- 1School of Medicine, University of Wollongong, Wollongong, NSW, Australia
- 2Illawarra Health and Medical Research Institute, Wollongong, NSW, Australia
- 3School of Physical Education, Sport and Exercise Sciences, University of Otago, Dunedin, New Zealand
We investigated whether substituting the final half within 60-min bouts of exercise with passive warm or cold water immersion would provide similar or greater benefits for cardiometabolic health. Thirty healthy participants were randomized to two of three short-term training interventions in a partial crossover (12 sessions over 14–16 days, 4 week washout): (i) EXS: 60 min cycling 70% maximum heart rate (HRmax), (ii) WWI: 30 min cycling then 30 min warm water (38–40°C) immersion, and/or (iii) CWI: 30 min cycling then 30 min cold water (10–12°C) immersion. Before and after, participants completed a 20 min cycle work trial, O2max test, and an Oral Glucose Tolerance Test during which indirect calorimetry was used to measure substrate oxidation and metabolic flexibility (slope of fasting to post-prandial carbohydrate oxidation). Data from twenty two participants (25 ± 5 year, BMI 23 ± 3 kg/m2, Female = 11) were analyzed using a fixed-effects linear mixed model. O2max increased more in EXS (interaction p = 0.004) than CWI (95% CI: 1.1, 5.3 mL/kg/min, Cohen’s d = 1.35), but not WWI (CI: −0.4, 3.9 mL/kg/min, d = 0.72). Work trial distance and power increased 383 ± 223 m and 20 ± 6 W, respectively, without differences between interventions (interaction both p > 0.68). WWI lowered post-prandial glucose ∼9% (CI −1.9, −0.5 mmol/L; d = 0.63), with no difference between interventions (interaction p = 0.469). Substituting the second half of exercise with WWI provides similar cardiometabolic health benefits to time matched exercise, however, substituting with CWI does not.
Introduction
Physical inactivity has been reported as the fourth leading cause of death from chronic disease worldwide (World Health Organization [WHO], 2009) and is a significant contributor to preventable morbidity and mortality in Australian adults (Australian Bureau of Statistics, 2018). Despite ample evidence supporting the health benefits of physical activity, alarmingly, only 15% of Australian adults meet the “Australian Physical Activity and Sedentary Guidelines” of 150–300 min of moderate intensity physical activity per week (Australian Bureau of Statistics, 2018; Australian Government Department of Health [AGDH], 2020). Alternate strategies that assist and encourage those who are unable and/or unwilling to engage in sufficient levels of physical activity are urgently needed.
Post-exercise water immersion has long been used by athletes in an attempt to improve recovery and to enhance exercise capacity (Versey et al., 2013; Machado et al., 2017; Broatch et al., 2018; Chow et al., 2018; Tavares et al., 2018). Cold water immersion (CWI: 5–16°C) has been shown to stimulate metabolic regulators such as mitochondrial biogenesis (Broatch et al., 2018). For example, Ihsan et al. (2014, 2015) found an increase in skeletal muscle PGC-1α mRNA concentration, an important regulator of mitochondrial biogenesis and oxidative metabolism, when CWI (10°C for 15 min) occurred post-treadmill running. Conversely, prior work by Broatch et al. (2017) showed post-exercise CWI did not improve O2 max or maximal uncoupled respiration (complexes I and II), compared to 6-weeks of exercise training. Literature is inconsistent, with some studies finding improvements with CWI (reviewed in Broatch et al., 2018), while others observe no additional benefit in mitochondrial function and endurance performance with post-exercise CWI (Shute et al., 2017; Broatch et al., 2018). Whilst the inconsistent findings may be due to various factors, including differences in methodologies and participants’ body composition, it is plausible that the reduction in limb blood flow with CWI, in part, contributes to impairments in vascular function, metabolic flexibility and performance (Yamane et al., 2006, 2015; Stephens et al., 2018).
In contrast to CWI, warm water immersion (WWI: 37–46°C) increases limb blood flow, and muscle heating activates regulators of mitochondrial biogenesis and glucose sensitivity, it thereby offers potential alternate or adjunct to exercise training and is currently being investigated by many researchers for its health and performance benefits. WWI alone has been found to improve fasting glucose, glucose uptake (Gupte et al., 2009; Sjøberg et al., 2017), insulin concentrations (Hoekstra et al., 2018), vascular function and structure (Green et al., 2010; Brunt et al., 2016), and O2max, comparably to exercise training (Bailey et al., 2016). The only study to date, Bailey et al. (2016), observed similar increases in cardiorespiratory fitness and cerebrovascular function following 8 weeks of half hourly WWI sessions when compared to time-matched exercise. In addition, just 10 WWI sessions in sedentary, overweight adults or 3 weeks of WWI in type 2 diabetes patients was found to increase nitric oxide availability and reduce fasting insulin and glucose concentrations (Stephens et al., 2018). Collectively, these studies indicate that WWI might be used as a viable substitute in achieving some of the benefits of exercise training (e.g., reduced risk of diabetes, improved heart health, improved cardiovascular fitness) particularly for those who cannot or will not exercise sufficiently. Furthermore, prior research has shown that the combination of passive heat and exercise (rather than complete substitution) results in additive increases in both metabolic enzyme and protein adaptations in the skeletal muscle of mice (Tamura et al., 2014). Together, the key elements of heat, coupled with the mechanical tension, substrate turnover and transient oxidative stress experienced during exercise, drive the wide-ranging and unparalleled health benefits of exercise. Therefore, the combination of WWI and exercise, for those who cannot perform sufficient volumes of exercise, may be more beneficial than WWI alone in achieving positive health outcomes associated with regular exercise (i.e., also including those for bone and muscle). Further, substituting the second half of exercise with WWI may allow those who are disinclined or less able to meet exercise recommendations to achieve comparable health benefits. The present study investigates the efficacy of post-exercise warm or cold WI in an apparently health population before aiming to apply this approach to clinical populations.
To date, no research has examined the chronic cardiometabolic health effects of replacing half the exercise duration with warm or cold water immersion as an alternate strategy to time-matched exercise. Based on previous research (Bailey et al., 2016), we hypothesized that post-exercise WWI would improve O2max and glucose regulation to a similar extent to time-matched exercise, and greater extent than CWI would.
Research Design and Methods
Experimental Design
A partial-crossover trial was used, employing a balanced, incomplete block design whereby participants were randomized to complete two of three training interventions (12 training sessions over 14–16 days, with 4 week washout between, Figure 1): (i) EXS—60 min of steady state cycling at 70% of maximum heart rate (HRmax) (ii) WWI—30 min of steady state cycling at 70% HRmax followed by 30-min warm water (∼39°C) immersion of the lower limbs, up to umbilicus, and/or iii) CWI—30 min of steady state cycling at 70% HRmax followed by 30 min cold water (∼11°C) immersion of the lower limbs, up to the umbilicus. Prior to session one, all participants completed a non-blinded familiarization cycling work trial to familiarize them to the ergometer (Wattbike) and the 20-min duration. To measure changes in performance, each participant completed a blinded 20-min cycling work trial during the first and last training sessions (instead of continuous cycling). The work trial protocol is outlined below. For each intervention, post-assessments were completed 24 h (OGTT) and 48 h (O2 max) following the final training session.
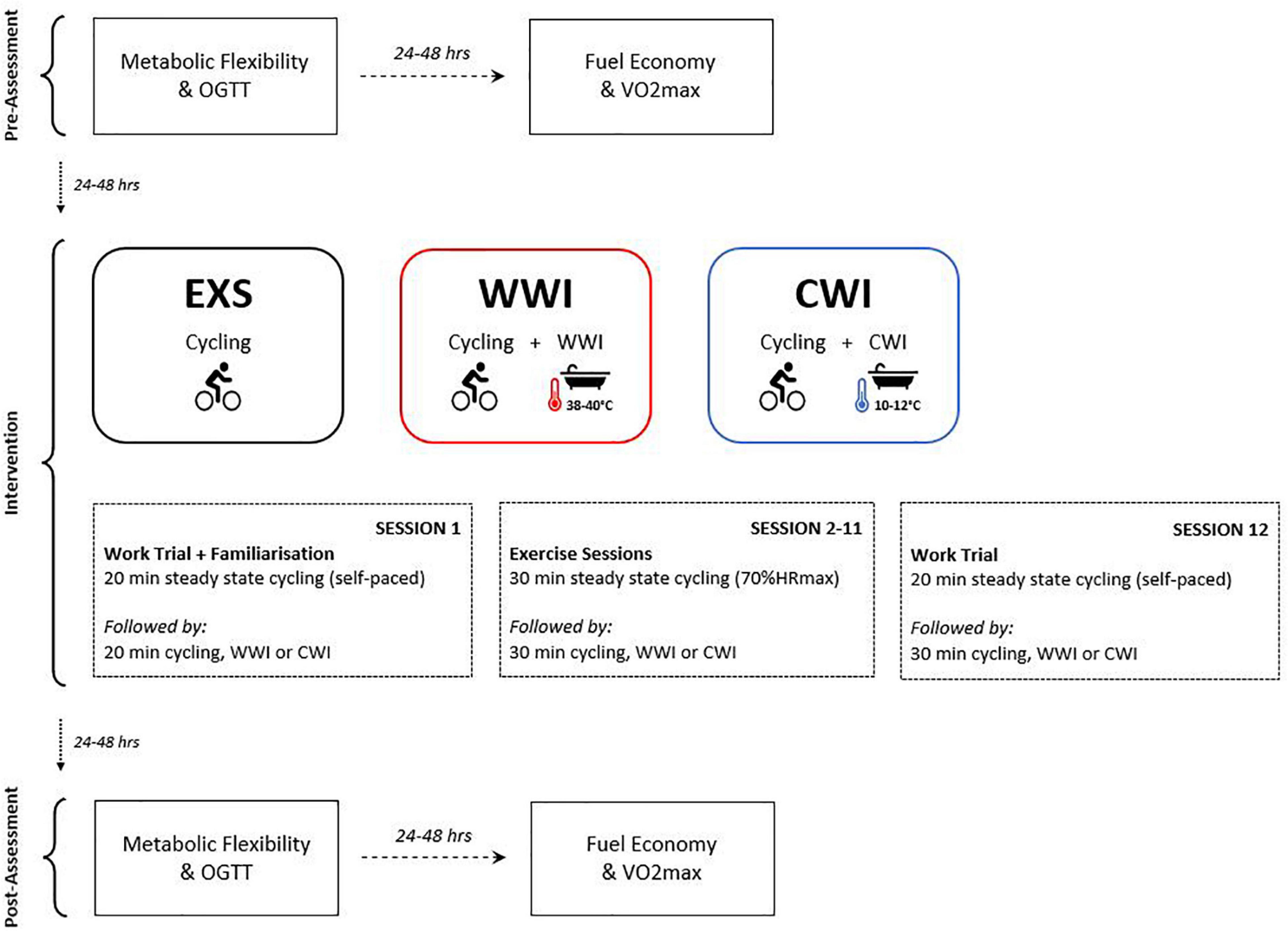
Figure 1. Experimental design. Pre- and post-assessments (OGTT and VO2max) were performed with 24–48 h between each and prior to session one, and after session 12. Sessions 2–11 included the full protocols [EXS (60-min moderate intensity cycling), CWI (30-min cold WI + 30-min moderate intensity cycling) or WWI (30-min warm WI + 30-min moderate intensity cycling)]. A 20-min work trial was completed in session one and 12 followed by EXS, CWI or WWI (time-matched). Participants completed two out of three interventions with a 4-week washout separating each intervention.
Participants
Thirty healthy adults were recruited to complete two of three interventions (Figure 2). Twenty two (age = 25 ± 5 y, BMI 23 ± 3 kg/m2, 11 females) completed both interventions (EXS: n = 17, CWI: n = 15, WWI: n = 16) and were included in analyses. The eight participants who did not complete both interventions stated time commitment, relocation, intolerance to exercise/cold, surgery or traveling as reasons for dropping out. Participants were recreationally active (participating in physical activity on ∼3 days per week but not in any formal exercise training program). Prospective participants were excluded if they had any previously diagnosed health conditions, smoked (in previous 3 years), were pregnant or had any injury preventing participation. Written informed consent was provided by all participants who were screened for contraindications to exercise using “The Physical Activity Readiness Questionnaire” at an initial consultation. Research protocols were approved by the UOW Human Research Ethics Committee (Ethics Number: 2018/322. Approval Date: 07/08/2018).
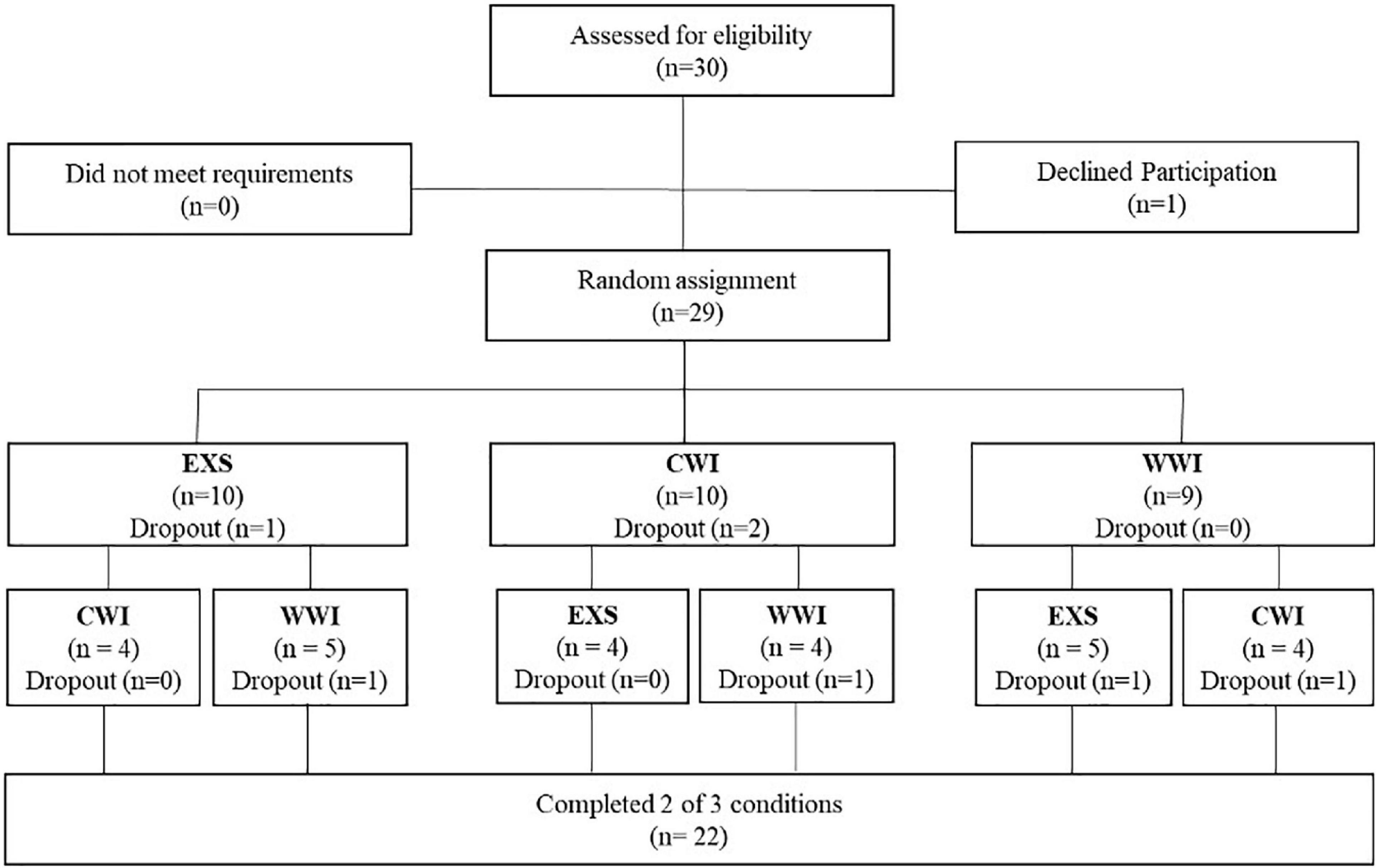
Figure 2. Study flow diagram. Partial randomized crossover trial where participants completed two of three training interventions (each 12 training sessions); 60 min of continuous exercise or 30 min of exercise followed by either 30 min of warm water (∼38–40°C) or cold water (∼10–12°C) immersion to the umbilicus.
Training Protocol
For each intervention, participants completed 12 sessions over 14–16 days, with a 4 week washout between (Figure 1). The 2 week duration was chosen to investigate the effects of short-term training whilst minimizing any potential confounding effect of changes in body composition. Several studies have shown benefits in cardiometabolic health after a similar exercise intervention period (Little et al., 2011; Reynolds et al., 2016). All twelve training sessions were supervised by an Accredited Exercise Physiologist. Blood pressure was taken before and after each training session, with heart rate measured via telemetry (Polar Electro, Kempele, Finland) and Rating of Perceived Exertion (RPE) recorded at 5-min intervals throughout each training session.
The first session of the EXS intervention involved 50 min of cycling only, with the first session of WI including 30 min of cycling followed by 20 min of immersion in either cold or warm water. Thereafter, each exercise training session involved continuous cycling at an intensity of 70% of HRmax (assessed via initial O2max test) for either 30 min (CWI and WWI) or 60 min (EXS) with WI interventions including 30 min of immersion so that all protocols were 60 min in total. All exercise training and water immersion interventions occurred in a room with an ambient 23°C temperature. Participants were able to drink water ad libitum during all interventions.
Water Immersion Interventions
The CWI and WWI interventions involved 30 min cycling at 70% HRmax followed by water immersion for 30 min. Participants sat with their legs fully extended, immersed in water up to their umbilicus between 10 and 12°C (CWI) or 38–40°C (WWI), i.e., temperatures routinely used in water immersion therapy for healthy and clinical populations (Versey et al., 2013). Water temperature was monitored (via a calibrated mercury thermometer) before and throughout immersion to ensure correct temperature was maintained. Hot water/ice was added to the bath and an external tap allowed removal of volume when necessary to maintaining correct immersion temperature and depth. Core temperature (auditory canal, using a Braun ThermoScan), thermal sensation and discomfort scales (Gagge et al., 1967) were measured every 5 min during immersion.
Pre- and Post-assessments
O2max
Participants fasted for 3 h prior to completing two stages of submaximal cycling, at a prescribed absolute and relative intensity, to assess metabolic flexibility (the ability to adapt substrate utilization to substrate availability; Aucouturier et al., 2011), immediately followed by a ramp cycle test to volitional exhaustion for the measurement of O2max. Stage one involved 10 min cycling at 50 W for females and 70 W for males (absolute: elicits a low-intensity response of approximately 30–40% of HRmax). In stage two, participants exercised for 10 min at 70% of predicted HRmax (220-age). Indirect Calorimetry (Gas Exchange via Parvo Medic’s TrueOneTM 2400) was used to measure participants’ energy use [oxygen uptake (O2), expired carbon dioxide (CO2)], and substrate utilization (carbohydrate/fat oxidation) (Péronnet and Massicotte, 1991). During the ramp stage, the load was increased by 35 W at 1-min intervals until volitional exhaustion. During the last 30 s of each stage, heart rate, via telemetry and RPE (Borg 6–20 scale; Borg, 1982) were recorded. HRmax was recorded at the completion of the ramp stage.
Work Trial
Participants completed a self-paced 20-min work trial on an air-braked ergometer (Wattbike, Nottingham, United Kingdom). Participants were encouraged to complete the maximum distance possible, with only their cadence and time used as feedback during the test. Heart rate, distance (km) and power (watts) were recorded at 5-min intervals. A minimum of 48 h separated the O2max and work trial sessions and the exercise training interventions.
Resting Metabolic Rate and Oral Glucose Tolerance Test
Participants fasted for 10 h before test initiation. RMR was measured using a Parvo Metabolic Cart, with a canopy hood to measure rates of CO2, O2 and RER. Participants were instructed to minimize activity prior to arrival at the laboratory. Following 10 min of supine rest, 20-min RMR data were collected with the final 10 min used for analyses. The same protocol was used for all comparison time points.
Fasting capillary glucose concentration was then measured using a standard lancing device and a HemoCue glucose analyzer (HemoCue Glucose 201 RT glucose; HemoCue AB, Sweden). A standard 75 g glucose tolerance drink was consumed, then RMR was measured for a further 20 min at 30- and 60-min post-consumption. Energy use (O2) and fuel utilization (CHO and fat oxidation, assuming minimal protein contribution) (Péronnet and Massicotte, 1991) were calculated at each time point. Capillary glucose concentration was measured 30, 60, 90, and 120 min post-prandially (PP).
Data Analysis
Data were first assessed for normality using Q-Q plots, histograms and the Shapiro-Wilk test. After outliers were removed (all data points for that measurement) using the default value of ± 3 standard deviations in SPSS, all assumption of normality conditions were met. The study was powered on effect size change in O2max (Bailey et al., 2016) and a linear mixed model (with time × intervention interaction, and main effects of time) was used to compare outcomes between interventions. To determine differences between interventions, post hoc tukey tests were performed following a significant interaction. Significance was set at P < 0.05. A statistician from UOW was consulted in the study design, performed the blocked randomization and assisted with statistical analyses. 95% CI were calculated using SPSS, and Cohen’s d were calculated for effect size [Cohen’s d 0.2–0.5 (small); 0.5–0.8 (moderate); 0.8 + (large)].
Results
All participants completed the 12 sessions in 14–16 days, with no more than two consecutive days between training sessions. HR profiles for the three interventions are shown in Figure 3. HR was not different between interventions for the first 30 min of cycling but was significantly higher for the total 60 min during EXS (143 ± 19 bpm) compared to CWI (114 ± 30 bpm) and WWI (121 ± 27 bpm) (interaction p < 0.001). HR was significantly higher during WWI than CWI (p = 0.002). RPE did not differ between interventions (EXS: 11 ± 1, CWI: 11 ± 1, and WWI: 11 ± 1, interaction p = 0.986). On average, auditory canal temperature was not different during the 30 min of WI between WWI (37.4 ± 1.3°C) and CWI (37.5 ± 1.3°C, interaction p = 0.931). As expected, thermal sensation differed between CWI (“cold”; 4.0 ± 0.3) and WWI (“slightly warm”; 8.0 ± 0.3) (interaction p < 0.001). Thermal discomfort was greater with CWI (“slightly uncomfortable”; 2.0 ± 0.2) compared to WWI (“comfortable”; 1.0 ± 0.1) (interaction p = 0.052).
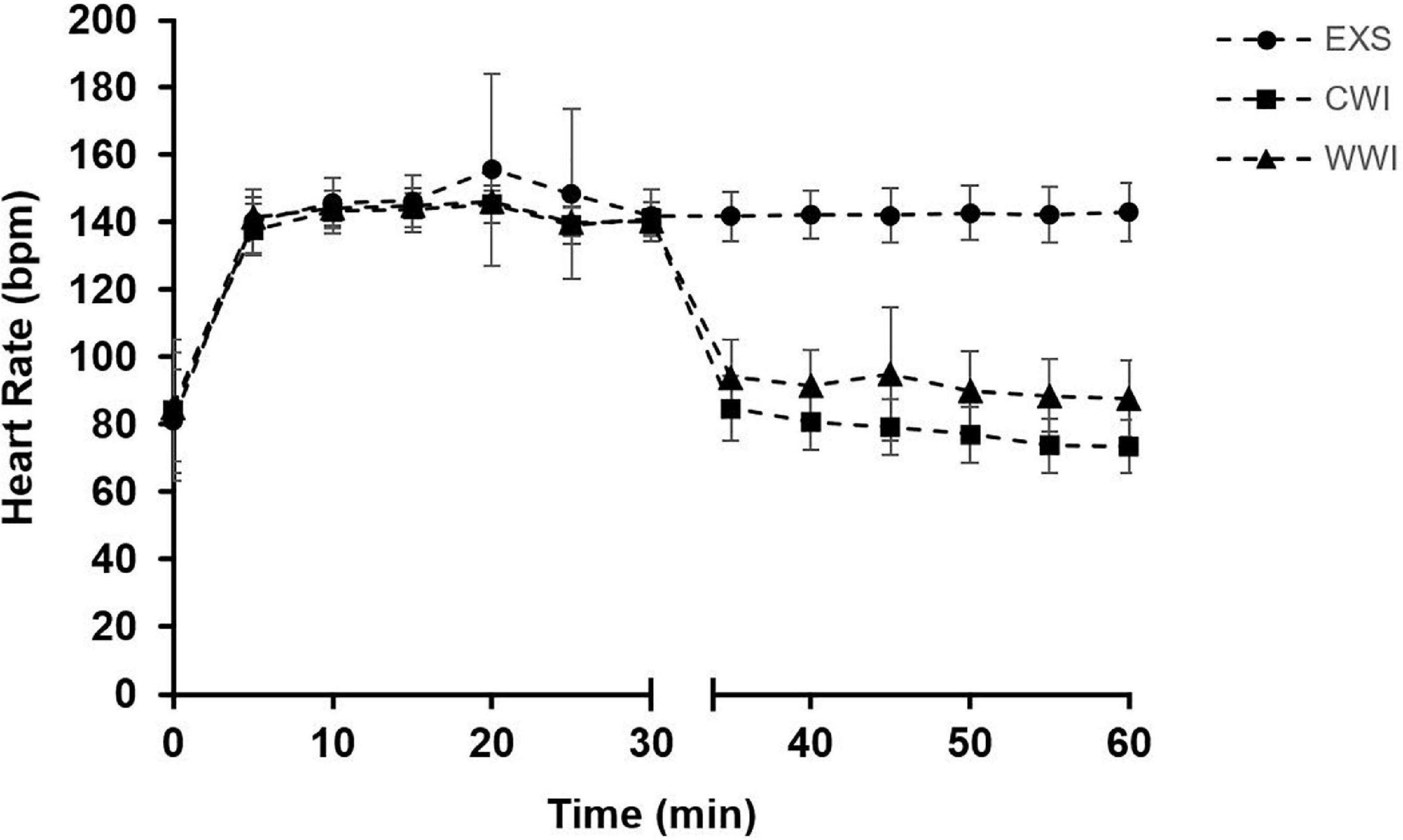
Figure 3. Mean heart rate [HR (bpm)] during time-matched exercise [EXS (n = 16)], post-exercise Cold Water Immersion [CWI (n = 14)] and post-exercise Warm Water Immersion [WWI (n = 14)]. After 30 min of cycling, CWI and WWI began 30 min of water immersion (indicated by gap in x-axis). HR was not significantly different between interventions from 0 to 30 min but was significantly higher with EXS than WI (p < 0.001) from 0 to 60 min. HR was significantly higher during the 30 min of WWI when compared to CWI (p = 0.002).
O2max
The change in O2max differed between interventions [interaction p = 0.004, Figure 4; EXS (Pre: 39.9 ± 7.4 mL/kg/min to Post: 43.6 ± 6.4 mL/kg/min, p = 0.001), CWI (Pre: 41.8 ± 7.7 mL/kg/min to Post: 41.3 ± 8.1 mL/kg/min, p = 0.495), WWI (Pre: 40.0 ± 8.9 mL/kg/min to Post: 41.3 ± 9.0 mL/kg/min, p = 0.103)]. EXS significantly increased O2max (large effect) by 3.2 mL/kg/min more than CWI (CI: 1.1, 5.3 mL/kg/min, d = 1.36), but not significantly more than WWI (CI: −0.4, 3.9 mL/kg/min, d = 0.73).
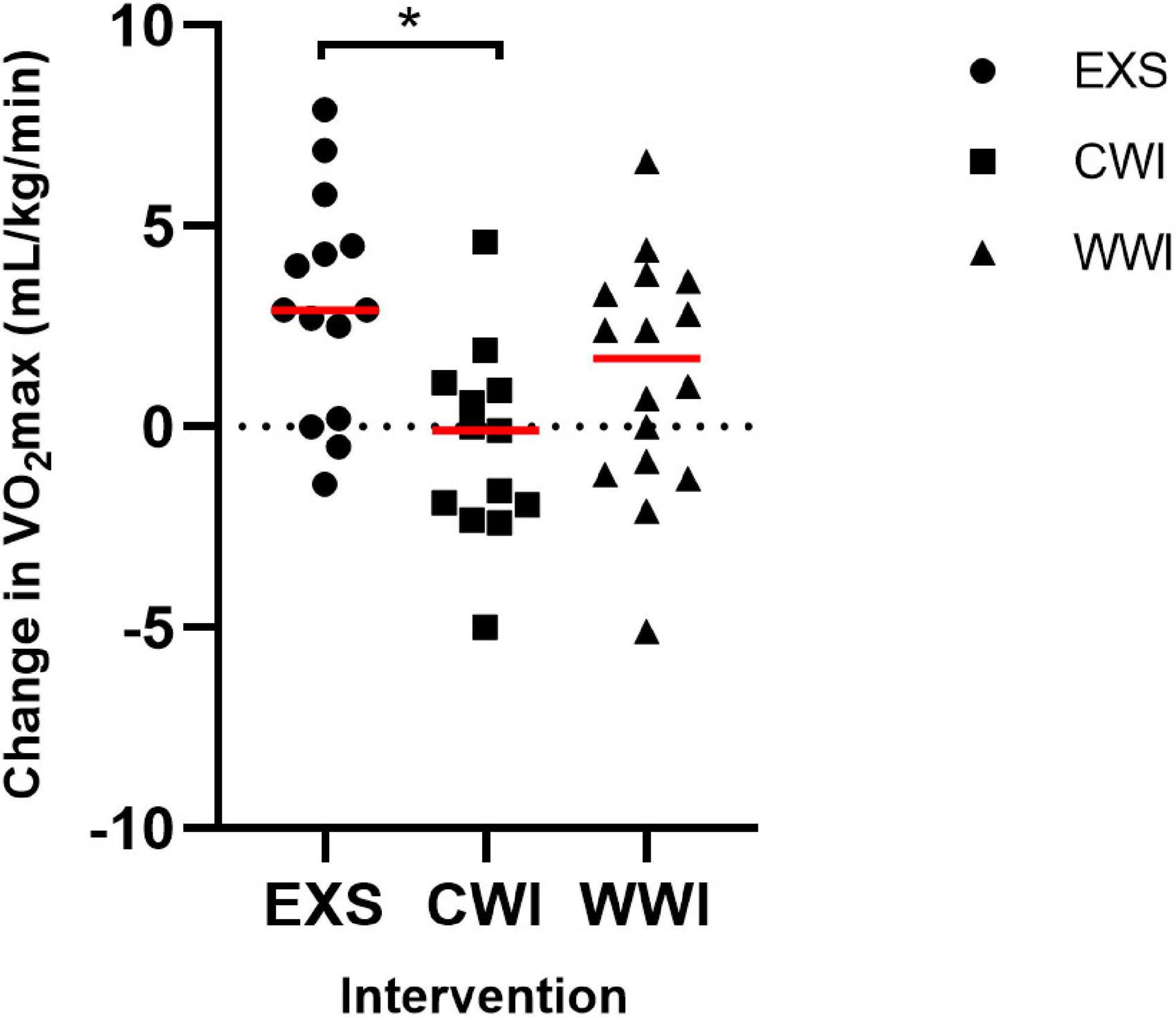
Figure 4. Change in cardiorespiratory fitness (O2max [mL/kg/min]) following 12 sessions of Exercise [EXS (n = 14)], post-exercise Cold Water Immersion [CWI (n = 14)] and post-exercise Warm Water Immersion [WWI (n = 16)]. *Indicates significant difference between EXS and CWI (post hoc, p = 0.002), following significant interaction (p = 0.004).
Work Trial
Distance (time effect p = 0.048) and power (time effect p = 0.008) increased for the 20-min work trial, by an average 380 m and 20 W, respectively, with no difference between interventions [interactions Distance (p = 0.692), Power (p = 0.812), Table 1].

Table 1. Total distance traveled, average heart rate (HR) and power during the 20-min cycle ergometer work trial.
Glucose Tolerance (Oral Glucose Tolerance Test)
Fasting (interaction p = 0.769), mean (interaction 0.193) and peak post-prandial glucose (PPG) (interaction = 0.149) were not significantly different between interventions. The change in mean PPG is shown in Figure 5A. Mean PPG changed by + 0.1 mmol/L following EXS (CI−0.5, 0.9 mmol/L; d = 0.19), −0.2 mmol/L following CWI (CI−0.5, 0.3 mmol/L; d = 0) and −0.6 mmol/L following WWI (CI−1.2, −0.03 mmol/L; d = 0.62). Peak PPG showed a small change after EXS (CI −0.6, 1.2 mmol/L, d = 0.21) and no change after CWI (CI −0.6, 0.6 mmol/L; d = 0.00), but WWI was found to moderately decrease peak PPG by −1.0 mmol/L (CI −1.9, −0.5 mmol/L; d = 0.63). The change in Peak PPG is shown in Figure 5B.
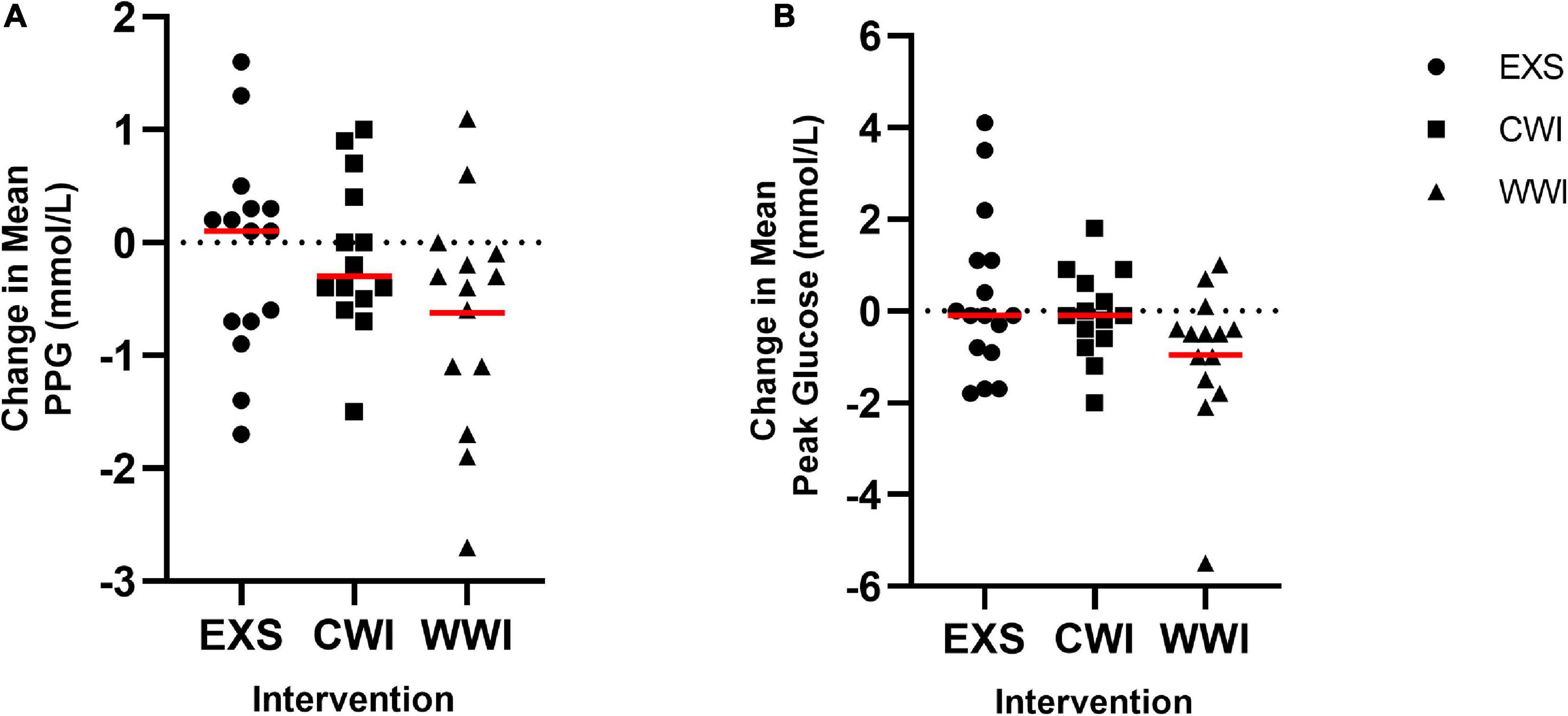
Figure 5. (A) Change in post-prandial Glucose (PPG) after 12 sessions of time-matched exercise [EXS (n = 15)], post-exercise Cold Water Immersion [CWI (n = 14)] and post-exercise Warm Water Immersion [WWI (n = 14)]. No significant interaction effect. (B) Change in Peak PPG after 12 sessions of EXS (n = 16), CWI (n = 14) and WWI (n = 14). No significant interaction effect.
Metabolic Flexibility
Fuel Utilization Glucose Challenge Oral Glucose Tolerance Test
Fasting (p = 0.67) and post-prandial RER (p = 0.71) were not significantly different between interventions, nor was fasting (p = 0.39) or post-prandial (p = 0.40) carbohydrate (CHO) utilization (Table 2).
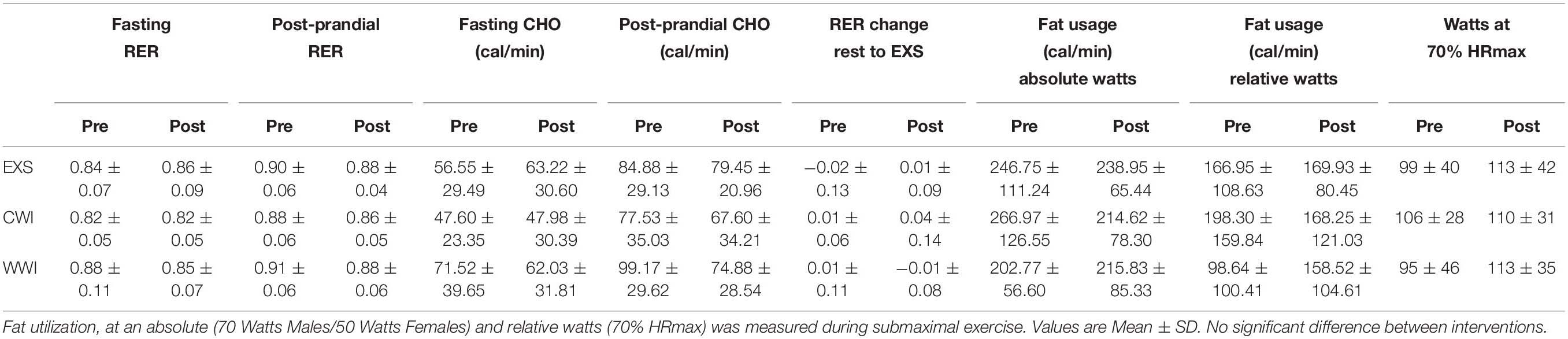
Table 2. Fasting and post-prandial (PP) respiratory exchange ratio (RER) and substrate use [Carbohydrate (CHO)], following 12 sessions of time-matched Exercise [EXS (n = 15)], post-exercise cold water immersion [CWI (n = 14)] and post-exercise warm water immersion [WWI (n = 14)] during a 2 h Oral Glucose Tolerance test.
Fuel Utilization Submaximal Exercise
Change in RER from rest to exercise was not significantly different between interventions (interaction p = 0.676) (Table 2). Fat utilization at an absolute (interaction p = 0.270) and relative power (interaction p = 0.515) was not significantly different between interventions, nor across time (time effect p = 0.687). Power output at 70% of HRmax was not significantly different between interventions (interaction p = 0.443) nor across time (p = 0.158).
Discussion
This study investigated whether substituting the final 30 min of each exercise bout with warm or cold water immersion could provide similar or greater benefits for cardiometabolic health and exercise performance. The main finding was that exercise training alone (EXS) results in the greatest increase in cardiovascular fitness (O2max), being significantly greater than the change following 2-weeks of post-exercise CWI but not post-exercise WWI. All short-term interventions improved performance related indices such as power output and distance attained during a 20 min work trial (albeit this study did not include a non-intervention control). WWI was also perceived as thermally comfortable, which may further facilitate its efficacy as an adjunct to exercise training, allowing for less exercise whilst still achieving cardiometabolic health benefits in people who cannot/will not perform sufficient volumes of exercise. Although the changes in metabolic indices were not significantly different between interventions, future research is needed given the non-significant but moderate decrease in peak post-prandial glucose after WWI. WWI used alone has previously been shown to improve glucose control (Hooper, 1999; Hoekstra et al., 2018), and thus warrants further research as an adjunct to exercise for improving glucose tolerance in those at risk. Together, our study adds to the current literature on hydrotherapy and highlights post-exercise WWI as a potential alternative to longer duration exercise. Given similar benefits to longer duration exercise, post-exercise WWI has important implications for those who are either unable or disinclined to exercise for 60 min, such as those with low exercise tolerance, chronic obstructive pulmonary disease, congestive heart failure, cardiomyopathy, peripheral vascular disease, obesity and Type 2 Diabetes.
Cardiorespiratory Fitness (O2max)
The benefits of ∼300 min/week of exercise training (physical activity guidelines) on fitness are well known. The present study found that, although O2max improved to a greater extent with EXS alone when compared to post-exercise CWI, there were no significant differences between EXS alone and when 30 min of exercise was substituted with 30 min of post-exercise WWI. This suggests WWI can be a partial substitute to exercise for achieving gains in fitness. Bailey et al. (2016) found comparable improvements in cardiovascular fitness after 30 min of WWI alone vs. time-matched moderate-intensity cycling, whereas we have shown here that substituting the second half of an exercise session with WWI also provides comparable benefits. In contrast, post-exercise CWI was less effective than EXS alone for improving cardiorespiratory fitness. Prior work by Broatch et al. (2017) also showed CWI did not improve O2 max or maximal uncoupled respiration (complexes I and II), compared to 6-weeks of exercise training, supporting the work of Yamane et al. (2006, 2015) in showing blunted vascular, endurance and strength effects of CWI. Future research on CWI is needed to determine the mechanisms by which CWI may blunt exercise adaptations (e.g., blood flow, inflammation and cellular metabolism) (Pedersen and Hoffman-Goetz, 2000; Mawhinney et al., 2017; Peake et al., 2017).
This is the first study to compare the effects of post-exercise WWI, CWI, and EXS. The duration and intensity of our interventions are in line with current physical activity recommendations that suggest 150–300 min of moderate intensity exercise per week for improvements in cardiorespiratory fitness (Australian Government Department of Health [AGDH], 2020). The larger increase in cardiorespiratory fitness with EXS than CWI presumably reflects at least partly a greater cardiovascular stimulus. Thomas et al. (2016) previously showed that WWI and exercise increase muscle temperature, limb blood flow and antegrade shear stress, with the increases following WWI being of a greater extent compared to exercise. Indeed, many key adaptations to exercise training are stimulated by elevations in muscle and body temperature, resulting in increases in blood flow and volume, metabolic rate, and the regulation of gene expression and signaling molecules i.e., for mitochondrial biogenesis (Thomas et al., 2016). Exercise remains superior for improving cardiovascular fitness, however, for those who are unable to perform larger volumes of exercise, WWI could be used as an alternate option to retain O2max or achieve some improvement.
Exercise Performance Work Trial
Exercise performance, measured as work trial distance and power, improved after all interventions. In contrast, prior research on exercise performance and WI (often used to promote recovery) has shown little to no effect on exercise training adaptations (Broatch et al., 2018). For example, Vaile et al. (2008) found that CWI following exercise led to modest improvements in time trial performance but did not find any benefits with WWI. Likewise, Malta et al. (2021) concluded that aerobic exercise performance, specifically, mean power and time-trial performance (duration) were not adversely effected by regular use of CWI. Our findings that post-exercise CWI and WWI had similar (but no reliably identifiable additive or detrimental) effects for performance indicators compared to time-matched exercise alone, suggest WI could be used as an alternate to exercise alone. We acknowledge that without a 30-min exercise alone intervention, it is unclear whether WI is additive in our study. The aim of this proof-of-concept study was to see if WI could provide comparable effects as an exercise substitute for those who cannot perform sufficient volumes of exercise. Previous research comparing 30–45 min of post-meal exercise has found similar benefits for post-prandial glucose, however, benefit to fitness and exercise performance needs to be further investigated (Bellini et al., 2021).
Metabolic Health
Continued research on hydrotherapy is needed to determine the impact of WI as an exercise adjunct for enhancing metabolic health. In contrast to Hoekstra et al. (2018), the current study found no intervention effect on fasting blood glucose, mean PPG or metabolic flexibility; despite similar intervention durations. This may be due to differences in water immersion depth (neck) and duration (60 min), which were used by Hoekstra et al. (2018) would likely elicited greater thermal stress and thus adaptation. Despite there being no significant difference between interventions, our WWI results are promising given the on average 1.0 mmol/L change in peak PPG following the short-term WWI intervention; particularly in an apparently healthy population where blood glucose improvements would be expected to be minimal. A 1 mmol/L (∼10%) has been associated with improved glycemic control, reduced risk of diabetes related complications and CVD (Mazzone, 2010; Hrubeniuk et al., 2020). Furthermore, prior studies have shown that WWI interventions improve HbA1c, fasting blood glucose and insulin sensitivity in at risk populations (McCarty et al., 2009; Hooper et al., 2014; Krause et al., 2015). The proposed mechanisms underlying these changes in other studies are an increase in heat shock protein (iHsp72) expression and reduced chronic low-grade inflammation (Hoekstra et al., 2020). Further investigation regarding the intensity of exercise preceding WWI, the required temperature of water and the duration of immersion, in metabolically compromised individuals, is required to better understand how WWI could be used as an adjunct therapy.
Future Directions
The aim of the present study was to investigate the feasibility and efficacy of post-exercise WI in an apparently healthy population before implementing this approach in clinical populations. In keeping with the physical activity recommendations and to allow translation of results to “free living,” insufficiently active adults, moderate intensity physical activity was chosen for the present study. Further to this, WI involved temperatures and depths that individuals could implement at home in the future. Improvements in work trial performance after all interventions suggest that partial substitution of exercise training, with either WWI or CWI, can improve performance indicators despite the reduced exercise volume. Whilst changes in O2max were similar between EXS and post-exercise WWI, CWI did not lead to improvements in cardiorespiratory fitness. Our findings, together with previous work, suggest WWI can be used in clinical populations to enhance their ability to perform physical activity. Adherence to WWI in Akerman et al. (2019) was ∼four times higher than the exercise only group. In the present study, discomfort experienced during CWI was significantly greater than with WWI. Together with the fitness benefits, this comfort indicates post-exercise WWI may be more efficacious than CWI due to the reduced discomfort experienced by participants, thus potentially improving exercise compliance. Future studies should focus on WWI as an adjunct to exercise training for health benefits.
Strengths and Limitations
A 4 week washout period was implemented, allowing participants to return to baseline for main outcomes of cardiorespiratory fitness and glucose control before beginning their second intervention and to ensure reversibility of training effect (Hecksteden et al., 2018). We also employed 30 min of WI, that together with exercise training is in line with the current physical activity guidelines of 150–300 min per week. Body mass was not a contributing factor and did not change significantly from baseline with any of the interventions; this was by design and thus expected. Ideally, participants would have completed all three trial interventions (complete crossover design), however, the associated time commitment and seasonal impact may have deterred participation and resulted in larger dropouts. Further to this, we acknowledge the limitations of prescribing exercise relative to fixed percentages of HRmax (Jamnick et al., 2020). As mentioned above, a limitation of the present study, was that it did not include a 30-min exercise only intervention, therefore it is unknown whether WI is indeed additive. In addition, because this proof-of-concept study involved young, healthy subjects, this may have contributed to an increased ceiling effect for significant differences in other metabolic outcomes, particularly for glucose tolerance. As such, duration (12 sessions) and intensity (moderate) of intervention may not have been enough to elicit significant differences.
Conclusion
This study adds to current literature highlighting the therapeutic potential of post-exercise WWI. Findings from this study show that 2-weeks of post-exercise WWI can provide similar benefits for cardiovascular fitness and time trial performance compared to 60-min of EXS alone, whereas EXS was superior to post-exercise CWI for cardiovascular fitness. For people who cannot exercise for the full 60 min, substituting 30 min of the exercise session with WWI provides a promising adjunct to achieve similar positive health outcomes. The combination of WWI and exercise in the present study is noteworthy given WI is not a full substitute for all exercise adaptations (e.g., bone density, calcium handling, neuromuscular junction maintenance, etc.). Results pertaining to metabolic health remain inconclusive and further investigation is required to determine if this could be used as a viable option for individuals with obesity or type 2 diabetes.
Data Availability Statement
The raw data supporting the conclusions of this article will be made available by the authors, without undue reservation.
Ethics Statement
The studies involving human participants were reviewed and approved by UOW Human Health and Medical Research Committee. The patients/participants provided their written informed consent to participate in this study.
Author Contributions
MF, TH, and JC designed the study. BR, CC, TH, and MF conducted the research. BR, CC, and MF analyzed the data. BR composed the manuscript. All authors edited the manuscript and approved the final draft.
Funding
This work was funded by an IHMRI Young Investigator Grant awarded to MF.
Conflict of Interest
The authors declare that the research was conducted in the absence of any commercial or financial relationships that could be construed as a potential conflict of interest.
Publisher’s Note
All claims expressed in this article are solely those of the authors and do not necessarily represent those of their affiliated organizations, or those of the publisher, the editors and the reviewers. Any product that may be evaluated in this article, or claim that may be made by its manufacturer, is not guaranteed or endorsed by the publisher.
Acknowledgments
Acknowledgment must go to all 30 study participants, along with Hannah Christie, Ander Oritz Erdocia, Jamie Duijvelshoff, Mather Burgers, and our undergraduate research students Thomas Bergen, Blake Charman, Macalla Farland, Cale Ryan, Taylor Johnson, Megan Greaves, Zoe Morell, Lachlan Greenfield and Ethan Macky, all of whom provided invaluable assistance with delivery of training sessions.
References
Akerman, A. P., Thomas, K. N., van Rij, A. M., Body, E. D., Alfadhel, M., and Cotter, J. D. (2019). Heat therapy vs. supervised exercise therapy for peripheral arterial disease: a 12-wk randomized, controlled trial. Am. J. Physiol. Heart Circ. Physiol. 316, H1495–H1506.
Aucouturier, J., Duché, P., and Timmons, B. W. (2011). Metabolic flexibility and obesity in children and youth. Obes. Rev. 12, e44–e53.
Australian Bureau of Statistics (2018). National Health Survey: First Results 2017-2018. Canberra: Australian Bureau of Statistics.
Bailey, T., Cable, N., Miller, G., Sprung, V., Low, D., and Jones, H. (2016). Repeated Warm Water Immersion Induces Similar Cerebrovascular Adaptations to 8 Weeks of Moderate-Intensity Exercise Training in Females. Int. J. Sports Med. 37, 757–765. doi: 10.1055/s-0042-106899
Bellini, A., Nicolo, A., Bazzucchi, I., and Sacchetti, M. (2021). Effects of Different Exercise Strategies to Improve Postprandial Glycemia in Healthy Individuals. Med. Sci. Sports Exerc. 53, 1334–1344. doi: 10.1249/mss.0000000000002607
Borg, G. A. (1982). Psychophysical bases of perceived exertion. Med. Sci. Sports Exerc. 14, 377–381.
Broatch, J. R., Petersen, A., and Bishop, D. J. (2017). Cold-water immersion following sprint interval training does not alter endurance signaling pathways or training adaptations in human skeletal muscle. Am. J. Physiol. Regul. Integr. Comp. Physiol. 313, R372–R384.
Broatch, J. R., Petersen, A., and Bishop, D. J. (2018). The Influence of Post-Exercise Cold-Water Immersion on Adaptive Responses to Exercise: a Review of the Literature. Sports Med. 48, 1369–1387. doi: 10.1007/s40279-018-0910-8
Brunt, V. E., Howard, M. J., Francisco, M. A., Ely, B. R., and Minson, C. T. (2016). Passive heat therapy improves endothelial function, arterial stiffness and blood pressure in sedentary humans. J. Physiol. 594, 5329–5342. doi: 10.1113/jp272453
Chow, G. C. C., Chung, J. W. Y., and Fong, S. S. M. (2018). Differential effects of post-exercise ice water immersion and room temperature water immersion on muscular performance, vertical jump, and agility in amateur rugby players: a randomized controlled trial. Sci. Sports 33, e271–e279.
Australian Government Department of Health [AGDH] (2020). Australia’s Physical Activity and Sedentary Behaviour Guidelines and the Australian 24-Hour Movement Guidelines. Australian Government Department of Health. Available online at: https://www1.health.gov.au/internet/main/publishing.nsf/Content/health-pubhlth-strateg-phys-act-guidelines. (Accessed July 30, 2020)
Gagge, A. P., Stolwijk, J. A. J., and Hardy, J. D. (1967). Comfort and thermal sensations and associated physiological responses at various ambient temperatures. Environ. Res. 1, 1–20. doi: 10.1016/0013-9351(67)90002-3
Green, D. J., Carter, H. H., Fitzsimons, M. G., Cable, N. T., Thijssen, D. H., and Naylor, L. H. (2010). Obligatory role of hyperaemia and shear stress in microvascular adaptation to repeated heating in humans. J. Physiol. 588, 1571–1577. doi: 10.1113/jphysiol.2010.186965
Gupte, A. A., Bomhoff, G. L., Swerdlow, R. H., and Geiger, P. C. (2009). Heat treatment improves glucose tolerance and prevents skeletal muscle insulin resistance in rats fed a high-fat diet. Diabetes 58, 567–578. doi: 10.2337/db08-1070
Hecksteden, A., Faude, O., Meyer, T., and Donath, L. (2018). How to Construct, Conduct and Analyze an Exercise Training Study? Front. Physiol. 9:1007. doi: 10.3389/fphys.2018.01007
Hoekstra, S. P., Bishop, N. C., Faulkner, S. H., Bailey, S. J., and Leicht, C. A. (2018). Acute and chronic effects of hot water immersion on inflammation and metabolism in sedentary, overweight adults. J. Appl. Physiol. 125, 2008–2018. doi: 10.1152/japplphysiol.00407.2018
Hoekstra, S. P., Bishop, N. C., and Leicht, C. A. (2020). Elevating body termperature to reduce low-grade inflammation: a welcome strategy for those unable to exercise? Exerc. Immunol. Rev. 26, 42–55.
Hooper, P. L. (1999). Hot-tub therapy for type 2 diabetes mellitus. N. Engl. J. Med. 341, 924–925. doi: 10.1056/nejm199909163411216
Hooper, P. L., Balogh, G., Rivas, E., Kavanagh, K., and Vigh, L. (2014). The importance of the cellular stress response in the pathogenesis and treatment of type 2 diabetes. Cell Stress Chaperones 19, 447–464. doi: 10.1007/s12192-014-0493-8
Hrubeniuk, T. J., Bouchard, D. R., Goulet, E. D. B., Gurd, B., and Sénéchal, M. (2020). The ability of exercise to meaningfully improve glucose tolerance in people living with prediabetes: a meta-analysis. Scand. J. Med. Sci. Sports 30, 209–216. doi: 10.1111/sms.13567
Ihsan, M., Markworth, J. F., Watson, G., Choo, H. C., Govus, A., Pham, T., et al. (2015). Regular postexercise cooling enhances mitochondrial biogenesis through AMPK and p38 MAPK in human skeletal muscle. Am. J. Physiol. Regul. Integr. Comp. Physiol. 309, R286–R294.
Ihsan, M., Watson, G., Choo, H. C., Lewandowski, P., Papazzo, A., Cameron-Smith, D., et al. (2014). Postexercise muscle cooling enhances gene expression of PGC-1α. Med. Sci. Sports Exerc. 46, 1900–1907. doi: 10.1249/mss.0000000000000308
Jamnick, N. A., Pettitt, R. W., Granata, C., Pyne, D. B., and Bishop, D. J. (2020). An Examination and Critique of Current Methods to Determine Exercise Intensity. Sports Med. 50, 1729–1756. doi: 10.1007/s40279-020-01322-8
Krause, M., Ludwig, M. S., Heck, T. G., and Takahashi, H. K. (2015). Heat shock proteins and heat therapy for type 2 diabetes: pros and cons. Curr. Opin. Clin. Nutr. Metab. Care 18, 374–380. doi: 10.1097/mco.0000000000000183
Little, J. P., Gillen, J. B., Percival, M. E., Safdar, A., Tarnopolsky, M. A., Punthakee, Z., et al. (2011). Low-volume high-intensity interval training reduces hyperglycemia and increases muscle mitochondrial capacity in patients with type 2 diabetes. J. Appl. Physiol. 111, 1554–1560. doi: 10.1152/japplphysiol.00921.2011
Machado, A. F., Almeida, A. C., Micheletti, J. K., Vanderlei, F. M., Tribst, M. F., Netto Junior, J., et al. (2017). Dosages of cold-water immersion post exercise on functional and clinical responses: a randomized controlled trial. Scand. J. Med. Sci. Sports 27, 1356–1363. doi: 10.1111/sms.12734
Malta, E. S., Dutra, Y. M., Broatch, J. R., Bishop, D. J., and Zagatto, A. M. (2021). The Effects of Regular Cold-Water Immersion Use on Training-Induced Changes in Strength and Endurance Performance: a Systematic Review with Meta-Analysis. Sports Med. 51, 161–174. doi: 10.1007/s40279-020-01362-0
Mawhinney, C., Jones, H., Low, D. A., Green, D. J., Howatson, G., and Gregson, W. (2017). Influence of cold-water immersion on limb blood flow after resistance exercise. Eur. J. Sport Sci. 17, 519–529. doi: 10.1080/17461391.2017.1279222
Mazzone, T. (2010). Intensive Glucose Lowering and Cardiovascular Disease Prevention in Diabetes: reconciling the Recent Clinical Trial Data. Circulation 122, 2201–2211. doi: 10.1161/circulationaha.109.913350
McCarty, M. F., Barroso-Aranda, J., and Contreras, F. (2009). Regular thermal therapy may promote insulin sensitivity while boosting expression of endothelial nitric oxide synthase–effects comparable to those of exercise training. Med. Hypotheses 73, 103–105. doi: 10.1016/j.mehy.2008.12.020
Peake, J. M., Neubauer, O., Della Gatta, P. A., and Nosaka, K. (2017). Muscle damage and inflammation during recovery from exercise. J. Appl. Physiol. 122, 559–570. doi: 10.1152/japplphysiol.00971.2016
Pedersen, B. K., and Hoffman-Goetz, L. (2000). Exercise and the Immune System: regulation, Integration, and Adaptation. Physiol. Rev. 80, 1055–1081. doi: 10.1152/physrev.2000.80.3.1055
Péronnet, F., and Massicotte, D. (1991). Table of nonprotein respiratory quotient: an update. Can. J. Sport Sci. 16, 23–29.
Reynolds, A. N., Mann, J. I., Williams, S., and Venn, B. J. (2016). Advice to walk after meals is more effective for lowering postprandial glycaemia in type 2 diabetes mellitus than advice that does not specify timing: a randomised crossover study. Diabetologia 59, 2572–2578.
Shute, R., Heesch, M., Laursen, T., and Slivka, D. (2017). Local muscle cooling does not impact expression of mitochondrial-related genes. J. Therm. Biol. 67, 35–39. doi: 10.1016/j.jtherbio.2017.04.008
Sjøberg, K. A., Frøsig, C., Kjøbsted, R., Sylow, L., Kleinert, M., Betik, A. C., et al. (2017). Exercise Increases Human Skeletal Muscle Insulin Sensitivity via Coordinated Increases in Microvascular Perfusion and Molecular Signaling. Diabetes 66, 1501–1510. doi: 10.2337/db16-1327
Stephens, J. M., Halson, S. L., Miller, J., Slater, G. J., and Askew, C. D. (2018). Influence of body composition on physiological responses to post-exercise hydrotherapy. J. Sports Sci. 36, 1044–1053. doi: 10.1080/02640414.2017.1355062
Tamura, Y., Matsunaga, Y., Masuda, H., Takahashi, Y., Takahashi, Y., Terada, S., et al. (2014). Postexercise whole body heat stress additively enhances endurance training-induced mitochondrial adaptations in mouse skeletal muscle. Am. J. Physiol. Regul. Integr. Comp. Physiol. 307, R931–R943.
Tavares, F., Beaven, C. M., Teles, J., Baker, D., Healy, P., Smith, T. B., et al. (2018). The Effects of Chronic Cold Water Immersion in Elite Rugby Players. Int. J. Sports Physiol. Perform. 14, 156–162. doi: 10.1123/ijspp.2018-0313
Thomas, K. N., van Rij, A. M., Lucas, S. J., Gray, A. R., and Cotter, J. D. (2016). Substantive hemodynamic and thermal strain upon completing lower-limb hot-water immersion; comparisons with treadmill running. Temperature 3, 286–297. doi: 10.1080/23328940.2016.1156215
Vaile, J., Halson, S., Gill, N., and Dawson, B. (2008). Effect of hydrotherapy on recovery from fatigue. Int. J. Sports Med. 29, 539–544. doi: 10.1055/s-2007-989267
Versey, N. G., Halson, S. L., and Dawson, B. T. (2013). Water immersion recovery for athletes: effect on exercise performance and practical recommendations. Sports Med. 43, 1101–1130. doi: 10.1007/s40279-013-0063-8
World Health Organization [WHO] (2009). Global health risks?: mortality and burden of disease attributable to selected major risks. Geneva: World Health Organization.
Yamane, M., Ohnishi, N., and Matsumoto, T. (2015). Does Regular Post-exercise Cold Application Attenuate Trained Muscle Adaptation? Int. J. Sports Med. 11:36.
Yamane, M., Teruya, H., Nakano, M., Ogai, R., Ohnishi, N., and Kosaka, M. (2006). Post-exercise leg and forearm flexor muscle cooling in humans attenuates endurance and resistance training effects on muscle performance and on circulatory adaptation. Eur. J. Appl. Physiol. 96, 572–580. doi: 10.1007/s00421-005-0095-3
Keywords: exercise, metabolic flexibility, glucose, hydrotherapy, water immersion
Citation: Russell BM, Chang CR, Hill T, Cotter JD and Francois ME (2021) Post-exercise Warm or Cold Water Immersion to Augment the Cardiometabolic Benefits of Exercise Training: A Proof of Concept Trial. Front. Physiol. 12:759240. doi: 10.3389/fphys.2021.759240
Received: 16 August 2021; Accepted: 05 October 2021;
Published: 03 November 2021.
Edited by:
Martin Burtscher, University of Innsbruck, AustriaReviewed by:
James Robert Broatch, Victoria University, AustraliaEmily C. LaVoy, University of Houston, United States
Copyright © 2021 Russell, Chang, Hill, Cotter and Francois. This is an open-access article distributed under the terms of the Creative Commons Attribution License (CC BY). The use, distribution or reproduction in other forums is permitted, provided the original author(s) and the copyright owner(s) are credited and that the original publication in this journal is cited, in accordance with accepted academic practice. No use, distribution or reproduction is permitted which does not comply with these terms.
*Correspondence: Monique E. Francois, francois@uow.edu.au