- Laboratorio de Reproducción y Fisiología Materno-Embrionaria, Instituto de Biodiversidad y Biología Experimental y Aplicada (IBBEA), Consejo Nacional de Investigaciones Científicas y Tecnológicas (CONICET), Departamento de Biodiversidad y Biología Experimental (DBBE), Facultad de Ciencias Exactas y Naturales, Universidad de Buenos Aires, Buenos Aires, Argentina
Adequate placentation, placental tissue remodeling and vascularization is essential for the success of gestation and optimal fetal growth. Recently, it was suggested that abnormal placenta induced by maternal alcohol consumption may participate in fetal growth restriction and relevant clinical manifestations of the Fetal Alcohol Spectrum Disorders (FASD). Particularly, periconceptional alcohol consumption up to early gestation can alter placentation and angiogenesis that persists in pregnancy beyond the exposure period. Experimental evidence suggests that abnormal placenta following maternal alcohol intake is associated with insufficient vascularization and defective trophoblast development, growth and function in early gestation. Accumulated data indicate that impaired vascular endothelial growth factor (VEGF) system, including their downstream effectors, the nitric oxide (NO) and metalloproteinases (MMPs), is a pivotal spatio-temporal altered mechanism underlying the early placental vascular alterations induced by maternal alcohol consumption. In this review we propose that the periconceptional alcohol intake up to early organogenesis (first trimester) alters the VEGF-NO-MMPs system in trophoblastic-decidual tissues, generating imbalances in the trophoblastic proliferation/apoptosis, insufficient trophoblastic development, differentiation and migration, deficient labyrinthine vascularization, and uncompleted remodelation and transformation of decidual spiral arterioles. Consequently, abnormal placenta with insufficiency blood perfusion, vasoconstriction and reduced labyrinthine blood exchange can be generated. Herein, we review emerging knowledge of abnormal placenta linked to pregnancy complications and FASD produced by gestational alcohol ingestion and provide evidence of the early abnormal placental angiogenesis-vascularization and growth associated to decidual-trophoblastic dysregulation of VEGF system after periconceptional alcohol consumption up to mid-gestation, in a mouse model.
Introduction
Normal growth and survival of the fetus depends on the adequate placentation. Besides providing sufficient amounts of nutrients and oxygen, the placenta establishes a privileged immune environment for fetal growth by orchestrating maternal adaptations to pregnancy and acting as a selective and protective barrier to prevent feto-maternal diseases (Creeth and John, 2020). Poor placentation and placental failure compromises fetal development leading to potential chronic diseases in adult (Sharma et al., 2016; Perez-Garcia et al., 2018; Woods et al., 2018). Experimental studies suggest that prenatal alcohol exposure disrupts the placenta (Carter et al., 2016), which may play a crucial etiologic role in alcohol-related fetal effects throughout pregnancy (Hoyme et al., 2016).
Maternal alcohol consumption can lead to the irreversible condition of fetal alcohol syndrome (FAS), the most severe form of the alcohol spectrum disorders (FASDs) (Guerri et al., 2009; de Sanctis et al., 2011; May and Gossage, 2011; Memo et al., 2013; Joya et al., 2015; Van Heertum and Rossi, 2017). FASD is characterized by fetal and birth anomalies, intrauterine growth retardation (IUGR), numerous physical, cognitive, and behavioral defects in newborns and children (Hoyme et al., 2016; Roozen et al., 2016). Prevalence of FASD was estimated at 1–30/1,000 live births in the United States (May et al., 2009; Lange et al., 2017; Popova et al., 2017), and of annual pregnancies, about 40% of women drink some alcoholic beverage during pregnancy while 3–5% of women drink heavily throughout pregnancy (Heller and Burd, 2013). In some Latin-American countries like Uruguay and Argentina, the prevalence of heavy alcohol consumption during pregnancy ranges at 40 to 75% (Magri et al., 2007; López, 2013; López et al., 2015). Despite public efforts to reduce prevalence of alcohol consumption, still high proportion of women often continues to drink moderate levels of alcohol (200 ml/day of wine containing ethanol 11%) during the early pregnancy while unaware they are pregnant (4 to 6 weeks after conception during early organogenesis) (Colvin et al., 2007). In this relation, recently we established a mouse model of perigestational moderate alcohol ingestion, previous and up to early gestation, to study the embryo developmental effects compatible with FASD. Perigestational alcohol intake up to organogenesis (equivalent to the first three-four weeks of human pregnancy) induces delayed embryo differentiation and growth, and dysmorphogenesis, by altering molecular pathways, genotoxicity, apoptosis and oxidative stress (OS) (Cebral et al., 2007, 2011; Coll et al., 2011, 2017). However, despite the direct effects of ethanol exposure on embryo-fetal outcomes, placental injury due to maternal alcohol ingestion was recently proposed as an indirect cause of fetal abnormalities and FASD (Gupta et al., 2016). Maternal alcohol-induced dysfunctional placenta was linked to IUGR, congenital defects, adulthood obesity, metabolic syndromes, cardiovascular disease (Davis et al., 2012a,b; Zhu et al., 2015; Linask and Han, 2016) and fetal programming diseases (Bada et al., 2005; Burd et al., 2007; Gundogan et al., 2008, 2015; Patra et al., 2011; Bosco and Diaz, 2012; Davis-Anderson et al., 2017; Tai et al., 2017). Nevertheless, the etiology of abnormal placenta associated to maternal alcohol consumption is proposed to be related to gestational windows of susceptibility: peri-implantation, gastrulation and/or organogenesis (first trimester in human) (Livy et al., 2004). Both early alcohol and acetaldehyde exposure may contribute to the pathogenesis of FASD by reducing placental growth and function on the first trimester (Lui et al., 2014). Particularly, perigestational moderate alcohol consumption up to peri-implantation prevents blastocyst implantation and results in early pregnancy loss (Perez-Tito et al., 2014). Since maternal alcohol exposure can decrease the trophoblast migration/invasion leading to abnormal placental vascularization (Han et al., 2012), the link between placental vasculopathy and failure in the early decidual-trophoblast development and dysregulation of angiogenesis-vascularization after perigestational alcohol ingestion up to organogenesis recently began to be studied in mouse models (Coll et al., 2018; Ventureira et al., 2019; Gualdoni G. S. et al., 2021).
At present different animal models provide insights into the alcohol-induced mechanisms on the placenta. Nevertheless, the impact of perigestational alcohol ingestion up to early gestation on placental angiogenic mechanisms involved in abnormal placenta has not been sufficiently clarified. Herein, we first provide a brief background on the gestational alcohol placental defects as the etiology of FASD, and then we extend the revision to the knowledge of emerging evidences on the effects of periconceptional consumption up to early organogenesis on placental development, highlighting the role of the trophoblast-decidual VEGF system in a mouse model.
Main Effects of Gestational Alcohol Consumption on Placenta
Normal placental vascular development is critical for optimal fetal growth, maintenance and successful pregnancy, and subsequent life course (Adamson et al., 2002; Reynolds et al., 2006). The placenta, the major organ determinant of intrauterine growth, is involved in nutrient transport and metabolism of several molecules (Martín-Estal et al., 2019), and in the synthesis and releases of hormones and other mediators into both maternal and fetal circulations (Guttmacher et al., 2014). Alcohol use throughout gestation can disrupt the normal hormonal interactions between mother and fetus, altering natural homeostasis and hence leading to poor pregnancy outcomes. Gestational alcohol exposure impaires the respiratory gases supply due to poor placental vascularity, leading to hypoxia, thus resulting in pregnancy complications, IUGR, and preeclampsia, malnutrition, or stillbirth (Aliyu et al., 2008, 2011; Salihu et al., 2011; Carter et al., 2016). Ethanol interferes with placental transport of nutrients, oxygen, and waste products (Gundogan et al., 2008, 2015; Kwan et al., 2020). Moreover, prenatal alcohol exposure alters the placental iron transport yielding to fetal iron deficiency anemia, condition that exacerbates alcohol-related growth restriction (Kwan et al., 2020). Also, animal and in vitro studies have suggested that chronic and heavy alcohol use in pregnancy may impair transport of folic acid across the placenta to the fetus by decreasing expression of transport proteins, thus contributing to the deficits observed in FASD (Hutson et al., 2012). In overall, alcohol use throughout pregnancy promotes poor fetal outcomes and relevant clinical manifestations of FASD by inducing abnormal placental morphogenesis and impairment of placental metabolism and hormonal function (Gundogan et al., 2008; Ramadoss and Magness, 2012c; Gupta et al., 2016).
Gestational alcohol intake produces the “alcohol-related placental associated syndrome” (Salihu et al., 2011) that includes miscarriage, hypertension, preeclampsia, preterm birth, placenta previa, placenta accreta and placental hemorrhage (Gundogan et al., 2010; Meyer-Leu et al., 2011; Avalos et al., 2014; Carter et al., 2016; Tai et al., 2017; Ohira et al., 2019; Orzabal et al., 2019; Odendaal et al., 2020). Moreover, high risk of placental abruption was observed after consumption of 7–21 drinks per week (a mean of two drinks per day and BAC of 5–100 mg/dL) (Burd et al., 2007). In human and animal models, alcohol exposure during pregnancy usually reduces placental weight and size, affecting directly the structure and function (Gundogan et al., 2008, 2015; Bosco and Diaz, 2012; Carter et al., 2016; Kwan et al., 2020). The most generalized effects of gestational alcohol intake on the placenta were on its vasculature, which is associated with uteroplacental malperfusion, resistance, and placental and umbilical cord contraction (Iveli et al., 2007). In mouse models, alcohol exposure at mid-gestation leads to severe embryo-placental growth retardation (Haycock and Ramsay, 2009).
Gundogan et al. (2008, 2010) reported, in animal model, that one major placental abnormality due to chronic gestational ethanol exposure is the failure of maternal decidual spiral artery remodeling by which the interaction between the invasive trophoblasts and maternal vessels is impared and leads to altered placental blood flow and nutrient exchange. Moderate or high-dose of ethanol intake during gestation also reduces the labyrinthine development (Gundogan et al., 2008, 2010, 2013, 2015). During third trimester of gestation, alcohol affects the uteroplacental vascular function (Rosenberg et al., 2010; Subramanian et al., 2014; Orzabal et al., 2019) by impairment of uterine spiral artery remodeling, angiogenesis and vasodilation (Radek et al., 2005), via altered endothelial angiogenic gene expression (Ramadoss and Magness, 2012b) and proteome defects (Ramadoss et al., 2011; Ramadoss and Magness, 2012c). Table 1 summarized the main relevant findings, on the placental effects produced by gestational alcohol ingestion in human and/or murine models.
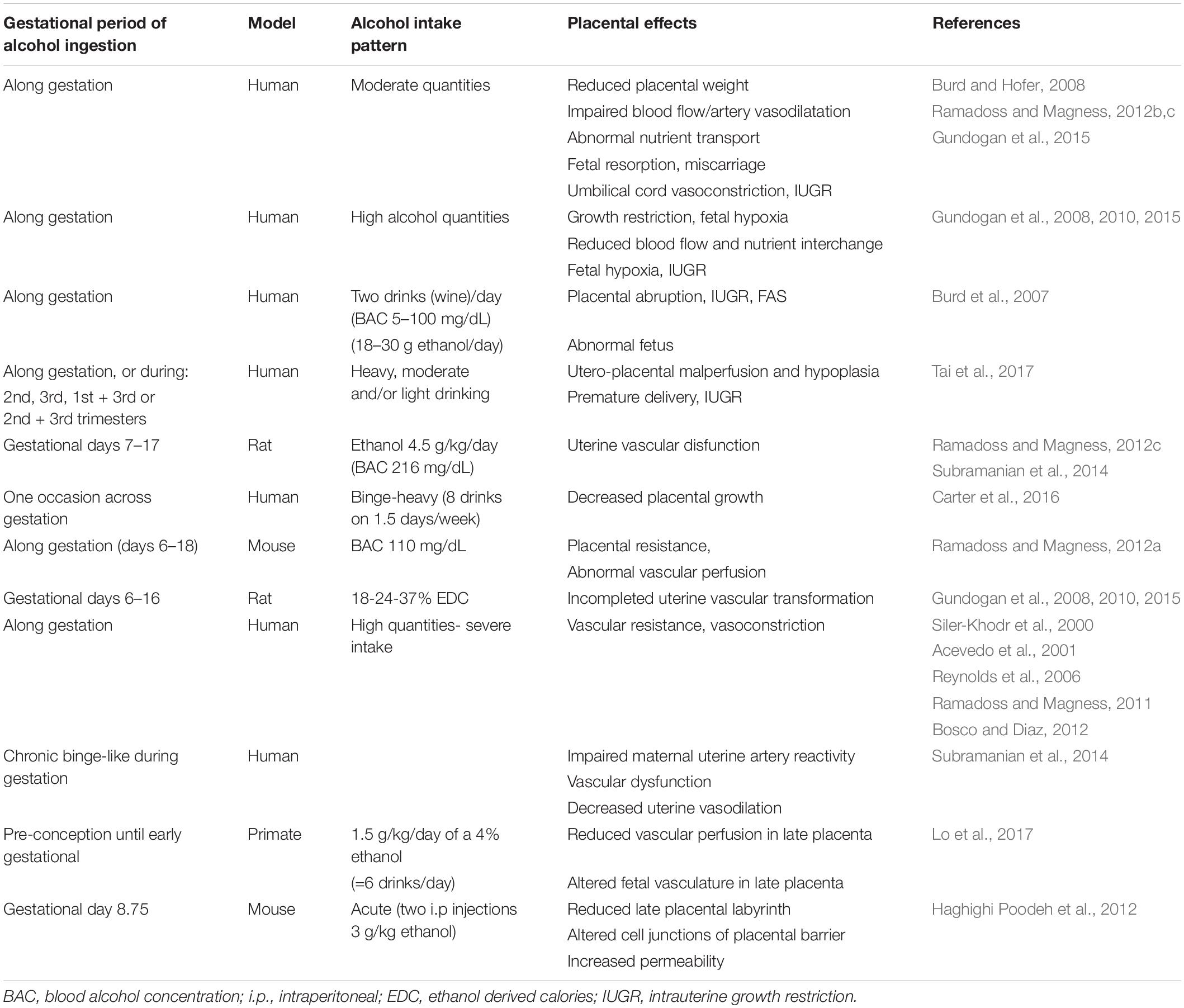
Table 1. Summary of the main findings on the placental effects produced by gestational alcohol ingestion.
Adequate mutual interactions between decidual and trophoblast tissues during early placentation determine the normal vascularization of the placenta at term. Placental defective growth and angiogenesis, linked to altered early maternal vascular remodeling and trophoblast invasion (Woods et al., 2017, 2018), may cause pregnancy failure, placental insufficiency, preeclampsia, fetal developmental disorders, and preterm birth (Cha et al., 2012). Advances in the understanding of placental abnormalities and the main mechanisms involved induced by maternal alcohol exposure are usually studied in the mouse model of placentation (Probyn et al., 2012), which briefly is given below.
Overview of Mouse Placental Development and Vascularization as a Model for Placental-Alcohol Effect Studies. Role of the Vascular Endothelial Growth Factor System
In mouse, placentation begins with implantation (days 4.5–6 of gestation), when blastocyst’s mural trophectodermal cells invade the uterine epithelium (Woods et al., 2018). After implantation, placental development goes through the gestational phases of gastrulation (days 6 to 8.5), organogenesis (days 9 to 11.5) and the fetal phase (days 12–19).
Decidual Development and Maternal Vascularization
Decidualization and maternal angiogenesis are pivotal to provide normal placental vascularization at term. Immediately with implantation, antimesometrial uterine stromal cells proliferate and transform in decidual cells, forming the avascular and densely packed decidual tissue (day 6 of gestation). Following decidualization extends toward mesometrial region of the implantation site (days 7.5–10), transformation of uterine spiral arteries occurs by angiogenesis (Das, 2009, 2010). The smooth muscle layer of spiral arteries close to the trophoblastic zone, normally largely remodel and disappear and thus, profound mesometrial decidual vascular growth takes place to allow uterine vascular elongation and provide maternal oxygenated blood flow to the fetal face of placenta (Cross et al., 2002; Kim et al., 2013; Ventureira et al., 2019). Maternal angiogenesis and vasodilation, involving also endothelial proliferation, are promoted mainly by decidual and uterine natural killer cells (uNKs) (Blois et al., 2011; Sojka et al., 2019). The uNKs have vital roles in decidual vascular remodeling and dilation of spiral arteries (Charalambous et al., 2012; Hofmann et al., 2014; Lima et al., 2014), in trophoblast invasion control (Ashkar and Croy, 2001; Ashkar et al., 2003; Croy et al., 2003; Felker and Croy, 2017; Meyer et al., 2017a,b), decidualization, and immune tolerance (Greenwood et al., 2000; Wallace et al., 2012; Rätsep et al., 2015). Mouse strains genetically ablated from uNK cells, not only fail to undergo smooth muscle spiral artery remodeling but also show abnormal branching of the vascular bed, leading to implantation sites with anomalous vascularization (Yougbaré et al., 2017). The VEGF of uNK cells is necessary to guide the maternal angiogenesis (Gargett et al., 2001; Wulff et al., 2002; Zygmunt et al., 2003; Heryanto et al., 2004; Taylor, 2004; Coultas et al., 2005).
Development of Placental-Trophoblast Layer
After implantation, in mouse gestational days 7–8.5, differentiated polyploid invasive primary trophoblast giant cells (TGCs) first invade the maternal microvasculature around the conceptus (Malassine et al., 2003), while the polar trophectoderm forms the extra-embryonic ectoderm and, from it, the chorionic cells and the ectoplacental cone (EPC). The allantois grows out, attaches and fuses to the chorionic trophoblast forming the chorio-allantoic placenta. Meanwhile, secondary TGC cells differentiate at the margin of the EPC, and become invasive to remodel mesometrially the maternal extracellular matrix (ECM) and microvasculature (Woods et al., 2018).
At organogenesis, the mouse placenta consists of three layers: the decidua, the junctional zone (JZ), and the labyrinth. The JZ, the outer layer limiting the decidua, is constituted by spongiotrophoblast cells (SpT), and the invasive cells: TGCs (P-TGC), spiral artery-associated TGCs (SpA-TGCs) and glycogen cells (GC) (Malassine et al., 2003; Woods et al., 2018). The labyrinth develops when the attached allantoic mesoderm invaginates and interdigitates into the chorion, to form the extensive vascular fetal network of definitive placenta. The labyrinth is composed of the fetal vessels, chorionic-mononuclear trophoblast cells and the allantoic mesenchyme. While the fetal vessels undergo extensive branching, the chorionic trophoblasts differentiate into sinusoidal-TGCs and the syncytiotrophoblast cells develop; all togheter form, with the fetal endothelium, the interhemal barrier (Watson and Cross, 2005; Hu and Cross, 2010; Rai and Cross, 2014; Woods et al., 2018).
When the definitive placental vascularization is established (around day 11 of gestation), major trophoblast invasion to maternal spiral arteries begins. While TGCs remodel the decidual ECM (interstitial invasion) (Knöfler et al., 2001; Rai and Cross, 2014), spiral artery-TGCs replace the endothelial cells of dilated spiral arteries (endovascular invasion). This maternal vessel transformation leads to distended, high flow and low-resistance maternal sinusoids at JZ (Cross et al., 2002), where the maternal blood is funneled in trophoblast-lined conduits in absence of arteriolar vasoconstriction. These vascular remodeling processes directed by trophoblast are major key for successful progression of pregnancy. Abnormal invasion (sub- or over-invasiveness) and maternal endothelial remodeling/replacement are key etiologic mechanisms associated to placental pathologies and pregnancy complications (Perez-Garcia et al., 2018). Placental angiogenesis-vascularization occurs under production and control of growth and vasoactive factors, such as the vascular endothelial growth factor (VEGF) (Hess et al., 2007).
Vascular Endothelial Growth Factor System During Placentation
The VEGF system is involved in angiogenesis-vascularization during placentation (Figure 1). VEGF is expressed in arteriolar smooth muscle, endothelium, in trophoblast, uNKs and decidual cells (Hoffmann et al., 2007; Zhang et al., 2011; Hofmann et al., 2014; Li et al., 2014; Ventureira et al., 2019). Its expression is regulated by hypoxia through transcription of the hypoxia inducible factor (HIF)-1α (Maltepe et al., 2005). During early gestation, HIF-1α regulates the trophoblast proliferation and differentiation during EPC development (Takeda et al., 2006). A hypoxic-oxidant environment induces the synthesis and release of anti-angiogenic factors, leading to failures in trophoblastic differentiation, invasion and placental angiogenesis (Perez-Garcia et al., 2018; Woods et al., 2018).
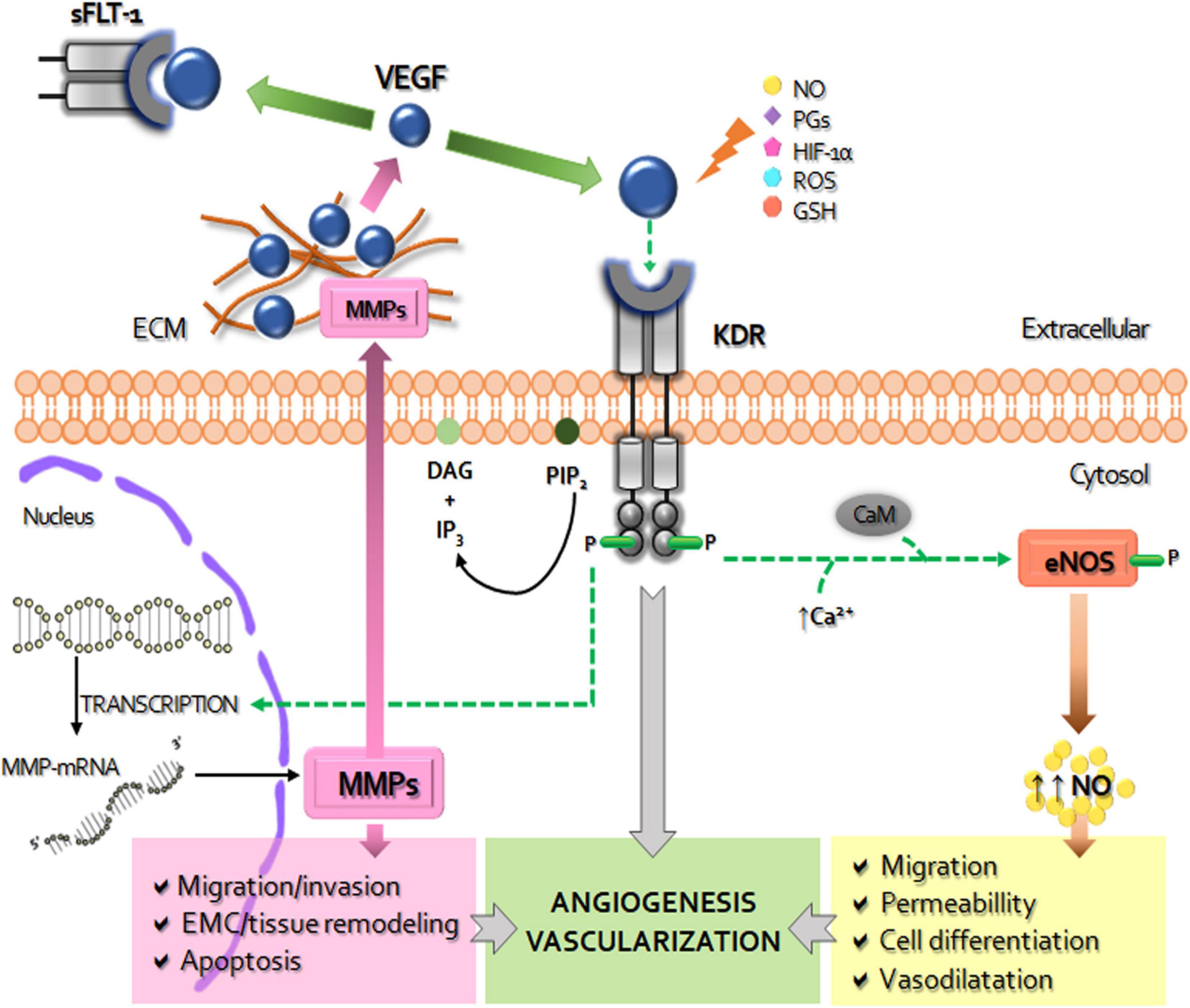
Figure 1. Schematic diagram of the role of VEGF system in the placentation. The vascular endothelial growth factor (VEGF) activates the membrane kinase insert domain receptor (KDR) by phosphorylation, triggering specific signaling cascades. By increasing intracellular calcium (Ca++) and calmodulin (CaM) VEGF-KDR binding induces the expression and activity of nitric oxide synthase (NOS) to produce increase of nitric oxide (NO), a strong inducer of increased vascular permeability, vasodilation, cell migration and differentiation. The other via of VEGF-KDR activation, related to fosfatidil inositol 4,5-bisfosfato (PIP-2) and diacylglycerol (DAG), leads to the transcription of metalloproteinases (MMPs). MMPs are secreted to the extracellular matrix (ECM) where, among other functions, degrade and remodel the ECM and release the ECM-sequestered VEGF by cleaving proteins that retain it in the matrix. The soluble receptor tyrosine kinase similar to fms-1 (sFLT-1) can prevent the binding of VEGF to KDR, thus decreasing it pro-angiogenic and survival activity. VEGF expression can be modified by NO, prostaglandins (PGs), hypoxia inducible factor (HIF-1α), reactive oxygen species (ROS), reduced glutathione (GSH), among other factors. In overall, placental activated VEGF system mediates the angiogenesis-vascularization during placentation.
Vascular Endothelial Growth Factor acts through binding to three receptors with intrinsic tyrosine kinase activity: VEGF-R1 (FLT-1), VEGF-R2 (KDR/Flk-1) and VEGF-R3 (FLT-3) (Kimura and Esumi, 2003). Main physiological effects of VEGF are attributed to KDR, whereas FLT-1 modulates VEGF signaling via ligand sequestration (Chung and Ferrara, 2011). KDR activation induces cell proliferation and migration (Lara et al., 2018), permeability (Yancopoulos et al., 2000), decidualization, trophoblastic differentiation and invasion, and nutrient uptake of the placenta (Reynolds and Redmer, 2001; Haghighi Poodeh et al., 2012). In pathological placentas, VEGF is reduced whereas the soluble form of FLT-1, responsible for the endothelial dysfunction, is increased (Roberts and Escudero, 2012; Li et al., 2014).
The VEGF-KDR binding activates signaling cascades that stimulate the production of at least 11 angiogenic factors (Apte et al., 2019), such as the endothelial nitric oxide synthase (eNOS) and matrix metalloproteinases (MMPs). The nitric oxide (NO), produced by oxidation of L-arginine, is an important regulator of the placental vasodilatation, participating in vascular smooth muscle relaxing, the increase of blood flow, and reduction of platelet aggregation and thrombosis (Krause et al., 2011).
The other major contributors to VEGF-mediated angiogenesis are the MMPs. These multigenic proteolytic zinc-dependent enzymes are composed by six classes (collagenases, gelatinases, stromelysins, matrilysins, membrane-type MMPs, and other MMPs) (Amălinei et al., 2007; Cui et al., 2017; Henriet and Emonard, 2019). MMPs are involved in proliferation, apoptosis, migration, differentiation, tissue and ECM remodeling, protein degradation (Lemaître and D’Armiento, 2006; Hamutoğlu et al., 2020), and trophoblast survival and invasion (Isaka et al., 2003; Agaoglu et al., 2016). MMP-2 (gelatinase A) and MMP-9 (gelatinase B) play a role in endometrial tissue remodeling at implantation (Novaro et al., 2002), in decidualization (Fontana et al., 2012), in trophoblast invasiveness (Staun-Ram et al., 2004; Plaks et al., 2013; Espino et al., 2017; Gualdoni G. et al., 2021), in endothelial cell morphogenesis (Chandrasekar et al., 2000). Expansion of the uterus to accommodate the growing embryo and the maternal vascular establishment depend on MMP-2 and -9 (Sternlicht and Werb, 2001). At early mouse gestation, TGCs are positive for MMP-2 and MMP-9 expression (Alexander et al., 1996; Bany et al., 2000; Bai et al., 2005), while during organogenesis mainly MMP-9 participates in TGC-invasion and labyrinthine vascularization (Fontana et al., 2012; Gualdoni G. et al., 2021; Gualdoni G. S. et al., 2021). VEGF up-regulates the MMP-2 and MMP-9 expression in human umbilical vein endothelial cells (Heo et al., 2010). Imbalances of VEGF system and subsequent alterations in MMP-2 and MMP-9 expression-activity take relevance in abnormal placentation (Gualdoni G. S. et al., 2021) and in various placentopathies (Amălinei et al., 2007; Cui et al., 2017).
Pathophysiologic Mechanisms Involved in Alcohol-Associated Placental Abnormalities and Role of the Angiogenic Vascular Endothelial Growth Factor System
The alcohol-induced abnormal placental mechanisms are multifactorial. The alcohol dispersed into placenta is primary detoxified by other placental systems (CYP2E1) different from the alcohol dehydrogenase (ADH) and aldehyde dehydrogenase (ALDH) enzymes (Gemma et al., 2007), because of their low affinity/activity in placental tissues (Heller and Burd, 2013). By this, gestational alcohol exposure impacts on the metabolism of placenta producing oxidative stress (OS) (Kay et al., 2000; Gundogan et al., 2008, 2010, 2015). Although antioxidant enzymatic and glutathione activities have been shown in the placenta after gestational alcohol ingestion (Qanungo and Mukherjea, 2000), OS impacts strongly on trophoblastic function and leads to pregnancy loss (Gundogan et al., 2010). Perigestational moderate alcohol ingestion up to organogenesis in mouse produces protein nitration, lipid peroxidation and DNA damage in trophoblast-decidual tissue (Coll et al., 2018). As a result of alcohol-induced OS and decreased bioavailability of NO, insufficient early placental vascularization and arteriolar vasoconstriction can cause placental hypoxia (Acevedo et al., 2001; Wareing et al., 2006; Gualdoni G. S. et al., 2021).
Stimulatory and inhibitory effects of ethanol on VEGF have been reported, depending on alcohol administration patterns (Radek et al., 2008; Haghighi Poodeh et al., 2012; Jegou et al., 2012). In chick extraembryonic tissues, moderate and heavy alcohol exposure for 24 or 48 h, impaires vascular development and downregulates VEGF and its receptors (Gu et al., 2001; Tufan and Satiroglu-Tufan, 2003). Together with enhanced permeability and altered placental barrier, VEGF was up-regulated in the CD-1 mouse placenta at 9.5–14.5 days of gestation after two 4-h interval intraperitoneal doses of 3 g/kg ethanol injected 8.75 days post-coitum (Haghighi Poodeh et al., 2012). Ethanol treatment decreases VEGF in yolk sac membranes by inhibition of angiogenic genes due to excess of alcohol-induced reactive oxygen species production (Wang et al., 2016). However, diminished placental vascular density after early alcohol exposure significantly decreased KDR expression in placenta at term (Holbrook et al., 2019). In addition, in alcohol-induced hypoxic placenta, the release of anti-angiogenic soluble receptor sFlt-1 (Reyes et al., 2012), is associated with maternal endothelial dysfunction (Roberts and Cooper, 2001; Roberts et al., 2011). Thus, alterations in the VEGF-VEGF-R, caused by oxidative stress, may be the main cause important imbalances in placental angiogenesis induced by alcohol.
The VEGF downstream molecular expression, such as NO and MMPs, may be altered by alcohol. Chronic binge-like alcohol decreases uterine arterial endothelial eNOS expression in an animal model of third trimester-equivalent of human pregnancy (Ramadoss et al., 2011), in a similar way as does the acute ethanol exposure in human placenta. However, chronic and acute ethanol exposure seems to induce eNOS activity in the fetoplacental unit in other model and HUVEC cells, respectively (Acevedo et al., 2001). Anyway, inhibition or stimulation of NO synthesis by alcohol use throughout pregnancy leads to vasoconstriction of the placenta and umbilical vessels and results in hypoxia and reduced fetal malnutrition (Lui et al., 2014; Gundogan et al., 2015; Lo et al., 2017; Holbrook et al., 2019; Ohira et al., 2019). Consequently, the impaired placental function leads to an increase in oxidative stress that compromises placentation as it alters trophoblast cell motility (Kay et al., 2000; Gundogan et al., 2008). Also, gestational alcohol exposure can alter transcription of MMPs and affects the maternal uterine vascular remodeling (Orzabal et al., 2019).
Perigestational Alcohol Consumption Up to Early Gestation: Effects on Mouse Placentation and Role of the Vascular Endothelial Growth Factor System
The origins of uteroplacental insufficiency and vasculopathy at late gestation may be caused by abnormal placentation during early pregnancy, including the peri-implantation period (Burton and Jauniaux, 2010; Kajantie et al., 2010). Evidence is currently lacking, in animal models, to explain the effects and cellular-molecular pathways responsible for late placental abnormalities induced by perigestational alcohol consumption (PAC) until early gestation (Gårdebjer et al., 2014; Kalisch-Smith and Moritz, 2017; Kalisch-Smith et al., 2019). Here, in a mouse model, we propose that PAC up to organogenesis disrupts the early decidual-trophoblastic development and vascularization (Figures 2A,B; Perez-Tito et al., 2014; Coll et al., 2018; Ventureira et al., 2019; Gualdoni G. S. et al., 2021), leading to incomplete maternal vascular remodeling due to trophoblast invasion defects (Figure 2C), and later, to abnormal placenta (Figure 2D).
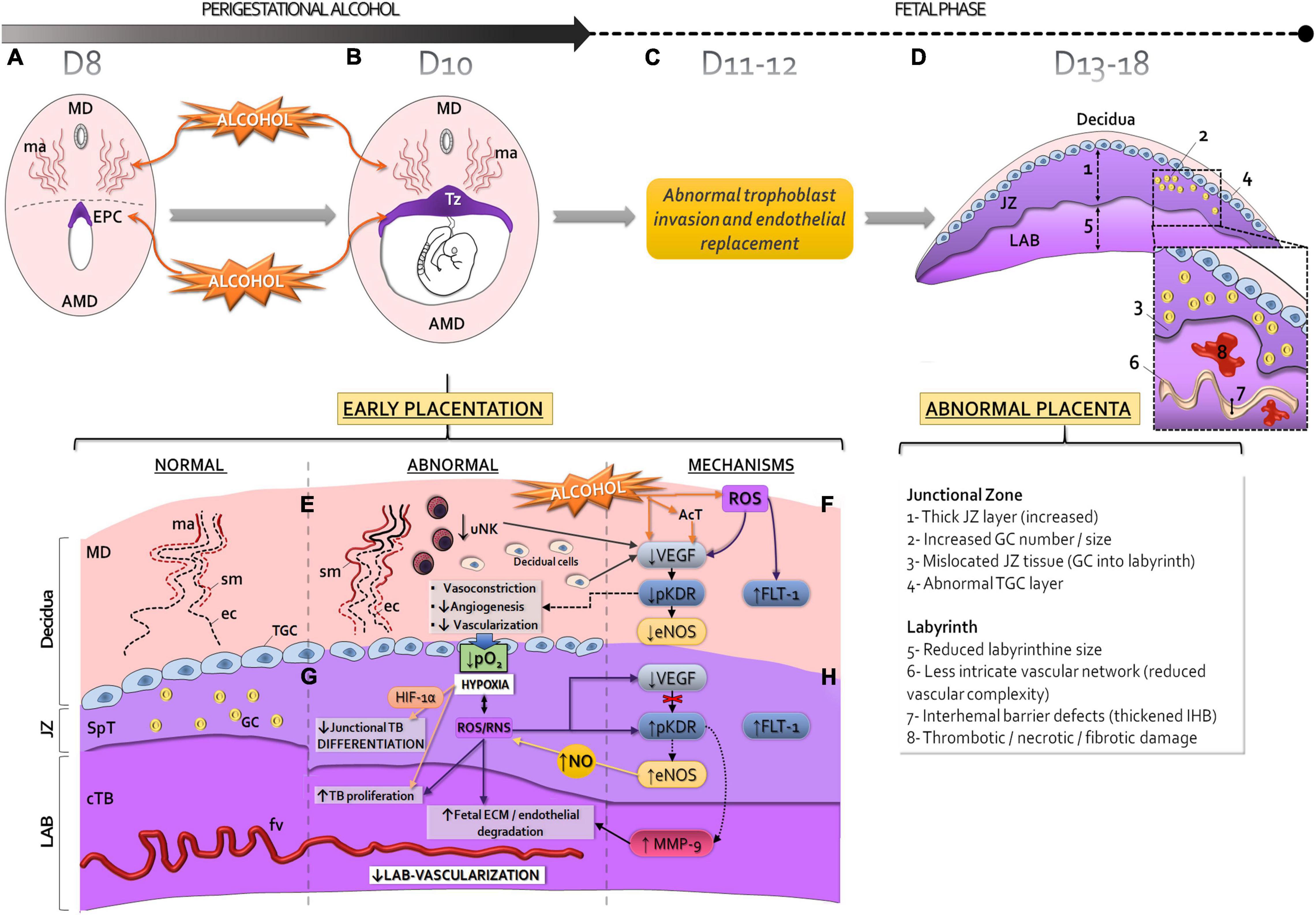
Figure 2. Proposed mechanisms of abnormal early placentation and vascularization and long-term impact to placenta produced by perigestational alcohol consumption, in the mouse model. Perigestational alcohol (OH) intake up to gastrulation-organogenesis [day 8 (D8) (A) and 10 (D10) (B), disrupts the maternal spiral artery (ma) remodeling of mesometrial decidua (MD), and alters the ectoplacental cone (EPC) (A) and subsequent development of trophoblastic zone (Tz) of the early placenta (B). Consequently, after cessation of alcohol ingestion (day 11, D11), abnormal trophoblast invasion and endothelial replacement may occur (C), leading to abnormal structure and function of the placenta at term (D). The placental abnormalities can be originated in altered angiogenesis-vascularization of decidual-trophoblastic tissues (E,G) during perigestational alcohol exposure (B). Alcohol and/or its metabolites [acetaldehyde, AcT, reactive oxygen species (ROS)] impact on the early placentation processes, including changes in uNK cells. Consequently, diminished junctional trophoblast differentiation and low labyrinthine growth and vascularization can occur (G). The decidual-trophoblastic abnormalities can be associated with altered endothelial nitric oxide synthase (eNOS) and metalloproteinases (MMP)-2 and/or MMP-9 expression/activity due to abnormal vascular endothelial growth factor (VEGF) expression and KDR activation (pKDR) or increased FLT-1 (F,H). The disrupted VEGF-receptor-NOS-MMPs mechanisms are linked to hypoxia and increased HIF-1α, and reactive oxygen species and/or reactive nitrogen species (ROS/RNS) production. Sm, smooth muscle cells of spiral arteries; ec, endothelial cells; cTB: chorionic trophoblastic cells; SpT, spongiotrophoblastic cells; GC, glycogen cells; TGC, trophoblast giant cells; fv, fetal vessels; Lab, labyrinth; JZ, junctional zone; TB, trophoblast; ECM, extracellular matrix; AMD, antimesometrial decidua.
After PAC up to day 10 of gestation, the lumen expansion of decidual spiral arteries is reduced (Figure 2E; Ventureira et al., 2019), producing a poor dilation of maternal vascular bed. Alcohol could alter the decidual artery endothelial organization and reduces cell proliferation. PAC disrupts the arterial smooth muscle cell remodeling and leads to permanent muscle wall in decidual vessels. These defects could be associated to decreased number of uNK cells in decidua, which may also be involved in low dilation and less branching of maternal spiral arteries (Figure 2E; Ventureira et al., 2019).
Reduced decidual angiogenesis after PAC can be explained in part by the decreased VEGF expression in decidual and uNK cells probably due to OS (Coll et al., 2018; Ventureira et al., 2019; Figure 2F). However, down-regulation of KDR expression in decidual and endothelial cells after PAC could also be involved in the abnormal decidual vascularization. FLT-1 drives anti-angiogenic effects by its binding to VEGF (Ferrara, 2004; Lima et al., 2014; Felker and Croy, 2017), and its increase was associated with oxidative factors (Kim et al., 2013). After PAC, OS may induce FLT-1 expression in decidual and uNK cells causing abnormal decidual angiogenesis (Ventureira et al., 2019; Figure 2F).
Impaired downstream VEGF signaling, due to reduced activation of KDR (pKDR) after alcohol consumption, leads to a decreased expression/activity of eNOS in the decidual endothelium, contributing to maternal artery vasoconstriction and reduced angiogenesis (Figure 2F). The immediate consequence of low decidual vascularization, because of unremodeled maternal vessels, is a deficient blood perfusion, increased blood pressure and flow velocity. Reduced tissue oxygenation increases HIF-1α expression levels in the trophoblastic interface, which is consistent with a hypoxic-oxidative state (Gualdoni G. S. et al., 2021; Figure 2G).
At organogenesis, the labyrinth is still growing in a low oxygen environment, but junctional trophoblastic cells become more differentiated and invasive with increased oxygenation (Cowden Dahl et al., 2005; Pringle et al., 2010). PAC produces trophoblastic growth deficiency and TGC and spongiotrophoblast cell abnormalities compatible with trophoblast differentiation alterations (Gualdoni G. S. et al., 2021; Figure 2G). Subsequent abnormal invasion (Figure 2C) can lead to placentopathy later (Woods et al., 2017; Figure 2D). Paralely, the PAC-induced deficient labyrinthine vasculogenesis is associated to a densely packed tissue due to increased chorionic trophoblastic cell proliferation (Gualdoni G. S. et al., 2021; Figure 2G). The early insufficient labyrinthine vascularization generates persistent hypoxia and OS and embryo growth restriction and malformations at organogenesis (Cebral et al., 2007; Coll et al., 2011, 2017; Gualdoni G. S. et al., 2021).
Although hypoxia is a strong stimulus for placental VEGF expression (Zhang et al., 2015), this expression decreased in the exposed-trophoblastic tissues (Gualdoni G. S. et al., 2021). Despite VEGF reduction, probably due to OS and/or to sequestering by FLT-1, alcohol induces high trophoblastic KDR phosphorylation (Figure 2H). Following PAC, downstream KDR activation results in increased trophoblastic eNOS expression, which over-produces NO, causing placental OS. However, eNOS expression could also be triggered by FLT-1 pathways (Bussolati et al., 2001) or induced by hypoxia since eNOS promoter contains hypoxia response elements (Schäffer et al., 2006). Once ethanol induces NO production, this factor is able to cause phosphorylation of KDR (Bussolati et al., 2001). Despite KDR activation in JZ and labyrinth, MMP-2 and MMP-9 expression decreased in the former but only MMP-9 increased in the later, resulting in tissue-dependent adverse alcohol effects in the exposed trophoblastic tissues (Gualdoni G. S. et al., 2021; Figure 2H).
In conclusion, PAC up to organogenesis leads to early abnormal placentation by defective decidual-trophoblastic development and disruption of complex angiogenic cellular processes, in which the VEGF system results one of the major affected mechanisms. PAC up to early pregnancy may lead to placental abnormalities and vasculopathy compatible with abnormal placentas associated to FASD.
Author Contributions
EC have proposed the topic of this revision and prepared the draft of the manuscript. GG and EC edited the text. GG designed the figures. PJ, CB, and MV co-wrote the manuscript. All authors approved the last version of the manuscript.
Funding
This study was supported by ANPCyT PICT 2008-2210 and PICT 2019-00129 grants.
Conflict of Interest
The authors declare that the research was conducted in the absence of any commercial or financial relationships that could be construed as a potential conflict of interest.
Publisher’s Note
All claims expressed in this article are solely those of the authors and do not necessarily represent those of their affiliated organizations, or those of the publisher, the editors and the reviewers. Any product that may be evaluated in this article, or claim that may be made by its manufacturer, is not guaranteed or endorsed by the publisher.
References
Acevedo, C. G., Carrasco, G., Burotto, M., Rojas, S., and Bravo, I. (2001). Ethanol inhibits L-arginine uptake and enhances NO formation in human placenta. Life Sci. 68, 2893–2903. doi: 10.1016/s0024-3205(01)01070-0
Adamson, S. L., Lu, Y., Whiteley, K. J., Holmyard, D., Hemberger, M., Pfarrer, C., et al. (2002). Interactions between trophoblast cells and the maternal and fetal circulation in the mouse placenta. Dev. Biol. 250, 358–373. doi: 10.1006/dbio.2002.0773
Agaoglu, O. K., Agaoglu, A. R., Guzeloglu, A., Aslan, S., Kurar, E., Kayis, S. A., et al. (2016). Gene expression profiles of some cytokines, growth factors, receptors, and enzymes (GM-CSF, IFNγ, MMP-2, IGF-II, EGF, TGF-β, IGF-IIR) during pregnancy in the cat uterus. Theriogenology 85, 638–644. doi: 10.1016/j.theriogenology.2015.10.001
Alexander, C. M., Hansell, E. J., Behrendtsen, O., Flannery, M. L., Kishnani, N. S., Hawkes, S. P., et al. (1996). Expression and function of matrix metalloproteinases and their inhibitors at the maternal-embryonic boundary during mouse embryo implantation. Development 122, 1723–1736. doi: 10.1242/dev.122.6.1723
Aliyu, M. H., Lynch, O., Nana, P. N., Alio, A. P., Wilson, R. E., Marty, P. J., et al. (2011). Alcohol consumption during pregnancy and risk of placental abruption and placenta previa. Matern. Child. Health. J 15, 670–676. doi: 10.1007/s10995-010-0615-6
Aliyu, M. H., Wilson, R. E., Zoorob, R., Chakrabarty, S., Alio, A., Kirby, R. S., et al. (2008). Alcohol consumption during pregnancy and the risk of early stillbirth among singletons. Alcohol 42, 369–374. doi: 10.1016/j.alcohol.2008.04.003
Amălinei, C., Căruntu, I. D., and Bãlan, R. A. (2007). Biology of metalloproteinases. Rom. J. Morphol. Embryol. 48, 323–334.
Apte, R. S., Chen, D. S., and Ferrara, N. (2019). VEGF in signaling and disease: beyond discovery and development. Cell 176, 1248–1264. doi: 10.1016/j.cell.2019.01.021
Ashkar, A. A., Black, G. P., Wei, Q., He, H., Liang, L., Head, J. R., et al. (2003). Assessment of requirements for IL-15 and IFN regulatory factors in uterine NK cell differentiation and function during pregnancy. J. Immunol. 171, 2937–2944. doi: 10.4049/jimmunol.171.6.2937
Ashkar, A. A., and Croy, B. A. (2001). Functions of uterine natural killer cells are mediated by interferon gamma production during murine pregnancy. Semin. Immunol. 13, 235–241. doi: 10.1006/smim.2000.0319
Avalos, L. A., Roberts, S. C. M., Kaskutas, L. A., Block, G., and Li, D. K. (2014). Volume and type of alcohol during early pregnancy and the risk of miscarriage. Subst. Use. Misuse. 49, 1437–1445. doi: 10.3109/10826084.2014.912228
Bada, H. S., Das, A., Bauer, C. R., Shankaran, S., Lester, B. M., Gard, C. C., et al. (2005). Low birth weight and preterm births: etiologic fraction attributable to prenatal drug exposure. J. Perinatol. 25, 631–637. doi: 10.1038/sj.jp.7211378
Bai, S. X., Wang, Y. L., Qin, L., Xiao, Z. J., Herva, R., and Piao, Y. S. (2005). Dynamic expression of matrix metalloproteinases (MMP-2, -9 and -14) and the tissue inhibitors of MMPs (TIMP-1, -2 and -3) at the implantation site during tubal pregnancy. Reproduction 129, 103–113. doi: 10.1530/rep.1.00283
Bany, B., Harvey, M., and Schultz, G. (2000). Expression of matrix metalloproteinases 2 and 9 in the mouse uterus during implantation and oil-induced decidualization. J. Reprod. Fertil. 120, 125–134. doi: 10.1530/reprod/120.1.125
Blois, S. M., Klapp, B. F., and Barrientos, G. (2011). Decidualization and angiogenesis in early pregnancy: unravelling the functions of DC and NK cells. J. Reprod. Immunol. 88, 86–92. doi: 10.1016/j.jri.2010.11.002
Bosco, C., and Diaz, E. (2012). Placental hypoxia and foetal development versus alcohol exposure in pregnancy. Alcohol Alcohol. 47, 109–117. doi: 10.1093/alcalc/agr166
Burd, L., and Hofer, R. (2008). Biomarkers for detection of prenatal alcohol exposure: a critical review of fatty acid ethyl esters in meconium. Birth Defects Res. A Clin. Mol. Teratol. 82, 487–493. doi: 10.1002/bdra.20464
Burd, L., Roberts, D., Olson, M., and Odendaal, H. J. (2007). Ethanol and the placenta: a review. J. Matern. Fetal Neonatal Med. 20, 361–375. doi: 10.1080/14767050701298365
Burton, G. J., and Jauniaux, E. (2010). “The maternal circulation and placental shape,” in The Placenta and Human Developmental Programming, eds G. J. Burton, D. J. P. Barker, A. Moffett, and K. Thornburg (Cambridge: Cambridge University Press), 161–174. doi: 10.1017/cbo9780511933806.014
Bussolati, B., Dunk, C., Grohman, M., Kontos, C. D., and Ahmed, J. M. (2001). Vascular endothelial growth factor receptor-1 modulates vascular endothelial growth factor- mediated angiogenesis via nitric oxide. Am. J. Pathol. 159, 993–1008.
Carter, R. C., Wainwright, H., Molteno, C. D., Georgieff, M. K., Dodge, N. C., Warton, F., et al. (2016). Alcohol, methamphetamine, and marijuana exposure have distinct effects on the human placenta. Alcohol. Clin. Exp. Res. 40, 753–764. doi: 10.1111/acer.13022
Cebral, E., Abrevaya, X. C., and Mudry, M. D. (2011). Male and female reproductive toxicity induced by sub-chronic ethanol exposure in CF-1 mice. Cell Biol. Toxicol. 27, 237–248. doi: 10.1007/s10565-011-9185-7
Cebral, E., Faletti, A., Jawerbaum, A., and Paz, D. (2007). Periconceptional alcohol consumption-induced changes in embryonic prostaglandin E levels in mouse organogenesis. Modulation by nitric oxide. Prostaglandins Leukot Essent. Fatty Acids. 76, 141–151. doi: 10.1016/j.plefa.2006.12.001
Cha, J., Sun, X., and Dey, S. K. (2012). Mechanisms of implantation: strategies for successful pregnancy. Nat. Med. 18, 1754–1767. doi: 10.1038/nm.3012
Chandrasekar, N., Jasti, S., Alfred-Yung, W. F., Ali-Osman, F., Dinh, D. H., Olivero, W. C., et al. (2000). Modulation of endothelial cell morphogenesis in vitro by MMP-9 during glial-endothelial cell interactions. Clin. Exp. Metastasis. 18, 337–342. doi: 10.1023/A:1010833730407
Charalambous, F., Elia, A., and Georgiades, P. (2012). Decidual spiral artery remodeling during early post-implantation period in mice: investigation of associations with decidual uNK cells and invasive trophoblast. Biochem. Biophys. Res. Commun. 417, 847–852. doi: 10.1016/j.bbrc.2011.12.057
Chung, A. S., and Ferrara, N. (2011). Developmental and pathological angiogenesis. Annu. Rev. Cell Dev. Biol. 27, 563–584. doi: 10.1146/annurev-cellbio-092910-154002
Coll, T. A., Chaufan, G., Pérez Tito, L. G., Ventureira, M. R., Ríos de Molina, M. C., and Cebral, E. (2018). Cellular and molecular oxidative stress-related effects in uterine myometrial and trophoblast-decidual tissues after perigestational alcohol intake up to early mouse organogenesis. Mol. Cell. Biochem. 440, 89–104. doi: 10.1007/s11010-017-3158-y
Coll, T. A., Chaufan, G., Pérez-Tito, L., Ventureira, M. R., Sobarzo, C. M. A., Ríos de Molina, M. D. C., et al. (2017). Oxidative stress and cellular and tissue damage in organogenic outbred mouse embryos after moderate perigestational alcohol intake. Mol. Reprod. Dev. 84, 1086–1099. doi: 10.1002/mrd.22865
Coll, T. A., Perez-Tito, L., Sobarzo, C. M. A., and Cebral, E. (2011). Embryo developmental disruption during organogenesis produced by CF-1 murine periconceptional alcohol consumption. Birth Defects Res. B. 92, 560–574. doi: 10.1002/bdrb.20329
Colvin, L., Payne, J., Parsons, D., Kurinczuk, J. J., and Bower, C. (2007). Alcohol consumption during pregnancy in nonindigenous west Australian women. Alcohol. Clin. Exp. Res. 31, 276–284. doi: 10.1111/j.1530-0277.2006.00303.x
Coultas, L., Chawengsaksophak, K., and Rossant, J. (2005). Endothelial cells and VEGF in vascular development. Nature 438, 937–945. doi: 10.1038/nature04479
Cowden Dahl, K. D., Fryer, B. H., Mack, F. A., Compernolle, V., Maltepe, E., Adelman, D. M., et al. (2005). Hypoxia-inducible factors 1alpha and 2alpha regulate trophoblast differentiation. Mol. Cell. Biol. 25, 10479–10491. doi: 10.1128/MCB.25.23
Creeth, H. D. J., and John, R. M. (2020). The placental programming hypothesis: placental endocrine insufficiency and the co-occurrence of low birth weight and maternal mood disorders. Placenta 98, 52–59. doi: 10.1016/j.placenta.2020.03.011
Cross, J. C., Hemberger, M., Lu, Y., Nozaki, T., Whiteley, K., Masutani, M., et al. (2002). Trophoblast functions, angiogenesis and remodeling of the maternal vasculature in the placenta. Mol. Cell Endocrinol. 187, 207–212. doi: 10.1016/s0303-7207(01)00703-1
Croy, B. A., Esadeg, S., Chantakru, S., van den Heuvel, M., Paffaro, V. A., He, H., et al. (2003). Update on pathways regulating the activation of uterine natural killer cells, their interactions with decidual spiral arteries and homing of their precursors to the uterus. J. Reprod. Immunol. 59, 175–191. doi: 10.1016/S0165-0378(03)00046-9
Cui, N., Hu, M., and Khalil, R. A. (2017). Biochemical and biological attributes of matrix metalloproteinases. Prog. Mol. Biol. Transl. Sci. 147, 1–73. doi: 10.1016/bs.pmbts.2017.02.005
Das, S. K. (2009). Cell cycle regulatory control for uterine stromal cell decidualization in implantation. Reproduction 137, 889–899. doi: 10.1530/REP-08-0539
Das, S. K. (2010). Regional development of uterine decidualization: molecular signaling by Hoxa-10. Mol. Reprod. Dev. 77, 387–396. doi: 10.1002/mrd.21133
Davis, E. F., Lazdam, M., Lewandowski, A. J., Worton, S. A., Kelly, B., Kenworthy, Y., et al. (2012a). Cardiovascular risk factors in children and young adults born to preeclamptic pregnancies: a systematic review. Pediatrics 129, e1552–e1561. doi: 10.1542/peds.2011-3093
Davis, E. F., Newton, L., Lewandowski, A. J., Lazdam, M., Kelly, B. A., Kyriakou, T., et al. (2012b). Pre-eclampsia and offspring cardiovascular health: mechanistic insights from experimental studies. Clin. Sci. 123, 53–72. doi: 10.1042/CS20110627
Davis-Anderson, K. L., Berger, S., Lunde-Young, E. R., Naik, V. D., Seo, H., Johnson, G. A., et al. (2017). Placental proteomics reveal insights into fetal alcohol spectrum disorders. Alcohol. Clin. Exp. Res. 41, 1551–1558. doi: 10.1111/acer.13448
de Sanctis, L., Memo, L., Pichini, S., Tarani, L., and Vagnarelli, F. (2011). Fetal alcohol syndrome: new perspectives for an ancient and underestimated problem. J. Matern. Fetal Neonatal Med. 24(Suppl. 1), 34–37. doi: 10.3109/14767058.2011.607576
Espino, Y., Sosa, S., Flores-Pliego, A., Espejel-Nuñez, A., Medina-Bastidas, D., Vadillo-Ortega, F., et al. (2017). New insights into the role of matrix metalloproteinases in preeclampsia. Int. J. Mol. Sci. 18:1448. doi: 10.3390/ijms18071448
Felker, A. M., and Croy, B. A. (2017). Natural cytotoxicity receptor 1 in mouse uNK cell maturation and function. Mucosal Immunol. 10, 1122–1132. doi: 10.1038/mi.2016.126
Ferrara, N. (2004). Vascular endothelial growth factor: basic science and clinical progress. Endocrine Rev. 25, 581–611. doi: 10.1210/er.2003-0027
Fontana, V., Coll, T. A., Sobarzo, C. M. A., Perez-Tito, L., Calvo, J. C., and Cebral, E. (2012). Matrix metalloproteinase expression and activity in trophoblast decidual tissues at organogenesis in CF-1 mouse. J. Mol. Histol. 43, 487–496. doi: 10.1007/s10735-012-9429-8
Gårdebjer, E. M., Cuffe, J. S. M., Pantaleon, M., Wlodek, M. E., and Moritz, K. M. (2014). Periconceptional alcohol consumption causes fetal growth restriction and increases glycogen accumulation in the late gestation rat placenta. Placenta 35, 50–57. doi: 10.1016/j.placenta.2013.10.008
Gargett, C. E., Lederman, F., Heryanto, B., Gambino, L. S., and Rogers, P. A. W. (2001). Focal vascular endothelial growth factor correlates with angiogenesis in human endometrium. Role of intravascular neutrophils. Hum. Reprod. 16, 1065–1075. doi: 10.1093/humrep/16.6.1065
Gemma, S., Vichi, S., and Testai, E. (2007). Metabolic and genetic factors contributing to alcohol induced effects and fetal alcohol syndrome. Neurosci. Biobehav. Rev. 31, 221–229. doi: 10.1016/j.neubiorev.2006.06.018
Greenwood, J. D., Minhas, K., di Santo, J. P., Makita, M., Kiso, Y., and Croy, B. A. (2000). Ultrastructural studies of implantation sites from mice deficient in uterine natural killer cells. Placenta 21, 693–702. doi: 10.1053/plac.2000.0556
Gu, J. W., Elam, J., Sartin, A., Li, W., Roach, R., and Adair, T. H. (2001). Moderate levels of ethanolinduce expression of vascular endothelial growth factor and stimulate angiogenesis. Am. J. Physiol. Regulat. Integrat. Comparat. Physiol. 281, R365–R372. doi: 10.1152/ajpregu.2001.281.1.R365
Gualdoni, G., Gomez Castro, G., Hernández, R., Barbeito, C., and Cebral, E. (2021). Comparative matrix metalloproteinase-2 and -9 expression and activity during endotheliochorial and hemochorial trophoblastic invasiveness. Tissue Cell 74:101698. doi: 10.1016/j.tice.2021.101698
Gualdoni, G. S., Ventureira, M. R., Coll, T. A., Palomino, W. A., Barbeito, C. G., and Cebral, E. (2021). Perigestational alcohol consumption induces altered early placentation and organogenic embryo growth restriction by disruption of trophoblast angiogenic factors. Reprod. BioMed. Online. 42, 481–504. doi: 10.1016/j.rbmo.2020.10.015
Guerri, C., Bazinet, A., and Riley, E. P. (2009). Foetal alcohol spectrum disorders and alterations in brain and behaviour. Alcohol Alcohol. 44, 108–114. doi: 10.1093/alcalc/agn105
Gundogan, F., Elwood, G., Longato, L., Tong, M., Feijoo, A., Carlson, R. I., et al. (2008). Impaired placentation in fetal alcohol syndrome. Placenta 29, 148–157. doi: 10.1016/j.placenta.2007.10.002
Gundogan, F., Elwood, G., Mark, P., Feijoo, A., Longato, L., Tong, M., et al. (2010). Ethanol-induced oxidative stress and mitochondrial dysfunction in rat placenta: relevance to pregnancy loss. Alcohol. Clin. Exp. Res. 34, 415–423. doi: 10.1111/j.1530-0277.2009.01106.x
Gundogan, F., Gilligan, J., Ooi, J. H., Sung, J., Qi, W., Naram, R., et al. (2013). Dual mechanisms of ethanolimpaired placentation: experimental model. J. Clin. Exp. Pathol. 3:142. doi: 10.4172/2161-0681.1000142
Gundogan, F., Gilligan, J., Qi, W., Chen, E., Naram, R., and de la Monte, S. M. (2015). Dose effect of gestational ethanol exposure on placentation and fetal growth. Placenta 36, 523–530. doi: 10.1016/j.placenta.2015.02.010
Gupta, K. K., Gupta, V. K., and Shirasaka, T. (2016). An update on Fetal alcohol syndrome—pathogenesis, risks, and treatment. Alcohol. Clin. Exp. Res. 40, 1594–1602. doi: 10.1111/acer.13135
Guttmacher, A. E., Maddox, Y. T., and Spong, C. Y. (2014). The human placenta project: placental structure, development, and function in real time. Placenta 35, 303–304. doi: 10.1016/j.placenta.2014.02.012
Haghighi Poodeh, S., Salonurmi, T., Nagy, I., Koivunen, P., Vuoristo, J., Räsänen, J., et al. (2012). Alcohol-induced premature permeability in mouse placenta-yolk sac barriers in vivo. Placenta 33, 866–873. doi: 10.1016/j.placenta.2012.07.008
Hamutoğlu, R., Bulut, H. E., Kaloğlu, C., Önder, O., Dağdeviren, T., Aydemir, M. N., et al. (2020). The regulation of trophoblast invasion and decidual reaction by matrix metalloproteinase-2, metalloproteinase-7, and metalloproteinase-9 expressions in the rat endometrium. Reprod. Med. Biol. 19, 385–397. doi: 10.1002/rmb2.12342
Han, M., Neves, A. L., Serrano, M., Brinez, P., Huhta, J. C., Acharya, G., et al. (2012). Effects of alcohol, lithium, and homocysteine on nonmuscle myosin-II in the mouse placenta and human trophoblasts. Am. J. Obstet. Gynecol. 207, 140.e7–140.e19. doi: 10.1016/j.ajog.2012.05.007
Haycock, P. C., and Ramsay, M. (2009). Exposure of mouse embryos to ethanol during preimplantation development: effect on DNA methylation in the h19 imprinting control region. Biol. Reprod. 81, 618–627. doi: 10.1095/biolreprod.108.074682
Heller, M., and Burd, L. (2013). Review of ethanol dispersion, distribution, and elimination from the fetal compartment. Birth Defects Res. Clin. Mol. Teratol. 100, 277–283. doi: 10.1002/bdra.23232
Henriet, P., and Emonard, H. (2019). Matrix metalloproteinase-2: not (just) a “hero” of the past. Biochimie 166, 223–232. doi: 10.1016/j.biochi.2019.07.019
Heo, S. H., Choi, Y. J., Ryoo, H. M., and Cho, J. Y. (2010). Expression profiling of ETS and MMP factors in VEGF-activated endothelial cells: role of MMP-10 in VEGF-induced angiogenesis. J. Cell. Physiol. 224, 734–742. doi: 10.1002/jcp.22175
Heryanto, B., Girling, J. E., and Rogers, P. A. (2004). Intravascular neutrophils partially mediate the endometrial endothelial cell proliferative response to oestrogen in ovariectomised mice. Reproduction 127, 613–620. doi: 10.1530/rep.1.00161
Hess, A. P., Hamilton, A. E., Talbi, S., Dosiou, C., Nyegaard, M., Nayak, N., et al. (2007). Decidual stromal cell response to paracrine signals from the trophoblast: amplification of immune and angiogenic modulators. Biol. Reprod. 76, 102–117. doi: 10.1095/biolreprod.106.054791
Hoffmann, P., Feige, J. J., and Alfaidy, N. (2007). Placental expression of EG-VEGF and its receptors PKR1 (prokineticin receptor-1) and PKR2 throughout mouse gestation. Placenta 28, 1049–1058. doi: 10.1016/j.placenta.2007.03.008
Hofmann, A. P., Gerber, S. A., and Croy, B. A. (2014). Uterine natural killer cells pace early development of mouse decidua basalis. Mol. Hum. Reprod. 20, 66–76. doi: 10.1093/molehr/gat060
Holbrook, B. D., Davies, S., Cano, S., Shrestha, S., Jantzie, L. L., Rayburn, W. F., et al. (2019). The association between prenatal alcohol exposure and protein expression in human placenta. Birth Defects Res. 111, 749–759. doi: 10.1002/bdr2.1488
Hoyme, H. E., Kalberg, W. O., Elliott, A. J., Blankenship, J., Buckley, D., Marais, A. S., et al. (2016). Updated clinical guidelines for diagnosing fetal alcohol spectrum disorders. Pediatrics 138:e20154256. doi: 10.1542/peds.2015-4256
Hu, D., and Cross, J. (2010). Development and function of trophoblast giant cells in the rodent placenta. Inter. J. Dev. Biol. 54, 341–354. doi: 10.1387/ijdb.082768dh
Hutson, J. R., Stade, B., Lehotay, D. C., Collier, C. P., and Kapur, B. M. (2012). Folic acid transport to the human fetus is decreased in pregnancies with chronic alcohol exposure. PLoS One. 7:e38057. doi: 10.1371/journal.pone.0038057
Isaka, K., Usuda, S., Ito, H., Sagawa, Y., Nakamura, H., Nishi, H., et al. (2003). Expression and activity of matrix metalloproteinase 2 and 9 in human trophoblasts. Placenta 24, 53–64. doi: 10.1053/plac.2002.0867
Iveli, M. F., Morales, S., Rebolledo, A., Savietto, V., Salemme, S., Apezteguia, M., et al. (2007). Effects of light ethanol consumption during pregnancy: increased frequency of minor anomalies in the newborn and altered contractility of umbilical cord artery. Pediatr. Res. 61, 456–461. doi: 10.1203/pdr.0b013e3180332c59
Jegou, S., El Ghazi, F., Kwetieu de Lendeu, P., Marret, S., Laudenbach, V., Uguen, A., et al. (2012). Prenatal alcohol exposure affects vasculature development in the neonatal brain. Ann. Neurol. 72, 952–960. doi: 10.1002/ana.23699
Joya, X., Garcia-Algar, O., Salat-Batlle, J., Pujades, C., and Vall, O. (2015). Advances in the development of novel antioxidant therapies as an approach for fetal alcohol syndrome prevention. Birth Defects Res. (Part A) 103, 163–177. doi: 10.1002/bdra.23290
Kajantie, E., Thornburg, K. L., Eriksson, J. G., Osmond, C., and Barker, D. J. (2010). In preeclampsia, the placenta grows slowly along its minor axis. Int. J. Dev. Biol. 54, 469–473. doi: 10.1387/ijdb.082833ek
Kalisch-Smith, J. I., and Moritz, K. M. (2017). Detrimental effects of alcohol exposure around conception: putative mechanisms. Biochem. Cell. Biol. 96, 107–116. doi: 10.1139/bcb-2017-0133
Kalisch-Smith, J. I., Steane, S. E., Simmons, D. G., Pantaleon, M., Anderson, S. T., Akison, L. K., et al. (2019). Periconceptional alcohol exposure causes female-specific perturbations to trophoblast differentiation and placental formation in the rat. Development 146:dev172205. doi: 10.1242/dev.172205
Kay, H. H., Grindle, K. M., and Magness, R. R. (2000). Ethanol exposure induces oxidative stress and impairs nitric oxide availability in the human placental villi: a possible mechanism of toxicity. Am. J. Obstetrics Gynecol. 182, 682–688. doi: 10.1067/mob.2000.104201
Kim, M., Park, H. J., Seol, J. W., Jang, J. Y., Cho, Y. I. S. I., Kim, K. R., et al. (2013). VEGF-A regulated by progesterone governs uterine angiogenesis and vascular remodeling during pregnancy. EMBO Mol. Med. 5, 1415–1430. doi: 10.1002/emmm.201302618
Kimura, H., and Esumi, H. (2003). Reciprocal regulation between nitric oxide and vascular endothelial growth factor in angiogenesis. Acta Biochim. Pol. 50, 49–59.
Knöfler, M., Vasicek, R., and Schreiber, M. (2001). Key regulatory transcription factors involved in placental trophoblast development - A review. Placenta 22, S83–S92. doi: 10.1053/plac.2001.0648
Krause, H., Hanson, M. A., and Casanello, P. (2011). Role of nitric oxide in placental vascular development and function. Placenta 32, 797–805. doi: 10.1016/j.placenta.2011.06.025
Kwan, S. T. C., Kezer, C. A., Helfrich, K. K., Saini, N., Huebner, S. M., and Flentke, G. R. (2020). Maternal iron nutriture modulates placental development in a rat model of fetal alcohol spectrum disorder. Alcohol 84, 57–66. doi: 10.1016/j.alcohol.2019.11.003
Lange, S., Probst, C., Gmel, G., Rehm, J., Burd, L., and Popova, S. (2017). Global prevalence of fetal alcohol spectrum disorder among children and youth: a systematic review and meta-analysis. JAMA Pediatr. 171, 948–956. doi: 10.1001/jamapediatrics.2017.1919
Lara, E., Acurio, J., Leon, J., Penny, J., Torres-Vergara, P., and Escudero, C. (2018). Are the cognitive alterations present in children born from preeclamptic pregnancies the result of impaired angiogenesis? focus on the potential role of the VEGF family. Front. Physiol. 9:1591. doi: 10.3389/fphys.2018.01591
Lemaître, V., and D’Armiento, J. (2006). Matrix metalloproteinases in development and disease. Birth. Defects. Res. C. Embryo Today. 78, 1–10. doi: 10.1002/bdrc.20065
Li, H., Qu, D., McDonald, A., Isaac, S. M., Whiteley, K. J., Sung, H. K., et al. (2014). Trophoblast-specific reduction of VEGFA alters placental gene expression and maternal cardiovascular function in mice. Biol. Reprod. 91:87. doi: 10.1095/biolreprod.114.118299
Lima, P. D., Zhang, J., Dunk, C., Lye, S. J., and Croy, B. A. (2014). Leukocyte driven-decidual angiogenesis in early pregnancy. Cell. Mol. Immunol. 11, 522–537. doi: 10.1038/cmi.2014.63
Linask, K. K., and Han, M. (2016). Acute alcohol exposure during mouse gastrulation alters lipid metabolism in placental and heart development: folate prevention. Birth Defects Res. A. 106, 749–760. doi: 10.1002/bdra.23526
Livy, D. J., Maier, S. E., and West, J. R. (2004). Long-term alcohol exposure prior to conception results in lower fetal body weights. Birth Defects Res. B. Dev. Reprod. Toxicol. 71, 135–141. doi: 10.1002/bdrb.20007
Lo, J., Schabel, M. C., Roberts, V. H. J., Wang, X., Lewandowski, K. S., Grant, K. A., et al. (2017). First trimester alcohol exposure alters placental perfusion and fetal oxygen availability affecting fetal growth and development in a non-human primate model. Am. J. Obstet. Gynecol. 216, 302.e1–302.e8. doi: 10.1016/j.ajog.2017.01.016
López, M. B. (2013). Saber, valorar y actuar: relaciones entre informacio‘n, actitudes y consumo de alcohol durante la gestacion. Health Addict. 13, 35–46.
López, M. B., Aran-Filippetti, V., and Cremonte, M. (2015). Consumo de alcohol antes y durante la gestación en Argentina: prevalencia y factores de riesgo. Revista Panamericana de Salud Publica 37, 211–217.
Lui, S., Jones, R. L., Robinson, N. J., Greenwood, S. L., Aplin, J. D., and Tower, C. L. (2014). Detrimental effects of ethanol and its metabolite acetaldehyde, on first trimester human placental cell turnover and function. PLoS One 9:e87328. doi: 10.1371/journal.pone.0087328
Magri, R., Mıguez, H., and Parodi, V. (2007). Consumo de alcohol y otras drogas en embarazadas. Arch. Pediatr. Urug. 78, 122–132.
Malassine, A., Frendo, J. L., and Evain-Brion, D. (2003). A comparison of placental development and endocrine functions between the human and mouse model. Hum. Reprod. Update. 9, 531–539. doi: 10.1093/humupd/dmg043
Maltepe, E., Krampitz, G. W., Okazaki, K. M., Red-Horse, K., Mak, W., Simon, M. C., et al. (2005). Hypoxia-inducible factor-dependent histone deacetylase activity determines stem cell fate in the placenta. Development 132, 3393–3403. doi: 10.1242/dev.01923
Martín-Estal, I., Rodriguez-Zambrano, M. A., and Castilla-Cortázar, I. (2019). “Biochemical assessment of placental function,” in Fetal Growth Restrict, eds L. Nardozza, E. Araujo Júnior, G. Rizzo, and R. Deter (Cham: Springer), 83–116.
May, P. A., and Gossage, J. P. (2011). Maternal risk factors for fetal alcohol spectrum disorders: not as simple as it might seem. Alcohol. Res. Health 34, 15–26.
May, P. A., Gossage, J. P., Kalberg, W. O., Robinson, L. K., Buckley, D., Manning, M., et al. (2009). Prevalence and epidemiologic characteristics of FASD from various research methods with an emphasis on recent in-school studies. Dev. Disabil. Res. Rev. 15, 176–192. doi: 10.1002/ddrr.68
Memo, L., Gnoato, E., Caminiti, S., Pichini, S., and Tarani, L. (2013). Fetal alcohol spectrum disorders and fetal alcohol syndrome: the state of the art and new diagnostic tools. Early Hum. Dev. 89(Suppl. 1), S40–S43. doi: 10.1016/S0378-3782(13)70013-6
Meyer, N., Schüler, T., and Zenclussen, A. C. (2017a). Simultaneous ablation of uterine natural killer cells and uterine mast cells in mice leads to poor vascularization and abnormal Doppler measurements that compromise fetal well-being. Front. Immunol. 8:1913. doi: 10.3389/fimmu.2017.01913
Meyer, N., Woidacki, K., Knöfler, M., Meinhardt, G., Nowak, D., Velicky, P., et al. (2017b). Chymase-producing cells of the innate immune system are required for decidual vascular remodeling and fetal growth. Sci. Rep. 7:45106. doi: 10.1038/srep45106
Meyer-Leu, Y., Lemola, S., Daeppen, J. B., Deriaz, O., and Gerber, S. (2011). Association of moderate alcohol use and binge drinking during pregnancy with neonatal health. Alcohol. Clin. Exp. Res. 35, 1669–1677. doi: 10.1111/j.1530-0277.2011.01513.x
Novaro, V., Pustovrh, C., Colman-Lerner, A., Radisky, D., Lo Nostro, F., Paz, D., et al. (2002). Nitric oxide induces gelatinase A (matrix metalloproteinase 2) during rat embryo implantation. Fertil. Steril. 78, 1278–1287. doi: 10.1016/s0015-0282(02)04240-1
Odendaal, H., Wright, C., Schubert, P., Boyd, T. K., Roberts, D. J., and Brink, L. (2020). Associations of maternal smoking and drinking with fetal growth and placental abruption. Eur. J. Obstet. Gynecol. Reprod. Biol. 253, 95–102. doi: 10.1016/j.ejogrb.2020.07.018
Ohira, S., Motoki, N., Shibazaki, T., Misawa, Y., Inaba, Y., and Kanai, M. (2019). Alcohol consumption during pregnancy and risk of placental abnormality: the Japan environment and Children’s study. Sci. Rep. 9:10259. doi: 10.1038/s41598-019-46760-1
Orzabal, M. R., Lunde-Younga, E. R., Ramireza, J. I., Naika, V. D., Hillhouseb, A., Kongantib, K., et al. (2019). Gestational binge alcohol-induced alterations in maternal uterine artery transcriptome. Reprod. Toxicol. 87, 42–49. doi: 10.1016/j.reprotox.2019.05.053
Patra, J., Bakker, R., Irving, H., Jaddoe, V. W., Malini, S., and Rehm, J. (2011). Dose-response relationship between alcohol consumption before and during pregnancy and the risks of low birthweight, preterm birth and small for gestational age (SGA)-a systematic review and meta-analyses. BJOG 118, 1411–1421. doi: 10.1111/j.1471-0528.2011.03050.x
Perez-Garcia, V., Fineberg, F., Wilson, R., Murray, A., Mazzeo, C. I., Tudor, C., et al. (2018). Placentation defects are highly prevalent in embryonic lethal mouse mutants. Nature 555, 463–468. doi: 10.1038/nature26002
Perez-Tito, L. G., Bevilacqua, E., and Cebral, E. (2014). Peri-implantational in vivo and in vitro embryo trophoblast development after perigestational alcohol exposure in the CD-1 mouse. Drug. Chem. Toxicol. 37, 184–197. doi: 10.3109/01480545.2013.834358
Plaks, V., Rinkenberger, J., Dai, J., Flannery, M., Sund, M., Kanasaki, K., et al. (2013). Matrix metalloproteinase-9 deficiency phenocopies features of preeclampsia and intrauterine growth restriction. Proc. Natl. Acad. Sci. U.S.A. 110, 11109–11114. doi: 10.1073/pnas.1309561110
Popova, S., Lange, S., Probst, C., Gmel, G., and Rehm, J. (2017). Estimation of national, regional, and global prevalence of alcohol use during pregnancy and fetal alcohol syndrome: a systematic review and meta-analysis. Lancet. Glob. Health 5, e290–e299. doi: 10.1016/S2214-109X(17)30021-9
Pringle, K. G., Kind, K. L., Sferruzzi-Perri, A. N., Thompson, J. G., and Roberts, C. T. (2010). Beyond oxygen: complex regulation and activity of hypoxia inducible factors in pregnancy. Hum. Reprod. Update 16, 415–431. doi: 10.1093/humupd/dmp046
Probyn, M. E., Zanini, S., Ward, L. C., Bertram, J. F., and Morit, K. M. (2012). A rodent model of low- to moderate-dose ethanol consumption during pregnancy: patterns of ethanol consumption and effects on fetal and offspring growth. Reprod. Fertil. Dev. 24, 859–870. doi: 10.1071/RD11200
Qanungo, S., and Mukherjea, M. (2000). Ontogenic profile of some antioxidants and lipid peroxidation in human placental and fetal tissues. Mol. Cell. Biochem. 215, 11–19. doi: 10.1023/a:1026511420505
Radek, K. A., Kovacs, E. J., Gallo, R. L., and DiPietro, L. A. (2008). Acute ethanol exposure disrupts VEGF receptor cell signaling in endothelial cells. Am. J. Physiol. Heart Circ. Physiol. 295, H174–H184. doi: 10.1152/ajpheart.00699.2007
Radek, K. A., Matthies, A. M., Burns, A. L., Heinrich, S. A., Kovacs, A. J., and DiPietro, L. A. (2005). Acute ethanol exposure impairs angiogenesis and the proliferative phase of wound healing. Am. J. Physiol. Heart. Circ. Physiol. 289, H1084–H1090. doi: 10.1152/ajpheart.00080.2005
Rai, A., and Cross, J. C. (2014). Development of the hemochorial maternal vascular spaces in the placenta through endothelial and vasculogenic mimicry. Dev. Biol. 387, 131–141. doi: 10.1016/j.ydbio.2014.01.015
Ramadoss, J., Jobe, S. O., and Magness, R. R. (2011). Alcohol and maternal uterine vascular adaptations during pregnancy-part I: effects of chronic in vitro binge-like alcohol on uterine endothelial nitric oxide system and function. Alcohol. Clin. Exp. Res. 35, 1686–1693. doi: 10.1111/j.1530-0277.2011.01515.x
Ramadoss, J., and Magness, R. R. (2011). 2-D DIGE uterine endothelial proteomic profile for maternal chronic binge-like alcohol exposure. J. Proteomics 74, 2986–2994. doi: 10.1016/j.jprot.2011.07.029
Ramadoss, J., and Magness, R. R. (2012c). Vascular effects of maternal alcohol consumption. Am. J. Physiol. Heart Circ. Physiol. 303, H414–H421. doi: 10.1152/ajpheart.00127.2012
Ramadoss, J., and Magness, R. R. (2012b). Multiplexed digital quantification of binge-like alcohol-mediated alterations in maternal uterine angiogenic mRNA transcriptome. Physiol. Genomics 44, 622–628. doi: 10.1152/physiolgenomics.00009.2012
Ramadoss, J., and Magness, R. R. (2012a). Alcohol-Induced alterations in maternal uterine endothelial proteome: a quantitative iTRAQ mass spectrometric approach. Reprod. Toxicol. 34, 538–544. doi: 10.1016/j.reprotox.2012.08.008
Rätsep, M. T., Felker, A. M., Kay, V. R., Tolusso, L., Hofmann, A. P., and Croy, B. A. (2015). Uterine natural killer cells: supervisors of vasculature construction in early decidua basalis. Reproduction 149, R91–R102. doi: 10.1530/REP-14-0271
Reyes, L., Garcia, R., Ruiz, S., Dehghan, M., and Lopez-Jaramillo, P. (2012). Nutritional status among women with pre-eclampsia and healthy pregnant and not-pregnant women in Latin American country. J. Obstet. Gynecol. Res. 38, 498–504. doi: 10.1111/j.1447-0756.2011.01763.x
Reynolds, L. P., Caton, J. S., Redmer, D. A., Grazul-Bilska, A. T., Vonnahme, K. A., Borowicz, P. P., et al. (2006). Evidence for altered placental blood flow and vascularity in compromised pregnancies. J. Physiol. 572, 51–58. doi: 10.1113/jphysiol.2005.104430
Reynolds, L. P., and Redmer, D. A. (2001). Minireview: angiogenesis in the placenta. Biol. Reprod. 64, 1033–1040. doi: 10.1095/biolreprod64.4.1033
Roberts, J. M., Bodnar, L. M., Patrick, T. E., and Powers, R. W. (2011). The role of obesity in preeclampsia. Pregnancy Hypertens. 1, 6–16. doi: 10.1016/j.preghy.2010.10.013
Roberts, J. M., and Cooper, D. W. (2001). Pathogenesis and geneticsof pre-eclampsia. Lancet 357, 53–56. doi: 10.1016/S0140-6736(00)03577-7
Roberts, J. M., and Escudero, C. (2012). The placenta in preeclampsia. Pregnancy Hypertens. 2, 72–83. doi: 10.1016/j.preghy.2012.01.001
Roozen, S., Peters, G. J., Kok, G., Townend, D., Nijhuis, J., and Curfs, L. (2016). Worldwide prevalence of fetal alcohol spectrum disorders: a systematic literature review including meta-analysis. Alcohol. Clin. Exp. Res. 40, 18–32. doi: 10.1111/acer.12939
Rosenberg, M. J., Wolff, C. R., El-Emawy, A., Staples, M. C., Perrone-Bizzozero, N. I., and Savage, D. D. (2010). Effects of moderate drinking during pregnancy on placental gene expression. Alcohol 44, 673–690. doi: 10.1016/j.alcohol.2009.10.002
Salihu, H. M., Kornosky, J. L., Lynch, O. N., Alio, A. P., August, E. M., and Marty, P. J. (2011). Impact of prenatal alcohol consumption on placenta-associated syndromes. Alcohol 45, 73–79. doi: 10.1016/j.alcohol.2010.05.010
Schäffer, L., Vogel, J., Breymann, C., Gassmann, M., and Marti, H. H. (2006). Preserved placental oxygenation and development during severe systemic hypoxia. Am. J. Physiol. Regul. Integr. Comp. Physiol. 290, R844–R851. doi: 10.1152/ajpregu.00237.2005
Sharma, D., Shastri, S., Farahbakhsh, N., and Sharma, P. (2016). Intrauterine growth restriction - Part 1. J. Matern. Fetal. Neonatal. Med. 29, 3977–3987. doi: 10.3109/14767058.2016.1152249
Siler-Khodr, T. M., Yang, Y., Grayson, M. H., Henderson, G. I., Lee, M., and Schenker, S. (2000). Effect of ethanol on thromboxane and prostacyclin production in the human placenta. Alcohol 21, 169–180. doi: 10.1016/S0741-8329(00)00084-7
Sojka, D. K., Yang, L., and Yokoyama, W. M. (2019). Uterine natural killer cells. Front. Imunol. 10:960. doi: 10.3389/fimmu.2019.00960
Staun-Ram, E., Goldman, S., Gabarin, D., and Shalev, E. (2004). Expression and importance of matrix metalloproteinases 2 and 9 (MMP-2 and MMP-9) in human trophoblast invasion. Reprod. Biol. Endocrinol. 2:59. doi: 10.1186/1477-7827-2-59
Sternlicht, M. D., and Werb, Z. (2001). How matrix metalloproteinases regulate cell behaviour. Annu. Rev. Cell. Dev. Biol. 17, 463–516. doi: 10.1146/annurev.cellbio.17.1.463
Subramanian, K., Naik, V. D., Sathishkumar, M. S. K., Yallampalli, C., Saade, G. R., Hankins, G. D., et al. (2014). Chronic binge alcohol exposure during pregnancy impairs rat maternal uterine vascular function. Alcohol. Clin. Exp. Res. 38, 1832–1838. doi: 10.1111/acer.12431
Tai, M., Piskorski, A., Kao, J. C. W., Hess, L. A., de la Monte, S. M., and Gündogan, F. (2017). Placental morphology in fetal alcohol spectrum disorders. Alcohol Alcohol. 52, 138–144. doi: 10.1093/alcalc/agw088
Takeda, K., Ho, V. C., Takeda, H., Duan, L. J., Nagy, A., and Fong, G. H. (2006). Placental but not heart defects are associated with elevated hypoxia-inducible factor alpha levels in mice lacking prolyl hydroxylase domain protein 2. Mol. Cell Biol. 26, 8336–8346. doi: 10.1128/MCB.00425-06
Taylor, H. S. (2004). Endometrial cells derived from donor stem cells in bone marrow transplant recipients. JAMA 292, 81–85. doi: 10.1001/jama.292.1.81
Tufan, A. C., and Satiroglu-Tufan, N. L. (2003). The effect of ethanol exposure on extraembryonic vascular development in the chick area vasculosa. Cells. Tissues. Organs. 175, 84–97. doi: 10.1159/000073752
Van Heertum, K., and Rossi, B. (2017). Alcohol and fertility: how much is too much? Fertil. Res. Pract. 3:10. doi: 10.1186/s40738-017-0037-x
Ventureira, M. R., Sobarzo, C., Argandoña, F., Palomino, W. A., Barbeito, C., and Cebral, C. (2019). Decidual vascularization in organogenesis after perigestational alcohol ingestion. Reproduction 158, 109–122. doi: 10.1530/REP-18-0230
Wallace, A. E., Fraser, R., and Cartwright, J. E. (2012). Extravillous trophoblast and decidual natural killer cells: a remodelling partnership. Hum. Reprod. Update 18, 458–471. dms015 doi: 10.1093/humupd/
Wang, G., Zhong, S., Zhang, S. Y., Ma, Z. L., Chen, J. L., and Lu, W. H. (2016). Angiogenesis is repressed by ethanol exposure during chick embryonic development. J. Appl. Toxicol. 36, 692–701. doi: 10.1002/jat.3201
Wareing, M., Greenwood, S. L., and Baker, P. N. (2006). Reactivity of human placental chorionic plate vessels is modified by level of oxygenation: differences between arteries and veins. Placenta 27, 42e–48e. doi: 10.1016/j.placenta.2004.11.008
Watson, E. D., and Cross, J. C. (2005). Development of structures and transport functions in the mouse placenta. Physiology 20, 180–193. doi: 10.1152/physiol.00001.2005
Woods, L., Perez-Garcia, V., and Hemberger, M. (2018). Regulation of placental development and its impact on fetal growth-New Insights From Mouse Models. Front. Endo. 9:570. doi: 10.3389/fendo.2018.00570
Woods, L., Perez-Garcia, V., Kieckbusch, J., Wang, X., DeMayo, F., Colucci, F., et al. (2017). Decidualisation and placentation defects are a major cause of age-related reproductive decline. Nat. Commun. 8:352. doi: 10.1038/s41467-017-00308-x
Wulff, C., Wilson, H., Dickson, S. E., Wiegand, S. J., and Fraser, H. M. (2002). Hemochorial placentation in the primate: expression of vascular endothelial growth factor, angiopoietins, and their receptors throughout pregnancy. Biol. Reprod. 66, 802–812. doi: 10.1095/biolreprod66.3.802
Yancopoulos, G. D., Davis, S., Gale, N. W., Rudge, J. S., Wiegand, S. J., and Holash, J. (2000). Vascular-specific growth factors and blood vessel formation. Nature 407, 242–248. doi: 10.1038/35025215
Yougbaré, I., Tai, W., Zdravic, D., Oswald, B. E., Lang, S., Zhu, G., et al. (2017). Activated NK cells cause placental dysfunction and miscarriages in fetal alloimmune thrombocytopenia. Nat. Commun. 8:224. doi: 10.1038/s41467-017-00269-1
Zhang, J., Chen, Z., Smith, G. N., and Croy, B. A. (2011). Natural killer cell-triggered vascular transformation: maternal care before birth? Cell. Mol. Immunol. 8, 1–11. doi: 10.1038/cmi.2010.38
Zhang, R., Pan, X. H., and Xiao, L. (2015). Expression of vascular endothelial growth factor (VEGF) under hypoxia in placenta with intrahepatic cholestasis of pregnancy and its clinically pathological significance. Int. J. Clin. Exp. Pathol. 8, 11475–11479.
Zhu, Y., Romitti, P. A., Caspers Conway, K. M., Shen, D. H., Sun, L., Browne, M. L., et al. (2015). Maternal periconceptional alcohol consumption and congenital heart defects. Birth Defects Res. A Clin. Mol. Teratol. 103, 617–629. doi: 10.1002/bdra.23352
Keywords: placenta, perigestational alcohol, vascular abnormality, VEGF system, mouse
Citation: Gualdoni GS, Jacobo PV, Barril C, Ventureira MR and Cebral E (2022) Early Abnormal Placentation and Evidence of Vascular Endothelial Growth Factor System Dysregulation at the Feto-Maternal Interface After Periconceptional Alcohol Consumption. Front. Physiol. 12:815760. doi: 10.3389/fphys.2021.815760
Received: 15 November 2021; Accepted: 28 December 2021;
Published: 02 February 2022.
Edited by:
Carlos Galaviz-Hernandez, Instituto Politécnico Nacional (IPN), MexicoReviewed by:
Sanjay Basak, National Institute of Nutrition (ICMR), IndiaCopyright © 2022 Gualdoni, Jacobo, Barril, Ventureira and Cebral. This is an open-access article distributed under the terms of the Creative Commons Attribution License (CC BY). The use, distribution or reproduction in other forums is permitted, provided the original author(s) and the copyright owner(s) are credited and that the original publication in this journal is cited, in accordance with accepted academic practice. No use, distribution or reproduction is permitted which does not comply with these terms.
*Correspondence: Elisa Cebral, ZWNlYnJhbEBob3RtYWlsLmNvbQ==