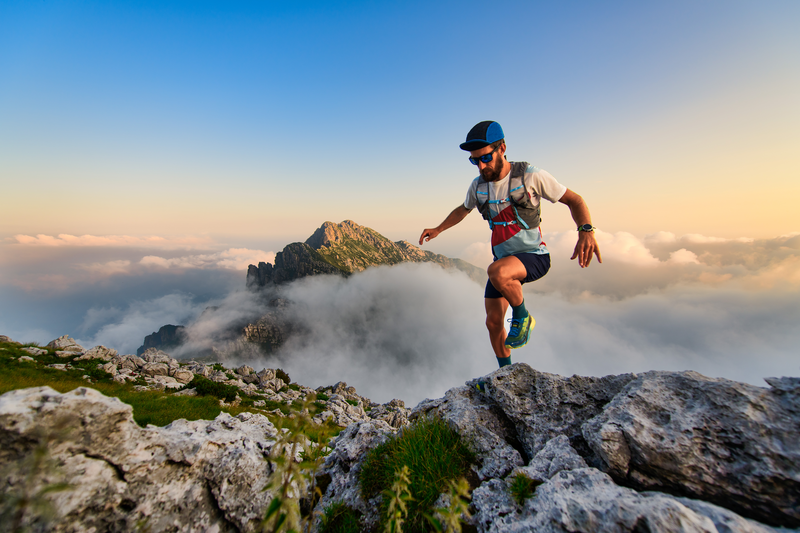
95% of researchers rate our articles as excellent or good
Learn more about the work of our research integrity team to safeguard the quality of each article we publish.
Find out more
ORIGINAL RESEARCH article
Front. Physiol. , 11 November 2021
Sec. Aquatic Physiology
Volume 12 - 2021 | https://doi.org/10.3389/fphys.2021.768122
This article is part of the Research Topic Homeostasis and Physiological Regulation in the Aquatic Animal during Osmotic Stress View all 7 articles
The local gill production of corticotropin releasing hormone (crh) and crh-receptor (crhr) is hypothesized to play important roles during seawater (SW) and freshwater (FW) acclimation in euryhaline black porgy (Acanthopagrus schlegelii). The mRNA expression of crh, crhr, and Na+/K+-ATPase (a-nka) was examined in SW and FW diencephalon (Dien) and in the gills at different exposure time by Q-PCR analysis. The in situ hybridization results indicate that crh mRNA hybridization signals were more abundant in FW fish in the gigantocellular (PMgc) and parvocellular (PMpc) part of the magnocellular preoptic nucleus versus SW fish. The crh and crhr-expressing cells were located in basal cells of gill filament. Furthermore, in vitro dexamethasone (DEX) treatment could increase the crh-system in the gill. Increased transcripts of the crh-system in the gill via in vitro and in vivo CRH treatments suggest that CRH may regulate the system in a local manner. The a-Nka cells were localized in the filament and secondary lamellae mitochondria rich cells (MRCs) of FW fish at 8 h and 1 day. a-Nka cells were seen in both filament and lamellae in the FW but much less in SW fish indicating that gills play key roles in black porgy osmoregulation. Gill crh and crhr play important roles in the response to salinity stress.
Stress stimulates the corticotrophin releasing hormone (crh) and crh-receptor (crhr) from the hypothalamus and pituitary by activation of the hypothalamic pituitary interrenal (HPI/fish) and/or hypothalamic pituitary adrenal (HPA/mammals)-axis (Dautzenberg and Hauger, 2002). In vertebrates, crh is the dominant hypothalamic neuropeptide controlling the HPI-axis (Vale et al., 1981; Rivier and Vale, 1983). Stress acts in the nervous system and converges at the hypothalamus, and the final products are corticosteroid hormones, particularly, cortisol, which is believed to regulate homeostasis. Furthermore, this same corticosteroid terminates the stress response by interacting directly with the hypothalamus or anterior pituitary to attenuate crh and crhr production (Slominski et al., 2000).
In mammals, the skin is a barrier between the external environment and the internal milieu and plays a critical role in maintaining internal homeostasis. Slominski and Wortsman (2000) suggest that the skin has a high sensory capability for stress stimuli and is tightly coupled to a local response system. During stress, the mammalian skin expressed crh and crhr and is highly reactive to common stressors such as immune cytokines, ultraviolet radiation, cutaneous pathology, or even the physiological changes associated with the hair cycle phase (Slominski et al., 2001). However, the body surfaces of teleosts are continuously exposed to an aquatic environment and are in intimately physiological contact with fish via the body fluid compartments and the epithelium of the gill, kidney, and intestine (Flik et al., 2006). Physiological processes, such as gas exchange, osmoregulation, excretion of nitrogenous waste products and acid-base balance, take place in the osmoregulatory organs (Evans et al., 2005). Thus, damage to these organs could result in the compromise of fish survival.
The gill is the only organ that is diffused by the entire cardiac output and has an extensive vascular surface area in contact with the plasma (Olson, 1998). The gills also play a dominant role in endocrine regulation. They are an endocrine target and are metabolically active tissue (Evans et al., 2005). The teleost gill possesses two morphologically distinct epithelia. One is a multilayered filament epithelium with pavement, mucous, and mitochondria rich cells (MRCs) which is largely involved in ion exchange. Another is a bi-layered lamellar epithelium made of undifferentiated, myoepithelial-like, granular, and neuroendocrine cells involved in gas exchange between the blood and the ambient water (Li et al., 1995). Versus terrestrial vertebrates, aquatic fish face a more challenging task to achieve an internal homeostasis due to the presence of ionic and/or osmotic gradients that are hostile to the body fluids of fish (Kaneko et al., 2002; Kaneko and Hiroi, 2008).
Black porgy, Acanthopagrus schlegelii, is an euryhaline teleost that maintains ion and water balances in a wide variety of environmental salinity via osmoregulatory mechanisms (Tomy et al., 2009). Thus, in fish, the gill is an important osmoregulatory organ that creates ionic and osmotic gradients between the body fluid and external environments (Marshall and Grosell, 2006). In the gill, MRCs or chloride cells present in gill epithelia are a major site of ion secretion and absorption. They are important in SW and FW adaptation, respectively (Kaneko et al., 2008). The chloride cells or MRCs are structurally characterized by many mitochondria including an abundant tubular system where ion-transporting enzymes such as Na+/K+-ATPase, Ca2+-ATPase, and Ca2+/Na+ exchanger is located (Wendelaar Bonga et al., 1990; Flik and Verbost, 1993; Marshall, 2002). The MRCs are the site of the active Ca2+ transport that underlies transepithelial Ca2+ uptake in both freshwater (FW) and seawater (SW) fish (Marshall et al., 1992; McCormick et al., 1992; Verbost et al., 1994).
Here, we hypothesize that the gill is an essential vital organ that directly faces the exterior and has an endocrine-like function to acclimate and adapt to environmental changes. To maintain homeostasis, a gill crh system (crh/crhr) may be activated by external stressors including in vivo and/or in vitro conditions. Locally produced crh and crhr in the black porgy gill can respond to ambient salinity stress versus the hypothalamic crh-system. Based on limited information available on the expression of crh and crhr in the gill of common carp, Cyprinus carpio, we study here the expression of crh, crhr, and Na+/K+-ATPase (a-nka) in the black porgy diencephalon (Dien) and gill by Q-PCR analysis (Mazon et al., 2006). This is also the first study to localize the transcripts of crh and crhr in SW and FW-acclimated black porgy gill at day 1 and day 30 by in situ hybridization. We further performed in vitro and in vivo dexamethasone (DEX) and CRH treatment to analyze the mRNA expression pattern of crh-system in Dien and gill. Immunohistochemical studies with a-Nka antibody have identified the a-Nka immunoreactivity (ir) cells in the gill at day 1 and day 30 during SW and FW acclimation.
Black porgy (6–7 months old, n = 190) (body weight = 17.69 ± 0.59 g, body length = 9.96 ± 0.37 cm) were procured from an aquaculture farm of southern Taiwan (Chiayi County) and allowed to acclimated for 1 month at the National Taiwan Ocean University aquarium in seawater (33 ppt) with a natural light system. The water temperature, pH and dissolved oxygen (DO) values of which ranged from 19 to 24°C, 8.11 to 8.18, and 7.7 to 7.8 mg/L, respectively. Seawater was obtained from the open sea and filtered through sand. Freshwater was obtained from the tap and was put in the tank for 1 week with aeration for dechlorination (pH and DO values were 7.91–8.08 and 7.8–8.0 mg/L, respectively). The fish tanks were aerated to maintain adequate dissolved oxygen. The water was continuously aerated through air stones. The level of the aeration was sufficient to sustain dissolved oxygen levels for all experiments. The sponge filters and gravel were used to filter the solids from fish waste and uneaten feeds. Fish were fed pelleted dry food ad libitum at a daily ration of 1% of their estimated body weight. For each experiment, the fish were anesthetized with 1% glycophenol monophenyl ether and decapitated. The diencephalon and gill samples were collected and snap frozen in liquid nitrogen at −80°C. All experiments were conducted in accordance with the principles and procedures approved by the Institutional Animal Care and Use Committee, National Taiwan Ocean University, Taiwan (# 99026).
To characterize the endocrine changes in osmoregulation in response to acute salinity stress, an experiment was conducted where fish were randomly divided into two groups and maintained in SW (33 ppt). After an initial acclimation period (30 days), fish were maintained in SW (n = 40) and transferred to FW (0 ppt). Respective control groups (n = 40) received the SW with no salinity changes. The diencephalon and gill samples (n = 10) were collected after 8 h, 1 day, 4 days, and 30 days of transfer.
The fish (n = 24) were cultured in 2,000 L tanks for 2 weeks. After an initial acclimation, the fish were randomly divided into three groups (n = 8 per group). The different doses of mammalian crh (Sigma, St. Louis, MO, United States) (10 and 40 μg/kg) were prepared by dissolving crh with phosphate buffered saline (PBS) (pH 7.4). Fish were injected intraperitoneally with crh twice on day 1 and day 4. Control fish were injected with coconut oil and treated as a control. We collected the samples on day 5.
The fish (n = 64) were cultured in 2,000 L tanks for 2 weeks. After an initial acclimation, the fish were randomly divided into eight groups (n = 8 per group). The serial dilutions of mammalian DEX (low 10–8 M, medium 10–6 M, and high 10–4 M) and crh (low 10–9, medium 10–7, and high 10–5 M) were prepared and the gill filaments were incubated for 2 and 4 days with the respective concentrations. A fish group was incubated with L-15 medium as a control. After in vitro culture, gill filaments were removed with forceps and then snap frozen in liquid nitrogen at −80°C until RNA isolation.
Primary gill filaments were excised from the arches and separated from one another. Five to six primary gill filaments were placed in each petri dish (n = 8 fish, each fish per petri dish) containing 1% penicillin-streptomycin in Leibovitz L-15 medium (Gibco, United States), this was incubated in ice for an hour. After incubation, the filaments were washed with 1X PBS to remove blood. The gill filaments were again rinsed with 5% fungizone in 1X PBS. Again, the filaments were rinsed with 5% penicillin-streptomycin in L-15 medium. Serial dilutions of DEX (10–8, 10–6, and 10–4 M) and CRH (10–9, 10–7, and 10–5 M) were prepared in L-15 medium. The incubation medium was removed and replaced with the serial dilutions of CRH, DEX, and/or vehicle in L-15 medium containing 50 units ml–1 penicillin and 50 μg ml–1 streptomycin equilibrated with a 99% oxygen and 1% carbon dioxide gas mixture. Gill filaments were incubated at 15°C for 48 h (day 2) and 96 h (day 4) in a humidified chamber with 99%:1% of O2:CO2 with gentle shaking. After culture, gill filaments were removed with forceps and then snap frozen in liquid nitrogen at −80°C until RNA isolation.
RNA was isolated from the Dien and gill by using TRIzol® reagent and reverse transcribed (Gibco BRL, Grand Island, NY, United States) according to the protocol of the manufacturer. The resulting cDNA was used as a template for the subsequent PCR amplification of the genes used here.
Genes involved in the stress response were cloned from cDNA of the black porgy gill. Multiple alignments of previously published sequence of the respective genes were constructed using CLUSTAL X program (version 1.81) to find the conserved region. Primers were designed from here (Table 1). PCR reactions were performed with 2.5 μl of 10X reaction buffer [200 mM Tris–HCl (pH 8.4), 500 mM KCl], 1 μl of 10 mM dNTP, 1 μl of 2 mM MgCl2, 0.5 μl each of 10 μM sense and antisense primers, 1 μl cDNA, and 0.2 μl superscript enzyme (Invitrogen, Carlsbad, CA, United States) in a final volume of 25 μl. The PCR conditions were set as follows: 94°C for 5 min, 94°C for 30 s, 50°C for 30 s, 72°C for 30 s for 35 cycles, and 72°C for 10 min. The PCR products were verified by electrophoresis on a 1.5% agarose gel and visualized using ethidium bromide staining DNA fragments were excised using a Gel-MTM Gel Extraction system Kit (Bio 101) (Viogene, La Jolla, CA, United States) and cloned into pGEM® – T Easy vector (Promega, Madison, WI, United States). Plasmids containing the insert were sequenced using a dye terminator cycle sequencing kit (Perkin Elmer, Foster City, CA, United States) and submitted to the Basic Local Alignment Search Tool (BLAST) for making comparison with the known sequences accessible in NCBI database.
Total RNA was extracted from the Dien and gill of black porgy (n = 3). The complementary DNA (cDNA) strand was synthesized with 1 μg of total RNA using SuperScript II with the oligo(dT)12–18 primers. Gene specific primers were employed for RT-PCR analysis (Table 1). The glyceraldehyde 3-phosphate dehydrogenase (gapdh) was used as an internal control. The gapdh transcripts did not significantly change between or among the treatments. The PCR conditions were as follows: 94°C for 1 min, 55°C for 1 min, and 72°C for 2 min for 30 cycles and the PCR products were separated on 1.5% agarose gel. Reactions in which reverse transcriptase was neglected were used as negative controls for SW and FW fish.
Q-PCR analysis was performed to analyze the gene expression of crh, crhr1, and α-nka in the Dien and gill during FW and SW acclimation with iQTM Multicolor Real Time-PCR Detection system (Bio-Rad). Primers were designed using primer expression software (Applied Biosystems, Foster City, CA, United States) (Table 1). Gene quantification of standards, samples, and controls was conducted simultaneously in a Q-PCR machine (iQTM Multicolor Real-Time PCR Detection System; Bio-Rad Co., Hercules, CA, United States) with iQTM SYBR green (Bio-Rad) as a dsDNA minor-groove binding agent, forward and reverse primers, and water according to our previous study (Aruna et al., 2012a). Calculation of PCR efficiency was based on the slope of the relationship between log input cDNA (transcript concentrations) vs. cycle threshold (Ct). We used an efficiency-corrected method to calculate the relative expression level of crh, crhr, and a-nka in the hypothalamus and the gill. The correlation of the standard curve was −0.999. The gapdh was used as a control gene to calibrate the mRNA expression level, because there were no significant changes in the expression levels between all controls and experimental groups.
The in situ hybridization was carried out to localize the crh and crhr transcripts in the hypothalamus and gill of black porgy. The tissues were fixed in a 4% paraformaldehyde in phosphate-buffered saline (PBS) at 4°C overnight and embedded in paraffin. Paraffin sections (5 μm) were collected on TESPA-treated slides (3-aminopropyltriethoxysilane, Sigma). For in situ hybridization, the digoxigenin (DIG)-labeled sense and anti-sense RNA probes of crh and crhr (primers in Table 1) were prepared in black porgy using T7 and Sp6 polymerase (Promega, Madison, WI, United States) on linear plasmid DNA containing respective inserts of the genes in the pGEM-T Easy vector in accordance with the methods described previously (Aruna et al., 2012a). The resulting PCR products purified (Viogene, Bio 101, La Jolla, CA, United States) and the quantity of the PCR amplification product was measured via spectrophotometry at 260 nm. The RNA probe quality was checked by spectrophotometry at 260 nm. The preparation of RNA probe by PCR amplification was described previously (Thisse and Thisse, 2008).
The serial sections proceeded to rehydration, prehybridization, and hybridization according to our previous study (Aruna et al., 2012a). The sections were incubated with an alkaline phosphatase-conjugated sheep anti-digoxigenin antibody (Roche, Penzberg, Germany) (dilution 1:2,000 in 2% blocking reagent) overnight at 4°C. The hybridization signals were visualized by NTMT (100 mM NaCl, 100 mM Tris–HCl, pH 9.5, 50 mM MgCl2, 0.1% Tween-20) and NBT/BCIP staining which was stopped by washing the sections with water.
Immunohistochemical analysis of the a-Nka was performed in the gill of black porgy. A few deparaffinized serial sections (5 μm) were incubated with 3% H2O2 in PBS. The sections were then incubated with 1.5% normal goat serum for 30 min and with commercial primary antibody (a-Nka-IgG) (Santa Cruz Biotechnology Inc., Santa Cruz, CA, United States) overnight at 4°C. This was followed by incubation with anti-rabbit IgG (Vector Laboratories Inc., Burlingame, CA, United States) for 1 h at room temperature. The sections were then visualized by an ABC kit (avidin-biotin, Vector Laboratories Inc.) and DAB (3,3′-diaminobenzidine, Sigma).
Data are expressed as means ± standard error of the mean. The values were analyzed by one-way ANOVA, followed by Student–Newman–Keuls multiple tests. Value with p < 0.05 indicating a significant difference and are denoted by “a” and “b.” Student’s t-test was also conducted to determine significant differences (p < 0.05) between SW and FW fish denoted by asterisks (∗).
The expected single band of crh, crhr, and a-nka was obtained in all the examined tissues except a-nka in Dien by RT-PCR, though with varying intensity in expression. A strong band of crh and crhr was found in the Dien and gill. In the gill, the expression of a-nka was strong, but no expression was observed in Dien (Figure 1A). crh, crhr, and a-nka mRNA levels were determined by RT-PCR in Dien and gill of SW and FW black porgy. gapdh was used as an internal control (Figure 1A).
Figure 1. (A) The black porgy corticotropin releasing hormone (crh), crh receptor (crhr), and a-nka cDNA consisted of 363 bp, 556 bp, and 968 bp. A single band of the expected size was obtained for crh, crhr, and α-nka mRNA in the tissue analyzed by RT-PCR. crh and crhr mRNA were detected in the diencephalon (Dien) and gill. a-nka mRNA was strongly detected in the gill. In Dien, the intensity of the band was very weak. The gapdh was used as an internal control. (B) Comparative analysis of crh, gene expressions as determined by quantitative real-time PCR in Dien of black porgy from a seawater (SW) to freshwater (FW) transfer at 8 h, day 1, day 4, and day 30. Each data had 10 fish. (C) Localization of crh in the hypothalamus gigantocellular (PMgc) (b,e) and parvocellular part of the magnocellular preoptic nucleus (PMpc) (c,f) was performed by in situ hybridization during SW (a–c) and FW (d–f) acclimation of black porgy on day 30. The representative fish is shown from one of 3 fish. There was no notable signal found in the representative sense pictures (not shown). PMgc, gigantocellular part of the magnocellular preoptic nucleus; PMmc, magnocellular part of the magnocellular preoptic nucleus; PMpc, parvocellular part of the magnocellular preoptic nucleus. (D) Comparative analysis of crh, gene expressions as determined by quantitative real-time PCR in gill of black porgy from SW to FW at 8 h, day 1, day 4, and day 30. Data (10 fish for each data) are expressed as means ± standard error of the mean. Student’s t test was conducted to determine significant differences (p < 0.05) between SW and FW fish are denoted by asterisks (∗). (E) The in situ hybridization was performed to localize crh transcripts in black porgy gill during SW and FW acclimation at day 1 (c,d) and day 30 (g,h). The transcripts of crh hybridization signal were weak in FW acclimated fish (c,d) in the branchial epithelium when compared to the SW acclimated fish (a,b) at day 1. And, the hybridization signals of crh were similar between SW (e,f) and FW (g,h) fish at day 30 in the branchial epithelium of the gill. The representative fish is shown from one of 3 fish. There was no notable signal found in the representative sense pictures (not shown). aa, afferent artery; be, branchial epithelium; ea, efferent artery; fi, filament; la, lamellae.
The expression of crh in FW was highly expressed in Dien at day 4 and day 30 versus the control (SW) fish (Figure 1B). In contrast, the expression of crhr (Figure 2A) was significantly (p < 0.05) downregulated in the Dien at day 4 FW when compared to their respective controls. No significant difference between SW and FW was observed in the expression of crhr in Dien at 8 h, day 4, and day 30 (Figure 2A).
Figure 2. (A) Comparative analysis of crhr, gene expressions as determined by quantitative real-time PCR in diencephalon (Dien) of black porgy from SW to FW at 8 h, day 1, day 4, and day 30. Each data had 10 fish. (B) Comparative analysis of crhr, gene expressions as determined by quantitative real-time PCR in the gill of black porgy from a SW to FW transfer at 8 h, day 1, day 4, and day 30. Each data had 10 fish. (C) The in situ hybridization was performed to localize crhr transcripts in black porgy gill during SW and FW acclimation on day 1 (a–f) and day 30 (g–j). The mRNA expression of crhr signals was more abundant throughout the gill in FW-acclimated fish (d–f) compared to the SW fish (a–c) on day 1. On day 30, the hybridization signals of crhr were almost similar between SW (g,h) and FW (i,j) fish in the branchial epithelium of the gill. The representative fish is shown from one of 3 fish. There was no notable signal found in the representative sense pictures (not shown). aa, afferent artery; be, branchial epithelium; ea, efferent artery; fi, filament; la, lamellae. Student’s t-test was conducted to determine significant differences (p < 0.05) between SW and FW denoted by asterisks (*).
A significant (p < 0.05) reduction was found in the gill crh at day 1 and day 4 in FW versus SW (Figure 1D). In contrast, the crhr mRNA levels were significantly (p < 0.05) increased in gill at day 1 in FW versus SW (Figure 2B). The expression levels of α-nka were 5, 6, 4.5, and 4.4-fold higher in FW at 8 h, day 1, day 4, and day 30, respectively, versus controls (Figure 3A). No significant difference was observed in the expression of crh and crhr in gill between SW and FW fish at 8 h and day 30 (Figures 1D, 2B).
Figure 3. (A) Comparative analysis of a-nka, gene expressions as determined by quantitative real-time PCR in diencephalon (Dien) of black porgy from SW to FW at 8 h, day 1, day 4, and day 30. Each data had 10 fish. (B) The immunohistochemistry was performed to localize the a-Nka cells in black porgy gill during SW and FW acclimation on day 1 and day 30. The expression of a-Nka in the gill was increased in FW at 8 hrs (b) and day 1 (d) compared to the respective SW fish (a,c). The Nka was expressed in the filaments and lamellae in the FW fish (b,d) but the a-Nka was expressed only in the gill filament not lamellae in SW fish (a,c). The representative fish is shown from one of 3 fish. There were no notable signals found in the control (not shown). (C) Localization pattern of a-Nka cells during SW (a) and FW (b) of black porgy. The a-Nka cells were also appeared in the lamellae (pointed with arrow heads) in addition to filament in FW fish (b). MRC: mitochondria rich cell, fi: filament, la: lamellae. Student’s t-test was conducted to determine significant differences (p < 0.05) between SW and FW denoted by asterisks (*).
The localization of crh transcripts was performed in the hypothalamus at day 30 SW (Figures 1Ca–c) and FW (Figures 1Cd–f) by in situ hybridization. The crh transcript hybridization signals were detected in both SW (Figure 1Ca) and FW fish (Figure 1Cd). The crh mRNA hybridization signals were more abundant in FW fish in the gigantocellular part of the magnocellular preoptic nucleus (PMgc) (Figure 1Ce) and parvocellular part of the magnocellular preoptic nucleus (PMpc) (Figure 1Cf) versus the SW fish (Figures 1Cb,c).
The expression of crh (Figure 1E) and crhr (Figure 2C) gene was examined by in situ hybridization in gill at day 1 and day 30. The hybridization signals of crh and crhr mRNA in the gill were located near and around the afferent filamental artery (Figures 1Ea–h, 2Ca–j). The transcripts of crh hybridization signals were decreased in FW acclimated fish in the branchial epithelium (Figures 1Ec,d) versus the SW acclimated fish (Figures 1Ea,b) at day 1. In contrast, the mRNA expression of crhr signals was more abundant throughout the gill in the FW acclimated fish (Figures 2Cd–f) versus the SW fish (Figures 2Ca–c) at day 1. The hybridization signals of crh and crhr were similar between SW (Figures 1Ee,f, 2Cg,h) and FW (Figures 1Eg,h, 2Ci,j) fish at day 30 in the branchial epithelium of the gill.
The expression of a-Nka was examined in the gill (Figures 3Ba–d). The a-Nka cells were mainly expressed in the MRCs. The expression of a-Nka genes in the gill was increased in FW at 8 h, day 1, day 4, and day 30. However, we performed the immunohistochemistry at 8 h and day 1 in both SW (Figures 3Ba,b) and FW (Figures 3Bc,d). The a-Nka cells were expressed in the filaments and lamellae in the FW fish at 8 h and day 1 (Figures 3Bb,d) but the a-Nka cells were expressed only in the gill filament but not lamellae in SW fish (Figures 3Ba,c). The fluorescent hybridization showed the pattern of a-Nka cells in SW and FW fish (Figures 3Ca,b). The a-Nka cells were localized in the filament at SW (Figure 3Ca). However, FW fish had a-Nka cells localized in both filament and lamellae (Figure 3Cb).
We further examined the co-localization of crh transcript cells vs. a-Nka cells and crhr transcript cells vs. a-Nka cells in the gill filament or lamellae of FW and SW fish by ISH and IHC (Figure 4). Our data found that crh and crhr transcript cells were not co-localized with a-Nka cells in either FW or SW fish (Figure 4). The crh and crhr cells are suggested to localize in the basal cells of the filament and a-Nka cells are localized in the filament epithelium (Figure 4).
Figure 4. There was no co-localization of crh transcript cells vs. a-Nka cells and crhr transcript cells vs. a-nka cells in the gill filament of FW and SW fish. Fi, filament; la, lamellae. (A) crh transcripts in the gill filament/lamellae of FW (a,b) and SW (c,d) fish. (B) crhr transcripts in filament/lamellae of FW (a,b) and SW (c,d) fish.
The transcripts of crh were significantly (p < 0.05) increased in the gill in vivo at low (10 μg/kg) and high doses (40 μg/kg) of crh (Figure 5A). No significant (p > 0.05) difference was observed in the mRNA expression of crhr (Figure 5B) and a-nka (Figure 5C) in the gill in vitro at both low and high doses of CRH. No significant (p > 0.05) difference was found in the transcripts of crh and crhr in the Dien at low and high doses of in vivo crh treatment (Figures 5D,E).
Figure 5. Q-PCR analysis was performed to examine the mRNA expression of crh, crhr, and a-nka in the gill (A–C) and diencephalon (Dien) (D,E) during in vivo CRH treatment with low (10 μg/kg) and high (40 μg/kg) doses. Data (n = 8 for each data) are expressed as means ± standard error of the mean. The values were analyzed by one-way ANOVA, followed by a Student–Newman–Keuls multiple test, with p < 0.05 indicating a significant difference, are denoted by “a” and “b.”
The transcripts of crh were significantly increased at medium (10–7 M) and high (10–5 M) doses of CRH (Figure 6A). The crhr was significantly (p < 0.05) increased only at high dose in the gill on day 2 (Figure 6B). The long duration of gill culture with CRH did not change the mRNA levels of CRH on day 4 (Figure 6C). However, high dose of crh significantly (p < 0.05) downregulated the crhr mRNA expression in the gill on day 4 (Figure 6D). In addition, the low dose (10–9 M) of CRH did not change the crh and crhr transcripts in the gill on day 2 and day 4 (Figures 6A–D).
Figure 6. (A–D) Q-PCR analysis was performed to examine the mRNA expression of crh and crhr in gill on day 2 (A,B) and day 4 (C,D) during in vitro gill culture with CRH low (10–9 M), medium (10–7 M) and high (10–6 M) doses. Data (n = 8 for each data) are expressed as means ± standard error of the mean. The values were analyzed by one-way ANOVA, followed by a Student–Newman–Keuls multiple test, with p < 0.05 indicating a significant difference, and are denoted by “a” and “b.” (E–H) Q-PCR analysis was examined the mRNA expression of crh and crhr in gill on day 2 (E,F) and day 4 (G,H) during in vitro gill culture with DEX low (10–8 M), medium (10–6 M), and high (10–4 M) doses. Data (n = 8 for each data) are expressed as means ± standard error of the mean. The values were subjected to analysis by one-way ANOVA, followed by a Student–Newman–Keuls multiple test, with p < 0.05 indicating a significant difference, are denoted by “a” and “b.”
The mRNA expression of crh (Figure 6E) and crhr (Figure 6F) was significantly (p < 0.05) increased at high dose (10–4 M). No significant (p > 0.05) difference was observed in the gill at low (10–8 M) and medium (10–6 M) doses of DEX versus their controls at day 2. In addition, there was no significant (p > 0.05) difference in the transcripts of crh (Figure 6G) and crhr (Figure 6H) at low, medium, and high dose of DEX at day 4.
We show that the mRNA expression of crh, crhr, and a-nka in the Dien and gill of black porgy during a SW to FW acclimation at 8 h, day 1, day 4, and day 30. In addition, we localized crh and crhr transcripts in SW and FW Dien and gill of black porgy by in situ hybridization at day 1 and day 30. The crh (in vitro, in vivo) and DEX (in vitro) treatments with different doses were performed to analyze the differential mRNA expression pattern of crh and crhr in Dien and gill. Furthermore, we localized the a-Nka cells in FW and SW-acclimated black porgy gill at day 1 and day 30 by immunohistochemistry. The mRNA expression of crh, crhr, and a-nka transcripts during FW transfer and in vivo and in vitro crh and DEX treatment at different days with different doses were elaborately framed in the current study.
The serum osmolality levels were significantly decreased in the FW black porgy at day 1 and 30 (unpublished data). In the SW fish, serum osmolality was significantly higher compared to the FW fish, as indicated in our previous study (Tomy et al., 2009). Serum cortisol was significantly increased at day 1 and returned to normal levels at 30 (unpublished data). According to this, it seems that black porgy may require only a short period for acclimation to osmotic stress. The total concentration of solutes such as the inorganic ions present in the fluid is the osmolality (Soegianto et al., 2017). However, at the time of initial acclimation the serum osmolality levels were significantly different between SW and FW fish; this reflects the acclimation. The euryhaline teleost reduced their ion secretion rapidly when quickly moved to a FW environment. Overall, the black porgy maintained its serum osmolality within the range of 270–420 mOsmol kg–1. This suggests efficient hyper or hypo osmoregulation in black porgy (Tomy et al., 2009).
Generally, the teleost crh system was activated through the HPI-axis during the initial stress (Huising et al., 2004; Doyon et al., 2005). The expression of crh could regulate itself in response to the stress induced by the elevation of glucocorticoids and neurogenic signals (Lachize et al., 2009). The increased cortisol levels could affect gill function via regulating gill ion transporters (Kiilerich et al., 2007). The elevated hypothalamic crh markedly downregulated the pituitary crhr expression because continuous release of the hypothalamic crh desensitized the pituitary corticotrope cells (Huising et al., 2004). Furthermore, repeated or chronic stress can increase the crh mRNA levels in mammalian hippocampus, paraventricular nucleus, and tilapia forebrain and hypothalamus (Makino et al., 1995; Aruna et al., 2015). This suggests that the intensity and duration of the salinity stress are important external factors regulating the brain crh system (Doyon et al., 2005; Aruna et al., 2012a).
Seawater and FW acclimation activated crh, crhr, and a-nka transcripts in the black porgy hypothalamus and gill. In agreement with Doyon et al. (2005), we show here that the expression of crh mRNA was significantly increased at day 4 and day 30 whereas the crhr transcripts were significantly downregulated at day 4 in the Dien of the black porgy. These data suggest that the transiently elevated levels of plasma cortisol may exert negative-feedback effects on the level hypothalamus (hypothalamic crhr) as suggested in carp pituitary (Huising et al., 2004). In addition, we detected the crh transcript hybridization signals in both SW and FW fish. Similar to Q-PCR data, the crh mRNA hybridization signals were more abundant in FW fish at PMgc and PMpc versus SW fish at day 30. The data suggest that the expression of crh mRNA is essential to the salinity stress.
Increased expression of crhr mRNA in the gill suggests that the gills are responsible for the stressors and regulate the ventilation rate and blood oxygen transport (Wendelaar Bonga, 1997; Mazon et al., 2006). Furthermore, the Q-PCR and in situ hybridization results show that the crh transcripts were significantly reduced in the black porgy gill at day 1 and day 4. Interestingly, the crhr was significantly increased in the gill at day 1. The local production of crh and/or crhr in the gill may be directly activated by external stressors.
There are very few studies on the expression and localization of crh and crhr in the gill during SW and FW acclimation. This is the first study in black porgy to localize the crh and crhr transcripts in SW and FW-acclimated gill at day 1 and day 30 by in situ hybridization. Similar to the Q-PCR results, the cellular transcripts of the crh and crhr were lower and higher in FW-acclimated fish, respectively. The transcripts of the crh and crhr were highly localized in the apical membrane of the branchial epithelium in both SW and FW black porgy. In addition, the crhr transcripts were localized in the basal cells of the gill filament as reported in common carp by immunohistochemistry (Mazon et al., 2006). Our previous studies showed glucocorticoid receptors and mineralocorticoid receptors in tilapia gill (Aruna et al., 2012b). Taken together, the expression and localization of crh and crhr in black porgy gill highlight that positive involvement of the crh system can balance the homeostasis during salinity stress.
The DEX (5 mg/kg)-treated pigs had no effect on the mRNA expression of crh in the hypothalamus after 3 h (Vellucci and Parrott, 2000). In mice, in vitro DEX treatment for 24–96 h caused a specific decrease in crh mRNA (Adler et al., 1988). Here, we elaborately framed out the mRNA expression of crh and crhr in the Dien and gill by in vitro and in vivo crh and DEX treatment. In contrast to Adler et al. (1988), a high in vitro dose of DEX increased the transcripts of the crh and crhr in black porgy gill on day 2. Pierson et al. (2004) showed that the high dose of DEX treatment leads to a chronic stress, which may activate the local crh-system in the gill.
We also found that the transcripts of crh were significantly increased in gill by in vitro and in vivo CRH treatment. In vivo CRH treatment did not cause any effects on the expression of crh and crhr transcripts in the black porgy Dien with different doses. Consistent with our findings, the in vitro and/or in vivo CRH treatment decreased and/or did not change the mRNA expression of CRHR in the mammalian anterior pituitary after acute or repeated immobilization stress (Hauger et al., 1977; Luo et al., 1995; Makino et al., 1995; Sakai et al., 1996; Roseboom et al., 2001). Sumitomo et al. (1987) and Yokoe et al. (1988) strongly suggested that the main source of plasma CRH was the hypothalamic paraventricular nucleus neurons with their terminals in the median eminence of mammals. In contrast to the mammals, the descending spinal crh-ir projections appear to be absent in teleosts (Lovejoy and Balment, 1999; Pepels et al., 2002). Taken together, the local gill crh system may respond more rapidly than the hypothalamic crh in black porgy.
The crh system is a powerful stress response tool in teleosts (HPI-axis/local crh). It can trigger cortisol release from the cascade signals of brain-pituitary to inter-renal gland to respond to the various stressors (Flik et al., 2006). Generally, the presence of crh in the brain (nucleus of preopticus) is associated with fear and anxiety (Majzoub, 2006). Pepels et al. (2002) found a crh in the heads of larvae of Mozambique tilapia 5 days post hatching, suggesting the importance of the crh system in teleosts. The cutaneous crh system may respond to external stressors in mammals (Slominski et al., 2001). We also found the changes in the expression of crh-crhr in the brain-pituitary and corticosteroid receptors in the gill during the stress response in tilapia (Aruna et al., 2012a,b, 2015). Gill tissue separates the internal and external environment and is constantly activated by external stressors or internal factors. The presence of local crh, crhr, and corticosteroid receptors in the gill may be important to respond to stress in fish. Thus, the activation of the local gill crh-crhr system may be regulated by the circulation (crh-acth system from the brain-pituitary, and cortisol from interrenal gland) or local crh during stress.
Consistent with the physiological model for ion secretion by gill epithelia, the FW transfer significantly increased the mRNA expression of a-nka at 8 h, day 1, day 4, and day 30 in black porgy gill. These findings suggest that gills actively adapt to FW with possible functional plasticity along with more rapid responses to environmental changes. Similar elevation in branchial a-nka mRNA transcripts has been reported in Anguilla anguilla (Cutler et al., 1995) and Salmo trutta (Madsen et al., 1995) for the transfer from FW to SW. Black porgy was used for the transfer from SW to FW (Tomy et al., 2009). During the SW to FW transfer, the expression of a-Nka was on the filaments and lamellae MRCs on day 1 in FW fish. On day 30, the a-Nka cells were increased in number and larger but were expressed only in the filament of FW fish when compared to the respective SW fish. These data suggest that the changes in the ionic composition of hypotonic media can induce dramatic modifications in the functions of the gills and alter the morphology of MRCs (Laurent et al., 1985; Perry and Laurent, 1989; Perry et al., 1992; Lee et al., 1996). Thus, there may be two types of MRC. A type whose function rules out ions in a marine environment and another type that absorbs Na+, Cl–, Ca2+ in a FW environment (Perry, 1997; Goss et al., 2001; Marshall, 2002). These MRCs were located along the filamental epithelium for many species and along the secondary lamellae (Wendelaar Bonga and van der Meij, 1989; Katoh and Kaneko, 2003; Carmona et al., 2004; Lima and Kültz, 2004; Allen and Cech, 2007). Consistent with these results, MRCs have been shown to localize on both the lamellae and filaments in hypo-osmotic environment (Cataldi et al., 1995; McKenzie et al., 1999). Furthermore, the expression and localization of crh, crhr and a-Nka cells in the gill showed that the brain-gill crh system might be involved in the osmoregulation.
This study demonstrated crh and crhr in the gill by in situ hybridization during SW and FW in black porgy. The differential expression pattern of crh mRNA was found in Dien and gill during FW transfer and in vivo treatment of CRH in black porgy suggesting that the crh mRNA in the gill is independently functioned and regulated during environmental stress. Increased transcripts of crh mRNA in the gill by in vitro and in vivo crh treatment provide evidence that the crh may be regulated by itself in an autocrine manner. In addition, the increased expression of a-nka mRNA with differential localization of a-Nka cells in the filament and lamellae at 8 h and day 1 in FW fish suggests that a-Nka cells play a key role in ionic homeostasis during FW acclimation. The changes in the localization of the a-Nka cells in the black porgy gill adaptively respond to changing levels of environmental ions. Thus, the presence of crh and crhr in fish gill is essential to respond to the ambient salinity and/or stress to maintain the ion/base regulation as well as homeostasis.
The raw data supporting the conclusions of this article will be made available by the authors, without undue reservation.
The animal study was reviewed and approved by the National Taiwan Ocean University (#99026).
C-FC conceived and designed the project, and supervised, wrote, reviewed, and edited the manuscript. AA and C-FC wrote the manuscript. AA, T-PW, J-CC, D-SL, and GN collected, measured, and analyzed the sample. AA and GN wrote the first draft of the manuscript. AA, GN, and C-FC worked for the revision. All authors contributed to the article and approved the submitted version.
This research was partially supported by the Academia Sinica project (AS-99-TP-B05 and AS-101-TP-B01), Taiwan.
The authors declare that the research was conducted in the absence of any commercial or financial relationships that could be construed as a potential conflict of interest.
All claims expressed in this article are solely those of the authors and do not necessarily represent those of their affiliated organizations, or those of the publisher, the editors and the reviewers. Any product that may be evaluated in this article, or claim that may be made by its manufacturer, is not guaranteed or endorsed by the publisher.
Adler, G. K., Smas, C. M., and Majzoub, J. A. (1988). Expression and dexamethasone regulation of the human corticotropin-releasing hormone gene in a mouse anterior pituitary cell line. J. Biol. Chem. 263, 5846–5852. doi: 10.1016/s0021-9258(18)60643-x
Allen, P. J., and Cech, J. J. (2007). Age/size effects on juvenile green sturgeon (Acipenser medirostris), oxygen consumption, growth, and osmoregulation in saline environments. Environ. Biol. Fish. 79, 211–229.
Aruna, A., Nagarajan, G., and Chang, C. F. (2012a). Involvement of corticotrophin-releasing hormone and corticosteroid receptors in the brain–pituitary–gill of tilapia during the course of seawater acclimation. J. Neuroendocrinol. 24, 818–830. doi: 10.1111/j.1365-2826.2012.02282.x
Aruna, A., Nagarajan, G., and Chang, C. F. (2012b). Diffferential expression patterns and location of glucocorticoids and mineralocorticoid receptor transcripts in the osmoregulatory organs of tilapia during salinity stress. Gen. Comp. Endocrinol. 179, 465–476. doi: 10.1016/j.ygcen.2012.08.028
Aruna, A., Nagarajan, G., and Chang, C. F. (2015). The acute salinity changes activate the dual pathways of endocrine responses in the brain and pituitary of tilapia. Gen. Comp. Endocrinol. 211, 154–164. doi: 10.1016/j.ygcen.2014.12.005
Carmona, R., Garcia-Gallego, M., Sanz, A., Domezain, A., and Ostos-Garrido, M. N. (2004). Chloride cells and pavement cells in gill epithelia of Acipenser naccarii: ultrastructural modifications in seawater acclimated specimens. J. Fish Biol. 64, 553–566.
Cataldi, E., Ciccotti, E., Di Marco, P., Di Santo, O., Bronzi, P., and Cataudella, S. (1995). Acclimation trials of juvenile Italian sturgeon to different salinities: morpho-physiological descriptors. J. Fish Biol. 47, 609–618. doi: 10.1111/j.1095-8649.1995.tb01927.x
Cutler, C. P., Sanders, I. L., Hazon, N., and Cramb, G. (1995). Primary sequence, tissue specificity and expression of the Na+K+-ATPase alpha 1 subunit in the European eel (Anguilla anguilla). Comp. Biochem. Physiol. B 111, 567–573.
Dautzenberg, F. M., and Hauger, R. L. (2002). The CRF peptide family and their receptors: yet more partners discovered. Trends Pharmacol. Sci. 23, 71–77. doi: 10.1016/s0165-6147(02)01946-6
Doyon, C., Trudeau, V. F., and Moon, T. W. (2005). Stress elevates corticotropin releasing factor and CRF-binding protein mRNA levels in rainbow trout (Oncorhynchus mykiss). J. Endocrinol. 186, 123–130. doi: 10.1677/joe.1.06142
Evans, D. H., Piermarini, P. M., and Choe, K. P. (2005). The multifunctional fish gill: dominant site of gas exchange, osmoregulation, acid-base regulation, and excretion of nitrogenous waste. Physiol. Rev. 85, 97–177. doi: 10.1152/physrev.00050.2003
Flik, G., Klaren, P. H. M., Van den Burg, E. H., Metz, J. R., and Huising, J. R. (2006). CRF and stress in fish. Gen. Comp. Endocrinol. 146, 36–44.
Flik, G., and Verbost, P. M. (1993). Calcium transport in fish gills and intestine. J. Exp. Biol. 184, 17–29. doi: 10.1242/jeb.184.1.17
Goss, G. G., Adamia, S., and Galvez, F. (2001). Peanut lectin binds to a subpopulation of mitochondria-rich cells in the rainbow trout epithelium. Am. J. Physiol. 281, R1718–R1725. doi: 10.1152/ajpregu.2001.281.5.R1718
Hauger, R. L., Dautzenberg, F. M., Flaccus, A., Liepold, T., and Spiess, J. (1977). Regulation of corticotrophin releasing factor receptor function in human Y-79 retinoblastoma cells: rapid and reversible homologous desensitization but prolonged recovery. J. Neurochem. 68, 2308–2316. doi: 10.1046/j.1471-4159.1997.68062308.x
Huising, M. O., Metz, J. R., van Schooten, C., Taverne-Thiele, A. J., Hermsen, T., Verburg-van Kemenade, B. M., et al. (2004). Structural characterisation of a cyprinid (Cyprinus carpio L.) CRH, CRH-BP and CRH-R1, and the role of these proteins in the acute stress response. J. Mol. Endocrinol. 32, 627–648. doi: 10.1677/jme.0.0320627
Kaneko, T., and Hiroi, J. (2008). “Osmo- and iono regulation,” in Fish Larval Physiology, eds R. N. Finn and B. G. Kapoor (Enfield: Science Publishers), 163–183.
Kaneko, T., Shiraishi, K., Katoh, F., Hasegawa, S., and Hiroi, J. (2002). Chloride cells in early life stages of fish and their functional differentiation. Fish. Sci. 68, 1–9. doi: 10.1046/j.1444-2906.2002.00382.x
Kaneko, T., Watanabe, S., and Lee, K. M. (2008). Functional morphology of mitochondrion-rich cells in euryhaline and stenohaline teleosts. Aqua Biosci. Monogr. 1, 1–62. doi: 10.1007/BF00221380
Katoh, F., and Kaneko, T. (2003). Short-term and long-term replacement of branchial chloride cells in killifish transferred from seawater to freshwater, revealed by morphofunctional observations and a newly established ‘time-differential double fluorescent staining’ technique. J. Exp. Biol. 206, 4113–4123. doi: 10.1242/jeb.00659
Kiilerich, P., Kristiansen, K., and Madsen, S. S. (2007). Cortisol regulation of ion transporter mRNA in Atlantic salmon gill and the effect of salinity on the signaling pathway. J. Endocrinol. 194, 417–427. doi: 10.1677/JOE-07-0185
Lachize, S., Apostolakis, E. M., van der Laan, S., Tijssen, A. M. I., Xu, J., Kloet, E. R., et al. (2009). Steroid receptor coactivator-1 is necessary for regulation of corticotropin-releasing hormone by chronic stress and glucocorticoids. Proc. Natl. Acad. Sci. U.S.A. 19, 8038–8042.
Laurent, P., Hobe, P., and Dunel-Erb, H. S. (1985). The role of environmental sodium chloride relative to calcium in gill morphology of freshwater salmonid fish. Cell Tissue Res. 240, 675–692. doi: 10.1007/bf00216356
Lee, T. H., Hwang, P. P., and Feng, S. H. (1996). Morphological studies of gill and mitochondria-rich cells in the stenohaline Cyprinid teleosts, Cyprinus carpio and Carassius auratus, adapted to various hypotonic environments. Zool. Stud. 35, 272–278.
Li, J., Eygensteyn, J., Lock, R. A. C., Verbost, P. M., Van Der Heijdden, A. J. H., Wendelaar Bonga, S. E., et al. (1995). Branchial chloride cells in larvae and juveniles of freshwater tilapia Oreochromis mossambicus. J. Exp. Biol. 198, 2177–2184. doi: 10.1242/jeb.198.10.2177
Lima, R., and Kültz, D. (2004). Laser scanning cytometry and tissue microarray analysis of salinity effects on killifish chloride cells. J. Exp. Biol. 207, 1729–1739. doi: 10.1242/jeb.00930
Lovejoy, D. A., and Balment, R. J. (1999). Evolution and physiology of the corticotropin-releasing factor (CRF) family of neuropeptides in vertebrates. Gen. Comp. Endocrinol. 115, 1–22. doi: 10.1006/gcen.1999.7298
Luo, X., Kiss, A., Rabadan-Diehl, C., and Aguilaera, G. (1995). Regulation of hypothalamic and pituitary corticotrophin-releasing hormone receptor messenger ribonucleic acid by adrenalectomy and glucocorticoids. Endocrinology 136, 3877–3883. doi: 10.1210/endo.136.9.7649095
Madsen, S. S., Jensen, M. K., Nhr, K., and Kristiansen, K. (1995). Expression of Na+K+- ATPase in the brown trout, Salmo trutta: in vivo modulation by hormones and seawater. Am. J. Physiol. 269, R1339–R1345.
Makino, S., Schulkin, J., Smith, M. A., Pacak, K., Palkovits, M., and Gold, P. W. (1995). Regulation of corticotrophin releasing hormone receptor messenger ribonucleic acid in the rat brain and pituitary by glucocorticoids and stress. Endocrinology 136, 4517–4525. doi: 10.1210/endo.136.10.7664672
Marshall, W. S. (2002). Na+, Cl–, Ca2+, and Zn2+ transport by fish gills: a retrospective review and prospective synthesis. J. Exp. Zool. 293, 264–283. doi: 10.1002/jez.10127
Marshall, W. S., Bryson, S. E., and Wood, C. M. (1992). Calcium transport by isolated skin of rainbow trout. J. Exp. Biol. 166, 297–316. doi: 10.1242/jeb.166.1.297
Marshall, W. S., and Grosell, M. (2006). “Ion transport, osmoregulation, and acid-base balance,” in The Physiology of Fishes, eds D. H. Evans and J. B. Claiborne (Boca Raton, FL: CRC Press), 177–230. doi: 10.1007/978-3-642-70613-4_16
Mazon, A. F., Verburg-van Kemenade, B. M., Flik, G., and Huising, M. O. (2006). Corticotropin-releasing hormone-receptor 1 (CRH-R1) and CRH-binding protein (CRH-BP) are expressed in the gills and skin of common carp Cyprinus carpio L. and respond to acute stress and infection. J. Exp. Biol. 209, 510–517. doi: 10.1242/jeb.01973
McCormick, S. D., Hasegawa, S., and Hirano, T. (1992). Calcium uptake in the skin of a freshwater teleost. Proc. Natl. Acad. Sci. U.S.A. 89, 3635–3638. doi: 10.1073/pnas.89.8.3635
McKenzie, D. J., Cataldi, E., Di Marco, P., Mandich, A., Romano, P., Ansferri, S., et al. (1999). Some aspects of osmotic and ionic regulation in Adriatic sturgeon Acipenser naccarii. II: morpho-physiological adjustments to hyperosmotic environments. J. Appl. Ichthyol. 15, 61–66. doi: 10.1111/j.1439-0426.1999.tb00208.x
Pepels, P., Meek, J., Wendelaar Bonga, S. E., and Balm, P. H. M. (2002). Distribution and quantification of corticotropin-releasing hormone (CRH) in the brain of the teleost fish Oreochromis mossambicus (tilapia). J. Comp. Neurol. 453, 247–268.
Perry, S. F. (1997). The chloride cell: structure and function in the gills of freshwater fishes. Ann. Rev. Physiol. 59, 325–347. doi: 10.1146/annurev.physiol.59.1.325
Perry, S. F., Goss, G., and Fenwick, C. (1992). Interrelationships between gill chloride cell morphology and calcium uptake in freshwater teleosts. Fish Physiol. Biochem. 10, 327–337. doi: 10.1007/BF00004482
Perry, S. F., and Laurent, P. (1989). Adaptational responses of rainbow trout to lowered external NaCI concentration: contribution of the branchial chloride cell. J. Exp. Biol. 147, 147–168. doi: 10.1242/jeb.147.1.147
Pierson, P. M., Lamers, A., Flik, G., and Mayer-Gostanc, N. (2004). The stress axis, stanniocalcin, and ion balance in rainbow trout. Gen. Comp. Endocrinol. 137, 263–271. doi: 10.1016/j.ygcen.2004.03.010
Rivier, C., and Vale, W. (1983). Modulation f stress-induced ACTH release by corticotropin-releasing factor, catecholamines and vasopressin. Nature 305, 325–327. doi: 10.1038/305325a0
Roseboom, P. H., Unben, C. M., and Kalin, N. H. (2001). Persistent corticotrophin-releasing factor 1 receptor desensitization in the human retinoblastoma cell line IMR-32. Mol. Brain Res. 92, 115–127. doi: 10.1016/s0169-328x(01)00162-0
Sakai, K., Horiba, N., Sakai, Y., Tozawa, F., Demura, H., and Suda, T. (1996). Regulation of corticotrophin releasing factor receptor messenger ribonucleic acid in rat anterior pituitary. Endocrinology 137, 1758–1763.
Slominski, A., Wortsman, J., Luger, T., Paus, R., and Solomon, S. (2000). Corticotropin releasing hormone and proopiomelanocortin involvement in the cutaneous response to stress. Physiol. Rev. 80, 979–1020. doi: 10.1152/physrev.2000.80.3.979
Slominski, A., Wortsman, J., Pisarchik, A., Zbytek, B., Linton, E. A., Mazurkiewicz, J. E., et al. (2001). Cutaneous expression of corticotropin-releasing hormone (CRH), urocortin, and CRH receptors. FASEB J. 15, 1678–1693. doi: 10.1096/fj.00-0850rev
Soegianto, A., Adhim, M. H., Zainuddin, A., Putranto, T. W. C., and Irawan, B. (2017). Effect of different salinity on serum osmolality, ion levels and hematological parameters of East Java strain tilapia Oreochromis niloticus. Mar. Fresh. Behav. Physiol. 50, 105–113. doi: 10.1080/10236244.2017.1333391
Sumitomo, T., Suda, T., Tomori, N., Yajima, F., Nakagami, Y., Ushiyama, T., et al. (1987). Immunoreactive corticotropin-releasing factor in rat plasma. Endocrinology 120, 1391–1396. doi: 10.1210/endo-120-4-1391
Thisse, C., and Thisse, B. (2008). High-resolution in situ hybridization to whole mount zebrafish embryos. Nat. Protocols 3, 59–69. doi: 10.1038/nprot.2007.514
Tomy, S., Chang, Y. M., Chen, Y. H., Cao, J. C., Wang, T. P., and Chang, C. F. (2009). Salinity effects on the expression of osmoregulatory genes in the euryhaline black porgy Acanthopagrus schlegeli. Gen. Comp. Endocrinol. 161, 123–132. doi: 10.1016/j.ygcen.2008.12.003
Vale, W., Spiess, J., Rivier, C., and Rivier, J. (1981). Characterization of a 41-residue ovine hypothalamic peptide that stimulates secretion of corticotropin and beta-endorphin. Science 213, 1394–1397. doi: 10.1126/science.6267699
Vellucci, S. V., and Parrott, R. F. (2000). Gene expression in the forebrain of dexamethazone-treated pigs: effect on stress neuropeptides in the hypothalamus and hippocampus and glutamate receptor subunits in the hippocampus. Res. Vet. Sci. 69, 25–31. doi: 10.1053/rvsc.2000.0380
Verbost, P. M., Schoenmakers, T. J., Flik, G., and Wendelaar Bonga, S. E. (1994). Kinetics of ATP- and Na+-gradient driven Ca2+ transport in basolateral membranes from gills of freshwater- and seawater-adapted tilapia. J. Exp. Biol. 186, 95–108. doi: 10.1242/jeb.186.1.95
Wendelaar Bonga, S. E., Flik, G., Balm, P. H. M., and Van Der Meij, J. C. A. (1990). The ultrastructure of chloride cells in the gills of the teleost Oreochromis mossambicus during exposure to acidified water. Cell Tissue Res. 259, 575–585. doi: 10.1007/bf01740786
Wendelaar Bonga, S. E., and van der Meij, C. J. M. (1989). Degeneration and death, by apoptosis and necrosis, of the pavement and chloride cells in the gills of the teleost Oreochromis mossambicus. Cell Tissue Res. 55, 235–243. doi: 10.1007/bf00229086
Yokoe, T., Audhya, T., Brown, C., Hutchinson, B., Passarelli, J., and Hollander, C. S. (1988). Corticotropin-releasing factor levels in the peripheral plasma and hypothalamus of the rat vary in parallel with changes in the pituitary-adrenal axis. Endocrinology 123, 1348–1354. doi: 10.1210/endo-123-3-1348
Keywords: crh, crhr, α-nka, gill, fish, brain, osmotic stress
Citation: Aruna A, Wang T-P, Cao J-C, Lan D-S, Nagarajan G and Chang C-F (2021) Differential Expression of Hypothalamic and Gill-crh System With Osmotic Stress in the Euryhaline Black Porgy, Acanthopagrus schlegelii. Front. Physiol. 12:768122. doi: 10.3389/fphys.2021.768122
Received: 31 August 2021; Accepted: 06 October 2021;
Published: 11 November 2021.
Edited by:
Xiaodan Wang, East China Normal University, ChinaReviewed by:
Ramon Lavado, Baylor University, United StatesCopyright © 2021 Aruna, Wang, Cao, Lan, Nagarajan and Chang. This is an open-access article distributed under the terms of the Creative Commons Attribution License (CC BY). The use, distribution or reproduction in other forums is permitted, provided the original author(s) and the copyright owner(s) are credited and that the original publication in this journal is cited, in accordance with accepted academic practice. No use, distribution or reproduction is permitted which does not comply with these terms.
*Correspondence: Ganesan Nagarajan, bmFkaW1vb2xhbUBrZnUuZWR1LnNh; Ching-Fong Chang, QjAwNDRAZW1haWwubnRvdS5lZHUudHc=
Disclaimer: All claims expressed in this article are solely those of the authors and do not necessarily represent those of their affiliated organizations, or those of the publisher, the editors and the reviewers. Any product that may be evaluated in this article or claim that may be made by its manufacturer is not guaranteed or endorsed by the publisher.
Research integrity at Frontiers
Learn more about the work of our research integrity team to safeguard the quality of each article we publish.