- 1School of Psychology, South China Normal University, Guangzhou, China
- 2Lab of Light and Physiopsychological Health, National Center for International Research on Green Optoelectronics, South China Normal University, Guangzhou, China
- 3Guangdong Provincial Key Laboratory of Optical Information Materials and Technology and Institute of Electronic Paper Displays, South China Normal University, South China Academy of Advanced Optoelectronics, Guangzhou, China
- 4Department of Counseling and Industrial/Organizational Psychology, Ming Chuan University, Taoyuan, Taiwan
- 5Department of Psychology, National Chengchi University, Taipei, Taiwan
- 6The Research Center for Mind, Brain, and Learning, National Chengchi University, Taipei, Taiwan
Light can induce an alertness response in humans. The effects of exposure to bright light vs. dim light on the levels of alertness during the day, especially in the afternoon, as reported in the literature, are inconsistent. This study employed a multiple measurement strategy to explore the temporal variations in the effects of exposure to bright light vs. regular office light (1,200 lx vs. 200 lx at eye level, 6,500 K) on the alertness of participants for 5 h in the afternoon. In this study, 20 healthy adults (11 female; mean age 23.25 ± 2.3 years) underwent the Karolinska sleepiness scale (KSS), the auditory psychomotor vigilance test (PVT), and the waking electroencephalogram (EEG) test for two levels of light intervention. The results yielded a relatively lower relative delta power and a relatively higher beta power for the 1,200 lx condition in comparison with the 200 lx condition. However, the light conditions elicited no statistically significant differences in the KSS scores and performance with respect to the PVT. The results suggested that exposure to bright light for 5 h in the afternoon could enhance physiological arousal while exerting insignificant effects on subjective feelings and performance abilities relating to the alertness of the participants.
Introduction
Since the discovery of intrinsically photosensitive retinal ganglion cells (ipRGCs), light is shown to have an influence on human physiological and psychological functions as a result of the so-called non-image forming (NIF) functions of light (Berson et al., 2002; Hattar et al., 2002), such as the effects of light on alertness (Cajochen, 2007; Figueiro et al., 2018; Pachito et al., 2018; Souman et al., 2018; Xu and Lang, 2018), mood (Bedrosian and Nelson, 2013, 2017), cognitive function (Vandewalle et al., 2009; Fisk et al., 2018; Ru et al., 2019, 2021), and the regulation of the circadian rhythm (LeGates et al., 2014; Prayag et al., 2019b; Tahkamo et al., 2019) and sleep (Figueiro et al., 2017; Boubekri et al., 2020; Peeters et al., 2021; Stefani et al., 2021). Alertness is a key topic of such research due to its importance to human life. Although the effects of light on alertness have been explored using both subjective and objective measures, the research results are far from consistent, especially the results for diurnal studies (Lok et al., 2018a; Souman et al., 2018; Xu and Lang, 2018; Sithravel and Ibrahim, 2021). Several factors may contribute to the discrepancies in research, such as the light level and the spectrum, the time of day/year, the duration of light exposure, as well as previous light history before the laboratory studies commenced. Optimization of the daytime functions of office workers with respect to artificial light is a primary concern in the interior design and ergonomics of buildings. The current literature suggests that manipulating the level of office light rather than correlation of the color temperature (CCT) can elicit greater non-visual effects during the daytime (Min et al., 2013; Park et al., 2013; Ru et al., 2019). Therefore, the current study is concerned with how high-intensity light influences the physiological and psychological alertness of participants during the daytime.
While the effects of the intensity of polychromatic white light on alertness during the daytime have been extensively studied, the results have revealed no conclusive or consistent findings based on subjective ratings, performance, and physiological indicators of alertness (Lok et al., 2018a; Souman et al., 2018). Some studies have reported positive effects of bright light vs. dim light on the subjective ratings of alertness (Akerstedt et al., 2003; Phipps-Nelson et al., 2003; Ruger et al., 2006; Vandewalle et al., 2006; Iskra-Golec and Smith, 2008; Smolders et al., 2012; Smolders and De Kort, 2014; Huiberts et al., 2017; Te Kulve et al., 2017), while others have reported no significant effects (Sahin et al., 2014; Huiberts et al., 2016). Additionally, the same situation applies regarding the effects of light levels on objective alertness as assessed by undertaking computerized tasks [e.g., the psychomotor vigilance test (PVT)] (Phipps-Nelson et al., 2003; Smolders et al., 2012; Smolders and De Kort, 2014; Huiberts et al., 2016, 2017; Maierova et al., 2016; Münch et al., 2016; Te Kulve et al., 2017), during which the duration of light exposure ranged from 15 min to 5 h. In addition, exposure to bright light vs. dim light is shown to moderate physical arousal as measured by the electroencephalogram (EEG), suggesting a decrease in lower power densities, such as the theta and the theta–alpha band (Vandewalle et al., 2006; Sahin et al., 2014; Smolders et al., 2016). These findings suggested that the alertness effects of light vary as a function of the waveband of the light for different areas of the brain (Smolders et al., 2016). For instance, the regulation of the theta wave is most prominent in the frontal area of the brain, which is associated with sleepiness and the homeostatic sleep drive (Cajochen et al., 2001; Strijkstra et al., 2003). Recently, two independent studies explored the dose-response relationships between the light level and alertness during the daytime (Lok et al., 2018b; Smolders et al., 2018). Unlike the dose-response relationships established during the nighttime (Cajochen et al., 2000), no conclusive findings were found for these two diurnal studies. It is speculated that the relatively short duration (about 1 h) of light exposure in these studies might have yielded results that were not conclusive (Smolders et al., 2018). Thus, whether the duration of light exposure would moderate the alertness effects in response to daytime light remains largely unknown.
The duration of light exposure has been considered a potential variable in moderating the NIF effects of daytime light on mental function (Khademagha et al., 2016; Lok et al., 2018a; Souman et al., 2018; Sithravel and Ibrahim, 2021). The time durations adopted in the previous studies ranged from 15 min (Iskra-Golec and Smith, 2008) to 10 h (Maierova et al., 2016). Indeed, the duration of light exposure was less than 1 h in 10 of 19 studies reviewed in a recent review article (Lok et al., 2018b). Although an exposure duration as short as 1 min has been shown to have significant NIF effects during the nighttime (Prayag et al., 2019a), these effects may not be subject to same controls during the daytime as melatonin levels are minimal during the biological day (Dijk et al., 1992; Hull et al., 2003; Akerstedt et al., 2017). A relatively short duration of light exposure during the daytime might thus not be enough to elicit an alertness response. For example, a recent study explored the effects of the CCT on subjective alertness, effort-related cardiac response, and cognitive performance during the daytime. The results showed that 4 min of exposure to light did not elicit significant changes in alertness and task performance (Lasauskaite et al., 2018). Similarly, several previous studies using 15 min (Iskra-Golec and Smith, 2008) or 55 min (Huiberts et al., 2016) of light exposure revealed non-significant effects on the performance or subjective indicators of alertness. Several recent studies found that bright light did not have an effect on alertness even when the duration of exposure was extended to 1 h (Lok et al., 2018b; Smolders et al., 2018) or 90 min (Lok et al., 2019; De Vries et al., 2020). However, bright light has been shown to increase subjective alertness in the case of exposure to it for 3 h (Münch et al., 2016), 4 h (Ruger et al., 2006), and 5 h (Phipps-Nelson et al., 2003). Although the aforementioned studies suggest that different durations of bright light exposure might lead to inconsistencies in results, it is still difficult to directly compare findings in different studies given the differences in study paradigm and the various light manipulations that were employed. Studies to discern the relationship between alertness and bright light exposure in the daytime for extended durations are, therefore, warranted. Thus, current research is concerned with exploring whether extended exposure durations of up to 5 h will moderate alertness response of an individual to bright light in the afternoon. In addition, the study design seeks to address whether participants experience more or less mental fatigue after exposure. The light exposure period of 5 h served to capture how alertness dynamically changes under bright light illumination. The results of the current study could provide evidence-based data in support of healthy lighting design and intervention applications.
In addition to the duration of light exposure, the various indicators employed to assess the alertness response of an individual to light reported in previous studies make it difficult to compare their findings directly. Additionally, it should be noted that assessment of previous studies has revealed a rather inconsistent pattern concerning the effects of daytime bright light vs. dim light on the subjective and objective indicators of alertness within a similar study paradigm (Smolders et al., 2012; Huiberts et al., 2015, 2016, 2017). Studies employing subjective indicators of alertness even revealed inconsistent findings (Smolders and De Kort, 2014; Leichtfried et al., 2015; Huiberts et al., 2016; Weisgerber et al., 2017). Only four studies, which involved three indicators of alertness, yielded consistent findings (one with no effect (Badia et al., 1991) and three with positive results (Phipps-Nelson et al., 2003; Huiberts et al., 2015; Münch et al., 2016). Maierova et al. (2016) and Te Kulve et al. (2017) reported a positive effect of bright light on subjective alertness but not on objective alertness. These results suggested that the various indicators of alertness with respect to sensitivity respond differently to light manipulation during the daytime. To address this problem, the use of a multi-measurement approach might be a feasible option for directly comparing the alertness effects caused by bright light on subjective ratings, task performance, and EEG activity.
To investigate the temporal dynamics of alertness during the daytime, the present study utilized extended exposure duration periods of up to 5 h, and a multi-measurement approach was adopted to trace the trajectory effects of bright light on the subjective, objective, and physiological alertness during the afternoon. Participants were assigned to the conditions that experienced exposure to bright light vs. regular office light for 5 h during the daytime, and during which the Karolinska sleepiness scale (KSS), an auditory PVT, and the waking EEG were repeatedly measured during light manipulation. It was hypothesized that exposure to bright light vs. office light in the afternoon would induce an exposure duration-dependent alertness effect, and with more pronounced effects on alertness indicators being experienced as the duration of exposure was extended.
Materials and Methods
Experimental Design
A within-subject design was used in the study with the light conditions (200 lx vs. 1,200 lx at 6,500 K at eye level) being the independent factor. All participants visited the laboratory twice at intervals of at least 1 week. The participants were exposed to light from a light box for 5 consecutive hours. The order of light condition was balanced across the participants with half of the participants receiving the bright light first.
Participants
Twenty participants (11 female, 20–30 years old, mean age 23.25 years, SD = 2.3) met all criteria for the laboratory-based study. The criteria for inclusion in the study were as follows: (1) no extreme chronotype according to the Munich Chronotype Questionnaire (Roenneberg et al., 2003), (2) no color blindness, (3) no diagnosis of physiological or psychological disease based on a subjective report, (4) had not traveled across time zones for a month before the commencement of the study, and (5) had had a regular sleep (7–9 h), which meant going to bed between 21:00 pm and 24:00 pm and waking up time between 6:00 am and 10:00 am. The research was approved by the National Chengchi university Ethical Committee and was conducted in accordance with the requisite university guidelines and the principles of the Helsinki Declaration.
Room and Lighting Settings
The study was conducted in a laboratory with two separate compartmentalized rooms. The rooms were soundproofed with no additional light source. The walls and ceilings of the rooms were all white, and the floors were solid wood. The dimensions of the rooms were 5.7 m × 2.5 m × 3.1 m and 3.7 m × 2.5 m × 3.1 m, respectively. Each room had a table on which a light box had been placed. The light box was a cuboid made of wooden materials. White paint inside the box caused the light to be evenly distributed. Bright white light and common light were directed to the corneas of participants by arrays of light-emitting diodes (LEDs) to avoid direct viewing of the light. The light was emitted from a hole in the top of the box.
The spectral power distributions (SPD) of the two light conditions (1,200 lx at 6,500 K and 200 lx at 6,500 K) are presented in Figure 1 and were measured by a TES-1335 digital illuminance meter (TES Electrical Electronic Corp., Taipei, Taiwan) at eye level. The light level was 100 lx at 6,500 K at eye level for baseline measurement for both light conditions. Then the light level was adjusted to either 1,200 lx (photon density: 1.04 × 1015 photons s–1 cm–2; irradiance: 375.14 μW cm–2) or 200 lx (photon density: 1.54 × 1014 photons s–1 cm–2; irradiance: 55.30 μW cm–2) at 6,500 K at eye level after the baseline phase. The effective irradiances of each retinal photoreceptor provided by the two light conditions are shown in Table 1 (Lucas et al., 2014; Spitschan et al., 2019). During the experiment, the temperature in the rooms was maintained at 25°C by air conditioning.
Procedure
Before commencing the study, all participants provided their informed consent and completed a screening questionnaire. Only the qualified participants were allowed to participate in the formal experiments. The laboratory sessions began at 13:00 pm and ended at 19:00 pm and included 30 min for the baseline measurement and 5 h for light exposure. An overview of the experiment procedure is given in Figure 2. During the baseline measurement, the KSS, a 10-min auditory PVT, and a 3-min waking EEG recording were conducted under 100 lx at 6,500 K (at eye level). After the baseline assessment, the light level was adjusted to either 200 lx or 1,200 lx (at 6,500 K at eye level) for 5 consecutive h (from 14:00 pm to 19:00 pm), during which time the subjective sleepiness of participants was measured every half hour (T1, T2, T3, T4, T5, T6, T7, T8, T9, and T10). The PVT and the waking EEG were assessed hourly from 14:00 pm to 19:00 pm [T2 (15:00), T4 (16:00), T6 (17:00), T8 (18:00), and T10 (19:00)].
The participants were required to maintain a fixed sitting posture in front of the light box and could not engage in activities, such as listening to music or reading to eliminate the interference of potentially interfering variables, such as activities and exposure to natural light, during the 5-h light intervention. The participants were permitted to drink water but coffee or other beverages containing caffeine and alcohol were forbidden on the days of the experiments. At about 18:00 pm, the participants were given a light meal that could be finished within about 5 min. During the EEG recording, the participants were asked to relax, keep their eyes open, and focus on a spot on the light box while avoiding frequent blinking and head movements (as shown in Figure 2 for an overview of the experimental procedure).
Measures
Subjective Alertness
The KSS was used to assess subjective alertness, the values ranging from 1, “very alert,” to 9, “extremely sleepy” (Akerstedt and Gillberg, 1990). The KSS values were collected every half hour.
Objective Alertness
The auditory PVT was employed to assess objective alertness (Dinges and Powell, 1985). For this test, audible beeps (100 ms, 1,000 Hz, 60 dB) were delivered through a pair of headphones at randomized intervals of 2–10 s. The participants were required to press the space bar on the keyboard as soon as they heard the beep. The average reaction speed in the overall trials, the fastest 10% trials, and the slowest 10% trials were calculated separately as objective indicators of alertness.
Physiological Alertness
The EEG was recorded during the Karolinska drowsiness test (KDT) (Akerstedt and Gillberg, 1990) at six referential channels—C3–A2, C4–A1, F3–A2, F4–A1, O1–A2, and O2–A1—by using the Embla digital system (Embla N7000 digital PSG system; Rembrandt Embla Company, Broomfield, CO, United States). During the EEG recordings, the participants were required to sit in front of the light boxes without moving their heads, look straight ahead at a sign, and not blink or shake the body for 3 min. All EEG signals were low-pass filtered at 35 Hz and high-pass filtered at 0.3 Hz at a sampling rate of 200 Hz. All subsequent EEG data were analyzed using the Brain Product Analyzer software. The differential standard has been used to classify the EEG band in previous studies (Cajochen et al., 2005; Besserve et al., 2007; Rahman et al., 2014; Sahin et al., 2014; Iskra-Golec et al., 2017; Hsiao et al., 2018). In the current study, the classification of the EEG band, as referred to in the study of Hsiao et al. (2018), corresponds to the frequency bands were defined as delta (0.5–2.5 Hz), theta (2.5–7.5 Hz), alpha (7.5–12 Hz), and beta (14–35 Hz). Expanding the range of theta bands enables one to explore how the low-frequency theta bands vary under bright light conditions (Makeig et al., 2000). The relative ratio of the relative EEG power to the power ratio obtained at the baseline was calculated in the statistical analysis. Similar to the PVT, the EEG data were collected hourly after the PVT. Light-induced moderations of the theta, alpha, and beta bands were used as indicators of physiological alertness (Lockley et al., 2006; Phipps-Nelson et al., 2009; Cajochen et al., 2011; Rahman et al., 2014).
Statistical Analysis
Before further analysis, the reaction speed recorded on the PVT was inverted to increase the normality of the raw data. Because of the nested structure of the data, the linear mixed model (LMM) analyses were performed to investigate the effects of light conditions on subjective alertness, task performance, and resting EEG. Given that the order for exposure by the two light conditions was balanced across the participants, the “order” of the light conditions was used as a fixed factor in the preliminary model. The results obtained did not reveal any significant effects, such as the interaction between the order and the light conditions or possible interactions between the order, light conditions, and test time. Moreover, the subjective alertness, task performance, and physiological indicators were measured multiple times for the two light conditions; and the test time was added as an additional fixed factor in the model. It was surmised that the multiple measurements may result in a correlation occurring between repeated measurements within one session thus chi-square tests were used to identify the differences in the outcomes between the models to verify whether their fitness had significantly improved (Field, 2013). AR(1) was used as a repeated covariance structure based on chi-square comparisons of the null models with different repeated covariance types [Scaled Identity, Autoregressive (1), and Diagonal].
An LMM was employed with light condition (200 lx vs. 1,200 lx) as a fixed factor and Participant ID was used as the random intercept for subjective alertness, PVT performance, and EEG activity to check the differences in the baseline measurements. Subsequently, LMM was used with light condition (200 lx vs. 1,200 lx) and test time (T1–T10) as a fixed factor, and participant ID and Session as the random intercept to evaluate the effect of light condition on the subjective alertness. The LMMs with light conditions (200 lx vs. 1,200 lx) and test time (T2, T4, T6, T8, and T10) as a fixed factor, and Participant ID and Session as the random intercept were employed to evaluate the effect of light conditions on alertness performance and resting EEG power. Effect sizes (one of the pseudo R2-values indicator ) were calculated by dividing the model chi-square, which represents the change from the null model by the null model—2log-likelihood. is a measure of the proportional reduction in the absolute value of the log-likelihood value, which is a determinant of the extent to which the fit improves as a result of the inclusion of the predictor variables (Field, 2013). The change in value is from 0 to 1, where 0 represents the condition whereby the predictors are unable to predict the outcome variable and 1 means that the model predicts the outcome variable perfectly (Hosmer and Lemeshow, 2013). The post-hoc tests with the Bonferroni correction were used to investigate differences in the dependent variables of the test time, the estimated marginal means (EMMs), and the SEs. All the statistical analyses were performed using SPSS Statistics 25.
Results
Baseline Comparisons
The LMM analyses of the KSS scores and performance on the PVT at the baseline revealed no significant difference between light conditions (p > 0.1). However, significant differences were found in several spectral bands in different channels between light conditions at the baseline. Thus, all waking EEG data were processed by dividing the original data for T2, T4, T6, T8, and T10 by the baseline T0. The original data were normalized before further statistical analysis.
Effects of Light Condition on Alertness
Subjective Alertness (Karolinska Sleepiness Scale)
The LMM analyses of the KSS scores revealed no significant effect of light condition [F(1, 64) = 0.188, p = 0.666], nor that of light condition × test time interaction [F(9, 220) = 0.521, p = 0.858]. Test time did have a significant main effect [F(9, 232) = 3.68, p < 0.001, R2pseudo = 0.032] (as shown in Figure 3). The post-hoc comparisons indicated that the KSS scores at T3 (EMMT3 = 4.28, SE = 0.24) and T5 (EMMT5 = 4.20, SE = 0.24) were both significantly lower than that at T10 (EMMT10 = 5.04, SE = 0.24, all p < 0.05), suggesting that the participants felt more alert after 1.5 and 2.5 h of light exposure than at the end of the 5-h exposure. The KSS score at T2 (EMMT2 = 4.86, SE = 0.24) was significantly higher than that at T3 (EMMT3 = 4.28, SE = 0.24, p = 0.045), suggesting that the participants felt more alert after 1.5 h of exposure than after 1 h.
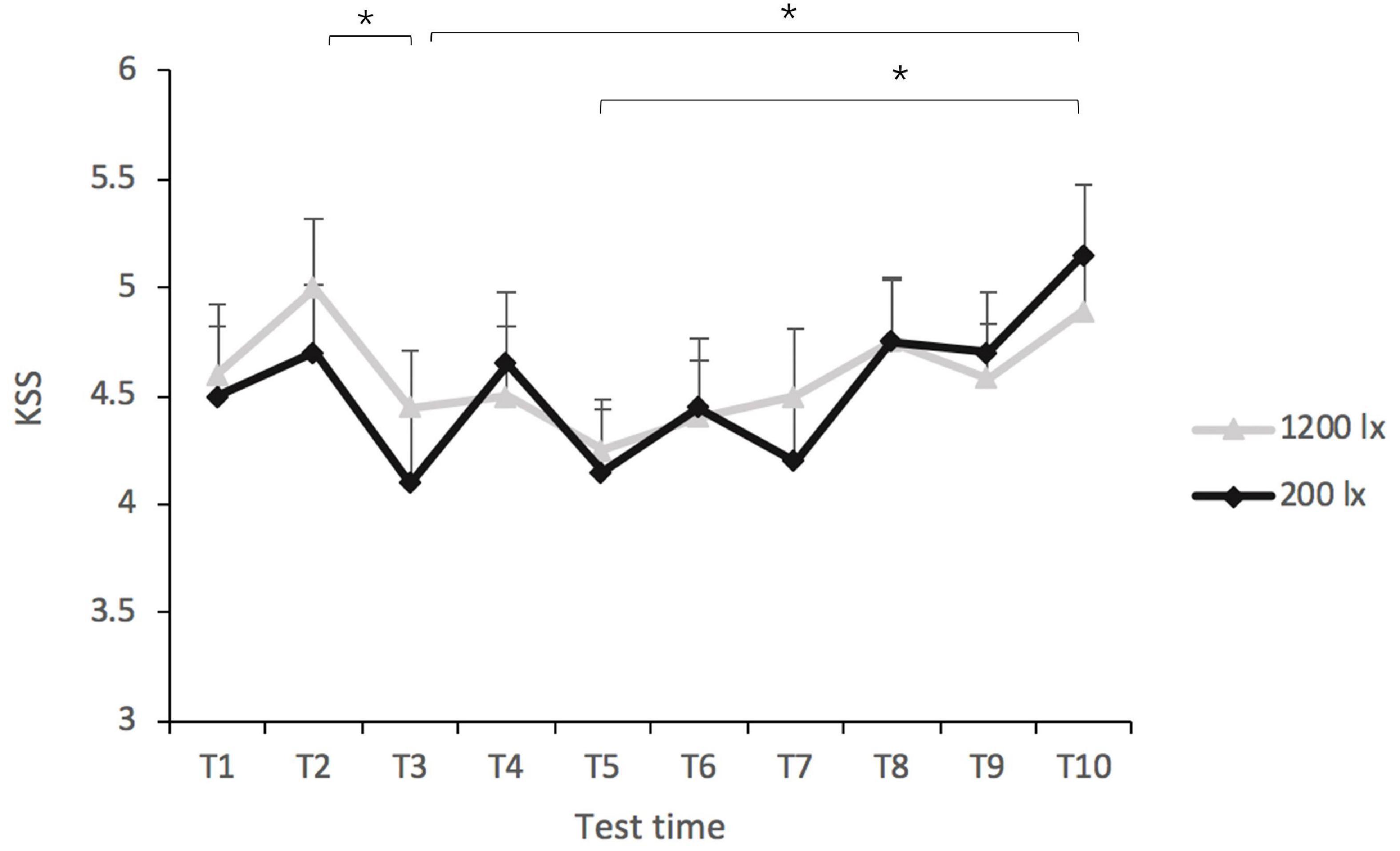
Figure 3. Scores of the Karolinska sleepiness scale (KSS) in the 200 lx (dim) and 1,200 lx (bright) conditions. *p < 0.05.
Objective Alertness (Psychomotor Vigilance Task)
Light condition [F(1, 41) = 0.77, p = 0.384] and light condition × test time had no significant effect ([F(1, 137) = 0.25, p = 0.911] on the overall reaction speed on the PVT. Test time did have a significant main effect on the overall reaction speed [F(4, 139) = 2.86, p < 0.05, R2pseudo = 0.160] (as shown in Figure 4). The post-hoc tests indicated that the reaction speed was faster at T2 (EMMT2 = 3.34, SE = 0.13) than at T10 (EMMT10 = 3.12, SE = 0.13), suggesting that the reaction time exhibited an increasing trend with time (as shown in Figure 4). The estimates of reaction speed at T4 (EMMT4 = 3.27, SE = 0.13), T6 (EMMT6 = 3.21, SE = 0.13), and T8 (EMMT8 = 3.18, SE = 0.13) were not significantly different.
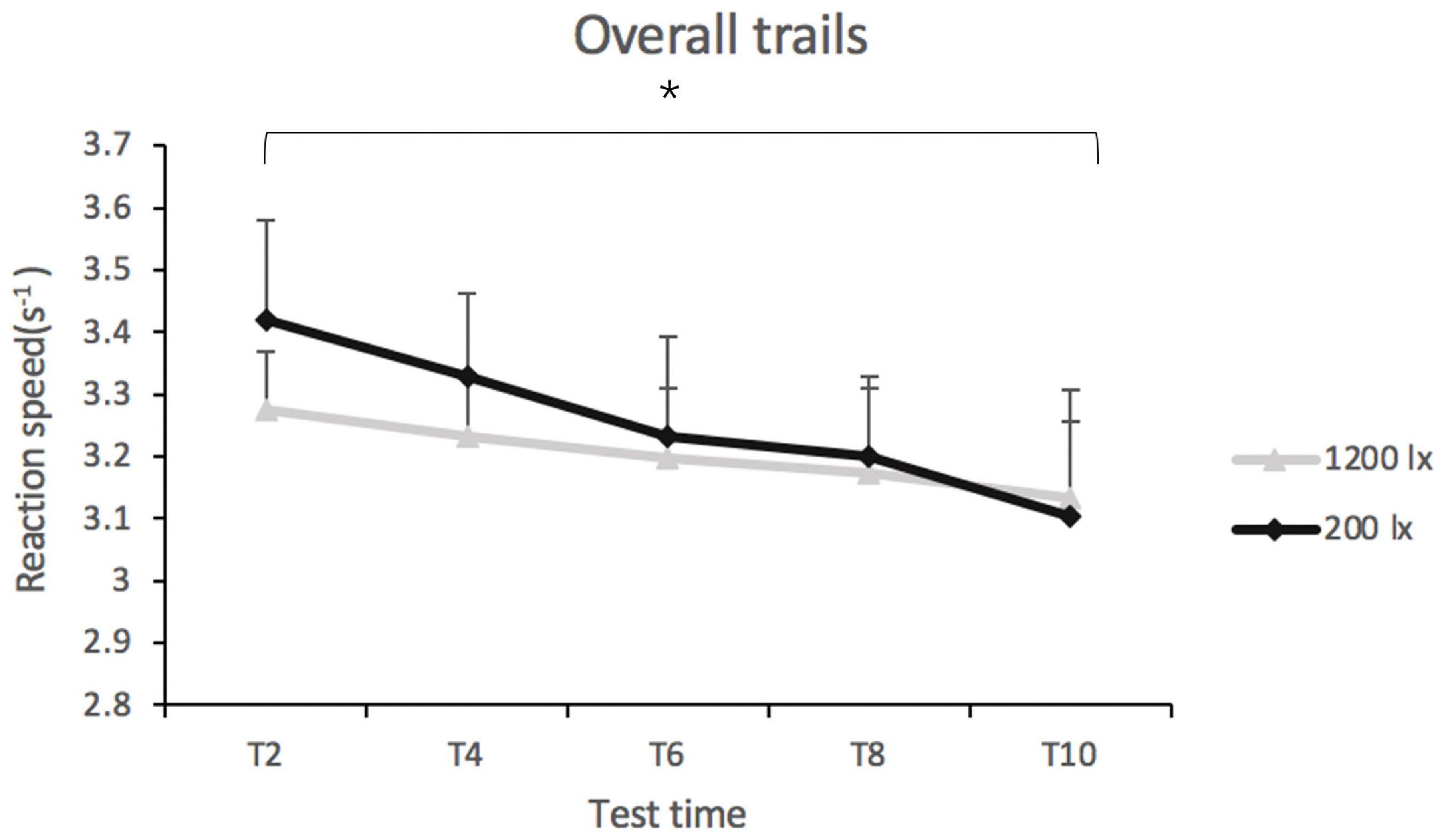
Figure 4. Overall reaction speed in trials of psychomotor vigilance test (PVT) in 200 lx (dim) and 1,200 lx (bright) conditions. *p < 0.05.
The LMM analysis of the fastest 10% of reaction speeds revealed no significant effect of light condition [F(1, 36) = 2.35, p = 0.134], test time [F(4, 137) = 1.89, p = 0.116], or that due to the interaction between light condition × test time [F(4, 122) = 0.089, p = 0.986]. Similarly, light condition, test time, and the interaction between them had no significant effect on the slowest 10% of reaction speeds [F(1, 40) = 0.07, p = 0.794; F(4, 139) = 2.23, p = 0.069; and F(4, 128) = 0.66, p = 0.621].
Physiological Alertness (Electroencephalogram)
Light condition had a significant main effect on the delta band at F3 [F(1, 42) = 8.46, p = 0.006, R2pseudo = 0.157], F4 [F(1, 78) = 7.86, p = 0.006, R2pseudo = 0.012], C3 [F(1, 48) = 5.79, p = 0.020, R2pseudo = 0.05], and O2 [F(1, 45) = 5.12, p = 0.028, R2pseudo = 0.057]. The post-hoc tests revealed a significantly lower delta band under 1,200 lx condition (EMM 1,200 lx = 0.84; SE = 0.08) compared with the 200 lx exposure (EMM 200 lx = 1.06; SE = 0.08) at F3 (as shown in Figure 5A); the same results were noted on channels F4, C3, and O2, with lower delta bands being observed for the 1,200 lx condition compared with the 200 lx condition. The effect of test time were also significant on the delta band at channels F3 [F(4, 154) = 3.36, p = 0.012] and F4 [F(4, 154) = 2.83, p = 0.027]. The post-hoc tests showed that the delta band was significantly higher for test time 2 (EMMT2 = 1.09; SE = 0.07) compared with test time 10 (EMMT10 = 0.83; SE = 0.07) at F3. At channel F4, the delta band was significantly higher for test time 2 (EMMT2 = 1.11; SE = 0.07) compared with test time 10 (EMMT10 = 0.84; SE = 0.07). No significant difference was found in the delta band at C4 [F(1, 53) = 2.99, p = 0.089] and O1 [F(1, 38) = 1.28, p = 0.265]. There was no significant interaction effect (all F < 1, p’s > 0.05) on the delta band either.
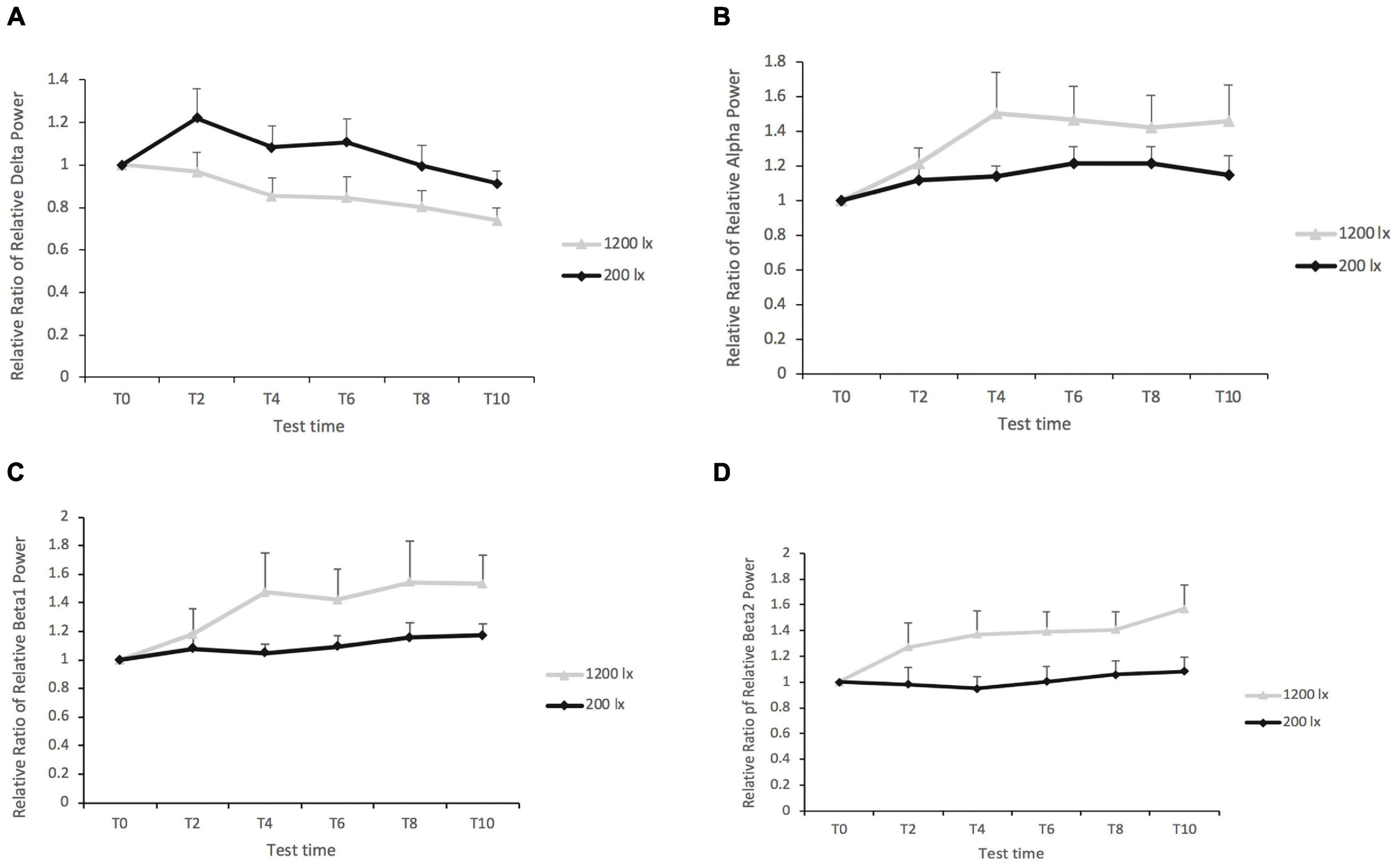
Figure 5. (A) The relative ratio of the relative delta power in region F3 between 1,200 and 200 lx. (B) The relative ratio of the relative alpha power in region O1 between 1,200 and 200 lx. (C) The relative ratio of the relative beta1 power in region F3 between 1,200 and 200 lx. (D) The relative ratio of relative beta2 power in region F3 between 1,200 and 200 lx. The figure shows only some of the significant results of the channels.
The results for the theta band revealed no significant main effects or interaction effects (all p > 0.05).
In the case of the alpha band, the results revealed a significant main effect for the light condition at channel O1 [F(1, 101) = 4.03, p = 0.047, R2pseudo = 0.06], with a higher alpha band in the 1,200 lx condition than in the 200 lx condition (as shown in Figure 5B). Light condition elicited no significant difference in the alpha power spectrum in the central and frontal areas of the brain (all p > 0.05). The main effect of test time (all p > 0.05) and its interaction effects (all ps > 0.05) did not have significance on the alpha band.
Light condition had significant effects on both the beta1 (as shown in Figure 5C) and the beta2 bands (as shown in Figure 5D) on all channels (all p < 0.05), except for the beta1 band on C4 (p = 0.066). The post-hoc tests revealed higher beta1 and beta2 for the 1,200 lx condition than the 200 lx light condition. The main effect of test time, and the interaction between the light condition and the test time, was not significant on the powers of beta1 and beta2 (all p > 0.05).
Discussion
The effects of exposure to bright light versus office light (1,200 vs. 200 lx at eye level, 6,500 K) for 5 h in the afternoon on the alertness of participants were examined using a multi-measurement experimental design strategy. The results revealed that prolonged exposure to a higher level of light in the afternoon lowered the relative delta power and increased the relative beta power in comparison with exposure to regular office light, indicating an increase in cortical arousal. However, the subjective measure of alertness (KSS) and performance on the vigilance test (PVT) remained unaffected as a result of light manipulation.
The findings revealed that 5 h of exposure to 1,200 vs. 200 lx at 6,500 K light in the afternoon elicited no statistically significant improvement of subjective alertness as assessed with the KSS, and this is consistent with the previous studies suggesting no significant effects of bright light vs. dim light on subjective alertness for relatively short (i.e., 55 min) or longer durations (i.e., 110 min) (Sahin et al., 2014; Huiberts et al., 2016; Lok et al., 2018b; Smolders et al., 2018; De Vries et al., 2020). In contrast, other studies have reported significant effects from exposure to bright light for 90 min–5 h during the daytime (Phipps-Nelson et al., 2003; Ruger et al., 2006; Maierova et al., 2016; Münch et al., 2016). Such discrepancies might have occurred due to the difference in light levels in the experimental sessions or the baseline measurements (ranging from ≤ 10–40 lx vs. 200 lx for the present study) (Akerstedt et al., 2003; Phipps-Nelson et al., 2003; Ruger et al., 2006; Vandewalle et al., 2006; Sahin et al., 2014; Maierova et al., 2016; Münch et al., 2016; Te Kulve et al., 2017). Additionally, the monotony of remaining for 5 h in front of a lamp without performing any tasks is probably the reason for finding no significant results related to subjective alertness. Moreover, the participants did feel more alert after 1.5 and 2.5 h of light exposure (T3 and T5) than at the end of the experimental session (T10) for both light conditions, indicating that participants did felt significantly less alert at the end of the 5 h session than earlier in the session regardless of the light conditions. We thus speculate that when participants lack any activities and are under exposure to 1,200 lx vs. 200 lx blue-enriched white light for 5 h, it is not possible to prevent a decline in subjective alertness over 5 h in the afternoon.
In addition to subjective alertness, the findings revealed that exposure to 5 h of 1,200 lx vs. 200 lx light exposure had no significant effect on the performance in terms of the overall response speed, or the fastest 10% or slowest 10% of reaction speeds in the PVT. The present results replicate certain previous findings (Huiberts et al., 2016; Maierova et al., 2016; Te Kulve et al., 2017; Smolders et al., 2018; Lok et al., 2019) while contradicting others (Phipps-Nelson et al., 2003; Smolders et al., 2012; Smolders and De Kort, 2014; Münch et al., 2016; Huiberts et al., 2017). These inconsistencies in the results of independent studies might reflect differences in methodology. For instance, the participants were sleep-restricted in the study by Phipps-Nelson et al. (2003), and the participants were subjected to mental fatigue before light manipulation in the study of Smolders and De Kort (2014). In contrast, the participants in the current study were all well-rested. Moreover, the variability in illumination parameters and exposure durations used in previous studies may partly explain the discrepancies. Münch et al. (2016) explored the influence of light of 750 lx vs. 40 lx at eye level for 3 h for 3 days, whereas exposure to 1,200 lx and 200 lx light for 5 h was adopted in the present study. The results of previous studies suggested a lasting and accumulative effect of daytime light on the later days (Gabel et al., 2015) or employing a relatively dimmer light (i.e., 40 lx Münch et al., 2016) as a control condition, although such a dim light level would not be typical for what office workers experienced in real-work situations. Besides, some previous studies have reported a time-of-day dependent alertness effect of bright light during the daytime (Smolders et al., 2012; Huiberts et al., 2017), with more pronounced effects on PVT performance in the morning than in the afternoon. However, whether the alertness effect of 5-h bright light exposure occurs for exposure in the morning remains unknown. Except for the insignificant differences in the PVT between the light conditions, the current findings revealed that the overall reaction speed in the PVT decreased at the end of the experimental session, which corresponded to an increasing trend in subjective sleepiness as indicated by the KSS scores. These effects might have occurred owing to an accumulated pressure for sleep as the waking time increased (Borbely, 1982; Borbely et al., 2016).
The theta, alpha, and beta bands were investigated as indicators of psychophysiological alertness (Santamaria and Chiappa, 1987; Aeschbach et al., 1999; Craig et al., 2012; Ishii et al., 2014). With this, the increased high-frequency spectral power (i.e., of the beta bands) and the decreased low-frequency spectral power (i.e., of the theta-alpha and theta bands) indicate higher physical alertness and arousal in the case of the former (Badia et al., 1991; Aeschbach et al., 1999; Strijkstra et al., 2003; Kaida et al., 2006a; Lockley et al., 2006; Phipps-Nelson et al., 2009; Cajochen et al., 2011; Craig et al., 2012; Rahman et al., 2014). The current results revealed that the ratio of the alpha band was significantly more for the 1,200 lx vs. 200 lx condition at O1, and the ratio of the beta band was significantly more for the 1,200 vs. 200 lx condition in all brain regions except the C4 channel. These findings indicated that exposure to 1,200 lx vs. 200 lx light moderated EEG activities somewhat differently across the spectral bands. Only three studies as reviewed by Lok et al. (2018a) assessed the effects of light exposure on the EEG-based beta activity during the daytime. None of these studies have reported a significant light-induced regularity effect on EEG activity (Badia et al., 1991; Sahin et al., 2014; Smolders et al., 2016). However, the comparative exposure levels increased both the relative beta1 and beta2 powers. In addition to the differences in the properties of the light, the multiple measurement strategy employed in this study may facilitate detection in view of the light-induced moderation of neurophysiological activity. However, the results reveal an insignificant effect of the light level on the power of theta, which is consistent with the results of certain previous studies (Badia et al., 1991; Akerstedt et al., 2003; Smolders et al., 2016) while contradicting the findings of others (Kaida et al., 2006a; Sahin et al., 2014). As mentioned above, the difference in the properties of light makes it difficult to directly compare present findings with those of independent studies. For instance, natural light (Kaida et al., 2006b) or dim light (<5 lx) was used as the control condition employed in the study of Sahin et al. (2014), while LED light (200 lx) was used as the control light condition in the present study. Most previous studies have not examined the delta band or reported the moderation of delta activity by light (Badia et al., 1991; Akerstedt et al., 2003; Sahin et al., 2014; Smolders et al., 2016) which has been documented mainly as an indicator of homeostatic sleep pressure. In the current study, the EEG delta activity decreased for 1,200 vs. 200 lx light in the afternoon. One earlier study also reported that daytime bright light (1,700 lx vs. 450 lx at the desk) during the daytime decreased the delta activity. It was speculated by Küller and Wetterberg (1993) that bright light might cause an alertness effect on the central nervous system. Iskra-Golec et al. (2017) investigated the effect of blue light on EEG activity in the daytime and found that the delta band decreased under blue light; it was concluded that decreases in the delta wave were correlated negatively with subjective sleepiness and fatigue (Lal and Craig, 2002).
The moderating effect of light on neural activity in different regions of the brain was examined. The results revealed that the delta band was lower for 1,200 lx light compared with 200 lx light for all channels except C4 (p = 0.085) and O1 (p = 0.437), indicating that the bright light-induced moderation of the delta band was subtly affected by these regions. A similar situation was observed for the beta1 and beta2 bands, with increased beta in the 1,200 lx vs. 200 lx condition for all channels except beta1 at C4 (p = 0.066). However, the relative power density of the theta band remained unaffected by the light condition for all channels. For the alpha band, the findings showed that the 1,200 lx vs. 200 lx condition elicited a higher density for the alpha band but only in the occipital region, which is consistent in part with the previous studies (Badia et al., 1991; Akerstedt et al., 2003; Smolders et al., 2016) while at the same time contradicting other research (Kaida et al., 2006b; Sahin et al., 2014). One factor which might contribute to these discrepancies is the differential analysis of the strategies considered. For example, the power density of a single channel (Kaida et al., 2006b; Sahin et al., 2014) or the average power density of multiple channels (Sahin et al., 2014; Smolders et al., 2016) were used as the dependent variable for EEG activity, which limited the ability to detect regional differences between the light conditions. Together, these findings suggest that bright light vs. the regular office light elicits a localization- and spectrum-dependent moderation of neurophysiological activities in the brain.
The current findings, however, did not reveal a consistent pattern that emerged between the effect of bright light on the subjective, objective, and physiological alertness. Both the subjective alertness and the vigilance performance were shown to decrease over time regardless of the light condition, however, the physiological alertness, as assessed by using the EEG power, was significantly influenced by the light condition. These findings suggest that the EEG activity might be more sensitive to the bright light induced alertness response during the daytime compared with the subjective ratings and task performance. These findings were partly in line with previous studies concerned with assessing the alertness effects of bright vs. dim light using multiple measures of alertness as described by Te Kulve et al. (2017). Nevertheless, the differences in exposure duration and time of day made it difficult to directly compare the findings of previous studies and present research. Previous studies suggested that the consistency of subjective and objective indicators would be affected by the degree of sleep deprivation, the experimental design (within or between subjects), and statistical approaches taken to compare associations across time (Zhou et al., 2012; Mulhall et al., 2019; Manousakis et al., 2021). Thus, for the moment, we can suggest that multiple measures of alertness are necessary for assessing the alertness effect of daytime light in other experimental scenarios (e.g., in the morning or the entire day) in the future.
The results of this study should be treated with some caution owing to several limitations. First, the early evening period (18:00 pm–19:00 pm) was included in the experimental session, where such endogenous factors as hormonal melatonin, although not measured here, should not be neglected. Second, the time-of-day effects of bright vs. dim light during daytime have been reported in several previous studies (Ruger et al., 2006; Smolders et al., 2012; Huiberts et al., 2015, 2017). This study was conducted only in the afternoon; hence the findings may not be transferrable to other times of the day. Third, some variables were not well controlled in this study, such as the light exposure history and sleep quality of the participants before commencing the laboratory study. Although the participants were asked to maintain a regular sleep routine and keep away from sunlight as much as possible before the test, the contribution of these additional variables cannot be ruled out. Fourth, lack of control of food intake at lunch on the experiment days leads to another limitation, which may affect the participant’s state of alertness in the afternoon. Last, the tasks used in the current study were rather monotonous, repetitive, and the lack of activities during the light intervention made it difficult for participants to stay awake. Such a situation would not reflect the activities of an actual workday for the participants. More experimental work is needed to evaluate the generalization of current findings.
Conclusion
The results of the current study revealed that, in general, prolonged exposure to bright light in the afternoon moderates the physiological level of arousal as measured by EEG activity; however, no statistically significant change in subjective ratings and performance measures of alertness were observed. Exposure to a blue-enriched white light (1,200 lx, 6,500 K) vs. regular office light (200 lx, 6,500 K) significantly lowered the relative delta power and increased the relative beta power. These findings suggest that exposure to bright light for 5 h in the afternoon compared with regular office light level enhances physiological arousal levels in terms of the delta power and beta power but at the same time exerts no influence on subjective alertness or performance with respect to vigilance.
Data Availability Statement
The original contributions presented in the study are included in the article/supplementary material, further inquiries can be directed to the corresponding author/s.
Ethics Statement
The studies involving human participants were reviewed and approved by the Ethical Committee of National Chengchi University. The patients/participants provided their written informed consent to participate in this study.
Author Contributions
XL: conceptualization, methodology, software, investigation, formal analysis, writing and original draft preparation, writing, reviewing, and editing. TR: supervision, conceptualization, methodology, validation, writing, reviewing, and editing. QC: data curation and visualization. F-CH and C-SH: subjects recruitment and clinical assessment. C-MY: conceptualization and methodology. GZ: project administration and funding acquisition. All authors reviewed the manuscript.
Funding
This work was supported by the National Key Research and Development Program of China (No. 2016YFB0401202), Program for Chang Jiang Scholars and Innovative Research Teams in Universities (No. IRT_17R40), Science and Technology Program of Guangzhou (No. 2019050001), Guangdong Provincial Key Laboratory of Optical Information Materials and Technology (Grant No. 2017B030301007), the National Center for International Research on Green Optoelectronics, MOE International Laboratory for Optical Information Technologies and the 111 Project, a program co-financed by Philips Lighting.
Conflict of Interest
The authors declare that the research was conducted in the absence of any commercial or financial relationships that could be construed as a potential conflict of interest.
Publisher’s Note
All claims expressed in this article are solely those of the authors and do not necessarily represent those of their affiliated organizations, or those of the publisher, the editors and the reviewers. Any product that may be evaluated in this article, or claim that may be made by its manufacturer, is not guaranteed or endorsed by the publisher.
References
Aeschbach, D., Matthews, J. R., Postolache, T. T., Jackson, M. A., Giesen, H. A., and Wehr, T. A. (1999). Two circadian rhythms in the human electroencephalogram during wakefulness. Am. J. Physiol. 277, R1771–R1779.
Akerstedt, T., and Gillberg, M. (1990). Subjective and objective sleepiness in the active individual. Int. J. Neurosci. 52, 29–37.
Akerstedt, T., Hallvig, D., and Kecklund, G. (2017). Normative data on the diurnal pattern of the Karolinska Sleepiness Scale ratings and its relation to age, sex, work, stress, sleep quality and sickness absence/illness in a large sample of daytime workers. J. Sleep Res. 26, 559–566. doi: 10.1111/jsr.12528
Akerstedt, T., Landstrom, U., Bystrom, M., Nordstrom, B., and Wibom, R. (2003). Bright light as a sleepiness prophylactic: a laboratory study of subjective ratings and EEG. Perceptual Motor Skills 97, 811–819. doi: 10.2466/pms.2003.97.3.811
Badia, P., Myers, B., Boecker, M., Culpepper, J., and Harsh, J. R. (1991). Bright light effects on body temperature, alertness, EEG and behavior. Physiol. Behav. 50, 583–588. doi: 10.1016/0031-9384(91)90549-4
Bedrosian, T. A., and Nelson, R. J. (2013). Influence of the modern light environment on mood. Mol. Psychiatry 18, 751–757. doi: 10.1038/mp.2013.70
Bedrosian, T. A., and Nelson, R. J. (2017). Timing of light exposure affects mood and brain circuits. Transl. Psychiatry 7:e1017. doi: 10.1038/tp.2016.262
Berson, D. M., Dunn, F. A., and Takao, M. (2002). Phototransduction by retinal ganglion cells that set the circadian clock. Science 295, 1070–1073. doi: 10.1126/science.1067262
Besserve, M., Jerbi, K., Laurent, F., Baillet, S., Martinerie, J., and Garnero, L. (2007). Classification methods for ongoing EEG and MEG signals. Biol. Res. 40, 415–437.
Borbely, A. A., Daan, S., Wirz-Justice, A., and Deboer, T. (2016). The two-process model of sleep regulation: a reappraisal. J. Sleep Res. 25, 131–143.
Boubekri, M., Lee, J., Macnaughton, P., Woo, M., Schuyler, L., Tinianov, B., et al. (2020). The impact of optimized daylight and views on the sleep duration and cognitive performance of office workers. Int. J. Environ. Res. Public Health 17:3219. doi: 10.3390/ijerph17093219
Cajochen, C., Frey, S., Anders, D., Spati, J., Bues, M., Pross, A., et al. (2011). Evening exposure to a light-emitting diodes (LED)-backlit computer screen affects circadian physiology and cognitive performance. J. Appl. Physiol. 110, 1432–1438. doi: 10.1152/japplphysiol.00165.2011
Cajochen, C., Knoblauch, V., Krauchi, K., Renz, C., and Wirz-Justice, A. (2001). Dynamics of frontal EEG activity, sleepiness and body temperature under high and low sleep pressure. Neuroreport 12, 2277–2281. doi: 10.1097/00001756-200107200-00046
Cajochen, C., Munch, M., Kobialka, S., Krauchi, K., Steiner, R., Oelhafen, P., et al. (2005). High sensitivity of human melatonin, alertness, thermoregulation, and heart rate to short wavelength light. J. Clin. Endocrinol. Metab. 90, 1311–1316. doi: 10.1210/jc.2004-0957
Cajochen, C., Zeitzer, J. M., Czeisler, C. A., and Dijk, D. J. (2000). Dose-response relationship for light intensity and ocular and electroencephalographic correlates of human alertness. Behav. Brain Res. 115, 75–83. doi: 10.1016/s0166-4328(00)00236-9
Craig, A., Tran, Y., Wijesuriya, N., and Nguyen, H. (2012). Regional brain wave activity changes associated with fatigue. Psychophysiology 49, 574–582. doi: 10.1111/j.1469-8986.2011.01329.x
De Vries, A., Souman, J. L., and De Kort, Y. A. W. (2020). Teasing apart office illumination: isolating the effects of task illuminance on office workers. Light. Res. Technol. 52, 944–958.
Dijk, D. J., Duffy, J. F., and Czeisler, C. A. (1992). Circadian and sleep/wake dependent aspects of subjective alertness and cognitive performance. J. Sleep Res. 1, 112–117. doi: 10.1111/j.1365-2869.1992.tb00021.x
Dinges, D. F., and Powell, J. W. (1985). Microcomputer analyses of performance on a portable, simple visual RT task during sustained operations. Behav. Res. Methods Instruments Comput. 17, 652–655. doi: 10.3758/bf03200977
Figueiro, M. G., Nagare, R., and Price, L. (2018). Non-visual effects of light: how to use light to promote circadian entrainment and elicit alertness. Light. Res. Technol. 50, 38–62. doi: 10.1177/1477153517721598
Figueiro, M. G., Steverson, B., Heerwagen, J., Kampschroer, K., Hunter, C. M., Gonzales, K., et al. (2017). The impact of daytime light exposures on sleep and mood in office workers. Sleep Health 3, 204–215. doi: 10.1016/j.sleh.2017.03.005
Fisk, A. S., Tam, S. K. E., Brown, L. A., Vyazovskiy, V. V., Bannerman, D. M., and Peirson, S. N. (2018). Light and cognition: roles for circadian rhythms, sleep and arousal. Front. Neurol. 9:56. doi: 10.3389/fneur.2018.00056
Gabel, V., Maire, M., Reichert, C. F., Chellappa, S. L., Schmidt, C., Hommes, V., et al. (2015). Dawn simulation light impacts on different cognitive domains under sleep restriction. Behav. Brain Res. 281, 258–266. doi: 10.1016/j.bbr.2014.12.043
Hattar, S., Liao, H. W., Takao, M., Berson, D. M., and Yau, K. W. (2002). Melanopsin-containing retinal ganglion cells: architecture, projections, and intrinsic photosensitivity. Science 295, 1065–1070. doi: 10.1126/science.1069609
Hosmer, D. W., and Lemeshow, S. (2013). Applied Logistic Regression. New York, NY: John Wiley & Sons.
Hsiao, F. C., Tsai, P. J., Wu, C. W., Yang, C. M., Lane, T. J., Lee, H. C., et al. (2018). The neurophysiological basis of the discrepancy between objective and subjective sleep during the sleep onset period: an EEG-fMRI study. Sleep 41:zsy056. doi: 10.1093/sleep/zsy056
Huiberts, L. M., Smolders, K., and De Kort, Y. A. W. (2017). Seasonal and time-of-day variations in acute non-image forming effects of illuminance level on performance, physiology, and subjective well-being. Chronobiol. Int. 34, 827–844. doi: 10.1080/07420528.2017.1324471
Huiberts, L. M., Smolders, K. C., and De Kort, Y. A. (2015). Shining light on memory: effects of bright light on working memory performance. Behav. Brain Res. 294, 234–245. doi: 10.1016/j.bbr.2015.07.045
Huiberts, L. M., Smolders, K. C., and De Kort, Y. A. (2016). Non-image forming effects of illuminance level: exploring parallel effects on physiological arousal and task performance. Physiol. Behav. 164, 129–139. doi: 10.1016/j.physbeh.2016.05.035
Hull, J. T., Wright, K. P. Jr., and Czeisler, C. A. (2003). The influence of subjective alertness and motivation on human performance independent of circadian and homeostatic regulation. J. Biol. Rhythms 18, 329–338. doi: 10.1177/0748730403253584
Ishii, A., Tanaka, M., and Watanabe, Y. (2014). Neural mechanisms of mental fatigue. Rev. Neurosci. 25, 469–479.
Iskra-Golec, I., Golonka, K., Wyczesany, M., Smith, L., Siemiginowska, P., and Watroba, J. (2017). Daytime effect of monochromatic blue light on EEG activity depends on duration and timing of exposure in young men. Adv. Cogn. Psychol. 13, 241–247. doi: 10.5709/acp-0224-0
Iskra-Golec, I., and Smith, L. (2008). Daytime intermittent bright light effects on processing of laterally exposed stimuli, mood, and light perception. Chronobiol. Int. 25, 471–479. doi: 10.1080/07420520802118103
Kaida, K., Takahashi, M., Akerstedt, T., Nakata, A., Otsuka, Y., Haratani, T., et al. (2006a). Validation of the Karolinska sleepiness scale against performance and EEG variables. Clin. Neurophysiol. 117, 1574–1581. doi: 10.1016/j.clinph.2006.03.011
Kaida, K., Takahashi, M., Haratani, T., Otsuka, Y., Fukasawa, K., and Nakata, A. (2006b). Indoor exposure to natural bright light prevents afternoon sleepiness. Sleep 29, 462–469. doi: 10.1093/sleep/29.4.462
Khademagha, P., Aries, M. B. C., Rosemann, A. L. P., and Van Loenen, E. J. (2016). Implementing non-image-forming effects of light in the built environment: a review on what we need. Build. Environ. 108, 263–272.
Küller, R., and Wetterberg, L. (1993). Melatonin, cortisol, EEG, ECG and subjective comfort in healthy humans: impact of two fluorescent lamp types at two light intensities. Light. Res. Technol. 25, 71–80. doi: 10.1177/096032719302500203
Lal, S. K., and Craig, A. (2002). Driver fatigue: electroencephalography and psychological assessment. Psychophysiology 39, 313–321.
Lasauskaite, R., Hazelhoff, E. M., and Cajochen, C. (2018). Four minutes might not be enough for light colour temperature to affect sleepiness, mental effort, and light ratings. Light. Res. Technol. 51, 1128–1138.
LeGates, T. A., Fernandez, D. C., and Hattar, S. (2014). Light as a central modulator of circadian rhythms, sleep and affect. Nat. Rev. Neurosci. 15, 443–454.
Leichtfried, V., Mair-Raggautz, M., Schaeffer, V., Hammerer-Lercher, A., Mair, G., Bartenbach, C., et al. (2015). Intense illumination in the morning hours improved mood and alertness but not mental performance. Appl. Ergon. 46(Pt A) 54–59. doi: 10.1016/j.apergo.2014.07.001
Lockley, S. W., Evans, E. E., Scheer, F. A., Brainard, G. C., Czeisler, C. A., and Aeschbach, D. (2006). Short-wavelength sensitivity for the direct effects of light on alertness, vigilance, and the waking electroencephalogram in humans. Sleep 29, 161–168.
Lok, R., Smolders, K., Beersma, D. G. M., and De Kort, Y. A. W. (2018a). Light, alertness, and alerting effects of white light: a literature overview. J. Biol. Rhythms 33, 589–601. doi: 10.1177/0748730418796443
Lok, R., Van Koningsveld, M. J., Gordijn, M. C. M., Beersma, D. G. M., and Hut, R. A. (2019). Daytime melatonin and light independently affect human alertness and body temperature. J. Pineal Res. 67:e12583. doi: 10.1111/jpi.12583
Lok, R., Woelders, T., Gordijn, M. C. M., Hut, R. A., and Beersma, D. G. M. (2018b). White light during daytime does not improve alertness in well-rested individuals. J. Biol. Rhythms 33, 637–648.
Lucas, R. J., Peirson, S. N., Berson, D. M., Brown, T. M., Cooper, H. M., Czeisler, C. A., et al. (2014). Measuring and using light in the melanopsin age. Trends Neurosci. 37, 1–9. doi: 10.1016/j.tins.2013.10.004
Maierova, L., Borisuit, A., and Scartezzini, J. L. (2016). Diurnal variations of hormonal secretion, alertness and cognition in extreme chronotypes under different lighting conditions. Sci. Rep. 6:33591. doi: 10.1038/srep33591
Makeig, S., Jung, T. P., and Sejnowski, T. J. (2000). Awareness during drowsiness: dynamics and electrophysiological correlates. Can. J. Exp. Psychol. 54, 266–273. doi: 10.1037/h0087346
Manousakis, J. E., Mann, N., Jeppe, K. J., and Anderson, C. (2021). Awareness of sleepiness: temporal dynamics of subjective and objective sleepiness. Psychophysiology 58:e13839. doi: 10.1111/psyp.13839
Min, B.-K., Jung, Y.-C., Kim, E., and Park, J. Y. (2013). Bright illumination reduces parietal EEG alpha activity during a sustained attention task. Brain Res. 1538, 83–92. doi: 10.1016/j.brainres.2013.09.031
Mulhall, M. D., Sletten, T. L., Magee, M., Stone, J. E., Ganesan, S., Collins, A., et al. (2019). Sleepiness and driving events in shift workers: the impact of circadian and homeostatic factors. Sleep 42:zsz074. doi: 10.1093/sleep/zsz074
Münch, M., Nowozin, C., Regente, J., Bes, F., De Zeeuw, J., Hädel, S., et al. (2016). Blue-Enriched morning light as a countermeasure to light at the wrong time: effects on cognition, sleepiness, sleep, and circadian phase. Neuropsychobiology 74, 207–218. doi: 10.1159/000477093
Pachito, D. V., Eckeli, A. L., Desouky, A. S., Corbett, M. A., Partonen, T., Rajaratnam, S. M., et al. (2018). Workplace lighting for improving alertness and mood in daytime workers. Cochrane Database Syst. Rev. 3:CD012243. doi: 10.1002/14651858.CD012243.pub2
Park, J. Y., Min, B. K., Jung, Y. C., Pak, H., Jeong, Y. H., and Kim, E. (2013). Illumination influences working memory: an EEG study. Neuroscience 247, 386–394. doi: 10.1016/j.neuroscience.2013.05.016
Peeters, S. T., Smolders, K. C. H. J., Vogels, I. M. L. C., and De Kort, Y. A. W. (2021). Less is more? Effects of more vs. less electric light on alertness, mood, sleep and appraisals of light in an operational office. J. Environ. Psychol. 74:101583. doi: 10.1016/j.jenvp.2021.101583
Phipps-Nelson, J., Redman, J. R., Dijk, D. J., and Rajaratnam, S. M. W. (2003). Daytime exposure to bright light, as compared to dim light, decreases sleepiness and improves psychomotor vigilance performance. Sleep 26, 695–700. doi: 10.1093/sleep/26.6.695
Phipps-Nelson, J., Redman, J. R., Schlangen, L. J., and Rajaratnam, S. M. (2009). Blue light exposure reduces objective measures of sleepiness during prolonged nighttime performance testing. Chronobiol. Int. 26, 891–912. doi: 10.1080/07420520903044364
Prayag, A. S., Jost, S., Avouac, P., Dumortier, D., and Gronfier, C. (2019a). Dynamics of non-visual responses in humans: as fast as lightning? Front. Neurosci. 13:126. doi: 10.3389/fnins.2019.00126
Prayag, A. S., Münch, M., Aeschbach, D., Chellappa, S., and Gronfier, C. (2019b). Light modulation of human clocks, wake, and sleep. Clocks Sleep 1, 193–208. doi: 10.3390/clockssleep1010017
Rahman, S. A., Flynn-Evans, E. E., Aeschbach, D., Brainard, G. C., Czeisler, C. A., and Lockley, S. W. (2014). Diurnal spectral sensitivity of the acute alerting effects of light. Sleep 37, 271–281. doi: 10.5665/sleep.3396
Roenneberg, T., Wirz-Justice, A., and Merrow, M. (2003). Life between clocks: daily temporal patterns of human chronotypes. J. Biol. Rhythms 18, 80–90. doi: 10.1177/0748730402239679
Ru, T., Smolders, K., Chen, Q., Zhou, G., and De Kort, Y. A. W. (2021). Diurnal effects of illuminance on performance: exploring the moderating role of cognitive domain and task difficulty. Light. Res. Technol. (in press). doi: 10.1177/1477153521990645
Ru, T. T., De Kort, Y. A. W., Smolders, K. C. H. J., Chen, Q. W., and Zhou, G. F. (2019). Non-image forming effects of illuminance and correlated color temperature of office light on alertness, mood, and performance across cognitive domains. Build. Environ. 149, 253–263. doi: 10.1016/j.buildenv.2018.12.002
Ruger, M., Gordijn, M. C., Beersma, D. G., De Vries, B., and Daan, S. (2006). Time-of-day-dependent effects of bright light exposure on human psychophysiology: comparison of daytime and nighttime exposure. Am. J. Physiol. Regul. Integr. Comp. Physiol. 290, R1413–R1420. doi: 10.1152/ajpregu.00121.2005
Sahin, L., Wood, B. M., Plitnick, B., and Figueiro, M. G. (2014). Daytime light exposure: effects on biomarkers, measures of alertness, and performance. Behav. Brain Res. 274, 176–185. doi: 10.1016/j.bbr.2014.08.017
Santamaria, J., and Chiappa, K. H. (1987). The EEG of drowsiness in normal adults. J. Clin. Neurophysiol. 4, 327–382. doi: 10.1097/00004691-198710000-00002
Sithravel, R., and Ibrahim, R. (2021). Identifying supportive daytime lighting characteristics for enhancing individuals’ psychophysiological wellbeing in windowless workplace in tropical Malaysia. Indoor Built Environ. 30, 298–312. doi: 10.1177/1420326x19889656
Smolders, K., Peeters, S. T., Vogels, I., and De Kort, Y. A. W. (2018). Investigation of dose-response relationships for effects of white light exposure on correlates of alertness and executive control during regular daytime working hours. J. Biol. Rhythms 33, 649–661. doi: 10.1177/0748730418796438
Smolders, K. C. H. J., and De Kort, Y. A. W. (2014). Bright light and mental fatigue: effects on alertness, vitality, performance and physiological arousal. J. Environ. Psychol. 39, 77–91. doi: 10.1016/j.jenvp.2013.12.010
Smolders, K. C. H. J., De Kort, Y. A. W., and Cluitmans, P. J. M. (2016). Higher light intensity induces modulations in brain activity even during regular daytime working hours. Light. Res. Technol. 48, 433–448. doi: 10.1177/1477153515576399
Smolders, K. C. H. J., Kort, D., and Cluitmans, P. J. M. (2012). A higher illuminance induces alertness even during office hours: findings on subjective measures, task performance and heart rate measures. Physiol. Behav. 107, 7–16. doi: 10.1016/j.physbeh.2012.04.028
Souman, J. L., Tinga, A. M., Te Pas, S. F., Van Ee, R., and Vlaskamp, B. N. S. (2018). Acute alerting effects of light: a systematic literature review. Behav. Brain Res. 337, 228–239. doi: 10.1016/j.bbr.2017.09.016
Spitschan, M., Stefani, O., Blattner, P., Gronfier, C., Lockley, S. W., and Lucas, R. J. (2019). How to report light exposure in human chronobiology and sleep research experiments. Clocks Sleep 1, 280–289. doi: 10.3390/clockssleep1030024
Stefani, O., Freyburger, M., Veitz, S., Basishvili, T., Meyer, M., Weibel, J., et al. (2021). Changing color and intensity of LED lighting across the day impacts on circadian melatonin rhythms and sleep in healthy men. J. Pineal Res. 70:e12714. doi: 10.1111/jpi.12714
Strijkstra, A. M., Beersma, D. G., Drayer, B., Halbesma, N., and Daan, S. (2003). Subjective sleepiness correlates negatively with global alpha (8-12 Hz) and positively with central frontal theta (4-8 Hz) frequencies in the human resting awake electroencephalogram. Neurosci. Lett. 340, 17–20. doi: 10.1016/s0304-3940(03)00033-8
Tahkamo, L., Partonen, T., and Pesonen, A. K. (2019). Systematic review of light exposure impact on human circadian rhythm. Chronobiol. Int. 36, 151–170. doi: 10.1080/07420528.2018.1527773
Te Kulve, M., Schlangen, L. J. M., Schellen, L., Frijns, A. J. H., and Van Marken Lichtenbelt, W. D. (2017). The impact of morning light intensity and environmental temperature on body temperatures and alertness. Physiol. Behav. 175, 72–81. doi: 10.1016/j.physbeh.2017.03.043
Vandewalle, G., Balteau, E., Phillips, C., Degueldre, C., Moreau, V., Sterpenich, V., et al. (2006). Daytime light exposure dynamically enhances brain responses. Curr. Biol. 16, 1616–1621. doi: 10.1016/j.cub.2006.06.031
Vandewalle, G., Maquet, P., and Dijk, D. J. (2009). Light as a modulator of cognitive brain function. Trends Cogn. Sci. 13, 429–438. doi: 10.1016/j.tics.2009.07.004
Weisgerber, D. M., Nikol, M., and Mistlberger, R. E. (2017). Driving home from the night shift: a bright light intervention study. Sleep Med. 30, 171–179. doi: 10.1016/j.sleep.2016.09.010
Xu, Q., and Lang, C. P. (2018). Revisiting the alerting effect of light: a systematic review. Sleep Med. Rev. 41, 39–49. doi: 10.1016/j.smrv.2017.12.001
Keywords: light, illumination, alertness, light exposure, electroencephalogram
Citation: Luo X, Ru T, Chen Q, Hsiao F-C, Hung C-S, Yang C-M and Zhou G (2021) Temporal Dynamics of Subjective and Objective Alertness During Exposure to Bright Light in the Afternoon for 5 h. Front. Physiol. 12:771605. doi: 10.3389/fphys.2021.771605
Received: 08 September 2021; Accepted: 08 November 2021;
Published: 07 December 2021.
Edited by:
Xi Long, Eindhoven University of Technology, NetherlandsReviewed by:
Arcady A. Putilov, Federal Research Center of Fundamental and Translational Medicine, RussiaClaudia Roberta de Castro Moreno, University of São Paulo, Brazil
Copyright © 2021 Luo, Ru, Chen, Hsiao, Hung, Yang and Zhou. This is an open-access article distributed under the terms of the Creative Commons Attribution License (CC BY). The use, distribution or reproduction in other forums is permitted, provided the original author(s) and the copyright owner(s) are credited and that the original publication in this journal is cited, in accordance with accepted academic practice. No use, distribution or reproduction is permitted which does not comply with these terms.
*Correspondence: Taotao Ru, dGFvdGFvLnJ1QG0uc2NudS5lZHUuY24=