- 1College of Plant Protection, Gansu Agricultural University, Lanzhou, China
- 2State Key Laboratory of Green Pesticide and Agricultural Bioengineering, Ministry of Education, Guizhou University, Guiyang, China
It is predicted that the current atmospheric CO2 level will be doubled by the end of this century. Here, we investigate the impacts of elevated CO2 (550 and 750 μL/L) on the development and nutrition status of the green pea aphid for six generations, which is longer than previous studies. All seven examined physiological parameters were not affected over six generations under the ambient CO2 level (380 μL/L). However, the elevated CO2 levels (550 and 750 μL/L) prolonged nymph duration, decreased adult longevity, female fecundity and protein content, and increased the contents of total lipid, soluble sugar and glycogen. There was a significant interaction between the effect of CO2 levels and the effect of generations on nymph duration, female fecundity and adult longevity. The elevated CO2 had immediate effects on the female fecundity and the contents of total protein, total lipid and soluble sugar, starting within F0 generation. The adult longevity decreased, and the glycogen content increased from the F1 generation. However, the significant effect on the nymph development was only observed after three generations. Our study indicates that the elevated CO2 levels first influence the reproduction, the nutrition and the energy supply, then initiate aphid emergency responses by shortening lifespan and increasing glucose metabolism, and finally result in the slow development under further persistent elevated CO2 conditions after three generations, possibly leading to population decline under elevated CO2 conditions. Our results will guide further field experiments under climate change conditions to evaluate the effects of elevated CO2 on the development of the pea aphids and other insects, and to predict the population dynamics of the green pea aphid.
Introduction
A rise in atmospheric carbon dioxide (CO2) concentration is the most conspicuous characteristics of global climate change in this century (Michael, 2019). CO2 concentration is predicted to continue to increase from the current 400 ppm to between 750 and 1,300 ppm by the end of this century (IPCC, 2014). The elevated CO2 will not only accelerate the process of global warming, sea level rise, and climate anomalies, but also affect the survival and distribution of plants and animals on the earth, thus having a profound impact on the entire ecosystem (Bezemer and Jones, 1998; Dury et al., 1998; Guo et al., 2013a).
The effects of elevated atmospheric CO2 concentration on plant nutritional and defensive chemistry, and their consequent effects on insect have received extensive attention (DeLucia et al., 2012; Facey et al., 2014). In particular, unprecedented increases in atmospheric CO2 have the capacity to change plant chemistry, thus, impacting plant nutrients and leading to changes in the synergistic relationship between insects and plants (Robinson et al., 2012; Zavala et al., 2013; Ode et al., 2014). For example, the effect of elevated CO2 on the physiological metabolism of aphid host plant alfalfa (Medicago sativa L.) has been shown previously (Sun et al., 2019), which discovered that the concentrations of soluble protein, soluble carbohydrate and starch were higher when plants grown under elevated CO2, also the concentration of flavone, total polyphenols and simple phenols increased significantly in alfalfa. The concentrations of phenolics, terpenoids, condense tannins, and gossypol were increased in Gossypium hirsutum by elevated CO2 (Coviella et al., 2002). The elevated CO2 mediated the decrease of plant N content resulting in a nutritional deficiency for protein-limited insect pests (Mattson, 1980; Coviella and Trumble, 1999) and reduced fecundity and fitness of most leaf-chewing insects (Coll and Hughes, 2008). The CO2-mediated lower nitrogen content and higher C: N ratio and thus a decrease nutritional quality of Zea mays caused a significant decline in the survival and weight gain as well as larval food consumption of Ostrinia furnacalis (Xie et al., 2015). The growth of Lymantria dispar larvae was significantly inhibited by elevated CO2 and CO2-induced changes in quality of leaves of Populus pseudosimonii and Betula platyphylla (Ji et al., 2011). Similarly, the population density and body mass of the vine weevils (Otiorhynchus sulcatus) decreased under elevated CO2 (Johnson et al., 2011). Johnson et al. (2020) reported that the elevated CO2 accelerated the growth rates of the cotton bollworm (Helicoverpa armigera). Furthermore, insect responses to rising CO2 are not uniform. Robinson et al. (2012) found that while Lepidoptera populations decreased with elevated CO2, the populations of other groups such as Homoptera and Acari actually increased.
Wu et al. (2006) found that the elevated CO2 concentration (750 μL/L) decreased the protein and total amino acid contents of Helicoverpa armigera. Similarly, the study on the larvae of Stegobium paniceum and Lasioderma serricorne showed that the contents of polysaccharide, soluble protein and lipids in the larvae as well as the utilization rate of the larvae decreased with increasing CO2 concentration (Cao et al., 2006). Some studies suggested that the elevated CO2 concentration can increase the relative feeding rate, prolong the development time, decrease the relative growth rate and pupa weight in chewing mouthparts insects (Bidart-Bouzat and Imeth-Nathaniel, 2008; Massad and Dyer, 2010). For example, the larva-to-adult emergence survival rate of Cnaphalocrocis medinalis decreased by 44.0%, and the egg hatching rate reduced by 26.8% under the elevated CO2 as compared to the ambient CO2 (Li et al., 2013).
Aphids are considered to be the most important group of insect pests in temperate regions because of its rapid parthenogenic reproduction and short life cycle. Hughes and Bazzaz (2001) investigated interactions between five species of phloem-feeding aphids (Homoptera: Aphididae) at elevated CO2 (700 μL/L) compared to the ambient CO2 (350 μL/L), and found that Acyrthosiphon pisum population were reduced by over 60% at elevated CO2, in contrast, Myzus persicae populations increased by 120% at elevated CO2, but the CO2 treatment did not significantly affect the populations of the remaining three species (Aphis nerii, Aphis oenotherae, Aulacorthum solani). Also, under elevated CO2, winged aphid adults were produced in all the aphid species except Myzus persicae, but the proportion of winged to wingless was not affected by CO2 levels. The pea aphid, A. pisum Harris, is a worldwide pest that feeds on leguminous crops and forages grasses such as peas, dry beans, alfalfa, clover and fresh beans (Harrington, 1945). Its rapid parthenogenic reproduction and short life cycle enable them to very quickly cause serious economic and production losses of host crops (Losey and Eubanks, 2000; Ryalls et al., 2013). In addition to the direct feeding damage, the pea aphids also transmit 25 types of viruses, including Alfalfa mosaic virus and Pea enation mosaicvirus (Makkouk, 1988). In previous studies, many factors that affect the growth and development of the pea aphid were investigated from different perspectives, including temperature (Hubhachen et al., 2018), photoperiod (Miquel et al., 2019), symbiont (Lv et al., 2018), genetics (Caillaud and Losey, 2010; Wang et al., 2012), and host plant quality (Kordan et al., 2018). Among them rising global CO2 concentrations has become an important climatic factor affecting pea aphid growth, for example, elevated CO2 concentrations altered plant phenolics and thus the performance of aphids (Hong et al., 2020). Studies found that the aphids grown at 380 ppm CO2 had the longest pre-reproductive period and the aphids grown at 1,050 ppm CO2 had the highest intrinsic rate of natural increase (Amiri-jami et al., 2012). Li and Liu (2017) found that developmental duration of three consecutive generations of green pea aphids was shortened, while larval weight, adult weight, weight difference and mean daily relative growth rate all displayed increasing trends under elevated (750 μL/L) CO2. However, the biochemistry and genetic bases of the long-term effect of elevated CO2 on aphid development and nutrients are not investigated.
The objectives of this study are to analyze the independent and interactive effects of two elevated CO2 levels on the development and nutrition content of the pea aphids over six generations. The aim of this study is to provide new and comprehensive insights into the function of CO2 as well as its potential role in control strategies of pea aphids in agricultural systems.
Materials and Methods
Insects and CO2 Treatment
The stock colony of the green pea aphid Acyrthosiphon pisum Harris was from a single parthenogenetic female collected from the alfalfa (Medicago sativa L) field in Experimental Station of the Gansu Agricultural University, Lanzhou, Gansu Province, China. Fresh and clean alfalfa leaves were placed on the filter paper in 9 cm petri dishes with the back facing up and moist absorbent cotton around the petioles and leaf edges to ensure the leaves fresh. Several small holes were drilled on the lid of the petri dish to facilitate the passage of CO2. Three levels of CO2, i.e., ambient CO2 (∼380 μL/L) and elevated CO2 (550 and 750 μL/L), were applied continuously to the petri dishes with the aphids in separate rearing chambers. The CO2 levels were chosen based on the current and predicted levels of CO2 in future years, respectively (Watson et al., 1996).
Determination of Aphid Development for Six Generations
Twenty newly emerged (<6 h) nymph from the aphid stocks were individually placed into a previously prepared petri dish and treated with one of the three CO2 levels for more than 30 days in a rearing chamber. The broad alfalfa leaves were changed every 3 days during the experiment. The observations were made daily at 8 h intervals until the adults died. Then another 20 nymphs (<6 h) in the petri dishes were individually transferred to new petri dishes with fresh leaves and kept for next run of the treatments and observations. This procedure was repeated six times thus six generations (F0–F5) were examined under each CO2 level. This made 20 replicates for each treatment at each generation. Each treatment was repeated three times for each CO2 level. A total of 1,080 nymphs (20 nymphs × 3 treatments × 6 generations × 3 replicates) was used.
The duration of each nymphal stage and the molting during the nymph stages in each petri dish were recorded daily at 8 h intervals until the adults died. The number of nymphs produced per aphid and the adult longevity were recorded when the adults died. Nymphal duration was between hatching and adult emergence.
Sample Preparation for Biochemistry Measurements
A total of 135 adult aphids was collected from each generation for the detections of total protein, soluble sugar, glycogen and lipid under the influence of CO2. Briefly, five adult aphids from each generation were placed into a 1.5 mL centrifuge tube containing 200 μL of the lysis buffer solution (100 mM KH2PO4, 1 mM ethylenediaminetetraacetic acid, and 1 mM dithiothreitol, pH 7.4). The aphids were homogenized and then centrifuged at 10,000 rpm for 10 min at 4°C. The supernatant was then used as one biological sample for the nutritional detections of total protein, sugar, glycogen and lipid. 20 μL of the biological sample was used for the protein content measurement and another 160 μL was used for the measurements of lipids, soluble sugar and glycogen content. This biological sample preparation of biochemistry measurements was repeated for nine times for each of three CO2 treatments (380, 550, and 750 μL/L).
Determination of Total Protein Content
The total protein content of the aphids was detected according to the method of Lowry et al. (1951). About 20 μL of the biological sample was transferred into a 96-well microplate and mixed with of 200 μL Coomassie brilliant blue G-250 for 15 min at room temperature. The optical density (OD) values were measured at 595 nm. Bovine serum albumin was dissolved in the same buffer and diluted into a series of concentrations, which was used as the standard. The total protein contents were calculated based on the standard curve of bovine serum albumin. The experiment was repeated nine times.
Sample Preparation for the Measurements of Total Lipid, Soluble Sugar, and Glycogen Content
Briefly, 160 μL of the biological sample was transferred into a 2 mL centrifuge tube, and 20 μL of 20% sodium sulfate solution (Na2SO4; Sigma) was added, then mixed with 1,500 μL of a chloroform–methanol solution (1:2v/v). This mixture was then centrifuged at 10,000 rpm for 15 min at 4°C. The supernatant was used for the nutritional detection of lipids and soluble sugar, and the precipitant was used for the glycogen content measurement. The preparation was prepared nine times for each CO2 treatment.
Determination of Total Lipids Content
The total lipid content was detected according to the methods of Handel (1985). Briefly, about 100 μL of the supernatant was transferred into a 1 mL centrifuge tube and heated at 90°C until solvent was completely evaporated. 10 μL of 98% concentrated sulfuric acid was then added to the tube and incubated at 90°C for 2 min in a water bath. After cooling the samples on ice, 190 μL of 1.2 g/L vanillin reagent was added and reacted for 15 min at room temperature. The OD value was measured at 525 nm, and the total lipid content was calculated based on the standard curve of triolein. The experiment was repeated nine times.
Determination of Soluble Sugar
The soluble sugar and glycogen were determined according to the methods of Handel (1965) and Handel and Day (1988). To detect the soluble sugar, 150 μL of the supernatant was transferred into a 1.5 mL centrifuge tube and completely evaporated it at room temperature. 10 μL of distilled water and 240 μL of anthrone reagent were added, kept for 15 min at room temperature and then incubated in boiling water for a further 15 min, followed by cooling at room temperature, then added to a 96-well microplate. The OD value at the 630 nm wavelength was measured. The soluble sugar content was calculated based on the standard curve of D-glucose. The experiment was repeated nine times.
Determination of Glycogen Content
The precipitant from pre-preparation was mixed with 400 μL of 80% methanol and sonicated for 10 min to make it turbid. The resultant homogenate was centrifuged again at 10,000 rpm for 4 min at 4°C. Next, the supernatant was transferred to a new 2 mL centrifuge tube and mixed with 1,200 μL of anthrone reagent and incubated for 15 min at room temperature. The samples were heated for 15 min at 90°C in a water bath. The reaction was stopped by cooling with cold water. Finally, the absorbance value of the samples was read at 630 nm and the glycogen content was calculated using D-glucose as standard. The experiment was repeated nine times.
Statistical Analyses
All data were analyzed with the IBM SPSS Statistics version 23.0 for Windows (Chicago, IL, United States). A two-way ANOVA was conducted to examine the effects of CO2 level, generation and the interaction between the CO2 level and the generation on the physiological parameters (nymphal duration, adult longevity, female fecundity and nutrition contents) followed by Tukey’s HSD test was applied to determine differences specific treatments. The general linear model (GML) procedure of SAS was used to compare the means there is a significant interaction effects between generation and CO2 level is identified. Duncan’s Multiple Range Test to compare the means of the physiological parameters if the interaction effect is not significant.
Results
There Is a Significant Interaction Between CO2 Levels and Generation
There was a statistically significant interaction between the effects of CO2 level and generation on the nymph duration (F = 36.3; df = 10; p < 0.001; Table 1). This indicates that the effect CO2 levels on nymph duration is dependent on the generation, and the nymph duration at each generation is dependent on the CO2 levels. Similarly, significant interactions were found for the measurements of adult longevity (F = 13.1; df = 10; p < 0.001), female fecundity (F = 17.3; df = 10; p < 0.001), protein content (F = 20.3; df = 10; p < 0.001) and sugar content (F = 9.5; df = 10; p < 0.001; Table 1). So, to interpret the effect of CO2 levels and generations without inference of each other, one-way ANOVA followed by Tukey’s HSD test was used to separately analyze the effect of CO2 level at each generation and the effect of generation under each CO2 level on each of the performance indices.

Table 1. Two factors ANOVA analysis of the effect of CO2 *generation interaction on performance indices in Acyrthosiphon pisum.
A. pisum Nymph Duration Is Increased by Elevated CO2
Figure 1A shows the Tukey’s HSD test results of the effect of CO2 levels on the nymph duration at each generation. It revealed that the nymph duration was shortened at F0 generation by the elevated CO2 levels [F(2, 6) = 9.5, p = 0.014; Figure 1A]. The nymph duration at F1 generation was not significantly affected by the elevated CO2 levels. It was significantly increased by the elevated CO2 levels only after F3 generation [F(2, 6) = 12.0, p = 0.008; Figure 1A]. At F5 generation, the nymph duration was increased significantly by the elevated CO2 levels from 7.3 ± 0.2 days at 380 μL/L to 10.7 ± 0.5 days at 550 μL/L and 13.1 ± 0.7 days at 750 μL/L [F(2, 6) = 91.5, p < 0.001; Figure 1A].
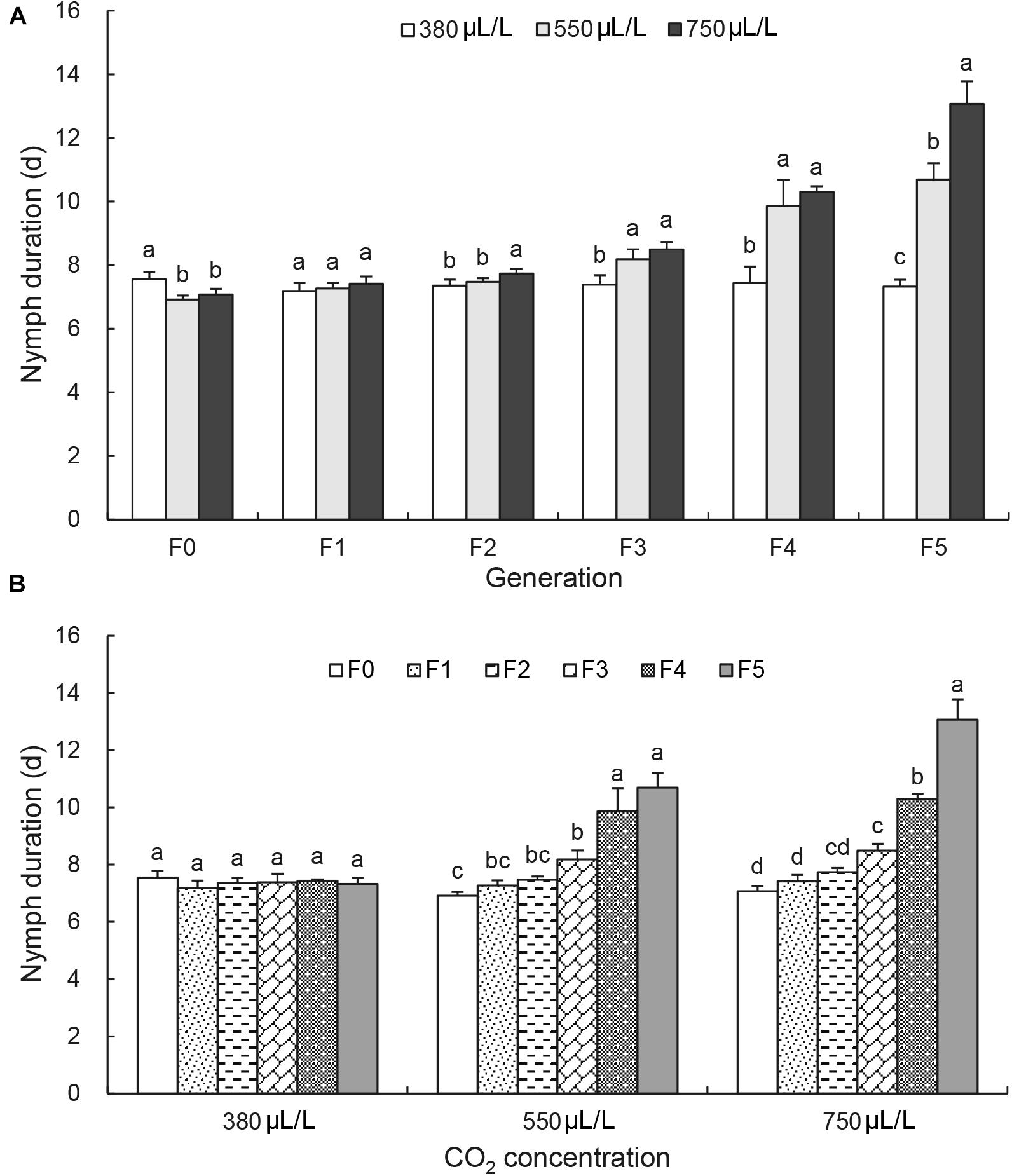
Figure 1. Effects of CO2 and generations on the nymph duration of Acyrthosiphon pisum. (A) Comparison of the effect of CO2 levels (380, 550, and 750 μL/L) at each of six generations (F0–F5). (B) Comparison of the effect of generations under each of CO2 levels (380 μL/L, elevated 550 and 750 μL/L). Different lowercase letters indicate significant differences between CO2 levels at each generation (A) and between generations at each CO2 level (B) as determined by one-way ANOVA Tukey’s HSD test at p < 0.05.
Figure 1B shows the Tukey’s HSD test results of the effect of generations on the nymph duration at each CO2 level. Under the ambient CO2 level 380 μL/L, the nymph duration was not changed and kept a similar length of 7.4 ± 0.3 days throughout 6 generations (Figure 1B). Under elevated CO2, the nymph duration had a significant increase at F4 and F5 generations [F(5, 12) = 38.3, under 550 μL/L CO2 level, p < 0.001; F(5, 12) = 135.5 under 750 μL/L CO2 level, p < 0.001].
A. pisum Adult Longevity Is Decreased by Elevated CO2
The adult longevity was not affected by CO2 levels and remained constant of 20.6 ± 0.8 days at F0 generation (Figure 2A). It was decreased significantly from F1 generation by elevated CO2 levels and reduced from 21.8 ± 0.2 days at 380 μL/L to 15.7 ± 0.6 days at 550 μL/L and 12.3 ± 1.2 days at 750 μL/L at F5 generation [F(2, 6) = 111.3, p < 0.001; Figure 2A]. Similarly, the adult longevity also remained constant days under the ambient CO2 level (380μL/L) over the generations (Figure 2B). However, it started to decrease significantly under the elevated CO2 levels. Under the elevated CO2 level of 750 μL/L, the adult longevity was significantly reduced to 12.3 ± 1.2 days by F5 generation [F(5, 12) = 22.8, p < 0.001; Figure 2B].
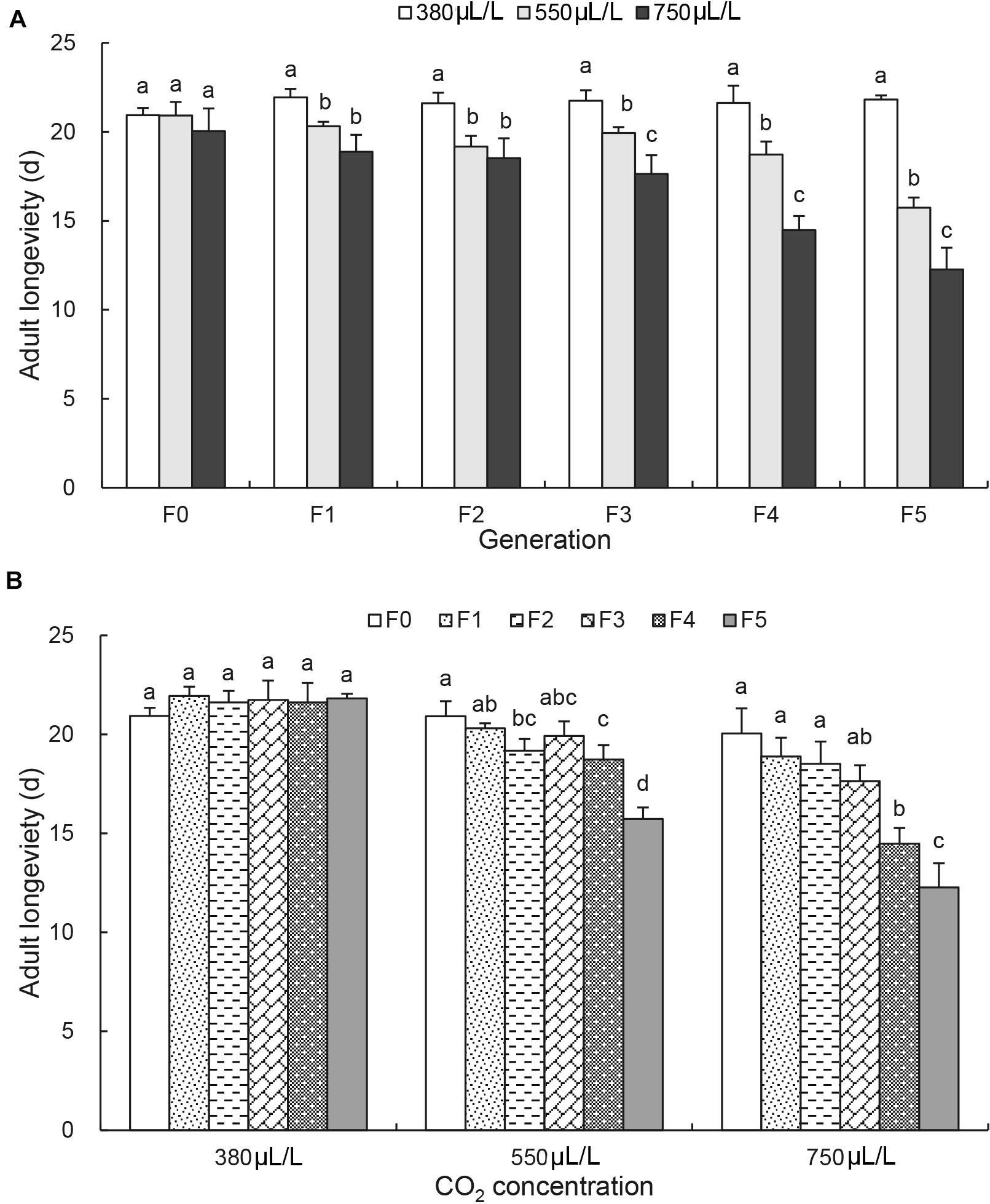
Figure 2. Effects of CO2 and generations on the adult longevity of Acyrthosiphon pisum. (A) Comparison of the effect of CO2 levels (380, 550, and 750 μL/L) at each of six generations (F0–F5). (B) Comparison of the effect of generations under each of CO2 levels (380 μL/L, elevated 550 and 750 μL/L). Different lowercase letters indicate significant differences between CO2 levels at each generation (A) and between generations at each CO2 level (B) as determined by one-way ANOVA Tukey’s HSD test at p < 0.05.
A. pisum Fecundity Is Significantly Reduced by Elevated CO2
At F0 generation, the number of nymphs per female was not affected significantly by 550 μL/L CO2 level but significantly reduced by 750 μL/L CO2 level (Figure 3A). At F1 generation, the reduction of the number of nymphs per female became more obvious under the elevated CO2 levels (Figure 3A). By F5 generation, the number of nymphs per female was reduced significantly from 68.4 ± 2.4 under the ambient CO2 level to 15.5 ± 0.5 under 550 μL/L CO2 level and to 8.5 ± 3.3 under 750 μL/L CO2 level [F(2, 6) = 558.2, p < 0.001; Figure 3A]. There was no reduction in the number of nymphs per female between generations under the ambient CO2 level 380 μL/L (Figure 3B). Significant reduction of the number of nymphs per female was observed between generations under elevated CO2 levels [F(5, 12) = 58.9 under 550 μL/L CO2 level, p < 0.001; F(5, 12) = 30.9 under 750 μL/L CO2 level, p < 0.001] when the effect of CO2 levels was analyzed (Figure 3B).
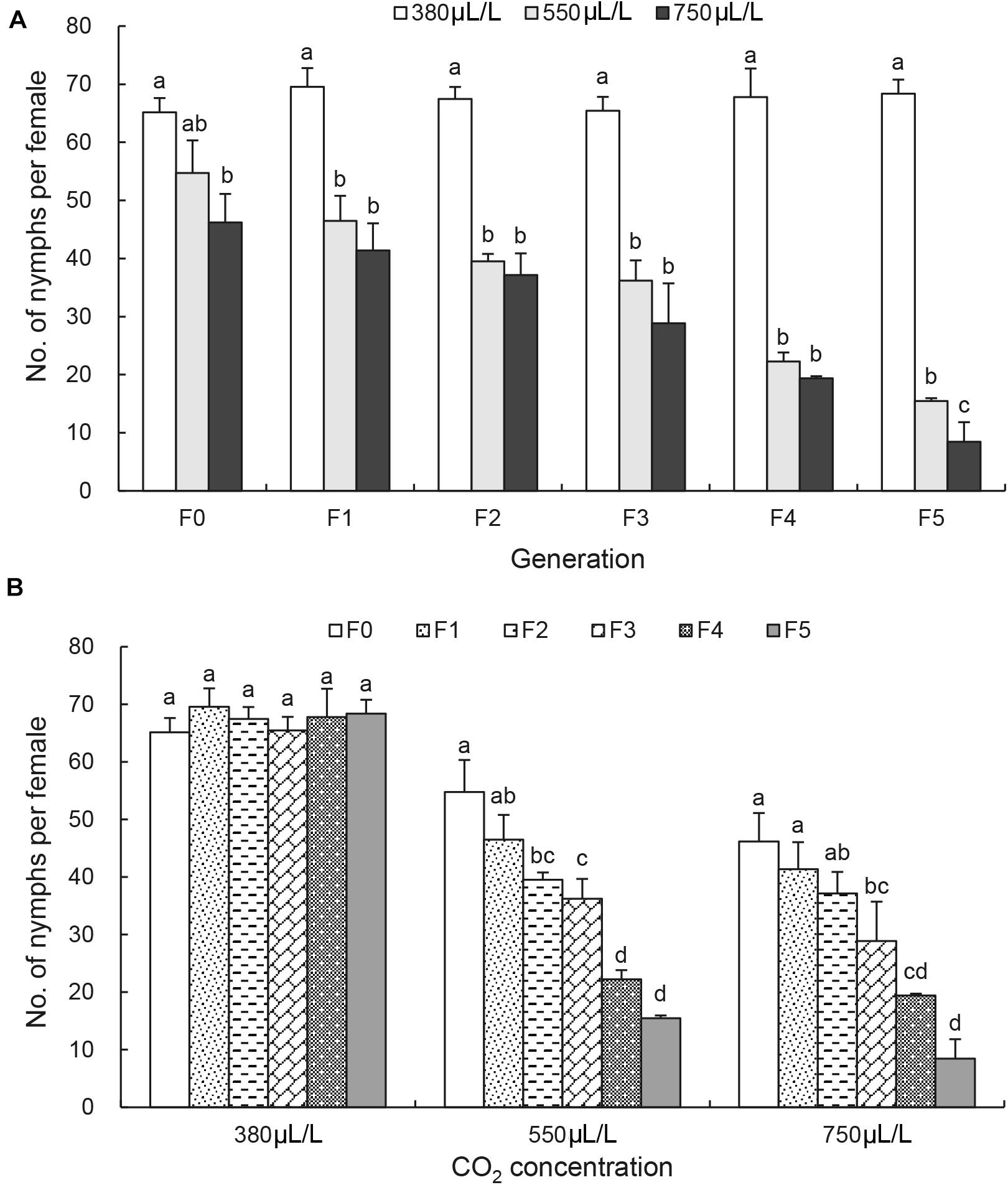
Figure 3. Effects of CO2 and generations on the female fecundity of Acyrthosiphon pisum. (A) Comparison of the effect of CO2 levels (380, 550, and 750 μL/L) at each of six generations (F0–F5). (B) Comparison of the effect of generations under each of CO2 levels (380 μL/L, elevated 550 and 750 μL/L). Different lowercase letters indicate significant differences between CO2 levels at each generation (A) and between generations at each CO2 level (B) as determined by one-way ANOVA Tukey’s HSD test at p < 0.05.
A. pisum Total Protein Content Is Reduced Under Elevated CO2
The elevated CO2 levels significantly reduced the total protein contents even at F0 generation [F(2, 24) = 127.6, p < 0.001; Table 2 and Supplementary Figure 1A]. The total protein content under the ambient CO2 was not affected throughout the generations (Table 2 and Supplementary Figure 1B). Under the elevated CO2 level of 550μL/L, the total protein contents were significantly reduced from 19.7 ± 0.6 μg/aphid at F0 generation to 13.6 ± 0.5 μg/aphid at F5 generation [F(5, 48) = 176.9, p < 0.001]. Under the elevated CO2 level of 750 μL/L, it was reduced from 16.6 ± 0.6 μg/aphid at F0 generation to 12.5 ± 0.8 μg/aphid at F3 generation and reached 11.4 ± 0.9 μg/aphid at F5 generation [F(5, 48) = 61.9, p < 0.001; Table 2 and Supplementary Figure 1B].
A. pisum Total Lipid Content Is Not Affected Under Elevated CO2
The total content of total lipids was significantly increased even at F0 generation by both elevated CO2 levels [F(2, 24) = 5.4, p = 0.012; Table 2 and Supplementary Figure 2A]. At F5 generation, it was increased from 6.4 ± 0.7 μg/aphid under 380 μL/L level to 7.9 ± 0.5 μg/aphid under 550 μL/L level and to 8.0 ± 0.6 μg/aphid under 750 μL/L level [F(2, 24) = 17.2, p < 0.001]. The total content of lipids was unchanged over the generations under the ambient and elevated CO2 levels (Table 2 and Supplementary Figure 2B). The total lipid contents increased by 14.9–23.4% and 13.8–25.0% across generations under the elevated CO2 levels of 550 and 750 μL/L, respectively, relative to those under the ambient CO2 level.
A. pisum Sugar Content Is Increased Under Elevated CO2
The content of soluble sugar and glycogen were significantly increased at each generation (Table 2 and Supplementary Figures 3A, 4A) but were not affected by the elevated CO2 levels over generations (Table 2 and Supplementary Figures 3B, 4B). The significant increase was observed at F0 generation for the soluble sugar content from 25.4 ± 0.6 μg/aphid at 380 μL/L level to 33.7 ± 1.0 μg/aphid by 550 μL/L level and to 36.8 ± 0.5 μg/aphid by 750 μL/L level [F(2, 24) = 557.8, p < 0.001]. At F5 generation, the soluble sugar was increased from 25.6 ± 0.4 μg/aphid under the ambient CO2 to approximately 40.3 ± 0.5 μg/aphid by the elevated CO2 750 μL/L (Table 2).
Under both elevated CO2 levels, the content of soluble sugar was slightly and significantly increased after F2 generation under 550 μL/L level [F(5, 48) = 24.8, p < 0.001] and after F4 generation under 750 μL/L level [F(5, 48) = 26.9, p < 0.001; Table 2 and Supplementary Figure 3B].
A. pisum Glycogen Content Is Significantly Increased Under Elevated CO2
The glycogen content was significantly increased from F1 generation (Table 2 and Supplementary Figure 4A). It was significantly increased from 2.0 ± 0.1 μg/aphid at 380 μL/L level to 2.6 ± 0.3 μg/aphid by 750 μL/L [F(2, 24) = 5.7, p = 0.010]. At F5 generation, the glycogen content increased from 2.0 ± 0.1 μg/aphid under the ambient CO2 to approximately 2.8 ± 0.1 μg/aphid by the elevated CO2 750 μL/L (Table 2). The glycogen content was not affected by the elevated CO2 levels over generations even under the higher elevated CO2 level 750 μL/L (Table 2 and Supplementary Figure 4B).
Discussion
The current study reports the long-term effects of elevated CO2 levels on the development and nutritional dynamics of the pea aphid A. pisum over six generations. It confirms that the pea aphid is well adapted to the current environmental CO2 level as all seven examined physiological parameters were not affected over 6 generations under the ambient CO2 level. However, the elevated CO2 levels (550 and 750 μL/L) prolonged the nymph duration (Figure 1), decreased the adult longevity, the female fecundity and the protein content (Figures 2, 3 and Table 2), and increased the contents of total lipid, soluble sugar and glycogen (Table 2).
The elevated CO2 had an immediate effect on the female fecundity and the contents of total protein, total lipid and sugar, starting within F0 generation. In the current study, only total protein and total lipid contents were analyzed. It is not known whether or not they are influenced by the changes of vitellogenin and triacylglycride. Triacylglycerols is a major lipid in aphid fat body as energy reserves (Ward et al., 1981) and accumulated in aphid fat body under stress (Bergman et al., 1991; Chen et al., 2005). Vitellogenin is a major yolk protein (Arrese and Soulages, 2010) and directly regulates egg maturation and affect ovary development and decrease ovulation (Ge et al., 2019; Huang et al., 2019).
The adult longevity decreased, and the glycogen content increased from F1 generation. However, the significant effect on the nymph development was only observed after three generations (Figure 1A). Furthermore, the interactions between the CO2 levels and the generations significant influenced nymph duration, female fecundity and adult longevity. The biggest effect of CO2 was found on the female fecundity by the generations (Figure 3A), indicating that the elevated CO2 may not be conducive to the reproduction of the aphids. The results are consistent with that of the study in Cnaphalocrocis medinalis, where elevated CO2 reduced the survival rate from larva to adult emergence by 44.0% compared with ambient CO2 (Li et al., 2013). Docherty et al. (1997) also reported a significantly reduced fecundity in Phyllaphis fagi under the elevated CO2 level of 600 μL/L. However, it was reported that elevated CO2 levels could increase Myzus persicae population (Hughes and Bazzaz, 2001) and Nilaparvata lugens population (Xiao et al., 2011). It is possible that the elevated CO2 levels first influence the reproduction, the energy supply and the nutrition status, and then initiation of shortening lifespan and increasing glycogen transition to glucoses for emergency escaping, and finally result in the slow development of the pea aphid under persistent elevated CO2 conditions, possibly leading to population decline under elevated CO2 conditions.
Insects store nutrition in the form of proteins, carbohydrates, glycogen and lipids (Ahsaei et al., 2014). Nutrition storage in insects has significant implications for their survival and reproduction (Makkouk, 1988). Our results show a significant reduction of protein content by the elevated CO2 levels (Table 2). This is consistent with previous reports that the fertility of the pea aphid was closely related to the protein contents in the body (Ahsaei et al., 2013). For example, the reproduction ability of the pea aphids was positively proportional to its protein content: the greater protein content, the higher the reproduction ability (Raikhel and Dhadialla, 1992). The protein content of Nilaparvata lugens declined under elevated CO2, speculated that it would be difficult for it to obtain nutrients and energy after feeding on rice which grown under high CO2 concentrations (Zeng et al., 2012). Similarly, elevated CO2 decreased the protein content of Helicoverpa armigera larvae by 14.16% (Wu et al., 2006). Therefore, we propose that the elevated CO2 inhibited protein synthesis in the aphids, and directly led to the reduction of protein contents, thus further caused the longer nymph duration and lower female fecundity of the pea aphids in elevated CO2 levels than those of the aphids under the ambient CO2.
The total lipid and soluble sugar contents were significantly increased by the elevated CO2 levels at F0 generation (Table 2). Interestingly, over the generations, they were not significantly changed under the elevated levels of CO2, neither generation nor its interaction with CO2 level had a significant effect on them (Table 2 and Supplementary Figures 2B, 3B). However, the total lipid and soluble sugar contents were significantly higher under the elevated CO2 levels than those under the ambient CO2 condition at each generation (Table 2 and Supplementary Figures 2A, 3A). The function of soluble sugar is to offer nutrition for the energy requirement of the muscles when an insect is walking or escaping (Hansford and Johnson, 1975). These increased responses to elevated CO2 could be the initiation for their escaping from unsuitable environment. Our study results show that glycogen content increased with the elevated CO2 concentration in the pea aphid, which could reduce the feeding rate of the aphids, thus longer nymph duration and reduced adult fecundity under elevated CO2 than that under the ambient CO2.
On other hands, elevated CO2 can lead to the changes of plant secondary metabolism, thus affect the insect physiology indirectly (Bidart-Bouzat and Imeth-Nathaniel, 2008; Todgham and Stillman, 2013). The soluble sugars in the aphid body are mainly from the sap of the phloem of the host plant (Li et al., 2008). Elevated CO2 could enhance plant biomass and leaf area, so soluble sugar levels increase, leading to enhance the behavior of the pea aphids (Curtis and Wang, 1998). The reduced metabolic efficiency of plants under elevated CO2 could weaken the feeding of insect herbivores and slow the growth of insects (Vuorinen et al., 2004). Elevated CO2 could also increase the concentrations of various phenolic compounds, which would act to reduce the pest population and affect natural enemies (Koricheva et al., 1998). For example, the content of flavone, phenolics and condensed tannins and gossypol were higher in the aphid host plant the alfalfa, Medicago sativa (L.) grown under elevated CO2 (Sun et al., 2019). Carbohydrate content in plants increased with elevated atmospheric CO2 due to higher photosynthetic rates (Reddy et al., 2010; Kimball, 2016). Elevated CO2 increased carbon to nitrogen ratio of plant tissues (Wilsey, 1996). This causes lower nitrogen concentrations in plants and leads to less amino acids available to aphids (Guo et al., 2013b; Ziska et al., 2016). It is widely recognized that the reproductive ability and abundance of aphids are largely determined by the availability of amino acids in their diet (Jansson and Smilowitz, 1986), and the nutritional quality and resistance of host plants (Guo et al., 2014).
In the current study, the significant effects of the elevated CO2 on the growth of the aphids fed with alfalfa leaves were studied for over 6 generations, which is longer than previous studies of 3 generations. Our study clearly demonstrates that the elevated CO2 affect some parameters such as nymph developmental duration after 3 generations and shows that the final consequence of the elevated CO2 on aphid population dynamics is combinatory and long time. The results will guide further field experiments to evaluate the effects of the elevated CO2 conditions on the development of the pea aphids and other insects under climate change conditions.
Data Availability Statement
The original contributions presented in the study are included in the article/Supplementary Material, further inquiries can be directed to the corresponding author/s.
Ethics Statement
Informed consent was obtained from all individual participants included in the study. The research project was conducted on invertebrate species that are not subjected to any specific ethical issue and legislation.
Author Contributions
CuL, QS, and CaL conceived and designed research. CuL, QS, and QZ conducted the experiments. YG and KZ contributed new reagents and analytical tools. CuL and J-JZ analyzed the data. CuL and CaL wrote the manuscript. J-JZ made critical revision, proofreading, and replying comments. All authors read and approved manuscript.
Funding
This work was financially supported by the National Science Foundation of China (31960351, 31660522, and 31960227) and Program of Introducing Talents to Chinese Universities (111 Program No. D20023) to J-JZ.
Conflict of Interest
The authors declare that the research was conducted in the absence of any commercial or financial relationships that could be construed as a potential conflict of interest.
Acknowledgments
We would like to thank all of the participants who volunteered their time in the study. We appreciate very much the valuable comments and suggestion by the reviewers and editors.
Supplementary Material
The Supplementary Material for this article can be found online at: https://www.frontiersin.org/articles/10.3389/fphys.2021.688220/full#supplementary-material
References
Ahsaei, S. M., Hosseininaveh, V., Talaei-Hassanlouei, R., and Bigham, M. (2014). Changes in energy content of Podisus maculiventris (Say) (Hemiptera: Pentatomidae) in response to different diets. Arthropods 3, 166–173.
Ahsaei, S. M., Tabadkani, S. M., Hosseininaveh, V., Allahyari, H., and Bigham, M. (2013). Differential accumulation of energy by the colour morphs of the pea aphid Acyrthosiphon pisum (Hemiptera: Aphididae) mirrors their ecological adaptations. Eur. J. Entomol. 110, 241–245. doi: 10.14411/eje.2013.035
Amiri-jami, A. R., Sadeghi, H., and Shoor, M. (2012). The performance of Brevicoryne brassicae on ornamental cabbages grown in CO2-enriched atmospheres. J. Asia Pac. Entomol. 15, 249–253. doi: 10.1016/j.aspen.2012.01.001
Arrese, E. L., and Soulages, J. L. (2010). Insect fat body: energy, metabolism, and regulation. Annu. Rev. Entomol. 55, 207–225. doi: 10.1146/annurev-ento-112408-085356
Bergman, D. K., Dillwith, J. W., and Berberet, R. C. (1991). Spotted alfalfa aphid, Therioaphis maculata, fatty acids relative to the condition and susceptibility of its host. Arch. Insect Biochem. Physiol. 18, 1–12. doi: 10.1002/arch.940180102
Bezemer, T. M., and Jones, T. H. (1998). Plant-insect herbivore interactions in elevated atmospheric CO2: quantitative analyses and guild effects. Oikos 82, 212–222. doi: 10.2307/3546961
Bidart-Bouzat, M., and Imeth-Nathaniel, A. (2008). Global change effects on plant chemical defenses against insect herbivores. J. Integr. Plant Biol. 50, 1339–1354. doi: 10.1111/j.1744-7909.2008.00751.x
Caillaud, M. C., and Losey, J. E. (2010). Genetics of color polymorphism in the pea aphid, Acyrthosiphon pisum. J. Insect Sci. 10:95. doi: 10.1673/031.010.9501
Cao, Y., Liu, Y., Wang, L., Ran, G., Shang, B., and Li, C. (2006). The influence of CO2 on relevant indexes of energy substance utilization in the larvae of Stegobium paniceum and Lasioderma serricorne. Acta Sci. Nat. Univ. Sunyatseni 55, 123–129.
Chen, Z., Madden, R. D., and Dillwith, J. W. (2005). Effect of precocene II on fatty acid metabolism in the pea aphid, Acyrthosiphon pisum, under cold stress. J. Insect Physiol. 51, 411–416. doi: 10.1016/j.jinsphys.2005.02.006
Coll, M., and Hughes, L. (2008). Effects of elevated CO2 on an insect omnivore: a test for nutritional effects mediated by host plants and prey. Agric. Ecosyst. Environ. 123, 271–279. doi: 10.1016/j.agee.2007.06.003
Coviella, C. E., Stipanovic, R. D., and Trumble, J. T. (2002). Plant allocation to defensive compounds: interactions between elevated CO2 and nitrogen in transgenic cotton plants. J. Exp. Bot. 53, 323–331. doi: 10.1093/jexbot/53.367.323
Coviella, C. E., and Trumble, J. T. (1999). Effects of elevated atmospheric carbon dioxide on insect-plant interactions. Conserv. Biol. 13, 700–712. doi: 10.1046/j.1523-1739.1999.98267.x
Curtis, P. S., and Wang, X. (1998). A meta-analysis of elevated CO2 effects on woody plant mass, form, and physiology. Oecologia 113, 299–313. doi: 10.1007/s004420050381
DeLucia, E. H., Nabity, P. D., Zavala, J. A., and Berenbaum, M. R. (2012). Climage change: resetting plant–insect interactions. Plant Physiol. 160, 1677–1685. doi: 10.1104/pp.112.204750
Docherty, M., Wade, F., Hurst, D., Whittaker, J., and Lea, P. (1997). Responses of tree sap-feeding herbivores to elevated CO2. Glob. Change Biol. 3, 51–59. doi: 10.1046/j.1365-2486.1997.00096.x
Dury, S. J., Good, J. E. G., Perrins, C. M., Buse, A., and Kaye, T. (1998). The effects of increasing CO2 and temperature on oak leaf palatability and the implications for herbivorous insects. Glob. Change Biol. 4, 55–61. doi: 10.1046/j.1365-2486.1998.00102.x
Facey, S. L., Ellsworth, D. S., Staley, J. T., Wright, D. J., and Johnson, S. N. (2014). Upsetting the order: how climate and atmospheric change affects herbivore-enemy interactions. Curr. Opin. Insect Sci. 5, 66–74. doi: 10.1016/j.cois.2014.09.015
Ge, L. Q., Zheng, S., Gu, H. T., Zhou, Y. K., Zhou, Z., Song, Q. S., et al. (2019). Jinggangmycin- induced UDP-glycosyltransferase 1-2-like is a positive modulator of fecundity and population growth in Nilaparvata lugens (Stål) (Hemiptera: Delphacidae). Front. Physiol. 10:747. doi: 10.3389/fphys.2019.00747
Guo, H., Liu, X. M., Guo, X. L., Liu, Z., Ma, M. M., Lyu, B., et al. (2013a). A preliminary analysis on the relationship between CO2 concentrations and global climate change. J. Subtrop. Resour. Environ. 8, 1–13.
Guo, H., Sun, Y., Li, Y., Liu, X., Zhang, W., and Ge, F. (2014). Elevated CO2 decreases the response of the ethylene signaling pathway in Medicago truncatula and increases the abundance of the pea aphid. New Phytol. 201, 279–291. doi: 10.1111/nph.12484
Guo, H. Y., Sun, Y., Li, B., Tong, M., Harris, K., Zhu-Salzman, K., et al. (2013b). Pea aphid promotes amino acid metabolism both in Medicago truncatula and bacteriocytes to favor aphid population growth under elevated CO2. Glob. Change Biol. 19, 3210–3223. doi: 10.1111/gcb.12260
Handel, V. E. (1965). Microseparation of glycogen, sugars, and lipids. Anal. Biochem. 11, 266–271. doi: 10.1016/0003-2697(65)90014-X
Handel, V. E. (1985). Rapid determination of total lipids in mosquitoes. J. Am. Mosq. Control Assoc. 1, 302–304.
Handel, V. E., and Day, J. (1988). Assay of lipids, glycogen and sugars in individual mosquitoes: correlations with wing length in field-collected Aedes vexans. J. Am. Mosq. Control Assoc. 4, 549–550.
Hansford, R. G., and Johnson, R. N. (1975). The nature and control of the tricarboxylate cycle in beetle flight muscle. Biochem. J. 148, 389–401. doi: 10.1042/bj1480389
Harrington, C. D. (1945). Biological races of the pea aphid. J. Econ. Entomol. 38, 12–22. doi: 10.1093/jee/38.1.12
Hong, Y. Y., Hong, G. G., Yu, C. S., and Feng, G. (2020). Plant phenolics mediated bottom-up effects of elevated CO2 on Acyrthosiphon pisum and its parasitoid Aphidius avenae. Insect Sci. 27, 170–184. doi: 10.1111/1744-7917.12627
Huang, B., Shi, Z. H., and Hou, Y. M. (2019). Tradeoff between triglyceride consumption and ovariole development in Plutella xylostella (L.) released in mixed-host environments. J. Integr. Agric. 18, 865–872. doi: 10.1016/S2095-3119(17)61842-2
Hubhachen, Z., Madden, R. D., and Dillwith, J. W. (2018). Influence of rearing temperature on triacylglycerol storage in the pea aphid, Acyrthosiphon pisum. Arch. Insect Biochem. Physiol. 99:e21495. doi: 10.1002/arch.21495
Hughes, L., and Bazzaz, F. A. (2001). Effects of elevated CO2 on five plant-aphid interactions. Entomol. Exp. Appl. 99, 87–96. doi: 10.1046/j.1570-7458.2001.00805.x
IPCC (2014). Contribution of Working Groups I, II and III to the 5th Assessment Report of the Intergovernmental Panel on Climate Change. Climate Change 2014: Synthesis Report. Geneva: IPCC.
Jansson, R. K., and Smilowitz, Z. (1986). Influence of nitrogen on population parameters of potato insects: abundance, population growth, and within-plant distribution of the green peach aphid, Myzus persicae (Homoptera: Aphididae). Environ. Entomol. 15, 49–55. doi: 10.1093/ee/15.1.49
Ji, L. Z., An, L. L., and Wang, X. W. (2011). Growth responses of gypsy moth larvae to elevated CO2: the influence of methods of insect rearing. Insect Sci. 18, 409–418. doi: 10.1111/j.1744-7917.2011.01419.x
Johnson, S. N., Barton, A. T., Clark, K. E., Gregory, P. J., Mcmenemy, L. S., and Hancock, R. D. (2011). Elevated atmospheric carbon dioxide impairs the performance of root-feeding vine weevils by modifying root growth and secondary metabolites. Glob. Change Biol. 17, 688–695. doi: 10.1111/j.1365-2486.2010.02264.x
Johnson, S. N., Waterman, J. M., and Hall, C. R. (2020). Author correction: increased insect herbivore performance under elevated CO2 is associated with lower plant defence signalling and minimal declines in nutritional quality. Sci. Rep. 10:21783. doi: 10.1038/s41598-020-70823-3
Kimball, B. A. (2016). Crop responses to elevated CO2 and interactions with H2O, N, and temperature. Curr. Opin. Plant Biol. 31, 36–43. doi: 10.1016/j.pbi.2016.03.006
Kordan, B., Stec, K., Słomiński, P., Giertych, M. J., Wróblewska-Kurdyk, A., and Gabrys, B. (2018). Susceptibility of forage legumes to infestation by the pea aphid Acyrthosiphon pisum (Harris) (Hemiptera: Aphididae). Crop Pasture Sci. 69, 775–784. doi: 10.1071/CP18065
Koricheva, J., Larsson, S., Haukioja, E., and Keinanen, M. (1998). Regulation of woody plant secondary metabolism by resource availability: hypothesis testing by means of meta-analysis. Oikos 83, 212–226. doi: 10.2307/3546833
Li, B. P., Guo, Q., and Meng, L. (2013). Effects of elevated CO2 concentration on development, reproduction and food utilization of the Cnaphalocrocis medinalis Guenée (Lepidoptera: Pyralidae). Sci. Agric. Sin. 46, 4464–4470.
Li, J. B., Fang, L. P., Lǘ, Z. Z., and Zhang, Z. (2008). Relationships between the cotton resistance to the cotton aphid (Aphis gossypii) and the content of soluble sugars. Plant Prot. 34, 26–30.
Li, R. H., and Liu, C. Z. (2017). Effects of elevated CO2 on development and reproduction of green pea aphid (Acythosiphon pisum). Acta Prataculturae Sin. 26, 111–120.
Losey, J. E., and Eubanks, M. D. (2000). Implications of pea aphid host-plant specialization for the potential colonization of vegetables following post-harvest emigration from forage crops. Environ. Entomol. 29, 1283–1288. doi: 10.1603/0046-225X-29.6.1283
Lowry, O. H., Rosebroug, N., Farr, A., and Randdall, R. J. (1951). Protein measurement with the Folin phenol reagent. J. Biol. Chem. 193, 267–275. doi: 10.1016/S0021-9258(19)52451-6
Lv, N., Wang, L., Sang, W., Liu, C. Z., and Qiu, B. L. (2018). Effects of endosymbiont disruption on the nutritional dynamics of the pea aphid Acyrthosiphon pisum. Insects 9, 161–171. doi: 10.3390/insects9040161
Makkouk, K. (1988). “Viruses and virus diseases of pea, lentil, faba bean and chickpea,” in World Crops: Cool Season Food Legumes, Vol. 5, eds R. J. Summerfield (Dordrecht: Springer), 591–615. doi: 10.1007/978-94-009-2764-3_49
Massad, T. J., and Dyer, L. A. (2010). A meta-analysis of the effects of global environmental change on plant-herbivore interactions. Arthropod Plant Interact. 4, 181–188. doi: 10.1007/s11829-010-9102-7
Mattson, W. J. (1980). Herbivory in relation to plant nitrogen content. Annu. Rev. Ecol. Syst. 11, 119–161. doi: 10.1146/annurev.es.11.110180.001003
Michael, A. (2019). Are changes in plants due to enhanced CO2 contributing to insect population declines? Environ. Entomol. 48, 274–275. doi: 10.1093/ee/nvz021
Miquel, B., Rubén, C. C., and David, M. T. (2019). Insulin-like peptides in volved in photoperiodism in the aphid Acyrthosiphon pisum. Insect Biochem. Mol. Biol. 112:103185. doi: 10.1016/j.ibmb.2019.103185
Ode, P. J., Johnson, S. N., and Moore, B. D. (2014). Atmospheric change and induced plant secondary metabolites-are we reshaping the building blocks of multi-trophic interactions? Curr. Opin. Insect Sci. 5, 57–65. doi: 10.1016/j.cois.2014.09.006
Raikhel, A. S., and Dhadialla, T. S. (1992). Accumulation of yolk proteins in insect oocytes. Annu. Rev. Entomol. 37, 217–251. doi: 10.1146/annurev.en.37.010192.001245
Reddy, A. R., Rasineni, G. K., and Raghavendra, A. S. (2010). The impact of global elevated CO2 concentration on photosynthesis and plant productivity. Curr. Sci. 99, 46–57.
Robinson, E. A., Ryan, G. D., and Newman, J. A. (2012). A meta-analytical review of the effects of elevated CO2 on plant-arthropod interactions highlights the importance of interacting environmental and biological variables. New Phytol. 194, 321–336. doi: 10.1111/j.1469-8137.2012.04074.x
Ryalls, J. M. W., Riegler, M., Moore, B. D., and Johnson, S. N. (2013). Biology and trophic interactions of lucerne aphids. Agric. For. Entomol. 15, 335–350. doi: 10.1111/afe.12024
Sun, Q., Zhang, T. W., Wei, J. Y., Lyu, Y. Q., and Liu, C. Z. (2019). Effects of elevated CO2 concentration on nutrients and secondary metabolites in Medicago sativa leaf under different damage degrees of pea aphid (Acyrthosiphon pisum, Hemiptera: Aphididae). Chin. J. Eco Agric. 27, 92–99.
Todgham, A. E., and Stillman, J. H. (2013). Physiological responses to shifts in multiple environmental 539 stressors: relevance in a changing world. Integr. Comp. Biol. 53, 539–544. doi: 10.1093/icb/ict086
Vuorinen, T., Nerg, A. M., Ibrahim, M. A., Reddy, G. V. P., and Holopainen, J. K. (2004). Emission of Plutella xylostella-induced compounds from cabbages grown at elevated CO2 and orientation behavior of the natural enemies. Plant Physiol. 135, 1984–1992. doi: 10.1104/pp.104.047084
Ward, J. P., Candy, D. J., and Smith, S. N. (1981). Lipid storage and changes during flight by triatomine bugs (Rhodnius prolixus and Triatoma infestans). J. Insect Physiol. 28, 527–534. doi: 10.1016/0022-1910(82)90033-6
Watson, R. T., Zinyowera, M. C., and Moss, R. H. (1996). Intergovernmental Panel on Climate Change. Climate Change1995: Impacts, Adaptations and Mitigation of Climate Change: Scientific-Technical Analysis. Cambridge: Cambridge University Press, 3–18.
Wang, G., Guo, Y. P., Guo, S. F., Zhou, J. J., and Liu, C. Z. (2012). RNA interference of trehalose-6-phosphate synthase and trehalase genes regulates chitin metabolism in two color morphs of Acyrthosiphon pisum Harris. Sci Rep. 11, 948. doi: 10.1038/s41598-020-80277-2
Wilsey, B. J. (1996). Plant responses to elevated atmospheric CO2 among terrestrial biomes. Oikos 76, 201–205. doi: 10.2307/3545764
Wu, G., Chen, F. J., and Ge, F. (2006). Direct effects of elevated CO2 on growth, development and reproduction of cotton bollworm Helicoverpa armigera Hǘbner. Acta Ecol. Sin. 26, 1732–1738.
Xiao, N. C., Wei, H., Neng, W. X., Jun, S. L., Lan, Z. H., and Fa, J. C. (2011). Effects of elevated CO2 and transgenic Bt rice on yeast-like endosymbiote and its host brown planthopper. J. Appl. Entomol. 135, 333–342. doi: 10.1111/j.1439-0418.2010.01558.x
Xie, H., Liu, K., Sun, D., Wang, Z., Lu, X., and He, K. (2015). A field experiment with elevated atmospheric CO2-mediated changes to C4 crop-herbivore interactions. Sci. Rep. 5:13923. doi: 10.1038/srep13923
Zavala, J. A., Nabity, P. D., and DeLucia, E. H. (2013). An emerging understanding of mechanisms governing insect herbivory under elevated CO2. Annu. Rev. Entomol. 58, 79–97. doi: 10.1146/annurev-ento-120811-153544
Zeng, Y. Y., Huang, W. K., Su, L., Wu, G., Zhuang, J., Zhao, W. Y., et al. (2012). Effects of high CO2 concentration on nutrients and enzyme activities of Nilaparvata lugens. Sci. Sin. Vitae 42, 739–745.
Keywords: Acyrthosiphon pisum, elevated CO2, generation, development, nutrition
Citation: Li C, Sun Q, Gou Y, Zhang K, Zhang Q, Zhou J-J and Liu C (2021) Long-Term Effect of Elevated CO2 on the Development and Nutrition Contents of the Pea Aphid (Acyrthosiphon pisum). Front. Physiol. 12:688220. doi: 10.3389/fphys.2021.688220
Received: 30 March 2021; Accepted: 12 May 2021;
Published: 04 June 2021.
Edited by:
Bin Tang, Hangzhou Normal University, ChinaReviewed by:
Ali R. Bandani, University of Tehran, IranYongliang Fan, Northwest A&F University, China
Copyright © 2021 Li, Sun, Gou, Zhang, Zhang, Zhou and Liu. This is an open-access article distributed under the terms of the Creative Commons Attribution License (CC BY). The use, distribution or reproduction in other forums is permitted, provided the original author(s) and the copyright owner(s) are credited and that the original publication in this journal is cited, in accordance with accepted academic practice. No use, distribution or reproduction is permitted which does not comply with these terms.
*Correspondence: Changzhong Liu, liuchzh@gsau.edu.cn