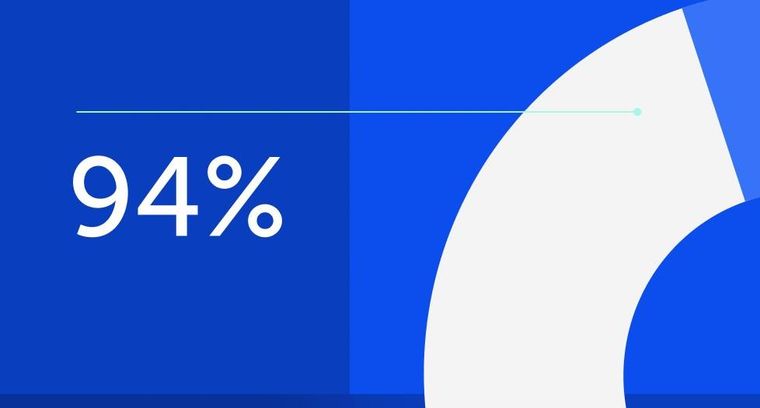
94% of researchers rate our articles as excellent or good
Learn more about the work of our research integrity team to safeguard the quality of each article we publish.
Find out more
REVIEW article
Front. Physiol., 08 October 2021
Sec. Gastrointestinal Sciences
Volume 12 - 2021 | https://doi.org/10.3389/fphys.2021.715852
This article is part of the Research TopicThe Microbiome in Hepatobiliary and Intestinal DiseaseView all 18 articles
Recent studies have identified the critical role of microbiota in the pathophysiology of autoimmune liver diseases (AILDs), including autoimmune hepatitis (AIH), primary biliary cholangitis (PBC), and primary sclerosing cholangitis (PSC). Metagenomic studies reveal significant decrease of gut bacterial diversity in AILDs. Although profiles of metagenomic vary widely, Veillonella is commonly enriched in AIH, PBC, and PSC. Apart from gut microbiome, the oral and bile microbiome seem to be associated with these diseases as well. The functional analysis of metagenomics suggests that metabolic pathways changed in the gut microbiome of the patients. Microbial metabolites, including short-chain fatty acids (SCFAs) and microbial bile acid metabolites, have been shown to modulate innate immunity, adaptive immunity, and inflammation. Taken together, the evidence of host–microbiome interactions and in-depth mechanistic studies needs further accumulation, which will offer more possibilities to clarify the mechanisms of AILDs and provide potential molecular targets for the prevention and treatment in the future.
Autoimmune liver diseases (AILDs) are chronic inflammatory conditions of the liver, including autoimmune hepatitis (AIH), primary biliary cholangitis (PBC), and primary sclerosing cholangitis (PSC) (Biewenga et al., 2020). Poor understanding of etiology makes the diagnosis and treatment of patients with AILDs challenging. In recent years, increasing studies have been focusing on microbiota-host interactions. Imbalanced microbial communities have been suggested to be related with aberrant immune response (Shi et al., 2017). Relationships have been established between the microbiome and autoimmune diseases, such as systemic lupus erythematosus (Katz-Agranov and Zandman-Goddard, 2017), inflammatory bowel disease (Franzosa et al., 2019), and rheumatoid arthritis (Bergot et al., 2019). Particularly, intestinal microbiome and liver could communicate through the biliary tract, portal vein, and systemic circulation, given the special anatomic and physiological relationships of liver and gut. Studies have discovered that liver diseases are intimately linked to the microbial communities of the human gut (Seki and Schnabl, 2012; Miyake and Yamamoto, 2013; LaRusso et al., 2017). Besides bacteria, the involvement of fungus and chlamydia has also been demonstrated in AILDs (Abdulkarim et al., 2004; Wang et al., 2017). Moreover, various metabolites of gut microbiome have been shown to participate in immune development and regulation (Levy et al., 2017).
Since the microbiota plays an important role in the development of the innate and adaptive immune system (Zheng et al., 2020), further study on the interaction between the diseases and microbiota may provide new insights on the etiology and management of AILDs. With the development of high throughput DNA sequencing, the diversity of the human microbiome has been greatly appreciated. The meta-omics approach, which consists of metagenomic, metatranscriptomic, and metabolomic analysis, has allowed for a more comprehensive characterization of the human microbiome (Zhang et al., 2019). Metagenome, a DNA sequencing method, aims to catalog all the genes from the samples (Wang et al., 2015). Metagenome reveals only the microbial composition of the community. The metatranscriptome could record expressed transcripts of the active members under a set of environmental conditions (Shakya et al., 2019). Changes in the composition or function of the gut microbiota lead to metabolite alterations. Through metabolomics, specific bacterial metabolic pathways and metabolites can be defined (Medina-Cleghorn and Nomura, 2014). Apparently, the microbiome is a rising star in the exploration for the prevention, diagnosis, and treatment of AILDs. Thus, we summarize a review of microbiome associated with AILDs from metagenomics and metabolomics, which may be the key for further understanding of the etiology and management of AILDs.
Metagenomics is a powerful tool that is helpful for the analysis of microbial heterogenicity. It mainly includes two sequencing strategies: amplicon sequencing, most often amplifying portions of the hypervariable regions of 16S rRNA; or shotgun sequencing, which sequences all given genomic DNA from a sample (Rausch et al., 2019). The shotgun metagenomics sequencing can achieve species-level and potentially strain-level of microorganisms (Walsh et al., 2018). In contrast, relative abundances of bacterial taxa derived from the general 16S rRNA is usually defined at the genus level (Walsh et al., 2018).
Studies of the microbiota often focus on the bacterial diversity in the feces of the patients. Collectively, alpha diversity of fecal microbial showed a downward trend in most AILDs patients, which was shown in Table 1 (Sabino et al., 2016; Tang et al., 2018; Wei et al., 2020). However, a German cohort study reported that the alpha-diversity of patients with PSC was similar to controls (Ruhlemann et al., 2019). The microbial communities of human gut were mainly composed of Firmicutes, Bacteroidetes, Actinobacteria, and Proteobacteria at the phylum level (Dekaboruah et al., 2020). Firmicutes and Proteobacteria were increased in both AIH and PBC, while Bacteroidetes showed no significant difference in AIH and PBC when compared to healthy control (HC) (Lv et al., 2020). Remarkably, PSC was characterized by abundant Bacteroidetes and Proteobacteria, whereas Firmicutes were underrepresented (Sabino et al., 2016; Ruhlemann et al., 2019). However, different results can be observed in salivary microbiome and bile microbiome. Statistically significant differences in the phylum level in Firmicutes, Bacteroidetes, and Proteobacteria of the salivary microbiota among the PBC, AIH, and HC groups were not detected (Abe et al., 2018), although the abundance of Bacteroidetes, Firmicutes, and Fusobacteria was also lower in bile microbiome of PSC patients. The abundance of Proteobacteria was higher when compared with patients from the control group (Tyc et al., 2020). These findings suggest that both gut and oral microbiome may be involved in AILDs pathogenesis.
Microbiome studies are relatively rare in AIH. We only found 6 related articles in PubMed, which are shown in Table 2. Wei et al. (2020) indicated increased abundance in Veillonella, Klebsiella, Streptococcus, and Lactobacillus in AIH compared to HC. Moreover, they created a model including Veillonella, Lactobacillus, Oscillospira, and Clostridiales to distinguish AIH from controls (Wei et al., 2020). Lachnospiraceae, Veillonella, Bacteroides, Roseburia, and Ruminococcaceae were selected as the AIH microbial biomarkers in another study (Lou et al., 2020). They also reported a higher relative abundance of Streptococcus in patients. In Africa, Elsherbiny et al. (2020) reported that Faecalibacterium, Blautia, Streptococcus, Haemophilus, Bacteroides, Veillonella, Eubacterium, Lachnospiraceae, and Butyricicoccus were enriched in AIH. Given together, most studies confirmed an overrepresentation of Veillonella in the gut microbiota of AIH patients. Microbes enriched in gut may aggravate the disease. However, no data support a causal relationship between Veillonella and AIH. The actual strains will need to be identified in future studies by shotgun metagenomic sequencing.
Probiotics were believed to restore the composition of the gut microbiome (Hemarajata and Versalovic, 2013). It can also participate in regulating the immune system (Liu et al., 2018). Bifidobacteria-based probiotics have been shown to confer health benefits on the host by regulating gut microbiota (O’Callaghan and van Sinderen, 2016). There is a depletion of Bifidobacterium in AIH (Lin et al., 2015; Lv et al., 2020). Furthermore, patients with lower Bifidobacterium failed to achieve remission (Lv et al., 2020). Zhang et al. (2020) reported that Bifidobacterium lactis 420 have beneficial functions in alleviating experimental autoimmune hepatitis. It suggested probiotics supplements may help to treat AIH in the future.
Except for gut microbiota, it is increasingly recognized that the oral cavity microbiota could also affect the host health (Arweiler and Netuschil, 2016). Dysbiosis of the oral microbiota has been found to be related to the pathogenesis of autoimmune diseases, such as inflammatory bowel diseases and systemic lupus erythematosus (van der Meulen et al., 2019; Elmaghrawy et al., 2020). There is a significant increase in Veillonella in the oral microbiota of AIH patients when compared with the HC, whereas Streptococcus is decreased (Abe et al., 2018). However, Veillonella is closely related to oral infectious diseases (Luo et al., 2020), which may influence the result. More research is needed. Notably, the change of Veillonella in oral bacterial community is consistent with that of fecal in AIH patients, although the relationship between the gut microbiota and the oral microbiota is still unknown. We can look forward to seeing that Veillonella strain may become a microbial marker in AIH.
Primary biliary cholangitis is a chronic occult disease which can progress to cirrhosis, and ultimately to liver failure and even death (Hirschfield et al., 2018). Lammert et al. (2021) found that fecal microbiota were related to the fibrosis and cirrhosis of PBC. Therefore, the analysis of microbiota composition in patients with PBC is meaningful. A study containing 60 UDCA treatment naive PBC patients and 80 healthy controls found 12 bacteria (Table 3) whose abundance changed in PBC compared with HC in China. Haemophilus, Veillonella, Clostridium, Lactobacillus, Streptococcus, Pseudomonas, Klebsiella, and an unknown genus in the family of Enterobacteriaceae were increased, and Bacteroidetes spp., Sutterella, Oscillospira, Faecalibacterium were decreased in PBC (Tang et al., 2018). These altered genera can help to discriminate PBC with HC (Tang et al., 2018). The relative abundance of Streptococcus was reported to be positively correlated with AST in alcoholic liver disease (Zhong et al., 2021). Regrettably, its role in PBC needs to be explored. Further, later study has reported that a decrease abundance of Faecalibacterium was associated with treatment non-responders, suggesting that Faecalibacterium may be beneficial for treatment response in patients with PBC (Furukawa et al., 2020). Another study from China also indicated more abundant genera including Haemophilus, Veillonella, Lactobacillus, Streptococcus, and Klebsiella in PBC (Lv et al., 2016). Besides Streptococcus and Lactobacillus, Bifidobacterium was proved to be more abundant in the PBC group compared with healthy control in Japanese (Furukawa et al., 2020). In addition, this study observed a significant reduction in the diversity of Clostridiales, which included amounts of butyric acid-producing symbiotic bacteria (Furukawa et al., 2020), although the findings above showed gut microbiota was strongly related to PBC. Evidence above also revealed that the change of microbial abundance is not just limited to one specie. This makes it more difficult to understand the potential mechanism between microbiome and disease. Present studies of metagenomics of gut microbiota are all from Asian patients and thus have certain limitations. In the future, more metagenomics studies of PBC are needed to identify the possible pathogens.
Gut microbiome is the hotspot in PBC research, whereas the investigation of microbiology at other body sites is also going on. As we all know, the biliary tract is traditionally considered sterile or has few bacteria. Previous study has reported that there are bacteria in PBC patients’ bile, mainly including Staphylococcus aureus, Enterococcus faecium, and Streptococcus pneumoniae (Hiramatsu et al., 2000). Additionally, it is worth noting that Propionibacterium acnes 16S rRNA gene has been detected in epithelioid granuloma of PBC livers, but less in adjacent hepatic parenchyma (Harada et al., 2001). Propionibacterium acnes has been suggested as a most likely infectious pathogen of sarcoidosis, a kind of autoimmune disease (Yamaguchi et al., 2021). This study indicated that Propionibacterium acnes may also play a role in PBC which required further investigation. Dysbiosis of the oral microbiome has also been defined in PBC. It was characterized by increased relative abundances in Eubacterium and Veillonella as well as decreased abundances in Fusobacterium (Abe et al., 2018). Above all, Veillonella is consistently overrepresented in both stool and saliva of patients with PBC, indicating that Veillonella is closely associated with PBC. Further studies are warranted to investigate how Veillonella interact with PBC.
The 16S rRNA gene analysis has also been a major method of bacterial analysis in PSC. Studies of the changes of microbiome in PSC were shown in Table 4. At present, there was only one study that has measured genetic diversity of fecal microbiota by shotgun metagenomic sequencing. It demonstrated the microbial gene richness reduced markedly in patients with PSC compared with HC (Kummen et al., 2020). Nine species showed an increased prevalence and 5 species were less prevalent in PSC compared to HC (Kummen et al., 2020). This study suggested that Veillonella atypica, Veillonella parvula, and an unclassified Veillonella species were more prevalent in PSC patients (Kummen et al., 2020). There is no study illustrating the effect of specific Veillonella strain in PSC. The relative abundance of Veillonella genera were also increased in children and teenagers with PSC (Cortez et al., 2020). Intriguingly, it has been proved that the abundance of Veillonella decreased after effective treatment of UDCA (Kummen and Hov, 2019). Evidence above suggested that the abundance of Veillonella was closely related to PSC, but it is not sufficient to distinguish PSC and controls (Ruhlemann et al., 2017). Then Ruhlemann et al. (2017) established a diagnosis model consisting of Veillonella, Clostridiales, Lachnospiraceae, and Coprococcus to help to differentiate PSC from HC.
To our knowledge, 70% of PSC patients have underlying inflammatory bowel disease (IBD) (Weismuller et al., 2017). Thus, it is necessary to distinguish the microbial profile of PSC with or without IBD. Published data suggested that the fecal microbiota of patients with PSC was significantly different from both HC and patients with IBD (Ruhlemann et al., 2019). At the genus level, Rothia, Lactobacillus, Streptococcus, and Veillonella were observed overrepresented specifically in PSC patients (Bajer et al., 2017). Coprobacillus, Escherichia, Corynebacterium, and Lactobacillus genera were related to PSC-IBD, but not PSC without IBD (Bajer et al., 2017). However, Rothia, Streptococcus, Enterococcus, Veillonella, Clostridium, and Haemophilus were more abundant in all subgroups of PSC (Bajer et al., 2017). Until now, studies have shown that all the treatments can’t change the natural history of PSC. Evaluating the difference of microbiota between PSC with or without IBD may help to find specific treatments for different subgroups of PSC. A randomized placebo-controlled crossover study including 14 PSC patients suggested that probiotics supplement didn’t alter the symptoms, liver biochemistry, or liver function in PSC (Vleggaar et al., 2008). However, evidence showed that fecal microbiota transplantation could increase bacterial diversity and was related with decreased alkaline phosphatase in patients with PSC (Allegretti et al., 2019).
Currently, composition of the bile microbiome in PSC is gradually emerging. In previous studies of bile microbiome in PSC, an over-representation of Enterococcus spp., Prevotella spp., Staphylococcus spp., Lawsonella spp., Veillonella dispar, and Cutibacterium was observed (Liwinski et al., 2020). Klebsiella spp. was also found in bile fluid of PSC patient (Liwinski et al., 2020). It has been reported that Klebsiella pneumoniae is associated with intestinal barrier dysfunction (Nakamoto et al., 2019). Nevertheless, the relationship between Klebsiella and PSC could not be confirmed in a cohort including 62 patients (Ruhlemann et al., 2019). On the contrary, Pereira et al. (2017) found similar bacterial communities of bile in non-PSC subjects and early stage PSC patients, which indicated that the initiation of PSC may not be associated with alteration in bile microbial communities.
The characteristic of oral microbiota has also been defined in PSC. Alpha-diversity of the salivary microbiome was not changed when comparing PSC with HC, but there is an overrepresentation of Streptococcus salivarius, Prevotella histicola, Rothia mucilaginosa, V. parvula, Actinomyces, Campylobacter concisus, Bifidobacterium stellenboschense, and Bacteroidales genus Phocaeicola (Lapidot et al., 2021). Furthermore, S. salivarius, V. parvula, Actinomyces, and Bifidobacterium were both significantly enriched in both the saliva and the fecal samples in patients with PSC compared with HC (Lapidot et al., 2021). Combining the analyses of fecal and oral microbiota studies may help to find out the specific bacterium which participates in the pathogenesis of disease.
As we all know, Helicobacter mainly colonizes in stomach. Helicobacter species may also partly participate in the pathogenesis of AILDs. In a previous study, Helicobacter species have been detected in livers from adults suffering from AIH, PSC, and PBC using PCR or DNA sequencing (Nilsson et al., 2000; Casswall et al., 2010). However, this study could not decide whether the Helicobacter specie play a pathogenetic role in AILDs because of the lack of healthy controls. Boomkens et al. (2005) in the Netherlands demonstrated that Helicobacter species do not play a causal role in the pathogenesis of the PBC and PSC, by comparing Helicobacter species-specific DNA in liver tissue of patients with PBC/PSC and a control group. But another study involving 25 patients with end-stage PSC and 31 controls suggests a contributory role of Helicobacter pylori in the pathogenesis of PSC (Krasinskas et al., 2007). H. pylori gene can be detected in liver tissue samples of patients with PBC (Krasinskas et al., 2007), whereas there is no correlation between H. pylori antibody and PBC (Durazzo et al., 2004). Cross-reaction can be observed between H. pylori and mitochondria antibody of the bile duct cells, although there is no evidence so far of a common epitope between H. pylori and bile duct cell (Bogdanos et al., 2004). In general, the correlation between H. pylori antibody and AILDs is still controversial.
In addition to bacteria, the role of chlamydia in AILDs has also been studied. Although Chlamydia pneumoniae specific 16S rRNA gene and antigen can be found in PBC liver (Leung et al., 2003). No difference of Chlamydia pneumoniae IgG was seen in PBC patients compared to post-hepatitis cirrhosis patients, suggesting that infection with chlamydia may not be the triggering agent of PBC (Liu et al., 2005). Recently, Lemoinne et al. (2020) found that the fungal microbiota of patients with PSC displayed an increased biodiversity. Moreover, their study suggested that PSC was associated with the increase of Exophiala genus and Sordariomycetes class, with a decrease of the Saccharomycetales order, Saccharomycetes class, Saccharomycetaceae family, and S. cerevisiae species (Lemoinne et al., 2020). Investigation of other microbiome such as chlamydia and fungi may provide a new direction for microbiome study in the future.
It is urgent to find related bacteria of AILDs, because of difficult diagnosis at the early stage in disease. Cumulative evidences show a linkage between microbiome and AILDs. The genera of Veillonella is predominant in the gut of AIH, PSC, and PBC patients, showing a vital role in AILDs. However, little is known about the potential mechanism of it in AILDs. The present result showed Veillonella may be enriched by suppression of bile acid synthesis (Loomba et al., 2021). Exploring the potential mechanism of microbiome in AILDs could be an opportunity for disease diagnosing and treating.
Exploring the response of microbiome may help to find the induction factor of AILDs. Metagenomics is limited to reveal the functional activities of microorganisms, while metatranscriptomics is applied to explore the rapid response and expressed biological signatures of microorganisms to the external stimuli (Franzosa et al., 2014; Ram-Mohan and Meyer, 2020). However, there is still no metatranscriptomics research in AILDs. By PICRUSt, a tool used to infer the functional profile of microbial community, the microbial function is shown in Figure 1. Elsherbiny et al. (2020) demonstrated that butyrate, tryptophan, branched-chain fatty acids, pantothenate, and coenzyme A metabolisms were improved in microbial communities of AIH. However, the metabolism associated with proline and arginine was reduced (Elsherbiny et al., 2020). Changes in the metabolites have been verified in metabolomics, which will be illustrated later. Furthermore, bacterial invasion of epithelial cells, peroxisome proliferator-activated receptors (PPAR) signaling pathway, and caprolactam degradation pathways were enriched in PBC (Tang et al., 2018). The selective destruction of biliary epithelial cells is the key step in the pathogenesis of PBC (Selmi et al., 2010). PPAR agonists is suggested to regulate bile acid pool, and reduce inflammation and fibrosis of liver (Gerussi et al., 2020). Intriguingly, UDCA use not only influences the relative abundance of microbial species in feces but also alters the metabolic pathways of microbiota. The metabolic pathway predicted by 16s rRNA sequencing data showed elevation in taurine and hypotaurine metabolism in PBC after UDCA treatment, whereas glycine metabolism pathway had no difference with that of UDCA-naive PBC (Chen et al., 2020). The change of bile acids in PBC has been confirmed by metabolomic study (Yang and Duan, 2016). As for PSC patients, evidence showed that inferred microbiome functions were significantly different between PSC and healthy controls. There were an increase of ‘biofilm formation by Escherichia coli,’ ‘lipopolysaccharide biosynthesis,’ ‘shigellosis,’ ‘Salmonella infection,’ ‘pathogenic E. coli infection’ and ‘bacterial invasion of epithelial cells,’ and a decrease of ‘tryptophan metabolism,’ ‘biosynthesis of amino acids’ in PSC (Liwinski et al., 2020). In addition, Kummen et al. (2020) demonstrated that patients with PSC had more metabolic pathways related to vitamin B6 synthesis and branched-chain amino acid synthesis compared to healthy controls. In short, pioneering work has indicated that metatranscriptomics had their functional potential in AILDs. Further study is needed in the field.
Figure 1. The changes of microbial function predicted by metagenomic in autoimmune liver diseases (AILDs).
Microbial metabolites are emerging to be important effectors mediating the impact of microbiota on host immune responses and are critical for host-microbiota interactions. The gut microbial metabolites contain a wide variety of molecules ranging from short-chain fatty acids (SCFAs) and vitamins to secondary bile acids and neurotransmitters (Kummen and Hov, 2019). Colonic microbiota can transform carbohydrates into SCFAs, including acetate, propionate, and butyrate (Topping and Clifton, 2001). SCFAs have been verified to participate in regulating both innate immunity and antigen-specific adaptive immunity (Kim, 2018). It cannot only suppress the proinflammatory activation of macrophage but also promote peripheral regulatory T-cell generation (Dohmen et al., 2002; Arpaia et al., 2013). Previous study indicated that SCFAs can be uptaken by liver (Cummings et al., 1987). Recently, Zhang et al. (2020) demonstrated that the fecal butyrate was decreased in AIH patients compared to HC. The cause of butyrate reduction in AIH is still unknown. Furukawa et al. (2020) demonstrated a significant reduction in the diversity of the order Clostridiales, which included butyric acid-producing bacteria. It may partly explain the reduction of butyrate. In addition, SFCAs supplementation has been proved to ameliorate experimental autoimmune hepatitis (Hu et al., 2018). Furthermore, Wu et al. (2017) have shown that butyrate could ameliorate experimental autoimmune hepatitis through maintaining the integrity of small intestine via inhibiting TLR4 signaling pathway. Altogether, SCFAs may have beneficial effects on liver health through various mechanisms.
Excessive intrahepatic accumulation of bile acids can aggravate liver injury in cholestatic diseases (Schmucker et al., 1990). Bile acids not only act as a stimulator of numerous inflammatory mediators but also induct mitochondrial reactive oxygen species, which may contribute to the progression of cholestatic liver diseases (Li et al., 2017). In addition, bile acids are crucial for the immune modulation and study has demonstrated bile acids metabolites play a pivotal role to regulate the balance of TH17 and Treg cells (Hang et al., 2019). Bile acid can regulate gut microbial composition and immune response (Ramirez-Perez et al., 2017). On the contrary, the microbiome also plays a central role in bile acid homeostasis. Primary bile acids are synthesized in the liver and metabolized into secondary bile acids by microbiota in the gut (Ridlon and Bajaj, 2015). Liwinski et al. (2020) detected decreased concentrations of most bile acids in bile of PSC patients, except for the secondary bile acid-taurolithocholic acid. Torres et al. (2018) also found PSC-IBD patients had a significant decrease in total stool bile acid pool compared to HC. Chen et al. (2020) identified decreased levels of lithocholic acid, glycodeoxycholic acid, and increased levels of cholic acid, taurochenodeoxycholic acid, chenodeoxycholic acid, and taurocholic acid in feces of PBC. UDCA, a kind of secondary bile acid, is just a small fraction of total bile acids. So far, it’s the main therapeutic drug for PBC, which has been proved to significantly improve the outcomes of patients with PBC (Santiago et al., 2018). The production of UDCA can be modified by intestinal bacteria in gut (Tonin and Arends, 2018). UDCA was absent in germ-free mice (Tabibian et al., 2016). Farnesoid X receptor, which plays a pivotal role in regulating liver inflammation and the extent of inflammatory responses, can be activated by bile acids (Ding et al., 2015). Oral vancomycin significantly reduced the concentration of secondary fecal bile acids, emphasizing the role of microbiota in transformation of primary bile acid (Vaughn et al., 2019). It’s worth noting that the abundance of Veillonella and Klebsiella have been proved to be negatively correlated with the level of secondary bile acids in serum (Chen et al., 2020).
As mentioned above, evidence showed the change of microbiota metabolomics was associated with AILDs. But there is much of the gut metabolome that remains uncharacterized. Untargeted metabolomics has great potential to identify novel molecules in AILDs in the future.
It’s widely recognized that genetic predisposition in combination with exposure to environmental triggers and immunity dysregulation play a vital role in the pathogenesis of AILDs (Arndtz and Hirschfield, 2016). We summarized the role of intestinal microbiota in AILDs pathogenesis as follows in Figure 2.
Several genome-wide association studies indicated that there is significant association between HLA DR3, DR4, and AIH (de Boer et al., 2014; van Gerven et al., 2015), HLA DRB1∗08, and PBC (Invernizzi et al., 2005). Moreover, early studies from Norway and the United Kingdom also identified HLA-DR3 (DRB1∗0301) as the susceptible gene of PSC (Schrumpf et al., 1982). HLA gene has been shown to affect the microbial composition of the late infant gut in a cohort study from southeast Sweden (Russell et al., 2019). The microbiome participates in regulating inflammatory and immune responses as trigger factors. However, the detailed relationship between HLA and microbiome in AILDs still needs further exploration.
To our knowledge, T lymphocyte plays a central role in the immunopathogenesis of AILDs (Ishibashi et al., 2003; Ichiki et al., 2005; Henriksen et al., 2017). Interestingly, Clostridium has been reported to regulate the induction of T regulatory cells by providing bacterial antigens and SCFAs (Atarashi et al., 2013). Bacterial metabolites SCFAs have been demonstrated to affect the activity of T regulatory cells and reduce the levels of pro-inflammatory cytokines such as IFN-γ, IL-6, IL-1b, MIP-2, and TNF-α (Felice et al., 2015; Bhaskaran et al., 2018). In addition, bile acid metabolites, which mainly regulated by microbiota, were reported to inhibit TH17 cells by binding to the key transcription factor retinoid-related orphan receptor-γ and regulate T regulatory cells through the production of mitochondrial reactive oxygen species (Hang et al., 2019).
It is currently believed that chronic bacterial infection might play a part in the initiation or development of the autoimmune status in patients with AILDs (Haruta et al., 2010). Many studies suggest the alteration of microbes in AILDs, but the causality of this relationship is unclear. In fact, additional intestinal barrier dysfunction has been proved to exacerbate liver injury in mice (Zhang et al., 2021). Bacteria and endotoxin would enter the systemic circulation and trigger immune response because of intestinal barrier dysfunction (Krentz and Allen, 2017). Recently, it has been reported that translocation of Enterococcus gallinarum to the liver in germ-free C57BL/6 mice could trigger autoimmune responses (Manfredo Vieira et al., 2018). Zhang et al. (2020) found that B. lactis 420 mitigated experimental autoimmune hepatitis through regulating intestinal barrier and liver immune cells (macrophage and Th17 cells). Specifically, the NOD.c3c4, which could spontaneously develop biliary inflammation, is a model of the human biliary disease primary biliary cirrhosis (Koarada et al., 2004). Biliary inflammation is ameliorated in antibiotic-treatment and germ-free NOD.c3c4 mice (Schrumpf et al., 2017). Nakamoto et al. (2019) demonstrated that gnotobiotic mice inoculated with PSC-derived microbiota was more susceptible to hepatobiliary injuries. Previous evidence shown that microbiome may be the promoting factors of AILDs. Antibacterial treatment may be effective options to suppress the development of the disease.
Molecular mimicry between immunodominant epitopes of the pathogen and self-peptides has been hypothesized to be the key event leading to the disease. It may provide clues to explain the relationship between microbes and disease. Investigations have detected that PDC-E2 of E. coli is molecularly similar to human PDC-E2, the immunodominant target of AMAs in PBC (Shimoda et al., 1995). Meanwhile, PDC-E2-like proteins of Novosphingobium aromaticivorans were more similar with human PDC-E2 than that of E. coli (Kaplan, 2004). Although N. aromaticivorans hasn’t been detected in the liver of patients with PBC (Tanaka et al., 1999), a study has reported that N. aromaticivorans is present in approximately 25% of fecal samples from patients and controls (Selmi et al., 2003). Lactobacillus delbrueckii and Mycobacterium gordonae might also induce loss of tolerance to human mitochondrial proteins in genetically susceptible individuals due to molecular mimicry and immunological cross-reactivity (Vilagut et al., 1994, 1997; Bogdanos et al., 2008). Recent findings also suggested that antibodies against Yersinia enterocolitica were present in PBC patients (Yamaguchi et al., 1994). Interestingly, Roesler et al. (2003) identified β-subunit of bacterial RNA-polymerase, a non-species-specific bacterial protein, as the target of antibodies in PBC. Besides bacterium, mycoplasma has also been suggested as a causative factor in the etiopathogenesis of PBC via ‘molecular mimicry’ (Berg et al., 2009). It is worth noting that PDC-E2 is highly conserved from prokaryotes to advanced organisms, which may be the reason why multiple microorganisms are related to AILDs. These results confirmed the hypothesis that autoimmunity in AILDs may be triggered by proteins specific for bacteria through ‘molecular mimicry,’ which provide a hopeful direction for further study on the pathogenesis of AILDs. In short, the relationship between the organisms and AILDs needs to be explored.
Increasing evidence has highlighted the crucial role of microbiome in AILDs. Research showed various changes of microbiome in metagenomic and metabolomic analyses. Although the studies so far didn’t clearly demonstrate the causation between microbiome and AILDs, they found the associated microbiome and even possible role in the pathogenesis providing direction for further study in the pathogenesis of AILDs. Moreover, mutual authentication among metagenomic and metabolomic of the microbiome could help us to understand the detailed and correct role of microbiota in AILDs. Nevertheless, the integrated analysis of gut microbiome and metabolite is lacking because of limited data of metagenomics and metabolomics in AILDs. With the application of metagenomics and metabolomics, it is possible to identify new microbial diagnostic markers in the early diagnosis and novel treatments of AILDs. The role of the microbiome, not only bacteria, in the mechanisms of AILDs needs more comprehensive and in-depth research to explore in the future.
BW and LZ guided the outline and carried out manuscript editing. YZ and YR collected data and drafted the manuscript. HZ performed manuscript review. Each of the co-authors has approved the final draft submitted. All authors contributed to the article and approved the submitted version.
This study was supported by grants (81860109 and 81470834) from the National Natural Science Foundation of China.
The authors declare that the research was conducted in the absence of any commercial or financial relationships that could be construed as a potential conflict of interest.
All claims expressed in this article are solely those of the authors and do not necessarily represent those of their affiliated organizations, or those of the publisher, the editors and the reviewers. Any product that may be evaluated in this article, or claim that may be made by its manufacturer, is not guaranteed or endorsed by the publisher.
We thank the National Natural Science Foundation of China for their financial support.
Abdulkarim, A. S., Petrovic, L. M., Kim, W. R., Angulo, P., Lloyd, R. V., and Lindor, K. D. (2004). Primary biliary cirrhosis: an infectious disease caused by Chlamydia pneumoniae? J. Hepatol. 40, 380–384. doi: 10.1016/j.jhep.2003.11.033
Abe, K., Takahashi, A., Fujita, M., Imaizumi, H., Hayashi, M., Okai, K., et al. (2018). Dysbiosis of oral microbiota and its association with salivary immunological biomarkers in autoimmune liver disease. PLoS One 13:e0198757. doi: 10.1371/journal.pone.0198757
Allegretti, J. R., Kassam, Z., Carrellas, M., Mullish, B. H., Marchesi, J. R., Pechlivanis, A., et al. (2019). Fecal microbiota transplantation in patients with primary sclerosing cholangitis: a pilot clinical trial. Am. J. Gastroenterol. 114, 1071–1079. doi: 10.14309/ajg.0000000000000115
Arndtz, K., and Hirschfield, G. M. (2016). The pathogenesis of autoimmune liver disease. Dig. Dis. 34, 327–333. doi: 10.1159/000444471
Arpaia, N., Campbell, C., Fan, X., Dikiy, S., van der Veeken, J., deRoos, P., et al. (2013). Metabolites produced by commensal bacteria promote peripheral regulatory T-cell generation. Nature 504, 451–455. doi: 10.1038/nature12726
Arweiler, N. B., and Netuschil, L. (2016). The oral microbiota. Adv. Exp. Med. Biol. 902, 45–60. doi: 10.1007/978-3-319-31248-4_4
Atarashi, K., Tanoue, T., Oshima, K., Suda, W., Nagano, Y., Nishikawa, H., et al. (2013). Treg induction by a rationally selected mixture of Clostridia strains from the human microbiota. Nature. 500, 232–236. doi: 10.1038/nature12331
Bajer, L., Kverka, M., Kostovcik, M., Macinga, P., Dvorak, J., Stehlikova, Z., et al. (2017). Distinct gut microbiota profiles in patients with primary sclerosing cholangitis and ulcerative colitis. World J. Gastroenterol. 23, 4548–4558. doi: 10.3748/wjg.v23.i25.4548
Berg, C. P., Kannan, T. R., Klein, R., Gregor, M., Baseman, J. B., Wesselborg, S., et al. (2009). Mycoplasma antigens as a possible trigger for the induction of antimitochondrial antibodies in primary biliary cirrhosis. Liver Int. 29, 797–809. doi: 10.1111/j.1478-3231.2008.01942.x
Bergot, A. S., Giri, R., and Thomas, R. (2019). The microbiome and rheumatoid arthritis. Best Pract. Res. Clin. Rheumatol. 33:101497. doi: 10.1016/j.berh.2020.101497
Bhaskaran, N., Quigley, C., Paw, C., Butala, S., Schneider, E., and Pandiyan, P. (2018). Role of short chain fatty acids in controlling tregs and immunopathology during mucosal infection. Front. Microbiol. 9:1995. doi: 10.3389/fmicb.2018.01995
Biewenga, M., Farina Sarasqueta, A., Tushuizen, M. E., de Jonge-Muller, E. S. M., van Hoek, B., and Trouw, L. A. (2020). The role of complement activation in autoimmune liver disease. Autoimmun. Rev. 19:102534. doi: 10.1016/j.autrev.2020.102534
Bogdanos, D. P., Baum, H., Grasso, A., Okamoto, M., Butler, P., Ma, Y., et al. (2004). Microbial mimics are major targets of crossreactivity with human pyruvate dehydrogenase in primary biliary cirrhosis. J. Hepatol. 40, 31–39. doi: 10.1016/s0168-8278(03)00501-4
Bogdanos, D., Pusl, T., Rust, C., Vergani, D., and Beuers, U. (2008). Primary biliary cirrhosis following Lactobacillus vaccination for recurrent vaginitis. J Hepatol. 49, 466–473. doi: 10.1016/j.jhep.2008.05.022
Boomkens, S. Y., de Rave, S., Pot, R. G., Egberink, H. F., Penning, L. C., Rothuizen, J., et al. (2005). The role of Helicobacter spp. in the pathogenesis of primary biliary cirrhosis and primary sclerosing cholangitis. FEMS Immunol. Med. Microbiol. 44, 221–225. doi: 10.1016/j.femsim.2004.11.002
Casswall, T. H., Nemeth, A., Nilsson, I., Wadstrom, T., and Nilsson, H. O. (2010). Helicobacter species DNA in liver and gastric tissues in children and adolescents with chronic liver disease. Scand. J. Gastroenterol. 45, 160–167. doi: 10.3109/00365520903426915
Chen, W., Wei, Y., Xiong, A., Li, Y., Guan, H., Wang, Q., et al. (2020). Comprehensive analysis of serum and fecal bile acid profiles and interaction with gut microbiota in primary biliary cholangitis. Clin. Rev. Allergy Immunol. 58, 25–38. doi: 10.1007/s12016-019-08731-2
Cortez, R. V., Moreira, L. N., Padilha, M., Bibas, M. D., Toma, R. K., Porta, G., et al. (2020). Gut microbiome of children and adolescents with primary sclerosing cholangitis in association with ulcerative colitis. Front. Immunol. 11:598152. doi: 10.3389/fimmu.2020.598152
Cummings, J. H., Pomare, E. W., Branch, W. J., Naylor, C. P., and Macfarlane, G. T. (1987). Short chain fatty acids in human large intestine, portal, hepatic and venous blood. Gut 28, 1221–1227. doi: 10.1136/gut.28.10.1221
de Boer, Y. S., van Gerven, N. M., Zwiers, A., Verwer, B. J., van Hoek, B., van Erpecum, K. J., et al. (2014). Genome-wide association study identifies variants associated with autoimmune hepatitis type 1. Gastroenterology 147, 443–52.e5. doi: 10.1053/j.gastro.2014.04.022
Dekaboruah, E., Suryavanshi, M. V., Chettri, D., and Verma, A. K. (2020). Human microbiome: an academic update on human body site specific surveillance and its possible role. Arch. Microbiol. 202, 2147–2167. doi: 10.1007/s00203-020-01931-x
Ding, L., Yang, L., Wang, Z., and Huang, W. (2015). Bile acid nuclear receptor FXR and digestive system diseases. Acta Pharm. Sin. B 5, 135–144. doi: 10.1016/j.apsb.2015.01.004
Dohmen, K., Shigematsu, H., Miyamoto, Y., Yamasaki, F., Irie, K., and Ishibashi, H. (2002). Atrophic corpus gastritis and Helicobacter pylori infection in primary biliary cirrhosis. Dig. Dis. Sci. 47, 162–169. doi: 10.1023/a:1013292210036
Durazzo, M., Rosina, F., Premoli, A., Morello, E., Fagoonee, S., Innarella, R., et al. (2004). Lack of association between seroprevalence of Helicobacter pylori infection and primary biliary cirrhosis. World J. Gastroenterol. 10, 3179–3181. doi: 10.3748/wjg.v10.i21.3179
Elmaghrawy, K., Hussey, S., and Moran, G. P. (2020). The oral microbiome in pediatric IBD: a source of pathobionts or biomarkers? Front Pediatr. 8:620254. doi: 10.3389/fped.2020.620254
Elsherbiny, N. M., Rammadan, M., Hassan, E. A., Ali, M. E., El-Rehim, A. S. A., Abbas, W. A., et al. (2020). Autoimmune hepatitis: shifts in gut microbiota and metabolic pathways among egyptian patients. Microorganisms 8:1011. doi: 10.3390/microorganisms8071011
Felice, C., Lewis, A., Armuzzi, A., Lindsay, J. O., and Silver, A. (2015). Review article: selective histone deacetylase isoforms as potential therapeutic targets in inflammatory bowel diseases. Aliment Pharmacol. Ther. 41, 26–38. doi: 10.1111/apt.13008
Franzosa, E. A., Morgan, X. C., Segata, N., Waldron, L., Reyes, J., Earl, A. M., et al. (2014). Relating the metatranscriptome and metagenome of the human gut. Proc. Natl. Acad. Sci. U.S.A. 111, E2329–E2338. doi: 10.1073/pnas.1319284111
Franzosa, E. A., Sirota-Madi, A., Avila-Pacheco, J., Fornelos, N., Haiser, H. J., Reinker, S., et al. (2019). Gut microbiome structure and metabolic activity in inflammatory bowel disease. Nat. Microbiol. 4, 293–305. doi: 10.1038/s41564-018-0306-4
Furukawa, M., Moriya, K., Nakayama, J., Inoue, T., Momoda, R., Kawaratani, H., et al. (2020). Gut dysbiosis associated with clinical prognosis of patients with primary biliary cholangitis. Hepatol. Res. 50, 840–852. doi: 10.1111/hepr.13509
Gerussi, A., Luca, M., Cristoferi, L., Ronca, V., Mancuso, C., Milani, C., et al. (2020). New therapeutic targets in autoimmune cholangiopathies. Front. Med. (Lausanne) 7:117. doi: 10.3389/fmed.2020.00117
Hang, S., Paik, D., Yao, L., Kim, E., Trinath, J., Lu, J., et al. (2019). Bile acid metabolites control TH17 and Treg cell differentiation. Nature 576, 143–148. doi: 10.1038/s41586-019-1785-z
Harada, K., Tsuneyama, K., Sudo, Y., Masuda, S., and Nakanuma, Y. (2001). Molecular identification of bacterial 16S ribosomal RNA gene in liver tissue of primary biliary cirrhosis: is Propionibacterium acnes involved in granuloma formation? Hepatology 33, 530–536. doi: 10.1053/jhep.2001.22653
Haruta, I., Kikuchi, K., Hashimoto, E., Nakamura, M., Miyakawa, H., Hirota, K., et al. (2010). Long-term bacterial exposure can trigger nonsuppurative destructive cholangitis associated with multifocal epithelial inflammation. Lab. Invest. 90, 577–588. doi: 10.1038/labinvest.2010.40
Hemarajata, P., and Versalovic, J. (2013). Effects of probiotics on gut microbiota: mechanisms of intestinal immunomodulation and neuromodulation. Therap. Adv. Gastroenterol. 6, 39–51. doi: 10.1177/1756283X12459294
Henriksen, E. K., Jorgensen, K. K., Kaveh, F., Holm, K., Hamm, D., Olweus, J., et al. (2017). Gut and liver T-cells of common clonal origin in primary sclerosing cholangitis-inflammatory bowel disease. J. Hepatol. 66, 116–122. doi: 10.1016/j.jhep.2016.09.002
Hiramatsu, K., Harada, K., Tsuneyama, K., Sasaki, M., Fujita, S., Hashimoto, T., et al. (2000). Amplification and sequence analysis of partial bacterial 16S ribosomal RNA gene in gallbladder bile from patients with primary biliary cirrhosis. J. Hepatol. 33, 9–18. doi: 10.1016/s0168-8278(00)80153-1
Hirschfield, G. M., Dyson, J. K., Alexander, G. J. M., Chapman, M. H., Collier, J., Hubscher, S., et al. (2018). The British Society of Gastroenterology/UK-PBC primary biliary cholangitis treatment and management guidelines. Gut 67, 1568–1594. doi: 10.1136/gutjnl-2017-315259
Hu, E. D., Chen, D. Z., Wu, J. L., Lu, F. B., Chen, L., Zheng, M. H., et al. (2018). High fiber dietary and sodium butyrate attenuate experimental autoimmune hepatitis through regulation of immune regulatory cells and intestinal barrier. Cell. Immunol. 328, 24–32. doi: 10.1016/j.cellimm.2018.03.003
Ichiki, Y., Aoki, C. A., Bowlus, C. L., Shimoda, S., Ishibashi, H., and Gershwin, M. E. (2005). T cell immunity in autoimmune hepatitis. Autoimmun. Rev. 4, 315–321. doi: 10.1016/j.autrev.2005.01.005
Invernizzi, P., Selmi, C., Mackay, I. R., Podda, M., and Gershwin, M. E. (2005). From bases to basis: linking genetics to causation in primary biliary cirrhosis. Clin. Gastroenterol. Hepatol. 3, 401–410. doi: 10.1016/s1542-3565(04)00678-0
Ishibashi, H., Nakamura, M., Shimoda, S., and Gershwin, M. E. (2003). T cell immunity and primary biliary cirrhosis. Autoimmun. Rev. 2, 19–24. doi: 10.1016/s1568-9972(02)00122-2
Kaplan, M. M. (2004). Novosphingobium aromaticivorans: a potential initiator of primary biliary cirrhosis. Am. J. Gastroenterol. 99, 2147–2149. doi: 10.1111/j.1572-0241.2004.41121.x
Katz-Agranov, N., and Zandman-Goddard, G. (2017). The microbiome and systemic lupus erythematosus. Immunol. Res. 65, 432–437. doi: 10.1007/s12026-017-8906-2
Kim, C. H. (2018). Immune regulation by microbiome metabolites. Immunology 154, 220–229. doi: 10.1111/imm.12930
Koarada, S., Wu, Y., Fertig, N., Sass, D. A., Nalesnik, M., Todd, J. A., et al. (2004). Genetic control of autoimmunity: protection from diabetes, but spontaneous autoimmune biliary disease in a nonobese diabetic congenic strain. J. Immunol. 173, 2315–2323. doi: 10.4049/jimmunol.173.4.2315
Krasinskas, A. M., Yao, Y., Randhawa, P., Dore, M. P., and Sepulveda, A. R. (2007). Helicobacter pylori may play a contributory role in the pathogenesis of primary sclerosing cholangitis. Dig. Dis. Sci. 52, 2265–2270. doi: 10.1007/s10620-007-9803-7
Krentz, T., and Allen, S. (2017). Bacterial translocation in critical illness. J. Small Anim. Pract. 58, 191–198. doi: 10.1111/jsap.12626
Kummen, M., and Hov, J. R. (2019). The gut microbial influence on cholestatic liver disease. Liver Int. 39, 1186–1196. doi: 10.1111/liv.14153
Kummen, M., Thingholm, L. B., Ruhlemann, M. C., Holm, K., Hansen, S. H., Moitinho-Silva, L., et al. (2020). Altered gut microbial metabolism of essential nutrients in primary sclerosing cholangitis. Gastroenterology 160, 1784.e–1798.e0. doi: 10.1053/j.gastro.2020.12.058
Lammert, C., Shin, A., Xu, H., Hemmerich, C., O’ Connell, T. M., and Chalasani, N. (2021). Short-chain fatty acid and fecal microbiota profiles are linked to fibrosis in primary biliary cholangitis. FEMS Microbiol. Lett. 368:fnab038. doi: 10.1093/femsle/fnab038
Lapidot, Y., Amir, A., Ben-Simon, S., Veitsman, E., Cohen-Ezra, O., Davidov, Y., et al. (2021). Alterations of the salivary and fecal microbiome in patients with primary sclerosing cholangitis. Hepatol. Int. 15, 191–201. doi: 10.1007/s12072-020-10089-z
LaRusso, N. F., Tabibian, J. H., and O’Hara, S. P. (2017). Role of the intestinal microbiome in cholestatic liver disease. Dig. Dis. 35, 166–168. doi: 10.1159/000450906
Lemoinne, S., Kemgang, A., Ben Belkacem, K., Straube, M., Jegou, S., Corpechot, C., et al. (2020). Fungi participate in the dysbiosis of gut microbiota in patients with primary sclerosing cholangitis. Gut 69, 92–102. doi: 10.1136/gutjnl-2018-317791
Leung, P. S., Park, O., Matsumura, S., Ansari, A. A., Coppel, R. L., and Gershwin, M. E. (2003). Is there a relation between Chlamydia infection and primary biliary cirrhosis? Clin. Dev. Immunol. 10, 227–233. doi: 10.1080/10446670310001642429
Levy, M., Blacher, E., and Elinav, E. (2017). Microbiome, metabolites and host immunity. Curr. Opin. Microbiol. 35, 8–15. doi: 10.1016/j.mib.2016.10.003
Li, Y., Tang, R., Leung, P. S. C., Gershwin, M. E., and Ma, X. (2017). Bile acids and intestinal microbiota in autoimmune cholestatic liver diseases. Autoimmun. Rev. 16, 885–896. doi: 10.1016/j.autrev.2017.07.002
Lin, R., Zhou, L., Zhang, J., and Wang, B. (2015). Abnormal intestinal permeability and microbiota in patients with autoimmune hepatitis. Int. J. Clin. Exp. Pathol. 8, 5153–5160.
Liu, H. Y., Deng, A. M., Zhang, J., Zhou, Y., Yao, D. K., Tu, X. Q., et al. (2005). Correlation of Chlamydia pneumoniae infection with primary biliary cirrhosis. World J Gastroenterol. 11, 4108–4110. doi: 10.3748/wjg.v11.i26.4108
Liu, Y., Alookaran, J. J., and Rhoads, J. M. (2018). Probiotics in autoimmune and inflammatory disorders. Nutrients 10:1537. doi: 10.3390/nu10101537
Liwinski, T., Zenouzi, R., John, C., Ehlken, H., Ruhlemann, M. C., Bang, C., et al. (2020). Alterations of the bile microbiome in primary sclerosing cholangitis. Gut 69, 665–672. doi: 10.1136/gutjnl-2019-318416
Loomba, R., Ling, L., Dinh, D. M., DePaoli, A. M., Lieu, H. D., Harrison, S. A., et al. (2021). The commensal microbe veillonella as a marker for response to an FGF19 analog in NASH. Hepatology 73, 126–143. doi: 10.1002/hep.31523
Lou, J., Jiang, Y., Rao, B., Li, A., Ding, S., Yan, H., et al. (2020). Fecal microbiomes distinguish patients with autoimmune hepatitis from healthy individuals. Front. Cell Infect. Microbiol. 10:342. doi: 10.3389/fcimb.2020.00342
Luo, Y. X., Sun, M. L., Shi, P. L., Liu, P., Chen, Y. Y., and Peng, X. (2020). [Research progress in the relationship between Veillonella and oral diseases]. Hua Xi Kou Qiang Yi Xue Za Zhi 38, 576–582. doi: 10.7518/hxkq.2020.05.018
Lv, L. X., Fang, D. Q., Shi, D., Chen, D. Y., Yan, R., Zhu, Y. X., et al. (2016). Alterations and correlations of the gut microbiome, metabolism and immunity in patients with primary biliary cirrhosis. Environ. Microbiol. 18, 2272–2286. doi: 10.1111/1462-2920.13401
Lv, T., Casar, C., Ruehlemann, M. C., Bang, C., Sebode, M., Hohenester, S., et al. (2020). A disease-specific decline of the relative abundance of Bifidobacterium in patients with autoimmune hepatitis. Aliment Pharmacol. Ther. 51, 1417–1428. doi: 10.1111/apt.15754
Manfredo Vieira, S., Hiltensperger, M., Kumar, V., Zegarra-Ruiz, D., Dehner, C., Khan, N., et al. (2018). Translocation of a gut pathobiont drives autoimmunity in mice and humans. Science 359, 1156–1161. doi: 10.1126/science.aar7201
Medina-Cleghorn, D., and Nomura, D. K. (2014). Exploring metabolic pathways and regulation through functional chemoproteomic and metabolomic platforms. Chem. Biol. 21, 1171–1184. doi: 10.1016/j.chembiol.2014.07.007
Miyake, Y., and Yamamoto, K. (2013). Role of gut microbiota in liver diseases. Hepatol. Res. 43, 139–146. doi: 10.1111/j.1872-034X.2012.01088.x
Nakamoto, N., Sasaki, N., Aoki, R., Miyamoto, K., Suda, W., Teratani, T., et al. (2019). Gut pathobionts underlie intestinal barrier dysfunction and liver T helper 17 cell immune response in primary sclerosing cholangitis. Nat. Microbiol. 4, 492–503. doi: 10.1038/s41564-018-0333-1
Nilsson, H. O., Taneera, J., Castedal, M., Glatz, E., Olsson, R., and Wadstrom, T. (2000). Identification of Helicobacter pylori and other Helicobacter species by PCR, hybridization, and partial DNA sequencing in human liver samples from patients with primary sclerosing cholangitis or primary biliary cirrhosis. J. Clin. Microbiol. 38, 1072–1076. doi: 10.1128/JCM.38.3.1072-1076.2000
O’Callaghan, A., and van Sinderen, D. (2016). Bifidobacteria and their role as members of the human gut microbiota. Front. Microbiol. 7:925. doi: 10.3389/fmicb.2016.00925
Pereira, P., Aho, V., Arola, J., Boyd, S., Jokelainen, K., Paulin, L., et al. (2017). Bile microbiota in primary sclerosing cholangitis: Impact on disease progression and development of biliary dysplasia. PLoS One 12:e0182924. doi: 10.1371/journal.pone.0182924
Ramirez-Perez, O., Cruz-Ramon, V., Chinchilla-Lopez, P., and Mendez-Sanchez, N. (2017). The role of the gut microbiota in bile acid metabolism. Ann. Hepatol. 16(Suppl. 1: s3-105.), s15–s20. doi: 10.5604/01.3001.0010.5494
Ram-Mohan, N., and Meyer, M. M. (2020). Comparative metatranscriptomics of periodontitis supports a common polymicrobial shift in metabolic function and identifies novel putative disease-associated ncRNAs. Front. Microbiol. 11:482. doi: 10.3389/fmicb.2020.00482
Rausch, P., Ruhlemann, M., Hermes, B. M., Doms, S., Dagan, T., Dierking, K., et al. (2019). Comparative analysis of amplicon and metagenomic sequencing methods reveals key features in the evolution of animal metaorganisms. Microbiome 7:133. doi: 10.1186/s40168-019-0743-1
Ridlon, J. M., and Bajaj, J. S. (2015). The human gut sterolbiome: bile acid-microbiome endocrine aspects and therapeutics. Acta Pharm. Sin. B 5, 99–105. doi: 10.1016/j.apsb.2015.01.006
Roesler, K. W., Schmider, W., Kist, M., Batsford, S., Schiltz, E., Oelke, M., et al. (2003). Identification of beta-subunit of bacterial RNA-polymerase–a non-species-specific bacterial protein–as target of antibodies in primary biliary cirrhosis. Dig. Dis. Sci. 48, 561–569. doi: 10.1023/a:1022501102877
Ruhlemann, M. C., Heinsen, F. A., Zenouzi, R., Lieb, W., Franke, A., and Schramm, C. (2017). Faecal microbiota profiles as diagnostic biomarkers in primary sclerosing cholangitis. Gut 66, 753–754. doi: 10.1136/gutjnl-2016-312180
Ruhlemann, M., Liwinski, T., Heinsen, F. A., Bang, C., Zenouzi, R., Kummen, M., et al. (2019). Consistent alterations in faecal microbiomes of patients with primary sclerosing cholangitis independent of associated colitis. Aliment Pharmacol. Ther. 50, 580–589. doi: 10.1111/apt.15375
Russell, J. T., Roesch, L. F. W., Ordberg, M., Ilonen, J., Atkinson, M. A., Schatz, D. A., et al. (2019). Genetic risk for autoimmunity is associated with distinct changes in the human gut microbiome. Nat. Commun. 10:3621. doi: 10.1038/s41467-019-11460-x
Sabino, J., Vieira-Silva, S., Machiels, K., Joossens, M., Falony, G., Ballet, V., et al. (2016). Primary sclerosing cholangitis is characterised by intestinal dysbiosis independent from IBD. Gut 65, 1681–1689. doi: 10.1136/gutjnl-2015-311004
Santiago, P., Scheinberg, A. R., and Levy, C. (2018). Cholestatic liver diseases: new targets, new therapies. Therap. Adv. Gastroenterol. 11:1756284818787400. doi: 10.1177/1756284818787400
Schmucker, D. L., Ohta, M., Kanai, S., Sato, Y., and Kitani, K. (1990). Hepatic injury induced by bile salts: correlation between biochemical and morphological events. Hepatology 12, 1216–1221. doi: 10.1002/hep.1840120523
Schrumpf, E., Fausa, O., Forre, O., Dobloug, J. H., Ritland, S., and Thorsby, E. (1982). HLA antigens and immunoregulatory T cells in ulcerative colitis associated with hepatobiliary disease. Scand. J. Gastroenterol. 17, 187–191. doi: 10.3109/00365528209182038
Schrumpf, E., Kummen, M., Valestrand, L., Greiner, T. U., Holm, K., Arulampalam, V., et al. (2017). The gut microbiota contributes to a mouse model of spontaneous bile duct inflammation. J. Hepatol. 66, 382–389. doi: 10.1016/j.jhep.2016.09.020
Seki, E., and Schnabl, B. (2012). Role of innate immunity and the microbiota in liver fibrosis: crosstalk between the liver and gut. J. Physiol. 590, 447–458. doi: 10.1113/jphysiol.2011.219691
Selmi, C., Balkwill, D. L., Invernizzi, P., Ansari, A. A., Coppel, R. L., Podda, M., et al. (2003). Patients with primary biliary cirrhosis react against a ubiquitous xenobiotic-metabolizing bacterium. Hepatology 38, 1250–1257. doi: 10.1053/jhep.2003.50446
Selmi, C., Meda, F., Kasangian, A., Invernizzi, P., Tian, Z., Lian, Z., et al. (2010). Experimental evidence on the immunopathogenesis of primary biliary cirrhosis. Cell Mol. Immunol. 7, 1–10. doi: 10.1038/cmi.2009.104
Shakya, M., Lo, C. C., and Chain, P. S. G. (2019). Advances and challenges in metatranscriptomic analysis. Front. Genet. 10:904. doi: 10.3389/fgene.2019.00904
Shi, N., Li, N., Duan, X., and Niu, H. (2017). Interaction between the gut microbiome and mucosal immune system. Mil Med Res. 4:14. doi: 10.1186/s40779-017-0122-9
Shimoda, S., Nakamura, M., Ishibashi, H., Hayashida, K., and Niho, Y. H. L. A. (1995). DRB4 0101-restricted immunodominant T cell autoepitope of pyruvate dehydrogenase complex in primary biliary cirrhosis: evidence of molecular mimicry in human autoimmune diseases. J. Exp. Med. 181, 1835–1845. doi: 10.1084/jem.181.5.1835
Tabibian, J. H., O’Hara, S. P., Trussoni, C. E., Tietz, P. S., Splinter, P. L., Mounajjed, T., et al. (2016). Absence of the intestinal microbiota exacerbates hepatobiliary disease in a murine model of primary sclerosing cholangitis. Hepatology 63, 185–196. doi: 10.1002/hep.27927
Tanaka, A., Prindiville, T. P., Gish, R., Solnick, J. V., Coppel, R. L., Keeffe, E. B., et al. (1999). Are infectious agents involved in primary biliary cirrhosis? APCR approach. J. Hepatol. 31, 664–671. doi: 10.1016/s0168-8278(99)80346-8
Tang, R., Wei, Y., Li, Y., Chen, W., Chen, H., Wang, Q., et al. (2018). Gut microbial profile is altered in primary biliary cholangitis and partially restored after UDCA therapy. Gut 67, 534–541. doi: 10.1136/gutjnl-2016-313332
Tonin, F., and Arends, I. W. C. E. (2018). Latest development in the synthesis of ursodeoxycholic acid (UDCA): a critical review. Beilstein J. Org. Chem. 14, 470–483. doi: 10.3762/bjoc.14.33
Topping, D. L., and Clifton, P. M. (2001). Short-chain fatty acids and human colonic function: roles of resistant starch and nonstarch polysaccharides. Physiol. Rev. 81, 1031–1064. doi: 10.1152/physrev.2001.81.3.1031
Torres, J., Palmela, C., Brito, H., Bao, X., Ruiqi, H., Moura-Santos, P., et al. (2018). The gut microbiota, bile acids and their correlation in primary sclerosing cholangitis associated with inflammatory bowel disease. U. Eur. Gastroenterol. J. 6, 112–122. doi: 10.1177/2050640617708953
Tyc, O., Jansen, C., Schierwagen, R., Uschner, F. E., Israelsen, M., Klein, S., et al. (2020). Variation in bile microbiome by the etiology of cholestatic liver disease. Liver Transpl. 26, 1652–1657. doi: 10.1002/lt.25882
van der Meulen, T. A., Harmsen, H. J. M., Vila, A. V., Kurilshikov, A., Liefers, S. C., Zhernakova, A., et al. (2019). Shared gut, but distinct oral microbiota composition in primary Sjogren’s syndrome and systemic lupus erythematosus. J. Autoimmun. 97, 77–87. doi: 10.1016/j.jaut.2018.10.009
van Gerven, N. M., de Boer, Y. S., Zwiers, A., Verwer, B. J., Drenth, J. P., van Hoek, B., et al. (2015). HLA-DRB1∗03:01 and HLA-DRB1∗04:01 modify the presentation and outcome in autoimmune hepatitis type-1. Genes Immun. 16, 247–252. doi: 10.1038/gene.2014.82
Vaughn, B. P., Kaiser, T., Staley, C., Hamilton, M. J., Reich, J., Graiziger, C., et al. (2019). A pilot study of fecal bile acid and microbiota profiles in inflammatory bowel disease and primary sclerosing cholangitis. Clin. Exp. Gastroenterol. 12, 9–19. doi: 10.2147/CEG.S186097
Vilagut, L., Pares, A., Vinas, O., Vila, J., Jimenez de Anta, M. T., and Rodes, J. (1997). Antibodies to mycobacterial 65-kD heat shock protein cross-react with the main mitochondrial antigens in patients with primary biliary cirrhosis. Eur. J. Clin. Invest. 27, 667–672. doi: 10.1046/j.1365-2362.1997.1690724.x
Vilagut, L., Vila, J., Vinas, O., Pares, A., Gines, A., Jimenez de Anta, M. T., et al. (1994). Cross-reactivity of anti-Mycobacterium gordonae antibodies with the major mitochondrial autoantigens in primary biliary cirrhosis. J. Hepatol. 21, 673–677. doi: 10.1016/s0168-8278(94)80117-7
Vleggaar, F. P., Monkelbaan, J. F., and van Erpecum, K. J. (2008). Probiotics in primary sclerosing cholangitis: a randomized placebo-controlled crossover pilot study. Eur. J. Gastroenterol. Hepatol. 20, 688–692. doi: 10.1097/MEG.0b013e3282f5197e
Walsh, A. M., Crispie, F., O’Sullivan, O., Finnegan, L., Claesson, M. J., and Cotter, P. D. (2018). Species classifier choice is a key consideration when analysing low-complexity food microbiome data. Microbiome 6:50. doi: 10.1186/s40168-018-0437-0
Wang, W. L., Xu, S. Y., Ren, Z. G., Tao, L., Jiang, J. W., and Zheng, S. S. (2015). Application of metagenomics in the human gut microbiome. World J. Gastroenterol. 21, 803–814. doi: 10.3748/wjg.v21.i3.803
Wang, Y., Zhao, Z., Lu, H., Zhang, J., and Huang, F. (2017). Fungal infection involvement in primary biliary cirrhosis: a review of 2 cases. Exp. Ther. Med. 13, 489–494. doi: 10.3892/etm.2016.3977
Wei, Y., Li, Y., Yan, L., Sun, C., Miao, Q., Wang, Q., et al. (2020). Alterations of gut microbiome in autoimmune hepatitis. Gut. 69, 569–577. doi: 10.1136/gutjnl-2018-317836
Weismuller, T. J., Trivedi, P. J., Bergquist, A., Imam, M., Lenzen, H., Ponsioen, C. Y., et al. (2017). Patient age, sex, and inflammatory bowel disease phenotype associate with course of primary sclerosing cholangitis. Gastroenterology 152, 1975–84 e8. doi: 10.1053/j.gastro.2017.02.038
Wu, J. L., Zou, J. Y., Hu, E. D., Chen, D. Z., Chen, L., Lu, F. B., et al. (2017). Sodium butyrate ameliorates S100/FCA-induced autoimmune hepatitis through regulation of intestinal tight junction and toll-like receptor 4 signaling pathway. Immunol. Lett. 190, 169–176. doi: 10.1016/j.imlet.2017.08.005
Yamaguchi, H., Miura, H., Ohsumi, K., Ishimi, N., Taguchi, H., Ishiyama, N., et al. (1994). Detection and characterization of antibodies to bacterial heat-shock protein 60 in sera of patients with primary biliary cirrhosis. Microbiol. Immunol. 38, 483–487. doi: 10.1111/j.1348-0421.1994.tb01813.x
Yamaguchi, T., Costabel, U., McDowell, A., Guzman, J., Uchida, K., Ohashi, K., et al. (2021). Immunohistochemical detection of potential microbial antigens in granulomas in the diagnosis of sarcoidosis. J. Clin. Med. 10:983. doi: 10.3390/jcm10050983
Yang, H., and Duan, Z. (2016). Bile acids and the potential role in primary biliary cirrhosis. Digestion 94, 145–153. doi: 10.1159/000452300
Zhang, H., Liu, M., Liu, X., Zhong, W., Li, Y., Ran, Y., et al. (2020). Bifidobacterium animalis ssp. Lactis 420 mitigates autoimmune hepatitis through regulating intestinal barrier and liver immune cells. Front. Immunol. 11:569104. doi: 10.3389/fimmu.2020.569104
Zhang, H., Liu, M., Zhong, W., Zheng, Y., Li, Y., Guo, L., et al. (2021). Leaky gut driven by dysbiosis augments activation and accumulation of liver macrophages via RIP3 signaling pathway in autoimmune hepatitis. Front. Immunol. 12:624360. doi: 10.3389/fimmu.2021.624360
Zhang, X., Li, L., Butcher, J., Stintzi, A., and Figeys, D. (2019). Advancing functional and translational microbiome research using meta-omics approaches. Microbiome 7:154. doi: 10.1186/s40168-019-0767-6
Zheng, D., Liwinski, T., and Elinav, E. (2020). Interaction between microbiota and immunity in health and disease. Cell Res. 30, 492–506. doi: 10.1038/s41422-020-0332-7
Keywords: autoimmune liver diseases, microbiome, metagenomic, metabolomic, bile acids
Citation: Zheng Y, Ran Y, Zhang H, Wang B and Zhou L (2021) The Microbiome in Autoimmune Liver Diseases: Metagenomic and Metabolomic Changes. Front. Physiol. 12:715852. doi: 10.3389/fphys.2021.715852
Received: 27 May 2021; Accepted: 24 August 2021;
Published: 08 October 2021.
Edited by:
Phillipp Hartmann, University of California, San Diego, United StatesReviewed by:
Zhi-Bin Zhao, Guangdong Provincial People’s Hospital, ChinaCopyright © 2021 Zheng, Ran, Zhang, Wang and Zhou. This is an open-access article distributed under the terms of the Creative Commons Attribution License (CC BY). The use, distribution or reproduction in other forums is permitted, provided the original author(s) and the copyright owner(s) are credited and that the original publication in this journal is cited, in accordance with accepted academic practice. No use, distribution or reproduction is permitted which does not comply with these terms.
*Correspondence: Lu Zhou, bHpob3UwMUB0bXUuZWR1LmNu; Bangmao Wang, bXdhbmcwMkB0bXUuZWR1LmNu
†These authors have contributed equally to this work
Disclaimer: All claims expressed in this article are solely those of the authors and do not necessarily represent those of their affiliated organizations, or those of the publisher, the editors and the reviewers. Any product that may be evaluated in this article or claim that may be made by its manufacturer is not guaranteed or endorsed by the publisher.
Research integrity at Frontiers
Learn more about the work of our research integrity team to safeguard the quality of each article we publish.