- 1Department of Medicinal Chemistry, Virginia Commonwealth University, Richmond, VA, United States
- 2Institute for Structural Biology, Drug Discovery and Development, Virginia Commonwealth University, Richmond, VA, United States
The human sirtuins are a group of NAD+-dependent protein deacylases. They “erase” acyl modifications from lysine residues in various cellular targets including histones, transcription factors, and metabolic enzymes. Through these far-reaching activities, sirtuins regulate a diverse array of biological processes ranging from gene transcription to energy metabolism. Human sirtuins have been intensely pursued by both academia and industry as therapeutic targets for a broad spectrum of diseases such as cancer, neurodegenerative diseases, and metabolic disorders. The last two decades have witnessed a flood of small molecule sirtuin regulators. However, there remain relatively few compounds targeting human sirtuins in clinical development. This reflects the inherent issues concerning the development of isoform-selective and potent molecules with good drug-like properties. In this article, small molecule sirtuin regulators that have advanced into clinical trials will be discussed in details as “successful” examples for future drug development. Special attention is given to the discovery of these compounds, the mechanism of action, pharmacokinetics analysis, formulation, as well as the clinical outcomes observed in the trials.
Introduction
Initially, sirtuins were classified as epigenetic “eraser” enzymes dedicated for the removal of acetyl groups from histone N-terminal lysine residues (Blander and Guarente, 2004; Trapp and Jung, 2006). The deacetylation of histones causes chromatin condensation, which is closely associated with transcription silencing (Shahbazian and Grunstein, 2007). Unlike the Zn2+-dependent histone deacetylases (HDACs), sirtuins carry out the chemical modifications in an NAD+-dependent fashion (Imai et al., 2000; Landry et al., 2000). Growing evidence suggests that sirtuins not only act on histone proteins, but also have other cellular targets such as transcription factors and metabolic enzymes (Sauve et al., 2006; Kosciuk et al., 2019). Furthermore, diverse catalytic activities have been uncovered for sirtuins, including, but not limited to, depropionylation, debutyrylation, desuccinylation, and de-fatty acylation (Du et al., 2011; Feldman et al., 2013; Jiang et al., 2013; Anderson et al., 2017). These pleiotropic enzymatic activities give sirtuins their far-reaching functions in maintaining genome integrity, regulating metabolism homeostasis, and promoting organismal longevity (Guarente and Picard, 2005; Cen et al., 2011; Watroba et al., 2017).
The human sirtuins, SIRT1-SIRT7, have been intensively investigated for their enzymatic activities and biological functions. There are numerous wonderful review articles highlighting the significance of these enzymes in regulating normal physiology and pathophysiology (Chang and Guarente, 2014; Herskovits and Guarente, 2014; Kumar and Lombard, 2018; Chang et al., 2020; Jaiswal et al., 2021). For example, overexpression of SIRT1 has been observed to increase carcinogenesis in prostate and thyroid tumors in mice with a deficiency of tumor suppressor PTEN (phosphatase and tensin homolog) (Herranz et al., 2013). SIRT1 also plays an important role in the development of drug resistance in chronic myeloid leukemia (CML) cells (Wang et al., 2013). It has been shown to activate error-prone DNA repair which can lead to increased incidence of genetic mutations (Wang et al., 2013). Thus, a SIRT1 inhibitor would be beneficial in combination with established chemotherapeutics to reduce drug resistance. Sirtuin activators can also play an important role in disease modulation. SIRT1 is considered to have a neuroprotective role in the brain, as it helps to regulate apoptosis and BDNF (brain-derived neurotrophic factor) expression (Luo et al., 2001; Zocchi and Sassone-Corsi, 2012). Activation of SIRT1 may be beneficial for the treatment of Alzheimer’s disease (AD), in which SIRT1 levels are typically reduced (Lutz et al., 2014). AD is characterized by the presence of amyloid plaques containing amyloid beta (Aβ) and neurofibrillary tangles (NFTs) containing hyperphosphorylated tau (Ittner and Götz, 2011). SIRT1 overexpression was shown to increase α-secretase activity, thus reducing the formation of Aβ (Endres and Fahrenholz, 2012). SIRT1 also prevents AD pathology through the deacetylation of tau (Min et al., 2010). Tau acetylation inhibits the degradation of tau and is detected in early stages of diseases with abnormal tau accumulation (Min et al., 2010). Therefore, SIRT1 serves as a possible therapeutic target for the treatment of AD.
Naturally, the development of small molecule regulators targeting human sirtuins has become a hot topic of research. Despite all the efforts over the last few decades, the success stories were scarce. Many small molecule sirtuin inhibitors and activators can only be called “chemical probes” at the present time due to the lack of isoform selectivity, moderate potency, limited bioavailability, and poor pharmacokinetic (PK) and pharmacodynamic (PD) profiles. There is a clear gap between the pre-clinical probe discovery and clinical drug candidate development. There is also a gap in the amount of research effort put into studying the various sirtuin isoforms. SIRT1 is by far the most studied isoform, with over 11,000 articles indexed in PubMed. In comparison, the other two most studied isoforms, SIRT2 and SIRT3, together have only around 3,700 articles. This disparity in research translates to fewer small molecule modulators targeting the other isoforms. Thus, the modulators that have entered clinical trials are primarily focused on SIRT1.
In this review, we will “tell the tales” of several human sirtuin regulators that have advanced into clinical investigation for the treatment of various diseases. The focus of the discussions will be the discovery of these compounds, their mechanism of action (MOA), and the rationale and outcome of the clinical trials. Although we brand these compounds as the “success” stories, they are not without controversy or limitation. On the flip side, the lessons we learn from these examples may help guide the design and development of the next generation of sirtuin regulators as therapeutic candidates.
For the benefit of the general audience, we would like to briefly discuss the basic theories behind the development of small molecule drug candidates toward clinical trials. The candidate compounds are normally small molecules, either natural products or synthetic compounds, with desired biological activity toward target proteins or enzymes in the in vitro setting. These candidates are the results of rounds of optimization for improved potency, selectivity, and solubility. For example, Lipinski’s rule of five (Ro5) has been the golden standard to prioritize the drug-like properties of orally active compounds (Lipinski et al., 2001). The “druggability” of the candidates will then be analyzed through ADME (absorption, distribution, metabolism and excretion) studies. These studies will assess the bioavailability, distribution, stability, and elimination of the candidate compounds. In the following sections, Cmax of certain sirtuin modulators will be discussed. This critical parameter in ADME analysis describes the maximum concentration of a candidate compound in targeted tissue/organ after administration. The results from ADME studies will guide the further optimization of the candidate molecules. The best dosage, administration route, and formulation need to be evaluated as well. The formulations of resveratrol (RSV) will be discussed in detail in the next section. The active ingredient, RSV, has been combined with variety of substances to improve its bioavailability.
Resveratrol and Related SRT Compounds
Resveratrol
In the 1980s, epidemiologists observed that developed countries with increased wine consumption had decreased deaths due to ischemic heart disease (IHD) (St Leger et al., 1979). This later came to be known as the “French Paradox” because of the lowered IHD mortality rates in France despite having no difference in saturated fat intake or blood cholesterol levels (Burr, 1995). In Bertelli et al. (1995) implicated resveratrol (RSV, Figure 1) as the mediator of the cardioprotective effects of wine, thus spurring interest in the molecule as a potential therapeutic. The association between RSV and SIRT1 was discovered in 2003 by high-throughput screening (HTS) utilizing a Fluor de Lys deacetylation assay on a library of plant-derived polyphenols (Howitz et al., 2003). Howitz et al. (2003) found that RSV activated SIRT1 deacetylase activity by decreasing the KM for both NAD+ and the acetylated peptide. In addition to its effects on SIRT1, RSV was also shown to act on a wide range of enzymes, including COX-1, cAMP degrading phosphodiesterases, and nuclear factor-κB (NF-κB) (Jang et al., 1997; Manna et al., 2000; Pezzuto, 2011; Park et al., 2012). Consequently, there is debate whether the observed effects of RSV treatment are due to SIRT1 activation. For example, RSV has been shown to promote autophagy and many have attributed this effect to SIRT1 activation (Morselli et al., 2010; Wu et al., 2011). But this assertion was disputed by a study that observed the direct inhibition of mTOR, an inhibitor of autophagy, by RSV (Park et al., 2016). Despite the promiscuous nature of RSV, a study by Price et al. (2012) found that the presence of SIRT1 was necessary for RSV-mediated mitochondrial biogenesis and AMPK activation.
After the discovery of RSV as a SIRT1 activator, the validity of the study and its use of the Fluor de Lys assay was disputed. As the assay uses a synthetic peptide substrate that contains a fluorophore, some argued that it was not physiologically relevant and could produce false positives. Kaeberlein et al. (2005) were able to replicate the activation of SIRT1 by RSV, but only when the fluorescent moiety was present on the peptide substrates. Other studies confirmed that the fluorophore was necessary for RSV-promoted activation of SIRT1 (Borra et al., 2005; Beher et al., 2009; Pacholec et al., 2010). In one such study, Pacholec et al. (2010) used NMR, SPR, and ITC to prove that the sirtuin-activating compounds (STACs) were directly interacting with the fluorophore attached to the peptide, even in the absence of SIRT1. Altogether, the contradictory results called into question the reliability of the fluorometric assay and raised serious doubts concerning RSV’s mechanism of action and its ability to bind to and activate SIRT1 (Schmidt, 2010).
In response to the controversy, scientists at Sirtris, a company established after the initial discovery of SIRT1 activation by RSV, provided an explanation for the importance of the fluorescent moiety. Dai et al. (2010) observed the formation of STAC-substrate complexes, but found no correlation between the potency of a STAC and its affinity for the fluorescent TAMRA group. They further found that the ability of a STAC to activate SIRT1 was dependent on the substrate structure and were able to induce RSV-activation of SIRT1 using substrates composed of natural amino acids (Dai et al., 2010). This finding was also seen by Lakshminarasimhan et al. (2013) in which they observed RSV-activation of SIRT1 after replacing the fluorophore with a large, hydrophobic residue. Hubbard et al. (2013) further found that the fluorophore or its hydrophobic replacement has a positional requirement and were able to show that hydrophobic motifs within PGC-1α and FOXO3a could facilitate SIRT1 activation. Additionally, they also determined that the conserved residue Glu230 was critical for RSV activation and found that the benefits of RSV were attenuated when SIRT1 Glu230 mutants were expressed in myoblasts (Hubbard et al., 2013).
After enough evidence was presented confirming an interaction between RSV and SIRT1, the focus shifted to identifying how RSV interacts with SIRT1. When the association between RSV and SIRT1 was first reported, RSV was categorized as an allosteric modulator of the “K system” type (Monod et al., 1965; Howitz et al., 2003). For “K system” modulators, the KM value is affected while the Vmax remains the same. Identifying the region of SIRT1 where RSV binds was the first step in characterizing the interaction. SIRT1 is comprised of three major structured regions: an N-terminal domain (183–229), a catalytic domain (229–516), and a C-terminal regulatory region (641–665) (Davenport et al., 2014). The apo enzyme undergoes a conformational change following the binding of NAD+ into a “closed” form that traps the substrate (Yuan and Marmorstein, 2012; Davenport et al., 2014). Using hydrogen deuterium exchange mass spectrometry (HDX-MS), Dai et al. (2015) were able to investigate the STAC binding domain (SBD) and determine that residues 183–229 were necessary for STAC activation of SIRT1. A crystal structure of a SIRT1/FdL peptide/RSV complex by Cao et al. (2015) indicated the binding of 3 RSV molecules for each SIRT1. Two of the RSV molecules form hydrogen bonds with the peptide and the SBD, thus bringing the two domains together in a “closed” conformation with improved substrate binding (Cao et al., 2015; Dai et al., 2018). The perspective of RSV acting as a stabilizing force between the substrate and SIRT1 has also been observed in a computational study in which RSV restored binding of “loose-binding” substrates (Hou et al., 2016).
Bioavailability is the primary obstacle in the development of RSV as a therapy. In human trials, Walle et al. (2004) observed that RSV had an absorption of ∼70%, but were unable to detect unmodified RSV 30 min after administration. After absorption, RSV is rapidly metabolized in the liver where it is conjugated to either sulfate or glucuronate (Springer and Moco, 2019). The RSV conjugates are produced by sulfotransferases (SULTs) and uridine-5′-diphospho-glucoronosyltransferases (UGTs) (Springer and Moco, 2019). Both SULTs and UGTs have genetic polymorphisms that can affect their ability to metabolize drugs, and thus can lead to high variability in levels of unmodified RSV between individuals (Ung and Nagar, 2007; Mehboob et al., 2017). The sulfate-conjugated metabolites, which are the primary conjugated form of RSV, were shown to have similar actions to RSV. Calamini et al. (2010) found that the sulfate conjugate of RSV was able to inhibit COX-1 and COX-2, and could activate SIRT1 only in the presence of a Fluor de Lys substrate. As the level of the conjugated forms of RSV surpass that of free RSV, it remains unclear which molecule is responsible for the experimentally observed effects of RSV supplementation.
Development of novel formulations of RSV emerged as its bioavailability became a prominent issue. One approach was to inhibit the enzymes responsible for conjugating RSV. Co-administration of RSV with quercetin was shown to decrease the formation of RSV-sulfate conjugates through the inhibition of SULT1A1, the major SULT isoform expressed in the liver and kidneys (De Santi et al., 2000). Multiple studies have observed the synergistic effect between RSV and quercetin from reducing adipose tissue weight following a high fat diet (Arias et al., 2016) to inhibiting the development of prostate cancer in a mouse model (Singh and Ahmad, 2015). In a similar fashion, Reen et al. (1993) combined RSV with piperine, an alkaloid previously shown to diminish the activity of UGTs in the intestines of rats. The study found that the addition of piperine to RSV resulted in a more than 1000% increase in the maximum serum concentration of RSV (Johnson et al., 2011). Despite the promising results seen in animal models, outcomes from human trials in healthy subjects were ambiguous (Table 1). Two trials co-administering RSV and quercetin found no improvement in RSV pharmacokinetics (la Porte et al., 2010; Huhn et al., 2018). Piperine supplementation was found to be useful in improving RSV bioavailability in one study examining cerebral blood flow (Wightman et al., 2014), but had no beneficial effect on serum levels of RSV in another study (Bailey et al., 2021).
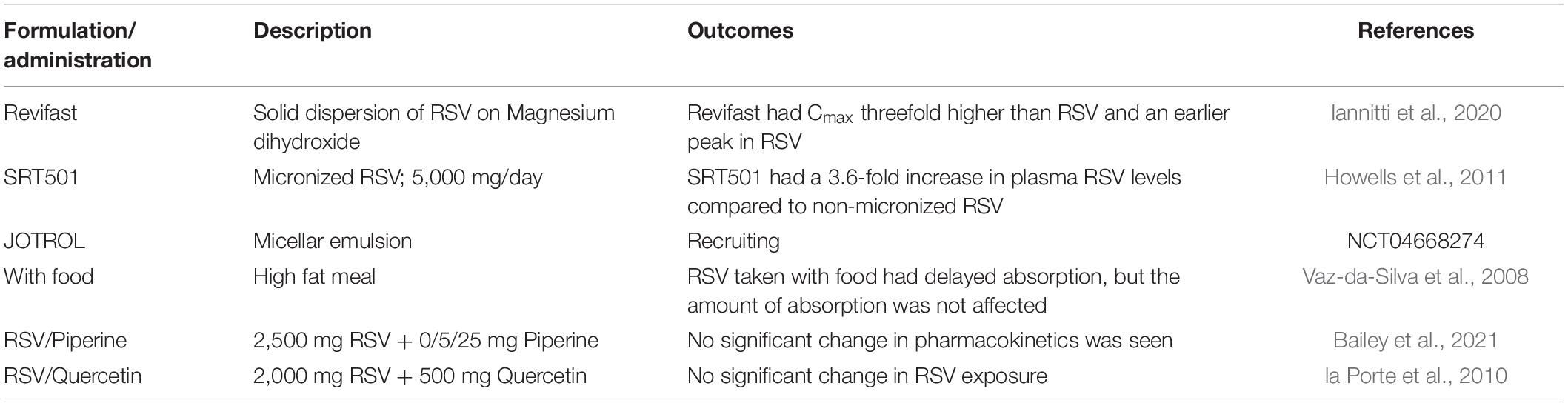
Table 1. Clinical trials examining pharmacokinetics of novel resveratrol formulations and administrations.
Other groups tried to solve the bioavailability issue by focusing on the drug delivery method. The Fioretti lab developed a novel formulation of RSV as a solid dispersion on Magnesium dihydroxide microparticles. In their early studies, they found that the new formulation (later termed Revifast) was three times more soluble than the unmodified RSV and had enhanced bioavailability in rabbits (Spogli et al., 2018). In human trials, Revifast displayed an earlier peak in RSV as well as a twofold increase in free-RSV levels in plasma (Iannitti et al., 2020). Another method used to increase bioavailability of RSV is micronization. Sirtris developed SRT501, in which they reduced the particle size below 5 μm in order to improve solubility by increasing the surface area of RSV. In an early clinical trial, SRT501 was well tolerated in colorectal cancer (CRC) patients and had an improved Cmax compared to conventional RSV (Howells et al., 2011). Following that success, SRT501 was used as a treatment for patients with refractory or relapsed multiple myeloma. The clinical trial was terminated early due to severe side effects including nephrotoxicity (Popat et al., 2013). SRT501 was later discontinued for development as the company focused on other SIRT1-activating drug candidates.
RSV has been extensively studied in clinical trials. In the NIH clinical trial registry, over 150 trials using RSV are in various stages of completion. The trials concerned with sirtuin activation and the resulting therapeutic effects have been compiled in Tables 2–9. When summarizing the clinical outcomes of RSV (Figure 2), the issues with RSV are apparent. For most disease states RSV had a neutral effect, suggesting that the bioavailability issues are a major obstacle. Most of the trials in which RSV had a positive effect had higher dosages, typically over 500 mg/day, and the more recent trials are trending toward higher dosages as well. Although it is unclear if RSV can directly activate sirtuins when taken orally, it is likely that work with RSV will continue, as it is a readily available natural product with limited adverse reactions. Despite the early promise seen in the lab, the current reality of RSV has shown the need for developing better, more selective activators of sirtuins.
Resveratrol-Related Activators
Following the discovery of RSV, Sirtris began to develop new small molecule activators of SIRT1. From HTS, they discovered SIRT1 activators that were structurally distinct from RSV and had improved SIRT1 activating abilities (Milne et al., 2007). One of the compounds, SRT1720 (Figure 1), activated SIRT1 with an EC1.5 = 2.9 μM and a maximum activation of approximately 4.5-fold, whereas RSV had an EC1.5 = 46.2 μM and a maximum activation of 2-fold (Milne et al., 2007). Using ITC, it was determined that the SRT STACs could only bind SIRT1 in the presence of the peptide substrate and they had a mechanism of action similar to RSV. It was further found that they used the same binding site as RSV. In a rodent model of insulin resistance, SRT1720 treatment resulted in a decrease in the blood glucose level and an increase in insulin sensitivity (Milne et al., 2007).
Thus far, clinical trials of three compounds related to SRT1720 have been completed. SRT2104 (Figure 1) has the greatest number of registered clinical trials (Table 10), but SRT2379 and SRT3025 (Figure 1) have also made it into the clinic (Table 11). The single clinical trial to assess the safety and pharmacokinetics of SRT3025 was interrupted after the researchers found a prolongation effect of SRT3025 on the corrected QT interval, a warning that continuation could lead to a potentially fatal proarrhythmia in the subjects (NCT01340911, GSK Study Register). Further development of SRT2379 was also terminated due to preclinical toxicities observed by the researchers (NCT01416376, GSK Study Register).
For the lead compound, SRT2104, 5 out of the 8 clinical trials that focused on clinical outcomes had neutral or statistically insignificant results. A pharmacokinetic study found that the bioavailability of SRT2104 was 14% and exposure was improved when administered with food (Hoffmann et al., 2013). Most trials observed highly variable pharmacokinetics, leading some to have inconsistent clinical outcomes. A few trials observed beneficial effects of SRT2104 treatment on lipid profiles (Baksi et al., 2014), histological examinations of subjects with psoriasis (Krueger et al., 2015), and inflammation (van der Meer et al., 2015). McCallum et al. (2014) tried to improve the pharmacokinetics of SRT2104 by using different release formulations, but were unsuccessful. As of current, it appears that SRT2104 is no longer in development. Despite issues observed in clinical trials, SRT2104 continues to be used in studies as a SIRT1 activator (Miller et al., 2021).
EX-527 (Selisistat)
Before 2005, many small molecule regulators targeting sirtuins showed modest potency, isoform selectivity, and solubility (Bedalov et al., 2001; Grozinger et al., 2001; Bitterman et al., 2002; Mai et al., 2005; Olaharski et al., 2005; Porcu and Chiarugi, 2005). Isoform-selective small molecule probes were highly sought after for a better understanding of the biological functions of these enzymes (Porcu and Chiarugi, 2005). Napper et al. (2005) performed an HTS of a library of 280,000 compounds against human recombinant SIRT1 using a fluorometric assay in order to expand available SIRT1 small-molecule probes. The initial screening identified indole as a viable scaffold for SIRT1 targeting. The subsequent SAR study led to the discovery of EX-527 (or Selisistat) and the structurally related compound CHIC-35 (Figure 3) as selective SIRT1 inhibitors with IC50 values of 0.098 and 0.063 μM, respectively (Napper et al., 2005). EX-527 exhibited 100-fold selectivity for SIRT1 over SIRT2/3, Class I/II HDACs, and NAD+ glycohydrolase (Napper et al., 2005). Kinetic studies indicated that EX-527 is an uncompetitive inhibitor of SIRT1 regarding NAD+; thus, inhibition depends on the concentration of NAD+ (Napper et al., 2005; Gertz et al., 2013). Additionally, EX-527 exhibits exceptional ADME properties such as oral bioavailability, metabolic stability, and membrane penetrability, thus elevating EX-527 from a small-molecule probe to a therapeutic candidate (Napper et al., 2005). In contrast, the study of CHIC-35 has been limited to pre-clinical investigations, primarily on its anti-inflammatory effects (Lugrin et al., 2013; Asad and Sachidanandan, 2020).
SAR studies of EX-527 revealed that the primary carboxamide was necessary for an effective inhibition and modifications to the lead compound were generally not well tolerated (Napper et al., 2005). The isoform selectivity of EX-527 was initially attributed to possible differences among the sirtuin active sites (Gertz et al., 2013; Broussy et al., 2020). A recent structural biology study suggested that EX-527 occupies the C-pocket (nicotinamide binding site) and a neighboring hydrophobic pocket which are highly conserved in the sirtuin family (Gertz et al., 2013). Further kinetic analysis indicated that the isoform selectivity of EX-527 stemmed from the differences in the kinetics of catalysis rather than any significant structural variation (Gertz et al., 2013; Broussy et al., 2020).
EX-527 has been explored as a potential therapeutic for Huntington’s disease (HD). HD is a neurodegenerative disease characterized by abnormal movements, personality changes, and cognitive decline (Bates et al., 2015). A hallmark of HD is the expression of the mutant Huntington gene (mHTT), which has an expansion of a cytosine-adenine-guanine (CAG) repeat (Bates et al., 2015; Tabrizi et al., 2020). This extension leads to the protein misfolding and the formation of protein aggregates in HD patients (Bates et al., 2015; Tabrizi et al., 2020). As such, a potential therapeutic approach has focused on the degradation and removal of these aggregates. Acetylation of mHTT can direct the protein to autophagosomes for degradation (Jeong et al., 2009), thus facilitating the removal of the mutant protein. SIRT1 has been pursued as a therapeutic target for the treatment of HD because it has been shown to deacetylate mHTT to prevent its degradation. Genetic loss or pharmacological inhibition of Sir2 (the Drosophila melanogaster homolog of human SIRT1) was found to be neuroprotective for mHTT-challenged fruit flies (Pallos et al., 2008). Indeed, Smith et al. (2014) demonstrated that in a Drosophila model of HD, 10 μM of EX-527 could rescue neurodegeneration at a comparable level as the genetic elimination of Sir2. Additionally, in the R6/2 mouse model of HD, EX-527 can restore neural functions at 5 and 20 mg/kg dosages (Smith et al., 2014). It is important to note that the role of SIRT1 in HD remains controversial, as some view it as protective in HD modulation (Duan, 2013).
The aforementioned preclinical animal studies were essential for clinical trials involving EX-527 as an HD treatment option (Table 12). In a Phase 1 trial (NCT01521832), EX-527 was assessed for its safety in healthy human subjects. The study employed healthy men and women with two separate dosage regimens: a single dosage of 5, 25, 75, 150, 300, or 600 mg, and multiple dosages of 100, 200, or 300 mg/day (Westerberg et al., 2015). In this study, EX-527 was found to be well-tolerated and safe after multiple doses of 300 mg/day and at a single dose of 600 mg. Based on the promising safety profile and dosing information, another clinical trial (NCT01485952) sought to investigate the feasibility of targeting SIRT1 as a potential treatment for HD (Sussmuth et al., 2015). In this study, human subjects with HD were treated with either 0, 10, or 100 mg/day of EX-527. There were improvements across the clinical, cognitive, and neuropsychiatric assessments from the baseline (day -1) to day 1 with no additional improvement at day 14 (Sussmuth et al., 2015).
A relatively new clinical application of EX-527 is improving in vitro fertilization (IVF) outcomes through the treatment of endometriosis (NCT04184323). Endometriosis is a chronic inflammatory reproductive disorder involving the growth of uterine endometrial cells outside of the uterine cavity (Zondervan et al., 2020). These delocalized endometrial growths can become lesions that lead to chronic localized pelvic pain and cramping with accompanying fertility issues. Standard treatment options for endometriosis involve mechanical lesion removal, hormonal therapy, or a combination of the two (Budinetz and Sanfilippo, 2010). However, reemergence of the lesions and complications associated with the hormonal therapy remain limitations. Thus, alternative treatment options are needed. A recent study demonstrated a KRAS activation-triggered SIRT1 overexpression in women with endometriosis, which has been suggested to contribute to infertility and the pathogenesis of endometriosis (Yoo et al., 2017). Targeting SIRT1 with small molecule inhibitors thus serves as a potential therapeutic treatment for endometriosis-mediated IVF failure. A planned clinical trial (NCT04184323) will seek to explore inhibition of SIRT1 by EX-527 as a possible treatment for the inflammation associated with endometriosis.
Quercetin
Quercetin (Figure 4) is a flavonoid phytoestrogen which has demonstrated activity in the management of brain, blood, salivary gland and uterine cancers (Dolatabadi, 2011; Sak, 2014; Sinaga et al., 2017), as well as viral infections such as HCV (Rojas et al., 2016) and Zika virus (Wong et al., 2017) and bacterial infections (Wang et al., 2018; Zeng et al., 2019; Oktyabrsky et al., 2020) in both in vivo and in vitro studies. The structure of quercetin consists of three rings and five hydroxyl groups at the 3, 5, 7, 3′ and 4′-positions of the basic flavanol skeleton (Figure 4). The name “quercetin” was derived from the Latin word “Quercetum” which means oak forest. It is one of the most abundant flavonoids found in fruits and vegetables, and was discovered alongside other bioflavonoids by Albert Szent Gyorgyi in 1936 (Moskaug, Carlsen et al., 2004).
In the same HTS that identified RSV, quercetin was observed to increase SIRT1 activity by fivefold (Howitz et al., 2003). Studies have shown the inhibitory role of quercetin on the progression of breast, colon, prostate, and lung cancers (Baghel et al., 2012; Smith et al., 2016). Quercetin has been found to alleviate kidney fibrosis, intervertebral disc degeneration, and diabetic encephalopathy via activation of SIRT1-mediated pathways (Dong et al., 2014; Hu et al., 2020; Liu et al., 2020). Quercetin treatment of Herpes simplex virus-1 infected neuronal cell lines increased the survival of the cells by inhibiting viral production and improved neurodegenerative markers via SIRT1 activation (Leyton et al., 2015). It was also found that quercetin inhibits oxidative injury in human endothelial cells through SIRT1 activation, leading to the upregulation of the SIRT1/AMPK pathway (Chen et al., 2013). In addition, quercetin regulates oxidative stress in the body by directly reducing the level of reactive oxygen species (Oboh et al., 2016). In myocardial/ischemia-reperfusion (MI/R) injury in rats, quercetin supplementation increased the expression levels of SIRT1 and PGC-1α, leading to the activation of the SIRT1/PGC-1α pathway and subsequent reduction in MI/R-induced myocardial damage (Tang et al., 2019). In addition to SIRT1, quercetin has a mild stimulating effect on SIRT6 (You et al., 2019). When modified with a bulky trihydroxy benzoyl group at the 3-OH group, as in catechin gallate, it inhibits SIRT6 activity (Rahnasto-Rilla et al., 2018; You et al., 2019).
Quercetin exists as a glycone or an aglycone in plants. When ingested, the glycone form can be hydrolyzed to the aglycone form that can be easily absorbed in the small intestine due to its hydrophobic nature (Massi et al., 2017). In human plasma, ingested quercetin glycosides are predominantly metabolized into quercetin 3-O-β-D-glucuronide and quercetin 3′-O-sulfate (Figure 4; D’Andrea, 2015; Moodi et al., 2021). Modifications such as glycosylation and methylation of the quercetin scaffold result in derivatives with distinct biological activities (Lesjak et al., 2018). For example, isoquercetin, the 3-O-glucoside of quercetin (Magar and Sohng, 2020), demonstrates SIRT6 stimulation activity with no influence on SIRT1 activity (You et al., 2019).
Quercetin’s therapeutic applications have been limited by its low bioavailability, poor solubility, and short half-life (Gugler et al., 1975; Ferry et al., 1996; KaŞıkcı and Bağdatlıoğlu, 2016). Modifications have been made to improve these properties (Massi et al., 2017). In one study, the bioavailability of quercetin was increased by about 20 times through a phytosome delivery system (Riva et al., 2019). Several other studies have utilized conjugation to various amino acids and nanoparticle delivery systems to improve the bioavailability of quercetin. Acylated quercetin analogs synthesized by Duan et al. (2017) were about 10-fold more soluble in water than quercetin. Of the several quercetin human clinical trials targeting various disease states, only one ongoing study (NCT03943459) is aimed at investigating the activation of SIRT1 by quercetin in coronary disease.
Conclusion and Perspectives
A simple search in PubMed provides hundreds of publications related to sirtuin inhibitor/activator development, demonstrating the critical roles these enzymes play in regulating diverse cellular events and the intense interest in pursuing them as therapeutic targets. Unfortunately, tremendous efforts have only resulted in a handful of small molecules in clinical studies as described in this review article. Translating sirtuin regulators from the bench to the clinics has been hampered by the lack of isoform-selective candidate compounds with favorable pharmacological profiles. The catalytic domain is highly conserved between sirtuins and therefore represents a promiscuous target for NAD+ or peptide-competitive inhibitors (Dai et al., 2018). In the case of activators, the binding sites are often not readily apparent by the inspection of a crystal structure, and there is no general and systematic strategy to identify and target these sites. Furthermore, for several human sirtuin isoforms, novel enzymatic activities were discovered recently (Du et al., 2011; Feldman et al., 2013; Jiang et al., 2013; Anderson et al., 2017). Potent regulators targeting specifically these new activities are still in the making. Pre-clinical investigations using animal models may differ in the genetic background or the assessment methods which have caused controversies and ambiguities that still need to be reconciled. In spite of numerous reports on the endogenous substrates of sirtuins and the pathways they regulate, our understanding of the biological functions of sirtuins is still in its infancy. For example, SIRT1 has been closely associated with cancer pathology, and has been suggested as either a tumor promoter or a suppressor in a context-dependent manner (Deng, 2009). All the research effort has only scratched the surface of sirtuin biology. A comprehensive and thorough picture of these intriguing enzymes still awaits description.
Of course, sirtuin-targeting drugs still hold great therapeutic potential, and progress in the field will accelerate the development of small molecule drug candidates. Apart from their highly conserved catalytic core, sirtuins harbor structurally unique N- or C-terminal extensions that can be targeted for selectivity or even specificity. The conformational plasticity of the active site that explains the isoform selectivity of EX-527 (Gertz et al., 2013; Broussy et al., 2020) has also been suggested as a novel target for inhibitor development. The clinical success of sirtuin-targeting medications requires a clear understanding of the “sirtuin-dependency” of the disease, robust lead compounds that are potent and selective with ideal drug-like properties, PK/PD profiling and improvement, as well as advances in formulation. The combined efforts from all these aspects will bring more sirtuin regulators into the clinic for treating diseases with considerable unmet medical needs.
Author Contributions
AC, DW, and YC: conceptualization. AC, DW, DD, and YC: writing. YC: project administration and funding acquisition. All authors contributed to the article and approved the submitted version.
Funding
This work was supported in part by CHE-1846785 from NSF (to YC), 2020 VCU CCTR Endowment Fund (sub-award of UL1TR002649 from National Center for Advancing Translational Sciences to VCU) (to YC), Jeffress Trust Award in Interdisciplinary Research from Thomas F. and Kate Miller Jeffress Memorial Trust and Bank of America (to YC), 2020 Presidential Research Quest Fund from VCU (to YC), and start-up funds from VCU (to YC).
Conflict of Interest
The authors declare that the research was conducted in the absence of any commercial or financial relationships that could be construed as a potential conflict of interest.
Publisher’s Note
All claims expressed in this article are solely those of the authors and do not necessarily represent those of their affiliated organizations, or those of the publisher, the editors and the reviewers. Any product that may be evaluated in this article, or claim that may be made by its manufacturer, is not guaranteed or endorsed by the publisher.
Abbreviations
A β, Amyloid beta; AD, Alzheimer’s disease; ADME, Absorptiondistribution, metabolism excretion; AE, Adverse effect; AMPK, AMP-activated protein kinase; BBB, Blood-brain barrier; BDNF, Brain-derived neurotrophic factor; Cmax, Maximum concentration of a drug in the intended organ/tissue after administration; CML, Chronic myeloid leukemia; COPD, Chronic obstructive pulmonary disease; COX, Cyclooxygenase; CRC, Colorectal cancer; EC1.5, Concentration at which the enzyme activity is increased by 50%; FOXO, Forkhead box transcription factor; GI, gastrointestinal; HD, Huntington’s disease; HDACS, Histone deacetylases; HDL, High density lipoprotein; HDX-MS, Hydrogen deuterium exchange mass spectrometry; HTS, High-throughput screening; IC50, Half-maximal inhibitory concentration; IGFBP, Insulin-like growth factor binding protein; IGF-I, Insulin-like growth factor I; IHD, Ischemic heart disease; ITC, Isothermal colorimetry; Km, Michaelis-Menten constant; LDL, Low density lipoprotein; mHTT, mutant Huntington gene; MI/R- Myocardial/ischemia-reperfusion, MOA, Mechanism of action; NAD, Nicotinamide adenine dinucleotide; NAFLD, Non-alcoholic fatty liver disease; NF- κ B, Nuclear factor κ B; NFTs, Neurofibrillary tangles; NMR, Nuclear magnetic resonance; PD, Pharmacodynamic; PGC, Peroxisome proliferator-activated receptor-gamma coactivator; PK, Pharmacokinetic; PTEN, Phosphatase and tensin homolog; RSV, Resveratrol; SAR, Structure-activity relationship; SBD, STAC binding domain; SPR, Surface plasmon resonance; STACs, Sirtuin-activating compounds; SULTs, Sulfotransferases; TAMRA, Tetramethylrhodamine; T2D, Type 2 diabetes mellitus; UC, Ulcerative colitis; UGTs, Uuridine-5′-diphospho-glucoronosyltransferases.
References
Aftanas, L. I., Markov, A. A., Rikita, M. V., and Danilenko, K. V. (2020). P.326 Efficacy of resveratrol in the treatment of unipolar depression: double-blind randomized placebo-controlled parallel-group study. Eur. Neuropsychopharmacol. 40:S189.
Agarwal, B., Campen, M. J., Channell, M. M., Wherry, S. J., Varamini, B., Davis, J. G., et al. (2013). Resveratrol for primary prevention of atherosclerosis: clinical trial evidence for improved gene expression in vascular endothelium. Int. J. Cardiol. 166, 246–248. doi: 10.1016/j.ijcard.2012.09.027
Anderson, K. A., Huynh, F. K., Fisher-Wellman, K., Stuart, J. D., Peterson, B. S., Douros, J. D., et al. (2017). SIRT4 is a lysine deacylase that controls leucine metabolism and insulin secretion. Cell Metab. 25, 838–855.e15. doi: 10.1016/j.cmet.2017.03.003
Anton, S. D., Embry, C., Marsiske, M., Lu, X., Doss, H., Leeuwenburgh, C., et al. (2014). Safety and metabolic outcomes of resveratrol supplementation in older adults: results of a twelve-week, placebo-controlled pilot study. Exp. Gerontol. 57, 181–187. doi: 10.1016/j.exger.2014.05.015
Arias, N., Macarulla, M. T., Aguirre, L., Milton, I., and Portillo, M. P. (2016). The combination of resveratrol and quercetin enhances the individual effects of these molecules on triacylglycerol metabolism in white adipose tissue. Eur. J. Nutr. 55, 341–348. doi: 10.1007/s00394-015-0854-9
Asad, Z., and Sachidanandan, C. (2020). Chemical screens in a zebrafish model of CHARGE syndrome identifies small molecules that ameliorate disease-like phenotypes in embryo. Eur. J. Med. Genet. 63:103661. doi: 10.1016/j.ejmg.2019.04.018
Asghari, S., Asghari-Jafarabadi, M., Somi, M.-H., Ghavami, S.-M., and Rafraf, M. (2018). Comparison of calorie-restricted diet and resveratrol supplementation on anthropometric indices, metabolic parameters, and serum Sirtuin-1 levels in patients with nonalcoholic fatty liver disease: a randomized controlled clinical trial. J. Am. Coll. Nutr. 37, 223–233. doi: 10.1080/07315724.2017.1392264
Baghel, S. S., Shrivastava, N., Baghel, R. S., Agrawal, P., and Rajput, S. (2012). A review of quercetin: antioxidant and anticancer properties. World J. Pharm. Pharm. Sci. 1, 146–160.
Bailey, H. H., Johnson, J. J., Lozar, T., Scarlett, C. O., Wollmer, B. W., Kim, K., et al. (2021). A randomized, double-blind, dose-ranging, pilot trial of piperine with resveratrol on the effects on serum levels of resveratrol. Eur. J. Cancer Prev. 30, 285–290.
Baksi, A., Kraydashenko, O., Zalevkaya, A., Stets, R., Elliott, P., Haddad, J., et al. (2014). A phase II, randomized, placebo-controlled, double-blind, multi-dose study of SRT2104, a SIRT1 activator, in subjects with type 2 diabetes. Br. J. Clin. Pharmacol. 78, 69–77. doi: 10.1111/bcp.12327
Baldassarre, M. E. Di Mauro, A. Labellarte, G. Pignatelli, M. Fanelli, M. Schiavi, E. Mastromarino, P., Capozza, M., Panza, R., and Laforgia, N. (2020). Resveratrol plus carboxymethyl-β-glucan in infants with common cold: a randomized double-blind trial. Heliyon 6:e03814. doi: 10.1016/j.heliyon.2020.e03814
Banaszewska, B., Wrotyńska-Barczyńska, J., Spaczynski, R. Z., Pawelczyk, L., and Duleba, A. J. (2016). Effects of resveratrol on polycystic ovary syndrome: a double-blind, randomized, placebo-controlled trial. J. Clin. Endocrinol. Metab. 101, 4322–4328. doi: 10.1210/jc.2016-1858
Bates, G. P., Dorsey, R., Gusella, J. F., Hayden, M. R., Kay, C., Leavitt, B. R., et al. (2015). Huntington disease. Nat. Rev. Dis. Primers 1:15005.
Batista-Jorge, G. C., Barcala-Jorge, A. S., Silveira, M. F., Lelis, D. F., Andrade, J. M. O., de Paula, A. M. B., et al. (2020). Oral resveratrol supplementation improves Metabolic Syndrome features in obese patients submitted to a lifestyle-changing program. Life Sci. 256:117962. doi: 10.1016/j.lfs.2020.117962
Bedalov, A., Gatbonton, T., Irvine, W. P., Gottschling, D. E., and Simon, J. A. (2001). Identification of a small molecule inhibitor of Sir2p. Proc. Natl. Acad. Sci. U.S.A. 98, 15113–15118.
Beher, D., Wu, J., Cumine, S., Kim, K. W., Lu, S.-C., Atangan, L., et al. (2009). Resveratrol is not a direct activator of SIRT1 enzyme activity. Chem. Biol. Drug Des. 74, 619–624.
Beijers, R. J., Gosker, H. R., Sanders, K. J., de Theije, C., Kelders, M., Clarke, G., et al. (2020). Resveratrol and metabolic health in COPD: a proof-of-concept randomized controlled trial. Clin. Nutr. 39, 2989–2997. doi: 10.1016/j.clnu.2020.01.002
Bertelli, A. A., Giovannini, L., Giannessi, D., Migliori, M., Bernini, W., Fregoni, M., et al. (1995). Antiplatelet activity of synthetic and natural resveratrol in red wine. Int. J. Tissue React. 17, 1–3.
Bitterman, K. J., Anderson, R. M., Cohen, H. Y., Latorre-Esteves, M., and Sinclair, D. A. (2002). Inhibition of silencing and accelerated aging by nicotinamide, a putative negative regulator of yeast sir2 and human SIRT1. J. Biol. Chem. 277, 45099–45107. doi: 10.1074/jbc.M205670200
Blander, G., and Guarente, L. (2004). The Sir2 family of protein deacetylases. Annu. Rev. Biochem. 73, 417–435.
Bo, S., Ciccone, G., Castiglione, A., Gambino, R., De Michieli, F., Villois, P., et al. (2013). Anti-inflammatory and antioxidant effects of resveratrol in healthy smokers a randomized, double-blind, placebo-controlled, cross-over trial. Curr. Med. Chem. 20, 1323–1331. doi: 10.2174/0929867311320100009
Bo, S., Togliatto, G., Gambino, R., Ponzo, V., Lombardo, G., Rosato, R., et al. (2018). Impact of sirtuin-1 expression on H3K56 acetylation and oxidative stress: a double-blind randomized controlled trial with resveratrol supplementation. Acta Diabetol. 55, 331–340. doi: 10.1007/s00592-017-1097-4
Borra, M. T., Smith, B. C., and Denu, J. M. (2005). Mechanism of human SIRT1 activation by resveratrol. J. Biol. Chem. 280, 17187–17195.
Broussy, S., Laaroussi, H., and Vidal, M. (2020). Biochemical mechanism and biological effects of the inhibition of silent information regulator 1 (SIRT1) by EX-527 (SEN0014196 or selisistat). J. Enzyme Inhib. Med. Chem. 35, 1124–1136. doi: 10.1080/14756366.2020.1758691
Brown, V. A., Patel, K. R., Viskaduraki, M., Crowell, J. A., Perloff, M., Booth, T. D., et al. (2010). Repeat dose study of the cancer chemopreventive agent resveratrol in healthy volunteers: safety, pharmacokinetics, and effect on the insulin-like growth factor axis. Cancer Res. 70, 9003–9011. doi: 10.1158/0008-5472.CAN-10-2364
Budinetz, T., and Sanfilippo, J. S. (2010). Treatment of endometriosis: a hormonal approach. Minerva Ginecol. 62, 373–380.
Calamini, B., Ratia, K., Malkowski, M. G., Cuendet, M., Pezzuto, J. M., Santarsiero, B. D., et al. (2010). Pleiotropic mechanisms facilitated by resveratrol and its metabolites. Biochem. J. 429, 273–282. doi: 10.1042/BJ20091857
Cao, D., Wang, M., Qiu, X., Liu, D., Jiang, H., Yang, N., et al. (2015). Structural basis for allosteric, substrate-dependent stimulation of SIRT1 activity by resveratrol. Genes Dev. 29, 1316–1325. doi: 10.1101/gad.265462.115
Cen, Y., Youn, D. Y., and Sauve, A. A. (2011). Advances in characterization of human sirtuin isoforms: chemistries, targets and therapeutic applications. Curr. Med. Chem. 18, 1919–1935.
Chang, A. R., Ferrer, C. M., and Mostoslavsky, R. (2020). SIRT6, a Mammalian Deacylase with Multitasking Abilities. Physiol. Rev. 100, 145–169. doi: 10.1152/physrev.00030.2018
Chang, H. C., and Guarente, L. (2014). SIRT1 and other sirtuins in metabolism. Trends Endocrinol. Metab. 25, 138–145.
Chen, Z., Shentu, T.-P., Wen, L., Johnson, D. A., and Shyy, J. Y. J. (2013). Regulation of SIRT1 by oxidative stress-responsive miRNAs and a systematic approach to identify its role in the endothelium. Antioxid. Redox Signal. 19, 1522–1538. doi: 10.1089/ars.2012.4803
Chow, H. H. S., Garland, L. L., Hsu, C.-H., Vining, D. R., Chew, W. M., Miller, J. A., et al. (2010). Resveratrol modulates drug- and carcinogen-metabolizing enzymes in a healthy volunteer study. Cancer Prev. Res. 3:1168. doi: 10.1158/1940-6207.CAPR-09-0155
Dai, H., Case, A. W., Riera, T. V., Considine, T., Lee, J. E., Hamuro, Y., et al. (2015). Crystallographic structure of a small molecule SIRT1 activator-enzyme complex. Nat. Commun. 6:7645. doi: 10.1038/ncomms8645
Dai, H., Kustigian, L., Carney, D., Case, A., Considine, T., Hubbard, B. P., et al. (2010). SIRT1 activation by small molecules: kinetic and biophysical evidence for direct interaction of enzyme and activator. J. Biol. Chem. 285, 32695–32703. doi: 10.1074/jbc.M110.133892
Dai, H., Sinclair, D. A., Ellis, J. L., and Steegborn, C. (2018). Sirtuin activators and inhibitors: Promises, achievements, and challenges. Pharmacol. Ther. 188, 140–154. doi: 10.1016/j.pharmthera.2018.03.004
D’Andrea, G. (2015). Quercetin: a flavonol with multifaceted therapeutic applications? Fitoterapia 106, 256–271. doi: 10.1016/j.fitote.2015.09.018
Dash, S., Xiao, C., Morgantini, C., Szeto, L., and Lewis, G. F. (2013). High-dose resveratrol treatment for 2 weeks inhibits intestinal and hepatic lipoprotein production in overweight/obese men. Arterioscler. Thromb. Vasc. Biol. 33, 2895–2901. doi: 10.1161/ATVBAHA.113.302342
Davenport, A. M., Huber, F. M., and Hoelz, A. (2014). Structural and functional analysis of human SIRT1. J. Mol. Biol. 426, 526–541.
de Ligt, M., Bergman, M., Fuentes, R. M., Essers, H., Moonen-Kornips, E., Havekes, B., et al. (2020). No effect of resveratrol supplementation after 6 months on insulin sensitivity in overweight adults: a randomized trial. Am. J. Clin. Nutr. 112, 1029–1038. doi: 10.1093/ajcn/nqaa125
de Ligt, M., Bruls, Y. M. H., Hansen, J., Habets, M. F., Havekes, B., Nascimento, E. B. M., et al. (2018). Resveratrol improves ex vivo mitochondrial function but does not affect insulin sensitivity or brown adipose tissue in first degree relatives of patients with type 2 diabetes. Mol. Metab. 12, 39–47.
De Santi, C., Pietrabissa, A., Spisni, R., Mosca, F., and Pacifici, G. M. (2000). Sulphation of resveratrol, a natural compound present in wine, and its inhibition by natural flavonoids. Xenobiotica 30, 857–866.
Deng, C. X. (2009). SIRT1, is it a tumor promoter or tumor suppressor? Int. J. Biol. Sci. 5, 147–152.
Djurica, D., Ren, J., Holt, R. R., Feng, X., Carlson, C. R., Shindel, A. W., et al. (2016). A single intake of a resveratrol-arginine conjugate improves microvascular function compared to trans-resveratrol in postmenopausal women. Pharma Nutr. 4, 132–138.
Dolatabadi, J. E. N. (2011). Molecular aspects on the interaction of quercetin and its metal complexes with DNA. Int. J. Biol. Macromol. 48, 227–233. doi: 10.1016/j.ijbiomac.2010.11.012
Dong, J., Zhang, X., Zhang, L., Bian, H. X., Xu, N., Bao, B., and Liu, J. (2014). Quercetin reduces obesity-associated ATM infiltration and inflammation in mice: a mechanism including AMPKalpha1/SIRT1. J. Lipid Res. 55, 363–374. doi: 10.1194/jlr.M038786
Du, J., Zhou, Y., Su, X., Yu, J. J., Khan, S., Jiang, H., et al. (2011). Sirt5 is a NAD-dependent protein lysine demalonylase and desuccinylase. Science 334, 806–809.
Duan, W. (2013). Targeting sirtuin-1 in Huntington’s disease: rationale and current status. CNS Drugs 27, 345–352. doi: 10.1007/s40263-013-0055-0
Duan, Y., Sun, N., Xue, M., Wang, X., and Yang, H. (2017). Synthesis of regioselectively acylated quercetin analogues with improved antiplatelet activity. Mol. Med. Rep. 16, 9735–9740. doi: 10.3892/mmr.2017.7781
Endres, K., and Fahrenholz, F. (2012). The role of the anti-amyloidogenic secretase ADAM10 in shedding the app-like proteins. Curr. Alzheimer Res. 9, 157–164. doi: 10.2174/156720512799361664
Faghihzadeh, F., Adibi, P., Rafiei, R., and Hekmatdoost, A. (2014). Resveratrol supplementation improves inflammatory biomarkers in patients with nonalcoholic fatty liver disease. Nutr. Res. 34, 837–843. doi: 10.1016/j.nutres.2014.09.005
Feldman, J. L., Baeza, J., and Denu, J. M. (2013). Activation of the protein deacetylase SIRT6 by long-chain fatty acids and widespread deacylation by mammalian sirtuins. J. Biol. Chem. 288, 31350–31356. doi: 10.1074/jbc.C113.511261
Ferry, D. R., Smith, A., Malkhandi, J., Fyfe, D. W., deTakats, P. G., Anderson, D., et al. (1996). Phase I clinical trial of the flavonoid quercetin: pharmacokinetics and evidence for in vivo tyrosine kinase inhibition. Clin. Cancer Res. 2, 659–668.
Gertz, M., Fischer, F., Nguyen, G. T., Lakshminarasimhan, M., Schutkowski, M., Weyand, M., et al. (2013). Ex-527 inhibits Sirtuins by exploiting their unique NAD+-dependent deacetylation mechanism. Proc. Natl. Acad. Sci. U.S.A. 110, E2772-E2781. doi: 10.1073/pnas.1303628110
Gliemann, L., Schmidt, J. F., Olesen, J., Biensø, R. S., Peronard, S. L., Grandjean, S. U., et al. (2013). Resveratrol blunts the positive effects of exercise training on cardiovascular health in aged men. J. Physiol. 591, 5047–5059. doi: 10.1113/jphysiol.2013.258061
Goh, K. P., Lee, H. Y., Lau, D. P., Supaat, W., Chan, Y. H., and Koh, A. F. (2014). Effects of resveratrol in patients with type 2 diabetes mellitus on skeletal muscle SIRT1 expression and energy expenditure. Int. J. Sport Nutr. Exerc. Metab. 24, 2–13. doi: 10.1123/ijsnem.2013-0045
Grozinger, C. M., Chao, E. D., Blackwell, H. E., Moazed, D., and Schreiber, S. L. (2001). Identification of a class of small molecule inhibitors of the sirtuin family of NAD-dependent deacetylases by phenotypic screening. J. Biol. Chem. 276, 38837–38843. doi: 10.1074/jbc.M106779200
Guarente, L., and Picard, F. (2005). Calorie restriction–the SIR2 connection. Cell 120, 473–482. doi: 10.1016/j.cell.2005.01.029
Gugler, R., Leschik, M., and Dengler, H. J. (1975). Disposition of quercetin in man after single oral and intravenous doses. Eur. J. Clin. Pharmacol. 9(2-3), 229–234. doi: 10.1007/BF00614022
Harper, S. A., Bassler, J. R., Peramsetty, S., Yang, Y., Roberts, L. M., Drummer, D., et al. (2021). Resveratrol and exercise combined to treat functional limitations in late life: a pilot randomized controlled trial. Exp. Gerontol. 143:111111.
Heebøll, S., Kreuzfeldt, M., Hamilton-Dutoit, S., Kjaer Poulsen, M., Stødkilde-Jørgensen, H., Møller, H. J., et al. (2016). Placebo-controlled, randomised clinical trial: high-dose resveratrol treatment for non-alcoholic fatty liver disease. Scand. J. Gastroenterol. 51, 456–464.
Herranz, D., Maraver, A., Cañamero, M., Gómez-López, G., Inglada-Pérez, L., Robledo, M., et al. (2013). SIRT1 promotes thyroid carcinogenesis driven by PTEN deficiency. Oncogene 32, 4052–4056. doi: 10.1038/onc.2012.407
Herskovits, A. Z., and Guarente, L. (2014). SIRT1 in neurodevelopment and brain senescence. Neuron 81, 471–483. doi: 10.1016/j.neuron.2014.01.028
Hodgin, K. S., Donovan, E. K., Kekes-Szabo, S., Lin, J. C., Feick, J., Massey, R. L., et al. (2021). A placebo-controlled, pseudo-randomized, crossover trial of botanical agents for gulf war illness: resveratrol (Polygonum cuspidatum), Luteolin, and Fisetin (Rhus succedanea). Int. J. Environ. Res. Public Health 18:2483. doi: 10.3390/ijerph18052483
Hoffmann, E., Wald, J., Lavu, S., Roberts, J., Beaumont, C., Haddad, J., et al. (2013). Pharmacokinetics and tolerability of SRT2104, a first-in-class small molecule activator of SIRT1, after single and repeated oral administration in man. Br. J. Clin. Pharmacol. 75, 186–196. doi: 10.1111/j.1365-2125.2012.04340.x
Hoseini, A., Namazi, G., Farrokhian, A., Reiner, Ž., Aghadavod, E., Bahmani, F., et al. (2019). The effects of resveratrol on metabolic status in patients with type 2 diabetes mellitus and coronary heart disease. Food Funct. 10, 6042–6051. doi: 10.1039/c9fo01075k
Hou, X., Rooklin, D., Fang, H., and Zhang, Y. (2016). Resveratrol serves as a protein-substrate interaction stabilizer in human SIRT1 activation. Sci. Rep. 6:38186. doi: 10.1038/srep38186
Howells, L. M., Berry, D. P., Elliott, P. J., Jacobson, E. W., Hoffmann, E., Hegarty, B., et al. (2011). Phase I randomized, double-blind pilot study of micronized resveratrol (SRT501) in patients with hepatic metastases–safety, pharmacokinetics, and pharmacodynamics. Cancer Prev. Res. 4, 1419–1425. doi: 10.1158/1940-6207.CAPR-11-0148
Howitz, K. T., Bitterman, K. J., Cohen, H. Y., Lamming, D. W., Lavu, S., Wood, J. G., et al. (2003). Small molecule activators of sirtuins extend Saccharomyces cerevisiae lifespan. Nature 425, 191–196. doi: 10.1038/nature01960
Hu, T., Lu, X. Y., Shi, J. J., Liu, X. Q., Chen, Q. B., Wang, Q., et al. (2020). Quercetin protects against diabetic encephalopathy via SIRT1/NLRP3 pathway in db/db mice. J. Cell. Mol. Med. 24, 3449–3459. doi: 10.1111/jcmm.15026
Hubbard, B. P., Gomes, A. P., Dai, H., Li, J., Case, A. W., Considine, T., et al. (2013). Evidence for a common mechanism of SIRT1 regulation by allosteric activators. Science 339, 1216–1219. doi: 10.1126/science.1231097
Huhn, S., Beyer, F., Zhang, R., Lampe, L., Grothe, J., Kratzsch, J., et al. (2018). Effects of resveratrol on memory performance, hippocampus connectivity and microstructure in older adults – A randomized controlled trial. Neuroimage 174, 177–190.
Iannitti, R. G., Floridi, A., Lazzarini, A., Tantucci, A., Russo, R., Ragonese, F., et al. (2020). Resveratrol supported on magnesium DiHydroxide (Resv@MDH) represents an oral formulation of resveratrol with better gastric absorption and bioavailability respect to pure resveratrol. Front. Nutr. 7:570047. doi: 10.3389/fnut.2020.570047
Imai, S., Armstrong, C. M., Kaeberlein, M., and Guarente, L. (2000). Transcriptional silencing and longevity protein Sir2 is an NAD-dependent histone deacetylase. Nature 403, 795–800.
Ittner, L. M., and Götz, J. (2011). Amyloid-β and tau — a toxic pas de deux in Alzheimer’s disease. Nat. Rev. Neurosci. 12, 67–72.
Jaiswal, A., Xudong, Z., Zhenyu, J., and Saretzki, G. (2021). Mitochondrial sirtuins in stem cells and cancer. FEBS J. doi: 10.1111/febs.15879 [Epub ahead of print].
Jang, M., Cai, L., Udeani, G. O., Slowing, K. V., Thomas, C. F., Beecher, C. W. W., et al. (1997). Cancer chemopreventive activity of resveratrol, a natural product derived from grapes. Science 275, 218–220.
Jeong, H., Then, F., Melia, T. J. Jr., Mazzulli, J. R., Cui, L., Savas, J. N., et al. (2009). Acetylation targets mutant huntingtin to autophagosomes for degradation. Cell 137, 60–72. doi: 10.1016/j.cell.2009.03.018
Jiang, H., Khan, S., Wang, Y., Charron, G., He, B., Sebastian, C., et al. (2013). SIRT6 regulates TNF-alpha secretion through hydrolysis of long-chain fatty acyl lysine. Nature 496, 110–113. doi: 10.1038/nature12038
Johnson, J. J., Nihal, M., Siddiqui, I. A., Scarlett, C. O., Bailey, H. H., Mukhtar, H., et al. (2011). Enhancing the bioavailability of resveratrol by combining it with piperine. Mol. Nutr. Food Res. 55, 1169–1176.
Kaeberlein, M., McDonagh, T., Heltweg, B., Hixon, J., Westman, E. A., Caldwell, S. D., et al. (2005). Substrate-specific activation of sirtuins by resveratrol. J. Biol. Chem. 280, 17038–17045.
Kantartzis, K., Fritsche, L., Bombrich, M., Machann, J., Schick, F., Staiger, H., et al. (2018). Effects of resveratrol supplementation on liver fat content in overweight and insulin-resistant subjects: a randomized, double-blind, placebo-controlled clinical trial. Diabetes Obes. Metab. 20, 1793–1797. doi: 10.1111/dom.13268
KaŞıkcı, M. B., and Bağdatlıoğlu, N. (2016). Bioavailability of quercetin. Curr. Res. Nutr. Food Sci. J. 4, 146–151.
Kennedy, D. O., Wightman, E. L., Reay, J. L., Lietz, G., Okello, E. J., Wilde, A., et al. (2010). Effects of resveratrol on cerebral blood flow variables and cognitive performance in humans: a double-blind, placebo-controlled, crossover investigation. Am. J. Clin. Nutr. 91, 1590–1597. doi: 10.3945/ajcn.2009.28641
Kjaer, T. N., Ornstrup, M. J., Poulsen, M. M., Stødkilde-Jørgensen, H., Jessen, N., Jørgensen, J. O. L., et al. (2017). No beneficial effects of resveratrol on the metabolic syndrome: a randomized placebo-controlled clinical trial. J. Clin. Endocrinol. Metab. 102, 1642–1651.
Knop, F. K., Konings, E., Timmers, S., Schrauwen, P., Holst, J. J., and Blaak, E. E. (2013). Thirty days of resveratrol supplementation does not affect postprandial incretin hormone responses, but suppresses postprandial glucagon in obese subjects. Diabet. Med. 30, 1214–1218.
Köbe, T., Witte, A. V., Schnelle, A., Tesky, V. A., Pantel, J., Schuchardt, J.-P., et al. (2017). Impact of resveratrol on glucose control, hippocampal structure and connectivity, and memory performance in patients with mild cognitive impairment. Front. Neurosci. 11:105. doi: 10.3389/fnins.2017.00105
Kosciuk, T., Wang, M., Hong, J. Y., and Lin, H. (2019). Updates on the epigenetic roles of sirtuins. Curr. Opin. Chem. Biol. 51, 18–29.
Krueger, J. G., Suárez-Fariñas, M., Cueto, I., Khacherian, A., Matheson, R., Parish, L. C., et al. (2015). A randomized, placebo-controlled study of SRT2104, a SIRT1 activator, in patients with moderate to severe psoriasis. PLoS One 10:e0142081. doi: 10.1371/journal.pone.0142081
Kumar, S., and Lombard, D. B. (2018). Functions of the sirtuin deacylase SIRT5 in normal physiology and pathobiology. Crit. Rev. Biochem. Mol. Biol. 53, 311–334. doi: 10.1080/10409238.2018.1458071
la Porte, C., Voduc, N., Zhang, G., Seguin, I., Tardiff, D., Singhal, N., et al. (2010). Steady-State pharmacokinetics and tolerability of trans-resveratrol 2000 mg twice daily with food, quercetin and alcohol (ethanol) in healthy human subjects. Clin. Pharmacokinet. 49, 449–454. doi: 10.2165/11531820-000000000-00000
Lakshminarasimhan, M., Rauh, D., Schutkowski, M., and Steegborn, C. (2013). SIRT1 activation by resveratrol is substrate sequence-selective. Aging 5, 151–154. doi: 10.18632/aging.100542
Landry, J., Sutton, A., Tafrov, S. T., Heller, R. C., Stebbins, J., Pillus, L., et al. (2000). The silencing protein SIR2 and its homologs are NAD-dependent protein deacetylases. Proc. Natl. Acad. Sci. U.S.A. 97, 5807–5811.
Lesjak, M., Beara, I., Simin, N., Pintać, D., Majkić, T., Bekvalac, K., et al. (2018). Antioxidant and anti-inflammatory activities of quercetin and its derivatives. J. Funct. Foods 40, 68–75.
Leyton, L., Hott, M., Acuña, F., Caroca, J., Nuñez, M., Martin, C., et al. (2015). Nutraceutical activators of AMPK/Sirt1 axis inhibit viral production and protect neurons from neurodegenerative events triggered during HSV-1 infection. Virus Res. 205, 63–72. doi: 10.1016/j.virusres.2015.05.015
Libri, V., Brown, A. P., Gambarota, G., Haddad, J., Shields, G. S., Dawes, H., et al. (2012). A pilot randomized, placebo controlled, double blind phase i trial of the novel SIRT1 activator SRT2104 in elderly volunteers. PLoS One 7:e51395. doi: 10.1371/journal.pone.0051395
Lipinski, C. A., Lombardo, F., Dominy, B. W., and Feeney, P. J. (2001). Experimental and computational approaches to estimate solubility and permeability in drug discovery and development settings. Adv. Drug Deliv. Rev. 46(1-3), 3–26. doi: 10.1016/s0169-409x(00)00129-0
Liu, T., Yang, Q., Zhang, X., Qin, R., Shan, W., Zhang, H., et al. (2020). Quercetin alleviates kidney fibrosis by reducing renal tubular epithelial cell senescence through the SIRT1/PINK1/mitophagy axis. Life Sci. 257:118116. doi: 10.1016/j.lfs.2020.118116
Løkken, N., Khawajazada, T., Storgaard, J., Raaschou-Pedersen, D., Ørngreen, M., and Vissing, J. (2019). P.58No effect of resveratrol supplementation in patients with mitochondrial myopathy - a randomized, double-blind, placebo-controlled, cross-over study. Neuromuscul. Disord. 29, S57–S58.
Lugrin, J., Ciarlo, E., Santos, A., Grandmaison, G., dos Santos, I., Le Roy, D., et al. (2013). The sirtuin inhibitor cambinol impairs MAPK signaling, inhibits inflammatory and innate immune responses and protects from septic shock. Biochim. Biophys. Acta 1833, 1498–1510. doi: 10.1016/j.bbamcr.2013.03.004
Luo, J., Nikolaev, A. Y., Imai, S.-I., Chen, D., Su, F., Shiloh, A., et al. (2001). Negative Control of p53 by Sir2α promotes cell survival under stress. Cell 107, 137–148.
Lutz, M. I., Milenkovic, I., Regelsberger, G., and Kovacs, G. G. (2014). Distinct patterns of sirtuin expression during progression of Alzheimer’s disease. Neuromol. Med. 16, 405–414.
Magar, R. T., and Sohng, J. K. (2020). A review on structure, modifications and structure-activity relation of quercetin and its derivatives. J. Microbiol. Biotechnol. 30, 11–20. doi: 10.4014/jmb.1907.07003
Mai, A., Massa, S., Lavu, S., Pezzi, R., Simeoni, S., Ragno, R., et al. (2005). Design, synthesis, and biological evaluation of sirtinol analogues as class III histone/protein deacetylase (Sirtuin) inhibitors. J. Med. Chem. 48, 7789–7795. doi: 10.1021/jm050100l
Mankowski, R. T., You, L., Buford, T. W., Leeuwenburgh, C., Manini, T. M., Schneider, S., et al. (2020). Higher dose of resveratrol elevated cardiovascular disease risk biomarker levels in overweight older adults – A pilot study. Exp. Gerontol. 131:110821. doi: 10.1016/j.exger.2019.110821
Manna, S. K., Mukhopadhyay, A., and Aggarwal, B. B. (2000). Resveratrol suppresses TNF-induced activation of nuclear transcription factors NF-kappa B, activator protein-1, and apoptosis: potential role of reactive oxygen intermediates and lipid peroxidation. J. Immunol. 164, 6509–6519. doi: 10.4049/jimmunol.164.12.6509
Marques, B., Trindade, M., Aquino, J. C. F., Cunha, A. R., Gismondi, R. O., Neves, M. F., et al. (2018). Beneficial effects of acute trans-resveratrol supplementation in treated hypertensive patients with endothelial dysfunction. Clin. Exp. Hypertens. 40, 218–223. doi: 10.1080/10641963.2017.1288741
Massi, A., Bortolini, O., Ragno, D., Bernardi, T., Sacchetti, G., Tacchini, M., et al. (2017). Research progress in the modification of quercetin leading to anticancer agents. Molecules 22:1270.
McCallum, S., Wald, J., Hoffmann, E., Jayaramachandran, S., Bhatt, K., Englehart, S., et al. (2014). “Modified Release Formulations Do Not Enhance the PK of the Novel SIRT1 Activator SRT2104 (GSK2245840B),” in Proceedings of the 2014 Annual Meeting & Exposition - American Association of Pharmaceutical Scientists, San Diego, CA.
McDermott, M. M., Leeuwenburgh, C., Guralnik, J. M., Tian, L., Sufit, R., Zhao, L., et al. (2017). Effect of resveratrol on walking performance in older people with peripheral artery disease: the RESTORE randomized clinical trial. JAMA Cardiol. 2, 902–907. doi: 10.1001/jamacardio.2017.0538
Mehboob, H., Tahir, I. M., Iqbal, T., Saleem, S., Perveen, S., and Farooqi, A. (2017). Effect of UDP-Glucuronosyltransferase (UGT) 1A Polymorphism (rs8330 and rs10929303) on Glucuronidation Status of Acetaminophen. Dose Response 15:1559325817723731. doi: 10.1177/1559325817723731
Mendes da Silva, D., Gross, L. A., Neto, E. D. P. G., Lessey, B. A., and Savaris, R. F. (2017). The use of resveratrol as an adjuvant treatment of pain in endometriosis: a randomized clinical trial. J. Endocr. Soc. 1, 359–369. doi: 10.1210/js.2017-00053
Méndez-del Villar, M., González-Ortiz, M., Martínez-Abundis, E., Pérez-Rubio, K. G., and Lizárraga-Valdez, R. (2014). Effect of resveratrol administration on metabolic syndrome, insulin sensitivity, and insulin secretion. Metab. Syndr. Relat. Disord. 12, 497–501.
Miller, J. J., Fink, A., Banagis, J. A., Nagashima, H., Subramanian, M., Lee, C. K., et al. (2021). Sirtuin activation targets IDH-mutant tumors. Neuro Oncol. 23, 53–62. doi: 10.1093/neuonc/noaa180
Milne, J. C., Lambert, P. D., Schenk, S., Carney, D. P., Smith, J. J., Gagne, D. J., et al. (2007). Small molecule activators of SIRT1 as therapeutics for the treatment of type 2 diabetes. Nature 450, 712–716.
Min, S.-W., Cho, S.-H., Zhou, Y., Schroeder, S., Haroutunian, V., Seeley, W. W., et al. (2010). Acetylation of tau inhibits its degradation and contributes to tauopathy. Neuron 67, 953–966.
Miraglia Del Giudice, M., Maiello, N., Decimo, F., Capasso, M., Campana, G., Leonardi, S., et al. (2014). Resveratrol plus carboxymethyl-β-glucan may affect respiratory infections in children with allergic rhinitis. Pediatr. Allergy Immunol. 25, 724–728. doi: 10.1111/pai.12279
Monod, J., Wyman, J., and Changeux, J.-P. (1965). On the nature of allosteric transitions: a plausible model. J. Mol. Biol. 12, 88–118.
Moodi, Z., Bagherzade, G., and Peters, J. (2021). Quercetin as a precursor for the synthesis of novel Nanoscale Cu (II) complex as a catalyst for alcohol oxidation with high antibacterial activity. Bioinorg. Chem. Appl. 2021:8818452. doi: 10.1155/2021/8818452
Morselli, E., Maiuri, M. C., Markaki, M., Megalou, E., Pasparaki, A., Palikaras, K., et al. (2010). Caloric restriction and resveratrol promote longevity through the Sirtuin-1-dependent induction of autophagy. Cell Death Dis. 1:e10. doi: 10.1038/cddis.2009.8
Moskaug, J., Carlsen, H., Myhrstad, M., and Blomhoff, R. (2004). Molecular imaging of the biological effects of quercetin and quercetin-rich foods. Mech. Ageing Dev. 125, 315–324. doi: 10.1016/j.mad.2004.01.007
Moussa, C., Hebron, M., Huang, X., Ahn, J., Rissman, R. A., Aisen, P. S., et al. (2017). Resveratrol regulates neuro-inflammation and induces adaptive immunity in Alzheimer’s disease. J. Neuroinflammation 14:1. doi: 10.1186/s12974-016-0779-0
Napper, A. D., Hixon, J., McDonagh, T., Keavey, K., Pons, J. F., Barker, J., et al. (2005). Discovery of Indoles as Potent and Selective Inhibitors of the Deacetylase SIRT1. J. Med. Chem. 48, 8045–8054.
Noh, R. M., Venkatasubramanian, S., Daga, S., Langrish, J., Mills, N. L., Lang, N. N., et al. (2017). Cardiometabolic effects of a novel SIRT1 activator, SRT2104, in people with type 2 diabetes mellitus. Open Heart 4:e000647. doi: 10.1136/openhrt-2017-000647
Novelle, M. G., Wahl, D., Diéguez, C., Bernier, M., and de Cabo, R. (2015). Resveratrol supplementation: Where are we now and where should we go? Ageing Res. Rev. 21, 1–15. doi: 10.1016/j.arr.2015.01.002
Oboh, G., Ademosun, A. O., and Ogunsuyi, O. B. (2016). Quercetin and its role in chronic diseases. Adv. Exp. Med. Biol. 929, 377–387.
Oktyabrsky, O. N., Bezmaternykh, K. V., Smirnova, G. V., and Tyulenev, A. V. (2020). Biotechnology, Effect of resveratrol and quercetin on the susceptibility of Escherichia coli to antibiotics. World J. Microbiol. Biotechnol. 36:167. doi: 10.1007/s11274-020-02934-y
Olaharski, A. J., Rine, J., Marshall, B. L., Babiarz, J., Zhang, L., Verdin, E., et al. (2005). The flavoring agent dihydrocoumarin reverses epigenetic silencing and inhibits sirtuin deacetylases. PLoS Genet. 1:e77. doi: 10.1371/journal.pgen.0010077
Ozemek, C., Hildreth, K. L., Blatchford, P. J., Hurt, K. J., Bok, R., Seals, D. R., et al. (2020). Effects of resveratrol or estradiol on postexercise endothelial function in estrogen-deficient postmenopausal women. J. Appl. Physiol. 128, 739–747.
Pacholec, M., Bleasdale, J. E., Chrunyk, B., Cunningham, D., Flynn, D., Garofalo, R. S., et al. (2010). SRT1720, SRT2183, SRT1460, and resveratrol are not direct activators of SIRT1. J. Biol. Chem. 285, 8340–8351.
Pallos, J., Bodai, L., Lukacsovich, T., Purcell, J. M., Steffan, J. S., Thompson, L. M., et al. (2008). Inhibition of specific HDACs and sirtuins suppresses pathogenesis in a Drosophila model of Huntington’s disease. Hum. Mol. Genet. 17, 3767–3775. doi: 10.1093/hmg/ddn273
Park, D., Jeong, H., Lee, M. N., Koh, A., Kwon, O., Yang, Y. R., et al. (2016). Resveratrol induces autophagy by directly inhibiting mTOR through ATP competition. Sci. Rep. 6:21772. doi: 10.1038/srep21772
Park, S.-J., Ahmad, F., Philp, A., Baar, K., Williams, T., Luo, H., et al. (2012). Resveratrol ameliorates aging-related metabolic phenotypes by inhibiting cAMP phosphodiesterases. Cell 148, 421–433. doi: 10.1016/j.cell.2012.01.017
Patel, K. R., Brown, V. A., Jones, D. J. L., Britton, R. G., Hemingway, D., Miller, A. S., et al. (2010). Clinical pharmacology of resveratrol and its metabolites in colorectal cancer patients. Cancer Res. 70, 7392–7399.
Pezzuto, J. M. (2011). The phenomenon of resveratrol: redefining the virtues of promiscuity. Ann. N.Y. Acad. Sci. 1215, 123–1230. doi: 10.1111/j.1749-6632.2010.05849.x
Pollack, R. M., Barzilai, N., Anghel, V., Kulkarni, A. S., Golden, A., O’Broin, P., et al. (2017). Resveratrol improves vascular function and mitochondrial number but not glucose metabolism in older adults. J. Gerontol. 72, 1703–1709. doi: 10.1093/gerona/glx041
Popat, R., Plesner, T., Davies, F., Cook, G., Cook, M., Elliott, P., et al. (2013). A phase 2 study of SRT501 (resveratrol) with bortezomib for patients with relapsed and or refractory multiple myeloma. Br. J. Haematol. 160, 714–717.
Porcu, M., and Chiarugi, A. (2005). The emerging therapeutic potential of sirtuin-interacting drugs: from cell death to lifespan extension. Trends Pharmacol. Sci. 26, 94–103. doi: 10.1016/j.tips.2004.12.009
Poulsen, M. K., Nellemann, B., Bibby, B. M., Stødkilde-Jørgensen, H., Pedersen, S. B., Grønbaek, H., et al. (2018). No effect of resveratrol on VLDL-TG kinetics and insulin sensitivity in obese men with nonalcoholic fatty liver disease. Diabetes Obes. Metab. 20, 2504–2509. doi: 10.1111/dom.13409
Poulsen, M. M., Vestergaard, P. F., Clasen, B. F., Radko, Y., Christensen, L. P., Stødkilde-Jørgensen, H., et al. (2013). High-dose resveratrol supplementation in obese men: an investigator-initiated, randomized, placebo-controlled clinical trial of substrate metabolism, insulin sensitivity, and body composition. Diabetes 62, 1186–1195. doi: 10.2337/db12-0975
Price, N. L., Gomes, A. P., Ling, A. J., Duarte, F. V., Martin-Montalvo, A., North, B. J., et al. (2012). sirt1 is required for AMPK activation and the beneficial effects of resveratrol on mitochondrial function. Cell Metab. 15, 675–690.
Rahnasto-Rilla, M., Tyni, J., Huovinen, M., Jarho, E., Kulikowicz, T., Ravichandran, S., et al. (2018). Natural polyphenols as sirtuin 6 modulators. Sci. Rep. 8:4163.
Reen, R. K., Jamwal, D. S., Taneja, S. C., Koul, J. L., Dubey, R. K., Wiebel, F. J., et al. (1993). Impairment of UDP-glucose dehydrogenase and glucuronidation activities in liver and small intestine of rat and guinea pig in vitro by piperine. Biochem. Pharmacol. 46, 229–238. doi: 10.1016/0006-2952(93)90408-o
Riva, A., Ronchi, M., Petrangolini, G., Bosisio, S., and Allegrini, P. (2019). Improved oral absorption of quercetin from quercetin phytosome®, a new delivery system based on food grade lecithin. Eur. J. Drug Metab. Pharmacokinet. 44, 169–177. doi: 10.1007/s13318-018-0517-3
Roggerio, A., Strunz, C. M. C., Pacanaro, A. P., Leal, D. P., Takada, J. Y., Avakian, S. D., et al. (2018). Gene expression of Sirtuin-1 and endogenous secretory receptor for advanced glycation end products in healthy and slightly overweight subjects after caloric restriction and resveratrol administration. Nutrients 10:937. doi: 10.3390/nu10070937
Rojas, Á., Del Campo, J. A., Clement, S., Lemasson, M., García-Valdecasas, M., Gil-Gómez, A., et al. (2016). Effect of quercetin on hepatitis C virus life cycle: from viral to host targets. Sci. Rep. 6:31777. doi: 10.1038/srep31777
Sak, K. (2014). Site-specific anticancer effects of dietary flavonoid quercetin. Nutr. Cancer 66, 177–193.
Saldanha, J. F., Leal, V. O., Rizzetto, F., Grimmer, G. H., Ribeiro-Alves, M., Daleprane, J. B., et al. (2016). Effects of Resveratrol Supplementation in Nrf2 and NF-κB Expressions in nondialyzed chronic kidney disease patients: a randomized, double-blind, placebo-controlled, crossover clinical trial. J. Ren. Nutr. 26, 401–406. doi: 10.1053/j.jrn.2016.06.005
Sands, B. E., Joshi, S., Haddad, J., Freudenberg, J. M., Oommen, D. E., Hoffmann, E., et al. (2016). Assessing colonic exposure, safety, and clinical activity of SRT2104, a Novel Oral SIRT1 activator, in patients with mild to moderate ulcerative colitis. Inflamm. Bowel Dis. 22, 607–614. doi: 10.1097/MIB.0000000000000597
Sauve, A. A., Wolberger, C., Schramm, V. L., and Boeke, J. D. (2006). The biochemistry of sirtuins. Annu. Rev. Biochem. 75, 435–465.
Schmidt, C. (2010). GSK/Sirtris compounds dogged by assay artifacts. Nat. Biotechnol. 28, 185–186. doi: 10.1038/nbt0310-185
Shahbazian, M. D., and Grunstein, M. (2007). Functions of site-specific histone acetylation and deacetylation. Annu. Rev. Biochem. 76, 75–100.
Sinaga, S., Sudarmi, S., Iksen, I., Kevin, K., and Sari, M. J. (2017). Evaluation of total phenolic, flavonoid content, antioxidant and in vitro antilithogenesis activities of chives leaf (Allium schoenoprasum, L.). Rasayan J. Chem. 11, 1604–1608.
Singh, C. K., and Ahmad, N. (2015). Abstract 2801: resveratrol-Quercetin combination significantly inhibits prostate cancer in TRAMP mice. Cancer Res. 75(15 Supplement):2801.
Smith, A. J., Oertle, J., Warren, D., and Prato, D. (2016). Quercetin: a promising flavonoid with a dynamic ability to treat various diseases, infections, and cancers. J. Cancer Ther. 7, 83–95.
Smith, M. R., Syed, A., Lukacsovich, T., Purcell, J., Barbaro, B. A., Worthge, S. A., et al. (2014). A potent and selective Sirtuin 1 inhibitor alleviates pathology in multiple animal and cell models of Huntington’s disease. Hum. Mol. Genet. 23, 2995–3007.
Spogli, R., Bastianini, M., Ragonese, F., Iannitti, R., Monarca, L., Bastioli, F., et al. (2018). Solid dispersion of resveratrol supported on magnesium DiHydroxide (Resv@MDH) microparticles improves oral bioavailability. Nutrients 10:1925.
Springer, M., and Moco, S. (2019). Resveratrol and its human metabolites-effects on metabolic health and obesity. Nutrients 11:143.
St Leger, A. S., Cochrane, A. L., and Moore, F. (1979). Factors associated with cardiac mortality in developed countries with particular reference to the consumption of wine. Lancet 1(8124), 1017–1020.
Sussmuth, S. D., Haider, S., Landwehrmeyer, G. B., Farmer, R., Frost, C., Tripepi, G., et al. (2015). An exploratory double-blind, randomized clinical trial with selisistat, a SirT1 inhibitor, in patients with Huntington’s disease. Br. J. Clin. Pharmacol. 79, 465–476.
Tabrizi, S. J., Flower, M. D., Ross, C. A., and Wild, E. J. (2020). Huntington disease: new insights into molecular pathogenesis and therapeutic opportunities. Nat. Rev. Neurol. 16, 529–546.
Tang, J., Lu, L., Liu, Y., Ma, J., Yang, L., Li, L., et al. (2019). Quercetin improve ischemia/reperfusion-induced cardiomyocyte apoptosis in vitro and in vivo study via SIRT1/PGC-1α signaling. J. Cell. Biochem. 120, 9747–9757.
Timmers, S., de Ligt, M., Phielix, E., van de Weijer, T., Hansen, J., Moonen-Kornips, E., et al. (2016). Resveratrol as add-on therapy in subjects with well-controlled type 2 diabetes: a randomized controlled trial. Diabetes Care 39, 2211–2217.
Trapp, J., and Jung, M. (2006). The role of NAD+ dependent histone deacetylases (sirtuins) in ageing. Curr. Drug Targets 7, 1553–1560.
Turner, R. S., Thomas, R. G., Craft, S., van Dyck, C. H., Mintzer, J., Reynolds, B. A., et al. (2015). A randomized, double-blind, placebo-controlled trial of resveratrol for Alzheimer disease. Neurology 85, 1383–1391. doi: 10.1212/WNL.0000000000002035
Ung, D., and Nagar, S. (2007). Variable sulfation of dietary polyphenols by recombinant human sulfotransferase (SULT) 1A1 genetic variants and SULT1E1. Drug Metab. Dispos. 35, 740–746. doi: 10.1124/dmd.106.013987
van der Made, S. M., Plat, J., and Mensink, R. P. (2015). Resveratrol does not influence metabolic risk markers related to cardiovascular health in overweight and slightly obese subjects: a randomized, placebo-controlled crossover trial. PLoS One 10:e0118393. doi: 10.1371/journal.pone.0118393
van der Meer, A. J., Scicluna, B. P., Moerland, P. D., Lin, J., Jacobson, E. W., Vlasuk, G. P., et al. (2015). The selective Sirtuin 1 activator SRT2104 reduces endotoxin-induced cytokine release and coagulation activation in humans. Crit. Care Med. 43, e199–e202. doi: 10.1097/CCM.0000000000000949
Vaz-da-Silva, M., Loureiro, A. I., Falcao, A., Nunes, T., Rocha, J. F., Fernandes-Lopes, C., et al. (2008). Effect of food on the pharmacokinetic profile of trans-resveratrol. Int. J. Clin. Pharmacol. Ther. 46, 564–570. doi: 10.5414/CPP46564
Venkatasubramanian, S., Noh, R. M., Daga, S., Langrish, J. P., Joshi, N. V., Mills, N. L., et al. (2013). Cardiovascular effects of a novel SIRT1 activator, SRT2104, in otherwise healthy cigarette smokers. J. Am. Heart Assoc. 2:e000042. doi: 10.1161/JAHA.113.000042
Venkatasubramanian, S., Noh, R. M., Daga, S., Langrish, J. P., Mills, N. L., Waterhouse, B. R., et al. (2016). Effects of the small molecule SIRT1 activator, SRT2104 on arterial stiffness in otherwise healthy cigarette smokers and subjects with type 2 diabetes mellitus. Open Heart 3:e000402. doi: 10.1136/openhrt-2016-000402
Vors, C., Couillard, C., Paradis, M. E., Gigleux, I., Marin, J., Vohl, M. C., et al. (2018). Supplementation with resveratrol and curcumin does not affect the inflammatory response to a high-fat meal in older adults with abdominal obesity: a randomized, placebo-controlled crossover trial. J. Nutr. 148, 379–388. doi: 10.1093/jn/nxx072
Walker, J. M., Eckardt, P., Aleman, J. O., da Rosa, J. C., Liang, Y., Iizumi, T., et al. (2018). The effects of trans-resveratrol on insulin resistance, inflammation, and microbiota in men with the metabolic syndrome: a pilot randomized, placebo-controlled clinical trial. J. Clin. Transl. Res. 4, 122–135.
Walle, T., Hsieh, F., DeLegge, M. H., and Oatis, J. E. Jr., and Walle, U. K. (2004). High absorption but very low bioavailability of oral resveratrol in humans. Drug Metab. Dispos. 32, 1377–1382. doi: 10.1124/dmd.104.000885
Wang, S., Yao, J., Zhou, B., Yang, J., Chaudry, M. T., Wang, M., et al. (2018). Bacteriostatic effect of quercetin as an antibiotic alternative in vivo and its antibacterial mechanism in vitro. J. Food Prot. 81, 68–78. doi: 10.4315/0362-028X.JFP-17-214
Wang, Z., Yuan, H., Roth, M., Stark, J. M., Bhatia, R., and Chen, W. Y. (2013). SIRT1 deacetylase promotes acquisition of genetic mutations for drug resistance in CML cells. Oncogene 32, 589–598. doi: 10.1038/onc.2012.83
Watroba, M., Dudek, I., Skoda, M., Stangret, A., Rzodkiewicz, P., and Szukiewicz, D. (2017). Sirtuins, epigenetics and longevity. Ageing Res. Rev. 40, 11–19. doi: 10.1016/j.arr.2017.08.001
Westerberg, G., Chiesa, J. A., Andersen, C. A., Diamanti, D., Magnoni, L., Pollio, G., et al. (2015). Safety, pharmacokinetics, pharmacogenomics and QT concentration-effect modelling of the SirT1 inhibitor selisistat in healthy volunteers. Br. J. Clin. Pharmacol. 79, 477–491. doi: 10.1111/bcp.12513
Wiewel, M. A., van der Meer, A. J., Haddad, J., Jacobson, E. W., Vlasuk, G. P., and van der Poll, T. (2013). SRT2379, a small-molecule SIRT1 activator, fails to reduce cytokine release in a human endotoxemia model. Crit. Care 17:8. doi: 10.1186/cc12909
Wightman, E. L., Reay, J. L., Haskell, C. F., Williamson, G., Dew, T. P., and Kennedy, D. O. (2014). Effects of resveratrol alone or in combination with piperine on cerebral blood flow parameters and cognitive performance in human subjects: a randomised, double-blind, placebo-controlled, cross-over investigation. Br. J. Nutr. 112, 203–213. doi: 10.1017/S0007114514000737
Wightman, E., Eschle, T. M., and Kennedy, D. (2019). The Cognitive effects of the polyphenol resveratrol in young, healthy humans: a review of six balanced crossover, placebo controlled, double blind trials. Int. J. Nutr. Health Food Saf. 1, 001–009.
Wong, G., He, S., Siragam, V., Bi, Y., Mbikay, M., Chretien, M., et al. (2017). Antiviral activity of quercetin-3-β-OD-glucoside against Zika virus infection. Virol. Sin. 32, 545–547. doi: 10.1007/s12250-017-4057-9
Wu, Y., Li, X., Zhu, J. X., Xie, W., Le, W., Fan, Z., et al. (2011). Resveratrol-activated AMPK/SIRT1/autophagy in cellular models of Parkinson’s disease. Neurosignals 19, 163–174. doi: 10.1159/000328516
Yiu, E., Tai, G., Peverill, R., Lee, K., Croft, K., Mori, T., et al. (2013). An open label clinical pilot study of resveratrol as a treatment for friedreich ataxia (S43.006). Neurology 80(7 Suppl.):S43.006.
Yoo, J. Y., Kim, T. H., Fazleabas, A. T., Palomino, W. A., Ahn, S. H., Tayade, C., et al. (2017). KRAS Activation and over-expression of SIRT1/BCL6 Contributes to the Pathogenesis of Endometriosis and Progesterone Resistance. Sci. Rep. 7:6765.
Yoshino, J., Conte, C., Fontana, L., Mittendorfer, B., Imai, S.-I., Schechtman, K. B., et al. (2012). Resveratrol supplementation does not improve metabolic function in nonobese women with normal glucose tolerance. Cell Metab. 16, 658–664. doi: 10.1016/j.cmet.2012.09.015
You, W., Zheng, W., Weiss, S., Chua, K. F., and Steegborn, C. (2019). Structural basis for the activation and inhibition of Sirtuin 6 by quercetin and its derivatives. Sci. Rep. 9:19176. doi: 10.1038/s41598-019-55654-1
Yuan, H., and Marmorstein, R. (2012). Structural basis for sirtuin activity and inhibition. J. Biol. Chem. 287, 42428–42435. doi: 10.1074/jbc.R112.372300
Zeng, Y., Nikitkova, A., Abdelsalam, H., Li, J., and Xiao, J. (2019). Activity of quercetin and kaemferol against Streptococcus mutans biofilm. Arch Oral Biol. 98, 9–16. doi: 10.1016/j.archoralbio.2018.11.005
Zhang, J. Y., Holbrook, M., Inagaki, E., Feng, B., Ko, D., Weisbrod, R., et al. (2017). The effects of resveratrol treatment on vascular function in type 2 diabetes mellitus. Arterioscler. Thromb. Vasc. Biol. 37:A164. doi: 10.1161/atvb.37.suppl_1.164
Zhu, C. W., Grossman, H., Neugroschl, J., Parker, S., Burden, A., and Luo, X. Sano, M. (2018). A randomized, double-blind, placebo-controlled trial of resveratrol with glucose and malate (RGM) to slow the progression of Alzheimer’s disease: a pilot study. Alzheimers Dement. 4, 609–616. doi: 10.1016/j.trci.2018.09.009
Zocchi, L., and Sassone-Corsi, P. (2012). SIRT1-mediated deacetylation of MeCP2 contributes to BDNF expression. Epigenetics 7, 695–700. doi: 10.4161/epi.20733
Zondervan, K. T., Becker, C. M., and Missmer, S. A. (2020). Endometriosis. N. Engl. J. Med. 382, 1244–1256. doi: 10.1056/NEJMra1810764
Keywords: sirtuin, inhibitor, activator, clinical trial, drug development
Citation: Curry AM, White DS, Donu D and Cen Y (2021) Human Sirtuin Regulators: The “Success” Stories. Front. Physiol. 12:752117. doi: 10.3389/fphys.2021.752117
Received: 02 August 2021; Accepted: 29 September 2021;
Published: 21 October 2021.
Edited by:
Vinod Kumar Bhaskara Pillai, University of Chicago, United StatesReviewed by:
Nejat Dalay, Istanbul University, TurkeyErik Alexander Blackwood, University of Arizona, United States
Gautham Yepuri, New York University Langone Medical Center, United States
Copyright © 2021 Curry, White, Donu and Cen. This is an open-access article distributed under the terms of the Creative Commons Attribution License (CC BY). The use, distribution or reproduction in other forums is permitted, provided the original author(s) and the copyright owner(s) are credited and that the original publication in this journal is cited, in accordance with accepted academic practice. No use, distribution or reproduction is permitted which does not comply with these terms.
*Correspondence: Yana Cen, ceny2@vcu.edu