- 1Department of Ultrasound Diagnostics, Tangdu Hospital, Fourth Military Medical University, Xi’an, China
- 2State Key Laboratory of Cancer Biology, Department of Biochemistry and Molecular Biology, Fourth Military Medical University, Xi’an, China
Adipose tissue functions importantly in the bodily homeostasis and systemic metabolism, while obesity links to multiple disorders. Beyond the canonical hormones, growth factors and cytokines, exosomes have been identified to play important roles in transmission of information from adipose tissue to other organs. Exosomes are nanoscale membrane vesicles secreted by donor cells, and transfer the genetic information to the recipient cells where the encapsulated nucleic acids and proteins are released. In this review, we elaborate the recent advances in the biogenesis and profiling of adipose tissue derived exosomes, and their physiological and pathological effects on different organs. Moreover, the potential significance of the exosomes as therapeutic vehicles or drugs is also discussed.
Introduction
In mammals, there are two main types of adipose tissues termed as white adipose tissue (WAT) and brown adipose tissue (BAT), respectively. WAT and BAT function significantly different (Zwick et al., 2018). WAT stores excess energy in the form of triglycerides (van Eenige et al., 2018), while BAT improves the energy expenditure by activating uncoupling protein 1 (UCP1) (Oelkrug et al., 2015). In WAT triglycerides (TAG) and other neutral lipids are stored in large lipid droplets. Hydrolysis of TAGs can provide substrates to meet systemic metabolic needs during negative energy balance (Scherer, 2006). WAT can be further divided into visceral adipose tissue (VAT) and subcutaneous adipose tissue (SAT) (Barreira et al., 2011), mainly distributed in the chest and abdominal cavity (Britton et al., 2013). Besides the commonly studied WAT and BAT, recent studies have identified beige adipose tissue, which is more closely related to WAT in development and similar to BAT in shape and function (Kaisanlahti and Glumoff, 2019). Beige adipose cells can also dissipate energy to fight obesity through uncoupled mitochondrial respiration (Cypess and Kahn, 2010; Nedergaard et al., 2011; Chen and Pfeifer, 2017).
Normal VAT functions importantly in maintaining normal metabolism, while excessive VAT is a risk factor for metabolic diseases (Fox et al., 2007). Previous studies have shown that visceral adipocytes promote systemic inflammation by secreting cytokines, such as interleukin IL-6 and tumor necrosis factor TNF-α (Fontana et al., 2007; Choi and Cohen, 2017). These mediators are secreted into the circulation and affect distant organs, increasing the risk of type 2 diabetes, atherosclerosis, cardiovascular disease, asthma (de Jong et al., 2002; Ji and Guo, 2019; Wallace et al., 2019), and several forms of cancer. Excessive adipose tissue also increases systemic TGF-β1, which switches fibroblasts to myofibroblasts and promotes fibrosis (Masszi et al., 2004). In addition to the above-mentioned secreted adipokines, extracellular vesicles (EVs) secreted by the adipose tissue, such as exosomes, also show the ability to regulate the pathophysiological level of the body (Li et al., 2015).
Extracellular vesicles refer to the collective name of micro vesicles with membrane structure that are actively secreted by cells (van Niel et al., 2018). According to physical and chemical properties and biochemical composition, EVs are mainly divided into exosomes, microvesicles and apoptotic bodies (Shao et al., 2018). Exosomes are nanoscale extracellular lipid bilayer vesicles with the diameter of 30–200 nm, which can be secreted by almost all types of cells (Wan et al., 2018; Wu et al., 2019). Exosome biogenesis usually starts from endosomal membrane invagination, forming the late endosomal multivesicular bodies (MVBs)/endosomes. During this process, cytosolic nucleic acids and proteins are incorporated into the lumen of MVBs. The exosomes are further secreted from the donor cells when the endosome fused with the plasma membrane (Wang et al., 2021). Almost all cells secrete EVs and play a pivotal role in intracellular communication and biological events. Exosomes contain various contents including proteins, lipids, and nucleic acids (DNA, RNA, and microRNA) (Lehmann et al., 2008; Guescini et al., 2010), which are widely involved in signal transduction and genetic information exchange. The exosome biogenesis process and intercellular communication are depicted in Figure 1. Due to the ubiquity of resources, stability in biological fluids, potent bioactivity, and continuous improvements in the quantitative and qualitative techniques, exosomes have become promising biomarkers and therapeutic carriers in clinical application (Zhang B. et al., 2019). For example, exosomes derived from tumor tissues can be used as biomarkers for cancer diagnosis. Exosomes are involved X-ray radiation and other canonical therapies in cancer treatment (Ahmadi and Rezaie, 2020). In addition, engineered exosomes could be used as vaccine or chemotherapy drug carrier (Tian et al., 2014). Beyond systemic delivery, exosomes could be also released by hydrogel for generation purposes.
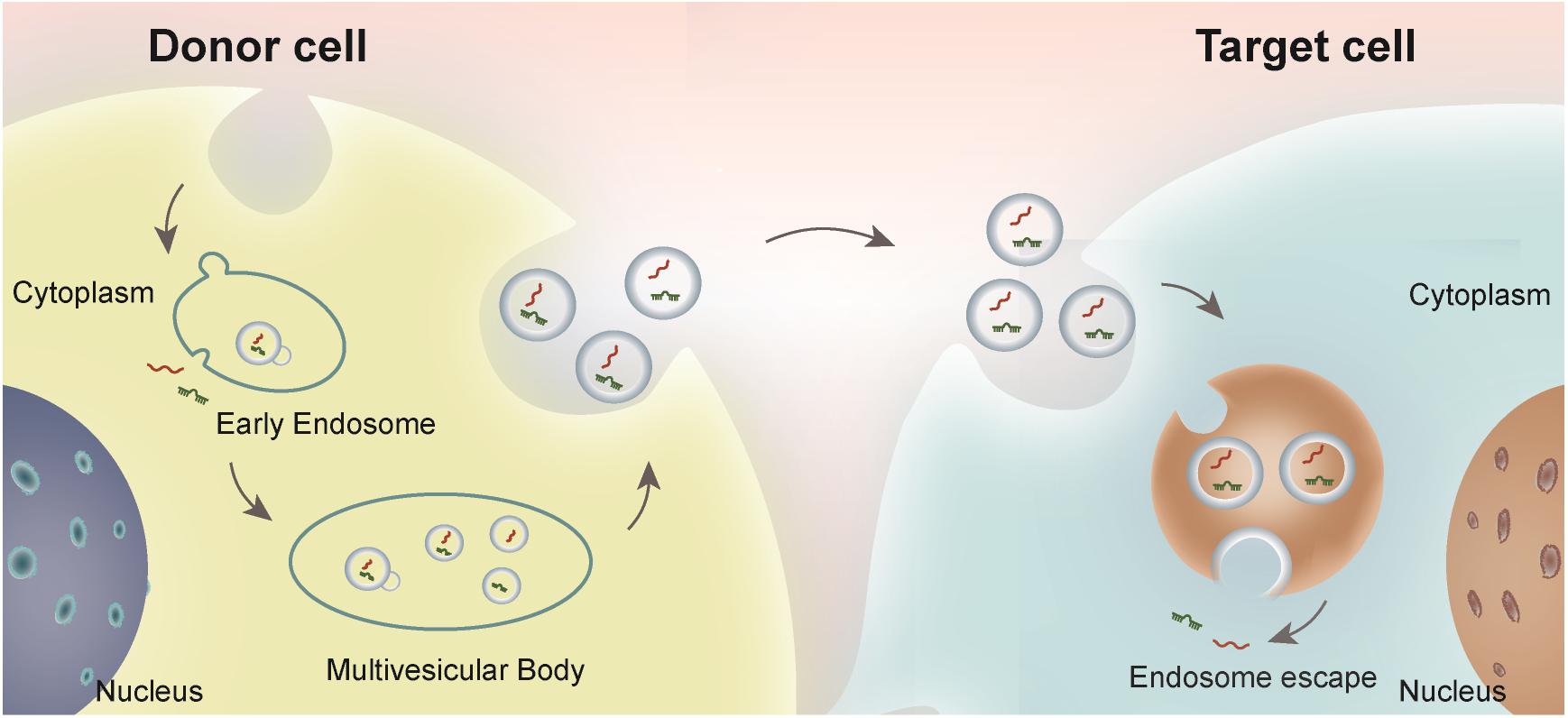
Figure 1. Schematic illustration of exosome biogenesis and intercellular communication. Exosomes, originated from the endosome in the donor cells, are secreted and endocytosed by the recipient cells either adjacent to or distal from the donor cells. The bioactive cargos could be escaped from the endosome in the recipient cells and play functional roles.
Adipose tissue constitutes an important source of circulating exosomes, which can regulate gene expression in adjacent or distant tissues via the encapsulated miRNAs (Deregibus et al., 2007; Thomou et al., 2017; Burkova et al., 2018). Accumulating studies have found that exosomes released by adipose tissue may act importantly in maintaining the body’s homeostasis (Ji and Guo, 2019).
Pathophysiological Roles of Adipose Tissue-Derived Exosomes
The homeostasis of the body is the result of the interaction between various tissues. As the largest secretory organ, adipose tissue releases various hormones and adipokines. The increased/enlarged adipose tissue in obese patients can cause various metabolic and cardiovascular complications (Labrecque et al., 2017). Recent studies identify adipose tissue derived exosomes as a type of intercellular communication mediator between adipose tissue and other tissues.
Adipose Tissue-Derived Exosomes in Lipid Metabolism
Similar as the parental cells, adipose tissue derived exosomes also play essential role in lipid metabolism. Adipose tissue is rich in rich in enzymes related to the lipogenesis, such as acetyl-CoA carboxylase, glucose-6-phosphate dehydrogenase dehydrogenase, and fatty acid synthase. Consistently, adipose tissue derived exosomes are also enrich of these enzymes. Notably, under hypoxic conditions, the total amount of exosomes and the encapsulated enzymes by the adipose tissue are increased. In turn, these enzymes may affect lipogenic activity in the recipient cells (Sano et al., 2014). Moreover, abundant miR-450a-5p expression was found in adipose tissue derived exosomes, which can promote adipogenesis by inhibiting the expression of WNT1 inducible signaling pathway protein 2 (WISP2) (Zhang Y. et al., 2017).
Obesity is a recognized risk factor for atherosclerosis. Obesity is linked to coronary plaque progression and a high rate of cardiovascular disease events (Kahn et al., 2019). It has been reported that adipokines and cytokines secreted from accumulated VAT can affect lipid metabolism and have an impact on the formation of atherosclerosis (Martinez and Andriantsitohaina, 2017; Akbar et al., 2019; Scheja and Heeren, 2019). It has been found that adipose tissue derived exosomes from obese individuals can down-regulate expression of ABCA1 and ABCG1, which function importantly in cholesterol efflux, resulting in reduced cholesterol efflux, polarization of M1 and enhanced activation of NF-κB. Ultimately, adipose tissue derived exosomes from obese individuals accelerate the formation of macrophage foam cells, promote inflammation, and increase the risk of atherosclerosis (Xie et al., 2018).
Adipose Tissue-Derived Exosomes in Regulation of Insulin Resistance
Adipose tissue functions importantly in insulin activity regulation. Specifically, adipose tissue in obesity dampens insulin sensitivity. Adipose tissue exosomes can directly interfere with insulin signaling in liver and muscle cells, which is related to o systemic insulin resistance (Kranendonk et al., 2014). For example, miR-27a derived from adipose tissue may inhibit PPARγ and its downstream genes, and play a key role in the occurrence of insulin resistance induced by obesity (Yu et al., 2018). The reduced miR-141-3p in adipose tissue exosomes of obese patients can significantly inhibit hepatocyte insulin sensitivity and glucose uptake (Dang et al., 2019). It has also been revealed that, exosomes from the adipose tissue macrophages (ATMs-Exos) in obese mice cause glucose tolerance and insulin resistance in lean mice via the miRNA cargos (Hubal et al., 2017). For example, miR-155 is highly expressed in the exosomes from obese ATMs-Exos. Exosomal miR-155 inhibits the target gene PPAR-γ together with several downstream PPAR-γ target genes in recipient liver cells, causing insulin resistance and abnormal glucose tolerance (Ying et al., 2017). Similarly, exosomal miR-29a causes insulin resistance via targeting PPAR-δ(Liu T. et al., 2019). Systemic inflammation is also considered as the leading cause of insulin resistance. Consistently, exosome-like vesicles (ELVs) released from adipose tissue are also found to play a role in macrophage activation and insulin resistance. ELVs are involved in the increased secretion of TNF-α and IL-6 by ATMs-Exos in obese mice. Mechanistically, ELVs induce insulin resistance induction at least partially through the TLR4/TRIF pathway (Deng et al., 2009).
Adipose Tissue-Derived Exosomes in Regulation of Inflammation and Immunity
Inflammation in adipose tissue is the main cause of obesity-related metabolic complications. However, the molecular link between fat adipose tissue and inflammatory immune cells remains elusive. MiR-34a is a key component of exosomes secreted by adipocytes. Studies have shown that mature adipocytes secrete exosomes and transport miR-34a to macrophages, transmitting nutrient overload signals to macrophages residing in fat. In the recipient macrophages, miR-34a inhibits M2 polarization by inhibiting the expression of Krüppel-like factor 4 (Klf4), exacerbating systemic inflammation and contributing to the metabolic abnormalities caused by obesity (Pan et al., 2019). In contrast to the pro-inflammatory exosomes from the obese adipose tissue, ADSC-derived exosomes are found to promote M2 polarization in macrophages (Zhao H. et al., 2018).
Neutrophils are transient cells of the innate immune system and participate in defense against pathogens by generating reactive oxygen species (ROS). Exosomes isolated from interstitial stromal cells of human adipose tissue are found to increase the vitality of the neutrophils via the encapsulated protein cargos, resulting in improved immunity (Mahmoudi et al., 2019).
Adipose Tissue-Derived Exosomes in Regulation of Other Systems
Besides the above regulation, accumulating evidence suggests that adipose tissue derived exosomes also function importantly in other systems. Exosomes of perivascular adipose tissue (PVAT) contain a large number of miRNAs. PVAT derived exosomes from the obese mice due to inflammation lead to vascular phenotypic transformation of the abdominal aorta in lean mice, which could be at least partially attributed to the increased expression of miR-221-3p. Mechanistically, miR-221-3p significantly enhances the proliferation and migration of vascular smooth muscle cell (VSMC) (Li X. et al., 2019).
A recent study profiled the proteomic content change of adipose tissue exosomes in obese women during pregnancy. Obese pregnant women’s exosomes are rich in proteins that associated with metabolism, such as mitochondrial dysfunction and SIRT signaling pathways (Jayabalan et al., 2019). Accordingly, the increased exosome release was positively correlated with the birth weight Z score, indicating that it may interact with the placenta and regulate fetal growth.
Obesity is linked with types of cancers. Recently Lu et al. (2017) revealed that the gluteal adipose exosome derived Hotair promote colon cancer cell proliferation via enhancing Wnt signal. In a pioneering study, Wei et al. (2020) revealed that high fat diet changed the miRNA profile of the visceral adipose exosomes, switching the exosome from anti-inflammatory to pro-inflammatory phenotype. In turn, these exosomes predisposed the intestine to inflammation via promoting macrophage M1 polarization. The study has established an exosomal pathway how obesity aggravates colitis, which well explained the strong association of obesity with the risk of colitis found in epidemic studies (Wei et al., 2020). We have also found that exosomes from the maternal adipose tissue could cross the placenta barrier, affecting the developmental embryos (Liu et al., 2021).
In addition, adipocytes are also one type of stromal cells of many different cancers. For example, hepatocellular carcinoma (HCC) is the main form of liver cancer and has shown an increased incidence and poor prognosis. Studies have shown that the effects of fatty exosomes promote tumor growth and reduce DNA damage by targeting deubiquitination-related USP7 via the exosomal circRNA (Zhang H. et al., 2019). In HCC patients with a body fat ratio, the expression of miR-23a/b in serum exosomes was significantly higher, compared with that in patients with low body fat. In vitro studies further suggest that exosomal miR-23a/b is most likely from fat cells, and it could promote the growth and migration of HCC cells when endocytosed (Liu Y. et al., 2019).
In summary, adipose tissue can regulate the function of multiple organs, such as the cardiovascular system, endocrine system, immune system, and reproductive system, in the form of exosomes (Table 1 and Figure 2). However, there are still too many to be revealed concerning the detailed function and the underlying mechanisms. With the deepening of research on the physiological and pathological effects of adipose tissue exosomes will help to better explore the mysteries of life and provide timely and effective clinical interventions.
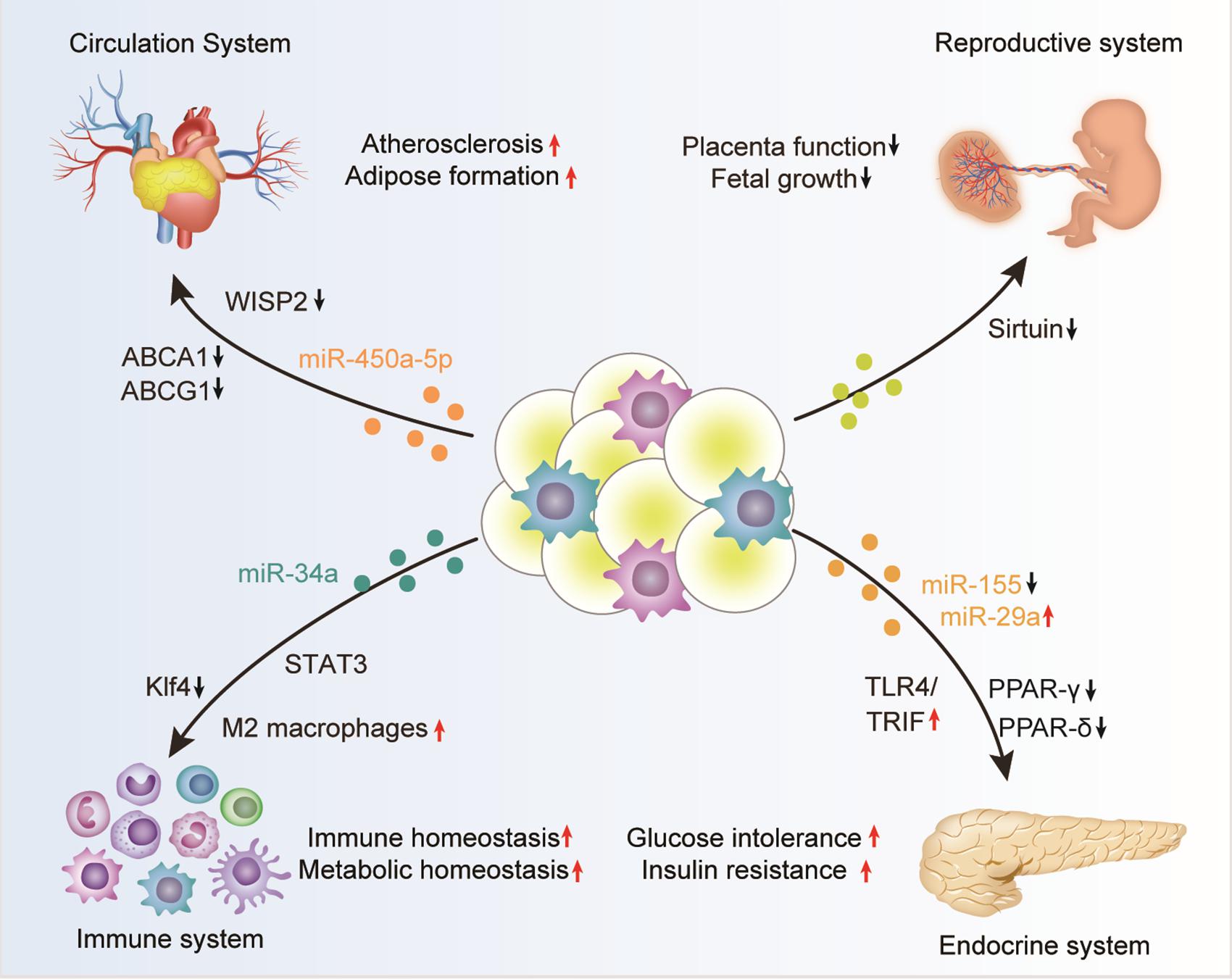
Figure 2. Adipose tissue-derived exosomes in the regulation of biological processes. Adipose tissue derived exosomes, either from the adipocytes, associated macrophages, or other stromal cells, can circulate into distant organs, such as the cardiovascular system, endocrine system, immune system, and reproductive system, where they can regulate the function of the recipient cells. The exosome-mediated crosstalk between adipose and other organs function importantly in many physiological and pathological contexts, especially obesity.
Therapeutic Implication of Adipose Tissue-Derived Exosomes
The encapsulated RNA, miRNA, proteins and lipids in exosomes share similar profile as the parental cells. To this end, exosomes are considered as potential biomarkers and therapeutic drugs for many diseases (Figure 3 and Table 2).
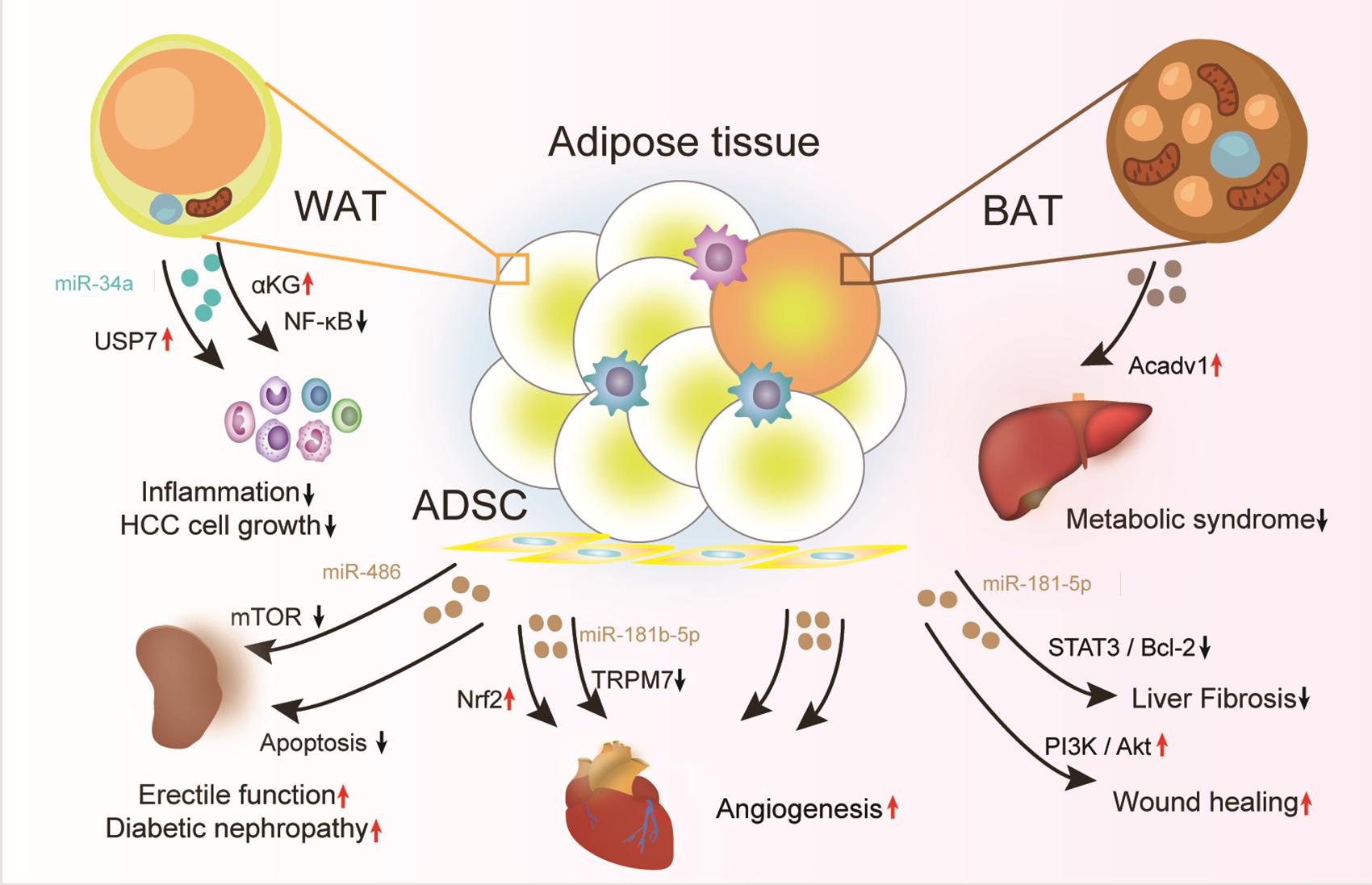
Figure 3. Therapeutic effects of adipose tissue-derived exosomes. Exosomes from healthy adipose tissue, specifically the adipose in lean, the brown adipocytes, and the ADSCs have great therapeutic potential for types of diseases, such as inflammation, metabolic syndrome, and endothelial dysfunction. Moreover, the adipose tissue should be engineered with chemical drugs or gene modification for production of exosomes with improved therapeutic effects.
Adipose Tissue-Derived Exosomes for Improvement of Endothelial Function
Adipose tissue-derived exosomes are considered to be an important regulator of cardiovascular health (Liang et al., 2016; Xu et al., 2019). Recently, PVAT is found to function importantly in the regulation of endothelial homeostasis (Schinzari et al., 2017). PVAT are the adipose tissue surrounding blood vessels, and factors secreted by adipocytes can easily target blood vessels. PVAT is considered a unique storage of adipose tissue, different from SAT and other VAT, especially in terms of regulation of vascular function (Brown et al., 2014). It has been revealed that exosomes derived from mangiferin stimulated PVAT ameliorate endothelial dysfunction, suggesting a potent benefit in the treatment of obesity-related cardiovascular diseases (Zhao Q. et al., 2019).
Adipose stem cells are a type of adult stem cells and have the potential to differentiate into other types of cells. Besides, adipose stem cells are of potent paracrine function. These features make them have broad prospects and potential in regenerative medicine. With the deepening of research, it has been proposed that the biological functions of adipose stem cells are closely related to their exosomes. ADSC-exos are considered to be a candidate drug for ischemic diseases (Zhao et al., 2017). Studies have shown that treatment of exosomes with from ADSCs overexpressing Nrf2 accelerate the healing of wound ulcers on the feet of diabetic rats, partially via enhanced angiogenesis (Li X. et al., 2018). Similarly, ADSC derived exosomes could promote angiogenesis via enhancing VEGF/VEGFR and/or miR-181b-5p/transient receptor potential melastatin 7 (TRPM7) axis (Yang et al., 2018; Han et al., 2019). The exosomes from miR-199-3p modified ADSCs promote the proliferation and migration of endothelial tip cells by down-regulating Sema3A (Du et al., 2018).
Adipose Tissue-Derived Exosomes in Treatment of Metabolic Syndrome
As adipose tissue-derived exosomes are fundamental regulator of insulin resistance, the role of exosomes in the treatment of diabetes is now intensively studied (Zhang B. et al., 2019; Jin et al., 2020). Type 2 diabetes and obesity are diseases related to excess energy in the body. The abnormal interaction between the hypothalamus and adipose tissue is a key trigger for energy metabolism dysfunction. Adipocyte derived exosomes can regulate POMC expression in vivo and in vitro through the hypothalamic mTOR signal, thereby affecting the body’s energy intake (Gao et al., 2020). In addition, ADSCs-Exo suppresses mTOR activation, improving the diabetes-related complications (Jin et al., 2019).
The main function of BAT is to produce heat through UCP1 located on the inner mitochondrial membrane. Transplanting BAT from donor mice to recipient mice significantly improves the whole body energy metabolism, with the weight gain caused by high-fat diet prevented and insulin resistance reversed (Liu et al., 2013, 2015; Stanford et al., 2013). BAT can dissipate energy and can also regulate the metabolism of other organs through exosomal miRNA (Chen and Pfeifer, 2017). Very recently, we have shown that high-fat diet mice treated with BAT exosomes had reduced body weight and blood glucose levels, while serum exosomes treatment had such effect (Zhou et al., 2020). Mechanistically, the BAT-exosomes are enriched in functional mitochondrial components, such as ACADV1, which can be transferred to the recipient cells (Zhou et al., 2020). The survival rate is the big concern for brown adipocytes/adipose tissue transplantation. In contrast, there is no such concern for BAT-Exo treatment, while BAT-Exos have similar function as the BATs.
Other Diagnostic and Therapeutic Implications of Adipose Tissue-Derived Exosomes
Besides the potential therapeutic effects on endothelial function and metabolism, exosomes could be also used in other contexts. For example, miR-181-5p in fat-derived mesenchymal stem cells (MSCs-Exo) can significantly down-regulate collagen I and vimentin in the liver to reduce liver damage (Qu et al., 2017). ASCs-Exos can optimize the characteristics of fibroblasts to achieve the purpose of promoting skin wound healing (Hu et al., 2016; Zhang W. et al., 2018) and improve erectile dysfunction in diabetic mice (Chen F. et al., 2017). Similarly, overexpression of miR-19b in VAT exosomes can reduce inflammation in placental cells (Liu et al., 2021). With the continuous deepening of related research, exosomes from adipose tissue or adipocytes or the precursor, are expected to widely used in different diseases.
Conclusion and Perspectives
Exosomes produced by adipose tissue participate in the regulation of almost all physiological activities in the body. Besides the intensively studied miRNAs in exosomes, other bioactive molecules, such as lncRNA and circRNA, are worthy of further exploration. It is important to note that isolation of the exosomes from the adipose tissue is prerequisite for the profiling of the cargos. Since adipose tissue consists of types of cells. Isolation of the exosomes with adipocyte specific antibodies would be a promising and accurate strategy for the purpose of profiling adipocyte specific exosomes. In addition to the encapsulated cargos, the targeting specificity and the underlying mechanisms are also worthy of exploration. The adipose-derived exosomes should carry special exosomal membrane proteins, responsible for their special biodistribution.
The exosomes from healthy adipose tissue or the ADSCs have great therapeutic potential. Compared with the cells, exosome-based therapy has low immunogenicity, convenience for transportation and manufacture. Currently, there are multiple clinical trials exploring the therapeutic and diagnostic value of exosomes derived from adipose tissue or the stromal cells. The safety and efficiency of aerosol inhalation of the exosomes derived from allogenic adipose MSCs-Exo in severe patients with novel coronavirus pneumonia (NCP) has been performed recently (NCT04276987). In addition, a Phase 1/2 clinical trial to explore the safety and efficacy of the exosomes derived from allogenic adipose MSCs-Exos in the treatment of mild to moderate dementia due to Alzheimer’s disease, is also undergoing (NCT04388982). The effect of adipose derived stem cells exosomes as an adjunctive therapy to scaling and root planning in the treatment of periodontitis is also undergoing (NCT04270006). Beyond the therapeutic purposes, roles of exosomes from fat tissue in lean and obese patients are also explored in clinical trial (NCT04167722). The clinical trials will open an avenue for the diagnosis and therapy based on adipose derived exosomes.
Beyond the native exosomes, exosomes from adipose tissue could be engineered to encapsulate cargos of interest. CD9-HuR engineered exosomes have found to successfully enrich the overexpressed RNA cargos with AREs (Li Z. et al., 2019). We have shown that exosomes-mediated delivery of Ldlr mRNA can treat diseases in Ldlr–/– mice (Li et al., 2021). Further engineering of exosomes from adipose MSCs-Exos would be a promising therapeutic strategy, especially when the wide source of adipose MSCs-Exo is considered.
The main limitations of in clinical application of adipose tissue-derived exosomes remain the resources and quality control among different batches. Isolation of the sufficient exosomes from the adipose tissue or establishment of the alternative resources is fundamental for potential clinical application. Techniques to ensure the exosome homogeneity during biogenesis, isolation, and quality control are also widely needed. The challenges could be solved when the fundamental procedures of exosome biogenesis and regulation are further clarified.
Author Contributions
LY and GY designed the framework, analyzed the data, and revised the manuscript. YL, CW, and MW contributed to data collection and manuscript drafting. All authors agreed to submit the final version.
Funding
This study was funded by NSFC 81671690 and 81871357 to LY, NSFC 31771507 and 81970737 to GY.
Conflict of Interest
The authors declare that the research was conducted in the absence of any commercial or financial relationships that could be construed as a potential conflict of interest.
References
Ahmadi, M., and Rezaie, J. (2020). Tumor cells derived-exosomes as angiogenenic agents: possible therapeutic implications. J. Transl. Med. 18:249. doi: 10.1186/s12967-020-02426-5
Akbar, N., Azzimato, V., Choudhury, R. P., and Aouadi, M. (2019). Extracellular vesicles in metabolic disease. Diabetologia 62, 2179–2187. doi: 10.1007/s00125-019-05014-5
Barreira, T. V., Harrington, D. M., Staiano, A. E., Heymsfield, S. B., and Katzmarzyk, P. T. (2011). Body adiposity index, body mass index, and body fat in white and black adults. JAMA 306, 828–830. doi: 10.1001/jama.2011.1189
Britton, K. A., Massaro, J. M., Murabito, J. M., Kreger, B. E., Hoffmann, U., and Fox, C. S. (2013). Body fat distribution, incident cardiovascular disease, cancer, and all-cause mortality. J. Am. Coll. Cardiol. 62, 921–925. doi: 10.1016/j.jacc.2013.06.027
Brown, N. K., Zhou, Z., Zhang, J., Zeng, R., Wu, J., Eitzman, D. T., et al. (2014). Perivascular adipose tissue in vascular function and disease: a review of current research and animal models. Arterioscler. Thromb. Vasc. Biol. 34, 1621–1630. doi: 10.1161/ATVBAHA.114.303029
Burkova, E. E., Dmitrenok, P. S., Bulgakov, D. V., Vlassov, V. V., Ryabchikova, E. I., and Nevinsky, G. A. (2018). Exosomes from human placenta purified by affinity chromatography on sepharose bearing immobilized antibodies against CD81 tetraspanin contain many peptides and small proteins. IUBMB Life 70, 1144–1155. doi: 10.1002/iub.1928
Chen, F., Zhang, H., Wang, Z., Ding, W., Zeng, Q., Liu, W., et al. (2017). Adipose-derived stem cell-derived exosomes ameliorate erectile dysfunction in a rat model of type 2 diabetes. J. Sex. Med. 14, 1084–1094. doi: 10.1016/j.jsxm.2017.07.005
Chen, Y., and Pfeifer, A. (2017). Brown fat-derived exosomes: small vesicles with big impact. Cell Metab. 25, 759–760. doi: 10.1016/j.cmet.2017.03.012
Choi, C. H. J., and Cohen, P. (2017). Adipose crosstalk with other cell types in health and disease. Exp. Cell Res. 360, 6–11. doi: 10.1016/j.yexcr.2017.04.022
Cypess, A. M., and Kahn, C. R. (2010). The role and importance of brown adipose tissue in energy homeostasis. Curr. Opin. Pediatr. 22, 478–484. doi: 10.1097/MOP.0b013e32833a8d6e
Dang, S. Y., Leng, Y., Wang, Z. X., Xiao, X., Zhang, X., Wen, T., et al. (2019). Exosomal transfer of obesity adipose tissue for decreased miR-141-3p mediate insulin resistance of hepatocytes. Int. J. Biol. Sci. 15, 351–368. doi: 10.7150/ijbs.28522
de Jong, P. E., Verhave, J. C., Pinto-Sietsma, S. J., Hillege, H. L., and Group, P. S. (2002). Obesity and target organ damage: the kidney. Int. J. Obes. Relat. Metab. Disord 26 Suppl 4, S21–S24. doi: 10.1038/sj.ijo.0802213
Deng, Z. B., Poliakov, A., Hardy, R. W., Clements, R., Liu, C., Liu, Y., et al. (2009). Adipose tissue exosome-like vesicles mediate activation of macrophage-induced insulin resistance. Diabetes 58, 2498–2505. doi: 10.2337/db09-0216
Deregibus, M. C., Cantaluppi, V., Calogero, R., Lo Iacono, M., Tetta, C., Biancone, L., et al. (2007). Endothelial progenitor cell derived microvesicles activate an angiogenic program in endothelial cells by a horizontal transfer of mRNA. Blood 110, 2440–2448. doi: 10.1182/blood-2007-03-078709
Du, L., Li, G., Yang, Y., Yang, G., Wan, J., Ma, Z., et al. (2018). Exosomes from microRNA-199-3p-modified adipose-derived stem cells promote proliferation and migration of endothelial tip cells by downregulation of semaphorin 3A. Int. J. Clin. Exp. Pathol. 11, 4879–4888.
Fontana, L., Eagon, J. C., Trujillo, M. E., Scherer, P. E., and Klein, S. (2007). Visceral fat adipokine secretion is associated with systemic inflammation in obese humans. Diabetes 56, 1010–1013. doi: 10.2337/db06-1656
Fox, C. S., Massaro, J. M., Hoffmann, U., Pou, K. M., Maurovich-Horvat, P., Liu, C. Y., et al. (2007). Abdominal visceral and subcutaneous adipose tissue compartments: association with metabolic risk factors in the framingham heart study. Circulation 116, 39–48. doi: 10.1161/CIRCULATIONAHA.106.675355
Gao, J., Li, X., Wang, Y., Cao, Y., Yao, D., Sun, L., et al. (2020). Adipocyte-derived extracellular vesicles modulate appetite and weight through mTOR signalling in the hypothalamus. Acta Physiol. (Oxf) 228:e13339. doi: 10.1111/apha.13339
Guescini, M., Genedani, S., Stocchi, V., and Agnati, L. F. (2010). Astrocytes and Glioblastoma cells release exosomes carrying mtDNA. J. Neural Transm. (Vienna) 117, 1–4. doi: 10.1007/s00702-009-0288-8
Han, Y., Ren, J., Bai, Y., Pei, X., and Han, Y. (2019). Exosomes from hypoxia-treated human adipose-derived mesenchymal stem cells enhance angiogenesis through VEGF/VEGF-R. Int. J. Biochem. Cell Biol. 109, 59–68. doi: 10.1016/j.biocel.2019.01.017
Hu, L., Wang, J., Zhou, X., Xiong, Z., Zhao, J., Yu, R., et al. (2016). Exosomes derived from human adipose mensenchymal stem cells accelerates cutaneous wound healing via optimizing the characteristics of fibroblasts. Sci. Rep. 6:32993. doi: 10.1038/srep32993
Hubal, M. J., Nadler, E. P., Ferrante, S. C., Barberio, M. D., Suh, J. H., Wang, J., et al. (2017). Circulating adipocyte-derived exosomal MicroRNAs associated with decreased insulin resistance after gastric bypass. Obesity (Silver Spring) 25, 102–110. doi: 10.1002/oby.21709
Jayabalan, N., Lai, A., Ormazabal, V., Adam, S., Guanzon, D., Palma, C., et al. (2019). Adipose tissue exosomal proteomic profile reveals a role on placenta glucose metabolism in gestational diabetes mellitus. J. Clin. Endocrinol. Metab. 104, 1735–1752. doi: 10.1210/jc.2018-01599
Ji, C., and Guo, X. (2019). The clinical potential of circulating microRNAs in obesity. Nat. Rev. Endocrinol. 15, 731–743. doi: 10.1038/s41574-019-0260-0
Jin, J., Shi, Y., Gong, J., Zhao, L., Li, Y., He, Q., et al. (2019). Exosome secreted from adipose-derived stem cells attenuates diabetic nephropathy by promoting autophagy flux and inhibiting apoptosis in podocyte. Stem Cell Res. Ther. 10:95. doi: 10.1186/s13287-019-1177-1
Jin, J., Wang, Y., Zhao, L., Zou, W., Tan, M., and He, Q. (2020). Exosomal miRNA-215-5p Derived from adipose-derived stem cells attenuates epithelial-mesenchymal transition of podocytes by inhibiting ZEB2. Biomed. Res. Int. 2020:2685305. doi: 10.1155/2020/2685305
Kahn, C. R., Wang, G., and Lee, K. Y. (2019). Altered adipose tissue and adipocyte function in the pathogenesis of metabolic syndrome. J. Clin. Invest. 129, 3990–4000. doi: 10.1172/JCI129187
Kaisanlahti, A., and Glumoff, T. (2019). Browning of white fat: agents and implications for beige adipose tissue to type 2 diabetes. J. Physiol. Biochem. 75, 1–10. doi: 10.1007/s13105-018-0658-5
Kranendonk, M. E., Visseren, F. L., Van Herwaarden, J. A., Nolte-’T Hoen, E. N., De Jager, W., Wauben, M. H., et al. (2014). Effect of extracellular vesicles of human adipose tissue on insulin signaling in liver and muscle cells. Obesity (Silver Spring) 22, 2216–2223. doi: 10.1002/oby.20847
Labrecque, J., Laforest, S., Michaud, A., Biertho, L., and Tchernof, A. (2017). Impact of bariatric surgery on white adipose tissue inflammation. Can. J. Diabetes 41, 407–417. doi: 10.1016/j.jcjd.2016.12.003
Lehmann, B. D., Paine, M. S., Brooks, A. M., Mccubrey, J. A., Renegar, R. H., Wang, R., et al. (2008). Senescence-associated exosome release from human prostate cancer cells. Cancer Res. 68, 7864–7871. doi: 10.1158/0008-5472.CAN-07-6538
Li, M., Li, C., Liu, Y., Chen, Y., Wu, X., Yu, D., et al. (2015). Decreased secretion of adiponectin through its intracellular accumulation in adipose tissue during tobacco smoke exposure. Nutr. Metab. (Lond) 12:15. doi: 10.1186/s12986-015-0011-8
Li, X., Ballantyne, L. L., Yu, Y., and Funk, C. D. (2019). Perivascular adipose tissue-derived extracellular vesicle miR-221-3p mediates vascular remodeling. FASEB J. 33, 12704–12722. doi: 10.1096/fj.201901548R
Li, X., Xie, X., Lian, W., Shi, R., Han, S., Zhang, H., et al. (2018). Exosomes from adipose-derived stem cells overexpressing Nrf2 accelerate cutaneous wound healing by promoting vascularization in a diabetic foot ulcer rat model. Exp. Mol. Med. 50:29. doi: 10.1038/s12276-018-0058-5
Li, Z., Zhao, P., Zhang, Y., Wang, J., Wang, C., Liu, Y., et al. (2021). Exosome-based ldlr gene therapy for familial hypercholesterolemia in a mouse model. Theranostics 11, 2953–2965. doi: 10.7150/thno.49874
Li, Z., Zhou, X., Wei, M., Gao, X., Zhao, L., Shi, R., et al. (2019). In vitro and in vivo RNA inhibition by CD9-hur functionalized exosomes encapsulated with miRNA or CRISPR/dCas9. Nano Lett. 19, 19–28. doi: 10.1021/acs.nanolett.8b02689
Liang, X., Zhang, L., Wang, S., Han, Q., and Zhao, R. C. (2016). Exosomes secreted by mesenchymal stem cells promote endothelial cell angiogenesis by transferring miR-125a. J. Cell Sci. 129, 2182–2189. doi: 10.1242/jcs.170373
Liu, T., Sun, Y. C., Cheng, P., and Shao, H. G. (2019). Adipose tissue macrophage-derived exosomal miR-29a regulates obesity-associated insulin resistance. Biochem. Biophys. Res. Commun. 515, 352–358. doi: 10.1016/j.bbrc.2019.05.113
Liu, X., Wang, S., You, Y., Meng, M., Zheng, Z., Dong, M., et al. (2015). Brown adipose tissue transplantation reverses obesity in Ob/Ob mice. Endocrinology 156, 2461–2469. doi: 10.1210/en.2014-1598
Liu, X., Zheng, Z., Zhu, X., Meng, M., Li, L., Shen, Y., et al. (2013). Brown adipose tissue transplantation improves whole-body energy metabolism. Cell Res. 23, 851–854. doi: 10.1038/cr.2013.64
Liu, Y., Tan, J., Ou, S., Chen, J., and Chen, L. (2019). Adipose-derived exosomes deliver miR-23a/b to regulate tumor growth in hepatocellular cancer by targeting the VHL/HIF axis. J. Physiol. Biochem. 75, 391–401. doi: 10.1007/s13105-019-00692-6
Liu, Y., Wang, Y., Wang, C., Shi, R., Zhou, X., Li, Z., et al. (2021). Maternal obesity increases the risk of fetal cardiac dysfunction via visceral adipose tissue derived exosomes. Placenta 105, 85–93. doi: 10.1016/j.placenta.2021.01.020
Lu, X., Bai, D., Liu, X., Zhou, C., and Yang, G. (2017). Sedentary lifestyle related exosomal release of Hotair from gluteal-femoral fat promotes intestinal cell proliferation. Sci. Rep. 7:45648. doi: 10.1038/srep45648
Mahmoudi, M., Taghavi-Farahabadi, M., Rezaei, N., and Hashemi, S. M. (2019). Comparison of the effects of adipose tissue mesenchymal stromal cell-derived exosomes with conditioned media on neutrophil function and apoptosis. Int. Immunopharmacol. 74:105689. doi: 10.1016/j.intimp.2019.105689
Martinez, M. C., and Andriantsitohaina, R. (2017). Extracellular vesicles in metabolic syndrome. Circ. Res. 120, 1674–1686. doi: 10.1161/CIRCRESAHA.117.309419
Masszi, A., Fan, L., Rosivall, L., Mcculloch, C. A., Rotstein, O. D., Mucsi, I., et al. (2004). Integrity of cell-cell contacts is a critical regulator of TGF-β1-induced epithelial-to-myofibroblast transition. Am. J. Pathol. 165, 1955–1967. doi: 10.1016/s0002-9440(10)63247-6
Nedergaard, J., Bengtsson, T., and Cannon, B. (2011). New powers of brown fat: fighting the metabolic syndrome. Cell Metab. 13, 238–240. doi: 10.1016/j.cmet.2011.02.009
Oelkrug, R., Polymeropoulos, E. T., and Jastroch, M. (2015). Brown adipose tissue: physiological function and evolutionary significance. J. Comp. Physiol. B 185, 587–606. doi: 10.1007/s00360-015-0907-7
Pan, Y., Hui, X., Hoo, R. L. C., Ye, D., Chan, C. Y. C., Feng, T., et al. (2019). Adipocyte-secreted exosomal microRNA-34a inhibits M2 macrophage polarization to promote obesity-induced adipose inflammation. J. Clin. Invest. 129, 834–849. doi: 10.1172/JCI123069
Qu, Y., Zhang, Q., Cai, X., Li, F., Ma, Z., Xu, M., et al. (2017). Exosomes derived from miR-181-5p-modified adipose-derived mesenchymal stem cells prevent liver fibrosis via autophagy activation. J. Cell Mol. Med. 21, 2491–2502. doi: 10.1111/jcmm.13170
Sano, S., Izumi, Y., Yamaguchi, T., Yamazaki, T., Tanaka, M., Shiota, M., et al. (2014). Lipid synthesis is promoted by hypoxic adipocyte-derived exosomes in 3T3-L1 cells. Biochem. Biophys. Res. Commun. 445, 327–333. doi: 10.1016/j.bbrc.2014.01.183
Scheja, L., and Heeren, J. (2019). The endocrine function of adipose tissues in health and cardiometabolic disease. Nat. Rev. Endocrinol. 15, 507–524. doi: 10.1038/s41574-019-0230-6
Scherer, P. E. (2006). Adipose tissue: from lipid storage compartment to endocrine organ. Diabetes 55, 1537–1545. doi: 10.2337/db06-0263
Schinzari, F., Tesauro, M., and Cardillo, C. (2017). Endothelial and perivascular adipose tissue abnormalities in obesity-related vascular dysfunction: novel targets for treatment. J. Cardiovasc. Pharmacol. 69, 360–368. doi: 10.1097/FJC.0000000000000469
Shao, H., Im, H., Castro, C. M., Breakefield, X., Weissleder, R., and Lee, H. (2018). New technologies for analysis of extracellular vesicles. Chem. Rev. 118, 1917–1950. doi: 10.1021/acs.chemrev.7b00534
Stanford, K. I., Middelbeek, R. J., Townsend, K. L., An, D., Nygaard, E. B., Hitchcox, K. M., et al. (2013). Brown adipose tissue regulates glucose homeostasis and insulin sensitivity. J. Clin. Invest. 123, 215–223. doi: 10.1172/JCI62308
Thomou, T., Mori, M. A., Dreyfuss, J. M., Konishi, M., Sakaguchi, M., Wolfrum, C., et al. (2017). Adipose-derived circulating miRNAs regulate gene expression in other tissues. Nature 542, 450–455. doi: 10.1038/nature21365
Tian, Y., Li, S., Song, J., Ji, T., Zhu, M., Anderson, G. J., et al. (2014). A doxorubicin delivery platform using engineered natural membrane vesicle exosomes for targeted tumor therapy. Biomaterials 35, 2383–2390. doi: 10.1016/j.biomaterials.2013.11.083
van Eenige, R., Van Der Stelt, M., Rensen, P. C. N., and Kooijman, S. (2018). Regulation of adipose tissue metabolism by the endocannabinoid system. Trends Endocrinol. Metab. 29, 326–337. doi: 10.1016/j.tem.2018.03.001
van Niel, G., D’angelo, G., and Raposo, G. (2018). Shedding light on the cell biology of extracellular vesicles. Nat. Rev. Mol. Cell Biol. 19, 213–228. doi: 10.1038/nrm.2017.125
Wallace, J. G., Bellissimo, C. J., Yeo, E., Fei Xia, Y., Petrik, J. J., Surette, M. G., et al. (2019). Obesity during pregnancy results in maternal intestinal inflammation, placental hypoxia, and alters fetal glucose metabolism at mid-gestation. Sci. Rep. 9:17621. doi: 10.1038/s41598-019-54098-x
Wan, Z., Gao, X., Dong, Y., Zhao, Y., Chen, X., Yang, G., et al. (2018). Exosome-mediated cell-cell communication in tumor progression. Am. J. Cancer Res. 8, 1661–1673.
Wang, C., Li, Z., Liu, Y., and Yuan, L. (2021). Exosomes in atherosclerosis: performers, bystanders, biomarkers, and therapeutic targets. Theranostics 11, 3996–4010. doi: 10.7150/thno.56035
Wei, M., Gao, X., Liu, L., Li, Z., Wan, Z., Dong, Y., et al. (2020). Visceral adipose tissue derived exosomes exacerbate colitis severity via pro-inflammatory MiRNAs in high fat diet fed mice. ACS Nano 14, 5099–5110. doi: 10.1021/acsnano.0c01860
Wu, X., Liu, Y., Wei, W., and Liu, M. L. (2019). Extracellular vesicles in autoimmune vasculitis - little dirts light the fire in blood vessels. Autoimmun. Rev. 18, 593–606. doi: 10.1016/j.autrev.2018.12.007
Xie, Z., Wang, X., Liu, X., Du, H., Sun, C., Shao, X., et al. (2018). Adipose-derived exosomes exert proatherogenic effects by regulating macrophage foam cell formation and polarization. J. Am. Heart Assoc. 7:e007442. doi: 10.1161/JAHA.117.007442
Xu, F., Xiang, Q., Huang, J., Chen, Q., Yu, N., Long, X., et al. (2019). Exosomal miR-423-5p mediates the proangiogenic activity of human adipose-derived stem cells by targeting Sufu. Stem Cell Res Ther 10, 106. doi: 10.1186/s13287-019-1196-y
Yang, Y., Cai, Y., Zhang, Y., Liu, J., and Xu, Z. (2018). Exosomes secreted by adipose-derived stem cells contribute to angiogenesis of brain microvascular endothelial cells following oxygen-glucose deprivation in vitro through microRNA-181b/TRPM7 axis. J. Mol. Neurosci. 65, 74–83. doi: 10.1007/s12031-018-1071-9
Ying, W., Riopel, M., Bandyopadhyay, G., Dong, Y., Birmingham, A., Seo, J. B., et al. (2017). Adipose tissue macrophage-derived exosomal miRNAs can modulate in vivo and in vitro insulin sensitivity. Cell 171, 372–384.e312. doi: 10.1016/j.cell.2017.08.035.
Yu, Y., Du, H., Wei, S., Feng, L., Li, J., Yao, F., et al. (2018). Adipocyte-derived exosomal MiR-27a induces insulin resistance in skeletal muscle through repression of PPARgamma. Theranostics 8, 2171–2188. doi: 10.7150/thno.22565
Zhang, B., Yang, Y., Xiang, L., Zhao, Z., and Ye, R. (2019). Adipose-derived exosomes: a novel adipokine in obesity-associated diabetes. J. Cell Physiol. 234, 16692–16702. doi: 10.1002/jcp.28354
Zhang, H., Deng, T., Ge, S., Liu, Y., Bai, M., Zhu, K., et al. (2019). Exosome circRNA secreted from adipocytes promotes the growth of hepatocellular carcinoma by targeting deubiquitination-related USP7. Oncogene 38, 2844–2859. doi: 10.1038/s41388-018-0619-z
Zhang, W., Bai, X., Zhao, B., Li, Y., Zhang, Y., Li, Z., et al. (2018). Cell-free therapy based on adipose tissue stem cell-derived exosomes promotes wound healing via the PI3K/Akt signaling pathway. Exp. Cell Res. 370, 333–342. doi: 10.1016/j.yexcr.2018.06.035
Zhang, Y., Yu, M., Dai, M., Chen, C., Tang, Q., Jing, W., et al. (2017). miR-450a-5p within rat adipose tissue exosome-like vesicles promotes adipogenic differentiation by targeting WISP2. J. Cell Sci. 130, 1158–1168. doi: 10.1242/jcs.197764
Zhao, H., Shang, Q., Pan, Z., Bai, Y., Li, Z., Zhang, H., et al. (2018). Exosomes from adipose-derived stem cells attenuate adipose inflammation and obesity through polarizing M2 macrophages and beiging in white adipose tissue. Diabetes 67, 235–247. doi: 10.2337/db17-0356
Zhao, L., Johnson, T., and Liu, D. (2017). Therapeutic angiogenesis of adipose-derived stem cells for ischemic diseases. Stem Cell Res. Ther. 8:125. doi: 10.1186/s13287-017-0578-2
Zhao, Q., Yang, J., Liu, B., Huang, F., and Li, Y. (2019). Exosomes derived from mangiferin-stimulated perivascular adipose tissue ameliorate endothelial dysfunction. Mol. Med. Rep. 19, 4797–4805. doi: 10.3892/mmr.2019.10127
Zhou, X., Li, Z., Qi, M., Zhao, P., Duan, Y., Yang, G., et al. (2020). Brown adipose tissue-derived exosomes mitigate the metabolic syndrome in high fat diet mice. Theranostics 10, 8197–8210. doi: 10.7150/thno.43968
Keywords: adipose tissue, exosomes, clinical application, physiological functions, pathological functions
Citation: Liu Y, Wang C, Wei M, Yang G and Yuan L (2021) Multifaceted Roles of Adipose Tissue-Derived Exosomes in Physiological and Pathological Conditions. Front. Physiol. 12:669429. doi: 10.3389/fphys.2021.669429
Received: 18 February 2021; Accepted: 23 March 2021;
Published: 20 April 2021.
Edited by:
Linda Ruth Peterson, Washington University School of Medicine in St. Louis, United StatesReviewed by:
Jafar Rezaie, Urmia University of Medical Sciences, IranHaiyan Fu, Southern Medical University, China
Copyright © 2021 Liu, Wang, Wei, Yang and Yuan. This is an open-access article distributed under the terms of the Creative Commons Attribution License (CC BY). The use, distribution or reproduction in other forums is permitted, provided the original author(s) and the copyright owner(s) are credited and that the original publication in this journal is cited, in accordance with accepted academic practice. No use, distribution or reproduction is permitted which does not comply with these terms.
*Correspondence: Guodong Yang, yanggd@fmmu.edu.cn; Lijun Yuan, yuanlj@fmmu.edu.cn