- 1College of Fisheries, Henan Normal University, Xinxiang, China
- 2Ninghai Institute of Mariculture Breeding and Seed Industry, Zhejiang Wanli University, Ninghai, China
- 3Key Laboratory of Aquatic Germplasm Resources of Zhejiang, College of Biological and Environmental Sciences, Zhejiang Wanli University, Ningbo, China
Increasing evidence has revealed accumulated ammonia will cause adverse effects on the growth, reproduction, and survival of aquatic animals. As a marine benthic mollusk, the razor clam Sinonovacula constricta shows better growth and survival under high ammonia nitrogen environment. However, little is known about its adaptation mechanisms to high ammonia stress in an integrated mariculture system. In this study, we analyzed the association between the polymorphism of glutamate dehydrogenase gene (GDH), a key gene involved in ammonia nitrogen detoxification, and ammonia tolerance. The results showed that 26 and 22 single-nucleotide polymorphisms (SNPs) of GDH in S. constricta (denoted as Sc-GDH) were identified from two geographical populations, respectively. Among them, two SNPs (c.323T > C and c.620C > T) exhibited a significant and strong association with ammonia tolerance, suggesting that Sc-GDH gene could serve as a potential genetic marker for molecular marker–assisted selection to increase survival rate and production of S. constricta. To observe the histological morphology and explore the histocellular localization of Sc-GDH, by paraffin section and hematoxylin–eosin staining, the gills were divided into gill filament (contains columnar and flattened cells) and gill cilia, whereas hepatopancreas was made up of individual hepatocytes. The results of immunohistochemistry indicated that the columnar cells of gill filaments and the endothelial cells of hepatocytes were the major sites for Sc-GDH secretion. Under ammonia stress (180 mg/L), the expression levels of Sc-GDH were extremely significantly downregulated at 24, 48, 72, and 96 h (P < 0.01) after RNA interference. Thus, we can speculate that Sc-GDH gene may play an important role in the defense process against ammonia stress. Overall, these findings laid a foundation for further research on the adaptive mechanisms to ammonia–nitrogen tolerance for S. constricta.
Introduction
In practice, some improper management methods such as high-density stocking and overfeeding lead to gradual ammonia nitrogen accumulation in rearing water, which are extremely toxic to the health of aquatic organisms. Ammonia is usually present in ionized () and unionized (NH3) states in water, and NH3 diffuses easily across membrane and into hemolymph of aquatic animals (Randall and Tsui, 2002). A number of studies have demonstrated that ammonia exposure resulted in histopathologic changes in gill and liver of Oreochromis niloticus (Benli et al., 2008), Cyprinus carpio (Peyghan and Takamy, 2002), and Portunus pelagicus (Romano and Zeng, 2006). Similarly, the gills of sliver catfish exposed to ammonia levels above LC50 (96 h) resulted in edema and fusion of the secondary lamellae (Miron et al., 2008). Additionally, the ammonia exposure induces oxidative stress and then further causes endoplasmic reticulum stress and apoptosis in the hepatopancreas of Litopenaeus vannamei (Liang et al., 2016). A similar pattern was observed in Ruditapes philippinarum showing that ammonia can destroy the stability of lysosomal membrane and cause cell apoptosis of the gills (Cong et al., 2017). However, the histocellular localization of genes associated with ammonia–nitrogen excretion in the gills and liver has been rarely reported. It should also be mentioned that Opsanus beta and Porichthys notatus exhibited remarkably different levels of the branchial expression of nitrogen transporters, both with regard to urea transporter proteins and Rhesus glycoprotein proteins, in accord with their disparate approaches to nitrogen metabolism (Bucking et al., 2013). Similarly, the changes of protein expression in the Na+/K+-ATPase and Rhesus glycoprotein C like of sea lamprey (Petromyzon marinus) gill during metamorphosis suggested that the methods of excreting ammonia were fundamentally changed (Sunga et al., 2020). Another study on immunofluorescence indicated that ammonium transporters might participate in ammonia excretion instead of ammonia absorption and assimilation in Tridacna squamosa (Boo et al., 2018). However, to date, little research focuses on the action site of genes related to detoxification metabolism of benthic mollusks exposed to high ammonia.
The razor clam Sinonovacula constricta is an economically important bivalve species along the coast of west Pacific Ocean, which has become one of the most popular seafood because of its delicious taste. In China, the clam yield is mainly obtained by polyculture with shrimps or crabs in seawater pond (Xie et al., 2011; Li et al., 2016). S. constricta, as a benthic bivalve, often lives in the sand mud from the surface to a depth of 40–50 cm in a comprehensive aquaculture system, so it always faces more severe ammonia nitrogen than other species because of the deposition and decay of surplus food and dead organisms in the sediment. However, it is still unclear about its underlying molecular mechanism to ammonia nitrogen detoxification. Long-term exposure to ammonia eventually leads to increased susceptibility of adult bivalves and embryos to contaminant stress or reduced reproductive capacity (Keppler, 2007), although mollusks have a high tolerance to pollutants (Saha et al., 2000). By comparing several marine bivalves, it has been found that the benthic species, such as R. philippinarum (Cong et al., 2019) and Corbicula fluminea (Zhang et al., 2019), had higher ammonia nitrogen tolerance than adhesive ones, such as oyster (Epifanio and Srna, 1975) and scallop (Widman et al., 2008). In this regard, different mollusks may have specific adaptive strategies to high concentration of ammonia.
It is commonly known that glutamate dehydrogenase (GDH) is a mitochondrial enzyme and widely exists in plants (Kanamori et al., 1972), animals (Spanaki and Plaitakis, 2012), and microorganisms (Kanamori et al., 1987). It can catalyze the reversible reaction α-ketoglutarate + NH3 + NADH ⇆ glutamate + NAD+ and is allosterically regulated by leucine, pyridine, adenine, and guanine nucleotides (Smith et al., 2001). In general, GDH maintains the low concentration of ammonia in the body by converting excess ammonia into glutamate when the concentration of ammonia nitrogen is higher than normal level (Cooper, 2012). Researches on the GDH gene in aquatic animals mainly focus on fish and crustaceans, such as Monopterus albus (Tng et al., 2010) and Penaeus monodon (Yang et al., 2015). By contrary, GDH gene has been rarely studied in mollusks. In our previous study, we found that the expression of Sc-GDH was significantly increased under acute ammonia stress (P < 0.05). Further, the results of quantitative real-time polymerase chain reaction (qRT-PCR) showed that the expression of Sc-GDH was higher in the gill and hepatopancreas than other tissues (P < 0.05) (Zhang et al., 2020). Therefore, we speculated that Sc-GDH gene is involved in ammonia tolerance by influencing the active site. To investigate this hypothesis, in this study, we identified the polymorphisms of Sc-GDH gene and analyzed its association with ammonia tolerance. Then, the histological structure of gills and hepatopancreas was observed, in order to further explore the histocellular localization of Sc-GDH protein by immunohistochemistry method. Furthermore, we performed an RNA interference (RNAi) experiment to analyze the expression levels of Sc-GDH. These works would help us understand ammonia adaptation mechanism and develop a viable source for the breeding of new ammonia-tolerant variety in S. constricta.
Materials and Methods
Ethics Statement
The razor clams S. constricta used in this work were collected from the genetic breeding research center of Zhejiang Wanli University, China. All experimental procedures were approved by the Institutional Animal Care and Use Committee of Zhejiang Wanli University, China.
Ammonia Challenge and Sample Collection
Two geographical populations of S. constricta with an average shell length of 63.26 ± 1.73 mm from the coastal areas of Ninghai, Zhejiang province (ZJ), and Changle, Fujian province (FJ), in China were collected and raised in the genetic breeding research center of Zhejiang Wanli University. All these clams were acclimatized in seawater with a salinity level of 22 at 22 ± 0.5°C with aeration and were fed with Chaetoceros muelleri in the morning and evening.
For ammonia challenge experiments, 1,200 individuals from each population were divided into five groups (240 clams per group): the control group (CG) and four ammonia stress groups (AG). The CG tank was filled with 500 L of natural seawater, while the four AG tanks were filled with 500 L of seawater with a high concentration of ammonia (180 mg/L), according to the 96-h LC50 values (Zhang et al., 2020). The ammonia concentration in the AG was adjusted by adding NH4Cl (Sangon, Shanghai, China) solution to the seawater, and the pH value was calibrated to 7.87 ± 0.23 by 10% NaOH solution. All the clams were monitored to eliminate the dead individuals every 2 h. The stress exposure lasted for 120 h. The surviving clams throughout the challenge experiment in the AGs of each population were classified as tolerant group (TG). Afterward, the gills of selected samples were dissected and immediately frozen in liquid nitrogen for RNA extraction. Moreover, the gills and hepatopancreas of six surviving individuals from the AG and CG from ZJ population were collected for paraffin section and immunohistochemistry test.
Primers and PCR Amplification
Total RNAs were extracted from gills using Trizol reagent (Omega, United States) according to the manufacturer’s instructions. RNA purity was measured using the NanoDrop 2000 spectrophotometers (Thermo Scientific, United States). RNA degradation and contamination were monitored on agarose electrophoresis. First-stand cDNA was synthesized from total RNA using PrimeScript RT reagent kit with gDNA Eraser (Takara, Japan). The thermal cycles were conducted in a PCR instrument (Bio-Rad, United States). Gene-specific primers were designed based on the sequence of Sc-GDH gene (GenBank accession no. MK451702) (Table 1). The amplifications were performed in a total volume of 25 μL, which contained 12.5 μL of Taq PCR Master Mix (Sangon, Shanghai, China), 1 μL of each primer (10 μM), 9.5 μL of PCR-grade water, and 1 μL of cDNA template. The PCR was carried out with the following conditions: 35 cycles of 95°C for 30 s, 58°C for 30 s, and 72°C for 1 min and a final extension of 72°C for 10 min. After amplification, the PCR products were detected by electrophoresis on 1% agarose gels. Specific PCR products were purified and sequenced.
Association Analysis Between the Single-Nucleotide Polymorphisms of Sc-GDH and Ammonia Tolerance
In the above ammonia challenge experiment, 96 and 132 survived individuals in FJ and ZJ population were regarded as the TGs, respectively. At the same time, 100 and 121 individuals in FJ and ZJ population were selected as CGs, respectively. Then, the total RNAs of all samples from the TGs and CGs were extracted, synthesized into cDNA, performed PCR amplification, and sequenced. The nucleotide sequences of the Sc-GDH from different individual clams were aligned using Mutation Surveyor. To detect the single-nucleotide polymorphisms (SNPs), χ2 of SPSS 22 software was employed to determine statistical significance. The observed heterozygosity (Ho), expected heterozygosity (He), effective allele (Ne), and polymorphism information content (PIC) of these SNPs were calculated by Popgen32. The Hardy–Weinberg equilibrium and associated loci linkage disequilibrium (LD) of these SNPs were analyzed by SHEsis online1 (Shi and He, 2006; Li et al., 2009).
Paraffin Section, Hematoxylin–Eosin Stain, and Immunofluorescence Staining
To test the cellular localization of Sc-GDH as well as the histological morphology in gills and hepatopancreas, the conventional paraffin sections, hematoxylin–eosin (H&E) staining, and immunofluorescence staining were performed. The tissues were fixed in 4% paraformaldehyde at 4°C for 24 h and then dehydrated with graded alcohol, transparent, infiltration paraffin, and paraffin-embedded. Paraffin sections (4 μm) were deparaffinized first and stained with hematoxylin (8 min) and eosin (1 min), respectively, and then photographed under an optical microscope (Nikon Eclipse 80i, Japan). In addition, paraffin sections were deparaffinized prior to immersion in 10 mM sodium citrate buffer for 20 min for antigen retrieval at a subboiling temperature (96°C). Sections were then blocked for 1 h in blocking solution (5% albumin from bovine serum) at room temperature, followed by primary antibody (antibody rabbit anti-GDH, produced privately by HuaBio, Zhejiang, 1:200) incubation (overnight, 4°C). Optimum primary antibody dilutions were predetermined by known positive control tissues. A known positive control section was included in each step to ensure proper staining. Primary antibody was detected by secondary antibodies Alexa Flour 488 donkey anti–rabbit immunoglobulin G (Invitrogen, A21206) and diluted at 1:250. Nuclei were stained with DAPI (Beyotime, Shanghai, China). The cells were observed and photographed under a fluorescence microscope (Nikon Eclipse 80i, Japan).
RNA Interference
Three hundred healthy adult clams selected from the genetic breeding research center of Zhejiang Wanli University (the average shell length was 62.43 ± 1.45 mm) were divided into three groups; the adductor muscles of all individuals of each group were injected with 5,000 ng diluted small interfering RNA (siRNA) of Sc-GDH (siRNA-GDH, Sangon, Shanghai, China), 5,000 ng diluted siRNA-negative control (NC), or 5,000 ng DEPC treated water (DEPC-W, blank control) and then were continuously cultured for 120-h exposure to 180 mg/L ammonia nitrogen seawater in three 500-L tanks (the management measures during the period are the same as above). The hepatopancreas tissues from six clams per treatment group were removed at 0, 24, 48, 72, 96, and 120 h postinjection.
For each sample, triplicate qRT-PCR reactions with iTaqTM universal SYBR® Green Supermix (Bio-Rad, CA, United States) following the manufacturer’s instructions were performed on Roche LightCycler® 480 System (Roche Diagnostics, Basel, Switzerland). Each containing 2 μg of total RNA as a template was performed with cycling conditions: 95°C for 10 min, 40 cycles of denaturation at 95°C for 10 s, annealing at 60°C for 1 min, and a final extension at 72°C for 10 s. The relative expression levels of Sc-GDH were calculated by the 2–Δ Δ Ct method. The ribosomal protein S9 (RS9) gene was used as an internal reference gene (Zhao et al., 2018). All PCR primers used in this study are listed in Table 1.
Results
Association Analysis Between SNPs of Sc-GDH and Ammonia Tolerance
In the ammonia challenge experiment, the first dead clam was observed in ZJ population at 24 h after ammonia stress, and the mortality rate was steadily increased and reached its apex level at 88–90 h. There were no mortalities found in the CG during the experiment. In this study, a total of 26 and 22 SNPs were detected by sequence comparisons in ZJ and FJ population, respectively (Supplementary Table 1). Each SNP was named after the rank-number of bases from the first base of mRNA sequence of Sc-GDH to the mutation locus for convenience. All loci of the two populations were synonymous mutations. The sample size, percent genotypes, and variation type of SNPs with significant differences of Sc-GDH in each population are shown in Table 2. The c.323T > C and c.620C > T were significantly different in genotype frequency between AG and TG in each population (P < 0.05) (Table 2), speculating that they are strongly associated with ammonia tolerance. The sequencing peak map showed that there were two highly consistent peaks at the mutation locus, which makes the c.323T > C and c.620C > T changes from homozygous to heterozygous (Figure 1).
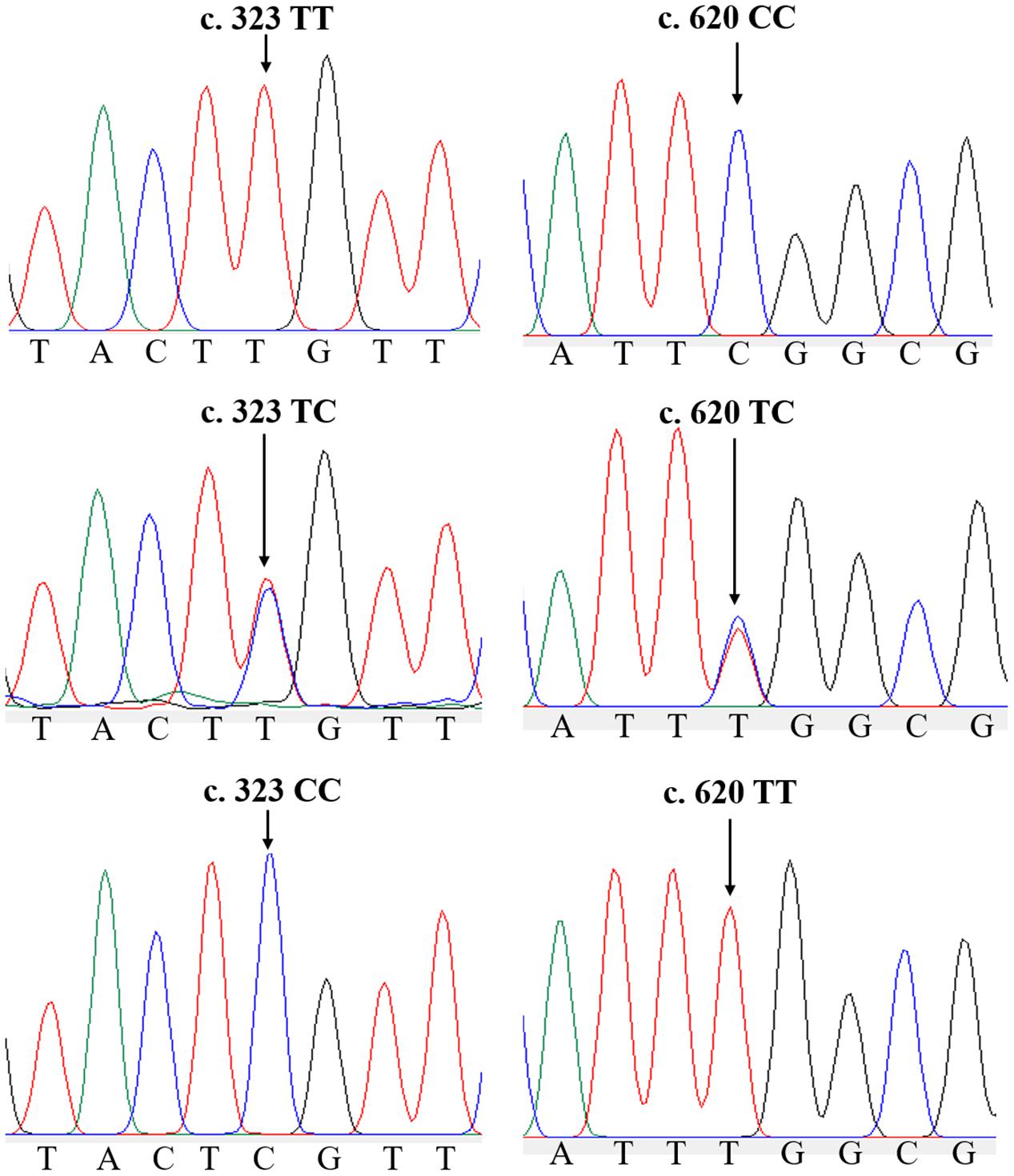
Figure 1. Two single-nucleotide polymorphisms (SNPs) of Sc-GDH gene. Peaks of normal and two mutated forms of c.323T > C and c.620C > T locus in Sc-GDH.
Of the 26 SNPs in ZJ population, 23 were transversions, whereas 20 transversions were found in 22 SNPs of FJ population (Supplementary Table 1). In addition, the Ho, He, Ne, and PIC values of these SNPs in two populations are shown in Supplementary Table 2. The c.323T > C represented a moderately polymorphic in ZJ population, whereas the c.620C > T indicated a moderately polymorphic in both two populations (Table 3). Further, the LD analysis revealed the linkage relationship (D’ > 0.75) between the c.323T > C and c.620C > T in both populations.
Histological Morphology and Histocellular Localization of Sc-GDH in Gills and Hepatopancreas
The histological observation of clam gills and hepatopancreas by paraffin section and H&E staining showed that nuclei were stained with hematoxylin (blue–purple), and the cytoplasm and components of the extracellular matrix were stained with eosin (red) (Figure 2). Gills were made up of cilia and filaments composed of a single layer of epithelial cells and their surrounding blood lumen. Among them, the epithelium of gill filaments was flat cells in about half of the area near the gill lumen; the remaining epithelial cells could be subdivided into columnar cells of frontal and lateral cilia according to the position of cilia (Figure 2A). Then, we proceeded to examine the expression and histocellular localization of Sc-GDH in the gills from S. constricta. The fluorescein isothiocyanate (FITC)–labeled antibody (antibody mouse anti-rabbit) selected in this study can specifically label Sc-GDH protein in tissues and display green fluorescence. Compared with the FITC staining (green) in the CG, the columnar cells in lateral cilia of gill in the AG showed a strong and positive signal (Figure 3).
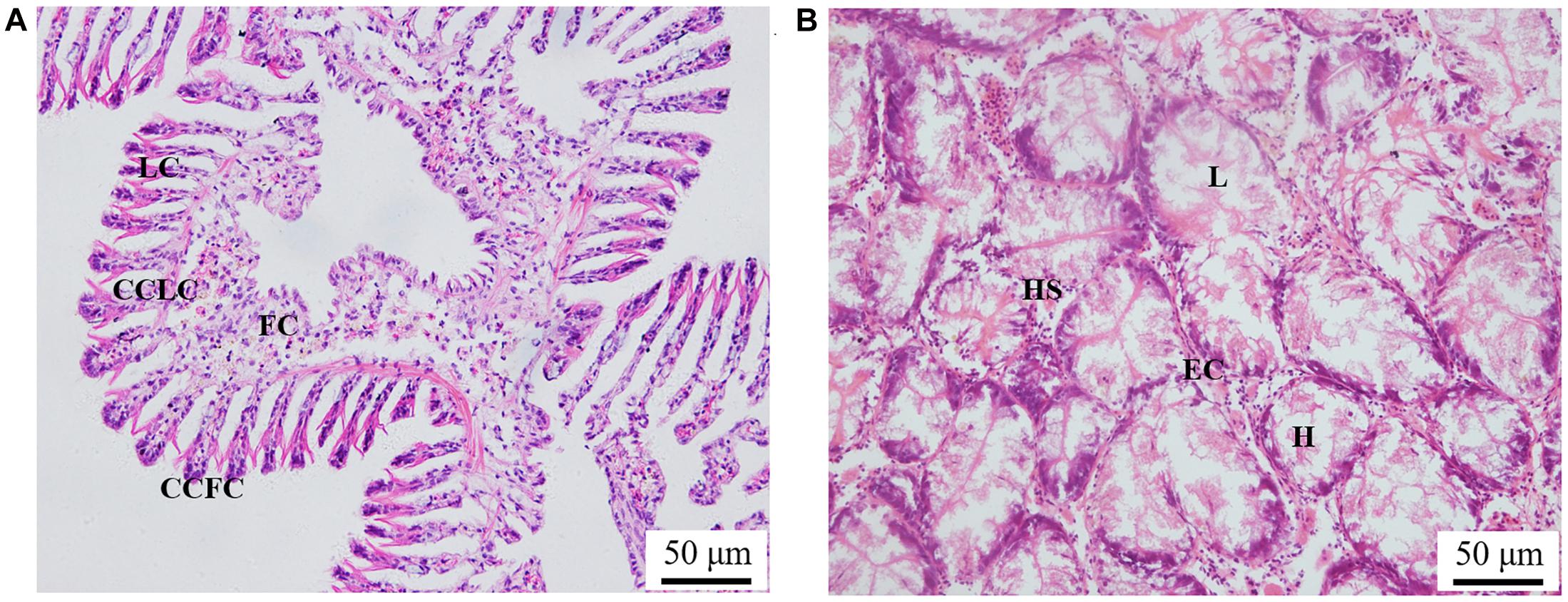
Figure 2. Paraffin section observation of gills in panel (A) and hepatopancreas in panel (B) in the Sinonovacula constricta. LC, lateral cilia; CCLC, columnar cells of lateral cilia; FC, flat cells; CCFC, columnar cells of frontal cilia; L, lumen; HS, hepatic sinusoid; EC, endothelial cells; H, hepatocytes. Scale bars were 50 μm.
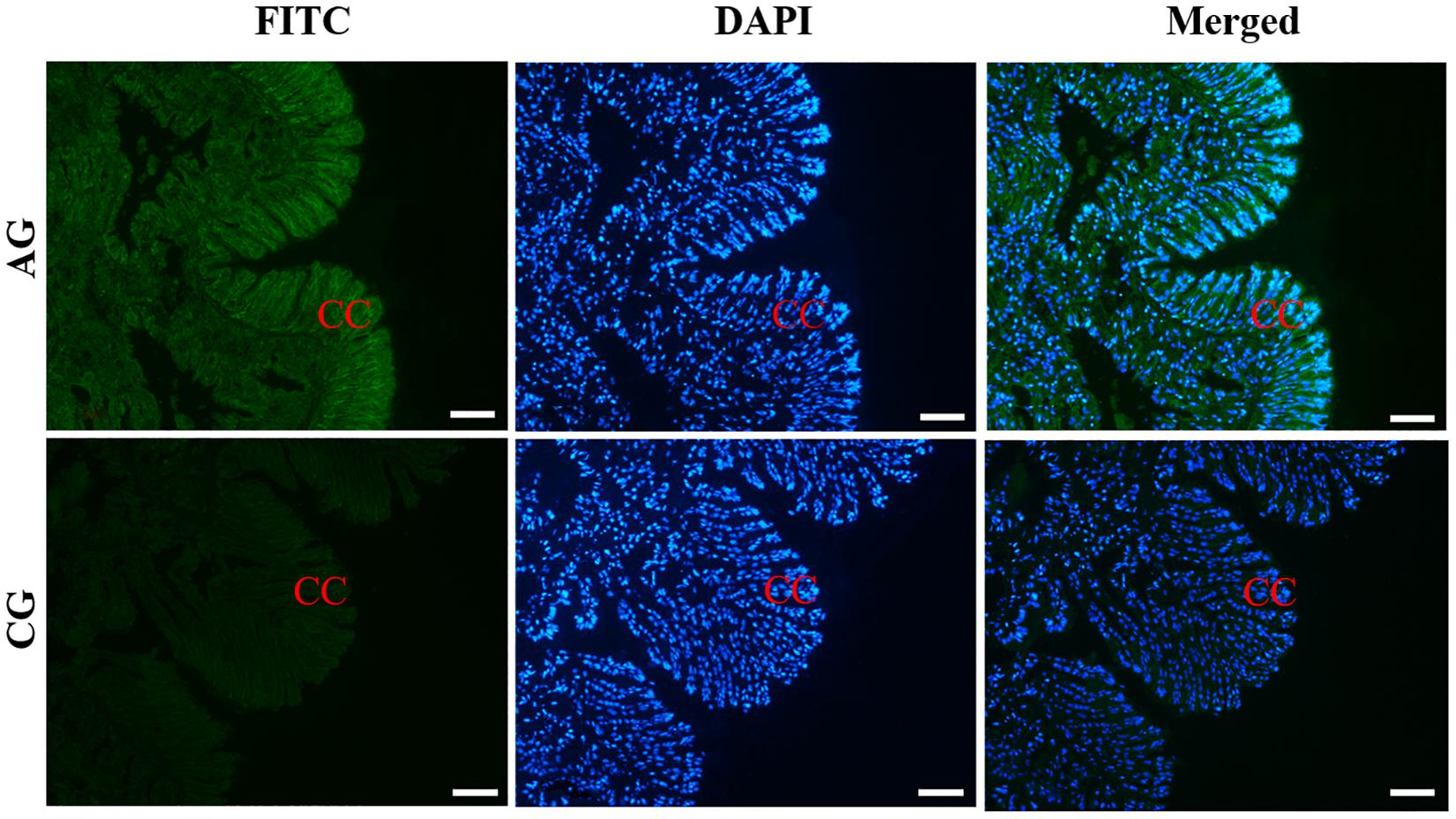
Figure 3. Immunofluorescence of Sc-GDH in the gills of S. constricta. Sc-GDH protein was stained with the anti-GDH antibody in green, and nuclei were stained with DAPI in blue. The merged images were co-localized with Sc-GDH protein (green) and DAPI (blue) in the columnar cells of lateral cilia of gills. CC, columnar cells. Scale bars were 50 μm.
Similarly, the histological structure of hepatopancreas was observed, and histocellular localization of Sc-GDH was performed. Hepatopancreas was made up of individual hepatocytes, which was irregularly elliptical sphere in shape, and hepatic sinusoid was formed between each hepatocyte cell. The wall of hepatic sinusoid was made up of endothelial cells, which enhances the permeability of hepatic sinusoid, and was conducive to the material exchange between hepatocyte cell and blood (Figure 2B). In addition, the different size of the lumens in the hepatocyte cells also increases the possibility of the material exchange between hepatocyte cell and blood (Figure 2B). On this basis, Sc-GDH was detected in endothelial cells of hepatic sinusoid in AG and showed positive signal, whereas CG exhibited extremely low expression of Sc-GDH (Figure 4). The combination diagram showed that the green and the blue signal did not coincide, indicating that Sc-GDH was not expressed in the nucleus (Figures 3, 4).
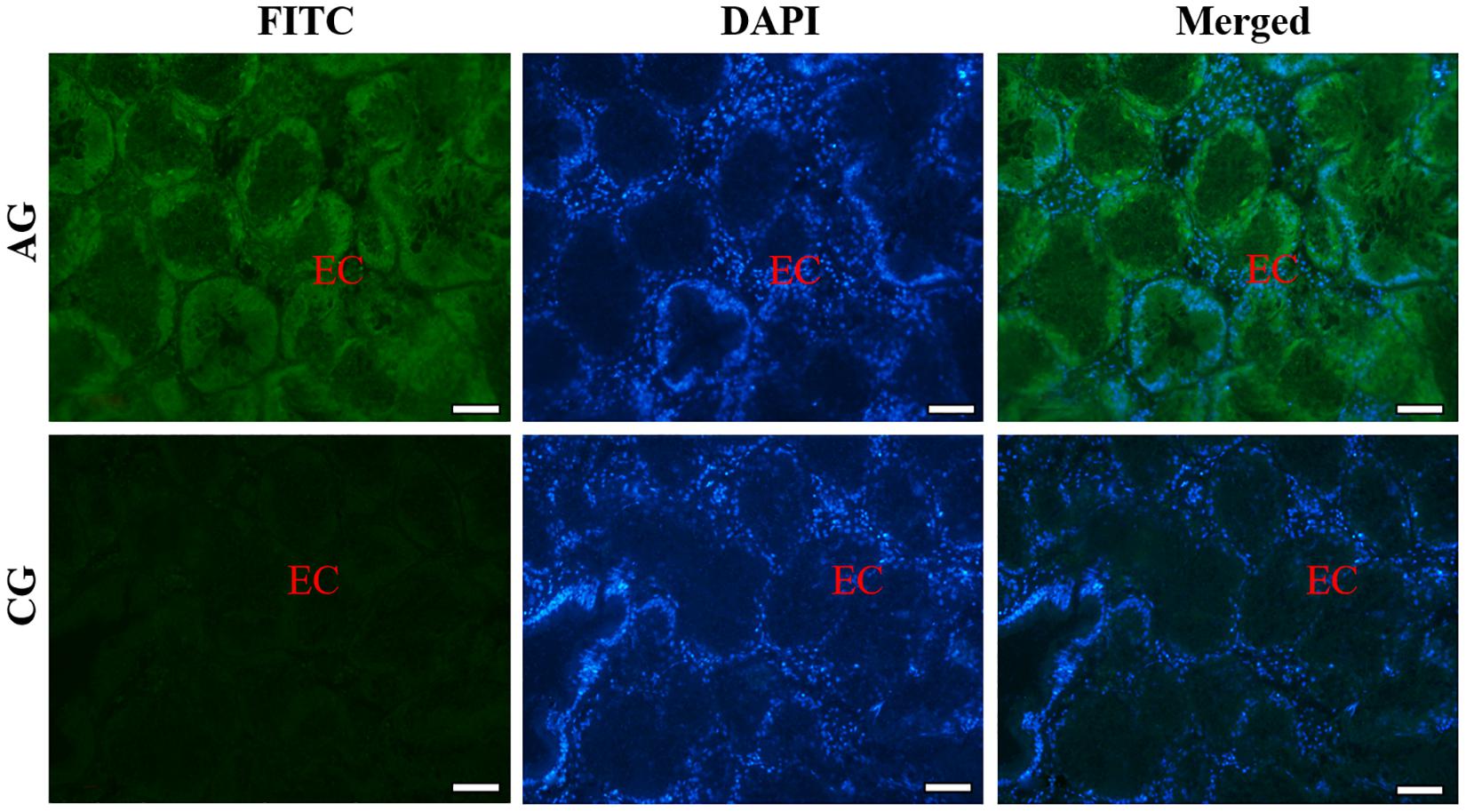
Figure 4. Immunofluorescence of Sc-GDH in the hepatopancreas of S. constricta. Sc-GDH protein was stained with the anti-GDH antibody in green, and nuclei were stained with DAPI in blue. The merged images were co-localized with Sc-GDH protein (green) and DAPI (blue) in the endothelial cells of hepatic sinusoid. EC, endothelial cells. Scale bars were 50 μm.
Expression of Sc-GDH After RNAi Silencing
RNA interference technology was used further investigate Sc-GDH function in the hepatopancreas. The results showed that a variation trend of the mRNA expression level of Sc-GDH was roughly the same in NC and DEPC-W. In addition, the mRNA expression level of Sc-GDH in siRNA-GDH was significantly lower than that in NC at 24, 48, 72, and 96 h (P < 0.01), which correspondingly downregulated to approximately 58.83, 71.41, 65.25, and 43.30% (Figure 5). However, there were no significant differences at 96–120 h.
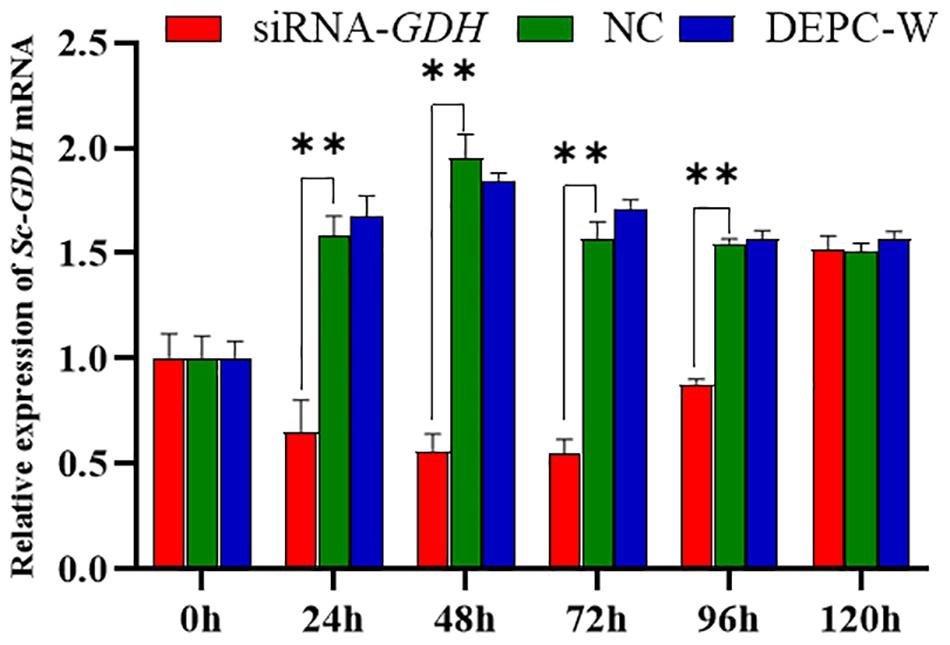
Figure 5. Relative expression of Sc-GDH genes in the hepatopancreas after RNA interference. Vertical bars represented the mean ± SD (n = 4). ∗∗Extremely significant difference between siRNA-GDH and NC (P < 0.01).
Discussion
Up to now, researches on the GDH gene in aquatic animals mainly focus on fish and crustaceans, such as Oncorhynchus mykiss (Wright et al., 2007), Periophthalmodon schlosseri, Boleophthalmus boddaerti (Ip et al., 2005), Clarias gariepinus (Wee et al., 2007), L. vannamei (Qiu et al., 2018), and Macrobrachium rosenbergii (Dong et al., 2020). However, the related studies on mollusks are very scarce. In this study, we inferred that Sc-GDH gene may be related with the antiammonia response of clams, and selected it as a candidate gene to detect the SNPs associated with ammonia tolerance traits in S. constricta. Furthermore, we explored the cellular localization of Sc-GDH in gill and hepatopancreas. In addition, to investigate the functions, the expression responses of Sc-GDH were determined under ammonia stress. To our knowledge, these studies were first carried out in S. constricta.
It has been proposed that SNP, a molecular genetic marker of the third generation, has a good application prospect in population genetic analysis of aquatic animal, molecular marker–assisted selection (MAS) and biological evolution (Wenne, 2017). Increasing evidence has shown that some SNPs are associated with growth, disease resistance, and other economic traits of clams (He et al., 2012; Siva et al., 2012, 2016; Nie et al., 2015; Niu et al., 2015; Yao et al., 2020; Zhao et al., 2020). For instance, a synonymous mutation SNP c.852A > G of insulin-like growth factor 2 mRNA-binding protein gene in Patinopecten yessoensis showed a significant association with the growth traits (Ning et al., 2018), while two SNPs HbIIA-E2-146 and HbIIB-E2-23 of the hemoglobin gene (Hb) in Tegillarca granosa were associated with the Vibrio parahaemolyticus resistance (Bao et al., 2013). The +2248T/C and +2365T/C of interferon regulatory factor 2 (IRF-2) gene in freshwater mussel Hyriopsis cumingii were significantly related to Aeromonas hydrophila resistance (Wang et al., 2013). Because of the advantages with high genetic stability and high detection accuracy among many molecular markers, SNP marker has been widely used in the MAS of mollusks varieties with economic traits (Syvänen, 2001). In this study, we found that two SNP loci (c.323T > C and c.620C > T) showed a significant association with the ammonia tolerance of razor clams. Given that the genetic selection program with the increasing ammonia tolerance in S. constricta is still in the infancy, our findings suggest that the SNP c.323T > C and c.620C > T of Sc-GDH gene could be served as a potential genetic marker for MAS to increase survival rate and clam production.
As we all know, GDH plays a vital role in the metabolic pathway of ammonia nitrogen detoxification (Spanaki and Plaitakis, 2012). In this study, 26 SNPs were found in the CDS region of Sc-GDH (1 per 61 bp) in ZJ population, whereas 22 SNPs (1 per 72 bp) were observed in FJ population. The difference in numbers of SNP loci between two populations may be due to the genetic divergence or deviation of sequencing. The screenings of SNP in bivalves indicated that there was a high frequency of SNPs (1 per 40 bp) and insertion/deletion (1 per 33 bp) polymorphisms in ESTs (Saavedra and Bachère, 2006). Several studies suggested that average density of SNPs was estimated to be 1 per 60 bp in coding regions and 1 per 40 bp in noncoding regions in Crassostrea gigas (Sauvage et al., 2007). Identical conclusions were obtained in this study, reflecting that bivalves have abundant genetic variation. Moreover, the frequency of C–T was much higher than A–G one, which can be explained by that C in C&G sequence spontaneously exchanging to T via methylation (Yebra and Bhagwat, 1995; Yoon et al., 2001). Furthermore, the preference for tRNA binding to degenerate cordons in gene translation is not random; synonymous mutations may alter or reduce gene translation efficiency (Kimchi-Sarfaty et al., 2007). It has been shown that the synonymous mutations can be used to encode additional information to affect the speed or accuracy of mRNA translation, mRNA folding, mRNA splicing, and protein folding through translation pausing, thus changing its function (Supek et al., 2014; Zwart et al., 2018). In this regard, the two synonymous mutations with significant trait differences found in this study suggest their promising applications in the selective breeding of new varieties.
Immunohistochemistry can visualize target protein to achieve histocellular localization, which has become the most effective method for protein expression localization (Lugos et al., 2020). As we all know, the hepatopancreas tissue is an essentially digestive and detoxification organ (Wu et al., 2013). Under a high concentration of ammonia situation, edema, serious vacuolization, and local necrosis occur in liver, which in turn affect its detoxification function and even cause the death of fish (Hargreaves and Kucuk, 2001; Mishra and Mohanty, 2008). Similarly, in this study, Sc-GDH protein distributions altered after ammonia stress, which were mostly expressed in the cytoplasm endothelial cells of hepatocytes. Identical conclusions were found in the livers of chicken and mouse (Akutsu and Miyazaki, 2002; Vázquez-Martínez et al., 2017). Collectively, these findings indicate that Sc-GDH in the hepatopancreas of razor clam plays a key role in response to external ammonia entering the bodies.
RNA interference has evolved into a powerful tool for probing the functions of genes (Hannon, 2002), which has successfully led to the silence of homologous genes (Fire et al., 1998). Studies have shown that RNAi inhibition of PmTNFR1 and PmTNFR5 downregulated the downstream genes, which suggested that they mediated the NF-κB signaling pathway and were closely related to immune defense in the pearl oyster (Wu et al., 2020). Similarly, the silencing experiment of LvGrx 2 expression indicated that it was involved in the regulation of oxidative defense and antioxidant system in shrimp under ammonia stress (Zheng et al., 2019). In our previous study, Sc-GDH gene expression in hepatopancreas after exposure to ammonia stress (180 mg/L) was significantly higher than that of the controls (Zhang et al., 2020). In this study, we successfully silenced the expression of Sc-GDH by injecting siRNA, with 71.41% interference efficiency in AG at 48 h. Therefore, we propose to evaluate the long-term interference by injecting siRNA-GDH in the razor clam every 48 h to explore the downstream genes related to detoxification metabolism of ammonia stress in the future.
In conclusion, two SNPs in the CDS region of Sc-GDH gene were significantly associated with ammonia tolerance in two geographical populations, which could serve as candidate markers for MAS selection of ammonia-tolerant clams. In addition, the main secretion location of Sc-GDH was observed in the columnar cells of gill filaments and the endothelial cells of hepatocytes. Finally, we found that 48 h was the best effect duration on inhibiting Sc-GDH gene expression. These findings would contribute to clarify the role of Sc-GDH in ammonia tolerance and provide a foundational knowledge on the detoxification metabolism of razor clams under ammonia stress.
Data Availability Statement
The datasets presented in this study can be found in online repositories. The names of the repository/repositories and accession number(s) can be found in the article/Supplementary Material.
Ethics Statement
The animal study was reviewed and approved by Institutional Animal Care and Use Committee (IACUC) of Zhejiang Wanli University, China.
Author Contributions
ZL, YD, and CS designed the study. GS performed the molecular analyses under the support of HY. Data analysis of the results was done by GS, YD, and CS. GS wrote the manuscript and all co-authors participated in the revisions of the manuscript. All authors contributed to the article and approved the submitted version.
Funding
This work was supported by National Key Research and Development Program of China (2018YFD0901405), Zhejiang Major Program of Science and Technology (2016C02055-9), Ningbo Major Project of Science and Technology (2019B10005), National Marine Genetic Resource Center Program, and Ningbo Top Discipline of Environmental Science and Engineering.
Conflict of Interest
The authors declare that the research was conducted in the absence of any commercial or financial relationships that could be construed as a potential conflict of interest.
Supplementary Material
The Supplementary Material for this article can be found online at: https://www.frontiersin.org/articles/10.3389/fphys.2021.664804/full#supplementary-material
Footnotes
References
Akutsu, S., and Miyazaki, J. (2002). Biochemical and immunohistochemical studies on tropomyosin and glutamate dehydrogenase in the chicken liver. Zool. Sci. 19, 275–286. doi: 10.2108/zsj.19.275
Bao, Y., Li, P., Dong, Y., Xiang, R., Gu, L., Yao, H., et al. (2013). Polymorphism of the multiple hemoglobins in blood clam Tegillarca granosa and its association with disease resistance to Vibrio parahaemolyticus. Fish Shellfish Immunol. 34, 1320–1324. doi: 10.1016/j.fsi.2013.02.022
Benli, A., Köksal, G., and Ozkul, A. (2008). Sublethal ammonia exposure of Nile tilapia (Oreochromis niloticus L.): effects on gill, liver and kidney histology. Chemosphere 72, 1355–1358. doi: 10.1016/j.chemosphere.2008.04.037
Boo, M., Hiong, K., Goh, E., Choo, C., Wong, W., Chew, S., et al. (2018). The ctenidium of the giant clam, Tridacna squamosa, expresses an ammonium transporter 1 that displays light-suppressed gene and protein expression and may be involved in ammonia excretion. J. Comp. Physiol. B Biochem. Syst. Environ. Physiol. 188, 765–777. doi: 10.1007/s00360-018-1161-6
Bucking, C., Edwards, S., Tickle, P., Smith, C., Mcdonald, M., and Walsh, P. (2013). Immunohistochemical localization of urea and ammonia transporters in two confamilial fish species, the ureotelic gulf toadfish (Opsanus beta) and the ammoniotelic plainfin midshipman (Porichthys notatus). Cell Tissue Res. 352, 623–637. doi: 10.1007/s00441-013-1591-0
Cong, M., Wu, H., Cao, T., Ji, C., and Lv, J. (2019). Effects of ammonia nitrogen on gill mitochondria in clam Ruditapes philippinarum. Environ. Toxicol. Pharmacol. 65, 46–52. doi: 10.1016/j.etap.2018.12.003
Cong, M., Wu, H., Yang, H., Zhao, J., and Lv, J. (2017). Gill damage and neurotoxicity of ammonia nitrogen on the clam Ruditapes philippinarum. Ecotoxicology 26, 459–469. doi: 10.1007/s10646-017-1777-4
Cooper, A. (2012). The role of glutamine synthetase and glutamate dehydrogenase in cerebral ammonia homeostasis. Neurochem. Res. 37, 2439–2455. doi: 10.1007/s11064-012-0803-4
Dong, X., Liu, Q., Kan, D., Zhao, W., Guo, H., and Lv, L. (2020). Effects of ammonia-N exposure on the growth, metabolizing enzymes, and metabolome of Macrobrachium rosenbergii. Ecotoxicol. Environ. Saf. 189:110046. doi: 10.1016/j.ecoenv.2019.110046
Epifanio, C., and Srna, R. (1975). Toxicity of ammonia, nitrite ion, nitrate ion, and orthophosphate to Mercenaria mercenaria and Crassostrea virginica. Mar. Biol. 33, 241–246. doi: 10.1007/BF00390928
Fire, A., Xu, S., Montgomery, M., Kostas, S., Driver, S., and Mello, C. (1998). Potent and specific genetic interference by double-stranded RNA in Caenorhabditis elegans. Nature 391, 806–811. doi: 10.1038/35888
Hargreaves, J., and Kucuk, S. (2001). Effects of diel un-ionized ammonia fluctuation on juvenile hybrid striped bass, channel catfish, and blue tilapia. Aquaculture 195, 163–181. doi: 10.1016/S0044-8486(00)00543-3
He, Y., Yu, H., Bao, Z., Zhang, Q., and Guo, X. (2012). Mutation in promoter region of a serine protease inhibitor confers Perkinsus marinus resistance in the eastern oyster (Crassostrea virginica). Fish Shellfish Immunol. 33, 411–417. doi: 10.1016/j.fsi.2012.05.028
Ip, Y., Leong, M., Sim, M., Goh, G., Wong, W., and Chew, S. (2005). Chronic and acute ammonia toxicity in mudskippers, Periophthalmodon schlosseri and Boleophthalmus boddaerti: brain ammonia and glutamine contents, and effects of methionine sulfoximine and MK801. J. Exp. Biol. 208, 1993–2004. doi: 10.1242/jeb.01586
Kanamori, K., Weiss, R., and Roberts, J. (1987). Role of glutamate dehydrogenase in ammonia assimilation in nitrogen-fixing Bacillus macerans. J. Bacteriol. 169, 4692–4695. doi: 10.1128/jb.169.10.4692-4695
Kanamori, T., Konishi, S., and Takahashi, E. (1972). Inducible formation of glutamate dehydrogenase in rice plant roots by the addition of ammonia to the media. Physiol. Plant. 26, 1–6. doi: 10.1111/j.1399-3054.1972.tb03536.x
Keppler, C. (2007). Effects of ammonia on cellular biomarker responses in oysters (Crassostrea virginica). Bull. Environ. Contamin. Toxicol. 78, 63–66. doi: 10.1007/s00128-007-9007-z
Kimchi-Sarfaty, C., Oh, J., Kim, I., Sauna, Z., Calcagno, A., Ambudkar, S., et al. (2007). A “silent” polymorphism in the MDR1 gene changes substrate specificity. Science 315, 525–528. doi: 10.1126/science.1135308
Li, L., Yan, B., Li, S., Xu, J., and An, X. (2016). A comparison of bacterial community structure in seawater pond with shrimp, crab, and shellfish cultures and in non-cultured pond in Ganyu, Eastern China. Ann. Microbiol. 66, 317–328. doi: 10.1007/s13213-015-1111-4
Li, Z., Zhang, Z., He, Z., Tang, W., Li, T., Zeng, Z., et al. (2009). A partition-ligation-combination-subdivision EM algorithm for haplotype inference with multiallelic markers: update of the SHEsis (http://analysis.bio-x.cn). Cell Res. 19, 519–523. doi: 10.1038/cr.2009.33
Liang, Z., Liu, R., Zhao, D., Wang, L., Sun, M., Wang, M., et al. (2016). Ammonia exposure induces oxidative stress, endoplasmic reticulum stress and apoptosis in hepatopancreas of pacific white shrimp (Litopenaeus vannamei). Fish Shellfish Immunol. 54, 523–528. doi: 10.1016/j.fsi.2016.05.009
Lugos, M., Davou, G., Choji, T., Jugu, K., Ashi, R., Oyero, S., et al. (2020). Using immunohistochemistry without linkers to determine the optimum concentrations of primary antibodies for immunofluorescence staining of formalin-fixed paraffin-embedded tissue sections. Appl. Immunohistochem. Mol. Morphol. 28, 249–257. doi: 10.1097/PAI.0000000000000718
Miron, D., Moraes, B., Becker, A., Crestani, M., Spanevello, R., Loro, V., et al. (2008). Ammonia and pH effects on some metabolic parameters and gill histology of silver catfish, Rhamdia quelen (Heptapteridae). Aquaculture 277, 192–196. doi: 10.1016/j.aquaculture.2008.02.023
Mishra, A., and Mohanty, B. (2008). Acute toxicity impacts of hexavalent chromium on behavior and histopathology of gill, kidney and liver of the freshwater fish, Channa punctatus (Bloch). Environ. Toxicol. Pharmacol. 26, 136–141. doi: 10.1016/j.etap.2008.02.010
Nie, Q., Yue, X., and Liu, B. (2015). Development of Vibrio spp. infection resistance related SNP markers using multiplex SNaPshot genotyping method in the clam Meretrix meretrix. Fish Shellfish Immunol. 43, 469–476. doi: 10.1016/j.fsi.2015.01.030
Ning, X., Feng, L., Li, X., Wang, S., Zhang, M., Wang, S., et al. (2018). The scallop IGF2 mRNA-binding protein gene PyIMP and association of a synonymous mutation with growth traits. Genes Genet. Syst. 93, 91–100. doi: 10.1266/ggs.17-00028
Niu, D., Wang, L., Bai, Z., Xie, S., Zhao, H., and Li, J. (2015). Identification and expression characterization of the myostatin (MSTN) gene and association analysis with growth traits in the razor clam Sinonovacula constricta. Gene 555, 297–304. doi: 10.1016/j.gene.2014.11.020
Peyghan, R., and Takamy, G. (2002). Histopathological, serum enzyme, cholesterol and urea changes in experimental acute toxicity of ammonia in common carp Cyprinus carpio and use of natural zeolite for prevention. Aquacult. Int. 10, 317–325. doi: 10.1023/A:1022408529458
Qiu, L., Shi, X., Yu, S., Han, Q., Diao, X., and Zhou, H. (2018). Changes of ammonia-metabolizing enzyme activity and gene expression of two strains in shrimp Litopenaeus vannamei under ammonia stress. Front. Physiol. 9:211. doi: 10.3389/fphys.2018.00211
Randall, D., and Tsui, T. (2002). Ammonia toxicity in fish. Mar. Pollut. Bull. 45, 17–23. doi: 10.1016/s0025-326x(02)00227-8
Romano, N., and Zeng, C. (2006). The effects of salinity on the survival, growth and haemolymph osmolality of early juvenile blue swimmer crabs, Portunus pelagicus. Aquaculture 260, 151–162. doi: 10.1016/j.aquaculture.2006.06.019
Saavedra, C., and Bachère, E. (2006). Bivalve genomics. Aquaculture 256, 1–14. doi: 10.1016/j.aquaculture.2006.02.023
Saha, N., Dutta, S., and Häussinger, D. (2000). Changes in free amino acid synthesis in the perfused liver of an air-breathing walking catfish, Clarias batrachus infused with ammonium chloride: a strategy to adapt under hyperammonia stress. J. Exp. Zool. 286, 13–23. doi: 10.1002/(sici)1097-010x(20000101)286:1<13::aid-jez2>3.0.co;2-x
Sauvage, C., Bierne, N., Lapègue, S., and Boudry, P. (2007). Single nucleotide polymorphisms and their relationship to codon usage bias in the Pacific oyster Crassostrea gigas. Gene 406, 13–22. doi: 10.1016/j.gene.2007.05.011
Shi, Y., and He, L. (2006). SHEsis, a powerful software platform for analyses of linkage disequilibrium, haplotype construction, and genetic association at polymorphism loci. Cell Res. 15, 97–98. doi: 10.1038/sj.cr.7290272
Siva, V., Wang, L., Qiu, L., Zhou, Z., Liu, C., Yang, J., et al. (2016). Polymorphism in a serine protease inhibitor gene and its association with the resistance of bay scallop (Argopecten irradians) to Listonella anguillarum challenge. Fish Shellfish Immunol. 59, 1–8. doi: 10.1016/j.fsi.2016.09.056
Siva, V., Yang, C., Yang, J., Wang, L., Wang, L., Zhou, Z., et al. (2012). Association between the polymorphism of CfPGRP-S1 gene and disease susceptibility/resistance of zhikong scallop (Chlamys farreri) to Listonella anguillarum challenge. Fish Shellfish Immunol. 33, 736–742. doi: 10.1016/j.fsi.2012.06.016
Smith, T., Peterson, P., Schmidt, T., Fang, J., and Stanley, C. (2001). Structures of bovine glutamate dehydrogenase complexes elucidate the mechanism of purine regulation. J. Mol. Biol. 307, 707–720. doi: 10.1006/jmbi.2001.4499
Spanaki, C., and Plaitakis, A. (2012). The role of glutamate dehydrogenase in mammalian ammonia metabolism. Neurotox. Res. 21, 117–127. doi: 10.1007/s12640-011-9285-4
Sunga, J., Wilson, J., and Wilkie, M. (2020). Functional re-organization of the gills of metamorphosing sea lamprey (Petromyzon marinus): preparation for a blood diet and the freshwater to seawater transition. J. Comp. Physiol. B 190, 701–715. doi: 10.1007/s00360-020-01305-1
Supek, F., Belén, M., Valcárcel, J., Gabaldón, T., and Lehner, B. (2014). Synonymous mutations frequently act as driver mutations in human cancers. Cell 156, 1324–1335. doi: 10.1016/j.cell.2014.01.051
Syvänen, A. (2001). Accessing genetic variation: genotyping single nucleotide polymorphisms. Nat. Rev. Genet. 2, 930–942. doi: 10.1038/35103535
Tng, Y., Chew, S., Wee, N., Wong, F., and Ip, Y. (2010). Acute ammonia toxicity and the protective effects of methionine sulfoximine on the swamp eel, Monopterus albus. J. Exp. Zool. A Ecol. Genet. Physiol. 311, 676–688. doi: 10.1002/jez.555
Vázquez-Martínez, O., Méndez, I., Turrubiate, I., Valente-Godínez, H., Pérez-Mendoza, M., García-Tejada, P., et al. (2017). Restricted feeding modulates the daily variations of liver glutamate dehydrogenase activity, expression, and histological location. Exp. Biol. Med. 242, 945–952. doi: 10.1177/1535370217699533
Wang, G., Li, X., and Li, J. (2013). Association between SNPs in interferon regulatory factor 2 (IRF-2) gene and resistance to Aeromonas hydrophila in freshwater mussel Hyriopsis cumingii. Fish Shellfish Immunol. 34, 1366–1371. doi: 10.1016/j.fsi.2013.02.006
Wee, N., Tng, Y., Cheng, H., Lee, S., Chew, S., and Ip, Y. (2007). Ammonia toxicity and tolerance in the brain of the African sharptooth catfish, Clarias gariepinus. Aquat. Toxicol. 82, 204–213. doi: 10.1016/j.aquatox.2007.02.015
Wenne, R. (2017). Single nucleotide polymorphism markers with applications in aquaculture and assessment of its impact on natural populations. Aquat. Living Resour. 31:2. doi: 10.1051/alr/2017043
Widman, J., Meseck, S., Sennefelder, G., and Veilleux, D. (2008). Toxicity of un-ionized ammonia, nitrite, and nitrate to juvenile bay scallops, Argopecten irradians irradians. Arch. Environ. Contam. Toxicol. 54, 460–465. doi: 10.1007/s00244-007-9051-z
Wright, P., Steele, S., Huitema, A., and Bernier, N. (2007). Induction of four glutamine synthetase genes in brain of rainbow trout in response to elevated environmental ammonia. J. Exp. Biol. 210, 2905–2911. doi: 10.1242/jeb.003905
Wu, H., Ji, C., Wei, L., Zhao, J., and Lu, H. (2013). Proteomic and metabolomic responses in hepatopancreas of Mytilus galloprovincialis challenged by Micrococcus luteus and Vibrio anguillarum. J. Proteom. 94, 54–67. doi: 10.1016/j.jprot.2013.09.001
Wu, Y., He, J., Yao, G., Liang, H., and Huang, X. (2020). Molecular cloning, characterization, and expression of two TNFRs from the pearl oyster Pinctada fucata martensii. Fish Shellfish Immunol. 98, 147–159. doi: 10.1016/j.fsi.2020.01.010
Xie, B., Jiang, W., and Yang, H. (2011). Growth performance and nutrient quality of Chinese shrimp Penaeus chinensis in organic polyculture with razor clam Sinonovacula constricta or hard clam Meretrix meretrix. Bulgarian J. Agric. Sci. 17, 851–858.
Yang, L., Yang, Q., Jiang, S., Li, Y., Zhou, F., Li, T., et al. (2015). Metabolic, immune responses in prawn (Penaeus monodon) exposed to ambient ammonia. Aquacult. Int. 23, 1049–1062. doi: 10.1007/s10499-014-9863-6
Yao, H., Liu, C., Lin, D., Liu, S., Lin, Z., and Dong, Y. (2020). Polymorphisms of LAP3 gene and their association with the growth traits in the razor clam Sinonovacula constricta. Mol. Biol. Rep. 47, 1257–1264. doi: 10.1007/s11033-019-05231-6
Yebra, M., and Bhagwat, A. (1995). A cytosine methyltransferase converts 5-methylcytosine in DNA to thymine. Biochemistry 34, 14752–14757. doi: 10.1021/bi00045a016
Yoon, J., Smith, L., Feng, Z., Tang, M., Lee, C., and Pfeifer, G. (2001). Methylated CpG dinucleotides are the preferential targets for G-to-T transversion mutations induced by benzo[a]pyrene diol epoxide in mammalian cells: similarities with the p53 mutation spectrum in smoking-associated lung cancers. Cancer Res. 61, 7110–7117. doi: 10.1097/00002820-200110000-00014
Zhang, H., Sun, G., Lin, Z., Yao, H., and Dong, Y. (2020). The razor clam Sinonovacula constricta uses the strategy of conversion of toxic ammonia to glutamine in response to high environmental ammonia exposure. Mol. Biol. Rep. 47, 9579–9593. doi: 10.1007/s11033-020-06018-w
Zhang, T., Yan, Z., Zheng, X., Fan, J., Wang, S., Wei, Y., et al. (2019). Transcriptome analysis of response mechanism to ammonia stress in Asian clam (Corbicula fluminea). Aquat. Toxicol. 214:105235. doi: 10.1016/j.aquatox.2019.105235
Zhao, J., Cui, B., Yao, H., Lin, Z., and Dong, Y. (2020). A potential role of bone morphogenetic protein 7 in shell formation and growth in the razor clam Sinonovacula constricta. Front. Physiol. 11:1059. doi: 10.3389/fphys.2020.01059
Zhao, X., Fu, J., Jiang, L., Zhang, W., Shao, Y., Jin, C., et al. (2018). Transcriptome-based identification of the optimal reference genes as internal controls for quantitative RT-PCR in razor clam (Sinonovacula constricta). Genes Genomics 40, 603–613. doi: 10.1007/s13258-018-0661-9
Zheng, P., Wang, L., Wang, A., Zhang, X., Ye, J., Wang, D., et al. (2019). cDNA cloning and expression analysis of glutaredoxin (Grx) 2 in the Pacific white shrimp Litopenaeus vannamei. Fish Shellfish Immunol. 86, 662–671. doi: 10.1016/j.fsi.2018.12.011
Keywords: Sinonovacula constricta, GDH, ammonia stress, SNP, immunohistochemistry, RNAi
Citation: Sun G, Dong Y, Sun C, Yao H and Lin Z (2021) Vital Role of Glutamate Dehydrogenase Gene in Ammonia Detoxification and the Association Between its SNPs and Ammonia Tolerance in Sinonovacula constricta. Front. Physiol. 12:664804. doi: 10.3389/fphys.2021.664804
Received: 06 February 2021; Accepted: 22 March 2021;
Published: 05 May 2021.
Edited by:
Youji Wang, Shanghai Ocean University, ChinaCopyright © 2021 Sun, Dong, Sun, Yao and Lin. This is an open-access article distributed under the terms of the Creative Commons Attribution License (CC BY). The use, distribution or reproduction in other forums is permitted, provided the original author(s) and the copyright owner(s) are credited and that the original publication in this journal is cited, in accordance with accepted academic practice. No use, distribution or reproduction is permitted which does not comply with these terms.
*Correspondence: Zhihua Lin, zhihua9988@126.com; Changsen Sun, changsen_1977@hotmail.com