- Department of Environmental Toxicology, University of California, Davis, Davis, CA, United States
An organism’s diet is a major route of exposure to both beneficial nutrients and toxic environmental chemicals and natural products. The uptake of dietary xenobiotics in the intestine is prevented by transporters of the Solute Carrier (SLC) and ATP Binding Cassette (ABC) family. Several environmental chemicals and natural toxins have been identified to induce expression of these defense transporters in fish and aquatic invertebrates, indicating that they are substrates and can be eliminated. However, certain environmental chemicals, termed Transporter-Interfering Chemicals or TICs, have recently been shown to bind to and inhibit fish and mammalian P-glycoprotein (ABCB1), thereby sensitizing cells to toxic chemical accumulation. If and to what extent other xenobiotic defense or nutrient uptake transporters can also be inhibited by dietary TICs is still unknown. To date, most chemical-transporter interaction studies in aquatic organisms have focused on ABC-type transporters, while molecular interactions of xenobiotics with SLC-type transporters are poorly understood. In this perspective, we summarize current advances in the identification, localization, and functional analysis of protective MXR transporters and nutrient uptake systems in the digestive system of fish and aquatic invertebrates. We collate the existing literature data on chemically induced transporter gene expression and summarize the molecular interactions of xenobiotics with these transport systems. Our review emphasizes the need for standardized assays in a broader panel of commercially important fish and seafood species to better evaluate the effects of TIC and other xenobiotic interactions with physiological substrates and MXR transporters across the aquatic ecosystem and predict possible transfer to humans through consumption.
Introduction
Fish and other aquatic animals can be exposed to water-soluble environmental chemicals via uptake through the gills and hydrophobic xenobiotics through ingestion of contaminated food. Gills are commonly considered a part of the respiratory system of aquatic animals (Collinder et al., 2009; Carvalho, 2011; Ray and Ringø, 2014). In addition to respiration, fish gills are critical for excretion of nitrous waste, pH regulation, hormone production, and osmoregulation (Foyle et al., 2020; Zhang et al., 2021). In most bivalves, gills have undergone a secondary adaptation to mainly serve as feeding structures while their function in osmoregulation and ion transport have been less studied (Riisgård et al., 2011, 2015; Moreira et al., 2015). Due to their permanent contact with the aquatic environment, the gills of fish and mollusks represent a crucial interface between the aquatic organism and the environment. As such, gills are typically equipped with selective (membrane) barriers that control nutrient uptake and toxic xenobiotic or metabolite elimination (Maetz and García Romeu, 1964; Erickson et al., 2006; Luckenbach and Epel, 2008; Hwang et al., 2011; Armitage et al., 2013; Wang and Wang, 2015). In fish, these barriers are present along the whole digestive system to provide precise control over small molecule uptake. While the digestive anatomy across terrestrial and aquatic vertebrates is highly similar, including a digestive tract with a basic segmentation into esophagus, stomach, midgut, and hindgut, the relative lengths and volumes of each section can differ according to diet and means of nutrient extraction and uptake (Chapman, 1997; Collinder et al., 2009; Karasov and Douglas, 2013; Furness et al., 2015).
In fish and mammals, most nutrients as well as water and ions in the diet are typically absorbed through the epithelial cells of the small and large intestines (Sundell and Rønnestad, 2011; Kiela and Ghishan, 2016). Thereby, macro- and micronutrient uptake is often mediated by simple diffusion, facilitated diffusion, or secondary active transport coupled to an electrochemical gradient (Sundell and Rønnestad, 2011). Conversely, the uptake of dietary toxins, toxicants and other harmful xenobiotics is prevented by a combination of primary active ATP-binding Cassette (ABC) and secondary active solute carrier (SLC) proteins lining the apical and basolateral membrane of the enterocytes (Dietrich et al., 2003; Müller et al., 2017; Nicklisch and Hamdoun, 2020). These membrane proteins effectively regulate xenobiotic bioavailability and often act in concert to either pump compounds into the blood stream for metabolic processing in the liver (primarily SLCs) or to efflux them back into the gut lumen (primarily ABCs) for immediate excretion.
Yet, despite the similarities in intestinal macro- and micro-anatomy between terrestrial and aquatic organisms, little is known about the molecular composition and levels of both nutrient uptake and xenobiotic defense systems along the digestive tract of fish and other aquatic organisms. In addition, there is limited data available on the molecular interactions of aqueous and food-borne contaminants with these transport systems and how these interactions could affect nutrient homeostasis and toxic contaminant bioaccumulation.
1. Epithelial Transport in the Digestive System of Aquatic Organisms
One of the key functions of the digestive system is to absorb nutrients from the diet that can be metabolized to provide energy for growth and development. At the same time, the intestinal tract serves as a major environmental barrier to prevent toxic xenobiotic uptake and accumulation. In the gills and intestine of fish and other aquatic organisms, several members of both the solute carrier (SLC) and ATP-Binding Cassette (ABC) family of transporters have been identified to participate in essential physiological functions such as nutrient uptake, ion flux, cell signaling processes, and toxic metabolite and xenobiotic efflux.
1.1 Nutrient and Endogenous Substrate Transporters
Transepithelial transport of nutrients in the gastrointestinal tract is typically mediated by secondary active transporters of the solute carrier (SLC) family. In humans, SLCs represent the largest group of secondary active membrane transporters (Hediger et al., 2004, 2013; Almén et al., 2009; Höglund et al., 2011). SLCs are capable of facilitating bidirectional transport (Kottra and Daniel, 2001; Kottra et al., 2002; Winter et al., 2011), though are primarily implicated in cellular uptake of nutrients and ions (Planchamp et al., 2007; Colas et al., 2016; Zhang et al., 2018; Felmlee et al., 2020; Song et al., 2020). Despite their important role in nutrient uptake and metabolic homeostasis, SLC transporters are notoriously understudied in humans and aquatic organisms (Verri et al., 2012; Schlessinger et al., 2013; César-Razquin et al., 2015; Barat et al., 2016).
In fish, SLCs play an important role in organismal development and transport of endogenous compounds to allow for proper development of swim bladders (Kim et al., 2020), inner ears (Liu et al., 2015), kidneys (Schoels et al., 2021), and normal development of embryos (Takesono et al., 2012). In addition, SLCs play a crucial role in intestinal absorption and transport of amino acids and other physiological compounds, including thyroid hormones (Arjona et al., 2011; Muzzio et al., 2014), iron (Cooper et al., 2007), zinc (Yan et al., 2012; Jiang et al., 2014), coenzyme-A (Kim et al., 2020), and amino acids and peptides (Romano et al., 2006, 2014; Verri et al., 2010, 2017; Margheritis et al., 2013; Rimoldi et al., 2015; Song et al., 2017; To et al., 2019; Vacca et al., 2019). Several SLC transporters have been shown to be involved in intestinal methionine absorption as well as iron transport and acid-base regulation in the gills (Cooper et al., 2007; Ivanis et al., 2008; To et al., 2019). For instance, in the intestine of rainbow trout, 8 SLC transporters (SLC1A5, SLC6A19, SLC6A15, SLC7A7, SLC43A1, SLC43A2, SLC3A1, and SLC3A2), which represent both high and low affinity methionine transporters, were found to have differential expression both along the length of the intestine and between the apical and basolateral membranes of the intestine (To et al., 2019). In the gills, sodium/hydrogen exchanging proteins SLC9A2 and SLC9A3, which are highly expressed in the gills and kidney, were found to localize to mitochondria rich cells that were also enriched with sodium/potassium ATPase, indirectly suggesting their involvement in acid-base regulation in rainbow trout (Oncorhynchus mykiss) gills (Ivanis et al., 2008). Furthermore, peptide transporting proteins of the SLC15 protein family are highly expressed in zebrafish (Danio rerio) intestines (Romano et al., 2006; Vacca et al., 2019). High intestinal expression was also shown for SLC15 proteins in tilapia (Oreochromis niloticus) (Huang et al., 2015). In Zebrafish, the expression of SLC22 proteins is low in the intestine, gills and heart while high in the liver and kidney (Mihaljevic et al., 2016). However, Organic Anion Transporting Proteins (OATPs), a subfamily of SLC22 proteins, are highly expressed in the gills and intestine (Popovic et al., 2010a). Intestinal SLC type transporters have also been described to transport vitamins, including vitamin E (alpha-tocopherol), vitamin B1 (thiamine), vitamin C (ascorbic acid), vitamin B7 (biotin), vitamin B9 (folate), and vitamin B2 (riboflavin) (Said and Nexo, 2018). Deficiencies in some of those vitamins have been shown to cause severe organ damage, oxidative stress, and developmental toxicity in fish (Wang et al., 2016; Pan et al., 2017; Harder et al., 2018).
1.2 Multidrug/Multixenobiotic Resistance Transporters
Members of both the ABC and SLC-type families have been shown to be part of a highly conserved defense mechanism against chemical insults, often referred to as multidrug-resistance (MDR) transporters (Ling, 1997; Gottesman et al., 2002; Glavinas et al., 2004; Leslie et al., 2005; Sharom, 2008; Giacomini et al., 2010; Hillgren et al., 2013; Nigam, 2015). The mammalian homologs of these transporter superfamilies have been extensively studied in the past, unraveling their precise molecular interactions with drugs and environmental chemicals (Juliano and Ling, 1976; Beck, 1987; Horio et al., 1988; Hediger et al., 2004; Oosterhuis et al., 2008; Staud et al., 2013; Nicklisch et al., 2016; Chedik et al., 2019; Guéniche et al., 2020; Nicklisch and Hamdoun, 2020).
In the early 90s, a mechanism similar to MDR was reported in aquatic organisms and since then referred to as multixenobiotic-resistance or MXR (Kurelec, 1992, 1997; Bard, 2000; Epel et al., 2008; Luckenbach and Epel, 2008; Ferreira et al., 2014). The MXR phenotype and its inducibility by environmental chemicals was first demonstrated in invertebrate species in the 1990s (Cornwall et al., 1995; Kurelec et al., 1995; Smital and Kurelec, 1998). Thereby, baseline levels of MXR inducibility and activity between different species have been correlated with the amount of pollution present in their habitat (Kurelec et al., 1995; Smital et al., 2000). Over the past three decades, fish and other aquatic invertebrates, such as sea urchins and mussels, have been instrumental in understanding MXR transporter interactions with xenobiotics and the chemosensitization effects of so called Transporter-Interfering Chemicals or TICs (Eufemia et al., 2002; Hamdoun et al., 2004; Luckenbach and Epel, 2005, 2008; Kwong et al., 2006; Stevenson et al., 2006; Faria et al., 2011; Martins et al., 2020; Nicklisch and Hamdoun, 2020; Protopopova et al., 2020; Nicklisch et al., 2021).
1.2.1 ABC-Type MDR/MXR Transporters
The ABC-type transporter P-glycoprotein (aka MDR1 or ABCB1) is one the best characterized mammalian MDR/MXR transporters that shows high expression in biological barriers such as the liver, brain, kidney and the intestine (Juliano and Ling, 1976; Beck, 1987; Horio et al., 1988). The identification of two P-glycoprotein genes in the winter flounder Pleuronectes americanus using Southern blot techniques represented the first set of P-glycoprotein orthologs reported in lower vertebrates (Chan et al., 1992; Luckenbach et al., 2014; Gordon et al., 2019). Since then, numerous ABC transporter proteins, which mediate the MXR phenotype, have been identified, characterized, and cloned in other fish species including Emerald rockcod (Zucchi et al., 2010), carp (Smital and Sauerborn, 2002), catfish (Liu et al., 2013), killifish (Christine Paetzold et al., 2009), mullet (Diaz de Cerio et al., 2012), Nile tilapia (Costa et al., 2012, 2013), rainbow trout (Lončar et al., 2010; Kropf et al., 2016; Love et al., 2021), zebrafish (Popovic et al., 2010b; Long et al., 2011; Fischer et al., 2013), and more recently yellowfin tuna (Nicklisch et al., 2021).
The mammalian MDR/MXR transporters include the ABCB, ABCC and ABCG subfamilies. Each of these subfamilies is comprised of members with physiological efflux function, xenobiotic efflux function, or both (Dean et al., 2001; Giacomini et al., 2010; König et al., 2013; Nicklisch and Hamdoun, 2020). For instance, in the human ABCB subfamily, ABCB1 has been characterized as a key drug efflux transporter in multiple barrier tissues while ABCB4 mainly serves as phosphatidylcholine transporter in the liver (Borst and Elferink, 2002; Dean and Annilo, 2005; Jonker et al., 2009). The human abcb5 gene has been suggested to confer drug resistance in malignant melanoma cells (Chen et al., 2005; Frank et al., 2005). Based on synteny analysis, the human abb1 and abcb4 genes are co-orthologs of zebrafish abcb4 (Ferreira et al., 2014; Luckenbach et al., 2014). Notably, several teleost fish, including zebrafish, lack the abcb1 ortholog but possess the two P-glycoproteins ABCB4 and ABCB5 (Fischer et al., 2013; Luckenbach et al., 2014). Thereby, the Zebrafish ABCB4 transporter has been described as major multixenobiotic efflux transporter while ABCB5 might be implicated in xenobiotic efflux in gill and skin ionocytes (Dymowska et al., 2012; Gordon et al., 2019). Yet, other fish species have been shown to possess abcb1 and abcb4 but lack the abcb5 ortholog (Liu et al., 2013). The confusion in annotating human versus fish MDR/MXR transporter genes mainly arises from lineage-specific genes, i.e., genes that have no detectable homologs in the other species (Dean and Annilo, 2005; Annilo et al., 2006; Gabaldón and Koonin, 2013). For the sake of clarity, in this perspective, we will refer to the aquatic species’ transporter genes as they have been annotated by the cited authors. Future combined synteny and phylogenetic analysis will likely revise those annotations and provide means to reduce the difficulty of inferring transporter function and nomenclature.
Studies on localization and expression levels of fish ABC-type MDR/MXR transporters have mostly focused on two model organisms, rainbow trout and zebrafish. For instance, the qPCR analysis of eight different ABC transporters (abcb1, abcb11, abcc1, abcc2, abcc3, abcc4, abcc5, and abcg2) in the liver, brain, gonads, kidney, gills, and intestine of rainbow trout revealed similar expression patterns to mammalian tissues (Lončar et al., 2010). Thereby, relative expression was higher in digestive and excretory tissues such as the liver, kidneys, and intestine compared to the brain or gills. Interestingly, the expression of the key efflux pump abcb1 was highest in brain but not detected in the gills (Lončar et al., 2010). The bile salt pump Abcb11 had very low expression across the tested tissues except for the liver.
The mRNA levels of abcb1a, abcb1b, abcc1, abcc2, abcc3, abcc4, abcc5, and abcg2 were also examined in earlier stages of rainbow trout embryo development and increased from 1 to 20 days post hatch. The predominant increases in abcb1a and abcb1b gene expression occurred in abdominal viscera (intestine, liver, kidney) and head (gills) of early larvae (Kropf et al., 2016). Gills of early larvae were also high in abcb5, abcc3, and abcg2 mRNA (Kropf et al., 2020). The ABC proteins abcb4, abcb5, abcc2 and abch1 have also been characterized in zebrafish and have been shown to confer the MXR phenotype (Popovic et al., 2010b; Long et al., 2011; Fischer et al., 2013). Cellular ABC transporter efflux activities have been detected in zebrafish embryos within 48 h of development (Long et al., 2011; Fischer et al., 2013). The abch1 transporter gene is unique to vertebrate fish and showed higher expression in tissues such as the gills and brain than in the intestine or liver (Popovic et al., 2010b). Expression of the abcc2 gene was highest in the intestine, followed by the kidney and liver (Long et al., 2011).
1.2.2 SLC-Type MDR/MXR Transporters
The SLC proteins are responsible for transporting a wide range of substrates including both organic and metal ions, endogenous metabolites, and xenobiotics such as pharmaceuticals and toxicants (Hediger et al., 2004; König et al., 2013; Lin et al., 2015; Bruyere et al., 2017; Chedik et al., 2019; Nicklisch and Hamdoun, 2020; Pizzagalli et al., 2021). In clinical research, there has been particular focus on members of the SLC22 family, which encompasses many organic ion transporters, and the SLC47 family, the multidrug and toxicant extrusion (MATE) proteins (Nigam, 2015, 2018). Yet, similar to nutrient uptake transporters, there have been very few studies on the tissue expression levels and localization of MDR/MXR-type SLC transporters in aquatic organisms. In the earlier stages of rainbow trout embryo development, the multidrug and toxin extrusion protein 1 (mate1) and organic anion transporting polypeptide 2 (oatp2) genes were detected in yolk sac epithelium, while the mRNA levels of the organic anion transporting polypeptide 1d gene (oatp1d) were high in abdominal viscera (Kropf et al., 2016). During the development of zebrafish embryos, mate6 and mate7 genes are highly expressed after 6 hpf (Lončar et al., 2016). Interestingly, tissue-specific mate gene expression in kidney, liver, intestine, and brain was generally higher in male than in female zebrafish, indicating gender differences in these important detoxification systems. The lowest gene expression of mate3, 4, 5, 6, 7, and 8 was found in the gills, irrespective of sex.
2. Regulation of Nutrient and MDR/MXR Transporter Expression
2.1 Diet and Adaptive Regulation of Intestinal Uptake
The quantity and quality of diet have been shown to influence transporter expression levels in the intestine of fish. For instance, fasting of Nile tilapia (Oreochromis niloticus) was shown to significantly decrease mRNA levels of the SLC15 peptide transporter gene family, but mRNA levels recovered upon refeeding (Huang et al., 2015). Similar results were seen in the Mummichog (Fundulus heteroclitus), where short-term fasting increased, long-term fasting decreased, and re-feeding restored SLC15 mRNA levels (Bucking and Schulte, 2012). Further, short-term starvation of rainbow trout induced an increase of P-glycoprotein concentration in the intestinal epithelia (Baumgarner et al., 2013).
Diet quality has also been shown to have a pronounced effect on fish transporters, and is particularly relevant in the field of aquaculture, where farmers have direct control over the primary source of nutrition as well as any form of dietary supplementation. Replacement of traditional fishmeal with meat and bone meal in the diet of juvenile turbot (Scophthalmus maximus) was shown to increase intestinal peptide transporter 1 (PepT1/SLC15A1) (Song et al., 2017). Similarly, large reductions in fishmeal content and replacement with vegetable meal in European sea bass (Dicentrarchus labrax) diet was shown to increase PepT1 gene expression but not affect the expression of the monocarboxylate transporter SLC6A19 (Rimoldi et al., 2015). Atlantic salmon (Salmo salar) fed diets supplemented with saponins and pea protein were shown to have several metabolic systems greatly altered, including differential expression of several SLC proteins (Kortner et al., 2012). Studies on dietary restrictions and feed supplementation that may decrease fitness of farmed fish are still in the early stages, but could serve as guideline to help elucidate mechanisms leading to various forms of intestinal pathology (Kortner et al., 2012).
2.2 Environmental Stressors
As a result of climate change and associated alterations in salinity, pH, and temperature of aquatic environments, the bioavailability and toxicity of environmental chemicals can increase (Noyes et al., 2009; Hasenbein et al., 2018; Ross and Behringer, 2019; Paul et al., 2020; Derby et al., 2021; Fulton et al., 2021). Recent studies have shown that these environmental stressor can also affect gene regulation, expression, and overall fitness of fish populations both within and between species (Jeffries et al., 2018, 2019; DeCourten et al., 2019; Romney et al., 2019; Mundy et al., 2020; Komoroske et al., 2021; Segarra et al., 2021). Most of these investigations have been conducted in fish and focused on SLC-type transporters and the effect of pH and temperature on substrate affinity and transport rate.
For instance, increased pH (pH > 7.4) was shown to cause a decrease in copper absorption in rainbow trout gut sacs as well as a decrease in iron absorption by SLC11 proteins isolated from rainbow trout gills and expressed in Xenopus laevis oocytes (Cooper et al., 2007; Nadella et al., 2007). The pH dependence of PepT1 in teleost fish has been thoroughly examined. In Zebrafish and other teleost fish, PepT1 tends to exhibit a higher substrate affinity with decreasing pH (Romano et al., 2014; Verri et al., 2017). Zebrafish PepT1 also exhibited an increase in maximal transport rate when extracellular pH transitioned from acidic (pH = 6.5) to alkaline (pH = 8.5), while other teleost fish Pept1 proteins exhibit little or no change in maximal transport rate in response to similar pH shifts (Romano et al., 2014; Verri et al., 2017).
The transport rate of SLC transporters at different temperatures has been shown to vary by species (Romano et al., 2014; Verri et al., 2017). Interestingly, teleost fish PepT1 proteins have higher transport rates at lower temperatures (22°C) than the mammalian PepT1 orthologs, which function better at temperatures around 30°C and higher. This possibly demonstrates an adaptative evolution between terrestrial and aquatic species living in different temperature regimens (Romano et al., 2014; Verri et al., 2017). The Antarctic icefish (Chionodraco hamatus) represents an extreme example, with structural changes to its PEPT1 protein facilitating in part its ability to function at very cold (−1.9°C) temperatures (Romano et al., 2014; Verri et al., 2017). In Delta smelt (Hypomesus transpacificus), the expression of the ion exchange transporter SLC8B1 was shown to have non-linear responses to increasing temperature, with decreased expression at 20°C and 25°C compared to the control (14°C) and re-elevated expression at 27°C (Jeffries et al., 2018). This u-shaped response was attributed to sublethal thermal threshold with partial downregulation of non-essential cellular processes during the period of stress.
If and to what extent ABC-type MDR/MXR transporters can be regulated by changes in salinity, temperature or pH is not well understood. For mammalian P-glycoprotein (ABCB1), a linear increase in transport rate with increasing temperature has been demonstrated (Litman et al., 1997; Lu et al., 2001; Clay and Sharom, 2013). The authors suggested that the increase in drug transport rate at higher temperatures can be attributed to several factors, including the increased partitioning of the drug/xenobiotic into the membrane and the structural changes in the protein that increase substrate affinity. Effects of other environmental stressors on the affinity and/or transport rate of ABC transporters are still elusive.
2.3 Chemical Inducers and Repressors
Multixenobiotic Resistance transporters represent a critical line of defense in preventing xenobiotic chemicals from accumulating and harming an aquatic organism. Initial investigations identifying MXR transporters as molecular targets of xenobiotics focused on chemical exposure experiments and subsequent evaluation of transporter mRNA and/or protein expression levels (Table 1). Thereby, transporter downregulation suggested a mechanism of direct inhibition or that upstream or downstream regulatory pathways might be targeted by the compound. Whereas upregulation of transporters indicated that they could be involved in the elimination of those compounds.
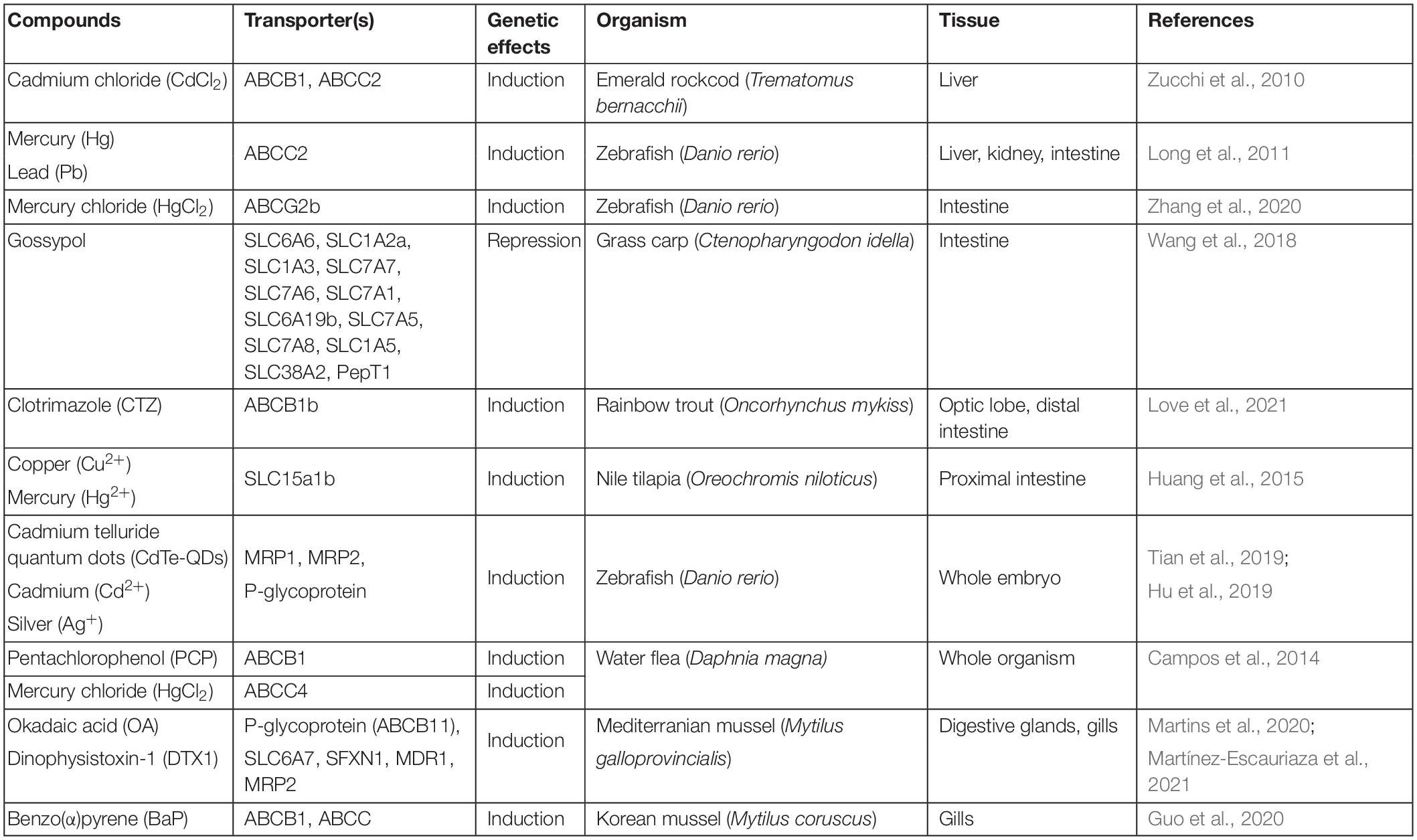
Table 1. List of tissue specific MXR transporter gene induction or repression by environmental chemicals, heavy metals, and natural toxins.
In rainbow trout, the expression of two P-glycoprotein isoforms, abcb1a and abcb1b, was significantly increased upon exposure to the antifungal agent clotrimazole (Love et al., 2021). In addition, several heavy metals were shown to induce the expression of ABC proteins and act as their substrates in fish. For instance, both cadmium and silver ions have been shown to induce pgp (ABCB4) and abcc2 expression in zebrafish embryos (Hu et al., 2019). Mercury and lead induced abcc2 expression in both larval and adult zebrafish, and the overexpression of abcc2 alleviated the accumulation of these metals along with cadmium (Long et al., 2011). In the Antarctic fish species Emerald rockcod (Trematomus bernacchii), the abcb1 and abcc2 genes are induced in the liver upon exposure to cadmium ions (Zucchi et al., 2010). Cadmium telluride quantum dots (CdTe-QDs) are nanoparticles that are often released into environmental water bodies and accumulate in aquatic biota. Zebrafish embryos exposed to CdTe quantum dots show delayed hatching and induce the expression of abcc1 and abcc2, indicating that quantum dots could be possible substrates for these ABCC-type transporters (Tian et al., 2019).
There has been limited studies on the inducing or repressing effects of xenobiotics on SLC transporter expression. In Nile tilapia (Oreochromis niloticus) the expression levels of peptide uptake transporters SLC15a1a, SLC15a1b, SLC15a2, and SLC15a5 decreased after fasting (Huang et al., 2015). Subsequent exposure to waterborne copper, but not mercury, prevented the restoration of the expression levels. In young grass carp (Ctenopharyngodon idella), the cotton derived phenol gossypol was shown to down-regulate several peptide and amino acid SLC transporters in the intestine, leading to intestinal damage and reduced growth (Wang et al., 2018). This is particularly important for aquaculture species where commercially available plant protein sources like rapeseed and cottonseed meal are used as cost effective feed (Deng et al., 2014). Unintentional exposure to gossypol in cottonseed meal could ultimately reduce feeding efficiency and overall percent weight gain.
3. Molecular Interactions of Xenobiotics With MXR Transporters
To date, the number of identified MXR transporter substrates and inhibitors among xeobiotics is low and assay protocols have yet to be standardized (Table 2; Nicklisch and Hamdoun, 2020). Still, several environmental chemicals, including pharmaceuticals, pesticides, herbicides, industrial chemicals, and polycyclic aromatic hydrocarbons (PAHs) have been shown to interact with and inhibit the function of MXR transporters in fish, bivalves, and crustaceans.
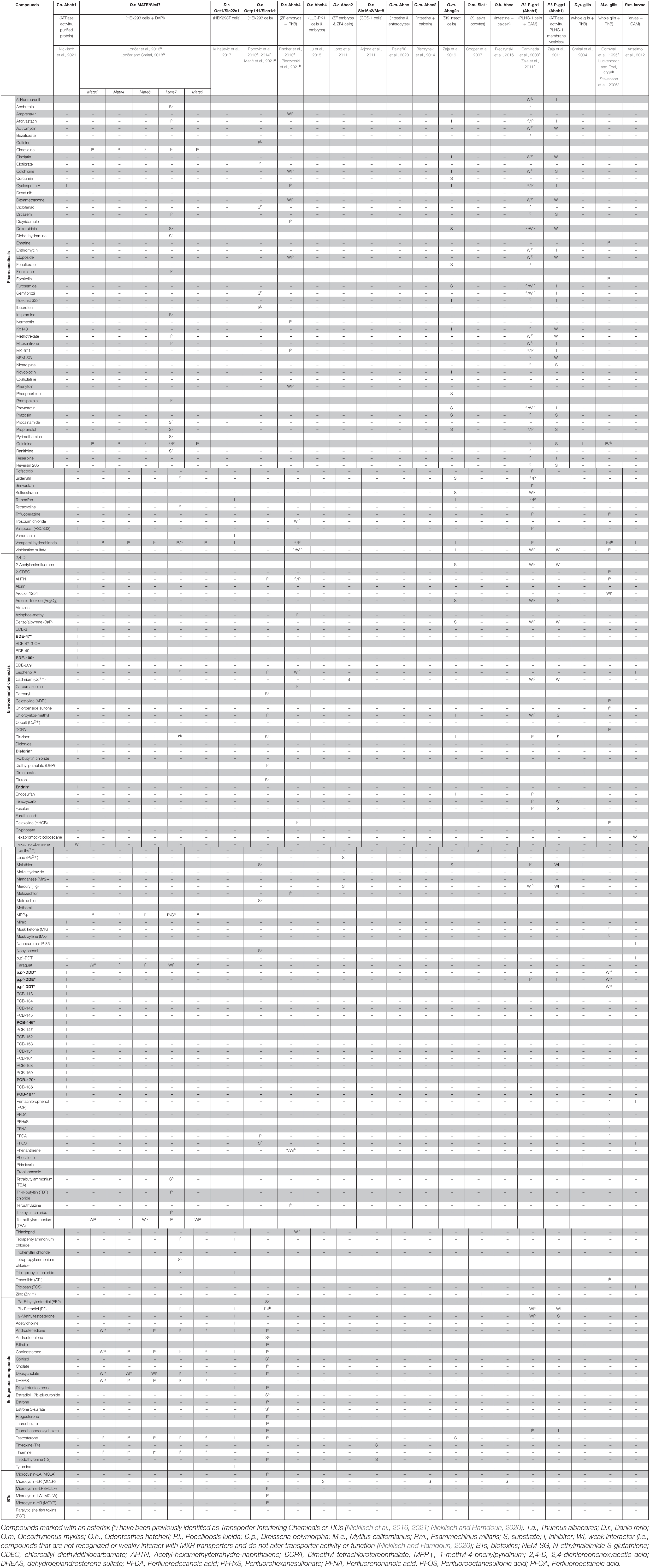
Table 2. Summary of known xenobiotic interactions with MXR transporters in fish and aquatic invertebrates.
For instance, in PLHC-1 (Poeciliopsis lucida hepatoma cells) the ABCB1 homolog P-gp1 was shown to be inhibited by a series of pharmaceuticals, with sildenafil and simvastatin showing highest inhibition potency (Caminada et al., 2008). In zebrafish, ABCB4 efflux function was inhibited by different environmental chemicals, including insecticides, fragrances, and pharmaceuticals (Fischer et al., 2013; Bieczynski et al., 2021). Divalent metals (Cd, Mn, Zn, Pb, and Co) have been shown to cause (competitive) inhibition of rainbow trout SLC11 iron uptake in Xenopus oocytes (Cooper et al., 2007). Using HEK293 cells, heterologously expressing zebrafish MATE7/SLC47a7 protein, interactions with 89 different environmental chemicals were investigated with the majority of compounds being inhibitors (Lončar and Smital, 2018). Similarly, the model substrate transport of zebrafish organic anion transporting polypeptide 1d1 (OATP1d1) and organic cation transporter 1 (OCT1) expressed in HEK293 cells was shown to be (competitively) inhibited by nearly all tested endo- and xenobiotics (Popovic et al., 2014; Mihaljević et al., 2017).
In bivalves, exposures to organic pesticides, pharmaceuticals, industrial chemicals, cadmium, and benzo(a)pyrene led to increased deformities, impaired growth, and increased toxicant accumulation. For instance, in the Californian mussel (Mytilus californianus), moderately hydrophobic pesticides and PFAS compounds have been shown to inhibit gill tissue efflux of Rhodamine B, a known P-glycoprotein substrate (Cornwall et al., 1995; McFadzen et al., 2000; Stevenson et al., 2006). In the thick shell mussel (Mytilus coruscus), gill tissue pre-exposure to benzo(α)pyrene (BaP) reduced the inhibitory effect of ABCB1- and ABCC1-specific inhibitors reversin 205 and MK572 on Calcein-AM efflux (Guo et al., 2020). This indicated that BaP could bind to both transporters and possible alter their detoxification function in these mussels.
Using mussels, sea urchin larvae, and the water flea Daphnia magna, it was shown that MXR transporter inhibition may also facilitate toxic synergism in environmental chemical mixtures (Faria et al., 2011; Anselmo et al., 2012; Campos et al., 2014). For instance, when early life stages of the green sea urchin (Psammechinus miliaris) were exposed to combinations of the toxic MXR transporter substrates vinblastine and triclosan (TCS) or P-85 nanoparticles, the toxicity increased by a factor of 2-8 (Anselmo et al., 2012). Similarly, when early life stages of the zebra mussel (Dreissena polymorpha) were exposed to a combination of vinblastine and model ABC transporter inhibitors, the toxicity increased super-additively (Faria et al., 2011).
Further, marine biotoxins and other naturally occurring marine products have been shown to upregulate ABC and SLC protein expression and function as substrates in various mussels species (Eufemia et al., 2002; Martins et al., 2020). In vitro and ex vivo experiments in rainbow trout and the Patagonian silverside (Odontesthes hatcheri) suggest that microcystin-LR may act as a substrate and competitive inhibitor of ABCC-like proteins in intestinal tissues (Bieczynski et al., 2014, 2016). Additional ex vivo and in vitro experiments in rainbow trout intestines demonstrated that paralytic shellfish toxin (PST) is capable of inhibiting ABCC-like transporters when absorbed by intestinal epithelial cells (Painefilú et al., 2020). The activity of ABC proteins can also be regulated by endogenous compounds. For instance, in the rectal salt glands of dogfish sharks (Squalus acanthias), ABCC2 activity was shown to be inhibited by the vasoconstrictor endothelin-1 via the ETB receptor and protein kinase C signaling (Miller et al., 2002).
4. Implications of Transporter Inhibition for Chemical Bioaccumulation and Nutrient Deficiencies
Many of the environmental chemicals that have been tested with MDR/MXR and nutrient uptake transporters were shown to be competitive substrates or inhibitors of the transporters in aquatic organisms. These so-called Transporter-Interfering Chemicals or TICs had been previously identified and shown to bind and inhibit mammalian and fish defense transporters, such as ABCB1 (Nicklisch et al., 2016, 2021; Nicklisch and Hamdoun, 2020). It has been hypothesized that the exact type of transporters also serve endogenous roles during development and for maintaining cellular and organismal homeostasis (Ahn and Nigam, 2009; Wu et al., 2011; Nigam, 2015, 2018). In addition, the known substrate overlaps and structural similarities between serval nutrient and MDR/MXR transporter subfamilies would likely make nutrient uptake systems another target for TICs (Kaler et al., 2007; Truong et al., 2008; Ahn and Nigam, 2009). This is critical since the inhibition of both xenobiotic defense systems and nutrient uptake transporters can potentiate detrimental effects on a developing aquatic organism. Nutrient deficient animals, specifically with vitamin deficiencies, have been shown to grow slower and suffer from other conditions such as hypoxia, neurological disorders, immunosuppression, and lower reproductive viability (Deng et al., 2014; Wang et al., 2016; Pan et al., 2017; Harder et al., 2018; Talukder Shefat, 2018). Likewise, the inhibition of xenobiotic efflux transporters will further promote the accumulation of toxic substrates that are otherwise eliminated.
Hence, contaminant-laden diets can represent a critical exposure pathway for fish to toxic environmental chemicals and natural compounds (Streit, 1998; Mackay and Fraser, 2000; Macdonald et al., 2002; Armitage et al., 2013; Mackay et al., 2018). This is particularly relevant in aquaculture, as it has been demonstrated that farmed fish can carry higher pollutant loads than wild caught fish (Hites et al., 2004a,b). Feed contamination is a prevalent issue and includes persistent organic pollutants, such as polybrominated diphenyl ethers (PBDEs) and polychlorinated biphenyls (PCBs), and heavy metals such as mercury, arsenic, cadmium, iron, and lead (Choi and Cech, 1998; Dórea, 2006; Kundu et al., 2017; Biancarosa et al., 2019; Li et al., 2019). While individual levels of these contaminants in fish feed or aquatic environments might be low, local tissue concentration in the digestive tract of these mostly hydrophobic compounds and the synergistic action of their mixtures can effectively act in concert to inhibit the protective efflux function of intestinal transport systems.
As such, it is important to both expand the panel of tested environmental chemicals and to include physiological transporters to evaluate the impacts on nutrient uptake, metabolism, and homeostasis. Furthermore, the transporter/chemical interaction assays need to be standardized and include co-exposures to chemical mixtures, representing environmental levels found in fish feed and/or the aquatic environment. Finally, given the differences in MDR/MXR transporter sequences between model and commercial fish species (Nicklisch et al., 2021), it is important to include MXR and nutrient transporters from commercial fish species to better predict and mitigate toxic chemical and heavy metal bioaccumulation fish and seafood species (Nicklisch et al., 2017a,b). Together, such studies will inform fish and shellfish advisories on guidelines for the selection of TIC-free aquaculture feed to develop safe eating guidelines.
Conclusion and Future Directions
To date only a handful of MDR/MXR transporters have been cloned and functionally characterized in fish and aquatic invertebrates. Among those, the least characterized MXR transporter superfamily are the solute carriers (SLCs). Transporter localization, gene expression levels and endogenous and xenobiotic substrate/inhibitor identification has mainly focused on two model organisms: zebrafish and rainbow trout. Furthermore, there have been only a few studies conducted on fish feed and dietary levels of contaminants and transporter-mediated uptake and bioaccumulation in commercial fish and other human-relevant aquaculture species. Finally, the extent to which other mutable environmental factors, such as salinity, temperature, and pH, may impair the levels and function of MXR and other nutrient transporters remains elusive. This perspective further highlights the urgent need to identify novel TICs, to determine their environmental levels in the natural diets and feeds of fish and other aquatic invertebrates, and to specify the transporter targets that regulate their uptake and disposition in aquatic organisms. Special emphasis should be placed on determining the interactions of TICs with protective MXR efflux transporters and essential nutrient uptake transporters lining the gills and intestinal epithelia to better predict toxic chemical accumulation and/or nutrient deprivation. The key strategies to mitigate toxicant and toxin accumulation in aquatic organisms include targeted chemical analysis of fish feed in aquaculture operations, a continuous biomonitoring of TIC levels in lower trophic level organisms that serve as prey or feed for commercial fish species and the development of regulatory guidelines that inform industrial chemical and agricultural pesticide management strategies to reduce or eliminate the use of environmentally persistent TIC compounds.
Data Availability Statement
The original contributions presented in the study are included in the article/supplementary material, further inquiries can be directed to the corresponding author/s.
Author Contributions
Both authors listed have made a substantial, direct, and intellectual contribution to the work, and approved it for publication.
Funding
This work of SCTN was supported by NIFA-USDA (CA-D-ETX-2526-H).
Conflict of Interest
The authors declare that the research was conducted in the absence of any commercial or financial relationships that could be construed as a potential conflict of interest.
Publisher’s Note
All claims expressed in this article are solely those of the authors and do not necessarily represent those of their affiliated organizations, or those of the publisher, the editors and the reviewers. Any product that may be evaluated in this article, or claim that may be made by its manufacturer, is not guaranteed or endorsed by the publisher.
References
Ahn, S. Y., and Nigam, S. K. (2009). Toward a systems level understanding of organic anion and other multispecific drug transporters: a remote sensing and signaling hypothesis. Mol. Pharmacol. 76, 481–490.
Almén, M. S., Nordström, K. J., Fredriksson, R., and Schiöth, H. B. (2009). Mapping the human membrane proteome: a majority of the human membrane proteins can be classified according to function and evolutionary origin. BMC Biol. 7:50. doi: 10.1186/1741-7007-7-50
Annilo, T., Chen, Z.-Q., Shulenin, S., Costantino, J., Thomas, L., Lou, H., et al. (2006). Evolution of the vertebrate ABC gene family: analysis of gene birth and death. Genomics 88, 1–11. doi: 10.1016/j.ygeno.2006.03.001
Anselmo, H. M. R., van den Berg, J. H. J., Rietjens, I. M. C. M., and Murk, A. J. (2012). Inhibition of cellular efflux pumps involved in multi xenobiotic resistance (MXR) in echinoid larvae as a possible mode of action for increased ecotoxicological risk of mixtures. Ecotoxicology 21, 2276–2287. doi: 10.1007/s10646-012-0984-2
Arjona, F. J., de Vrieze, E., Visser, T. J., Flik, G., and Klaren, P. H. M. (2011). Identification and functional characterization of zebrafish solute carrier Slc16a2 (Mct8) as a thyroid hormone membrane transporter. Endocrinology 152, 5065–5073. doi: 10.1210/en.2011-1166
Armitage, J. M., Arnot, J. A., Wania, F., and Mackay, D. (2013). Development and evaluation of a mechanistic bioconcentration model for ionogenic organic chemicals in fish. Environ. Toxicol. Chem. 32, 115–128. doi: 10.1002/etc.2020
Barat, A., Sahoo, P. K., Kumar, R., and Pande, V. (2016). Solute carriers (SLCs) identified and characterized from kidney transcriptome of golden mahseer (Tor putitora) (Fam: cyprinidae). Comparat. Biochem. Physiol. Part B: Biochem. Mol. Biol. 200, 54–61. doi: 10.1016/j.cbpb.2016.06.003
Bard, S. M. (2000). Multixenobiotic resistance as a cellular defense mechanism in aquatic organisms. Aquatic Toxicol. 48, 357–389. doi: 10.1016/S0166-445X(00)00088-6
Baumgarner, B. L., Bharadwaj, A. S., Inerowicz, D., Goodman, A. S., and Brown, P. B. (2013). Proteomic analysis of rainbow trout (Oncorhynchus mykiss) intestinal epithelia: physiological acclimation to short-term starvation. Comparat. Biochem. Physiol. Part D, Genomics Proteomics 8, 58–64. doi: 10.1016/j.cbd.2012.11.001
Beck, W. T. (1987). The cell biology of multiple drug resistance. Biochem. Pharmacol. 36, 2879–2887. doi: 10.1016/0006-2952(87)90198-5
Biancarosa, I., Sele, V., Belghit, I., Ørnsrud, R., Lock, E.-J., and Amlund, H. (2019). Replacing fish meal with insect meal in the diet of Atlantic salmon (Salmo salar) does not impact the amount of contaminants in the feed and it lowers accumulation of arsenic in the fillet. Food Additives Contaminants: Part A 36, 1191–1205. doi: 10.1080/19440049.2019.1619938
Bieczynski, F., Burkhardt-Medicke, K., Luquet, C. M., Scholz, S., and Luckenbach, T. (2021). Chemical effects on dye efflux activity in live zebrafish embryos and on zebrafish Abcb4 ATPase activity. FEBS Lett. 595, 828–843. doi: 10.1002/1873-3468.14015
Bieczynski, F., De Anna, J. S., Pirez, M., Brena, B. M., Villanueva, S. S. M., and Luquet, C. M. (2014). Cellular transport of microcystin-LR in rainbow trout (Oncorhynchus mykiss) across the intestinal wall: possible involvement of multidrug resistance-associated proteins. Aquatic Toxicol. (Amsterdam, Netherlands) 154, 97–106. doi: 10.1016/j.aquatox.2014.05.003
Bieczynski, F., Torres, W. D. C., Painefilu, J. C., Castro, J. M., Bianchi, V. A., Frontera, J. L., et al. (2016). Alterations in the intestine of Patagonian silverside (Odontesthes hatcheri) exposed to microcystin-LR: changes in the glycosylation pattern of the intestinal wall and inhibition of multidrug resistance proteins efflux activity. Aquatic Toxicol. 178, 106–117. doi: 10.1016/j.aquatox.2016.07.016
Borst, P., and Elferink, R. O. (2002). Mammalian ABC transporters in health and disease. Annu. Rev. Biochem. 71, 537–592. doi: 10.1146/annurev.biochem.71.102301.093055
Bruyere, A., Hubert, C., Le Vee, M., Chedik, L., Sayyed, K., Stieger, B., et al. (2017). Inhibition of SLC drug transporter activities by environmental bisphenols. Toxicol. Vitro: Int. J. Published Assoc. ith BIBRA 40, 34–44. doi: 10.1016/j.tiv.2016.12.009
Bucking, C., and Schulte, P. M. (2012). Environmental and nutritional regulation of expression and function of two peptide transporter (PepT1) isoforms in a euryhaline teleost. Comparat. Biochem. Physiol. Part A: Mol. Integrat. Physiol. 161, 379–387. doi: 10.1016/j.cbpa.2011.12.008
Caminada, D., Zaja, R., Smital, T., and Fent, K. (2008). Human pharmaceuticals modulate P-gp1 (ABCB1) transport activity in the fish cell line PLHC-1. Aquatic Toxicol. 90, 214–222. doi: 10.1016/j.aquatox.2008.08.013
Campos, B., Altenburger, R., Gómez, C., Lacorte, S., Piña, B., Barata, C., et al. (2014). First evidence for toxic defense based on the multixenobiotic resistance (MXR) mechanism in Daphnia magna. Aquatic Toxicol. 148, 139–151. doi: 10.1016/j.aquatox.2014.01.001
Carvalho, O. (2011). Comparative physiology of the respiratory system in the animal kingdom. Open Biol. J. 4, 35–46. doi: 10.2174/1874196701104010035
César-Razquin, A., Snijder, B., Frappier-Brinton, T., Isserlin, R., Gyimesi, G., Bai, X., et al. (2015). A call for systematic research on solute carriers. Cell 162, 478–487. doi: 10.1016/j.cell.2015.07.022
Chan, K. M., Davies, P. L., Childs, S., Veinot, L., and Ling, V. (1992). P-glycoprotein genes in the winter flounder, Pleuronectes americanus: isolation of two types of genomic clones carrying 3’ terminal exons. Biochim. Biophys. Acta 1171, 65–72. doi: 10.1016/0167-4781(92)90140-u
Chapman, H. W. (1997). Comparative physiology of the vertebrate digestive system, 2nd ed. Can. Vet. J. 38, 576–577.
Chedik, L., Bruyere, A., and Fardel, O. (2019). Interactions of organophosphorus pesticides with solute carrier (SLC) drug transporters. Xenobiotica 49, 363–374. doi: 10.1080/00498254.2018.1442030
Chen, K. G., Szakács, G., Annereau, J.-P., Rouzaud, F., Liang, X.-J., Valencia, J. C., et al. (2005). Principal expression of two mRNA isoforms (ABCB 5α and ABCB 5β) of the ATP-binding cassette transporter gene ABCB 5 in melanoma cells and melanocytes. Pigment Cell Res. 18, 102–112. doi: 10.1111/j.1600-0749.2005.00214.x
Choi, M. H., and Cech, J. J. Jr. (1998). Unexpectedly high mercury level in pelleted commercial fish feed. Environ. Toxicol. Chem. 17, 1979–1981. doi: 10.1002/etc.5620171013
Christine Paetzold, S., Ross, N. W., Richards, R. C., Jones, M., Hellou, J., and Bard, S. M. (2009). Up-regulation of hepatic ABCC2, ABCG2, CYP1A1 and GST in multixenobiotic-resistant killifish (Fundulus heteroclitus) from the Sydney Tar Ponds, Nova Scotia, Canada. Mar. Environ. Res. 68, 37–47. doi: 10.1016/j.marenvres.2009.04.002
Clay, A. T., and Sharom, F. J. (2013). Lipid bilayer properties control membrane partitioning, binding, and transport of P-Glycoprotein substrates. Biochemistry 52, 343–354. doi: 10.1021/bi301532c
Colas, C., Ung, P. M.-U., and Schlessinger, A. (2016). SLC transporters: structure, function, and drug discovery. MedChemComm (RSC Publishing) 7, 1069–1081. doi: 10.1039/C6MD00005C
Collinder, E., Björnhag, G., Cardona, M., Norin, E., Rehbinder, C., and Midtvedt, T. (2009). Gastrointestinal host-microbial interactions in mammals and fish: comparative studies in man, mice, rats, pigs, horses, cows, elk, reindeer, salmon and cod. Microb. Ecol. Health. Dis. 15, 66–78. doi: 10.1080/08910600310014980
Cooper, C. A., Shayeghi, M., Techau, M. E., Capdevila, D. M., MacKenzie, S., Durrant, C., et al. (2007). Analysis of the rainbow trout solute carrier 11 family reveals iron import ⩽ pH 7.4 and a functional isoform lacking transmembrane domains 11 and 12. FEBS Lett. 581, 2599–2604. doi: 10.1016/j.febslet.2007.04.081
Cornwall, R., Toomey, B. H., Bard, S., Bacon, C., Jarman, W. M., and Epel, D. (1995). Characterization of multixenobiotic/multidrug transport in the gills of the mussel Mytilus californianus and identification of environmental substrates. Aquatic Toxicol. 31, 277–296. doi: 10.1016/0166-445X(94)00070-7
Costa, J., Reis-Henriques, M. A., Castro, L. F. C., and Ferreira, M. (2012). Gene expression analysis of ABC efflux transporters, CYP1A and GSTα in Nile tilapia after exposure to benzo(a)pyrene. Comparat. Biochem. Physiol. Part C: Toxicol. Pharmacol. 155, 469–482. doi: 10.1016/j.cbpc.2011.12.004
Costa, J., Reis-Henriques, M. A., Wilson, J. M., and Ferreira, M. (2013). P-glycoprotein and CYP1A protein expression patterns in Nile tilapia (Oreochromis niloticus) tissues after waterborne exposure to benzo(a)pyrene (BaP). Environ. Toxicol. Pharmacol. 36, 611–625. doi: 10.1016/j.etap.2013.05.017
Dean, M., and Annilo, T. (2005). Evolution of the ATP-binding cassette (ABC) transporter superfamily in vertebrates. Annu. Rev. Genomics Hum. Genet. 6, 123–142. doi: 10.1146/annurev.genom.6.080604.162122
Dean, M., Hamon, Y., and Chimini, G. (2001). The human ATP-binding cassette (ABC) transporter superfamily. J. Lipid Res. 42, 1007–1017. doi: 10.1016/S0022-2275(20)31588-1
DeCourten, B. M., Connon, R. E., and Brander, S. M. (2019). Direct and indirect parental exposure to endocrine disruptors and elevated temperature influences gene expression across generations in a euryhaline model fish. PeerJ 7:e6156. doi: 10.7717/peerj.6156
Deng, J., Zhang, X., Long, X., Tao, L., Wang, Z., Niu, G., et al. (2014). Effects of dietary cholesterol supplementation on growth and cholesterol metabolism of rainbow trout (Oncorhynchus mykiss) fed diets with cottonseed meal or rapeseed meal. Fish. Physiol. Biochem. 40, 1827–1838. doi: 10.1007/s10695-014-9971-2
Derby, A. P., Fuller, N. W., Huff Hartz, K. E., Segarra, A., Connon, R. E., Brander, S. M., et al. (2021). Trophic transfer, bioaccumulation and transcriptomic effects of permethrin in inland silversides, Menidia beryllina, under future climate scenarios. Environ. Pollut. 275:116545. doi: 10.1016/j.envpol.2021.116545
Diaz de Cerio, O., Bilbao, E., Cajaraville, M. P., and Cancio, I. (2012). Regulation of xenobiotic transporter genes in liver and brain of juvenile thicklip grey mullets (Chelon labrosus) after exposure to Prestige-like fuel oil and to perfluorooctane sulfonate. Gene 498, 50–58. doi: 10.1016/j.gene.2012.01.067
Dietrich, C. G., Geier, A., and Oude Elferink, R. P. J. (2003). ABC of oral bioavailability: transporters as gatekeepers in the gut. Gut 52, 1788–1795.
Dórea, J. G. (2006). Fish meal in animal feed and human exposure to persistent bioaccumulative and toxic substances. J. Food Protect. 69, 2777–2785. doi: 10.4315/0362-028X-69.11.2777
Dymowska, A. K., Hwang, P.-P., and Goss, G. G. (2012). Structure and function of ionocytes in the freshwater fish gill. Respiratory Physiol. Neurobiol. 184, 282–292. doi: 10.1016/j.resp.2012.08.025
Epel, D., Luckenbach, T., Stevenson, C. N., Macmanus-Spencer, L. A., Hamdoun, A., and Smital, T. (2008). Efflux Transporters: newly appreciated roles in protection against pollutants. Environ. Sci. Technol. 42, 3914–3920.
Erickson, R. J., McKim, J. M., Lien, G. J., Hoffman, A. D., and Batterman, S. L. (2006). Uptake and elimination of ionizable organic chemicals at fish gills: II. Observed and predicted effects of pH, alkalinity, and chemical properties. Environ. Toxicol. Chem. 25, 1522–1532. doi: 10.1897/05-359R.1
Eufemia, N., Clerte, S., Girshick, S., and Epel, D. (2002). Algal products as naturally occurring substrates for p-glycoprotein in Mytilus californianus. Mar. Biol. 140, 343–353. doi: 10.1007/s002270100693
Faria, M., Navarro, A., Luckenbach, T., Piña, B., and Barata, C. (2011). Characterization of the multixenobiotic resistance (MXR) mechanism in embryos and larvae of the zebra mussel (Dreissena polymorpha) and studies on its role in tolerance to single and mixture combinations of toxicants. Aquatic Toxicol. 101, 78–87. doi: 10.1016/j.aquatox.2010.09.004
Felmlee, M. A., Jones, R. S., Rodriguez-Cruz, V., Follman, K. E., and Morris, M. E. (2020). Monocarboxylate transporters (SLC16): function, regulation, and role in health and disease. Pharmacol. Rev. 72, 466–485. doi: 10.1124/pr.119.018762
Ferreira, M., Costa, J., and Reis-Henriques, M. A. (2014). ABC transporters in fish species: a review. Front. Physiol. 5:266. doi: 10.3389/fphys.2014.00266
Fischer, S., Klüver, N., Burkhardt-Medicke, K., Pietsch, M., Schmidt, A.-M., Wellner, P., et al. (2013). Abcb4 acts as multixenobiotic transporter and active barrier against chemical uptake in zebrafish (Danio rerio) embryos. BMC Biol. 11:69. doi: 10.1186/1741-7007-11-69
Foyle, K. L., Hess, S., Powell, M. D., and Herbert, N. A. (2020). What is gill health and what is its role in marine finfish aquaculture in the face of a changing climate? Front. Mar. Sci. 7:400. doi: 10.3389/fmars.2020.00400
Frank, N. Y., Margaryan, A., Huang, Y., Schatton, T., Waaga-Gasser, A. M., Gasser, M., et al. (2005). ABCB5-mediated doxorubicin transport and chemoresistance in human malignant melanoma. Cancer Res. 65, 4320–4333. doi: 10.1158/0008-5472.CAN-04-3327
Fulton, C. A., Huff Hartz, K. E., Fuller, N. W., Kent, L. N., Anzalone, S. E., Miller, T. M., et al. (2021). Fitness costs of pesticide resistance in Hyalella azteca under future climate change scenarios. Sci. Total Environ. 753:141945. doi: 10.1016/j.scitotenv.2020.141945
Furness, J. B., Cottrell, J. J., and Bravo, D. M. (2015). Comparative gut physiology symposium: comparative physiology of digestion. J. Anim. Sci. 93, 485–491. doi: 10.2527/jas.2014-8481
Gabaldón, T., and Koonin, E. V. (2013). Functional and evolutionary implications of gene orthology. Nat. Rev. Genet. 14, 360–366. doi: 10.1038/nrg3456
Giacomini, K. M., Huang, S.-M., Tweedie, D. J., Benet, L. Z., Brouwer, K. L. R., Chu, X., et al. (2010). Membrane transporters in drug development. Nat. Rev. Drug Discov. 9, 215–236. doi: 10.1038/nrd3028
Glavinas, H., Krajcsi, P., Cserepes, J., and Sarkadi, B. (2004). The role of ABC transporters in drug resistance, metabolism and toxicity. Curr. Drug Delivery 1, 27–42. doi: 10.2174/1567201043480036
Gordon, W. E., Espinoza, J. A., Leerberg, D. M., Yelon, D., and Hamdoun, A. (2019). Xenobiotic transporter activity in zebrafish embryo ionocytes. Aquatic Toxicol. 212, 88–97. doi: 10.1016/j.aquatox.2019.04.013
Gottesman, M. M., Fojo, T., and Bates, S. E. (2002). Multidrug resistance in cancer: role of ATP-dependent transporters. Nat. Rev. Cancer 2, 48–58. doi: 10.1038/nrc706
Guéniche, N., Bruyere, A., Ringeval, M., Jouan, E., Huguet, A., Hégarat, L. L., et al. (2020). Differential interactions of carbamate pesticides with drug transporters. Xenobiotica 50, 1380–1392. doi: 10.1080/00498254.2020.1771473
Guo, B., Xu, Z., Yan, X., Buttino, I., Li, J., Zhou, C., et al. (2020). Novel ABCB1 and ABCC transporters are involved in the detoxification of Benzo(α)pyrene in thick shell mussel, mytilus coruscus. Front. Mar. Sci. 7:119. doi: 10.3389/fmars.2020.00119
Hamdoun, A. M., Cherr, G. N., Roepke, T. A., and Epel, D. (2004). Activation of multidrug efflux transporter activity at fertilization in sea urchin embryos (Strongylocentrotus purpuratus). Dev. Biol. 276, 452–462. doi: 10.1016/j.ydbio.2004.09.013
Harder, A. M., Ardren, W. R., Evans, A. N., Futia, M. H., Kraft, C. E., Marsden, J. E., et al. (2018). Thiamine deficiency in fishes: causes, consequences, and potential solutions. Rev. Fish Biol. Fisheries 28, 865–886. doi: 10.1007/s11160-018-9538-x
Hasenbein, S., Poynton, H., and Connon, R. E. (2018). Contaminant exposure effects in a changing climate: how multiple stressors can multiply exposure effects in the amphipod Hyalella azteca. Ecotoxicology (London, England) 27, 845–859. doi: 10.1007/s10646-018-1912-x
Hediger, M. A., Clémençon, B., Burrier, R. E., and Bruford, E. A. (2013). The ABCs of membrane transporters in health and disease (SLC series): introduction. Mol. Aspects Med. 34, 95–107. doi: 10.1016/j.mam.2012.12.009
Hediger, M. A., Romero, M. F., Peng, J.-B., Rolfs, A., Takanaga, H., and Bruford, E. A. (2004). The ABCs of solute carriers: physiological, pathological and therapeutic implications of human membrane transport proteins. Pflügers Archiv. 447, 465–468. doi: 10.1007/s00424-003-1192-y
Hillgren, K. M., Keppler, D., Zur, A. A., Giacomini, K. M., Stieger, B., Cass, C. E., et al. (2013). Emerging transporters of clinical importance: an update from the International Transporter Consortium. Clin. Pharmacol. Therapeutics 94, 52–63. doi: 10.1038/clpt.2013.74
Hites, R. A., Foran, J. A., Carpenter, D. O., Hamilton, M. C., Knuth, B. A., and Schwager, S. J. (2004a). Global assessment of organic contaminants in farmed salmon. Science 303, 226–229. doi: 10.1126/science.1091447
Hites, R. A., Foran, J. A., Schwager, S. J., Knuth, B. A., Hamilton, M. C., and Carpenter, D. O. (2004b). Global assessment of polybrominated diphenyl ethers in farmed and wild salmon. Environ. Sci. Technol. 38, 4945–4949. doi: 10.1021/es049548m
Höglund, P. J., Nordström, K. J. V., Schiöth, H. B., and Fredriksson, R. (2011). The solute carrier families have a remarkably long evolutionary history with the majority of the human families present before divergence of bilaterian species. Mol. Biol. Evol. 28, 1531–1541. doi: 10.1093/molbev/msq350
Horio, M., Gottesman, M. M., and Pastan, I. (1988). ATP-dependent transport of vinblastine in vesicles from human multidrug-resistant cells. Proc. Natl. Acad. Sci. U.S.A. 85, 3580–3584.
Hu, J., Tian, J., Zhang, F., Wang, H., and Yin, J. (2019). Pxr- and Nrf2- mediated induction of ABC transporters by heavy metal ions in zebrafish embryos. Environ. Pollut. 255:113329. doi: 10.1016/j.envpol.2019.113329
Huang, Q., Vera Delgado, J. M., Seni Pinoargote, O. D., and Llaguno, R. A. (2015). Molecular evolution of the Slc15 family and its response to waterborne copper and mercury exposure in tilapia. Aquatic Toxicol. 163, 140–147. doi: 10.1016/j.aquatox.2015.04.011
Hwang, P.-P., Lee, T.-H., and Lin, L.-Y. (2011). Ion regulation in fish gills: recent progress in the cellular and molecular mechanisms. Am. J. Physiol.-Regulatory Integrat. Comparat. Physiol. 301, R28–R47. doi: 10.1152/ajpregu.00047.2011
Ivanis, G., Esbaugh, A. J., and Perry, S. F. (2008). Branchial expression and localization of SLC9A2 and SLC9A3 sodium/hydrogen exchangers and their possible role in acid-base regulation in freshwater rainbow trout (Oncorhynchus mykiss). J. Exp. Biol. 211(Pt 15), 2467–2477. doi: 10.1242/jeb.017491
Jeffries, K. M., Connon, R. E., Verhille, C. E., Dabruzzi, T. F., Britton, M. T., Durbin-Johnson, B. P., et al. (2019). Divergent transcriptomic signatures in response to salinity exposure in two populations of an estuarine fish. Evolutionary Appl. 12, 1212–1226. doi: 10.1111/eva.12799
Jeffries, K. M., Fangue, N. A., and Connon, R. E. (2018). Multiple sub-lethal thresholds for cellular responses to thermal stressors in an estuarine fish. Comparat. Biochem. Physiol. Part A: Mol. Integrat. Physiol. 225, 33–45. doi: 10.1016/j.cbpa.2018.06.020
Jiang, Y., Zhang, S., Feng, S., Sun, J., and Xu, P. (2014). Genome wide identification, phylogeny and expression of zinc transporter genes in common carp. PLoS One 9:e116043. doi: 10.1371/journal.pone.0116043
Jonker, J. W., Stedman, C. A. M., Liddle, C., and Downes, M. (2009). Hepatobiliary ABC transporters: physiology, regulation and implications for disease. Front. Biosci. 4, 4904–4920. doi: 10.2741/3576
Juliano, R. L., and Ling, V. (1976). A surface glycoprotein modulating drug permeability in Chinese hamster ovary cell mutants. Biochim. Biophys. Acta (BBA) - Biomembranes 455, 152–162. doi: 10.1016/0005-2736(76)90160-7
Kaler, G., Truong, D. M., Khandelwal, A., Nagle, M., Eraly, S. A., Swaan, P. W., et al. (2007). Structural variation governs substrate specificity for Organic Anion Transporter (OAT) homologs: potential remote sensing by oat family members*. J. Biol. Chem. 282, 23841–23853. doi: 10.1074/jbc.M703467200
Karasov, W. H., and Douglas, A. E. (2013). Comparative digestive physiology. Comprehensive Physiol. 3, 741–783. doi: 10.1002/cphy.c110054
Kiela, P. R., and Ghishan, F. K. (2016). Physiology of intestinal absorption and secretion. Best Pract. Res. Clin. Gastroenterol. 30, 145–159. doi: 10.1016/j.bpg.2016.02.007
Kim, Y.-I., Nam, I.-K., Lee, D.-K., Bhandari, S., Charton, L., Kwak, S., et al. (2020). Slc25a17 acts as a peroxisomal coenzyme A transporter and regulates multiorgan development in zebrafish. J. Cell. Physiol. 235, 151–165. doi: 10.1002/jcp.28954
Komoroske, L. M., Jeffries, K. M., Whitehead, A., Roach, J. L., Britton, M., Connon, R. E., et al. (2021). Transcriptional flexibility during thermal challenge corresponds with expanded thermal tolerance in an invasive compared to native fish. Evolutionary Appl. 14, 931–949. doi: 10.1111/eva.13172
König, J., Müller, F., and Fromm, M. F. (2013). Transporters and drug-drug interactions: important determinants of drug disposition and effects. Pharmacol. Rev. 65, 944–966. doi: 10.1124/pr.113.007518
Kortner, T. M., Skugor, S., Penn, M. H., Mydland, L. T., Djordjevic, B., Hillestad, M., et al. (2012). Dietary soyasaponin supplementation to pea protein concentrate reveals nutrigenomic interactions underlying enteropathy in Atlantic salmon (Salmo salar). BMC Vet. Res. 8:101. doi: 10.1186/1746-6148-8-101
Kottra, G., and Daniel, H. (2001). Bidirectional electrogenic transport of peptides by the proton-coupled carrier PEPT1 in Xenopus laevis oocytes: its asymmetry and symmetry. J. Physiol. 536, 495–503. doi: 10.1111/j.1469-7793.2001.0495c.xd
Kottra, G., Stamfort, A., and Daniel, H. (2002). PEPT1 as a paradigm for membrane carriers that mediate electrogenic bidirectional transport of anionic, cationic, and neutral substrates *. J. Biol. Chem. 277, 32683–32691. doi: 10.1074/jbc.M204192200
Kropf, C., Fent, K., Fischer, S., Casanova, A., and Segner, H. (2020). ABC transporters in gills of rainbow trout (Oncorhynchus mykiss). J. Exp. Biol. 223:jeb221069.
Kropf, C., Segner, H., and Fent, K. (2016). ABC transporters and xenobiotic defense systems in early life stages of rainbow trout (Oncorhynchus mykiss). Comparat. Biochem. Physiol. Part C: Toxicol. Pharmacol. 18, 45–56. doi: 10.1016/j.cbpc.2016.02.006
Kundu, G. K., Alauddin, M., Akter, M. S., Khan, M. S., Islam, M. M., Mondal, G., et al. (2017). Metal contamination of commercial fish feed and quality aspects of farmed tilapia (Oreochromis niloticus) in Bangladesh. Biores. Commun.-(BRC) 3, 345–353.
Kurelec, B. (1992). The multixenobiotic resistance mechanism in aquatic organisms. Crit. Rev. Toxicol. 22, 23–43. doi: 10.3109/10408449209145320
Kurelec, B. (1997). A new type of hazardous chemical: the chemosensitizers of multixenobiotic resistance. Environ. Health Perspect. 105(Suppl. 4), 855–860. doi: 10.1289/ehp.97105s4855
Kurelec, B., Lucić, D., Pivćević, B., and Krća, S. (1995). Induction and reversion of multixenobiotic resistance in the marine snail Monodonta turbinata. Mar. Biol. 123, 305–312. doi: 10.1007/BF00353622
Kwong, R. W. M., Wang, W.-X., Lam, P. K. S., and Yu, P. K. N. (2006). The uptake, distribution and elimination of paralytic shellfish toxins in mussels and fish exposed to toxic dinoflagellates. Aquatic Toxicol. 80, 82–91. doi: 10.1016/j.aquatox.2006.07.016
Leslie, E. M., Deeley, R. G., and Cole, S. P. C. (2005). Multidrug resistance proteins: role of P-glycoprotein, MRP1, MRP2, and BCRP (ABCG2) in tissue defense. Toxicol. Appl. Pharmacol. 204, 216–237. doi: 10.1016/j.taap.2004.10.012
Li, X., Dong, S., Wang, P., Su, X., and Fu, J. (2019). Polychlorinated biphenyls are still alarming persistent organic pollutants in marine-origin animal feed (fishmeal). Chemosphere 233, 355–362. doi: 10.1016/j.chemosphere.2019.05.250
Lin, L., Yee, S. W., Kim, R. B., and Giacomini, K. M. (2015). SLC transporters as therapeutic targets: emerging opportunities. Nat. Rev. Drug Discov. 14, 543–560. doi: 10.1038/nrd4626
Ling, V. (1997). Multidrug resistance: molecular mechanisms and clinical relevance. Cancer Chemother. Pharmacol. 40, S3–S8. doi: 10.1007/s002800051053
Litman, T., Zeuthen, T., Skovsgaard, T., and Stein, W. D. (1997). Structure-activity relationships of P-glycoprotein interacting drugs: kinetic characterization of their effects on ATPase activity. Biochim. Biophys. Acta (BBA) - Mol. Basis Dis. 1361, 159–168. doi: 10.1016/S0925-4439(97)00026-4
Liu, F., Xia, W., Hu, J., Wang, Y., Yang, F., Sun, S., et al. (2015). Solute carrier family 26 member a2 (slc26a2) regulates otic development and hair cell survival in zebrafish. PLoS One 10:e0136832. doi: 10.1371/journal.pone.0136832
Liu, S., Li, Q., and Liu, Z. (2013). Genome-wide identification, characterization and phylogenetic analysis of 50 Catfish ATP-Binding Cassette (ABC) transporter genes. PLoS One 8:e63895. doi: 10.1371/journal.pone.0063895
Lončar, J., Popović, M., Krznar, P., Zaja, R., and Smital, T. (2016). The first characterization of multidrug and toxin extrusion (MATE/SLC47) proteins in zebrafish (Danio rerio). Sci. Rep. 6:28937. doi: 10.1038/srep28937
Lončar, J., Popović, M., Zaja, R., and Smital, T. (2010). Gene expression analysis of the ABC efflux transporters in rainbow trout (Oncorhynchus mykiss). Comparat. Biochem. Physiol. Part C: Toxicol. Pharmacol. 151, 209–215. doi: 10.1016/j.cbpc.2009.10.009
Lončar, J., and Smital, T. (2018). Interaction of environmental contaminants with zebrafish (Danio rerio) multidrug and toxin extrusion protein 7 (Mate7/Slc47a7). Aquatic Toxicol. 205, 193–203. doi: 10.1016/j.aquatox.2018.10.016
Long, Y., Li, Q., Zhong, S., Wang, Y., and Cui, Z. (2011). Molecular characterization and functions of zebrafish ABCC2 in cellular efflux of heavy metals. Comparative Biochem. Physiol. Toxicol. Pharmacol.: CBP 153, 381–391. doi: 10.1016/j.cbpc.2011.01.002
Love, R. C., Osachoff, H. L., and Kennedy, C. J. (2021). Short communication: tissue-specific transcript expression of P-glycoprotein isoforms abcb1a and abcb1b in rainbow trout (Oncorhynchus mykiss) following induction with clotrimazole. Comparat. Biochem. Physiol. Part B, Biochem. Mol. Biol. 252:110538. doi: 10.1016/j.cbpb.2020.110538
Lu, P., Liu, R., and Sharom, F. J. (2001). Drug transport by reconstituted P-glycoprotein in proteoliposomes. Eur. J. Biochem. 268, 1687–1697. doi: 10.1046/j.1432-1327.2001.02041.x
Lu, X., Long, Y., Sun, R., Zhou, B., Lin, L., Zhong, S., et al. (2015). Zebrafish Abcb4 is a potential efflux transporter of microcystin-LR. Comp. Biochem. Physiol. C Toxicol. Pharmacol. 167, 35–42. doi: 10.1016/j.cbpc.2014.08.005
Luckenbach, T., and Epel, D. (2005). Nitromusk and polycyclic musk compounds as long-term inhibitors of cellular xenobiotic defense systems mediated by multidrug transporters. Environ. Health Perspect. 113, 17–24. doi: 10.1289/ehp.7301
Luckenbach, T., and Epel, D. (2008). ABCB- and ABCC-type transporters confer multixenobiotic resistance and form an environment-tissue barrier in bivalve gills. Am. J. Physiol.-Regulat. Integrat. Comparat. Physiol. 294, R1919–R1929. doi: 10.1152/ajpregu.00563.2007
Luckenbach, T., Fischer, S., and Sturm, A. (2014). Current advances on ABC drug transporters in fish. Comparat. Biochem. Physiol. Part C: Toxicol. Pharmacol. 165, 28–52. doi: 10.1016/j.cbpc.2014.05.002
Macdonald, R., Mackay, D., and Hickie, B. (2002). Contaminant amplification in the environment. Environ. Sci. Technol. 36, 456A–462A. doi: 10.1021/es022470u
Mackay, D., Celsie, A. K. D., Powell, D. E., and Parnis, J. M. (2018). Bioconcentration, bioaccumulation, biomagnification and trophic magnification: a modelling perspective. Environ. Sci.: Processes Impacts 20, 72–85. doi: 10.1039/C7EM00485K
Mackay, D., and Fraser, A. (2000). Bioaccumulation of persistent organic chemicals: mechanisms and models. Environ. Pollut. 110, 375–391. doi: 10.1016/S0269-7491(00)00162-7
Maetz, J., and García Romeu, F. (1964). The mechanism of sodium and chloride uptake by the gills of a fresh-water fish, carassius auratus: II. Evidence for NH4+ / NA+ and HCO3- / Cl- exchanges. J. Gen. Physiol. 47, 1209–1227. doi: 10.1085/jgp.47.6.1209
Margheritis, E., Terova, G., Oyadeyi, A. S., Renna, M. D., Cinquetti, R., Peres, A., et al. (2013). Characterization of the transport of lysine-containing dipeptides by PepT1 orthologs expressed in Xenopus laevis oocytes. Comparat. Biochem. Physiol. Part A: Mol. Integrat. Physiol. 164, 520–528. doi: 10.1016/j.cbpa.2012.12.016
Marić, P., Ahel, M. Maraković, N., Lončar, J. Mihaljević, I., and Smital, T. (2021). Selective interaction of microcystin congeners with zebrafish (Danio rerio) Oatp1d1 transporter. Chemosphere 283:131155. doi: 10.1016/j.chemosphere.2021.131155
Martínez-Escauriaza, R., Lozano, V., Pérez-Parallé, M. L., Blanco, J., Sánchez, J. L., and Pazos, A. J. (2021). Expression analyses of genes related to multixenobiotic resistance in mytilus galloprovincialis after exposure to okadaic acid-producing dinophysis acuminata. Toxins 13:614. doi: 10.3390/toxins13090614
Martins, J. C., Domínguez-Pérez, D., Azevedo, C., Braga, A. C., Costa, P. R., Osório, H., et al. (2020). Molecular responses of mussel mytilus galloprovincialis associated to accumulation and depuration of marine biotoxins okadaic acid and Dinophysistoxin-1 revealed by shotgun proteomics. Front. Mar. Sci. 7:589822. doi: 10.3389/fmars.2020.589822
McFadzen, I., Eufemia, N., Heath, C., Epel, D., Moore, M., and Lowe, D. (2000). Multidrug resistance in the embryos and larvae of the mussel Mytilus edulis. Mar. Environ. Res. 50, 319–323. doi: 10.1016/S0141-1136(00)00057-X
Mihaljević, I., Popović, M., Žaja, R., Maraković, N., Šinko, G., and Smital, T. (2017). Interaction between the zebrafish (Danio rerio) organic cation transporter 1 (Oct1) and endo- and xenobiotics. Aquatic Toxicol. 187, 18–28. doi: 10.1016/j.aquatox.2017.03.012
Mihaljevic, I., Popovic, M., Zaja, R., and Smital, T. (2016). Phylogenetic, syntenic, and tissue expression analysis of slc22 genes in zebrafish (Danio rerio). BMC Genomics 17:626. doi: 10.1186/s12864-016-2981-y
Miller, D. S., Masereeuw, R., and Karnaky, K. J. (2002). Regulation of MRP2-mediated transport in shark rectal salt gland tubules. Am. J. Physiol. Regulat. Integrat. Comparat. Physiol. 282, R774–R781. doi: 10.1152/ajpregu.00333.2001
Moreira, R., Pereiro, P., Canchaya, C., Posada, D., Figueras, A., and Novoa, B. (2015). RNA-Seq in Mytilus galloprovincialis: comparative transcriptomics and expression profiles among different tissues. BMC Genomics 16:728. doi: 10.1186/s12864-015-1817-5
Müller, J., Keiser, M., Drozdzik, M., and Oswald, S. (2017). Expression, regulation and function of intestinal drug transporters: an update. Biol. Chem. 398, 175–192. doi: 10.1515/hsz-2016-0259
Mundy, P. C., Jeffries, K. M., Fangue, N. A., and Connon, R. E. (2020). Differential regulation of select osmoregulatory genes and Na+/K+-ATPase paralogs may contribute to population differences in salinity tolerance in a semi-anadromous fish. Comparat. Biochem. Physiol. Part A: Mol. Integrat. Physiol. 240:110584. doi: 10.1016/j.cbpa.2019.110584
Muzzio, A. M., Noyes, P. D., Stapleton, H. M., and Lema, S. C. (2014). Tissue distribution and thyroid hormone effects on mRNA abundance for membrane transporters Mct8, Mct10, and organic anion-transporting polypeptides (Oatps) in a teleost fish. Comparat. Biochem. Physiol. Part A: Mol. Integrat. Physiol. 167, 77–89. doi: 10.1016/j.cbpa.2013.09.019
Nadella, S. R., Grosell, M., and Wood, C. M. (2007). Mechanisms of dietary Cu uptake in freshwater rainbow trout: evidence for Na-assisted Cu transport and a specific metal carrier in the intestine. J. Comparat. Physiol. B, Biochem. Syst. Environ. Physiol. 177, 433–446. doi: 10.1007/s00360-006-0142-3
Nicklisch, S. C. T., Bonito, L. T., Sandin, S., and Hamdoun, A. (2017a). Geographic differences in persistent organic pollutant levels of yellowfin tuna. Environ. Health Perspect. 125:067014. doi: 10.1289/EHP518
Nicklisch, S. C. T., Bonito, L. T., Sandin, S., and Hamdoun, A. (2017b). Mercury levels of yellowfin tuna (Thunnus albacares) are associated with capture location. Environ. Pollut. 229, 87–93. doi: 10.1016/j.envpol.2017.05.070
Nicklisch, S. C. T., and Hamdoun, A. (2020). Disruption of small molecule transporter systems by Transporter-Interfering Chemicals (TICs). FEBS Lett. 594, 4158–4185. doi: 10.1002/1873-3468.14005
Nicklisch, S. C. T., Pouv, A. K., Rees, S. D., McGrath, A. P., Chang, G., and Hamdoun, A. (2021). Transporter-interfering chemicals inhibit P-glycoprotein of yellowfin tuna (Thunnus albacares). Comparat. Biochem. Physiol. Part C: Toxicol. Pharmacol. 248:109101. doi: 10.1016/j.cbpc.2021.109101
Nicklisch, S. C. T., Rees, S. D., McGrath, A. P., Gökirmak, T., Bonito, L. T., Vermeer, L. M., et al. (2016). Global marine pollutants inhibit P-glycoprotein: environmental levels, inhibitory effects, and cocrystal structure. Sci. Adv. 2:e1600001. doi: 10.1126/sciadv.1600001
Nigam, S. K. (2015). What do drug transporters really do? Nat. Rev. Drug Discov. 14, 29–44. doi: 10.1038/nrd4461
Nigam, S. K. (2018). The SLC22 transporter family: a paradigm for the impact of drug transporters on metabolic pathways, signaling, and disease. Annu. Rev. Pharmacol. Toxicol. 58, 663–687. doi: 10.1146/annurev-pharmtox-010617-052713
Noyes, P. D., McElwee, M. K., Miller, H. D., Clark, B. W., Van Tiem, L. A., Walcott, K. C., et al. (2009). The toxicology of climate change: environmental contaminants in a warming world. Environ. Int. 35, 971–986. doi: 10.1016/j.envint.2009.02.006
Oosterhuis, B., Vukman, K., Vági, E., Glavinas, H., Jablonkai, I., and Krajcsi, P. (2008). Specific interactions of chloroacetanilide herbicides with human ABC transporter proteins. Toxicology 248, 45–51. doi: 10.1016/j.tox.2008.03.003
Painefilú, J. C., Bianchi, V. A., Krock, B., De Anna, J. S., Kristoff, G., and Luquet, C. M. (2020). Effects of paralytic shellfish toxins on the middle intestine of Oncorhynchus mykiss: glutathione metabolism, oxidative status, lysosomal function and ATP-binding cassette class C (ABCC) proteins activity. Ecotoxicol. Environ. Safety 204:111069. doi: 10.1016/j.ecoenv.2020.111069
Pan, J.-H., Feng, L., Jiang, W.-D., Wu, P., Kuang, S.-Y., Tang, L., et al. (2017). Vitamin E deficiency depressed fish growth, disease resistance, and the immunity and structural integrity of immune organs in grass carp (Ctenopharyngodon idella): referring to NF-κB, TOR and Nrf2 signaling. Fish Shellfish Immunol. 60, 219–236. doi: 10.1016/j.fsi.2016.11.044
Paul, T., Shukla, S. P., Kumar, K., Poojary, N., Manickavasagam, S., and Kumar, S. (2020). Effects of temperature and pH on acute toxicity of triclosan in pangasianodon hypophthalmus (Sauvage, 1878). Proc. Natl. Acad. Sci. India Section B: Biol. Sci. 90, 677–685. doi: 10.1007/s40011-019-01143-4
Pizzagalli, M. D., Bensimon, A., and Superti-Furga, G. (2021). A guide to plasma membrane solute carrier proteins. FEBS J. 288, 2784–2835. doi: 10.1111/febs.15531
Planchamp, C., Hadengue, A., Stieger, B., Bourquin, J., Vonlaufen, A., Frossard, J.-L., et al. (2007). Function of both sinusoidal and canalicular transporters controls the concentration of organic anions within hepatocytes. Mol. Pharmacol. 71, 1089–1097. doi: 10.1124/mol.106.030759
Popovic, M., Zaja, R., Fent, K., and Smital, T. (2013). Molecular characterization of zebrafish Oatp1d1 (Slco1d1), a novel organic anion-transporting polypeptide. J. Biol. Chem. 288, 33894–33911. doi: 10.1074/jbc.M113.518506
Popovic, M., Zaja, R., Fent, K., and Smital, T. (2014). Interaction of environmental contaminants with zebrafish organic anion transporting polypeptide, Oatp1d1 (Slco1d1). Toxicol. Appl. Pharmacol. 280, 149–158. doi: 10.1016/j.taap.2014.07.015
Popovic, M., Zaja, R., Loncar, J., and Smital, T. (2010b). A novel ABC transporter: the first insight into zebrafish (Danio rerio) ABCH1. Mar. Environ. Res. 69, S11–S13. doi: 10.1016/j.marenvres.2009.10.016
Popovic, M., Zaja, R., and Smital, T. (2010a). Organic anion transporting polypeptides (OATP) in zebrafish (Danio rerio): phylogenetic analysis and tissue distribution. Comparat. Biochem. Physiol. Part A, Mol. Integrat. Physiol. 155, 327–335. doi: 10.1016/j.cbpa.2009.11.011
Protopopova, M. V., Pavlichenko, V. V., and Luckenbach, T. (2020). Changes of cellular stress response related hsp70 and abcb1 transcript and Hsp70 protein levels in Siberian freshwater amphipods upon exposure to cadmium chloride in the lethal concentration range. PeerJ 8:e8635. doi: 10.7717/peerj.8635
Ray, A. K., and Ringø, E. (2014). “The gastrointestinal tract of fish,” in Aquaculture Nutrition, eds D. Merrifield and E. Ringo (Hoboken, NJ: John Wiley & Sons, Ltd), 1–13.
Riisgård, H. U., Egede, P. P., and Barreiro Saavedra, I. (2011). Feeding behaviour of the mussel, mytilus edulis: new observations, with a minireview of current knowledge. J. Mar. Biol. 2011:e312459. doi: 10.1155/2011/312459
Riisgård, H. U., Funch, P., and Larsen, P. S. (2015). The mussel filter–pump – present understanding, with a re-examination of gill preparations. Acta Zool. 96, 273–282. doi: 10.1111/azo.12110
Rimoldi, S., Bossi, E., Harpaz, S., Cattaneo, A. G., Bernardini, G., Saroglia, M., et al. (2015). Intestinal B0AT1 (SLC6A19) and PEPT1 (SLC15A1) mRNA levels in European sea bass (Dicentrarchus labrax) reared in fresh water and fed fish and plant protein sources. J. Nutr. Sci. 4:e21. doi: 10.1017/jns.2015.9
Romano, A., Barca, A., Storelli, C., and Verri, T. (2014). Teleost fish models in membrane transport research: the PEPT1(SLC15A1) H+–oligopeptide transporter as a case study. J. Physiol. 592(Pt 5), 881–897. doi: 10.1113/jphysiol.2013.259622
Romano, A., Kottra, G., Barca, A., Tiso, N., Maffia, M., Argenton, F., et al. (2006). High-affinity peptide transporter PEPT2 (SLC15A2) of the zebrafish Danio rerio: functional properties, genomic organization, and expression analysis. Physiol. Genomics 24, 207–217. doi: 10.1152/physiolgenomics.00227.2005
Romney, A. L. T., Yanagitsuru, Y. R., Mundy, P. C., Fangue, N. A., Hung, T.-C., Brander, S. M., et al. (2019). Developmental staging and salinity tolerance in embryos of the delta smelt, Hypomesus transpacificus. Aquaculture 511:634191. doi: 10.1016/j.aquaculture.2019.06.005
Ross, E., and Behringer, D. (2019). Changes in temperature, pH, and salinity affect the sheltering responses of Caribbean spiny lobsters to chemosensory cues. Sci. Rep. 9:4375. doi: 10.1038/s41598-019-40832-y
Said, H. M., and Nexo, E. (2018). “Chapter 54 - intestinal absorption of water-soluble vitamins: cellular and molecular mechanisms,” in Physiology of the Gastrointestinal Tract, 6th Edn, ed. H. M. Said (Cambridge, MA: Academic Press), 1201–1248.
Schlessinger, A., Yee, S. W., Sali, A., and Giacomini, K. M. (2013). SLC classification: an update. Clin. Pharmacol. Therapeutics 94, 19–23. doi: 10.1038/clpt.2013.73
Schoels, M., Zhuang, M., Fahrner, A., Küchlin, S., Sagar, Franz, H., et al. (2021). Single-cell mRNA profiling reveals changes in solute carrier expression and suggests a metabolic switch during zebrafish pronephros development. Am. J. Physiol.-Renal Physiol. 320, F826–F837. doi: 10.1152/ajprenal.00610.2020
Segarra, A., Mauduit, F., Amer, N. R., Biefel, F., Hladik, M. L., Connon, R. E., et al. (2021). Salinity changes the dynamics of pyrethroid toxicity in terms of behavioral effects on newly hatched delta smelt larvae. Toxics 9:40. doi: 10.3390/toxics9020040
Sharom, F. J. (2008). ABC multidrug transporters: structure, function and role in chemoresistance. Pharmacogenomics 9, 105–127. doi: 10.2217/14622416.9.1.105
Smital, T., and Kurelec, B. (1998). The chemosensitizers of multixenobiotic resistance mechanism in aquatic invertebrates: a new class of pollutants. Mutation Res. 399, 43–53. doi: 10.1016/s0027-5107(97)00265-0
Smital, T., Luckenbach, T., Sauerborn, R., Hamdoun, A. M., Vega, R. L., and Epel, D. (2004). Emerging contaminants—pesticides, PPCPs, microbial degradation products and natural substances as inhibitors of multixenobiotic defense in aquatic organisms. Mutat. Res. 552, 101–117. doi: 10.1016/j.mrfmmm.2004.06.006
Smital, T., and Sauerborn, R. (2002). Measurement of the activity of multixenobiotic resistance mechanism in the common carp Cyprinus carpio. Mar. Environ. Res. 54, 449–453. doi: 10.1016/S0141-1136(02)00155-1
Smital, T., Sauerborn, R., Pivcević, B., Krca, S., and Kurelec, B. (2000). Interspecies differences in P-glycoprotein mediated activity of multixenobiotic resistance mechanism in several marine and freshwater invertebrates. Comparat. Biochem. Physiol. Toxicol. Pharmacol.: CBP 126, 175–186. doi: 10.1016/s0742-8413(00)00110-9
Song, F., Xu, D., Zhou, H., Xu, W., Mai, K., and He, G. (2017). The differences in postprandial free amino acid concentrations and the gene expression of PepT1 and amino acid transporters after fishmeal partial replacement by meat and bone meal in juvenile turbot (Scophthalmus maximus L.). Aquaculture Res. 48, 3766–3781. doi: 10.1111/are.13203
Song, W., Li, D., Tao, L., Luo, Q., and Chen, L. (2020). Solute carrier transporters: the metabolic gatekeepers of immune cells. Acta Pharmaceutica Sin. B 10, 61–78. doi: 10.1016/j.apsb.2019.12.006
Staud, F., Cerveny, L., Ahmadimoghaddam, D., and Ceckova, M. (2013). Multidrug and toxin extrusion proteins (MATE/SLC47); role in pharmacokinetics. Int. J. Biochem. Cell Biol. 45, 2007–2011. doi: 10.1016/j.biocel.2013.06.022
Stevenson, C. N., MacManus-Spencer, L. A., Luckenbach, T., Luthy, R. G., and Epel, D. (2006). New perspectives on perfluorochemical ecotoxicology: inhibition and induction of an efflux transporter in the marine mussel, mytilus californianus. Environ. Sci. Technol. 40, 5580–5585. doi: 10.1021/es0602593
Streit, B. (1998). “Bioaccumulation of contaminants in fish,” in Fish Ecotoxicology, eds T. Braunbeck, D. E. Hinton, and B. Streit (Basel: Birkhäuser).
Sundell, K. S., and Rønnestad, I. (2011). “integrated function and control of the gut | intestinal absorption,” in Encyclopedia of Fish Physiology, ed. A. P. Farrell (Cambridge, MA: Academic Press), 1311–1321.
Takesono, A., Moger, J., Farooq, S., Cartwright, E., Dawid, I. B., Wilson, S. W., et al. (2012). Solute carrier family 3 member 2 (Slc3a2) controls yolk syncytial layer (YSL) formation by regulating microtubule networks in the zebrafish embryo. Proc. Natl. Acad. Sci. U.S.A. 109, 3371–3376. doi: 10.1073/pnas.1200642109
Talukder Shefat, S. H. (2018). Nutritional fish disease and public health concern. Poultry Fisheries Wildlife Sci. 6:199. doi: 10.4172/2375-446X.1000199
Tian, J., Hu, J., Liu, G., Yin, H., Chen, M., Miao, P., et al. (2019). Altered Gene expression of ABC transporters, nuclear receptors and oxidative stress signaling in zebrafish embryos exposed to CdTe quantum dots. Environ. Pollut. 244, 588–599. doi: 10.1016/j.envpol.2018.10.092
To, V. P. T. H., Masagounder, K., and Loewen, M. E. (2019). SLC transporters ASCT2, B0AT1-like, y+LAT1, and LAT4-like associate with methionine electrogenic and radio-isotope flux kinetics in rainbow trout intestine. Physiol. Rep. 7:e14274. doi: 10.14814/phy2.14274
Truong, D. M., Kaler, G., Khandelwal, A., Swaan, P. W., and Nigam, S. K. (2008). Multi-level analysis of organic anion transporters 1, 3, and 6 reveals major differences in structural determinants of antiviral discrimination*. J. Biol. Chem. 283, 8654–8663. doi: 10.1074/jbc.M708615200
Vacca, F., Barca, A., Gomes, A. S., Mazzei, A., Piccinni, B., Cinquetti, R., et al. (2019). The peptide transporter 1a of the zebrafish Danio rerio, an emerging model in nutrigenomics and nutrition research: molecular characterization, functional properties, and expression analysis. Genes Nutr. 14:33. doi: 10.1186/s12263-019-0657-3
Verri, T., Barca, A., Pisani, P., Piccinni, B., Storelli, C., and Romano, A. (2017). Di- and tripeptide transport in vertebrates: the contribution of teleost fish models. J. Comparat. Physiol. B 187, 395–462. doi: 10.1007/s00360-016-1044-7
Verri, T., Romano, A., Barca, A., Kottra, G., Daniel, H., and Storelli, C. (2010). Transport of di- and tripeptides in teleost fish intestine. Aquaculture Res. 41, 641–653. doi: 10.1111/j.1365-2109.2009.02270.x
Verri, T., Terova, G., Romano, A., Barca, A., Pisani, P., Storelli, C., et al. (2012). “The SoLute Carrier (SLC) family series in teleost fish,” in Functional Genomics in Aquaculture, eds M. Saroglia and Z. Liu (Hoboken, NJ: John Wiley & Sons, Ltd), 219–320.
Wang, K., Jiang, W., Wu, P., Liu, Y., Jiang, J., Kuang, S., et al. (2018). Gossypol reduced the intestinal amino acid absorption capacity of young grass carp (Ctenopharyngodon idella). Aquaculture 492, 46–58. doi: 10.1016/j.aquaculture.2018.03.061
Wang, K., Wang, E., Qin, Z., Zhou, Z., Geng, Y., and Chen, D. (2016). Effects of dietary vitamin E deficiency on systematic pathological changes and oxidative stress in fish. Oncotarget 7, 83869–83879. doi: 10.18632/oncotarget.13729
Wang, X., and Wang, W.-X. (2015). Physiologically based pharmacokinetic model for inorganic and methylmercury in a marine fish. Environ. Sci. Technol. 49, 10173–10181. doi: 10.1021/acs.est.5b02301
Winter, T. N., Elmquist, W. F., and Fairbanks, C. A. (2011). OCT2 and MATE1 provide bidirectional agmatine transport. Mol. Pharmaceutics 8, 133–142. doi: 10.1021/mp100180a
Wu, W., Dnyanmote, A. V., and Nigam, S. K. (2011). Remote communication through solute carriers and ATP binding cassette drug transporter pathways: an update on the remote sensing and signaling hypothesis. Mol. Pharmacol. 79, 795–805. doi: 10.1124/mol.110.070607
Yan, G., Zhang, Y., Yu, J., Yu, Y., Zhang, F., Zhang, Z., et al. (2012). Slc39a7/zip7 plays a critical role in development and zinc homeostasis in zebrafish. PLoS One 7:e42939. doi: 10.1371/journal.pone.0042939
Zaja, R. Lončar, J., Popovic, M., and Smital, T. (2011). First characterization of fish P-glycoprotein (abcb1) substrate specificity using determinations of its ATPase activity and calcein-AM assay with PLHC-1/dox cell line. Aquat. Toxicol. 103, 53–62. doi: 10.1016/j.aquatox.2011.02.005
Zaja, R. Popović, M., Lončar, J., and Smital, T. (2016). Functional characterization of rainbow trout (Oncorhynchus mykiss) Abcg2a (Bcrp) transporter. Comp. Biochem. Physiol. Toxicol. Pharmacol. CBP, 190, 15–23. doi: 10.1016/j.cbpc.2016.07.005
Zhang, Q.-L., Dong, Z.-X., Luo, Z.-W., Zhang, M., Deng, X.-Y., Guo, J., et al. (2020). The impact of mercury on the genome-wide transcription profile of zebrafish intestine. J. Hazardous Mater. 389:121842. doi: 10.1016/j.jhazmat.2019.121842
Zhang, T., Yao, J., Xu, D., Ma, X., Jin, W., Lv, G., et al. (2021). Gill physiological and transcriptomic response of the threatened freshwater mussel Solenaia oleivora to salinity shift. Comparat. Biochem. Physiol. Part D, Genomics Proteomics 40:100913. doi: 10.1016/j.cbd.2021.100913
Zhang, Y., Zhang, Y., Sun, K., Meng, Z., and Chen, L. (2018). The SLC transporter in nutrient and metabolic sensing, regulation, and drug development. J. Mol. Cell Biol. 11, 1–13. doi: 10.1093/jmcb/mjy052
Zucchi, S., Corsi, I., Luckenbach, T., Bard, S. M., Regoli, F., and Focardi, S. (2010). Identification of five partial ABC genes in the liver of the Antarctic fish Trematomus bernacchii and sensitivity of ABCB1 and ABCC2 to Cd exposure. Environ. Pollut. 158, 2746–2756. doi: 10.1016/j.envpol.2010.04.012
Keywords: multixenobiotic resistance, intestine, ABC transporters, aquatic, transporter-interfering chemicals (TICs), induction, SLC transporters
Citation: Romersi RF and Nicklisch SCT (2022) Interactions of Environmental Chemicals and Natural Products With ABC and SLC Transporters in the Digestive System of Aquatic Organisms. Front. Physiol. 12:767766. doi: 10.3389/fphys.2021.767766
Received: 31 August 2021; Accepted: 18 November 2021;
Published: 13 January 2022.
Edited by:
Flavia Bieczynski, Consejo Nacional de Investigaciones Científicas y Técnicas (CONICET), ArgentinaReviewed by:
Till Luckenbach, Helmholtz Centre for Environmental Research, Helmholtz Association of German Research Centres (HZ), GermanyJovica Loncar, Rudjer Boskovic Institute, Croatia
Copyright © 2022 Romersi and Nicklisch. This is an open-access article distributed under the terms of the Creative Commons Attribution License (CC BY). The use, distribution or reproduction in other forums is permitted, provided the original author(s) and the copyright owner(s) are credited and that the original publication in this journal is cited, in accordance with accepted academic practice. No use, distribution or reproduction is permitted which does not comply with these terms.
*Correspondence: Sascha C. T. Nicklisch, bmlja2xpc2NoQHVjZGF2aXMuZWR1