailments, such as dengue, Zika, yellow fever, and chikungunya. These diseases are more prevalent in subtropical and tropics regions of the world, although the severity and risks involved are under the influence of climatic, social, and environmental factors. In the last two decades, the incidences of dengue have amplified over 8-fold. In 2000, a total of 505,430 dengue cases were recorded which increased to more than 2.4 million in 2010, reaching 5.2 million in 2019 (World Health Organization, 2022). Nevertheless, the total number of cases and deaths apparently declined during the years 2020 and 2021 which might be due to the under-reporting of cases during the COVID-19 pandemic (World Health Organization, 2022).
In India, dengue fever is pervasive in nearly every single region. The disease has been reported from 36 states/union territories with a total of 44,585 dengue incidences and 56 fatalities in 2020, rising to 193,245 incidences and 346 deaths in 2021 (NVBDCP, 2022). The maximum outbreak was documented in Uttar Pradesh (29,750 cases) followed by Punjab and Rajasthan. Likewise, India encountered a total of 119,070 chikungunya cases in 2021, the highest in the last few years (NVBDCP, 2022).
Mosquito-borne diseases can currently be kept under check only through mosquito control by employing various interventions among which the main control tool is the application of synthetic insecticides in the form of space sprays, indoor residual sprays (IRS), insecticide-treated nets (ITNs), and long-lasting insecticide-treated nets (LLINs) (Dulacha et al., 2022). These measures have not only created serious health and environmental issues but also made mosquitoes resistant to insecticides, forcing the use of higher doses of chemicals at a higher frequency. These issues have severely impacted the survival of non-target organisms and disturbed our ecosystem, specifically due to the bio-magnification of these toxicants in the food chain. Thus, the identification of effective and eco-friendly control methods is necessitated, which can be used safely and effectively.
In recent years, metallic nanoparticles formulated with diverse metals possessing infrequent and varied physical and chemical properties have engrossed substantial interest among researchers (Sabri et al., 2016). Several kinds of nano-formulations have been prepared using various agents, especially plants and microbes, which have emerged as probable bio-pesticides. Thus, attempts have been made to formulate metallic nanoparticles by employing different metals, such as iron, zinc, silver, gold, platinum, and titanium. Among these, silver nanoparticles have been investigated at a larger scale and reported to exhibit toxic potential against bacteria, fungi, and mosquito larvae (Ga’al et al., 2018). The formulation of these composites using phytoconstituents is considered a highly advantageous and rapid approach in comparison to their fabrication using chemical or physical methods. This is because phytomediated measures do not require any toxicants, high temperature, energy, pressure, and purification steps; phytoconstituents can act as reducing agents and capping agents and control the biophysical parameters of nanoparticles (Govindarajan et al., 2016; Sabri et al., 2016).
Various biological agents have been studied for a few years in an effort to produce inexpensive, facile, and effective metallic nanoparticles against mosquitoes (Thakkar et al., 2010; Salunkhe et al., 2011; Tran and Le, 2013; Shah et al., 2015; Ahmed et al., 2016). Silver nanoparticles have been synthesized using many plants, such as extracts of neem (Azadirachta indica), green tea (Camellia sinensis), natural rubber, Aloe vera, and lemongrass (Cymbopogon citratus) (Vijayaraghavan et al., 2012); Ambrosia arborescens leaf extract (Morejón et al., 2018); and Annona reticulata leaf extract (Parthiban et al., 2019). Various plants used in the formulation of silver nanoparticles and possessing toxic activity against larvae of different mosquito species have been reviewed and documented (Kabtiyal et al., 2022). Apart from this, the impact of silver nanoparticles prepared from Hedychium coronarium (Kalimuthu et al., 2017) and Cymodocea serrulata (Amutha et al., 2019) aqueous extracts has also been evaluated on the mid-gut histoarchitecture of the A. aegypti, Culex quinquefasciatus, and Anopheles stephensi larvae.
The leaf, flower, and seed extracts of a perennial herb A. aspera have been reported to exhibit biological activity against A. aegypti, A. subpictus, and C. tritaeniorhynchus, having diverse effects on each development stage (Bagavan et al., 2008). The plant has been used to treat malaria, pneumonia, cough, asthma, snake bites, stomach aches, skin eruptions, boils, and heaps (Amaladhas et al., 2013; Elumalai et al., 2016; Sharma et al., 2016). The larvicidal efficacy of silver nanoparticles synthesized from the leaf and stem extracts of A. aspera has been assessed against A. aegypti, revealing respective LC50 values of 5.819 and 9.119 μg/ml after 24 h (Sharma et al., 2017; Sharma et al., 2020). The current study estimated the impact of these nanocomposites on the morphology, behavior, and development of the early fourth instar of A. aegypti. The effect of the nanocomposites on the mid-gut cellular architecture of larvae was evaluated through light microscopy, ultra-histology, and transmission electron microscopy. It is believed that the outcomes of the study would assist in safe and effective mosquito management.
Materials and methods
The investigations were carried out with silver nanocomposites prepared from A. aspera leaf and stem extracts against the early fourth instars of a laboratory-reared strain of A. aegypti. The larvae were exposed to pre-determined lethal dosages of each nanocomposite for 24 h. The effect of nanocomposites on the behavior, morphology, growth, and development of larvae was assessed. The toxic impact on the mid-gut histological architecture of larvae was also studied.
Rearing of A. aegypti
The dengue vector, A. aegypti, used in the current investigations, has been maintained in a dedicated laboratory at Acharya Narendra Dev College, University of Delhi, India. Rearing is carried out under the controlled condition of relative humidity (75 ± 10%), temperature (27 ± 2°C), and photoperiod (14 L:10 D). Adults are kept in a muslin cloth cage and fed sugar syrup of water-soaked deseeded raisins. The females, provided with blood meals on alternate days for egg maturation, lay eggs in a water-filled bowl lined with strips of Whatman filter paper No. 1. The eggs are hatched in a plastic or enamel tray half-filled with de-chlorinated water. The larvae are provided dog biscuits and yeast (3:2 w/w) for nutrition and are maintained carefully. The pupae were separated out and kept in the screened cloth cages for adult emergence.
Preparation of A. aspera extract
The fresh leaves and stems of A. aspera were gathered from the areas surrounding the institute. The plant was identified by experts in the Department of Botany, University of Delhi, India, and a sample was submitted in their herbarium with accession no. 14376. The collected parts were washed thoroughly and cut into small pieces. The 10 g pieces of each part were added to 100 ml of double-distilled water and heated at 60°C for 15–20 min. The aqueous extracts formed were left undisturbed for 3 h and then filtered to obtain a clear solution (Sharma et al., 2017).
Formulation of A. aspera silver nanocomposites
The silver nanocomposites were synthesized from the leaf and stem aqueous extracts of A. aspera following a standardized protocol used in our earlier studies (Sharma et al., 2017; Sharma et al., 2020). The 15 ml A. aspera leaf extract (AALE) and 18 ml A. aspera stem extract (AASE) were separately added to 100 ml of 4 mM silver nitrate solution. The silver nitrate–AALE mixture was incubated at room temperature in the dark for 24 h, while that of the AASE was first boiled at 85–90°C in a water bath for 30 min. The alteration in the color of the solution was recorded to confirm the synthesis of silver nanocomposites which were subsequently incubated in the dark for 24 h and centrifuged at 12,000 rpm for 10–15 min. The supernatant was collected and re-centrifuged, resulting in the formation of powdery pellets which were stored at room temperature for conducting bioassays.
Effect of A. aspera silver nanocomposites on larval growth and development
Our earlier studies have estimated the LC50 values of AgNCs formulated with leaf (AL-AgNCs) and stem (AS-AgNCs) extracts of A. aspera (Sharma et al., 2017; Sharma et al., 2020). The respective LC30, LC50, and LC90 values obtained with exposure of A. aegypti larvae to AL-AgNCs were recorded as 1.681, 5.819, and 21.068 μg/ml, and with AS-AgNC exposure as 1.750, 9.119, and 15.48 μg/ml. The effect of all these dosages on the growth and development of A. aegypti larvae was estimated. A total of 20 larvae were exposed to individual dosages for 24 h. The larvae which survived were monitored until adult emergence. The developmental period and adult emergence were recorded to assess the latent effects of NCs. The studies were conducted in three replicates with each dosage.
Effect of A. aspera silver nanocomposites on larval behavior
A total of 20 early fourth instars of A. aegypti were exposed to median lethal doses of leaf and stem AA-AgNCs, separately. The larvae were observed at specific time intervals of 1, 3, 6, and 9 h, followed by final observations at 24 h. The changes in their behavior pattern such as wriggling, swiftness, excitation, agitation, aggression, aggregation, horizontal or vertical movements, inability to rise up to the water surface, unnatural positions, uncertain movements, and convulsions were documented. The study was conducted with each dosage in three replicates.
Effect of A. aspera silver nanocomposites on larval morphology
The A. aegypti early fourth instars were exposed to the median lethal dosage of leaf and stem AA-AgNCs for 24 h, 20 larvae in three replicates. The dead larvae were examined carefully under a zoom stereo binocular microscope (Magnus; MSZ-B1) for morphological alterations. The changes in the head, thorax, abdomen, and external organs, such as the eyes, antennae, mouth brushes, saddle, setae, siphon, and anal gills, were scrutinized. The larvae were also observed critically for changes in their body pigmentation or any other visible aberrations. The observed morphological features in the AA-AgNC-exposed larvae were compared with those of the controls and assessed.
Effect of A. aspera silver nanocomposites on the larval mid-gut histological architecture
The effect of LC50 dosages of A. aspera silver nanocomposites on the mid-gut histological architecture of 24 h-exposed A. aegypti early fourth instars was also evaluated. Control sets were exposed to the aqueous solution of AgNO3. The studies were conducted using light microscopy, ultra-histology, and transmission electron microscopy.
Light microscopy
Post-exposure, the middle body portion of 3–5 moribund larvae was excised of their anterior and posterior portions and fixed in 10% neutral buffered formalin for 24 h. The fixed larvae were washed thoroughly under running water and dehydrated by passing through the ascending series of alcohol grades, 30%, 50%, 70%, 90%, and 100% alcohol (Merck), for 60 min in each. The dehydrated larvae were treated with acetone for 30 min and xylene solution for 2 h. These larvae were embedded in paraffin wax at 55°C for overnight infiltration.
The 4–6 μm thick longitudinal sections of the larval mid-gut were cut using a microtome (Weswox Optik). The sections were made free of wrinkles/folds by treating them with warm water (40–50°C) and dried on albumin-smeared microscope glass slides. The sections were de-waxed in xylene solution for 30 min, followed by one change in acetone and hydration in the descending series of degraded alcohol (100%, 90%, 70%, 50%, and 30%) and distilled water. The hydrated sections were stained with hematoxylin stain and dehydrated again until 90% alcohol concentration. These sections were now counter-stained with eosin for 2–3 min, following which they were kept in absolute alcohol followed by two changes in absolute alcohol: xylene (1:1) for 10 min each. The sections were mounted on a slide with a drop of DPX mountant. The mid-gut tissues were observed critically under a Nikon light microscope (E600) at 40X, examined for alterations relative to the control, and photographed using a Canon Power Shot Digital Camera (SX50HS).
Ultra-thin histology and transmission electron microscopy
The NC-exposed moribund larvae were fixed in Karnovsky’s fixative (4% paraformaldehyde and 1% glutaraldehyde solution in 0.1 M of phosphate buffer; pH = 7.4) at 4°C for 24 h. Subsequently, they were washed thrice with 0.1 M phosphate buffered saline (PBS) for 15–30 min at 4°C. After fixation, the larvae were treated with 1% (w/v) osmium tetroxide for 15 min at 4°C followed by 3× washing again with PBS at 4°C. Each larva was dehydrated at room temperature by keeping it in the ascending graded series of acetone for 15 min each, treated with absolute alcohol twice for 30 min each, and cleared with toluene twice for 15–20 min. Infiltration was carried out by placing the sample for 2 h in each toluene and raisin mixture (3:1, 2:2, and 1:3), one by one, followed by keeping in pure raisin for 2 h.
The embedded tissue was longitudinally sectioned at 0.5 μm thickness (for slide preparation) and 70 nm thickness (for copper grid preparation) using ultra-microtome (Leica EM UC6). The sections were stained with toluidine blue, observed meticulously, and photographed using a transmission electron microscope (TECNAI 200 Kv ) (Fei, Electron Optics).
Results
The current investigations used early fourth instar larvae of A. aegypti reared in the laboratory and exposed to lethal dosages of leaf and stem AA-AgNCs for 24 h. The exposure effect on the growth, development, behavior, morphology, and mid-gut histological structure of larvae was assessed.
Effect of A. aspera silver nanocomposites on larval growth and development
The larvae exposed to sub-lethal, median-lethal, and lethal dosages of AA-AgNCs showed delayed development and reduced adult emergence in a dose-dependent manner. The development duration from larvae to adults in control sets was 6.00 days which increased significantly by 4.0, 7.0, and 8.67% on exposure to LC30, LC50, and LC90 dosages (p < 0.05), respectively, of A. aspera leaf nanocomposites (Table 1). The more intense delaying effects on the development duration were imparted by AS-AgNCs, which increased the development duration of larvae by 6.0, 9.0, and 11.66% at LC30, LC50, and LC90 dosages, respectively, compared to controls (Table 2).
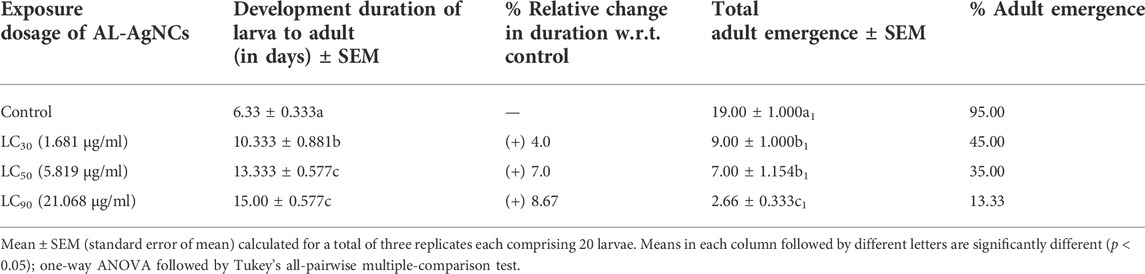
TABLE 1. Development duration and the adults emerged from the early fourth instar larvae of Aedes aegypti exposed to the silver nanocomposites synthesized from the leaf extracts of Achyranthes aspera (AL-AgNCs) for 24 h.
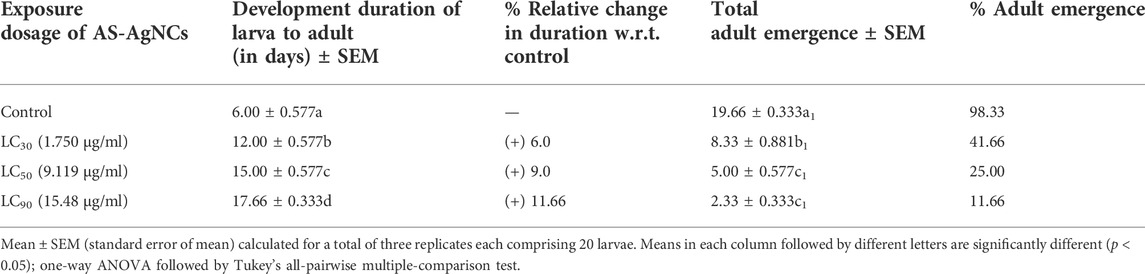
TABLE 2. Development duration and the adults emerged from the early fourth instar larvae of Aedes aegypti exposed to the silver nanocomposites synthesized from the stem extracts of Achyranthes aspera (AS-AgNCs) for 24 h.
Likewise, a significant and dose-dependent effect of AA-AgNCs (p < 0.05) was recorded on the number of adults that emerged from the exposed larvae indicating the latent effects of formulations. Compared to 95–98.33% of adults which emerged in the control sets, just 13.33–11.66% adults emerged on AA-AgNC exposure at the LC90 dosage (Tables 1, 2). Even other dosages negatively impacted emergence at a significant level; however, greater effects were imparted by AS-AgNCs.
Effect of A. aspera silver nanocomposites on the larval behavior
The 24 h exposure with LC50 values of leaf and stem AA-AgNCs could not induce any behavioral modifications in the early fourth instars of A. aegypti. The larvae showed normal wriggling movements without any restlessness or excitation. Nevertheless, larval mortality of 48.33% and 51.66% was recorded with AL-AgNCs and AS-AgNCs, respectively (Table 3).
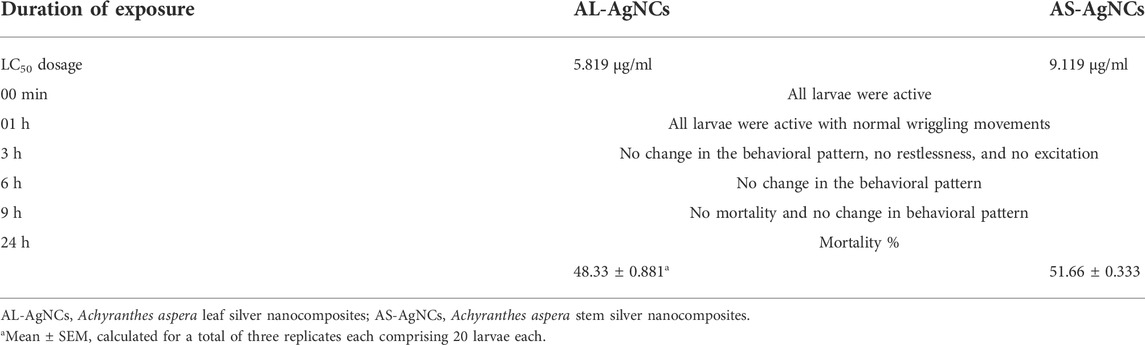
TABLE 3. Impact of the silver nanocomposites synthesized from leaf and stem extracts of Achyranthes aspera on the behavior of early fourth instars of Aedes aegypti.
Effect of A. aspera silver nanocomposites on the larval morphology
Observation of the early fourth instars of A. aegypti exposed to A. aspera leaf and stem mediated-AgNCs under a light microscope revealed no aberrations in their external organs. The larval cuticle, eyes, antennae, mouth, setae, and ventral brushes were normally shaped, similar to that observed in the control larvae (Figure 1).
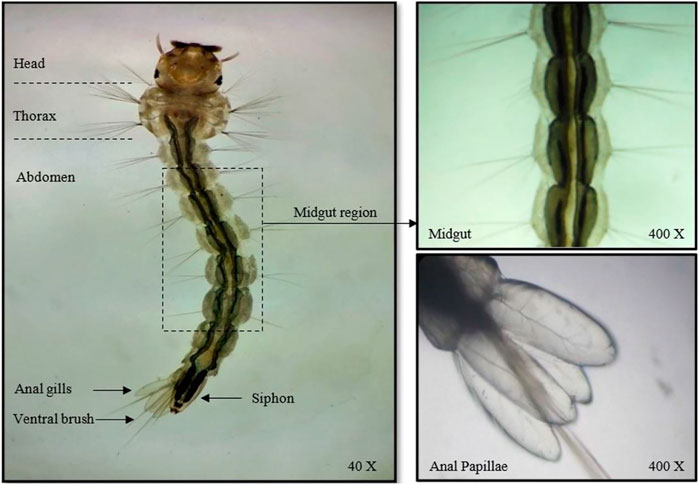
FIGURE 1. Light photomicrograph of unexposed early fourth instars of Aedes aegypti with an enlarged view of the mid-gut and anal papillae.
Nevertheless, increased pigmentation and blackening of the gut was observed in the AA-AgNC-exposed larvae (Figures 2A,B). The larval body was shrunk with impaired, destroyed, and disorganized gut portions. In addition, distinct and severe damage in the body cuticular membrane was recorded along with slight shrinkage in the internal membrane of anal papillae. A more pronounced effect was observed when the larvae were exposed to AS-AgNCs (Figure 2B).
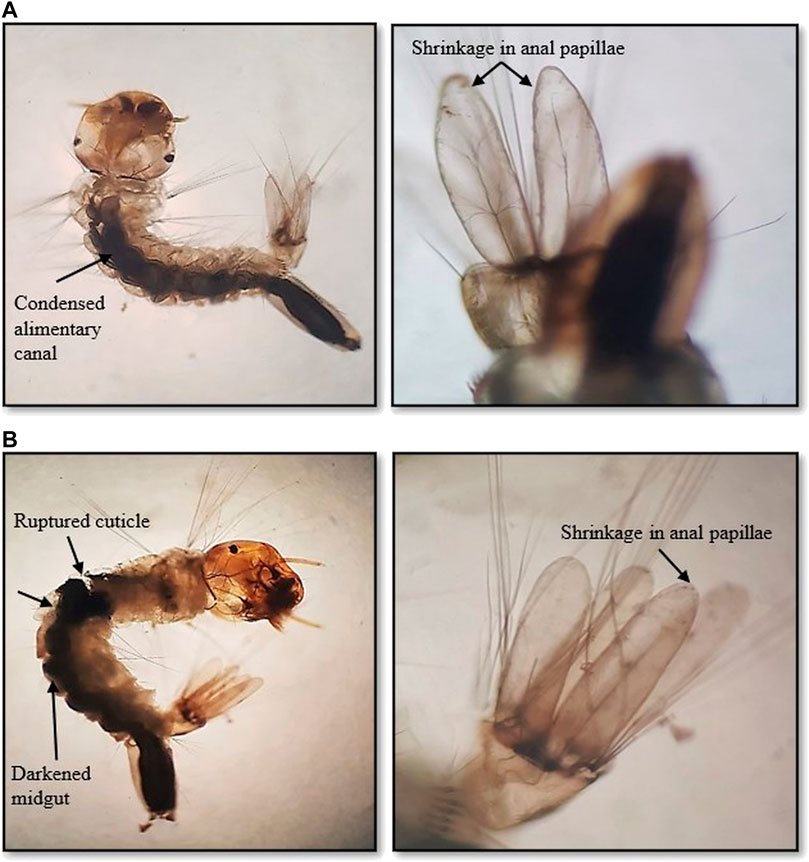
FIGURE 2. Light photomicrographs of early fourth instars of Aedes aegypti with an enlarged view of mid-gut and anal papillae on exposure to (A) Achyranthes aspera leaf extract-mediated silver nanocomposites (AL-AgNCs) and (B) Achyranthes aspera stem extract-mediated silver nanocomposites (AS-AgNCs) (→) indicates damaged area showing high pigmentation and blackening of the mid-gut region, ruptured cuticle, and deformed body with shrinkage in anal papillae.
Effect of A. aspera silver nanocomposites on the larval mid-gut histological architecture
Light microscopy of the longitudinal sections of the mid-gut of control larvae showed an unbroken and undamaged mid-gut lined evenly and entirely with epithelial cells, an intact peritrophic and basement membrane, and normal muscle forms (Figure 3A). On the other hand, the larvae exposed to AL-AgNCs and AS-AgNCs for 24 h had a relatively deformed and darkly pigmented mid-gut, containing blackened (Figure 3B) or brownish content (Figure 3C), respectively. The partial or complete destruction of mid-gut epithelial cells resulted in the widening of intercellular spaces, cytoplasmic vacuolization, destruction of the epithelial layer including nuclei and nucleoli, altered structure of microvilli, and detachment of the peritrophic membrane from the basement membrane (Figures 3B,C). These detrimental alterations in the organism’s mid-gut indicate that these changes are a conjoint response to cellular intoxication.
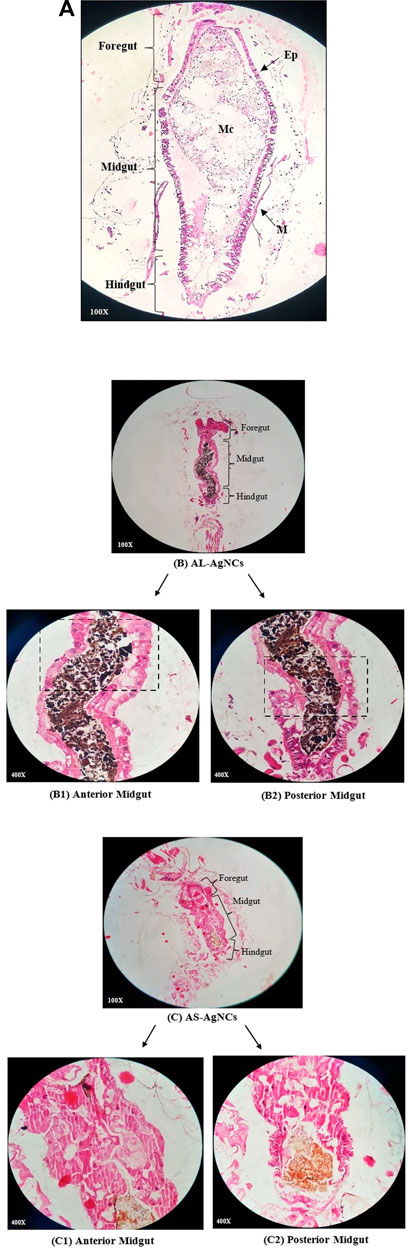
FIGURE 3. Photomicrographs of the longitudinal section of early fourth instar larvae of Aedes aegypti exposed for 24 h to the LC50 dosage of silver nanocomposites: (A) control, (B) Exposed to Achyranthes aspera leaf extract-mediated silver nanocomposites (AL-AgNCs), and (C) Exposed to Achyranthes aspera stem extract-mediated silver nanocomposites (AS-AgNCs).
Similar observations were recorded with ultrathin-histology and transmission electron microscopy (TEM) of the mid-gut sections of the A. aegypti larvae. The control larvae showed a normal organization of the gut epithelium and surrounding muscles (Figures 4A, 5A). In contrast, the larvae exposed to AL-AgNCs and AS-AgNCs possessed significantly disrupted gut histoarchitecture and cellular organization (Figures 4B,C, 5B,C).
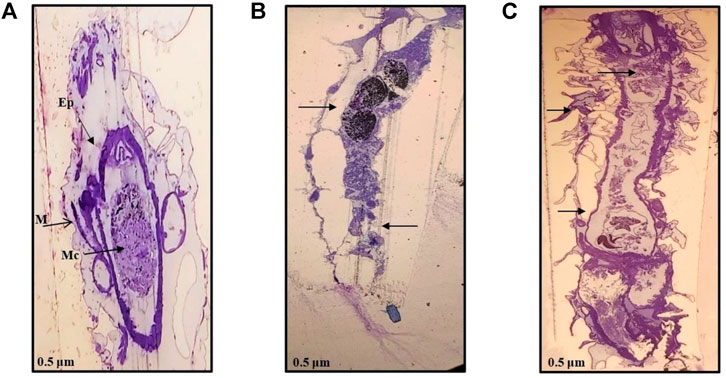
FIGURE 4. Ultra-thin histological sections of early fourth instars of Aedes aegypti: (A) control larva; (B) larva exposed to Achyranthes aspera leaf extract-mediated silver nanocomposites; (C) larva exposed to Achyranthes aspera stem extract-mediated silver nanocomposites. (→) indicates damaged area, Ep—epithelium layer, M—muscle, Mc—mid-gut content (magnification: ×100).
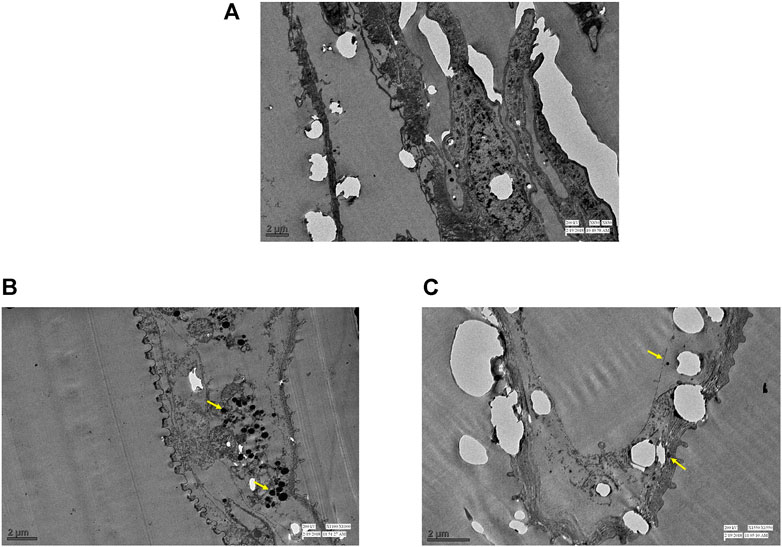
FIGURE 5. TEM images of mid-guts of early fourth instars of Aedes aegypti; (A) control; (B) larva exposed to Achyranthes aspera leaf extract-mediated silver nanocomposites (AL-AgNCs); (C) larva exposed to Achyranthes aspera stem extract-mediated silver nanocomposites (AS-AgNCs); (→) indicates deposition of black spots.
The adverse impact was observed on almost all the parts of the larval mid-gut; that is, peritrophic membrane, microvilli, epithelial layer, and basement membrane. Severe damages to the cuticle membrane of the gut with a disintegrated epithelium, vacuolated cytoplasm, and desquamation of epithelial cells with intercellular spaces were noticed. The remnants of the damaged cells and body muscles were documented. The blackening of the gut content was visible. Similar observations, although more distinct, were observed with the TEM micrographs of the larval mid-gut. The control A. aegypti larvae showed normal cellular organization of the mid-gut architecture with intact cells (Figure 6A) as against cellular disorganization and ruptured mid-gut organization in experimental larvae (Figures 6B,C). The most significant observation was the presence of black spots (marked with yellow arrows), possibly indicating the deposition of silver particles.
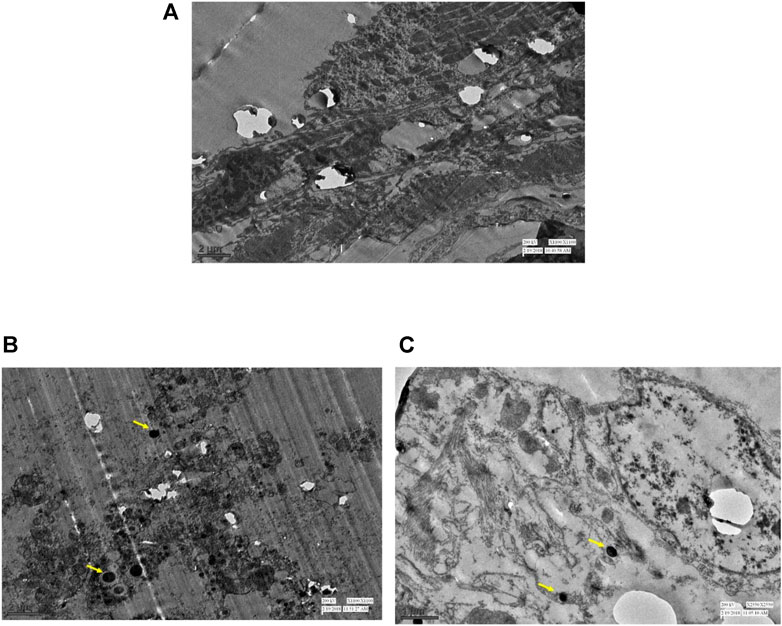
FIGURE 6. TEM images of the cellular organization of early fourth instars of Aedes aegypti mid-gut: (A) control; (B) larva exposed to Achyranthes aspera leaf extract-mediated silver nanocomposites (AL-AgNCs); (C) larva exposed to Achyranthes aspera stem extract-mediated silver nanocomposites (AS-AgNCs); and (→) indicates deposition of black spots.
Discussion
Management of mosquitoes is of high concern nowadays because of the worldwide spread of mosquito-borne diseases. Global transmission of dengue and chikungunya has necessitated the development of an eco-friendly and efficient intervention measure which can control the Aedes population and diseases in the absence of an effectual vaccine and medication. Since the use of chemical insecticides has raised environmental and human health concerns, the formulation and utilization of effective nanoformulations with low toxicity to humans and high ecological safety are recommended in the management programs. The use of plant-borne compounds in the form of metallic nanocomposites is considered safe and effective against mosquitos in juvenile stages (Benelli and Mehlhorn, 2016). In light of this, attempts were undertaken to study the impact of silver nanocomposites, synthesized using A. aspera leaf and stem extracts, on the development, behavior, morphology, and gut histological structure of the larvae of A. aegypti.
The current study revealed that larval exposure to A. aspera nanocomposites caused dose-dependent prolonged development of larvae and decreased emergence of adults, indicating the latent effects of NCs. An enhanced effect was imparted by AS-AgNCs compared to that of the AL-AgNCs. Similar effects, causing retarded growth of larvae, have been induced by the exposure of A. albopictus and A. vagus larvae to zinc oxide nanocomposites (ZnO-NCs), suggesting the delayed effects of the NCs (Gunathilaka et al., 2021). Compared to the developmental duration in controls (8 days), they recorded an extended duration of 13 days (at LC25), 14.67 days (at LC50), 16.67 days (at LC75), and 17 days (at LC100), indicating the dose-dependent effects in the current study (Gunathilaka et al., 2021). Nevertheless, the effect of phytomediated nanocomposites has not been assessed as the modifiers of larval behavior although several studies have documented behavioral alterations in mosquito larvae on exposure to the plant extracts, revealing restlessness, excitation, coiling movements, and formation of a ring-like structure. Keeping that in mind, investigations were conducted on the impact of AA-AgNCs on the larval behavior of A. aegypti which did not show any noticeable behavior change except the induced mortality after 24 h. This is in contrast with the previously reported results on the effects of A. aspera leaf and stem extracts on A. aegypti larval behavior (Sharma et al., 2016) inducing aggression, convulsions, and paralysis. This indicates that although A. aspera crude extracts imparted effects similar to those of nerve poisons, the nanocomposites formulated with these extracts did not act as so. Thus, to estimate their plausible mode of action as contact and stomach toxicants, further investigations were conducted on the effects of NCs on the morphology and gut histology of larvae.
The morphological study of the early fourth instars of A. aegypti exposed to a median lethal dosage of AA-AgNCs, whether formulated with leaves or stem extracts, revealed observable effects, such as pigmentation and blackening of the gut, shrunk body, impaired and disorganized gut portion, ruptured cuticle, and a slight contraction of the internal membrane of anal papillae. These effects indicate the contact and systemic toxic effects of nanocomposites impacting both the body cuticle and gut of larvae. Our earlier studies have shown the toxic effect of these NCs on the survival of A. aegypti larvae (Sharma et al., 2017; Sharma et al., 2020). It is hypothesized that a damaged gut may have impaired digestion, growth, and thus, larval survival, which is also supported by the pigmented gut content induced by AA-AgNC exposure. Similar effects of Lobelia leschenaultiana-fabricated ZnO nanocomposites have been reported by Banumathi et al. (2017) on the external morphology of A. aegypti larvae. In addition, they also observed the highly shrunk abdominal region of the larvae along with the loss of antenna and mouth brush, unlike in the current study.
In order to understand the cause of pigmented gut content induced by AA-AgNCs, the current study also examined the effect of AA-AgNCs on the mid-gut histology of A. aegypti larvae through sectional observations. It is known that the dynamic structure of the mid-gut epithelium plays a significant role in every stage of the insect lifecycle, from beginning to end (Hixson et al., 2021). In addition, the peritrophic membrane (PM), a semipermeable and non-cellular lining of the mid-gut, protects the underlying epithelium against food abrasion, chemicals, and pathogenic attacks, and facilitates the digestive process by compartmentalization of enzymes (Gusmão et al., 2002). It has been proposed that ingested toxicants and phytochemicals may cross passively and adversely affect the insect mid-gut architecture and damage the cellular structure. Furthermore, these xenobiotics may also disrupt the PM structure, aiding their transport across the mid-gut lining and imparting toxic effects on the mid-gut. The exposure of A. aegypti early fourth instars to A. aspera leaf and stem nanocomposites induced extensive damage to the mid-gut structure leading to vacuolization, disintegration of the epithelial layer, and desquamation of epithelial cells with intercellular spaces, supporting these propositions.
The deleterious effects of NCs on the larval mid-gut indicate the high systemic toxicity of AA-AgNCs impairing the digestion, absorption, and assimilation of nutrients in larvae impacting their growth and survival. Similar effects of phytomediated NCs on the mid-gut of the A. aegypti larvae when exposed to H. coronarium-synthesized silver nanoparticles (Kalimuthu et al., 2017), Ulva lactuca-fabricated ZnO-NCs (Ishwarya et al., 2018), C. serrulata-assisted Ag, Pd, and TiO2 NCs (Amutha et al., 2019), and Leonotis nepetifolia silver nanoparticles (Manimegalai et al., 2020) have been reported. Likewise, silver nanoparticles biofabricated using essential oils of Aquilaria sinensis (AsEO) and Pogostemon cablin (PcEO) caused significant damage to the epithelial and brush border cells of the larval mid-gut of A. albopictus (Ga´al et al., 2018).
The current results are in corroboration with those of Sultana et al. (2020) who investigated the effects of silver nanocomposites formulated with Cyperus rotundus extracts on the gut histology of A. albopictus, A. stephensi, and C. quinquefasciatus, and observed the complete destruction of the larval gut. Similar effects of Solanum tuberosum carbon-dot-silver nanohybrids against C. quinquefasciatus larvae leading to blackening of the gut with disintegrated cellular organization and intensely damaged cuticle (Sultana et al., 2018) have been recorded. The TEM analysis of A. aegypti larval mid-gut exposed to the leaf extract of Schinus terebinthifolius displayed drastic cell disruption, disintegrated microvilli, and electron-lucent and vacuolated cytoplasm (Procópio et al., 2015). Likewise, Barua et al. (2016) put forward the fact that on the treatment of C. quinquefasciatus with dot–silver nanohybrid fabricated using the roots of C. rotundus, the hybrids entered the larval body by rupturing the cuticular membrane and subsequently destroyed the intestinal system. They suggested that cuticle rupture in the larvae lessened the membrane penetrability and affected the proton motive forces, which disturbed the intercellular functions and caused cell death.
The TEM images of A. aegypti larvae treated with AL-AgNCs and AS-AgNCs also showed the presence of black spots inside the mid-gut, denoting the deposition of silver particles used in the formulation of nanocomposites. These observations are in accordance with those of Sultana et al. (2018) who demonstrated the presence of silver (up to 2.98 atom%) in the S. tuberosum-carbon-dot-silver nanohybrid-exposed body cells of C. quinquefasciatus larvae through electro diffraction X-ray. Similar conclusions have been made by Sap-Iam et al. (2010) who reported shrunk larvae, penetration of polymethacrylate-stabilized AgNCs through the cuticle of A. aegypti larvae, and the presence of dark spots throughout the larval body indicating deposition of silver particles.
It is proposed that the small size of the AgNCs allows their passage through the insect cuticle and entry into the gut and individual cells, where they interfere with growth, molting, and other physiological processes (Govindarajan and Benelli, 2016; Sultana et al., 2020). The increased toxic and growth regulatory potential of phytomediated AgNCs may also be ascribed to their interaction with the extracellular lipoprotein matrix increasing the membrane penetrability. Similar conclusions have been made by Sundaravadivelan and Nalini (2012) who attributed the probable cause of larval death to the penetration of AgNCs through the larval membrane by interaction with cell molecules. They may probably form bonds with sulfur- or phosphorus-containing compounds present in the mid-gut, such as DNA or proteins, causing denaturation of enzymes and affecting the functions of organelles (Gnanadesigan et al., 2011; Srikar et al., 2016; Thakur et al., 2017; Sultana et al., 2018). They are also reported to generate peroxides in the mid-gut, leading to cell death (Raffi et al., 2008; Nalini et al., 2017). Another proposition is that NCs plausibly decrease the membrane permeability by reducing ion exchange and ATP synthesis leading to cell death (Sap-Iam et al., 2010).
These studies are of immense importance in the field of Aedes management. The field studies can ascertain the use of A. aspera-mediated nanocomposites in mosquito management programs.
Conclusion
The silver nanocomposites synthesized from leaf and stem extracts of A. aspera adversely affected growth, morphology, and mid-gut histoarchitecture of early fourth instars of A. aegypti. The AgNC-exposed larvae did not show any particular change in their behavior on exposure to nanocomposites. The larvae showed shrinkage in the internal membrane of anal papillae, pigmented mid-gut, and shrunk body with an intensely damaged cuticle membrane. The mid-gut had significantly disordered internal architecture with lysed cells, damaged peritrophic membrane and microvilli, a disintegrated epithelial layer, and a ruptured and displaced basement membrane. TEM visualization of the larval mid-gut showed severe cellular damage and presence of aggregated AgNCs inside the larval body. These outcomes recommend the possible use of A. aspera nanocomposites as an effective and safe intervention against A. aegypti. Further investigations, however, would help to trace the AgNC path and understand their mode of action.
Data availability statement
The original contributions presented in the study are included in the article/Supplementary Material; further inquiries can be directed to the corresponding author.
Author contributions
AS and SK conceived and designed the study. AS, MM, and VD performed the experiments; AS and SK sourced for funds and wrote the manuscript. All authors were involved in the finalization of the manuscript.
Acknowledgments
Authors are thankful to the Principal, Acharya Narendra Dev College, University of Delhi, and the School of Sciences, Indira Gandhi National Open University, for providing infrastructure and research facilities.
Conflict of interest
The authors declare that the research was conducted in the absence of any commercial or financial relationships that could be construed as a potential conflict of interest.
Publisher’s note
All claims expressed in this article are solely those of the authors and do not necessarily represent those of their affiliated organizations, or those of the publisher, the editors, and the reviewers. Any product that may be evaluated in this article, or claim that may be made by its manufacturer, is not guaranteed or endorsed by the publisher.
References
Ahmed S., Ahmad M., Swami B. L., Ikram S. (2016). A review on plants extract mediated synthesis of silver nanoparticles for antimicrobial applications: A green expertise. J. Adv. Res. 7, 17–28. doi:10.1016/j.jare.2015.02.007
Amaladhas P. T., Usha M., Naveen S. (2013). Sunlight induced rapid synthesis and kinetics of silver nanoparticles using leaf extract of Achyranthes aspera L. and their antimicrobial applications. Adv. Mat. Lett. 4, 779–785. doi:10.5185/amlett.2013.2427
Amutha V., Deepak P., Kamaraj C., Balasubramani G., Aiswarya D., Arul D., et al. (2019). Mosquito-larvicidal potential of metal and oxide nanoparticles synthesized from aqueous extract of the seagrass, Cymodocea serrulata. J. Clust. Sci. 30, 797–812. doi:10.1007/s10876-019-01542-7
Bagavan A., Rahuman A. A., Kamaraj C., Geetha K. (2008). Larvicidal activity of saponin from Achyranthes aspera against Aedes aegypti and Culex quinquefasciatus (Diptera: Culicidae). Parasitol. Res. 103, 223–229. doi:10.1007/s00436-008-0962-z
Banumathi B., Vaseeharan B., Ishwarya R., Govindarajan M., Alharbi N. S., Kadaikunnan S., et al. (2017). Toxicity of herbal extracts used in ethno-veterinary medicine and green-encapsulated ZnO nanoparticles against Aedes aegypti and microbial pathogens. Parasitol. Res. 116, 1637–1651. doi:10.1007/s00436-017-5438-6
Barua S., Raul P. K., Gopalakrishnan R., Das B., Veer V. (2016). Sustainable-resource-based carbon dot–silver nanohybrid: A strong tool against Culex quinquefasciatus, a common disease vector. ACS Sustain. Chem. Eng. 4, 2345–2350. doi:10.1021/acssuschemeng.6b00015
Benelli G., Mehlhorn H. (2016). Declining malaria, rising of dengue and zika virus: Insights for mosquito vector control. Parasitol. Res. 115, 1747–1754. doi:10.1007/s00436-016-4971-z
Dulacha D., Were V., Oyugi E., Kiptui R., Owiny M., Boru W., et al. (2022). Reduction in malaria burden following the introduction of indoor residual spraying in areas protected by long-lasting insecticidal nets in Western Kenya, 2016–2018. PLoS One 17 (4), e0266736. doi:10.1371/journal.pone.0266736
Elumalai D., Kaleena P. K., Ashok K., Suresh A., Hemavathi M. (2016). Green synthesis of silver nanoparticle using Achyranthes aspera and its larvicidal activity against three major mosquito vectors. Eng. Agric. Environ. Food 9, 1–8. doi:10.1016/j.eaef.2015.08.002
Ga’al H., Fouad H., Mao G., Tian J., Jianchu M. (2018). Larvicidal and pupicidal evaluation of silver nanoparticles synthesized using Aquilaria sinensis and Pogostemon cablin essential oils against dengue and zika viruses vector Aedes albopictus mosquito and its histopathological analysis. Artif. Cells Nanomed. Biotechnol. 46, 1171–1179. doi:10.1080/21691401.2017.1365723
Gnanadesigan M., Anand M., Ravikumar S., Maruthupandy M., Vijaykumar V., Selvam , et al. (2011). Biosynthesis of silver nanoparticles by using mangrove plant extract and their potential mosquito larvicidal property. Asian pac. J. Trop. Med. 4, 799–803. doi:10.1016/S1995-7645(11)60197-1
Govindarajan M., Benelli G. (2016). A facile one-pot synthesis of eco-friendly nanoparticles using Carissa carandas: Ovicidal and larvicidal potential on malaria, dengue and filariasis mosquito vectors. J. Clust. Sci. 28, 15–36. doi:10.1007/s10876-016-1035-6
Govindarajan M., Hoti S. L., Benelli G. (2016). Facile fabrication of eco-friendly nano mosquitocides: Biophysical characterization and effectiveness on neglected tropical mosquito vectors. Enzyme Microb. Technol. 95, 155–163. doi:10.1016/j.enzmictec.2016.05.005
Gunathilaka U. M. T. M., de Silva W. A. P. P., Dunuweera S. P., Rajapakse R. M. G. (2021). Effect of morphology on larvicidal activity of chemically synthesized zinc oxide nanoparticles against mosquito vectors. RSC Adv. 11, 8857–8866. doi:10.1039/d1ra00014d
Gusmão D. S., Páscoa V., Mathias L., Vieira I. J. C., Braz-Filho R., Lemos F. J. A. (2002). Derris (Lonchocarpus) urucu (leguminosae) extract modifies the peritrophic matrix structure of Aedes aegypti (Diptera: Culicidae). Mem. Inst. Oswaldo Cruz 97, 371–375. doi:10.1590/s0074-02762002000300017
Hixson B., Taracena M. L., Buchon N. (2021). Midgut epithelial dynamics are central to mosquitoes’ physiology and fitness, and to the transmission of vector-borne disease. Front. Cell. Infect. Microbiol. 11, 653156. doi:10.3389/fcimb.2021.653156
Ishwarya R., Vaseeharan B., Kalyani S., Banumathi B., Govindarajan M., Alharbi N. S., et al. (2018). Facile green synthesis of zinc oxide nanoparticles using Ulva lactuca seaweed extract and evaluation of their photocatalytic, antibiofilm and insecticidal activity. J. Photochem. Photobiol. B 178, 249–258. doi:10.1016/j.jphotobiol.2017.11.006
Kabtiyal N., Bayas R., Shinde L., Goyal M. (2022). Review article on the synthesis of silver nanoparticles from plant extract and its larvicidal activity on the mosquito. Int. J. Mosq. Res. 9, 01–12. doi:10.22271/23487941.2022.v9.i3a.608
Kalimuthu K., Panneerselvam C., Chou C., Tseng L. C., Murugan K., Tsai K. H., et al. (2017). Control of dengue and zika virus vector Aedes aegypti using the predatory copepod Megacyclops formosanus: Synergy with Hedychium coronarium-synthesized silver nanoparticles and related histological changes in targeted mosquitoes. Process Saf. Environ. Prot. 109, 82–96. doi:10.1016/j.psep.2017.03.027
Manimegalai T., Raguvaran K., Kalpana M., Maheswaran R. (2020). Green synthesis of silver nanoparticle using Leonotis nepetifolia and their toxicity against vector mosquitoes of Aedes aegypti and Culex quinquefasciatus and agricultural pests of Spodoptera litura and Helicoverpa armigera. Environ. Sci. Pollut. Res. Int. 27, 43103–43116. doi:10.1007/s11356-020-10127-1
Morejón B., Pilaquinga F., Domenech F., Ganchala D., Debut A., Neira M. (2018). Larvicidal activity of silver nanoparticles synthesized using extracts of Ambrosia arborescens (asteraceae) to control Aedes aegypti L. (Diptera: Culicidae). J. Nanotechnol. 2018, 1–8. Article ID 6917938. doi:10.1155/2018/6917938
Nalini M., Lena M., Sumathi P., Sundaravadivelan C. (2017). Effect of phyto-synthesized silver nanoparticles on developmental stages of malaria vector, Anopheles stephensi and dengue vector, Aedes aegypti. Egypt. J. Basic Appl. Sci. 4, 212–218. doi:10.1016/j.ejbas.2017.04.005
National Vector Borne Disease Control Programme (2022). Dengue cases and deaths in the country since. Online. Available at: https://nvbdcp.gov.in/index4.php?lang=1&level=0&linkid=431&lid=3715 (Accessed on July 19, 2022).
Parthiban E., Manivannan N., Ramanibai R., Mathivanan N. (2019). Green synthesis of silver-nanoparticles from Annona reticulata leaves aqueous extract and its mosquito larvicidal and anti-microbial activity on human pathogens. Biotechnol. Rep. 21, e00297. doi:10.1016/j.btre.2018.e00297
Procópio T. F., Fernandes K. M., Pontual E. V., Ximenes R. M., de Oliveira A. R., de Santana Souza C., et al. (2015). Schinus terebinthifolius leaf extract causes midgut damage, interfering with survival and development of Aedes aegypti larvae. PloS One 10, e0126612. doi:10.1371/journal.pone.0126612
Raffi M., Hussain F., Bhatti T. M., Akhter J. I., Hameed A., Hasan M. M. (2008). Antibacterial characterization of silver nanoparticles against E. coli ATCC-15224. J. Mat. Sci. Technol. 24, 192–196.
Sabri M. A., Umer A., Awan G. H., Hassan M. F., Hasnain A. (2016). Selection of suitable biological method for the synthesis of silver nanoparticles. Nanomater. Nanotechnol. 6, 29. doi:10.5772/62644
Salunkhe R. B., Patil S. V., Patil C. D., Salunke B. K. (2011). Larvicidal potential of silver nanoparticles synthesized using fungus Cochliobolus lunatus against Aedes aegypti (Linnaeus, 1762) and Anopheles stephensi Liston (Diptera; Culicidae). Parasitol. Res. 109, 823–831. doi:10.1007/s00436-011-2328-1
Sap-Iam N., Homklinchan C., Larpudomlert R., Warisnoicharoen W., Sereemaspun A., Dubas S. T. (2010). UV irradiation-induced silver nanoparticles as mosquito larvicides. J. Appl. Sci. 10, 3132–3136. doi:10.3923/jas.2010.3132.3136
Shah M., Fawcett D., Sharma S., Tripathy S. K., Poinern G. E. J. (2015). Green synthesis of metallic nanoparticles via biological entities. Materials 8, 7278–7308. doi:10.3390/ma8115377
Sharma A., Kumar S., Tripathi P. (2016). Evaluation of the larvicidal efficacy of five indigenous weeds against an Indian strain of dengue vector, Aedes aegypti L. (Diptera: Culicidae). J. Parasitol. Res. 2016, 2857089. 1–8. doi:10.1155/2016/2857089
Sharma A., Kumar S., Tripathi P. (2017). A facile and rapid method for green synthesis of Achyranthes aspera stem extract-mediated silver nanocomposites with cidal potential against Aedes aegypti L. Saudi J. Biol. Sci. 26, 698–708. doi:10.1016/j.sjbs.2017.11.001
Sharma A., Tripathi P., Kumar S. (2020). One-pot synthesis of silver nanocomposites from Achyranthes aspera: An eco-friendly larvicide against Aedes aegypti L. Asian pac. J. Trop. Biomed. 10, 54–64. doi:10.4103/2221-1691.275420
Srikar S. K., Giri D. D., Pal D. B., Mishra P. K., Upadhyay S. N. (2016). Green synthesis of silver nanoparticles: A review. Green sustain. Chem. 6, 34–56. doi:10.4236/gsc.2016.61004
Sultana N., Raul P. K., Goswami D., Das B., Gogoi H. K., Raju P. S. (2018). Nanoweapon: Control of mosquito breeding using carbon-dot-silver nanohybrid as a biolarvicide. Environ. Chem. Lett. 16, 1017–1023. doi:10.1007/s10311-018-0712-0
Sultana N., Raul P. K., Goswami D., Das D., Islam S., Tyagi V., et al. (2020). Bio-nanoparticle assembly: A potent on-site biolarvicidal agent against mosquito vectors. RSC Adv. 10, 9356–9368. doi:10.1039/c9ra09972g
Sundaravadivelan C., Nalini M. (2012). Biolarvicidal effect of phyto-synthesized silver nanoparticles using Pedilanthus tithymaloides (L.) Poit stem extract against the dengue vector Aedes aegypti L. (Diptera; Culicidae). Asian pac. J. Trop. Biomed. 17, 1–8.
Thakkar K. N., Mhatre S. S., Parikh R. Y. (2010). Biological synthesis of metallic nanoparticles. Nanomedicine 6, 257–262. doi:10.1016/j.nano.2009.07.002
Thakur S., Mohan K. G., Rani S. M. (2017). Plant-mediated synthesis of silver nanoparticles – a critical review. Int. J. Pharmacogn. Phytochem. Res. 9, 947–956. doi:10.25258/phyto.v9i07.11161
Tran Q. H., Le A. T. (2013). Silver nanoparticles: Synthesis, properties, toxicology, applications and perspectives. Adv. Nat. Sci. Nanosci. Nanotechnol. 4, 033001. doi:10.1088/2043-6262/4/3/033001
Vijayaraghavan K., Nalini S. K., Prakash N. U., Madhankumar D. (2012). Biomimetic synthesis of silver nanoparticles by aqueous extract of Syzygium aromaticum. Mat. Lett. 75, 33–35. doi:10.1016/j.matlet.2012.01.083
World Health Organization (WHO) (2022). Dengue and severe dengue. Available at: https://www.who.int/news-room/fact-sheets/detail/dengue-and-severe-dengue.
Keywords: Achyranthes aspera, Aedes aegypti, development, histological architecture, morphology, silver nanocomposites, stomach poison, TEM
Citation: Sharma A, Mishra M, Dagar VS and Kumar S (2022) Morphological and physiological changes induced by Achyranthes aspera-mediated silver nanocomposites in Aedes aegypti larvae. Front. Physiol. 13:1031285. doi: 10.3389/fphys.2022.1031285
Received: 29 August 2022; Accepted: 23 September 2022;
Published: 12 October 2022.
Edited by:
Vincenzo Parrino, University of Messina, ItalyReviewed by:
Sengodan Karthi, Manonmaniam Sundaranar University, IndiaMohamed Hamed, Al Azhar University, Egypt
Copyright © 2022 Sharma, Mishra, Dagar and Kumar. This is an open-access article distributed under the terms of the Creative Commons Attribution License (CC BY). The use, distribution or reproduction in other forums is permitted, provided the original author(s) and the copyright owner(s) are credited and that the original publication in this journal is cited, in accordance with accepted academic practice. No use, distribution or reproduction is permitted which does not comply with these terms.
*Correspondence: Sarita Kumar, saritakumar@andc.du.ac.in
†These authors have contributed equally to this work