- 1Institute for Biomedical Research and Innovation, National Research Council, Palermo, Italy
- 2Pediatric Division, Department of Surgical Sciences, Dentistry, Gynecology and Pediatrics, University of Verona, Verona, Italy
Leptin is a pleiotropic adipocytokine involved in several physiologic functions, with a known role in innate and adaptive immunity as well as in tissue homeostasis. Long- and short-isoforms of leptin receptors are widely expressed in many peripheral tissues and organs, such as the respiratory tract. Similar to leptin, microbiota affects the immune system and may interfere with lung health through the bidirectional crosstalk called the “gut-lung axis.” Obesity leads to impaired protective immunity and altered susceptibility to pulmonary infections, as those by severe acute respiratory syndrome coronavirus 2 (SARS-CoV-2). Although it is known that leptin and microbiota link metabolism and lung health, their role within the SARS-CoV2 coronavirus disease 2019 (COVID-19) deserves further investigations. This review aimed to summarize the available evidence about: (i) the role of leptin in immune modulation; (ii) the role of gut microbiota within the gut-lung axis in modulating leptin sensitivity; and (iii) the role of leptin in the pathophysiology of COVID-19.
Introduction
Leptin adipocytokine is a pleiotropic hormone involved into widespread physiologic function, such as appetite and metabolic rate (Münzberg and Morrison, 2015; Mancuso et al., 2018), and in maintaining the homeostasis of immune system (La Cava and Matarese, 2004; Pérez-Pérez et al., 2017; Maurya et al., 2018; de Candia et al., 2021; Salum et al., 2021). The lung has been known as a sensitive and leptin-producing organ for more than 20 years with extensive research published for the role of leptin in the respiratory system, both in animals (Wang et al., 1996; De Matteis et al., 1998; Tsuchiya et al., 1999; Bergen et al., 2002) and humans (Bruno et al., 2005a,2009, 2011; Unal et al., 2006; Vernooy et al., 2009; Malli et al., 2010; Brandao-Rangel et al., 2021; Figure 1A).
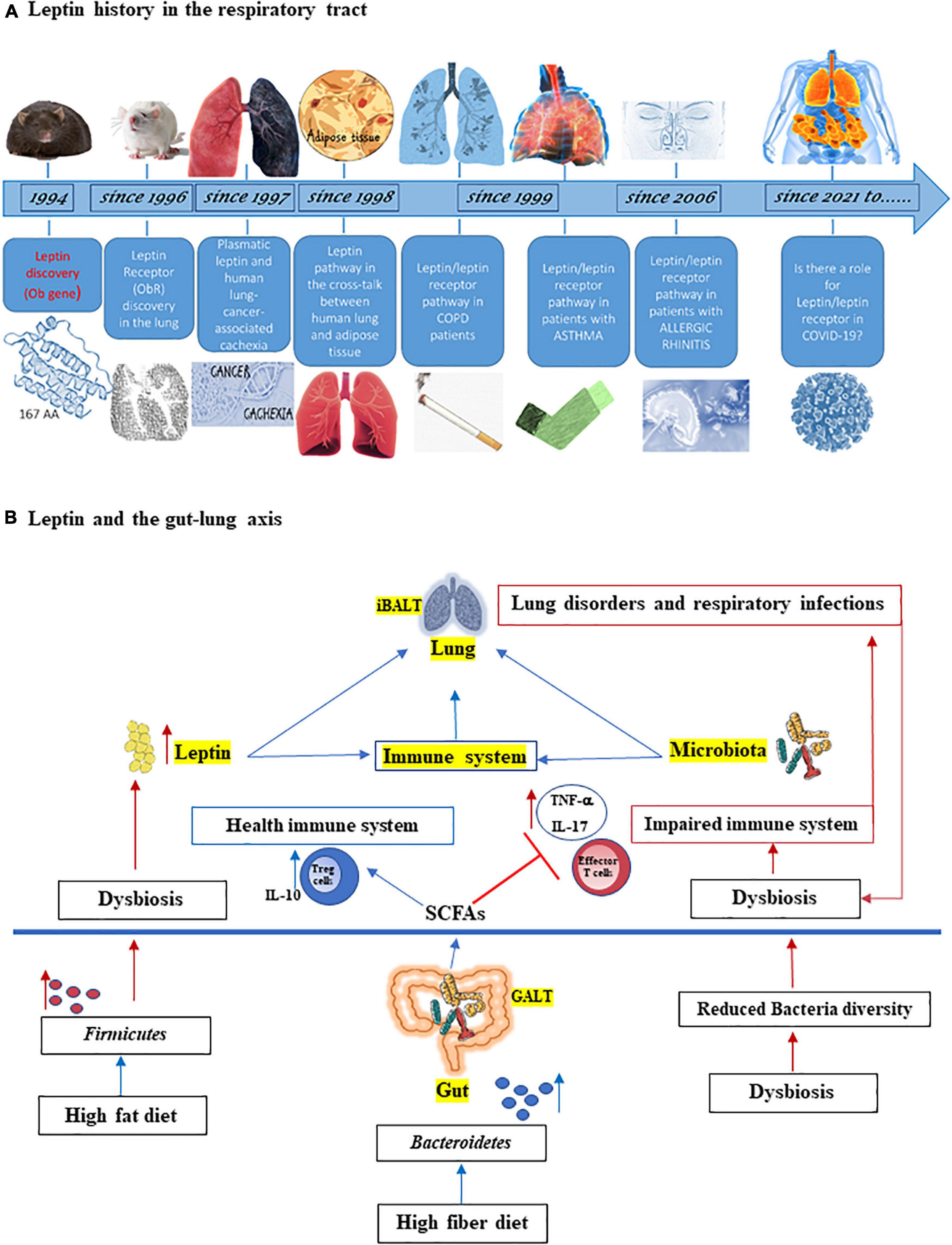
Figure 1. (A) Leptin history in the respiratory tract. Since its discovery 27 years ago, the adipocytokine leptin has provided a revolutionary framework for studying the physiological role of adipose tissue as an endocrine organ, also on respiratory tract. Leptin was discovered in mouse by Friedman group (Zhang et al., 1994) and soon leptin receptor (ObR) expression was found in the lung from rat (Wang et al., 1996). In 1997, it was studied as the correlation between serum leptin and lung cancer cachexia (Simons et al., 1997) and in 1998, the cross-talk between human lung and adipose tissue started to be identified (Kielar et al., 1998; Tankersley et al., 1998). Since 1999, leptin and its receptor are studied in patients with chronic obstructive pulmonary disease (COPD), asthma (Heuck and Wolthers, 1999; Takabatake et al., 1999; Bruno et al., 2005a,2009), and since 2006 also in patients with allergic rhinitis (Unal et al., 2006; Bruno et al., 2019). With coronavirus disease 2019 (COVID-19) pandemic, it has been hypothesized a role of leptin in severity disease (Guglielmi et al., 2021; Wang et al., 2021). (B) Leptin and the gut-lung axis. Both leptin and microbiota influence innate and adaptive immune system and are critical for maintaining homeostasis of the immune system in the lungs (iBALT = inducible bronchus-associated lymphoid tissue) and in the gut (GALT = gut-associated lymphoid tissue). High fiber diet can increase the prevalence of Bacteroidetes species as well as the production of short chain fatty acids (SCFAs), which maintain the health immune system through the induction of regulatory T cells (Tregs) and interleukin-10 (IL-10) production and by inhibiting inflammation. On the other hand, high fat diet can increase both adipose tissue and Firmicutes species, associated with dysbiosis, inflammation, and with increased of effector T cells and IL-17 and tumor necrosis factor-α (TNF-α) production as well as the level of circulating leptin. This latter is also increased by dysbiosis. In turn, lung disorders and respiratory infections boots dysbiosis.
High leptin concentrations are directly associated with obesity and/or the subsequent development of metabolic disease sequelae, such as insulin resistance, type 2 diabetes, and cardiovascular diseases (Ghadge and Khaire, 2019), all key risk factors associated with increased coronavirus disease 2019 (COVID-19) mortality (Kim et al., 2021; Mohammad et al., 2021). In addition, it has been assessed that an increased leptinemia is observed not only in patients with obesity and metabolic syndrome but also in patients who are not obese but affected by other inflammatory diseases, such as sepsis and respiratory infections (Vernooy et al., 2013; Birlutiu and Boicean, 2021; Karampela et al., 2021). Furthermore, increased value of leptin is one of the factors that raised the risk of non-alcoholic fatty liver disease presence in patients with prediabetes (Vesa et al., 2020) as well, after adjusting for body mass index (BMI) or fat mass, serum leptin levels result positively and independently associated with peripheral artery disease (Zahner et al., 2019).
Angiotensin converting enzyme 2 (ACE-2), a receptor required for the entry of severe acute respiratory syndrome coronavirus 2 (SARS-CoV-2) into the cells, is expressed on lungs, gut, pancreas, kidneys, heart, in vessels blood, and adipose tissue. Emerging evidence indicates that the ACE-2 expression is increased in individuals who are obese and overweight (Mohammad et al., 2021). Indeed, obesity appears to be a risk factor for worsening the severity of COVID-19 or of SARS-CoV-2 infection: large retrospective studies report a greater rates of obesity among patients with severe COVID-19 (Guerson-Gil et al., 2021; Zhou et al., 2021).
Similar to leptin, the gut microbiota is involved in the development and preservation of the immune system, energy homeostasis, and nutritional status (Belkaid and Hand, 2014; D’Argenio and Salvatore, 2015). Dysbiosis, altered microbiota composition, is associated with an increase in the proportion of bacteria with a pro-inflammatory profile, with a low-grade, persistent and systemic inflammation, and with poor outcomes in patients with COVID-19 (Magalhães et al., 2021; Moreira-Rosário et al., 2021). Interestingly, the gut microbiota may affect lung health through a crosstalk called the “gut-lung axis.”
The contribution of pro-inflammatory adipocytokine as leptin, together with the host microbiota, in modulating the immune system is a topic of interest in this research field.
Leptin, at a Glance
Leptin is a 16-kD non-glycosylated hormone of 167 amino acids discovered in 1994 (Zhang et al., 1994) with a tertiary structure resembling that of members of the long-chain helical cytokine family. Leptin production is related to the amount of fat tissue (Considine et al., 1996), despite variability in plasma leptin concentration is independent from fat (Behnes et al., 2012) as leptin is produced by placenta, gastric mucosa, mammary gland, skeletal muscle, brain, intestine, bone marrow, and lymphoid tissues (Wolsk et al., 2012; Vernooy et al., 2013; Li et al., 2017; Pan et al., 2017; Pérez-Pérez et al., 2018).
Leptin receptor was found in lungs both from human and animals (Wang et al., 1996; De Matteis et al., 1998; Bruno et al., 2005a,2009) and in human inferior turbinates (Bruno et al., 2019). Since a while, it has been assessed that leptin displays many faces in the respiratory system, from lung embryogenic to maturation and to the control of ventilation (Tankersley et al., 1998; Takabatake et al., 1999; Jutant et al., 2021). All these functions are mainly related to the leptin-signaling pathway involvement in immune modulation (La Cava and Matarese, 2004; Procaccini et al., 2017).
Leptin modulates both innate and adaptive immune responses in monocytes/macrophages (Curat et al., 2004), neutrophils, eosinophils, effector, and regulatory T lymphocytes (Bruno et al., 2005b; Conus et al., 2005; Lourenço et al., 2016). Furthermore, a large amount of literature reports an important association between leptin and inflammation (Naylor and Petri, 2016; Becerril et al., 2018; Ziegler et al., 2019; Schoeman and Fielding, 2021).
Leptin modulation of inflammatory responses can directly contribute to the pathophysiology of COVID-19. Leptin activates monocytes promoting cytokine storm that contribute to severe respiratory distress syndrome and multiple-organ failure in COVID-19. Upon infection, patients with excessive fat mass would be prone to produce more leptin. High levels of leptin were associated with inflammatory mediators and disease severity in obese and not obese patients (Vernooy et al., 2013; Birlutiu and Boicean, 2021; Karampela et al., 2021; Wang et al., 2021). A state of low-grade, chronic inflammation, as observed in obesity, has been associated with altered leptin levels, impaired immune system, and host defenses: obese subjects are more susceptible to respiratory virus infection, to a greater severity of illness prolonged viral shed, increased viral diversity, and adverse endpoints after diseases, until death (Andersen et al., 2016; Aquino-Junior et al., 2018).
Leptin together with other cytokines, could be promising new biological markers and therapeutic targets in obesity-related diseases (Ghadge and Khaire, 2019; Bruno et al., 2021). As leptin deficiency/resistance has been associated with immune dysregulation and altered cytokine production, impairments of leptin signaling may hinder the cooperative interplay of the immunologic, metabolic, and neuro-endocrinologic processes (White et al., 2013; Guglielmi et al., 2021), potentially playing a role in driving the COVID-19 cytokine storm. In addition, leptin sensitivity may be modulated by gut microbiota (Heiss and Olofsson, 2018).
The Microbiota as a Modulator of Leptin Sensitivity
The term “microbiota” identifies all microorganisms, mainly bacteria and a small number of fungi, archaea, and viruses, that live on the surface and inside our body (D’Argenio and Salvatore, 2015). Most of the adult human microbiota lives in the gut but it also colonizes the oral cavity, skin, vagina, and the lung (Dickson et al., 2016; Blum, 2017; Maschirow et al., 2019; Campisciano et al., 2021). These communities of microorganisms are essential for human physiology and survival and for this reason the microbiota is called the “forgotten organ” (O’Hara and Shanahan, 2006).
There is a strong link between the diet, the gut microbiota, and the effects on the metabolism of host (Tremaroli and Bäckhed, 2012). The gut microbiota regulates the host energy homeostasis as it is involved in the absorption of host nutrients, in maintaining the integrity of the intestinal immune barrier, in the regulation of host fat storage genes, and pathways that modulate appetite, intestinal motility, and energy expenditure (Backhed et al., 2004; Duca and Lam, 2014; Bagarolli et al., 2017). Alterations of the gut microbiota are associated with obesity and vice versa. Studies have shown that a high-fat diet can cause imbalances in the composition of the gut microbiota with a decrease of phylogenetic diversity and that germ-free animals are protected from this (Cani et al., 2008; Duncan et al., 2008; Bagarolli et al., 2017). At the same time, other studies have showed that the obese phenotype can be transferred by gut microbiota transplantation (Turnbaugh et al., 2006; Heiss and Olofsson, 2018).
Leptin sensitivity can be influenced by multiple factors, such as diet and gut homeostasis (Gabriel and Fantuzzi, 2019), which in turn is profoundly affected by gut microbiota asset. Microbiota metabolites, such as short-chain fatty acids (SCFAs) as acetate, propionate, and butyrate, can activate the specific signaling pathways in the host and regulate the secretion of hormones, such as glucagon-like peptide, peptide YY, and leptin itself, affecting the gut motility and the fat storage in the adipose tissue (Heiss and Olofsson, 2018). It was found that SCFAs, produced by the gut microbiota, stimulate the production of leptin in mouse adipocyte cultures through the activation of the G-protein coupled receptor (GPR) 41 and their oral administration in mice also increases the concentrations of circulating leptin (Xiong et al., 2004). In a study on diet-induced obese and type 2 diabetic mice, it has been assessed that prebiotics (good food for microbiota) improve leptin sensitivity in an altered gut microbiota composition, suggesting that gut microbiota modulations could be a novel therapeutic target to reset leptin sensitivity (Everard et al., 2011). Furthermore, it has been reported that leptin receptor deficient mice (db/db) have lower Bacteroidetes and higher Firmicutes proportions than wild-type mice (Rajala et al., 2014) as both leptin and adiponectin supplementation throughout the suckling period are able to modify both the intraepithelial lymphocytes and gut microbiota composition in mice (Grases-Pintó et al., 2019). An experimental study performed in humans in overweight/obese mothers, with high leptin concentration in their breast milk, reported a lower abundance of Proteobacteria phylum in the infant gut microbiota (Lemas et al., 2016). Specific bacterial strains are associated with the release of leptin. A study demonstrates that amounts of Bifidobacterium and Lactobacillus correlate positively with plasma concentrations of leptin (Queipo-Ortuno et al., 2013). Intestinal dysbiosis has been reported to be associated with chronically increased leptin levels and decreased sensitivity to leptin, through the induction of the suppressor of cytokine signaling 3 (SOCS3) and the suppression of the brain-derived neurotrophic factor (BDNF) expression in the hypothalamus (Schéle et al., 2013), and decreased expression of obesity-suppressing neuropeptides in the central nervous system (Yao et al., 2020). All evidence aimed to assess a possible modulation by microbiota on leptin expression/sensitivity and vice versa.
Gut microbiota, as leptin, influences and modulates inflammation and immune systems (Macpherson and Harris, 2004; Lynch and Pedersen, 2016; Bizzoca et al., 2020; Mohammadi et al., 2020; Yang et al., 2020). Commensal bacteria are recognized by the innate immune system and thus the microbiota plays a role in regulating the development, homeostasis, and function of innate and adaptive immune cells (Brestoff and Artis, 2013), avoids inflammation and bacterial translocation and hinders the colonization of pathogens (Maschirow et al., 2019).
In addition, gut microbiota dysbiosis is associated with lung disorders and respiratory infections (Trompette et al., 2014; Shukla et al., 2017). Changes in species and the proportion of bacteria in the gut are associated with asthma, lung disease, and allergic inflammation (Kalliomaki et al., 2001; Russell et al., 2013). Conversely, changes in the lung microbial community due to viral infections modify the composition of the gut microbiota leading to intestinal dysbiosis (Ichinohe et al., 2011). All these findings confirm that gut microbiota systematically influences the lung microbiota and this strictly interconnection is termed “gut-lung axis” (Marsland and Gollwitzer, 2014; McAleer and Kolls, 2018; Dang and Marsland, 2019).
Leptin and Gut-Lung Axis
A balance between host and gut microbiota is crucial to keep a healthy intestinal barrier and for healthy metabolism. Microbiota is believed to contribute to metabolism in humans as it has been reported that differences in the composition of the microbiota are related to obese or lean individuals (Turnbaugh et al., 2009). Moreover, microbiota plays a fundamental role in optimal immune homeostasis (Wu and Wu, 2012).
Gut-lung axis communicates through a bi-directional pathway in which endotoxins, or microbial metabolites, may affect the lung through the blood and, conversely, the lung inflammation affects the gut microbiota. To further support this axis in pathological contexts, it has been demonstrated a link between bowel and lung inflammatory diseases (Wypych et al., 2019; Raftery et al., 2020). This axis is established because there can be a direct seeding of intestinal bacteria into the lung through reflux and aspiration, but also because some products of the intestinal bacterial metabolism and nutrition can influence the composition and functionality of the lung microbiota. The production of SCFAs from dietary fiber by the intestinal microbiota increases the presence in the lung of dendritic cells with high phagocytic capacity and reduces the ability to promote the effector function of Th2 cells, thereby improving the allergic airway inflammation (Trompette et al., 2014). Multiple mechanisms could be postulated to explain how gut microbiota modulates lung immune responses. In this regard, it has been shown that human lung tissues expressed SCFAs receptor, free fatty acids receptor 2 and 3 (FFAR2 and FFAR3) (Liu et al., 2021), and the activation of these receptors influences the expression interleukin-1β (IL-1β) and in turn lung immune tone (Mizuta et al., 2020) and airway hyperactivity. Some micronutrients exert a relevant effect on gut microbiota leading to the production of specific metabolites that affect immune systems and in turn chronic disease development or evolution (Espírito Santo et al., 2021). High fiber intake can limit emphysema progression and mitigates the inflammatory response in cigarette smoke-exposed emphysema mice (Jang et al., 2021). A systematic review (Gabriel and Fantuzzi, 2019) analyses the relationship between SCFAs and leptin metabolism: it concludes that body fat, rather than SCFAs, remains the main driver for leptin synthesis in vivo and that the activation of FFAR3 increases leptin release and expression in vitro.
Furthermore, a cross-sectional study design (Yang et al., 2017) reports that the gut microbiota is associated with cardiorespiratory fitness in women, regardless of age and dietary intakes, with increased Eubacterium rectale-Clostridium coccoides (EreC) and Enterobacteria but lower Bacteroides and with low aerobic fitness and low maximum oxygen uptake (VO2 max). While VO2 max is negatively correlated with fat percentage and leptin, EreC is positively associated with fat percentage and leptin, but the relationship between VO2 max and EreC is confused by body fatness as the observed differences disappeared after adjusting of the fat percentage.
Recent evidence supports a relevant role of gut-lung axis in acute respiratory distress syndrome (ARDS) (Dickson et al., 2016), COVID-19 (Allali et al., 2021), and chronic obstructive pulmonary disease (COPD) pathogenesis (Lai et al., 2021). Several current studies are elucidating the mechanisms of how microbiota regulate lung inflammation and are providing useful information for considering the use of probiotic, prebiotic, and postbiotic therapies for lung disease, such as COVID-19 (Tsai et al., 2019; Gasmi et al., 2021). Microbial-derived components (postbiotics) elicit the activation of downstream cascades capable to modulate both local and systemic immune responses.
Leptin, Respiratory Health, and Sars-CoV-2 Infection
According to increasing scientific evidence, leptin can modulate respiratory health through pleiotropic actions (Jutant et al., 2021). First, leptin has been reported to play a role in lung development and in the maturation of fetal lungs, as it seems to be involved in surfactant proteins production by fetal type II cells (Torday et al., 2002). In addition, leptin can modulate bronchial diameter, by counteracting the parasympathetic effect on the airways (Arteaga-Solis et al., 2013). Finally, congenital leptin-deficient patients show defects in immunity and are at risk of death due to infections (Díez et al., 2008). Dysregulated leptin production and activity could be involved in the pathogenesis of several pulmonary diseases, such as COPD, idiopathic pulmonary fibrosis, lung cancer, and pulmonary arterial hypertension. Interestingly, its role appears to be both protective through bronchodilation and negative by promoting inflammation in patients with asthma. Furthermore, leptin appears to be protective against respiratory infections. In patients hospitalized for pneumonia, leptin levels were inversely correlated with markers of inflammation (Jutant et al., 2021). More recently, a significant association between high plasma leptin levels and risk of severe respiratory infections was found in a cohort of ambulatory patients, independent on BMI and other risk factors (Ubags et al., 2016). In obese patients with a viral infection, altered leptin sensitivity may contribute to a dramatic pro-inflammatory cytokine response and to an inefficient response to infection (Alti et al., 2018). It has been suggested that leptin could be involved in the etiology of several effects commonly observed in patients with COVID-19. For instance, the frequently reported anosmia (Gane et al., 2020) may be partly ascribed to the ability of leptin to alter the olfactory epithelium (Savigner et al., 2009). One study recently reported a positive correlation between serum leptin levels and BMI of adult patients infected with SARS-CoV-2 (van der Voort et al., 2020). Wang et al. observed that patients with COVID-19 with a high BMI had significantly high levels of leptin, which were associated with inflammatory mediators and disease severity in such patients. Of note, leptin levels are increased in patients with COVID-19 compared with controls as well as in severe patients with COVID-19 compared with mild patients (Wang et al., 2021). Therefore, it seems that, upon infection, patients with excessive fat mass are prone to produce more leptin, which in turn activates monocytes promoting that cytokine storm that has been recognized to contribute to severe respiratory distress syndrome and multiple-organ failure in COVID-19. Furthermore, leptin is inhibited by ACE-2 via alamandine production and activation of the MrgD-receptor/c/Src/p38MAPK pathway (Uchiyama et al., 2017). Therefore, it has been hypothesized that in obese patients infected by SARS-CoV-2, the impaired ACE-2 function after the viral binding may increase leptin levels. This may contribute to the hyperinflammatory pulmonary response frequently observed in obese patients infected with SARS-CoV-2 (Guglielmi et al., 2021).
In summary, a weakened immune response can end in a heightened cytokine release that can prove fatal. However, the role of leptin in the pathogenesis of SARS-CoV-2 needs further investigation to be fully clarified.
Discussion and Conclusion
The COVID-19 pandemic continues to represent the worst health threat worldwide and to cause morbidity and mortality with more than 5,000,000 death cases reported to the WHO by November 2021.1
It has been assessed that the healthy microbiota of upper and lower respiratory tract plays several important roles in the development and maintenance of respiratory tract and whole organism homeostasis and the viral infections, such as that caused by SARS-CoV-2 may perpetuate a systemic inflammation via gut-lung axis (Banerjee et al., 2020; Belanger et al., 2020; Gheblawi et al., 2020). Indeed, gut dysbiosis may be linked to the onset of several pulmonary diseases, such as asthma (Huang et al., 2021.), COPD (Raftery et al., 2020), cystic fibrosis (Thavamani et al., 2021), and lung infections (Bajinka et al., 2021). A connection between the lungs and gut has been widely demonstrated in both human and mouse studies. Inducible bronchus-associated lymphoid tissue (iBALT) and gut-associated lymphoid tissue (GALT) are strictly interconnected and both leptin and microbiota are important factors responsible for interactions between these two sites (Figure 1B).
Leptin could represent an important player in the gut-lung axis (Di Renzo et al., 2020). It has been hypothesized that in obese patients infected by SARS-CoV-2, the impaired ACE-2 function after the viral binding may increase leptin levels, thereby contributing to the hyperinflammatory pulmonary response (Guglielmi et al., 2021). This framework may explain the occurrence of respiratory failure which has been commonly observed in overweight/obese patients. It has been widely assessed that obesity has an adverse effect on respiratory physiology both for mechanical factors and for impaired adipocyte-mediated immune function by increased levels of pro-inflammatory cytokines and by decreased anti-inflammatory adipokines. Majority of the observational and retrospective cohort studies on thousands of patients with COVID-19 report that obese subjects are at increased risk of severe disease and increased mortality due to COVID-19 (Asare et al., 2020; Gazzaruso et al., 2020; Pettit et al., 2020; Guerson-Gil et al., 2021; Rapp et al., 2021). Since respiratory failure usually takes place at 8–12 days from the initial signs of infection, there would be a window of opportunity to intervene, for instance by downregulating the leptin production (van der Voort et al., 2020). A relevant aspect also concerns the role of the leptin-immune axis, as leptin can impair antibodies production and class switching of immunoglobulin. Indeed, increased leptin levels are recognized as the mediating factor linking metabolism and immunity and are thought to predispose to increased morbidity and mortality for SARS-CoV-2 infection through an impairment of the immune response (Rebello et al., 2020).
On the basis of the evidence here provided, it could be possible to hypothesize a relevant role of leptin in the increased levels of pro-inflammatory mediators in the obese patients with COVID-19. Leptin can disrupt the release of anti-inflammatory cytokines and anti-inflammatory adipokines, leading to the impairment of the normal immune function and perpetuating the progression and the severity of chronic diseases as well as infections, such as COVID-19. However, nowadays, it is still to be explored whether leptin could be used in clinical practice as a pro-inflammatory biomarker of disease progression and severity to predict the patient prognosis (Wang et al., 2021).
At the same time, the interest of the scientific pre-clinical and clinical research in gut microbiota is growing, but the experimental studies in this field are still at the beginning. Our review is illustrative and aim to focus the next-future research for lung diseases and COVID-19 specifically in the field of the interaction between leptin and microbiota, as both widely involved in the regulation and immune and inflammatory systems. Anyway, the presence of the functional leptin receptor in the lung together with evidence of local leptin production, supports the concept that leptin plays an important role in lung health (Vernooy et al., 2013).
In conclusion, obesity-associate chronic inflammation impairs immune function and increases ACE-2 expression resulting in an increased disease severity and worse clinical outcome in obese subjects with COVID-19. The goal in this field is to understand the contribution of pro-inflammatory adipocytokine as leptin, together with the host microbiota in modulating the immune system. We strongly suggest that the next prospective studies in lung infections may be integrated and be given an interdisciplinary approach, included nutritional status, and gut microbiota, as these new insights could be translated into preventive and therapeutic measures for COVID-19 (Figure 2).
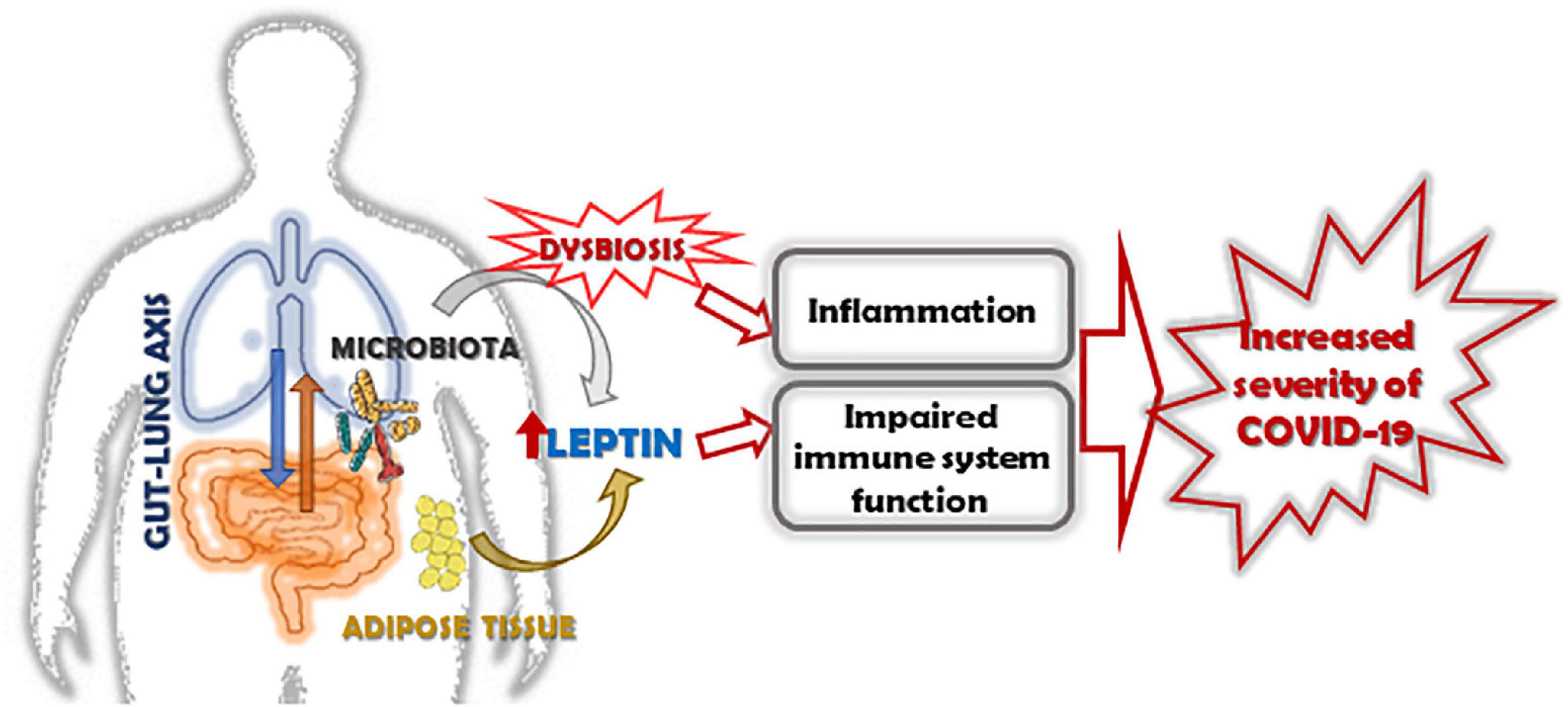
Figure 2. Role of leptin in increasing the severity of COVID-19 in obese subjects. The altered expression of leptin/leptin receptor pathway and the increase of leptin resistance in obese subjects, together with the alteration of microbiota, induces inflammation, and impairs the function of the immune system. In this context, leptin and dysbiosis could be key factors associated with increased severity of COVID-19 in obese subjects.
Author Contributions
AB and SD: conceptualization. AB, EP, SD, and GF: writing original draft. AB, EP, GF, and SL: review and editing. All authors contributed to the article and approved the submitted version.
Conflict of Interest
The authors declare that the research was conducted in the absence of any commercial or financial relationships that could be construed as a potential conflict of interest.
Publisher’s Note
All claims expressed in this article are solely those of the authors and do not necessarily represent those of their affiliated organizations, or those of the publisher, the editors and the reviewers. Any product that may be evaluated in this article, or claim that may be made by its manufacturer, is not guaranteed or endorsed by the publisher.
Footnotes
References
Allali, I., Bakri, Y., Amzazi, S., and Ghazal, H. (2021). Gut-Lung Axis in COVID-19. Interdiscip. Perspect. Infect Dis. 2021:6655380. doi: 10.1155/2021/6655380
Alti, D., Sambamurthy, C., and Kalangi, S. K. (2018). Emergence of leptin in infection and immunity: scope and challenges in vaccines formulation. Front. Cell Infect. Microbiol. 8:147. doi: 10.3389/fcimb.2018.00147
Andersen, C. J., Murphy, K. E., and Fernandez, M. L. (2016). Impact of obesity and metabolic syndrome on immunity. Adv. Nutr. 7, 66–75. doi: 10.3945/an.115.010207
Aquino-Junior, J. C. J., MacKenzie, B., Almeida-Oliveira, A. R., Martins, A. C., Oliveira-Junior, M. C., and Britto, A. A. (2018). Aerobic exercise inhibits obesity-induced respiratory phenotype. Cytokine 104, 46–52. doi: 10.1016/j.cyto.2017.12.025
Arteaga-Solis, E., Zee, T., Emala, C. W., Vinson, C., Wess, J., and Karsenty, G. (2013). Inhibition of leptin regulation of parasympathetic signaling as a cause of extreme body weight-associated asthma. Cell Metab. 17, 35–48. doi: 10.1016/j.cmet.2012.12.004
Asare, S., Sandio, A., Opara, I. N., Riddle-Jones, L., Palla, M., Renny, N., et al. (2020). Higher obesity trends among african americans are associated with increased mortality in infected COVID-19 patients within the city of detroit. SN Compr. Clin. Med. Online ahead of print. doi: 10.1007/s42399-020-00385-y
Backhed, F., Ding, H., Wang, T., Hooper, L. V., Koh, G. Y., Nagy, A., et al. (2004). The gut microbiota as an environmental factor that regulates fat storage. Proc. Natl. Acad. Sci. U S A 101, 15718–15723. doi: 10.1073/pnas.0407076101
Bagarolli, R. A., Tobar, N., Oliveira, A. G., Araújo, T. G., Carvalho, B. M., and Rocha, G. Z. (2017). Probiotics modulate gut microbiota and improve insulin sensitivity in DIO mice. J. Nutr. Biochem. 50, 16–25. doi: 10.1016/j.jnutbio.2017.08.006
Bajinka, O., Simbilyabo, L., Tan, Y., Jabang, J., and Saleem, S. A. (2021). Lung-brain axis. Crit. Rev. Microbiol. 4, 1–13. doi: 10.1080/1040841X.2021.1960483
Banerjee, M., Gupta, S., Sharma, P., Shekhawat, J., and Gauba, K. (2020). Obesity and COVID-19: a fatal alliance. Indian J. Clin. Biochem. 35, 1–8. doi: 10.1007/s12291-020-00909-2
Becerril, S., Rodríguez, A., Catalán, V., Méndez-Giménez, L., Ramírez, B., Sáinz, N., et al. (2018). Targeted disruption of the iNOS gene improves adipose tissue inflammation and fibrosis in leptin-deficient ob/ob mice: role of tenascin C. Int. J. Obes. 42, 1458–1470. doi: 10.1038/s41366-018-0005-5
Behnes, M., Brueckmann, M., Lang, S., Putensen, C., Saur, J., Borggrefe, M., et al. (2012). Alterations of leptin in the course of inflammation and severe sepsis. BMC Infect. Dis. 12:217. doi: 10.1186/1471-2334-12-217
Belanger, M. J., Hill, M. A., Angelidi, A. M., Dalamaga, M., Sowers, J. R., and Mantzoros, C. S. (2020). Covid-19 and disparities in nutrition and obesity. N. Engl. J. Med. 383:e69. doi: 10.1056/NEJMp2021264
Belkaid, Y., and Hand, T. W. (2014). Role of the microbiota in immunity and inflammation. Cell 157, 121–141. doi: 10.1016/j.cell.2014.03.011
Bergen, H. T., Cherlet, T. C., Manuel, P., and Scott, J. E. (2002). Identification of leptin receptors in lung and isolated fetal type II cells. Am. J. Respir. Cell Mol. Biol. 27, 71–77. doi: 10.1165/ajrcmb.27.1.4540
Birlutiu, V., and Boicean, L. C. (2021). Serum leptin level as a diagnostic and prognostic marker in infectious diseases and sepsis: a comprehensive literature review. Medicine 100:e25720. doi: 10.1097/md.0000000000025720
Bizzoca, D., Solarino, G., Vicenti, G., Moretti, L., Nappi, V. S., Belluati, A., et al. (2020). Novel directions in the study of osteoporosis: focus on gut microbiota as a potential therapeutic target. J. Biol. Regul. Homeost. Agents 34, 29–35.
Blum, H. E. (2017). The human microbiome. Adv. Med. Sci. 62, 414–420. doi: 10.1016/j.advms.2017.04.005
Brandao-Rangel, M. A. R., Moraes-Ferreira, R., Oliveira-Junior, M. C., Santos-Dias, A., Bachi, A. L. L., and Gabriela-Pereira, G. (2021). Pulmonary function changes in older adults with and without metabolic syndrome. Sci. Rep. 11:17337. doi: 10.1038/s41598-021-96766-x
Brestoff, J. R., and Artis, D. (2013). Commensal bacteria at the interface of host metabolism and the immune system. Nat. Immunol. 14, 676–684. doi: 10.1038/ni.2640
Bruno, A., Chanez, P., Chiappara, G., Siena, L., Giammanco, S., Gjomarkaj, M., et al. (2005a). Does leptin play a cytokine-like role within the airways of COPD patients? Eur. Respir. J. 26, 398–405. doi: 10.1183/09031936.05.00092404
Bruno, A., Conus, S., Schmid, I., and Simon, H. U. (2005b). Apoptotic pathways are inhibited by leptin receptor activation in neutrophils. J. Immunol. 174, 8090–8096. doi: 10.4049/jimmunol.174.12.8090
Bruno, A., Di Sano, C., Lorusso, F., Dino, P., Russo, D., and Ballacchino, A. (2019). Notch-1 decreased expression contributes to leptin receptor downregulation in nasal epithelium from allergic turbinates. Biochim. Biophys. Acta Mol. Basis Dis. 1865, 1642–1650. doi: 10.1016/j.bbadis.2019.03.016
Bruno, A., Di Sano, C., Simon, H. U., Chanez, P., Patti, A. M., Di Vincenzo, S., et al. (2021). Leptin and TGF-beta1 downregulate PREP1 expression in human adipose-derived mesenchymal stem cells and mature adipocytes. Front. Cell Dev. Biol. 9:700481. doi: 10.3389/fcell.2021.700481
Bruno, A., Pace, E., Chanez, P., Gras, D., Vachier, I., Chiappara, G., et al. (2009). Leptin and leptin receptor expression in asthma. J. Allergy Clin. Immunol. 124, 230–237.e1-4. doi: 10.1016/j.jaci.2009.04.032
Bruno, A., Siena, L., Gerbino, S., Ferraro, M., Chanez, P., Giammanco, M., et al. (2011). Apigenin affects leptin/leptin receptor pathway and induces cell apoptosis in lung adenocarcinoma cell line. Eur. J. Cancer 47, 2042–2051. doi: 10.1016/j.ejca.2011.03.034
Campisciano, G., Quadrifoglio, M., Comar, M., De Seta, F., Zanotta, N., Ottaviani, C., et al. (2021). Evidence of bacterial DNA presence in chorionic villi and amniotic fluid in the first and second trimester of pregnancy. Future Microbiol. 16, 801–810. doi: 10.2217/fmb-2020-0243
Cani, P. D., Bibiloni, R., Knauf, C., Waget, A., Neyrinck, A. M., Delzenne, N. M., et al. (2008). Changes in gut microbiota control metabolic endotoxemia-induced inflammation in highfat diet-induced obesity and diabetes in mice. Diabetes 57, 1470–1481. doi: 10.2337/db07-1403
Considine, R. V., Sinha, M. K., Heiman, M. L., Kriauciunas, A., Stephens, T. W., Nyce, M. R., et al. (1996). Serum immunoreactive-leptin concentrations in normal-weight and obese humans. N. Engl. J. Med. 334, 292–295. doi: 10.1056/NEJM199602013340503
Conus, S., Bruno, A., and Simon, H. U. (2005). Leptin is an eosinophil survival factor. J. Allergy Clin. Immunol. 116, 1228–1234. doi: 10.1016/j.jaci.2005.09.003
Curat, C. A., Miranville, A., Sengenès, C., Diehl, M., Tonus, C., Busse, R., et al. (2004). From blood monocytes to adipose tissue-resident macrophages: induction of diapedesis by human mature adipocytes. Diabetes Metab. Res. Rev. 53, 1285–1292. doi: 10.2337/diabetes.53.5.1285
Dang, A. T., and Marsland, B. J. (2019). Microbes, metabolites, and the gut-lung axis. Mucosal Immunol. 12, 843–850. doi: 10.1038/s41385-019-0160-6
D’Argenio, V., and Salvatore, F. (2015). The role of the gut microbiome in the healthy adult status. Clin. Chim. Acta 451, 97–102. doi: 10.1016/j.cca.2015.01.003
de Candia, P., Prattichizzo, F., Garavelli, S., Alviggi, C., La Cava, A., and Matarese, G. (2021). The pleiotropic roles of leptin in metabolism, immunity, and cancer. J. Exp. Med. 218:e20191593. doi: 10.1084/jem.20191593
De Matteis, R., Dashtipour, K., Ognibene, A., and Cinti, S. (1998). Localization of leptin receptor splice variants in mouse peripheral tissues by immunohistochemistry. Proc. Nutr. Soc. 57, 441–448. doi: 10.1079/pns19980063
Di Renzo, L., Gualtieri, P., Pivari, F., Soldati, L., Attinà, A., and Leggeri, C. (2020). COVID-19: is there a role for immunonutrition in obese patient? J. Transl. Med. 18:415. doi: 10.1186/s12967-020-02594-4
Dickson, R. P., Erb-Downward, J. R., Martinez, F. J., and Huffnagle, G. B. (2016). The microbiome and the respiratory tract. Annu. Rev. Physiol. 78, 481–504. doi: 10.1146/annurev-physiol-021115-105238
Díez, M. L., Santolaria, F., Tejera, A., Alemán, M. R., González-Reimers, E., Milena, A., et al. (2008). Serum leptin levels in community acquired pneumonia (CAP) are related to nutritional status and not to acute phase reaction. Cytokine 42, 156–160. doi: 10.1016/j.cyto.2008.02.013
Duca, F. A., and Lam, T. K. (2014). Gut microbiota, nutrient sensing and energy balance. Diabetes Obes. Metab. 16(Suppl. 1), 68–76. doi: 10.1111/dom.12340
Duncan, S. H., Lobley, G. E., Holtrop, G., Ince, J., Johnstone, A. M., Louis, P., et al. (2008). Human colonic microbiota associated with diet, obesity and weight loss. Int. J. Obes. 32, 1720–1724.
Espírito Santo, C., Caseiro, C., Martins, M. J., Monteiro, R., and Brandão, I. (2021). Gut Microbiota, in the halfway between nutrition and lung function. Nutrients 13:1716. doi: 10.1038/ijo.2008.155
Everard, A., Lazarevic, V., Derrien, M., Girard, M., Muccioli, G. G., Neyrinck, A. M., et al. (2011). Responses of gut microbiota and glucose and lipid metabolism to prebiotics in genetic obese and diet-induced leptin-resistant mice. Diabetes 60, 2775–2786. doi: 10.3390/nu13051716
Gabriel, F. C., and Fantuzzi, G. (2019). The association of short-chain fatty acids and leptin metabolism: a systematic review. Nutr. Res. 72, 18–35. doi: 10.1016/j.nutres.2019.08.006
Gane, S. B., Kelly, C., and Hopkins, C. (2020). Isolated sudden onset anosmia in COVID-19 infection. a novel syndrome? Rhinology 58, 299–301. doi: 10.4193/Rhin20.114
Gasmi, A., Tippairote, T., Mujawdiya, P. K., Peana, M., Menzel, A., Dadar, M., et al. (2021). The microbiota-mediated dietary and nutritional interventions for COVID-19. Clin. Immunol. 226:108725. doi: 10.1016/j.clim.2021.108725
Gazzaruso, C., Paolozzi, E., Valenti, C., Brocchetta, M., Naldani, D., Grignani, C., et al. (2020). Association between antithrombin and mortality in patients with COVID-19. a possible link with obesity. Nutr. Metab. Cardiovasc. Dis. 30, 1914–1919. doi: 10.1016/j.numecd.2020.07.040
Ghadge, A. A., and Khaire, A. A. (2019). Leptin as a predictive marker for metabolic syndrome. Cytokine 121:154735. doi: 10.1016/j.cyto.2019.154735
Gheblawi, M., Wang, K., Viveiros, A., Nguyen, Q., Zhong, J. C., Turner, A. J., et al. (2020). Angiotensin-converting enzyme 2: SARS-CoV-2 receptor and regulator of the renin-angiotensin system: celebrating the 20th anniversary of the discovery of ACE2. Circ. Res. 126, 1456–1474. doi: 10.1161/CIRCRESAHA.120.317015
Grases-Pintó, B., Abril-Gil, M., Castell, M., Rodríguez-Lagunas, M. J., Burleigh, S., Fåk Hållenius, F., et al. (2019). Influence of leptin and adiponectin supplementation on intraepithelial lymphocyte and microbiota composition in suckling rats. Front. Immunol. 10:2369. doi: 10.3389/fimmu.2019.02369
Guerson-Gil, A., Palaiodimos, L., Assa, A., Karamanis, D., Kokkinidis, D., Chamorro-Pareja, N., et al. (2021). Sex-specific impact of severe obesity in the outcomes of hospitalized patients with COVID-19: a large retrospective study from the Bronx, New York. Eur. J. Clin. Microbiol. Infect. Dis. 40, 1963–1974.
Guglielmi, V., Colangeli, L., D’Adamo, M., and Sbraccia, P. (2021). Susceptibility and severity of viral infections in obesity: lessons from influenza to covid-19. does leptin play a role? Int. J. Mol. Sci. 22:3183.
Heiss, C. N., and Olofsson, L. E. (2018). Gut microbiota-dependent modulation of energy metabolism. J. Innate Immun. 10, 163–171.
Heuck, C., and Wolthers, O. D. (1999). Serum leptin in children with asthma treated with inhaled budesonide. Respir. Med. 93, 268–271. doi: 10.1016/S0954-6111(99)90023-9
Huang, Y. C., Wu, M. C., Wang, Y. H., and Wei, J. C. (2021). The influence of constipation on asthma: a real-world, population-based cohort study. Int. J. Clin. Pract. 16:e14540. doi: 10.1111/ijcp.14540
Ichinohe, T., Pang, I. K., Kumamoto, Y., Peaper, D. R., Ho, J. H., Murray, T. S., et al. (2011). Microbiota regulates immune defense against respiratory tract influenza A virus infection. Proc. Natl. Acad. Sci. U.S.A. 108, 5354–5359. doi: 10.1073/pnas.1019378108
Jang, Y. O., Kim, O. H., Kim, S. J., Lee, S. H., Yun, S., Lim, S. E., et al. (2021). High-fiber diets attenuate emphysema development via modulation of gut microbiota and metabolism. Sci. Rep. 11:7008.
Jutant, E. M., Tu, L., Humbert, M., Guignabert, C., and Huertas, A. (2021). The thousand faces of leptin in the lung. Chest 159, 239–248. doi: 10.1016/j.chest.2020.07.075
Kalliomaki, M., Kirjavainen, P., Eerola, E., Kero, P., Salminen, S., and Isolauri, E. (2001). Distinct patterns of neonatal gut microflora in infants in whom atopy was and was not developing. J. Allergy Clin. Immunol. 107, 129–134. doi: 10.1067/mai.2001.111237
Karampela, I., Chrysanthopoulou, E., Skyllas, G., Christodoulatos, G. S., Kandri, E., Antonakos, G., et al. (2021). Circulating leptin, soluble leptin receptor and free leptin index in critically ill patients with sepsis: a prospective observational study. Minerva Anestesiol. 87, 880–890.
Kielar, D., Clark, J. S., Ciechanowicz, A., Kurzawski, G., Sulikowski, T., and Naruszewicz, M. (1998). Leptin receptor isoforms expressed in human adipose tissue. Metabolism 47, 844–847. doi: 10.1016/S0026-0495(98)90124-X
Kim, C. W., Aronow, W. S., and Frishman, W. H. (2021). COVID-19 and cardiometabolic disease. Cardiol. Rev. doi: 10.1097/CRD.0000000000000405
La Cava, A., and Matarese, G. (2004). The weight of leptin in immunity. Nat. Rev. Immunol. 4, 371–379.
Lai, H. C., Lin, T. L., Chen, T. W., Kuo, Y. L., Chang, C. J., Wu, T. R., et al. (2021). Gut microbiota modulates COPD pathogenesis: role of anti-inflammatory Parabacteroides goldsteinii lipopolysaccharide. Gut Online ahead of print. doi: 10.1136/gutjnl-2020-322599
Lemas, D. J., Young, B. E., Baker, P. R., Tomczik, A. C., Soderborg, T. K., and Hernandez, T. L. (2016). Alterations in human milk leptin and insulin are associated with early changes in the infant intestinal microbiome. Am. J. Clin. Nutr. 103, 1291–1300. doi: 10.3945/ajcn.115.126375
Li, F., Li, Y., Duan, Y., Hu, C. A., Tang, Y., and Yin, Y. (2017). Myokines and adipokines: involvement in the crosstalk between skeletal muscle and adipose tissue. Cytokine Growth Factor Rev. 33, 73–82. doi: 10.1016/j.cytogfr.2016.10.003
Liu, Q., Tian, X., Maruyama, D., Arjomandi, M., and Prakash, A. (2021). Lung immune tone via gut-lung axis: gut-derived LPS and short-chain fatty acids’ immunometabolic regulation of lung IL-1β, FFAR2, and FFAR3 expression. Am. J. Physiol. Lung. Cell Mol. Physiol. 321, L65–L78.
Lourenço, E. V., Liu, A., Matarese, G., and La Cava, A. (2016). Leptin promotes systemic lupus erythematosus by increasing autoantibody production and inhibiting immune regulation. Proc. Natl. Acad. Sci. U.S.A. 113, 10637–10642. doi: 10.1073/pnas.1607101113
Lynch, S. V., and Pedersen, O. (2016). The human intestinal microbiome in health and disease. N. Engl. J. Med. 375, 2369–2379.
Macpherson, A. J., and Harris, N. L. (2004). Interactions between commensal intestinal bacteria and the immune system. Nat. Rev. Immunol. 4, 478–485. doi: 10.1038/nri1373
Magalhães, N. S., Savino, W., Silva, P. M. R., Martins, M. A., and Carvalho, V. F. (2021). Gut microbiota dysbiosis is a crucial player for the poor outcomes for COVID-19 in elderly, diabetic and hypertensive patients. Front. Med. 8:644751.
Malli, F., Papaioannou, A. I., Gourgoulianis, K. I., and Daniil, Z. (2010). The role of leptin in the respiratory system: an overview. Respir. Res. 11:152. doi: 10.1186/1465-9921-11-152
Mancuso, P., Curtis, J. L., Freeman, C. M., Peters-Golden, M., Weinberg, J. B., and Myers, M. G. (2018). Ablation of the leptin receptor in myeloid cells impairs pulmonary clearance of Streptococcus pneumoniae and alveolar macrophage bactericidal function. Am. J. Physiol. Lung. Cell Mol. Physiol. 315, L78–L86.
Marsland, B. J., and Gollwitzer, E. S. (2014). Host-microorganism interactions in lung diseases. Nat. Rev. Immunol. 14, 827–835. doi: 10.1038/nri3769
Maschirow, L., Suttorp, N., and Opitz, B. (2019). Microbiota-dependent regulation of antimicrobial immunity in the lung. Am. J. Respir. Cell Mol. Biol. 61, 284–289.
Maurya, R., Bhattacharya, P., Dey, R., and Nakhasi, H. L. (2018). Leptin functions in infectious diseases. Front. Immunol. 9:2741. doi: 10.3389/fimmu.2018.02741
McAleer, J. P., and Kolls, J. K. (2018). Contributions of the intestinal microbiome in lung immunity. Eur. J. Immunol. 48, 39–49.
Mizuta, K., Sasaki, H., Zhang, Y., Matoba, A., and Emala, C. W. (2020). The short-chain free fatty acid receptor FFAR3 is expressed and potentiates contraction in human airway smooth muscle. Am. J. Physiol. Lung. Cell Mol. Physiol. 318, L1248–L1260. doi: 10.1152/ajplung.00357.2019
Mohammad, S., Aziz, R., Al Mahri, S., Malik, S. S., Haji, E., Khan, A. H., et al. (2021). Obesity and COVID-19: what makes obese host so vulnerable? Immun. Ageing 18:1.
Mohammadi, S. O., Yadegar, A., Kargar, M., Mirjalali, H., and Kafilzadeh, F. (2020). The impact of Helicobacter pylori infection on gut microbiota-endocrine system axis; modulation of metabolic hormone levels and energy homeostasis. J. Diabetes Metab. Disord. 19, 1855–1861. doi: 10.1007/s40200-020-00608-y
Moreira-Rosário, A., Marques, C., Pinheiro, H., Araújo, J. R., Ribeiro, P., and Rocha, R. (2021). Gut microbiota diversity and c-reactive protein are predictors of disease severity in COVID-19 Patients. Front. Microbiol. 12: 705020.
Münzberg, H., and Morrison, C. D. (2015). Structure, production and signaling of leptin. Metabolism 64, 13–23. doi: 10.1016/j.metabol.2014.09.010
Naylor, C., and Petri, W. A. (2016). Leptin regulation of immune responses. Trends Mol. Med. 22, 88–98.
O’Hara, A. M., and Shanahan, F. (2006). The gut flora as a forgotten organ. EMBO Rep. 7, 688–693. doi: 10.1038/sj.embor.7400731
Pan, Y., Zhou, F., He, C., Hui, L., Huang, T., and Wei, Y. (2017). Leptin-LepRb expressed in gastric cancer patients and related to cancer-related depression. Biomed. Res. Int. 2017:6482842.
Pérez-Pérez, A., Toro, A., Vilariño-García, T., Maymó, J., Guadix, P., Dueñas, J. L., et al. (2018). Leptin action in normal and pathological pregnancies. J. Cell Mol. Med. 22, 716–727. doi: 10.1111/jcmm.13369
Pérez-Pérez, A., Vilariño-García, T., Fernández-Riejos, P., Martín-González, J., Segura-Egea, J. J., and Sánchez-Margalet, V. (2017). Role of leptin as a link between metabolism and the immune system. Cytokine Growth Factor Rev. 35, 71–84.
Pettit, N. N., MacKenzie, E. L., Ridgway, J. P., Pursell, K., Ash, D., Patel, B., et al. (2020). Obesity is associated with increased risk for mortality among hospitalized patients with COVID-19. Obesity 28, 1806–1810. doi: 10.1002/oby.22941
Procaccini, C., La Rocca, C., Carbone, F., De Rosa, V., Galgani, M., and Matarese, G. (2017). Leptin as immune mediator: interaction between neuroendocrine and immune system. Dev. Comp. Immunol. 66, 120–129. doi: 10.1016/j.dci.2016.06.006
Queipo-Ortuno, M. I., Seoane, L. M., Murri, M., Pardo, M., Gomez-Zumaquero, J. M., Cardona, F., et al. (2013). Gut microbiota composition in male rat models under different nutritional status and physical activity and its association with serum leptin and ghrelin levels. PLoS One 8:e65465. doi: 10.1371/journal.pone.0065465
Raftery, A. L., Tsantikos, E., Harris, N. L., and Hibbs, M. L. (2020). Links between inflammatory bowel disease and chronic obstructive pulmonary disease. Front. Immunol. 11:2144. doi: 10.3389/fimmu.2020.02144
Rajala, M. W., Patterson, C. M., Opp, J. S., Foltin, S. K., Young, V. B., and Myers, M. G. (2014). Leptin acts independently of food intake to modulate gut microbial composition in male mice. Endocrinology 155, 748–757. doi: 10.1210/en.2013-1085
Rapp, J. L., Lieberman-Cribbin, W., Tuminello, S., and Taioli, E. (2021). Male sex, severe obesity, older age, and chronic kidney disease are associated with COVID-19 severity and mortality in New York City. Chest 159, 112–115.
Rebello, C. J., Kirwan, J. P., and Greenway, F. L. (2020). Obesity, the most common comorbidity in SARS-CoV-2: is leptin the link? Int. J. Obes. 44, 1810–1817. doi: 10.1038/s41366-020-0640-5
Russell, S. L., Gold, M. J., Willing, B. P., Thorson, L., and Mcnagny, K. M. (2013). Finlay B. B. Perinatal antibiotic treatment affects murine microbiota, immune responses and allergic asthma. Gut Microbes 4, 158–164. doi: 10.4161/gmic.23567
Salum, K. C. R., Rolando, J. M., Zembrzuski, V. M., Carneiro, J. R. I., Mello, C. B., and Maya-Monteiro, C. M. (2021). When leptin is not there: a review of what nonsyndromic monogenic obesity cases tell us and the benefits of exogenous leptin. Front. Endocrinol. 12:722441.
Savigner, A., Duchamp-Viret, P., Grosmaitre, X., Chaput, M., Garcia, S., Ma, M., et al. (2009). Modulation of spontaneous and odorant-evoked activity of rat olfactory sensory neurons by two anorectic peptides, insulin and leptin. J. Neurophysiol. 101, 2898–2906. doi: 10.1152/jn.91169.2008
Schéle, E., Grahnemo, L., Anesten, F., Hallén, A., Bäckhed, F., and Jansson, J. O. (2013). The gut microbiota reduces leptin sensitivity and the expression of the obesity-suppressing neuropeptides proglucagon (Gcg) and brain-derived neurotrophic factor (Bdnf) in the central nervous system. Endocrinology 154, 3643–3651. doi: 10.1210/en.2012-2151
Schoeman, D., and Fielding, B. C. (2021). Leptin deficiency, caused by malnutrition, makes you susceptible to SARS-CoV-2 infection but could offer protection from severe COVID-19. mSphere 6:e31-21. doi: 10.1128/mSphere.00031-21
Shukla, S. D., Budden, K. F., Neal, R., and Hansbro, P. M. (2017). Microbiome effects on immunity, health and disease in the lung. Clin. Transl. Immunol. 6:e133. doi: 10.1038/cti.2017.6
Simons, J. P., Schols, A. M., Campfield, L. A., Wouters, E. F., and Saris, W. H. (1997). Plasma concentration of total leptin and human lung-cancer-associated cachexia. Clin. Sci. 93, 273–277.
Takabatake, N., Nakamura, H., Abe, S., Hino, T., Saito, H., Yuki, H., et al. (1999). Circulating leptin in patients with chronic obstructive pulmonary disease. Am. J. Respir. Crit. Care Med. 159, 1215–1219. doi: 10.1164/ajrccm.159.4.9806134
Tankersley, C. G., O’Donnell, C., Daood, M. J., Watchko, J. F., Mitzner, W., Schwartz, A., et al. (1998). Leptin attenuates respiratory complications associated with the obese phenotype. J. Appl. Physiol. 85, 2261–2269. doi: 10.1152/jappl.1998.85.6.2261
Thavamani, A., Salem, I., Sferra, T. J., and Sankararaman, S. (2021). Impact of altered gut microbiota and its metabolites in cystic fibrosis. Metabolites 11:123. doi: 10.3390/metabo11020123
Torday, J. S., Sun, H., Wang, L., Torres, E., Sunday, M. E., and Rubin, L. P. (2002). Leptin mediates the parathyroid hormone-related protein paracrine stimulation of fetal lung maturation. Am. J. Physiol. Lung. Cell Mol. Physiol. 282, L405–L410. doi: 10.1152/ajplung.2002.282.3.L405
Tremaroli, V., and Bäckhed, F. (2012). Functional interactions between the gut microbiota and host metabolism. Nature 489, 242–249. doi: 10.1038/nature11552
Trompette, A., Gollwitzer, E. S., Yadava, K., Sichelstiel, A. K., Sprenger, N., and Ngom-Bru, C. (2014). Gut microbiota metabolism of dietary fiber influences allergic airway disease and hematopoiesis. Nat. Med. 20, 159–166. doi: 10.1038/nm.3444
Tsai, Y. L., Lin, T. L., Chang, C. J., Wu, T. R., Lai, W. F., Lu, C. C., et al. (2019). Probiotics, prebiotics and amelioration of diseases. J. Biomed. Sci. 26:3. doi: 10.1186/s12929-018-0493-6
Tsuchiya, T., Shimizu, H., Horie, T., and Mori, M. (1999). Expression of leptin receptor in lung: leptin as a growth factor. Eur. J. Pharmacol. 365, 273–279. doi: 10.1016/S0014-2999(98)00884-X
Turnbaugh, P. J., Hamady, M., Yatsunenko, T., Cantarel, B. L., Duncan, A., and Ley, R. E. (2009). A core gut microbiome in obese and lean twins. Nature 457, 480–484. doi: 10.1038/nature07540
Turnbaugh, P. J., Ley, R. E., Mahowald, M. A., Magrini, V., Mardis, E. R., and Gordon, J. I. (2006). An obesity-associated gut microbiome with increased capacity for energy harvest. Nature 444, 1027–1031. doi: 10.1038/nature05414
Ubags, N. D., Stapleton, R. D., Vernooy, J. H., Burg, E., Bement, J., and Hayes, C. M. (2016). Hyperleptinemia is associated with impaired pulmonary host defense. JCI Insight 1:e82101. doi: 10.1172/jci.insight.82101
Uchiyama, T., Okajima, F., Mogi, C., Tobo, A., Tomono, S., and Sato, K. (2017). Alamandine reduces leptin expression through the c-Src/p38 MAP kinase pathway in adipose tissue. PLoS One 12:e0178769. doi: 10.1371/journal.pone.0178769
Unal, M., Eskandari, G., Muşlu, N., Pata, Y. S., and Akbaş, Y. (2006). Serum leptin levels in patients with allergic rhinitis. Otolaryngol. Head Neck Surg. 134, 331–333. doi: 10.1016/j.otohns.2005.11.021
van der Voort, P. H. J., Moser, J., Zandstra, D. F., Muller Kobold, A. C., Knoester, M., and Calkhoven, C. F. (2020). Leptin levels in SARS-CoV-2 infection related respiratory failure: a cross-sectional study and a pathophysiological framework on the role of fat tissue. Heliyon 6:e04696. doi: 10.1016/j.heliyon.2020.e04696
Vesa, C. M., Behl, T., Nemeth, S., Bratu, O. G., Diaconu, C. C., Moleriu, R. D., et al. (2020). Prediction of NAFLD occurrence in prediabetes patients. Exp. Ther. Med. 20:190. doi: 10.3892/etm.2020.9320
Vernooy, J. H., Drummen, N. E., van Suylen, R. J., Cloots, R. H., Möller, G. M., and Bracke, K. R. (2009). Wouters EF. enhanced pulmonary leptin expression in patients with severe COPD and asymptomatic smokers. Thorax 64, 26–32. doi: 10.1136/thx.2007.085423
Vernooy, J. H., Ubags, N. D., Brusselle, G. G., Tavernier, J., Suratt, B. T., Joos, G. F., et al. (2013). Leptin as regulator of pulmonary immune responses: involvement in respiratory diseases. Pulm Pharmacol. Ther. 26, 464–472. doi: 10.1016/j.pupt.2013.03.016
Wang, J., Xu, Y., Zhang, X., Wang, S., Peng, Z., and Guo, J. (2021). Leptin correlates with monocytes activation and severe condition in COVID-19 patients. J. Leukoc. Biol. 110, 9–20. doi: 10.1002/JLB.5HI1020-704R
Wang, M. Y., Zhou, Y. T., Newgard, C. B., and Unger, R. H. (1996). A novel leptin receptor isoform in rat. FEBS Lett. 392, 87–90. doi: 10.1016/0014-5793(96)00790-9
White, S. J., Taylor, M. J., Hurt, R. T., Jensen, M. D., and Poland, G. A. (2013). Leptin-based adjuvants: an innovative approach to improve vaccine response. Vaccine 31, 1666–1672. doi: 10.1016/j.vaccine.2013.01.032
Wolsk, E., Mygind, H., Grøndahl, T. S., Pedersen, B. K., and van Hall, G. (2012). Human skeletal muscle releases leptin in vivo. Cytokine 60, 667–673. doi: 10.1016/j.cyto.2012.08.021
Wu, H. J., and Wu, E. (2012). The role of gut microbiota in immune homeostasis and autoimmunity. Gut Microbes 3, 4–14. doi: 10.4161/gmic.19320
Wypych, T. P., Wickramasinghe, L. C., and Marsland, B. J. (2019). The influence of the microbiome on respiratory health. Nat. Immunol. 20, 1279–1290. doi: 10.1038/s41590-019-0451-9
Xiong, Y., Miyamoto, N., Shibata, K., Valasek, M. A., Motoike, T., and Kedzierski, R. M. (2004). Short-chain fatty acids stimulate leptin production in adipocytes through the G protein-coupled receptor GPR41. Proc. Natl. Acad. Sci. U.S.A. 101, 1045–1050. doi: 10.1073/pnas.2637002100
Yang, D., Xing, Y., Song, X., and Qian, Y. (2020). The impact of lung microbiota dysbiosis on inflammation. Immunology 159, 156–166. doi: 10.1111/imm.13139
Yang, Y., Shi, Y., Wiklund, P., Tan, X., Wu, N., Zhang, X., et al. (2017). The association between cardiorespiratory fitness and gut microbiota composition in premenopausal women. Nutrients 9:792. doi: 10.3390/nu9080792
Yao, H., Fan, C., Fan, X., Lu, Y., Wang, Y., Wang, R., et al. (2020). Effects of gut microbiota on leptin expression and body weight are lessened by high-fat diet in mice. Br. J. Nutr. 124, 396–406. doi: 10.1017/S0007114520001117
Zahner, G. J., Ramirez, J. L., Spaulding, K. A., Khetani, S. A., Gasper, W. J., and Grunfeld, C. (2019). Leptinemia is associated with peripheral artery disease. J. Surg. Res. 238, 48–56. doi: 10.1016/j.jss.2019.01.023
Zhang, Y., Proenca, R., Maffei, M., Barone, M., Leopold, L., and Friedman, J. M. (1994). Positional cloning of the mouse obese gene and its human homologue. Nature 372, 425–332. doi: 10.1038/372425a0
Zhou, Y., Chi, J., Lv, W., and Wang, Y. (2021). Obesity and diabetes as high-risk factors for severe coronavirus disease 2019 (Covid-19). Diabetes Metab. Res. Rev. 37:e3377. doi: 10.1002/dmrr.3377
Keywords: immunity, leptin, microbiota, obesity, SARS-CoV-2
Citation: Bruno A, Ferrante G, Di Vincenzo S, Pace E and La Grutta S (2021) Leptin in the Respiratory Tract: Is There a Role in SARS-CoV-2 Infection? Front. Physiol. 12:776963. doi: 10.3389/fphys.2021.776963
Received: 14 September 2021; Accepted: 26 November 2021;
Published: 22 December 2021.
Edited by:
Deanne Helena Hryciw, Griffith University, AustraliaReviewed by:
Ke Wang, Suzhou Wuzhong People’s Hospital, ChinaFernanda Lima, Federal University of Santa Catarina, Brazil
Copyright © 2021 Bruno, Ferrante, Di Vincenzo, Pace and La Grutta. This is an open-access article distributed under the terms of the Creative Commons Attribution License (CC BY). The use, distribution or reproduction in other forums is permitted, provided the original author(s) and the copyright owner(s) are credited and that the original publication in this journal is cited, in accordance with accepted academic practice. No use, distribution or reproduction is permitted which does not comply with these terms.
*Correspondence: Serena Di Vincenzo, c2VyZW5hLmRpdmluY2Vuem9AaXJpYi5jbnIuaXQ=