- 1Biologie des Organismes et Ecosystèmes Aquatiques (BOREA), MNHN, CNRS UMR 8067, SU, IRD 207, UCN, UA, Paris, France
- 2Sorbonne Université, CNRS, Biologie Intégrative des Organismes Marins (BIOM), Banyuls-sur-Mer, France
- 3Department of Genetics, Physiology and Microbiology, Complutense University of Madrid (UCM), Madrid, Spain
- 4Equipe de Neuro-Ethologie Sensorielle, ENES/CRNL, CNRS UMR 5292, UMR-S 1028, Faculté des Sciences et Techniques, Université Jean-Monnet (UJM), Saint-Étienne, France
- 5Station Ifremer de Palavas, Palavas-les-Flots, Nantes, France
In fish, most hormonal productions of the pituitary gland display daily and/or seasonal rhythmic patterns under control by upstream regulators, including internal biological clocks. The pineal hormone melatonin, one main output of the clocks, acts at different levels of the neuroendocrine axis. Melatonin rhythmic production is synchronized mainly by photoperiod and temperature. Here we aimed at better understanding the role melatonin plays in regulating the pituitary hormonal productions in a species of scientific and economical interest, the euryhaline European sea bass Dicentrarchus labrax. We investigated the seasonal variations in mRNA abundance of pituitary hormones in two groups of fish raised one in sea water (SW fish), and one in brackish water (BW fish). The mRNA abundance of three melatonin receptors was also studied in the SW fish. Finally, we investigated the in vitro effects of melatonin or analogs on the mRNA abundance of pituitary hormones at two times of the year and after adaptation to different salinities. We found that (1) the reproductive hormones displayed similar mRNA seasonal profiles regardless of the fish origin, while (2) the other hormones exhibited different patterns in the SW vs. the BW fish. (3) The melatonin receptors mRNA abundance displayed seasonal variations in the SW fish. (4) Melatonin affected mRNA abundance of most of the pituitary hormones in vitro; (5) the responses to melatonin depended on its concentration, the month investigated and the salinity at which the fish were previously adapted. Our results suggest that the productions of the pituitary are a response to multiple factors from internal and external origin including melatonin. The variety of the responses described might reflect a high plasticity of the pituitary in a fish that faces multiple external conditions along its life characterized by marked daily and seasonal changes in photoperiod, temperature and salinity.
Introduction
In vertebrates, the neuroendocrine regulations are controlled by the brain-pituitary (BP) axis. The hypothalamus receives information from external and internal origin, which is converted into messages that regulate the hormonal productions of the pituitary gland. The pituitary hormones then act on downstream peripheral targets, thus controlling functions as important as reproduction, growth, osmoregulation (aquatic vertebrates), feeding, lactation (mammals), pigmentation, immunity or stress. As for most functions of the organism, the neuroendocrine regulations display daily and/or annual rhythmic patterns (Ekström and Meissl, 1997; Cowan et al., 2017; Falcón and Zohar, 2018). The alternation of light (L) and darkness (D) over the 24 h cycle (photoperiod) plays a major role in synchronizing these rhythms, while other factors are also involved (e.g., temperature, water salinity, food availability, rainfall) (Falcón et al., 2010). Synchronization to these factors generally involves internal daily (circadian) and seasonal (circannual) clocks (Migaud et al., 2010).
In fish, virtually all cells possess the molecular machinery that makes a functional biological clock (Whitmore et al., 2000a,b), which constitute a network of more or less potent oscillators. In this network, the photosensitive pineal organ plays a pivotal role (Underwood, 1989; Falcón et al., 2007; Falcón and Zohar, 2018). It produces melatonin – a time-keeping molecule – in higher amounts at night than during day. The amplitude of the nocturnal increase depends on various inputs (e.g., external temperature, internal neurotransmitters and neuromodulators). Previous studies indicated that melatonin may directly regulate the pituitary hormonal secretions: (1) Messenger RNA corresponding to melatonin receptors and/or melatonin binding sites have been identified in the pituitary of several fish species (Gaildrat and Falcón, 1999, 2000; Gaildrat et al., 2002; Park et al., 2006; Ikegami et al., 2009; Confente et al., 2010; Shin et al., 2011; Hong et al., 2014; Ciani et al., 2019; Sakai et al., 2019); (2) in isolated cultured pituitary glands melatonin modulates (i) the production of the 2nd messenger cyclic AMP (Gaildrat and Falcón, 1999, 2000; Falcón et al., 2003), (ii) the release of luteinizing hormone (LH) (Khan and Thomas, 1996), growth hormone (GH) and prolactin (PRL; Falcón et al., 2003), and (iii) the abundance of the clock genes mRNA Cry1 and Cry2 (Herrero and Lepesant, 2014). Melatonin acts also upstream the pituitary, as its receptors have been identified in the preoptic area (POA) and hypothalamus (Ekström and Vaněček, 1992; Davies et al., 1994; Oliveira et al., 2008; Ikegami et al., 2009; Herrera-Pérez et al., 2010), and modulates the secretion of gonadotropin releasing hormone (GnRH; Khan and Thomas, 1996; Sebert et al., 2008; Servili et al., 2013).
The role of melatonin as a neuroendocrine regulator in fish is no more questioned. However, a clear-cut picture is still missing perhaps because a sum of factors needs to be considered including the species investigated (and within the same species, the genetic diversity, sex, age, geographical location, previous history…), as well as the experimental conditions (in vivo vs. in vitro, photoperiod, temperature, salinity, time of day and time of year). Here we focused attention on the European sea bass – Dicentrarchus labrax – a euryhaline species of great economical interest in aquaculture. D. labrax is the matter of intensive molecular, physiological and behavioral investigations, which provide useful basic knowledge and molecular tools. Most of the studies on the sea bass have focussed on the BP-gonadal and BP-interrenal axis, including investigations on the daily and annual variations in melatonin and reproductive hormones plasma levels as well as in pituitary mRNA abundance of the latter (Rodriguez et al., 2001; Bayarri et al., 2004, 2009, Varsamos et al., 2006; Cowan et al., 2017; Sánchez-Vázquez et al., 2019). Here we investigated the annual variations in mRNA abundance corresponding to the three melatonin receptors cloned in the Mediterranean sea bass, and a range of pituitary hormones, including the reproductive hormones LH (or gonadotropin II, β subunit) and FSH (follicle-stimulating hormone or gonadotropin I, β subunit), as well as GH, PRL, POMC (proopiomelanocortin), SL (somatolactin) and TSH (thyroid stimulating hormone, β subunit). We also investigated in vitro the impact of melatonin and of melatonin analogs on the mRNA abundance of these pituitary hormones at two times of the year and in fish previously adapted to two different salinities.
Animals, Materials and Methods
Animals and Rearing Protocols
Dicentrarchus labrax used in this study originated from commercial aquaculture plants (Supplementary Figure 1A). Most of the experiments were performed with fish from “Méditerranée Pisciculture” [Salses-Le-Chateau, France; 42°50′ North (N), E2°55′ east (E)]. At the aquaculture plant the fish were reared outdoors, under natural conditions of photoperiod. The tanks were supplied with a mixture of seawater (from the Mediterranean Sea) and freshwater (from a local resurgence), so that the resulting salinity and temperature were maintained constant all year long (∼7‰ salinity and 16 ± 2°C, respectively). Random samplings indicated that most of the fish were males, in agreement with previous studies showing the sex ratio of farmed sea bass is highly biased, with a high proportion of males (ranging between 75 and 100%: Piferrer et al., 2005; Vandeputte et al., 2012, 2014, 2020), particularly at the water temperature at the “Méditerranée Pisciculture” facilities.
Upon arrival at the lab facilities of Banyuls-sur-Mer (France; 42°28′ N, 3°12′ E) the fish were placed for 3 weeks in 1 m3 indoors tanks (35 fish per tank) supplied with Mediterranean Sea water (∼37‰ salinity along the French Mediterranean coast) provided by an open circuit, at a flow rate of ∼400 l/h (Supplementary Figure 1B). In the experiments investigating the effects of salinity, the seawater was replaced by freshwater (4‰ salinity), all other conditions remaining similar [natural temperature and simulated natural photoperiod (light provided by fluorescent bulbs)] (Supplementary Figure 1B). To minimize stress induced by sampling, the fish were evenly distributed in different tanks, isolated one from each other by a double layer of thick black plastic curtains, so that the sampling was carried out in an alternate manner. Fish were fed ad libitum. Dark sampling was performed under dim red light. Mortality was close to 0 in the lab facilities. From now on these fish are named BW fish as they had been reared in brackish water before their arrival at the lab. As specified in Supplementary Figure 1, these fish were used for the in vivo (annual variations and acclimation to water salinities), and in vitro (melatonin challenges), experiments.
A second group of fish was made available to us, which originated from “Cannes aquaculture” (Cannes, France; 43°33′ N, 7°00′ E) and had been maintained for more than a year at the IFREMER station of Palavas-les-Flots (France; 43°31′ N, 3°55′ E). At the IFREMER station, they were placed in 10,000 l indoors round tanks, under simulated natural photoperiod (light provided by fluorescent bulbs) and natural temperature (see details below and Supplementary Figure 1A). Two major differences distinguished these fish from the BW fish described above: (1) they experienced seawater only (salinity ∼37‰) and (2) the same pool of fish was used from the beginning until the end of the experiment, so that they weighed 172 ± 37 g (mean ± S.E.) at the beginning, and 388 ± 77 g at the end, of the experiment (i.e., 12 months later). When brought to the lab the fish were kept in seawater, and were thus named SW fish (Supplementary Figure 1B); all other lab conditions were similar to those described above for the BW fish. These fish were used to investigate the annual variations in abundance of pituitary hormones and melatonin receptors mRNA (Supplementary Figure 1).
All experiments were performed according to the European Union regulations concerning the protection of experimental animals, and in agreement with the protocols approved by the Animal Care and Use Committee of the Observatoire Océanologique of Banyuls sur Mer (CNRS-UPMC; authorization # A-66-01-601).
Daily and Annual Variations of Pituitary Hormones and Melatonin Receptors
The fish were sacrificed by decapitation every 4 h of a 24 h LD cycle (starting at 08:00) (Supplementary Figure 1C). The sampling was performed in April (13°C, water temperature), June (22°C), October (20°C), January (13°C) and March (13°C). After removal, the pituitary glands were dipped in RNAlater ® (Applied Biosystems, Courtaboeuf, France). At each sampling time, 5 pools of 3 pituitary glands each were obtained for the BW fish. For technical reasons (size and number of fish available) only two 2 pools of 5 pituitary glands each were collected for the SW fish. The pools were frozen and kept at −80°C until mRNA extraction (as indicated below).
Organ Culture and Pharmacological Treatments
After dissection, pituitary glands from BW fish (Supplementary Figure 1C) were cultured individually as detailed elsewhere (Falcón et al., 2003). Briefly, the pituitary glands were rinsed and cultured for 2 days in 750 μL of HEPES-buffered RPMI-1640 culture medium (one pituitary per well of a 24-well culture plate) at the temperature at which the fish had been acclimated. At noon of day 3, the pituitaries were challenged for 24 h with melatonin concentrations ranging from 10–12 to 10–8 M, which correspond to the plasma physiological concentrations measured during daytime and night-time in D. labrax (Garcia-Allegue et al., 2001; López-Olmeda et al., 2009). The effects of S20304 (10–8 M) and luzindole (10–7 M), respectively, a non-selective agonist and a non-selective antagonist at the mammalian melatonin receptors, were also investigated. At the end of the treatment, pools of 3 glands each were dipped in RNAlater ® and stored at −80°C. The in vitro experiments investigating the effects of melatonin were carried out in February and August, i.e., during the reproductive and resting seasons, respectively. The experiments conducted in February were performed with pituitaries of fish adapted to salinities of 37 or 4‰ salinities, and those in August with pituitaries of the 37‰, salinity adapted fish. Data are expressed as the mean ± S.E.M. More details are provided in the figure legends and in Supplementary Figure 1.
Total RNA Extraction, cDNA Synthesis, PCR and Real Time Quantitative PCR
Total RNA from the pituitary glands was extracted using the TRIZOL reagent (Life technologies SAS; Saint Aubin, France) according to the manufacturer’s instructions. The concentration and quality of the RNA was checked by spectrophotometry (Bio-photometer, Eppendorf, Le Pecq, France) and after migration in a MOPS gel. One μg of RNA was reversed transcribed using the Superscript Reverse transcriptase (Clontech, Saint-Germain-en-Laye, France). The cDNA obtained was stored at −20°C.
Real time quantitative PCR (qPCR) was performed using a Light Cycler 1.5 (Roche Diagnostics, Meylan, France) and the Light Cycler Fast Start DNA Master SYBR Green I kit (Roche Molecular Biochemicals, Meylan, France). The conditions of the reaction were 10 min at 95°C followed by 10 s at 95°C, 5 s at 60°C and 15 s at 72°C, for 30 cycles. The melting curves were analysed for each sample, to check that only a single sequence was amplified. They were obtained after increasing temperature progressively to 95°C, decreasing it to 65°C for 20 s, and finally increasing it to 95°C. Negative controls included cDNA replacement by water and the use of non-transcribed total RNA. In order to ensure the specificity of the amplification, some controls also included running the obtained PCR products in an agarose gel, followed by extraction, sub-cloning and sequencing (Sauzet et al., 2008). The 2–ΔΔCt method was used to determine the relative mRNA abundance (Livak and Schmittgen, 2001), with the L17 ribosomal protein mRNA as the reference gene (Herrera-Pérez et al., 2011). The primers (Table 1) were designed using the Light Cycler Probe Design software (Roche, Meylan, France). Their efficiency was tested using serial dilutions of cDNA from pituitary extracts; the calibration curves exhibited slopes and efficiencies close to −3.32 and 100%, respectively.
Statistical Analysis
Data were analysed using the one-way or two-way analysis of variance (ANOVA) followed by Holm-Sidak’s post hoc test for multiple comparisons. Individual means were compared using the Student’s t test (Two-tailed). Prism 6.01 (GraphPad™ software, La Jolla, CA, United States) was used to perform the statistics (level of significance set at P < 0.05) and plot the graphs.
Chemicals
Unless otherwise specified, the products were from Sigma Aldrich (Saint Quentin Fallavier, France). S20304 was a kind gift of the ‘Servier Labs’ (Neuilly-sur-Seine, France).
Results
Annual Variations of Pituitary Hormones mRNA in vivo
Sampling started in April and ended in March of the following year. Relative abundance of mRNA from all the pituitary hormones investigated displayed significant annual variations.
FSH and LH: The profiles obtained were similar for both hormones and independent of the fish origin (BW or SW fish) (Figure 1 and Table 2). Levels were low from April to October, and then increased to reach a peak value in January and resume low levels in March.
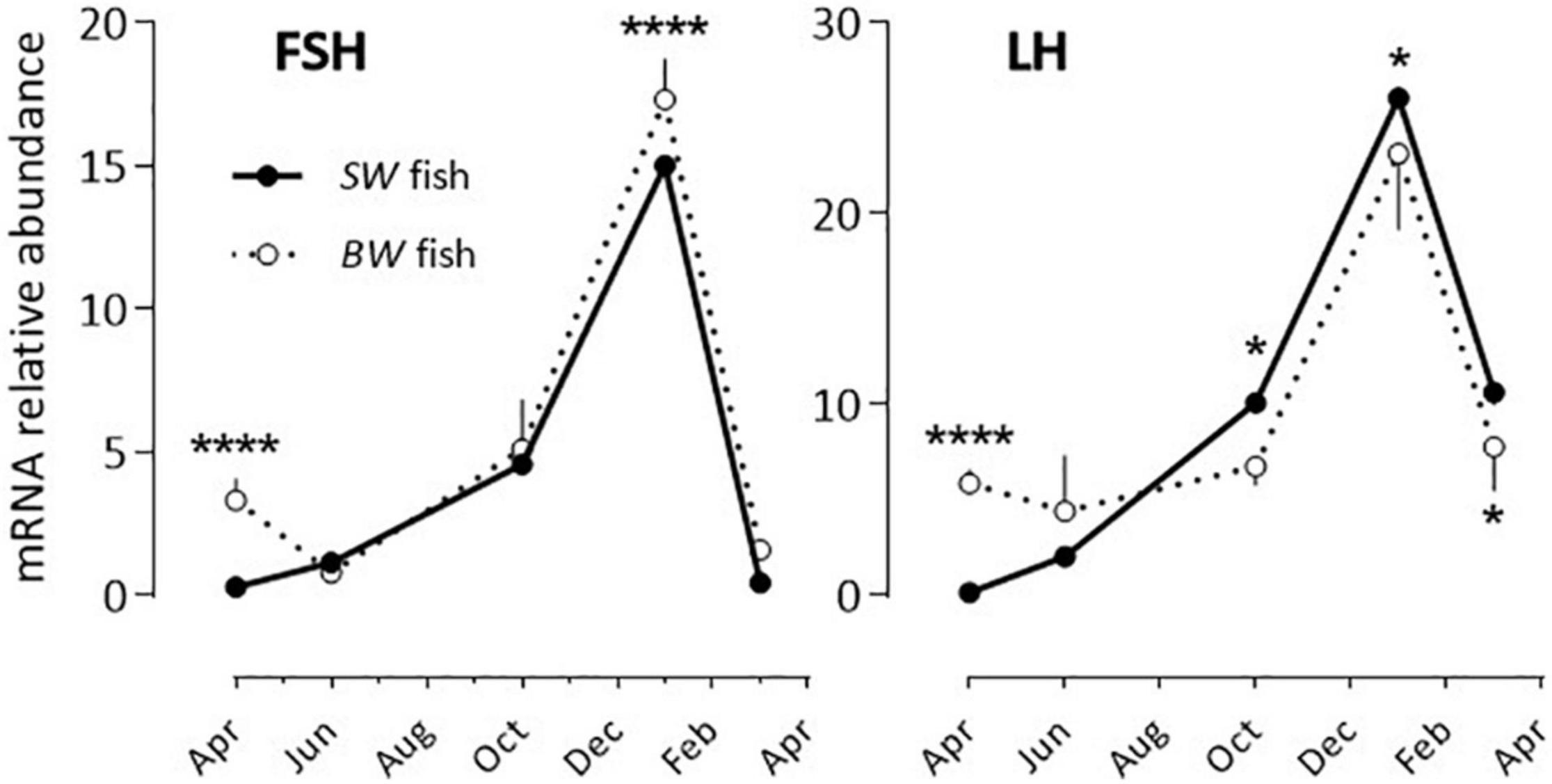
Figure 1. Relative annual variations in FSH and LH mRNA abundance in sea bass pituitaries in vivo. The pituitaries were collected from April until March of the following year. As detailed in the Materials and Methods section each value represents the mean ± S.E.M. (n = 6) of the values obtained from the 6 sampling hours (08:00, 12:00, 16:00, 20:00, 00:00, 04:00) of one light/dark cycle. At each sampling hour the sampling consisted of 5 pools of 3 pituitaries each (BW fish) or 2 pools of 5 pituitaries each (SW fish). All values are normalized to the value obtained from the SW fish in April (plotted as 1 in the Y axis). Two-way ANOVA indicated that the annual variations were statistically significant (Table 2) and the Holm-Sidak post hoc test was used for comparison of means between the SW and BW fish (Significant *: P < 0.05; ****: P < 0.0001).
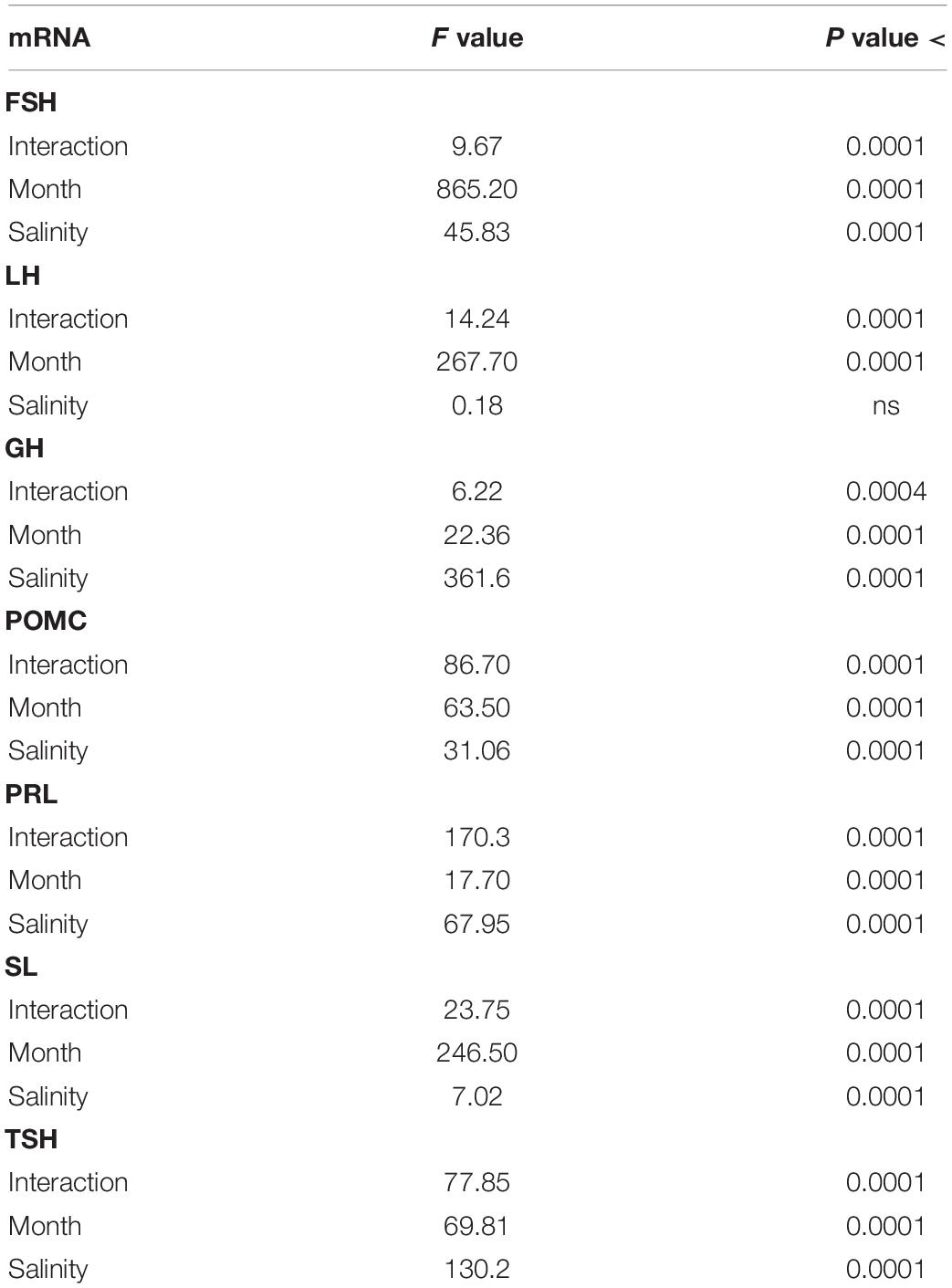
Table 2. Two-way ANOVA data from Figure 1 (Annual variations in the abundance of mRNA of sea bass pituitary hormones).
POMC and GH: POMC mRNA abundance displayed a bell-shape response (peak in October) in the SW fish, while no significant annual rhythm was seen in the BW fish (Figure 2 and Table 2). The opposite situation was observed with GH, which exhibited a U-shape profile in the BW fish (high values in October and January), and no significant variation in the SW fish (Figure 2 and Table 2).
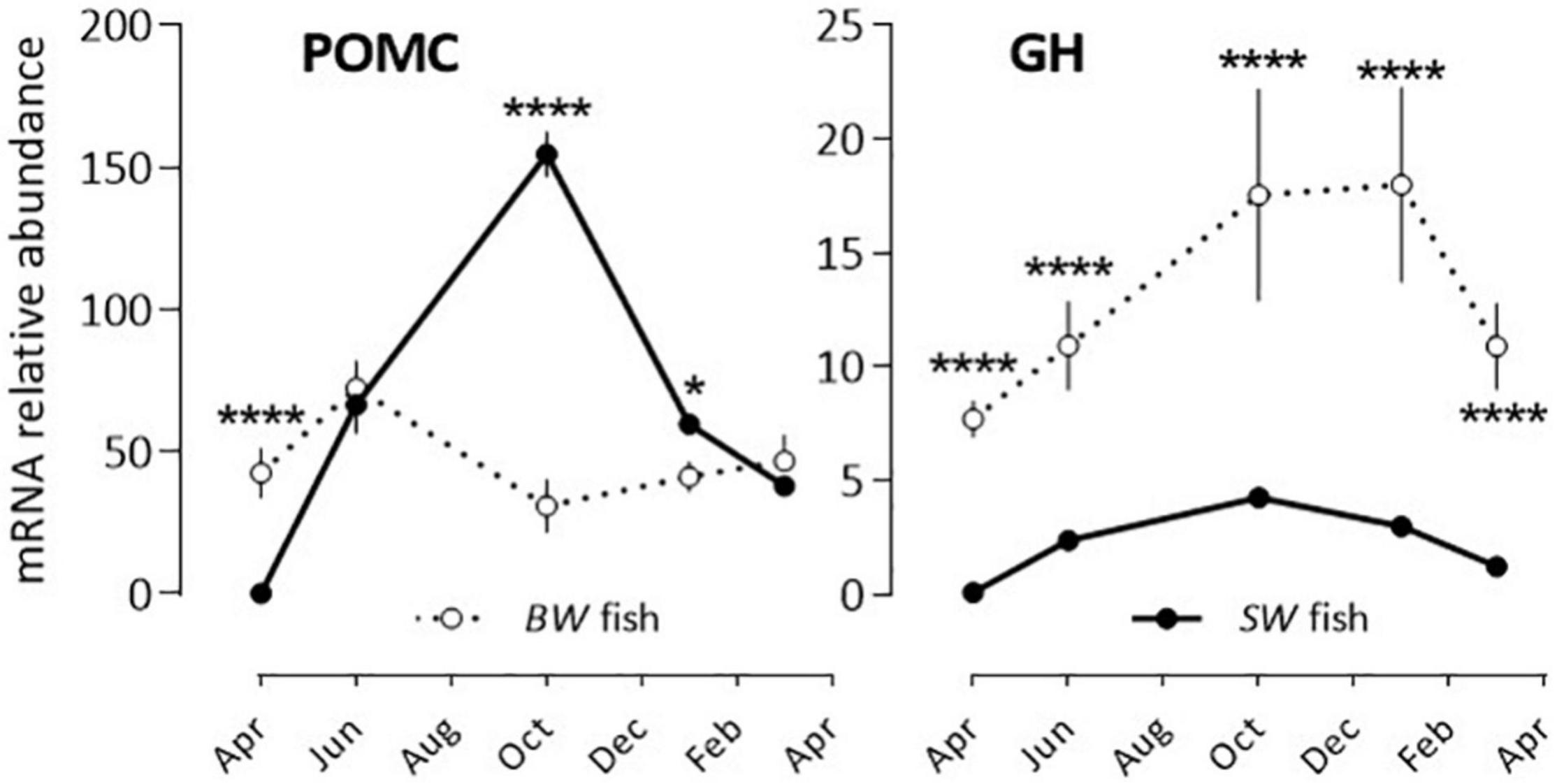
Figure 2. Relative annual variations in POMC and GH mRNA abundance in sea bass pituitaries in vivo. Same conditions as indicate in the legend of Figure 1. Mean ± S.E.M. (n = 6). Two-way ANOVA (Table 2) and Holm-Sidak’s post hoc test was used for comparison of means between the SW and BW fish (Significant *: P < 0.05; ****: P < 0.0001).
PRL, SL and TSH: Two situations were observed after comparing the BW to the SW fish as the annual variations displayed 180° out of phase profiles (Figure 3 and Table 2). In the BW fish, PRL mRNA relative abundance decreased significantly from April to October, and increased from October to March, while SL and TSH levels were high in March and low at the other months investigated.
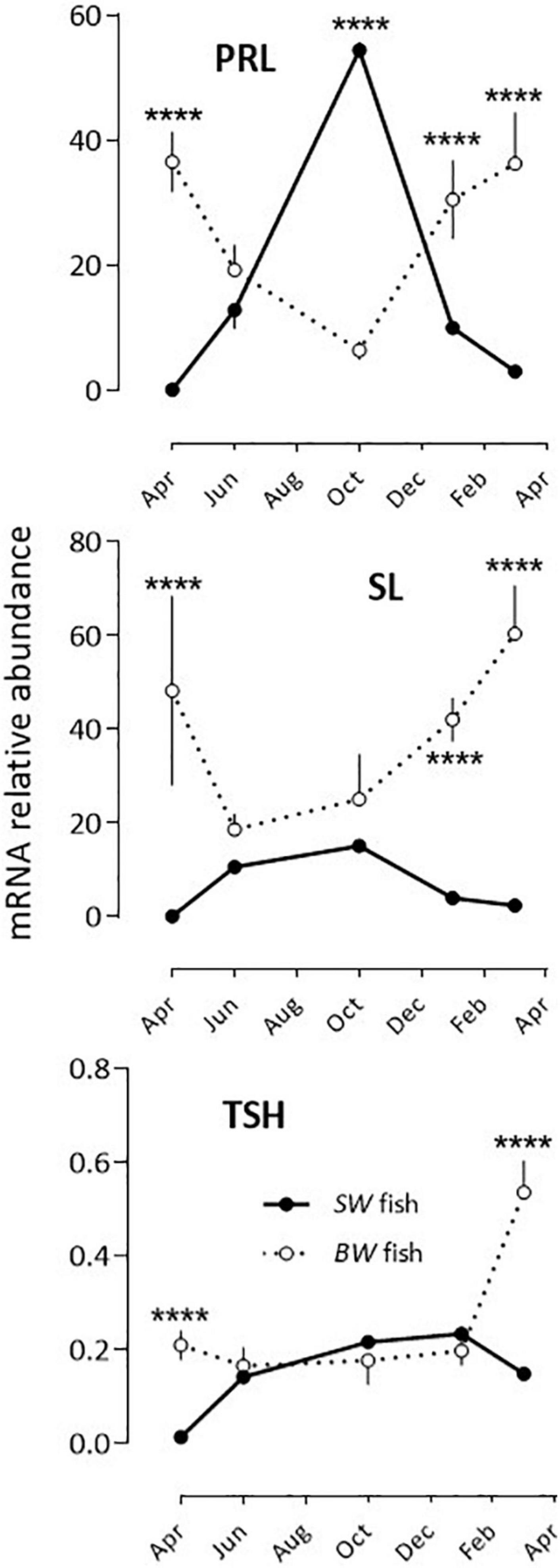
Figure 3. Relative annual variations in PRL, SL and TSH mRNA abundance in sea bass pituitaries in vivo. Same conditions as indicate in the legend of Figure 1. Mean ± S.E.M. (n = 6). Two-way ANOVA (Table 2) and Holm-Sidak’s post hoc test was used for comparison of means between the SW and BW fish (Significant *: P < 0.05; ****: P < 0.0001).
Daily and Annual Variations of Melatonin Receptors mRNA in vivo
The three melatonin receptor subtypes cloned in the sea bass displayed daily variations in abundance in the SW fish, significant in March (MT1, MT2, Mel1c), January and October (MT1, Mel1c), but not in June (Figure 4A). They also displayed annual variations. The highest abundance was found in June and October for MT2, October for MT1 and March for Mel1c (Figure 4B).
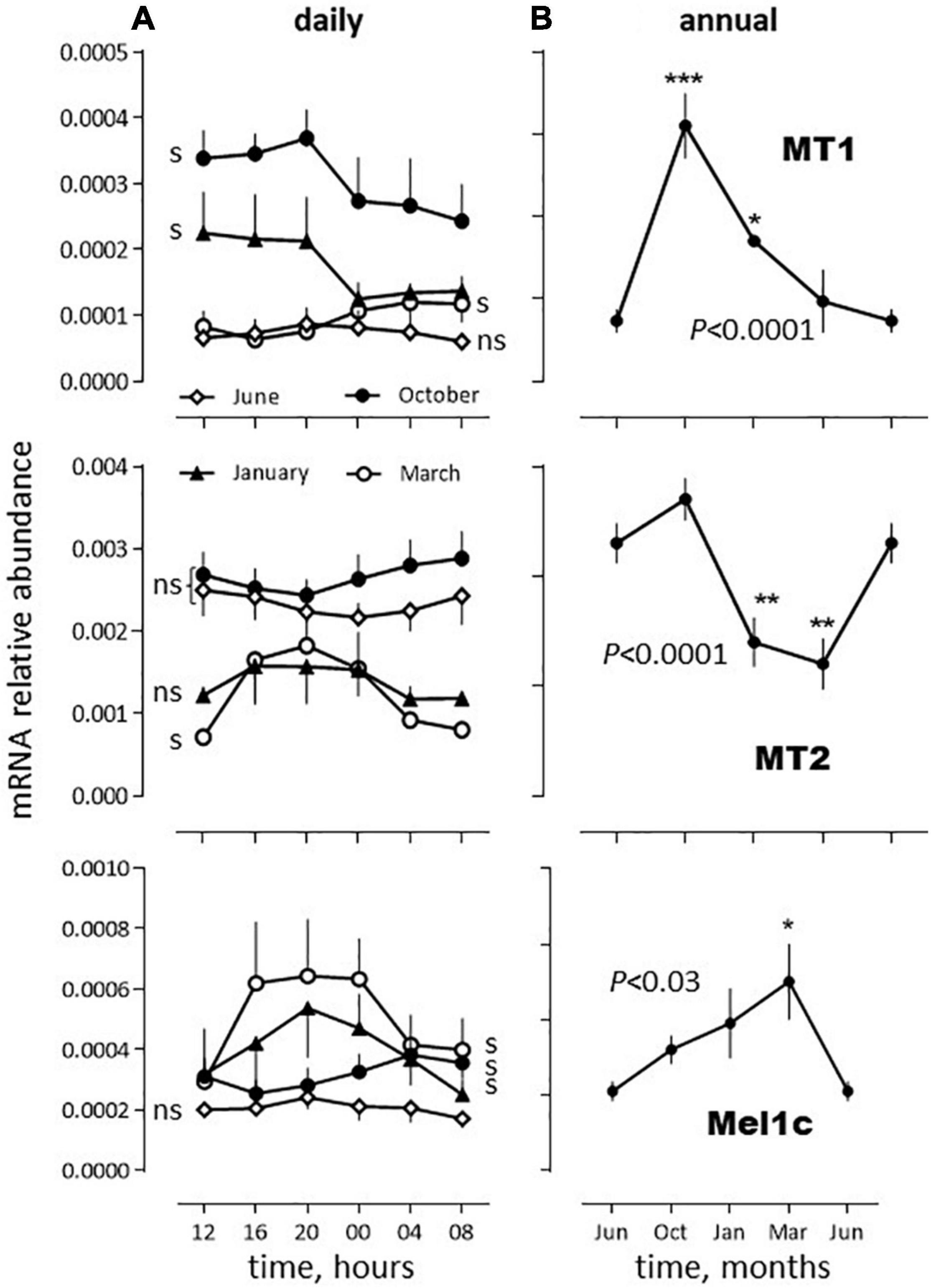
Figure 4. Relative daily (A) and annual (B) variations in mRNA abundance of sea bass melatonin receptors mRNA in vivo. The data were obtained from the same SW fish pituitaries extracts as used in Figures 1–3. (A) Daily variations: The moving average method was used because each sampling time was made of only 2 pools of 5 pituitaries each (as indicated in the Materials and Methods section). Thus, the plot at time tx represents the mean of the values obtained at tx–1, tx and tx+1. Mean ± S.E.M. (n = 6). Photoperiod was 14L(07:00–21:00)/10D (June), 12L(08:00–20:00)/12D, (October), 10L(08:00–18:00)/14D (January) and 12L(08:00–20:00)/12D (March). One-way ANOVA indicated P < 0.005 for MT1 in January, March and October, P < 0.0001 for MT2 in March and P < 0.01 for Mel1c in January, March and October; s: significant, ns: non-significant. (B) Annual variations: For each month the value obtained corresponds to the mean obtained at each sampling hour (in a) (mean ± S.E.M., n = 6). The June data are plotted twice. One way ANOVA level of significance is indicated in the graphs. Individual means are compared to the corresponding value in June using the Holm-Sidak’s post hoc test (Significant *P < 0.05; **P < 0.01; ***P < 0.005).
Impact of Salinity on Pituitary Hormones mRNA Abundance in vivo
After acclimation of the seabass to 4 or 37‰ salinities for 3 weeks in the lab, the mRNA abundance of pituitary hormones changed significantly for PRL (more than a 4-fold difference), GH, POMC and FSH (∼3-fold difference) (Figure 5). In the case of PRL and POMC, the levels were high under the 4‰ condition and low under the 37‰ condition, while the opposite held true for GH and FSH mRNA levels. SL levels were also 30% lower under the 4 vs. 37‰ condition, a difference not statistically significant. No difference was seen for LH and TSH (Figure 5).
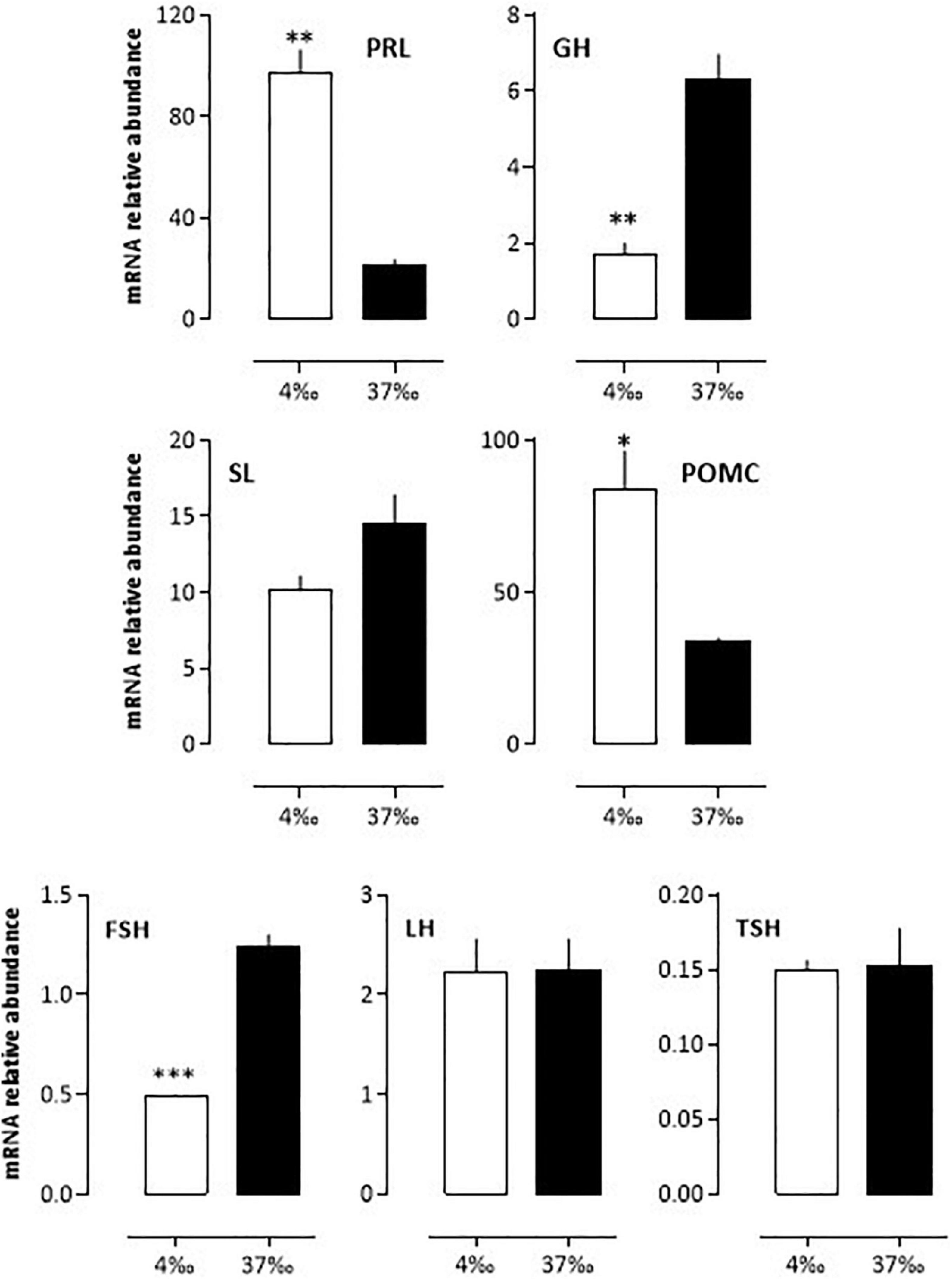
Figure 5. In vivo relative variations of mRNA abundance from bass pituitary hormones after a 3 weeks adaptation to 4 or 37‰ salinities. BW fish were maintained at the lab for 3 weeks in either sea- or freshwater as indicated in the Materials and Methods section. Mean ± S.E.M. (n = 5 samples, each sample containing a pool of 3 pituitary glands). Student’s t-test *P < 0.01, **P < 0.001 and ***P < 0.0001.
Effects of Melatonin on Pituitary Hormones mRNA Abundance in vitro
The effects of melatonin on the pituitary hormones mRNA levels were investigated in February and August, in BW fish acclimated for 3 weeks to a 37 or a 4‰ salinity (see experimental protocol design in Supplementary Figure 1).
-February, 37‰ salinity. Melatonin had no significant effect on PRL, SL and LH mRNA abundance (Figures 6–8). The hormone induced dramatic effects on GH and POMC, inhibitory for the former (3-fold inhibition at 10–12 M; Figure 6) and stimulatory for the latter (4-fold stimulation at 10–9 M; Figure 7). The FSH mRNA relative abundance was slightly inhibited by increasing concentrations of melatonin (Figure 8). The effect on TSH was complex as the stimulation observed at 10–12 and 10–10 M was reversed at 10–9 and 10–8 M (Figure 7). The effects were mimicked by the melatonin analogs S20304 and luzindole used at the concentration used (insets in Figures 6–8).
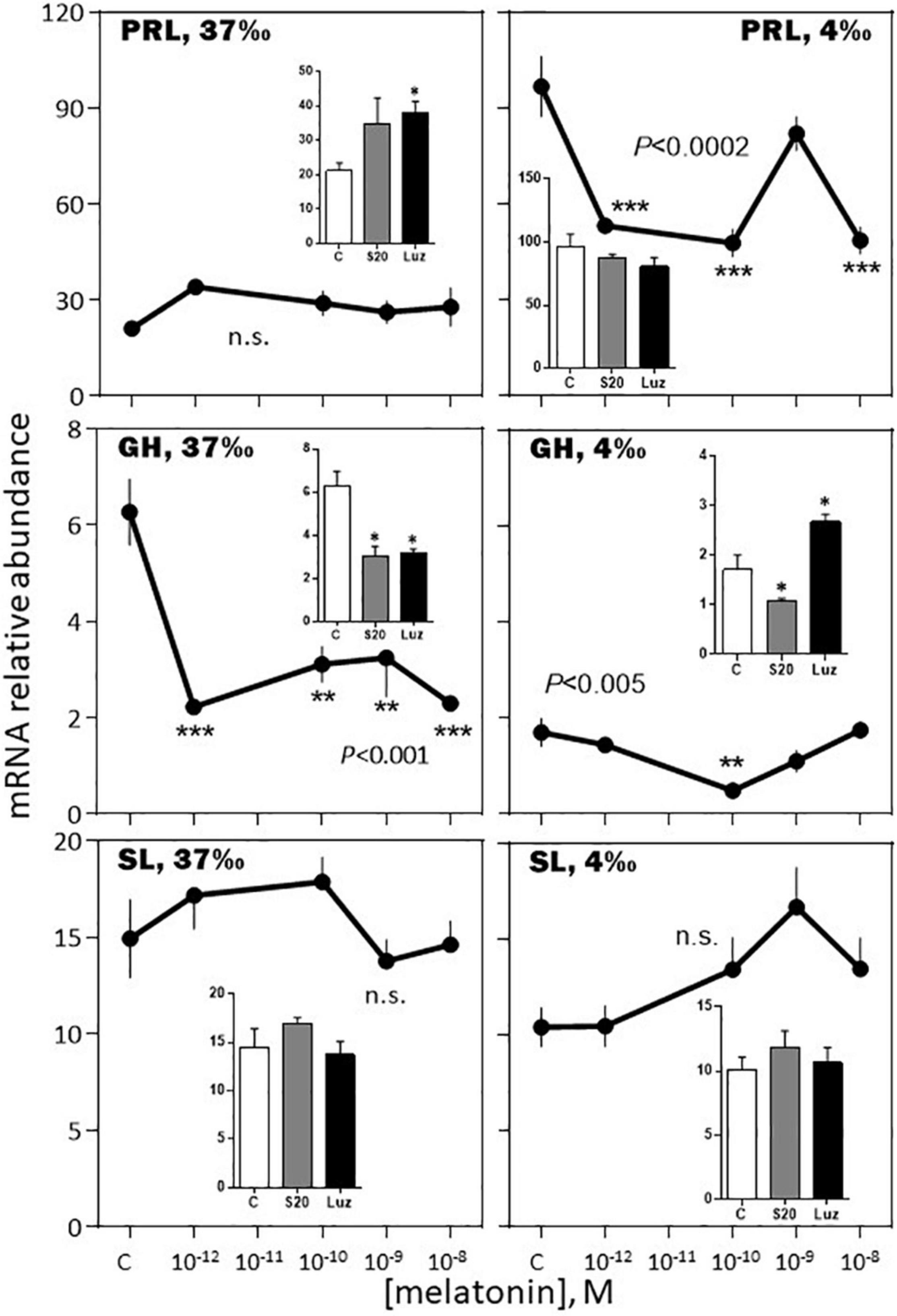
Figure 6. Effects of melatonin and melatonin analogs on PRL, GH and SL mRNA relative abundance in vitro. The experiment was performed in February. The BW fish were acclimated to either seawater (37‰; left column) or freshwater (4‰; right column) 3 weeks before sacrifice. The pituitaries were challenged for 12 h with the different concentrations of melatonin indicated in the abscissae (see Materials and Methods section for details). The controls (C in the abscissae) received an equivalent amount of solvent present at the highest melatonin concentration; it did not exceed 0.0001% in the final solution. Mean ± S.E.M. of n = 3 pools (containing 5 glands each). One-Way ANOVA (P gives the level of significance; n.s.: non-significant). Means were compared to the corresponding control using the Holm-Sidak’s post hoc test: *P < 0.05, **P < 0.005, ***P < 0.0005. The insets show the 12 h effects of two melatonin analogs S20304 (S20 at 10– 8 M) and luzindole (Luz at 10– 7 M). Mean ± S.E.M. of n = 3 pools (containing 5 glands each). Values are compared to the controls using the Student’s t-test at Significant *P < 0.01.
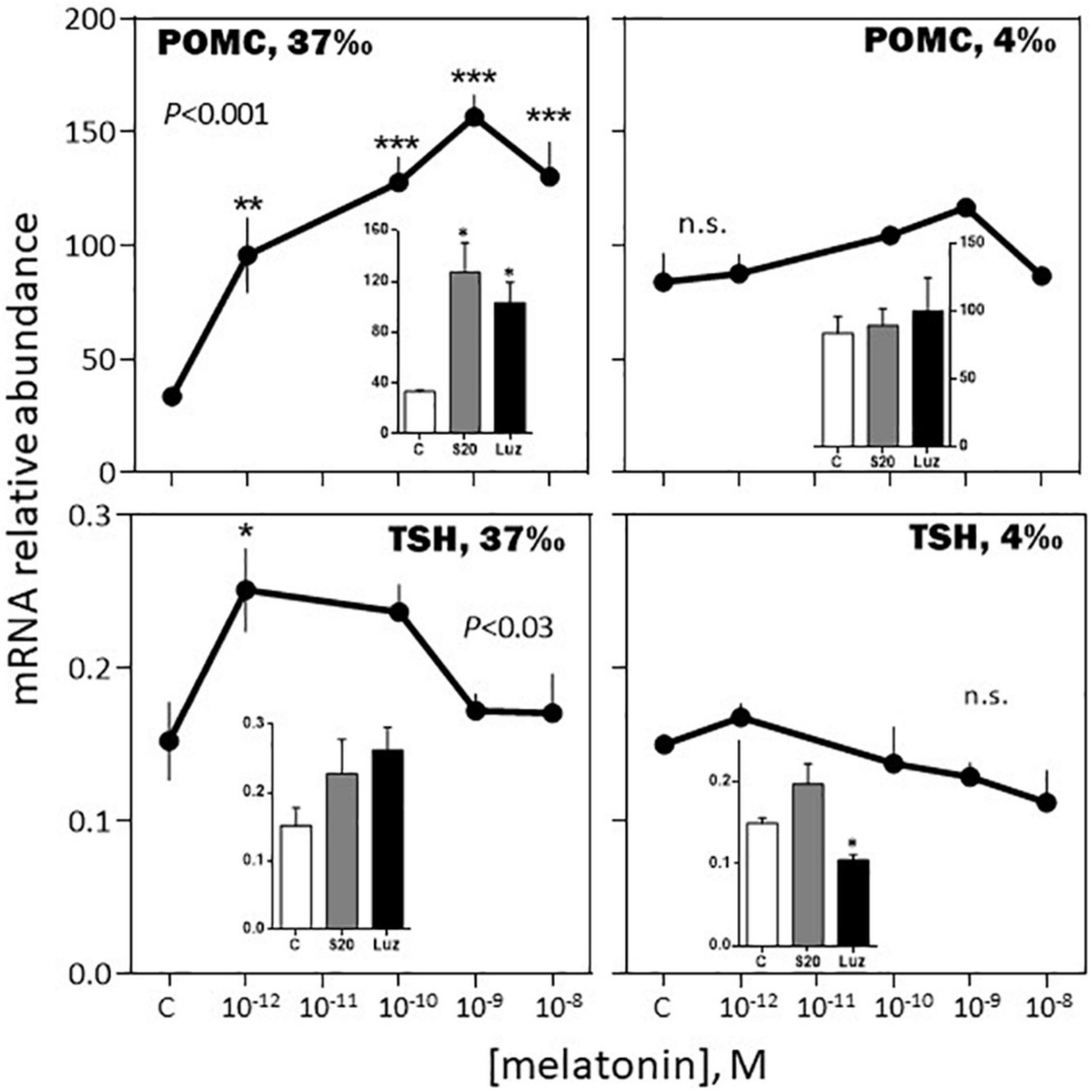
Figure 7. Effects of melatonin and melatonin analogs on POMC and TSH mRNA relative abundance in vitro. Mean ± S.E.M. of n = 3 pools (containing 5 glands each). One-Way ANOVA (P gives the level of significance; n.s.: non-significant). Means were compared to the corresponding control using the Holm-Sidak’s post hoc test: *P < 0.05, **P < 0.005, ***P < 0.0005. The insets show the 12 h effects of two melatonin analogs S20304 (S20 at 10– 8 M) and luzindole (Luz at 10– 7 M). Mean ± S.E.M. of n = 3 pools (containing 5 glands each). Values are compared to the controls using the Student’s t-test at P < 0.01. See Figure 6 for more details.
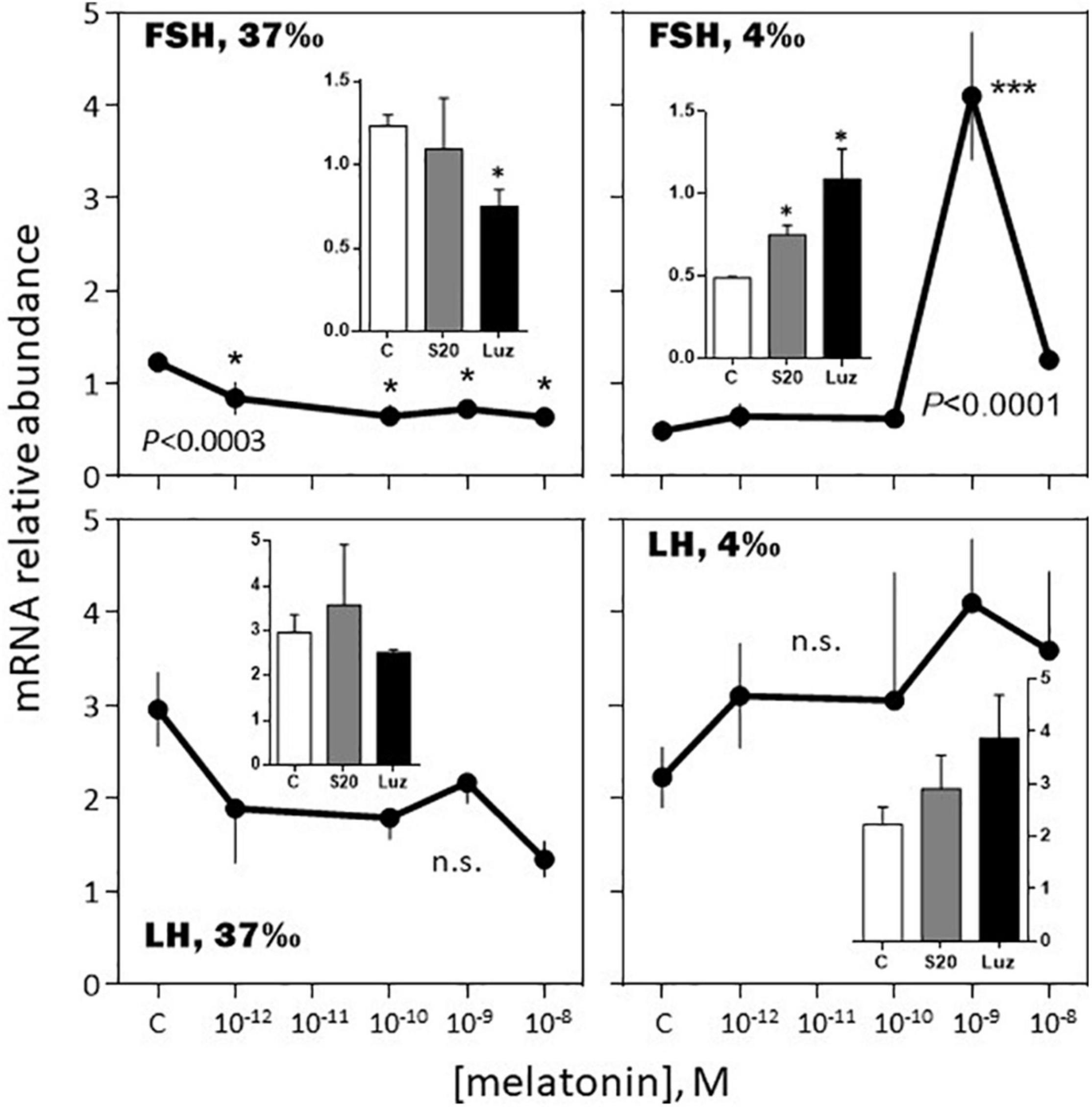
Figure 8. Effects of melatonin and melatonin analogs on FSH and LH mRNA relative abundance in vitro. Mean ± S.E.M. of n = 3 pools (containing 5 glands each). One-Way ANOVA (P gives the level of significance; n.s.: non-significant). Means were compared to the corresponding control using the Holm-Sidak’s post hoc test: *P < 0.05, ***P < 0.0005. The insets show the 12 h effects of two melatonin analogs S20304 (S20 at 10– 8 M) and luzindole (Luz at 10– 7 M). Mean ± S.E.M. of n = 3 pools (containing 5 glands each). Values are compared to the controls using the Student’s t-test at P < 0.01. See Figure 6 for more details.
-February, 4‰ salinity. In the fish adapted to the 4‰ salinity, melatonin had no significant effects on SL, POMC, TSH and LH mRNA abundances (Figures 6–8). A v-shaped dose-response curve was obtained with GH (highest inhibition at 10–10 M of melatonin; Figure 6), while FSH mRNA levels were increased at the single concentration of 10–9 M (Figure 8). In a general manner, the effects were mimicked by the melatonin analogs S20304 and luzindole used at one single concentration (insets in Figures 6–8).
-August, 37‰ salinity. While TSH mRNA abundance was not affected by melatonin, POMC, GH, SL mRNA relative levels were inhibited in a dose-dependent manner (Figures 9, 10). PRL and LH mRNA were also affected, however, the response was more complex, stimulatory at the low (10–12, 10–10 M), and inhibitory at the high (10–9 M), concentrations (Figures 9, 10). In a general manner, the effects of the melatonin agonist S20304 (10–8 M), were in agreement with those obtained with melatonin, except for POMC (inset Figure 9). For comparison the data obtained in February and August (at the salinity of 37‰) are plotted together in Supplementary Figures 2, 3.
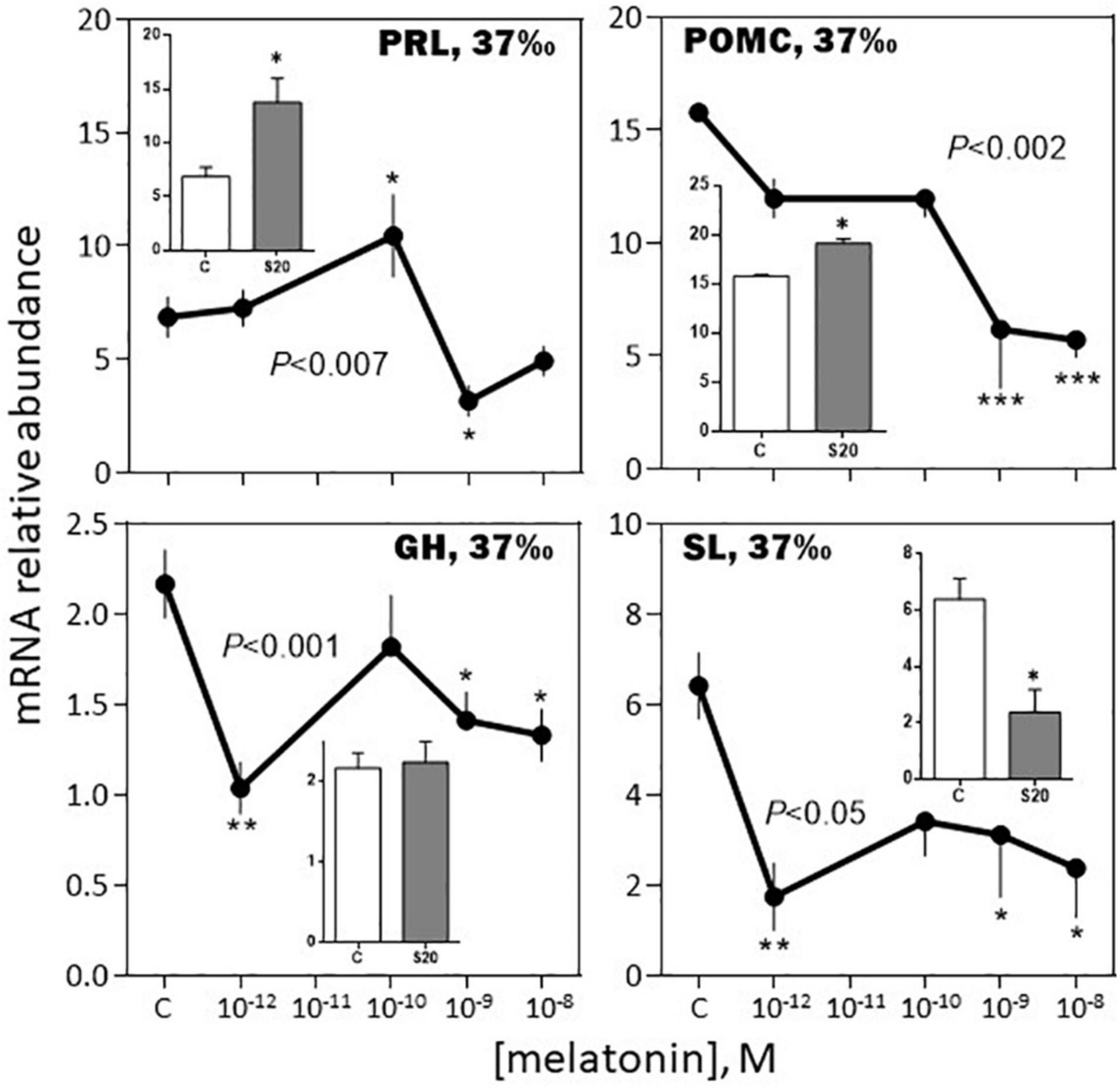
Figure 9. Effects of melatonin and melatonin analogs on PRL, POMC, GH and SL mRNA relative abundance in vitro. The experiment was performed in August using BW fish acclimated to a 37‰ salinity. Other conditions are as indicated in Figure 6 and in the Materials and Methods section. Mean ± S.E.M. of n = 5 pools (containing 3 glands each). One-Way ANOVA (P level of significance is indicated within the graph; n.s.: non-significant); values are compared to their respective control (C) using the Holm-Sidak’s pos-hoc test (*P < 0.05, **P < 0.005, ***P < 0.0005). The insets show the 12 h effects of the melatonin analogs S20304 (S20 at 10– 8 M). Mean ± S.E.M. of n = 5 pools (containing 3 glands each); values are compared to their respective control (C) using the Student’s t-test at *P < 0.01.
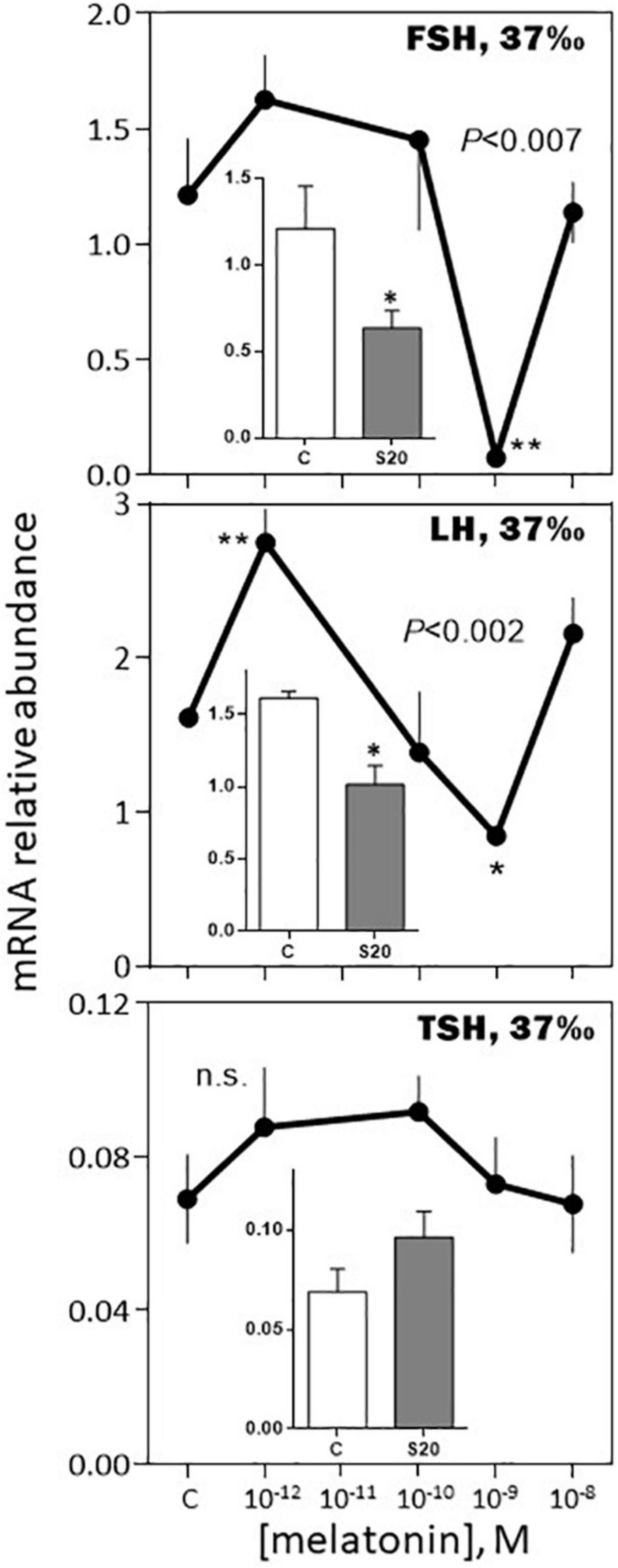
Figure 10. Effects of melatonin and melatonin analogs on FSH, LH and TSH mRNA relative abundance in vitro. Mean ± S.E.M. of n = 3 pools (containing 5 glands each). One-Way ANOVA (P gives the level of significance; n.s.: non-significant). Means were compared to the corresponding control using the Holm-Sidak’s post hoc test: *P < 0.05, **P < 0.005. The insets show the 12 h effects of two melatonin analogs S20304 (S20 at 10– 8 M) and luzindole (Luz at 10– 7 M). Mean ± S.E.M. of n = 3 pools (containing 5 glands each). Values are compared to the controls using the Student’s t-test at P < 0.01. See Figure 9 for more details.
Discussion
Annual Variations of Pituitary Hormones mRNA Abundance
Most of the studies reporting on the daily and annual variations of pituitary hormones focus on FSH and LH (Mateos et al., 2003; Cowan et al., 2017). Here we provide the first information on a panel of pituitary hormones in the European sea bass. Previous studies have shown a clear annual rhythm in the abundance of FSH and LH mRNA in the sea bass pituitary, with a peak occurrence in January/February, and lowest abundance from May to October (Mateos et al., 2003). This rhythm was paralleled by variations in the gonado-somatic index and plasma LH protein levels, in keeping with the annual rhythm of reproduction in this species (Kara, 1997; Alvarado et al., 2013). Our data agree fully with these previous findings. In addition, we found that similar profiles were obtained independent of the fish origin and previous rearing conditions, which brings strong support to the conclusion that the seasonal control of reproduction is a tightly locked photoperiod-dependent process in the European sea bass, as suggested earlier (Bayarri et al., 2004; Falcón et al., 2010).
A contrasting situation occurred with the other pituitary hormones, in which the annual rhythms of mRNA abundance differed in phase (TSH, SL, PRL) or amplitude (GH, POMC), in the SW vs. BW fish. The reason for these differences could lie in the fact that the fish originated from two different locations and had different historical backgrounds. The BW fish originated from distinct fertilization processes, and had all similar standard commercial sale size at each sampling month. In contrast, the SW fish were all from a single pool of fish that were followed during whole duration of the experiment. In addition, the SW fish were raised in a 37‰ salinity all life on, while the BW fish experienced a transition from a ∼7‰ to a ∼37‰ salinity 3 weeks before sacrifice. The differences may have impacted differently PRL, SL, GH, TSH, and POMC. These hormones have pleiotropic effects [control of skin pigmentation (POMC, SL), immunity and stress (POMC, GH, PRL), food intake (POMC, GH), growth (GH, TSH), osmotic regulation (GH, PRL), sexual maturation (GH, PRL, TSH)], and this involves a complex network of interactions within the pituitary, as well as the whole neuroendocrine loops (Agustsson and Bjornsson, 2000; Harris and Bird, 2000; Boeuf and Falcón, 2001; Bhandari et al., 2003; Deane and Woo, 2009; Laiz-Carrión et al., 2009; Blanco, 2020); in this regard, their regulation implies more flexibility than is the case for the reproductive hormones.
Part of the annual life cycle of the European sea bass involves salinity changes, which needs rapid adaptive neuroendocrine responses. We indeed observed that a 3 weeks adaptation to either low (4‰) or high (37‰) salinity impacted mRNA levels of GH, FSH, PRL, and POMC, while those of LH, SL, and TSH remained unaffected. In the case of PRL, POMC, SL, and GH, our data agree with those obtained in this (Varsamos et al., 2006) and other (Laiz-Carrión et al., 2009; Lu et al., 2016; Yamaguchi et al., 2016; Yuan et al., 2017; Liu et al., 2020; Vargas-Chacoff et al., 2021) fish species maintained under low or high salinities. They are also in line with the known roles of these hormones, essential in the processes of acclimation to different salinities (PRL, SL, GH, or rather the GH/IGF-I axis); both, PRL and GH/IGF-I interact with cortisol to control ionic balance (Takei and McCormick, 2013). The production of cortisol by the interrenal gland is controlled by ACTH (adrenocorticotropic hormone), a cleavage product of POMC. Here we found that POMC mRNA levels were higher under the freshwater than under the seawater condition, as observed in the ayu, an anadromous species (Lu et al., 2016), but in contrast to the situation in the tilapia, mainly a fresh water fish (Aruna et al., 2015). The case of SL is interesting as we found a slight non-significant increase in mRNA relative abundance, as is the case in the gilthead seabream (Laiz-Carrión et al., 2009); however, in the latter intermediate salinities (12‰) induced a dramatic decrease in SL mRNA compared to the low and high salinities (6 and 38‰, respectively), which highlights the complexity of the mechanisms studied.
Daily and Annual Variations of Melatonin Receptors mRNA Abundance in the Pituitary
Melatonin receptors have been identified in the pituitary of several fish species (Gaildrat and Falcón, 1999, 2000; Gaildrat et al., 2002; Sauzet et al., 2008; Ikegami et al., 2009; Confente et al., 2010; Hong et al., 2014; Ciani et al., 2019; Sakai et al., 2019). Three subtypes have been identified in the sea bass (MT1, MT2, Mel1c), MT2 displaying the higher relative abundance in the pituitary (Sauzet et al., 2008; Herrera-Pérez et al., 2010; and this study). They also exhibited daily and seasonal variations as reported earlier for both, mRNA relative abundance and melatonin binding sites, in the pituitary and brain of other fish species (Falcón et al., 1996; Gaildrat et al., 1998; Amano et al., 2003; Park et al., 2006; Bayarri et al., 2010; Confente et al., 2010; Ciani et al., 2019). These variations are controlled by circadian clocks in the pike (Gaildrat et al., 1998) and circannual clocks in the European sea bass (Bayarri et al., 2010) as they were observed under constant conditions. Thus, a good understanding of the time-dependent effects of melatonin (as discussed below) requires that not only its cyclic production, but also the rhythmic availability and affinity of its receptors to be considered.
Melatonin Effects on Pituitary Hormones
The current study supports previous investigations indicating melatonin treatment impacts LH, GH, and PRL productions in fish (Khan and Thomas, 1996; Falcón et al., 2003), and extends to POMC, SL, FSH, and TSH the range of hormones affected. In most cases, the conclusions were supported by the observed effects of the melatonin receptor agonist S20304 and antagonist luzindole. As characterized in mammals, luzindole is supposed to be an antagonist. However, paradoxical effects as the ones described in this study have been reported. In rats, luzindole may also act as a partial agonist at the MT2 receptor subtype (Legros et al., 2020) or exert direct proper effects (i.e., not mediated through melatonin receptors) on plasma membrane K+ currents (Zhou et al., 2003) or [Ca2+]i stores (Estaras et al., 2020). In the medaka luzindole acted as a partial antagonist or a full agonist depending on the receptor subtype (Sakai et al., 2019). It has been emphasized that “ligands that target melatonin receptor subtypes in one species does not have the same affinity and specificity in another species” (ViviD and Bentley, 2018), which might be particularly true in fish, in which the receptors display proper structural and functional characteristics different from those of mammals.
In brief, the responses to the melatonin challenges appeared complex, depending for each hormone on several factors, including dose, time of year and previous adaptation to salinity.
The in vitro Response to Melatonin Depended on the Month of the Year
Melatonin had a moderate inhibitory effect on FSH and no effect on LH mRNA abundance in February. In August, the effect on FSH was amplified at the nanomolar concentration of melatonin, while LH mRNA displayed a biphasic response, stimulatory at the picomolar, and inhibitory at the nanomolar, concentrations of melatonin. Considering the sea bass annual reproductive cycle (Kara, 1997; Alvarado et al., 2013), these data are consistent with the idea that melatonin contributes to exert an inhibitory role on reproduction during the resting phase.
A novel finding of this study is the reported seasonal dependent impact of melatonin on the other hormones investigated. Strong in February (up to a 5-fold increase at 10–9 M melatonin), the increase in POMC mRNA relative abundance was no more observed in August. Similarly, the bell-shaped biphasic response of TSH was reduced in amplitude in August, compared to February. A reverse situation was observed for PRL and SL mRNA levels, no effect being seen in February, compared to an inhibitory response in August. Finally, the melatonin induced reduction of GH mRNA levels was less pronounced in August compared to February. We conclude that in the sea bass melatonin impacts all the pituitary hormones and the effects depend on the dose and month of the year. More studies at other months of the year are needed to get a more complete picture.
The in vitro Responses to Melatonin Depended on the Previous Fish Adaptation to Salinity
Previous investigations indicated that salinity changes affect both, melatonin production and melatonin binding sites in fish (Gern et al., 1984; Kleszczynska et al., 2006; López-Patiño et al., 2011), including in the Mediterranean sea bass, in which plasma melatonin levels, retina and brain maximal 2-[125I]-iodomelatonin binding (Bmax) were higher under low vs. high salinity adaptation. We have indication that the effects depend on the previous duration of adaption of the fish to a given salinity, and that this also affects the affinity (kD) of the binding sites for melatonin (authors’ unpublished data). These observations together with those discussed above concerning the modulation by salinity of mRNA levels of PRL, GH, TSH or POMC before the melatonin challenge, might in part explain that melatonin treatment resulted in different effects in the 4 vs. 37‰ acclimated fish. Compared to the 37‰ acclimated fish, in those acclimated to the 4‰ salinity the amplitudes of the dose-dependent responses to melatonin were dramatically reduced (POMC and GH), no more observed (TSH), or reversed (FSH). Whatever the situation, we conclude that melatonin effects on the pituitary depend highly on the salinity at which the fish had previously been adapted.
Conclusion
This study on the European sea bass brings important new information on the control of neuroendocrine regulations in fish: (1) all the pituitary hormones investigated displayed annual variations, bringing together and in a single species, previous fragmentary information. (2) The pattern of the FSH and LH annual variations is robust, independent of the year investigated, fish origin and historical background, including previous salinity conditions. This is a strong support to the idea that the control of the annual cycle of reproduction is a robust and tightly regulated process where photoperiod is likely to play a major role; whether this involves a circannual clock remains an open question. (3) The other pituitary hormones (POMC, GH, PRL, SL, and TSH) deviate from this strictly controlled pattern as they displayed more flexible profiles depending on whether the fish had been reared under brackish or sea water. (4) Melatonin has pleiotropic effects in the pituitary of the European sea bass: we confirm, at the mRNA level, previously identified effects of melatonin on LH, GH and PRL proteins, and extend to FSH, POMC, SL, and TSH the range of hormones modulated by melatonin. At this stage it is not yet possible to determine whether the effects are direct and/or indirect. The cellular localization of the different melatonin receptor subtypes will help identifying the pituitary cellular targets. Also, more investigations are needed in order to determine whether the effects on mRNA are followed or not by effects at the level of the corresponding proteins for each of the hormones investigated. (5) The in vitro effects of melatonin depended on the month investigated as described before in trout (Falcón et al., 2003); this is in line with previous in vivo studies investigating the effects of pinealectomy on fish reproduction (Falcón et al., 2010; ViviD and Bentley, 2018). This highlights the necessity to run seasonal investigations when studying the effects of melatonin on neuroendocrine regulations, whether in vivo or in vitro. The effects depend on the daily and annual patterns of both, the circulating levels of the hormone as well as the abundance and affinity of its receptor subtypes. (6) Salinity appears as an important factor to consider when studying neuroendocrine responses in euryhaline species. Salinity modifies basal pituitary productions and melatonin plasma levels, as well as abundance of melatonin receptors. We believe the European sea bass pituitary exemplifies the statement that “countless endogenous and plastic physiological factors, as well as the perception and transduction of predictable and unpredictable environmental cues, coordinate… to adapt to ever-changing environments” (ViviD and Bentley, 2018). This is of crucial importance; it probably explains the observed versatility concerning the responses to melatonin from a species to another or even within the same species as evidenced here, making generalizations extremely difficult.
Data Availability Statement
The original contributions presented in the study are included in the article/Supplementary Material, further inquiries can be directed to the corresponding author.
Ethics Statement
The animal study was reviewed and approved by CNRS-UPMC; authorization #A-66-01-601.
Author Contributions
JF: project coordinator, search for funds, experimental design, experiments, data analysis, writing, training students, and submission. MH: experiments, data analysis, and reading proofs. LN: experiments, data analysis, and reading proofs. EI: experiments and data analysis. EP: experiments. MB: experimental design, experiments, and reading proofs. JA: statistical analysis. DC: fish maintenance. MF: fish maintenance and samplings. MD: search for funds, students training, and reading proofs. LB: students training and reading proofs. All authors contributed to the article and approved the submitted version.
Funding
This work was supported by the GDR2821 (CNRS, IFREMER, UJM, UPMC). EP was supported by a Ph.D. grant from the UPMC. LN and EI were supported by the EU FP7 program “ASSEMBLE”. MH was supported by a post-doctoral grant from the “Martin Escudero” Foundation (Spain).
Conflict of Interest
The authors declare that the research was conducted in the absence of any commercial or financial relationships that could be construed as a potential conflict of interest.
Publisher’s Note
All claims expressed in this article are solely those of the authors and do not necessarily represent those of their affiliated organizations, or those of the publisher, the editors and the reviewers. Any product that may be evaluated in this article, or claim that may be made by its manufacturer, is not guaranteed or endorsed by the publisher.
Acknowledgments
We are grateful and address our deep thank to Sandrine Sauzet, for her technical assistance and coaching of EP and MH, to the SOMLIT (CNRS, Service d’Observation en Milieu Littoral; http://somlit.epoc.u-bordeaux1.fr/fr/) for providing the temperature data, and to Gilbert Dutto and Eric Gasset for their assistance in the management of the SW fish at the IFREMER of Palavas-Les-Flots.
Supplementary Material
The Supplementary Material for this article can be found online at: https://www.frontiersin.org/articles/10.3389/fphys.2021.774975/full#supplementary-material
Abbreviations
BW, brackish water; D, dark; FW, freshwater; FSH, follicle-stimulating hormone or gonadotropin I- β; GH, growth hormone; L, light; LH, luteinizing hormone or gonadotropin II- β; PRL, prolactin; POMC, proopiomelanocortin; qPCR, real time quantitative PCR (qPCR); SW, sea water; SL, somatolactin; TSH, thyroid stimulating hormone or thyrotropin β.
References
Agustsson, T., and Bjornsson, B. T. (2000). Growth hormone inhibits growth hormone secretion from the rainbow trout pituitary in vitro. Comp. Biochem. Physiol. C Toxicol. Pharmacol. 126, 299–303. doi: 10.1016/s0742-8413(00)00120-1
Alvarado, M., Carrillo, M., and Felip, A. (2013). Expression of kisspeptins and their receptors, GnRH-1/GnRH-II-1a and gonadotropin genes in the brain of adult male and female European sea bass during different gonadal stages. Gen. Comp. Endocrinol. 187, 104–116. doi: 10.1016/j.ygcen.2013.03.030
Amano, M., Iigo, M., Ikuta, K., Kitamura, S., and Yamamori, K. (2003). Daily variations in melatonin binding sites in the masu salmon brain. Neurosci. Lett. 350, 9–12.
Aruna, A., Nagarajan, G., and Chang, C.-F. (2015). The acute salinity changes activate the dual pathways of endocrine responses in the brain and pituitary of tilapia. Gen. Comp. Endocrinol. 211, 154–164. doi: 10.1016/j.ygcen.2014.12.005
Bayarri, M. J., Falcón, J., Zanuy, S., and Carrillo, M. (2010). Continuous light and melatonin: daily and seasonal variations of brain binding sites and plasma concentration during the first reproductive cycle of sea bass. Gen. Comp. Endocrinol. 169, 58–64. doi: 10.1016/j.ygcen.2010.07.007
Bayarri, M. J., Rodriguez, L., Zanuy, S., Madrid, J. A., Sánchez-Vázquez, F. J., Kagawa, H., et al. (2004). Effect of photoperiod manipulation on the daily rhythms of melatonin and reproductive hormones in caged European sea bass (Dicentrarchus labrax). Gen. Comp. Endocrinol. 136, 72–81. doi: 10.1016/j.ygcen.2003.12.004
Bayarri, M. J., Zanuy, S., Yilmaz, O., and Carrillo, M. (2009). Effects of continuous light on the reproductive system of european sea bass gauged by alterations of circadian variations during their first reproductive cycle. Chronobiol. Int. 26, 184–199. doi: 10.1080/07420520902758311
Bhandari, R. K., Taniyama, S., Kitahashi, T., Ando, H., Yamauchi, K., Zohar, Y., et al. (2003). Seasonal changes of responses to gonadotropin-releasing hormone analog in expression of growth hormone/prolactin/somatolactin genes in the pituitary of masu salmon. Gen. Comp. Endocrinol. 130, 55–63. doi: 10.1016/s0016-6480(02)00536-1
Blanco, A. M. (2020). Hypothalamic- and pituitary-derived growth and reproductive hormones and the control of energy balance in fish. Gen. Comp. Endocrinol. 287:113322. doi: 10.1016/j.ygcen.2019.113322
Boeuf, G., and Falcón, J. (2001). Photoperiod and growth in fish. Vie et Milieu-Life Environ. 51, 247–266.
Ciani, E., Fontaine, R., Maugars, G., Mizrahi, N., Mayer, I., Levavi-Sivan, B., et al. (2019). Melatonin receptors in Atlantic salmon stimulate cAMP levels in heterologous cell lines and show season-dependent daily variations in pituitary expression levels. J. Pineal Res. 67:e12590. doi: 10.1111/jpi.12590
Confente, F., Rendón, M. C., Besseau, L., Falcón, J., and Muñoz-Cueto, J. A. (2010). Melatonin receptors in a pleuronectiform species, Solea senegalensis: cloning, tissue expression, day-night and seasonal variations. Gen. Comp. Endocrinol. 167, 202–214. doi: 10.1016/j.ygcen.2010.03.006
Cowan, M., Azpeleta, C., and López-Olmeda, J. F. (2017). Rhythms in the endocrine system of fish: a review. J. Comp. Physiol. B 187, 1057–1089. doi: 10.1007/s00360-017-1094-5
Davies, B., Hannah, L. T., Randall, C. F., Bromage, N., and Williams, L. M. (1994). Central melatonin binding-sites in rainbow-trout (Onchorhynchus mykiss). Gen. Comp. Endocrinol. 96, 19–26. doi: 10.1006/gcen.1994.1155
Deane, E. E., and Woo, N. Y. S. (2009). Modulation of fish growth hormone levels by salinity, temperature, pollutants and aquaculture related stress: a review. Rev. Fish Biol. Fish. 19, 97–120.
Ekström, P., and Meissl, H. (1997). The pineal organ of teleost fishes. Rev. Fish Biol. Fish. 7, 199–284.
Ekström, P., and Vaněček, J. (1992). Localization of 2-[125l]l-iodomelatonin binding sites in the brain of the Atlantic salmon, Salmo salar L. Neuroendocrinology 55, 529–537. doi: 10.1159/000126166
Estaras, M., Marchena, A. M., Fernández-Bermejo, M., Mateos, J. M., Vara, D., Roncero, V., et al. (2020). The melatonin receptor antagonist luzindole induces the activation of cellular stress responses and decreases viability of rat pancreatic stellate cells. J. Appl. Toxicol. 40, 1554–1565. doi: 10.1002/jat.4018
Falcón, J., Besseau, L., Fazzari, D., Attia, J., Gaildrat, P., Beauchaud, M., et al. (2003). Melatonin modulates secretion of growth hormone and prolactin by trout pituitary glands and cells in culture. Endocrinology 144, 4648–4658. doi: 10.1210/en.2003-0707
Falcón, J., Besseau, L., Sauzet, S., and Boeuf, G. (2007). Melatonin effects on the hypothalamo-pituitary axis in fish. Trends Endocrinol. Metab. 18, 81–88. doi: 10.1016/j.tem.2007.01.002
Falcón, J., Migaud, H., Muñoz-Cueto, J. A., and Carrillo, M. (2010). Current knowledge on the melatonin system in teleost fish. Gen. Comp. Endocrinol. 165, 469–482. doi: 10.1016/j.ygcen.2009.04.026
Falcón, J., Molina-Borja, M., Collin, J. P., and Oaknin, S. (1996). Age-related changes in 2-[I125]-Iodomelatonin binding sites in the brain of sea breams (Sparus aurata, L). Fish Physiol. Biochem. 15, 401–411.
Falcón, J., and Zohar, Y. (2018). “Photoperiodism in fish,” in Encyclopedia of Reproduction, ed. M. K. Skinner (Cambridge, Massachusetts: Academic Press), 400–408. doi: 10.1016/b978-0-12-809633-8.20584-0
Gaildrat, P., Becq, F., and Falcón, J. (2002). First cloning and functional characterization of a melatonin receptor in fish brain: a novel one? J. Pineal Res. 32, 74–84. doi: 10.1034/j.1600-079x.2002.1817.x
Gaildrat, P., and Falcón, J. (1999). “Expression of melatonin receptors and 2-[125]I-iodomelatonin binding sites in the pituitary of a teleost fish,” in Melatonin after Four Decades: an Assessment of Its Potential, ed. J. Olcese (Heidelberg: Springer), 61–72.
Gaildrat, P., and Falcón, J. (2000). Melatonin receptors in the pituitary of a teleost fish: mRNA expression, 2-[125]I-iodomelatonin binding and cyclic AMP response. Neuroendocrinology 72, 57–66.
Gaildrat, P., Ron, B., and Falcón, J. (1998). Daily and circadian variations in 2-[125]I-iodomelatonin binding sites in the pike brain (Esox lucius). J. Neuroendocrinol. 10, 511–517. doi: 10.1046/j.1365-2826.1998.00240.x
Garcia-Allegue, R., Madrid, J. A., and Sánchez-Vázquez, F. J. (2001). Melatonin rhythms in European sea bass plasma and eye: influence of seasonal photoperiod and water temperature. J. Pineal Res. 31, 68–75. doi: 10.1034/j.1600-079x.2001.310110.x
Gern, W., Dickhoff, W. W., and Folmar, L. C. (1984). Increases in plasma melatonin titers accompanying seawater adaptation of coho salmon (Oncorhynchus kisutch). Gen. Comp. Endocrinol. 55, 458–462. doi: 10.1016/0016-6480(84)90018-2
Harris, J., and Bird, D. J. (2000). Modulation of the fish immune system by hormones. Vet. Immunol. Immunopathol. 77, 163–176.
Herrera-Pérez, P., Servili, A., Rendón, M. C., Sánchez-Vázquez, F. J., Falcón, J., and Muñoz-Cueto, J. A. (2011). The pineal complex of the European sea bass (Dicentrarchus labrax): I. Histological, immunohistochemical and qPCR study. J. Chem. Neuroanat. 41, 170–180. doi: 10.1016/j.jchemneu.2011.01.006
Herrera-Pérez, P., Rendón, M. C., Besseau, L., Sauzet, S., Falcón, J., and Muñoz-Cueto, J. A. (2010). Melatonin receptors in the brain of the European sea bass: an in situ hybridization and autoradiographic study. J. Comp. Neurol. 518, 3495–3511. doi: 10.1002/cne.22408
Herrero, M. J., and Lepesant, J. M. J. (2014). Daily and seasonal expression of clock genes in the pituitary of the European sea bass (Dicentrarchus labrax). Gen. Comp. Endocrinol. 208, 30–38. doi: 10.1016/j.ygcen.2014.08.002
Hong, L. Y., Hong, W. S., Zhu, W. B., Shi, Q., You, X. X., and Chen, S. X. (2014). Cloning and expression of melatonin receptors in the mudskipper Boleophthalmus pectinirostris: their role in synchronizing its semilunar spawning rhythm. Gen. Comp. Endocrinol. 195, 138–150. doi: 10.1016/j.ygcen.2013.11.004
Ikegami, T., Motohashi, E., Doi, H., Hattori, A., and Ando, H. (2009). Synchronized diurnal and circadian expressions of four subtypes of melatonin receptor genes in the diencephalon of a puffer fish with lunar-related spawning cycles. Neurosci. Lett. 462, 58–63. doi: 10.1016/j.neulet.2009.06.076
Kara, M. H. (1997). Cycle sexuel et fécondité du Loup Dicentrarchus labrax (Poisson Moronidé) du golfe d’Annaba. Cah. Biol. Mar. 38, 161–168.
Khan, I. A., and Thomas, P. (1996). Melatonin influences gonadotropin II secretion in the Atlantic croaker (Micropogonias undulatus). Gen. Comp. Endocrinol. 104, 231–242. doi: 10.1006/gcen.1996.0166
Kleszczynska, A., Vargas-Chacoff, L., Gozdowska, M., Kalamarz, H., Martinez-Rodriguez, G., Mancera, J. M., et al. (2006). Arginine vasotocin, isotocin and melatonin responses following acclimation of gilthead sea bream (Sparus aurata) to different environmental salinities. Comp. Biochem. Physiol. A Mol. Integr. Physiol. 145, 268–273. doi: 10.1016/j.cbpa.2006.06.037
Laiz-Carrión, R., Fuentes, J., Redruello, B., Guzman, J. M., del Rio, M. P. M., Power, D., et al. (2009). Expression of pituitary prolactin, growth hormone and somatolactin is modified in response to different stressors (salinity, crowding and food-deprivation) in gilthead sea bream Sparus auratus. Gen. Comp. Endocrinol. 162, 293–300. doi: 10.1016/j.ygcen.2009.03.026
Legros, C., Dupre, C., Brasseur, C., Bonnaud, A., Bruno, O., Valour, D., et al. (2020). Characterization of the various functional pathways elicited by synthetic agonists or antagonists at the melatonin MT1 and MT2 receptors. Pharmacol. Res. Perspect. 8:e00539.
Liu, Z. F., Ma, A. J., Zhang, J. S., Yang, S. S., Cui, W. X., Xia, D. D., et al. (2020). Cloning and molecular characterization of PRL and PRLR from turbot (Scophthalmus maximus) and their expressions in response to short-term and long-term low salt stress. Fish Physiol. Biochem. 46, 501–517. doi: 10.1007/s10695-019-00699-2
Livak, K. J., and Schmittgen, T. D. (2001). Analysis of relative gene expression data using real-time quantitative PCR and the 2(T)(-Delta Delta C) method. Methods 25, 402–408. doi: 10.1006/meth.2001.1262
López-Olmeda, J. F., Oliveira, C., Kalamarz, H., Kulczykowska, E., Delgado, M. J., and Sánchez-Vázquez, F. J. (2009). Effects of water salinity on melatonin levels in plasma and peripheral tissues and on melatonin binding sites in European sea bass (Dicentrarchus labrax). Comp. Biochem. Physiol. A Mol. Integr. Physiol. 152, 486–490. doi: 10.1016/j.cbpa.2008.12.003
López-Patiño, M. A., Rodriguez-Illamola, A., Gesto, M., Soengas, J. L., and Miguez, J. M. (2011). Changes in plasma melatonin levels and pineal organ melatonin synthesis following acclimation of rainbow trout (Oncorhynchus mykiss) to different water salinities. J. Exp. Biol. 214, 928–936. doi: 10.1242/jeb.051516
Lu, X.-J., Hao, Z., Yang, G.-J., Li, M.-Y., and Chen, J. (2016). Comparative transcriptome analysis on the alteration of gene expression in ayu (Plecoglossus altivelis) larvae associated with salinity change. Zool. Res. 37, 126–135. doi: 10.13918/j.issn.2095-8137.2016.3.126
Mateos, J., Mañanos, E., Martínez-Rodriguez, G., Carrillo, M., Querat, B., and Zanuy, S. (2003). Molecular characterization of sea bass gonadotropin subunits (alpha, FSH beta, and LH beta) and their expression during the reproductive cycle. Gen. Comp. Endocrinol. 133, 216–232. doi: 10.1016/s0016-6480(03)00164-3
Migaud, H., Davie, A., and Taylor, J. F. (2010). Current knowledge on the photoneuroendocrine regulation of reproduction in temperate fish species. J. Fish Biol. 76, 27–68. doi: 10.1111/j.1095-8649.2009.02500.x
Oliveira, C., López-Olmeda, J. F., Delgado, M. J., Alonso-Gomez, A. L., and Sánchez-Vázquez, F. J. (2008). Melatonin binding sites in Senegal sole: day/night changes in density and location in different regions of the brain. Chronobiol. Int. 25, 645–652. doi: 10.1080/07420520802254437
Park, Y.-J., Park, J.-G., Kim, S.-J., Lee, Y.-D., Rahman, M. S., and Takemura, A. (2006). Melatonin receptor of a reef fish with lunar-related rhythmicity: cloning and daily variations. J. Pineal Res. 41, 166–174. doi: 10.1111/j.1600-079x.2006.00350.x
Piferrer, F., Blàzquez, M., Navarro, L., and Gonzàlez, A. (2005). Genetic, endocrine, and environmental components of sex determination and differentiation in the European sea bass (Dicentrarchus labrax L.). Gen. Comp. Endocrinol. 142, 102–110. doi: 10.1016/j.ygcen.2005.02.011
Rodriguez, L., Begtashi, I., Zanuy, S., Shaw, M., and Carrillo, M. (2001). Changes in plasma levels of reproductive hormones during first sexual maturation in European male sea bass (Dicentrarchus labrax L.) under bartificial day lengths. Aquaculture 202, 235–248. doi: 10.1016/s0044-8486(01)00774-8
Sakai, K., Yamamoto, Y., and Ikeuchi, T. (2019). Vertebrates originally possess four functional subtypes of G protein-coupled melatonin receptor. Sci. Rep. 9:9465. doi: 10.1038/s41598-019-45925-2
Sánchez-Vázquez, F. J., López-Olmeda, J. F., Vera, L. M., Migaud, H., López-Patino, M. A., and Miguez, J. M. (2019). Environmental cycles, melatonin, and circadian control of stress response in fish. Front. Endocrinol. 10:279. doi: 10.3389/fendo.2019.00279
Sauzet, S., Besseau, L., Pérez, P. H., Coves, D., Chatain, B., Peyric, E., et al. (2008). Cloning and retinal expression of melatonin receptors in the European sea bass, Dicentrarchus labrax. Gen. Comp. Endocrinol. 157, 186–195. doi: 10.1016/j.ygcen.2008.04.008
Sebert, M. E., Legros, C., Weltzien, F. A., Malpaux, B., Chemineau, P., and Dufour, S. (2008). Melatonin activates brain dopaminergic systems in the eel with an inhibitory impact on reproductive function. J. Neuroendocrinol. 20, 917–929. doi: 10.1111/j.1365-2826.2008.01744.x
Servili, A., Herrera-Pérez, P., Rendon, M. D., and Munoz-Cueto, J. A. (2013). Melatonin inhibits GnRH-1, GnRH-3 and GnRH receptor expression in the brain of the European sea bass, Dicentrarchus labrax. Int. J. Mol. Sci. 14, 7603–7616. doi: 10.3390/ijms14047603
Shin, H. S., Kim, N. N., Lee, J., Kil, G. S., and Choi, C. Y. (2011). Diurnal and circadian regulations by three melatonin receptors in the brain and retina of olive flounder Paralichthys olivaceus: profiles following exogenous melatonin. Mar. Freshw. Behav. Physiol. 44, 223–238.
Takei, Y., and McCormick, S. D. (2013). “Hormonal control of fish euryhalinity,” in Euryhaline Fishes, eds S. D. McCormick, A. P. Farrell, and C. J. Brauner (Waltham,MA: Academic Press), 69–123.
Underwood, H. (1989). The pineal and melatonin: regulators of circadian function in lower vertebrates. Experientia 45, 914–922.
Vandeputte, M., Clota, F., Sadoul, B., Blanc, M.-O., Blondeau-Bidet, E., Bégout, M.-L., et al. (2020). Low temperature has opposite effects on sex determination in a marine fish at the larval/postlarval and juvenile stages. Ecol. Evol. 10, 13825–13835. doi: 10.1002/ece3.6972
Vandeputte, M., Garouste, R., Dupont-Nivet, M., Haffray, P., Vergnet, A., Chavanne, H., et al. (2014). Multi-site evaluation of the rearing performances of 5 wild populations of European sea bass (Dicentrarchus labrax). Aquaculture 424, 239–248. doi: 10.1016/j.aquaculture.2014.01.005
Vandeputte, M., Quillet, E., and Chatain, B. (2012). Are sex ratios in wild European sea bass (Dicentrarchus labrax) populations biased? Aquat. Living Resour. 25, 77–81.
Vargas-Chacoff, L., Dann, F., Paschke, K., Oyarzun-Salazar, R., Nualart, D., Martinez, D., et al. (2021). Freshening effect on the osmotic response of the Antarctic spiny plunderfish Harpagifer antarcticus. J. Fish Biol. 98, 1558–1571. doi: 10.1111/jfb.14676
Varsamos, S., Xuereb, B., Commes, T., Flik, G., and Spanings-Pierrot, C. (2006). Pituitary hormone mRNA expression in European sea bass Dicentrarchus labrax in seawater and following acclimation to fresh water. J. Endocrinol. 3, 473–480. doi: 10.1677/joe.1.06847
ViviD, D., and Bentley, G. E. (2018). Seasonal reproduction in vertebrates: melatonin synthesis, binding, and functionality using Tinbergen’s four questions. Molecules 23:652. doi: 10.3390/molecules23030652
Whitmore, D., Cermakian, N., Crosio, C., Foulkes, N. S., Pando, M. P., Travnickova, Z., et al. (2000a). A clockwork organ. Biol. Chem. 381, 793–800.
Whitmore, D., Foulkes, N. S., and Sassone-Corsi, P. (2000b). Light acts directly on organs and cells in culture to set the vertebrate circadian clock. Nature 404, 87–91. doi: 10.1038/35003589
Yamaguchi, Y., Moriyama, S., Lerner, D. T., Grau, E. G., and Seale, A. P. (2016). Autocrine positive feedback regulation of prolactin release from tilapia prolactin cells and its modulation by extracellular osmolality. Endocrinology 157, 3505–3516. doi: 10.1210/en.2015-1969
Yuan, M. Z., Jia, Q. Q., Wang, T., Lu, Q., Tang, L. L., Wang, Y. J., et al. (2017). Dynamic responses of prolactin, growth hormone and their receptors to hyposmotic acclimation in the olive flounder Paralichthys olivaceus. Gen. Comp. Endocrinol. 254, 8–13. doi: 10.1016/j.ygcen.2017.09.005
Keywords: sea bass, pituitary, hormones, annual variations, melatonin, photoperiod, salinity
Citation: Falcón J, Herrero MJ, Nisembaum LG, Isorna E, Peyric E, Beauchaud M, Attia J, Covès D, Fuentès M, Delgado MJ and Besseau L (2021) Pituitary Hormones mRNA Abundance in the Mediterranean Sea Bass Dicentrarchus labrax: Seasonal Rhythms, Effects of Melatonin and Water Salinity. Front. Physiol. 12:774975. doi: 10.3389/fphys.2021.774975
Received: 13 September 2021; Accepted: 12 November 2021;
Published: 15 December 2021.
Edited by:
José A. Muñoz-Cueto, University of Cádiz, SpainReviewed by:
Mairi Elizabeth Cowan, Scottish Association For Marine Science, United KingdomJesús M. Míguez, University of Vigo, Spain
Copyright © 2021 Falcón, Herrero, Nisembaum, Isorna, Peyric, Beauchaud, Attia, Covès, Fuentès, Delgado and Besseau. This is an open-access article distributed under the terms of the Creative Commons Attribution License (CC BY). The use, distribution or reproduction in other forums is permitted, provided the original author(s) and the copyright owner(s) are credited and that the original publication in this journal is cited, in accordance with accepted academic practice. No use, distribution or reproduction is permitted which does not comply with these terms.
*Correspondence: Jack Falcón, j.falcon-pro@orange.fr
†These authors have contributed equally to this work
‡Present address: Maria Jesus Herrero, Campus Los Leones, School of Medicine, Universidad San Sebastian, Santiago, Chile