- 1MOE Key Laboratory of Marine Genetics and Breeding, College of Marine Life Sciences, Ocean University of China, Qingdao, China
- 2Laboratory for Marine Fisheries Science and Food Production Processes, Pilot Qingdao National Laboratory for Marine Science and Technology, Qingdao, China
- 3Laboratory of Tropical Marine Germplasm Resources and Breeding Engineering, SANYA Oceanographic Institution of the Ocean University of CHINA, Sanya, China
Mitogen-activated protein kinase (MAPK) cascades are fundamental signal transduction modules in all eukaryotic organisms, participating growth and development, as well as stress response. In the present study, three MAPK genes were successfully identified from the genome of Chlamys farreri, respectively, named CfERK1/2, CfJNK, and Cfp38, and only one copy of ERK, JNK, and p38 were detected. Domain analysis indicated that CfMAPKs possessed the typical domains, including S_TKc, Pkinase, and PKc_like domain. Phylogenetic analysis showed that three CfMAPKs of MAPK subfamilies exists in the common ancestor of vertebrates and invertebrates. All CfMAPKs specifically expressed during larval development and in adult tissues, and the expression level of CfERK1/2 and Cfp38 was apparently higher than that of CfJNK. Under heat stress, the expression of CfERK1/2 and Cfp38 were significantly downregulated and then upregulated in four tissues, while the expression of CfJNK increased in all tissues; these different expression patterns suggested a different molecular mechanism of CfMAPKs for bivalves to adapt to temperature changes. The diversity of CfMAPKs and their specific expression patterns provide valuable information for better understanding of the functions of MAPK cascades in bivalves.
Introduction
The Zhikong scallop, Chlamys farreri (Jones, 1904, also known as Chinese scallop), naturally distributes along the coasts of Northern China, Korea, Japan, and Eastern Russia, which usually live a semisessile lifestyle by attaching itself to rocks and other hard surfaces with byssal threads (Shumway and Parsons, 2016). C. farreri is one of commercially important scallops for aquaculture in China (Guo and Luo, 2016). The production of scallops in China showed a declining tendency for the last few years, which was 2.01 million tons in 2017, 1.92 million tons in 2018, and 1.83 million tons in 2019 (China Fishery Statistical Yearbook 2019 and 2020). In recent years, extreme weather especially high temperatures happened frequently worldwide, which threatened the survival of almost all marine organisms. Stress caused by sudden changes in temperature or chronic heat stimuli above optimum conditions can interrupt cellular homeostasis and result in serious deficiency in development and growth and even large-scale death in bivalves, including scallop, mussel, and oyster (Liu et al., 2004; Xiao et al., 2005; Hornstein et al., 2018; Rahman et al., 2019; Zhang et al., 2020).
High temperature affects the physiological and biochemical responses of marine organisms, which are driven by the regulation of gene expression (Hornstein et al., 2018; Rahman et al., 2019; Zhang et al., 2020). Stress-activated protein kinases have been proven to play significant roles in the thermal response of marine organisms (Anestis et al., 2008; Tomanek and Zuzow, 2010; Dong et al., 2011; Yao and Somero, 2012). Mitogen-activated protein kinases (MAPKs) are protein Ser/Thr kinases that transmit extracellular signals and cause many intracellular reactions (Cargnello and Roux, 2011). This process is realized by the cascade reaction of conservative MAPK, mitogen-activated protein kinase (MAPKK), and mitogen-activated protein kinase (MAPKKK). The MAPK mainly has three subfamilies, including extracellular signal regulated kinase (ERK), c-Jun NH2-terminal kinase (JNK), and p38 (Johnson and Lapadat, 2002). The classification of subfamily is based on the Thr-X-Tyr sequence of the tripeptide motif: ERK is Thr-Glu-Tyr, JNK is Thr-Pro-Tyr, and p38 is Thr-Gly-Tyr. The numbers of gene copy in different subfamilies are different among species. In vertebrates, ERK subfamily includes ERK1 and ERK2 (Boulton et al., 1990, 1991); JNK subfamily includes JNK1, JNK2, and JNK3 (Kyriakis et al., 1994); and p38 subfamily includes p38α, p38β, p38γ, and p38δ (Cuadrado and Nebreda, 2010). There are also some atypical subfamilies, such as ERK3/4 and ERK7/8, whose activation process is not a typical three-level cascade reaction (Lau and Xu, 2019). The MAPK signaling pathway is highly conservative in evolution and closely related to a variety of signal transmission and feedback (Yang et al., 2010). Many studies have found that MAPK played important roles in controlling the response of cells to the environment, extensively participating in various physiological processes such as cell growth, cell differentiation, proliferation, and apoptosis (Johnson and Lapadat, 2002; Morrison, 2012).
Extracellular signal regulated kinase signaling pathway is the first discovered MAPK signaling pathway including ERK1/2 and ERK5. ERK pathway is essential in the regulation of cell proliferation and differentiation in all organisms (Jing and Anning, 2005). In starfish (Asterina miniata) and sea urchin (Strongylocentrotus purpuratus), ERK pathway can regulate the meiotic process of oocytes (Carroll et al., 2000). In Chaetopleura, Tectura, and Lymnaea, ERK pathway has been proven to participate in the cell division of blastomere (Lambert and Nagy, 2003). In the study of Ciona intestinalis, ERK pathway can induce the formation of ascidian notochord precursor (Picco et al., 2007), embryonic nerve, and brain (Akanuma and Nishida, 2004). Besides, many studies showed that ERK pathway also participate in the immune response of organism. For example, ERK was found to be a downstream target of immune signal transmission in the hemocytes of gastropod snail (Lymnaea stagnalis), which played a crucial role in the Molluscan innate defense response (Lacchini et al., 2006). The JNK signal pathway is another signaling pathway whose activation site is located in the amino-terminal active region (Weston and Davis, 2007). JNK is also called stress-activated protein kinase (SAPK), and its upstream kinases mitogen-activated protein kinase 4 (MEK4) and mitogen-activated protein kinase 7 (MEK7) are key nodes in the JNK signal pathway (Dhanasekaran and Reddy, 2008). The different functions of JNK1/2/3 mainly depend on the specific site of their binding to the substrate (Ye et al., 2009). The currently known substrates of JNK are Ets-like protein 1 (Elk-1), activating transcription factor 2 (ATF-2), and c-Jun. p38 also belongs to SAPK, which can be activated by many stress conditions and mediates various stress responses (Johnson and Lapadat, 2002). Stresses such as high temperature, hypoxia, and hypertonicity can activate JNK and p38 pathways of M. edulis (Gaitanaki et al., 2004). In Drosophila hemocytes, JNK signaling pathway mediated the immune response to endotoxin through a rapid activation of JNK during a bacterial lipopolysaccharide (LPS) infection (Sluss et al., 1996). In red swamp crayfish (Procambarus clarkii), the expression of p38 gene regulated the distribution and accumulation of cadmium (Cd) in different tissues under Cd-stressed condition (Shui et al., 2020).
All above studies emphasize the important functions of MAPK signaling pathway in environmental response, cell proliferation and differentiation, etc. However, the knowledge regarding MAPK in invertebrate responding to environmental stress is still unclear, and further researches are necessary. In the present study, we carried out the systematic identification of MAPK in the genome of C. farreri and phylogenetic analysis and examined their expression profiles during larval development stages, in adult tissues, and under heat stress. The results will contribute to better understand the function of MAPK and provide valuable information for elucidating environmental adaptation of bivalves.
Materials and Methods
Genome-Wide Identification and Sequence Analysis of MAPK Genes in C. farreri
The whole-genome of C. farreri (PRJAN185456) (Li et al., 2017) were used to search with the typical MAPK protein sequences of other species, including ERK (Caenorhabditis elegans, Patinopecten yessoensis, Crassostrea gigas, Crassostrea virginica, and Ciona intestinalis), JNK (Apostichopus japonicas, Danio rerio, Xenopus tropicalis, and Gallus gallus), and p38 (Mus musculus and Homo sapiens), retrieved from the National Center for Biotechnology Information (NCBI)1, Wormbase2, and Flybase3 (Supplementary Table 1). The open reading frame (ORF) finder was used to predict their gene structure4. The amino acid sequences were verified by BLASTP against the NCBI non-redundant protein sequence database. The conserved domains were predicted by SMART5. The putative isoelectric point (PI) and molecular weight were predicted by ExPASy-Prot6. The secondary structures was predicted by software Geneious 4.8.47.
Phylogenetic Analysis
Multiple protein sequences alignments were performed on identified MAPK protein sequences from C. farreri and other selected organisms using the ClustalW28. The phylogenetic tree was constructed utilizing software MEGA-X with the neighbor-joining (NJ) method (Kumar et al., 2016). Bootstrapping with 5,000 replications was used to evaluate the phylogenetic tree.
Spatiotemporal Expression of MAPK Genes in C. farreri
The expression profiles of CfMAPKs were analyzed using RNA-seq datasets (SRX2444844-SRX2444876 and SRX2510167-SRX2510176) of C. farreri (Li et al., 2017). The expression level was described by transcripts per kilobase per million mapped reads (TPM). The TPM values from the RNA-seq datasets, including different developmental stages (zygote, multicell, blastula, gastrula, trochophore, D-shaped larvae, early umbo, middle umbo, post umbo, eyespots larvae, and juvenile) and adult tissues (adductor muscle, smooth muscle, foot, mantle, eye, gill, hemolymph, digestive gland, kidney, female gonad, male gonad, cerebral ganglia, and visceral ganglia) were Log2 transformed and subsequently used to perform a visualization of expression analysis with heat map by software TBtools (Chen et al., 2020).
Expression Analysis of CfMAPKs Under Heat Stress
The transcriptome databases of C. farreri in response to heat stress were independently constructed by our laboratory (unpublished data). In simple terms, 27°C was used as thermal stimulus condition, which is close to the maximum temperature at sea area of scallop distribution, and 20°C was used as control temperature. Transcriptome databases at eight time points (3, 6, 12, and 24 h, 3, 6, 15, and 30 days) in four tissues (three individual replications were set), including mantle, gill, heart and hemolymph, were used to reveal the expression levels of CfMAPKs under heat stress. Statistical analyses were conducted with one-way analysis of variance followed by post hoc comparison of means bases Tukey honestly significant difference (HSD) with software IBM SPSS Statistics 20; significant level was set at p < 0.05.
Results
Gene Identification and Sequence Analysis
Three CfMAPKs were identified from the transcriptome and genome of C. farreri, which showed high sequence similarity with ERK, JNK, and p38 of species from invertebrate to vertebrate. Three genes were named separately as CfERK1/2, CfJNK, and Cfp38. As shown in Table 1, the length of CfERK, CfJNK, and Cfp38 was 1,962, 2,075, and 630 bp, encoding 363, 408, and 209 amino acids, respectively. CfERK1/2 and CfJNK were composed of 11 exons and 10 introns; Cfp38 was composed of 8 exons and 7 introns (Figure 1). Some introns of CfERK are extremely long, such as the last three introns that were longer than 10 kb (11,279, 11,104, and 21,079 bp). The length of some exons was similar among the three CfMAPKs. For example, the length of second exons was similar 124, 127, and 114 bp, respectively. More strikingly, the exon 6 in CfERK and exon 7 in CfJNK were the same in length (71 bp). Both exon 4 of CfJNK and exon 6 of Cfp38 were of the same length. The conserved domain (S_TKc) in all CfMAPKs was predicted by SMART (Figure 2). The secondary structure showed that all three CfMAPK genes have four structures including α helix, β sheet, random coil, and turn. However, the number of different structures among members was pretty different (Table 1 and Figure 2).
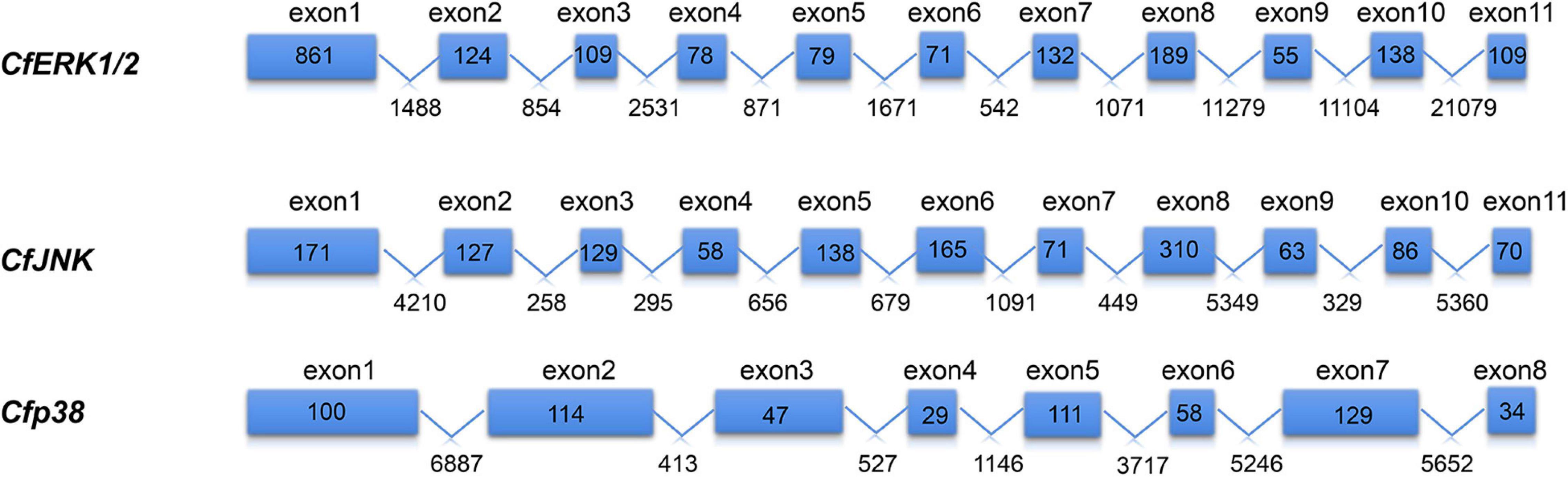
Figure 1. Gene structure of CfMAPKs. The blue boxes indicate the exons, and the polylines indicate the introns. The numbers in the boxes indicate the length of exon; the numbers under the line indicate the length of intron.
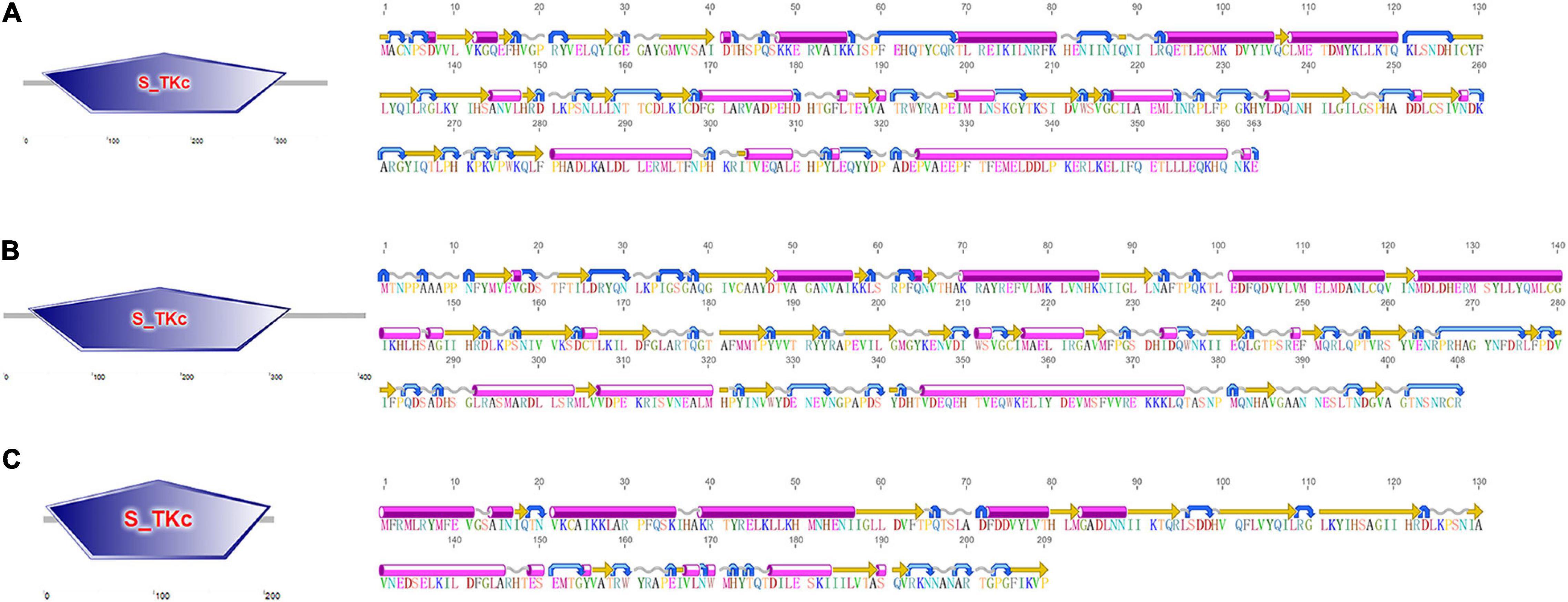
Figure 2. The conserved domain (left) and secondary structure (right) of (A) CfERK1/2, (B) CfJNK, and (C) Cfp38. The pink cylinders stand for alpha helixes, the orange straight arrows stand for beta strands, the gray wavy lines stand for coil, and the blue curved arrows stand for turns.
Based on multiple sequences alignment of the MAPK amino acid sequences among C. farreri and other species including H. sapiens, D. rerio, and P. yessoensis, the dual phosphorylation T-X-Y motif located and some functional sites, such as HRDLK-XX-N, DFG, and APE motif, were identified, which were similar to the MAPK proteins reported in other species (Figure 3). In addition to the conserved structures shared by most MAPKs, three specific signature sequences were identified in CfERK (marked with green box), and two specific signature sequences were identified in CfJNK (marked with yellow box) and Cfp38 (marked with orange box), respectively.
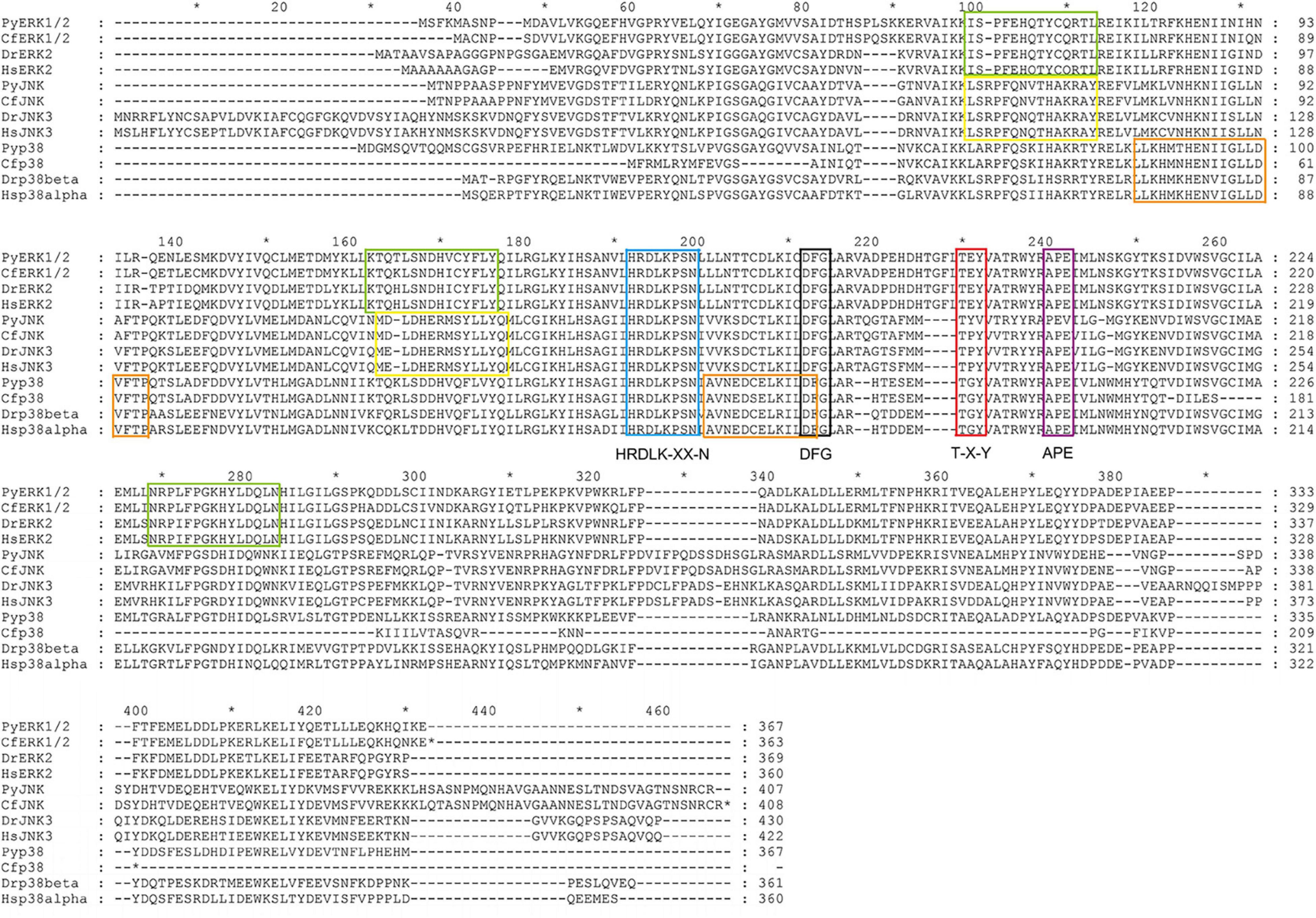
Figure 3. Alignment of the amino acid sequences of CfMAPK with other MAPKs. The blue box indicates the HRDLK-XX-N motif; the black and the purple boxes indicate the DFG and APE motif, respectively; the red box indicates the phosphorylation T-X-Y motif; and the green, yellow, and orange boxes indicate the conserved signature sequences of ERK, JNK, and p38, respectively.
Phylogenetic Analysis
A NJ phylogenetic tree was constructed using the amino acid sequence of CfMAPKs and MAPK members of other species (Figure 4). As a whole, the phylogenetic tree was classified into three subfamilies, comprising p38, JNK, and ERK, which indicated that ERK, JNK, and p38 were more conserved between homologs of other species than other subfamilies. The three CfMAPKs, respectively, located within one clade and first clustered with MAPKs of other bivalves including P. yessoensis, C. gigas, and C. virginica.
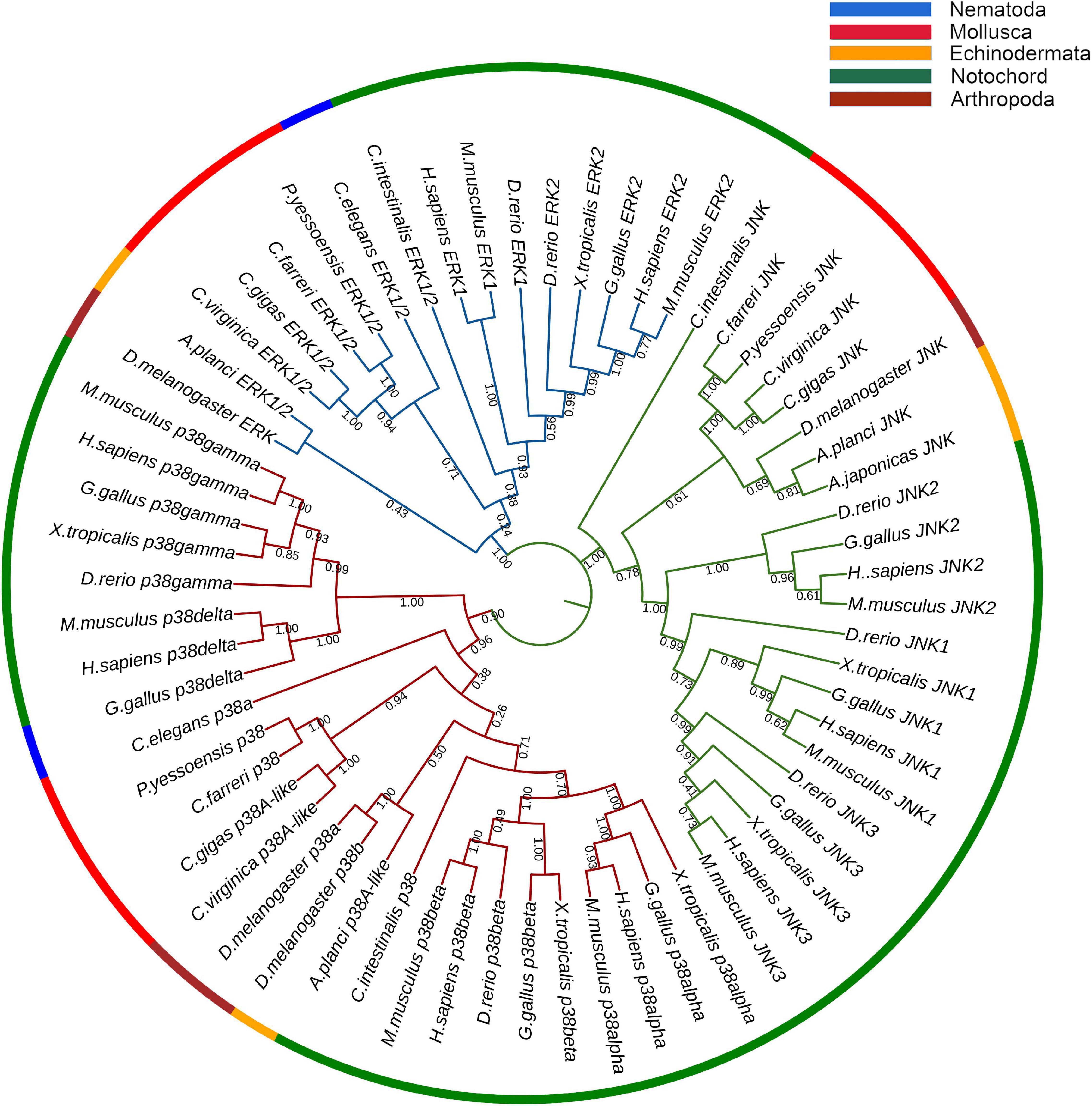
Figure 4. Phylogenetic tree of CfERK, CfJNK, and Cfp38 with other MAPKs. The blue branches are ERK, the green branches are JNK, and the red branches are p38. The colors of the outermost rings represent different phyla, blue represents Nematoda, red represents Mollusca, orange represents Echinodermata, green represents Notochord, and crimson represents Arthropoda. The numbers under the tree branches indicate the bootstrap values from 5,000 replicates.
Spatiotemporal Expression of CfMAPKs
RNA-seq datasets for different developmental periods and adult tissues of C. farreri were analyzed to detect the expression patterns of MAPK genes. During developmental process, CfERK1/2 and Cfp38 showed high expression level, but CfJNK showed a low expression level (Figure 5A). Specifically, expression of CfERK1/2 gradually increased from gastrula and maintained a high expression level from D-shaped larvae to juvenile. The Cfp38 was the highest expression member among CfMAPKs and remained continuously express high level during developmental stages, especially blastula, gastrula, trochophore, eyespots larva, and juvenile. In all tested tissues, the expressions of CfERK and Cfp38 were ubiquitous, whereas CfJNK was barely expressed (Figure 5B), which showed similar expression pattern with developmental stages. Specifically, CfERK showed the highest expression in the visceral ganglia, followed by cerebral ganglia, hemolymph, and eye. Cfp38 expressed highest in the kidney, followed by hemolymph and smooth muscle.
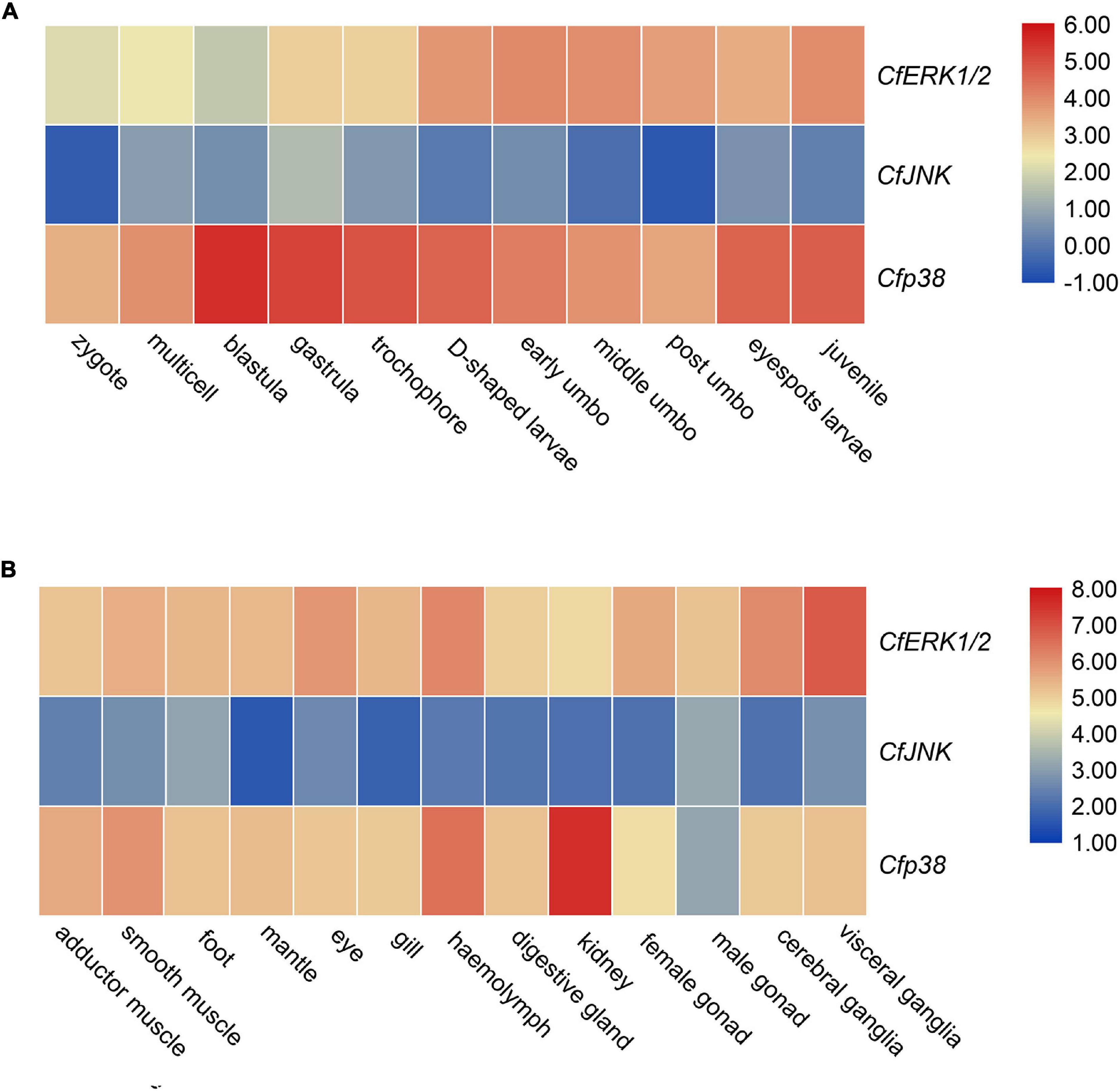
Figure 5. Expression analysis of CfERK1/2, CfJNK, and Cfp38 during (A) different developmental stages and (B) in adult tissues of C. farreri based on the log2TPM.
Expression Profiles of CfMAPKs in Response to Heat Stress
To examine the expression patterns of CfMAPK in response to environmental stresses, RNA-seq datasets from C. farreri under heat stress were analyzed. In general, the expression of CfMAPK changed apparently and responded frequently in mantle, gill, heart, and hemolymph. Specifically, CfERK1/2 and Cfp38 showed general downregulated trends, whereas CfJNK upregulated at some time points in the mantle, gill, heart, and hemolymph under heat stress (Figure 6). Comparing to control, CfERK1/2 declined significantly at 3, 6 h, and 3 days; Cfp38 declined significantly at 6, 12 h, and 3, 15 days, while CfJNK increased markedly at 3 h in mantle (p < 0.05). Except for CfJNK (only increased significantly at 15 days), the expression pattern of CfERK1/2 and Cfp38 in the hemolymph was similar with that in the mantle, just with different time points of significant change. Besides, a down–up–down expression pattern CfERK1/2 and Cfp38 was found in gill, while CfJNK increased significantly at 6 days. Comparing to other three tissues, the expression of CfERK1/2 and Cfp38 was not significantly changed in the heart, while CfJNK increased significantly at 3 h, 15, and 30 days. Overall, the expression of CfERK1/2 and Cfp38 showed a downregulated tendency, while CfJNK was upregulated at some time points under heat stress. Moreover, CfERK1/2 and Cfp38 had more significant changes than CfJNK, which indicated a different function of CfMAPKs in response to heat stress.
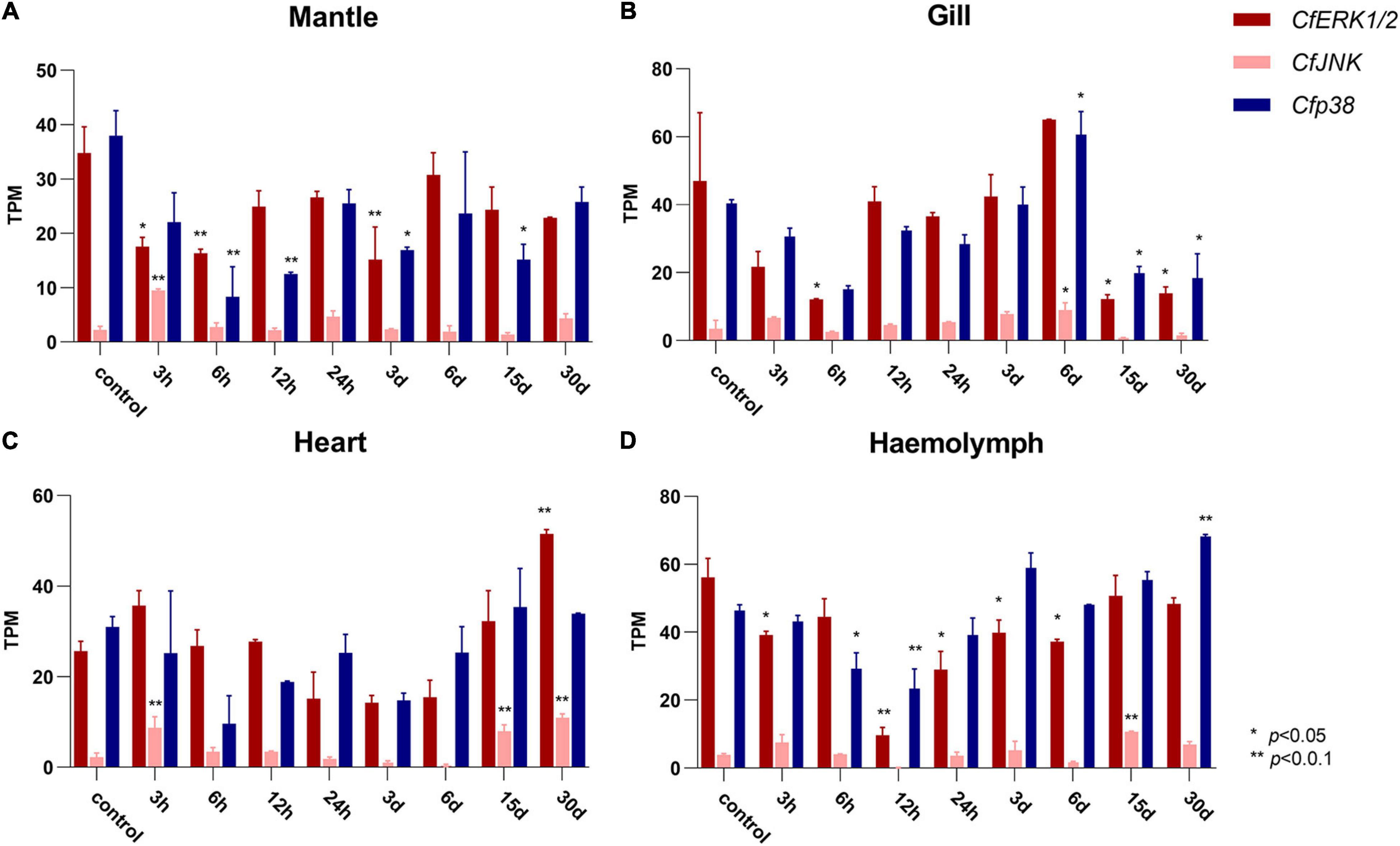
Figure 6. Expression of CfERK1/2, CfJNK, and Cfp38 in (A) mantle, (B) gills, (C) heart, and (D) hemolymph of C. farreri under heat stress at different time points based on the TPM value. The vertical bars represent the mean ± SD (N = 3). The expression of CfMAPKs at 0 h was used as the control. Values marked with asterisks indicate significant differences from the control expression (*p < 0.05; **p < 0.01).
Discussion
Mitogen-activated protein kinase signaling pathway is one of the ancient and evolutionarily conservative signaling pathways from yeast to human, which is involved in a vast array of physiological processes such as growth and development, stress response, and apoptosis (Johnson and Lapadat, 2002; Jing and Anning, 2005; Cargnello and Roux, 2011; Morrison, 2012). Until now, the features and functions of MAPK have been widely reported in vertebrate species, especially in mammals, while limited studies have been carried out in Mollusca. In the present study, based on the whole genome sequences and transcriptome databases of C. farreri, we systematically conducted the identification and evolution analysis of CfMAPK and examined their expression profiles during larval development stages, in adult tissues, and under heat stress.
In vertebrates, such as M. musculus and H. sapiens, there are four MAPK signal cascades including ERK1/2, JNK, p38, and ERK5 signaling pathway (Cargnello and Roux, 2011). At the same time, the genes of these pathways generally have multiple copies, such as two homologous ERK isoforms (ERK1 and ERK2), three JNK isoforms (JNK1, JNK1 and JNK3), and four p38 isoforms (p38α, p38β, p38γ, and p38δ) (Boulton et al., 1990, 1991; Kyriakis et al., 1994; Cuadrado and Nebreda, 2010). In the present study, three MAPK genes including CfERK1/2, CfJNK, and Cfp38 were successfully identified in the genome of C. farreri, but ERK5 gene is absent. In addition, only one copy of ERK, JNK, and p38 was detected in C. farreri genome. Moreover, all CfMAPK genes were conserved with other species in the same subfamily. The similar genes number and copy pattern of MAPKs were also reported in other invertebrates, such as C. elegans (Galbadage et al., 2016), P. yessoensis (Sun et al., 2016), and C. gigas (Qu et al., 2016, 2017). Thus, it is likely that only one copy for each of three MAPK subfamilies exists in the common ancestor of vertebrates and invertebrates, and multiple homologous isoforms of MAPK genes in vertebrates originated from the result of ancestral gene expansion during the evolution process.
During the developmental stages from zygote to juvenile scallop, CfERK1/2 and Cfp38 expressed apparently higher than CfJNK, which indicated that CfERK1/2 and Cfp38 may be more involved in the regulation of growth and development in C. farreri. CfERK maintained a relatively high level from D-shaped larvae to juvenile stages, indicating that it may be involved in the organ development and metamorphic process of C. farreri larvae, such as the disappearance of the velizer, the development of the gills and foot, and the formation of the secondary shell. In previous researches, the expression of ERK was essential for the tail degeneration of C. intestinalis (Chambon et al., 2002). During zebrafish embryonic development, ERK was activated by fibroblast growth factor (FGF), and it could regulate the development and formation of organs, including eye, somites, and limbs (Wong et al., 2019). In addition, in the study of Schistosoma mansoni, Ressurreição et al. (2011) reported that p38 of embryo significantly increased 3.7-fold after 28 h larval culture, and activated p38 was associated with regions of the tegument, neural mass, and germinal cells. In our study, similar expression pattern of p38 was observed in the development of C. farreri, and the expression of Cfp38 was mainly higher in blastula, gastrula, and trochophore than other stages, suggesting that p38 may be involved in blastocyst formation and layer differentiation of bivalve. These expression patterns revealed diverse regulation of CfMAPKs during developmental stages, which may suggest their fundamental role in larval growth and development of bivalve.
Many studies have found that MAPK played important roles in various physiological processes (Johnson and Lapadat, 2002; Jing and Anning, 2005; Cargnello and Roux, 2011; Morrison, 2012). In our study, CfMAPKs were ubiquitously expressed in all the investigated tissues, and the expression levels of CfERK and Cfp38 were higher than that of CfJNK, which indicated that CfERK and Cfp38 could play an important role in the biological processes of C. farreri. In previous studies, Napoli et al. (2012) reported that ERK signaling could orchestrate nerve repair in Schwann cells of mice after suffering from damage of the peripheral nerves. Ciccarelli and Giustetto (2014) found that ERK governed the transmission of neuronal intracellular signaling between pre- and postsynaptic targets related to regulators of gene expression such as transcription factors and histone proteins to control transcription. These studies demonstrated that ERK may be involved in the nerve function and signal transmission. In our study, CfERK was specifically highly expressed in the ganglia, which suggested its potentially regulated role in the nerve function of bivalves. Previous researches have shown that MAPK signaling play an important role in regulating the innate immunity. In Nile tilapia (Oreochromis niloticus), ERK signaling regulates proliferation of primordial T cells to participate in adaptive immune response during bacterial infection (Wei et al., 2020). In the clam (Meretrix petechialis), Zhang et al. (2019) reported that p38 participated in clam immunity by activating microphthalmia-associated transcription factor (MITF) to regulate the expression of the immune-related gene phenoloxidase (PO). In P. yessoensis, the expression of ERK and JNK significantly upregulated after infection with bacteria, revealing the involvement of ERK and JNK in defense against bacterial infection (Sun et al., 2016). In our study, CfERK and Cfp38 was highly expressed in hemolymph, known to have an important role in the immune system. In addition, Tian et al. (2020) reported that MAPKs mediated the immune defense ability of C. farreri hemocytes after Benzo[a]pyrene exposure. Hence, high expression of ERK and p38 may be related to the immune function of the bivalve. In addition, Cfp38 was significantly expressed in the kidney. For filter-feeding bivalve, the kidney has not only the function of secretion and excretion but also the function of storage, such as the accumulation of heavy metal ion in M. galloprovincialis and the accumulation of potent neurotoxins in C. farreri (Wilbur and Yonge, 2013; Li et al., 2017; Wakashin et al., 2018). Thus, high expression of Cfp38 in the kidney may better protect bivalve from accumulation of toxins by modulating the defense mechanisms, which revealed a molecular adaptation to lifestyle.
To provide insight into the function of CfMAPKs in response to environmental stress, the expression of CfMAPKs was, respectively, examined in four adult tissues after exposure to heat stress. Temperature is an important environmental factor affecting the physiological and biochemical responses of organisms, and stress caused by sudden changes in temperature interrupts cellular homeostasis, metabolism, and growth (Liu et al., 2004; Hornstein et al., 2018; Rahman et al., 2019; Zhang et al., 2020). Previous studies have shown that the level of MAPK pathway could be induced by cold and heat stress in the gill tissue, mantle tissue, and hemocytes of mussels (Anestis et al., 2008; Yao and Somero, 2012; Wang et al., 2018). In Takifugu fasciatus, the MAPK pathways on muscles were also activated under cold stress, including ERK, JNK, and p38, and it could regulate lipid metabolism in response to cold stress (Chu et al., 2021). In the study of chicken fibroblast cells, MAPK pathway genes (ERK, JNK, and p38) were downregulated under heat stress condition, and then, curcumin has the ability to ease the oxidative damage through activating the MAPK signaling pathway (Wu et al., 2019). In general, the expression of CfERK1/2 and Cfp38 significantly downregulated and then upregulated in four tissues under heat stress. These results indicated that the heat stress significantly affected the MAPK signaling pathway, which was involved in various tissues of C. farreri. In terms of time of thermal stimulation, the expression of CfMAPK was inhibited in the short term but gradually recovered in the long term. This unique expression pattern of MAPKs suggested a molecular mechanism for ectotherms such as bivalve to adapt to temperature changes. In addition, we also found a special phenomenon that the expression of CfJNK in all tissues could be increased within 3 h, which may be an acute stress mechanism of bivalve, and this was the first report in aquatic animals.
In summary, three MAPKs, including CfERK, CfJNK, and Cfp38, were successfully identified from the genome of C. farreri, and only one copy of ERK, JNK, and p38 was detected, respectively. During larval developmental stages and in adult tissues, CfERK1/2 and Cfp38 expressed apparently higher than CfJNK, which indicated that CfERK1/2 and Cfp38 may be more involved in the regulation of growth, development, and physiological processes in C. farreri. Under heat stress, the expression of CfERK1/2 and Cfp38 were significantly downregulated and then upregulated in four tissues, while the expression of CfJNK increased in all tissues; these different expression pattern suggested a different molecular mechanism of CfMAPKs for bivalve to adapt to temperature changes. The findings of this study provide insight into understanding the diverse functions of CfMAPKs and elucidating MAPK evolution in invertebrates. Further investigation on the functions of CfMAPKs will provide a more detailed explanation about the molecular adaptation mechanisms of bivalve under environmental stress.
Data Availability Statement
The original contributions presented in the study are included in the article/Supplementary Material, further inquiries can be directed to the corresponding author/s.
Author Contributions
ZL and ZY: conceptualization, methodology, data curation, investigation, formal analysis, visualization, writing – original draft, and writing – review and editing. XH: conceptualization, writing – original draft, writing – review and editing, supervision, project administration, and funding acquisition. CP: methodology, investigation, data curation, formal analysis, and visualization. HY, CC, YH, and XW: methodology, data curation, investigation, fanalysis, and visualization. QX and JH: conceptualization, supervision, and project administration. ZB: supervision, project administration, and funding acquisition. All authors contributed to the article and approved the submitted version.
Funding
The project was supported by the grants of the Chinese Ministry of Science and Technology through the National Key Research and Development Program of China (2018YFD0900304 and 2018YFD0901402), the Earmarked Fund for Modern Agro-industry Technology Research System (CARS-49), and Earmarked Fund for Agriculture Seed Improvement Project of Shandong Province (2020LZGC016).
Conflict of Interest
The authors declare that the research was conducted in the absence of any commercial or financial relationships that could be construed as a potential conflict of interest.
Publisher’s Note
All claims expressed in this article are solely those of the authors and do not necessarily represent those of their affiliated organizations, or those of the publisher, the editors and the reviewers. Any product that may be evaluated in this article, or claim that may be made by its manufacturer, is not guaranteed or endorsed by the publisher.
Supplementary Material
The Supplementary Material for this article can be found online at: https://www.frontiersin.org/articles/10.3389/fphys.2021.688626/full#supplementary-material
Footnotes
- ^ https://www.ncbi.nlm.nih.gov/guide/proteins/
- ^ https://wormbase.org/
- ^ http://flybase.org/
- ^ http://www.ncbi.nlm.nih.gov/orffinder/
- ^ http://smart.embl.de/
- ^ https://web.expasy.org/protparam/
- ^ http://www.geneious.com/
- ^ http://www.ebi.ac.uk/Tools/msa/clustalo/
References
Akanuma, T., and Nishida, H. (2004). Ets-mediated brain induction in embryos of the ascidian Halocynthia roretzi. Dev. Genes Evo. 214, 1–9. doi: 10.1007/s00427-003-0368-y
Anestis, A., Pörtner, H. O., Lazou, A., and Michaelidis, B. (2008). Metabolic and molecular stress responses of sublittoral bearded horse mussel Modiolus barbatus to warming seawater: implications for vertical zonation. J. Exp. Biol. 211, 2889–2898. doi: 10.1242/jeb.016782
Boulton, T. G., Nye, S. H., Robbins, D. J., Ip, N. Y., Radzlejewska, E., Morgenbesser, S. D., et al. (1991). ERKs: a family of protein-serine/threonine kinases that are activated and tyrosine phosphorylated in response to insulin and NGF. Cell 65, 663–675. doi: 10.1016/0092-8674(91)90098-J
Boulton, T. G., Yancopoulos, G. D., Gregory, J. S., Slaughter, C., Moomaw, C., Hsu, J., et al. (1990). An insulin-stimulated protein kinase similar to yeast kinases involved in cell cycle control. Science 249, 64–67.
Cargnello, M., and Roux, P. P. (2011). Activation and function of the MAPKs and their substrates, the MAPK-activated protein kinases. Microbiol. Mol. Biol. Rev. 75, 50–83. doi: 10.1128/MMBR.00031-10
Carroll, D. J., Albay, D. T., Hoang, K. M., O’Neill, F. J., Kumano, M., and Foltz, K. R. (2000). The relationship between calcium, MAP kinase, and DNA synthesis in the sea urchin egg at fertilization. Dev. Biol. 217, 179–191. doi: 10.1006/dbio.1999.9526
Chambon, J. P., Soule, J., Pomies, P., Fort, P., and Baghdiguian, S. (2002). Tail regression in Ciona intestinalis (Prochordate) involves a Caspase-dependent apoptosis event associated with ERK activation. Devlopment 129, 3105–3114. doi: 10.1242/dev.129.13.3105
Chen, C., Chen, H., Zhang, Y., Thomas, H. R., Frank, M. H., He, Y., et al. (2020). TBtools: an integrative toolkit developed for interactive analyses of big biological data. Mol. Plant 13, 1194–1202. doi: 10.1016/j.molp.2020.06.009
Chu, P., Wang, T., Sun, Y., Chu, M., Wang, H., Zheng, X., et al. (2021). Effect of cold stress on the MAPK pathway and lipidomics on muscle of Takifugu fasciatus. Aquaculture 540:736691. doi: 10.1016/j.aquaculture.2021.736691
Ciccarelli, A., and Giustetto, M. (2014). Role of ERK signaling in activity-dependent modifications of histone proteins. Neuropharmacology 80, 34–44. doi: 10.1016/j.neuropharm.2014.01.039
Cuadrado, A., and Nebreda, A. R. (2010). Mechanisms and functions of p38 MAPK signalling. Biochem. J. 429, 403–417. doi: 10.1042/BJ20100323
Dhanasekaran, D. N., and Reddy, E. P. (2008). JNK signaling in apoptosis. Oncogene 27, 6245–6251. doi: 10.1038/onc.2008.301
Dong, Y., Yu, S., Wang, Q., and Dong, S. (2011). Physiological responses in a variable environment: relationships between metabolism, hsp and thermotolerance in an intertidal-subtidal species. PLoS One 6:e26446. doi: 10.1371/journal.pone.0026446
Gaitanaki, C., Kefaloyianni, E., Marmari, A., and Beis, I. (2004). Various stressors rapidly activate the p38-MAPK signaling pathway in Mytilus galloprovincialis (Lam.). Mol. Cell Biochem. 260, 119–127. doi: 10.1023/B:MCBI.0000026064.73671.91
Galbadage, T., Shepherd, T. F., Cirillo, S. L., Gumienny, T. L., and Cirillo, J. D. (2016). The Caenorhabditis elegans p38 MAPK Gene plays a key role in protection from mycobacteria. Microbiologyopen 5, 436–452. doi: 10.1002/mbo3.341
Guo, X., and Luo, Y. (2016). Scallops and scallop aquaculture in China. Dev. Aquac. Fish. Sci. 40, 937–952. doi: 10.1016/B978-0-444-62710-0.00022-5
Hornstein, J., Espinosa, E. P., Cerrato, R. M., Lwiza, K. M., and Allam, B. (2018). The influence of temperature stress on the physiology of the Atlantic surfclam, Spisula solidissima. Comp. Biochem. Phys. A 222, 66–73. doi: 10.1016/j.cbpa.2018.04.011
Jing, L., and Anning, L. (2005). Role of JNK activation in apoptosis: a double-edged sword. Cell Res. 15, 36–42. doi: 10.1038/sj.cr.7290262
Johnson, G. L., and Lapadat, R. (2002). Mitogen-activated protein kinase pathways mediated by ERK, JNK, and p38 protein kinases. Science 298, 1911–1912. doi: 10.1126/science.1072682
Kumar, S., Stecher, G., and Tamura, K. (2016). MEGA7: molecular evolutionary genetics analysis version 7.0 for bigger datasets. Mol. Biol. Evol. 33, 1870–1874. doi: 10.1093/molbev/msw054
Kyriakis, J. M., Banerjee, P., Nikolakaki, E., Dai, T., Rubie, E. A., Ahmad, M. F., et al. (1994). The stress-activated protein kinase subfamily of c-Jun kinases. Nature 369, 156–160. doi: 10.1038/369156a0
Lacchini, A. H., Davies, A. J., Mackintosh, D., and Walker, A. J. (2006). β-1, 3-glucan modulates PKC signalling in Lymnaea stagnalis defence cells: a role for PKC in H2O2 production and downstream ERK activation. J. Exp. Biol. 209, 4829–4840. doi: 10.1242/jeb.02561
Lambert, J. D., and Nagy, L. M. (2003). The MAPK cascade in equally cleaving spiralian embryos. Dev. Biol. 263, 231–241. doi: 10.1016/j.ydbio.2003.07.006
Lau, A. T. Y., and Xu, Y. (2019). Regulation of human mitogen-activated protein kinase 15 (extracellular signal-regulated kinase 7/8) and its functions: a recent update. J. Cell Physiol. 234, 75–88. doi: 10.1002/jcp.27053
Li, Y., Sun, X., Hu, X., Xun, X., Zhang, J., Guo, X., et al. (2017). Scallop genome reveals molecular adaptations to semi-sessile life and neurotoxins. Nat. Commun. 8, 1–11. doi: 10.1038/s41467-017-01927-0
Liu, S., Jiang, X., Hu, X., Gong, J., Hwang, H., and Mai, K. (2004). Effects of temperature on non-specific immune parameters in two scallop species: argopecten irradians (Lamarck 1819) and Chlamys farreri (Jones & Preston 1904). Aquac. Res. 35, 678–682. doi: 10.1111/j.1365-2109.2004.01065.x
Morrison, D. K. (2012). MAP kinase pathways. Cold Spring Harb. Perspect. Biol. 4:a011254. doi: 10.1101/cshperspect.a011254
Napoli, I., Noon, L. A., Ribeiro, S., Kerai, A. P., Parrinello, S., Rosenberg, L. H., et al. (2012). A central role for the ERK-signaling pathway in controlling Schwann cell plasticity and peripheral nerve regeneration in vivo. Neuron 73, 729–742. doi: 10.1016/j.neuron.2011.11.031
Picco, V., Hudson, C., and Yasuo, H. (2007). Ephrin-Eph signalling drives the asymmetric division of notochord/neural precursors in Ciona embryos. Development 134, 1491–1497. doi: 10.1242/dev.003939
Qu, F., Xiang, Z., Li, J., Xiao, S., Mao, F., Qin, Y., et al. (2017). A molluscan extracellular signal-regulated kinase is involved in host response to immune challenges in vivo and in vitro. Fish Shellfish Immun. 62, 311–319. doi: 10.1016/j.fsi.2017.01.038
Qu, F., Xiang, Z., Zhang, Y., Li, J., Xiao, S., Zhang, Y., et al. (2016). A novel p38 MAPK indentified from Crassostrea hongkongensis and its involvement in host response to immune challenges. Mol. Immunol. 79, 113–124. doi: 10.1016/j.molimm.2016.10.001
Rahman, M., Henderson, S., Miller-Ezzy, P., Li, X., and Qin, J. (2019). Immune response to temperature stress in three bivalve species: pacific oyster Crassostrea gigas, Mediterranean mussel Mytilus galloprovincialis and mud cockle Katelysia rhytiphora. Fish Shellfish Immun. 86, 868–874. doi: 10.1016/j.fsi.2018.12.017
Ressurreição, M., Rollinson, D., Emery, A. M., and Walker, A. J. (2011). A role for p38 mitogen-activated protein kinase in early post-embryonic development of Schistosoma mansoni. Mol. Biochem. Parasitol. 180, 51–55. doi: 10.1016/j.molbiopara.2011.07.002
Shui, Y., Xie, J., Zhou, Y., Li, J., and Gan, J. (2020). Molecular characterization of p38 MAPK and tissue-specific expression under cadmium stress in red swamp crayfish (Procambarus clarkii). Sci. Total Environ. 720:137325. doi: 10.1016/j.scitotenv.2020.137325
Shumway, S. E., and Parsons, G. J. (2016). Scallops: Biology, Ecology, Aquaculture, And Fisheries. Amsterdam: Elsevier.
Sluss, H. K., Han, Z., Barrett, T., Davis, R. J., and Ip, Y. T. (1996). A JNK signal transduction pathway that mediates morphogenesis and an immune response in Drosophila. Gene Dev. 10, 2745–2758. doi: 10.1101/gad.10.21.2745
Sun, Y., Zhang, L., Zhang, M., Li, R., Li, Y., Hu, X., et al. (2016). Characterization of three mitogen-activated protein kinases (MAPK) genes reveals involvement of ERK and JNK, not p38 in defense against bacterial infection in Yesso scallop Patinopecten yessoensis. Fish Shellfish Immun. 54, 507–515. doi: 10.1016/j.fsi.2016.04.139
Tian, Y., Liu, J., and Pan, L. (2020). The mechanism of Mitogen-Activated Protein Kinases to mediate apoptosis and immunotoxicity induced by Benzo [a] pyrene on hemocytes of scallop Chlamys farreri in vitro. Fish Shellfish Immun. 102, 64–72. doi: 10.1016/j.fsi.2020.04.006
Tomanek, L., and Zuzow, M. J. (2010). The proteomic response of the mussel congeners Mytilus galloprovincialis and M. trossulus to acute heat stress: implications for thermal tolerance limits and metabolic costs of thermal stress. J. Exp. Biol. 213, 3559–3574. doi: 10.1242/jeb.041228
Wakashin, H., Seo, E., and Seo, Y. (2018). Accumulation and excretion of manganese ion in the kidney of Mytilus galloprovincialis. J. Exp. Biol. 221:jeb185439. doi: 10.1242/jeb.185439
Wang, J., Dong, B., Yu, Z., and Yao, C. (2018). The impact of acute thermal stress on green mussel Perna viridis: oxidative damage and responses. Comp. Biochem. Phys. A 222, 7–15. doi: 10.1016/j.cbpa.2018.04.001
Wei, X., Zhang, Y., Li, C., Ai, K., Li, K., Li, H., et al. (2020). The evolutionarily conserved MAPK/Erk signaling promotes ancestral T-cell immunity in fish via c-Myc-mediated glycolysis. J. Biol. Chem. 295, 3000–3016. doi: 10.1074/jbc.RA119.012231
Weston, C. R., and Davis, R. J. (2007). The JNK signal transduction pathway. Curr. Opin. Cell Biol. 19, 142–149. doi: 10.1016/j.ceb.2007.02.001
Wilbur, K. M., and Yonge, C. M. (2013). Physiology of Mollusca: Volume II. Cambridge, Massachusetts: Academic Press.
Wong, K.-L., Akiyama, R., Bessho, Y., and Matsui, T. (2019). ERK activity dynamics during zebrafish embryonic development. Int. J. Mol. Sci. 20:109. doi: 10.3390/ijms20010109
Wu, J., Ibtisham, F., Niu, Y., Wang, Z., Li, G., Zhao, Y., et al. (2019). Curcumin inhibits heat-induced oxidative stress by activating the MAPK-Nrf2/ARE signaling pathway in chicken fibroblasts cells. J. Therm. Biol. 79, 112–119. doi: 10.1016/j.jtherbio.2018.12.004
Xiao, J., Ford, S. E., Yang, H., Zhang, G., Zhang, F., and Guo, X. (2005). Studies on mass summer mortality of cultured zhikong scallops (Chlamys farreri Jones et Preston) in China. Aquaculture 250, 602–615. doi: 10.1016/j.aquaculture.2005.05.002
Yang, D., Zhao, R., Lin, S., and Sheng, J. (2010). MAPK Response under Low-temperature Stress and Bioinformatic Prediction of LeMAPK Functions. Food Sci. 31, 292–296. doi: 10.1017/S0004972710001772
Yao, C., and Somero, G. N. (2012). The impact of acute temperature stress on hemocytes of invasive and native mussels (Mytilus galloprovincialis and Mytilus californianus): DNA damage, membrane integrity, apoptosis and signaling pathways. J. Exp. Biol. 215, 4267–4277. doi: 10.1242/jeb.073577
Ye, D., Gao, W., Yan, F., Qian, T., and Zhang, Y. (2009). Astragalus injection inhibits c-Jun N terminal kinase mRNA expression following oxygen-glucose deprivation and reintroduction in rat hippocampal neurons. Neural Regen. Res. 4, 879–884. doi: 10.3969/j.issn.1673-5374.2009.11.014
Zhang, S., Yu, J., Wang, H., Liu, B., and Yue, X. (2019). p38 MAPK is involved in the immune response to pathogenic Vibrio in the clam Meretrix petechialis. Fish Shellfish Immun. 95, 456–463. doi: 10.1016/j.fsi.2019.10.048
Keywords: mitogen-activated protein kinase, Chlamys farreri, heat stress, molecular response, bivalve adaptation
Citation: Liu Z, Huang X, Yang Z, Peng C, Yu H, Cui C, Hu Y, Wang X, Xing Q, Hu J and Bao Z (2021) Identification, Characterization, and Expression Analysis Reveal Diverse Regulated Roles of Three MAPK Genes in Chlamys farreri Under Heat Stress. Front. Physiol. 12:688626. doi: 10.3389/fphys.2021.688626
Received: 31 March 2021; Accepted: 04 May 2021;
Published: 28 July 2021.
Edited by:
Guangxu Liu, Zhejiang University, ChinaReviewed by:
Wei Shi, Zhejiang University, ChinaXiaotong Wang, Ludong University, China
Aibin Zhan, Research Center for Eco-environmental Sciences (CAS), China
Copyright © 2021 Liu, Huang, Yang, Peng, Yu, Cui, Hu, Wang, Xing, Hu and Bao. This is an open-access article distributed under the terms of the Creative Commons Attribution License (CC BY). The use, distribution or reproduction in other forums is permitted, provided the original author(s) and the copyright owner(s) are credited and that the original publication in this journal is cited, in accordance with accepted academic practice. No use, distribution or reproduction is permitted which does not comply with these terms.
*Correspondence: Zujing Yang, yzj102553@163.com