Erratum: Assessment of sublethal and transgenerational effects of spirotetramat, on population growth of cabbage aphid, Brevicoryne brassicaeL. (Hemiptera: Aphididae)
- 1Entomological Research Institute, Ayub Agricultural Research Institute, Faisalabad, Pakistan
- 2Department of Entomology, Faculty of Crop and Food Sciences, Pir Mehr Ali Shah, Arid Agriculture University, Rawalpindi, Pakistan
- 3Department of Entomology, Faculty of Agricultural Sciences, University of the Punjab, Lahore, Pakistan
- 4Department of Zoology, University of Okara, Okara, Pakistan
- 5Department of Agriculture, Mir Chakar Khan Rind University, Sibi, Pakistan
- 6State Key Laboratory of Rice Biology and Ministry of Agriculture Key Lab of Molecular Biology of Crop Pathogens and Insects, Institute of Insect Sciences, Zhejiang University, Hangzhou, China
- 7Key Laboratory of Plant Genetics and Breeding, College of Agriculture, Guangxi University, Nanning, China
- 8Department of Zoology, Faculty of Basic Sciences, University of the Punjab, Lahore, Pakistan
- 9Food Engineering Department, Faculty of Food Science and Technology, University of Agricultural Science and Veterinary Medicine Cluj-Napoca, Cluj-Napoca, Romania
- 10Biology Department, Faculty of Science, King Khalid University, Abha, Saudi Arabia
- 11Department of Biology, College of Science, King Khalid University, Abha, Saudi Arabia
- 12Department of Biology, Saudi Arabia Research Center for Advanced Materials Science (RCAMS), College of Science, King Khalid University, Abha, Saudi Arabia
The cabbage aphid (Brevicoryne brassicae L.) is a devastating pest of cruciferous crops causing economic damage worldwide and notably owing to its increasing resistance to commonly used pesticides. Such resistance prompts the development of integrated pest management (IPM) programs that include novel pesticides being effective against the aphids. Spirotetramat is a novel insecticide used against sap-sucking insect pests, particularly aphids. This study evaluated the toxicity of spirotetramat to adult apterous B. brassicae after 72 h using the leaf dipping method. According to the toxicity bioassay results, the LC50 value of spirotetramat to B. brassicae was 1.304 mgL−1. However, the sublethal concentrations (LC5 and LC15) and transgenerational effects of this novel insecticide on population growth parameters were estimated using the age-stage, two-sex life table theory method. The sublethal concentrations (LC5; 0.125 mgL−1 and LC15; 0.298 mgL−1) of spirotetramat reduced the adult longevity and fecundity of the parent generation (F0). These concentrations prolonged the preadult developmental duration while decreasing preadult survival, adult longevity and reproduction of the F1 generation. The adult pre-reproductive period was also extended by spirotetramat treatment groups. Subsequently, the population growth parameters such as the intrinsic rate of increase r, finite rate of increase λ and net reproductive rate R0 of the F1 generation were decreased in spirotetramat treatment groups whereas, the mean generation time T of the F1 generation was not affected when compared to the control. These results indicated the negative effect of sublethal concentrations of spirotetramat on the performance of B. brassicae by reducing its nymphal survival, extending the duration of some immature stages and suppressing the population growth of B. brassicae. Overall, we demonstrated that spirotetramat is a pesticide showing both sublethal activities, and transgenerational effects on cabbage aphid; it may be useful for implementation in IPM programs against this aphid pest.
Introduction
The cabbage aphid, Brevicoryne brassicae Linnaeus (Hemiptera: Aphididae), is one of the most destructive pests of the Brassicaceae family and can be found around the globe (Anzabi et al., 2014; Sarhozaki and Safavi, 2014; Ahmed et al., 2020; Shonga and Getu, 2021a; Shonga and Getu, 2021b) including Pakistan (Abbas et al., 2017). It causes damage directly by sucking the plants almost in all growth stages and indirectly by transmitting diseases or secreting honeydew (Bashir and Azim, 2013). It is also known to be the vector of various plant viruses (Dáder et al., 2017). Although various management technologies have been developed and implemented against B. brassicae (e.g., promoting biocontrol services, (Lu et al., 2012), its management still primarily relies on the application of pesticides (Shang et al., 2012; Roh et al., 2015).
Many new insecticides have been developed and commercially available that are safer for the environment and human health and control insect pests more effectively (Babcock et al., 2011). Spirotetramat, a tetramic acid-based insecticide with a novel mode of action, belonging to a new cyclic keto-enol compound developed by Bayer Crop Science is being used worldwide against aphids, mites and other piercing-sucking pests of crops (Brück et al., 2009; Wang et al., 2016). It has distinctive translocation properties in that after foliar application; it is simultaneously translocated upwards by the xylem and downwards through the phloem (Brück et al., 2009). Spirotetramat acts as a lipid biosynthesis inhibitor that reduces the fecundity and fertility of sucking insect pests (Gong et al., 2016a; Salazar-López et al., 2016). The lipids are of vibrant significance to many insects for metamorphosis, embryogenesis and flight (Arrese et al., 2001). Due to the absence of cross-resistance to prevailing classes of chemical insecticides, spirotetramat may be a significant tool to achieve insecticide resistance in many crop pests around the globe (Ouyang et al., 2012; Döker et al., 2021). This has prompted the development of new pest management strategies and products, for example exploring and developing novel pesticides.
One of the main challenges in toxicology is how to evaluate the overall effect of toxic substances on insect populations (Lashkari et al., 2007). Traditional approaches to determining the lethal concentration of insecticides on insects are centered on assessing individual mortality in the short term; however, the elucidation of acquired data at the population level is inadequate due to the inadequacy of the number of endpoints (Stark and Banks, 2003; Desneux et al., 2007). After the application of insecticides in agricultural systems, insecticides may degrade to sublethal and low lethal doses over time in the field due to which some target pests do not show rapid mortality to the lethal dose (Mahmoodi et al., 2020; Ullah et al., 2020; Jie et al., 2021). Sublethal effects have been described as effects on the physiology and behavior of an individual that survives exposure to an insecticide or toxin at the sublethal or lethal dose/concentration (Desneux et al., 2007). Numerous studies have been carried out on this problem which showed that insect pests exposed to these lethal or sublethal doses or concentrations of toxicants go through several physiological and behavior impairments, hormesis, and better tolerance for chemical materials (Desneux et al., 2006; Tan et al., 2012; Guedes et al., 2016; Yousaf et al., 2018; Lv et al., 2021; Zhang et al., 2021). Besides mortality, sublethal effects of insecticides may be manifested in many ways, such as biological and behavioral parameters including developmental time, fecundity, longevity, sex ratio, feeding activity, predation rate, orientation, and mobility. (Desneux et al., 2007; Guedes et al., 2016). and may result not only from direct contact with herbicides but also as a result of feeding on contaminated food. Moreover, positive and negative effects triggered by the sublethal doses of insecticides can be transmitted from offspring to several filial generations (Hercus and Hoffmann, 2000; Shikano, 2017; Mahmoodi et al., 2020).
It is necessary to assess the sublethal effects to optimize the application of insecticides along with their toxic effects to evaluate their effectiveness precisely (Stark and Banks, 2003). Attaining this evidence can help in explaining the cases of insect outbreaks and pest reappearance as a result of prior pesticide applications. Another advantage of the complete assessment of the sublethal effects of pesticides would be getting the capability to develop more effective and environment-friendly procedures (Desneux et al., 2007; Rahmani and Bandani, 2013; Sohrabi et al., 2013). Demographic toxicology is an ecotoxicological approach that assimilates the life history and life table in the circumstantial of toxicology (Sarhozaki and Safavi, 2014). It helps appraise the sublethal special effects of a pesticide on the anticipated population development of the embattled pests (Stark and Banks, 2003; Lashkari et al., 2007). Insect life tables are helpful and dynamic tools for the estimation of sublethal effects of synthetic and natural insecticides on insect pests (Cutler et al., 2009; Saska et al., 2016; Liang et al., 2019). This is because their limitations openly imitate the wide-ranging effect of biological features (i.e., survival, reproduction, development, and sex ratio) on population fitness. Sublethal effects of many classes of insecticides, i.e., pymetrozine, imidacloprid, thiamethoxam, thiamethoxam-lambda cyhalothrin, buprofezin, and acetamiprid (Lashkari et al., 2007; Sarhozaki and Safavi, 2014) and (Mahmoodi et al., 2020) has been studied on cabbage aphid.
To date, no reports have been found regarding sublethal and transgenerational effects of spirotetramat on B. brassicae. In the present study we investigated within- and transgenerational (maternal) effects of sublethal insecticide stress on several fitness-associated traits; survival, and development time to understand the relations between the exposure doses of spirotetramat and insect response at both the individual and population levels.
Materials and methods
Insect rearing
The cabbage aphid was used as a study insect and was collected from brassica crop (Brassica napus L. var. canola) grown at the research area of Ayub Agricultural Research Institute, Faisalabad (31.4041° N, 730,487° E). The stock culture of B. brassicae was established on insecticide-free leaves of brassica plants under standard circumstances (24 ± 1°C temperature, 70 ± 5% relative humidity and 16:8 h light-dark period) at Entomological Research Institute, Faisalabad. Insecticide-free brassica plants were replaced every week.
Chemical and toxicity bioassays
The spirotetramat (CAS No. 203313-25-1; Movento® 240 SC; 240 g/L active ingredients) was obtained from Bayer Crop Science Co. Ltd. (Australia). The insecticide tested in this study is registered and being used in brassica crops in Pakistan to manage aphids. Toxicity bioassays were conducted with apterous adult aphids using the leaf dip method described by (Ullah et al., 2020) to measure the lethal and sublethal toxicity of spirotetramat. The distilled water was utilized to make spirotetramat concentrations (6, 3, 1.5, 0.75 and 0.375 mgL−1). Distilled water dipped leaf discs were used only for the control group. Brassica leaf discs were cut by a sharp metal cylinder and dipped in the dilutions of respective insecticide solution for 30 s. The treated leaf discs were placed at room temperature for 30 min to dry residual solution droplets on the leaves. The treated leaves were placed in plastic Petri dishes (size: 3 × 1.5 cm, with a biaxial surface downward) lined with moistened filter paper. Each concentration comprised three replications of 30 apterous adult aphids (≤24 h old) and five leaves were used for each replication. Mortality data were estimated at 72 h of spirotetramat exposure. Aphids were scored as dead if they did not exhibit repetitive (i.e., non-reflex) movement when gently probed with a soft camel hair brush (Moores et al., 1996). The lethal concentrations LC5, LC15, and LC50 were calculated by using PoloPlus 2.0 software (LeOra Software Inc. Berkely, CA).
Sublethal response of spirotetramat on F0 generation
Approximately 650 adult aphids were transferred to insecticide-free brassica leaves. All adult apterous aphids were removed after 72 h, while the neonate nymphs were retained on the leaves for 08 days to become adults. This procedure was applied to ensure the same age group of aphids before exposure to the insecticide. Therefore, the LC5 (0.125 mgL−1), as well as LC15 (0.298 mgL−1) concentrations of spirotetramat, were used in this study to evaluate their impact on the F0 generation of B. brassicae and distilled water was used as control. Brassica leaf discs were uniformly dipped in LC5 and LC15 of spirotetramat and control solution for 30 s, air-dried for 30 min. Then the dry treated leaf discs were placed in plastic Petri dishes with their biaxial surface downward containing moistened filter paper to maintain humidity. Adult apterous aphids were released to feed on these treated leaves and control solution for 72 h. After that, sixty healthy and surviving aphids were transferred to untreated fresh leaf discs in plastic Petri dishes individually. Adults of the F0 generation were inspected daily for recording longevity and fecundity, while the newborn nymphs were removed until the adult died. The leaf discs were changed every 3–4 days to prevent fungal growth until the adult aphids died.
Sublethal response of spirotetramat on the F1 generation
New-born nymphs (age ˂24 h) obtained from F0 adults, were gathered as F1 generation and then transferred to Petri-dishes independently. These aphids (F1 generation) were individually reared on insecticide-free brassica leaf discs, as described in the previous F0 generation. This method was repeated 60 times for the spirotetramat treatments (LC5 and LC15) and the control, treating each aphid as a single replication. Survival, development and reproduction were noted on daily basis. During the reproductive period, newborn nymphs were counted-up and then removed. Fresh leaf discs were changed every 3–4 days until the death of the adult aphid.
Statistical analysis
The bioassay data were used to calculate the lethal (LC50) and sublethal (LC5 and LC15) concentrations of spirotetramat by using Probit analysis (Finney, 1971) in PoloPlus 2.0 (Software, 2005). The life-history data of cabbage aphids exposed to sublethal concentrations of spirotetramat and the control were subjected to the computer-based program software (TWO SEX-MSChart) (Chi H, 2022) and analyzed by employing the age-stage two-sex life table theory (Chi and Liu, 1985; Chi, 1988). The life table parameters sxj, lx, mx, exj and vxj (age-stage survival rate, age-specific survival rate, age-specific fecundity, age-stage life expectancy and age-stage reproductive value, respectively) were estimated (where x is the age and j is the stage of insect). The population parameters including r, λ, R0 and T (intrinsic rate of increase, finite rate of increase, net reproductive rate and mean generation time, respectively) were also estimated. The life expectancy (exj) was determined according to (Chi and Su, 2006). The reproductive value (vxj) was calculated according to (Tuan et al., 2014a; 2014b). The standard bootstrap method was used with 100,000 resampling to calculate the variance as well as standard errors for biological and population growth parameters (Efron and Tibshirani, 1993; Akköprü et al., 2015). A paired-bootstrap-test at a 5% significance level based on the confidence interval of differences was used to analyze differences among treatments. The bootstrap along with the paired bootstrap test was also included in the TWO SEX-MSChart computer program.
Results
Toxicity of spirotetramat to apterous adult B. brassicae
The toxicity of spirotetramat against apterous adult cabbage aphid was determined after exposure for 72 h (Table 1). The estimated value of LC50 with a 95% confidence interval was 1.304 mgL−1, while LC5 and LC15 values were 0.125 mgL−1 and 0.298 mgL−1, respectively. The LC5 and LC15 values of spirotetramat obtained were selected to further estimate the sublethal as well as transgenerational effects of spirotetramat on demographic parameters of B. brassicae.
Sublethal response of spirotetramat on longevity and fecundity of parent F0 generation of B. brassicae
The longevity and fecundity of test individuals (F0 generation) were affected when exposed for 72 h to the two sublethal concentrations of spirotetramat (LC5 and LC15) as compared with the control (Table 2). The longevity of B. brassicae adults was significantly reduced when treated with Spirotetramat at LC15 as compared to the control (p < 0.00001), while that recorded on adults treated with insecticide at LC5 did not show a significant difference. A similar trend of the longevity of B. brassicae adults was found between LC5 and LC15 (p < 0.00053). Furthermore, F0 adults showed significantly reduced fecundity in both treatments (LC5 and LC15) as compared to the control (p < 0.00001). It was 31.75 nymphs/female in the control group, 17.31 nymph/female and 14.68 nymphs/female in the adults treated at LC5 and LC15 concentrations of the spirotetramat insecticide, respectively. There was a non-significant difference in fecundity between LC5 and LC15 treated groups.
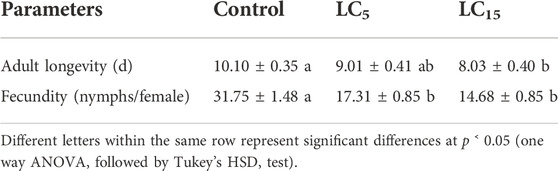
TABLE 2. Effect of exposure of parent adults (F0 generation) of Brevicoryne brassicae to Spirotetramat at LC5 and LC15 on their longevity and fecundity (Mean ± SE).
Transgenerational sublethal effects of spirotetramat on biological parameters of the F1 generation of B. brassicae
Table 3 indicates the developmental duration, longevity and fecundity of the subsequent progeny generation (F1) of B. brassicae exposed to sublethal concentrations (LC5 and LC15) of spirotetramat. However, the duration of immature developmental stages, pre-adult duration, pre-adult survival, adult pre-reproductive period (APRP) and total pre-reproductive period (TPRP) of the F1 generation did not show significant differences among treated groups. The values of adult longevity, total longevity and fecundity were recorded higher in control treatment as compared to the populations treated with sublethal concentrations of spirotetramat. Significantly lower values of adult longevity and total longevity between LC5 and LC15 as compared to the control (p < 0.00001), similarly significantly lower fecundity were observed in populations treated with spirotetramat at LC5 and LC15 as compared to the control (p < 0.00001).
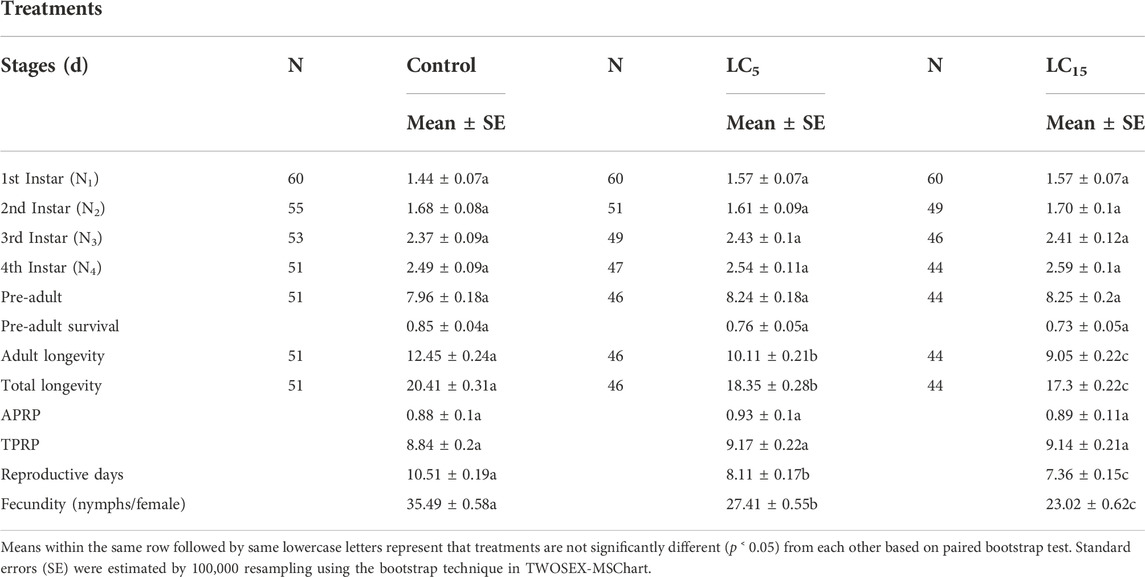
TABLE 3. Developmental duration, longevity and fecundity of different stages for F1 generation B. brassicae after exposure of parental adult (F0) to the LC5 and LC15 of Spirotetramat.
Transgenerational sublethal effects of spirotetramat on population growth parameters of the F1 generation of B. brassicae
The stage differentiation and overlapping survival curves in the F1 generation of B. brassicae exposed to LC5 and LC15 of spirotetramat are shown in Figure 1(A–C). Age-stage specific survival rate sxj is the expected duration of neonate nymphs that will survive to age x and stage j. The probability of reaching the adult stage was 0.73, 0.75, and 0.83 for a neonate nymph from LC15, LC5 and control groups, respectively. Age-specific survival rate (lx), age-specific fecundity (mx) and age-specific net maternity (lxmx) for LC5, LC15 and control treatments of B. brassicae are presented in Figure 2(A–C). The age-specific survival rate (lx) of B. brassicae decreased with age x and the maximum survival period for LC5 and LC15 concentrations of spirotetramat were 15 days and 14 days, respectively. This was lower than the maximum survival period of the control group (16 days). The age-specific fecundity (mx) curves for LC5 (4.08 offspring and 3.00 offspring) at age of 12 and 21 days while mx curve for LC15 (3.52 offspring) occurred at the age of 12 days, compared with the control (4.39 offspring) at age of 11 days. The maximum values of age-specific maternity (lxmx) for LC5, LC15 and control were 3.73, 3.13, and 2.58 offspring, respectively.
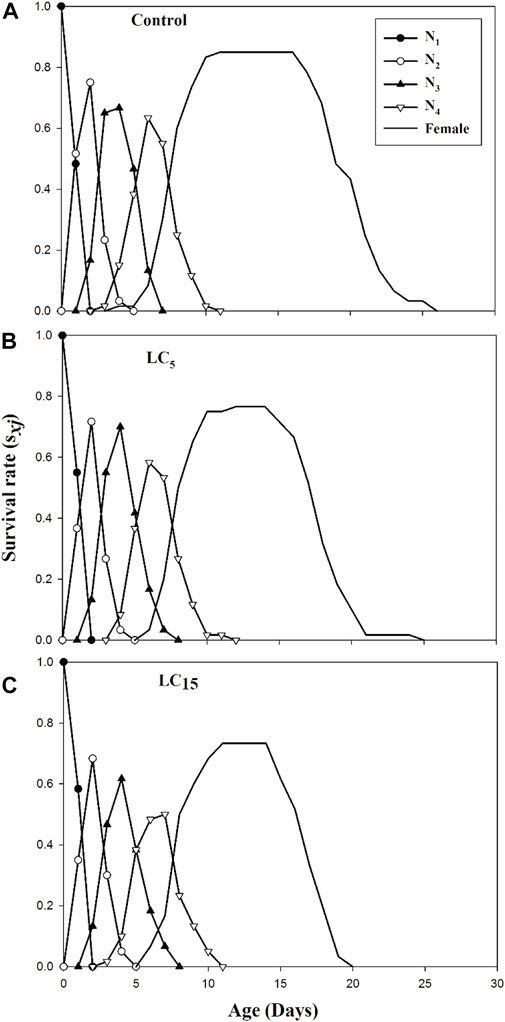
FIGURE 1. (A–C): Age-stage specific survival rate (sxj) of control group (A) and B. brassicae treated with LC5 (B) and LC15(C) of Spirotetramat in F1 generation.
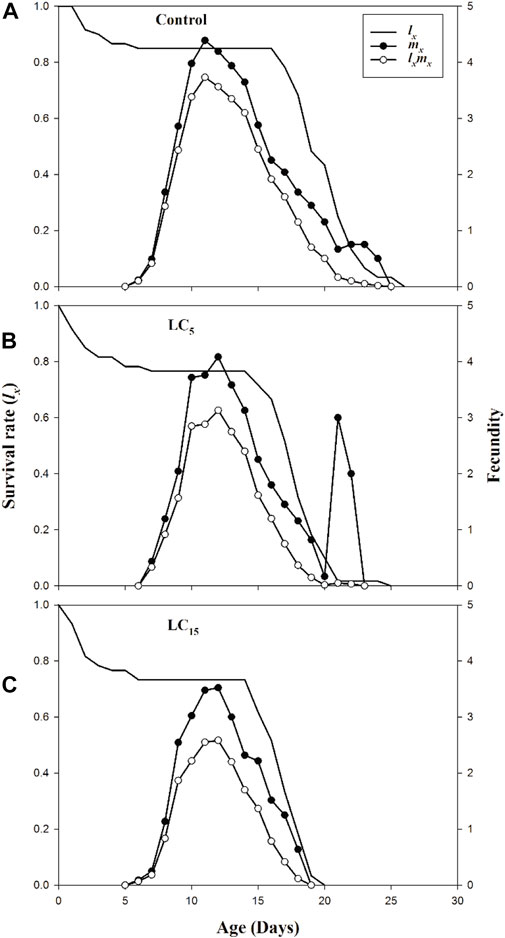
FIGURE 2. (A–C): Age specific survival rate (lx), age specific fecundity (mx) and net maternity (lxmx) of control group (A) and B. brassicae treated with LC5 (B) and LC15 (C) of Spirotetramat in F1 generation.
The age-stage specific life expectancy (exj) represents the expected lifespan of individual B. brassicae exposed to spirotetramat treated populations (LC5 and LC15) and control (Figures 3A–C).
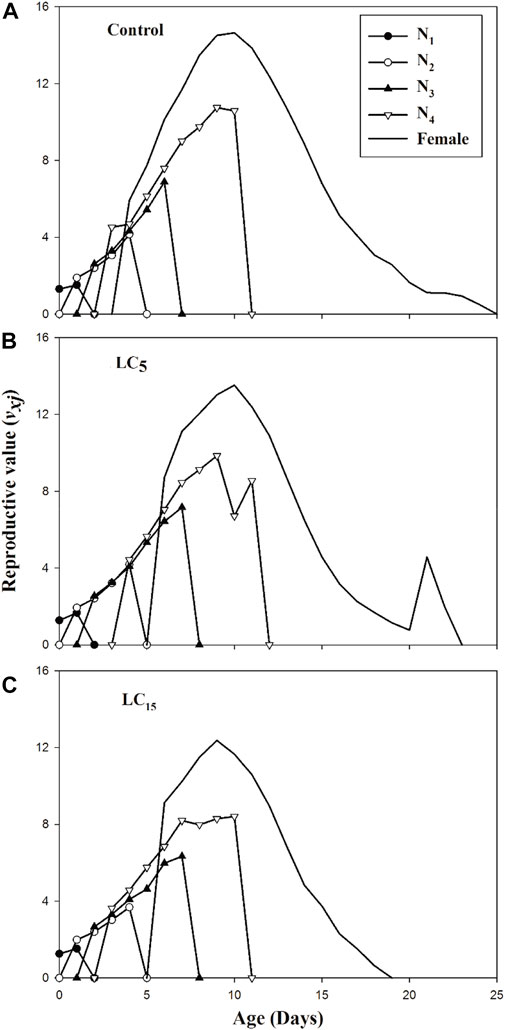
FIGURE 3. (A–C): Age-Stage specific reproductive value (vxj) of control group (A) and B. brassicae treated with LC5 (B) and LC15 (C) of Spirotetramat in F1 generation.
The exj curves showed that individuals in the control group of F1 generation are expected to survive longer than the spirotetramat treated population (LC5 and LC15). The age-stage specific reproductive value (vxj) exhibits the prediction of future offspring for individuals of B. brassicae from age x to stage j (Figures 4A–C). The highest reproductive value (vxj) peak was identified in the control group (v10 = 14.64), the peak value obtained for LC5 treated population was different from the control group although occurred on the same day (v10 = 13.52) while the earliest peak was observed in LC15 treated population (v9 = 12.36).
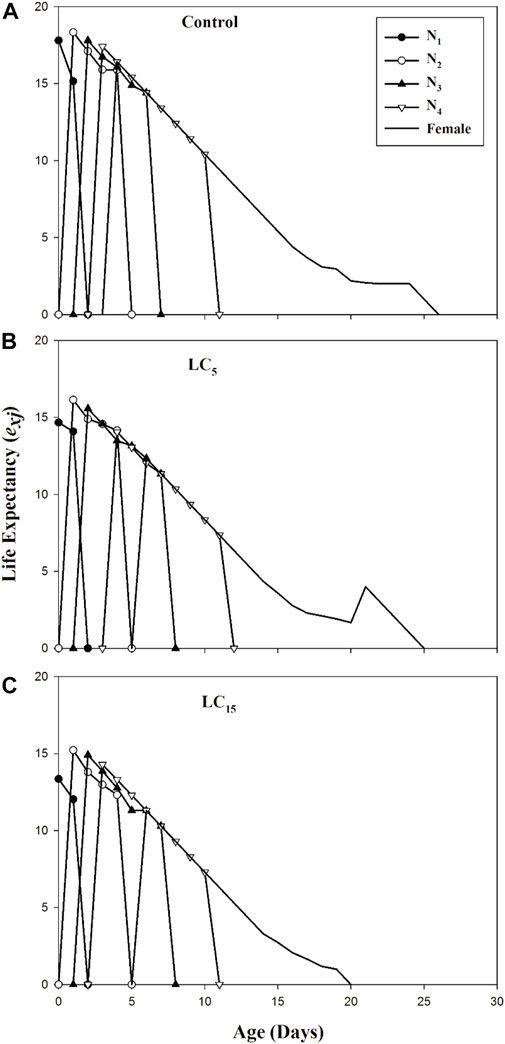
FIGURE 4. (A–C): Age-stage specific life expectancy (exj) of control group (A) and B. brassicae treated with LC5 (B) and LC15 (C) of Spirotetramat in F1 generation.
Population growth parameters of the F1 generation of B. brassicae treated with sublethal concentrations of the insecticide are shown in Table 4. The mean generation time (T) did not affect all the treatments. Moreover, the net reproductive rate (R0) was reduced from 30.16 offspring/individual in the control group to 16.88 offspring/individual in the population treated with LC5 and LC15 of the insecticide (p < 0.00001). The intrinsic rate of increase (r) (p < 0.00743) and finite rate of increase (λ) (0.00727) of F1 individuals were reduced at both sublethal concentrations of insecticide (LC5 and LC15) in comparison to those of the control group.
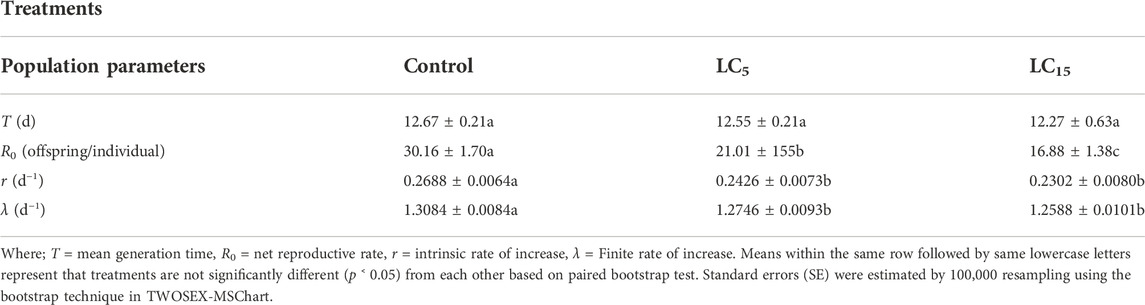
TABLE 4. Sublethal effects of Spirotetramat on population growth parameters (Mean ± SE) of F1 generation of B. brassicae.
Discussion
Resistance of B. brassicae has been reported to many organophosphates and pyrethroids (Ahmad and Akhtar, 2013). Due to this farmers have to increase the frequency of insecticide application which intern causes more resistance to these insecticides and also increases environmental pollution (Liang et al., 2019). Thus the study of this innovative substitute, Spirotetramat, is crucial in adjourning the increase of resistance to B. brassicae. The information regarding sublethal responses of this novel chemical has been reported on various insects, including Myzus persicae (Wang et al., 2016), Aphis gossypii (Gong et al., 2016b), Tetranychus urticae (Marcic et al., 2012), Encarsia Formosa (Drobnjaković and Marčić, 2021) and Cryptolaemus montrozuieri (Planes et al., 2013) but the information regarding resistance to a novel mode of insecticides action is lacking (Ahmed et al., 2020). In the present study, potential sublethal and transgenerational effects of spirotetramat on B. brassicae were assessed for two succeeding generations (F0 and F1). In a previous study, spirotetramat acts gentler, however, with the main influence on undeveloped phases and expressively disturbs the fertility and fecundity of M. persicae by triggering a high fraction of nonviable nymphs (Wang et al., 2016).
In the current study, all life table parameters of the filial generation, r, λ, and R0, as well as development, fecundity, duration of oviposition period, longevity and survival of both the treated and filial generations, were negatively and progressively affected by the LC5 and LC15 concentration of the spirotetramat insecticide. Similar adverse effects were also reported when sublethal concentrations of flupyradifurone were exposed in the F0 generation of M. persicae (Tang et al., 2019) and F1 generation of cotton aphid, A. gossypii (Liang et al., 2019). (Qu et al., 2015) reported that the fecundity and longevity of apterous aphid A. gossypii was expressively lowered after exposure to sublethal concentration of imidacloprid. Furthermore, the progeny of apterous female adults of A. gossypii was reduced when exposed to cycloxaprid and nitenpyram (Wang et al., 2017; Yuan et al., 2017; Cui et al., 2018). Sulfoxaflor also reduced the fecundity in the F0 generation of Sogatella frucifera (Xiang et al., 2019) and Nilaparvata lugens (Liao et al., 2019). Moreover, negative effects such as fecundity of Apolygus lucorum and longevity of Bemisia tabaci were decreased drastically when exposed to low or sublethal concentrations of cycloxaprid and buprofezin (Sohrabi et al., 2011; Pan et al., 2014). All information thoroughly described the negative impact of insecticide concentrations (low or sublethal), which most probably occurred in the field after the degradation of insecticide over time (Desneux et al., 2005; Desneux et al., 2007; Hafeez et al., 2021b). This mechanism might be linked with the vigor coordination in insects after exposure to insecticides and additional drive has been subjugated by insects to manage the insecticide compression, resulting in a shortage of energy for productivity. Also, the diminution trend in fecundity and longevity specified a dearth of hormetic effects, which develops an imperative sublethal outcome of insecticides (Liang et al., 2019). Hormesis can be explained as a dose-response association categorizing over the reverse of the reaction between low and high-stress doses (Jager et al., 2013; Guedes and Cutler, 2014). In the previous study, hormesis has been observed in various insect species and insecticides, likely the higher fecundity in M. persicae exposed to imidacloprid (Ayyanath et al., 2013) and the outbursts in Oligonchus ilicis tempted by pyrethroid (Cordeiro et al., 2013). Sublethal and transgenerational effects of spirotetramat affected developmental physiology, indicating the role of sublethal concentrations in larval growth, development, and sensitivity to spirotetramat. In previous studies, Physiological and biochemical studies have shown that P450 enzymes are vital to in insect hormone metabolism pathways but details of the molecular processes remain unknown (Iga and Kataoka, 2012; Hafeez et al., 2022). The growth and developmental physiology of B. brassicae was hindered after being treated with sublethal concentrations of spirotetramat, but how these sublethal concentrations regulate this process requires further study.
In the current study, the evaluation of transgenerational effects in the filial F1 cohort of B. brassicae, imitated that the exposure to LC5 and LC15 of spirotetramat in the parent generation (F0) inclined the F1 generation population growth, particularly through an amplified preadult developmental length and total pre-reproductive period (TPRP). Comparable outcomes originated in A. gossypii when treated with methyl benzoate, thiamethoxam, and flonicamid (Mostafiz et al., 2020; Ullah et al., 2020; Shi et al., 2022). In previous studies, sublethal effects of thiamethoxam insecticides have also been reported on the population development of Bradysia odorriphaga (Zhang et al., 2014) and Hippodamia variegate (Rahmani and Bandani, 2013). A similar trend of sublethal effects of imidacloprid was described on B. tabaci (He et al., 2013a), A. lucorum (Tan et al., 2012), M. persicae and B. brassicae (Lashkari et al., 2007; Wang et al., 2008). Some neonicotinoid insecticides (such as nitenpyram, clothianidin, acetamiprid and thiacloprid) can lead to substantial adverse effects on the biological traits of A. gossypii (Shi et al., 2011). (Lashkari et al., 2007) stated that treatment with imidacloprid lowered the average cohort time in B. brassicae. In B. tabaci, a low dose of imidacloprid did not disturb the biological and population growth factors but extended the mean generation time (Sohrabi et al., 2011). All these conclusions predicted that the effects of insecticide can vary intensely reliant on several aspects e.g., the amount of insecticide used, insecticide class, the insect species, the definite application circumstances, and the physiological state of the targeted organism (Shi et al., 2011; Han et al., 2012; Zhang et al., 2012; Guo et al., 2013; Xiao et al., 2015; Haddi et al., 2016).
The other parameters including sxj, lx and vxj were to clarify the conflicting effects of insecticides on population growth and the development of various insect pests (He et al., 2013b; Iftikhar et al., 2020; Hafeez et al., 2021a). Because lx is an elementary form of sxj, the lx curve of the insecticide-exposed cluster could only exemplify that spirotetramat reserved the survival rate in the immature stages (Liang et al., 2019). (Chen et al., 2016) reported that a higher survival rate after age 33 days in the flupyradifurone-treated aphids exhibited a thinkable concealed hormesis. In our study, we demonstrated that hormesis is not a key factor in terms of effects of spirotetramat on B. brassicae. In addition to this, the decrease in mx and lxmx curves of insecticide-treated groups reflected that the productiveness of the filial F1 generation is affected by insecticide (Tang et al., 2015; Liang et al., 2019). However, considering all biological processes at play when arthropods are exposed to pesticides, arthropods may develop, ultimately, hormesis and/or resistance responses to such chemicals (Kendig et al., 2010; Liang et al., 2012). In our experiment assessing the transgenerational effects in the F1 generation of B. brassicae, we showed that the exposure to the LC5 and LC15 of spirotetramat in the parent generation (F0) significantly affected the F1 generation population growth, notably through an increased duration of the preadult stage, of TPOP, and of the mean generation time (T). These effects translate to a lower intrinsic rate of increase (ri), finite rate of increase (λ), net reproductive rate (R0). Such effects on population growth have been reported when treated with lethal and sublethal concentrations of various insecticides such as also in A. gossypii (Chen et al., 2016), M. persicae (Tang et al., 2015) H. variegata (Goeze) (Rahmani and Bandani, 2013) and Plutella xylostella (Guo et al., 2013). In general, the life table parameters estimated in this study are somewhat similar to the published data on different insect pests including aphids (Chen et al., 2016; Ullah et al., 2019; Hafeez et al., 2021a). The results of the current study suggest that sublethal concentrations of spirotetramat reduced the productiveness of the parent generation (F0) of B. brassicae and had a transgenerational effect on the descendants by extending the preadult developmental length, reducing the survival rate of undeveloped phases and also overwhelming the fecundity of F1 generation.
Conclusion
Sublethal concentrations can interfere with the growth and overwhelm the population growth of the B. brassicae offspring. In practice, the results of the present study (under laboratory conditions) stressed the importance of assessing sublethal effects of the pesticide on this B. brassicae and also assessing how these effects may translate to the population level in the field. Therefore, further studies using various low-lethal and sublethal concentrations may be needed to provide a more comprehensive evaluation of putative hormesis responses to spirotetramat in B. brassicae. Our study hinted at the need to study further possible effects of spirotetramat on B. brassicae, in the aim to develop optimized IPM packages including this new pesticide (Table 4).
Data availability statement
The original contributions presented in the study are included in the article/supplementary material, further inquiries can be directed to the corresponding authors.
Author contributions
AI, FH, and MAA contributed to conception and design of the study. MH organized the database. AI and MH performed the statistical analysis. AI, AN, HKY, and MJS wrote the first draft of the manuscript. AI, SH, QA, MR, AS, RAM, YSM, KMS, and FAS wrote sections of the manuscript. All authors contributed to manuscript revision, read, and approved the submitted version.
Funding
The authors extend their appreciation to the Deanship of Scientific Research at King Khalid University, Abha, KSA for funding this work through General Research Project under grant number (G.R.P/23/43).
Conflict of interest
The authors declare that the research was conducted in the absence of any commercial or financial relationships that could be construed as a potential conflict of interest.
Publisher’s note
All claims expressed in this article are solely those of the authors and do not necessarily represent those of their affiliated organizations, or those of the publisher, the editors and the reviewers. Any product that may be evaluated in this article, or claim that may be made by its manufacturer, is not guaranteed or endorsed by the publisher.
References
Abbas, Q., Hussain, M., Hasnain, M., Akram, M., Raza, A., and Shahid, M. R. (2017). Population distribution and species richness of canola aphids and their natural enemies in different areas of Punjab, Pakistan. J. Entomol. Zool. Stud. 5, 1302–1308.
Ahmad, M., and Akhtar, S. (2013). Development of insecticide resistance in field populations of Brevicoryne brassicae (Hemiptera: Aphididae) in Pakistan. J. Econ. Entomol. 106, 954–958. doi:10.1603/EC12233
Ahmed, M., Qin, P., Ji, M., An, R., Guo, H., and Shafi, J. (2020). Spinasterol, 22, 23-dihydrospinasterol and fernenol from Citrullus Colocynthis L. With aphicidal activity against cabbage aphid Brevicoryne Brassicae L. Molecules 25, 2184. doi:10.3390/molecules25092184
Akköprü, E. P., Atlihan, R., Okut, H., and Chi, H. (2015). Demographic assessment of plant cultivar resistance to insect pests: A case study of the dusky-veined walnut aphid (Hemiptera: Callaphididae) on five walnut cultivars. J. Econ. Entomol. 108, 378–387. doi:10.1093/jee/tov011
Anzabi, S. H. M., Eivazi, A., Zargaran, M. R., and Gasemi-Kahrizeh, A. (2014). Effect of seven canola genotypes on cabbage aphid Brevicoryne brassicae growth parameters. Rom. Agric. Res. 13, 75–80.
Arrese, E. L., Canavoso, L. E., Jouni, Z. E., Pennington, J. E., Tsuchida, K., and Wells, M. A. (2001). Lipid storage and mobilization in insects: Current status and future directions. Insect biochem. Mol. Biol. 31, 7–17. doi:10.1016/S0965-1748(00)00102-8
Ayyanath, M. M., Cutler, G. C., Scott-Dupree, C. D., and Sibley, P. K. (2013). Transgenerational shifts in reproduction hormesis in green peach aphid exposed to low concentrations of imidacloprid. PLoS One 8, e74532. doi:10.1371/journal.pone.0074532
Babcock, J. M., Gerwick, C. B., Huang, J. X., Loso, M. R., Nakamura, G., Nolting, S. P., et al. (2011). Biological characterization of sulfoxaflor, a novel insecticide. Pest Manag. Sci. 67, 328–334. doi:10.1002/ps.2069
Bashir, F., and Azim, M. N. (2013). Effects of host plants on the biology of cabbage aphid, Brevicoryne brassicae (L.). J. Entomol. Res. 37, 83–85.
Brück, E., Elbert, A., Fischer, R., Krueger, S., Kühnhold, J., Klueken, A. M., et al. (2009). Movento®, an innovative ambimobile insecticide for sucking insect pest control in agriculture: Biological profile and field performance. Crop Prot. 28, 838–844. doi:10.1016/j.cropro.2009.06.015
Chen, X., Ma, K., Li, F., Liang, P., Liu, Y., Guo, T., et al. (2016). Sublethal and transgenerational effects of sulfoxaflor on the biological traits of the cotton aphid, Aphis gossypii Glover (Hemiptera: Aphididae). Ecotoxicology 25, 1841–1848. doi:10.1007/s10646-016-1732-9
Chi, H. (2022). TWOSEX-MSChart: A computer program for the age-stage, two-sex life table analysis. Taichung, Taiwan: National Chung Hsing University. Available at: http://140.120.197.173/Ecology/Download/Twosex-MsChart.rar.
Chi, H. (1988). Life-table Analysis incorporating both sexes and variable development rates among individuals. Environ. Entomol. 17, 26–34. doi:10.1093/ee/17.1.26
Chi, H., and Liu, H. (1985). Two new methods for the study of insect population ecology. Bull. Inst. Zool. Acad. Sin. 24, 225–240.
Chi, H., and Su, H. Y. (2006). Age-stage, two-sex life tables of Aphidius gifuensis (Ashmead) (Hymenoptera: Braconidae) and its host Myzus persicae (Sulzer) (Homoptera: Aphididae) with mathematical proof of the relationship between female fecundity and the net reproductive rate. Environ. Entomol. 35, 10–21. doi:10.1603/0046-225X-35.1.10
Cordeiro, E. M. G., de Moura, I. L. T., Fadini, M. A. M., and Guedes, R. N. C. (2013). Beyond selectivity: Are behavioral avoidance and hormesis likely causes of pyrethroid-induced outbreaks of the southern red mite oligonychus ilicis? Chemosphere 93, 1111–1116. doi:10.1016/j.chemosphere.2013.06.030
Cui, L., Yuan, H., Wang, Q., Wang, Q., and Rui, C. (2018). Sublethal effects of the novel cis-nitromethylene neonicotinoid cycloxaprid on the cotton aphid Aphis gossypii Glover (Hemiptera: Aphididae). Sci. Rep. 8, 8915–8919. doi:10.1038/s41598-018-27035-7
Cutler, G. C., Ramanaidu, K., Astatkie, T., and Isman, M. B. (2009). Green peach aphid, Myzus persicae (Hemiptera: Aphididae), reproduction during exposure to sublethal concentrations of imidacloprid and azadirachtin. Pest Manag. Sci. 65, 205–209. doi:10.1002/ps.1669
Dáder, B., Then, C., Berthelot, E., Ducousso, M., Ng, J. C. K., and Drucker, M. (2017). Insect transmission of plant viruses: Multilayered interactions optimize viral propagation. Insect Sci. 24, 929–946. doi:10.1111/1744-7917.12470
Desneux, N., Decourtye, A., and Delpuech, J.-M. (2006). The sublethal effects of pesticides on beneficial arthropods. Annu. Rev. Entomol. 52, 81–106. doi:10.1146/annurev.ento.52.110405.091440
Desneux, N., Decourtye, A., and Delpuech, J.-M. (2007). The sublethal effects of pesticides on beneficial arthropods. Annu. Rev. Entomol. 52, 81–106. doi:10.1146/annurev.ento.52.110405.091440
Desneux, N., Fauvergue, X., Dechaume-Moncharmont, F. X., Kerhoas, L., Ballanger, Y., and Kaiser, L. (2005). Diaeretiella rapae limits Myzus persicae populations after applications of deltamethrin in oilseed rape. J. Econ. Entomol. 98, 9–17. doi:10.1093/jee/98.1.9
Döker, İ., Kazak, C., and Ay, R. (2021). Resistance status and detoxification enzyme activity in ten populations of Panonychus citri (Acari: Tetranychidae) from Turkey. Crop Prot. 141, 105488–105497. doi:10.1016/j.cropro.2020.105488
Drobnjaković, T., and Marčić, D. (2021). Effects of spirotetramat insecticide on life history traits and population growth of Encarsia formosa (Hymenoptera: Aphelinidae). Biocontrol Sci. Technol. 31, 604–618. doi:10.1080/09583157.2021.1873248
Efron, B., and Tibshirani, R. J. (1993). “An introduction to the bootstrap,” in Monographs on statistics and applied probability, No. 57 (London: Chapman & Hall), 436. doi:10.1111/1467-9639.00050
Gong, Y., Shi, X., Desneux, N., and Gao, X. (2016a). Effects of spirotetramat treatments on fecundity and carboxylesterase expression of Aphis gossypii Glover. Ecotoxicology 25, 655–663. doi:10.1007/s10646-016-1624-z
Gong, Y., Shi, X., Desneux, N., and Gao, X. (2016b). Effects of spirotetramat treatments on fecundity and carboxylesterase expression of Aphis gossypii Glover. Ecotoxicology 52, 655–663. doi:10.1007/s10646-016-1624-z
Guedes, R. N. C., and Cutler, G. C. (2014). Insecticide-induced hormesis and arthropod pest management. Pest Manag. Sci. 70, 690–697. doi:10.1002/ps.3669
Guedes, R. N. C., Smagghe, G., Stark, J. D., and Desneux, N. (2016). Pesticide-Induced stress in arthropod pests for optimized integrated pest management programs. Annu. Rev. Entomol. 61, 43–62. doi:10.1146/annurev-ento-010715-023646
Guo, L., Desneux, N., Sonoda, S., Liang, P., Han, P., and Gao, X. W. (2013). Sublethal and transgenerational effects of chlorantraniliprole on biological traits of the diamondback moth, Plutella xylostella L. Plutella xylostella L. Crop Prot. 48, 29–34. doi:10.1016/j.cropro.2013.02.009
Haddi, K., Mendes, M. V., Barcellos, M. S., Lino-Neto, J., Freitas, H. L., Guedes, R. N. C., et al. (2016). Sexual success after stress? Imidacloprid-induced hormesis in males of the neotropical stink bug euschistus heros. PLoS One 11, e0156616. doi:10.1371/journal.pone.0156616
Hafeez, M., Li, X., Yousaf, H. K., Khan, M. M., Imran, M., Zhang, Z., et al. (2021a). Sublethal effects of bistrifluron on key biological traits, macronutrients contents and vitellogenin (SeVg) expression in Spodoptera exigua (Hübner). Pestic. Biochem. Physiol. 174, 104802. doi:10.1016/j.pestbp.2021.104802
Hafeez, M., Ullah, F., Khan, M. M., Li, X., Zhang, Z., Shah, S., et al. (2021b). Metabolic-based insecticide resistance mechanism and ecofriendly approaches for controlling of beet armyworm Spodoptera exigua: A review. Environ. Sci. Pollut. Res. Int. 29, 1746–1762. doi:10.1007/s11356-021-16974-w
Hafeez, M., Li, X., Ullah, F., Zhang, Z., Zhang, J., Huang, J., et al. (2022). Down-regulation of P450 genes enhances susceptibility to indoxacarb and alters physiology and development of fall armyworm, Spodoptera frugipreda (Lepidoptera: Noctuidae). Front. Physiol. 13, 884447–884465. doi:10.3389/fphys.2022.884447
Han, W., Zhang, S., Shen, F., Liu, M., Ren, C., and Gao, X. (2012). Residual toxicity and sublethal effects of chlorantraniliprole on Plutella xylostella (Lepidoptera: Plutellidae). Pest Manag. Sci. 68, 1184–1190. doi:10.1002/ps.3282
He, Y., Zhao, J., Zheng, Y., Weng, Q., Biondi, A., Desneux, N., et al. (2013a). Assessment of potential sublethal effects of various insecticides on key biological traits of the tobacco whitefly, Bemisia tabaci. Int. J. Biol. Sci. 9, 246–255. doi:10.7150/ijbs.5762
He, Y., Zhao, J., Zheng, Y., Weng, Q., Biondi, A., Desneux, N., et al. (2013b). Assessment of potential sublethal effects of various insecticides on key biological traits of the tobacco whitefly, Bemisia tabaci. Int. J. Biol. Sci. 9, 246–255. doi:10.7150/ijbs.5762
Hercus, M. J., and Hoffmann, A. A. (2000). Maternal and grandmaternal age influence offspring fitness in Drosophila. Proc. Biol. Sci. 267, 2105–2110. doi:10.1098/rspb.2000.1256
Iftikhar, A., Hafeez, F., Hafeez, M., Farooq, M., Asif Aziz, M., Sohaib, M., et al. (2020). Sublethal effects of a juvenile hormone analog, Pyriproxyfen on demographic parameters of non-target predator, Hippodamia convergens Guerin-Meneville (Coleoptera: Coccinellidae). Ecotoxicology 29, 1017–1028. doi:10.1007/s10646-020-02159-7
Iga, M., and Kataoka, H. (2012). Recent studies on insect hormone metabolic pathways mediated by cytochrome P450 enzymes. Biol. Pharm. Bull. 35, 838–843. doi:10.1248/bpb.35.838
Jager, T., Barsi, A., and Ducrot, V. (2013). Hormesis on life-history traits: Is there such thing as a free lunch? Ecotoxicology 22, 263–270. doi:10.1007/s10646-012-1022-0
Jie, M., Gao, Y., Kuang, D., Shi, Y., Wang, H., and Jing, W. (2021). Relationship between imidacloprid residues and control effect on cotton aphids in arid region. Environ. Geochem. Health 43, 1941–1952. doi:10.1007/s10653-020-00776-z
Kendig, E. L., Le, H. H., and Belcher, S. M. (2010). Defining Hormesis: Evaluation of a complex concentration response phenomenon. Int. J. Toxicol. 29 (3), 235–246. doi:10.1177/1091581810363012
Lashkari, M. R., Sahragard, A., and Ghadamyari, M. (2007). Sublethal effects of imidacloprid and pymetrozine on population growth parameters of cabbage aphid, Brevicoryne brassicae on rapeseed, Brassica napus L. Insect Sci. 14, 207–212. doi:10.1111/j.1744-7917.2007.00145.x
Liang, P., Tian, Y. A., Biondi, A., Desneux, N., and Gao, X.-W. (2012). Short-term and transgenerational effects of the neonicotinoid nitenpyram on susceptibility to insecticides in two whitefly species. Ecotoxicol. 21, 1889–1898. doi:10.1007/s10646-012-0922-3
Liang, P. Z., Ma, K. S., Chen, X. W., Tang, C. Y., Xia, J., Chi, H., et al. (2019). Toxicity and sublethal effects of flupyradifurone, a novel butenolide insecticide, on the development and fecundity of Aphis gossypii (Hemiptera: Aphididae). J. Econ. Entomol. 112, 852–858. doi:10.1093/jee/toy381
Liao, X., Ali, E., Li, W., He, B., Gong, P., Xu, P., et al. (2019). Sublethal effects of sulfoxaflor on the development and reproduction of the Brown planthopper, Nilaparvata lugens (Stål). Crop Prot. 118, 6–14. doi:10.1016/j.cropro.2018.12.005
Lu, Y., Wu, K., Jiang, Y., Guo, Y., and Desneux, N. (2012). Widespread adoption of Bt cotton and insecticide decrease promotes biocontrol services. Nature 487, 362–365. doi:10.1038/nature11153
Lv, N., Ma, K., Li, R., Liang, P., Liang, P., and Gao, X. (2021). Sublethal and lethal effects of the imidacloprid on the metabolic characteristics based on high-throughput non-targeted metabolomics in Aphis gossypii Glover. Ecotoxicol. Environ. Saf. 212, 111969. doi:10.1016/j.ecoenv.2021.111969
Mahmoodi, L., Mehrkhou, F., Guz, N., Forouzan, M., and Atlihan, R. (2020). Sublethal effects of three insecticides on fitness parameters and population projection of Brevicoryne brassicae (Hemiptera: Aphididae). J. Econ. Entomol. 113, 2713–2722. doi:10.1093/jee/toaa193
Marcic, D., Petronijevic, S., Drobnjakovic, T., Prijovic, M., Peric, P., and Milenkovic, S. (2012). The effects of spirotetramat on life history traits and population growth of Tetranychus urticae (Acari: Tetranychidae). Exp. Appl. Acarol. 56, 113–122. doi:10.1007/s10493-011-9500-2
Moores, G. D., Gao, X., Denholm, I., and Devonshire, A. L. (1996). Characterisation of insensitive acetylcholinesterase in insecticide-resistant cotton aphids, Aphis gossypii Glover (Homoptera: Aphididae). Pestic. Biochem. Physiol. 56, 102–110. doi:10.1006/pest.1996.0064
Mostafiz, M. M., Alam, M. B., Chi, H., Hassan, E., Shim, J. K., and Lee, K. Y. (2020). Effects of sublethal doses of methyl benzoate on the life history traits and acetylcholinesterase (AChE) activity of Aphis gossypii. Agron. (Basel). 10, 1313. doi:10.3390/agronomy10091313
Ouyang, Y., Montez, G. H., Liu, L., and Grafton-Cardwell, E. E. (2012). Spirodiclofen and spirotetramat bioassays for monitoring resistance in citrus red mite, Panonychus citri (Acari: Tetranychidae). Pest Manag. Sci. 68, 781–787. doi:10.1002/ps.2326
Pan, H., Liu, Y., Liu, B., Lu, Y., Xu, X., Qian, X., et al. (2014). Lethal and sublethal effects of cycloxaprid, a novel cis-nitromethylene neonicotinoid insecticide, on the mirid bug Apolygus lucorum. J. Pest Sci. (2004) 87, 731–738. doi:10.1007/s10340-014-0610-6
Planes, L., Catalán, J., Tena, A., Porcuna, J. L., Jacas, J. A., Izquierdo, J., et al. (2013). Lethal and sublethal effects of spirotetramat on the mealybug destroyer, Cryptolaemus montrouzieri. J. Pest Sci. (2004) 86, 321–327. doi:10.1007/s10340-012-0440-3
Qu, Y., Xiao, D., Li, J., Chen, Z., Biondi, A., Desneux, N., et al. (2015). Sublethal and hormesis effects of imidacloprid on the soybean aphid Aphis glycines. Ecotoxicology 24, 479–487. doi:10.1007/s10646-014-1396-2
Rahmani, S., and Bandani, A. R. (2013). Sublethal concentrations of thiamethoxam adversely affect life table parameters of the aphid predator, Hippodamia variegata (Goeze) (Coleoptera: Coccinellidae). Crop Prot. 54, 168–175. doi:10.1016/j.cropro.2013.08.002
Roh, H. S., Kim, J., Shin, E. S., Lee, D. W., Choo, H. Y., and Park, C. G. (2015). Bioactivity of sandalwood oil (Santalum austrocaledonicum) and its main components against the cotton aphid, Aphis gossypii. J. Pest Sci. (2004) 88, 621–627. doi:10.1007/s10340-014-0631-1
Salazar-López, N.-J., Aldana-Madrid, M.-L., Silveira-Gramont, M.-I., and Aguiar, J.-L. (2016). Spirotetramat — an alternative for the control of parasitic sucking insects and its fate in the environment. Insectic. Resist, 41–54. doi:10.5772/61322
Sarhozaki, M. T., and Safavi, S. A. (2014). Sub-lethal effects of tiametoxam on life table parameters of the cabbage aphid, Brevicoryne brassicae (L.) (Hemiptera: Aphididae) under laboratory conditions. Archives Phytopathology Plant Prot. 47, 508–515. doi:10.1080/03235408.2013.813145
Saska, P., Skuhrovec, J., Lukáš, J., Chi, H., Tuan, S. J., and Honek, A. (2016). Treatment by glyphosate-based herbicide alters life history parameters of the rose-grain aphid Metopolophium dirhodum. Sci. Rep. 6, 27801. doi:10.1038/srep27801
Shang, Q., Pan, Y., Fang, K., Xi, J., and Brennan, J. A. (2012). Biochemical characterization of acetylcholinesterase, cytochrome P450 and cross-resistance in an omethoate-resistant strain of Aphis gossypii Glover. Crop Prot. 31, 15–20. doi:10.1016/j.cropro.2011.09.014
Shi, X., Jiang, L., Wang, H., Qiao, K., Wang, D., and Wang, K. (2011). Toxicities and sublethal effects of seven neonicotinoid insecticides on survival, growth and reproduction of imidacloprid-resistant cotton aphid, Aphis gossypii. Pest Manag. Sci. 67, 1528–1533. doi:10.1002/ps.2207
Shi, D., Luo, C., Lv, H., Zhang, L., Desneux, N., You, H., et al. (2022). Impact of sublethal and low lethal concentrations of flonicamid on key biological traits and population growth associated genes in melon aphid, Aphis gossypii Glover. Crop Prot. 152, 105863. doi:10.1016/j.cropro.2021.105863
Shikano, I. (2017). Evolutionary ecology of multitrophic interactions between plants, insect herbivores and entomopathogens. J. Chem. Ecol. 43, 586–598. doi:10.1007/s10886-017-0850-z
Shonga, E., and Getu, E. (2021a). Efficacy of plant derived and synthetic insecticides against cabbage aphid, Brevicoryne brassicae (L.) (Homoptera: Aphididae) and their effect on coccinellid predators. SINET Ethiop. J. Sci. 44, 27–37. doi:10.4314/sinet.v44i1.3
Shonga, E., and Getu, E. (2021b). Population dynamics of cabbage aphid, Brevicoryne brassicae L. (Homoptera: Aphididae) in relation to weather factors on major brassica crops in central rift valley of Ethiopia: Baseline studies for the management of the pest. Int. J. Trop. Insect Sci. 41, 455–462. doi:10.1007/s42690-020-00226-4
Sohrabi, F., Shishehbor, P., Saber, M., and Mosaddegh, M. S. (2011). Lethal and sublethal effects of buprofezin and imidacloprid on Bemisia tabaci (Hemiptera: Aleyrodidae). Crop Prot. 30, 1190–1195. doi:10.1016/j.cropro.2011.05.004
Sohrabi, F., Shishehbor, P., Saber, M., and Mosaddegh, M. S. (2013). Lethal and sublethal effects of imidacloprid and buprofezin on the sweetpotato whitefly parasitoid Eretmocerus mundus (Hymenoptera: Aphelinidae). Crop Prot. 45, 98–103. doi:10.1016/j.cropro.2012.11.024
Stark, J. D., and Banks, J. E. (2003). Population-level effects of pesticides and other toxicants on arthropods. Annu. Rev. Entomol. 48, 505–519. doi:10.1146/annurev.ento.48.091801.112621
Tan, Y., Biondi, A., Desneux, N., and Gao, X. W. (2012). Assessment of physiological sublethal effects of imidacloprid on the mirid bug Apolygus lucorum (Meyer-Dür). Ecotoxicology 21, 1989–1997. doi:10.1007/s10646-012-0933-0
Tang, Q., Xiang, M., Hu, H., An, C., and Gao, X. (2015). Evaluation of sublethal effects of sulfoxaflor on the green peach aphid (Hemiptera: Aphididae) using life table parameters. J. Econ. Entomol. 108, 2720–2728. doi:10.1093/jee/tov221
Tang, Q., Ma, K., Chi, H., Hou, Y., and Gao, X. (2019). Transgenerational hormetic effects of sublethal dose of flupyradifurone on the green peach aphid, Myzus persicae (Sulzer) (Hemiptera: Aphididae). PLoS One 14, e0208058. doi:10.1371/journal.pone.0208058
Tuan, S. J., Lee, C. C., and Chi, H. (2014a). Erratum to Population and damage projection of Spodoptera litura (F.) on peanuts (Arachis hypogaea L.) under different conditions using the age-stage, two-sex life table Pest Manag Science, 70 (2014), 805-813, 10.1002/ps.3618]. Pest Manag. Sci. 70, 805–813. doi:10.1002/ps.3618
Tuan, S. J., Lee, C. C., and Chi, H. (2014b). Population and damage projection of Spodoptera litura (F.) on peanuts (Arachishypogaea L.) under different conditions using the age-stage, two-sex life table. Pest Manag. Sci. 70, 805–813. doi:10.1002/ps.3618
Ullah, F., Gul, H., Desneux, N., Gao, X., and Song, D. (2019). Imidacloprid-induced hormesis effects on demographic traits of the melon aphid, aphis gossypii. Entomol. Gen. 39, 325–337. doi:10.1127/entomologia/2019/0892
Ullah, F., Gul, H., Tariq, K., Desneux, N., Gao, X., and Song, D. (2020). Functional analysis of cytochrome P450 genes linked with acetamiprid resistance in melon aphid, Aphis gossypii. Pestic. Biochem. Physiol. 170, 104687. doi:10.1016/j.pestbp.2020.104687
Wang, X. Y., Yang, Z. Q., Shen, Z. R., Lu, J., and Xu, W. B. (2008). Sublethal effects of selected insecticides on fecundity and wing dimorphism of green peach aphid (Hom., Aphididae). J. Appl. Entomol. 132, 135–142. doi:10.1111/j.1439-0418.2007.01225.x
Wang, Z. H., Gong, Y. J., Jin, G. H., Zhu, L., and Wei, S. J. (2016). Effects of spirotetramat on development and reproduction of Myzus persicae (Hemiptera: Aphididae). Aust. Entomol. 55, 235–241. doi:10.1111/aen.12174
Wang, S. Y., Qi, Y. F., Desneux, N., Shi, X. Y., Biondi, A., and Gao, X. W. (2017). Sublethal and transgenerational effects of short-term and chronic exposures to the neonicotinoid nitenpyram on the cotton aphid Aphis gossypii. J. Pest Sci. (2004) 90, 389–396. doi:10.1007/s10340-016-0770-7
Xiang, X., Liu, S., Wang, X., Zhang, Y., Gong, C., Chen, L., et al. (2019). Sublethal effects of sulfoxaflor on population projection and development of the white-backed planthopper, Sogatella furcifera (Hemiptera: Delphacidae). Crop Prot. 120, 97–102. doi:10.1016/j.cropro.2019.02.016
Xiao, D., Yang, T., Desneux, N., Han, P., and Gao, X. (2015). Assessment of sublethal and transgenerational effects of pirimicarb on the wheat aphids Rhopalosiphum padi and Sitobion avenae. PLoS One 10, e0128936. doi:10.1371/journal.pone.0128936
Yousaf, H. K., Shan, T., Chen, X., Ma, K., Shi, X., Desneux, N., et al. (2018). Impact of the secondary plant metabolite Cucurbitacin B on the demographical traits of the melon aphid, Aphis gossypii. Sci. Rep. 8, 16473. doi:10.1038/s41598-018-34821-w
Yuan, H. B., Li, J. H., Liu, Y. Q., Cui, L., Lu, Y. H., Xu, X. Y., et al. (2017). Lethal, sublethal and transgenerational effects of the novel chiral neonicotinoid pesticide cycloxaprid on demographic and behavioral traits of Aphis gossypii (Hemiptera: Aphididae). Insect Sci. 24, 743–752. doi:10.1111/1744-7917.12357
Zhang, Z., Li, J. H., and Gao, X. W. (2012). Sublethal effects of metaflumizone on Plutella xylostella (Lepidoptera: Plutellidae). J. Integr. Agric. 11, 1145–1150. doi:10.1016/S2095-3119(12)60108-7
Zhang, P., Liu, F., Mu, W., Wang, Q., Li, H., and Chen, C. (2014). Life table study of the effects of sublethal concentrations of thiamethoxam on Bradysia odoriphaga Yang and Zhang. Pestic. Biochem. Physiol. 111, 31–37. doi:10.1016/j.pestbp.2014.04.003
Keywords: cabbage aphid, population growth, spirotetramat, sublethal concentrations, transgenerational effects
Citation: Iftikhar A, Hafeez F, Aziz MA, Hashim M, Naeem A, Yousaf HK, Saleem MJ, Hussain S, Hafeez M, Ali Q, Rehman M, Akhtar S, Marc RA, Syaad KMA, Mostafa YS and Saeed FAA (2022) Assessment of sublethal and transgenerational effects of spirotetramat, on population growth of cabbage aphid, Brevicoryne brassicae L. (Hemiptera: Aphididae). Front. Physiol. 13:1014190. doi: 10.3389/fphys.2022.1014190
Received: 08 August 2022; Accepted: 09 November 2022;
Published: 08 December 2022.
Edited by:
Xun Zhu, Institute of Plant Protection, Chinese Academy of Agricultural Sciences, ChinaCopyright © 2022 Iftikhar, Hafeez, Aziz, Hashim, Naeem, Yousaf, Saleem, Hussain, Hafeez, Ali, Rehman, Akhtar, Marc, Syaad, Mostafa and Saeed. This is an open-access article distributed under the terms of the Creative Commons Attribution License (CC BY). The use, distribution or reproduction in other forums is permitted, provided the original author(s) and the copyright owner(s) are credited and that the original publication in this journal is cited, in accordance with accepted academic practice. No use, distribution or reproduction is permitted which does not comply with these terms.
*Correspondence: Ayesha Iftikhar, aaishaiftkhr@yahoo.com; Muhammad Hafeez, 0621598@zju.edu.cn