- 1Section of Cardiology, Department of Medicine, Tulane University School of Medicine, New Orleans, LA, United States
- 2Tulane University Heart and Vascular Institute, New Orleans, LA, United States
Heart failure with preserved ejection fraction is a growing epidemic and accounts for half of all patients with heart failure. Increasing prevalence, morbidity, and clinical inertia have spurred a rethinking of the pathophysiology of heart failure with preserved ejection fraction. Unlike heart failure with reduced ejection fraction, heart failure with preserved ejection fraction has distinct clinical phenotypes. The obese-diabetic phenotype is the most often encountered phenotype in clinical practice and shares the greatest burden of morbidity and mortality. Left ventricular remodeling plays a major role in its pathophysiology. Understanding the interplay of obesity, diabetes mellitus, and inflammation in the pathophysiology of left ventricular remodeling may help in the discovery of new therapeutic targets to improve clinical outcomes in heart failure with preserved ejection fraction. Anti-diabetic agents like glucagon-like-peptide 1 analogs and sodium-glucose co-transporter 2 are promising therapeutic modalities for the obese-diabetic phenotype of heart failure with preserved ejection fraction and aggressive weight loss via lifestyle or bariatric surgery is still key to reverse adverse left ventricular remodeling. This review focuses on the obese-diabetic phenotype of heart failure with preserved ejection fraction highlighting the interaction between obesity, diabetes, and coronary microvascular dysfunction in the development and progression of left ventricular remodeling. Recent therapeutic advances are reviewed.
Introduction
Heart failure with preserved ejection fraction (HFpEF) is a growing epidemic (Owan and Redfield, 2005). Unlike heart failure with reduced ejection fraction (HFrEF), myocardial contractility is near normal in HFpEF, and impaired left ventricular (LV) relaxation/increased stiffness leads to pulmonary congestion and thereby dyspnea, pulmonary hypertension, and exercise intolerance (Becher et al., 2013; Andersson and Vasan, 2014; Borlaug, 2014). Currently, HFpEF is the leading cause of hospitalizations in patients > 65 years. It will overcome HFrEF as the leading cause of heart failure (HF) within the next 10 years (Lam et al., 2011; Liu et al., 2013). The increasing prevalence of HFpEF and lack of guideline-directed therapy, has rekindled interest in its pathophysiology (Borlaug, 2020; Mishra and Kass, 2021).
The cornerstone of HF is LV remodeling. In HFrEF, systolic dysfunction leads to eccentric hypertrophy with LV wall thinning and replacement fibrosis. In HFpEF, the LV wall thickens leading to concentric hypertrophy (LVH) (Heinzel et al., 2015) with impaired myocardial relaxation/increased stiffness leading to LV diastolic dysfunction (LVDD) (LeWinter and Meyer, 2013) and ultimately HFpEF. Age (Cheng et al., 2009), hypertension (Verdecchia et al., 1995), obesity (Woodiwiss et al., 2008), diabetes (T2D) (Eguchi et al., 2005), and renal dysfunction (Pluta et al., 2015) contribute to LV concentric remodeling. Distinct clinical HFpEF phenotypes are increasingly being recognized (Samson et al., 2016). Phenotyping HFpEF allows tailoring therapeutic modalities for concentric LV remodeling reversal and eventually, better outcomes. The obese-diabetic phenotype of HFpEF is extremely common (Samson et al., 2016) and associated with poor outcomes (Yusuf et al., 2003).
Obesity is the main driver of T2D with 90–95% of patients with T2D being obese (Mozaffarian et al., 2015). Obesity and T2D overlap in the development and progression of HFpEF (Altara et al., 2017). In the present review, we reviewed articles related to HFpEF and T2D. We conducted a literature search using PubMed, Embase, Ovid, and Cochrane databases and searched terms like “HF,” “T2D,” “HFpEF,” “Obesity,” “LVDD,” “epicardial adipose tissue (EAT),” and “visceral adipose tissue (VAT).” Arranged by hierarchy we reviewed randomized clinical trials, followed by registries and then cohort studies. This review first addresses how obesity affects LV remodeling and fosters low-grade systemic inflammation/microvascular dysfunction and thereby HFpEF (McHugh et al., 2019; Piche et al., 2020). Specific contributions of T2D to inflammation (Tsalamandris et al., 2019), coronary microvascular dysfunction (CMD) (Di Carli et al., 2003), and cardiac myocytes diastolic Ca2+ handling (Eisner et al., 2020) are then reviewed. Last, we address the clinical implications of obesity and T2D on HFpEF outcomes before reviewing emerging therapeutic options.
Effects of Obesity on the Heart
Obesity and Left Ventricular Concentric Remodeling
The obesity-LV concentric remodeling association was first reported in observational studies and later confirmed in several community-based cohorts (Peterson et al., 2004b; Wong et al., 2004; Powell et al., 2006; Avelar et al., 2007; Woodiwiss et al., 2008; Turkbey et al., 2010; Gidding et al., 2013; Kishi et al., 2014; Reis et al., 2014; Bello et al., 2016; Fliotsos et al., 2018; Razavi et al., 2020; Yan et al., 2020). The correlation between weight loss and decrease in LV mass and not between weight loss and decline in blood pressure (BP) after metabolic surgery is further evidence of the central role of obesity in the pathogenesis of LV concentric remodeling (Jhaveri et al., 2009; Rider et al., 2009; Owan et al., 2011; Kurnicka et al., 2018). However, the loose correlation between obesity-induced LV concentric remodeling and LVDD suggests that obesity may impair LV diastolic function through other mechanisms than obesity heightened cardiac pre-and afterload (Russo et al., 2011). Obesity-induced increase in myocardial triglycerides (TGs) content and myocardial energetics impairment may worsen LVDD (Peterson et al., 2004a; Rider et al., 2013; Piche and Poirier, 2018; Rayner et al., 2018).
Not unexpectedly, obesity is now a recognized risk factor for incident HFpEF (Packer and Kitzman, 2018; Pandey et al., 2018; Savji et al., 2018). Incident HFpEF correlates more closely with visceral adipose tissue (VAT) mass than body mass index (BMI) (Neeland et al., 2013; Cordola Hsu et al., 2021). Peak aerobic capacity is inversely and independently related to intra-abdominal fat, abdominal adiposity is a strong risk factor for all-cause mortality, and CT measured VAT predicts incident hospitalization in patients with HFpEF (Tsujimoto and Kajio, 2017; Haykowsky et al., 2018; Rao et al., 2018). In the Irbesartan in heart failure with preserved ejection fraction (I-PRESERVE) trial (Massie et al., 2008) 71% of the patients had a BMI > 26.5 kg/m2 and 55% of the patients in the Phosphodiesterase-5 inhibition to improve clinical status and exercise capacity in HFpEF (RELAX) trial had a BMI > 35 kg/m2 (Haass et al., 2011; Reddy et al., 2019). Women had a relatively greater waist circumference (an indirect measure of VAT) than men in the prospective comparison of angiotensin receptor -neprilysin inhibitor with ARB global outcomes in HFpEF (PARAGON-HF) trial (McMurray et al., 2020). A table regarding the salient features of important trials in HFpEF has been listed in Table 1.
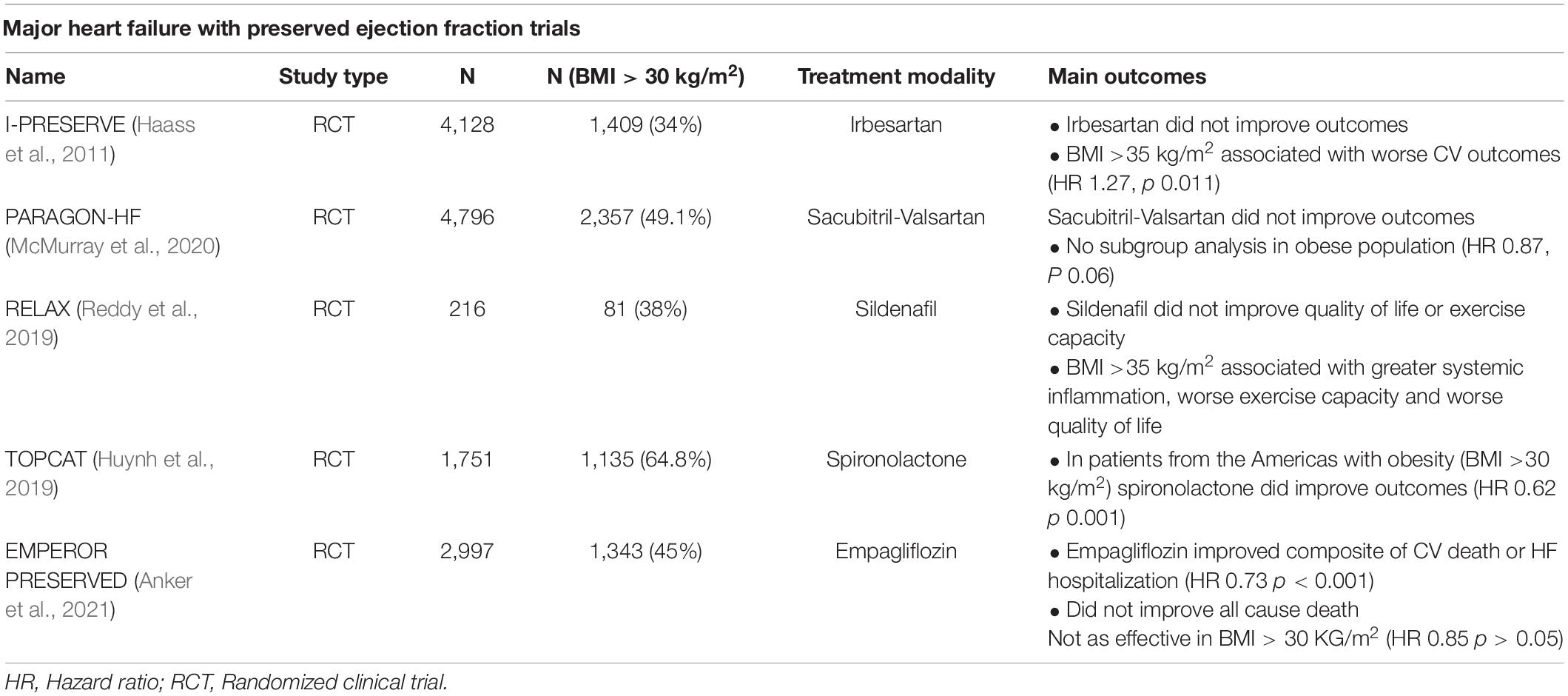
Table 1. Major heart failure with preserved ejection fraction trials with role of obesity in outcomes.
Obesity and Sodium Retention
Obesity leads to HFpEF by increasing renal tubular sodium reabsorption and plasma volume expansion (Bickel et al., 2001; Kotsis et al., 2010; Obokata et al., 2017). The overproduction of aldosterone in obesity occurs through 2 pathways: 1- renin-angiotensin system activation stimulates aldosterone secretion from the adrenal cortex and the adipocytes (Faulkner et al., 2018). 2-leptin directly stimulates adrenal cortical cells (Faulkner et al., 2018). Natriuretic peptides reduce aldosterone levels but in obesity, there is increased neprilysin activity that curtails their impact on reducing aldosterone secretion (Wang et al., 2004).
Hyperaldosteronism also stimulates the accumulation and inflammation of EAT leading to increased loco-regional and systemic inflammation (Iacobellis et al., 2016; Packer, 2018b).
Obesity and Low-Grade Systemic Inflammation
White adipose tissue (AT) accumulates in multiple depots. The subcutaneous depot accounts for around 80% of the total AT (Chait and den Hartigh, 2020). Visceral and other ectopic AT depots (EAT, perivascular, hepatic pancreas renal, and skeletal muscle) accounts for the remaining 20% (Chait and den Hartigh, 2020). Visceral AT refers to the intra-abdominal accumulation of mesenteric and omental AT that can be measured by single-slice CT at the level of L4–L5 or the umbilicus and by multiple slice imaging by MRI (Le Jemtel et al., 2018).
Weight gain leads to AT accumulation through adipocyte hypertrophy or hyperplasia. While expanding VAT becomes dysfunctional and inflamed thereby promoting low-grade systemic inflammation (Lumeng et al., 2007). Increasing BMI correlates with a circulating level of inflammatory markers like C-reactive protein (CRP), interleukin (IL) -6, P selectin, vascular cell adhesion molecule 1, plasminogen activator inhibitor 1, and tumor necrotic factor-alpha (TNF-α) (Osborn and Olefsky, 2012; McNelis and Olefsky, 2014). However, circulating inflammatory markers do not reliably reflect the degree of VAT and systemic inflammation (Le Jemtel et al., 2018).
After undergoing hypertrophy, VAT shifts from an anti-inflammatory state that facilitates AT angiogenesis and lipid storage to a pro-inflammatory state with production of monocyte chemoattractant protein- (MCP1), C-X-C motif chemokine 12 leukotriene B4, and colony-stimulating factor 1 that promote proliferation of classically activated macrophages and macrophages AT infiltration (McLaughlin et al., 2017; Reilly and Saltiel, 2017).
Adipogenesis modulates the AT remodeling process and hypoxia is the trigger behind angiogenesis, extracellular matrix remodeling, and inflammation (Crewe et al., 2017; Vishvanath and Gupta, 2019). Inflammatory VAT mediates the production of reactive oxygen species (ROS) and low nitric oxide (NO) that induce mitochondrial dysfunction and activate Nod-like receptor protein 3 (NLRP3) inflammasome (Abad-Jimenez et al., 2020) (Figure 1).
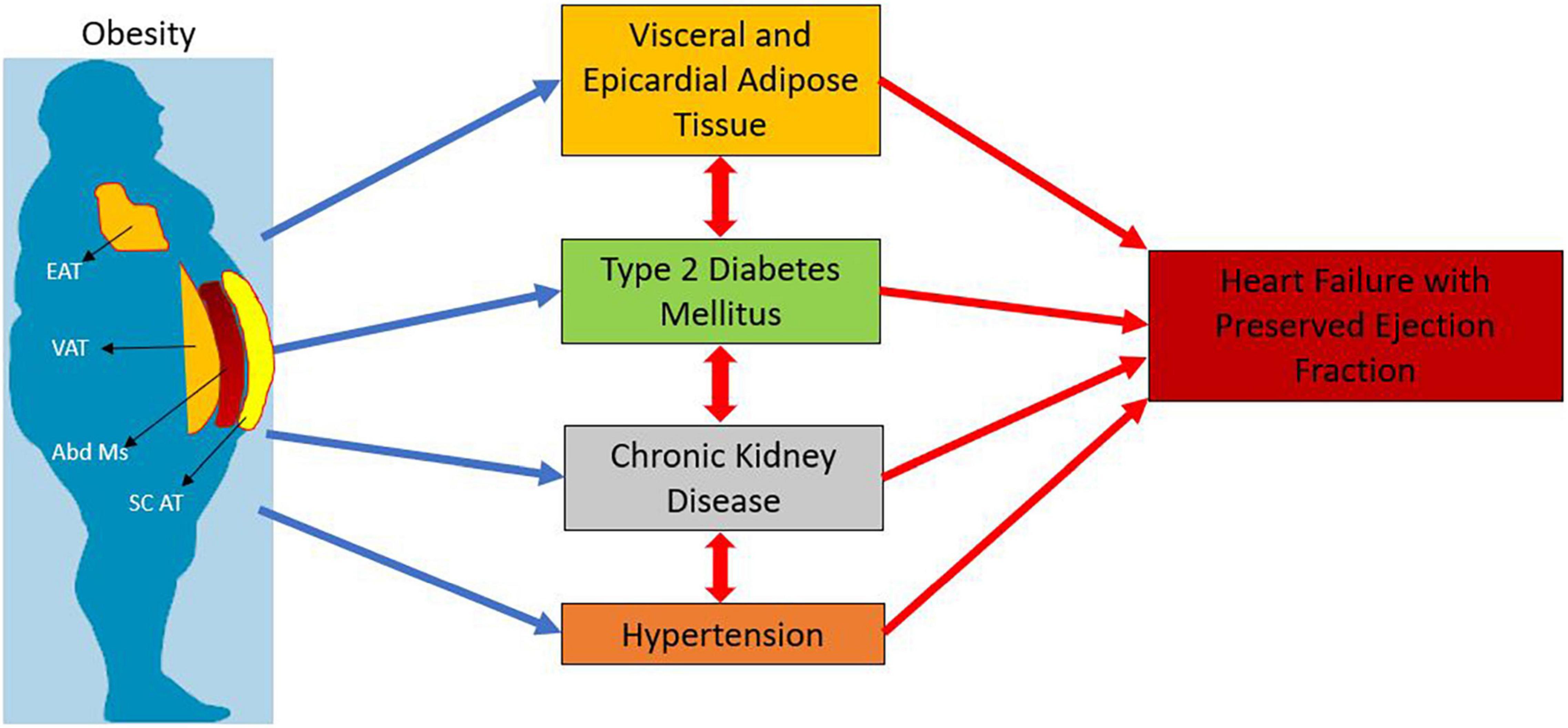
Figure 1. Interplay of obesity and type 2 diabetes with heart failure with preserved ejection fraction. EAT: Epicardial Adipose Tissue; VAT: Visceral Adipose Tissue; Abd Ms : Abdominal Muscles; SC AT : Subcutaneous Adipose Tissue.
Low-Grade Inflammation and Microvascular Dysfunction
Low-grade systemic inflammation worsens cardiovascular diseases (Dhorepatil et al., 2019; Ghoneim et al., 2020a,b). It triggers/heightens an endothelial inflammatory response in the coronary microvasculature (Paulus and Tschope, 2013). In turn, inflammation of the coronary microvascular endothelium alters cardiomyocyte elasticity/function and increases myocardial deposition of collagen that impairs myocardial relaxation and enhances myocardial fibrosis resulting in LVDD and HFpEF (Franssen et al., 2016). Endothelial adhesion molecules enable the infiltration of inflammatory cells that generate hydrogen peroxide (H2O2). High oxidative stress uncouples NO synthase (eNOS), reduces NO availability, and decreases soluble guanylate cyclase (sGC) stimulation that lowers the activity of cyclic guanosine monophosphate (cGMP) and protein kinase G (PKG). Low PKG activity leads to cardiomyocytes hypertrophy and decreases titin phosphorylation that increases LV stiffness (Franssen et al., 2016).
Microvascular inflammation is associated with increased production of inducible NOS (iNOS that reduces the protein unfolded response (Paulus, 2020). Suppression of the unfolded protein response may lead to interstitial accumulation of destabilized protein (Paulus, 2020). Microvascular inflammation with macrophages and secretion of transforming growth factor-beta (TGF) results in LV deposition of high tensile collagen (Paulus, 2020).
Lastly, microvascular rarefaction and Sirtuin 3 (SIRT 3) dependent defect in the endothelial cell metabolic programing and angiogenesis may affect the progression of perivascular and myocardial fibrosis in HFpEF (Zeng and Chen, 2019) (Figure 1).
Adipocyte Dysfunction and Heart Failure With Preserved Ejection Fraction
The role of adipocyte dysfunction in the development of obese-HFpEF is still evolving. Adipocyte homeostasis is maintained by the modulation of pro-inflammatory and anti-inflammatory cytokines. Obesity leads to an excess of pro-inflammatory cytokines and adipokine dysregulation. Adipose tissue exerts an endocrine effect via adipokines. Adipocyte dysfunction caused by obesity leads to alteration in adipokine levels that promotes LV remodeling and ultimately, HfpEF (Berezin et al., 2020). Elevated leptin levels in obesity are associated with cardiac/renal fibrosis (Packer, 2018a) and increased aldosterone production and sodium retention.
Low adiponectin levels in obesity contribute to an increase in the risk for cardiovascular (CV) disease (Shibata et al., 2009). Adiponectin levels are reduced HFpEF and elevated in HFrEF. In vitro adiponectin has multiple beneficial effects such as stimulation of AMP-activated protein kinase (AMPK)-dependent and extracellular-signal-regulated kinase (Barouch et al., 2003) signaling in cardiac myocytes and endothelial cells. Adiponectin reduces LVH and fibrosis, activates endothelial nitric oxide synthase system to and increases NO production (Kobayashi et al., 2004; Ouchi et al., 2004). These beneficial effects have led to an increasing interest in adiponectin as a therapeutic target (Achari and Jain, 2017).
Resistin is an adipocytokine secreted in macrophages by pro-inflammatory cytokines (Lau et al., 2017). Increased resistin levels promote microvascular inflammation, endothelial dysfunction, and vascular smooth muscle proliferation (Acquarone et al., 2019). In elderly patients without HF, serum levels of resistin predict incident HFpEF and HFrEF (Butler et al., 2009). Resistin levels are elevated in patients with HF, but it does not independently predict an adverse outcome (Brankovic et al., 2018). The roles of visfatin, omentin, and other adipocytokines are less well established and an area of active research (Berezin et al., 2020).
Visceral Adipose Tissue and Heart Failure With Preserved Ejection Fraction
Accumulation of VAT when obesity worsens plays a major role in the development and progression of cardiometabolic conditions. In T2D, VAT is a strong predictor of insulin resistance (Lebovitz and Banerji, 2005) and increased cardiometabolic risk (Rawshani et al., 2020). The inability of the body to cope with unrestricted energy intake leads to VAT expansion that mediates most of the detrimental impact of obesity on clinical outcomes.
In the Multi-Ethnic Study of Atherosclerosis (MESA) (Rao et al., 2018), patients with increased VAT had an independently increased risk of incident HFpEF hospitalization (HR 2.24; 95% C.I. 1.44–3.49). Subcutaneous AT (Sc AT) was not associated with HFpEF. Both VAT and EAT were associated with incident HFpEF hospitalization in the Jackson Heart Study population of African Americans (Rao et al., 2021). Epicardial AT was the only significant variable which predicted all-cause mortality and there was a trend toward increased all-cause mortality seen in VAT (Rao et al., 2021). There was no significant trend seen with S c. AT (Rao et al., 2021) (Table 2). These findings point toward the additive effects of VAT and EAT in the obese-HFpEF phenotype.
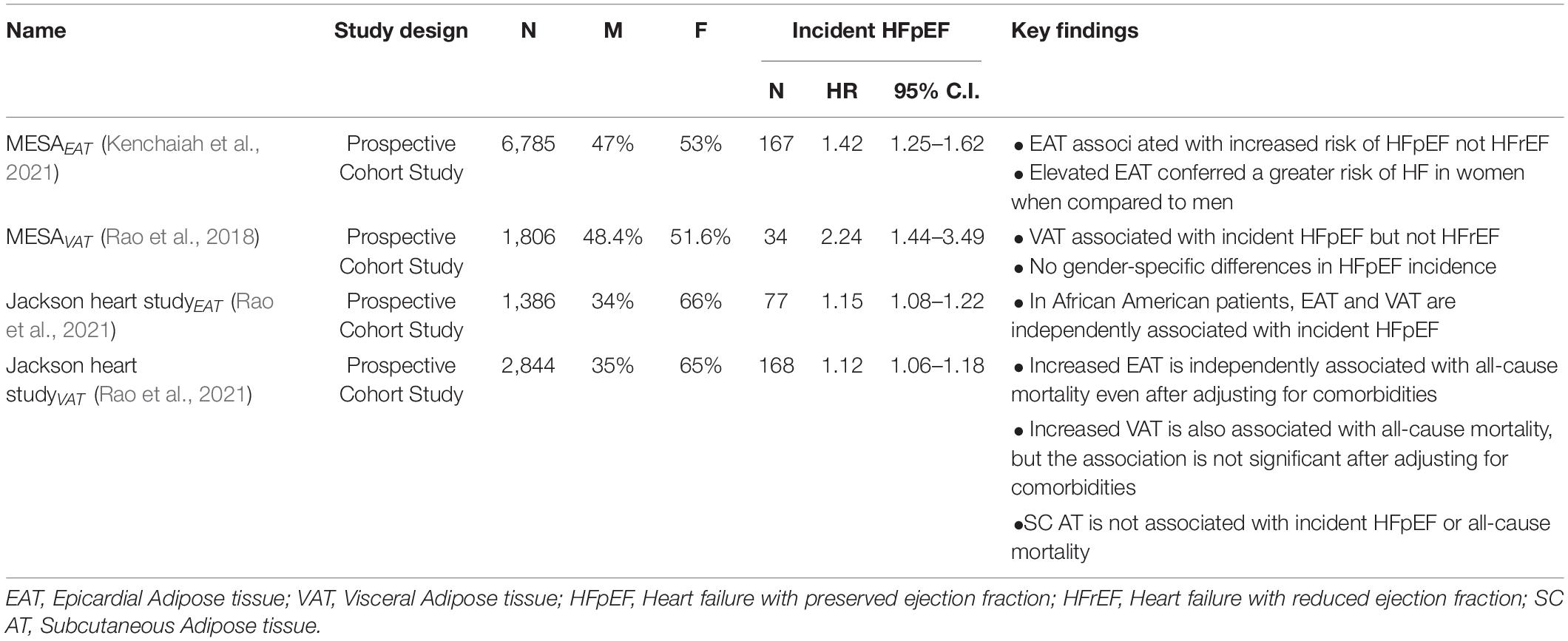
Table 2. Relationship of visceral and epicardial adipose tissue with incident heart failure with preserved ejection fraction.
In patients with obese-HfpEF, VAT accumulation is associated with LVDD and positively correlates with increased LV mass (Abbasi et al., 2015), sphericity, and lower end-diastolic volumes (Neeland et al., 2013). Effects of VAT are also gender specific, with women at baseline tending to have higher VAT% and in HFpEF having worse hemodynamics (Sorimachi et al., 2021). Women with increased VAT and HFpEF have higher exercise-induced LV filling pressures compared with their counterparts with lesser VAT (Sorimachi et al., 2021).
Pericardial/Epicardial Adipose Tissue and Heart Failure With Preserved Ejection Fraction
Increased pericardial/EAT is independently associated with both obesity and T2D (Yafei et al., 2019). EAT is twice as metabolically active as normal white AT and is involved a great degree of lipolysis and free fatty acid release (FFA) (Marchington et al., 1989). Excess circulating FFA levels lead to increased cardiac TG deposition. As EAT directly lies on the myocardium, FFAs released by EAT may have a direct effect on the myocytes and coronaries due to a complete lack of a fibrous fascial layer between the two. A large release of FFA may lead to cardiac lipotoxicity (Iacobellis et al., 2011).
Patients with increased EAT (measured on computed tomography; CT) have increased LV mass index (LVMI), large left atrial size (LA), and high E/e’ velocity by echocardiography (Butler et al., 2009; Brankovic et al., 2018; Acquarone et al., 2019). The association between EAT and LV parameters persist upon adjusting for obesity markers (BMI, waist circumference), and traditional CV risk factors (Kim et al., 2021). Epicardial AT may increase the myocardial fat content and interstitial fibrosis that likely affects myocardial contractility as evidenced by reduced global longitudinal strain (Ng et al., 2018). Elevated EAT also results in reduced peak VO2 consumption and peripheral extraction in patients with HfpEF, indicating a worse hemodynamic profile in these patients (Pugliese et al., 2021b).
Finally, in a recent analysis of MESA, EAT also was associated with an increased risk of incident HF (Kenchaiah et al., 2021). High EAT volumes defined as > 70 cm3 for women and > 120 cm3 for men correlated with a twofold increased incidence of HF in women and 53% higher risk in men. Increased EAT predominantly enhanced the risk of HFpEF (p < 0.001) and not HFrEF (p.31) (Table 2).
Role of Chronic Kidney Disease in Heart Failure With Preserved Ejection Fraction
Nearly 50% of patients with HFpEF have chronic kidney disease (CKD) (Redfield et al., 2003; Yancy et al., 2006). The etiology of CKD is multifactorial in HFpEF (van de Wouw et al., 2019). Comorbidities and HF contribute to microvascular dysfunction that causes and perpetuates both renal dysfunction and LV remodeling (van de Wouw et al., 2019). Chronic kidney disease is associated with premature vascular aging (Laurent et al., 2006) leading to macrovascular and microvascular dysfunction. Advanced atherosclerosis and arteriosclerosis worsen HTN that increases LV workload and exacerbates LVH and LVDD (Borlaug and Kass, 2011). Arteriosclerosis also leads to pulsatility (Mitchell, 2008) in the coronary microvascular bed that promotes microvascular disruption and CMD (Safar et al., 2015; van de Wouw et al., 2019). At a molecular level, CKD worsens the above mentioned pro-inflammatory pathways leading to increased ROS production, reduced local NO availability, and CMD (Rosner et al., 2012; Paulus and Tschope, 2013).
Obesity itself leads to a glomerulopathy, i.e., obesity-related glomerulopathy (ORG) that is characterized by maladaptive glomerular hypertrophy and focal segmental glomerulosclerosis (D’Agati et al., 2016). Other pathways of obesity-related CKD involve alteration of adipokines (Briffa et al., 2013), activation of Renin-Angiotensin-Aldosterone System (RAAS) (Upadhya et al., 2020), and ectopic lipid accumulation within the kidneys (Escasany et al., 2019) (Figure 2).
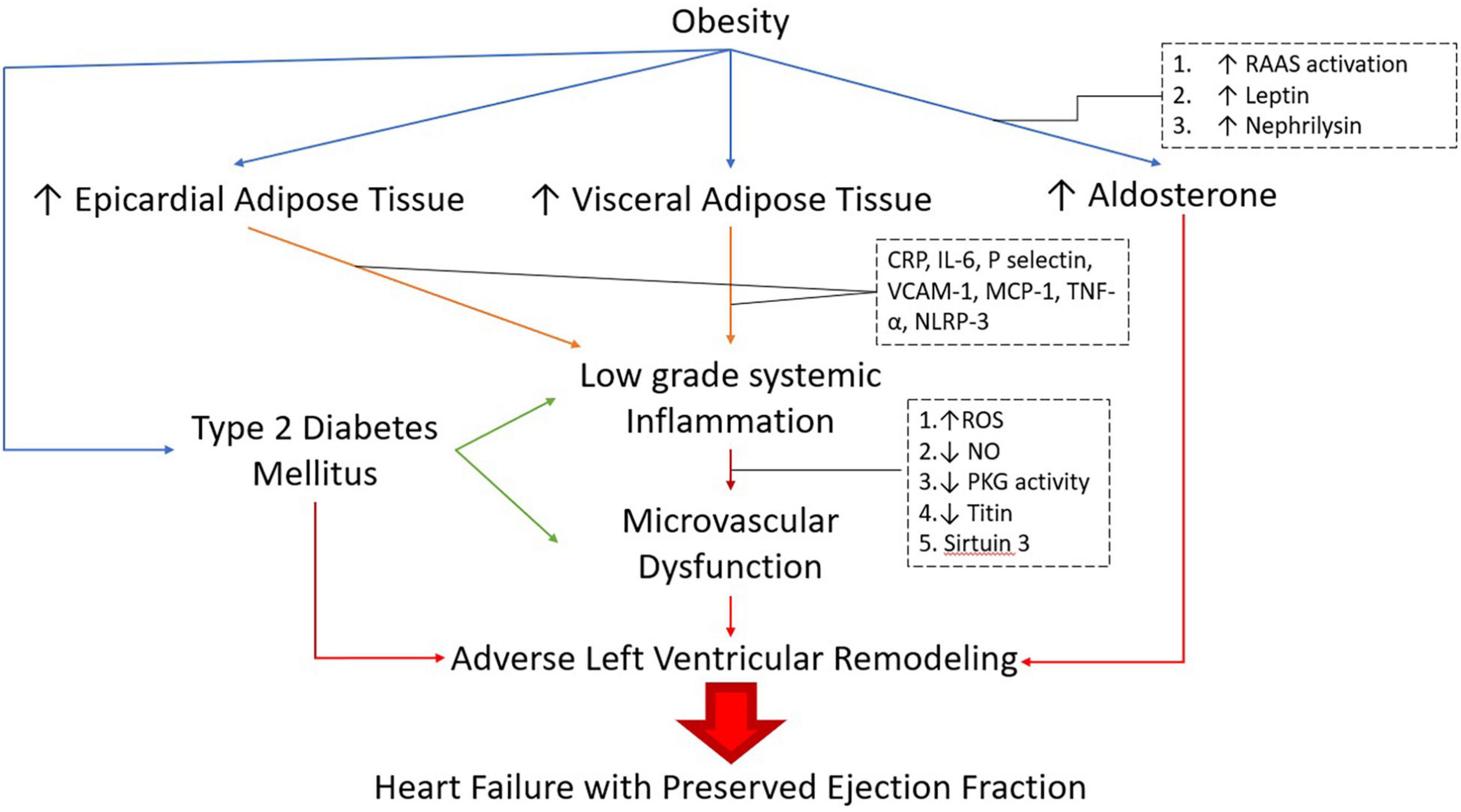
Figure 2. Pathogenesis of obese-DM-HFpEF. RAAS: Renin-Angiotensin-Aldosterone system, CRP: C-Reactive Protein, IL -6: lnterleukin-6, VCAM-1: Vascular cell adhesion molecule 1, MCP-1: monocycte chemoattractant protein-1,TNF-α: Tumor Necrosis Factor alpha, NLRP-3: Nod-Like receptor protein 3, ROS: Reactive oxygen species, NO: Nitric Oxide, PKG: Protein Kinase G.
Management of CKD in patients with obesity and HFpEF is challenging. Targeting the RAAS showed promise in retrospective studies with a greater reduction of proteinuria seen in obese than non-obese individuals (Praga et al., 2001; Mallamaci et al., 2011; Tsuboi et al., 2013). However, there is still resistance in initiating RAAS inhibitors due to the fear of downstream CKD progression and hyperkalemia.
Weight loss improves proteinuria and has a favorable effect on the estimated glomerular filtration rate (Saiki et al., 2005; Shen et al., 2010; Friedman et al., 2013). Bariatric surgery markedly reduces proteinuria (Fowler et al., 2009; Huan et al., 2009). However, bariatric surgery is associated with long-term renal complications like nephrolithiasis and oxalate nephropathy (Turgeon et al., 2012; Lieske et al., 2015).
Linking Diabetes Mellitus, Obesity, and Heart Failure With Preserved Ejection Fraction: A Clinical Perspective
Besides T2D macrovascular complications, the direct effects of T2D on the myocardium have received increased attention over the last decade (Jia et al., 2018).
At a molecular level, patients with T2D-HFpEF have increased t-tubule density and lower collagen deposition when compared with HFrEF. Patients with T2D-HFpEF also have impaired diastolic calcium homeostasis including reduced sarco/endoplasmic reticulum Ca2+-ATPase activity indicating a different pathophysiological process when compared to non-T2D HFpEF (Frisk et al., 2021).
Though BMI is a poor marker of VAT and EAT (Le Jemtel et al., 2018), elevated BMI indirectly suggests a high prevalence of both. MESA, BMI, and VAT was significantly elevated in patients with incident HFpEF (29.9 vs. 27.8, p 0.01; 230.7 cm3 vs. 162.6 cm3 p < 0.001, respectively) (Rao et al., 2018). Patients with EAT have elevated BMIs and increased VAT, further linking BMI as an indirect measure of VAT and EAT (Kenchaiah et al., 2021).
In HFpEF, patients with T2D commonly have higher BMIs than their non-diabetic counterparts. In an ancillary study of the Phosphodiesterase-5 inhibition to improve clinical status and Exercise capacity in Diastolic HF (RELAX) trial (Redfield et al., 2013; Reddy et al., 2019), BMI were 37.1 and 30.7 kg/m2 vs. 30.7 kg/m2 in patients with and without T2D. Unsurprisingly, patients with T2D-HFpEF had more severe initial presentations and more frequent hospitalizations. They also had more LVH and higher filling pressures (E/e’) by echocardiography. Cardiac Magnetic Resonance Imaging (CMR) reveals a trend toward higher LV Mass and higher levels of fibrosis in T2D than patients with non-T2D HFpEF (Lejeune et al., 2021). Patients with diabetes had high BMIs (31 vs. 27 kg/m2, p 0.001) and had an increased rate of mortality and hospitalization for HF (HR 1.72 95% C.I. 1.1–2.6, p 0.011).
A common feature of both T2D and HFpEF is exercise intolerance (EI) (Upadhya et al., 2015a; Pandey et al., 2021). The cause of exercise training (ET) is multifactorial (Pandey et al., 2021) in T2D and HFpEF with impairment in cardiac performance (Pugliese et al., 2021a) and skeletal muscle metabolism/perfusion (Espino-Gonzalez et al., 2021). Obesity and T2D significantly contribute to EI in HFpEF. Obesity-induced sarcopenia exacerbates muscle mass loss due to aging and worsens EI (Upadhya et al., 2015b). Type 2 diabetes lowers exercise capacity through impaired cardiac energetics (Levelt et al., 2016) and skeletal muscle oxygen extraction and metabolism (Nesti et al., 2020). Clinically, EI leads to poor quality of life (Salzano et al., 2021), frequent re-hospitalizations, and early mortality in T2D and HFpEF (Pugliese et al., 2020). Thus, reversal of EI is an important therapeutic target in patients with T2D-HFpEF. improves peak VO2 (Demopoulos et al., 1997) and quality of life in patients with systolic dysfunction (Fleg et al., 2015). In HFpEF, ET improves peak VO2 and quality of life independent of improvement in cardiac systolic or diastolic function (Pandey et al., 2015b). The effects of ET on skeletal muscle perfusion and metabolism warrant investigation in T2D-HFpEF.
Patients with T2D HFpEF have an increased risk of mortality (Yusuf et al., 2003; MacDonald et al., 2008; Massie et al., 2008). In the I-PRESERVE trial, patients with T2D also had a higher prevalence of coronary artery disease (CAD) and percutaneous coronary intervention/coronary artery bypass graft (PCI/CABG) indicating an increased risk of macrovascular disease (Massie et al., 2008). Of note, patients with and without T2D had similar LVEFs, and patients with T2D had significantly greater LV Mass and DD despite having a lower prevalence of hypertension (HTN) (Kristensen et al., 2017). The greater LV remodeling in patients with T2D was likely related to greater BMIs (31 + /6 vs. 29 ± 5 kg/m2, respectively). Moreover, in the T2D cohort, 52% of the patients had a BMI > 30 kg/m2 vs. 38% of the patients in the non-T2D cohort (Kristensen et al., 2017).
Lastly, the duration and severity of T2D in HFpEF affect outcomes. In a sub-analysis of the Treatment Of Preserved Cardiac function heart failure with an Aldosterone Antagonist (TOPCAT) trial (Pitt et al., 2014), patients from the Americas (n = 1765 patients) were analyzed into 3 subgroups, patients with T2D treated with insulin (ITDM, n = 390 patients), patients with T2D not on insulin (NITDM, n = 406 patients), and patients withoutT2D (n = 969 patients) (Huynh et al., 2019). The ITDM cohort had a longer duration of T2D and higher BMI when compared with NITDM and non-T2D patients. The ITDM cohort also had worse LVDD and increased LV Mass. Unsurprisingly, ITDM patients had the worst outcome profile with a 50% increase in all-cause and CV mortality that was elevated when compared to NITDM patients alone. The risk of adverse outcomes was similar in NITDM and non-T2D. Thus, obesity and T2D are additive risk factors in patients with T2D-HFpEF (Huynh et al., 2019). However, obesity directly impacts the severity of T2D as well as HFpEF. Obesity clearly worsens outcomes in T2D. Increasing insulin resistance leads to increased production of insulin from pancreatic β-cells that eventually cannot meet glycemic demands. The ectopic pancreatic fat deposition also contributes to β-cell dysfunction and thereby to T2D (Ishibashi et al., 2020). Thus, treatment of the obese HFpEF phenotype needs to target obesity and T2D.
Therapeutic Advances for Obese-T2D-Heart Failure With Preserved Ejection Fraction Phenotype
Targeting Coronary Microvascular Dysfunction
Therapy in HfpEF, specifically in the obese-T2D-HfpEF phenotype is searching for novel therapeutic approaches. Targeting CMD is an innovative approach but so far results have not been promising (Redfield et al., 2013, 2015; Borlaug et al., 2018). Increasing NO availability and enhancing cGMP have been disappointing. In multiple trials looking at phosphodiesterase inhibitors and oral nitrates, increasing NO has failed to improve quality of life or exercise capacity in HfpEF. Most trials recruited patients with high BMI, severe LV concentric remodeling, and advanced LVDD at baseline. Hence, extensive collagen deposition and LV stiffness may account for the neutral findings (Samson and Le Jemtel, 2021).
Given the neutral findings of the above trials, increasing NO may not be the most effective way to remedy endothelial dysfunction. Vericiguat, an sGC stimulator bypasses NO production and can stimulate the production of cGMP that as previously mentioned prevents further LV remodeling. Vericiguat did not improve the primary endpoints of NT-ProBNP levels and left atrial volumes but did improve quality of life in a clinical trial (Pieske et al., 2017). A lower BMI than in prior trials (∼30 kg/m2 in all groups) hints at a low prevalence of VAT and EAT in this population.
Regardless, despite the high prevalence of CMD in HFpEF (Shah et al., 2018), targeting CMD does not seem to be therapeutically fruitful.
Targeting Mineralocorticoid Receptors
Sodium retention secondary to increased aldosterone production plays a major role in obese-HFpEF. It accounts for the responsiveness to diuretics but excess natriuresis can accelerate renal dysfunction (Gupta et al., 2012). Experimentally, MRAs reduce oxidative stress (Gorini et al., 2019), cardiac inflammation (Tesch and Young, 2017) and fibrosis (Borlaug and Kass, 2011), and improve diastolic LV filling pressures (Pandey et al., 2015a). Spironolactone improved LV filling pressures and exercise capacity in patients with HFpEF. In T2D, spironolactone improves insulin resistance (Olatunji et al., 2017) and albuminuria (Makhlough et al., 2014; Selvaraj et al., 2018). The effects on diabetic nephropathy are mixed with delayed progression in type 1 (Schjoedt et al., 2005) but not T2D (Tofte et al., 2020).
In the TOPCAT trial, patients with obesity and T2D benefited the most from spironolactone (Cohen et al., 2020). Maximum reduction of the primary endpoint (All-cause death and HF hospitalization) was noted in patients with a BMI > 33 kg/m2 (Elkholey et al., 2021). A similar benefit was seen in patients with high waist circumference (HWC) (Men > 102 cm and women > 88 cm) indicating that spironolactone was more beneficial in patients with increased VAT. The beneficial effect of spironolactone in obese and HWC patients is a reduction in HF hospitalization. Quantification of VAT may help better risk stratify patients who benefit from MRAs. The promising pre-clinical favorable metabolic effects of finerenone (Marzolla et al., 2020) suggest that MRAs may benefit adjunct obese-T2D-HFpEF phenotype.
Targeting Obesity and Diabetes Mellitus
The pathophysiology of HFrEF highlights worsening LVEF due to the progression of eccentric LV remodeling which leads to symptom deterioration and eventual patient decline. The success of neurohormonal modulation in HFrEF is based on the ability of pharmacotherapy and device therapy ability to reserve LV eccentric remodeling. In contrast, neurohormonal modulation does not reverse LV concentric remodeling in HFpEF (Lam et al., 2018; Upadhya and Kitzman, 2020). Hence, the most effective therapies in HFrEF do not lower mortality in HFpEF (Massie et al., 2008; Pitt et al., 2014; Solomon et al., 2019). As previously mentioned, obesity, specifically VAT and EAT, drive LV remodeling (Yan et al., 2020). Obesity leads to T2D hence aggressive weight management will benefit patients with HFpEF and T2D.
Treating obesity is complex and involves lifestyle/behavioral modifications (LBM) as the first step, then pharmacotherapy and bariatric surgery as the next step. Newer advances in anti-diabetic medications have led to a successful strategy of targeting obesity and HF in patients with T2D changing the management paradigm for these patients.
Glucagon-Like Peptide-1 Analogs
Glucagon-like peptide-1 (GLP-1) analogs are coming back in T2D and recent trials demonstrate efficacy in CV disease (Verma et al., 2018) and weight loss (Wilding et al., 2021). GLP-1 receptors are expressed in various organs like the heart, kidney, and pancreas. GLP-1 agonists reduce ROS production by the endothelium and systemic inflammation. It may contribute to their beneficial effect on LV diastolic function studies (Nguyen et al., 2018; Bizino et al., 2019).
GLP-1 agonists have also been shown to be effective in reducing EAT which is a target in HFpEF (Dutour et al., 2016; Iacobellis et al., 2017). In 95 patients with T2D, liraglutide plus metformin was associated with a 36% reduction in EAT when compared with metformin alone (Iacobellis et al., 2017). In 44 patients, exenatide was also associated with a ∼10% reduction in EAT when compared to 1.2% in the standard of care arm (Dutour et al., 2016).
Reduction of adiposity is an essential therapeutic aim in obese-T2D-HFpEF. Before recent semaglutide trials, pharmaceutical agents approved for weight loss by the Food and Drug Administration (FDA) at best resulted in 7% weight loss (Srivastava and Apovian, 2018). GLP-I analogs have been shown to cause an average weight loss of 2.9 kg 95% C.I. 2.2–3.6 kg in 21 trials and 6,411 patients (Vilsboll et al., 2012). The finding of the recent Four Semaglutide Treatment Effect in People with Obesity (STEP 1–4) trials was more promising (Davies et al., 2021; Rubino et al., 2021; Wadden et al., 2021; Wilding et al., 2021). Subcutaneous semaglutide was compared with intensive LBM in successive steps in patients with and without T2D. Semaglutide reduced body weight by 10% in 75% of patients, 15% in 56% of patients, and 20% in 36% of patients. In contrast in a veteran’s affairs study (Maciejewski et al., 2016), patients with gastric bypass (GB) reduced weight by 27.5% (95% C.I. 23.8–31.2%), sleeve gastrectomy (SG) by 17.8% (95% C.I. 9.7–25.9%) underlining the magnitude of weight loss achieved by semaglutide. The cardiovascular and outcome effects of GLP-1 analogs need to be investigated in patients with obese-T2D-HFpEF.
Sodium-Glucose Co-transporter 2 Inhibitors
Sodium-glucose co-transporter 2 inhibitors (SGLT-2i) are extremely beneficial in HFrEF (McMurray et al., 2019; Packer et al., 2020). The actions are multiple (Lopaschuk and Verma, 2020) and include weight loss, increased diuresis, improved endothelial function, reduced inflammation, and cardiac remodeling prevention. Weight loss is modest (Mean 1.5–2 kg) (Liu et al., 2015; Maruthur et al., 2016; Zaccardi et al., 2016), and slightly greater in patients with T2D than those non-T2D (Pereira and Eriksson, 2019). Weight-loss lasts up to 4 years (Del Prato et al., 2015) and is dose-dependent (Cai et al., 2018). However, the weight loss induced by glycosuria leads to a compensatory increase in appetite and thereby caloric intake (Ferrannini et al., 2015). Thus, SGLT-2i must be combined with other medications for a lasting effect on weight (Leibel et al., 1995). SGLT-2i reduces perivascular AT thereby lowering leptin release and loco-regional inflammation (Iborra-Egea et al., 2019). In patients with T2D with CAD, SGLT2i reduces EAT, TNF-α, and plasminogen activator inhibitor-1 (Sato et al., 2018). SGLT2i effect on TNF-α leads to the improved endothelial secretion of NO and reduced CMD (Juni et al., 2019). Several experimental models have demonstrated the benefits of SGLT-2i on cardiac remodeling (Lambers Heerspink et al., 2013; Verma et al., 2016; Connelly et al., 2019). In a randomized clinical trial, patients treated with empagliflozin had a significantly lower LV mass index when compared with placebo at 6 months (Connelly et al., 2019). Reduced cardiac fibrosis and inhibition of the mammalian target of rapamycin pathway may alleviate LV remodeling (Lee et al., 2019; Kang et al., 2020).
Recently, in the empagliflozin in HFpEF (EMPEROR-PRESERVED) trial (Anker et al., 2021), empagliflozin did reduce the combined endpoint of CV death or hospitalization but did not reduce significantly reduce CV death alone. The results were underwhelming for patients with typical HFpEF as the benefits were mostly noted in patients with LVEF < 50% compared to LVEF > 60% i.e., HF mid-range-EF. Of note, patients with BMI > 30 kg/m2 (HR 0.85 C.I 0.7–1.03) did not derive as much benefit as those with BMI < 30 kg/m2 (HR.7 C.I 0.62–0.88). The findings of the awaited dapagliflozin trials (Solomon et al., 2021) may strengthen EMPEROR-HF (Packer et al., 2020).
Role of Metabolic Surgery
Metabolic surgery, specifically GB, is the most effective intervention for weight loss. It prevents the occurrence of HFpEF in patients with severe obesity. However metabolic surgery has complications and requires careful and long-term monitoring. Compared with LBM, metabolic surgery results in a greater weight loss and a 23% risk reduction in HF (Sundstrom et al., 2017). Exercise-induced weight loss, an integral part of LBM, reduces EAT and thereby, the incidence of HF (Kim et al., 2009).
Weight loss has not been so far a therapeutic target in the management of HFpEF. Of note, regular aerobic exercise training is an arduous undertaking for severely or morbidly obese patients. Weight loss improved LV mass index (LVMI) in MESA with every 5% weight loss being associated with a 1.3% decrease in LVMI and LV mass-to volume ratio (p < 0.0001) measured by cardiac MRI (Shah et al., 2015). The Utah obesity study examined patients undergoing metabolic surgery and compared them with control patients with morbid obesity who did not undergo surgery. All patients underwent 2D echocardiography, and close monitoring (Adams et al., 2005). Mean baseline BMI was 48 and 44 kg/m2 in metabolic surgery and control patients. At 2 years, BMI was 32 and 44 kg/m2 in metabolic surgery and control patients. Patients who underwent metabolic surgery had significant reductions in LVMI and increases in right ventricular (RV) fractional area change at 2 years (Owan et al., 2011). Smaller studies reported similar findings (Karason et al., 1997; Ippisch et al., 2008; Aggarwal et al., 2016). The effects of caloric restriction and/or exercise were reported in older patients with obesity and HFpEF. After 20 weeks, body weight decreased by 4 kg (3%) in the exercise group, 7 kg (7%) in the caloric restriction group, and 11 kg (10%) in the combined group while it increased by 1 kg (1%) in the control group. Both caloric restriction and exercise independently improved exercise capacity [as measured by peak oxygen consumption (VO2)] and the effects of caloric restriction and exercise were additive. However, there was no difference in the quality of life (as reported on the Minnesota Living with Heart Failure Questionnaire) or LVMI in either group. High intensity and moderate continuous exercise regimens do not significantly improve in peak VO2 compared with guideline-directed exercise regimens (Mueller et al., 2021) (Table 3).
Adherence to LBM is strongly recommended for patients with obesity, T2D, and HFpEF and physicians need to be pro-active to effectively help patients lose weight. Metabolic surgery though beneficial is marred by strict indications (Ayinapudi et al., 2020) and multiple complications (Ma and Madura, 2015; Surve et al., 2018). Randomized controlled trials of metabolic surgery and LVM are clearly needed in patients with severe and morbid obesity with HFpEF.
Conclusion
Heart failure with preserved ejection fraction remains a therapeutic conundrum. The obese-T2D phenotype has distinct pathophysiology encompassing inflammation, CMD, and LV remodeling. Obesity is at the crux of the pathophysiology and weight reduction must be prioritized in these patients. Quantification of VAT and EAT may better help risk-stratify patients at greatest risk of HFpEF and further studies are needed to assess their impact on management. Mineralocorticoid receptor antagonists and anti-diabetic agents like semaglutide and SGLT-2 inhibitors hold promise as useful adjunct agents for obese-T2D-HFpEF and should be studied in randomized clinical trials. Lifestyle and behavioral modifications should be offered to all patients and metabolic surgery may be considered in patients with BMI > 35 kg/m2.
Author Contributions
AD-P and TL contributed to conception and design of the article, wrote the manuscript, and drafted the figures. TT and RS edited certain sections of the manuscript. All authors contributed to manuscript revision, read, and approved the submitted version.
Conflict of Interest
The authors declare that the research was conducted in the absence of any commercial or financial relationships that could be construed as a potential conflict of interest.
Publisher’s Note
All claims expressed in this article are solely those of the authors and do not necessarily represent those of their affiliated organizations, or those of the publisher, the editors and the reviewers. Any product that may be evaluated in this article, or claim that may be made by its manufacturer, is not guaranteed or endorsed by the publisher.
References
Abad-Jimenez, Z., Lopez-Domenech, S., Diaz-Rua, R., Iannantuoni, F., Gomez-Abril, S. A., Perianez-Gomez, D., et al. (2020). Systemic Oxidative Stress and Visceral Adipose Tissue Mediators of NLRP3 Inflammasome and Autophagy Are Reduced in Obese Type 2 Diabetic Patients Treated with Metformin. Antioxidants 9:892. doi: 10.3390/antiox9090892
Abbasi, S. A., Hundley, W. G., Bluemke, D. A., Jerosch-Herold, M., Blankstein, R., Petersen, S. E., et al. (2015). Visceral adiposity and left ventricular remodeling: the Multi-Ethnic Study of Atherosclerosis. Nutr. Metab. Cardiovasc. Dis. 25, 667–676. doi: 10.1016/j.numecd.2015.03.016
Achari, A. E., and Jain, S. K. (2017). Adiponectin. Int. J. Mol. Sci. 18:1321. doi: 10.3390/ijms18061321
Acquarone, E., Monacelli, F., Borghi, R., Nencioni, A., and Odetti, P. (2019). Resistin: a reappraisal. Mech. Ageing Dev. 178, 46–63. doi: 10.1016/j.mad.2019.01.004
Adams, T. D., Avelar, E., Cloward, T., Crosby, R. D., Farney, R. J., Gress, R., et al. (2005). Design and rationale of the Utah obesity study. A study to assess morbidity following gastric bypass surgery. Contemp. Clin. Trials 26, 534–551. doi: 10.1016/j.cct.2005.05.003
Aggarwal, R., Harling, L., Efthimiou, E., Darzi, A., Athanasiou, T., and Ashrafian, H. (2016). The Effects of Bariatric Surgery on Cardiac Structure and Function: a Systematic Review of Cardiac Imaging Outcomes. Obes. Surg. 26, 1030–1040. doi: 10.1007/s11695-015-1866-5
Altara, R., Giordano, M., Norden, E. S., Cataliotti, A., Kurdi, M., Bajestani, S. N., et al. (2017). Targeting Obesity and Diabetes to Treat Heart Failure with Preserved Ejection Fraction. Front. Endocrinol. 8:160. doi: 10.3389/fendo.2017.00160
Andersson, C., and Vasan, R. S. (2014). Epidemiology of heart failure with preserved ejection fraction. Heart Fail. Clin. 10, 377–388. doi: 10.1016/j.hfc.2014.04.003
Anker, S. D., Butler, J., Filippatos, G., Ferreira, J. P., Bocchi, E., Bohm, M., et al. (2021). Empagliflozin in Heart Failure with a Preserved Ejection Fraction. N. Engl. J. Med. 385, 1451–1461. doi: 10.1056/NEJMoa2107038
Avelar, E., Cloward, T. V., Walker, J. M., Farney, R. J., Strong, M., Pendleton, R. C., et al. (2007). Left ventricular hypertrophy in severe obesity: interactions among blood pressure, nocturnal hypoxemia, and body mass. Hypertension 49, 34–39. doi: 10.1161/01.HYP.0000251711.92482.14
Ayinapudi, K., Samson, R., Le Jemtel, T. H., Marrouche, N. F., and Oparil, S. (2020). Weight Reduction for Obesity-Induced Heart Failure with Preserved Ejection Fraction. Curr. Hypertens. Rep. 22:47. doi: 10.1007/s11906-020-01074-w
Barouch, L. A., Berkowitz, D. E., Harrison, R. W., O’Donnell, C. P., and Hare, J. M. (2003). Disruption of leptin signaling contributes to cardiac hypertrophy independently of body weight in mice. Circulation 108, 754–759. doi: 10.1161/01.CIR.0000083716.82622.FD
Becher, P. M., Lindner, D., Fluschnik, N., Blankenberg, S., and Westermann, D. (2013). Diagnosing heart failure with preserved ejection fraction. Expert Opin. Med. Diagn. 7, 463–474. doi: 10.1517/17530059.2013.825246
Bello, N. A., Cheng, S., Claggett, B., Shah, A. M., Ndumele, C. E., Roca, G. Q., et al. (2016). Association of Weight and Body Composition on Cardiac Structure and Function in the ARIC Study (Atherosclerosis Risk in Communities). Circ. Heart Fail. 9:e002978. doi: 10.1161/CIRCHEARTFAILURE.115.002978
Berezin, A. E., Berezin, A. A., and Lichtenauer, M. (2020). Emerging Role of Adipocyte Dysfunction in Inducing Heart Failure Among Obese Patients With Prediabetes and Known Diabetes Mellitus. Front. Cardiovasc. Med. 7:583175. doi: 10.3389/fcvm.2020.583175
Bickel, C. A., Verbalis, J. G., Knepper, M. A., and Ecelbarger, C. A. (2001). Increased renal Na-K-ATPase, NCC, and beta-ENaC abundance in obese Zucker rats. Am. J. Physiol. Renal Physiol. 281, F639–F648. doi: 10.1152/ajprenal.2001.281.4.F639
Bizino, M. B., Jazet, I. M., Westenberg, J. J. M., van Eyk, H. J., Paiman, E. H. M., Smit, J. W. A., et al. (2019). Effect of liraglutide on cardiac function in patients with type 2 diabetes mellitus: randomized placebo-controlled trial. Cardiovasc. Diabetol. 18:55. doi: 10.1186/s12933-019-0857-6
Borlaug, B. A. (2014). Mechanisms of exercise intolerance in heart failure with preserved ejection fraction. Circ. J. 78, 20–32. doi: 10.1253/circj.CJ-13-1103
Borlaug, B. A. (2020). Evaluation and management of heart failure with preserved ejection fraction. Nat. Rev. Cardiol. 17, 559–573. doi: 10.1038/s41569-020-0363-2
Borlaug, B. A., and Kass, D. A. (2011). Ventricular-vascular interaction in heart failure. Cardiol. Clin. 29, 447–459. doi: 10.1016/j.ccl.2011.06.004
Borlaug, B. A., Anstrom, K. J., Lewis, G. D., Shah, S. J., Levine, J. A., Koepp, G. A., et al. (2018). Effect of Inorganic Nitrite vs Placebo on Exercise Capacity Among Patients With Heart Failure With Preserved Ejection Fraction: the INDIE-HFpEF Randomized Clinical Trial. JAMA 320, 1764–1773. doi: 10.1001/jama.2018.14852
Brankovic, M., Akkerhuis, K. M., Mouthaan, H., Brugts, J. J., Manintveld, O. C., van Ramshorst, J., et al. (2018). Cardiometabolic Biomarkers and Their Temporal Patterns Predict Poor Outcome in Chronic Heart Failure (Bio-SHiFT Study). J. Clin. Endocrinol. Metab. 103, 3954–3964. doi: 10.1210/jc.2018-01241
Briffa, J. F., McAinch, A. J., Poronnik, P., and Hryciw, D. H. (2013). Adipokines as a link between obesity and chronic kidney disease. Am. J. Physiol. Renal. Physiol. 305, F1629–F1636. doi: 10.1152/ajprenal.00263.2013
Butler, J., Kalogeropoulos, A., Georgiopoulou, V., de Rekeneire, N., Rodondi, N., Smith, A. L., et al. (2009). Serum resistin concentrations and risk of new onset heart failure in older persons: the health, aging, and body composition (Health ABC) study. Arterioscler. Thromb. Vasc. Biol. 29, 1144–1149. doi: 10.1161/ATVBAHA.109.186783
Cai, X., Yang, W., Gao, X., Chen, Y., Zhou, L., Zhang, S., et al. (2018). The Association Between the Dosage of SGLT2 Inhibitor and Weight Reduction in Type 2 Diabetes Patients: a Meta-Analysis. Obesity 26, 70–80. doi: 10.1002/oby.22066
Chait, A., and den Hartigh, L. J. (2020). Adipose Tissue Distribution, Inflammation and Its Metabolic Consequences, Including Diabetes and Cardiovascular Disease. Front. Cardiovasc. Med. 7:22. doi: 10.3389/fcvm.2020.00022
Cheng, S., Fernandes, V. R., Bluemke, D. A., McClelland, R. L., Kronmal, R. A., and Lima, J. A. (2009). Age-related left ventricular remodeling and associated risk for cardiovascular outcomes: the Multi-Ethnic Study of Atherosclerosis. Circ. Cardiovasc. Imaging 2, 191–198. doi: 10.1161/CIRCIMAGING.108.819938
Cohen, J. B., Schrauben, S. J., Zhao, L., Basso, M. D., Cvijic, M. E., Li, Z., et al. (2020). Clinical Phenogroups in Heart Failure With Preserved Ejection Fraction: detailed Phenotypes, Prognosis, and Response to Spironolactone. JACC Heart Fail. 8, 172–184. doi: 10.1016/j.jchf.2019.09.009
Connelly, K. A., Zhang, Y., Visram, A., Advani, A., Batchu, S. N., Desjardins, J. F., et al. (2019). Empagliflozin Improves Diastolic Function in a Nondiabetic Rodent Model of Heart Failure With Preserved Ejection Fraction. JACC Basic Transl. Sci. 4, 27–37. doi: 10.1016/j.jacbts.2018.11.010
Cordola Hsu, A. R., Xie, B., Peterson, D. V., LaMonte, M. J., Garcia, L., Eaton, C. B., et al. (2021). Metabolically Healthy/Unhealthy Overweight/Obesity Associations With Incident Heart Failure in Postmenopausal Women: the Women’s Health Initiative. Circ. Heart Fail. 14:e007297. doi: 10.1161/CIRCHEARTFAILURE.120.007297
Crewe, C., An, Y. A., and Scherer, P. E. (2017). The ominous triad of adipose tissue dysfunction: inflammation, fibrosis, and impaired angiogenesis. J. Clin. Invest. 127, 74–82. doi: 10.1172/JCI88883
D’Agati, V. D., Chagnac, A., de Vries, A. P., Levi, M., Porrini, E., Herman-Edelstein, M., et al. (2016). Obesity-related glomerulopathy: clinical and pathologic characteristics and pathogenesis. Nat. Rev. Nephrol. 12, 453–471. doi: 10.1038/nrneph.2016.75
Davies, M., Faerch, L., Jeppesen, O. K., Pakseresht, A., Pedersen, S. D., Perreault, L., et al. (2021). Semaglutide 2.4 mg once a week in adults with overweight or obesity, and type 2 diabetes (STEP 2): a randomised, double-blind, double-dummy, placebo-controlled, phase 3 trial. Lancet 397, 971–984. doi: 10.1016/S0140-6736(21)00213-0
Del Prato, S., Nauck, M., Duran-Garcia, S., Maffei, L., Rohwedder, K., Theuerkauf, A., et al. (2015). Long-term glycaemic response and tolerability of dapagliflozin versus a sulphonylurea as add-on therapy to metformin in patients with type 2 diabetes: 4-year data. Diabetes Obes. Metab. 17, 581–590. doi: 10.1111/dom.12459
Demopoulos, L., Bijou, R., Fergus, I., Jones, M., Strom, J., and LeJemtel, T. H. (1997). Exercise training in patients with severe congestive heart failure: enhancing peak aerobic capacity while minimizing the increase in ventricular wall stress. J. Am. Coll. Cardiol. 29, 597–603. doi: 10.1016/S0735-1097(96)00526-8
Dhorepatil, A., Ball, S., Ghosh, R. K., Kondapaneni, M., and Lavie, C. J. (2019). Canakinumab: promises and Future in Cardiometabolic Diseases and Malignancy. Am. J. Med. 132, 312–324. doi: 10.1016/j.amjmed.2018.10.013
Di Carli, M. F., Janisse, J., Grunberger, G., and Ager, J. (2003). Role of chronic hyperglycemia in the pathogenesis of coronary microvascular dysfunction in diabetes. J. Am. Coll. Cardiol. 41, 1387–1393. doi: 10.1016/S0735-1097(03)00166-9
Dutour, A., Abdesselam, I., Ancel, P., Kober, F., Mrad, G., Darmon, P., et al. (2016). Exenatide decreases liver fat content and epicardial adipose tissue in patients with obesity and type 2 diabetes: a prospective randomized clinical trial using magnetic resonance imaging and spectroscopy. Diabetes Obes. Metab. 18, 882–891. doi: 10.1111/dom.12680
Eguchi, K., Kario, K., Hoshide, S., Ishikawa, J., Morinari, M., and Shimada, K. (2005). Type 2 diabetes is associated with left ventricular concentric remodeling in hypertensive patients. Am. J. Hypertens. 18, 23–29. doi: 10.1016/j.amjhyper.2004.08.024
Eisner, D. A., Caldwell, J. L., Trafford, A. W., and Hutchings, D. C. (2020). The Control of Diastolic Calcium in the Heart: basic Mechanisms and Functional Implications. Circ. Res. 126, 395–412. doi: 10.1161/CIRCRESAHA.119.315891
Elkholey, K., Papadimitriou, L., Butler, J., Thadani, U., and Stavrakis, S. (2021). Effect of Obesity on Response to Spironolactone in Patients With Heart Failure With Preserved Ejection Fraction. Am. J. Cardiol. 146, 36–47. doi: 10.1016/j.amjcard.2021.01.018
Escasany, E., Izquierdo-Lahuerta, A., and Medina-Gomez, G. (2019). Underlying Mechanisms of Renal Lipotoxicity in Obesity. Nephron 143, 28–32. doi: 10.1159/000494694
Espino-Gonzalez, E., Tickle, P. G., Benson, A. P., Kissane, R. W. P., Askew, G. N., Egginton, S., et al. (2021). Abnormal skeletal muscle blood flow, contractile mechanics and fibre morphology in a rat model of obese-HFpEF. J. Physiol. 599, 981–1001. doi: 10.1113/JP280899
Faulkner, J. L., Bruder-Nascimento, T., and Belin de Chantemele, E. J. (2018). The regulation of aldosterone secretion by leptin: implications in obesity-related cardiovascular disease. Curr. Opin. Nephrol. Hypertens. 27, 63–69. doi: 10.1097/MNH.0000000000000384
Ferrannini, G., Hach, T., Crowe, S., Sanghvi, A., Hall, K. D., and Ferrannini, E. (2015). Energy Balance After Sodium-Glucose Cotransporter 2 Inhibition. Diabetes Care 38, 1730–1735. doi: 10.2337/dc15-0355
Fleg, J. L., Cooper, L. S., Borlaug, B. A., Haykowsky, M. J., Kraus, W. E., Levine, B. D., et al. (2015). Blood Institute Working, Exercise training as therapy for heart failure: current status and future directions. Circ. Heart Fail. 8, 209–220. doi: 10.1161/CIRCHEARTFAILURE.113.001420
Fliotsos, M., Zhao, D., Rao, V. N., Ndumele, C. E., Guallar, E., Burke, G. L., et al. (2018). Body Mass Index From Early-, Mid-, and Older-Adulthood and Risk of Heart Failure and Atherosclerotic Cardiovascular Disease: MESA. J. Am. Heart Assoc. 7:e009599. doi: 10.1161/JAHA.118.009599
Fowler, S. M., Kon, V., Ma, L., Richards, W. O., Fogo, A. B., and Hunley, T. E. (2009). Obesity-related focal and segmental glomerulosclerosis: normalization of proteinuria in an adolescent after bariatric surgery. Pediatr. Nephrol. 24, 851–855. doi: 10.1007/s00467-008-1024-6
Franssen, C., Chen, S., Unger, A., Korkmaz, H. I., De Keulenaer, G. W., Tschope, C., et al. (2016). Myocardial Microvascular Inflammatory Endothelial Activation in Heart Failure With Preserved Ejection Fraction. JACC Heart Fail. 4, 312–324. doi: 10.1016/j.jchf.2015.10.007
Friedman, A. N., Chambers, M., Kamendulis, L. M., and Temmerman, J. (2013). Short-term changes after a weight reduction intervention in advanced diabetic nephropathy. Clin. J. Am. Soc. Nephrol. 8, 1892–1898. doi: 10.2215/CJN.04010413
Frisk, M., Le, C., Shen, X., Roe, A. T., Hou, Y., Manfra, O., et al. (2021). Etiology-Dependent Impairment of Diastolic Cardiomyocyte Calcium Homeostasis in Heart Failure With Preserved Ejection Fraction. J. Am. Coll. Cardiol. 77, 405–419. doi: 10.1016/j.jacc.2020.11.044
Ghoneim, S., Dhorepatil, A., Shah, A. R., Ram, G., Ahmad, S., Kim, C., et al. (2020a). Non-alcoholic steatohepatitis and the risk of myocardial infarction: a population-based national study. World J. Hepatol. 12, 378–388. doi: 10.4254/wjh.v12.i7.378
Ghoneim, S., Shah, A., Dhorepatil, A., Butt, M. U., and Waghray, N. (2020b). The Risk of Cerebrovascular Accidents in Inflammatory Bowel Disease in the United States: a Population-Based National Study. Clin. Exp. Gastroenterol. 13, 123–129. doi: 10.2147/CEG.S250182
Gidding, S. S., Liu, K., Colangelo, L. A., Cook, N. L., Goff, D. C., Glasser, S. P., et al. (2013). Longitudinal determinants of left ventricular mass and geometry: the Coronary Artery Risk Development in Young Adults (CARDIA) Study. Circ. Cardiovasc. Imaging 6, 769–775. doi: 10.1161/CIRCIMAGING.112.000450
Gorini, S., Kim, S. K., Infante, M., Mammi, C., La Vignera, S., Fabbri, A., et al. (2019). Role of Aldosterone and Mineralocorticoid Receptor in Cardiovascular Aging. Front. Endocrinol. 10:584. doi: 10.3389/fendo.2019.00584
Gupta, A., Braunwald, E., McNulty, S., Felker, G. M., Gilbert, E. M., Alharethi, R., et al. (2012). Obesity and the response to intensified diuretic treatment in decompensated heart failure: a DOSE trial substudy. J. Card. Fail. 18, 837–844. doi: 10.1016/j.cardfail.2012.09.005
Haass, M., Kitzman, D. W., Anand, I. S., Miller, A., Zile, M. R., Massie, B. M., et al. (2011). Body mass index and adverse cardiovascular outcomes in heart failure patients with preserved ejection fraction: results from the Irbesartan in Heart Failure with Preserved Ejection Fraction (I-PRESERVE) trial. Circ. Heart Fail. 4, 324–331. doi: 10.1161/CIRCHEARTFAILURE.110.959890
Haykowsky, M. J., Nicklas, B. J., Brubaker, P. H., Hundley, W. G., Brinkley, T. E., Upadhya, B., et al. (2018). Regional Adipose Distribution and its Relationship to Exercise Intolerance in Older Obese Patients Who Have Heart Failure With Preserved Ejection Fraction. JACC Heart Fail. 6, 640–649. doi: 10.1016/j.jchf.2018.06.002
Heinzel, F. R., Hohendanner, F., Jin, G., Sedej, S., and Edelmann, F. (2015). Myocardial hypertrophy and its role in heart failure with preserved ejection fraction. J. Appl. Physiol. 119, 1233–1242. doi: 10.1152/japplphysiol.00374.2015
Huan, Y., Tomaszewski, J. E., and Cohen, D. L. (2009). Resolution of nephrotic syndrome after successful bariatric surgery in patient with biopsy-proven FSGS. Clin. Nephrol. 71, 69–73. doi: 10.5414/CNP71069
Huynh, T., Harty, B. J., Claggett, B., Fleg, J. L., McKinlay, S. M., Anand, I. S., et al. (2019). Comparison of Outcomes in Patients With Diabetes Mellitus Treated With Versus Without Insulin+Heart Failure With Preserved Left Ventricular Ejection Fraction (from the TOPCAT Study). Am. J. Cardiol. 123, 611–617. doi: 10.1016/j.amjcard.2018.11.022
Iacobellis, G., Malavazos, A. E., and Corsi, M. M. (2011). Epicardial fat: from the biomolecular aspects to the clinical practice. Int. J. Biochem. Cell Biol. 43, 1651–1654.
Iacobellis, G., Mohseni, M., Bianco, S. D., and Banga, P. K. (2017). Liraglutide causes large and rapid epicardial fat reduction. Obesity 25, 311–316. doi: 10.1002/oby.21718
Iacobellis, G., Petramala, L., Marinelli, C., Calvieri, C., Zinnamosca, L., Concistre, A., et al. (2016). Epicardial Fat Thickness and Primary Aldosteronism. Horm. Metab. Res. 48, 238–241. doi: 10.1055/s-0035-1559769
Iborra-Egea, O., Santiago-Vacas, E., Yurista, S. R., Lupon, J., Packer, M., Heymans, S., et al. (2019). Unraveling the Molecular Mechanism of Action of Empagliflozin in Heart Failure With Reduced Ejection Fraction With or Without Diabetes. JACC Basic Transl. Sci. 4, 831–840. doi: 10.1016/j.jacbts.2019.07.010
Ippisch, H. M., Inge, T. H., Daniels, S. R., Wang, B., Khoury, P. R., Witt, S. A., et al. (2008). Reversibility of cardiac abnormalities in morbidly obese adolescents. J. Am. Coll. Cardiol. 51, 1342–1348. doi: 10.1016/j.jacc.2007.12.029
Ishibashi, C., Kozawa, J., Hosakawa, Y., Yoneda, S., Kimura, T., Fujita, Y., et al. (2020). Pancreatic fat is related to the longitudinal decrease in the increment of C-peptide in glucagon stimulation test in type 2 diabetes patients. J. Diabetes Investig. 11, 80–87. doi: 10.1111/jdi.13108
Jhaveri, R. R., Pond, K. K., Hauser, T. H., Kissinger, K. V., Goepfert, L., Schneider, B., et al. (2009). Cardiac remodeling after substantial weight loss: a prospective cardiac magnetic resonance study after bariatric surgery. Surg. Obes. Relat. Dis. 5, 648–652. doi: 10.1016/j.soard.2009.01.011
Jia, G., Hill, M. A., and Sowers, J. R. (2018). Diabetic Cardiomyopathy: an Update of Mechanisms Contributing to This Clinical Entity. Circ. Res. 122, 624–638. doi: 10.1161/CIRCRESAHA.117.311586
Juni, R. P., Kuster, D. W. D., Goebel, M., Helmes, M., Musters, R. J. P., van der Velden, J., et al. (2019). Cardiac Microvascular Endothelial Enhancement of Cardiomyocyte Function Is Impaired by Inflammation and Restored by Empagliflozin. JACC Basic Transl. Sci. 4, 575–591. doi: 10.1016/j.jacbts.2019.04.003
Kang, S., Verma, S., Hassanabad, A. F., Teng, G., Belke, D. D., Dundas, J. A., et al. (2020). Direct Effects of Empagliflozin on Extracellular Matrix Remodelling in Human Cardiac Myofibroblasts: novel Translational Clues to Explain EMPA-REG OUTCOME Results. Can. J. Cardiol. 36, 543–553. doi: 10.1016/j.cjca.2019.08.033
Karason, K., Wallentin, I., Larsson, B., and Sjostrom, L. (1997). Effects of obesity and weight loss on left ventricular mass and relative wall thickness: survey and intervention study. BMJ 315, 912–916. doi: 10.1136/bmj.315.7113.912
Kenchaiah, S., Ding, J., Carr, J. J., Allison, M. A., Budoff, M. J., Tracy, R. P., et al. (2021). Pericardial Fat and the Risk of Heart Failure. J. Am. Coll. Cardiol. 77, 2638–2652. doi: 10.1016/j.jacc.2021.04.003
Kim, J. S., Kim, S. W., Lee, J. S., Lee, S. K., Abbott, R., Lee, K. Y., et al. (2021). Association of pericardial adipose tissue with left ventricular structure and function: a region-specific effect? Cardiovasc. Diabetol. 20:26. doi: 10.1186/s12933-021-01219-4
Kim, M. K., Tomita, T., Kim, M. J., Sasai, H., Maeda, S., and Tanaka, K. (2009). Aerobic exercise training reduces epicardial fat in obese men. J. Appl. Physiol. 106, 5–11. doi: 10.1152/japplphysiol.90756.2008
Kishi, S., Armstrong, A. C., Gidding, S. S., Colangelo, L. A., Venkatesh, B. A., and Jacobs, D. R. Jr., et al. (2014). Association of obesity in early adulthood and middle age with incipient left ventricular dysfunction and structural remodeling: the CARDIA study (Coronary Artery Risk Development in Young Adults). JACC Heart Fail. 2, 500–508. doi: 10.1016/j.jchf.2014.03.001
Kobayashi, H., Ouchi, N., Kihara, S., Walsh, K., Kumada, M., Abe, Y., et al. (2004). Selective suppression of endothelial cell apoptosis by the high molecular weight form of adiponectin. Circ. Res. 94, e27–e31. doi: 10.1161/01.RES.0000119921.86460.37
Kotsis, V., Stabouli, S., Papakatsika, S., Rizos, Z., and Parati, G. (2010). Mechanisms of obesity-induced hypertension. Hypertens. Res. 33, 386–393. doi: 10.1038/hr.2010.9
Kristensen, S. L., Mogensen, U. M., Jhund, P. S., Petrie, M. C., Preiss, D., Win, S., et al. (2017). Clinical and Echocardiographic Characteristics and Cardiovascular Outcomes According to Diabetes Status in Patients With Heart Failure and Preserved Ejection Fraction: a Report From the I-Preserve Trial (Irbesartan in Heart Failure With Preserved Ejection Fraction). Circulation 135, 724–735. doi: 10.1161/CIRCULATIONAHA.116.024593
Kurnicka, K., Domienik-Karlowicz, J., Lichodziejewska, B., Bielecki, M., Kozlowska, M., Goliszek, S., et al. (2018). Improvement of left ventricular diastolic function and left heart morphology in young women with morbid obesity six months after bariatric surgery. Cardiol. J. 25, 97–105. doi: 10.5603/CJ.a2017.0059
Lam, C. S. P., Voors, A. A., de Boer, R. A., Solomon, S. D., and van Veldhuisen, D. J. (2018). Heart failure with preserved ejection fraction: from mechanisms to therapies. Eur. Heart J. 39, 2780–2792. doi: 10.1093/eurheartj/ehy301
Lam, C. S., Donal, E., Kraigher-Krainer, E., and Vasan, R. S. (2011). Epidemiology and clinical course of heart failure with preserved ejection fraction. Eur. J. Heart Fail. 13, 18–28. doi: 10.1093/eurjhf/hfq121
Lambers Heerspink, H. J., de Zeeuw, D., Wie, L., Leslie, B., and List, J. (2013). Dapagliflozin a glucose-regulating drug with diuretic properties in subjects with type 2 diabetes. Diabetes Obes. Metab. 15, 853–862. doi: 10.1111/dom.12127
Lau, W. B., Ohashi, K., Wang, Y., Ogawa, H., Murohara, T., Ma, X. L., et al. (2017). Role of Adipokines in Cardiovascular Disease. Circ. J. 81, 920–928. doi: 10.1253/circj.CJ-17-0458
Laurent, S., Cockcroft, J., Van Bortel, L., Boutouyrie, P., Giannattasio, C., Hayoz, D., et al. (2006). Expert consensus document on arterial stiffness: methodological issues and clinical applications. Eur. Heart J. 27, 2588–2605. doi: 10.1093/eurheartj/ehl254
Le Jemtel, T. H., Samson, R., Milligan, G., Jaiswal, A., and Oparil, S. (2018). Visceral Adipose Tissue Accumulation and Residual Cardiovascular Risk. Curr. Hypertens. Rep. 20:77. doi: 10.1007/s11906-018-0880-0
Lebovitz, H. E., and Banerji, M. A. (2005). Point: visceral adiposity is causally related to insulin resistance. Diabetes Care 28, 2322–2325. doi: 10.2337/diacare.28.9.2322
Lee, H. C., Shiou, Y. L., Jhuo, S. J., Chang, C. Y., Liu, P. L., Jhuang, W. J., et al. (2019). The sodium-glucose co-transporter 2 inhibitor empagliflozin attenuates cardiac fibrosis and improves ventricular hemodynamics in hypertensive heart failure rats. Cardiovasc. Diabetol. 18:45. doi: 10.1186/s12933-019-0849-6
Leibel, R. L., Rosenbaum, M., and Hirsch, J. (1995). Changes in energy expenditure resulting from altered body weight. N. Engl. J. Med. 332, 621–628. doi: 10.1056/NEJM199503093321001
Lejeune, S., Roy, C., Slimani, A., Pasquet, A., Vancraeynest, D., Vanoverschelde, J. L., et al. (2021). Diabetic phenotype and prognosis of patients with heart failure and preserved ejection fraction in a real life cohort. Cardiovasc. Diabetol. 20:48. doi: 10.1186/s12933-021-01242-5
Levelt, E., Rodgers, C. T., Clarke, W. T., Mahmod, M., Ariga, R., Francis, J. M., et al. (2016). Cardiac energetics, oxygenation, and perfusion during increased workload in patients with type 2 diabetes mellitus. Eur. Heart J. 37, 3461–3469. doi: 10.1093/eurheartj/ehv442
LeWinter, M. M., and Meyer, M. (2013). Mechanisms of diastolic dysfunction in heart failure with a preserved ejection fraction: if it’s not one thing it’s another. Circ. Heart Fail. 6, 1112–1115. doi: 10.1161/CIRCHEARTFAILURE.113.000825
Lieske, J. C., Mehta, R. A., Milliner, D. S., Rule, A. D., Bergstralh, E. J., and Sarr, M. G. (2015). Kidney stones are common after bariatric surgery. Kidney Int. 87, 839–845. doi: 10.1038/ki.2014.352
Liu, X. Y., Zhang, N., Chen, R., Zhao, J. G., and Yu, P. (2015). Efficacy and safety of sodium-glucose cotransporter 2 inhibitors in type 2 diabetes: a meta-analysis of randomized controlled trials for 1 to 2years. J. Diabetes Complications 29, 1295–1303. doi: 10.1016/j.jdiacomp.2015.07.011
Liu, Y., Haddad, T., and Dwivedi, G. (2013). Heart failure with preserved ejection fraction: current understanding and emerging concepts. Curr. Opin. Cardiol. 28, 187–196. doi: 10.1097/HCO.0b013e32835c5492
Lopaschuk, G. D., and Verma, S. (2020). Mechanisms of Cardiovascular Benefits of Sodium Glucose Co-Transporter 2 (SGLT2) Inhibitors: a State-of-the-Art Review. JACC Basic Transl. Sci. 5, 632–644. doi: 10.1016/j.jacbts.2020.02.004
Lumeng, C. N., Bodzin, J. L., and Saltiel, A. R. (2007). Obesity induces a phenotypic switch in adipose tissue macrophage polarization. J. Clin. Invest. 117, 175–184. doi: 10.1172/JCI29881
Ma, I. T., and Madura, J. A. II (2015). Gastrointestinal Complications After Bariatric Surgery. Gastroenterol. Hepatol. 11, 526–535.
MacDonald, M. R., Petrie, M. C., Varyani, F., Ostergren, J., Michelson, E. L., Young, J. B., et al. (2008). Impact of diabetes on outcomes in patients with low and preserved ejection fraction heart failure: an analysis of the Candesartan in Heart failure: assessment of Reduction in Mortality and morbidity (CHARM) programme. Eur. Heart J. 29, 1377–1385. doi: 10.1093/eurheartj/ehn153
Maciejewski, M. L., Arterburn, D. E., Van Scoyoc, L., Smith, V. A., Yancy, W. S. Jr., Weidenbacher, H. J., et al. (2016). Bariatric Surgery and Long-term Durability of Weight Loss. JAMA Surg. 151, 1046–1055. doi: 10.1001/jamasurg.2016.2317
Makhlough, A., Kashi, Z., Akha, O., Zaboli, E., and Yazdanicharati, J. (2014). Effect of spironolactone on diabetic nephropathy compared to the combination of spironolactone and losartan. Nephrourol. Mon. 6:e12148. doi: 10.5812/numonthly.12148
Mallamaci, F., Ruggenenti, P., Perna, A., Leonardis, D., Tripepi, R., Tripepi, G., et al. (2011). ACE inhibition is renoprotective among obese patients with proteinuria. J. Am. Soc. Nephrol. 22, 1122–1128. doi: 10.1681/ASN.2010090969
Marchington, J. M., Mattacks, C. A., and Pond, C. M. (1989). Adipose tissue in the mammalian heart and pericardium: structure, foetal development and biochemical properties. Comp. Biochem. Physiol. B 94, 225–232. doi: 10.1016/0305-0491(89)90337-4
Maruthur, N. M., Tseng, E., Hutfless, S., Wilson, L. M., Suarez-Cuervo, C., Berger, Z., et al. (2016). Diabetes Medications as Monotherapy or Metformin-Based Combination Therapy for Type 2 Diabetes: a Systematic Review and Meta-analysis. Ann. Intern. Med. 164, 740–751. doi: 10.7326/M15-2650
Marzolla, V., Feraco, A., Gorini, S., Mammi, C., Marrese, C., Mularoni, V., et al. (2020). The novel non-steroidal MR antagonist finerenone improves metabolic parameters in high-fat diet-fed mice and activates brown adipose tissue via AMPK-ATGL pathway. FASEB J. 34, 12450–12465. doi: 10.1096/fj.202000164R
Massie, B. M., Carson, P. E., McMurray, J. J., Komajda, M., McKelvie, R., Zile, M. R., et al. (2008). Irbesartan in patients with heart failure and preserved ejection fraction. N. Engl. J. Med. 359, 2456–2467. doi: 10.1056/NEJMoa0805450
McHugh, K., DeVore, A. D., Wu, J., Matsouaka, R. A., Fonarow, G. C., Heidenreich, P. A., et al. (2019). Heart Failure With Preserved Ejection Fraction and Diabetes: JACC State-of-the-Art Review. J. Am. Coll. Cardiol. 73, 602–611. doi: 10.1016/j.jacc.2018.11.033
McLaughlin, T., Ackerman, S. E., Shen, L., and Engleman, E. (2017). Role of innate and adaptive immunity in obesity-associated metabolic disease. J. Clin. Invest. 127, 5–13. doi: 10.1172/JCI88876
McMurray, J. J. V., Jackson, A. M., Lam, C. S. P., Redfield, M. M., Anand, I. S., Ge, J., et al. (2020). Effects of Sacubitril-Valsartan Versus Valsartan in Women Compared With Men With Heart Failure and Preserved Ejection Fraction: insights From PARAGON-HF. Circulation 141, 338–351. doi: 10.1161/CIRCULATIONAHA.119.044491
McMurray, J. J. V., Solomon, S. D., Inzucchi, S. E., Kober, L., Kosiborod, M. N., Martinez, F. A., et al. (2019). Dapagliflozin in Patients with Heart Failure and Reduced Ejection Fraction. N. Engl. J. Med. 381, 1995–2008. doi: 10.1056/NEJMoa1911303
McNelis, J. C., and Olefsky, J. M. (2014). Macrophages, immunity, and metabolic disease. Immunity 41, 36–48. doi: 10.1016/j.immuni.2014.05.010
Mishra, S., and Kass, D. A. (2021). Cellular and molecular pathobiology of heart failure with preserved ejection fraction. Nat. Rev. Cardiol. 18, 400–423. doi: 10.1038/s41569-020-00480-6
Mitchell, G. F. (2008). Effects of central arterial aging on the structure and function of the peripheral vasculature: implications for end-organ damage. J. Appl. Physiol. 105, 1652–1660. doi: 10.1152/japplphysiol.90549.2008
Mozaffarian, D., Benjamin, E. J., Go, A. S., Arnett, D. K., Blaha, M. J., Cushman, M., et al. (2015). Heart disease and stroke statistics–2015 update: a report from the American Heart Association. Circulation 131, e29–e322. doi: 10.1161/CIR.0000000000000152
Mueller, S., Winzer, E. B., Duvinage, A., Gevaert, A. B., Edelmann, F., Haller, B., et al. (2021). Effect of High-Intensity Interval Training, Moderate Continuous Training, or Guideline-Based Physical Activity Advice on Peak Oxygen Consumption in Patients With Heart Failure With Preserved Ejection Fraction: a Randomized Clinical Trial. JAMA 325, 542–551. doi: 10.1001/jama.2020.26812
Neeland, I. J., Gupta, S., Ayers, C. R., Turer, A. T., Rame, J. E., Das, S. R., et al. (2013). Relation of regional fat distribution to left ventricular structure and function. Circ. Cardiovasc. Imaging 6, 800–807. doi: 10.1161/CIRCIMAGING.113.000532
Nesti, L., Pugliese, N. R., Sciuto, P., and Natali, A. (2020). Type 2 diabetes and reduced exercise tolerance: a review of the literature through an integrated physiology approach. Cardiovasc. Diabetol. 19:134. doi: 10.1186/s12933-020-01109-1
Ng, A. C. T., Strudwick, M., van der Geest, R. J., Ng, A. C. C., Gillinder, L., Goo, S. Y., et al. (2018). Impact of Epicardial Adipose Tissue, Left Ventricular Myocardial Fat Content, and Interstitial Fibrosis on Myocardial Contractile Function. Circ. Cardiovasc. Imaging 11:e007372. doi: 10.1161/CIRCIMAGING.117.007372
Nguyen, T. D., Shingu, Y., Amorim, P. A., Schenkl, C., Schwarzer, M., and Doenst, T. (2018). GLP-1 Improves Diastolic Function and Survival in Heart Failure with Preserved Ejection Fraction. J. Cardiovasc. Transl. Res. 11, 259–267. doi: 10.1007/s12265-018-9795-z
Obokata, M., Reddy, Y. N. V., Pislaru, S. V., Melenovsky, V., and Borlaug, B. A. (2017). Evidence Supporting. Circulation 136, 6–19. doi: 10.1161/CIRCULATIONAHA.116.026807
Olatunji, L. A., Usman, T. O., Akinade, A. I., Adeyanju, O. A., Kim, I., and Soladoye, A. O. (2017). Low-dose spironolactone ameliorates insulin resistance and suppresses elevated plasminogen activator inhibitor-1 during gestational testosterone exposure. Arch. Physiol. Biochem. 123, 286–292. doi: 10.1080/13813455.2017.1320681
Osborn, O., and Olefsky, J. M. (2012). The cellular and signaling networks linking the immune system and metabolism in disease. Nat. Med. 18, 363–374. doi: 10.1038/nm.2627
Ouchi, N., Kobayashi, H., Kihara, S., Kumada, M., Sato, K., Inoue, T., et al. (2004). Adiponectin stimulates angiogenesis by promoting cross-talk between AMP-activated protein kinase and Akt signaling in endothelial cells. J. Biol. Chem. 279, 1304–1309. doi: 10.1074/jbc.M310389200
Owan, T. E., and Redfield, M. M. (2005). Epidemiology of diastolic heart failure. Prog. Cardiovasc. Dis. 47, 320–332. doi: 10.1016/j.pcad.2005.02.010
Owan, T., Avelar, E., Morley, K., Jiji, R., Hall, N., Krezowski, J., et al. (2011). Favorable changes in cardiac geometry and function following gastric bypass surgery: 2-year follow-up in the Utah obesity study. J. Am. Coll. Cardiol. 57, 732–739. doi: 10.1016/j.jacc.2010.10.017
Packer, M. (2018b). Epicardial Adipose Tissue May Mediate Deleterious Effects of Obesity and Inflammation on the Myocardium. J. Am. Coll. Cardiol. 71, 2360–2372. doi: 10.1016/j.jacc.2018.03.509
Packer, M. (2018a). Do sodium-glucose co-transporter-2 inhibitors prevent heart failure with a preserved ejection fraction by counterbalancing the effects of leptin? A novel hypothesis. Diabetes Obes. Metab. 20, 1361–1366. doi: 10.1111/dom.13229
Packer, M., and Kitzman, D. W. (2018). Obesity-Related Heart Failure With a Preserved Ejection Fraction: the Mechanistic Rationale for Combining Inhibitors of Aldosterone, Neprilysin, and Sodium-Glucose Cotransporter-2. JACC Heart Fail. 6, 633–639. doi: 10.1016/j.jchf.2018.01.009
Packer, M., Anker, S. D., Butler, J., Filippatos, G., Pocock, S. J., Carson, P., et al. (2020). Cardiovascular and Renal Outcomes with Empagliflozin in Heart Failure. N. Engl. J. Med. 383, 1413–1424. doi: 10.1056/NEJMoa2022190
Pandey, A., Parashar, A., Kumbhani, D., Agarwal, S., Garg, J., Kitzman, D., et al. (2015b). Exercise training in patients with heart failure and preserved ejection fraction: meta-analysis of randomized control trials. Circ. Heart Fail. 8, 33–40. doi: 10.1161/CIRCHEARTFAILURE.114.001615
Pandey, A., Garg, S., Matulevicius, S. A., Shah, A. M., Garg, J., Drazner, M. H., et al. (2015a). Effect of Mineralocorticoid Receptor Antagonists on Cardiac Structure and Function in Patients With Diastolic Dysfunction and Heart Failure With Preserved Ejection Fraction: a Meta-Analysis and Systematic Review. J. Am. Heart Assoc. 4:e002137. doi: 10.1161/JAHA.115.002137
Pandey, A., Patel, K. V., Vaduganathan, M., Sarma, S., Haykowsky, M. J., Berry, J. D., et al. (2018). Physical Activity, Fitness, and Obesity in Heart Failure With Preserved Ejection Fraction. JACC Heart Fail. 6, 975–982. doi: 10.1016/j.jchf.2018.09.006
Pandey, A., Shah, S. J., Butler, J., Kellogg, D. L. Jr., Lewis, G. D., Forman, D. E., et al. (2021). Exercise Intolerance in Older Adults With Heart Failure With Preserved Ejection Fraction: JACC State-of-the-Art Review. J. Am. Coll. Cardiol. 78, 1166–1187. doi: 10.1016/j.jacc.2021.07.014
Paulus, W. J. (2020). Unfolding Discoveries in Heart Failure. N. Engl. J. Med. 382, 679–682. doi: 10.1056/NEJMcibr1913825
Paulus, W. J., and Tschope, C. (2013). A novel paradigm for heart failure with preserved ejection fraction: comorbidities drive myocardial dysfunction and remodeling through coronary microvascular endothelial inflammation. J. Am. Coll. Cardiol. 62, 263–271. doi: 10.1016/j.jacc.2013.02.092
Pereira, M. J., and Eriksson, J. W. (2019). Emerging Role of SGLT-2 Inhibitors for the Treatment of Obesity. Drugs 79, 219–230. doi: 10.1007/s40265-019-1057-0
Peterson, L. R., Waggoner, A. D., Schechtman, K. B., Meyer, T., Gropler, R. J., Barzilai, B., et al. (2004b). Alterations in left ventricular structure and function in young healthy obese women: assessment by echocardiography and tissue Doppler imaging. J. Am. Coll. Cardiol. 43, 1399–1404. doi: 10.1016/j.jacc.2003.10.062
Peterson, L. R., Herrero, P., Schechtman, K. B., Racette, S. B., Waggoner, A. D., Kisrieva-Ware, Z., et al. (2004a). Effect of obesity and insulin resistance on myocardial substrate metabolism and efficiency in young women. Circulation 109, 2191–2196. doi: 10.1161/01.CIR.0000127959.28627.F8
Piche, M. E., and Poirier, P. (2018). Obesity, ectopic fat and cardiac metabolism. Expert Rev. Endocrinol. Metab. 13, 213–221. doi: 10.1080/17446651.2018.1500894
Piche, M. E., Tchernof, A., and Despres, J. P. (2020). Obesity Phenotypes, Diabetes, and Cardiovascular Diseases. Circ. Res. 126, 1477–1500. doi: 10.1161/CIRCRESAHA.120.316101
Pieske, B., Maggioni, A. P., Lam, C. S. P., Pieske-Kraigher, E., Filippatos, G., Butler, J., et al. (2017). Vericiguat in patients with worsening chronic heart failure and preserved ejection fraction: results of the SOluble guanylate Cyclase stimulatoR in heArT failurE patientS with PRESERVED EF (SOCRATES-PRESERVED) study. Eur. Heart J. 38, 1119–1127. doi: 10.1093/eurheartj/ehw593
Pitt, B., Pfeffer, M. A., Assmann, S. F., Boineau, R., Anand, I. S., Claggett, B., et al. (2014). Spironolactone for heart failure with preserved ejection fraction. N. Engl. J. Med. 370, 1383–1392. doi: 10.1056/NEJMoa1313731
Pluta, A., Strozecki, P., Krintus, M., drowaz-Sypniewska, G. O., and Manitius, J. (2015). Left ventricular remodeling and arterial remodeling in patients with chronic kidney disease stage 1-3. Ren. Fail. 37, 1105–1110. doi: 10.3109/0886022X.2015.1061669
Powell, B. D., Redfield, M. M., Bybee, K. A., Freeman, W. K., and Rihal, C. S. (2006). Association of obesity with left ventricular remodeling and diastolic dysfunction in patients without coronary artery disease. Am. J. Cardiol. 98, 116–120. doi: 10.1016/j.amjcard.2006.01.063
Praga, M., Hernandez, E., Morales, E., Campos, A. P., Valero, M. A., Martinez, M. A., et al. (2001). Clinical features and long-term outcome of obesity-associated focal segmental glomerulosclerosis. Nephrol. Dial. Transplant. 16, 1790–1798. doi: 10.1093/ndt/16.9.1790
Pugliese, N. R., De Biase, N., Gargani, L., Mazzola, M., Conte, L., Fabiani, I., et al. (2020). Predicting the transition to and progression of heart failure with preserved ejection fraction: a weighted risk score using bio-humoural, cardiopulmonary, and echocardiographic stress testing. Eur. J. Prev. Cardiol. doi: 10.1093/eurjpc/zwaa129 [Online ahead of print]
Pugliese, N. R., Paneni, F., Mazzola, M., De Biase, N., Del Punta, L., Gargani, L., et al. (2021b). Impact of epicardial adipose tissue on cardiovascular haemodynamics, metabolic profile, and prognosis in heart failure. Eur. J. Heart Fail. 23, 1858–1871. doi: 10.1002/ejhf.2337
Pugliese, N. R., De Biase, N., Conte, L., Gargani, L., Mazzola, M., Fabiani, I., et al. (2021a). Cardiac Reserve and Exercise Capacity: insights from Combined Cardiopulmonary and Exercise Echocardiography Stress Testing. J. Am. Soc. Echocardiogr. 34, 38–50. doi: 10.1016/j.echo.2020.08.015
Rao, V. N., Bush, C. G., Mongraw-Chaffin, M., Hall, M. E., Clark, D. III, Fudim, M., et al. (2021). Regional Adiposity and Risk of Heart Failure and Mortality: the Jackson Heart Study. J. Am. Heart Assoc. 10:e020920. doi: 10.1161/JAHA.121.020920
Rao, V. N., Zhao, D., Allison, M. A., Guallar, E., Sharma, K., Criqui, M. H., et al. (2018). Adiposity and Incident Heart Failure and its Subtypes: MESA (Multi-Ethnic Study of Atherosclerosis). JACC Heart Fail. 6, 999–1007. doi: 10.1016/j.jchf.2018.07.009
Rawshani, A., Eliasson, B., Rawshani, A., Henninger, J., Mardinoglu, A., Carlsson, A., et al. (2020). Adipose tissue morphology, imaging and metabolomics predicting cardiometabolic risk and family history of type 2 diabetes in non-obese men. Sci. Rep. 10:9973. doi: 10.1038/s41598-020-66199-z
Rayner, J. J., Banerjee, R., Holloway, C. J., Lewis, A. J. M., Peterzan, M. A., Francis, J. M., et al. (2018). The relative contribution of metabolic and structural abnormalities to diastolic dysfunction in obesity. Int. J. Obes. 42, 441–447. doi: 10.1038/ijo.2017.239
Razavi, A. C., Bazzano, L. A., He, J., Fernandez, C., Whelton, S. P., Krousel-Wood, M., et al. (2020). Novel Findings From a Metabolomics Study of Left Ventricular Diastolic Function: the Bogalusa Heart Study. J. Am. Heart Assoc. 9:e015118. doi: 10.1161/JAHA.119.015118
Reddy, Y. N. V., Lewis, G. D., Shah, S. J., Obokata, M., Abou-Ezzedine, O. F., Fudim, M., et al. (2019). Characterization of the Obese Phenotype of Heart Failure With Preserved Ejection Fraction: a RELAX Trial Ancillary Study. Mayo Clin. Proc. 94, 1199–1209. doi: 10.1016/j.mayocp.2018.11.037
Redfield, M. M., Anstrom, K. J., Levine, J. A., Koepp, G. A., Borlaug, B. A., Chen, H. H., et al. (2015). Isosorbide Mononitrate in Heart Failure with Preserved Ejection Fraction. N. Engl. J. Med. 373, 2314–2324. doi: 10.1056/NEJMoa1510774
Redfield, M. M., Chen, H. H., Borlaug, B. A., Semigran, M. J., Lee, K. L., Lewis, G., et al. (2013). Effect of phosphodiesterase-5 inhibition on exercise capacity and clinical status in heart failure with preserved ejection fraction: a randomized clinical trial. JAMA 309, 1268–1277. doi: 10.1001/jama.2013.2024
Redfield, M. M., Jacobsen, S. J., Burnett, J. C. Jr., Mahoney, D. W., Bailey, K. R., and Rodeheffer, R. J. (2003). Burden of systolic and diastolic ventricular dysfunction in the community: appreciating the scope of the heart failure epidemic. JAMA 289, 194–202. doi: 10.1001/jama.289.2.194
Reilly, S. M., and Saltiel, A. R. (2017). Adapting to obesity with adipose tissue inflammation. Nat. Rev. Endocrinol. 13, 633–643. doi: 10.1038/nrendo.2017.90
Reis, J. P., Allen, N., Gibbs, B. B., Gidding, S. S., Lee, J. M., Lewis, C. E., et al. (2014). Association of the degree of adiposity and duration of obesity with measures of cardiac structure and function: the CARDIA study. Obesity 22, 2434–2440. doi: 10.1002/oby.20865
Rider, O. J., Cox, P., Tyler, D., Clarke, K., and Neubauer, S. (2013). Myocardial substrate metabolism in obesity. Int. J. Obes. 37, 972–979. doi: 10.1038/ijo.2012.170
Rider, O. J., Francis, J. M., Ali, M. K., Petersen, S. E., Robinson, M., Robson, M. D., et al. (2009). Beneficial cardiovascular effects of bariatric surgical and dietary weight loss in obesity. J. Am. Coll. Cardiol. 54, 718–726.
Rosner, M. H., Ronco, C., and Okusa, M. D. (2012). The role of inflammation in the cardio-renal syndrome: a focus on cytokines and inflammatory mediators. Semin. Nephrol. 32, 70–78. doi: 10.1016/j.semnephrol.2011.11.010
Rubino, D., Abrahamsson, N., Davies, M., Hesse, D., Greenway, F. L., Jensen, C., et al. (2021). Effect of Continued Weekly Subcutaneous Semaglutide vs Placebo on Weight Loss Maintenance in Adults With Overweight or Obesity: the STEP 4 Randomized Clinical Trial. JAMA 325, 1414–1425.
Russo, C., Jin, Z., Homma, S., Rundek, T., Elkind, M. S., Sacco, R. L., et al. (2011). Effect of obesity and overweight on left ventricular diastolic function: a community-based study in an elderly cohort. J. Am. Coll. Cardiol. 57, 1368–1374. doi: 10.1016/j.jacc.2010.10.042
Safar, M. E., Plante, G. E., and Mimran, A. (2015). Arterial stiffness, pulse pressure, and the kidney. Am. J. Hypertens. 28, 561–569. doi: 10.1093/ajh/hpu206
Saiki, A., Nagayama, D., Ohhira, M., Endoh, K., Ohtsuka, M., Koide, N., et al. (2005). Effect of weight loss using formula diet on renal function in obese patients with diabetic nephropathy. Int. J. Obes. 29, 1115–1120. doi: 10.1038/sj.ijo.0803009
Salzano, A., De Luca, M., Israr, M. Z., Crisci, G., Eltayeb, M., Debiec, R., et al. (2021). Exercise Intolerance in Heart Failure with Preserved Ejection Fraction. Heart Fail. Clin. 17, 397–413. doi: 10.1016/j.hfc.2021.03.004
Samson, R., and Le Jemtel, T. H. (2021). Therapeutic Stalemate in Heart Failure With Preserved Ejection Fraction. J. Am. Heart Assoc. 10:e021120. doi: 10.1161/JAHA.121.021120
Samson, R., Jaiswal, A., Ennezat, P. V., Cassidy, M., and Le Jemtel, T. H. (2016). Clinical Phenotypes in Heart Failure With Preserved Ejection Fraction. J. Am. Heart Assoc. 5:e002477. doi: 10.1161/JAHA.115.002477
Sato, T., Aizawa, Y., Yuasa, S., Kishi, S., Fuse, K., Fujita, S., et al. (2018). The effect of dapagliflozin treatment on epicardial adipose tissue volume. Cardiovasc. Diabetol. 17:6. doi: 10.1186/s12933-017-0658-8
Savji, N., Meijers, W. C., Bartz, T. M., Bhambhani, V., Cushman, M., Nayor, M., et al. (2018). of Obesity and Cardiometabolic Traits With Incident HFpEF and HFrEF. JACC Heart Fail. 6, 701–709. doi: 10.1016/j.jchf.2018.05.018
Schjoedt, K. J., Rossing, K., Juhl, T. R., Boomsma, F., Rossing, P., Tarnow, L., et al. (2005). Beneficial impact of spironolactone in diabetic nephropathy. Kidney Int. 68, 2829–2836. doi: 10.1111/j.1523-1755.2005.00756.x
Selvaraj, S., Claggett, B., Shah, S. J., Anand, I., Rouleau, J. L., O’Meara, E., et al. (2018). Prognostic Value of Albuminuria and Influence of Spironolactone in Heart Failure With Preserved Ejection Fraction. Circ. Heart Fail. 11:e005288. doi: 10.1161/CIRCHEARTFAILURE.118.005288
Shah, R. V., Murthy, V. L., Abbasi, S. A., Eng, J., Wu, C., Ouyang, P., et al. (2015). Weight loss and progressive left ventricular remodelling: the Multi-Ethnic Study of Atherosclerosis (MESA). Eur. J. Prev. Cardiol. 22, 1408–1418. doi: 10.1177/2047487314541731
Shah, S. J., Lam, C. S. P., Svedlund, S., Saraste, A., Hage, C., Tan, R. S., et al. (2018). Prevalence and correlates of coronary microvascular dysfunction in heart failure with preserved ejection fraction: PROMIS-HFpEF. Eur. Heart J. 39, 3439–3450. doi: 10.1093/eurheartj/ehy531
Shen, W. W., Chen, H. M., Chen, H., Xu, F., Li, L. S., and Liu, Z. H. (2010). Obesity-related glomerulopathy: body mass index and proteinuria. Clin. J. Am. Soc. Nephrol. 5, 1401–1409. doi: 10.2215/CJN.01370210
Shibata, R., Ouchi, N., and Murohara, T. (2009). Adiponectin and cardiovascular disease. Circ. J. 73, 608–614. doi: 10.1253/circj.CJ-09-0057
Solomon, S. D., de Boer, R. A., DeMets, D., Hernandez, A. F., Inzucchi, S. E., Kosiborod, M. N., et al. (2021). Dapagliflozin in heart failure with preserved and mildly reduced ejection fraction: rationale and design of the DELIVER trial. Eur. J. Heart Fail. 23, 1217–1225. doi: 10.1002/ejhf.2249
Solomon, S. D., McMurray, J. J. V., Anand, I. S., Ge, J., Lam, C. S. P., and Maggioni, A. P. (2019). Angiotensin-Neprilysin Inhibition in Heart Failure with Preserved Ejection Fraction. N. Engl. J. Med. 381, 1609–1620. doi: 10.1056/NEJMoa1908655
Sorimachi, H., Obokata, M., Takahashi, N., Reddy, Y. N. V., Jain, C. C., Verbrugge, F. H., et al. (2021). Pathophysiologic importance of visceral adipose tissue in women with heart failure and preserved ejection fraction. Eur. Heart J. 42, 1595–1605. doi: 10.1093/eurheartj/ehaa823
Srivastava, G., and Apovian, C. M. (2018). Current pharmacotherapy for obesity. Nat. Rev. Endocrinol. 14, 12–24. doi: 10.1038/nrendo.2017.122
Sundstrom, J., Bruze, G., Ottosson, J., Marcus, C., Naslund, I., and Neovius, M. (2017). Weight Loss and Heart Failure: a Nationwide Study of Gastric Bypass Surgery Versus Intensive Lifestyle Treatment. Circulation 135, 1577–1585. doi: 10.1161/CIRCULATIONAHA.116.025629
Surve, A., Cottam, D., Sanchez-Pernaute, A., Torres, A., Roller, J., Kwon, Y., et al. (2018). The incidence of complications associated with loop duodeno-ileostomy after single-anastomosis duodenal switch procedures among 1328 patients: a multicenter experience. Surg. Obes. Relat. Dis. 14, 594–601. doi: 10.1016/j.soard.2018.01.020
Tesch, G. H., and Young, M. J. (2017). Mineralocorticoid Receptor Signaling as a Therapeutic Target for Renal and Cardiac Fibrosis. Front. Pharmacol. 8:313. doi: 10.3389/fphar.2017.00313
Tofte, N., Lindhardt, M., Adamova, K., Bakker, S. J. L., Beige, J., Beulens, J. W. J., et al. (2020). Early detection of diabetic kidney disease by urinary proteomics and subsequent intervention with spironolactone to delay progression (PRIORITY): a prospective observational study and embedded randomised placebo-controlled trial. Lancet Diabetes Endocrinol. 8, 301–312. doi: 10.1016/S2213-8587(20)30026-7
Tsalamandris, S., Antonopoulos, A. S., Oikonomou, E., Papamikroulis, G. A., Vogiatzi, G., Papaioannou, S., et al. (2019). The Role of Inflammation in Diabetes: current Concepts and Future Perspectives. Eur. Cardiol. 14, 50–59. doi: 10.15420/ecr.2018.33.1
Tsuboi, N., Koike, K., Hirano, K., Utsunomiya, Y., Kawamura, T., and Hosoya, T. (2013). Clinical features and long-term renal outcomes of Japanese patients with obesity-related glomerulopathy. Clin. Exp. Nephrol. 17, 379–385. doi: 10.1007/s10157-012-0719-y
Tsujimoto, T., and Kajio, H. (2017). Abdominal Obesity Is Associated With an Increased Risk of All-Cause Mortality in Patients With HFpEF. J. Am. Coll. Cardiol. 70, 2739–2749. doi: 10.1016/j.jacc.2017.09.1111
Turgeon, N. A., Perez, S., Mondestin, M., Davis, S. S., Lin, E., Tata, S., et al. (2012). The impact of renal function on outcomes of bariatric surgery. J. Am. Soc. Nephrol. 23, 885–894. doi: 10.1681/ASN.2011050476
Turkbey, E. B., McClelland, R. L., Kronmal, R. A., Burke, G. L., Bild, D. E., Tracy, R. P., et al. (2010). The impact of obesity on the left ventricle: the Multi-Ethnic Study of Atherosclerosis (MESA). JACC Cardiovasc. Imaging 3, 266–274. doi: 10.1016/j.jcmg.2009.10.012
Upadhya, B., Amjad, A., and Stacey, R. B. (2020). Optimizing The Management of Obese HFpEF Phenotype: can We Mind Both The Heart and The Kidney? J. Card. Fail. 26, 108–111. doi: 10.1016/j.cardfail.2019.11.018
Upadhya, B., and Kitzman, D. W. (2020). Heart failure with preserved ejection fraction: new approaches to diagnosis and management. Clin. Cardiol. 43, 145–155. doi: 10.1002/clc.23321
Upadhya, B., Haykowsky, M. J., Eggebeen, J., and Kitzman, D. W. (2015a). Exercise intolerance in heart failure with preserved ejection fraction: more than a heart problem. J. Geriatr. Cardiol. 12, 294–304.
Upadhya, B., Haykowsky, M. J., Eggebeen, J., and Kitzman, D. W. (2015b). Sarcopenic obesity and the pathogenesis of exercise intolerance in heart failure with preserved ejection fraction. Curr. Heart Fail. Rep. 12, 205–214. doi: 10.1007/s11897-015-0257-5
van de Wouw, J., Broekhuizen, M., Sorop, O., Joles, J. A., Verhaar, M. C., Duncker, D. J., et al. (2019). Chronic Kidney Disease as a Risk Factor for Heart Failure With Preserved Ejection Fraction: a Focus on Microcirculatory Factors and Therapeutic Targets. Front. Physiol. 10:1108. doi: 10.3389/fphys.2019.01108
Verdecchia, P., Schillaci, G., Borgioni, C., Ciucci, A., Battistelli, M., Bartoccini, C., et al. (1995). Adverse prognostic significance of concentric remodeling of the left ventricle in hypertensive patients with normal left ventricular mass. J. Am. Coll Cardiol. 25, 871–878. doi: 10.1016/0735-1097(94)00424-O
Verma, S., Garg, A., Yan, A. T., Gupta, A. K., Al-Omran, M., Sabongui, A., et al. (2016). Effect of Empagliflozin on Left Ventricular Mass and Diastolic Function in Individuals With Diabetes: an Important Clue to the EMPA-REG OUTCOME Trial? Diabetes Care 39, e212–e213. doi: 10.2337/dc16-1312
Verma, S., Poulter, N. R., Bhatt, D. L., Bain, S. C., Buse, J. B., Leiter, L. A., et al. (2018). Effects of Liraglutide on Cardiovascular Outcomes in Patients With Type 2 Diabetes Mellitus With or Without History of Myocardial Infarction or Stroke. Circulation 138, 2884–2894. doi: 10.1161/CIRCULATIONAHA.118.034516
Vilsboll, T., Christensen, M., Junker, A. E., Knop, F. K., and Gluud, L. L. (2012). Effects of glucagon-like peptide-1 receptor agonists on weight loss: systematic review and meta-analyses of randomised controlled trials. BMJ 344:d7771. doi: 10.1136/bmj.d7771
Vishvanath, L., and Gupta, R. K. (2019). Contribution of adipogenesis to healthy adipose tissue expansion in obesity. J. Clin. Invest. 129, 4022–4031. doi: 10.1172/JCI129191
Wadden, T. A., Bailey, T. S., Billings, L. K., Davies, M., Frias, J. P., Koroleva, A., et al. (2021). Effect of Subcutaneous Semaglutide vs Placebo as an Adjunct to Intensive Behavioral Therapy on Body Weight in Adults With Overweight or Obesity: the STEP 3 Randomized Clinical Trial. JAMA 325, 1403–1413. doi: 10.1001/jama.2021.1831
Wang, T. J., Larson, M. G., Levy, D., Benjamin, E. J., Leip, E. P., Wilson, P. W., et al. (2004). Impact of obesity on plasma natriuretic peptide levels. Circulation 109, 594–600. doi: 10.1161/01.CIR.0000112582.16683.EA
Wilding, J. P. H., Batterham, R. L., Calanna, S., Davies, M., Van Gaal, L. F., Lingvay, I., et al. (2021). Once-Weekly Semaglutide in Adults with Overweight or Obesity. N. Engl. J. Med. 384:989. doi: 10.1056/NEJMoa2032183
Wong, C. Y., O’Moore-Sullivan, T., Leano, R., Byrne, N., Beller, E., and Marwick, T. H. (2004). Alterations of left ventricular myocardial characteristics associated with obesity. Circulation 110, 3081–3087. doi: 10.1161/01.CIR.0000147184.13872.0F
Woodiwiss, A. J., Libhaber, C. D., Majane, O. H., Libhaber, E., Maseko, M., and Norton, G. R. (2008). Obesity promotes left ventricular concentric rather than eccentric geometric remodeling and hypertrophy independent of blood pressure. Am. J. Hypertens. 21, 1144–1151. doi: 10.1038/ajh.2008.252
Yafei, S., Elsewy, F., Youssef, E., Ayman, M., Elshafei, M., and Abayazeed, R. (2019). Echocardiographic association of epicardial fat with carotid intima-media thickness in patients with type 2 diabetes. Diab. Vasc. Dis. Res. 16, 378–384. doi: 10.1177/1479164119827602
Yan, Y., Li, S., Guo, Y., Fernandez, C., Bazzano, L., He, J., et al. (2020). Life-Course Cumulative Burden of Body Mass Index and Blood Pressure on Progression of Left Ventricular Mass and Geometry in Midlife: the Bogalusa Heart Study. Circ. Res. 126, 633–643. doi: 10.1161/CIRCRESAHA.119.316045
Yancy, C. W., Lopatin, M., Stevenson, L. W., De Marco, T., Fonarow, G. C., and ADHERE Scientific Advisory Committee and Investigators (2006). Clinical presentation, management, and in-hospital outcomes of patients admitted with acute decompensated heart failure with preserved systolic function: a report from the Acute Decompensated Heart Failure National Registry (ADHERE) Database. J. Am. Coll. Cardiol. 47, 76–84. doi: 10.1016/j.jacc.2005.09.022
Yusuf, S., Pfeffer, M. A., Swedberg, K., Granger, C. B., Held, P., McMurray, J. J., et al. (2003). Effects of candesartan in patients with chronic heart failure and preserved left-ventricular ejection fraction: the CHARM-Preserved Trial. Lancet 362, 777–781. doi: 10.1016/S0140-6736(03)14285-7
Zaccardi, F., Webb, D. R., Htike, Z. Z., Youssef, D., Khunti, K., and Davies, M. J. (2016). Efficacy and safety of sodium-glucose co-transporter-2 inhibitors in type 2 diabetes mellitus: systematic review and network meta-analysis. Diabetes Obes. Metab. 18, 783–794. doi: 10.1111/dom.12670
Keywords: obesity, heart failure with preserved ejection fraction, diabetes mellitus, weight loss surgery, visceral adipose tissue, epicardial adipose tissue
Citation: Dhore-patil A, Thannoun T, Samson R and Le Jemtel TH (2022) Diabetes Mellitus and Heart Failure With Preserved Ejection Fraction: Role of Obesity. Front. Physiol. 12:785879. doi: 10.3389/fphys.2021.785879
Received: 29 September 2021; Accepted: 03 December 2021;
Published: 15 February 2022.
Edited by:
Laurent Metzinger, University of Picardie Jules Verne, FranceReviewed by:
Alexander E. Berezin, Zaporizhia State Medical University, UkraineIndranee Rajapreyar, University of Alabama at Birmingham, United States
Nicola Riccardo Pugliese, University of Pisa, Italy
Copyright © 2022 Dhore-patil, Thannoun, Samson and Le Jemtel. This is an open-access article distributed under the terms of the Creative Commons Attribution License (CC BY). The use, distribution or reproduction in other forums is permitted, provided the original author(s) and the copyright owner(s) are credited and that the original publication in this journal is cited, in accordance with accepted academic practice. No use, distribution or reproduction is permitted which does not comply with these terms.
*Correspondence: Thierry H. Le Jemtel, bGVqZW10ZWxAdHVsYW5lLmVkdQ==