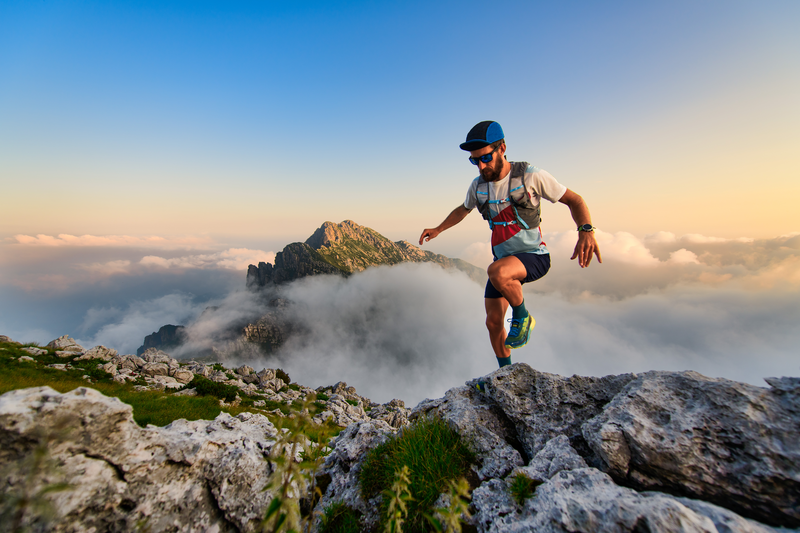
94% of researchers rate our articles as excellent or good
Learn more about the work of our research integrity team to safeguard the quality of each article we publish.
Find out more
ORIGINAL RESEARCH article
Front. Physiol. , 01 October 2021
Sec. Exercise Physiology
Volume 12 - 2021 | https://doi.org/10.3389/fphys.2021.738063
This article is part of the Research Topic Women and Men in Physical Activity View all 8 articles
This study aimed to examine sex differences in oxygen saturation in respiratory (SmO2-m.intercostales) and locomotor muscles (SmO2-m.vastus lateralis) while performing physical exercise. Twenty-five (12 women) healthy and physically active participants were evaluated during an incremental test with a cycle ergometer, while ventilatory variables [lung ventilation (E), tidal volume (Vt), and respiratory rate (RR)] were acquired through the breath-by-breath method. SmO2 was acquired using the MOXY® devices on the m.intercostales and m.vastus lateralis. A two-way ANOVA (sex × time) indicated that women showed a greater significant decrease of SmO2-m.intercostales, and men showed a greater significant decrease of SmO2-m.vastus lateralis. Additionally, women reached a higher level of ΔSmO2-m.intercostales normalized to E (L⋅min–1) (p < 0.001), whereas men had a higher level of ΔSmO2-m.vastus lateralis normalized to peak workload-to-weight (watts⋅kg–1, PtW) (p = 0.049), as confirmed by Student’s t-test. During an incremental physical exercise, women experienced a greater cost of breathing, reflected by greater deoxygenation of the respiratory muscles, whereas men had a higher peripheral load, indicated by greater deoxygenation of the locomotor muscles.
Physiological sex-related differences during dynamic physical exercise have been a subject of recent interest. A primary difference is the greater oxygen consumption of the respiratory muscles (O2–RM), such as the m.intercostales, in women (13.8 vs. 9.4% in men) (Lomauro and Aliverti, 2018), requiring higher lung ventilation (E) in response to metabolic demands (Guenette et al., 2009; Welch et al., 2018). This greater O2–RM is attributed to increased resistance and elasticity load of the respiratory system, consequent to a smaller rib cage (Bellemare et al., 2003), a mechanical disadvantage of m.diaphragma, lower airway diameter (Lomauro and Aliverti, 2018) and higher recruitment of the accessory respiratory muscles (Sheel et al., 2004; Mitchell et al., 2018). The increase of O2–RM requires increased blood flow to the respiratory muscles, which may restrict nutrient and oxygen supply to peripheral exercising muscles, thereby limiting the continued performance of exercise (metareflex) (Guenette and Sheel, 2007; Dominelli et al., 2017).
The oxygen saturation of muscles (SmO2) reflects the supply and demand of oxygen in this tissue. This can be determined using continuous-wave near-infrared spectroscopy (NIRS, 630–850 nm) that measures changes in oxygenated hemoglobin (O2 Hb) and myoglobin (mHb) at a microvascular level (Perrey and Ferrari, 2018; Alvares et al., 2020). A previous study reported high reliability of the measurement protocol of SmO2 in m.intercostales and m.vastus lateralis during maximal incremental treadmill exercise in male long-distance runners (Contreras-Briceño et al., 2019). However, investigations of sex-related differences and how SmO2 levels change in these muscles during exercise in women is scarce. Therefore, potential sex-related differences need to be explored further to define their impact on sports performance and in rehabilitation.
This study aimed to evaluate the sex differences of SmO2 in m.intercostales and m.vastus lateralis during an incremental cycle test until exhaustion and to analyze the changes in SmO2 in relative to ventilatory variables, peripheral workload, and aerobic capacity (O2–peak). To our knowledge, this is the first study that evaluates sex-related differences of SmO2 in the m.intercostales and m.vastus lateralis in highly recruited muscles of healthy subjects while performing an incremental maximal exercise.
Twenty-five physically active participants (12 women) were assessed. Anthropometric and pulmonary functional characteristics of the subjects are shown in Table 1. The participants reported no history of respiratory, cardiovascular, metabolic, musculoskeletal, neoplastic diseases or acute infections for at least 2 weeks before testing. They did not take anti-inflammatory medications, illicit drugs, antioxidants, or any other dietary supplements. All participants were thoroughly informed (in verbal and written forms) of the study procedures, and all of them signed informed consent forms. This study followed the Declaration of Helsinki (Harriss et al., 2017) and was approved by the ethics committee of the Faculty of Medicine of Pontificia Universidad Católica of Chile (project no. 19042213).
The participants were evaluated at the Laboratory of Exercise Physiology of Pontificia Universidad Católica of Chile during two sessions, separated by an interval of 24 h interval. All procedures were performed under laboratory environmental conditions (temperature, 22 ± 2°C; relative humidity, 40 ± 2%) and within a similar time frame (9:00 a.m.–2:00 p.m.). Participants were asked to avoid physical activities for 24 h before the measurements and to avoid alcohol, caffeine, and other stimulants and food for at least 3 h prior to the evaluations.
Anthropometric evaluations were measured (weight, height, and body mass index). Subsequently, spirometry (Microlab, model ML3500, CareFusion®, San Diego, United States) was performed according to the American Thoracic Society (ATS)⋅European Respiratory Society (ERS) protocol (Gibson et al., 2002), utilizing the reference values of Knudson et al. (1983). Finally, maximal inspiratory pressures (MIP) were evaluated using a pneumometer (Micro MRC, CareFusion®, Traunstein, Germany) according to the protocol proposed by the American Thoracic Society (ATS) and the European Respiratory Society (Gibson et al., 2002), utilizing the reference values of Black and Hyatt (Hyatt and Black, 1969). Respiratory resistance was also measured using the Test of Incremental Respiratory Endurance (TIRE) with POWERbreathe® threshold loading devices (IMT Technologies Ltd., Birmingham, United Kingdom) according to the modified protocol of Cahalin and Arena (2015), which consisted of a series of 90 s at a respiratory rate (RR) of 30 breaths⋅min–1, with an initial load of 10% of the MIP and increased by 10% until task failure or inability to maintain the RR.
Peak oxygen consumption (O2–peak) was evaluated using an ergospirometer (MasterScreen CPX, Jaeger®, Germany) via the breath-by-breath method during an incremental cycle ergometer protocol (ViaSprint 150P, Ergoline GmH, Traunstein, Germany). The protocol consisted of a 1-min rest, 5-min warm-up period at 40 watts, followed by an increase of 20 watts every 2 min until exhaustion, despite standardized verbal stimuli (respiratory quotient, 1.20 ± 0.05) (see Supplementary Material I; Contreras-Briceño et al., 2020). The participants were requested to maintain a cadence between 70 and 90 rpm. The O2–peak was calculated as the highest value obtained during the last 30 s of the incremental test, despite increasing the exercise intensity (<150 ml⋅min–1 of exercise) (Day et al., 2003). A cool down of 4 min of submaximal exercise was performed before allowing the patients to rest. At baseline and throughout the test, the heart rate, pulse oxygen saturation, and blood pressure were measured. Before every test, the gas analyzer was calibrated according to the instructions provided by the manufacturer.
The saturation of oxygen in muscles (SmO2) was evaluated using continuous-wave near-infrared spectroscopy (NIRS, 630–850 nm), a non-invasive method (MOXY®, Fortiori, Desing LLC, Minnesota, United States). This device measures the absorbance of infrared light by oxygenated (O2 Hb), deoxygenated hemoglobin (HHb), and myoglobin (mHb) at a microvascular level (Austin et al., 2005). From these values, SmO2 was calculated using PeriPedal (PeriPedal®, IN, United States) at a sampling frequency of 2 Hz (McManus et al., 2018) from the m.intercostales (SmO2-m.intercostales) and m.vastus lateralis (SmO2-m.vastus lateralis), according to a previous protocol (Contreras-Briceño et al., 2019). In brief, for m.intercostales, a MOXY® device was placed on the seventh intercostal space at the anterior axillary line in the right thoracic area. To determine the level of SmO2 in the locomotor muscles, a second MOXY® device was placed over the m.vastus lateralis, 5 cm lateral to the midline of the thigh and landmarked midway between the upper edge of the patella and the greater trochanter of the right femur. The devices were fixed to the skin with double-sided sticky tape and hypoallergenic skin tape (see Figure 1).
Each participant had an initial record of 90 s on the cycle ergometer, followed by 60 s of a baseline resting phase, during which data acquisition began was synchronized. For data analysis, data were compared across percentages of the test duration (0–100% of task), with the time of O2–peak set as 100%. To complement the analysis, a triphasic model of exercise intensity was used, where ventilatory thresholds (VT1 and VT2) were calculated by two expert researchers using a visual method (Guazzi et al., 2012). An opinion of a third expert was considered in cases of discrepancy during the analysis (Vainshelboim et al., 2017). The values used for ventilatory variables [E, RR, and tidal volume (Vt)] and SmO2 were obtained from the average of the last 30 s during each phase. At the end of the protocol, the exhaustion of the participants was evaluated using the Borg Modified Rating Perceived Scale (RPE) (see Supplementary Material I).
To compare differences of muscle oxygen levels between men and women, data are reported as: (i) maximum changes or differences (Δ), (e.g., ΔSmO2-m.intercostales = SmO2-m.intercostales rest—SmO2-m.intercostales peak phase) and (ii) percentage of change, dividing the SmO2 value of each phase by the SmO2 of rest phase (%-change), (e.g., SmO2-m.intercostales (%-change) = (SmO2-m.intercostales phase⋅SmO2-m.intercostales rest phase–1). In addition, ΔSmO2-m.intercostales was normalized by ΔE (SmO2-m.intercostales ⋅ΔE–1) and the ΔSmO2-m.vastus lateralis was normalized to peak workload-to-weight (watts⋅kg–1) (SmO2-m.vastus lateralis⋅ PtW–1).
Normality of the data was evaluated using the Shapiro-Wilk test. The descriptive characteristics of the participants were compared using the Student’s t-test. The differences between sexes with regard to SmO2-m.intercostales, SmO2-m.vastus lateralis, SmO2 m.intercostales—m.vastus lateralis ratio, and ventilatory variables (E, RR, and Vt), which are expressed according to the % task, were analyzed using the two-way ANOVA test, reporting differences between conditions when the interaction of the factors (sex × time) was significant (p < 0.05). Subsequent multiple comparisons were analyzed used the Sidak post-hoc test. Comparisons of ΔSmO2-m.intercostales⋅ΔE–1 and ΔSmO2-m.vastus lateralis⋅PtW–1 were performed using the Student’s t-test. The correlation between ΔSmO2-m.vastus lateralis (%) and ventilatory variables were assessed using Pearson’s correlation coefficient. Statistical significance was set at p < 0.05. The statistical analysis was performed using the GraphPad Prism (version 8.0; San Diego, California, United States).
The interaction of factors (sex × time) was different in the SmO2-m.intercostales expressed as a percentage of the performed task (p < 0.001). The SmO2-m.intercostales (% change) decreased more significantly in women than in men (between 40 and 100%, p < 0.001) and between VT1 and VT2 (p < 0.001) (see Figure 2A). The ΔSmO2-m.intercostales was greater in women than in men (39 ± 9 vs. 27 ± 19, respectively, p = 0.048) (see Supplementary Material I).
Figure 2. Changes in SmO2 between men and women. (A) SmO2-m.intercostales (%-change), (B) SmO2-m.vastus lateralis (%-change), (C) SmO2 ratio (absolute values) and (D) SmO2 ratio (%-change). #p < 0.05: comparison between sex with respect to percentage of task. The two vertical lines represent ventilatory thresholds (VT1, 61 ± 5% to VO2–peak and VT2, 84% ± 3% to VO2–peak). The interaction between factors (sex × time) is represented by the p-value.
The interaction of factors (sex × time) was different in the SmO2-m.vastus lateralis expressed as a percentage of the performed task (p < 0.001). The SmO2-m.vastus lateralis (% change) decreased more significantly in men than in women (between 60 and 100%, p < 0.001) and between VT1, VT2, and O2–peak phases (p < 0.001) (see Figure 2B). The ΔSmO2-m.vastus lateralis was greater in men than in women (34 ± 18 vs. 16 ± 9, respectively, p < 0.001) (see Supplementary Material I).
The interaction of factors (sex × time) was different in the SmO2 ratio (absolute values) expressed as a percentage of the performed task (p < 0.001) and the SmO2 ratio (%-change) expressed as a percentage of the performed task (p < 0.001). In terms of absolute values, the decrease was more in women than in men (between 80 and 100%, p < 0.001) and in VT2 and O2–peak phases (p < 0.001) (see Figure 2C). In terms of %-change, the decrease was more in women than in men (between 70 and 100%, p < 0.001) and in VT2 and O2–peak phases (p < 0.001) (see Figure 2D).
There were no differences in THb in the m.intercostales and m.vastus lateralis in both groups (see Supplementary Material I).
Compared to women, men had higher absolute (3470 ± 436 ml⋅min–1 vs. 2156 ± 189 ml⋅min–1, respectively, p < 0.001) and relative (51.0 ± 5.3 ml⋅kg⋅min–1 vs. 39.3 ± 3.0 ml⋅kg⋅min–1, respectively, p < 0.001) O2–peak values. Regarding the workload (watts), men demonstrated greater values in absolute terms (256 ± 23 vs. 164 ± 17, p < 0.001) and when normalized to body weight (PtW) (3.8 ± 0.4 vs. 3.0 ± 0.3, p < 0.001). Regarding the ventilatory variables, men reached greater Emax expressed in absolute terms (L⋅min–1) (142.9 ± 21.6 vs. 98.9 ± 14.9, p < 0.001) and in %-change (15.6 ± 4.3 vs. 9.9 ± 2.2, p = 0.037) (see Supplementary Material I). The interaction of factors (sex × time) was different in the E (L⋅min–1) (p < 0.001) and E (%-change) (p < 0.001). When expressing this variable regarding the % task in absolute terms (see Figure 3A) and %-change, men reached a greater value from 60 to 100% of the protocol (p < 0.001) (see Figure 3B). Concerning the RRmax expressed in absolute terms (bpm), there were no differences between sexes (p = 0.457). The interaction of factors (sex × time) was not different in the RR (breaths⋅min–1) (p = 0.857), but RR (%-change) was different (p < 0.001). The level of RR (%-change) in the peak phase was greater in men than in women (4.1 ± 1.2 vs. 2.9 ± 0.4, p < 0.001). When expressing this variable in relation to the % task, men reached a greater value from 90 to 100% of the protocol (p < 0.001) (see Figure 3F). The level of Vtmax expressed in absolute terms (L) was greater in men than in women (2.9 ± 0.3 vs. 2.0 ± 0.3, respectively, p < 0.010). The interaction of factors (sex × time) was different in the Vt (L) (p < 0.001), but not in the Vt (%-change) (p = 0.070). When expressing absolute Vtmax in relation to the % task, it was greater in men (between 30 and 100% during the protocol, p < 0.001) (see Figure 3B). Regarding the Vt (%-change), the value was greater in men (between 80 and 90% of the test, p < 0.027) (see Figure 3E).
Figure 3. Changes in ventilatory variables between men and women. The panels on the right indicate absolute values, (A) E (L⋅min–1), (B) Vt (L) and (C) RR (breaths⋅min–1). The panels on the left indicate the percentage to rest, (D) E (%-change), (E) Vt (%-change) and (F) RR (%-change). #p < 0.05: comparison between sex with respect to percentage of task. The two vertical lines represent the ventilatory thresholds (VT1, 61 ± 5% to VO2–peak and VT2, 84 ± 3% to VO2–peak). The interaction between factors (sex × time) is represented by the p-value.
The variables mentioned above that consider the triphasic model of exercise intensity are presented in Supplementary Material I.
The ΔSmO2-m.intercostales⋅E–1 was greater in women than in men (0.5 ± 0.1 vs. 0.2 ± 0.1, respectively, p < 0.001) (see Figure 4A), while the ΔSmO2-m.vastus lateralis⋅PtW–1 was greater in men than in women (10.1 ± 4.8 vs. 7.2 ± 4.0, respectively, p = 0.049) (see Figure 4B).
Figure 4. Comparison in SmO2 between men and women standardized by (A) ΔE (E peak—E rest phase, ΔSmO2-m.intercostales⋅ΔE–1) and (B) PtW (peak workload-to-weight, ΔSmO2-m.vastus lateralis⋅PtW–1). *p < 0.05.
In men, ΔSmO2-m.intercostales was directly associated with O2–peak (r = 0.72, p = 0.020) (see Figure 5A), ΔE (r = 0.65, p = 0.019) (see Figure 5B), and ΔRR (r = 0.80, p < 0.010) (see Figure 5C), and inversely associated with ΔVt (r = –0.58, p = 0.035) (see Figure 5D). In women, ΔSmO2-m.intercostales was associated with O2–peak (r = 0.68, p = 0.012) (see Figure 5E), ΔE (r = 0.81, p = 0.003) (see Figure 5F) and ΔRR (r = 0.58, p = 0.046) (see Figure 5G), but not with ΔVt (p = 0.129) (see Figure 5H).
Figure 5. Correlation in men and women between ΔSmO2-m.intercostales with: (A,E) O2–peak(ml⋅kg–1⋅min–1), (B,F) E (E peak—E rest phase, L⋅min–1), (C,G) RR (RR peak – RR rest phase, breaths⋅min–1) and (D,H) Vt (Vt peak – Vt rest phase, L).
Our main findings are that women have a greater decrease in SmO2-m.intercostales, in spite of achieving a lower maximal change in lung ventilation, and that men experience a consistent decrease in the SmO2-m.vastus lateralis induced by a greater peripheral workload.
Women show a greater decrease of SmO2-m.intercostales (%-change) from 40 to 100% of the maximal exercise test, possibly attributed to the great relative recruitment of the respiratory musculature, required to reach the E level to meet the exercise metabolic demands (Contreras-Briceño et al., 2021). This is consistent with previous findings that reported that the contractile activity of accessory respiratory muscles (m.sternocleidomastoideus and m.scalenus) was greater in women during a bicycle endurance test at a workload of 85%. This is attributed to their thoracic and pulmonary characteristics and dependence on thoracic and accessory respiratory muscles during intense exercise has been postulated to be an adaptation that diminishes the risk of diaphragmatic fatigue (Mitchell et al., 2018). Women have increased resistive respiratory (WOBR) and elastic (WOBe) work, lower forced vital capacity, and high limited expiratory flow (Sheel and Guenette, 2008; Sheel and Romer, 2012). A similar study reported that this greater level of WOB increases the respiratory oxygen consumption in women during maximal exercise (13.8 vs. 9.4% in men) (Lomauro and Aliverti, 2018). Our findings are aligned with these data, as we found a greater ΔSmO2-m.intercostales in women than in men (39% ± 9% vs. 27% ± 19%, respectively) (see Supplementary Material I).
In both groups, the participants who achieved greater E values induced greater deoxygenation in m.intercostales, which is consistent with the finding of a previous study (Contreras-Briceño et al., 2019). Interestingly, even though women achieved a lower E than men (88.7 ± 14.5 vs. 133.1 ± 21.8 L⋅min–1, respectively), they induced a higher level of ΔSmO2-m.intercostales normalized by E; this is consistent with a previous study that reported women reaching a higher level of O2–RM in spite of only achieving a E that was 25% of men’s values (Lomauro and Aliverti, 2018). Another related observation was that participants who demonstrated a greater decrease of ΔSmO2-m.intercostales also showed a larger change in RR, for which may be an effect of ventilatory strategy during exercise.
Considering the triphasic model of exercise intensity proposed by Skinner et al. (Skinner and McLellan, 1980), the SmO2-m.intercostales showed early changes that were lower in women at VT1 (0.8 ± 0.1 vs. 1.0 ± 0.1 in men) and VT2 (0.6 ± 0.4 vs. 0.8 ± 0.1 in men). Similarly, in women, exercise intensities on VT1 lead to a greater level of oxygen consumption by the respiratory muscles (O2–RM), a phenomenon recognized as a limiting factor in physical performance progression in women (Guenette and Sheel, 2007). However, there are no differences between sexes at the peak exercise phase, which could be explained by the exponential increase of E at the expense of RR (Wanke et al., 1991).
Our results show that men have greater decreases in SmO2-m.vastus lateralis from 60 to 100% of the workload, possibly because of a greater contraction speed and cross-sectional area in locomotor muscles, leading to a sustained high intensity of exercise requiring a higher level of oxygen consumption level in peripheral muscles (Hakkinen, 1993; Fulco et al., 1999). In addition, as expected, men reached a higher absolute peripheral workload (256 vs. 164 watts in women) and PtW (3.8 vs. 3.0 watts⋅kg–1 in women), which is in agreement with previous reports, wherein it was found that men perform more peripheral muscle work that is supported by a higher neuromuscular activity and IIx fiber recruitment (Miller et al., 1993). Thus, our study found that greater decreased ΔSmO2-m.vastus lateralis normalized by relative peripheral workload was higher in men (see Figure 4B). Further, women have a larger fiber I ratio and a higher capillary density in peripheral muscles, which provides better oxygen supply allowing them to sustain muscle work with a higher oxygen consumption capacity. Such related muscle mass and fiber type characteristics are worthy of investigation in future studies (Simoneau and Bouchard, 1989; Glenmark et al., 2004).
Another aspect to discuss is the length of time of exercise protocol used. The literature suggests that peak oxygen consumption tests should not exceed 10 min of duration to limit muscle fatigue as a cause of exercise cessation (Buchfuhrer et al., 1983). However, this finding has been questioned by Midgley et al. (2008) who showed that longer test generates higher O2–peak values, suggesting that the cycloergometer tests should last between 7 and 26 min (Midgley et al., 2008). In this sense, incremental exercises by 20 watts⋅min–1 had been associated with progressive deoxygenation in the m.vastus lateralis, m.rectus femoris, and m.vastus medialis, similarly to our findings (Chin et al., 2011). Consequently, utilization of this time-stage protocol can induce higher power and total work than ramp tests (Zuniga et al., 2012). Based on these previous findings, we used an exercise protocol with a longer 2-min incremental stage, without discarding that the fiber recruitments associated with exercising could affect the results obtained; this is a relevant aspect to elucidate in future research.
Synchronous evaluation of both muscle groups determined the SmO2 ratio by calculating the preponderance of the muscle group with a higher oxygen demand during the study protocol. In this regard, we found that women had fewer changes than men (absolute, between 80 and 100%; relative, from 70 to 100%) (see Figures 3C,D). These findings could be explained by the increase in the cost of breathing, which leads to a higher blood flow to respiratory muscles, probably restricting oxygen supply to the locomotor muscles (metabolic reflex) (Boushel, 2010). Moreover, women reach a higher level of O2–RM because of the increase in E, which limits oxygen supply to the locomotor muscles (Dominelli et al., 2015, 2017), also consistent with the study by Guenette et al. (2007). Nonetheless, other authors have reported a homogeneous distribution of blood flow in women, resulting from a lower sympathetic stimulation and vascular resistance in lower extremities, which does not negatively impact exercise progression (Smith et al., 2016). These conflicting data require further examination that would include evaluation methods to clarify of the effect of metabolic reflex on sports performance in women.
By device limitations, it was not possible to completely assess the respiratory and locomotor muscles in a single test. The lack of adipose thickness measures by skinfold and/or ultrasonography reports limits verification that the muscle tissue was within the measuring range of the MOXY device (12.5 mm) (McManus et al., 2018). However, our participants were at the lower end of the normal BMI range. In addition, cyclic hormonal variations should be considered in women participants, given that edema, dehydration, and altered thermoregulation are factors affecting physical performance by modifying the ventilatory center response (Kilbride et al., 2003); this is an aspect that requires further study. Future studies could consider the stage of the menstrual cycle; however, menstrual cycle is not consistently found to influence exercise performance (McNulty et al., 2020). Recently, it was reported that 36% of high-performance female athletes stated that their menstrual cycle impacted negatively on their performance for at least some or most of the time (Heather et al., 2021). In contrast, it did not affect the production of muscle strength and power (McNulty et al., 2020; Dasa et al., 2021). Furthermore, we recommend to future research could consider the confounding influence of physical fitness levels of participants.
This study compared the differences in oxygen saturation (SmO2) of m.intercostales and m.vastus lateralis between men and women during maximal physical exercise. Our main findings indicate that women show a greater decrease in SmO2-m.intercostales, which could be attributed to anatomical and functional difference of their lungs and thoraces. In contrast, men reach a greater decrease in SmO2-m.vastus lateralis, which is associated with a higher peripheral workload. These results provide new knowledge on the primary muscle groups recruited during maximal effort and therefore shed light on the sex-differences between subjects of similar physical condition. In future studies, we suggest studying the effects of different respiratory muscle training methods in SmO2-m.intercostales during physical exercise and their impact on sports performance. Consequently, optimal guidelines for adequate training protocols could be formulated, for athletes and clinical settings.
The original contributions presented in the study are included in the article/Supplementary Material, further inquiries can be directed to the corresponding author/s.
This study was reviewed and approved by the Ethics Committee of the Faculty of Medicine of Pontificia Universidad Católica of Chile (Project no. 19042213). The participants provided their written informed consent to participate in this study. Written informed consent was obtained from the individual(s) for the publication of any potentially identifiable images or data included in this article.
ME-R, GV, OA, LG, and FC-B substantially contributed to the concept and design of the study. WR and EM-G contributed to the assay setup. ME-R, FA-R, SR-S, GR-G, and FC-B contributed to data acquisition, analysis, and interpretation. ME-R, WR, GV, OA, LG, and FC-B contributed to the data interpretation, discussion, and manuscript preparation. ME-R, EM-G, and FC-B wrote the manuscript. All authors contributed to and critically revised the manuscript for important intellectual content and approved the submitted version.
This work was supported by the grants from the Fondo Nacional de Ciencia y Tecnología (FONDECYT 1170963 to LG, FONDAP 15130011 to LG from the Agencia Nacional de Investigación y Desarrollo [ANID], Chile) and funds for translating and editing from the Pontificia Universidad Católica de Chile (VRI) to FC-B.
The authors declare that the research was conducted in the absence of any commercial or financial relationships that could be construed as a potential conflict of interest.
All claims expressed in this article are solely those of the authors and do not necessarily represent those of their affiliated organizations, or those of the publisher, the editors and the reviewers. Any product that may be evaluated in this article, or claim that may be made by its manufacturer, is not guaranteed or endorsed by the publisher.
We would like to thank all the participants, and Mr. Francisco Cerda, Mr. Gonzalo Hevia, Mr. Miguel Carrasco, and Mr. Diego Llambias for technical support in the measurements done at Laboratory of Exercise Physiology from Pontificia Universidad Catoìlica de Chile.
The Supplementary Material for this article can be found online at: https://www.frontiersin.org/articles/10.3389/fphys.2021.738063/full#supplementary-material
Alvares, T., Oliveira, G., Soares, R., and Murias, J. (2020). Near-infrared spectroscopy-derived total haemoglobin as an indicator of changes in muscle blood flow during exercise-induced hyperaemia. J. Sports Sci. 38, 751–758. doi: 10.1080/02640414.2020.1733774
Austin, K., Daigle, K., Patterson, P., Cowman, J., Chelland, S., Haymes, E., et al. (2005). Reliability of near-infrared spectroscopy for determining muscle oxygen saturation during exercise. Res. Q. Exerc. Sport 76, 440–449. doi: 10.1080/02701367.2005.10599317
Bellemare, F., Jeanneret, A., and Couture, J. (2003). Sex differences in thoracic dimensions and configuration. Am. J. Respir. Crit. Care Med. 168, 305–312. doi: 10.1164/rccm.200208-876OC
Boushel, R. (2010). Muscle metaboreflex control of the circulation during exercise. Acta Physiol. 199, 367–383. doi: 10.1111/j.1748-1716.2010.02133.x
Buchfuhrer, M., Hansen, J., Robinson, T., Sue, D., Wasserman, K., and Whipp, B. (1983). Optimizing the exercise protocol for cardipulmonary assessment. J. Appl. Physiol. Respir. Environ. Exerc. Physiol. 55, 1558–1564. doi: 10.1152/jappl.1983.55.5.1558
Cahalin, L., and Arena, R. (2015). Novel methods of inspiratory muscle training via the test of incremental respiratory endurance (TIRE). Exerc. Sport Sci. Rev. 43, 84–92. doi: 10.1249/JES.0000000000000042
Chin, L. M. K., Kowalchuk, J. M., Barstow, T. J., Kondo, N., Amano, T., Shiojiri, T., et al. (2011). The relationship between muscle deoxygenation and activation in different muscles of the quadriceps during cycle ramp exercise. J. Appl. Physiol. 111, 1259–1265. doi: 10.1152/japplphysiol.01216.2010
Contreras-Briceño, F., Espinosa-Ramírez, M., Llambias, D., Carrasco, M., Cerda, F., López, A., et al. (2019). Reliability of NIRS portable device for measuring intercostal muscles oxygenation during exercise. J. Sports Sci. 37, 2653–2659. doi: 10.1080/02640414.2019.1653422
Contreras-Briceño, F., Espinosa-Ramírez, M., Moya-Gallardo, E., Fuentes-Kloss, R., Gabrielli, L., Araneda, O. F., et al. (2021). Intercostal muscles oxygenation and breathing pattern during exercise in competitive marathon runners. Int. J. Environ. Res. Public Health 18:8287. doi: 10.3390/ijerph18168287
Contreras-Briceño, F., Espinosa-Ramírez, M., Viscor, G., and Araneda, O. F. (2020). Humidity prevents the exercise-induced formation of hydrogen peroxide and nitrite in exhaled breath condensate in recreational cyclists. Eur. J. Appl. Physiol. 120, 2339–2348. doi: 10.1007/s00421-020-04456-9
Dasa, M. S., Kristoffersen, M., Ersvær, E., Bovim, L. P., Bjørkhaug, L., Moe-Nilssen, R., et al. (2021). The female menstrual cycles effect on strength and power parameters in high-level female team athletes. Front. Physiol. 12:600668. doi: 10.3389/fphys.2021.600668
Day, J., Rossiter, H., Coats, E., Skasick, A., and Whipp, B. (2003). The maximally attainable V̇O2 during exercise in humans: the peak vs. maximum issue. J. Appl. Physiol. 95, 1901–1907. doi: 10.1152/japplphysiol.00024.2003
Dominelli, P., Archiza, B., Ramsook, A., Mitchell, R., Peters, C., Molgat-Seon, Y., et al. (2017). Effects of respiratory muscle work on respiratory and locomotor blood flow during exercise. Exp. Physiol. 102, 1535–1547. doi: 10.1113/EP086566
Dominelli, P., Render, J., Molgat-Seon, Y., Foster, G., Romer, L., and Sheel, A. W. (2015). Oxygen cost of exercise hyperpnoea is greater in women compared with men. J. Physiol. 593, 1965–1979. doi: 10.1113/jphysiol.2014.285965
Fulco, C., Rock, P., Muza, S., Lammi, E., Cymerman, A., Butterfield, G., et al. (1999). Slower fatigue and faster recovery of the adductor pollicis muscle in women matched for strength with men. Acta Physiol. Scand. 167, 233–239. doi: 10.1046/j.1365-201x.1999.00613.x
Gibson, G., Whitelaw, W., Siafakas, N., Supinski, G., Fitting, J., Bellemare, F., et al. (2002). ATS/ERS statement on respiratory muscle testing. Am. J. Respir. Crit. Care Med. 166, 518–624. doi: 10.1164/rccm.166.4.518
Glenmark, B., Nilsson, M., Gao, H., Gustafsson, J. A., Dahlman-Wright, K., and Westerblad, H. (2004). Difference in skeletal muscle function in males vs. females: role of estrogen receptor-β. Am. J. Physiol. Endocrinol. Metab. 287, 1125–1131. doi: 10.1152/ajpendo.00098.2004
Guazzi, M., Adams, V., Conraads, V., Halle, M., Vanhees, L., Co-chair, R., et al. (2012). Clinical recommendations for cardiopulmonary exercise testing data assessment in specific patient populations. Circulation 126, 2261–2274. doi: 10.1161/CIR.0b013e31826fb946
Guenette, J., Querido, J., Eves, N., Chua, R., and Sheel, A. W. (2009). Sex differences in the resistive and elastic work of breathing during exercise in endurance-trained athletes. Am. J. Physiol. Regul. Integr. Comp. Physiol. 297, 166–175. doi: 10.1152/ajpregu.00078.2009
Guenette, J., and Sheel, A. W. (2007). Physiological consequences of a high work of breathing during heavy exercise in humans. J. Sci. Med. Sport 10, 341–350. doi: 10.1016/j.jsams.2007.02.003
Guenette, J., Witt, J., McKenzie, D., Road, J., and Sheel, A. W. (2007). Respiratory mechanics during exercise in endurance-trained men and women. J. Physiol. 581, 1309–1322. doi: 10.1113/jphysiol.2006.126466
Hakkinen, K. (1993). Neuromuscular fatigue and recovery in male and female athletes during heavy resistance exercise. Int. J. Sports Med. 14, 53–59. doi: 10.1055/s-2007-1021146
Harriss, D., Macsween, A., and Atkinson, G. (2017). Standards for ethics in sport and exercise science research: 2018 update. Int. J. Sports Med. 38, 1126–1131. doi: 10.1055/s-0043-124001
Heather, A. K., Thorpe, H., Ogilvie, M., Sims, S. T., Beable, S., Milsom, S., et al. (2021). Biological and socio-cultural factors have the potential to influence the health and performance of elite female athletes: a cross sectional survey of 219 elite female athletes in Aotearoa New Zealand. Front. Sports Act. Living 3:601420. doi: 10.3389/fspor.2021.601420
Hyatt, R., and Black, L. (1969). Maximal respiratory pressures: normal values and relationship to age and sex. Am. Rev. Respir. Dis. 99, 696–702.
Kilbride, E., McLoughlin, P., Gallagher, C., and Harty, H. (2003). Do gender differences exist in the ventilatory response to progressive exercise in males and females of average fitness? Eur. J. Appl. Physiol. 89, 595–602. doi: 10.1007/s00421-003-0853-z
Knudson, R., Lebowitz, M., Holberg, C., and Burrows, B. (1983). Changes in the normal maximal expiratory flow-volume curve with growth and aging. Am. Rev. Respir. Dis. 127, 725–734.
Lomauro, A., and Aliverti, A. (2018). Sex differences in respiratory function. Breathe 14, 131–140. doi: 10.1183/20734735.000318
McManus, C., Collison, J., and Cooper, C. (2018). Performance comparison of the MOXY and PortaMon near-infrared spectroscopy muscle oximeters at rest and during exercise. J. Biomed. Opt. 23, 1–14. doi: 10.1117/1.JBO.23.1.015007
McNulty, K. L., Elliott-Sale, K. J., Dolan, E., Swinton, P. A., Ansdell, P., Goodall, S., et al. (2020). The effects of menstrual cycle phase on exercise performance in eumenorrheic women: a systematic review and meta-analysis. Sports Med. 50, 1813–1827. doi: 10.1007/s40279-020-01319-3
Midgley, A. W., Bentley, D. J., Luttikholt, H., McNaughton, L. R., Miller, G. P. (2008). Challenging a dogma of exercise physiology: does an incremental exercise test for valid VO2max determination really need to last between 8 and 12 minutes? Sports Med. 38, 441–447. doi: 10.2165/00007256-200838060-00001
Miller, A., MacDougall, J., Tarnopolsky, M., and Sali, D. (1993). Gender differences in strength and muscle fiber characteristics. Eur. J. Appl. Physiol. 66, 254–262. doi: 10.1007/BF00235103
Mitchell, R., Schaeffer, M., Ramsook, A., Wilkie, S., and Guenette, J. (2018). Sex differences in respiratory muscle activation patterns during high-intensity exercise in healthy humans. Respir. Physiol. Neurobiol. 247, 57–60. doi: 10.1016/j.resp.2017.09.002
Perrey, S., and Ferrari, M. (2018). Muscle oximetry in sports science: a systematic review. Sports Med. 48, 597–616. doi: 10.1007/s40279-017-0820-1
Sheel, A. W., and Guenette, J. (2008). Mechanics of breathing during exercise in men and women: sex versus body size differences? Exerc. Sport Sci. Rev. 36, 128–134. doi: 10.1097/JES.0b013e31817be7f0
Sheel, A. W., Richards, J., Foster, G., and Guenette, J. (2004). Sex differences in respiratory exercise physiology. Sports Med. 34, 567–579. doi: 10.2165/00007256-200434090-00002
Sheel, A. W., and Romer, L. (2012). Ventilation and respiratory mechanics. Compr. Physiol. 2, 1093–1142. doi: 10.1002/cphy.c100046
Simoneau, J., and Bouchard, C. (1989). Human variation in skeletal muscle fiber-type proportion and enzyme activities. Am. J. Physiol. Endocrinol. Metab. 257, 567–572. doi: 10.1152/ajpendo.1989.257.4.E567
Skinner, J. S., and McLellan, T. H. (1980). The transition from aerobic to anaerobic metabolism. Res. Q. Exerc. Sport 51, 234–248. doi: 10.1080/02701367.1980.10609285
Smith, J., Broxterman, R., Hammer, S., Alexander, A., Didier, K., Kurti, S., et al. (2016). Sex differences in the cardiovascular consequences of the inspiratory muscle metaboreflex. Am. J. Physiol. Regul. Integr. Comp. Physiol. 311, 574–581. doi: 10.1152/ajpregu.00187.2016
Vainshelboim, B., Rao, S., Chan, K., Lima, R., Ashley, E., and Myers, J. (2017). A comparison of methods for determining the ventilatory threshold: implications for surgical risk stratification. Can. J. Anesthesia 64, 634–642. doi: 10.1007/s12630-017-0862-8
Wanke, T., Formanek, D., Schenz, G., Popp, W., Gatol, H., and Zwick, H. (1991). Mechanical load on the ventilatory muscles during an incremental cycle ergometer test. Eur. Respir. J. 4, 385–392.
Welch, J., Archiza, B., Guenette, J., West, C., and Sheel, A. W. (2018). Sex differences in diaphragmatic fatigue: the cardiovascular response to inspiratory resistance. J. Physiol. 596, 4017–4032. doi: 10.1113/JP275794
Keywords: cost of breathing, exercise, near-infrared spectroscopy, respiratory muscles, sex
Citation: Espinosa-Ramírez M, Moya-Gallardo E, Araya-Román F, Riquelme-Sánchez S, Rodriguez-García G, Reid WD, Viscor G, Araneda OF, Gabrielli L and Contreras-Briceño F (2021) Sex-Differences in the Oxygenation Levels of Intercostal and Vastus Lateralis Muscles During Incremental Exercise. Front. Physiol. 12:738063. doi: 10.3389/fphys.2021.738063
Received: 08 July 2021; Accepted: 10 September 2021;
Published: 01 October 2021.
Edited by:
Simone Luti, Università degli Studi di Firenze, ItalyReviewed by:
Christoph Zinner, Hessian College of Police and Administration, GermanyCopyright © 2021 Espinosa-Ramírez, Moya-Gallardo, Araya-Román, Riquelme-Sánchez, Rodriguez-García, Reid, Viscor, Araneda, Gabrielli and Contreras-Briceño. This is an open-access article distributed under the terms of the Creative Commons Attribution License (CC BY). The use, distribution or reproduction in other forums is permitted, provided the original author(s) and the copyright owner(s) are credited and that the original publication in this journal is cited, in accordance with accepted academic practice. No use, distribution or reproduction is permitted which does not comply with these terms.
*Correspondence: Felipe Contreras-Briceño, ZmNvbnRyZXJhc2JAdWMuY2w=
†ORCID: Maximiliano Espinosa-Ramírez, orcid.org/0000-0002-0840-1904; Eduardo Moya-Gallardo, orcid.org/0000-0001-9356-9009; W. Darlene Reid, orcid.org/0000-0001-9980-8699; Ginés Viscor, orcid.org/0000-0003-4942-2346; Oscar F. Araneda, orcid.org/0000-0002-9501-3159; Luigi Gabrielli, orcid.org/0000-0002-1551-7147; Felipe Contreras-Briceño, orcid.org/0000-0002-0674-7506
Disclaimer: All claims expressed in this article are solely those of the authors and do not necessarily represent those of their affiliated organizations, or those of the publisher, the editors and the reviewers. Any product that may be evaluated in this article or claim that may be made by its manufacturer is not guaranteed or endorsed by the publisher.
Research integrity at Frontiers
Learn more about the work of our research integrity team to safeguard the quality of each article we publish.