- 1Clinical Exercise Science, University Outpatient Clinic Potsdam, Department Sports and Health Sciences, University of Potsdam, Potsdam, Germany
- 2University Outpatient Clinic, Sports Medicine and Sports Orthopaedics, Department Sports and Health Sciences, University of Potsdam, Potsdam, Germany
- 3Department of Rehabilitation Medicine, Faculty of Health Science Brandenburg, University of Potsdam, Potsdam, Germany
- 4Department of Cardiology, Klinik am See, Rüdersdorf, Germany
Electrical muscle stimulation (EMS) is an increasingly popular training method and has become the focus of research in recent years. New EMS devices offer a wide range of mobile applications for whole-body EMS (WB-EMS) training, e.g., the intensification of dynamic low-intensity endurance exercises through WB-EMS. The present study aimed to determine the differences in exercise intensity between WB-EMS-superimposed and conventional walking (EMS-CW), and CON and WB-EMS-superimposed Nordic walking (WB-EMS-NW) during a treadmill test. Eleven participants (52.0 ± years; 85.9 ± 7.4 kg, 182 ± 6 cm, BMI 25.9 ± 2.2 kg/m2) performed a 10 min treadmill test at a given velocity (6.5 km/h) in four different test situations, walking (W) and Nordic walking (NW) in both conventional and WB-EMS superimposed. Oxygen uptake in absolute (VO2) and relative to body weight (rel. VO2), lactate, and the rate of perceived exertion (RPE) were measured before and after the test. WB-EMS intensity was adjusted individually according to the feedback of the participant. The descriptive statistics were given in mean ± SD. For the statistical analyses, one-factorial ANOVA for repeated measures and two-factorial ANOVA [factors include EMS, W/NW, and factor combination (EMS*W/NW)] were performed (α = 0.05). Significant effects were found for EMS and W/NW factors for the outcome variables VO2 (EMS: p = 0.006, r = 0.736; W/NW: p < 0.001, r = 0.870), relative VO2 (EMS: p < 0.001, r = 0.850; W/NW: p < 0.001, r = 0.937), and lactate (EMS: p = 0.003, r = 0.771; w/NW: p = 0.003, r = 0.764) and both the factors produced higher results. However, the difference in VO2 and relative VO2 is within the range of biological variability of ± 12%. The factor combination EMS*W/NW is statistically non-significant for all three variables. WB-EMS resulted in the higher RPE values (p = 0.035, r = 0.613), RPE differences for W/NW and EMS*W/NW were not significant. The current study results indicate that WB-EMS influences the parameters of exercise intensity. The impact on exercise intensity and the clinical relevance of WB-EMS-superimposed walking (WB-EMS-W) exercise is questionable because of the marginal differences in the outcome variables.
Introduction
Electrical muscle stimulation (EMS) is an increasingly popular training method and has become the focus of research in recent years. EMS is the electrical excitation and contraction of a muscle or a muscle group through an external applied electric field (Teschler and Mooren, 2019). Compared to voluntary exercise, which represents a progressive muscle fiber recruitment from small to large muscles according to the intensity of considered contraction, EMS represents a non-selective recruitment pattern of primarily fast-twitch motor units (muscle fibers type 2) at relatively low force levels (Henneman et al., 1965; Gregory and Bickel, 2005). Therefore, greater strength and power adaptation by synchronous recruitment of muscle fibers and a higher firing rate is possible (Gregory and Bickel, 2005). Otherwise, it is assumed that the application of EMS is just beneficial for submaximal and not for maximal exercise intensities (Paillard, 2018).
Electrical muscle stimulation devices are in a constant state of technological development. Compared to the devices used in previous studies (Buuren et al., 2013, 2015; Kemmler et al., 2013, 2016b,c), which require a connection between the main station, vest, and belts worn by the patient for power transfer via cable, newer EMS-devices work wirelessly via battery and apps for intensity adjusting. These new EMS devices offer a wide range of mobile applications for whole-body EMS (WB-EMS) training. Therefore, the use of superimposed WB-EMS for intensification of dynamic exercises has become more prominent in research (Wahl et al., 2014) with contradictory results. The previous studies have shown that superimposed WB-EMS can intensify voluntary bodyweight resistance training (Watanabe et al., 2019) and lead to the higher metabolic and respiratory response in its application during ergometer cycling (Mathes et al., 2017) and increased metabolic stress and hormonal responses (Wahl et al., 2015). In contrast, beneficial effects regarding the strength and power parameters are debatable. Superimposed dynamic WB-EMS resistance training seems to provide minor or no benefits when compared with dynamic resistance training alone (Micke et al., 2018).
Apart from the changing of dietary habits, endurance training is an important part of the management of several diseases and metabolic disorders such as type 2 diabetes mellitus (T2DM) (Hopps et al., 2011; Beavers et al., 2013). However, different reasons could lead to the physical inactivity of DMT2 patients. Previous studies identified walking as the most popular and most preferred exercise among these patients (Ford and Herman, 1995; Thomas et al., 2004). As a low-intensity exercise, it has various advantages compared with running and cycling. However, only minor beneficial metabolic effects for this patient group have been shown, indicating that the exercise intensity of normal walking might be insufficient (Karstoft et al., 2013). Furthermore, Nordic walking (NW) represents another approach for exercise in T2DM patients. At a defined speed, Nordic walking involves more muscles in various body segments and induces greater exercise intensity in terms of higher absolute oxygen uptake (VO2) compared with level walking (Sugiyama et al., 2013). Different reviews examining the health benefits of NW have confirmed these results. NW exerts beneficial effects on resting heart rate, blood pressure, exercise capacity, maximal oxygen consumption, and quality of life in patients with various diseases and can thus be recommended to a wide range of people as primary and secondary prevention (Tschentscher et al., 2013; Mathieson and Lin, 2014).
However, as already shown in other dynamic exercises, the question arises whether superimposed WB-EMS has also the potential to intensify W and NW resulting in higher metabolic response with potential therapeutic application in different patient cohorts. Therefore, the present study aimed to determine the differences in exercise intensity between WB-EMS-superimposed and conventional walking (WB-EMS-CON-W) and CON and WB-EMS-superimposed Nordic walking (WB-EMS-NW) during a treadmill test with a constant given velocity in a cohort of healthy men. We assume that WB-EMS-superimposed walking and Nordic walking (WB-EMS-W/NW) result in higher VO2 compared with the CON exercise execution. We also assume that due to the higher recruitment in various body segments during NW, WB-EMS has a higher impact on NW compared to W.
Materials and Methods
Study Design
The study was conducted as a randomized crossover design with a total of four measurement appointments. To avoid WB-EMS-induced delayed onset muscle soreness (DOMS) as a confounding factor on test performance (Nosaka et al., 2002; Mackey et al., 2008; Vanderthommen et al., 2012; Kemmler et al., 2015), an interval of 1 week between each of the four measurement appointments (M1–M4) was chosen. During the four measurements, the four test situations (CON-W, EMS- W, CON-NW, and EMS-NW) were conducted in random order. Randomization was carried out with randomization software (www.randomizer.org).
After participants were informed about the study background, aims, procedure, risks of the participation, and signing the written informed consent, every participant had a medical entrance examination during the first appointment (M1) to ensure their ability to perform the trial. During this examination, body weight (BW) and height were measured, and a physician performed a basic orthopedic and cardiopulmonary checkup which included a resting electrocardiogram. Blood samples were also taken to exclude acute infection.
Subjects
The recruitment of participants took place in the environment of the research facility and a local sports center. Healthy, non-smoking men between the age of 35 and 70 years with a moderate activity profile (<3 h/week) were included. Regular drug consumption, cardiac arrhythmias (Lown classification ≥ 3), heart failure (New York Heart Association classification ≥ II), and wearing an implantable cardiac device were exclusion criteria. Additional exclusion criteria were diseases, such as chronic obstructive pulmonary disease (COPD), movement-restricting diseases, and rheumatic and neurological diseases. Eleven healthy men (52.0 ± years; 85.9 ± 7.4 kg, 182 ± 6 cm, BMI 25.9 ± 2.2 kg/m2) volunteered to participate. The ethics committee of the University of Potsdam approved the study (application number: 19/2018).
Whole-Body EMS
The wireless WB-EMS device of Easy Motion Skin® (EasyMotionSkin GmbH, Leipzig, Germany) with a low-frequency neuromuscular electrical stimulation with interval stimulation pattern (pulse width 350 μs, current interval 9 s 85 Hz, and pause interval 1 s 7 Hz) was used. For WM-EMS, the participants had to wear a trunk vest, a waist belt, and cuffs on/over the thighs and upper arms for the stimulation of the eight muscle groups (upper arm, chest, shoulder, upper back, lower back, abdominal, gluteal, and upper legs). Due to the different electrical conductivity of the skin and tissues, WB-EMS intensity was adjusted individually and separately for each muscle group and the overall intensity during the WB-EMS test situations until the individual tolerated maximum amperage was reached. In contrast to previous studies that recommend a WB-EMS familiarization to achieve higher currents by the participants (Kemmler et al., 2016a, 2018; Teschler and Mooren, 2019), in this study, due to the question of the acute influence of EMS, a WB-EMS familiarization was not used.
Test Protocol and Measurement
At the beginning of each measurement, participants were asked for their subjective ability to perform the test. Current body weight was measured for the calculation of relative VO2 (VO2 in relation to BW). Depending on the test situation, the participant was prepared with the WB-EMS equipment and if applicable NW sticks, which were adjusted in relation to body height (height sticks = body height × 0.68). Afterward, the treadmill test (hp cosmos® saturn 250/100 r, incline 0.4%) took place (see Figure 1 for measurement protocol). After a 4 min warm-up at a walking velocity of 5 km/h, a 1-min break took place. Afterward, the 3-min WB-EMS-adjusting period at a test velocity of 6.5 km/h and the 10 min walking test at constant velocity were performed. During the WB-EMS adjusting, stimulation intensities for the specific muscle groups and the whole body were adjusted and increased until maximum tolerated amperage of the participant. WB-EMS set intensities were noted as a dimensionless quantity (given by the system).
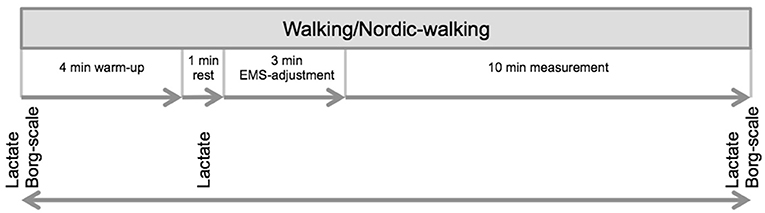
Figure 1. Study design. The study consisted of overall four appointments, one appointment for medical examination and first measurement and three more measurement appointments. An interval of 1 week between each measurement was implemented. During the four measurements, each condition (conventional walking, electrical muscle stimulation (EMS)-supported W, conventional Nordic walking, and EMS-supported Nordic walking) was performed in random order.
Outcome Measures
Outcome measures of interest were VO2, relative VO2, blood lactate concentration, and the rate of perceived exhaustion (RPE). For assessment of the respiratory values, the wireless portable breath-by-breath system MetaMax 3B by Cortex was used (Cortex Biophysik GmbH, Walter-Köhn-Straße 2d, 04356 Leipzig/Germany, measurement accuracy ± 2.2%). The MetaMax 3B system measures volume using a bidirectional digital turbine. Using the analysis software Metasoft version 3.9.5, the respiratory data, inspiration and expiration volume, and concentration of O2 and CO2 were calculated. The analyzing system was calibrated concerning volume, pressure, and gas (calibration gas: 15% O2 and 5% CO2) before every test. An air-conditioning system guaranteed stable conditions during the whole test in the lab (STPD). VO2 and relative VO2 were reported as an average value over the 10 min test.
Capillary blood samples were taken from the earlobe before the test, in the 1 min break after warm-up and after finishing the test. Blood lactate concentration was determined with in-house used analysis devices (Biosen S-line, EKF Diagnostic Sales, Magdeburg, Germany; enzymatic-amperometric method). Subjective perception of participants (RPE) of exercise intensity was assessed using the Borg scale (6–20) before and after the test (Scherr et al., 2012).
The reliability for VO2peak- and RPE measurements during WB-EMS-W were determined within earlier in-house testing using the 10 m incremental shuttle walk test. Results revealed good reliability for VO2 peak measurements (ICC = 0.886) with a test-retest variability within the range of biological day-to-day variability for VO2 measurements (± 12%) and good reliability of RPE measurements (ICC = 0.859).
Statistical Analysis
Results are presented descriptively (mean ± SD). The Kolmogorov–Smirnov test was used to test data for normality. Differences in outcome parameters between WB-EMS-W and CON-W to NW were presented relatively. BW differences between the four tests were analyzed using a one-factorial ANOVA test for repeated measures. To identify differences between WB-EMS-supported and CON-W respectively NW, two-factorial ANOVA with the single factors “EMS application” (EMS) and “(W/NW)” and its combination (EMS*W/NW) were performed. Paired t-test was used to identify differences in WB-EMS current intensities to the individual WB-EMS current intensities of the participants as well as in the mean current intensity of the eight muscle groups between the two measurement conditions. For all tests, the statistical software SPSS 23.0 was used, a significance level of α = 0.05 was assumed.
Results
All subjects completed the measurements successfully and were included for data analyses. There were no dropouts and no missing data. The results of body weight, the four outcome measurements, and results of the statistical analyses are presented in Table 1. Repeated measures ANOVA test showed a p-value of 0.194 and revealed no statistically significant difference in BW between the four test situations. Results for VO2 ranged from 1.72 ± 0.15 ml/min to 2.15 ± 0.21 ml/min with p-values of 0.006 (EMS), 0.000 (W/NW), and 0.935 (EMS*W/NW). The temporal course of VO2 for the four test conditions is given in Figure 2. Similar results can be seen for relative VO2 with mean values from 20.11 ± 1.05 to 25.18 ± 1.83 ml/min/kg and p-values of p < 0.001 (EMS), p < 0.000 (W/NW), and 0.935 (EMS*W/NW).
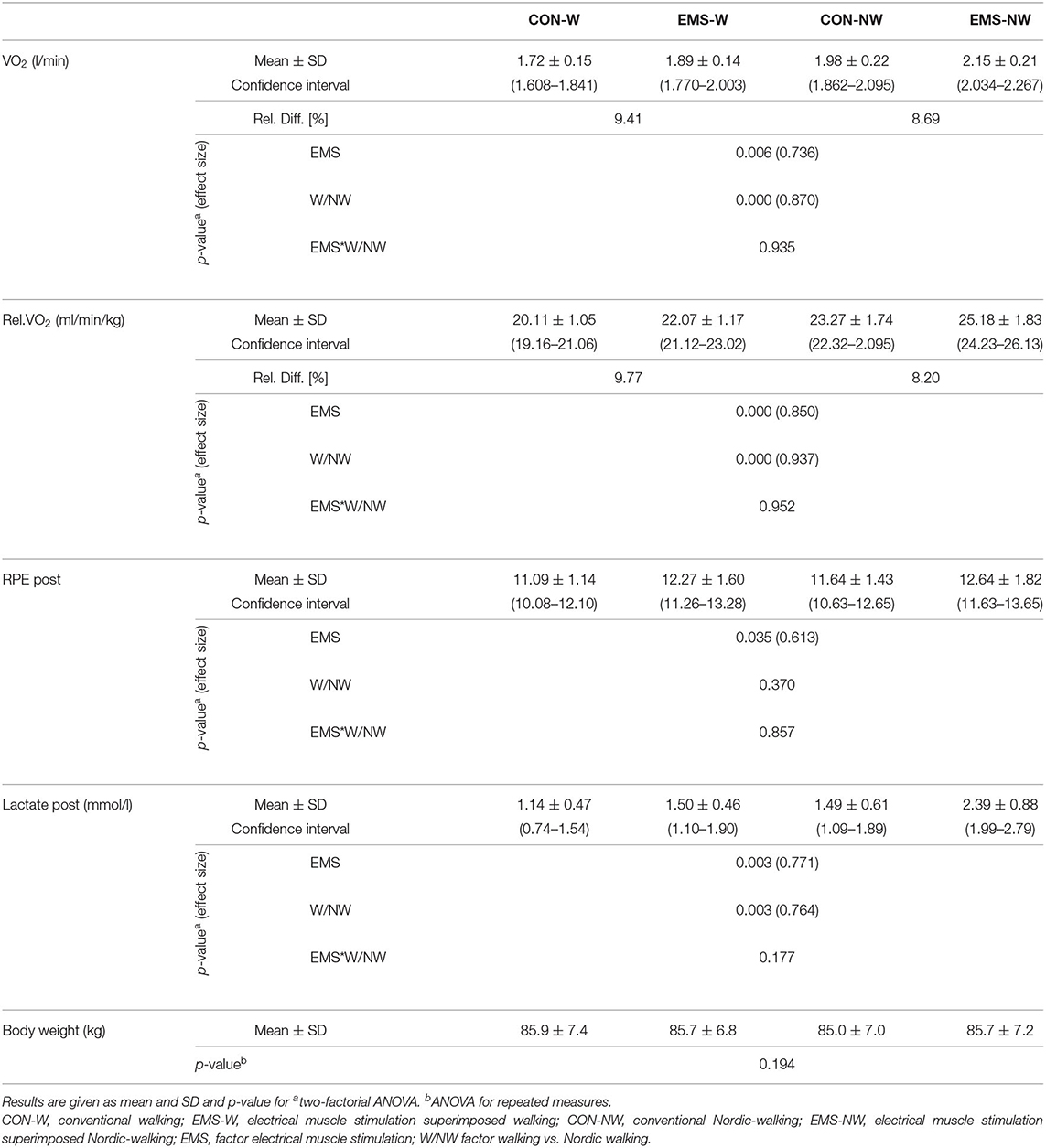
Table 1. Result for the body weight, absolute (VO2) and relative VO2 (rel.VO2; in relation to body weight) oxygen intake, rate of perceived exhaustion (RPE; Borg-scale), and post-exercise blood lactate concentration of the outcome variables.
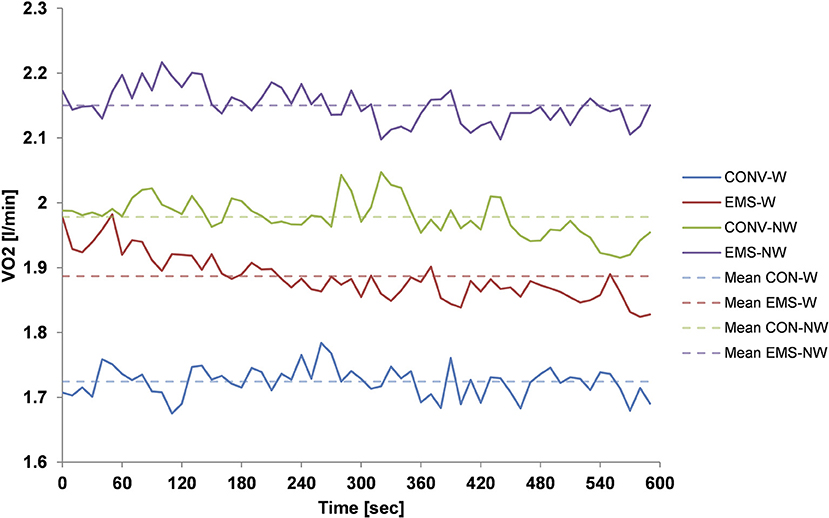
Figure 2. VO2 uptake during a 10 min treadmill test for the four different test situations: blue line indicates conventional walking (CON-W); red line indicates electrical muscle stimulation (EMS)-superimposed walking (EMS-W); green line indicates conventional Nordic walking (CON-NW); and the purple line indicates EMS-superimposed Nordic walking (EMS-NW). The solid line represents the mean on 10 s intervals for all subjects and the dashed line represents the mean value for all subjects over the 10 min measurement time. Solid shows continue steady progress in VO2 uptake for all measurement conditions with overlaps between two measurement conditions indicating differences in VO2 uptake between them. Differences in dashed lines support these results.
Post-exercise RPE ranged from 11.09 to 12.64 with a statistically significant difference for EMS as an influencing factor (p = 0.035). However, W/NW (p = 0.370) and the factor combination EMS*W/NW (p = 8.57) revealed no significant difference. The results for blood lactate concentration are in line with the results for VO2 and relative VO2 with a range of the mean values from 1.14 to 2.39 mmol−1, statistically significant results of the 2-factorial ANOVA for EMS (p = 0.003) and W/NW (p = 0.003), and a statistically non-significant result for EMS*W/NW (p = 0.117).
Results of WB-EMS current intensity analysis are given in Table 2. Individual WB-EMS current intensity of participants ranged from 19.9 to 29.8 with a mean ± SD of 24.7 ± 3.4 for W and from 19.5 to 32.8 with a mean ± SD of 27.7 ± 3.8 for NW. The t-test revealed no significant difference (p = 0.100). Muscle group WB-EMS current intensity ranged from 15.6 to 43.7 with a mean ± SD of 24.7 ± 8.4 for W and from 15.6 to 46.6 with a mean ± SD of 25.7 ± 3.8 for NW. The t-test revealed no significant difference (p = 0.054).
Discussion
The major finding of our study is that superimposed WB-EMS affect metabolic demand during W and NW significantly. VO2 uptake was higher in both EMS-W/NW when compared with CON exercises. In addition, WB-EMS leads to higher blood lactate concentrations when applied to exercise. However, clinical relevance for VO2 uptake and blood lactate concentration is debatable. The second finding of our study is that WB-EMS-superimposed exercises are perceived as more strenuous compared with CON exercise.
To our knowledge, this is the first investigation of a possible intensification of low-intensity exercises through superimposed WB-EMS. However, a wide range of studies already showed that EMS has the potential to increase the metabolic demands of different types of exercise, whether limited to a single muscle or related to the whole body (Buuren et al., 2015; Mathes et al., 2017). Measuring oxygen uptake relative to body weight during exercise represents the gold standard for measurements of exercise intensity. However, different outcome measures are useful to define metabolic demands and metabolic stress to identify the intensification potential of WB-EMS respectively EMS isolated for specific muscles.
As a prerequisite for evaluating the relative oxygen uptake, we analyzed the body weight difference of the participants between the four measurement appointments. ANOVA for repeated measures resulted in a p-value of 0.94 being synonymous with a statistically non-significant body weight difference between the four measurement appointments. Therefore, we can conclude that reported differences in relative VO2 are founded by differences in VO2 and not by variations in body weight. Amaro-Gahete et al. (2018) investigated performance-related parameters in runners and concluded that 6 weeks of WB-EMS training, coupled with a reduction in running endurance training, improved relativeVO2max. While VO2 did not improve after the intervention period, body weight reduction during that time was statistically significant. Therefore, the conclusion of a relativeVO2max improvement through WB-EMS training implementation compared with the control group training conventionally with non-significant differences in VO2 and body weight is worth challenging. However, due to our body weight data, we can conclude the effect of WB-EMS-W/NW on relative VO2.
Regarding WB-EMS-superimposed cycling, several studies support our findings regarding exercise intensification through WB-EMS. Wahl et al. (2012) investigated the physiological responses and perceived exertion during an incremental cycle ergometer test. Compared to our study, EMS was just applied to the thigh and calf muscles. However, they found higher metabolic stress at 75%, respectively, 100% of peak power output when superimposed EMS was applied. In a further study, they found similar results during a 60 min constant workload test on the cycle ergometer (Wahl et al., 2014). Mathes et al. (2017) investigated the chronic effects of superimposed WB-EMS during ergometer cycling. Participants in the WB-EMS-superimposed cycling group performed the training sessions during the 4 week training intervention with a significantly higher VO2peak (7% higher) compared with the cycling group. Even though participants performed more demanding exercises for the metabolic and respiratory system with applied WB-EMS, a 4 week training intervention of WB-EMS-superimposed cycling exercise did not result in superior improvements of endurance capacity than cycling alone did. This fact is in line with our results that the increase of 9.41% (VO2) compared to 9.77% (relative VO2) for WB-EMS-W and 8.69% (VO2) compared to 8.2% (relative VO2) for WB-EMS-NW is within the range of biological day-to-day variability. Therefore, regardless of the fact that the differences we obtained are statistically significant (WB-EMS as factor of 2-factorial ANOVA), the intensification of W and NW through WB-EMS seems to be clinically irrelevant for this population. However, the results for combined factor analyses (EMS*W/NW) of the 2-factorial ANOVA test indicate that WB-EMS does not have a different impact on VO2 between WB-EMS-W/NW. This result contradicts our hypothesis that due to the higher muscle recruitment in various body segments during NW, WB-EMS has a higher impact on NW compared to W.
This conclusion is also confirmed by the results of the EMS current intensity analysis. Various studies recommend a WB-EMS familiarization for a higher WB-EMS current intensity toleration by the participants and ultimately higher impact of the WB-EMS (Kemmler et al., 2016a, 2018; Teschler and Mooren, 2019). Since none of the study participants has had any experience with EMS so far, we did not do this familiarization to investigate the acute impact of WB-EMS. The analysis of the EMS current intensities showed no significant differences in the individual current strength of the participants or the current strength related to the eight muscle groups.
In addition to the use of superimposed WB-EMS in endurance training, it is also used as an intensification tool in strength training. Watanabe et al. (2019) investigated metabolic differences of WB-EMS, voluntary exercise, and its combination during body weight resistance training. They found an increase in relative VO2 of ≈23% during WB-EMS-superimposed body weight resistance training. Compared to the difference for VO2, we obtained during WB-EMS-W respectively NW, the VO2 increase during WB-EMS-superimposed resistance training seems to be clinically relevant. One reason for this difference might be differences in the focus of the participants on the exercise. During the WB-EMS resistance training, a 4 s exercise, 4 s rest intervals were used, whereas WB-EMS was just applied during continuous dynamic exercise. Therefore, it might be possible that participants were more focused on their muscle contraction during the 4 s intervals compared with the ongoing walking tasks on the treadmill, and a higher WB-EMS intensity was tolerable leading to a higher VO2 increase.
An interesting result of our study is the difference in VO2 between W and NW. We obtained a 15.12% higher VO2 for CON-W vs. NW, respectively, 13.76% for WB-EMS-superimposed exercise. For relative VO2, differences are 15.71% (CON) and 14.09% (WB-EMS superimposed). NW has been the subject of research in previous studies, for example, Schiffer et al. (2006) investigated differences in VO2 between W and NW. In contrast to our results, they found a VO2 difference between W and NW of 8% for a similar velocity of 1.8 m/s (≈ 6.48 km/h). In contrast, Church et al. (2002) found a ≈20% higher VO2 during a 1,600 m walking field test at self-selected walking speed. Figard-Fabre et al. (2009) investigated the impact of a 4 week learning period of the NW technique on VO2 intake during a 5 min treadmill test (v = 4 km/h). In line with our results, they found an initial difference of ≈12.2% in VO2 between NW and W and beyond an increase of ≈17.6% after the learning period. Our participants were only fundamentally familiar with the NW technique. Therefore, it could not be excluded that a familiarization of the participants with the NW technique might have enlarged the difference in VO2 intake between W and NW. It can only be speculated about a stronger WB-EMS impact after an NW technique-learning period. However, a longer period of NW with additional WB-EMS might increase the VO2 uptake and relative VO2 uptake.
In addition to the measurable difference in exercise intensity, the RPE is an important factor during the investigation of new training methods. Our results suggest that both WB-EMS-superimposed exercises, W and NW, are felt as more exhausting compared to the CON exercise. The application of WB-EMS on exercise seems to be a significant influencing factor regarding RPE. In contrast to this, Wahl et al. (2012) found conflicting results for EMS-superimposed cycling on an ergometer. While there were no differences in a first examinationKlicken oder tippen Sie hier, um Text einzugeben., another study came to the result that EMS-superimposed cycling was perceived as more strenuous than cycling alone (Wahl et al., 2014). Therefore, an evaluation of our results is difficult. It is different from the comparison between W and NW for both CON and EMS superimposed. In line with the previous studies, we did not find a statistically significant difference in RPE between the two exercises for both test situations. Barberan-Garcia et al. (2015) reported that NW generates a higher exercise intensity at the same RPE compared with standard W. They investigated VO2 uptake and RPE (using modified Borg scale) in a group of chronic obstructive pulmonary disease patients and compared the results of two 6 min walking tests, one time during a standard W test and other time during NW. Participants did not report any differences in RPE between W and NW. Figard-Fabre et al. (2011) investigated RPE between W and NW in a group of obese middle-aged women. Results confirmed our findings of similar RPE between W and NW.
Lactate measurements represent another tool for exercise intensity evaluation. As a result of our investigation, we were able to show that WB-EMS-W/NW leads to higher lactate formation in the muscles compared with CON exercise. However, it is debatable whether the extent of this increase is relevant or not. This result supports the assumption that EMS primarily excites type 2 muscle fibers, which have a higher lactate formation compared to type 1 muscle fibers. In line with our results, Wahl et al. (2012, 2014) also found that WB-EMS-superimposed cycling leads to a significantly higher lactate formation compared with cycling alone during a 60 min constant workload and an incremental test. Due to the additional non-selective recruitment pattern of primarily fast-twitch motor units, they concluded that an intensifying impact of WB-EMS on low-intensity endurance exercise could be a result of the mild eccentric work induced by the simultaneous activation of agonist and antagonist during the cycling movement. Further research seems to be necessary to investigate the impact and type of contraction modes of WB-EMS during dynamic exercises.
There are some limitations of the current study. The high variability in the age of the included participants within this sample can be stated as a limitation of the study with regard to the results, since it would be expected that there would also be a high scatter, especially with VO2 and relative VO2. The decision for this inclusion criterion was based on an assessment of the age of a manifestation of T2DM (35 years) and the occurrence of accompanying diseases (70 years), which could influence the outcome measures. However, the low SD in the examined variables suggests that age should not be regarded as an influencing factor. This could be because the selected exercise intensity for the W and NW, both conventionally and under WB-EMS, was a low-intensity exercise in a range in which the age and the associated VO2 capacity of the test persons have no influence.
Our results show that the use of WB-EMS to intensify exercise is limited, at least in our subject group. An influence of the WB-EMS on exercise intensity is statistically shown but is below the threshold for clinical relevance. Therefore, the high cost of acquiring the device should be considered when using WB-EMS in different patient groups, such as T2DM and assessing training success. However, habituation of the subjects with the WB-EMS and the presumed higher tolerance to the current intensity of the WB-EMS and associated higher intensification may still change this cost-benefit consideration and indicate WB-EMS-W or NW as an effective training method in different patient groups such as T2DM.
Conclusion
Our results indicate that WB-EMS influences parameters of exercise intensity. The impact on exercise intensity and the suitability for intensifying low-intensity exercise is questionable because of the low differences in the outcome variables. Regarding our results, CON-NW seems to be a more intensive training method than WB-EMS-W. Further studies seem to be necessary to confirm our results. An application of WB-EMS with more intensive ergometer exercises, such as elliptical machine training, could be another approach for WB-EMS-superimposed training. We acknowledge the support of the Deutsche Forschungsgemeinschaft and Open Access Publishing Fund of University of Potsdam.
Data Availability Statement
The raw data supporting the conclusions of this article will be made available by the authors, without undue reservation.
Ethics Statement
The studies involving human participants were reviewed and approved by Ethics Committee of the University of Potsdam. The patients/participants provided their written informed consent to participate in this study.
Author Contributions
RV and JS conceived, designed the research, and analyzed the data. RV conducted the experiments and wrote the manuscript. JS, MH, AQ, and HV revised the manuscript. All authors read and approved the manuscript.
Conflict of Interest
The authors declare that the research was conducted in the absence of any commercial or financial relationships that could be construed as a potential conflict of interest.
Publisher's Note
All claims expressed in this article are solely those of the authors and do not necessarily represent those of their affiliated organizations, or those of the publisher, the editors and the reviewers. Any product that may be evaluated in this article, or claim that may be made by its manufacturer, is not guaranteed or endorsed by the publisher.
References
Amaro-Gahete, F. J., De-la-O, A., Sanchez-Delgado, G., Robles-Gonzalez, L., Jurado-Fasoli, L., et al. (2018). Whole-body electromyostimulation improves performance-related parameters in runners. Front. Physiol. 9:1576. doi: 10.3389/fphys.2018.01576
Barberan-Garcia, A., Arbillaga-Etxarri, A., Gimeno-Santos, E., Rodríguez, D. A., Torralba, Y., Roca, J., et al. (2015). Nordic walking enhances oxygen uptake without increasing the rate of perceived exertion in patients with chronic obstructive pulmonary disease. Respiration 89, 221–225. doi: 10.1159/000371356
Beavers, K. M., Ambrosius, W. T., Nicklas, B. J., and Rejeski, W. J. (2013). Independent and combined effects of physical activity and weight loss on inflammatory biomarkers in overweight and obese older adults. J. Am. Geriatr. Soc. 61, 1089–1094. doi: 10.1111/jgs.12321
Buuren, F., van Horstkotte, D., Mellwig, K. P., Fründ, A., Vlachojannis, M., Bogunovic, N., et al. (2015). Electrical myostimulation (EMS) improves glucose metabolism and oxygen uptake in type 2 diabetes mellitus patients—results from the EMS study. Diabetes Technol.Therap. 17, 413–419. doi: 10.1089/dia.2014.0315
Buuren, F., van Mellwig, K. P., Prinz, C., Körber, B., Fründ, A., Fritzsche, D., et al. (2013). Electrical myostimulation improves left ventricular function and peak oxygen consumption in patients with chronic heart failure: results from the exEMS study comparing different stimulation strategies. Clinical Research in Cardiology 102, 523–534. doi: 10.1007/s00392-013-0562-5
Church, T. S., Earnest, C. P., and Morss, G. M. (2002). Field testing of physiological responses associated with Nordic Walking. Res. Q. Exerc. Sport 73, 296–300. doi: 10.1080/02701367.2002.10609023
Figard-Fabre, H., Fabre, N., Leonardi, A., and Schena, F. (2009). Physiological and perceptual responses to Nordic walking in obese middle-aged women in comparison with the normal walk. Eur. J. Appl. Physiol. 108, 1141–1151. doi: 10.1007/s00421-009-1315-z
Figard-Fabre, H., Fabre, N., Leonardi, A., and Schena, F. (2011). Efficacy of Nordic Walking in Obesity Management. Int. J. Sports Med. 32, 407–414. doi: 10.1055/s-0030-1268461
Ford, E. S., and Herman, W. H. (1995). Leisure-time physical activity patterns in the U.S. diabetic population. Findings from the 1990 National Health Interview Survey—Health Promotion and Disease Prevention Supplement. Diabetes care 18, 27–33.
Gregory, C. M., and Bickel, C. S. (2005). Recruitment patterns in human skeletal muscle during electrical stimulation. Phys. Ther. 85, 358–364.
Henneman, E., Somjen, G., and Carpenter, D. O. (1965). Functional significance of cell size in spinal motoneurons. J. Neurophysiol. 28, 560–580. doi: 10.1152/jn.1965.28.3.560
Hopps, E., Canino, B., and Caimi, G. (2011). Effects of exercise on inflammation markers in type 2 diabetic subjects. Acta Diabetol. 48, 183–189. doi: 10.1007/s00592-011-0278-9
Karstoft, K., Winding, K., Knudsen, S. H., Nielsen, J. S., Thomsen, C., Pedersen, B. K., et al. (2013). The effects of free-living interval-walking training on glycemic control, body composition, and physical fitness in type 2 diabetic patients: a randomized, controlled trial. Diabetes Care 36, 228–236. doi: 10.2337/dc12-0658
Kemmler, W., Bebenek, M., Engelke, K., Stengel, S., and von (2013). Impact of whole-body electromyostimulation on body composition in elderly women at risk for sarcopenia: the Training and ElectroStimulation Trial (TEST-III). AGE 36, 395–406. doi: 10.1007/s11357-013-9575-2
Kemmler, W., Froehlich, M., Stengel, S., and von Kleinöder, H. (2016a). Whole-body electromyostimulation—the need for common sense! rationale and guideline for a safe and effective training. Deuts. Z. Sportmed. 2016, 218–221. doi: 10.5960/dzsm.2016.246
Kemmler, W., Kohl, M., Stengel, S., and von (2016b). Effects of high intensity resistance training versus whole-body electromyostimulation on cardio-metabolic risk factors in untrained middle-aged males. A randomized controlled trial. J. Sports Res. 2016, 44–55. doi: 10.18488/journal.90/2016.3.2/90.2.44.55
Kemmler, W., Teschler, M., Bebenek, M., Stengel, S., and von (2015). (Very) high Creatinkinase concentration after exertional whole-body electromyostimulation application: health risks and longitudinal adaptations. Wiener Med. Wochen. 165, 427–435. doi: 10.1007/s10354-015-0394-1
Kemmler, W., Teschler, M., Weißenfels, A., Bebenek, M., Fröhlich, M., Kohl, M., et al. (2016c). Effects of whole-body electromyostimulation versus high-intensity resistance exercise on body composition and strength: a randomized controlled study. Evid. Based Complem. Altern. Med. 2016, 1–9. doi: 10.1155/2016/9236809
Kemmler, W., Weißenfels, A., Willert, S., Shojaa, M., Stengel, S., von Filipovic, A., et al. (2018). Efficacy and safety of low frequency whole-body electromyostimulation (WB-EMS) to improve health-related outcomes in non-athletic adults. a systematic review. Front. Physiol. 9:1594. doi: 10.3389/fphys.2018.00573
Mackey, A. L., Bojsen-Moller, J., Qvortrup, K., Langberg, H., Suetta, C., Kalliokoski, K. K., et al. (2008). Evidence of skeletal muscle damage following electrically stimulated isometric muscle contractions in humans. J. Appl. Physiol. 105, 1620–1627. doi: 10.1152/japplphysiol.90952.2008
Mathes, S., Lehnen, N., Link, T., Bloch, W., Mester, J., and Wahl, P. (2017). Chronic effects of superimposed electromyostimulation during cycling on aerobic and anaerobic capacity. Eur. J. Appl. Physiol. 117, 881–892. doi: 10.1007/s00421-017-3572-6
Mathieson, S., and Lin, C.-W. C. (2014). Health benefits of Nordic walking; a systematic review. Br. J. Sports Med. 48, 1577–1578. doi: 10.1136/bjsports-2013-093294
Micke, F., Kleinöder, H., Dörmann, U., Wirtz, N., and Donath, L. (2018). Effects of an eight-week superimposed submaximal dynamic whole-body electromyostimulation training on strength and power parameters of the leg muscles: a randomized controlled intervention study. Front. Physiol. 9:1719. doi: 10.3389/fphys.2018.01719
Nosaka, K., Newton, M., and Sacco, P. (2002). Delayed-onset muscle soreness does not reflect the magnitude of eccentric exercise-induced muscle damage. Scand. J. Med. Sci. Sports 12, 337–346. doi: 10.1034/j.1600-0838.2002.10178.x
Paillard, T. (2018). Training based on electrical stimulation superimposed onto voluntary contraction would be relevant only as part of submaximal contractions in healthy subjects. Front. Physiol. 9:1428. doi: 10.3389/fphys.2018.01428
Scherr, J., Wolfarth, B., Christle, J. W., Pressler, A., Wagenpfeil, S., and Halle, M. (2012). Associations between Borg's rating of perceived exertion and physiological measures of exercise intensity. Eur. J. Appl. Physiol. 113, 147–155. doi: 10.1007/s00421-012-2421-x
Schiffer, T., Knicker, A., Hoffman, U., Harwig, B., Hollmann, W., and Strüder, H. K. (2006). Physiological responses to nordic walking, walking and jogging. European Journal of Applied Physiology 98, 56–61. doi: 10.1007/s00421-006-0242-5
Sugiyama, K., Kawamura, M., Tomita, H., and Katamoto, S. (2013). Oxygen uptake, heart rate, perceived exertion, and integrated electromyogram of the lower and upper extremities during level and Nordic walking on a treadmill. J. Physiol. Anthropol. 32:2. doi: 10.1186/1880-6805-32-2
Teschler, M., and Mooren, F. C. (2019). (Whole-Body) Electromyostimulation, muscle damage, and immune system: a mini review. Front. Physiol. 10:1461. doi: 10.3389/fphys.2019.01461
Thomas, N., Alder, E., and Leese, G. P. (2004). Barriers to physical activity in patients with diabetes. Postgrad. Med. J. 80:287. doi: 10.1136/pgmj.2003.010553
Tschentscher, M., Niederseer, D., and Niebauer, J. (2013). Health benefits of Nordic walking: a systematic review. Am. J. Prev. Med. 44, 76–84. doi: 10.1016/j.amepre.2012.09.043
Vanderthommen, M., Triffaux, M., Demoulin, C., Crielaard, J.-M., and Croisier, J.-L. (2012). Alteration of muscle function after electrical stimulation bout of knee extensors and flexors. J. Sports Sci. Med. 11, 592–599.
Wahl, P., Hein, M., Achtzehn, S., Bloch, W., and Mester, J. (2014). Acute metabolic, hormonal and psychological responses to cycling with superimposed electromyostimulation. Eur. J. Appl. Physiol. 114, 2331–2339. doi: 10.1007/s00421-014-2952-4
Wahl, P., Hein, M., Achtzehn, S., Bloch, W., and Mester, J. (2015). Acute effects of superimposed electromyostimulation during cycling on myokines and markers of muscle damage. J. Musculoskelet. Neuron. Interac. 15, 53–59.
Wahl, P., Schaerk, J., Achtzehn, S., Kleinöder, H., Bloch, W., and Mester, J. (2012). Physiological responses and perceived exertion during cycling with superimposed electromyostimulation. J. Strength Condition. Res. 26, 2383–2388. doi: 10.1519/jsc.0b013e31823f2749
Keywords: electrical muscle stimulation, walking, Nordic walking, treadmill, exercise intensity
Citation: Verch R, Stoll J, Hadzic M, Quarmby A and Völler H (2021) Whole-Body EMS Superimposed Walking and Nordic Walking on a Treadmill—Determination of Exercise Intensity to Conventional Exercise. Front. Physiol. 12:715417. doi: 10.3389/fphys.2021.715417
Received: 26 May 2021; Accepted: 06 August 2021;
Published: 04 October 2021.
Edited by:
Wolfgang Kemmler, Friedrich-Alexander-University Erlangen-Nürnberg, GermanyReviewed by:
Alvaro De Pano, Universitat de Lleida, SpainPaula Ricci, Federal University of São Carlos, Brazil
Copyright © 2021 Verch, Stoll, Hadzic, Quarmby and Völler. This is an open-access article distributed under the terms of the Creative Commons Attribution License (CC BY). The use, distribution or reproduction in other forums is permitted, provided the original author(s) and the copyright owner(s) are credited and that the original publication in this journal is cited, in accordance with accepted academic practice. No use, distribution or reproduction is permitted which does not comply with these terms.
*Correspondence: Ronald Verch, verch@uni-potsdam.de