- 1Department of Sport Science, Faculty of Humanities, Otto von Guericke University, Magdeburg, Germany
- 2Department of Occupational Medicine, Faculty of Medicine, Otto von Guericke University, Magdeburg, Germany
Introduction: Heart rate variability (HRV), the beat-to-beat variation of adjacent heartbeats, is an indicator of the function of the autonomic nervous system (ANS). Increased HRV reflects well-functioning of autonomic control mechanism and cardiovascular health. The aim of this systematic review is to provide a systematic overview of the effects of different physical training modalities on resting HRV and cardiovascular health and risk factors (i.e., baroreflex sensitivity, body fat, body mass, body mass index, blood pressure, heart rate recovery, VO2 max, and VO2 peak) in young and middle-aged (mean age of the studies samples up to 44 years), healthy adults.
Methods: A systematic review in accordance with the PRISMA guidelines was performed. Studies investigating the effects of different physical interventions (endurance, resistance, high-intensity, coordinative, or multimodal training) on HRV were included. Trials were considered eligible if the intervention lasted for at least 4 weeks and participants were regarded as general healthy. Five electronic databases were searched from 2005 to September 8th, 2020. The methodological quality of eligible studies was assessed by two study quality and reporting assessment scales (TESTEX and STARDHRV). PROSPERO registration number: CRD42020206606.
Results: Of 3,991 retrieved records, 26 were considered eligible and analyzed. Twelve studies used an endurance training (of which three included high-intensity sessions), six studies resistance training, four studies coordinative training, two studies high-intensity training, and two studies used a multimodal intervention. Overall, the results showed for all types of intervention an improvement in linear and non-linear HRV parameters and cardiovascular health and risk factors. However, quality assessment revealed some methodological and reporting deficits.
Conclusion: This systematic review highlights the benefits of different types of physical training interventions on autonomic function and health parameters in young and middle-aged, healthy adults. In conclusion, higher training intensities and frequencies are more likely to improve HRV. For future studies, we recommend adhering to the criteria of methodological standards of exercise interventions and HRV measurements and encourage the use of non-linear HRV parameters.
Introduction
Cardiac autonomic control describes the regulation of heart function by the sympathetic and parasympathetic branches of the autonomic nervous system (ANS) (Gordan et al., 2015). Fluctuations in the time intervals between consecutive heart beats are characterized as heart rate variability (HRV) and regarded as an indicator of the functional state of the ANS and the adaptability to internal or external stimuli. These beat-to-beat variations of successive NN intervals are the result of complex interactions between sympathetic and parasympathetic influences on the heart rate (McCraty and Shaffer, 2015; Draghici and Taylor, 2016). Release of norepinephrine triggers sympathetic activation, leading to an increase of heart rate and contractility, an enhancement of conductivity for electrical signals in the heart facilitating physical and mental demands. At rest, however, the parasympathetic activity dominates through release of acetylcholine, slowing down heart rate, reducing its contractility, and inhibiting conduction velocity (Shaffer et al., 2014; Gordan et al., 2015). Parasympathetic activity is important for restorative processes and is the main contributor of fluctuations in heart rate (Thayer et al., 2010). While the parasympathetic activation occurs immediately and has only a short-term effect, sympathetic activation has a time delay of a few seconds, though exerts longer lasting effects (Pumprla et al., 2002; Shaffer et al., 2014). The sympathetic and parasympathetic nervous systems do not work exactly in opposite directions. Instead, they can be active simultaneously, e.g., when parasympathetic activity increases, sympathetic activity does not automatically decrease (Billman, 2013; Shaffer et al., 2014; Ernst, 2017).
Reduced HRV indicates relatively high sympathetic activation, disturbed regulation of the ANS, and inadequate adaptation of the cardiovascular system. It is also a sign of chronic stress, depletion of energy reserves, and autonomic imbalance (Shaffer et al., 2014). Relatively high HRV reflects an adaptive organism, optimal energy reserves, and a good state of autonomic control mechanisms (Thayer et al., 2010; Shaffer et al., 2014; Draghici and Taylor, 2016; Ernst, 2017). Thus, HRV is used as a non-invasive marker to help assessing the risk of sudden death, cardiovascular events, or subsequent mortality in post-infarct patients (Kleiger et al., 1987; Malik et al., 1989; Cripps et al., 1991; Tsuji et al., 1994; DeGiorgio et al., 2010), assessing the degree of diabetic autonomic neuropathy (Voss et al., 2015; Villafaina et al., 2017), as a monitoring tool in athletic training, and to prevent the state of overtraining (Plews et al., 2012; Buchheit et al., 2013; Makivić et al., 2013; Botek et al., 2014).
Regular physical activity reduces risk of cardiovascular diseases and premature mortality, and positively affects cardiovascular health and the functional state of the ANS (Sandercock et al., 2005; Hottenrott et al., 2006; Routledge et al., 2010). Especially aerobic training has been described as an optimal form to reduce risks of cardiovascular diseases and enhancing parasympathetic activity. Numerous studies have shown an increase in HRV after aerobic exercise interventions in healthy adults (Carter et al., 2003; Iwasaki et al., 2003; Tulppo et al., 2003), in elderly (Levy et al., 1998; Schuit et al., 1999; Stein et al., 1999; Albinet et al., 2010), and in people with various diseases (Zoppini et al., 2007; Borghi-Silva et al., 2015; Quiles et al., 2019). However, the effects of other types of physical interventions on HRV is still not clear. Especially, there is no consensus about the effects of combined, multimodal training programs and coordinative training on HRV. We define multimodal training interventions as programs including at least two types of modalities, mainly a combination of aerobic and resistance training. The focus of coordinative training interventions is not the improvement of aerobic capacity but the mediation of emotions and the improvement of sport specific skills. Discrepancies in sample characteristics, training types, and parameters such as duration, frequency, or intensity, and methodological differences in HRV assessment in literature, make it difficult to elucidate the effects on HRV in healthy people. Therefore, a comprehensive overview of the effects of different physical interventions on HRV in healthy adults is required. The objective of the present review is to provide an up-to-date analysis of studies on the effects of different physical interventions on HRV in healthy adults without HRV related diseases in the age of 18–44 years. In fact, regarding the upper age limit of 44 years, the average age of the sample of studies has to be considered. The range of age was chosen to focus on young and early middle-aged adults as they are underrepresented in literature regarding prevention of cardiovascular diseases. Instead, the majority of studies on the effects of exercise training is focused on diseased and/or elderly individuals (Bouaziz et al., 2017; Villafaina et al., 2017; Pearson and Smart, 2018; Raffin et al., 2019; Belvederi Murri et al., 2020; Palma et al., 2020). Investigations with elite athletes were excluded as the primary aim of training programs in professional sports is not to improve HRV but the sport specific performance (Plews et al., 2013). In our review of different training protocols and training types on HRV we distinguish between five types of interventions: endurance (aerobic), resistance, high-intensity, coordinative, and multimodal training programs consisting of at least two types of training modalities. Secondary to HRV, cardiovascular and health risk factors such as baroreflex sensitivity (BR), heart rate recovery after a physical test (HRR), blood pressure (BP), body fat (BF), body mass (BM), body mass index (BMI), and VO2 max or VO2 peak are considered. Based on literature (Carter et al., 2003; Iwasaki et al., 2003; Tulppo et al., 2003), we hypothesized exercise interventions have a positive influence on HRV and secondary outcomes. In particular, aerobic training should demonstrate a pronounced positive effect on HRV.
Methods
This systematic review provides a summary and qualitative analysis of recent studies on the effects of various exercise interventions on resting state HRV and selected cardiovascular and health risk factors in young and middle-aged healthy adults. We focused on the descriptive methodological aspects regarding intervention design and HRV recording, processing, as well as analysis. The Preferred Reporting Items for Systematic Reviews and Meta-Analysis (PRISMA) (Moher et al., 2009) was employed to carry out this review. Further, methodological and reporting quality of the studies were analyzed via two quality assessment tools (TESTEX and STARDHRV).
Data Sources and Search Strategy
The electronic databases PubMed, Scopus (Elsevir), SPORTDiscus, Ovid, and Cochrane Library were searched for studies from January 1st, 2005, to September 8th, 2020, using the following terms: (resistance training OR resistance exercise OR strength training OR strength exercise OR aerobic training OR aerobic exercise OR physical training OR physical exercise OR multimodal training OR multimodal exercise OR coordinative training OR coordinative exercise) AND (heart rate variability OR HRV OR cardiac autonomic control OR autonomic function OR parasympathetic activity OR parasympathetic nervous system OR cardiac vagal tone OR autonomic cardiac modulation OR vagus nerve OR vagal tone OR vagal activity).
Inclusion and Exclusion Criteria
The inclusion criteria for relevant studies were: (1) involving at least 10 healthy subjects aged between 18 and 44 years in average without diseases relevant for HRV analysis in the training group; (2) physical training intervention with a minimum of 4 weeks with eight training sessions; (3) randomized controlled trials, quasi-experimental trials, cross-over controlled trials, or controlled trials without randomization; (4) measurement of at least one HRV parameter at resting position before (pre) and after (post) the intervention through Holter ECG or chest belt; (5) studies with 24-h ECG measurements when a short-term recording segment at resting position was analyzed; (6) full-text in English or German language; and (7) human subjects. Exclusion criteria were: (1) studies with subjects with diagnosis of dementia, mental diseases, neurological diseases, endocrinological diseases (diabetes, thyroid gland disease), cardiac diseases, hypertension, Parkinson, or other health-related diseases; (2) measuring acute exercise effects or HRV during exercise; (3) single-case studies, Review articles, short communications, letters with insufficient information to analyze the results, guidelines, theses, dissertations, qualitative studies, scientific conference abstracts, or studies on animals; (4) 24-h ECG recording without short-term analysis at resting position; (5) HRV assessment through recording the pulse rate manually or through photoplethysmography; and (6) studies with professional athletes.
Data Collection and Analysis
Selection of Studies
Retrieved articles were transferred to the Citavi 6 reference manager (Swiss Academic Software, Wädenswil, Switzerland). Duplicates were removed. Two authors (B.G. and B.T.) independently screened titles and abstracts according to criteria for inclusion and exclusion. Thereafter, the full-text of each relevant article was obtained. The same two authors independently screened the full-text of these articles. If no full-text was available, the authors were contacted. The references of the eligible articles were screened to retrieve further articles. Disagreements were resolved through discussion with a third reviewer (I.B.).
Data Extraction
Using the PICOS approach (Liberati et al., 2009) two authors (B.G. and B.T.) independently extracted the following data: sample characteristics (sample size, age, gender), HRV protocol [method (ECG or chest belt), respiration (paced or spontaneous), position (supine, sitting, standing), sampling frequency), extracted HRV parameters, secondary outcomes (BF, BM, BMI, BP, BR, HRR, VO2 max, and VO2 peak], recording length, characteristics of intervention (type, duration of intervention, sessions per week), and control group.
Quality Assessment
The methodological quality of the studies was examined using two assessment tools. The first tool, “Tool for the Assessment of Study Quality and reporting in Exercise (TESTEX) scale,” is designed for exercise training studies (Smart et al., 2015). It assesses study quality and study reporting by using 12 parameters with a maximum score of 15 points. Higher scores represent higher methodological quality. The second tool, “Standard for Reporting Diagnostic Accuracy Studies Guidelines for Heart Rate Variability Research” (STARDHRV), is designed to assess the methodological quality of studies with the focus on HRV measurement (Dobbs et al., 2019). This tool includes 25 parameters with a maximum score of 25 points. The two authors (B.G. and B.T.) evaluated the quality of included studies independently. Disagreement was solved by discussion or mediated through a third party (I.B.). Based on our research questions and the included studies, the assessment tools were slightly modified. Tables 1, 2 describes the applied criteria for TESTEX and STARDHRV, respectively.
Data Synthesis and Analysis
For each selected study, changes in all HRV parameters and the selected cardiovascular and health risk factors from pre to post intervention were collected to compare for within-subject or between-subject changes, respectively. Increases were marked with an upward arrow, decreases with a downward arrow, and no change with a horizontal arrow. Significant changes, based on the criteria of the studies, were marked with an asterisk.
Results
Study Selection
The initial search identified 5,068 records. After removal duplicates and adding one record through other sources, 3,991 records were screened. Of these, 3,895 were excluded based on title and abstract. The remaining 96 records were assessed for eligibility by full-text assessment of which 26 studies fulfilled the inclusion criteria and thus included in this review. The selection process is shown in the PRISMA flow diagram in Figure 1.
Study Characteristics
Study characteristics of the eligible studies are summarized in Table 3. A detailed description of variables is available in the electronic Supplementary Table 1.
The selected 26 studies comprised a total of 843 participants (312 women; 531 men) with 569 in exercising groups and 274 in control groups. Sample sizes ranged from 10 (Gamelin et al., 2007) to 73 subjects (Brown et al., 2014). Eight studies did not include a control group (Gamelin et al., 2007; Heffernan et al., 2007, 2009; Martinmaki et al., 2008; Buchheit et al., 2010; McHugh et al., 2012; Pal et al., 2014; Nuuttila et al., 2017). The mean age of the collated sample was 27.37 ± 7.05 years and ranged between 19.2 (Duarte et al., 2015) and 42 years (Brown et al., 2014). The mean age of the exercising groups was 26.86 ± 6.81 years and ranged from 19.2 (Duarte et al., 2015) to 42.8 years (Brown et al., 2014) whereas the mean age of the control groups was 25.80 ± 6.58 years and ranged from 19 (Duarte et al., 2015) to 40.2 years (Brown et al., 2014). In one trial, only the age range was reported (Pal et al., 2014). Two trials did not differentiate the ages between training and control group (Heydari et al., 2013; Schumann et al., 2017). Six trials included only women (Wu et al., 2011; Badrov et al., 2013; Boutcher et al., 2013; Chu et al., 2015; de Rezende Barbosa et al., 2016; da Silva et al., 2019), 14 trials included only men (Gamelin et al., 2007; Heffernan et al., 2007, 2009; Martinmaki et al., 2008; Bond et al., 2009; Buchheit et al., 2010; Heydari et al., 2013; Pal et al., 2014; Duarte et al., 2015; Kim et al., 2017; Moreira et al., 2017; Nuuttila et al., 2017; Schumann et al., 2017; Sousa Fortes et al., 2018), and six trials included both genders (Cooke and Carter, 2005; Kiviniemi et al., 2010; McHugh et al., 2012; Cheema et al., 2013; Brown et al., 2014; Varela-Sanz et al., 2017). Based on our inclusion criteria, all studies involved healthy participants. Three studies also included a few overweight subjects (Boutcher et al., 2013; Heydari et al., 2013; Brown et al., 2014). In one study, two participants had asthma and one had mild scoliosis (Chu et al., 2015), and another study included only smokers (Kim et al., 2017). Four studies assessed moderately trained (Buchheit et al., 2010), moderately active (Kiviniemi et al., 2010), or recreationally trained subjects (Nuuttila et al., 2017; Schumann et al., 2017).
Half of the trials used an ECG with 1–12 leads and the other half used chest belts to record the NN intervals. The most frequently used recording position was a supine position (19 of 26 studies). Four of these trials also measured ECG at standing position (Kiviniemi et al., 2010; Schumann et al., 2017; Varela-Sanz et al., 2017) and one trial in supine, sitting, and standing position (Martinmaki et al., 2008). In four trials, HRV was recorded in sitting position (Wu et al., 2011; Brown et al., 2014; Kim et al., 2017; Moreira et al., 2017), and in one trial in standing position (da Silva et al., 2019). Two trials did not provide any information about position (Bond et al., 2009; Sousa Fortes et al., 2018).
Spontaneous breathing protocols were used in 10 studies. One study used a paced breathing with six breaths/min (Bond et al., 2009), four studies with twelve breaths/min (Gamelin et al., 2007; Heffernan et al., 2007, 2009; McHugh et al., 2012), and two studies with 15 breaths/min (Cooke and Carter, 2005; Kim et al., 2017). Nine studies did not report their breathing protocol (Wu et al., 2011; Brown et al., 2014; Pal et al., 2014; Chu et al., 2015; Moreira et al., 2017; Nuuttila et al., 2017; Schumann et al., 2017; Varela-Sanz et al., 2017; Sousa Fortes et al., 2018).
Thirteen Studies provided no information about ECG signals sampling frequency. Ten studies (Gamelin et al., 2007; Heffernan et al., 2007, 2009; Martinmaki et al., 2008; McHugh et al., 2012; Badrov et al., 2013; Heydari et al., 2013; Chu et al., 2015; Duarte et al., 2015; Sousa Fortes et al., 2018) recorded ECG with an ideal sampling rate (Sammito and Böckelmann, 2015) of at least 1.000 Hz. One study recorded at 256 Hz (Kim et al., 2017). The recording length was reported in all except one study (Varela-Sanz et al., 2017) and varied between 2 min (Schumann et al., 2017) and 4 h during a nocturnal measurement (Nuuttila et al., 2017). Two studies did not analyze using a fixed time range. Instead, they analyzed at least 200 NN intervals (Heffernan et al., 2007) or an epoch between 220 and 260 s (Heffernan et al., 2009) depending on the number of valid NN intervals. According to recent recommendations, duration of recording should last for at least 5 min to ensure comparability of results across studies and capture the lower frequency waves (Malik, 1996; Laborde et al., 2017).
All studies evaluated cardiac autonomic function using various combinations of time-domain, frequency-domain, or non-linear HRV parameters. Time-domain parameters were assessed in 19 studies, frequency-domain in 20 studies, and non-linear parameters in eight studies. Resting heart rate was assessed in 17 studies, while five studies reported the mean NN interval instead of heart rate (Cooke and Carter, 2005; Gamelin et al., 2007; Heffernan et al., 2007, 2009; de Rezende Barbosa et al., 2016), with two studies reported both (Pal et al., 2014; Moreira et al., 2017). RMSSD or its natural logarithm was the most frequently used time-domain parameter (Gamelin et al., 2007; Heffernan et al., 2009; Buchheit et al., 2010; Badrov et al., 2013; Cheema et al., 2013; Heydari et al., 2013; Duarte et al., 2015; Kim et al., 2017; Moreira et al., 2017; Nuuttila et al., 2017; Schumann et al., 2017; da Silva et al., 2019). HF (ms2, nu, or ln) was used in all studies that reported frequency-domain parameters. Regarding non-linear parameters, SampEn (Heffernan et al., 2007, 2009; Badrov et al., 2013), α1 (Badrov et al., 2013), SD1 (Gamelin et al., 2007; Kiviniemi et al., 2010; de Rezende Barbosa et al., 2016; Kim et al., 2017; Moreira et al., 2017), SD2 (Gamelin et al., 2007; de Rezende Barbosa et al., 2016), LZEn (Heffernan et al., 2007), and SD1/SD2 (de Rezende Barbosa et al., 2016; Kim et al., 2017) were reported.
Regarding cardiovascular and health risk factors, we decided to consider only factors, reported in at least three articles: baroreflex sensitivity (BR), blood pressure (BP), body fat (BF), body mass (BM), body mass index (BMI), heart rate recovery (HRR), and VO2 max or VO2 peak. Four studies did not assess the effects on any health parameter (Cheema et al., 2013; Chu et al., 2015; de Rezende Barbosa et al., 2016; Sousa Fortes et al., 2018). VO2 max or VO2 peak were the most commonly used factors (13 of 22 studies) (Gamelin et al., 2007; Heffernan et al., 2007, 2009; Martinmaki et al., 2008; Bond et al., 2009; Kiviniemi et al., 2010; McHugh et al., 2012; Boutcher et al., 2013; Heydari et al., 2013; Kim et al., 2017; Nuuttila et al., 2017; Schumann et al., 2017; Varela-Sanz et al., 2017), while only three studies assessed BR (Cooke and Carter, 2005; Heffernan et al., 2009; Heydari et al., 2013).
The majority of trials utilized endurance training interventions (11 of 26 studies) (Gamelin et al., 2007; Martinmaki et al., 2008; Bond et al., 2009; Buchheit et al., 2010; Kiviniemi et al., 2010; Wu et al., 2011; McHugh et al., 2012; Duarte et al., 2015; Kim et al., 2017; Schumann et al., 2017; da Silva et al., 2019). Brown et al. (2014) was also included as endurance training intervention since the effects of a walking program for employees were investigated. Six trials used a form of resistance training (Cooke and Carter, 2005; Heffernan et al., 2007, 2009; Badrov et al., 2013; de Rezende Barbosa et al., 2016; Sousa Fortes et al., 2018), three trials used high intensity training (HIT) (Boutcher et al., 2013; Heydari et al., 2013; Nuuttila et al., 2017), three further trials included HIT sessions (running intervals) in their endurance training programs (Gamelin et al., 2007; Buchheit et al., 2013; da Silva et al., 2019). Interestingly, Kim et al. (2017) used a training group running at moderate (60% of heart rate reserve) and one group running at high intensity (75% of heart rate reserve). Non-standard interventions were also used in a number of studies. These included Yoga (Cheema et al., 2013; Pal et al., 2014; Chu et al., 2015) and Capoeira, a Brazilian martial art/dance (Moreira et al., 2017). Finally, two studies combined endurance and resistance training (Moreira et al., 2017; Nuuttila et al., 2017; Varela-Sanz et al., 2017). Interventions duration ranged from six (Heffernan et al., 2007, 2009; Wu et al., 2011) to 14 weeks (Martinmaki et al., 2008). One (Moreira et al., 2017) to six sessions per week were performed (Pal et al., 2014; Nuuttila et al., 2017; Schumann et al., 2017). A detailed description of the interventions can be found in the Supplementary Table 1.
Outcome Heart Rate Variability
See Table 4 for the detailed outcome of all HRV measures, cardiovascular and health risk factors, TESTEX, and STARDHRV scores of the included studies. The majority of studies (16/26) reported significant positive adaptations in HRV after physical interventions (Cheema et al., 2013). Five studies showed non-significant improvements (Cooke and Carter, 2005; Martinmaki et al., 2008; Bond et al., 2009; Wu et al., 2011; McHugh et al., 2012; Badrov et al., 2013; Brown et al., 2014; Chu et al., 2015; Schumann et al., 2017). Three studies showed small deteriorations (McHugh et al., 2012; Brown et al., 2014; Chu et al., 2015). Only one study reported significant negative adaptations of HRV after the intervention compared to a control group (Cheema et al., 2013) and one study reported no clear changes in HRV (Schumann et al., 2017). Time-domain parameters improved significantly in 10 of 19 studies reporting them (Buchheit et al., 2010; Heydari et al., 2013; Pal et al., 2014; Duarte et al., 2015; de Rezende Barbosa et al., 2016; Kim et al., 2017; Moreira et al., 2017; Nuuttila et al., 2017; Sousa Fortes et al., 2018; da Silva et al., 2019), frequency-domain parameters in seven of 20 studies reporting them (Gamelin et al., 2007; Boutcher et al., 2013; Heydari et al., 2013; Pal et al., 2014; Duarte et al., 2015; Kim et al., 2017; Nuuttila et al., 2017), and non-linear parameters improved significantly in six of eight studies reporting them (Heffernan et al., 2007, 2009; Kiviniemi et al., 2010; de Rezende Barbosa et al., 2016; Kim et al., 2017; Moreira et al., 2017).
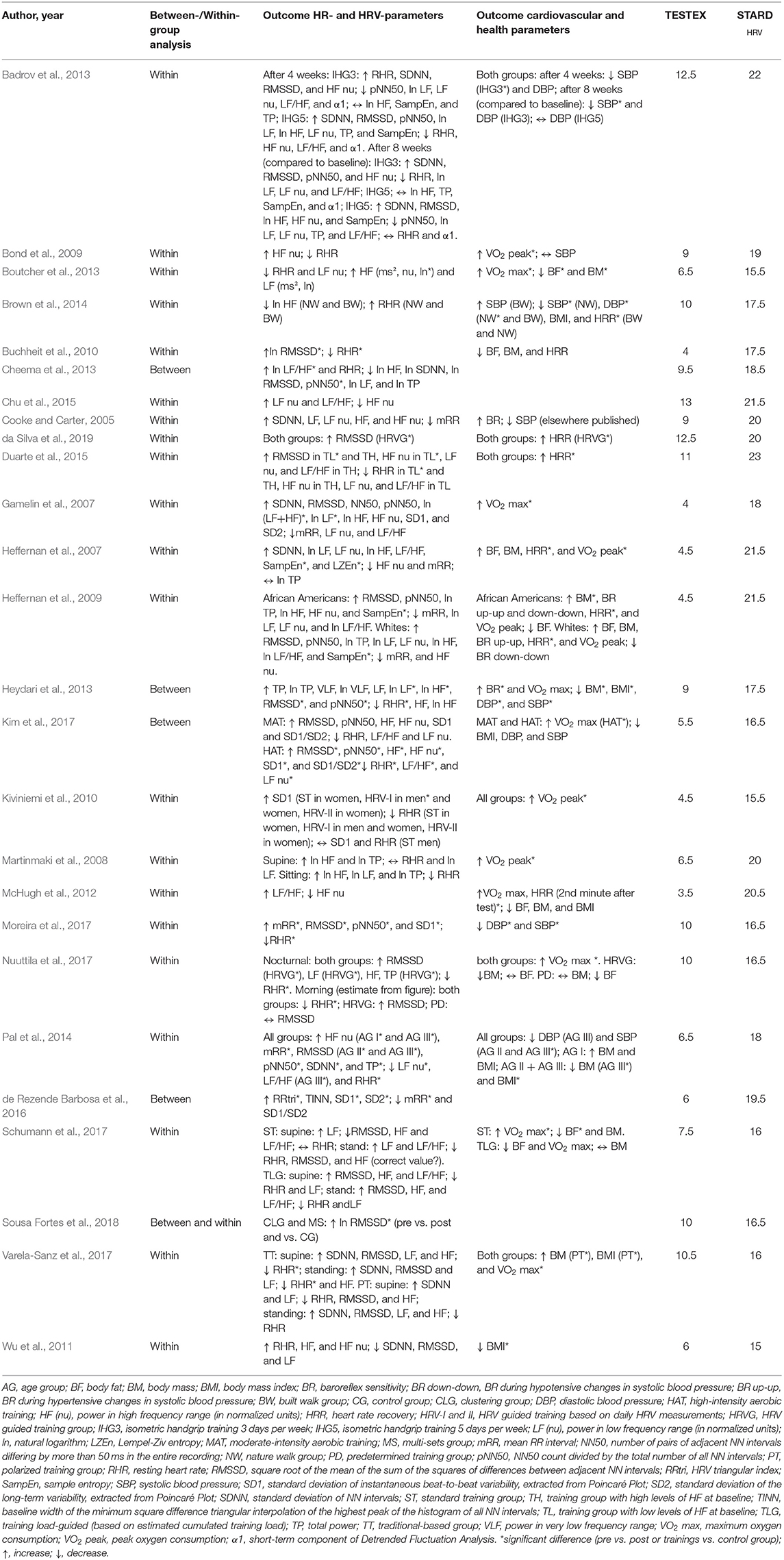
Table 4. Outcome of HRV measures, cardiovascular and health risk factors, TESTEX, and STARDHRV score.
Outcome Cardiovascular Health and Risk Factors
Significant reductions in blood pressure (systolic and/or diastolic) were detected in five studies (Cooke and Carter, 2005; Buchheit et al., 2010; Badrov et al., 2013; Cheema et al., 2013; Heydari et al., 2013; Brown et al., 2014; Pal et al., 2014; Chu et al., 2015; de Rezende Barbosa et al., 2016; Moreira et al., 2017; Sousa Fortes et al., 2018). VO2 max or peak significantly improved in 9 of 13 studies (Gamelin et al., 2007; Heffernan et al., 2007; Martinmaki et al., 2008; Bond et al., 2009; Kiviniemi et al., 2010; Boutcher et al., 2013; Kim et al., 2017; Nuuttila et al., 2017; Schumann et al., 2017). BF, BM, or BMI significantly decreased in five studies (Wu et al., 2011; Boutcher et al., 2013; Heydari et al., 2013; Pal et al., 2014; Schumann et al., 2017). Heart rate recovery was significantly improved in six trials (Heffernan et al., 2007, 2009; McHugh et al., 2012; Brown et al., 2014; Duarte et al., 2015; da Silva et al., 2019). Lastly, BR significantly improved in one study (Heydari et al., 2013). In contrast, BM increased in the investigation of Heffernan et al. (2009). BM and BMI increased in the trial of Varela-Sanz et al. (2017). Four studies did not assess any health parameter of any kind pre and post intervention (Cheema et al., 2013; Chu et al., 2015; de Rezende Barbosa et al., 2016; Sousa Fortes et al., 2018).
Quality Assessment
The TESTEX scale was used to assess the methodological quality and reporting of the selected studies (Smart et al., 2015). The 26 studies scored from 3.5 (McHugh et al., 2012) to 13 points (Chu et al., 2015) with an average of 7.9 (Table 4). The scoring of each study is detailed in the electronic Supplementary Table 2. However, it has to be mentioned that trials with only one group could not achieve more than eight points. Most studies provided adequate information regarding eligibility criteria, randomization, between-group statistics, and outcome measures. In contrast, most of the studies lacked an intention-to-treat analysis (23/26) and an activity monitoring in the control group (16/21). A subset of studies provided blinding of assessors (Bond et al., 2009; Cheema et al., 2013; Heydari et al., 2013; Chu et al., 2015). Furthermore, only seven of 26 studies achieved three points in subscale number six (“Outcome measures assessed in at least 85% of patients”) (Martinmaki et al., 2008; Badrov et al., 2013; Chu et al., 2015; Nuuttila et al., 2017; Varela-Sanz et al., 2017; Sousa Fortes et al., 2018; da Silva et al., 2019).
The HRV methodology in these studies was assessed with STARDHRV (Dobbs et al., 2019). The scoring of each study is detailed in the electronic Supplementary Table 3. The average score for all studies was 18.44/25 and ranged from 15 (Wu et al., 2011) to 23 points (Duarte et al., 2015). A number of studies failed to provide any information regarding sample size calculation (18/26) and failed to report full information about reasons for missing data, along with percentage missing (24/26), as well as artifact cleaning method and percentage of corrected beats (24/26). Information about pre-testing guidelines, hard- and software they used, stabilization period, and a description of analyzed HRV indices were provided in nearly all studies.
Discussion
Purpose and Main Findings
The aim of this systematic review was to summarize the existing literature on the effects of different physical training interventions on HRV in healthy adults. In the current review, we focused on healthy adults aged between 18 and 44 years. The upper age limit concerns the average age of the sample. A variety of physical interventions, such as endurance (aerobic), resistance, high intensity, coordinative, and multimodal interventions were considered. The majority of the studies demonstrated a significant positive effect on HRV after training intervention. Only in one study, the experimental group (Yoga) showed significantly increased LF/HF and reduced pNN50 after the intervention compared to the control group, implying a reduced parasympathetic activity (Cheema et al., 2013). For all five training modalities, significant positive effects on HRV were reported. However, due to the unequal number of studies for each exercise modality, a clear identification of exercise modalities to improve HRV is challenging. Nonetheless, all interventions with HIIT (n = 3) and multimodal training (n = 2) showed significant improvements in HRV. Four of six studies with resistance training reported significant improvements. Two studies failed to detect any significant change in HRV after the resistance training intervention. Nine of twelve studies with endurance training showed a significant or slightly improved HRV. One study with endurance training did not present clear results and two studies showed a small decline of HRV. Finally, a significant improvement in HRV was shown in two interventions with coordinative training. However, this result was contrasted by two other studies showing an impaired HRV after the same type of modality.
In conclusion, endurance and resistance training are very appropriate exercise modalities to improve resting HRV. Regarding HIIT and multimodal exercise modalities, a definite conclusion cannot be made as supporting data is still limited. For coordinative training, the intensity levels appear to be an important factor in order to stimulate effects on autonomic function. Based on our inclusion of all HRV indices in the respective studies for this review, RMSSD is one of the most used parameters. Further, RMSSD is the most affected parameter after endurance, HIIT, and coordinative training interventions. Therefore, our results are consistent with the model of RMSSD as an indicator of vagally mediated changes in HRV based on the increase of vagal influence after exercise training (Shaffer and Ginsberg, 2017).
Cardiovascular and health risk factors were shown to be improved after the interventions in most of the reviewed studies. Two studies demonstrated an increase of BM (Heffernan et al., 2009) after a resistance intervention and an increase of BM and BMI after a combined endurance and resistance intervention (Varela-Sanz et al., 2017). A number of trials also showed clear deficits in methodological and study reporting quality.
Endurance Training
Heart Rate Variability
Twelve studies applying endurance training interventions were identified. Three of them combined aerobic sessions (continuous runs) with HIT sessions (Gamelin et al., 2007; Buchheit et al., 2010; da Silva et al., 2019). Two studies focused exclusively on women (Wu et al., 2011; da Silva et al., 2019), three studies on women and men (Kiviniemi et al., 2010; McHugh et al., 2012; Brown et al., 2014), and the remaining seven studies included only male participants. While six studies showed significant improvements in at least one HRV parameter (Gamelin et al., 2007; Buchheit et al., 2010; Kiviniemi et al., 2010; Duarte et al., 2015; Kim et al., 2017; da Silva et al., 2019), three trials a tendency of improvement (Martinmaki et al., 2008; Bond et al., 2009; Wu et al., 2011), two trials a tendency of reduced HRV in at least one parameter (McHugh et al., 2012; Brown et al., 2014), one study revealed no clear changes in both training groups from pre to post measurement (Schumann et al., 2017).
The lack of improvement in HRV in two studies could be the result of a relatively low training volume. In the study of Brown et al. (2014), employees had to walk two times per week during their lunch time for about 20 minutes. McHugh et al. (2012) applied a 2-day per week “recreational jogging program”.
Martinmaki et al. (2008) revealed significant improvements in HRV during a submaximal exercise but only very small improvements during rest after the intervention. However, a decrease in resting plasma noradrenaline concentration was found, suggesting a slight decrease of sympathetic control at rest. The unchanged vagal heart rate control could be the result of low training volume (two sessions/week) or the relatively small sample size (n = 11). No improvements in HRV at rest but during mental and physical stressors were found in the investigation of Bond et al. (2009) and explained by the lesser vagal withdrawal after the aerobic intervention. Kiviniemi et al. (2010) and da Silva et al. (2019) analyzed the effects of HRV-guided training interventions. The training load in these investigations was determined based on HRV-values. While da Silva et al. (2019) revealed stronger improvements in the HRV-guided group compared to the control group, this effect was only significant in male subjects in the study of Kiviniemi et al. (2010). In contrast, women also could improve their HRV levels. These contrasting results could be explained by the applied sample characteristics. The latter study involved moderately trained subjects with an already good autonomic control, where the former study involved untrained subjects who may have benefitted already from low training loads. Since VO2 peak (Kiviniemi et al., 2010) and time to complete running 5 km improved (da Silva et al., 2019) after a HRV-guided training, it is still sensible to monitor autonomic functions by measuring HRV regularly and adapt training load based on the HRV results. In this context, Buchheit et al. (2010) showed an improvement in HRV along with a reduction in time to complete running 10 km. In this study, participants measured their HRV daily. No changes in HRV were detected for the first 4 weeks, showing that 4 weeks are insufficient to improve autonomic function. Hottenrott et al. (2006) recommended at least 3 months of aerobic training to induce modifications in vagal activity. Indeed, 3 months of aerobic training were applied in the studies of Duarte et al. (2015) and Gamelin et al. (2007). Heart rate variability improved significantly in subjects with lower resting vagal activity at baseline but not in subjects with an already high vagal activity (Duarte et al., 2015), confirming the results of Kiviniemi et al. (2010). This implies that autonomic adaptation not only relies on the training volume and intensity but also on the initial autonomic activity of the subject. A significant increase in total power and LF nu in combination with only a moderate increase in HF suggests a quantitative adaptation of autonomic control rather than a qualitative modification of sympathovagal balance (Gamelin et al., 2007). However, the small sample size (n = 10) is one limitation of this trial.
In summary, endurance training is appropriate to increase HRV. Higher intensities (Kiviniemi et al., 2010; Kim et al., 2017) and a combination of moderate- and high-intensity interventions (Gamelin et al., 2007; Buchheit et al., 2010; da Silva et al., 2019) seems to be appropriate to improve autonomic function. Since these interventions mainly applied running or running on a treadmill, we recommend running as an appropriate type of intervention. In contrast, low-intensity or low-volume training is not sufficient to significantly improve autonomic function (Martinmaki et al., 2008; Bond et al., 2009; Wu et al., 2011; McHugh et al., 2012; Brown et al., 2014). Compared to lower intensities, high-intensity sessions stimulate nitric oxide syntheses stronger, resulting in a stronger vagal activity (de Abreu et al., 2019). Furthermore, exercise training suppresses angiotensin II expression and thus improves cardiac vagal activity (Routledge et al., 2010). Consequently, to achieve improvements in autonomic functioning, the performance level, HRV at baseline, and HRV behavior in response to training have to be considered (Hottenrott et al., 2006; Kiviniemi et al., 2006). The role of gender in the improvement of autonomic functions needs to be further investigated, as only two studies exclusively examined female subjects. The majority of studies with male participants showed an improvement in HRV. Similarly, the study of Kiviniemi et al. (2010) showed a significant improvement in male participants but only a slight improvement in female participants. This finding could also be due to the already higher vagal activity in premenopausal women compared to men of the same age (Kuo et al., 1999).
Cardiovascular Health and Risk Factors
Cardiovascular and health risk factors could be significantly improved upon in all studies with endurance training, except in Buchheit et al. (2010). Here, BF, BM, and HRR showed only a slightly decrease. However, since moderately trained runners were participants, no effects regarding BF and BM were expected. Heart rate recovery was significantly improved in all of the four studies investigating this factor (McHugh et al., 2012; Brown et al., 2014; Duarte et al., 2015; da Silva et al., 2019). A rapid decline in heart rate after a (sub)maximal exercise could be the result of an improved vagal reactivation after an endurance intervention. Duarte et al. (2015) recorded RMSSD after a maximal treadmill test and found significant improvements, indicating that indices of vagal reactivity after exercise would be more sensible for autonomic adaptations after physical interventions than resting HRV alone.
Blood pressure was significantly lowered only in Brown et al. (2014). In addition, BF (Schumann et al., 2017) and BMI (Wu et al., 2011) were significantly reduced only in these studies whereas the other studies failed to report any significant changes. This could be due to the healthy sample pool, as in this review only relatively young subjects without severe health problems were considered. Therefore, a reduction of these risk factors, was not expected.
In contrast, VO2 max or peak were shown to be improved in six studies (Gamelin et al., 2007; Martinmaki et al., 2008; Bond et al., 2009; Kiviniemi et al., 2010; Kim et al., 2017; Schumann et al., 2017). McHugh et al. (2012) failed to show significant improvements possibly due to the low training volume (7 weeks, two sessions/week) and low intensity (recreational jogging).
In summary, a few health parameters could be improved upon in nearly all studies, though healthy and relatively young subjects (<45 years) participated in these studies. These improvements were accompanied by improvements in autonomic function and enhanced running performance (Gamelin et al., 2007; Schumann et al., 2017; da Silva et al., 2019).
Resistance Training
Heart Rate Variability
Six studies applying resistance training are included in this review. Two studies included female participants only (Badrov et al., 2013; de Rezende Barbosa et al., 2016), one study included both genders (Cooke and Carter, 2005), and the remaining three studies examined only male subjects (Heffernan et al., 2007, 2009; Sousa Fortes et al., 2018). While two studies reported no significant improvements in HRV (Cooke and Carter, 2005; Badrov et al., 2013), four studies revealed significant improvements (Heffernan et al., 2007, 2009; de Rezende Barbosa et al., 2016; Sousa Fortes et al., 2018).
Isometric handgrip training (IHG) failed to induce HRV modifications, possibly due to its localized effect on handgrip strength (Badrov et al., 2013). Heart rate variability was also unaffected after strength training intervention in the study of Cooke and Carter (2005). Critically, aerobic fitness was not assessed at baseline. Some subjects may have been aerobically fit and therefore differed in their baseline autonomic response. This, in combination to the small sample size of the training group (n = 12) may have caused to show a significant improvement on HRV.
Significant improvements in HRV were observed in non-linear parameters but not in time- or frequency-domain indices in the studies of Heffernan et al. (2007, 2009). These findings support the hypothesis of Bhati et al. (2019), that non-linear measures are more sensitive for detecting training-induced autonomic adaptations. The authors supposed an increase of autonomical mediated heart rate complexity (i.e., an increase of the unpredictability of the heart rate time series) after resistance training, reflecting increased parasympathetic and/or reduced sympathetic cardiac autonomic control (Heffernan et al., 2007). de Rezende Barbosa et al. (2016) reported significant improvements in geometric indices following a whole-body resistance training in young women compared to a control group. Sousa Fortes et al. (2018) showed a significant increase of ln RMSSD and a decrease of resting heart rate after two different methods in resistance training (clustering vs. multi-sets) during a 8-week intervention with three sessions per week.
While all three studies with male subjects found improvements in HRV (Heffernan et al., 2007, 2009; Sousa Fortes et al., 2018), the studies with both female and male subjects (Cooke and Carter, 2005) and with only female subjects showed no clear results (Badrov et al., 2013). These findings may indicate that women respond to resistance training with a less pronounced improvement in autonomic functioning compared to men. However, as the studies with endurance training already showed, the higher vagal activity of female subjects before menopause could also be a reason for a lack of training-induced improvement in HRV. Again, more extensive studies with participants comprised of young men and premenopausal women are necessary to investigate gender-specific effects of physical activity on HRV.
Adaptations in autonomic function can be caused by several possible physiological mechanism. For example, reduction in concentration of metabolites and pro-inflammatory cytokines is one mechanism (Sousa Fortes et al., 2018). The optimization of parasympathetic activation and reduction of sympathetic tone, explained by a decrease in plasma concentration of norepinephrine, is another explanation for the increase of HRV following resistance training (Sousa Fortes et al., 2018). Enhanced responsiveness of the baroreflex, vascular adjustments, and increased nitric oxide bioavailability may also cause positive adaptations (Selig et al., 2004; Macedo et al., 2016). Moreover, changes in body composition and enhanced lactate tolerance can also positively influence autonomic function (Bhati et al., 2019).
Cardiovascular Health and Risk Factors
Systolic blood pressure was significantly reduced following IHG, regardless of the number of sessions per week (Badrov et al., 2013). In contrast to earlier studies, no reductions in diastolic and mean arterial blood pressure were found. However, Badrov et al. (2013) argued that in these studies the subjects had higher initial BP-values. Isometric handgrip training may be a reasonable method in the prevention of hypertension. Heart rate recovery was improved in two studies and related to a prompt reactivation of parasympathetic activity and sympathetic withdrawal after the exercise (Heffernan et al., 2007, 2009). The authors suggested an increased acetylcholine release from the vagus, increased number of receptors, and their improved sensitivity, induced by resistance training, as possible mediating mechanisms. The small increase of VO2 peak was regarded as not physiologically relevant, although a modest improvement of aerobic capacity was possible (Heffernan et al., 2007). A significant increase in BM as reported in Heffernan et al. (2009) could be the result of a hypertrophic effect of the resistance training without detrimental health risks.
High Intensity (Interval) Training
Heart Rate Variability
HIIT on a cycle ergometer was used in two studies (Boutcher et al., 2013; Heydari et al., 2013). Both showed positive effects on vagally mediated HRV. The suggestion of that higher intensities cause an increased effect on HRV has been confirmed by other endurance interventions that included running HIT sessions (Gamelin et al., 2007; Buchheit et al., 2010; Kim et al., 2017; da Silva et al., 2019). Significant improvements were detected in short-term recordings (Gamelin et al., 2007; da Silva et al., 2019) as well as in longer recordings (Heydari et al., 2013). However, none of these studies used ECG, only chest belts for recording the NN intervals. As already mentioned previously, compared to continuous training, high intensities produce a stronger stimulus on nitric oxide syntheses, thus favoring the bioavailability of it and induce greater distensibility of the carotid artery, favoring baroreflex sensitivity (de Abreu et al., 2019). The results here also demonstrate that both women (Boutcher et al., 2013) and men (Heydari et al., 2013) can benefit from high-intensity training. However, interventions with participants of both genders would more clearly demonstrate a gender-specific adaptations to HIIT.
Cardiovascular Health and Risk Factors
VO2 max was significantly improved upon in two trials (Gamelin et al., 2007; Boutcher et al., 2013). It is supposed that HIIT increases stroke volume by enhanced cardiac contractility, enhanced mitochondrial oxidative capacity, and increased skeletal muscle diffuse capacity (Wisløff et al., 2007; Boutcher et al., 2013; Boutcher and Boutcher, 2017). HIIT is also suitable to reduce BF and BM in overweight women (Boutcher et al., 2013), as well as to reduce BP and improve BR in inactive young men (Heydari et al., 2013). These positive adaptations could be the result of an enhanced vasodilatory capacity, endothelial adaptation, or increase in endothelial NO synthase activity (Heydari et al., 2013).
Coordinative Training
Heart Rate Variability
Regarding coordinative training interventions, three studies applied Yoga (Cheema et al., 2013; Pal et al., 2014; Chu et al., 2015) and one study Capoeira (Moreira et al., 2017). While one study failed to detect any significant changes in female participants (Chu et al., 2015), HRV of male participants improved in one study (Pal et al., 2014), and decreased significantly in another study comprised of men and women (Cheema et al., 2013). In the last study, ln LF/HF increased and pNN50 decreased following 10 weeks of Yoga. The authors explained this result with a short intervention, small sample size, and low statistical power. Although the duration of intervention was shorter and the number of sessions per week even lower, no significant decline but a stable HRV was detected in Chu et al. (2015). These contradictory results are in line with a meta-analysis, showing no strong evidence for the effectiveness of yoga to improve HRV (Posadzki et al., 2015). A longer intervention and higher volume with six sessions per week might be appropriate to induce positive adaptations of the ANS (Pal et al., 2014). The authors concluded that yogic practice improves autonomic function coinciding with increased levels of serotonin and dopamine, and lowered levels of epinephrine and norepinephrine.
One session of Capoeira per week for 10 weeks was enough to increase HRV in young men (Moreira et al., 2017). These improvements may be caused by the additional benefits such as lowering psychological stress and anxiety, socialization, friendship, and relaxation promoted by the capoeira rhythm (Moreira et al., 2017). The inconsistent results could also be due to the gender of the subjects, as in both studies with positive effects on HRV, only men participated, whereas in the intervention of Chu et al. (2015) only women were examined or the majority of participants were women in Cheema et al. (2013).
To summarize, the HRV benefits from coordinative training may be the result of psychological factors, such as social contacts and the elicitation of positive emotions. These factors should be further investigated in future studies with interventions of subjects suffering from psychological problems, and programs with adequate volume and intensity, challenging the cardiovascular system. Additionally, cardiovascular effects could be elicited through the sum of acyclic movements in the coordinative programs. These aspects should be carefully considered, when planning physical interventions with untrained subjects.
Cardiovascular Health and Risk Factors
The reduction of BP after the Capoeira intervention may be due to increased parasympathetic tone and reduced sympathetic nerve activity. This is of relevance, since participants showed pre-hypertension prior to the intervention (Moreira et al., 2017). Improvement of BP was also detected after the Yoga intervention in the study of Pal et al. (2014) and was accompanied by improvements of other risk factors (levels of catecholamine, BDNF, and cholesterol).
Multimodal Training
Heart Rate Variability
Nuuttila et al. (2017) investigated the effects of an HIT consisting of endurance training with low- and high-intensities and strength training sessions in young men. Strength training was comprised of maximal and explosive exercises. Therefore, we considered this intervention as a multimodal training. In addition, the authors compared two training groups: HRVG (HRV-guided) and PD (predetermined training group). Heart rate variability was recorded at night (a 4 h block was analyzed) and every morning. The morning measurement was averaged weekly. The results showed significant improvements of the nocturnal HRV in the HRVG. The improvements in the PD were not significant. RMSSD of the morning measurements improved in HRVG and was stable in PD. These results support earlier findings of da Silva et al. (2019). In contrast, Varela-Sanz et al. (2017) could detect no significant improvements in HRV, neither during standing nor supine position in a pool of 30 men and five women. A decline in resting heart rate was the only significant result of this study. The authors argued, that training frequency and duration may have been too low to provoke changes in HRV. In addition, participants were already physically active before the intervention. Thus, higher intensities would be necessary to induce a vagal enhancement.
Cardiovascular Health and Risk Factors
VO2 max was improved following the HIT in both groups of the intervention in Nuuttila et al. (2017). This enhancement was accompanied by an improved performance in a 3,000 m running test. Therefore, HRV-guided HIT is an effective method to improve autonomic function and running performance. BM and BMI increased after the multimodal training program in the study of Varela-Sanz et al. (2017) but BF remained unchanged. This resulted in an overall improved maximum strength (Varela-Sanz et al., 2017).
Quality Assessment
TESTEX
The methodological quality of the studies ranged from 3.5 to 13, with an average score of 7.90. When trials without a between-group analysis (six studies) were excluded, the score ranged from 4.5 to 13, with an average score of 8.93. The following criteria were fulfilled by most of the studies: specification of the eligibility criteria and randomization, similar groups at baseline, and providing point estimates for all outcomes. However, allocation concealment, blinding of assessors, outcome measures (including reporting adverse events and percentage of exercises completed), intention-to-treat analysis, and activity monitoring in the control groups were fulfilled by only a small number of studies. Therefore, future studies should adhere to these methodological recommendations more closely to allow for better cross-comparison between studies.
STARDHRV
STARDHRV was devised as an assessment tool for trials measuring HRV. The score of the included studies ranged from 15 to 23, with an average score of 18.44. The first three criteria (identification as a study of validation; structured summary; and scientific and practical background) are fulfilled in all studies. In addition, a description of how estimates were calculated and baseline demographics of participants were provided in all studies. Main deficits were detected in the description of environmental conditions and in the ECG processing (reasons for missing data, along with percentage missing and how it was handled; artifact identification method; artifact cleaning method and percentage of beats corrected). For future studies, we recommend to follow the criteria as stated in TESTEX and STARDHRV to overcome methodological limitations. This will allow better comparability between studies and a standardization of HRV measurement.
Strengths and Limitations
Several limitations to this review have to be mentioned. First, not only randomized controlled trials were included to provide a broad overview of existing literature. Though, we want to emphasize that randomization is an extremely important aspect in clinical trials. Second, a meta-analysis could provide a quantitative analysis of studies and comparison of the effects on HRV between different types of interventions. However, a quantitative analysis is limited by the heterogeneity of studies, in relation to the exercise intervention protocols, and methods of HRV analysis. Therefore, we decided to refrain from a meta-analysis and conducted a qualitative analysis instead. The applied methodological assessment tools identified some deficits as none of the studies scored the maximum points for TESTEX or STARDHRV. This highlights the urgent need of complying to already existing criteria for HRV measurements and exercise training intervention studies. Third, the study sample pool was restricted to healthy adults up to the age of 44 years in average. The conclusions presented here are not generalizable to older adults or diseased samples. Fourth, although we did not limit our literature search to a single gender, only six studies were found that examined exclusively female subjects and six studies that examined female and male subjects together. Additionally, the gender distribution was not balanced in all of these studies. Lastly, only original articles in English and German (no article in German was included in the final sample) were included.
To our knowledge, this is the first systematic review dedicated to examining the effects of a broad range of physical interventions on autonomic function and selected cardiovascular and health risk factors in healthy adults. A key aspect of our review is the inclusion of different training modalities. Although, prevention of cardiovascular and health risk factors is typical in older age ranges, most of the included studies showed already an improvement of autonomic function and cardiovascular and health risk factors in relatively young and untrained adults. Our results highlight the potential of different types of physical modalities to improve autonomic function and to prevent cardiovascular diseases. As measuring HRV at rest alone is not sufficient to evaluate autonomic adaptation, we also considered investigations measuring cardiovascular and health risk factors providing a more comprehensive view of the ANS and cardiovascular system (Bhati et al., 2019).
Practical Implications
This review highlights the potential of different physical training interventions to improve HRV and health parameters (e.g., reducing blood pressure or increasing VO2 max) in young and middle-aged adults. Although aerobic training is the most common modality to improve autonomic functions (Routledge et al., 2010), resistance and high-intensity training interventions improve autonomic function as well (Bhati et al., 2019; de Abreu et al., 2019). Even coordinative training, with adequate exercise frequency and intensity, can enhance HRV (Pal et al., 2014; Moreira et al., 2017). A recent meta-regression has proved training frequency as a key factor influencing HRV positively in older adults. Due to the higher cardiovascular risk induced by higher intensities in the elderly, interventions with elderly should focus on training frequency, rather than intensity (de Abreu et al., 2019; Raffin et al., 2019). However, based on our results, we would recommend higher intensities for healthy, young people. The huge variety in methodological quality highlights the necessity to adhere to quality standards as a critical factor to minimize possible confounding factors and increase the comparability between studies (da Silva et al., 2014).
Since we were not able to formulate an optimal dose of exercise training for enhancing HRV, future studies should investigate the effects of different training doses. Currently, resting and post-exercise HRV are used to monitor the training progress (Makivić et al., 2013) and in the assessment of overreaching and to prevent overtraining (da Silva et al., 2015). Future studies could also employ HRV as an indicator of internal load to directly prescribe exercise intensity (Herold et al., 2020). Non-linear indices, such as DFA1, appear to be potential markers for diagnostics and monitoring of the training process (Gronwald et al., 2020). Therefore, we recommend to utilize not only traditional linear HRV parameters but also to analyze non-linear parameters to gain further insight into the complexity of the ANS. Due to the predominance of studies with male participants, the positive effects of any physical activity on HRV in men can be confirmed. The influence of physical activity in women needs further investigation since studies with exclusively female subjects and studies comparing male and female subjects are the minority. Therefore, this gender gap has to be closed and we encourage to investigate the influence of endurance, resistance, coordinative, multimodal, and HIT in premenopausal women. The effects of the hormonal balance and the menstrual cycle on autonomic modulation should also be considered.
Conclusion
As hypothesized, this review demonstrated the positive effectiveness of different physical training modalities on autonomic function in young and early middle-aged healthy adults, as indicated by an increase of different HRV parameters at rest. In addition to an increased HRV, selected cardiovascular, and health risk factors (baroreflex sensitivity, body fat, body mass, body mass index, blood pressure, heart rate recovery, and VO2 max and peak) improved as well. Several physiological mechanisms are involved in the adaptation of autonomic function. Aerobic training is supposed to decrease plasma noradrenaline concentration resulting in a decrease of sympathetic influence on heart rate (Kiviniemi et al., 2010; Kim et al., 2017). Furthermore, suppression of angiotensin II expression leads to an increase of the parasympathetic influence on the heart rate (Routledge et al., 2010). Additional high-intensity sessions favor nitric oxide synthesis and induce greater distensibility of the carotid artery, which improves baroreflex functional capacity (Bhati et al., 2019). Finally, the reduction of sympathetic tone by decreased plasma concentration of norepinephrine as well as the reduced concentration of metabolites (such as ammonia and urea) and pro-inflammatory cyokines (such as TNF-alpha and interleukin 2) are suggested as probable mechanisms for HRV improvement after resistance training. However, the underlying mechanisms have to be elucidated in future studies. Although we did not assess the quantitative effect of training intensity and frequency on HRV and health parameters by conducting a meta-analysis, our observations strongly suggested higher training intensities and frequencies to improve HRV. However, we recommend for future studies to adhere to the criteria of methodological standards for exercise training studies and HRV measurements and encourage to investigate the gender-related differences in the adaptation of autonomic function.
Data Availability Statement
The original contributions presented in the study are included in the article/Supplementary Material, further inquiries can be directed to the corresponding author/s.
Author Contributions
BG developed the concept for this review and designed the review process. BG and BT performed the literature search and analysis. BG wrote and edited the manuscript. BT, IB, and AH reviewed the drafted versions. All authors contributed to the article and approved the submitted version.
Funding
BG was financed by the European Regional Development Fund (grant number: ZS/2018/08/94206). The funder has no role in the design or conduct of this review.
Conflict of Interest
The authors declare that the research was conducted in the absence of any commercial or financial relationships that could be construed as a potential conflict of interest.
Supplementary Material
The Supplementary Material for this article can be found online at: https://www.frontiersin.org/articles/10.3389/fphys.2021.657274/full#supplementary-material
References
Albinet, C. T., Boucard, G., Bouquet, C. A., and Audiffren, M. (2010). Increased heart rate variability and executive performance after aerobic training in the elderly. Eur. J. Appl. Physiol. 109, 617–624. doi: 10.1007/s00421-010-1393-y
Badrov, M. B., Bartol, C. L., DiBartolomeo, M. A., Millar, P. J., McNevin, N. H., and McGowan, C. L. (2013). Effects of isometric handgrip training dose on resting blood pressure and resistance vessel endothelial function in normotensive women. Eur. J. Appl. Physiol. 113, 2091–2100. doi: 10.1007/s00421-013-2644-5
Belvederi Murri, M., Folesani, F., Zerbinati, L., Nanni, M. G., Ounalli, H., Caruso, R., et al. (2020). Physical activity promotes health and reduces cardiovascular mortality in depressed populations: a literature overview. Int. J. Environ. Res. Public Health. 17:5545. doi: 10.3390/ijerph17155545
Bhati, P., Moiz, J. A., Menon, G. R., and Hussain, M. E. (2019). Does resistance training modulate cardiac autonomic control? A systematic review and meta-analysis. Clin. Auton. Res. 29, 75–103. doi: 10.1007/s10286-018-0558-3
Billman, G. E. (2013). The LF/HF ratio does not accurately measure cardiac sympatho-vagal balance. Front. Physiol. 4:26. doi: 10.3389/fphys.2013.00026
Bond, V., Bartels, M. N., Sloan, R. P., Millis, R. M., Zion, A. S., Andrews, N., et al. (2009). Exercise training favourably affects autonomic and blood pressure responses during mental and physical stressors in African-American men. J. Hum. Hypertens. 23, 267–273. doi: 10.1038/jhh.2008.125
Borghi-Silva, A., Mendes, R. G., Trimer, R., Oliveira, C. R., Fregonezi, G. A., Resqueti, V. R., et al. (2015). Potential effect of 6 versus 12-weeks of physical training on cardiac autonomic function and exercise capacity in chronic obstructive pulmonary disease. Eur. J. Phys. Rehabil. Med. 51, 211–221.
Botek, M., McKune, A. J., Krejci, J., Stejskal, P., and Gaba, A. (2014). Change in performance in response to training load adjustment based on autonomic activity. Int. J. Sports Med. 35, 482–488. doi: 10.1055/s-0033-1354385
Bouaziz, W., Vogel, T., Schmitt, E., Kaltenbach, G., Geny, B., and Lang, P. O. (2017). Health benefits of aerobic training programs in adults aged 70 and over: a systematic review. Arch. Gerontol. Geriatr. 69, 110–127. doi: 10.1016/j.archger.2016.10.012
Boutcher, S. H., Park, Y., Dunn, S. L., and Boutcher, Y. N. (2013). The relationship between cardiac autonomic function and maximal oxygen uptake response to high-intensity intermittent-exercise training. J. Sports Sci. 31, 1024–1029. doi: 10.1080/02640414.2012.762984
Boutcher, Y. N., and Boutcher, S. H. (2017). Exercise intensity and hypertension: what's new? J. Hum. Hypertens. 31, 157–164. doi: 10.1038/jhh.2016.62
Brown, D. K., Barton, J. L., Pretty, J., and Gladwell, V. F. (2014). Walks4Work: assessing the role of the natural environment in a workplace physical activity intervention. Scand. J. Work Environ. Health 40, 390–399. doi: 10.5271/sjweh.3421
Buchheit, M., Chivot, A., Parouty, J., Mercier, D., Al Haddad, H., Laursen, P. B., et al. (2010). Monitoring endurance running performance using cardiac parasympathetic function. Eur. J. Appl. Physiol. 108, 1153–1167. doi: 10.1007/s00421-009-1317-x
Buchheit, M., Racinais, S., Bilsborough, J. C., Bourdon, P. C., Voss, S. C., Hocking, J., et al. (2013). Monitoring fitness, fatigue and running performance during a pre-season training camp in elite football players. J. Sci. Med. Sport. 16, 550–555. doi: 10.1016/j.jsams.2012.12.003
Carter, J. B., Banister, E. W., and Blaber, A. P. (2003). The effect of age and gender on heart rate variability after endurance training. Med. Sci. Sports Exerc. 35, 1333–1340. doi: 10.1249/01.MSS.0000079046.01763.8F
Cheema, B. S., Houridis, A., Busch, L., Raschke-Cheema, V., Melville, G. W., Marshall, P. W., et al. (2013). Effect of an office worksite-based yoga program on heart rate variability: outcomes of a randomized controlled trial. BMC Complement. Altern. Med. 13:82. doi: 10.1186/1472-6882-13-82
Chu, I.-H., Lin, Y.-J., Wu, W.-L., Chang, Y.-K., and Lin, I.-M. (2015). Effects of yoga on heart rate variability and mood in women: a randomized controlled trial. J. Altern. Complement. Med. 21, 789–795. doi: 10.1089/acm.2015.0138
Cooke, W. H., and Carter, J. R. (2005). Strength training does not affect vagal-cardiac control or cardiovagal baroreflex sensitivity in young healthy subjects. Eur. J. Appl. Physiol. 93, 719–725. doi: 10.1007/s00421-004-1243-x
Cripps, T. R., Malik, M., Farrell, T. G., and Camm, A. J. (1991). Prognostic value of reduced heart rate variability after myocardial infarction: clinical evaluation of a new analysis method. Br. Heart J. 65, 14–19. doi: 10.1136/hrt.65.1.14
da Silva, C. C., Goldberg, T. B., Soares-Caldeira, L. F., Oliveira, R. D., De Paula Ramos, S., and Nakamura, F. Y. (2015). The effects of 17 weeks of ballet training on the autonomic modulation, hormonal and general biochemical profile of female adolescents. J. Hum. Kinet. 47, 61–71. doi: 10.1515/hukin-2015-0062
da Silva, D. F., Ferraro, Z. M., Adamo, K. B., and Machado, F. A. (2019). Endurance running training individually guided by HRV in untrained women. J. Strength Cond. Res. 33, 736–746. doi: 10.1519/JSC.0000000000002001
da Silva, D. F., Verri, S. M., Nakamura, F. Y., and Machado, F. A. (2014). Longitudinal changes in cardiac autonomic function and aerobic fitness indices in endurance runners: a case study with a high-level team. Eur. J. Sport Sci. 14, 443–451. doi: 10.1080/17461391.2013.832802
de Abreu, R. M., Rehder-Santos, P., Simões, R. P., and Catai, A. M. (2019). Can high-intensity interval training change cardiac autonomic control? A systematic review. Braz. J. Phys. Ther. 23, 279–289. doi: 10.1016/j.bjpt.2018.09.010
de Rezende Barbosa, M., Júnior, J. N., Cassemiro, B. M., Bernardo, A., França da Silva, A. K., Vanderlei, F. M., et al. (2016). Effects of functional training on geometric indices of heart rate variability. J. Sport Health Sci. 5, 183–189. doi: 10.1016/j.jshs.2014.12.007
DeGiorgio, C. M., Miller, P., Meymandi, S., Chin, A., Epps, J., Gordon, S., et al. (2010). RMSSD, a measure of vagus-mediated heart rate variability, is associated with risk factors for SUDEP: the SUDEP-7 inventory. Epilepsy Behav. 19, 78–81. doi: 10.1016/j.yebeh.2010.06.011
Dobbs, W. C., Fedewa, M. V., MacDonald, H. V., Holmes, C. J., Cicone, Z. S., Plews, D. J., et al. (2019). The accuracy of acquiring heart rate variability from portable devices: a systematic review and meta-analysis. Sports Med. 49, 417–435. doi: 10.1007/s40279-019-01061-5
Draghici, A. E., and Taylor, J. A. (2016). The physiological basis and measurement of heart rate variability in humans. J. Physiol. Anthropol. 35:22. doi: 10.1186/s40101-016-0113-7
Duarte, A., Soares, P. P., Pescatello, L., and Farinatti, P. (2015). Aerobic training improves vagal reactivation regardless of resting vagal control. Med. Sci. Sports Exerc. 47, 1159–1167. doi: 10.1249/MSS.0000000000000532
Ernst, G. (2017). Heart-rate variability-more than heart beats? Front. Public Health. 5:240. doi: 10.3389/fpubh.2017.00240
Gamelin, F. X., Berthoin, S., Sayah, H., Libersa, C., and Bosquet, L. (2007). Effect of training and detraining on heart rate variability in healthy young men. Int. J. Sports Med. 28, 564–570. doi: 10.1055/s-2007-964861
Gordan, R., Gwathmey, J. K., and Xie, L.-H. (2015). Autonomic and endocrine control of cardiovascular function. World J. Cardiol. 7, 204–214. doi: 10.4330/wjc.v7.i4.204
Gronwald, T., Rogers, B., and Hoos, O. (2020). Fractal correlation properties of heart rate variability: a new biomarker for intensity distribution in endurance exercise and training prescription? Front. Physiol. 11:550572. doi: 10.3389/fphys.2020.550572
Heffernan, K. S., Fahs, C. A., Shinsako, K. K., Jae, S. Y., and Fernhall, B. (2007). Heart rate recovery and heart rate complexity following resistance exercise training and detraining in young men. Am. J. Physiol. Heart Circ. Physiol. 293, H3180–H3186. doi: 10.1152/ajpheart.00648.2007
Heffernan, K. S., Jae, S. Y., Vieira, V. J., Iwamoto, G. A., Wilund, K. R., Woods, J. A., et al. (2009). C-reactive protein and cardiac vagal activity following resistance exercise training in young African-American and white men. Am. J. Physiol. Regul. Integr. Comp. Physiol. 296, R1098–R1105. doi: 10.1152/ajpregu.90936.2008
Herold, F., Gronwald, T., Scholkmann, F., Zohdi, H., Wyser, D., Müller, N. G., et al. (2020). New directions in exercise prescription: is there a role for brain-derived parameters obtained by functional near-infrared spectroscopy? Brain Sci. 10:342. doi: 10.3390/brainsci10060342
Heydari, M., Boutcher, Y. N., and Boutcher, S. H. (2013). High-intensity intermittent exercise and cardiovascular and autonomic function. Clin. Auton. Res. 23, 57–65. doi: 10.1007/s10286-012-0179-1
Hottenrott, K., Hoos, O., and Esperer, H. D. (2006). Heart rate variability and physical exercise. Herz. 31, 544–552. doi: 10.1007/s00059-006-2855-1
Iwasaki, K.-I., Zhang, R., Zuckerman, J. H., and Levine, B. D. (2003). Dose-response relationship of the cardiovascular adaptation to endurance training in healthy adults: how much training for what benefit? J. Appl. Physiol. (1985). 95, 1575–1583. doi: 10.1152/japplphysiol.00482.2003
Kim, C.-S., Kim, M.-K., Jung, H.-Y., and Kim, M.-J. (2017). Effects of exercise training intensity on cardiac autonomic regulation in habitual smokers. Ann. Noninvasive Electrocardiol. 22:e12434. doi: 10.1111/anec.12434
Kiviniemi, A. M., Hautala, A. J., Kinnunen, H., Nissilä, J., Virtanen, P., Karjalainen, J., et al. (2010). Daily exercise prescription on the basis of HR variability among men and women. 1. Nur Standard-Training betrachten. Med. Sci. Sports Exerc. 42, 1355–1363. doi: 10.1249/mss.0b013e3181cd5f39
Kiviniemi, A. M., Hautala, A. J., Mäkikallio, T. H., Seppänen, T., Huikuri, H. V., and Tulppo, M. P. (2006). Cardiac vagal outflow after aerobic training by analysis of high-frequency oscillation of the R-R interval. Eur. J. Appl. Physiol. 96, 686–692. doi: 10.1007/s00421-005-0130-4
Kleiger, R. E., Miller, J. P., Bigger, J. T., and Moss, A. J. (1987). Decreased heart rate variability and its association with increased mortality after acute myocardial infarction. Am. J. Cardiol. 59, 256–262. doi: 10.1016/0002-9149(87)90795-8
Kuo, T. B., Lin, T., Yang, C. C., Li, C. L., Chen, C. F., and Chou, P. (1999). Effect of aging on gender differences in neural control of heart rate. Am. J. Physiol. 277, H2233–H2239. doi: 10.1152/ajpheart.1999.277.6.H2233
Laborde, S., Mosley, E., and Thayer, J. F. (2017). Heart rate variability and cardiac vagal tone in psychophysiological research - recommendations for experiment planning, data analysis, and data reporting. Front. Psychol. 8:213. doi: 10.3389/fpsyg.2017.00213
Levy, W. C., Cerqueira, M. D., Harp, G. D., Johannessen, K.-A., Abrass, I. B., Schwartz, R. S., et al. (1998). Effect of endurance exercise training on heart rate variability at rest in healthy young and older men. Am. J. Cardiol. 82, 1236–1241. doi: 10.1016/s0002-9149(98)00611-0
Liberati, A., Altman, D. G., Tetzlaff, J., Mulrow, C., Gøtzsche, P. C., Ioannidis, J. P. A., et al. (2009). The PRISMA statement for reporting systematic reviews and meta-analyses of studies that evaluate health care interventions: explanation and elaboration. PLoS Med. 6:e1000100. doi: 10.1371/journal.pmed.1000100
Macedo, F. N., Mesquita, T. R. R., Melo, V. U., Mota, M. M., Silva, T. L. T. B., Santana, M. N., et al. (2016). Increased nitric oxide bioavailability and decreased sympathetic modulation are involved in vascular adjustments induced by low-intensity resistance training. Front. Physiol 7:265. doi: 10.3389/fphys.2016.00265
Makivić, B., Nikić, M. D., and Willis, M. S. (2013). Heart Rate Variability (HRV) as a tool for diagnostic and monitoring performance in sport and physical activities. J. Exerc. Physiol. 16, 103–131.
Malik, M. (1996). Heart rate variability: standards of measurement, physiological interpretation, and clinical use. Circulation 93, 1043–1065. doi: 10.1161/01.CIR.93.5.1043
Malik, M., Farrell, T., Cripps, T., and Camm, A. J. (1989). Heart rate variability in relation to prognosis after myocardial infarction: selection of optimal processing techniques. Eur. Heart J. 10, 1060–1074. doi: 10.1093/oxfordjournals.eurheartj.a059428
Martinmaki, K., Hakkinen, K., Mikkola, J., and Rusko, H. (2008). Effect of low-dose endurance training on heart rate variability at rest and during an incremental maximal exercise test. Eur. J. Appl. Physiol. 104, 541–548. doi: 10.1007/s00421-008-0804-9
McCraty, R., and Shaffer, F. (2015). Heart rate variability: new perspectives on physiological mechanisms, assessment of self-regulatory capacity, and health risk. Glob. Adv. Health Med. 4, 46–61. doi: 10.7453/gahmj.2014.073
McHugh, A., Esco, M. R., Bloomquist, B. E., and Williford, H. N. (2012). Effects of a low volume recreational jogging program on cardiovascular-autonomic control. J. Exerc. Physiol. Online 15, 18–25
Moher, D., Liberati, A., Tetzlaff, J., and Altman, D. G. (2009). Preferred reporting items for systematic reviews and meta-analyses: the PRISMA statement. J. Clin. Epidemiol. 62, 1006–1012. doi: 10.1016/j.jclinepi.2009.06.005
Moreira, S. R., Teixeira-Araujo, A. A., Dos Santos, A. O., and Simões, H. G. (2017). Ten weeks of capoeira progressive training improved cardiovascular parameters in male practitioners. J. Sports Med. Phys. Fitness 57, 289–298. doi: 10.23736/S0022-4707.16.06030-8
Nuuttila, O. P., Nikander, A., Polomoshnov, D., Laukkanen, J. A., and Hakkinen, K. (2017). Effects of HRV-guided vs. predetermined block training on performance, HRV and serum hormones. Int. J. Sports Med. 38, 909–920. doi: 10.1055/s-0043-115122
Pal, R., Singh, S. N., Chatterjee, A., and Saha, M. (2014). Age-related changes in cardiovascular system, autonomic functions, and levels of BDNF of healthy active males: role of yogic practice. Age (Dordr.). 36:9683. doi: 10.1007/s11357-014-9683-7
Palma, S., Keilani, M., Hasenoehrl, T., and Crevenna, R. (2020). Impact of supportive therapy modalities on heart rate variability in cancer patients - a systematic review. Disabil. Rehabil. 42, 36–43. doi: 10.1080/09638288.2018.1514664
Pearson, M. J., and Smart, N. A. (2018). Exercise therapy and autonomic function in heart failure patients: a systematic review and meta-analysis. Heart Fail. Rev. 23, 91–108. doi: 10.1007/s10741-017-9662-z
Plews, D. J., Laursen, P. B., Kilding, A. E., and Buchheit, M. (2012). Heart rate variability in elite triathletes, is variation in variability the key to effective training? A case comparison. Eur. J. Appl. Physiol. 112, 3729–3741. doi: 10.1007/s00421-012-2354-4
Plews, D. J., Laursen, P. B., Stanley, J., Kilding, A. E., and Buchheit, M. (2013). Training adaptation and heart rate variability in elite endurance athletes: opening the door to effective monitoring. Sports Med. 43, 773–781. doi: 10.1007/s40279-013-0071-8
Posadzki, P., Kuzdzal, A., Lee, M. S., and Ernst, E. (2015). Yoga for heart rate variability: a systematic review and meta-analysis of randomized clinical trials. Appl. Psychophysiol. Biofeedback. 40, 239–249. doi: 10.1007/s10484-015-9291-z
Pumprla, J., Howorka, K., Groves, D., Chester, M., and Nolan, J. (2002). Functional assessment of heart rate variability: physiological basis and practical applications. Int. J. Cardiol. 84, 1–14. doi: 10.1016/s0167-5273(02)00057-8
Quiles, N., Taylor, B. S., and Ortiz, A. (2019). Effectiveness of an 8-week aerobic exercise program on autonomic function in people living with HIV taking Anti-retroviral therapy: a pilot randomized controlled trial. AIDS Res. Hum. Retroviruses. doi: 10.1089/AID.2019.0194
Raffin, J., Barthélémy, J.-C., Dupré, C., Pichot, V., Berger, M., Féasson, L., et al. (2019). Exercise frequency determines heart rate variability gains in older people: a meta-analysis and meta-regression. Sports Med. 49, 719–729. doi: 10.1007/s40279-019-01097-7
Routledge, F. S., Campbell, T. S., McFetridge-Durdle, J. A., and Bacon, S. L. (2010). Improvements in heart rate variability with exercise therapy. Can. J. Cardiol. 26, 303–312. doi: 10.1016/S0828-282X(10)70395-0
Sammito, S., and Böckelmann, I. (2015). Analysis of heart rate variability. Mathematical description and practical application. Herz. 40(Suppl. 1), 76–84. doi: 10.1007/s00059-014-4145-7
Sandercock, G. R. H., Bromley, P. D., and Brodie, D. A. (2005). Effects of exercise on heart rate variability: inferences from meta-analysis. Med. Sci. Sports Exerc. 37, 433–439. doi: 10.1249/01.mss.0000155388.39002.9d
Schuit, A. J., van Amelsvoort, L. G., Verheij, T. C., Rijneke, R. D., Maan, A. C., Swenne, C. A., et al. (1999). Exercise training and heart rate variability in older people. Med. Sci. Sports Exerc. 31, 816–821. doi: 10.1097/00005768-199906000-00009
Schumann, M., Botella, J., Karavirta, L., and Hakkinen, K. (2017). Training-load-guided vs standardized endurance training in recreational runners. Int. J. Sports Physiol. Perform. 12, 295–303. doi: 10.1123/ijspp.2016-0093
Selig, S. E., Caery, M., Menzies, D. G., Patterson, J., Geerling, R. H., Williams, A. D., et al. (2004). Moderate-intensity resistance exercise training in patients with chronic heart failure improves strength, endurance, heart rate variability, and forearm blood flow. J. Card. Fail. 10, 21–30. doi: 10.1016/s1071-9164(03)00583-9
Shaffer, F., and Ginsberg, J. P. (2017). An overview of heart rate variability metrics and norms. Front. Public Health 5:258. doi: 10.3389/fpubh.2017.00258
Shaffer, F., McCraty, R., and Zerr, C. L. (2014). A healthy heart is not a metronome: an integrative review of the heart's anatomy and heart rate variability. Front. Psychol. 5:1040. doi: 10.3389/fpsyg.2014.01040
Smart, N. A., Waldron, M., Ismail, H., Giallauria, F., Vigorito, C., Cornelissen, V., et al. (2015). Validation of a new tool for the assessment of study quality and reporting in exercise training studies: TESTEX. Int. J. Evid. Based Healthc. 13, 9–18. doi: 10.1097/XEB.0000000000000020
Sousa Fortes, L., de Pinheiro Paes, P., Tavares Paes, S., Oliveira Carvalho, F., and Serpeloni Cyrino, E. (2018). Clustering vs multi-sets method in resistance training: effect on heart rate variability. Asian J. Sports Med. 9, 1–6. doi: 10.5812/asjsm.14576
Stein, P. K., Ehsani, A. A., Domitrovich, P. P., Kleiger, R. E., and Rottman, J. N. (1999). Effect of exercise training on heart rate variability in healthy older adults. Am. Heart J. 138, 567–576. doi: 10.1016/S0002-8703(99)70162-6
Thayer, J. F., Yamamoto, S. S., and Brosschot, J. F. (2010). The relationship of autonomic imbalance, heart rate variability and cardiovascular disease risk factors. Int. J. Cardiol. 141, 122–131. doi: 10.1016/j.ijcard.2009.09.543
Tsuji, H., Venditti, F. J., Manders, E. S., Evans, J. C., Larson, M. G., Feldman, C. L., et al. (1994). Reduced heart rate variability and mortality risk in an elderly cohort: the Framingham heart study. Circulation 90, 878–883. doi: 10.1161/01.CIR.90.2.878
Tulppo, M. P., Hautala, A. J., Mäkikallio, T. H., Laukkanen, R. T., Nissilä, S., Hughson, R. L., et al. (2003). Effects of aerobic training on heart rate dynamics in sedentary subjects. J. Appl. Physiol. (1985). 95, 364–372. doi: 10.1152/japplphysiol.00751.2002
Varela-Sanz, A., Tuimil, J. L., Abreu, L., and Boullosa, D. A. (2017). Does concurrent training intensity distribution matter? J. Strength Cond. Res. 31, 181–195. doi: 10.1519/JSC.0000000000001474
Villafaina, S., Collado-Mateo, D., Fuentes, J. P., Merellano-Navarro, E., and Gusi, N. (2017). Physical exercise improves heart rate variability in patients with type 2 diabetes: a systematic review. Curr. Diab. Rep. 17:110. doi: 10.1007/s11892-017-0941-9
Voss, A., Schroeder, R., Heitmann, A., Peters, A., and Perz, S. (2015). Short-term heart rate variability - influence of gender and age in healthy subjects. PLoS ONE 10. doi: 10.1371/journal.pone.0118308
Wisløff, U., Støylen, A., Loennechen, J. P., Bruvold, M., Rognmo, Ø., Haram, P. M., et al. (2007). Superior cardiovascular effect of aerobic interval training versus moderate continuous training in heart failure patients: a randomized study. Circulation 115, 3086–3094. doi: 10.1161/CIRCULATIONAHA.106.675041
Wu, S.-D., Gau, J.-T., and Wang, Y.-H. (2011). Ryodoraku as a tool monitoring the effects of walking exercise. Chin. J. Integr. Med. 9, 1319–1325. doi: 10.3736/jcim20111207
Keywords: heart rate variability, autonomic nervous system, physical intervention, cardiovascular risk factors, healthy adults
Citation: Grässler B, Thielmann B, Böckelmann I and Hökelmann A (2021) Effects of Different Training Interventions on Heart Rate Variability and Cardiovascular Health and Risk Factors in Young and Middle-Aged Adults: A Systematic Review. Front. Physiol. 12:657274. doi: 10.3389/fphys.2021.657274
Received: 22 January 2021; Accepted: 30 March 2021;
Published: 23 April 2021.
Edited by:
Urs Granacher, University of Potsdam, GermanyReviewed by:
Kuno Hottenrott, Martin Luther University of Halle-Wittenberg, GermanyJeremy Raffin, Université Jean Monnet, France
Copyright © 2021 Grässler, Thielmann, Böckelmann and Hökelmann. This is an open-access article distributed under the terms of the Creative Commons Attribution License (CC BY). The use, distribution or reproduction in other forums is permitted, provided the original author(s) and the copyright owner(s) are credited and that the original publication in this journal is cited, in accordance with accepted academic practice. No use, distribution or reproduction is permitted which does not comply with these terms.
*Correspondence: Bernhard Grässler, bernhard.graessler@ovgu.de