- Laboratory of Functional Reserves of Human Organism, Scientific Research Institute of Neurosciences and Medicine, Novosibirsk, Russia
Coordination of cardiovascular and respiratory systems enables a wide range of human adaptation and depends upon the functional state of an individual organism. Hypoxia is known to elicit changes in oxygen and carbon dioxide sensitivity, while training alters cardiorespiratory coordination (CRC). The delayed effect of high altitude (HA) acclimatization on CRC in mountaineers remains unknown. The objective of this study was to compare CRC in acute hypercapnia in mountaineers before and after a HA expedition. Nine trained male mountaineers were investigated at sea level before (Pre-HA) and after a 20-day sojourn at altitudes of 4,000–7,000 m (Post-HA) in three states (Baseline, Hypercapnic Rebreathing, and Recovery). A principal component (PC) analysis was performed to evaluate the CRC. The number of mountaineers with one PC increased Post-HA (nine out of nine), compared to Pre-HA (five out of nine) [Chi-square (df = 1) = 5.14, P = 0.023]; the percentage of total variance explained by PC1 increased [Pre-HA median 65.6 (Q1 64.9/Q3 74.9), Post-HA 75.6 (73.3/77.9), P = 0.028]. Post-HA, the loadings of the expired fraction of O2, CO2, and ventilation onto PC1 did not change, and the loading of heart rate increased [Pre-HA 0.64 (0.45/0.68) and Post-HA 0.76 (0.65/0.82), P = 0.038]. During the Recovery, the percentage of total variance explained by PC1 was higher than during the Baseline. Post-HA, there was a high correlation between the Exercise addiction scores and the eigenvalues of PC1 (r = 0.9, P = 0.001). Thus, acute hypercapnic exposure reveals the Post-HA increase in cardiorespiratory coordination, which is highly related to the level of exercise addiction.
Introduction
Ascent to high altitude (HA) is accompanied by the process of acclimatization to chronic hypoxia. Hypoxia is known to cause changes in the brain regulatory circuits, leading to alterations in blood flow and ventilatory sensitivity not only to oxygen but also to carbon dioxide (Lindsey et al., 2018). Six hours of hypoxic exposure is sufficient to lower the peripheral and central CO2 threshold (Richard et al., 2014). Moreover, ventilatory and cerebrovascular hypercapnic response patterns show similar plasticity in CO2 sensitivity following hypoxic acclimatization, suggesting an integrated control mechanism (Smith et al., 2017). Cerebral blood flow influences ventilation by altering central chemoreceptor stimulation via central CO2 washout at high altitudes (Hoiland et al., 2018). There are studies that describe the hematological (He et al., 2013) and hormonal changes (Liu et al., 2017), increased hypoxic tolerance, hypoxic ventilatory response and cerebral oxygenation (Cheung et al., 2014), and elevated sympathetic nervous system activity (Sander, 2016) after return to sea level. However, we are not aware of any studies that concern the delayed effects of HA acclimatization on the integration of cardiovascular and respiratory systems.
In general, the integration of the cardiovascular and respiratory systems can provide a wide range of adaptation of the human organism to varying environmental conditions and depends upon the individual functional state. The research approaches of modern physiology demonstrate the transition from the study of the organs and systems functioning to their interaction and integration, which help to improve prediction, particularly in sports physiology (Balagué et al., 2020). Cardiorespiratory coordination (CRC), based on the co-variation among cardiorespiratory variables is a new sensitive approach to studying intersystem integration. A principal component analysis of time series of cardiovascular and respiratory variables registered during cardiorespiratory exercise testing has been used for cardiorespiratory coordination determining to reveal training-specific physiological adaptations (Balagué et al., 2016; Garcia-Retortillo et al., 2019a) and manipulation-specific physiological state (Garcia-Retortillo et al., 2017, 2019b).
We have previously demonstrated that in swimming and skiing correlations between the responses of the cardiovascular and respiratory systems to acute hypoxic and hypercapnic tests are training-specific (Divert et al., 2015, 2017). Later we revealed that cardiorespiratory coherence changes in response to hypoxic exposure depend upon fitness status (Uryumtsev et al., 2020). The study of cardiorespiratory interactions is in its initial stage, and the influencing CRC factors are to be investigated. We suppose that acute hypercapnic exposure may reveal the peculiarities of coordination in the cardiorespiratory system due to the stimulation of central chemoreceptors in mountaineers several weeks after the return from HA.
In addition, we suggested that exercise addiction, which has been found in extreme sports (Heirene et al., 2016), could be correlated with cardiorespiratory coordination. Rock climbing athletes appear to experience withdrawal symptoms when they are abstinent from their sport comparable to individuals with substance and behavioral addictions (Heirene et al., 2016). Mountaineers ascent HA despite all the risks associated with this process. We assumed that the period between HA expeditions could be considered similar to the period of exercise deprivation in athletes with exercise addiction. Krivoschekov and Lushnikov (2017) described increased levels of anxiety and sympathetic nervous system activity assessed by Baevsky’s Stress Index (Baevsky and Chernikova, 2017) in athletes addicted to exercises during exercise deprivation (withdrawal states). Jerath et al. (2016) suggested that alteration in cardiorespiratory synchronization is the mechanism that underlies the symptoms of anxiety. Therefore, we expected that the higher the addiction score is, the worse the cardiorespiratory coordination would be during the period between HA expeditions.
Thus, the purpose of this study was threefold: (1) to compare the CRC in the hypercapnic test before and after the high-altitude expedition; (2) to compare the CRC before (at baseline) and right after the acute hypercapnia (during the recovery stage); and (3) to evaluate the correlation between the level of exercise addiction and CRC.
Materials and Methods
Subjects
The study included nine experienced healthy non-smoking male mountaineers aged 25–42 years. All subjects provided written informed consent prior to participation. The study protocol was approved by the Ethics Committee of the Scientific Research Institute of Neurosciences and Medicine (Novosibirsk) and performed in accordance with the Declaration of Helsinki.
Procedure
The mountaineers were examined twice in June and September at an altitude of 164 m above sea level (Novosibirsk). The first round of investigations took place prior to ascending to a HA (Pre-HA). Then the subjects sojourned for 20 days in the mountains, living under the camp conditions at the altitude of 4,100 m with short-term ascents to the altitude of 6,500–7,000 m (Khan-Tengri, Tien Shan). The second investigation, similar to the first one, was performed on average 2 weeks (range 8–28 days) after descending the HA (Post-HA).
The mountaineers began the first visit by signing informed consent, measuring anthropometric characteristics, filling out the Exercise Addiction Inventory, and measuring office blood pressure. After a 30-minute rest including adaptation to breathing through a mask, we began recording gas exchange, ventilation, heart rate, and blood oxygen saturation. Physiological testing was performed in a sitting position through a facemask. All investigations were performed in the morning 2 h after a light breakfast by the same research assistant at an air temperature of 25°C in three functional states: breathing the ambient air (Baseline, 7 min of steady-state ventilation), hyperoxic hypercapnic rebreathing from a 5-L bag (Rebreathing, 3 min) and breathing the ambient air again (Recovery, 7 min). The entire measurement procedure hence took about 17 min. The Read modified rebreathing procedure was used to create hypercapnia (Read, 1967). An initial concentration of 5% CO2 and 40% O2 was created in the bag. To avoid the increased activity of peripheral chemoreceptors, the hyperoxic O2 concentration in the bag was maintained. The hyperoxic mixture was prepared using a NewLife (AirSep, United States) oxygen concentrator. The technique is described in detail elsewhere (Divert et al., 2015).
Data Recording
A spiroergometric system Oxycon Pro (Erich Jaeger, Germany) was used for recording the following respiratory parameters: minute ventilation (VE), inspired and expired fraction of O2 (FiO2 and FeO2), inspired and expired fraction of CO2 (FiCO2 and FeCO2), and end-tidal O2 and CO2 partial pressure (PETO2 and PETCO2). Heart rate (HR) and blood oxygen saturation (SpO2) data were recorded by Pulse Oximeter BCI 3304 Autocorr (Smiths Medical, United States) and then automatically transferred to the Oxycon Pro. Office blood pressure was obtained by use of a sphygmomanometer (OMRON, Japan). We measured skeletal muscle mass by a multi-frequency tetrapolar bioelectrical impedance analysis device (InBody 370, Korea).
We used the Exercise Addiction Inventory (EAI) (Terry et al., 2004) to identify subjects at-risk from exercise addiction. The EAI consists of six statements based on a modified version of the components of behavioral addiction. Each statement has a five-point response option from “Strongly disagree” (1) to “Strongly agree” (5). The six statements that make up the inventory are: “Exercise is the most important thing in my life,” “Conflicts have arisen between me and my family and/or my partner about the amount of exercise I do,” “I use exercise as a way of changing my mood,” “Over time I have increased the amount of exercise I do in a day,” “If I have to miss an exercise session I feel moody and irritable,” and “If I cut down the amount of exercise I do, and then start again, I always end up exercising as often as I did before.” The EAI cut-off score for individuals considered at-risk of exercise addiction is 24.
Data Analysis
Data analysis was carried out using the STATISTICA10 software package (StatSoft). To evaluate the effect of hypercapnia on the separate cardiorespiratory variables we averaged the data that we received during the last 2 min of the baseline and recovery periods, taking into account the low-frequency fluctuations of the cardiorespiratory variables with a period of about 2 min (Grishin et al., 2019). To describe a hypercapnic ventilatory response, we calculated CO2 sensitivity during rebreathing as the slope in the regression line of VE vs. PETCO2 above the ventilatory threshold PETCO2.
To study cardiorespiratory coordination, we used principal component (PC) analysis, which reflects the degree of coincidence of temporal patterns of physiological responses, that is, how much their increase and decrease are statistically synchronized. The total variance allows us to represent the time patterns of selected cardiorespiratory variables with fewer coordinating variables or PCs. The PC is extracted in descending order of importance. The number of PC reflects the dimension of the system, so a decrease in the number of PCs indicates greater coordination and vice versa. The PC number changes when the system undergoes reconfiguration. PC analysis was performed for each mountaineer on the time series of the following selected cardiorespiratory Pre- and Post-HA variables: VE, FeO2, FeCO2, and HR. Other recorded variables were excluded from the analysis due to their mathematical relationship with the above variables.
The number of PCs was determined by the Kaiser criterion, which considers a significant PC with eigenvalues ≥ 1.00. In the tables, we give the eigenvalues as a percentage of the total variance. The greater this percentage is, the greater the coordination of the variables projected onto PC appears. To analyze the effect of HA, the PC eigenvalues pre- and post- HA were compared over the entire 17-minute measurement. To find out the effect of hypercapnia on CRC, the PC eigenvalues for the time series of two states (Baseline and Recovery) were compared. A Wilcoxon Matched Pairs Test was performed to assess statistically significant differences in the cardiorespiratory variables, eigenvalues and PC loads Pre- vs. Post-HA and between the Baseline and Recovery states. The frequencies of occurrence were compared by the chi-square criterion. To test the suitability of the selected cardiorespiratory data for structure detection, Bartlett’s test of sphericity and the Kaiser-Meyer-Olkin Measure of sampling adequacy was used. The relationship between the level of exercise addiction and the eigenvalues of PC1 was evaluated by the Pearson correlation coefficient. To evaluate whether the Post-HA results were influenced by the delay following the high-altitude exposure, we calculated the Pearson correlation coefficient between the days of delay and the variables characterizing the CRC. Statistical significance was considered at a P-value < 0.05.
Results
Anthropometric Characteristics
The anthropometric descriptive characteristics of the subjects expressed as medians (Q1/Q3) are as follows: height 176 (171/180) cm, body weight 70 (65.7/83.1) kg, body mass index 23.8 (21.7/25.7) kg/m2, and muscle weight 34.6 (31.9/38.8) kg. The Exercise Addiction score was 18.0 (17/19) with a range 15–21.
Effect of HA and Rebreathing on the Cardiorespiratory Parameters
Post-HA baseline blood pressure and recovery heart rate decreased significantly as compared to pre-HA (Table 1). There were no differences between studied respiratory variables as well as rebreathing data pre- and Post-HA. When comparing the variables at the recovery and the baseline stage, only one significant difference was found pre-HA. Recovery FeCO2 was lower than that at baseline, but the difference was less than 4%. Post-HA parameters at recovery and baseline were not different.
Principal Component Analysis
Bartlett’s test of sphericity (p < 0.01) and the Kaiser-Meyer-Olkin Measure of sampling adequacy (min 0.53, max 0.74) showed the suitability of the selected cardiorespiratory data for structure detection. The PC analysis of the entire Pre-HA measurement revealed five mountaineers with one PC and four mountaineers with two PCs. Post-HA, the number of mountaineers with one PC significantly increased to nine [Chi-square (df = 1) = 5.14, p = 0.023]. There were no participants with two PCs Post-HA. Since we used the Kaiser criterion to determine the number of PCs in the model, the following PC (PC2, etc.) had eigenvalues < 1, i.e., they explained less variance than the original variables. Since one PC includes coordinated variables, one can conclude that the coordination of cardio-respiratory variables in the hypercapnic test increases Post-HA.
Post-HA, the percentage of total variance explained by PC1 significantly increased (Table 2). This also confirms an increase in cardiorespiratory coordination.
The loadings of VE, FeO2, and FeCO2 onto PC1 did not change Post-HA, and the heart rate loading significantly increased Post-HA (Table 3). This indicates that the coordination of respiratory variables with heart rate increased. Pre-HA, VE, FeO2, and FeCO2 formed PC1, whereas HR was involved in forming PC2 in four mountaineers with two PCs. Post-HA, in all nine mountaineers, all the selected variables (VE, FeO2, FeCO2, and HR) formed PC1.
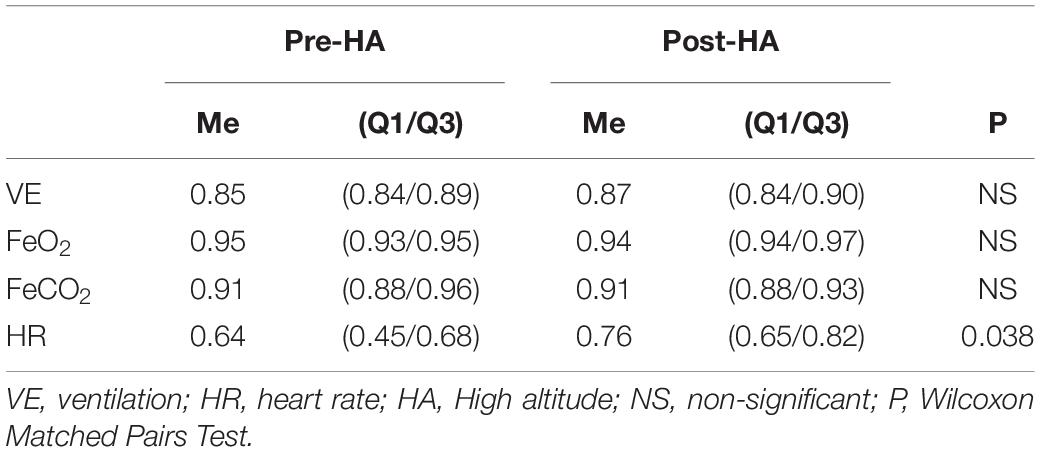
Table 3. Projection of the cardiorespiratory variables onto PC1 pre- and post-HA (entire measurement).
The entire measurement segmentation by the states Baseline, Rebreathing, and Recovery revealed the maximal percentage of total variance explained by PC1 during Rebreathing (Table 2). During the Recovery stage, this percentage was significantly higher than that during the Baseline state. We did not find any significant differences in these percentages before and after the HA expedition.
Relation of Exercise Addiction and Percentage of Total Variance Explained by PC1
Pre-HA, the Pearson correlation coefficients (r) between the exercise addiction score and the percentage of total variance explained by PC1 was 0.67 (p = 0.049), Post-HA r = 0.90 (p = 0.001) (Figure 1). The slopes in the regression equations both pre-HA and Post-HA were about 3. The exercise addiction score as well as the percentage of total variance explained by PC1 Pre- and Post-HA did not correlate with age (r = 0.31, 0.32, 0.31, respectively; NS), weight (r = −0.23, 0.01, −0.33; NS), BMI (r = −0.20, −0.22, −0.25; NS), and muscle mass (r = −0.11, −0.02, −0.26; NS).
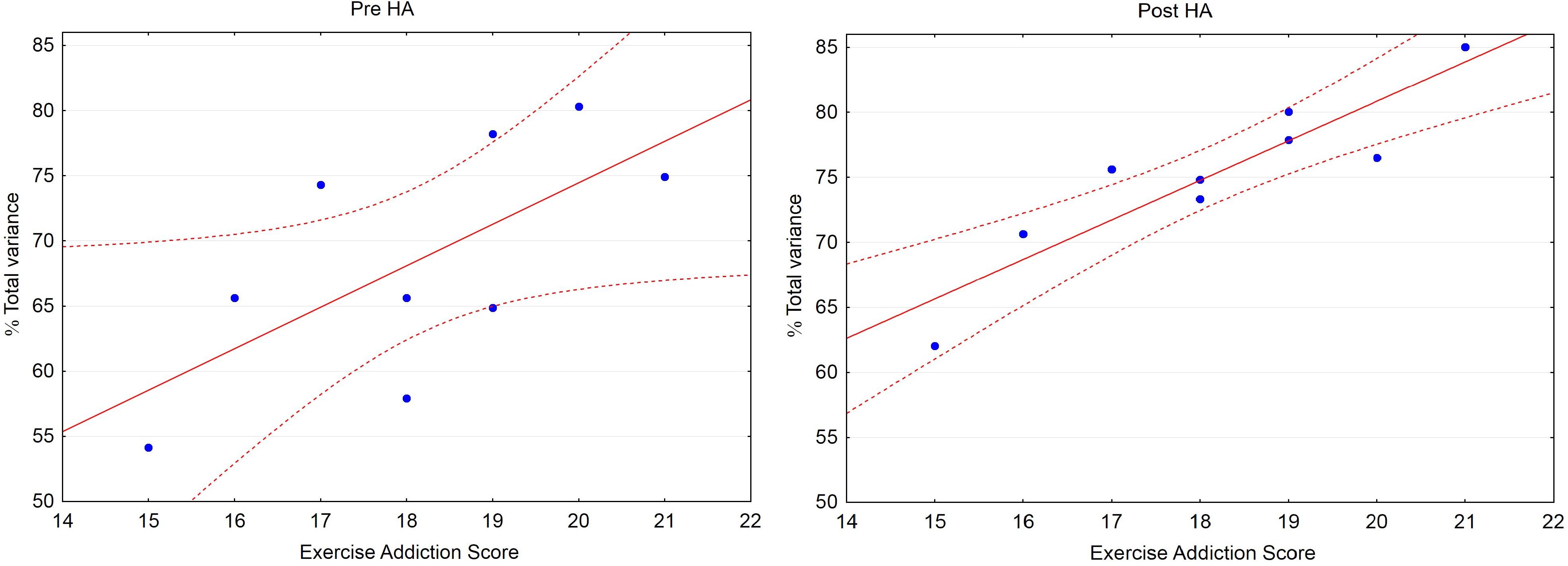
Figure 1. Scatterplot: Percentage of total variance explained by PC1 (% Total variance) vs. Exercise Addiction Score (EAS). Pre-HA: Correlation: r = 0.67 (p = 0.049), % Total variance = 10.79 + 3.18 • EAS. Post-HA: r = 0.90 (p = 0.001), % Total variance = 20.03 + 3.04 • EAS. Dashed lines depict 95% confidence interval.
Correlation Between the Delay Following the HA Expedition and the Variables Characterizing the CRC Changes
There was a negative correlation between Post- and Pre-HA difference in the percentage of total variance explained by PC1 (Δ% Total variance) on the one hand and the delay following the high-altitude exposure during Rebreathing on the other hand (r = −0.86; p = 0.003; regression: Δ% Total variance = 16.9–1.3 ∗ Days Post-HA). The regression line crosses the zero level (i.e., CRC return to the Pre-HA value) on the 13th day. We did not find any significant correlation between Δ% Total variance and the delay following the HA exposure during Baseline (r = −0.02; NS), Recovery (r = 0.51; NS) and Entire measurement (r = −0.01; NS). There were no significant correlations between the loadings of VE, HR, FeO2, FeCO2 onto PC1 and the delay after HA expedition (r = 0.37, 0.10, 0.16, 0.30, respectively; NS).
Discussion
Coordination among subsystems is a hallmark of physiologic state and function (Ivanov et al., 2016). Gas exchange, which is the outcome of a more or less coordinated work of the cardiovascular and respiratory systems, is regulated with the participation of the central and peripheral chemoreceptors that respond to the changes in the blood O2 and CO2 concentration as well as the pH in the intercellular fluid. The peculiarities of the cardiorespiratory coordination largely determine the individual reserves and physical ability (Balagué et al., 2016; Garcia-Retortillo et al., 2019a). They are of particular importance for mountaineers. Mountaineering is associated with hypoxic high-altitude sojourn, cold, and intense physical activity. The present study was aimed at studying the delayed effect of high-altitude exposure on the peculiarities of cardiorespiratory coordination revealed during central chemoreceptors stimulation. To the best of our knowledge, the results of this pilot study show for the first time an increase of cardiorespiratory coordination revealed by hypercapnic exposure in mountaineers several weeks after the high-altitude expedition. The second result concerns the increase in CRC during the recovery period after acute hypercapnia, which indicates a coordinating effect of acute hypercapnia. The third result demonstrates a high correlation between the exercise addiction scores and the CRC values after the high-altitude expedition.
The value of the ventilation threshold to CO2 in our study coincides with the value of the central chemoreflex recruitment threshold for ventilation in healthy young men of 46 mmHg (Keir et al., 2019). Previously, researchers considered the features of the influence of hypercapnia on ventilation, using the concepts of threshold, response, reactivity, and sensitivity (slope). This approach has shown some specific ventilatory responses to hypercapnia and hypoxia possibly because of adaptation to frequent high-altitude climbing (Puthon et al., 2016) or training-specific physiological adaptation (Divert et al., 2017), genetic factors (Goldberg et al., 2017), and even the presence of patent foramen ovale (Davis et al., 2019). We have not found any differences in the considered respiratory parameters at rest, as well as ventilatory thresholds and CO2 sensitivities before and after the high-altitude expedition. Probably, the delayed effect of altitude acclimatization on the studied respiratory parameters varies greatly between individuals. At the same time, heart rate and blood pressure significantly reduced after the expedition. According to He (He et al., 2013) the entire process of deacclimatization may take ≥ 100 days.
We revealed the remaining effect of acclimatization on cardiorespiratory coordination several weeks after return from high altitude. Using the principal component analysis, we were able to analyze the cardiorespiratory coordination over the entire measurement, including the transition periods between the baseline, rebreathing, and recovery states. This approach allows obtaining additional information about the CRC, even with the respect to non-stationary states. Various indices obtained as a result of the PC analysis for the entire 17-minute measurement showed the CRC increase Post-HA: the increase in the number of mountaineers with one PC (all the subjects), the increase in the percentage of variance explained by PC1 (eigenvalues), and an increase in the heart rate loading onto PC1. This is consistent with the results obtained by the same method, which has shown the higher sensitivity and responsiveness of cardiorespiratory coordination to exercise effects compared to isolated cardiorespiratory outcomes (Balagué et al., 2016; Garcia-Retortillo et al., 2017, 2019a). The last studies were performed during physical exercise testing. Increasing metabolic demand can be the coordinating factor for cardiorespiratory variables during exercise. In mountaineers Post-HA, changes in cardiorespiratory coordination are presumably associated with the plasticity of medullar regulatory circuits in response to long-term hypoxic stimulation of chemoreceptors (Lindsey et al., 2018).
The negative correlation between the Post- and Pre-HA difference in the percentage of total variance explained by PC1 and days Post-HA during Rebreathing indicates that the Post-HA rebreathing CRC was influenced by the delay following the high-altitude exposure. Meanwhile, we did not find any relationship between Post- and Pre-HA difference in CRC and days Post-HA during Baseline, Recovery, and Entire measurement. Thus, on the one hand, the large variability in the Post-HA exposure re-evaluation delay is a limitation of the study, but on the other hand, this allowed us to estimate the duration of the effects of HA acclimatization on the CRC after returning to the sea level.
The second result concerns an increase in CRC during the recovery period after acute hypercapnia, indicating an acute coordinating effect of hypercapnia. It has long been known that ventilation begins to increase linearly when the concentration of CO2 increases above the ventilatory threshold (Read, 1967; Duffin, 1990). This fact is used in the rebreathing test. However, we found for the first time that in the period immediately after the rebreathing, the percentage of the total variance, explaining PC1, also increases compared to the baseline level. In addition to ventilation and FeCO2, FeO2, and heart rate are included in the PC in our study. It should be mentioned that in our CRC study, we do not concern another type of cardiorespiratory coupling, respiratory sinus arrhythmia (RSA). However, hypercapnia is known to increase the amplitude of RSA (Tzeng et al., 2007; Brown et al., 2014), which also indicates an increase in cardiorespiratory coupling.
The third result relates the cardiorespiratory coordination to the exercise addiction score. When we included the EAI in our methods, we expected to identify subjects at-risk of exercise addiction similar to those found in extreme sports (Heirene et al., 2016). We assumed that the higher the addiction score is, the worse the cardiorespiratory coordination would be during the period between HA expeditions. However, the results obtained turned out to be the opposite. There was a high positive correlation between CRC and EAI scores. Moreover, none of the investigated climbers has reached the cut-off score of 24 points to be at-risk of exercise addiction. All examined subjects had a medium EAI score. Therefore, it is not possible to talk about withdrawal states. In our study, the level of exercise addiction as well as CRC did not correlate with age, weight, BMI, and muscle mass. An increase in the exercise addiction score indicates a greater commitment to a training lifestyle. We could assume that the EAI score is a psychological correlate of the fitness level in mountaineers. Therefore, the obtained high positive correlation between exercise addiction scores and CRC could be explained by the interdependence of these indices on the level of fitness. This is confirmed by the fact that it is after the high-altitude expedition that there is a high correlation. The concept of fitness for mountaineers includes the adaptation of the chemoreflex regulation circuit, as well as physical ability. However, this assumption requires further verification.
The practical relevance of the results obtained is that the cardiorespiratory coordination could serve as an additional marker of specific physiological adaptations to different hypoxic states, including high-altitude hypoxia in mountaineers and the pathological state accompanied by hypoxia.
Limitations
The main limitation of the study is the lack of blood pressure time series, which could significantly improve the PC model. Another limitation is the lack of blood and biochemical analyses, including changes in pH, and indices of autonomic nervous system regulation, which could provide a more detailed integration of dynamics across different systems. Large variability in the Post-HA exposure re-evaluation delay could be a limitation of the study, but this allowed us to estimate the duration of the effects of HA acclimatization on the CRC after returning to the sea level. Moreover, this study investigates nine subjects only, which may limit the statistical power of the study.
Further Considerations
In the future, we consider it appropriate to investigate the effect of autonomic nervous regulation on cardiorespiratory coordination.
Conclusion
Acute hypercapnic exposure reveals the Post-HA increase in cardiorespiratory coordination, which is highly related to the level of exercise addiction. Acute hypercapnia provides a coordinating effect on the cardiorespiratory system. The CRC after the acute hypercapnia during the recovery stage is significantly higher than at baseline. These facts require further investigation.
Data Availability Statement
The raw data supporting the conclusions of this article will be made available by the authors, without undue reservation.
Ethics Statement
The studies involving human participants were reviewed and approved by Ethics Committee of the Scientific Research Institute of Neurosciences and Medicine (Novosibirsk). The patients/participants provided their written informed consent to participate in this study.
Author Contributions
SK: conceptualized the research question, study design, and supervised the entire project. DU: performed the data analysis. VG and MZ: drafted the manuscript. VM and NB: collected data. All authors interpreted the results and critically reviewed and significantly contributed to the manuscript and approved the final version.
Funding
The study was supported by Budgetary Funding for Basic Scientific Research (theme no. AAAA-A21-121011990040-8).
Conflict of Interest
The authors declare that the research was conducted in the absence of any commercial or financial relationships that could be construed as a potential conflict of interest.
Acknowledgments
We would like to thank Helen Wiebe and Nina Poleeva for their support in the translation of the manuscript, Taissia Chinina-Kelly for her support in the translation of the abstract, and Tamara Komlyagina and Victor Divert for their helpful assistance with data collection.
References
Baevsky, R. M., and Chernikova, A. G. (2017). Heart rate variability analysis: physiological foundations and main methods. Cardiometry 10, 66–67. doi: 10.12710/cardiometry.2017.10.6676
Balagué, N., González, J., Javierre, C., Hristovski, R., Aragonés, D., Álamo, J., et al. (2016). Cardiorespiratory coordination after training and detraining. A principal component analysis approach. Front. Physiol. 7:35. doi: 10.3389/fphys.2016.00035
Balagué, N., Hristovski, R., Almarcha, M., Garcia-Retortillo, S., and Ivanov, P. C. (2020). Network physiology of exercise: vision and perspectives. Front. Physiol. 11:611550. doi: 10.3389/fphys.2020.611550
Brown, S. J., Barnes, M. J., and Mündel, T. (2014). Effects of hypoxia and hypercapnia on human HRV and respiratory sinus arrhythmia. Acta Physiol. Hung. 101, 263–272. doi: 10.1556/APhysiol.101.2014.3.1
Cheung, S. S., Mutanen, N. E., Karinen, H. M., Koponen, A. S., Kyröläinen, H., Tikkanen, H. O., et al. (2014). Ventilatory chemosensitivity, cerebral and muscle oxygenation, and total hemoglobin mass before and after a 72-day mt. Everest expedition. High. Alt. Med. Biol. 15, 331–340. doi: 10.1089/ham.2013.1153
Davis, J. T., Boulet, L. M., Hardin, A. M., Chang, A. J., Lovering, A. T., and Foster, G. E. (2019). Ventilatory responses to acute hypoxia and hypercapnia in humans with a patent foramen ovale. J. Appl. Physiol. 126, 730–738. doi: 10.1152/japplphysiol.00741.2018
Divert, V. E., Komlyagina, T. G., Krasnikova, N. V., Martynov, A. B., Timofeev, S. I., and Krivoschekov, S. G. (2017). Cardiorespiratory responses of swimmers to hypoxia and hypercapnia. Novosibirsk State Pedagog. Univer. Bul. 7, 207–224. doi: 10.15293/2226-3365.1705.14
Divert, V. E., Krivoshchekov, S. G., and Vodyanitsky, S. N. (2015). Individual-typological assessment of cardiorespiratory responses to hypoxia and hypercapnia in young healthy men. Hum. Physiol. 41, 166–174. doi: 10.3389/fphys.2019.0004510.1134/S036211971502005X
Duffin, J. (1990). The chemoreflex control of breathing and its measurement. Can. J. Anaesth. 37, 933–942. doi: 10.1007/BF03006641
Garcia-Retortillo, S., Javierre, C., Hristovski, R., Ventura, J. L., and Balagué, N. (2017). Cardiorespiratory coordination in repeated maximal exercise. Front. Physiol. 8:387. doi: 10.3389/fphys.2017.00387
Garcia-Retortillo, S., Javierre, C., Hristovski, R., Ventura, J. L., and Balagué, N. (2019a). Cardiorespiratory coordination reveals training-specific physiological adaptations. Eur. J. Appl. Physiol. 119, 1701–1709. doi: 10.1007/s00421-019-04160-3
Garcia-Retortillo, S., Javierre, C., Hristovski, R., Ventura, J. L., and Balagué, N. (2019b). Principal component analysis as a novel approach for cardiorespiratory exercise testing evaluation. Physiol. Meas. 40:084002. doi: 10.1088/1361-6579/ab2ca0
Goldberg, S., Ollila, H. M., Lin, L., Sharifi, H., Rico, T., Andlauer, O., et al. (2017). Analysis of hypoxic and hypercapnic ventilatory response in healthy volunteers. PLoS One 12:e0168930. doi: 10.1371/journal.pone.0168930
Grishin, V. G., Grishin, O. V., Gultyaeva, V. V., Ziinchenko, M. I., and Uryumtsev, D. Y. (2019). Very-low-frequency oscillations of oxygen transport in resting humans. Russ. J. Physiol. 105, 1154–1162. doi: 10.1134/S0869813919090061
He, B., Wang, J., Qian, G., Hu, M., Qu, X., Wei, Z., et al. (2013). Analysis of high-altitude de-acclimatization syndrome after exposure to high altitudes: a cluster-randomized controlled trial. PLoS One 8:e62072. doi: 10.1371/journal.pone.0062072
Heirene, R. M., Shearer, D., Roderique-Davies, G., and Mellalieu, S. D. (2016). Addiction in extreme sports: an exploration of withdrawal states in rock climbers. J. Behav. Addict. 5, 332–341. doi: 10.1556/2006.5.2016.039
Hoiland, R. L., Howe, C. A., Coombs, G. B., and Ainslie, P. N. (2018). Ventilatory and cerebrovascular regulation and integration at high-altitude. Clin. Auton. Res. 28, 423–435. doi: 10.1007/s10286-018-0522-2
Ivanov, P. C., Liu, K. K. L., and Bartsch, R. P. (2016). Focus on the emerging new fields of network physiology and network medicine. New J. Phys. 18:100201. doi: 10.1088/1367-2630/18/10/100201
Jerath, R., Cearley, S. M., and Jensen, M. (2016). Widespread membrane potential changes and cardiorespiratory synchronization involved in anxiety and sleep-wake transitions. J. Biol. Regul. Homeost. Agents 30, 935–944.
Keir, D. A., Duffin, J., Millar, P. J., and Floras, J. S. (2019). Simultaneous assessment of central and peripheral chemoreflex regulation of muscle sympathetic nerve activity and ventilation in healthy young men. J. Physiol. 597, 3281–3296. doi: 10.1113/JP277691
Krivoschekov, S. G., and Lushnikov, O. N. (2017). The functional state of athletes addicted to exercises during exercise deprivation. Hum. Physiol. 43, 678–685. doi: 10.1134/S0362119717040077
Lindsey, B. G., Nuding, S. C., Segers, L. S., and Morris, K. F. (2018). Carotid bodies and the integrated cardiorespiratory response to hypoxia. Physiology 33, 281–297. doi: 10.1152/physiol.00014.2018
Liu, C., Chen, J., Liu, B., Liao, W. T., Liu, J., Xu, G., et al. (2017). Activated corticosterone synthetic pathway is involved in poor responses to re-oxygenation after prolonged hypoxia. Int. J. Clin. Exp. Pathol. 10, 8414–8423.
Puthon, L., Bouzat, P., Rupp, T., Robach, P., Favre-Juvin, A., and Verges, S. (2016). Physiological characteristics of elite high-altitude climbers. Scand. J. Med. Sci. Sports 26, 1052–1059. doi: 10.1111/sms.12547
Read, D. J. (1967). A clinical method for assessing the ventilatory response to carbon dioxide. Australas. Ann. Med. 16, 20–32. doi: 10.1111/imj.1967.16.1.20
Richard, N. A., Sahota, I. S., Widmer, N., Ferguson, S., Sheel, A. W., and Koehle, M. S. (2014). Acute mountain sickness, chemosensitivity, and cardiorespiratory responses in humans exposed to hypobaric and normobaric hypoxia. J. Appl. Physiol. 116, 945–952. doi: 10.1152/japplphysiol.00319.2013
Sander, M. (2016). Does the sympathetic nervous system adapt to chronic altitude exposure? Adv. Exp. Med. Biol. 903, 375–393. doi: 10.1007/978-1-4899-7678-9_25
Smith, Z. M., Krizay, E., Sá, R. C., Li, E. T., Scadeng, M., and Powell, F. L. Jr., et al. (2017). Evidence from high-altitude acclimatization for an integrated cerebrovascular and ventilatory hypercapnic response but different responses to hypoxia. J. Appl. Physiol. 123, 1477–1486. doi: 10.1152/japplphysiol.00341.2017
Terry, A., Szabo, A., and Griffiths, M. D. (2004). The exercise addiction inventory: a new brief screening tool. Addict. Res. Theory 12, 489–499. doi: 10.1080/16066350310001637363
Tzeng, Y. C., Larsen, P. D., and Galletly, D. C. (2007). Effects of hypercapnia and hypoxia on respiratory sinus arrhythmia in conscious humans during spontaneous respiration. Am. J. Physiol. Heart Circ. Physiol. 292, H2397–H2407. doi: 10.1152/ajpheart.00817.2006
Keywords: high altitude, mountaineers, cardiorespiratory coordination, hypercapnia, rebreathing, principal component analysis, exercise addiction
Citation: Gultyaeva VV, Uryumtsev DY, Zinchenko MI, Melnikov VN, Balioz NV and Krivoschekov SG (2021) Cardiorespiratory Coordination in Hypercapnic Test Before and After High-Altitude Expedition. Front. Physiol. 12:673570. doi: 10.3389/fphys.2021.673570
Received: 27 February 2021; Accepted: 16 April 2021;
Published: 24 May 2021.
Edited by:
Nicolas Voituron, Université Paris 13, FranceReviewed by:
Samuel Verges, Université Grenoble Alpes, FranceVittore Verratti, G. d’Annunzio University of Chieti and Pescara, Italy
Martin Burtscher, University of Innsbruck, Austria
Copyright © 2021 Gultyaeva, Uryumtsev, Zinchenko, Melnikov, Balioz and Krivoschekov. This is an open-access article distributed under the terms of the Creative Commons Attribution License (CC BY). The use, distribution or reproduction in other forums is permitted, provided the original author(s) and the copyright owner(s) are credited and that the original publication in this journal is cited, in accordance with accepted academic practice. No use, distribution or reproduction is permitted which does not comply with these terms.
*Correspondence: Valentina V. Gultyaeva, Z3VsdHlhZXZhdnZAcGh5c2lvbC5ydQ==