- 1National Center for Genetic Engineering and Biotechnology (BIOTEC), Thailand Science Park, Pathum Thani, Thailand
- 2Department of Agricultural and Food Sciences, Alma Mater Studiorum, University of Bologna, Cesena, Italy
Transcriptomes associated with wooden breast (WB) were characterized in broilers at two different market ages. Breasts (Pectoralis major) were collected, 20-min postmortem, from male Ross 308 broilers slaughtered at 6 and 7 weeks of age. The breasts were classified as “non-WB” or “WB” based on palpation hardness scoring (non-WB = no abnormal hardness, WB = consistently hardened). Total RNA was isolated from 16 samples (n = 3 for 6 week non-WB, n = 3 for 6 week WB; n = 5 for 7 week non-WB, n = 5 for 7 week WB). Transcriptome was profiled using a chicken gene expression microarray with one-color hybridization technique, and compared between non-WB and WB samples of the same age. Among 6 week broilers, 910 transcripts were differentially expressed (DE) (false discovery rate, FDR < 0.05). Pathway analysis underlined metabolisms of glucose and lipids along with gap junctions, tight junction, and focal adhesion (FA) signaling as the top enriched pathways. For the 7 week broilers, 1,195 transcripts were identified (FDR < 0.05) with regulation of actin cytoskeleton, mitogen-activated protein kinase (MAPK) signaling, protein processing in endoplasmic reticulum and FA signaling highlighted as the enriched affected pathways. Absolute transcript levels of eight genes (actinin-1 – ACTN1, integrin-linked kinase – ILK, integrin subunit alpha 8 – ITGA8, integrin subunit beta 5 – ITGB5, protein tyrosine kinase 2 – PTK2, paxillin – PXN, talin 1 – TLN1, and vinculin – VCL) of FA signaling pathway were further elucidated using a droplet digital polymerase chain reaction. The results indicated that, in 6 week broilers, ITGA8 abundance in WB was greater than that of non-WB samples (p < 0.05). Concerning 7 week broilers, greater absolute levels of ACTN1, ILK, ITGA8, and TLN1, accompanied with a reduced ITGB5 were found in WB compared with non-WB (p < 0.05). Transcriptional modification of FA signaling underlined the potential of disrupted cell-cell communication that may incite aberrant molecular events in association with development of WB myopathy.
Introduction
Wooden breast (WB) abnormality, one of the emerging growth-related myopathies affecting chicken pectoral muscle, is characterized by hardened ridges extending from the cranial to the caudal regions of the breast (Sihvo et al., 2014). The abnormal breasts often exhibit pale color, surface hemorrhagic lesions, and clear viscous exudate on the surface (Barbut, 2019). Identified shortly after white striping (WS) abnormality, WB has abruptly raised a wide concern in the broiler industry worldwide (Sihvo et al., 2014; Velleman and Clark, 2015; de Carvalho et al., 2020; Xing et al., 2020) as it exerts remarkable adverse impacts on technological properties, including water holding capacity and texture, of chicken breasts (Chatterjee et al., 2016; Soglia et al., 2016; Tasoniero et al., 2016; Tijare et al., 2016; Cai et al., 2018; Malila et al., 2018; Madruga et al., 2019). Hence, it has caused tremendous economic losses. In United States alone, it was estimated that the growth-related myopathies had costed approximately 70,000 USD losses daily (Zanetti et al., 2018).
The actual etiology and the chronological events underlying development of WB are under extensive investigation. A growing evidence collected from scientific reports have pointed out the association between WB myopathy with artificial selection for rapid growth rate, enlarged muscle mass, and accelerated metabolism (Malila et al., 2018; Barbut, 2019; Petracci et al., 2019). The disproportionate muscles outgrew their supportive systems, particularly vascularization and oxygenation, leading to a series of molecular and ultrastructural disturbances that negatively affected myocellular integrity (Papah et al., 2018; Papah and Abasht, 2019; Maharjan et al., 2020; Abasht et al., 2021). Signs of phlebitis along with muscle damage and regeneration were observed as soon as 14 days of age in broilers (Papah et al., 2017; Radaelli et al., 2017). Damaged muscle fibers were replaced by collagen and adipose tissue and exhibited altered sarcomeric structure and functions (Papah et al., 2017; Velleman et al., 2017; Liu et al., 2020). Metabolic processes, ion homeostasis, as well as oxidative stress response mechanisms can be profoundly disrupted (Mutryn et al., 2015; Abasht et al., 2016; Kang et al., 2020). Soglia et al. (2020) recently demonstrated an increase in vimentin (VIM) and desmin (DES) in WB muscles compared with those of normal counterparts, suggesting the occurrence of intensive regenerative processes within these muscles. Muscle regeneration requires the donation of nuclei from satellite cells, the myogenic stem cells required in post-hatch skeletal muscle growth and regeneration (Velleman, 2015). In agreement with the on-going regenerative process in the presence of WB myopathy, numbers of total nuclei and mitotically active satellite cells were increased in the 43 day broilers compared with those of non-WB (Meloche et al., 2018). However, the mitotic activity of the satellite cell population was significantly altered in WB broiler, suggesting the compromised reparative capacity of the satellite cells in the abnormal birds (Ferreira et al., 2020).
Previous studies demonstrated the effects of age and hypertrophy on increasing the severity of muscle degeneration (Radaelli et al., 2017) and on the losses of satellite cells at both quantitative and functional characteristics, hence impairing muscle regeneration (Daughtry et al., 2017) in broilers. As described by Domingues-Faria et al. (2016), when skeletal muscle undergoes damage, pro-inflammatory cytokines recruit immune cells to the lesion site thus promoting migration and proliferation of satellite cells. Neutrophils take parts in the lysis of damaged cells as well as in phagocytosis to remove cell debris through apoptosis. The pro-inflammatory macrophages are then converted into anti-inflammatory phenotype to initiate differentiation of satellite cells, hence muscle repair. Overall, the repair process requires a well-regulated crosstalk between the muscle cells and the immune cells. In this regard, it is worth mentioning that, although affected by WB at a similar extent, broilers slaughtered at different ages might exhibit different molecular responses. Based on our previous study (Malila et al., 2018), the greater numbers of WB cases were found in the commercial broilers slaughtered at the age of 7 weeks in comparison to those slaughtered at 6 weeks. The increased WB severity was significantly related with the increased proportion of breast mass relative to carcass weight which was observed more in the group of 7-week-old broilers (Malila et al., 2018). Thus, performing further investigations may improve the knowledge relating to key biological processes associated with the development of WB. Here, the transcriptional profiles of non-WB and WB samples collected from commercial broilers at 6 and 7 weeks of age were assessed in order to investigate the progression of the muscular alterations occurring when the slaughter age was postponed.
Materials and Methods
Samples and Sample Collection
The breast samples used in this study were collected from carcasses of Ross 308 broilers, males, reared in a commercial farm until the age of 6 weeks (6wk) or 7 weeks (7wk). All birds were slaughtered at a local industrial-scale slaughterhouse. After the scalding step, the carcasses were randomly collected from the processing line, the breasts were dissected, and classified as “non-WB” or “WB” based on the presence of hardened bulge ridges assessed by palpation (Sihvo et al., 2017). The WB samples used in this study could be classified as “severe WB” (>75% of the breast being extremely rigid and with diffuse coverage) (Sihvo et al., 2017; Petracci et al., 2019). A sample was excised from the cranial portion (approximately 1 cm deep from the ventral surface) of the muscle, cut into 1 cm3 cubes, snap frozen in liquid nitrogen and stored at −80°C until RNA isolation.
Among the samples collected from 6 and 7-week-old birds, three and five samples were identified as WB, respectively. It is worth noting that all WB samples showed macroscopic characteristics of WS. The equal numbers of 6 and 7 week breast samples exhibiting no abnormal hardness were randomly selected to represent the non-WB group in this study. Hence, 16 selected samples were classified into four groups based on age and abnormality, i.e., 6wk; non-WB (n = 3), 6wk; WB (n = 3), 7wk; non-WB (n = 5), and 7wk; WB (n = 5).
Total RNA Isolation
Total RNA was isolated using TRIzolTM Reagent (Invitrogen), subsequently treated with DNase I (Thermo Scientific, Inc.) and re-purified using GeneJET RNA Cleanup and Concentration Micro Kit (Thermo Scientific, Inc.). The samples with an RNA Integrity Number exceeding 7.0 were analyzed by microarray hybridization at Molecular Genomics Pte., Ltd., (Singapore), quantitative real-time polymerase chain reaction (qPCR), and droplet digital PCR (ddPCR).
Microarray Hybridization and Data Analysis
The Agilent SurePrint G3 Custom GE 8 × 60 K chicken gene expression microarray (Agilent Technologies, Inc.) used in this study was designed based on the National Center for Biotechnology Information (NCBI) Gallus gallus Annotation Release 103.
One-color microarray hybridization was conducted as described in Malila et al. (2020). Briefly, total RNA was reverse transcribed into cDNA using an oligo(dT) as a primer. The cDNA was in vitro transcribed using T7 RNA polymerases to produce cyanine 3-CTP-labeled complementary RNA using a one-color low input quick amp labeling kit (Agilent) following the company’s instruction. The purified cRNA (600 ng) was hybridized onto the Agilent SurePrint array at 65°C for 17 h. The array was subsequently scanned using an Agilent High Resolution Microarray Scanner (C Model, Agilent). The TIFF image was exported and analyzed using an Agilent Feature Extraction Software version V10.7.1.1 (Agilent).
Microarray probe quality control was processed using GeneSpring software. The signal values of probes that were not met the quality control were excluded from the analysis (Malila et al., 2020). The filtered raw data were normalized using quantile normalization (Bolstad, 2017) and combat normalization afterward (Müller et al., 2016; Leek et al., 2017) in R version 3.4.3 (R Core Team, 2017). Log2 transformation was applied to normalized data for statistical analysis. Significant differences in the transcript abundance between non-WB vs WB samples belonging to each slaughter age group (6wk and 7wk) were assessed by t-test analyses for independent samples. Positive and negative fold change (FC) values represent increased or decreased expression of a particular gene in the WB samples relative to non-WB of the same slaughter age. The differentially expressed (DE) transcripts were identified using combined criteria of false discovery rate (FDR) ≤ 0.05 and absolute fold change (|FC|) ≥ 1.2. All DEGs of each comparison were mapped onto Kyoto Encyclopedia of Genes and Genomes (KEGG) pathway (Kanehisa et al., 2012) to identify possible biological interactions among them.
Confirmation of Differential Gene Expression
Changes in the expression patterns of the selected genes observed in the current microarray study were confirmed using qPCR (Malila et al., 2015). A total of 30 genes were chosen for confirming the microarray data. Primers (Supplementary Table 1) were designed using Primer-BLAST1. Threshold cycle (Ct) was analyzed using BioRad CFX Manager 2.1 software (BioRad). Hypoxanthine-guanine phosphoribosyltransferase (HPRT) was assigned as a reference gene as it showed no differences in expression due to WB development (data not shown). For each gene, relative transcript abundance in WB samples relative to non-WB of the same slaughter age was calculated based on 2–ΔΔCt method (Livak and Schmittgen, 2001) and plot against FC analyzed from microarrays.
Absolute Expression of Genes in Focal Adhesion Signaling Pathway
Absolute expression of eight key genes involved in focal adhesion (FA) signaling pathway was evaluated using an EvaGreen droplet digital polymerase chain reaction (ddPCR) assay of cDNA produced from reverse-transcription reactions. The ddPCR primer sequences are shown in Supplementary Table 1. The 20-μL ddPCR mixture contained 1X EvaGreen® supermix (Bio-Rad Laboratories, Inc., Hercules, CA, United States), 0.25 μM of each forward and reverse primer, and cDNA template. The concentration of the template for each gene was specified in Supplementary Table 1. The template was replaced by an equal volume of nuclease-free water for no template control. The water-in-oil emulsion droplets were generated using a QX100TM droplet generator (Bio-Rad Laboratories, Inc.) according to the manufacturer’s instruction. The droplets were transferred to a 96-well plate, heat sealed and placed into a conventional thermocycler (model T100TM, Bio-Rad Laboratories, Inc.). The reaction conditions were 95°C for 5 min; followed by 40 cycles of 95°C for 30 s, 58°C for 1 min, and 4°C for 5 min; and 90°C for 5 min. After the amplification was completed, the plate was transferred to a QX200TM droplet reader (Bio-Rad Laboratories, Inc.) where fluorescent signal intensity of the droplets was measured. The fluorescence amplitude threshold was set under the high amplitude droplet cluster to distinguish positive and negative droplets. The detected droplets were analyzed in copies per 20-μL reaction by QuantaSoftTM software (Bio-Rad Laboratories, Inc.) and divided by the amount of cDNA added to the reaction to obtain the absolute copy numbers per nanogram of template.
The effects of slaughter age and occurrence of WB condition on absolute expression was analyzed based on 2 × 2 factorial analysis of variance. The significant level was set at α = 0.05. The statistical analysis was performed using the R package version 3.4.3.
Results
Differential Gene Expression in Chicken Skeletal Muscle Associated With Wooden Breast Abnormality
A total of 2,070 transcripts were differentially expressed between non-WB and WB samples (Figure 1). Among the broilers slaughtered at the age of 6 weeks, 509 transcripts were up-regulated, and 401 transcripts were down-regulated in WB in comparison with non-WB samples. As for 7wk, 547 transcripts were up-regulated whereas 648 transcripts were down-regulated upon development of WB myopathy. Top 10 most up-regulated and down-regulated transcripts of the 6wk and 7wk samples are shown in Tables 1, 2, respectively. As illustrated in Venn diagram (Figure 1), only 35 out of 2,070 DE transcripts were identified in both 6 and 7 week comparisons with comparable FC (Table 3), except for the transcript encoding four and a half LIM domains 5 (FHL5) which showed 1.6-fold decrease in 6wk WB but 1.4-fold increase in 7wk WB compared to the expression of their non-WB counterparts. The complete DE transcripts lists are available in Supplementary Table 2.
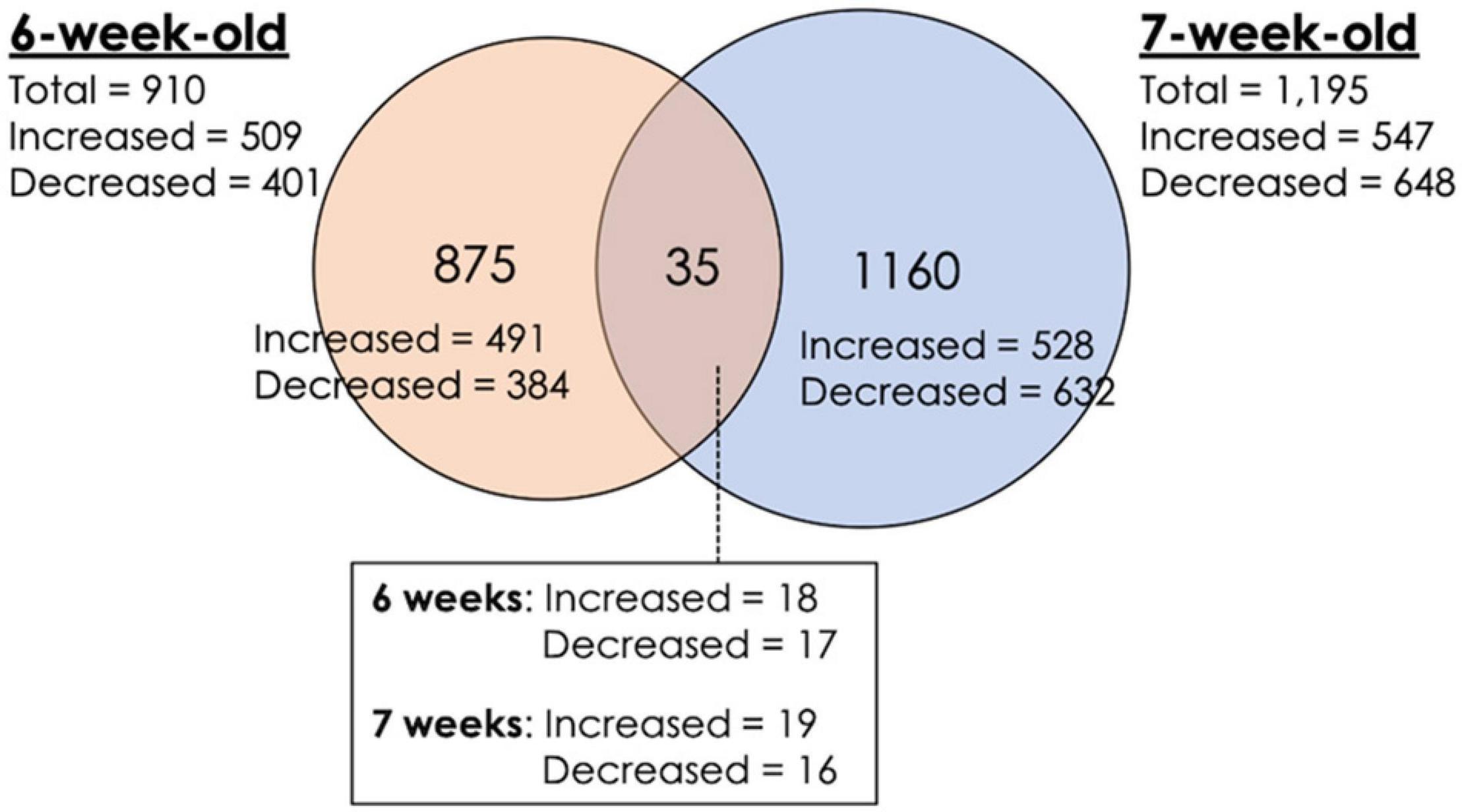
Figure 1. Differential expression of transcripts associated with wooden breast (WB) myopathy in 6-week-old and 7-week-old broilers. Venn diagram depicts number of differentially expressed transcripts obtained from microarray study. Increased expression indicates the greater transcript abundance of the particular genes in WB sample relative to that of non-WB within the same slaughter age, vice versa for decreased expression. The criteria used in identification of differentially expressed transcripts are a combination between false discovery rate ≤ 0.05 and absolute fold change (|FC|) ≥ 1.2.
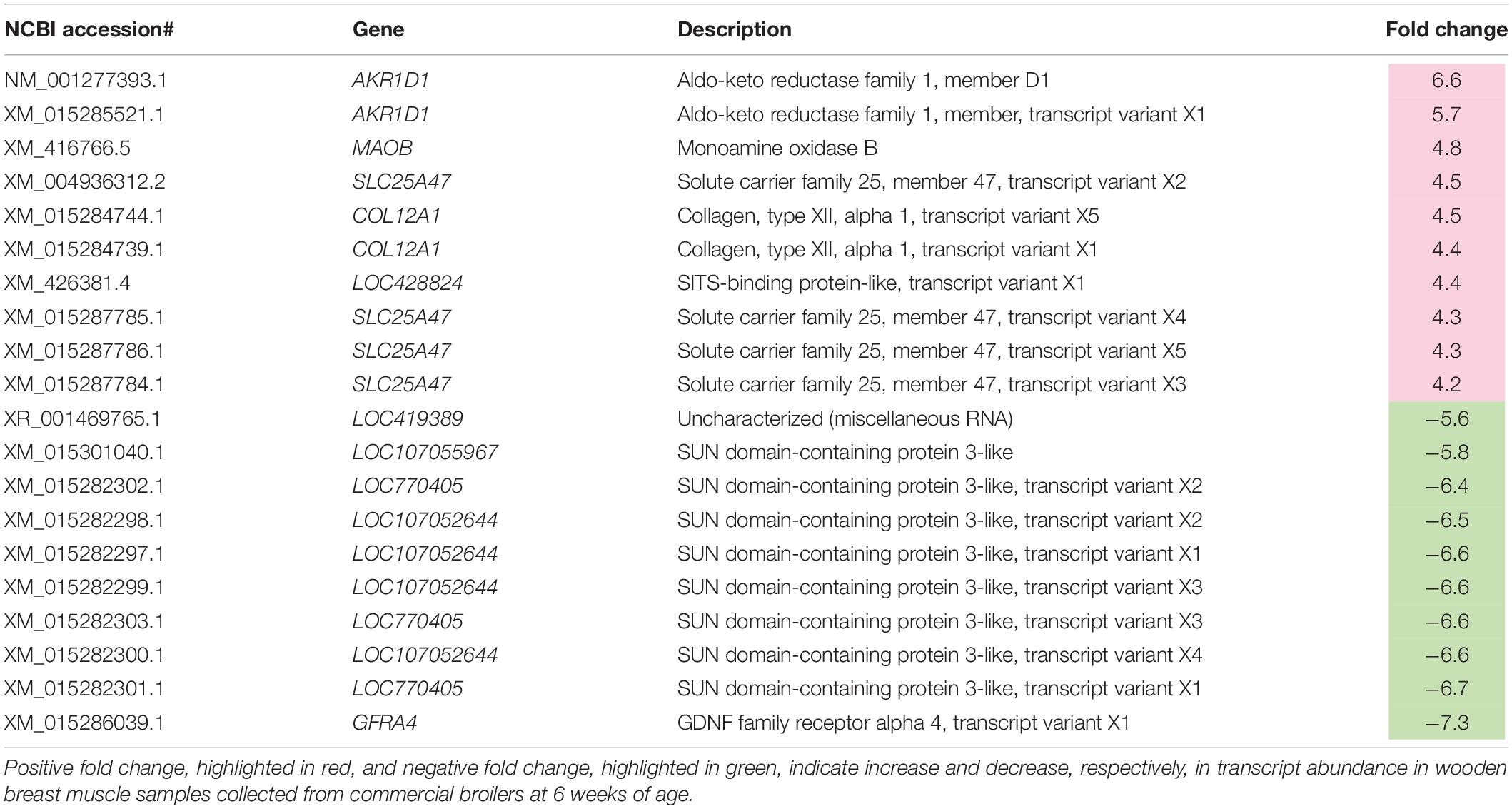
Table 1. Top 10 most differentially expressed transcripts associated with wooden breast in 6-week-old broilers.
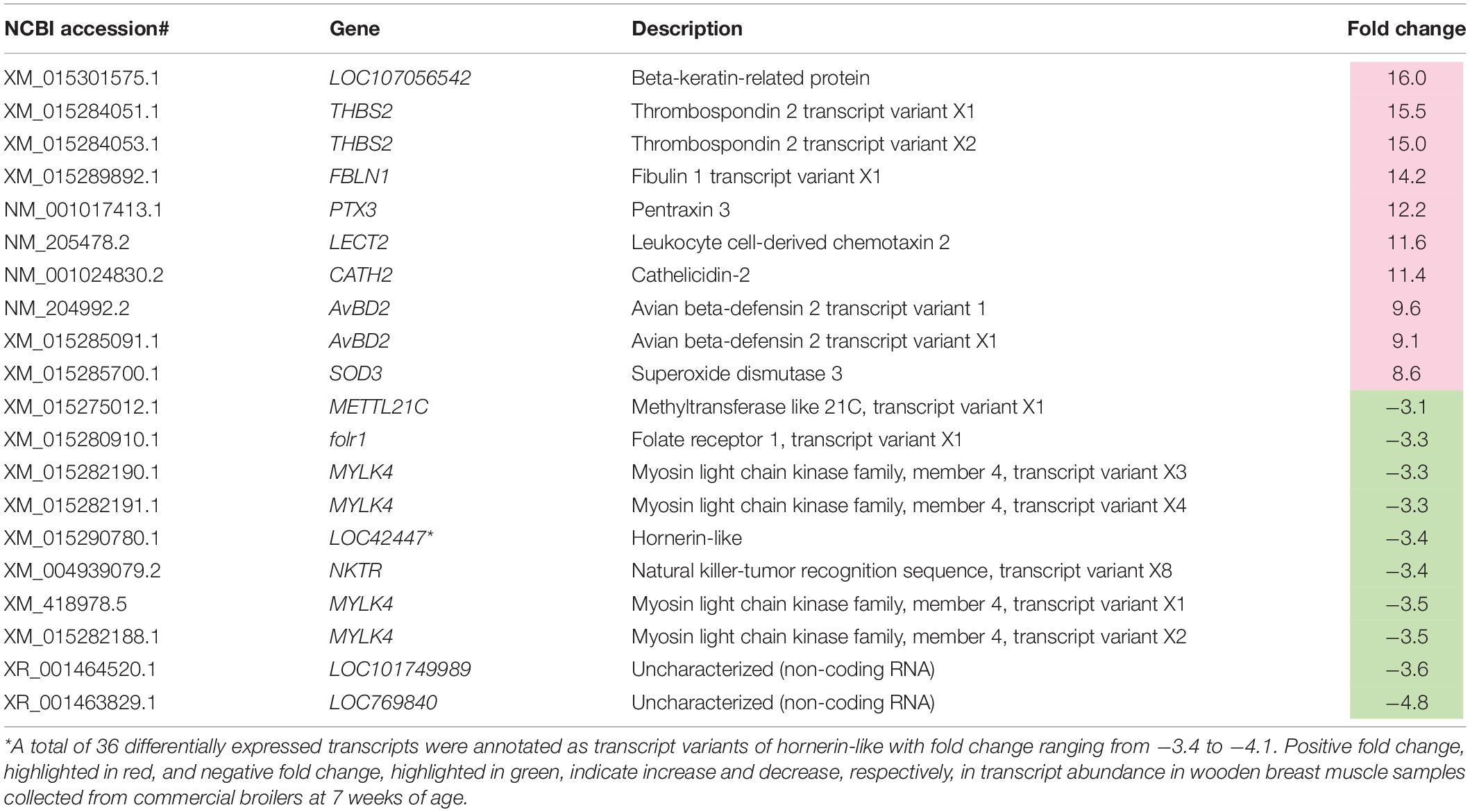
Table 2. Top 10 most differentially expressed transcripts associated with wooden breast in 7-week-old broilers.
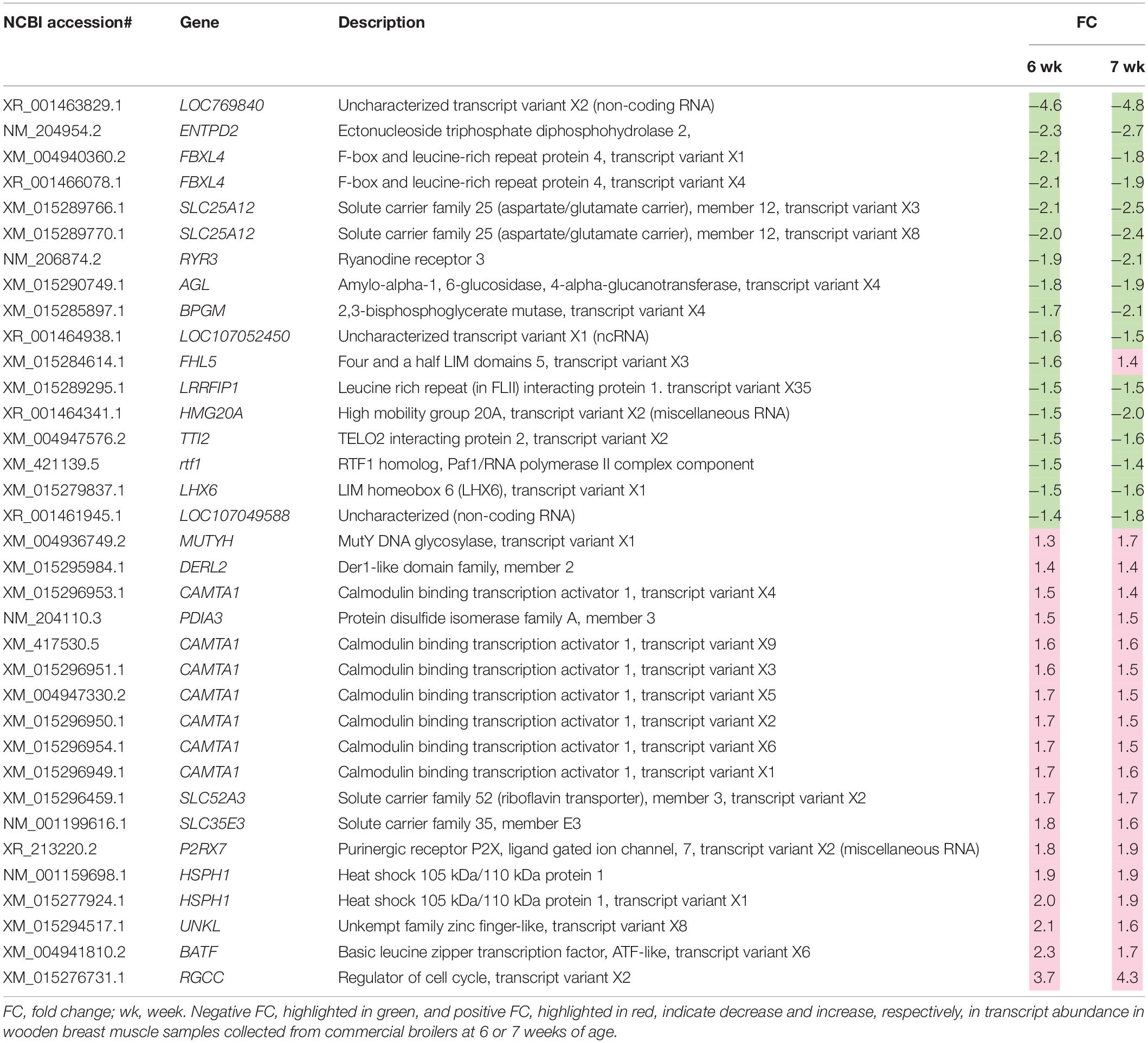
Table 3. Differentially expressed transcripts associated with development of wooden breast myopathies identified in skeletal muscles of both 6-week-old and 7-week-old commercial broilers.
Differential expression from microarray analysis was further confirmed by using a qPCR (Figure 2). As for 6 wk samples, 40 DE transcripts were tested. The findings indicated that 35 counterparts, accounting for 87.5% of the total, exhibited similar trends (Figure 2A). For the 7wk broilers, 84 out of 88 FC values, accounting for 95.5%, obtained from qPCR showed similar trend with the microarray results (Figure 2B).
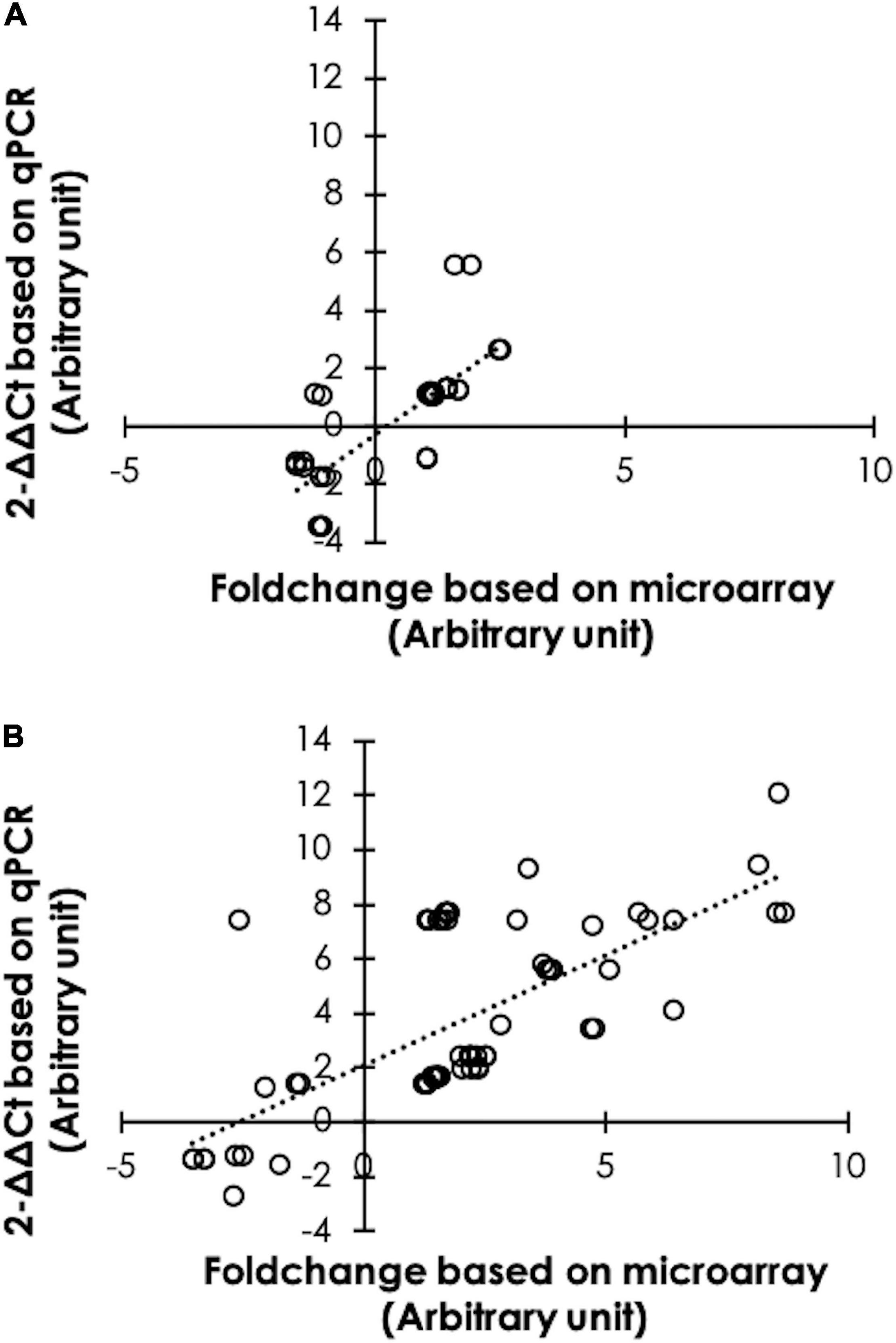
Figure 2. Confirmation of differential expression. Scatter plots illustrate the comparisons between fold changes of 40 differential expressed (DE) transcripts selected from 6-week-old broilers (A), and of 88 DE transcripts from 7-week-old broilers (B) obtained from microarray and quantitative real-time polymerase chain reaction (qPCR). The results indicated that 36 (accounted for 87.5%) and 84 (accounted for 95.5%) counterparts show comparable results between microarray and qPCR.
Functional and Pathway Analysis
Based on pathway analysis (Figure 3), alterations of metabolic pathways, cellular processes, particular transport and catabolism through phagosome, as well as cell communication via tight junction and FA, were highlighted in both 6wk and 7wk broilers. In 6wk group, metabolisms of purine and pyrimidine together with apoptosis, communication among cells through tight junction, gap junction, and FA were enriched altered pathways. Regulation of actin cytoskeleton signaling, mitogen−activated protein kinase (MAPK) signaling, protein processing in endoplasmic reticulum, FA, phagosome, and lysosome were enriched in the 7wk group.
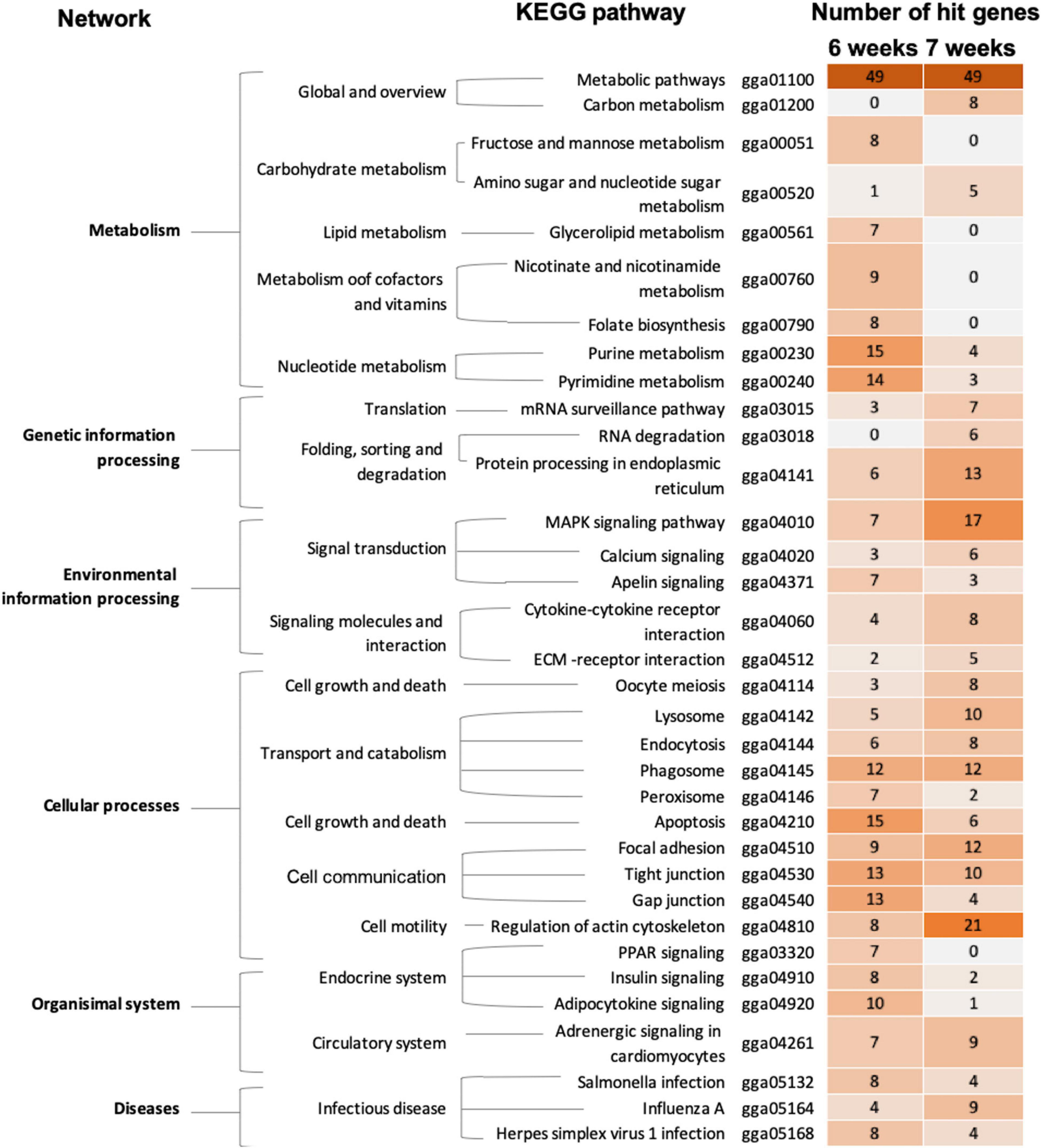
Figure 3. The enriched biological pathways associated with wooden breast (WB) myopathy in 6-week-old and 7-week-old broilers. Heatmap depicts the enriched altered biological pathways, which were further grouped into biological networks, based on KEGG database. The darker color indicated more numbers of differentially expressed genes mapped into the pathway.
Changes in Absolute Expression of Key Genes in Focal Adhesion Signaling Pathway
Absolute expressions of eight genes in FA signaling were further elucidated using ddPCR (Figure 4). For 6 wk, only two genes, including integrin subunit alpha 8 (ITGA8, FC = 2.0) and protein tyrosine kinase 2 (PTK2, FC = −2.0), were found differentially expressed upon development of WB abnormality (p < 0.05). On the other hand, increases in actinin-1 (ACTN1, FC = 3.7), integrin-linked kinase (ILK, FC = 1.3), ITGA8 (FC = 2.4), integrin beta subunit 5 (ITGB5, FC = 2.3), and talin 1 (TLN1, FC = 1.6) were observed in 7wk WB muscles in respect to their non-WB counterparts (p < 0.05). In addition, effects of different slaughtered ages on absolute transcript levels in WB muscles were detected for ACTN1, PXN, and VCL whereas such effects in non-WB muscles were observed for ILK, and VCL (p < 0.05).
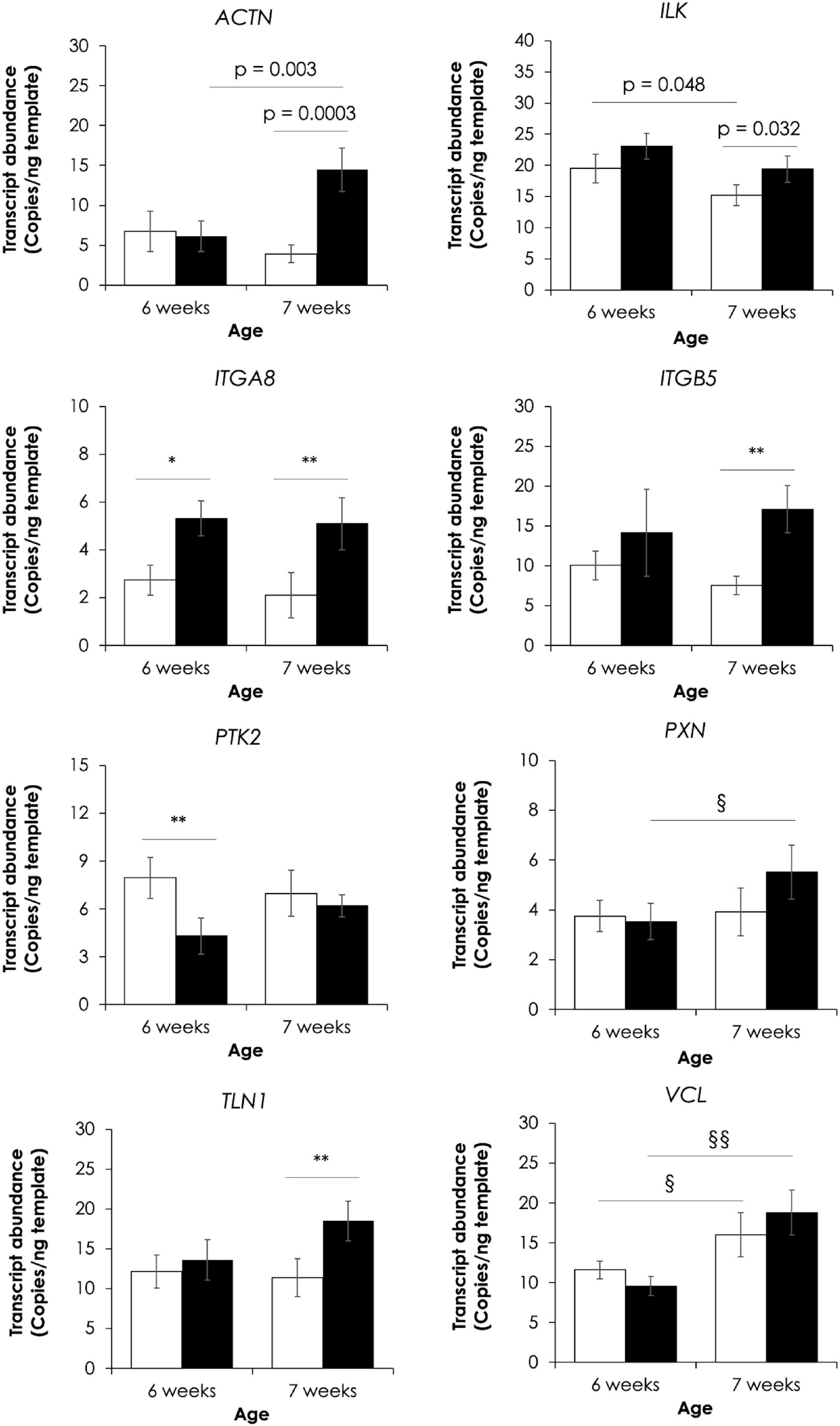
Figure 4. Effects of wooden breast myopathy on absolute expression of eight genes in focal adhesion signaling. The genes include actinin-1 (ACTN1), integrin-linked kinase (ILK), integrin subunit alpha 8 (ITGA8), integrin subunit beta 5 (ITGB5), protein tyrosine kinase 2 (PTK2), paxillin (PXN), talin 1 (TLN1), and vinculin (VCL). Bars and error bars depict average and standard error in copies per nanogram cDNA template. *p < 0.05, **p < 0.01 between non-WB and WB samples. §p < 0.05, §§p < 0.01 between different ages.
Discussion
Six-Week-Old Broilers
Metabolic processes of carbohydrates, glycerolipids, cofactors and vitamins, and nucleotides (i.e., purine and pyrimidine) were identified as enriched pathways associated with the development of WB in 6wk broilers (Table 4 and Figure 3). These findings are in agreement with those obtained in previous studies performed on WB muscles (Mutryn et al., 2015; Abasht et al., 2016; Zambonelli et al., 2016). In detail, among the DE transcripts listed in the pathways (Supplementary Table 3), aldo-keto reductase (ARK), family 1 member D1 (AKR1D1, FC = 6.6) was also listed as the top up-regulated genes in 6wk WB if compared to their unaffected counterparts. Another gene encoding ARK family 1 member 10 (ARK1B10) was also increased for 2.6-fold. The ARK superfamily catalyzes oxidation-reduction reactions to detoxify aldehydes and ketones generated during metabolisms or in response to stresses (Huang et al., 2016). Increased expressions of those enzymes could be a consequence of the muscle fibers encountering stressful conditions associated with WB myopathy. In addition, an overexpression of ARK1B10 was shown to elevate β-catenin, an activator of canonical Wnt signaling, in breast cancer cell lines (Qu et al., 2019). Within this context, the findings of the present study further support the hypothesis formulated by Phillips et al. (2020) in which, in the presence of WB myopathy, satellite cells could lose their capability of proliferation and differentiation due in part to an excessive activation of Wnt signaling pathway that, ultimately, results in the disruption of the muscle repair process.
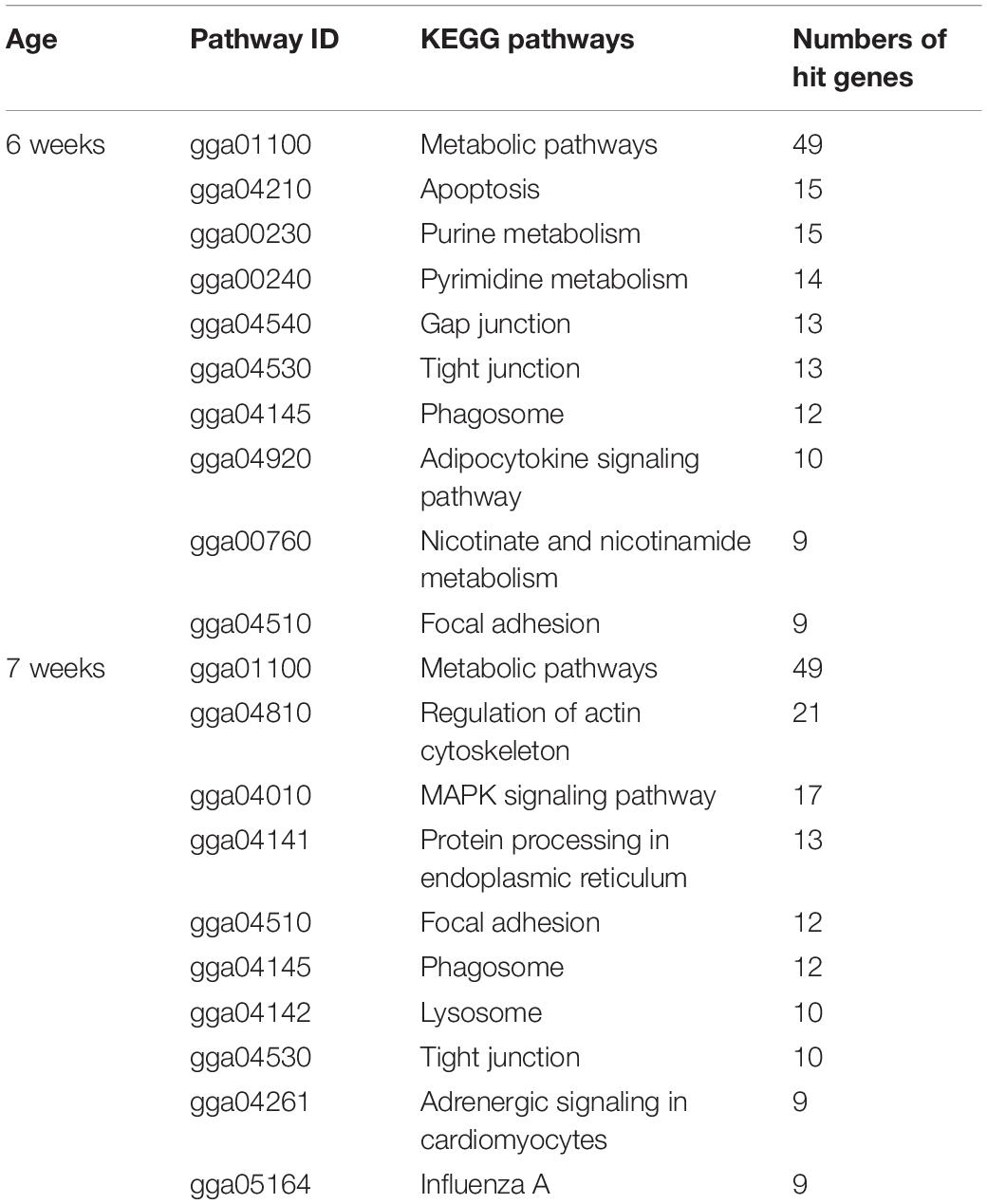
Table 4. Top 10 enriched biological pathways associated with development of wooden breast myopathies in 6-week-old and 7-week-old commercial broilers.
Grouped in the category of endocrine signaling, alteration of insulin, peroxisome-proliferator-activated receptors (PPAR), and adipocytokine signaling were more pronounced in the 6wk broilers compared to 7wk samples (Figure 3). Dysfunction of those signaling pathways caused by, or in association with, excess adipose tissues and insulin resistance leads to negative metabolic effects (Mashek et al., 2007; Contreras et al., 2019). Lake and Abasht (2020) addressed the resemblance between metabolic complications and muscle structural features of WB myopathy in broilers and that of diabetes in smooth and cardiac muscles of mammals. In this study (Supplementary Table 3), expressions of long-chain acyl-CoA ligase 1 (ACSL1), encoding the enzyme catalyzing conversion of fatty acid to acyl-CoA, and solute carrier family 27 member 2 (SLC27A2), which encodes fatty acid transporter protein (FATP), were reduced in WB at both ages for 1.7 and 1.9-fold, respectively, compared with those of non-WB samples. These results indicated a decreased β-oxidation likely associated with the accumulation of long-chain and very-long chain fatty acids (Mashek et al., 2007) in WB broilers which were well in agreement with the compromised glucose and lipid metabolisms, impaired energy production and fat deposition observed in the affected muscle (Abasht et al., 2016; Lake et al., 2019; Malila et al., 2019; Papah and Abasht, 2019; Lake and Abasht, 2020). Such impairment was further emphasized by an increased transcript abundance of the gene encoding 5′-AMP-activated protein kinase (AMPK) subunit beta-1 (PRKAB1, FC = 1.7), the conserved domain which acts as a positive regulator of AMPK complex (Supplementary Table 3).
Apart from metabolism of biochemical molecules, pathway enrichment analysis also reveals the manifestation of apoptosis in 6wk WB-affected broilers (Table 4). An impaired programmed cell death in the birds exhibiting growth-related myopathies was previously addressed (Malila et al., 2020; Phillips et al., 2020). In the present study, increased transcriptional levels of cathepsin B (CTSB, FC = 2.0), α-tubulin 1C (TUBA1C, FC = 1.7) along with a decrease in serine/threonine protein kinase B (AKT3, FC = −1.3) were observed in 6 week WB samples. The up-regulation of CTSB was previously associated with an enhanced activity of cathepsin B and L in WB breasts measured 12 h postmortem (Hasegawa et al., 2020). In addition, Mizunoe et al. (2020) recently reported that an overexpressed CTSB caused dysfunction of lipid metabolism in obese white adipose tissue potentially through a chronic state of low-grade inflammation, which was relevant to the condition of WB myopathy. Tubulins, dynamic polymers of microtubules, play important roles in various cellular functions through continual cytoskeletal reorganization in response to developmental and environmental cues (Ambriz et al., 2018). Chemical suppression of microtubules dynamic affected signaling processes that ultimately interfered apoptosis (Mollinedo and Gajate, 2003). It is interesting that Lake et al. (2019) reported an almost 1.7-fold decreased expression of gene encoding α-tubulin 8b in 2-week-old chicks that later (at 7 weeks of age) exhibited WB abnormality in comparison with unaffected broilers (Lake et al., 2019). The opposite directional changes of TUBA expression observed in a previous as well as in the current study might be an implication of a difference in the microenvironment at the different stage of WB myopathy (Ambriz et al., 2018). In addition, knockdown of AKT3 was shown to limit cell proliferation and attenuated mammalian target of rapamycin (mTOR) signaling in the brain of the mouse (Easton et al., 2005), suggesting that the mediated apoptosis in 6wk WB muscle might be attributed to a modified mTOR signaling.
The other enriched altered pathways in the 6wk broilers included gap junction, tight junction and FA (Table 4), which are the adhesive structures of cell adhesion molecules in the cell surface that regulate of cell polarity and cell-cell communication (Rao and Wu, 2021). Dysregulation of those cell adhesions together with extracellular matrix (ECM) promotes pathological conditions associated with several diseases in humans (Rao and Wu, 2021). The DE transcripts enlisted in those pathways (Supplementary Table 3) include TUBA1C (FC = 1.7), Platelet-derived growth factor (PDGF) subunit A (PDGFA, FC = −1.4), and subunit B (PDGFB, FC = −1.5), serine/threonine-protein phosphatase PP1 catalytic subunit (LOC100858156, FC = −1.4), AKT3 (FC = −1.3) and laminin gamma 1 (LAMC1, FC = −1.3). PDGF is considered one of the growth factors regulating cell proliferation, differentiation and angiogenesis through the binding with its receptor located on cell surface (Okonkwo et al., 2020). Up-regulations of its PDGF receptor (PDGFR) at gene level were addressed to be associated with increased fibrosis in WB fillets compared with non-WB counterparts (Papah et al., 2018; Pampouille et al., 2019). Although no differences in transcriptional level of PDGFR were identified in the 6wk WB broilers, we observed, in the affected muscles, a reduction of both PDGFA and PDGFB, which in mice has been reported to disrupt the wound repair process potentially through the perturbation of angiogenesis as previously observed in diabetic skin wound healing (Beer et al., 1997; Okonkwo et al., 2020). Furthermore, reduced expression of LAMC1 was previously reported in patients with abnormal coronary atherogenesis (Hebbel et al., 2020), suggesting maladaptive activities of the cells when LAMC1 expression was low. The importance of LAMC1 in WB myopathy was recently evidenced in the study of Bordini et al. (2021) as it was defined as one of the hub genes interconnecting the DE gene network in WB and WS affected broilers.
Overall, the intensive perturbations of metabolic processes observed in 6wk broilers, with special reference to glucose and lipid metabolisms, are pronounced in WB broilers along with the convergence of cell-cell communication that regulates various cellular activities, including the processes of muscle repair.
Seven-Week-Old Broilers
Metabolic pathway was one of the top enriched altered pathways observed in 7wk broilers (Table 4). Considering the DE transcripts listed in the metabolic pathways (Supplementary Table 4), however, the DE transcripts in such pathway were different from those observed in 6 week birds. Indeed, for 7wk samples, a number of those DE transcripts was involved in oxidative phosphorylation, and metabolisms of amino acids (argininosuccinate synthase, ASS1, FC = 2.5), amino sugars (glutamine-fructose-6-phosphate transaminase, GFPT1, FC = 2.0; sialic acid synthase, NANS, FC = 1.7; hexosaminidase, HEXB, FC = 2.1), proteoglycans (alpha-1,2-mannosyltransferase, ALG11, FC = −2.7; ALG12, FC = 1.9; alpha-1,6-mannosylglycoprotein 6-beta-N-acetylglucosaminyltransferase A, MGAT5, FC = 1.3; carbohydrate 4-sulfotransferase 9, CHST9, FC = 1.9), and precursor molecules required for biosynthesis and function of lysosomes (acid ceramidase, ASAH1, FC = 1.6; adenosine triphosphatase, ENTPD2, FC = −2.7). Regarding the biological roles of those DE transcripts, the profound disruption in the molecular events responsible for cell communication, cell transportation, and catalytic processes in the 7wk WB-affected samples is anticipated. The speculation is supported by the presence of phagosome, lysosome, and FA, regulation of actin cytoskeleton, apoptosis, endocytosis and ECM-receptor interaction in the enriched altered pathways (Table 4 and Figure 3). In addition, mitogen-activated protein kinase (MAPK) signaling, protein processing in endoplasmic reticulum, and cytokine-cytokine receptor interaction were among those enriched pathways.
The molecular events in the 7wk affected broilers were corresponded with the perturbed processes of muscle regeneration (Domingues-Faria et al., 2016). Expression of C-C motif chemokine ligand 19 (CCL19) was increased for 3.7-fold in 7wk WB-affected broilers if compared to their non-WB counterpart, corresponding with the findings of Hughes et al. (2020) in which up-regulated CCL19 was identified in human skins in response to inflammation. CCL19 binds to C-C chemokine receptor (CCR) type 7 (CCR7), playing roles in trafficking of immune cells (Yoshida et al., 1997). Cathepsin S (CTSS), another lysosomal protease participating in degradation of ECM and inflammatory processes, was increased for more than 2.4-fold in 7wk WB-affected broilers. Increased expression of CTSS was demonstrated in response to the secreted pro-inflammatory cytokines (Fu et al., 2020). Elevated abundances of CC7 (FC = 2.3), CCR5 (FC = 2.1), and tumor necrosis factor receptor superfamily member 18 (TNFRSF18, FC = 2.4), and neutrophil cytosolic factor 4 (NCF4, FC = 2.0) were observed in the affected birds. Overall, these findings supported the recruitment of immune cells to the affected breast muscle.
It is evident that the main components of cytoskeleton were manifested under WB condition. Actin gamma 1 (ACTG1, FC = 1.8), beta-keratin related protein (LOC107056542, FC = 16.0), α-tubulin 3 (LOC416695, FC = 3.0), and α-tubulin 4a (TUBA4A, FC = −1.5), the consisting proteins in those structures, were differentially expressed between WB and non-WB samples in the 7 week broilers (Supplementary Table 2). Muscle RAS oncogene homolog (MRAS), a member of small G proteins responsible for signal transduction and reorganization of actin cytoskeleton (Matsumoto et al., 1997; Kimmelman et al., 2002), was increased for 3.6-fold in the abnormal samples. Decreased transcript abundances of fibroblast growth factor 16 (FGF16, FC = −2.7) and son of sevenless (SOS2, FC = −1.5), Ras-guanine exchange factor, were detected in the 7wk affected broilers. As observed in the previous study of Sofronescu et al. (2010), activation of FGF16 at transcriptional level was essential for cardiac growth and postnatal development of human and mice while Guittard et al. (2015) reported limited peripheral T-cell migration, hence impairing immune response when SOS2 was absent.
Regarding the altered processes of cell communication (Table 4), transcript abundance of the gene encoding for one of the epidermal growth factor receptors (EGFR) belonging to the ErbB family of receptor tyrosine kinases, ERBB2, was decreased for 1.5-fold in respect to WB myopathy. This receptor is involved in the stabilization of peripheral microtubules in cell adhesion, triggering a rich network of signaling pathways (Yarden and Sliwkowski, 2001). Fibronectin 1 (FN1) was up-regulated for 2.7-fold in WB-affected 7wk broilers. Widely distributed in inflammatory lesions, fibronectins, a group of fibroblast surface glycoproteins, generally binds to ECM proteins, were also found to play a major role during wound healing (Grinnell et al., 1981). An extensive localization of FN1 at endomysial and perimysial levels was found in WB coupled with an increased content of this glycoprotein (Praud et al., 2020). In addition, grouped in phagosome, FA (Table 4), and ECM receptor interaction (Supplementary Table 4), thrombospondin 2 (THBS2), disulfide-linked homotrimeric glycoprotein, was highlighted as one of the most highly increased transcripts (FC = 15) in 7wk WB-affected broilers if compared to their non-WB counterparts (Table 2). This transient matrix glycoprotein inhibits microvascular endothelial cells proliferation thus resulting in impaired angiogenetic processes and limiting vascularization (Zhang et al., 2020). Intriguingly, these features overlap with the microscopic traits observed in WB affected muscles (Abasht et al., 2021).
A profound impairment of muscle regeneration in 7wk WB-affected broilers was further supported by the expression levels of genes responsible for protein processing within the endoplasmic reticulum (ER, Table 4 and Figure 3). Indeed, increased expressions of genes encoding for several chaperone proteins, including heat shock protein (HSP) 110 kDa (HSPA4L, FC = 1.5) and 70 kDa (HSPA5, FC = 1.7) were found. In particular, a 1.5-fold increase in protein disulfide-isomerase A3 (PDAI3) was found in WB muscles (Supplementary Table 4). This multifunctional chaperone is originally located within ER but can escape to cell surface and interacts with integrins. Wang et al. (2020) recently demonstrated that a chemical inhibition of PDIA3 reduces myoblast differentiation in injured skeletal muscle, ultimately impairing muscle regeneration. An activation of ER-associated degradation, the process to eliminate misfolded proteins retained within the ER lumen through the proteasome to restore normal ER function, might be speculated. Under severe stress condition in which ER function was not recovered, the affected cells were entered programmed cell death.
The impaired muscle regeneration in 7wk affected broilers perfectly matches with the altered muscular structure observed associated to WB condition (Velleman, 2020). In this study, a decreased transcript abundance of myopalladin (MYPN, FC = −1.4) and myomesin 1 (MYOM1, FC = −1.7) together with increased expressions of several genes encoding collagens (COL1A1, FC = 2.1, COL8A1, FC = 2.0, COL9A2, FC = 2.2, LOC101750377, FC = 2.1) were found in 7wk affected broilers in comparison with non-WB ones (Supplementary Table 2). MYPN tethers nebulin to actinin at Z-line, maintaining sarcomeric integrity (Bang et al., 2001) whereas MYOM1, a major component of myofibrillar M band expressed in almost all striated muscles, stabilizes the three-dimensional structure of the thick filaments by linking together myosin, titin, and light meromyosin (Agarkova and Perriard, 2005). Overall, gene expression is in agreement with the severe disruption of muscular structure coupled with accumulation of collagen observed in WB muscle (Velleman, 2020).
Collectively, differential expression of those genes underlined an extensive molecular alteration among the biological networks playing roles in response to severe muscle damage in the 7wk WB-affected broilers.
Transcriptional Modification of Focal Adhesion Signaling
The main enriched biological pathways associated with the development of WB myopathy in 6wk and 7wk was somewhat different. The molecular disruption in 6wk was more toward metabolic pathways for glucose and lipids whereas an aberrant muscle repair process has a more relevant importance in 7wk samples. Aside from that, the presence of FA signaling was similarly found in both groups, suggesting the relevance of this process in WB development. Here, we further compared absolute transcript abundances of the eight protein components of FA signaling between non-WB and WB samples within each slaughter age using ddPCR (Figure 4). The ddPCR technique permits the transcriptional quantification with good precision and sensitivity (Taylor et al., 2017). Therefore, the results could extend the comparative analysis of microarray which normally showed some limitation based on fixed dynamic range of fluorescence (Sharov et al., 2004). Increases or decreases in transcript abundance detected by both microarray and ddPCR studies in 6wk and 7wk were summarized in Figure 5.
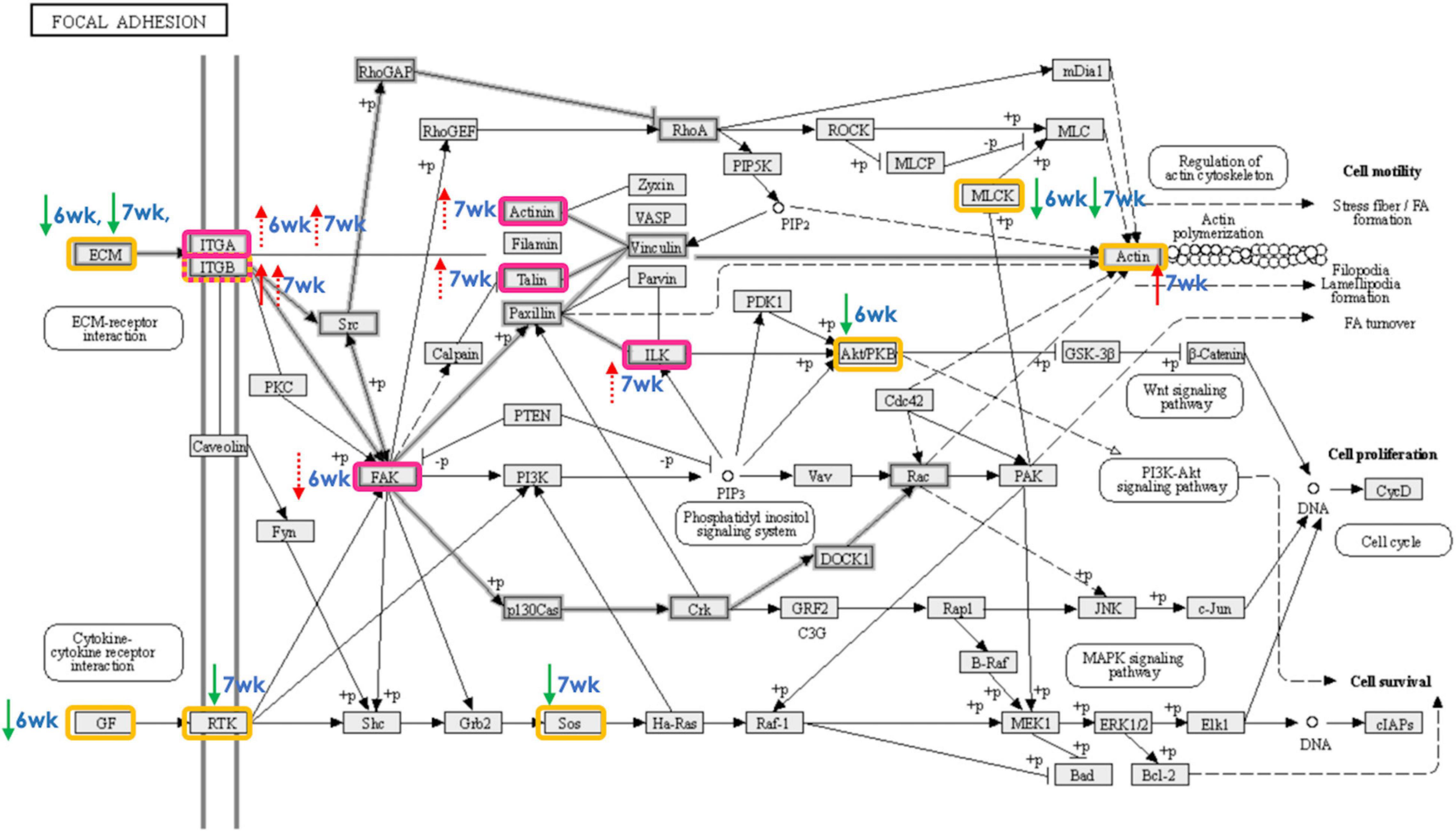
Figure 5. Focal adhesion signaling pathway as affected by development of wooden breast (WB) in 6-week-old and 7-week-old broilers. The proteins highlighted in yellow and pink boxes exhibited differential expression, as detected using microarray and droplet digital polymerase chain reaction, respectively, in skeletal muscle of broilers affected WB myopathy in comparison with their non-defective counterparts. The red and green arrows (solid = microarray, dashed = ddPCR) indicate the increased and decreased expression, respectively, of the gene-encoded protein in WB relative to non-WB of 6-week-old (6wk) or 7-week-old (7wk) broilers.
Focal adhesion, the integrins-mediated contact sites between cell and ECM, acts as the linking points that mediates mechanical and biochemical signaling from the ECM (Turner, 2000; Wozniak et al., 2004; Huveneers and Danen, 2009). The reorganization of actin cytoskeletal structure regulated by FA signal leads to a series of intracellular events in response to the extracellular initiator. The crosstalk is crucial for controlling several cellular mechanisms, from fundamental development through recovery from any injuries or infection, and, in turn, maintaining tissue homeostasis, thus organism’s survival (Zaidel-Bar and Geiger, 2010). A disrupted FA signaling accompanied by defective ECM and insufficient angiogenesis, which results in poor tissue oxygenation (condition consistently observed within the breast muscles of commercial broilers exhibiting growth-related myopathies), was associated with the impaired wound healing that led to an aberrant deposition of ECM molecules (e.g., collagen and decorin) and ultimately resulting in fibrosis (Koivisto et al., 2014).
Corresponded with the current results, up-regulated ITGA8 was previously reported in broiler breast muscle affected by WS abnormality (Marchesi et al., 2019), and in specimen of 90 patients with hepatic fibrosis (Nishimichi et al., 2021). In addition, the same authors found that ITGA8 was minimally expressed in healthy liver, underlying its relevance with liver fibrosis. Induced ITGB5 was found to directly interact with β-catenin, facilitating Wnt/β-catenin activity in hepatocellular carcinoma (Lin et al., 2018). Deletion of FAK in mouse liver accelerated liver regeneration through the increased TNFα as well as the activation of apoptosis (Shang et al., 2016). Decreased PTK2, gene encoding for FAK, found in 6wk WB broilers may be the result of an adaptive response through FAK-mediated FA to accelerate the removal of damaged cells through apoptosis, and partly mitigate the development of fibrosis within the muscle (Lagares et al., 2012; Velleman, 2020).
Reduced transcript abundances of genes encoding for ECM components (LAMC1 in 6wk, FN1 in 7wk), and growth factors (PDGFA in 6wk) were identified, based on the current microarray study, in the WB-affected muscles. In contrast, the majority of the genes encoding for FA components tested exhibited increased expression levels. Those genes encode intracellular proteins that mediate integrin binding to actin microfilament, maintain stability of the integrin adhesion, and sense matrix rigidity (Klapholz and Brown, 2017). Most of the transcriptional changes in this pathway were detected in 7wk broilers. The findings suggested an altered crosstalk between immune cell and muscle cells may be hypothesized as a consequence of the chronic inflammatory processes, leading to failure in muscle regeneration (Domingues-Faria et al., 2016).
In conclusion, our findings were congruent with the proposed model of ECM disorders in the pathogenesis of growth-related myopathies in broilers (Bordini et al., 2021). The present study further provides an observation on the alteration of ECM-mediated FA signaling. Such impairment exerted the deleterious effects on immune response and muscle regeneration process at a greater extent in 7wk broilers. Furthermore, molecular perturbation pattern associated with WB development differed between 6wk and 7wk birds. In 6wk broilers, the metabolic processes of glucose and lipid, in particular, were deviated between WB and non-WB group. On the other hand, the importance of impaired muscle regeneration processes was evident in the 7wk affected broilers. Whether the altered FA signaling was a cause or effects of those dysregulated biological pathways remained to be further investigated.
Data Availability Statement
The datasets presented in this study can be found in online repositories. The names of the repository/repositories and accession number(s) can be found below: https://www.ncbi.nlm.nih.gov/, GPL24307; https://www.ncbi.nlm.nih.gov/, GSE107362.
Ethics Statement
Ethical review and approval was not required for the animal study because all samples used in this study were purchased in the form of whole carcasses from the commercial processing plant without any experimental treatments subjected to the living animals. Thereby, the ethical approval was exempted.
Author Contributions
YM, WR, and WV conceived and designed the experiments as well as acquired the funding. YM, TU, YS, SA, and KT performed the experiments and analyzed the data. TU performed bioinformatics. KT performed ddPCR. YM drafted the manuscript with revisions provided by TU, WR, MP, and FS. All authors read and approved the final manuscript.
Funding
This research was financially supported by Cluster and Program Management, National Science and Technology Development Agency (Thailand; project number P15-50668), and by Office of the Permanent Secretary, Ministry of Higher Education, Science, Research and Innovation (Thailand; P20-50946 and P21-50165).
Conflict of Interest
The authors declare that the research was conducted in the absence of any commercial or financial relationships that could be construed as a potential conflict of interest.
Acknowledgments
The authors would like to acknowledge Juthawut U-chupaj and Premask Chaiwiwattrakul for their assistance during sample collection.
Supplementary Material
The Supplementary Material for this article can be found online at: https://www.frontiersin.org/articles/10.3389/fphys.2021.691194/full#supplementary-material
Footnotes
References
Abasht, B., Mutryn, M. F., Michalek, R. D., and Lee, W. R. (2016). Oxidative stress and metabolic perturbations in wooden breast disorder in chickens. PLoS One 11:e0153750. doi: 10.1371/journal.pone.0153750
Abasht, B., Papah, M. B., and Qiu, J. (2021). Evidence of vascular endothelial dysfunction in Wooden Breast disorder in chickens: insights through gene expression analysis, ultra-structural evaluation and supervised machine learning methods. PLoS One 16:e0243983. doi: 10.1371/journal.pone.0243983
Agarkova, I., and Perriard, J.-C. (2005). The M-band: an elastic web that crosslinks thick filaments in the center of the sarcomere. Trends Cell Biol. 15, 477–485. doi: 10.1016/j.tcb.2005.07.001
Ambriz, X., de Lanerolle, P., and Ambrosio, J. R. (2018). The mechanobiology of the actin cytoskeleton in stem cells during differentiation and interaction with biomaterials. Stem Cells Int. 2018:2891957. doi: 10.1155/2018/2891957
Bang, M. L., Mudry, R. E., McElhinny, A. S., Trombitás, K., Geach, A. J., Yamasaki, R., et al. (2001). Myopalladin, a novel 145-kilodalton sarcomeric protein with multiple roles in Z-disc and I-band protein assemblies. J. Cell. Biol. 153, 413–427. doi: 10.1083/jcb.153.2.413
Barbut, S. (2019). Recent myopathies in broiler’s breast meat fillets. Worlds Poult. Sci. J. 75, 559–582. doi: 10.1017/S0043933919000436
Beer, H.-D., Longaker, M. T., and Werner, S. (1997). Reduced expression of PDGF and PDGF receptors during impaired wound healing. J. Invest. Dermatol. 109, 132–138. doi: 10.1111/1523-1747.ep12319188
Bolstad, B. (2017). PreprocessCore: A Collection of Pre-Processing Functions. R Package Version 1.40.0. Available online at: https://github.com/bmbolstad/preprocessCore (accessed October 23, 2019).
Bordini, M., Zappaterra, M., Soglia, F., Petracci, M., and Davoli, R. (2021). Weighted gene co-expression network analysis identifies molecular pathways and hub genes involved in broiler White Striping and Wooden Breast myopathies. Sci. Rep. 11:1776. doi: 10.1038/s41598-021-81303-7
Cai, K., Shao, W., Chen, X., Campbell, Y. L., Nair, M. N., Suman, S. P., et al. (2018). Meat quality traits and proteome profile of woody broiler breast (pectoralis major) meat. Poult. Sci. 97, 337–346. doi: 10.3382/ps/pex284
Chatterjee, D., Zhuang, H., Bowker, B. C., Sanchez-Brambila, G., and Rincon, A. M. (2016). Instrumental texture characteristics of broiler pectoralis major with the wooden breast condition. Poult. Sci. 95, 2449–2454. doi: 10.3382/ps/pew204
Contreras, O., Cruz-Soca, M., Theret, M., Soliman, H., Tung, L. W., Groppa, E., et al. (2019). Cross-talk between TGF-β and PDGFRα signaling pathways regulates the fate of stromal fibro-adipogenic progenitors. J. Cell Sci. 132:jcs232157. doi: 10.1242/jcs.232157
Daughtry, M. R., Berio, E., Shen, Z., Suess, E. J. R., Shah, N., Geiger, A. E., et al. (2017). Satellite cell-mediated breast muscle regeneration decreases with broiler size. Poult. Sci. 96, 3457–3464. doi: 10.3382/ps/pex068
de Carvalho, L. M., Madruga, M. S., Estévez, M., Badaró, A. T., and Barbin, D. F. (2020). Occurrence of wooden breast and white striping in Brazilian slaughtering plants and use of near-infrared spectroscopy and multivariate analysis to identify affected chicken breasts. J. Food Sci. 85, 3102–3112. doi: 10.1111/1750-3841.15465
Domingues-Faria, C., Vasson, M.-P., Goncalves-Mendes, N., Boirie, Y., and Walrand, S. (2016). Skeletal muscle regeneration and impact of aging and nutrition. Ageing Res. Rev. 26, 22–36. doi: 10.1016/j.arr.2015.12.004
Easton, R. M., Cho, H., Roovers, K., Shineman, D. W., Mizrahi, M., Forman, M. S., et al. (2005). Role for Akt3/protein kinase Bgamma in attainment of normal brain size. Mol. Cell Biol. 25, 1869–1878. doi: 10.1128/MCB.25.5.1869-1878.2005
Ferreira, T. Z., Kindlein, L., Flees, J. J., Shortnacy, L. K., Vieira, S. L., Nascimento, V. P., et al. (2020). Characterization of pectoralis major muscle satellite cell population heterogeneity, macrophage density, and collagen infiltration in broiler chickens affected by wooden breast. Front. Physiol. 11:529. doi: 10.3389/fphys.2020.00529
Fu, R., Guo, H., Janga, S., Choi, M., Klinngam, W., Edman, M. C., et al. (2020). Cathepsin S activation contributes to elevated CX3CL1 (fractalkine) levels in tears of a Sjögren’s syndrome murine model. Sci. Rep. 10:1455. doi: 10.1038/s41598-020-58337-4
Grinnell, F., Billingham, R. E., and Burgess, L. (1981). Distribution of fibronectin during wound healing in vivo. J. Invest. Dermatol. 76, 181–189. doi: 10.1111/1523-1747.ep12525694
Guittard, G., Kortum, R. L., Balagopalan, L., Çuburu, N., Nguyen, P., Sommers, C. L., et al. (2015). Absence of both Sos-1 and Sos-2 in peripheral CD4(+) T cells leads to PI3K pathway activation and defects in migration. Eur. J. Immunol. 45, 2389–2395. doi: 10.1002/eji.201445226
Hasegawa, Y., Hara, T., Kawasaki, T., Yamada, M., Watanabe, T., and Iwasaki, T. (2020). Effect of wooden breast on postmortem changes in chicken meat. Food Chem. 315:126285. doi: 10.1016/j.foodchem.2020.126285
Hebbel, R. P., Wei, P., Milbauer, L., Corban Michel, T., Solovey, A., Kiley, J., et al. (2020). Abnormal endothelial gene expression associated with early coronary atherosclerosis. J. Am. Heart Assoc. 9:e016134. doi: 10.1161/JAHA.120.016134
Huang, L., He, R., Luo, W., Zhu, Y.-S., Li, J., Tan, T., et al. (2016). Aldo-keto reductase family 1 member B10 inhibitors: potential drugs for cancer treatment. Recent Pat. Anticancer Drug Discov. 11, 184–196. doi: 10.2174/1574892811888160304113346
Hughes, T. K., Wadsworth, M. H., Gierahn, T. M., Do, T., Weiss, D., Andrade, P. R., et al. (2020). Second-strand synthesis-based massively parallel scRNA-Seq reveals cellular states and molecular features of human inflammatory skin pathologies. Immunity 53, 878–894.e7. doi: 10.1016/j.immuni.2020.09.015
Huveneers, S., and Danen, E. H. J. (2009). Adhesion signaling – crosstalk between integrins, Src and Rho. J. Cell Sci. 122(Pt 8), 1059–1069. doi: 10.1242/jcs.039446
Kanehisa, M., Goto, S., Sato, Y., Furumichi, M., and Tanabe, M. (2012). KEGG for integration and interpretation of large-scale molecular data sets. Nucleic Acids Res. 40, D109–D114. doi: 10.1093/nar/gkr988
Kang, S. W., Kidd, M. T., Kadhim, H. J., Shouse, S., Orlowski, S. K., Hiltz, J., et al. (2020). Characterization of stress response involved in chicken myopathy. Gen. Comp. Endocrinol. 295:113526. doi: 10.1016/j.ygcen.2020.113526
Kimmelman, A. C., Nuñez Rodriguez, N., and Chan, A. M. (2002). R-Ras3/M-Ras induces neuronal differentiation of PC12 cells through cell-type-specific activation of the mitogen-activated protein kinase cascade. Mol. Cell. Biol. 22, 5946–5961. doi: 10.1128/mcb.22.16.5946-5961.2002
Klapholz, B., and Brown, N. H. (2017). Talin – the master of integrin adhesions. J. Cell Sci. 130, 2435–2446. doi: 10.1242/jcs.190991
Koivisto, L., Heino, J., Häkkinen, L., and Larjava, H. (2014). Integrins in wound healing. Adv. Wound Care 3, 762–783. doi: 10.1089/wound.2013.0436
Lagares, D., Busnadiego, O., García-Fernández, R. A., Kapoor, M., Liu, S., Carter, D. E., et al. (2012). Inhibition of focal adhesion kinase prevents experimental lung fibrosis and myofibroblast formation. Arthritis Rheum. 64, 1653–1664. doi: 10.1002/art.33482
Lake, J. A., and Abasht, B. (2020). Glucolipotoxicity: a proposed etiology for wooden breast and related myopathies in commercial broiler chickens. Front. Physiol. 11:169. doi: 10.3389/fphys.2020.00169
Lake, J. A., Papah, M. B., and Abasht, B. (2019). Increased expression of lipid metabolism genes in early stages of wooden breast links myopathy of broilers to metabolic syndrome in humans. Genes 10:746. doi: 10.3390/genes10100746
Leek, J., McShane, B. B., Gelman, A., Colquhoun, D., Nuijten, M. B., and Goodman, S. N. (2017). Five ways to fix statistics. Nature 551, 557–559. doi: 10.1038/d41586-017-07522-z
Lin, Z., He, R., Luo, H., Lu, C., Ning, Z., Wu, Y., et al. (2018). Integrin-β5, a miR-185-targeted gene, promotes hepatocellular carcinoma tumorigenesis by regulating β-catenin stability. J. Exp. Clin. Cancer Res. 37:17. doi: 10.1186/s13046-018-0691-9
Liu, J., Puolanne, E., Schwartzkopf, M., and Arner, A. (2020). Altered sarcomeric structure and function in woody breast myopathy of avian pectoralis major muscle. Front. Physiol. 11:287. doi: 10.3389/fphys.2020.00287
Livak, K. J., and Schmittgen, T. D. (2001). Analysis of relative gene expression data using real-time quantitative PCR and the 2-ΔΔCt method. Methods 25, 402–408. doi: 10.1006/meth.2001.1262
Madruga, M. S., da Rocha, T. C., de Carvalho, L. M., Sousa, A. M. B. L., de Sousa Neto, A. C., Coutinho, D. G., et al. (2019). The impaired quality of chicken affected by the wooden breast myopathy is counteracted in emulsion-type sausages. J. Food Sci. Technol. 56, 1380–1388. doi: 10.1007/s13197-019-03612-0
Maharjan, P., Hilton, K., Weil, J., Suesuttajit, N., Beitia, A., Owens, C. M., et al. (2020). Characterizing woody breast myopathy in a meat broiler line by heat production, microbiota, and plasma metabolites. Front. Vet. Sci. 6:497. doi: 10.3389/fvets.2019.00497
Malila, Y., Srimarut, Y., U-Chupaj, J., Strasburg, G., and Visessanguan, W. (2015). Monitoring of chicken RNA integrity as a function of prolonged postmortem duration. Asian Australas J. Anim. Sci. 28, 1649–1656. doi: 10.5713/ajas.15.0167
Malila, Y., Thanatsang, K., Arayamethakorn, S., Uengwetwanit, T., Srimarut, Y., Petracci, M., et al. (2019). Absolute expressions of hypoxia-inducible factor-1 alpha (HIF1A) transcript and the associated genes in chicken skeletal muscle with white striping and wooden breast myopathies. PLoS One 14:e0220904. doi: 10.1371/journal.pone.0220904
Malila, Y., U-Chupaj, J., Srimarut, Y., Chaiwiwattrakul, P., Uengwetwanit, T., Arayamethakorn, S., et al. (2018). Monitoring of white striping and wooden breast cases and impacts on quality of breast meat collected from commercial broilers (Gallus gallus). Asian Australas J. Anim. Sci. 31, 1807–1817. doi: 10.5713/ajas.18.0355
Malila, Y., Uengwetwanit, T., Arayamethakorn, S., Srimarut, Y., Thanatsang, K. V., Soglia, F., et al. (2020). Transcriptional profiles of skeletal muscle associated with increasing severity of white striping in commercial broilers. Front. Physiol. 11:580. doi: 10.3389/fphys.2020.00580
Marchesi, J. A. P., Ibelli, A. M. G., Peixoto, J. O., Cantão, M. E., Pandolfi, J. R. C., Marciano, C. M. M., et al. (2019). Whole transcriptome analysis of the pectoralis major muscle reveals molecular mechanisms involved with white striping in broiler chickens. Poult. Sci. 98, 590–601. doi: 10.3382/ps/pey429
Mashek, D. G., Li, L. O., and Coleman, R. A. (2007). Long-chain acyl-CoA synthetases and fatty acid channeling. Future Lipidol. 2, 465–476. doi: 10.2217/17460875.2.4.465
Matsumoto, K., Asano, T., and Endo, T. (1997). Novel small GTPase M-Ras participates in reorganization of actin cytoskeleton. Oncogene 15, 2409–2417. doi: 10.1038/sj.onc.1201416
Meloche, K. J., Dozier, W. A., Brandebourg, T. D., and Starkey, J. D. (2018). Skeletal muscle growth characteristics and myogenic stem cell activity in broiler chickens affected by wooden breast. Poult. Sci. 97, 4401–4414. doi: 10.3382/ps/pey287
Mizunoe, Y., Kobayashi, M., Hoshino, S., Tagawa, R., Itagawa, R., Hoshino, A., et al. (2020). Cathepsin B overexpression induces degradation of perilipin 1 to cause lipid metabolism dysfunction in adipocytes. Sci. Rep. 10:634. doi: 10.1038/s41598-020-57428-6
Mollinedo, F., and Gajate, C. (2003). Microtubules, microtubule-interfering agents and apoptosis. Apoptosis 8, 413–450. doi: 10.1023/A:1025513106330
Müller, C., Schillert, A., Röthemeier, C., Trégouët, D.-A., Proust, C., Binder, H., et al. (2016). Removing batch effects from longitudinal gene expression – quantile normalization plus comBat as best approach for microarray transcriptome data. PLoS One 11:e0156594. doi: 10.1371/journal.pone.0156594
Mutryn, M. F., Brannick, E. M., Fu, W., Lee, W. R., and Abasht, B. (2015). Characterization of a novel chicken muscle disorder through differential gene expression and pathway analysis using RNA-sequencing. BMC Genomics 16:399. doi: 10.1186/s12864-015-1623-0
Nishimichi, N., Tsujino, K., Kanno, K., Sentani, K., Kobayashi, T., Chayama, K., Sheppard, D., and Yokosaki, Y. (2021). Induced hepatic stellate cell integrin, α8β1, enhances cellular contractility and TGFβ activity in liver fibrosis. J. Pathol. 253, 3660–373. doi: 10.1002/path.5618
Okonkwo, U. A., Chen, L., Ma, D., Haywood, V. A., Barakat, M., Urao, N., et al. (2020). Compromised angiogenesis and vascular integrity in impaired diabetic wound healing. PLoS One 15:e0231962. doi: 10.1371/journal.pone.0231962
Pampouille, E., Hennequet-Antier, C., Praud, C., Juanchich, A., Brionne, A., Godet, E., et al. (2019). Differential expression and co-expression gene network analyses reveal molecular mechanisms and candidate biomarkers involved in breast muscle myopathies in chicken. Sci. Rep. 9:14905. doi: 10.1038/s41598-019-51521-1
Papah, M. B., and Abasht, B. (2019). Dysregulation of lipid metabolism and appearance of slow myofiber-specific isoforms accompany the development of Wooden Breast myopathy in modern broiler chickens. Sci. Rep. 9:17170. doi: 10.1038/s41598-019-53728-8
Papah, M. B., Brannick, E. M., Schmidt, C. J., and Abasht, B. (2018). Gene expression profiling of the early pathogenesis of wooden breast disease in commercial broiler chickens using RNA-sequencing. PLoS One 13:e0207346. doi: 10.1371/journal.pone.0207346
Papah, M. B., Brannick, E. M., Schmidt, C. J., and Abasht, B. (2017). Evidence and role of phlebitis and lipid infiltration in the onset and pathogenesis of Wooden Breast Disease in modern broiler chickens. Avian Pathol. 46, 623–643. doi: 10.1080/03079457.2017.1339346
Petracci, M., Soglia, F., Madruga, M., Carvalho, L., Ida, E., and Estévez, M. (2019). Wooden-breast, white striping, and spaghetti meat: causes, consequences and consumer perception of emerging broiler meat abnormalities. Compr. Rev. Food Sci. Food Saf. 18, 565–583. doi: 10.1111/1541-4337.12431
Phillips, C. A., Reading, B. J., Livingston, M., Livingston, K., and Ashwell, C. M. (2020). Evaluation via supervised machine learning of the broiler pectoralis major and liver transcriptome in association with the muscle myopathy wooden breast. Front. Physiol. 11:101. doi: 10.3389/fphys.2020.00101
Praud, C., Jimenez, J., Pampouille, E., Couroussé, N., Godet, E., Le Bihan-Duval, E., and Berri, C. (2020). Molecular phenotyping of white striping and wooden breast myopathies in chicken. Front. Physiol. 11:633. doi: 10.3389/fphys.2020.00633
Qu, J., Liu, X., Li, J., Gong, K., Duan, L., Luo, W., et al. (2019). [AKR1B10 promotes proliferation of breast cancer cells by activating Wnt/β-catenin pathway]. Xi bao yu fen zi mian yi xue za zhi. 35, 1094–1100.
R Core Team (2017). R: A Language and Environment for Statistical Computing. In R Foundation for Statistical Computing. Available online at: https://www.R-project.org (accessed October 23, 2019).
Radaelli, G., Piccirillo, A., Birolo, M., Bertotto, D., Gratta, F., Ballarin, C., et al. (2017). Effect of age on the occurrence of muscle fiber degeneration associated with myopathies in broiler chickens submitted to feed restriction. Poult. Sci. 96, 309–319. doi: 10.3382/ps/pew270
Rao, R. K., and Wu, Z. (2021). “Cell adhesion and the extracellular matrix,” in Goodman’s Medical Cell Biology, 4th Edn, ed. S. R. Goodman (Cambridge, MA: Academic Press), 203–247. doi: 10.1016/b978-0-12-817927-7.00007-7
Shang, N., Arteaga, M., Chitsike, L., Wang, F., Viswakarma, N., Breslin, P., et al. (2016). FAK deletion accelerates liver regeneration after two-thirds partial hepatectomy. Sci. Rep. 6:34316. doi: 10.1038/srep34316
Sharov, V., Kwong, K. Y., Frank, B., Chen, E., Hasseman, J., Gaspard, R., et al. (2004). The limits of log-ratios. BMC Biotechnol. 4:3. doi: 10.1186/1472-6750-4-3
Sihvo, H. K., Immonen, K., and Puolanne, E. (2014). Myodegeneration with fibrosis and regeneration in the pectoralis major muscle of broilers. Vet. Pathol. 51, 619–623. doi: 10.1177/0300985813497488
Sihvo, H. K., Linden, J., Airas, N., Immonen, K., Valaja, J., and Puolanne, E. (2017). Wooden breast myodegeneration of pectoralis major muscle over the growth period in broilers. Vet. Pathol. 54, 119–128. doi: 10.1177/0300985816658099
Sofronescu, A. G., Detillieux, K. A., and Cattini, P. A. (2010). FGF-16 is a target for adrenergic stimulation through NF-κB activation in postnatal cardiac cells and adult mouse heart. Cardiovasc. Res. 87, 102–110. doi: 10.1093/cvr/cvq025
Soglia, F., Mazzoni, M., Zappaterra, M., Di Nunzio, M., Babini, E., Bordini, M., et al. (2020). Distribution and expression of vimentin and desmin in broiler pectoralis major affected by the growth-related muscular abnormalities. Front. Physiol. 10:1581. doi: 10.3389/fphys.2019.01581
Soglia, F., Mudalal, S., Babini, E., Di Nunzio, M., Mazzoni, M., Sirri, F., et al. (2016). Histology, composition, and quality traits of chicken Pectoralis major muscle affected by wooden breast abnormality. Poult. Sci. 95, 651–659. doi: 10.3382/ps/pev353
Tasoniero, G., Cullere, M., Cecchinato, M., Puolanne, E., and Zotte, A. D. (2016). Technological quality, mineral profile, and sensory attributes of broiler chicken breasts affected by white striping and wooden breast myopathies. Poult. Sci. 95, 2707–2714. doi: 10.3382/ps/pew215
Taylor, S. C., Laperriere, G., and Germain, H. (2017). Droplet digital PCR versus qPCR for gene expression analysis with low abundant targets: from variable nonsense to publication quality data. Sci. Rep. 7:2409. doi: 10.1038/s41598-017-02217-x
Tijare, V. V., Yang, F. L., Kuttappan, V. A., Alvarado, C. Z., Coon, C. N., and Owens, C. M. (2016). Meat quality of broiler breast fillets with white striping and woody breast muscle myopathies. Poult. Sci. 95, 2167–2173. doi: 10.3382/ps/pew129
Turner, C. E. (2000). Paxillin and focal adhesion signalling. Nat. Cell Biol. 2, E231–E236. doi: 10.1038/35046659
Velleman, S. G. (2015). Relationship of skeletal muscle development and growth to breast muscle myopathies: a review. Avian Dis. 59, 525–531. doi: 10.1637/11223-063015-Review.1
Velleman, S. G. (2020). Pectoralis major (Breast) muscle extracellular matrix fibrillar collagen modifications associated with the wooden breast fibrotic myopathy in broilers. Front. Physiol. 11:461. doi: 10.3389/fphys.2020.00461
Velleman, S. G., and Clark, D. L. (2015). Histopathologic and myogenic gene expression changes associated with wooden breast in broiler breast muscles. Avian Dis. 59, 410–418. doi: 10.1637/11097-042015-Reg.1
Velleman, S. G., Clark, D. L., and Tonniges, J. R. (2017). Fibrillar collagen organization associated with broiler wooden breast fibrotic myopathy. Avian Dis. 61, 481–490. doi: 10.1637/11738-080217-Reg.1
Wang, C., Zhu, Y., Wu, D., Wang, Z., Xu, X., Shi, Y., et al. (2020). The role of PDIA3 in myogenesis during muscle regeneration. Exp. Mol. Med. 52, 105–117. doi: 10.1038/s12276-019-0368-2
Wozniak, M. A., Modzelewska, K., Kwong, L., and Keely, P. J. (2004). Focal adhesion regulation of cell behavior. Biochim. Biophys. Acta Mol. Cell Res. 1692, 103–119. doi: 10.1016/j.bbamcr.2004.04.007
Xing, T., Zhao, X., Zhang, L., Li, J. L., Zhou, G. H., Xu, X. L., et al. (2020). Characteristics and incidence of broiler chicken wooden breast meat under commercial conditions in China. Poult. Sci. 99, 620–628. doi: 10.3382/ps/pez560
Yarden, Y., and Sliwkowski, M. X. (2001). Untangling the ErbB signalling network. Nat. Rev. Mol. Cell Biol. 2, 127–137. doi: 10.1038/35052073
Yoshida, R., Imai, T., Hieshima, K., Kusuda, J., Baba, M., Kitaura, M., et al. (1997). Molecular cloning of a novel human CC chemokine EBI1-ligand chemokine that is a specific functional ligand for EBI1, CCR7∗. J. Biol. Chem. 272, 13803–13809. doi: 10.1074/jbc.272.21.13803
Zaidel-Bar, R., and Geiger, B. (2010). The switchable integrin adhesome. J. Cell Sci. 123(Pt 9), 1385–1388. doi: 10.1242/jcs.066183
Zambonelli, P., Zappaterra, M., Soglia, F., Petracci, M., Sirri, F., Cavani, C., et al. (2016). Detection of differentially expressed genes in broiler pectoralis major muscle affected by white striping – wooden breast myopathies. Poult. Sci. 95, 2771–2785. doi: 10.3382/ps/pew268
Zanetti, M. A., Tedesco, D. C., Schneider, T., Teixeira, S. T. F., Daroit, L., Pilotto, F., et al. (2018). Economic losses associated with wooden breast and white striping in broilers. Semin. Cienc. Agrar. 39, 887–892. doi: 10.5433/1679-0359.2018v39n2p887
Keywords: broiler chicken, wooden breast, transcriptome profile, droplet digital polymerase chain reaction, focal adhesion
Citation: Malila Y, Uengwetwanit T, Thanatsang KV, Arayamethakorn S, Srimarut Y, Petracci M, Soglia F, Rungrassamee W and Visessanguan W (2021) Insights Into Transcriptome Profiles Associated With Wooden Breast Myopathy in Broilers Slaughtered at the Age of 6 or 7 Weeks. Front. Physiol. 12:691194. doi: 10.3389/fphys.2021.691194
Received: 05 April 2021; Accepted: 07 June 2021;
Published: 28 June 2021.
Edited by:
Xiquan Zhang, South China Agricultural University, ChinaReviewed by:
Takeshi Kawasaki, Research Office Concerning the Health of Humans and Birds, JapanEero Puolanne, University of Helsinki, Finland
Copyright © 2021 Malila, Uengwetwanit, Thanatsang, Arayamethakorn, Srimarut, Petracci, Soglia, Rungrassamee and Visessanguan. This is an open-access article distributed under the terms of the Creative Commons Attribution License (CC BY). The use, distribution or reproduction in other forums is permitted, provided the original author(s) and the copyright owner(s) are credited and that the original publication in this journal is cited, in accordance with accepted academic practice. No use, distribution or reproduction is permitted which does not comply with these terms.
*Correspondence: Yuwares Malila, eXV3YXJlcy5tYWxAYmlvdGVjLm9yLnRo