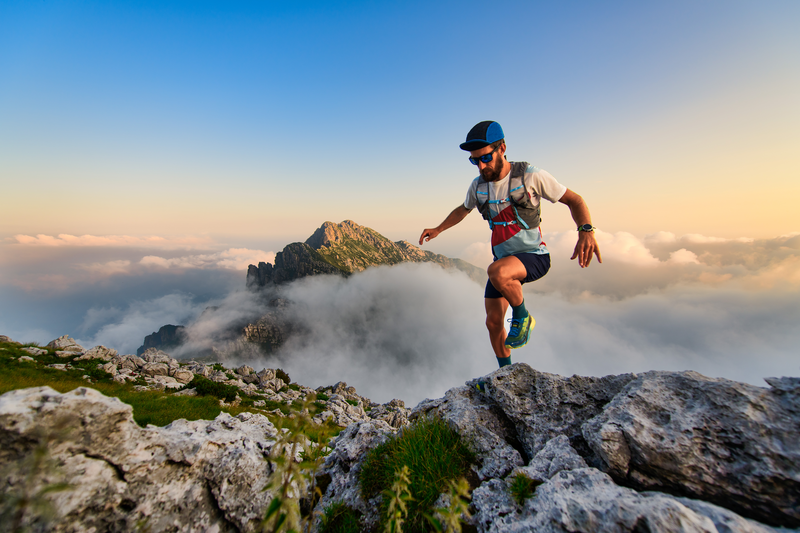
94% of researchers rate our articles as excellent or good
Learn more about the work of our research integrity team to safeguard the quality of each article we publish.
Find out more
ORIGINAL RESEARCH article
Front. Physiol. , 07 April 2021
Sec. Cardiac Electrophysiology
Volume 12 - 2021 | https://doi.org/10.3389/fphys.2021.658790
Background: Combined treatment with anthracyclines (e.g., doxorubicin; Dox) and trastuzumab (Trz), a humanized anti-human epidermal growth factor receptor 2 (HER2; ErbB2) antibody, in patients with HER2-positive cancer is limited by cardiotoxicity, as manifested by contractile dysfunction and arrhythmia. The respective roles of the two agents in the cardiotoxicity of the combined therapy are incompletely understood.
Objective: To assess cardiac performance, T-tubule organization, electrophysiological changes and intracellular Ca2+ handling in cardiac myocytes (CMs) using an in vivo rat model of Dox/Trz-related cardiotoxicity.
Methods and Results: Adult rats received 6 doses of either Dox or Trz, or the two agents sequentially. Dox-mediated left ventricular (LV) dysfunction was aggravated by Trz administration. Dox treatment, but not Trz, induced T-tubule disarray. Moreover, Dox, but not Trz monotherapy, induced prolonged action potential duration (APD), increased incidence of delayed afterdepolarizations (DADs) and beat-to-beat variability of repolarization (BVR), and slower Ca2+ transient decay. Although APD, DADs, BVR and Ca2+ transient decay recovered over time after the cessation of Dox treatment, subsequent Trz administration exacerbated these abnormalities. Trz, but not Dox, reduced Ca2+ transient amplitude and SR Ca2+ content, although only Dox treatment was associated with SERCA downregulation. Finally, Dox treatment increased Ca2+ spark frequency, resting Ca2+ waves, sarcoplasmic reticulum (SR) Ca2+ leak, and long-lasting Ca2+ release events (so-called Ca2+ “embers”), partially reproduced by Trz treatment.
Conclusion: These results suggest that in vivo Dox but not Trz administration causes T-tubule disarray and pronounced changes in electrical activity of CMs. While adaptive changes may account for normal AP shape and reduced DADs late after Dox administration, subsequent Trz administration interferes with such adaptive changes. Intracellular Ca2+ handling was differently affected by Dox and Trz treatment, leading to SR instability in both cases. These findings illustrate the specific roles of Dox and Trz, and their interactions in cardiotoxicity and arrhythmogenicity.
Anthracyclines (e.g., doxorubicin; Dox) are among the most efficient and frequently used chemotherapeutic agents, being prescribed to more than 40% of women with breast cancer (Giordano et al., 2012). Following anthracyclines and cyclophosphamide treatment, the human epidermal growth factor receptor 2 (HER2; ErbB2)/neu inhibitor trastuzumab (Trz), in combination with paclitaxel, improves outcomes in women with surgically removed HER2-positive breast cancer (Romond et al., 2005). However, both anthracyclines-related cardiotoxicity, including chronic congestive heart failure and Trz-related cardiotoxicity (Mor-Avi et al., 2011; Sawaya et al., 2012; Thavendiranathan et al., 2013), limit the clinical use of these agents. Because the simultaneous delivery of the two drugs results in enhanced cardiotoxicity, currently used clinical protocols involve their sequential administration. However, Dox/Trz combined therapy is still associated with a risk of left ventricular (LV) dysfunction in up to one-quarter of breast cancer patients (Ewer and Ewer, 2015; Advani et al., 2016; Ghigo et al., 2016), along with an increased risk of arrhythmia (Muraru et al., 2014; Santoro et al., 2017). In this regard, acute arrhythmogenicity of Dox administration has been reported (Steinberg et al., 1987). In a recent study from the Mayo Clinic, episodes of non-sustained ventricular tachycardia (VT), atrial fibrillation and sustained VT or ventricular fibrillation were seen respectively in 73.9, 56.6, and 30.4% of patients with anthracycline-related cardiomyopathy who had implantable cardioverter defibrillators (Mazur et al., 2017). Rare cases of malignant ventricular arrhythmias associated with Trz treatment have also been reported (Piotrowski et al., 2012). However, electrophysiological changes induced by Dox/Trz combined therapy are poorly characterized.
The underlying mechanisms of anthracyclines-induced cardiotoxicity are incompletely understood but increased oxidative stress, abnormal intracellular Ca2+ homeostasis and mitochondrial energetics, degradation of ultrastructural proteins, direct DNA damage via inhibition of topoisomerase 2β, and inhibition of pro-survival pathways such as neuregulin 1 (NRG) and ErbB (Dubey et al., 2016; Cappetta et al., 2017a) may be involved. In this regard, ErbB2 overexpression protected against Dox-related cardiotoxicity (Belmonte et al., 2015), whereas Trz-mediated inhibition of ErbB2 signaling interfered with the protective effects of ErbB2 and NRG, potentiating Dox-related toxicity in rat ventricular cardiac myocytes (CMs) (Sawyer et al., 2002).
The respective roles of Dox and Trz in cardiotoxicity induced by their combined administration remain to be fully elucidated. Here, we investigated these roles in T-tubule (TT) disarray, electrophysiological alterations and changes in intracellular Ca2+ handling using an in vivo rat model that mimics currently applied clinical regimens, specifically with respect to the sequential delivery of the two agents. Electrical measurements were performed at a single cell level. Dox treatment induced severe TT disarray, significant electrical abnormalities with a preserved Ca2+ handling. Although Trz monotherapy did not affect electrical activity and TT organization, administration of this agent following Dox pre-treatment exacerbated the abnormalities observed after the initial Dox treatment. These results suggest that CMs pre-stressed by Dox may become susceptible to Trz-mediated toxicity, especially electrical instability.
The animal protocol was approved by the Committee on the Ethics of Animal Experiments of the Canton Ticino, Switzerland (TI32/18). The study was carried out in strict accordance with the recommendations in the Guide for the Care and Use of Laboratory Animals of the Directive 2010/63/EU. The study protocol is depicted schematically in Figure 1A. Sprague Dawley female rats (10–12 weeks old; from Charles River Laboratories) were subdivided into four groups. In the Dox group, rats were injected i.p. with 6 doses of Dox hydrochloride (Sigma-Aldrich), one dose each other day (from d1 to d11), for a cumulative dosage of 20 mg/kg, followed by six doses of phosphate-buffered saline (PBS; pH 7.4), one dose each other day (from d19 to d29), as described (Milano et al., 2014). In the Trz group, rats received 6 doses of PBS (from d1 to d11) followed by 6 doses of Trz (Roche), one dose each other day (from d19 to d29), for a cumulative dosage of 20 mg/kg. In the combined Dox/Trz group, rats received 6 doses of Dox hydrochloride (from d1 to d11) followed by 6 doses of Trz (from d19 to d29). Control (Ctrl) rats received 12 doses of PBS at the time points corresponding to drug administration in treated groups (from d1 to d11, and from d19 to d29). Notably, Trz monotherapy was started at d19 of the study protocol to match the time point of Trz administration in the combined therapy group. Trz-mediated changes in LV function at later points were measured in a separate series of experiments (Supplementary Figure 1).
Figure 1. Effects of Dox and Trz on LV function. (A) Schema depicting the experimental protocol. Dox and Trz were administered sequentially (from d1 to d11, and from d19 to d29, respectively). The timing of Trz administration was the same in the Dox/Trz and Trz groups. (B) Echocardiographic results for LV end-systolic volume (LVESV), LV end-diastolic volume (LVEDV), LV ejection fraction (LVEF), and LV fractional shortening (LVFS) in different groups at varying time points. Two-way ANOVA for multiple groups has been used. Data are mean ± SEM; *p < 0.05 vs. Ctrl; §p < 0.05 vs. Trz (Ctrl, n = 5; Dox, n = 6; Trz, n = 8; Dox/Trz, n = 6). Additional data generated at later time points after Trz administration are shown in Supplementary Figure 1.
Heart function was monitored by echocardiography using a VEVO 2100 high-resolution imaging system (VisualSonics) at d0, d12, d19, d30, and d37, as described. Anesthesia was induced using 2% isoflurane mixed with 100% oxygen in an induction chamber. Rats were then placed on a heat pad in the supine position and kept at 37°C to minimize fluctuations of body temperature. Data acquisition was performed in rats lightly anesthetized with 0.5–1% isoflurane in order to maintain HR ≥ 350 bpm. Two-dimensional short-axis M-mode echocardiography was performed and LV end-systolic (LVESV) and end-diastolic (LVEDV) volumes, ejection fraction (LVEF) and fractional shortening (LVFS) were determined, as previously described (Barile et al., 2018).
Isolated CMs from in vivo treated rats were analyzed at d19 (early Dox time point), and at d37 (late Dox time point, early Trz time point, combined Dox/Trz treatment). For CMs isolation, rats were anesthetized using a cocktail of ketamine (100 mg/kg) and xylazine (75 mg/kg) and humanly euthanized by cervical dislocation. Hearts were harvested and perfused ex vivo in a Langendorff mode, as previously described (Rocchetti et al., 2014). CMs were isolated separately from LV and right ventricular (RV) free walls. Rod-shaped, Ca2+ tolerant CMs were used for patch-clamp measurements and confocal microscopy less than 12 h after tissue dissociation.
To investigate the impact of each treatment on the organization of TT, sarcolemmal membranes were marked by incubating CMs with 20 μM 3-di-ANEPPDHQ (Life Technologies, Carlsbad, CA, United States) and TT oriented transversely along z-lines were visualized (Rocchetti et al., 2014). 3-di-ANEPPDHQ was dissolved in the following solution (in mM): 40 KCl, 3 MgCl2, 70 KOH, 20 KH2PO4, 0.5 EGTA, 50 L-Glutamic acid, 20 Taurine, 10 HEPES, 10 D-glucose (pH 7.4) for 10 min at RT (Sacconi et al., 2012). Cell contraction was prevented by adding blebbistatin (17 μM; Sigma). CMs were washed with the same solution before confocal microscopy analysis. Images of loaded CMs were acquired by laser-scanning microscopy (images: 1,024 × 1,024 pxls, 78 μm × 78 μm) using a confocal microscope (Nikon C2 plus) with a 40× oil-immersion objective. Eight-bit gray-scaled images were analyzed by spatial Fast Fourier Transform analysis to quantify periodic components of pixel variance. To compensate for staining differences among cells, a raw power spectrum was generated with ImageJ (v.1.4) and normalized to its central peak. TT density was quantified by normalizing the area under the harmonic relative to the spatial frequency of 0.5 μm–1 (between 0.3 and 0.7 μm–1) to the area of the entire spectrum (Rocchetti et al., 2014).
Action potentials (AP) were recorded by pacing CMs at 1 Hz in current-clamp conditions. AP duration measured at 90% and 50% of the repolarization phase (APD90 and APD50, respectively), diastolic potential (Ediast) and maximal AP phase 0 depolarization velocity (dV/dtmax) were determined. Single cells were superfused with standard Tyrode’s solution containing (in mM): 154 NaCl, 4 KCl, 2 CaCl2, 1 MgCl2, 5.5 D-glucose, and 5 HEPES-NaOH (pH 7.35). Experiments were carried out in whole-cell configuration; the pipette solution contained (in mM): 23 KCl, 110 KAsp, 0.4 CaCl2, 3 MgCl2, 5 HEPES-KOH, 1 EGTA-KOH, 0.4 NaGTP, 5 Na2ATP, 5 Na2PC (pH 7.3). Delayed afterdepolarizations (DADs) were defined as diastolic depolarizations with amplitude ≥1 mV. The percentage of cells exhibiting DADs was quantified. Beat-to-beat variability of repolarization (BVR) was expressed as the short-term variability (STV) of APD90 (i.e., the mean orthogonal deviation from the identity line (Heijman et al., 2013; Altomare et al., 2015), calculated as follows:
for 30 consecutive APs (nbeats) at steady-state level. STV data are shown using APDn versus APDn + 1 (Poincaré) plots.
Cardiac myocytes were incubated in Tyrode’s solution for 45 min with the membrane-permeant form of the dye, Fluo4-AM (10 μmol/L), and then washed for 30 min to allow for the de-esterification process. Fluo4 emission was collected through a 535 nm band pass filter, converted to voltage, low-pass filtered (100 Hz) and digitized at 2 kHz after further low-pass digital filtering (FFT, 50 Hz). Intact CMs were field-stimulated at 1, 2 and 4 Hz at 37°C during superfusion with standard Tyrode’s solution. Ca2+ transient (CaT) amplitude at steady-state and the sarcoplasmic reticulum (SR) Ca2+ content (CaSR) estimated by an electronically timed 10 mmol/L caffeine pulse were evaluated at each cycle length. The diastolic fluorescence was used as reference (F0) for signal normalization (F/F0) after subtraction of background luminescence. For intergroup comparisons, Ca2+ handling parameters measured at each cycle length in a treated group were normalized to values measured in Ctrl. Rate-dependency of CaT decay kinetic was expressed as half-time decay (T0.5). Na+/Ca2+ exchanger (NCX) function was estimated by mono-exponential fit of caffeine-induced CaT. Frequency of resting Ca2+ waves was assessed under 1 min resting conditions before pacing. A Ca2+ wave was defined as a Ca2+ oscillation occurring at rest with an amplitude >3 SD over resting fluorescence levels (Frest). Comparable results were obtained using amplitude cutoffs up to 5 Frest SD. Frequency of spontaneous CaT occurring at rest (resting CaT) was assessed as an additional parameter of SR instability and Ca2+ overload.
Spontaneous unitary Ca2+ release events (Ca2+ sparks) were recorded at RT in Fluo4-AM (10 μM)-loaded CMs under resting conditions. Tyrode bath solution contained 2 mM CaCl2. Images were acquired at 60× magnification in line-scan mode (xt) at 0.5 kHz by confocal Nikon A1R microscope. Each cell was scanned along a longitudinal line and #10 xt frames (512 × 512 pxls) were acquired. Background fluorescence was measured. Confocal setting parameters were kept constant among experimental groups. Images were analyzed by SparkMaster plugin (Fiji) software (Picht et al., 2007). Automatic spark detection threshold was 3.8. Only in-focus Ca2+ sparks (amplitude > 0.3) were included in analyses. The following spark parameters were measured: frequency (event number/s/100 μm), amplitude (ΔF/F0), full width at half-maximal amplitude (FWHM; μm), full duration at half-maximal amplitude (FDHM; ms), full width (FW; μm) and full duration (FD; ms), time-to-peak (TtP, ms), and decay time constant (τ; ms). Spark mass (ΔF/F0∗μm3), an index of Ca2+ spark volume (Hollingworth et al., 2001), was calculated as spark amplitude∗1.206∗ FWHM3. Spark-mediated SR Ca2+ leak was calculated as the product of spark mass and frequency.
Proteins from CMs extracts were separated by SDS-polyacrylamide gel electrophoresis (4–12% Bis-Tris Criterion BIO-RAD gels), blotted for 1 h, incubated with polyclonal anti-SERCA2 primary antibody (N-19; Santa Cruz Biotechnology) at 4°C overnight, followed by incubation with a specific secondary antibody labeled with fluorescent markers (Alexa Fluor or IRDye) for 1 h. Signal intensity was quantified by Odyssey Infrared Imaging System (LI-COR). SERCA2 protein levels were normalized to actin levels, as measured using polyclonal anti-actin Ab (Sigma). Data are shown as percent changes vs. Ctrl.
Results are shown as mean ± SE. Unpaired Student’s t-test was used to test for significant differences in two-group analyses. One-way and two-way ANOVA were used to test for significance among multiple groups, with post hoc comparison analyses using Bonferroni’s multiple comparison test. Chi2-test was used for comparison of categorical variables. The statistical test used in each analysis is mentioned in the respective figure legends. Statistical significance was defined as p < 0.05.
Echocardiographic results are shown in Figure 1B. Compared to Ctrl, Dox-treated animals showed increases in LVESV at d19, d30, and d37, and in LVEDV at d19 and d37, along with decreases in LVEF and LVFS at all time points. In the absence of Dox pre-treatment, Trz-treated animals showed an increase in LVESV at d30 (i.e., one day after administration of the last Trz dose), and decreases in LVEF and LVFS. Of note, in this study Trz was administered from d19 to d29 to mimic clinical protocols that involve the sequential administration of the two agents to attenuate toxicity. In a separate study, we evaluated the effects of Trz on LV function for up to 37 days (i.e., the same time frame used for Dox). The results are shown in Supplementary Figure 1. Trz induced a transient decrease in LVFS at d12 and a transient increase in LVESV at d19. Animals receiving Dox/Trz combined therapy showed increases in LVESV and LVEDV, along with decreases in LVEF and LVFS at d30 and d37. Significant differences between Trz monotherapy and the combined Dox/Trz treatment were observed for LVESV and LVEF at d37, consistent with additive toxic effects by the two agents. Body weight, tibia length, and heart weight did not significantly differ among groups (Supplementary Table 1).
Representative confocal region of interest (ROI) of 3-di-ANEPPDHQ–treated CMs in the different groups are shown in Figure 2A. Disruption of TT architecture in the Dox and Dox/Trz groups, but not in the Trz group, can be appreciated visually. Representative spatial Fast Fourier Transform analyses of TT-power in LV and RV CMs are shown in Figure 2B. Quantitative analysis confirmed that Dox, but not Trz, treatment induces TT disarray (Figure 2C).
Figure 2. Effects of Dox and Trz on TT organization. (A) Confocal regions of interest (ROI) of a 3-di-ANEPPDHQ–treated LV CM from a Ctrl rat, and representative higher-magnification LV CM confocal ROI in all groups. Disruption of TT organization in the Dox and Dox/Trz groups, but not in the Trz group, is apparent. (B) Mean TT power spectra in LV and RV CMs in Dox (red), Trz (black) and Dox/Trz groups (green) at the indicated time points, superimposed to those in the Ctrl group (blue). (C) Quantitative analysis of the TT periodic component in LV and RV CMs. Data on the Dox group is shown at d19 and at d37. Unpaired Student’s t-test and one-way ANOVA were used to test for significant differences at d19 and d37, respectively. Bars are mean ± SEM (n = ≥15 cells from ≥ 3 rats/group; *p < 0.05; **p < 0.01).
LV and RV CMs were analyzed separately because electrical measurements are influenced by ventricular loading conditions. AP recordings in isolated CMs from either ventricle showed increases in both APD50 and APD90 in the Dox group at d19, but not at d37 (Figures 3A,B), indicating negligible effects at later time points. In the absence of Dox pre-treatment, Trz did not affect APD, whereas it significantly prolonged it in Dox pre-treated rats (Supplementary Table 2). Depolarizing events during diastole and systole were recorded as DADs and early afterdepolarizations (EADs), respectively. The percentage of cells exhibiting DADs at 1 Hz-stimulation was increased in Dox-treated animals at d19, but not at d37. In analogy to its effect on APD, Trz increased the frequency of DADs selectively in rats pre-treated with Dox (Figures 3C,D). Similar changes were found for beat-to-beat variability of repolarization (BVR), which reflects APD90 time-variability (i.e., electrical instability) and represents a pro-arrhythmic parameter (Johnson et al., 2010). The dispersion of APD90 values around the identity line in Poincaré plots was increased in the Dox group at d19 and in the Dox/Trz group, but not in the Dox group at d37 neither in Trz monotherapy (Figure 4A). Quantitative STV data are shown in Figure 4B. The slope of the linear correlation between STV and APD90 was comparable in all groups (Supplementary Figure 2), indicating that increases in BVR were strictly dependent on APD prolongation. These results indicate pro-arrhythmic conditions in both the Dox and Dox/Trz groups.
Figure 3. Effects of Dox and Trz on electrical activity. (A) Electrical recordings of stimulated (1 Hz) APs in LV and RV CMs in all groups. Measurements were performed at d19 and at d37 (before and after Trz administration, respectively). (B) Quantitative analysis of APD50 and APD90 showing APD prolongation in the Dox group (at d19) and in the Dox/Trz group (at d37). (C) Electrical recordings showing DAD at 1 Hz-stimulation, as well as EAD and trigger activity (TA) at 2 Hz-stimulation in the Dox/Trz group. (D) Quantitative analysis of cells (%) exhibiting DADs at 1 Hz-stimulation. Data were acquired at d19 and at d37 (see above). Unpaired Student’s t-test and one-way ANOVA was used to test for significant differences at d19 and d37, respectively, in panel (B). Chi2-test was used for comparison of categorical variables in panel (D). Bars are mean ± SEM (n = ≥12 cells from ≥3 rats/group; *p < 0.05; **p < 0.01).
Figure 4. Effects of Dox and Trz on beat-to-beat variability of repolarization (BVR). (A) Poincaré plots showing the dispersion of APD90 values around the identity line in LV and RV CMs in all groups. (B) Quantitative analysis of short-term variability (STV) of APD values. STV was increased in the Dox group at d19 and in the Dox/Trz group at d37. Data are shown at d19 and at d37. Unpaired Student’s t-test and one-way ANOVA was used to test for significant differences at d19 and d37, respectively. Bars are mean ± SEM (n = ≥12 from ≥3 rats/group; ∗p < 0.05).
In principle, TT disarray and changes in APD, DADs, and BVR can impact intracellular Ca2+ handling. We analyzed evocated Ca2+ transients (CaT) at 1, 2 and 4 Hz in field-stimulation, as well as caffeine-induced CaT which reflects SR Ca2+ content (CaSR; Supplementary Figure 3). Trz treatment resulted in significant decreases in CaT amplitude and CaSR in LV CMs, and to a slightly lesser extent in RV CMs. Unaltered or even increased CaT amplitudes were observed in Dox and Dox/Trz CMs (Figure 5A). Studies of the CaT decay kinetics showed a smaller decay half-time (T0.5) in Ctrl LV CMs compared to Ctrl RV CMs, in line with faster SR Ca2+ sequestration in LV CMs compared to RV CMs (Sathish et al., 2006). CaT decay in Dox d19 and to a lesser extent in Dox/Trz LV CMs was slower than in Ctrl (Figure 5B), suggesting Dox-induced changes in Ca2+ removal kinetics; a similar trend was observed in RV CMs. Accordingly, SERCA protein levels were significantly decreased in LV and RV CMs in both Dox and Dox/Trz CMs (Figure 5C). Trz alone did not significantly affect CaT decay kinetic and SERCA protein levels. To assess removal of intracellular Ca2+ through NCX, caffeine-induced CaT decay kinetic was evaluated. As shown in Supplementary Figure 4, rate-dependent NCX activity was observed in LV but not in RV Ctrl CMs, accordingly to previous data on chamber-specific NCX expression (Correia Pinto et al., 2006). However, rate-dependent NCX activity was absent in CMs from treated animals, particularly in the Trz group, suggesting faster NCX-dependent Ca2+ removal under these conditions, especially at slow pacing rates. The percentage of LV CMs exhibiting Ca2+ waves at resting was increased in all treated groups, with a similar increase in RV CMs from Dox d37 rats. The frequency of spontaneous CaT at resting in LV CMs was increased in both Dox and Dox/Trz groups (Figure 5D). These findings are in good agreement with our results on DAD frequency (Figures 3C,D).
Figure 5. Effects of Dox and Trz on intracellular Ca2+ handling. (A) Quantitative analysis of Ca2+ transient (CaT) amplitude and SR Ca2+ content (CaSR) estimated by a caffeine pulse at different pacing rates (1, 2, and 4 Hz) in field stimulated LV and RV CMs. Dox increased both CaT amplitude and CaSR in RV CMs but not in LV CMs, whereas Trz reduced them in both CMs. (B) Rate dependency of CaT decay kinetics (CaT decay half time, T0.5) in both RV and LV CMs. LV CaT decay was slower in the Dox and Dox/Trz groups, but not in the Trz group, compared to Ctrl. *p < 0.05 vs. Ctrl, §p < 0.05 vs. Trz. (C) Immunoblots showing SERCA protein levels (n = 2–4 rats/group; data in the Trz group and respective Ctrl were analyzed separately). Densitometric analysis. (D) Left panel: Example of Ca2+ wave (arrow) and spontaneous CaT occurring at resting. Quantitative analysis of cells (%) exhibiting spontaneous Ca2+ waves and resting CaT in both LV and RV CMs. Data on the Dox group are shown at d19 and at d37. One-way ANOVA was used to test for significant differences among multiple groups in panel (A,C). Mixed effect model of two-way ANOVA was used in panel (B) for statistical evaluation of CaT decay of multiple groups vs. pacing rate. Chi2-test was used for comparison of categorical variables in panel (D). Bars are mean ± SEM (n > 17 cells/group; *p < 0.05).
To sum up, in spite of SERCA downregulation, global Ca2+ handling was preserved in field-stimulated CMs in Dox and Dox/Trz groups, while SR instability was observed mainly at resting. This suggests that compensative mechanisms (i.e., increased Ca2+ influx during prolonged APs) may take place in Dox and Dox/Trz groups. Trz alone did not affect electrical activity while directly affect intracellular Ca2+ handling.
The effects of Dox on Ca2+ waves and resting CaT led us to investigate SR stability by quantifying spontaneous SR Ca2+ release events visualized as Ca2+ sparks. Representative images of Ca2+ sparks in the different LV groups are shown in Figure 6A. A similar pattern was observed in RV CMs. Increased frequencyof Ca2+ sparks was readily apparent in the Dox and Dox/Trz group, whereas Trz alone did not significantly impact this parameter. Spark mass was increased in LV CMs from Trz-treated animals, with similar trends in Dox-treated ones (Llach et al., 2019), but not in the Dox/Trz group. Dox, and to a lesser extent Trz, induced an increased spark-mediated SR Ca2+ leak quantified by the spark mass∗spark frequency index (Figure 6B). Dox, and to a lesser extent Trz, increased the number of so-called Ca2+ “embers,” defined as Ca2+ sparks with FDHM > 20 ms, the peak value of the FDHM distribution (Louch et al., 2013; Figure 6C and Supplementary Figure 5). Moreover, FDHM and the decay time constant (taudecay), two markers of altered RyR openings, were increased in LV myocytes of Dox-treated animals (Supplementary Table 3), supporting Dox-induced SR instability.
Figure 6. Effects of Dox and Trz on Ca2+ sparks. (A) Confocal xt-images of FLuo4-AM loaded LV CMs in all groups. (B) Quantitative analyses of Ca2+ sparks frequency, spark mass, and sparks-mediated SR Ca2+ leak (n = 14–24 cells from 3 to 5 rats per group). (C) Quantitative analysis of Ca2+ “embers” (i.e., Ca2+ sparks with FDHM > 20 ms). One-way ANOVA was used to test for significant differences between multiple groups in panel (B), and Chi2-test was used for comparison of categorical variables in panel (C). Bars are mean ± SEM (*p < 0.05).
Cardiotoxicity limits the application of anthracycline-based chemotherapy and anti-HER2 therapy combined regimens. Here, we investigated selected aspects of this condition including TT organization, electrophysiological changes, and intracellular Ca2+ handling using an in vivo rat model that mimics clinically used combined regimens. Dox treatment induced LV dysfunction lasting for more than 37 days. Trz monotherapy likewise impaired contractile function, albeit less severely than Dox, and for a shorter time period. The sequential administration of the two agents is reported to increase ROS levels and cardiac fibrosis, and to impair LV function to greater extents than did either agent alone, consistent with additive toxicity (Milano et al., 2014). Several mechanisms may be responsible for these findings. First, previous data in Erb2-mutated mice (Ozcelik et al., 2002), as well as in human induced pluripotent stem cell (hiPSC)-derived CMs suggested that pre-existing cellular stress induced by Dox may exacerbate Trz-related cardiotoxicity by inhibiting protective pathways including Erb2/4 (Tocchetti et al., 2012; Hsu et al., 2018; Kurokawa et al., 2018). It also has been shown that lapatinib, another HER2 inhibitor, potentiates Dox-related cardiotoxicity via iNOS signaling (Hsu et al., 2018).
Dox treatment, but not Trz, induced sustained TT disarray that accounted for, at least in part, LV dysfunction in this group. An association between TT disarray and heart failure of various etiologies including dilated cardiomyopathy has been reported previously in both human patients and animal models (Heinzel et al., 2008; Crocini et al., 2014; Crossman et al., 2015). Time course studies revealed that TT disruption preceded the development of heart failure, suggesting a causative role for the former in disease progression (Wei et al., 2010). In addition, altered TT structure has been shown to impair AP propagation (Crocini et al., 2017; Manfra et al., 2017). In the present study, Dox treatment resulted in APD prolongation in CMs. These results are in line with previous data on Dox-induced QT-interval prolongation in guinea pigs and reduced IKs component in stably transfected HEK293 cells (Ducroq et al., 2010). Another study reported increased INaL leading to changes in Ca2+ and Na+ handling in a model of Dox-induced LV diastolic dysfunction (Cappetta et al., 2017b). Moreover, Keung EC and coworkers (Keung et al., 1991) showed increased L-type Ca2+ current (ICaL) density and fast decay time constant of ICaL inactivation in LV CMs from Dox-treated rats, due to altered TT organization and excitation-contraction (EC)-coupling mechanisms. Thus, Dox-induced increases in ICaL and INaL could potentially account for the huge drug-induced APD prolongation in treated rats. Alternatively, K+ channels downregulation cannot be ruled out.
In our model, Dox-mediated APD prolongation was associated with increases in the frequency of DADs as a reflection of spontaneously released Ca2+ being extruded by NCX, as well as in BVR as an index of arrhythmogenicity. Trz treatment did not significantly impact APD, DADs, and BVR in the absence of Dox pre-treatment. In pre-treated rats, however, it induced changes in these parameters similar to those induced by Dox itself. Of note, these Trz-induced changes in pre-treated rats were observed after a full recovery in these electrical parameters following Dox treatment, suggesting that the latter exacerbated subsequent Trz toxicity. Clinical evidence of arrhythmia in Trz-treated patients has been reported (Piotrowski et al., 2012), although infrequently.
The analysis of intracellular Ca2+ handling revealed a preserved global Ca2+ handling in paced CMs from both LV and RV. Indeed, in spite of the slower SR Ca2+ uptake, SERCA downregulation and SR instability, CaT amplitude and CaSR were preserved in Dox and Dox/Trz LV CMs and even increased in RV CMs. These counterintuitive findings may reflect compensative mechanisms taking place in intact field-stimulated cells. Under such conditions, evocated CaT are influenced by changes in electrical activity. Especially in Dox19 and Dox/Trz group, AP prolongation may result in an increase in Ca2+ influx, which may compensate changes dependent on SERCA downregulation. CaT decay was slower after Dox/Trz combined therapy, and to a lesser extent after Dox monotherapy, but not after Trz monotherapy.
Unlike Dox, Trz treatment resulted in a decrease in both the amplitude of CaT and CaSR in LV CMs. Trz treatment didn’t affect APD, indicating that its effect on Ca2+ handling was independent on changes in electrical activity. While we did not analyze the precise mechanisms underlying Trz effects in this study, we hypothesize that the effect of this agent on Ca2+ handling might reflect metabolic changes, as previously described in hiPSC-derived CMs (Kitani et al., 2019). Moreover, sorafenib, a distinct tyrosine kinase inhibitor, is reported to reduce CaT amplitude and CaSR in human atrial CMs and in mouse ventricular CMs, inducing a reversible negative inotropic effect (Schneider et al., 2018).
During cell relaxation, released Ca2+ is recycled into the SR by the action of SERCA and extruded from the cell by NCX membrane protein, which plays a central role in the induction of Ca2+ waves. Dox treatment induced increases and in spontaneous Ca2+ waves and Ca2+ sparks which reflect SR instability. Previous studies have shown that low density of poorly organized TT favors de-synchronized and protracted Ca2+ release in failing CMs (Louch et al., 2004; Lyon et al., 2009), which has been linked to the slowed and decreased amplitude of contraction typical of the failing heart (Bokenes et al., 2008; Mork et al., 2009). Ca2+ spark mass, which reflects the amount of Ca2+ released within an individual spark, was increased by Trz in LV CMs, with a trend in the same direction for Dox. Previous data in isolated CMs suggest that ROS-dependent activation of CAMKII pathway may result in CaMKII-dependent SR Ca2+ leak contributing to Dox-mediated impairment of Ca2+ handling (Sag et al., 2011). Accordingly, in the present study, Dox induced an increase in prolonged spontaneous Ca2+ events (so-called Ca2+ “embers”) previously described in congestive heart failure (Louch et al., 2013), but not in chemotherapy-related cardiotoxicity. Ca2+ “embers” likely contributed to spontaneous SR Ca2+ leak, which was increased in all treated groups.
In conclusion, our data using an in vivo rat model of chemotherapy-related cardiotoxicity suggest that Dox treatment induces LV dysfunction, TT disarray, APD prolongation, electrical and SR instability, which are associated with a global preserved intracellular Ca2+ content regardless of SR abnormalities. Trz treatment alone induces a lesser degree of LV dysfunction, no TT disarray, no significant electrical changes. On the other hand, Trz affects intracellular Ca2+ handling, a finding that warrants further mechanistic characterization. However, Dox pre-treatment exacerbates Trz-related cardiotoxicity. While descriptive, our results highlight distinct yet interrelated cardiotoxic effects, including arrhythmogenicity, by the two agents when administered in combination.
The original contributions presented in the study are included in the article/Supplementary Material, further inquiries can be directed to the corresponding authors.
The animal study was reviewed and approved by the Ethics of Animal Experiments of the Canton Ticino, Switzerland (TI32/18).
CA, MR, LB, and GV contributed to the experimental design. GM and VB carried out the echocardiographic measurements and analysis. CA, SB, and NP performed patch-clamp experiments and data analysis. CA, NP, and LB performed confocal experiments. AL, ET, and MA carried out Ca2+ handling and sparks experiments with data analysis. EL evaluated the intracellular ROS. MF performed the western blot analysis. CA wrote the draft of the manuscript. LB critically revised the manuscript. GV and MR supervised the general project and wrote the manuscript. All authors read and approved the submitted version.
This work was supported by grants of the Swiss Cancer League, as well as of the Cecilia-Augusta Foundation and the San Salvatore Foundation (Lugano, Switzerland) to GV and Fondo Ricerca di Ateneo of University of Milano-Bicocca to MR.
MF was employed by company Windtree Therapeutics Inc.
The remaining authors declare that the research was conducted in the absence of any commercial or financial relationships that could be construed as a potential conflict of interest.
The Supplementary Material for this article can be found online at: https://www.frontiersin.org/articles/10.3389/fphys.2021.658790/full#supplementary-material
Advani, P. P., Ballman, K. V., Dockter, T. J., Colon-Otero, G., and Perez, E. A. (2016). Long-term cardiac safety analysis of NCCTG N9831 (Alliance) adjuvant Trastuzumab trial. J. Clin. Oncol. 34, 581–587. doi: 10.1200/JCO.2015.61.8413
Altomare, C., Bartolucci, C., Sala, L., Bernardi, J., Mostacciuolo, G., Rocchetti, M., et al. (2015). IKr impact on repolarization and its variability assessed by dynamic clamp. Circ. Arrhythm. Electrophysiol. 8, 1265–1275. doi: 10.1161/CIRCEP.114.002572
Barile, L., Cervio, E., Lionetti, V., Milano, G., Ciullo, A., Biemmi, V., et al. (2018). Cardioprotection by cardiac progenitor cell-secreted exosomes: role of pregnancy-associated plasma protein-A. Cardiovasc. Res. 114, 992–1005. doi: 10.1093/cvr/cvy055
Belmonte, F., Das, S., Sysa-Shah, P., Sivakumaran, V., Stanley, B., Guo, X., et al. (2015). ErbB2 overexpression upregulates antioxidant enzymes, reduces basal levels of reactive oxygen species, and protects against doxorubicin cardiotoxicity. Am. J. Physiol. Heart Circ. Physiol. 309, H1271–H1280. doi: 10.1152/ajpheart.00517.2014
Bokenes, J., Aronsen, J. M., Birkeland, J. A., Henriksen, U. L., Louch, W. E., Sjaastad, I., et al. (2008). Slow contractions characterize failing rat hearts. Basic Res. Cardiol. 103, 328–344. doi: 10.1007/s00395-008-0719-y
Cappetta, D., De Angelis, A., Sapio, L., Prezioso, L., Illiano, M., Quaini, F., et al. (2017a). Oxidative stress and cellular response to doxorubicin: a common factor in the complex milieu of Anthracycline Cardiotoxicity. Oxid. Med. Cell. Longev. 2017:1521020. doi: 10.1155/2017/1521020
Cappetta, D., Esposito, G., Coppini, R., Piegari, E., Russo, R., Ciuffreda, L. P., et al. (2017b). Effects of ranolazine in a model of doxorubicin-induced left ventricle diastolic dysfunction. Br. J. Pharmacol. 174, 3696–3712. doi: 10.1111/bph.13791
Correia Pinto, J., Henriques-Coelho, T., Roncon-Albuquerque, R. Jr., and Leite-Moreira, A. F. (2006). Differential right and left ventricular diastolic tolerance to acute afterload and NCX gene expression in Wistar rats. Physiol. Res. 55, 513–526.
Crocini, C., Coppini, R., Ferrantini, C., Yan, P., Loew, L. M., Tesi, C., et al. (2014). Defects in T-tubular electrical activity underlie local alterations of calcium release in heart failure. Proc. Natl. Acad. Sci. U.S.A. 111, 15196–15201. doi: 10.1073/pnas.1411557111
Crocini, C., Ferrantini, C., Coppini, R., and Sacconi, L. (2017). Electrical defects of the transverse-axial tubular system in cardiac diseases. J. Physiol. 595, 3815–3822. doi: 10.1113/JP273042
Crossman, D. J., Young, A. A., Ruygrok, P. N., Nason, G. P., Baddelely, D., Soeller, C., et al. (2015). T-tubule disease: relationship between t-tubule organization and regional contractile performance in human dilated cardiomyopathy. J. Mol. Cell Cardiol. 84, 170–178. doi: 10.1016/j.yjmcc.2015.04.022
Dubey, R., Lebensohn, A. M., Bahrami-Nejad, Z., Marceau, C., Champion, M., Gevaert, O., et al. (2016). Chromatin-remodeling complex SWI/SNF controls multidrug resistance by transcriptionally regulating the drug efflux pump ABCB1. Cancer Res. 76, 5810–5821. doi: 10.1158/0008-5472.CAN-16-0716
Ducroq, J., Moha ou Maati, H., Guilbot, S., Dilly, S., Laemmel, E., Pons-Himbert, C., et al. (2010). Dexrazoxane protects the heart from acute doxorubicin-induced QT prolongation: a key role for I(Ks). Br. J. Pharmacol. 159, 93–101. doi: 10.1111/j.1476-5381.2009.00371.x
Ewer, M. S., and Ewer, S. M. (2015). Cardiotoxicity of anticancer treatments. Nat. Rev. Cardiol. 12, 547–558. doi: 10.1038/nrcardio.2015.65
Ghigo, A., Li, M., and Hirsch, E. (2016). New signal transduction paradigms in anthracycline-induced cardiotoxicity. Biochim. Biophys. Acta 1863(7 Pt B), 1916–1925. doi: 10.1016/j.bbamcr.2016.01.021
Giordano, S. H., Lin, Y. L., Kuo, Y. F., Hortobagyi, G. N., and Goodwin, J. S. (2012). Decline in the use of anthracyclines for breast cancer. J. Clin. Oncol. 30, 2232–2239. doi: 10.1200/JCO.2011.40.1273
Heijman, J., Zaza, A., Johnson, D. M., Rudy, Y., Peeters, R. L., Volders, P. G., et al. (2013). Determinants of beat-to-beat variability of repolarization duration in the canine ventricular myocyte: a computational analysis. PLoS Comput. Biol. 9:e1003202. doi: 10.1371/journal.pcbi.1003202
Heinzel, F. R., Bito, V., Biesmans, L., Wu, M., Detre, E., von Wegner, F., et al. (2008). Remodeling of T-tubules and reduced synchrony of Ca2+ release in myocytes from chronically ischemic myocardium. Circ. Res. 102, 338–346. doi: 10.1161/CIRCRESAHA.107.160085
Hollingworth, S., Peet, J., Chandler, W. K., and Baylor, S. M. (2001). Calcium sparks in intact skeletal muscle fibers of the frog. J. Gen. Physiol. 118, 653–678. doi: 10.1085/jgp.118.6.653
Hsu, W. T., Huang, C. Y., Yen, C. Y. T., Cheng, A. L., and Hsieh, P. C. H. (2018). The HER2 inhibitor lapatinib potentiates doxorubicin-induced cardiotoxicity through iNOS signaling. Theranostics 8, 3176–3188. doi: 10.7150/thno.23207
Johnson, D. M., Heijman, J., Pollard, C. E., Valentin, J. P., Crijns, H. J., Abi-Gerges, N., et al. (2010). I(Ks) restricts excessive beat-to-beat variability of repolarization during beta-adrenergic receptor stimulation. J. Mol. Cell Cardiol. 48, 122–130. doi: 10.1016/j.yjmcc.2009.08.033
Keung, E. C., Toll, L., Ellis, M., and Jensen, R. A. (1991). L-type cardiac calcium channels in doxorubicin cardiomyopathy in rats morphological, biochemical, and functional correlations. J. Clin. Invest. 87, 2108–2113. doi: 10.1172/JCI115241
Kitani, T., Ong, S. G., Lam, C. K., Rhee, J. W., Zhang, J. Z., Oikonomopoulos, A., et al. (2019). Human-induced pluripotent stem cell model of trastuzumab-induced cardiac dysfunction in patients with breast cancer. Circulation 139, 2451–2465. doi: 10.1161/CIRCULATIONAHA.118.037357
Kurokawa, Y. K., Shang, M. R., Yin, R. T., and George, S. C. (2018). Modeling trastuzumab-related cardiotoxicity in vitro using human stem cell-derived cardiomyocytes. Toxicol. Lett. 285, 74–80. doi: 10.1016/j.toxlet.2018.01.001
Llach, A., Mazevet, M., Mateo, P., Villejouvert, O., Ridoux, A., Rucker-Martin, C., et al. (2019). Progression of excitation-contraction coupling defects in doxorubicin cardiotoxicity. J. Mol. Cell Cardiol. 126, 129–139. doi: 10.1016/j.yjmcc.2018.11.019
Louch, W. E., Bito, V., Heinzel, F. R., Macianskiene, R., Vanhaecke, J., Flameng, W., et al. (2004). Reduced synchrony of Ca2+ release with loss of T-tubules-a comparison to Ca2+ release in human failing cardiomyocytes. Cardiovasc. Res. 62, 63–73. doi: 10.1016/j.cardiores.2003.12.031
Louch, W. E., Hake, J., Mork, H. K., Hougen, K., Skrbic, B., Ursu, D., et al. (2013). Slow Ca(2)(+) sparks de-synchronize Ca(2)(+) release in failing cardiomyocytes: evidence for altered configuration of Ca(2)(+) release units? J. Mol. Cell Cardiol. 58, 41–52. doi: 10.1016/j.yjmcc.2013.01.014
Lyon, A. R., MacLeod, K. T., Zhang, Y., Garcia, E., Kanda, G. K., Lab, M. J., et al. (2009). Loss of T-tubules and other changes to surface topography in ventricular myocytes from failing human and rat heart. Proc. Natl. Acad. Sci. U.S.A. 106, 6854–6859. doi: 10.1073/pnas.0809777106
Manfra, O., Frisk, M., and Louch, W. E. (2017). Regulation of Cardiomyocyte T-tubular structure: opportunities for therapy. Curr. Heart Fail. Rep. 14, 167–178. doi: 10.1007/s11897-017-0329-9
Mazur, M., Wang, F., Hodge, D. O., Siontis, B. L., Beinborn, D. S., Villarraga, H. R., et al. (2017). Burden of cardiac arrhythmias in patients with anthracycline-related cardiomyopathy. JACC Clin. Electrophysiol. 3, 139–150. doi: 10.1016/j.jacep.2016.08.009
Milano, G., Raucci, A., Scopece, A., Daniele, R., Guerrini, U., Sironi, L., et al. (2014). Doxorubicin and trastuzumab regimen induces biventricular failure in mice. J. Am. Soc. Echocardiogr. 27, 568–579. doi: 10.1016/j.echo.2014.01.014
Mor-Avi, V., Lang, R. M., Badano, L. P., Belohlavek, M., Cardim, N. M., Derumeaux, G., et al. (2011). Current and evolving echocardiographic techniques for the quantitative evaluation of cardiac mechanics: ASE/EAE consensus statement on methodology and indications endorsed by the Japanese Society of Echocardiography. J. Am. Soc. Echocardiogr. 24, 277–313. doi: 10.1016/j.echo.2011.01.015
Mork, H. K., Sjaastad, I., Sejersted, O. M., and Louch, W. E. (2009). Slowing of cardiomyocyte Ca2+ release and contraction during heart failure progression in postinfarction mice. Am. J. Physiol. Heart Circ. Physiol. 296, H1069–H1079. doi: 10.1152/ajpheart.01009.2008
Muraru, D., Cucchini, U., Mihaila, S., Miglioranza, M. H., Aruta, P., Cavalli, G., et al. (2014). Left ventricular myocardial strain by three-dimensional speckle-tracking echocardiography in healthy subjects: reference values and analysis of their physiologic and technical determinants. J. Am. Soc. Echocardiogr. 27, 858–871.e1. doi: 10.1016/j.echo.2014.05.010
Ozcelik, C., Erdmann, B., Pilz, B., Wettschureck, N., Britsch, S., Hubner, N., et al. (2002). Conditional mutation of the ErbB2 (HER2) receptor in cardiomyocytes leads to dilated cardiomyopathy. Proc. Natl. Acad. Sci. U.S.A. 99, 8880–8885. doi: 10.1073/pnas.122249299
Picht, E., Zima, A. V., Blatter, L. A., and Bers, D. M. (2007). SparkMaster: automated calcium spark analysis with ImageJ. Am. J. Physiol. Cell Physiol. 293, C1073–C1081. doi: 10.1152/ajpcell.00586.2006
Piotrowski, G., Gawor, R., Slomka, R., Banasiak, M., Strzelecki, P., Gawor, Z., et al. (2012). [Cardioverter-defibrillator in the treatment of arrhythmia induced by trastuzumab used in the adjuvant setting in a patient with positive human epidermal growth factor receptor type-2 breast cancer]. Kardiol. Pol. 70, 756–757.
Rocchetti, M., Sala, L., Rizzetto, R., Staszewsky, L. I., Alemanni, M., Zambelli, V., et al. (2014). Ranolazine prevents INaL enhancement and blunts myocardial remodelling in a model of pulmonary hypertension. Cardiovasc. Res. 104, 37–48. doi: 10.1093/cvr/cvu188
Romond, E. H., Perez, E. A., Bryant, J., Suman, V. J., Geyer, C. E. Jr., Davidson, N. E., et al. (2005). Trastuzumab plus adjuvant chemotherapy for operable HER2-positive breast cancer. N. Engl. J. Med. 353, 1673–1684. doi: 10.1056/NEJMoa052122
Sacconi, L., Ferrantini, C., Lotti, J., Coppini, R., Yan, P., Loew, L. M., et al. (2012). Action potential propagation in transverse-axial tubular system is impaired in heart failure. Proc. Natl. Acad. Sci. U.S.A. 109, 5815–5819. doi: 10.1073/pnas.1120188109
Sag, C. M., Kohler, A. C., Anderson, M. E., Backs, J., and Maier, L. S. (2011). CaMKII-dependent SR Ca leak contributes to doxorubicin-induced impaired Ca handling in isolated cardiac myocytes. J. Mol. Cell Cardiol. 51, 749–759. doi: 10.1016/j.yjmcc.2011.07.016
Santoro, C., Arpino, G., Esposito, R., Lembo, M., Paciolla, I., Cardalesi, C., et al. (2017). 2D and 3D strain for detection of subclinical anthracycline cardiotoxicity in breast cancer patients: a balance with feasibility. Eur. Heart J. Cardiovasc. Imaging 18, 930–936. doi: 10.1093/ehjci/jex033
Sathish, V., Xu, A., Karmazyn, M., Sims, S. M., and Narayanan, N. (2006). Mechanistic basis of differences in Ca2+ -handling properties of sarcoplasmic reticulum in right and left ventricles of normal rat myocardium. Am. J. Physiol. Heart Circ. Physiol. 291, H88–H96. doi: 10.1152/ajpheart.01372.2005
Sawaya, H., Sebag, I. A., Plana, J. C., Januzzi, J. L., Ky, B., Tan, T. C., et al. (2012). Assessment of echocardiography and biomarkers for the extended prediction of cardiotoxicity in patients treated with anthracyclines, taxanes, and trastuzumab. Circ. Cardiovasc. Imaging 5, 596–603. doi: 10.1161/CIRCIMAGING.112.973321
Sawyer, D. B., Zuppinger, C., Miller, T. A., Eppenberger, H. M., and Suter, T. M. (2002). Modulation of anthracycline-induced myofibrillar disarray in rat ventricular myocytes by neuregulin-1beta and anti-erbB2: potential mechanism for trastuzumab-induced cardiotoxicity. Circulation 105, 1551–1554. doi: 10.1161/01.cir.0000013839.41224.1c
Schneider, C., Wallner, M., Kolesnik, E., Herbst, V., Machler, H., Pichler, M., et al. (2018). The anti-cancer multikinase inhibitor sorafenib impairs cardiac contractility by reducing phospholamban phosphorylation and sarcoplasmic calcium transients. Sci. Rep. 8:5295. doi: 10.1038/s41598-018-23630-w
Steinberg, J. S., Cohen, A. J., Wasserman, A. G., Cohen, P., and Ross, A. M. (1987). Acute arrhythmogenicity of doxorubicin administration. Cancer 60, 1213–1218. doi: 10.1002/1097-0142(19870915)60:6<1213::aid-cncr2820600609<3.0.co;2-v
Thavendiranathan, P., Grant, A. D., Negishi, T., Plana, J. C., Popovic, Z. B., and Marwick, T. H. (2013). Reproducibility of echocardiographic techniques for sequential assessment of left ventricular ejection fraction and volumes: application to patients undergoing cancer chemotherapy. J. Am. Coll. Cardiol. 61, 77–84. doi: 10.1016/j.jacc.2012.09.035
Tocchetti, C. G., Ragone, G., Coppola, C., Rea, D., Piscopo, G., Scala, S., et al. (2012). Detection, monitoring, and management of trastuzumab-induced left ventricular dysfunction: an actual challenge. Eur. J. Heart Fail. 14, 130–137. doi: 10.1093/eurjhf/hfr165
Keywords: doxorubicin, trastuzumab, cardiotoxicity, T-tubules, electrophysiology, calcium handling
Citation: Altomare C, Lodrini AM, Milano G, Biemmi V, Lazzarini E, Bolis S, Pernigoni N, Torre E, Arici M, Ferrandi M, Barile L, Rocchetti M and Vassalli G (2021) Structural and Electrophysiological Changes in a Model of Cardiotoxicity Induced by Anthracycline Combined With Trastuzumab. Front. Physiol. 12:658790. doi: 10.3389/fphys.2021.658790
Received: 28 January 2021; Accepted: 16 March 2021;
Published: 07 April 2021.
Edited by:
Victor A. Maltsev, National Institute on Aging (NIH), United StatesReviewed by:
Dmitry Terentyev, Brown University, United StatesCopyright © 2021 Altomare, Lodrini, Milano, Biemmi, Lazzarini, Bolis, Pernigoni, Torre, Arici, Ferrandi, Barile, Rocchetti and Vassalli. This is an open-access article distributed under the terms of the Creative Commons Attribution License (CC BY). The use, distribution or reproduction in other forums is permitted, provided the original author(s) and the copyright owner(s) are credited and that the original publication in this journal is cited, in accordance with accepted academic practice. No use, distribution or reproduction is permitted which does not comply with these terms.
*Correspondence: Giuseppe Vassalli, Z2l1c2VwcGUudmFzc2FsbGlAY2FyZGlvY2VudHJvLm9yZw==; Claudia Altomare, Y2xhdWRpYS5hbHRvbWFyZUBjYXJkaW9jZW50cm8ub3Jn
†These authors have contributed equally to this work and share senior authorship
Disclaimer: All claims expressed in this article are solely those of the authors and do not necessarily represent those of their affiliated organizations, or those of the publisher, the editors and the reviewers. Any product that may be evaluated in this article or claim that may be made by its manufacturer is not guaranteed or endorsed by the publisher.
Research integrity at Frontiers
Learn more about the work of our research integrity team to safeguard the quality of each article we publish.