- 1Peptide and Proteomics Division, Defence Institute of Physiology and Allied Sciences, New Delhi, India
- 2Neurophysiology Department, Defence Institute of Physiology and Allied Sciences, New Delhi, India
Acute exposure to high altitude perturbs physiological parameters and induces an array of molecular changes in healthy lowlanders. However, activation of compensatory mechanisms and biological processes facilitates high altitude acclimatization. A large number of lowlanders stay at high altitude regions from weeks to months for work and professional commitments, and thus are vulnerable to altitude-associated disorders. Despite this, there is a scarcity of information for molecular changes associated with long-term stay at high altitudes. In the present study, we evaluated oxygen saturation (SpO2), heart rate (HR), and systolic and diastolic blood pressure (SBP and DBP) of lowlanders after short- (7 days, HA-D7) and long-term (3 months, HA-D150) stay at high altitudes, and used TMT-based proteomics studies to decipher plasma proteome alterations. We observed improvements in SpO2 levels after prolonged stay, while HR, SBP, and DBP remained elevated as compared with short-term stay. Plasma proteomics studies revealed higher levels of apolipoproteins APOB, APOCI, APOCIII, APOE, and APOL, and carbonic anhydrases (CA1 and CA2) during hypoxia exposure. Biological network analysis also identified profound alterations in lipoprotein-associated pathways like plasma lipoprotein assembly, VLDL clearance, chylomicron assembly, chylomicron remodeling, plasma lipoprotein clearance, and chylomicron clearance. In corroboration, lipid profiling revealed higher levels of total cholesterol (TC), triglycerides (TGs), low-density lipoprotein (LDL) for HA-D150 whereas high density lipoproteins (HDL) levels were lower as compared with HA-D7 and sea-level indicating dyslipidemia. We also observed higher levels of proinflammatory cytokines IL-6, TNFα, and CRP for HA-D150 along with oxidized LDL (oxLDL), suggesting vascular inflammation and proartherogenic propensity. These results demonstrate that long-term stay at high altitudes exacerbates dyslipidemia and associated disorders.
Introduction
Human beings experience compromised oxygen delivery at high altitudes (≥ 2,500 m) due to decreased atmospheric pressure and partial pressure of oxygen. This condition of hypobaric hypoxia is the unavoidable, unmodifiable, and uniform environmental stress for everyone at any given altitude. In addition, extreme cold, solar radiation, and aridity are other major stressors for lowlanders at high altitudes, at least for the first few days upon the arrival. Lowlanders elicit an integrated physiological (including respiratory and cardio-pulmonary) and hematological response for acclimatization to high altitude (Houston and Riley, 1947; Bartsch and Gibbs, 2007; Naeije, 2010). It is generally accepted that increased ventilation and hemoconcentration facilitate near normalization of the arterial oxygen content of lowlanders after an approximately 7-day residence at a high altitude (Muza et al., 2010). More recently, several proteins and biochemical pathways have been reported facilitating high altitude acclimatization (Padhy et al., 2016; Tang et al., 2018; Gangwar et al., 2019; Pooja et al., 2020). Failure in such responses may lead to high altitude illnesses, ranging from acute mountain sickness (AMS) to severe and life-threatening forms like high altitude pulmonary edema (HAPE), high altitude cerebral edema (HACE), thromboembolism, and high altitude polycythemia (HAPC) (Reynafarje et al., 1959; Hackett and Roach, 2001; Gallagher and Hackett, 2004; Palmer, 2010; Gupta and Ashraf, 2012). Both animal and human studies have reported that hypobaric hypoxia-induced oxidative stress and inflammation are important factors for the genesis of these high altitude maladies (Hartmann et al., 2000; Sarada et al., 2008; Himadri et al., 2010; Siervo et al., 2014; Boos et al., 2016; Pena et al., 2020a; Pham et al., 2021).
More than 40 million people including thousands of army personnel, government officials, miners, pilgrims, trekkers, and porters visit high altitude regions annually and are at risk of suffering from altitude illness and potentially dying from it (Moore, 2001; Basnyat, 2018). The working conditions and duration of high altitude residence differ among lowlanders depending on their professional requirements. On average trekkers, porters, sportsperson, pilgrims, and tourists spend few days to weeks at high altitudes whereas military personnel, diplomats, miners, and scientists spend months to years at high altitudes. Both acute and chronic exposure to high altitude induces several metabolic alterations and adjustments (Murray, 2016; Murray et al., 2018). More specifically, hypobaric hypoxia exposure alters lipid profiles (Gangwar et al., 2019) inducing hypercholesterolemia and hypertriglyceridemia (Mohanna et al., 2006; Sherpa et al., 2011; Vats et al., 2013) and associated cardiovascular disorders above 3,000 m (Virues-Ortega et al., 2009; Mallet et al., 2021). In addition, extended stay of young and healthy lowlanders at high and extreme altitudes is reportedly associated with a higher risk of spontaneous vascular thrombosis (Anand et al., 2001), massive infarcts, and stroke (Jha et al., 2002). Hence, studying lowlander molecular response above 3,000 m and extended residence period (weeks to months) is a pertinent scientific endeavor that can save both money and manpower.
Blood plasma is a highly accessible sample for monitoring the health status of an individual (Geyer et al., 2016), and high-throughput plasma proteome analysis is a popular omics method to investigate system-level protein alterations that may be rooted from basic science or clinical perspective (Pernemalm and Lehtio, 2014; Ignjatovic et al., 2019). Several research groups including ours have used plasma proteomics for gaining insight into high altitude acclimatization (Ahmad et al., 2011, 2013; Levett et al., 2011, 2015; Julian et al., 2014; Yang et al., 2014; Lu et al., 2018; Wang et al., 2019) and high altitude disorders like AMS (Julian et al., 2014; Lu et al., 2018), HAPE (Ahmad et al., 2011; Yang et al., 2014), polycythemia (Wang et al., 2019), and CMS (Zhang et al., 2021). Despite these studies, no information is available for plasma proteome level alterations of lowlanders during prolonged (months) stay at high altitude. Hence, we sought to investigate global plasma proteome alterations of lowlanders during a 3-month stay at 4,176 m as compared to 7 days stay at high altitude and sea level. In addition, we have also monitored physiological indices, proinflammatory cytokines, and lipid profiles. To the best of our knowledge, this is the first plasma proteomics investigation reporting molecular pathways perturbed during prolonged exposure to high altitude. We have further used biochemical estimation, ELISA studies, and Western blot analysis to validate our proteomics observations. Our present results indicate that acute exposure to high altitude induces dyslipidemia among lowlanders chronic exposure.
Materials and Methods
Study Groups
We studied 105 healthy, nonsmoking, male military volunteers (age: 22–37 years, height: 170 ± 4 cm, weight: 64 ± 3 kg) at sea level (Pathankot, India, altitude 331 m). All the volunteers are lowlanders-born, living at sea level, and have not been exposed to high altitude for the last 1 year. Subsequently, all the volunteers traveled to Leh (altitude 3,520 m) by road and stayed there for 7 days (high altitude–day seven group, (HA-D7 group; n = 55). Then, the volunteers spent 1 day reaching Fukche (4,176 m) and were stationed there for 3 months (high altitude–day 150 group (HA-D150 group; n = 40). All the volunteers followed the same diet regimen during the entire study period. The present study was performed according to the Declaration of Helsinki, and the experimental design and procedures for conducting the experiment were approved by the institutional ethics committee (IEC/DIPAS/B2/1). Informed written consent was obtained from all the participants and each volunteer was informed of the possible risk and discomforts involved in the study. During the whole study duration, regular medical examinations were performed to determine health status.
Evaluation of Physiological Parameters
Physiological parameters including oxygen saturation (SpO2), heart rate (HR), systolic blood pressure (SBP), and diastolic blood pressure (DBP) were recorded at sea level and regularly at high altitudes. SpO2 was measured using a pulse oximeter (Smart Oxy Lite, BPL, India) from the right index finger as the average of three readings. Systolic blood pressure (SBP) and diastolic blood pressure (DBP) were recorded using a standard sphygmomanometer.
Collection of Blood Plasma
Overnight fasting venous blood samples were drawn in EDTA vacutainer at Pathankot (sea level), Leh (HA-D7), and Fukche (HA-D150 groups). Plasma was separated by centrifugation at 1,500 x g for 15 min at 4°C and was stored at −80°C with mammalian protease inhibitor (P8340, Sigma-Aldrich) for further studies.
TMT-Labeling and Plasma Protein Profiling
High abundant plasma proteins from each group (Sea level, HA-D7, and HA-D150) were depleted using High-Select™ Top14 Abundant Protein Depletion Resin (A36370, Thermo Scientific) according to the instructions of the manufacturer. An equal amount (100 μl) of the depleted sample (n = 8) was pooled together to comprise 800 μl of total volume for each group. Total protein content was determined with the ToPA Bradford Protein Assay kit (Cat No. K-0014, ITSI Biosciences, USA), and the consistency of protein profiles was checked using SDS-PAGE for each group. Subsequently, a 400 μg depleted protein sample was mixed with 75 μl of 8 M urea buffered in 1 M tetraethylammonium bromide (TEAB). The sample reduction was performed with 5 mM DL-dithiothreitol (DTT) for 30 min at 56°C, followed by alkylation with 11 mM iodoacetamide (IAM) for 15 min at room temperature in darkness. The sample was diluted by adding 200 mM TEAB and digested at a trypsin-to-protein mass ratio of 1:50 for the first digestion overnight and 1:100 for a 4-h digestion. Finally, each group of digested peptides was labeled with the TMT reagents following manufacturer protocols (Thermo Fisher Scientific, Torrance, CA, USA). Sample labeling was as follows: Sea level: 128, HA-D7: 129, and HA-D150: 130.
For multidimensional protein identification technology (MudPIT) analysis, all the labeled samples were combined and then fractionated by strong cation exchange (SCX). The fractions eluted at 50, 75, 150, 250, and 450 mM of ammonium acetate were collected. The samples were desalted using ZipTip, the desalted sample were dried in a speedvac, then resuspended in the appropriate mobile phase. For LC-MS/MS, the chromatography was performed with a Thermo EASY-nLCsystem. Peptides were eluted from the column using a linear acetonitrile gradient from 5 to 32% acetonitrile over 90 min followed by high and low organic washes for another 20 min coupled to Q Exactive™ mass spectrometer (Thermo Scientific) via a nanospray source with the spray voltage set to 2.0 kV and the ion transfer capillary set at 250°C. A data-dependent top 15 method was used where a full MS scan from m/z 350–1,600 was followed by MS/MS scans of the 15 most abundant ions. Each ion was subjected to Higher energy C trap dissociation (HCD) for fragmentation, peptide identification, and TMT reporter ion detection. Raw data files of each SCX fraction were searched against the most recent database for humans downloaded from UniProt using the MudPIT option in Proteome Discoverer 2.2 (Thermo Scientific) and the Sequest HT search algorithm. For protein identification results, only peptides identified with high confidence were used. For confidence, the Percolator algorithm was used for peptide spectrum match validation in database searches. The false discovery rate (FDR) threshold calculated in Proteome Discoverer Percolator with high confidence peptides (0.01) were used for protein identification.
Pathway and Network Analysis
The identified proteins were analyzed according to GO terms for biological process, cellular component, and molecular function using the Reactome database (http://www.reactome). Pathway enrichment analysis was assessed using the Kyoto Encyclopedia of Genes and Genomes (KEGG) pathway database (http://www.genome.jp/kegg/ or http://www.kegg.jp/).
Validation of Protein Levels by Immunoblotting
The protein levels in study groups were further validated with immunoblot analysis. Plasma containing 20 μg of protein was separated on 10% SDS-PAGE gels and transferred onto nitrocellulose membranes. The membranes were blocked in 5% BSA blocking buffer in PBS-0.1% Tween-20 (PBST) overnight at 4°C. Subsequently, the membranes were washed with PBST three times for 5 min each. It was followed by incubation with the Apo-B (PA5-86101, Invitrogen), Apo-CIII (701238, Thermo Fischer Scientific), and β-tubulin (MA516308, Invitrogen) for 2 h and secondary antibodies for 1.5 h at room temperature, respectively. The blots were washed again with PBST three times for 5 min each and were observed by adding chemiluminescent peroxidase substrate (Cat. No. 34095, ThermoFisher Scientific, USA) on UVP Biospectrum. Densitometry analysis was performed using Image J software.
Lipid Profiling
Plasma lipid parameters including cholesterol, HDL, LDL, and triglycerides were analyzed on a Randox Monaco clinical chemistry analyzer (Randox Laboratories, Crumlin, UK).
Evaluation of Inflammatory Cytokines
The plasma CRP level was evaluated using a human C-reactive protein ELISA Kit (E0829h, EIAab Science, Wuhan, CHINA) as per the instructions of the manufacturer. The levels of IL-6, TNFα, and ox-LDL were evaluated using Human IL-6 PicoKine™ ELISA Kit (EK0410, Boster Biologicals), Human TNFα ELISA Kit (950.090.096, Diaclone, Besancon Cedex, France), and human oxidized low density lipoprotein (OxLDL) ELISA Kit (E-EL-H0124, Elabscience), respectively, as per the recommendations of the manufacturer.
Statistical Analysis
All the values were represented as mean ± SD. Statistical analysis was performed using ANOVA with Newman–Keuls post-hoc tests, and a p-value of < 0.05 was considered significant. All analysis was performed using GraphPad Prism software version 7.0 (GraphPad Software, California, USA). Pearson correlation analysis was performed for depicting the correlation between physiological and biochemical parameters.
Results
Evaluation of Physiological Parameters
Physiological parameters including SpO2, HR, SBP, DBP, and Hb were recorded for all the volunteers at sea level (lowlander) and also at HA (HA-D7 and HA-D150), and are represented in Table 1. Ascent to HA resulted in a decrease in SpO2 level for both HA-D7 (87.47 ± 2.85, p < 0.001) and HA-D150 (95.42 ± 0.78) as compared with sea level (98.99 ± 0.001). Comparison between HA-D7 and HA-D150 revealed an 8% increase (p < 0.001) of SpO2 during a long-term stay at HA. The HR increased upon HA stay, irrespective of duration, and the highest HR was observed for HA-D150 (83.14 ± 12.81 bpm, p < 0.001) as compared with both sea level (76.60 ± 8.26 bpm) and HA-D7 (77.51 ± 12.09 bpm). Similarly, SBP and DBP also increased upon HA ascent, and HA-D150 possessed the highest SBP (127.44 ± 12.99 mmHg, p < 0.001) and DBP (84.55 ± 9.16 mmHg, p < 0.001) as compared with both lowlander and HA-D7. HA exposure also resulted in increased hemoglobin for both HA-D7 (15.91 ± 0.62 g/dL, p < 0.01) and HA-D150 (15.30 ± 1.05, p < 0.05) as compared with lowlanders (14.07 ± 0.16 g/dL).
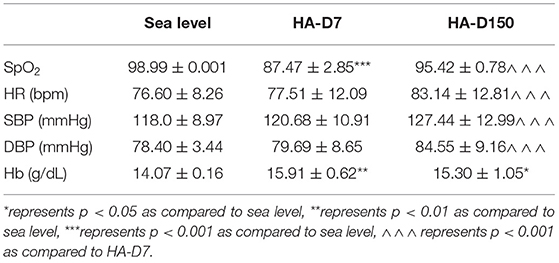
Table 1. Physiological parameters of volunteers at sea level (n = 105) who were subsequently exposed to HA for 7 days (HA-D7, n = 55) and 3 months (HA-D150, n = 40).
Plasma Proteomics Study
Plasma proteomics studies were performed to identify global perturbations in plasma proteins and pathways during short- and long-term stay at HA. Plasma was first depleted for abundant proteins followed by TMT labeling and LC-MS/MS-based quantitative proteomics studies. A total of 17,336 peptides were identified in this experiment including low confidence peptides. However, with higher stringency (0.01% FDR), a total of 377 differentially abundant proteins were identified (Figure 1A; Supplementary Table 1). The frequency distribution of the 377 quantitative proteins, log2-transformed ratios fitted normality distribution (Figure 1B). For the HA-D7 group, a total of 75 proteins were found to be downregulated (< 0.8-fold) and 62 proteins were found to be upregulated (>1.2-fold), whereas 56 proteins were downregulated (<0.8-fold) and 79 proteins were upregulated (>1.2-fold) for HA-D150. Comparing both HA-D7 and HA-D150, we observed 32 downregulated proteins (<0.8-fold) and 27 upregulated proteins (>1.2-fold) common between short- and long term stay at high altitude (Supplementary Tables 2, 3).
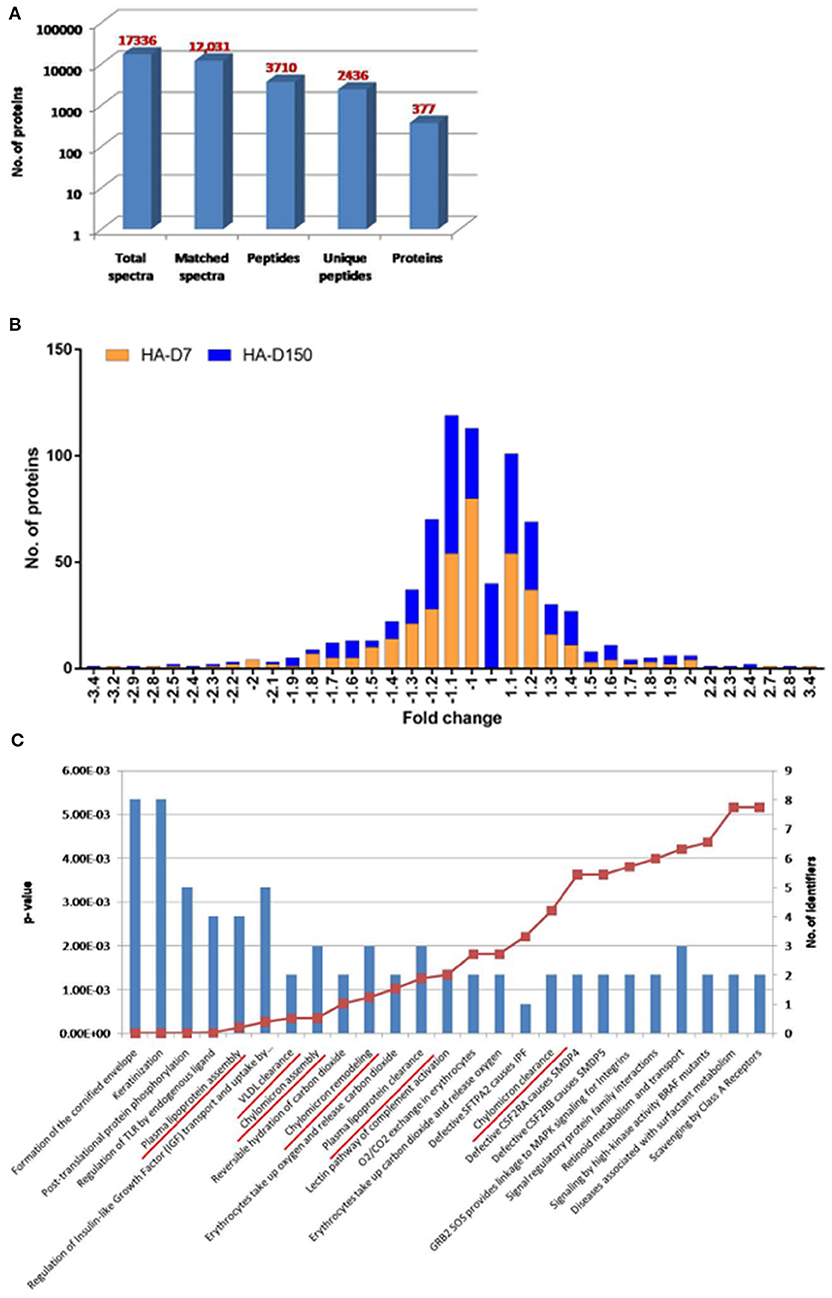
Figure 1. TMT based plasma proteomics analysis. (A) Bar graph representing spectra, peptides, and proteins identified from TMT-based LC-MS/MS. (B) The quantitative ratio histogram of quantitative proteins identified in the study groups (HA-D7 and HA-D150 with respect to sea level). (C) Top 25 significant pathways for the identified common upregulated proteins in HA-D7 and HA-D150 groups representing a number of identified proteins along with their p-value. Underlined signify identified pathways involved in lipoprotein metabolism.
Identified proteins were uploaded to a curated database “Reactome” (https://reactome.org) to identify underlying pathways altered during high altitude exposure. Interestingly, the top 25 significant pathways identified from common upregulated proteins at high altitude included plasma lipoprotein assembly, VLDL clearance, chylomicron assembly, chylomicron remodeling, plasma lipoprotein clearance, and chylomicron clearance (Figure 1C). In corroboration, we observed higher levels of apolipoproteins like APOB (1.32-fold), APOCI (1.36-fold), APOCIII (1.97-fold), APOCIV (1.34-fold), APOCIV + APOCII (1.15-fold), APOE (1.41-fold), and APOL (1.42-fold) for HA-D7. Similarly, we observed higher levels of Apo-AII (1.35-fold), Apo-B (1.34-fold), Apo-CI (1.39-fold), Apo-CIII (1.79-fold), Apo-CIV (1.17-fold), Apo-CIV + Apo-CII (1.20-fold), Apo-E (1.35-fold), and Apo-L (1.36-fold) for HA-D150. Comparing both the groups, we observed higher levels of carbonic anhydrase 1 (CA1, 2.30-fold) and carbonic anhydrase 2 (CA2, 2.36-fold) for HA-D150. We also observed a lower abundance of several cytoskeletal proteins like Profilin-1 (PROF-1), actin, cytoplasmic 2 (ACTG1), Talin-1 (TLN1), tubulin alpha chain, tubulin beta chain, myosin-9 (MYH9), and alpha-actinin-1 (ACTN1) in both the study groups (Supplementary Tables 1, 3).
Validation of Plasma Proteomics Analysis
Validation of protein levels by western blot revealed that APOB levels were higher in HA-D7 (1.33-fold) and HA-D150 (2.36-fold) as compared with sea level. Similar higher levels of Apo-CIII (5.06-fold for HA-D7, p < 0.001, and 2.17-fold for HA-D150) were observed as compared to sea level (Figures 2A,B).
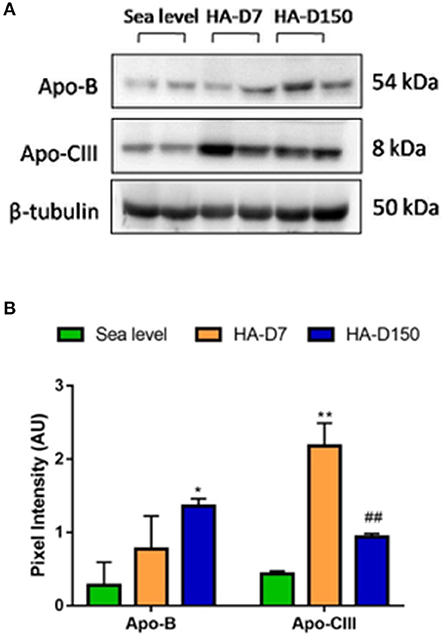
Figure 2. Validation of plasma proteomics data (n = 6). (A) Western blot analysis of Apo-B and Apo-CIII along with tubulin as a loading control. (B) Densitometry analysis of Apo-B and Apo-CIII with respect to tubulin. *represents p < 0.05, **represents p < 0.01 with respect to sea level and ##represents p < 0.01 with respect to HA-D7.
Altered Lipid Profile During a Prolonged Stay at HA
To support the proteomics-based observations of altered apolipoproteins, lipid profiles of all the study groups were evaluated (Table 2). Elevated levels of plasma total cholesterol (225.63 ± 83.38 mg/dL, p < 0.05), LDL (135.1 ± 72.53 mg/dL, p < 0.05) and triglycerides (147.23 ± 76.74 mg/dL, p < 0.001) were observed for HAD-150 as compared with sea-level values. Concomitantly, higher plasma LDL level (135.1 ± 72.53mg/dL, p < 0.05) and lower HDL level (41.75 ± 4.98 mg/dL) was observed for HA-D150 as compared with sea-level values (112.17 ± 37.3 mg/dL and 47.19 ± 8.32 mg/dL, respectively). Consequently, the LDL/HDL ratio and total cholesterol/HDL ratio were elevated for HA-D150 (3.24 ± 14.58 and 5.40 ± 16.76, respectively) as compared to both HA-D7 and sea level. Moreover, the atherogenecity index of plasma (AIP) represented as log10 (TG/HDL-C) was also found to be highest in HA-D150 (Table 2).
Evaluation of Inflammatory Markers
We evaluated levels of inflammatory markers CRP, IL-6 and TNFα along with ox-LDL in all the study groups. We observed higher CRP levels in altitude exposed groups (1.99-fold for HA-D7, p < 0.05 and 1.79-fold for HA-D150) as compared to sea level values (Figure 3D). We observed similar higher levels of IL-6 and TNFα for HA-D7 (1.97-fold, p < 0.01 and 1.11-fold, p < 0.05) and HA-D150 (2.11-fold, p < 0.01 and 1.14-fold, p < 0.01) as compared to sea level values (Figures 3A,B). Interestingly, plasma level of ox-LDL was 2.29-fold (p < 0.001) and 2.18-fold (p < 0.001) higher in HA-D150 (4626 ± 520 pg/ml) as compared to sea level values (2018 ± 256 pg/ml) and HA-D7 (2119 ± 419.8 pg/ml) respectively (Figure 3C).
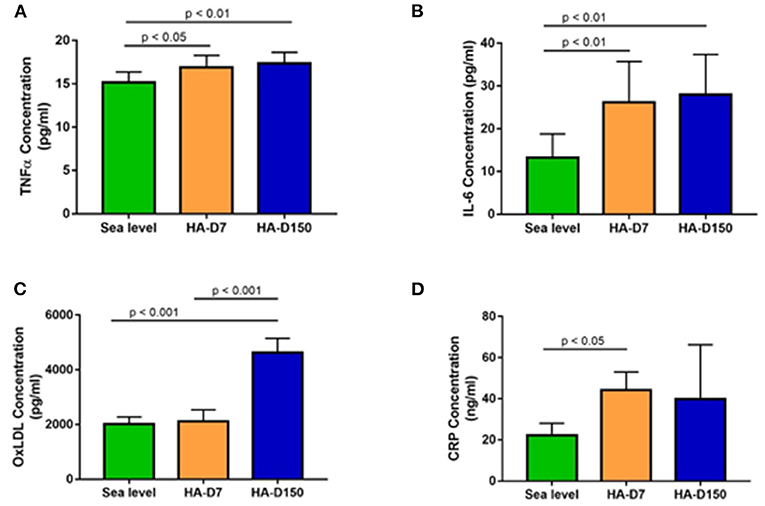
Figure 3. Assessment of inflammatory cytokines in the study groups (n = 8). ELISA-based estimation of (A) TNF-α, (B) IL-6, (C) ox-LDL, and (D) CRP for sea level, HA-D7, and HA-D150 groups.
Discussion
The present study reports the physiological and plasma proteome level changes for lowlanders during short- and long-term stay at high altitudes. Comparing the short-term and long-term plasma proteomes with sea level plasma proteome, the present study highlights activation of proatherogenic lipoproteins at high altitude that exacerbates during longer stay durations.
The arterial oxygen saturation level of lowlanders decreased upon acute exposure to high altitude (HA-D7) and increased significantly after long-term stay (HA-D150), though the levels were lower than sea level. This signifies healthy acclimatization to HA (Calbet et al., 2003; Soria et al., 2016) as severe and prolonged oxygen desaturation can lead to AMS (Mandolesi et al., 2014). As expected, the hemoglobin levels also increased significantly at high altitudes and persistently high levels were maintained irrespective of the duration of the stay at HA. Both the systolic and diastolic blood pressure along with heart rate increased upon HA exposure and was more pronounced during a long-term stay at HA. This is majorly due to massive activation of the sympathetic nervous system, contributed by noradrenaline despite enhanced hemoglobin levels and concomitant improved arterial oxygen content (Bartsch and Gibbs, 2007; Hainsworth et al., 2007).
Our TMT-based plasma proteomics studies identified a differential abundance of 377 proteins in HA-D7 and HA-D150 as compared to sea level. Using a cut-off of 0.8-fold for downregulation and 1.2-fold for upregulation, we identified 75 and 56 down-regulated proteins for HA-D7 and HA-D150 groups respectively. Similarly, we identified 62 and 79 upregulated proteins for HA-D7 and HA-D150 groups respectively. Bioinformatics analysis revealed alterations in several lipoprotein-associated pathways like plasma lipoprotein assembly, VLDL clearance, chylomicron assembly, plasma lipoprotein clearance, and chylomicron clearance. We observed higher levels of lipoproteins like APOB, APOCI, APOCIII, APOE, and APOL for both HA-D7 and HA-D150 in accordance with our previous studies (Gangwar et al., 2019). Interestingly, we observed higher levels of Apo-B for both HA-D7 and HA-D150 representing higher levels of low-density lipoprotein (LDL). Findings from several large studies indicate that elevated triglyceride (TG) levels along with increased levels of small dense LDL particles with concomitantly decreased levels of HDL cholesterol are often a component of atherogenic dyslipidemia (Reiner, 2017). We also observed similar high levels of APOCI, the endogenous inhibitor of cholesterol ester transfer protein (CETP) that limits the exchange of lipids has reportedly enhanced the risk of atherosclerosis (Westerterp et al., 2007). Our plasma proteomics and western blot studies identified higher APOCIII levels in high-altitude exposed groups. APOCIII is now recognized as a key regulator in severe hypertriglyceridemia due to its inhibition of lipoprotein lipase (LPL) and hepatic lipase. ApoCIII gain of function mutations are associated with atherosclerosis and coronary heart disease (CHD) and contribute to the development of hypertriglyceridemia, whereas loss of function mutations are associated with lower levels of plasma triglycerides and attenuation of vascular inflammatory processes (Pollin et al., 2008; Tg et al., 2014; Natarajan et al., 2015; Rocha et al., 2017).
Lipid profiling revealed higher levels of cholesterol, triglycerides, LDL, and lower levels of HDL were observed for HA-D150 as compared to HA-D7 and sea level groups (Table 2). It is noteworthy that sea-level values for total cholesterol, triglycerides (TGs), and LDL were within the physiological range, increased after high altitude exposure, and levels were further elevated during the prolonged stay. We also observed a moderate correlation of diastolic blood pressure (DBP) with LDL (R-squared = 0.46, p = 0.04) for HA-D7 group. Persistent hypoxia at high altitude induces HIF-1α that upregulates stearoyl-CoA desaturase (SCD)-1 in the sterol regulatory element-binding protein (SREBP)-1c pathway resulting in increased hepatic de novo TGs synthesis (Siques et al., 2020). In contrast, we observed the lowest HDL levels for HA-D150. High altitude exposure compromises HDL maturation and alters the levels of HDL associated proteins limiting its protective functions (Gangwar et al., 2019). Consequently, ratios of LDL/HDL, cholesterol/HDL, and AIP, potential risk factors for coronary artery disease (CAD) were elevated for HA-D150. Both animal and human studies have reported that hypoxia increases plasma triglycerides by decreasing tissue uptake (Barnholt et al., 2006; Siques et al., 2014). Epidemiological studies have reported a high prevalence of hypercholesterolemia and low HDL levels for indigenous high land native populations of Peru (Mohanna et al., 2006), Chile (Santos et al., 2001), and Tibet (Sherpa et al., 2011). These studies along with our current observation suggest that extended stay at high altitude results in dyslipidemia and elevates proatherogenic lipoprotein levels.
There is accumulating evidence that HA exposure is associated with an inflammatory response and associated endothelial activation/dysfunction (Hartmann et al., 2000; Bruno et al., 2014; Boos et al., 2016) that aggravates many forms of cardiovascular diseases (Riley and Gavin, 2017; Parati et al., 2018). Healthy young lowlanders with no preexisting risk factors develop massive infarct and ischemic stroke during a prolonged stay at HA (above 4,270 m) (Jha et al., 2002). Acclimatized lowlanders exhibit increased levels of coronary risk factors after 15–18 months stay at the Indian trans-Himalayan Ladakh region (altitude more than 3,500 m) (Dhar et al., 2018). Hence, we measured proinflammatory cytokines IL-6, CRP, TNFα, and oxidized LDL (oxLDL) levels in all three groups. The levels of IL-6 increased after altitude exposure (HA-D7) and levels were further increased after prolonged exposure (HA-D150). Studying cytokines after high altitude exposure, Hartmann et al. have reported increased IL-6 levels indicating considerable inflammation (Hartmann et al., 2000). Increased IL-6 level serves as an independent predictor of AMS (Boos et al., 2016) and plays a role in the pathogenesis of HAPE (Kubo et al., 1998). Similar higher levels of TNFα were observed after high altitude exposure and the highest levels were recorded for the HA-D150 group. Studying inflammatory cytokines in BAL fluid of HAPE patients, Kubo et al. have reported higher TNFα levels (Kubo et al., 1998) supporting our present observations. We also observed increased CRP levels for HA-D7 and the levels subsequently decreased for HA-D150 but remained higher than the sea level. Increased CRP levels have been reported for both acute (Hartmann et al., 2000; Gangwar et al., 2019) and prolonged high altitude exposure (Hu et al., 2016). Elevated levels of plasma CRP are associated with the risk of atherosclerotic events in general populations and show a predictive value even in terms of secondary prevention (Libby and Ridker, 2004; Calabro et al., 2009). Hence, we measured oxLDL levels that contribute to atherosclerotic plaque formation and progression by several mechanisms, including the induction of endothelial cell activation and dysfunction, macrophage foam cell formation, and smooth muscle cell migration and proliferation (Pirillo et al., 2013; Poznyak et al., 2020). We observed significantly higher oxLDL levels after a prolonged stay at high altitudes as compared to HA-D7 and sea level. Our present observations of elevated proinflammatory cytokines and oxLDL levels after prolonged exposure to high altitude indicate vascular inflammation leading to a proatherosclerotic state. A recent study evaluating long-term chronic intermittent hypobaric hypoxia-induced right ventricular hypertrophy has reported upregulation of lectin-like oxidized low-density lipoprotein receptor-1 (LOX-1), a major OxLDL receptor on endothelial cell surface supports our current observations (Pena et al., 2020b).
We identified CA1 and CA2 as the topmost upregulated proteins for HA-D150, on average 2-fold higher than the HA-D7 group. These two are a member of the CA family that reversibly catalyzes the hydration of CO2 to form , which then rapidly binds to calcium ions to form calcium carbonate (Supuran, 2008). This well-known reaction is involved in a range of physiologic processes, ranging from CO2 metabolism to cell proliferation and glucose/lipid metabolism (Gilmour, 2010). It is important to note that acetazolamide and other related CA inhibitors have been effectively used for the prevention and treatment of AMS and remain the standard of care for this indication (Swenson, 2006; Nieto Estrada et al., 2017). While there exists no difference between red blood cell CA activity between lowlanders and native highlanders (Gamboa et al., 2000), increased activity has been reported in patients with obstructive sleep apnea (OSA) (Hoff et al., 2020). Inhibition of CA activity curtails inflammation and experimental hypertension (Hudalla et al., 2019) suggesting that CAs are involved in the inflammation and vascular calcification (Adeva-Andany et al., 2015; Yuan et al., 2019). Though our results do not provide any direct evidence for CA-mediated vascular inflammation and calcification, their profound upregulation and existence of a proatherogenic propensity during long-term stay at high altitude suggests a possible link between CA and high altitude-induced dyslipidemia.
The present study is limited by studying only plasma samples and blood parameters for understanding the body response to hypoxia, whereas at organ and tissue level the response is more complex. All global plasma proteomics studies are constrained by a high dynamic range of plasma proteins, and even after several high abundance protein depletion steps, it is not possible to detect very low abundance proteins that may be of critical importance. We have studied only male volunteers for 3 months in the present study for specific reasons. A vast number of studies have reported sexual dimorphism for cardiovascular response and disorders particularly due to the sex hormone estrogen (Hester et al., 2019; Horiuchi et al., 2019; Hou et al., 2019; Shen et al., 2020; Ndzie Noah et al., 2021). It is important to note that estrogen levels widely vary between both the sex (Khosla et al., 1998), and cardiac adaptive responses significantly vary between males and females during chronic hypoxia (Bohuslavova et al., 2010). Hence to minimize variations, women were consciously excluded from the present study. However, such proteomics studies with women and longer durations of high altitude stay (6 months or more) could provide additional molecular information.
In summary, the present study reports elevated levels of SpO2, HR, SBP, and DBP after long-term exposure to high altitude as compared to short-term exposure whereas the hemoglobin levels remained the same. Global plasma proteomics studies revealed upregulation of several apolipoproteins, and subsequent bioinformatics analysis revealed perturbation in assembly, remodeling, and clearance of plasma lipoproteins including VLDL and chylomicrons. In corroboration, we also observed higher TC, TGs, and LDL after a prolonged stay at high altitude indicating dyslipidemia. These observations were further supported by higher levels of inflammatory cytokines IL-6, TNFα, CRP as well as oxLDL. These cumulative results indicate persistent vascular inflammation after long-term exposure to high altitude leading to dyslipidemia and a proatherosclerotic condition.
Data Availability Statement
The original contributions presented in the study are publicly available. This data can be found here: The mass spectrometry proteomics data have been deposited to the ProteomeXchange Consortium via the PRIDE partner repository with the dataset identifier PXD028070.
Ethics Statement
The studies involving human participants were reviewed and approved by Ethics Committee of Defence Institute of Physiology and Allied Sciences (IEC/DIPAS/B2/1). The patients/participants provided their written informed consent to participate in this study.
Author Contributions
NK designed the experiments and wrote the manuscript with inputs from P. P and VS performed the experiments. RM, KR, UP, and NK collected samples at sea level and high altitude as well as recorded physiological parameters. P, RV, and NK analyzed the data. All authors contributed to the article and approved the submitted version.
Funding
This study was supported by Defence Research and Development Organisation (Project number DIP-263).
Conflict of Interest
The authors declare that the research was conducted in the absence of any commercial or financial relationships that could be construed as a potential conflict of interest.
Publisher's Note
All claims expressed in this article are solely those of the authors and do not necessarily represent those of their affiliated organizations, or those of the publisher, the editors and the reviewers. Any product that may be evaluated in this article, or claim that may be made by its manufacturer, is not guaranteed or endorsed by the publisher.
Acknowledgments
The authors acknowledge Defense Research and Development Organisation for funding the project and the Indian Army for providing logistic support for this study.
Supplementary Material
The Supplementary Material for this article can be found online at: https://www.frontiersin.org/articles/10.3389/fphys.2021.730601/full#supplementary-material
References
Adeva-Andany, M. M., Fernandez-Fernandez, C., Sanchez-Bello, R., Donapetry-Garcia, C., and Martinez-Rodriguez, J. (2015). The role of carbonic anhydrase in the pathogenesis of vascular calcification in humans. Atherosclerosis 241, 183–191. doi: 10.1016/j.atherosclerosis.2015.05.012
Ahmad, Y., Sharma, N. K., Garg, I., Ahmad, M. F., Sharma, M., and Bhargava, K. (2013). An insight into the changes in human plasma proteome on adaptation to hypobaric hypoxia. PLoS ONE 8:e67548. doi: 10.1371/journal.pone.0067548
Ahmad, Y., Shukla, D., Garg, I., Sharma, N. K., Saxena, S., Malhotra, V. K., et al. (2011). Identification of haptoglobin and apolipoprotein A-I as biomarkers for high altitude pulmonary edema. Funct. Integr. Genom. 11, 407–417. doi: 10.1007/s10142-011-0234-3
Anand, A. C., Jha, S. K., Saha, A., Sharma, V., and Adya, C. M. (2001). Thrombosis as a complication of extended stay at high altitude. Natl. Med. J. India 14, 197–201.
Barnholt, K. E., Hoffman, A. R., Rock, P. B., Muza, S. R., Fulco, C. S., Braun, B., et al. (2006). Endocrine responses to acute and chronic high-altitude exposure (4,300 meters): modulating effects of caloric restriction. Am. J. Physiol. Endocrinol. Metabol. 290, E1078–1088. doi: 10.1152/ajpendo.00449.2005
Bartsch, P., and Gibbs, J. S. (2007). Effect of altitude on the heart and the lungs. Circulation 116, 2191–2202. doi: 10.1161/CIRCULATIONAHA.106.650796
Basnyat, B. (2018). The potential usefulness of serum biomarkers in high-altitude medicine. J. Travel Med. 25, 1–2. doi: 10.1093/jtm/tay083
Bohuslavova, R., Kolar, F., Kuthanova, L., Neckar, J., Tichopad, A., and Pavlinkova, G. (2010). Gene expression profiling of sex differences in HIF1-dependent adaptive cardiac responses to chronic hypoxia. J. Appl. Physiol. 109, 1195–1202. doi: 10.1152/japplphysiol.00366.2010
Boos, C. J., Woods, D. R., Varias, A., Biscocho, S., Heseltine, P., and Mellor, A. J. (2016). High altitude and acute mountain sickness and changes in circulating Endothelin-1, Interleukin-6, and Interleukin-17a. High Alt. Med. Biol. 17, 25–31. doi: 10.1089/ham.2015.0098
Bruno, R. M., Cogo, A., Ghiadoni, L., Duo, E., Pomidori, L., Sharma, R., et al. (2014). Cardiovascular function in healthy Himalayan high-altitude dwellers. Atherosclerosis 236, 47–53. doi: 10.1016/j.atherosclerosis.2014.06.017
Calabro, P., Golia, E., and Yeh, E. T. (2009). CRP and the risk of atherosclerotic events. Semin. Immunopathol. 31, 79–94. doi: 10.1007/s00281-009-0149-4
Calbet, J. A., Boushel, R., Radegran, G., Sondergaard, H., Wagner, P. D., and Saltin, B. (2003). Why is VO2 max after altitude acclimatization still reduced despite normalization of arterial O2 content? Am. J. Physiol. Regul. Integr. Comp. Physiol. 284, R304–R316. doi: 10.1152/ajpregu.00156.2002
Dhar, P., Sharma, V. K., Das, S. K., Barhwal, K., Hota, S. K., and Singh, S. B. (2018). Differential responses of autonomic function in sea level residents, acclimatized lowlanders at >3500m and Himalayan high altitude natives at >3500m: a cross-sectional study. Respir. Physiol. Neurobiol. 254, 40–48. doi: 10.1016/j.resp.2018.04.002
Gallagher, S. A., and Hackett, P. H. (2004). High-altitude illness. Emerg. Med. Clin. North Am. 22, 329–355. doi: 10.1016/j.emc.2004.02.001
Gamboa, J., Caceda, R., Gamboa, A., and Monge, C. C. (2000). Carbonic anhydrase activity in the red blood cells of sea level and high altitude natives. Biol. Res. 33, 207–208. doi: 10.4067/S0716-97602000000300006
Gangwar, A., Pooja, Sharma, M., Singh, K., Patyal, A., Bhaumik, G., et al. (2019). Intermittent normobaric hypoxia facilitates high altitude acclimatization by curtailing hypoxia-induced inflammation and dyslipidemia. Pflugers Arch. 471, 949–959. doi: 10.1007/s00424-019-02273-4
Geyer, P. E., Kulak, N. A., Pichler, G., Holdt, L. M., Teupser, D., and Mann, M. (2016). Plasma proteome profiling to assess human health and disease. Cell Syst. 2, 185–195. doi: 10.1016/j.cels.2016.02.015
Gilmour, K. M. (2010). Perspectives on carbonic anhydrase. Comp. Biochem. Physiol. A. Mol. Integr. Physiol. 157, 193–197. doi: 10.1016/j.cbpa.2010.06.161
Gupta, N., and Ashraf, M. Z. (2012). Exposure to high altitude: a risk factor for venous thromboembolism? Semin. Thromb. Hemost. 38, 156–163. doi: 10.1055/s-0032-1301413
Hackett, P. H., and Roach, R. C. (2001). High-altitude illness. N. Engl. J. Med. 345, 107–114. doi: 10.1056/NEJM200107123450206
Hainsworth, R., Drinkhill, M. J., and Rivera-Chira, M. (2007). The autonomic nervous system at high altitude. Clin. Auton. Res. 17, 13–19. doi: 10.1007/s10286-006-0395-7
Hartmann, G., Tschop, M., Fischer, R., Bidlingmaier, C., Riepl, R., Tschop, K., et al. (2000). High altitude increases circulating interleukin-6, interleukin-1 receptor antagonist and C-reactive protein. Cytokine 12, 246–252. doi: 10.1006/cyto.1999.0533
Hester, J., Ventetuolo, C., and Lahm, T. (2019). Sex, gender, and sex hormones in pulmonary hypertension and right ventricular failure. Compr. Physiol. 10, 125–170. doi: 10.1002/cphy.c190011
Himadri, P., Kumari, S. S., Chitharanjan, M., and Dhananjay, S. (2010). Role of oxidative stress and inflammation in hypoxia-induced cerebral edema: a molecular approach. High Alt. Med. Biol. 11, 231–244. doi: 10.1089/ham.2009.1057
Hoff, E., Zou, D., Schiza, S., Demir, Y., Grote, L., Bouloukaki, I., et al. (2020). Carbonic anhydrase, obstructive sleep apnea and hypertension: effects of intervention. J. Sleep Res. 29:e12956. doi: 10.1111/jsr.12956
Horiuchi, M., Kirihara, Y., Fukuoka, Y., and Pontzer, H. (2019). Sex differences in respiratory and circulatory cost during hypoxic walking: potential impact on oxygen saturation. Sci. Rep. 9:9550. doi: 10.1038/s41598-019-44844-6
Hou, Y. P., Wu, J. L., Tan, C., Chen, Y., Guo, R., and Luo, Y. J. (2019). Sex-based differences in the prevalence of acute mountain sickness: a meta-analysis. Mil. Med. Res. 6:38. doi: 10.1186/s40779-019-0228-3
Houston, C. S., and Riley, R. L. (1947). Respiratory and circulatory changes during acclimatization to high altitude. Am. J. Physiol. 149, 565–588. doi: 10.1152/ajplegacy.1947.149.3.565
Hu, S. L., Xiong, W., Dai, Z. Q., Zhao, H. L., and Feng, H. (2016). Cognitive changes during prolonged stay at high altitude and its correlation with C-reactive protein. PLoS ONE 11:e0146290. doi: 10.1371/journal.pone.0146290
Hudalla, H., Michael, Z., Christodoulou, N., Willis, G. R., Fernandez-Gonzalez, A., Filatava, E. J., et al. (2019). Carbonic anhydrase inhibition ameliorates inflammation and experimental pulmonary hypertension. Am. J. Respir. Cell Mol. Biol. 61, 512–524. doi: 10.1165/rcmb.2018-0232OC
Ignjatovic, V., Geyer, P. E., Palaniappan, K. K., Chaaban, J. E., Omenn, G. S., Baker, M. S., et al. (2019). Mass spectrometry-based plasma proteomics: considerations from sample collection to achieving translational data. J. Proteome Res. 18, 4085–4097. doi: 10.1021/acs.jproteome.9b00503
Jha, S. K., Anand, A. C., Sharma, V., Kumar, N., and Adya, C. M. (2002). Stroke at high altitude: Indian experience. High Alt. Med. Biol. 3, 21–27. doi: 10.1089/152702902753639513
Julian, C. G., Subudhi, A. W., Hill, R. C., Wilson, M. J., Dimmen, A. C., Hansen, K. C., et al. (2014). Exploratory proteomic analysis of hypobaric hypoxia and acute mountain sickness in humans. J. Appl. Physiol. 116, 937–944. doi: 10.1152/japplphysiol.00362.2013
Khosla, S., Melton, L. J. 3rd, Atkinson, E. J., O'Fallon, W. M., Klee, G. G., and Riggs, B. L. (1998). Relationship of serum sex steroid levels and bone turnover markers with bone mineral density in men and women: a key role for bioavailable estrogen. J. Clin. Endocrinol. Metabol. 83, 2266–2274. doi: 10.1210/jc.83.7.2266
Kubo, K., Hanaoka, M., Hayano, T., Miyahara, T., Hachiya, T., Hayasaka, M., et al. (1998). Inflammatory cytokines in BAL fluid and pulmonary hemodynamics in high-altitude pulmonary edema. Respir. Physiol. 111, 301–310. doi: 10.1016/S0034-5687(98)00006-1
Levett, D. Z., Fernandez, B. O., Riley, H. L., Martin, D. S., Mitchell, K., Leckstrom, C. A., et al. (2011). The role of nitrogen oxides in human adaptation to hypoxia. Sci. Rep. 1:109. doi: 10.1038/srep00109
Levett, D. Z., Vigano, A., Capitanio, D., Vasso, M., De Palma, S., Moriggi, M., et al. (2015). Changes in muscle proteomics in the course of the Caudwell Research Expedition to Mt. Everest. Proteomics 15, 160–171. doi: 10.1002/pmic.201400306
Libby, P., and Ridker, P. M. (2004). Inflammation and atherosclerosis: role of C-reactive protein in risk assessment. Am. J. Med.116(Suppl. 6A), 9S−16S. doi: 10.1016/j.amjmed.2004.02.006
Lu, H., Wang, R., Li, W., Xie, H., Wang, C., Hao, Y., et al. (2018). Plasma proteomic study of acute mountain sickness susceptible and resistant individuals. Sci. Rep. 8:1265. doi: 10.1038/s41598-018-19818-9
Mallet, R. T., Burtscher, J., Richalet, J. P., Millet, G. P., and Burtscher, M. (2021). Impact of high altitude on cardiovascular health: current perspectives. Vasc. Health Risk Manag. 17, 317–335. doi: 10.2147/VHRM.S294121
Mandolesi, G., Avancini, G., Bartesaghi, M., Bernardi, E., Pomidori, L., and Cogo, A. (2014). Long-term monitoring of oxygen saturation at altitude can be useful in predicting the subsequent development of moderate-to-severe acute mountain sickness. Wilderness Environ. Med. 25, 384–391. doi: 10.1016/j.wem.2014.04.015
Mohanna, S., Baracco, R., and Seclen, S. (2006). Lipid profile, waist circumference, and body mass index in a high altitude population. High Alt. Med. Biol. 7, 245–255. doi: 10.1089/ham.2006.7.245
Moore, L. G. (2001). Human genetic adaptation to high altitude. High Alt. Med. Biol. 2, 257–279. doi: 10.1089/152702901750265341
Murray, A. J. (2016). Energy metabolism and the high-altitude environment. Exp. Physiol. 101, 23–27. doi: 10.1113/EP085317
Murray, A. J., Montgomery, H. E., Feelisch, M., Grocott, M. P. W., and Martin, D. S. (2018). Metabolic adjustment to high-altitude hypoxia: from genetic signals to physiological implications. Biochem. Soc. Trans. 46, 599–607. doi: 10.1042/BST20170502
Muza, S. R., Beidleman, B. A., and Fulco, C. S. (2010). Altitude preexposure recommendations for inducing acclimatization. High Alt. Med. Biol. 11:87–92. doi: 10.1089/ham.2010.1006
Naeije, R. (2010). Physiological adaptation of the cardiovascular system to high altitude. Prog. Cardiovasc. Dis. 52, 456–466. doi: 10.1016/j.pcad.2010.03.004
Natarajan, P., Kohli, P., Baber, U., Nguyen, K. H., Sartori, S., Reilly, D. F., et al. (2015). Association of APOC3 loss-of-function mutations with plasma lipids and subclinical atherosclerosis: the multi-ethnic bioimage study. J. Am. College Cardiol. 66, 2053–2055. doi: 10.1016/j.jacc.2015.08.866
Ndzie Noah, M. L., Adzika, G. K., Mprah, R., Adekunle, A. O., Adu-Amankwaah, J., and Sun, H. (2021). Sex-gender disparities in cardiovascular diseases: the effects of estrogen on eNOS, lipid profile, and NFATs during catecholamine stress. Front. Cardiovasc. Med. 8:639946. doi: 10.3389/fcvm.2021.639946
Nieto Estrada, V. H., Molano Franco, D., Medina, R. D., Gonzalez Garay, A. G., Marti-Carvajal, A. J., and Arevalo-Rodriguez, I. (2017). Interventions for preventing high altitude illness: Part 1. Commonly-used classes of drugs. Cochrane Database Syst. Rev. 6:CD009761. doi: 10.1002/14651858.CD009761.pub2
Padhy, G., Gangwar, A., Sharma, M., Himashree, G., Singh, K., Bhaumik, G., et al. (2016). Plasma kallikrein-bradykinin pathway promotes circulatory nitric oxide metabolite availability during hypoxia. Nitric Oxide 55–56, 36–44. doi: 10.1016/j.niox.2016.02.009
Palmer, B. F. (2010). Physiology and pathophysiology with ascent to altitude. Am. J. Med. Sci. 340, 69–77. doi: 10.1097/MAJ.0b013e3181d3cdbe
Parati, G., Agostoni, P., Basnyat, B., Bilo, G., Brugger, H., Coca, A., et al. (2018). Clinical recommendations for high altitude exposure of individuals with pre-existing cardiovascular conditions: a joint statement by the European Society of Cardiology, the Council on Hypertension of the European Society of Cardiology, the European Society of Hypertension, the International Society of Mountain Medicine, the Italian Society of Hypertension and the Italian Society of Mountain Medicine. Eur. Heart J. 39, 1546–1554. doi: 10.1093/eurheartj/ehx720
Pena, E., Brito, J., El Alam, S., and Siques, P. (2020a). oxidative stress, kinase activity and inflammatory implications in right ventricular hypertrophy and heart failure under hypobaric hypoxia. Int. J. Mol. Sci. 21:6421. doi: 10.3390/ijms21176421
Pena, E., Siques, P., Brito, J., Arribas, S. M., Boger, R., Hannemann, J., et al. (2020b). Nox2 upregulation and p38alpha MAPK activation in right ventricular hypertrophy of rats exposed to long-term chronic intermittent hypobaric hypoxia. Int. J. Mol. Sci. 21:8576. doi: 10.3390/ijms21228576
Pernemalm, M., and Lehtio, J. (2014). Mass spectrometry-based plasma proteomics: state of the art and future outlook. Expert Rev. Proteomics 11, 431–448. doi: 10.1586/14789450.2014.901157
Pham, K., Parikh, K., and Heinrich, E. C. (2021). Hypoxia and inflammation: insights from high-altitude physiology. Front. Physiol. 12:676782. doi: 10.3389/fphys.2021.676782
Pirillo, A., Norata, G. D., and Catapano, A. L. (2013). LOX-1, OxLDL, and atherosclerosis. Mediators Inflamm. 2013:152786. doi: 10.1155/2013/152786
Pollin, T. I., Damcott, C. M., Shen, H., Ott, S. H., Shelton, J., Horenstein, R. B., et al. (2008). A null mutation in human APOC3 confers a favorable plasma lipid profile and apparent cardioprotection. Science 322, 1702–1705. doi: 10.1126/science.1161524
Pooja, S. M., Singh, K., Himashree, G., Bhaumik, G., Kumar, B., et al. (2020). Estrogen receptor (ESR1 and ESR2)-mediated activation of eNOS-NO-cGMP pathway facilitates high altitude acclimatization. Nitric Oxide 102, 12–20. doi: 10.1016/j.niox.2020.05.003
Poznyak, A. V., Nikiforov, N. G., Markin, A. M., Kashirskikh, D. A., Myasoedova, V. A., Gerasimova, E. V., et al. (2020). Overview of OxLDL and its impact on cardiovascular health: focus on atherosclerosis. Front. Pharmacol. 11:613780. doi: 10.3389/fphar.2020.613780
Reiner, Z. (2017). Hypertriglyceridaemia and risk of coronary artery disease. Nat. Rev. Cardiol. 14, 401–411. doi: 10.1038/nrcardio.2017.31
Reynafarje, C., Lozano, R., and Valdivieso, J. (1959). The polycythemia of high altitudes: iron metabolism and related aspects. Blood 14, 433–455. doi: 10.1182/blood.V14.4.433.433
Riley, C. J., and Gavin, M. (2017). Physiological changes to the cardiovascular system at high altitude and its effects on cardiovascular disease. High Alt. Med. Biol. 18, 102–113. doi: 10.1089/ham.2016.0112
Rocha, N. A., East, C., Zhang, J., and McCullough, P. A. (2017). ApoCIII as a cardiovascular risk factor and modulation by the novel lipid-lowering agent volanesorsen. Curr. Atheroscler. Rep. 19:62. doi: 10.1007/s11883-017-0697-3
Santos, J. L., Perez-Bravo, F., Carrasco, E., Calvillan, M., and Albala, C. (2001). Low prevalence of type 2 diabetes despite a high average body mass index in the Aymara natives from Chile. Nutrition 17, 305–309. doi: 10.1016/S0899-9007(00)00551-7
Sarada, S., Himadri, P., Mishra, C., Geetali, P., Ram, M. S., and Ilavazhagan, G. (2008). Role of oxidative stress and NFkB in hypoxia-induced pulmonary edema. Exp. Biol. Med. 233, 1088–1098. doi: 10.3181/0712-RM-337
Shen, Y., Yang, Y. Q., Liu, C., Yang, J., Zhang, J. H., Jin, J., et al. (2020). Association between physiological responses after exercise at low altitude and acute mountain sickness upon ascent is sex-dependent. Mil. Med. Res. 7:53. doi: 10.1186/s40779-020-00283-3
Sherpa, L. Y., Deji, S. H., Chongsuvivatwong, V., Luobu, O., Thelle, D. S., et al. (2011). Lipid profile and its association with risk factors for coronary heart disease in the highlanders of Lhasa, Tibet. High Alt. Med. Biol. 12, 57–63. doi: 10.1089/ham.2010.1050
Siervo, M., Riley, H. L., Fernandez, B. O., Leckstrom, C. A., Martin, D. S., Mitchell, K., et al. (2014). Effects of prolonged exposure to hypobaric hypoxia on oxidative stress, inflammation and gluco-insular regulation: the not-so-sweet price for good regulation. PLoS ONE 9:e94915. doi: 10.1371/journal.pone.0094915
Siques, P., Brito, J., Naveas, N., Pulido, R., De la Cruz, J. J., Mamani, M., et al. (2014). Plasma and liver lipid profiles in rats exposed to chronic hypobaric hypoxia: changes in metabolic pathways. High Alt. Med. Biol. 15:388–395. doi: 10.1089/ham.2013.1134
Siques, P., Brito, J., Ordenes, S., and Pena, E. (2020). Involvement of overweight and lipid metabolism in the development of pulmonary hypertension under conditions of chronic intermittent hypoxia. Pulmonary Circ. 10, 42–49. doi: 10.1177/2045894020930626
Soria, R., Egger, M., Scherrer, U., Bender, N., and Rimoldi, S. F. (2016). Pulmonary artery pressure and arterial oxygen saturation in people living at high or low altitude: systematic review and meta-analysis. J. Appl. Physiol. 121, 1151–1159. doi: 10.1152/japplphysiol.00394.2016
Supuran, C. T. (2008). Carbonic anhydrases–an overview. Curr. Pharm. Des. 14, 603–614. doi: 10.2174/138161208783877884
Swenson, E. R. (2006). Carbonic anhydrase inhibitors and hypoxic pulmonary vasoconstriction. Respir. Physiol. Neurobiol. 151, 209–216. doi: 10.1016/j.resp.2005.10.011
Tang, X. G., Wen, J., Zhang, X. S., and Jiang, D. C. (2018). Association between decreased osteopontin and acute mountain sickness upon rapid ascent to 3500 m among young Chinese men. J. Travel Med. 25, 1–6. doi: 10.1093/jtm/tay075
Tg Hdl Working Group of the Exome Sequencing Project, N.H.L. Blood I. Crosby, J., Peloso, G. M., and Auer, P. L.. (2014). Loss-of-function mutations in APOC3, triglycerides, and coronary disease. N. Engl. J. Med. 371, 22–31. doi: 10.1056/NEJMoa1307095
Vats, P., Ray, K., Majumadar, D., Amitabh, J. D. A., Bayen, S., Akunov, A., et al. (2013). Changes in cardiovascular functions, lipid profile, and body composition at high altitude in two different ethnic groups. High Alt. Med. Biol. 14, 45–52. doi: 10.1089/ham.2012.1071
Virues-Ortega, J., Hogan, A. M., Baya-Botti, A., Kirkham, F. J., Baldeweg, T., Mahillo-Fernandez, I., et al. (2009). Survival and mortality in older adults living at high altitude in Bolivia: a preliminary report. J. Am. Geriatr. Soc. 57, 1955–1956. doi: 10.1111/j.1532-5415.2009.02468.x
Wang, Z., Liu, F., Ye, S., Jiang, P., Yu, X., Xu, J., et al. (2019). Plasma proteome profiling of high-altitude polycythemia using TMT-based quantitative proteomics approach. J. Proteomics 194, 60–69. doi: 10.1016/j.jprot.2018.12.031
Westerterp, M., Berbée, J. F. P., Pires, N. M. M., Mierlo, G. J. D., v, Kleemann, R., et al. (2007). Apolipoprotein C-I Is crucially involved in lipopolysaccharide-induced atherosclerosis development in apolipoprotein E-Knockout Mice. Circulation 116, 2173–2181. doi: 10.1161/CIRCULATIONAHA.107.693382
Yang, Y., Ma, L., Guan, W., Wang, Y., Du, Y., Ga, Q., et al. (2014). Differential plasma proteome analysis in patients with high-altitude pulmonary edema at the acute and recovery phases. Exp. Ther. Med. 7, 1160–1166. doi: 10.3892/etm.2014.1548
Yuan, L., Wang, M., Liu, T., Lei, Y., Miao, Q., Li, Q., et al. (2019). Carbonic anhydrase 1-mediated calcification is associated with atherosclerosis, and methazolamide alleviates its pathogenesis. Front. Pharmacol. 10:766. doi: 10.3389/fphar.2019.00766
Keywords: high altitude, hypobaric hypoxia, acclimatization, plasma proteomics, inflammation, dyslipidemia
Citation: Pooja, Sharma V, Meena RN, Ray K, Panjwani U, Varshney R and Kumar Sethy N (2021) TMT-Based Plasma Proteomics Reveals Dyslipidemia Among Lowlanders During Prolonged Stay at High Altitudes. Front. Physiol. 12:730601. doi: 10.3389/fphys.2021.730601
Received: 25 June 2021; Accepted: 02 September 2021;
Published: 15 October 2021.
Edited by:
Sandro Malacrida, Eurac Research, ItalyReviewed by:
Simona Mrakic-Sposta, Italian National Research Council, ItalyIngrid Eftedal, Norwegian University of Science and Technology, Norway
Copyright © 2021 Pooja, Sharma, Meena, Ray, Panjwani, Varshney and Kumar Sethy. This is an open-access article distributed under the terms of the Creative Commons Attribution License (CC BY). The use, distribution or reproduction in other forums is permitted, provided the original author(s) and the copyright owner(s) are credited and that the original publication in this journal is cited, in accordance with accepted academic practice. No use, distribution or reproduction is permitted which does not comply with these terms.
*Correspondence: Niroj Kumar Sethy, bmtzZXRoeSYjeDAwMDQwO2dtYWlsLmNvbQ==; bmlyb2omI3gwMDA0MDtkaXBhcy5kcmRvLmlu