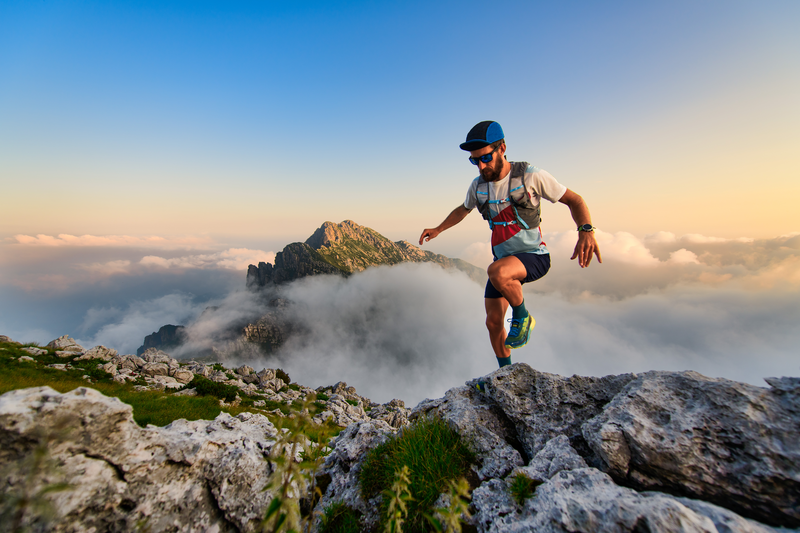
94% of researchers rate our articles as excellent or good
Learn more about the work of our research integrity team to safeguard the quality of each article we publish.
Find out more
ORIGINAL RESEARCH article
Front. Physiol. , 09 July 2021
Sec. Vascular Physiology
Volume 12 - 2021 | https://doi.org/10.3389/fphys.2021.644903
Aortic dissection (AD) is one of the most fatal cardiovascular emergency. At the anatomical level, AD occurs due to the formation of intimal tears. However, the molecular mechanism underlying this phenomenon remains unknown. Angiotensin II (Ang II) is a important effector in the development of cardiovascular disease that acts through binding to angiotensin type 1 receptor (AT1R). Yes-associated protein (YAP) was recently recognized as a key protein in macrophage activation. To determine whether AT1R and YAP are involved in macrophage-induced endothelial cell (EC) inflammation and AD incidence, we co-cultured THP-1 cells and HAECs in transwell chambers under different culture conditions and apply different conditions to the AD mice model. The results showed that Ang II promoted macrophage M1 polarization and adhesion, upregulated YAP phosphorylation, and induced EC injury that was related to increased levels of multiple pro-inflammatory chemokines. Blocking AT1R function pharmacologically or by transfection with AT1R siRNA can reduce the pro-inflammatory effect induced by Ang II. In addition, siRNA knock down of YAP expression further aggravated the pro-inflammatory effects of Ang II. Treatment with ARB effectively alleviated these pro-inflammatory effects. In the mice AD model, ARB effectively reduced the incidence of AD in mice, decreased M1 macrophages infiltration and AT1R content in the aortic wall and increased the tissue content of YAP. We found that AT1R induces YAP phosphorylation through binding to Ang II, and further promotes macrophage M1 polarization and adhesion to ECs. ARB reduces the incidence of AD in mice and affect macrophage polarization in mice aorta.
- Angiotensin II regulates YAP through angiotensin type I receptors in macrophages, and reduced aortic dissection incidence in mice.
- YAP affects macrophage polarization after being regulated by angiotensin type I receptor.
Aortic dissection (AD) is a lethal disease that causes a large number of aortic-related deaths (Hiratzka et al., 2010). Epidemiological surveys showed that the incidence of thoracic aortic dissection is 3–4 per 100,000 individuals per year (Olsson et al., 2006; Kochanek et al., 2011). AD is defined as blood flow that enters the aortic media through intimal tears, followed by formation of a true lumen (TL) and a false lumen (FL) with or without communication (Erbel et al., 2014). Clinically, AD can cause various serious complications, such as aortic rupture, visceral ischemia and etc. The majority of patients with non-operative ascending AD (Stanford type A) die within 2 weeks (Chen et al., 1997). Unfortunately, due to its unpredictable nature, little is known about pathological and molecular mechanisms that occur before or after onset of AD. Clinical treatment options for AD include optimal medical treatment (OMT), aortic replacement, and thoracic endovascular aorta repair (TEVAR) (Jia et al., 2013). However, the AD patients mortality rate after treatment remains high (Khayat et al., 2018). The surgical mortality for AD ranges from 10 to 35%, even at experienced medical centers (Nienaber and Clough, 2015). OMT is commonly used to treat AD in the clinic, mainly to protect the fragile aortic wall by reducing heart rate and blood pressure. However, there is no uniform standard for the selection of antihypertensive drugs, with most doctors relying on their own experience to select the most appropriate drugs. There have been no studies addressing which antihypertensive drugs are most effective in treating AD. Therefore, AD pathogenesis and the best treatment options are still poorly characterized.
Previous studies have shown that the occurrence of AD is associated with mutations in certain genes, such as FBN1, COL3A1, TGBFR1, and ACTA2. In addition to these genetic factors, there are other risk factors for AD such as hypertension, dyslipidemia, and smoking (Ohno-Urabe et al., 2018). Degeneration of the aortic media is now widely recognized as a histopathological risk factor for AD formation. This degeneration is caused by loss and disruption of elastic fibers and increased deposition of proteoglycans (Hiratzka et al., 2010; Kurihara et al., 2012). Degeneration only weakens the media layer, while most cases of AD begin with formation of an intimal tear (Erbel et al., 2014).
The occurrence of these non-genetic risk factors suggests that inflammation and intimal damage may contribute to the formation of intimal tears. Angiotensin II (Ang II) is the main effector peptide of the renin-angiotensin system, which can induce vasoconstriction, hypertrophy and extracellular remodeling through Ang II type 1 receptor (AT1R) (Schluter and Wenzel, 2008). Moreover, Ang II also regulates macrophage recruitment and polarization, which in turn triggers inflammation of local tissues (Yamamoto et al., 2011; Kaji, 2018). More importantly, studies have shown that Ang II contributes to the development of AD in humans and experimental animals (Daugherty et al., 2000). Angiotensin receptor blocker (ARB) is an antihypertensive drug that is commonly used to control blood pressure of AD patients through blocking AT1R. Therefore, we sought to determine whether ARB has other effects on AD in addition to lowering blood pressure and maintaining aortic wall stability.
Yes-associated protein (YAP) is a pluripotent intracellular connexin, which can act as a transcriptional co-activator and the main effector of the Hippo-YAP signaling pathway. Nuclear YAP binds to transcriptional enhancer associated domain transcription factors (TEADs) to affects cell proliferation and apoptosis through regulating transcriptional processes (Valis et al., 2011). Mammalian sterile 20-like kinase-1 and mammalian sterile 20-like kinase-2 (Mst1/2) act as the main mediators of the Hippo YAP pathway, and up-regulate YAP phosphorylation level. Since phosphorylated YAP (p-YAP) cannot enter the nucleus, it remains in the cytoplasm, so it cannot participate in regulating transcription (Ren et al., 2008). YAP plays a key role in mediating the proliferation, migration, apoptosis, and phenotypic transition of vascular smooth muscle cell (Feng et al., 2016). In addition, a recent study found that Hippo-YAP signaling in hepatocytes inhibits macrophage infiltration during formation of the protumoral microenvironment by inhibiting YAP, thereby maintaining normal liver growth (Kim et al., 2018). This prompted us to ask whether YAP plays a role in macrophage-induced endothelial cell (EC) inflammation and injury, and whether YAP is regulated by AT1R in macrophages. Therefore, the purpose of this study is to investigate the regulation of Ang II on AT1R-mediated YAP and the mechanism of macrophage-induced EC damage.
Forty-eight wild type mice (male, 22–24 g) were purchased from the experimental animal center of the Academy of Military Medical Sciences of PLA, Beijing, China. Twelve mice were randomly selected as the control group. The remaining 36 mice were given 1 g/kg β-aminopropionitrile (BAPN) (Xiya reagent, 151-18-8) water daily, and were randomly divided into three groups after 2 weeks of feeding. Group 1 continued BAPN feeding for 2 weeks; Group 2 and Group 3 continued BAPN feeding while telmisartan (T8949-10MG, Sigma-Aldrich, St. Louis, MO, United States) and nifedipine were fed separately for 2 weeks. The mice were fed with nifedipine (N7634-1G, Sigma-Aldrich, St. Louis, MO, United States) in order to set up an animal model with similar blood pressure levels to the telmisartan feeding group. The reason is that YAP is regulated by mechanical signals (Endlich et al., 2017), so we try to avoid the influence of different blood pressure level on YAP regulation by feeding with nifedipine. All mice were fed for 4 weeks, and each group of mice took Tail-cuff tail pressure method at five time points: 1 day before feeding, after feeding for 1, 2, 3, and 4 w to monitor changes in systolic blood pressure. Feeding mice with nifedipine and monitoring the molecular tail pressure is because that YAP is a pressure-sensitive protein. In order to eliminate the difference between the groups caused by the difference in aortic wall pressure, the blood pressure of the mice is controlled at the same level to observe the effect of ARB drugs on YAP.
Human monocytic THP-1cells (Shanghai Zhong Qiao Xin Zhou Biotechnology Co., Ltd., ZQ0086) were cultured in RPMI-1640 medium (Life Technologies, Carlsbad, CA, United States) supplemented with 10% FBS, 2 mM L-Glutamine, and 1% antibiotics (penicillin, streptomycin, and amphotericin-B) (Sigma-Aldrich, St. Louis, MO, United States). THP-1 were incubated with 100 mg/ml phorbol 12-myristate 13-acetate (PMA, Solarbio, P6741)for 48 h to induce differentiation into quiescent M0 macrophages. When the cells were adherent, they were transferred to PMA-free medium to obtain resting macrophages (M0). Then, M0 cells were seeded into the lower wells of transwell chamber with 0.4 μm pores (6 × 104 cells/well) (Corning, Corning, NY, United States), and human aortic endothelial cells (HAECs) were seeded into the upper wells (5 × 105 cells/well). Different treatment for M0 macrophages were placed in the lower wells after 24 h incubation as follows: Control group, THP-1 + HAECs only; group 1, THP-1 + HAECs with Ang II (1 μM) treatment; group 2, THP-1 + HAECs with Ang II (1 μM) and small interfering RNA (siRNA) negative control (NC) transfection; group 3, THP-1 + HAECs with Ang II (1 μM) and AT1R siRNA transfection; group 4, THP-1 + HAECs with Ang II (1 μM) and 1 μl DMSO; and group 5, THP-1 + HAECs with Ang II (1 μM) and the ARB 1 μl (20 mM) telmisartan treatment. After applying various intervention conditions, the cells were further cultured at 37°C and 5% CO2 for 72 h, and the total incubation time was 96 h.
THP-1 and HAECs were inoculated into multi-well plates (5 × 103 cells/well). Cells were inoculated with three replicate sets per experimental group. We followed the CCK-8 method in our previous study (Wang et al., 2020).
Endothelin (ET)-1 levels in supernatant from each group at the end of the 96 h treatment and in the mice serum from different group after 4 week feed were tested through competitive inhibition ELISA with ET-1 Antibody ELISA kit (USCN Life Sciences, Inc.), and interleukin (IL)-6 and matrix metallopeptidase (MMP)-9 in the cell solutions and mice serum were detected by double antibody ELISA with IL-6 ELISA Kit (USCN Life Sciences, Inc.) and MMP9 ELISA Kit (USCN Life Sciences, Inc.), respectively. We follow our previous research for ELISA (Wang et al., 2020).
The macrophages were collected from the lower chamber for each group, counted, resuspended, and the cell number was adjusted to approximately 1 × 105 per sample. Fluorescent antibodies were diluted with FACS buffer at the following ratios: anti-CD86-PE (1:200, BioLegend, Inc., 305406), anti-CD68-FITC (1:100, BioLegend, Inc., 305206), and anti-CD206-APC (1:200, BioLegend, Inc., 321110). Fifty microliters of each diluted antibody working solution was added to each cell sample, after which the cells were incubated at 4°C for 30 min in the dark. After incubation, 200 μl of FACS buffer was added to each sample. The mixture was centrifuged at 1,000 rpm for 10 min at 10°C, and the supernatant was discarded. Cell samples were analyzed using a FACS Calibur flow cytometer and Cell Quest software (Becton Dickinson, Franklin Lakes, NJ, United States).
Each group of THP-1 after 96 h co-culture and AD mice aorta tissue was collected for Western blotting test. Membranes were subsequently incubated overnight at 4°C with the following primary antibodies: Anti-YAP (cat. no. AF6328; polyclonal, rabbit anti-human and mouse; 1:1,000; Affinity Biosciences), anti-p-YAP (Ser127) (cat. no. AF3328; polyclonal, rabbit anti-human and mouse; 1:1,000; Affinity Biosciences), anti-AT1R (cat. no. DF4910; polyclonal, rabbit anti-human and mouse; 1:1,000; Affinity Biosciences), anti-GAPDH (cat. no. ab9485; polyclonal, rabbit anti-human and mouse; 1:2,500; Abcam). The specific operations and reagents are the same as our previous research (Wang et al., 2020). ImageJ (V1.8.0, National Institutes of Health) was used to evaluate the density of bands by quantitative densitometry.
Shanghai GenePharma Co., Ltd., designed and synthesized AT1R siRNA as previous study (Wang et al., 2020). YAP siRNA was also designed and synthesized be the same company as follows: YAP – 860, GCAUCUUCGACAGUCUUCUTT; YAP – 941, GGUCAGAGAUACUUCUUAATT; YAP – 1662, GGUGAUACUAUCAACCAAATT; NC, UUCUCCGAACGUG UCACGUTT. AT1R siRNAs and YAP siRNA were transfected into cells with Lipofectamine® 2000 (Thermo Fisher Scientific, Waltham, MA, United States) following the manufacturer’s protocol. M0 THP-1cells were inoculated into a 6-well plate at 5 × 105 cells/well, and cultured overnight at 37°C in 5% CO2 incubator. Two hours before transfection, they were mixed with serum-free MEMα medium (Gibco; Thermo Fisher Scientific).
RT-qPCR for siRNA selection: At 48 h after siRNA transfection, as described in the previous section, AT1R relative and YAP relative mRNA were collected from the transfected THP-1 cells. The RT-qPCR was performed as the same way as our previous study (Wang et al., 2020). Based on the effectiveness of AT1R siRNA shown in Figure 4A, SiRNA-165 was used in subsequent experiments. And, siRNA-1662 was chosen to knockdown YAP which is exhibit in Figure 6A. RT-qPCR for CTGF and CYR61: After 96 h co-culture, CTGF and CYR61 were collected from different group of THP-1. RevertAid First Strand cDNA Synthesis kit (Lot:U9126, TIANGEN, Inc.) were used according to the manufacturer’s protocol in order to reverse transcribe 1 μg RNA into first-strand cDNA. FastStart Universal SYBR Green Master (Rox; 04913914001, Hoffmann-La Roche, Ltd.) was then used to perform quantitative PCR as follows: 2 min at 50°C, 10 min at 95°C, and then 45 cycles of 30 s at 95°C and 30 s at 60°C. Amplification was performed on a PCR System 7300 (Applied Biosystems; Thermo Fisher Scientific, Inc.). The 2−ΔΔ Cq method was used to analyze the results.
M0 macrophages were labeled with calcein acetoxymethyl ester (MedChemExpress, HY-D0041) and incubated with HAECs at a density of 2 × 105 for 2 h. Depending on the experimental group, the cells were then transfected with the AT1R siRNA for 48 h or treated with Ang II and telmisartan for 24 h. Then, the cells were lightly rinsed with 1% BSA in PBS to remove unadhered cells. All cells were labeled with DAPI, and the proportion of macrophages adhered to each group was analyzed by fluorescence microscopy. The proportion of adherent cells (%) = the number of macrophages (green fluorescence)/the total number of cells (blue fluorescence) × 100.
The mice aortas were separated and fixed in the 4% paraformaldehyde for 48 h after 4 w of treatment with different drugs. Serial paraffin cross section (5 μm) of mice aorta were prepared for histological analysis. Aorta sections were stained with eosin and hematoxylin for morphological assessment. Immunostaining of CD68 and polarization markers (CD86, CD86, 1:1,000, Santa Cruz, CA, United States) was performed to detect macrophages infiltration in mice aorta. The images of immunofluorescence were digitally captured on a Carl Zeiss LSM880 laser scanning confocal microscope (Carl zeiss, Jena, Germany). CD68 + cell number or average integral optical density of CD 86 and CD 206 in the mice aortic wall were quantitative analyzed from 10 samples per group using Image Pro Plus 6.0 software (Media Cybernetics, Silver Spring, MD, United States).
Statistical analysis was performed using SPSS software (v19.0; IBM Corp.) to evaluate differences among groups. Comparisons among >2 groups were performed using one-way ANOVA followed by Tukey’s post hoc test. Data are presented as mean ± standard deviation, and P < 0.05 was considered to indicate a statistically significant difference. All experiments were repeated in triplicates (Wang et al., 2020).
We first divided the co-cultured cells (THP-1 cells and HAECs) into four groups: control group, co-cultured cells only; group 1, co-cultured cells with Ang II; group 2, co-cultured cells with Ang II and DMSO; group 3, co-cultured cells with Ang II and telmisartan (an ARB); group 4, co-cultured cells with Ang II and nifedipine (a calcium channel blocker). Then, we tested the proliferation in each group using a CCK-8 assay (Figure 1A). After Ang II treatment, the cell proliferation activity was significantly lower than the control group (P < 0.01). Compared with the control group, cell proliferation was significantly increased after treatment with telmisartan (group 3) (P < 0.01). Based on these results, we speculated that Ang II damages HAECs, and that this damage is likely due to Ang II–mediated promotion of macrophage infiltration and adhesion, which cause an inflammatory reaction. Ang II is known to upregulate ET-1, IL-6 and MMP-9 to promote endothelial inflammation (Nurden, 2011; Pacurari et al., 2014). Therefore, we measured ET-1, IL-6, and MMP-9 levels in supernatants from each group (Figures 1B–D), and found that the circulating levels of these proteins were all significantly higher in group 1 and group 2 than in the control group and group 3 (P < 0.05). These findings indicate that Ang II promotes ECs inflammation and that ARB can alleviate these pro-inflammatory effects.
Figure 1. Ang II inhibits cell proliferation and promotes ECs inflammation in co-cultured HAECs and THP-1 cells, and telmisartan counteracts this effect. (A) M0 macrophages were first co-cultured with HAECs for 24 h, and then the intervention conditions of each group were added to the lower chamber and continued to co-culture for 72 h. CCK-8 detection of cell proliferation in THP-1 cell and HAEC co-cultures at 0, 24, 48, 72, and 96 h. After 96 h of co-culture, Ang II (1 μM, 24 h) significantly decreased the proliferation of HAECs and THP-1 cells (P < 0.01), and this phenomenon was significantly reduced by telmisartan treatment (20 μM, 24 h) (P < 0.01); (B) ELISA results of IL-6 in supernatants from different samples after 96 h co-culture; (C) ELISA results of ET-1 in supernatants from different samples after 96 h co-culture; (D) ELISA results of MMP-9 in supernatants from different samples after 96 h co-culture. Ang II (1 μM, 24 h) significantly increased the expression of IL-6, ET-1, and MMP 9 in the supernatants compared with the control group (which was not treated with Ang II). IL-6, ET-1, and MMP-9 levels were significantly lower in the telmisartan (20 μM, 24 h) group compared with the DMSO group; (n = 3, mean and S.D., t-test) **P < 0.05 compared with the HAECs + THP-1 group; ∧∧P < 0.05 compared with the HAECs + THP-1 + Ang II group; ##P < 0.05 compared with the HAECs + THP-1 + DMSO group.
Pro-inflammatory M1 (CD68 +, CD86 +) macrophage polarization can cause acute inflammation, while M2 (CD206 +) polarization is associated with cell proliferation and fibrosis (Guo et al., 2017). Therefore, the proportion of M1 cells was assayed to determine the severity of inflammation in each group. To do this, we analyzed the proportion of CD68 +, CD86 +, and CD206 + cells in the lower chamber of each sample by flow cytometry (Figure 2A). Compared with the control group, Ang II had a positive effect on M1 polarization in group 1 and group 2 (P < 0.05). However, the proportion of M1 cells was significantly decreased (P < 0.01), while the proportion of CD206 + cells was significantly increased (P < 0.01) after treatment with telmisartan in group 3 (Figures 2B–D). This led us to hypothesize that the higher number of pro-inflammatory macrophages present causes more macrophages to adhere to endothelial cells, producing inflammation and intimal damage (Ding et al., 2016). To verify this hypothesis, we examined the adhesion rate of THP-1 cells to HAECs by immunocytochemistry (Figure 2E). As expected, the results showed that the adhesion rate of THP-1 cells in group 1 and group 2, which contained Ang II, was significantly higher than that of the control group (Figure 2F). This increase in adhesion rate due to Ang II was reduced in group 3 due to treatment with ARB. This suggests that Ang II promotes the development of an inflammatory response and intimal injury, while treatment with ARB can alleviate these effects and may promote reconstruction of the disrupted tissue by increasing the proportion of M2 cells (Ivanova et al., 2019).
Figure 2. Ang II promotes THP-1 polarization to a CD68 + CD86 + phenotype and increases THP-1 adhesion. Telmisartan attenuates these effects and promotes THP-1 polarization to a CD206 + phenotype. (A) Flow cytometry analysis of CD68, CD86, and CD206 expression in the different groups after 96 h co-culture. The data shown in the one-factor histogram for each group represent the cells in the red circle in the upper scatter plot. HAECs + THP-1 group: CD68, 12.51%; CD86, 12.54%; CD206, 7.11%. HAECs + THP-1 + Ang II (1 μM, 24 h) group: CD68, 53.30%; CD86, 48.09%; CD206, 9.64%. HAECs + THP-1 + Ang II (1 μM, 24 h) + DMSO (20 μM, 24 h) group: CD68, 48.19%; CD86, 46.24%; CD206, 12.66%. HAECs + THP-1 + Ang II + telmisartan (20 μM, 24 h) group: CD68, 19.42%; CD86, 21.89%; CD206, 23.34%; (B,C) The proportion of CD68 + and CD86 + cells, respectively [n = 3, mean and Standard Deviation (S.D.), t-test, **P < 0.05 compared with the HAECs + THP-1 group; ∧∧P < 0.05 compared with the HAECs + THP-1 + Ang II group; ##P < 0.05 compared with the HAECs + THP-1 + DMSO group]. The proportion of CD68 + and CD86 + cells was significantly increased by treatment with Ang II (P < 0.01), and telmisartan reversed this increase. (D) The proportion of CD206 + cells (n = 3, mean and S.D., t-test, symbols are the same as in B,C). The proportion of CD206 + cells increased in response to treatment with Ang II (P < 0.05), and telmisartan strongly promoted THP-1 polarization to a CD206 + phenotype (P < 0.01); (E) Fluorescence staining of adherent macrophages in the different groups. All cells were labeled with DAPI (blue), and the number of adherent macrophages increased significantly in response to treatment with Ang II. This effect was not seen in cells treated with telmisartan; (F) The ratio between the number of macrophages and the total number of cells (n = 3, mean and S.D., t-test, symbols are the same as in B,C).
In order to identify the changes in the expression levels of YAP and AT1R in different groups, we used western blotting to detect the expression of AT1R, YAP, and p-YAP in each group of THP-1 cells (Figure 3A). Compared with the control group, YAP expression was decreased in THP-1 cells from group 1 and group 2, which were treated with Ang II (Figure 3C), while AT1R (Figure 3B), and p-YAP (Figure 3D) expression were increased in these groups (P < 0.05). In group 3, treatment with telmisartan alleviated these changes in expression (Figures 3B–D). These data indicate that Ang II promotes YAP phosphorylation, preventing it from entering the nucleus. Next, we used siRNA transfection to provide further evidence for the role of AT1R in this process.
Figure 3. Ang II promotes AT1R expression and YAP phosphorylation, and treatment with telmisartan or transfection with AT1R siRNA counteract this effect. (A) Western bolt analysis of six independent lysates from 96 h co-cultured HAECS + THP-1 cells that were untreated, treated with Ang II, treated with Ang II and siRNA NC, treated with Ang II and AT1R siRNA, treated with Ang II and DMSO, or treated with Ang II and telmisartan. Ang II treatment up-regulated the content of AT1R and p-YAP, and this effect was alleviated by AT1R siRNA transfection or telmisartan treatment. (B–D) The ratio between AT1R and GAPDH, YAP and GAPDH, p-YAP and GAPDH or p-YAP and YAP expression (n = 3, mean and S.D., t-test, **P < 0.05 compared with the HAECs + THP-1 group; ∧∧P < 0.05 compared with the HAECs + THP-1 + Ang II group; ##P < 0.05 compared with the HAECs + THP-1 + DMSO group).
To further confirm that Ang II regulation of macrophages is mediated by AT1R, we used an siRNA to knock down AT1R expression. RT-PCR analysis was performed to select the optimal AT1R siRNA, AT1R siRNA-165 (Figure 4A). The cells were divided into two groups: THP-1 cells + HAECs + Ang II + control siRNA and THP-1 cells + HAECs + Ang II + AT1R siRNA. Then, we tested cell proliferation, macrophage polarization, macrophage adhesion, and YAP phosphorylation as described above. THP-1 M1 polarization and adhesion were inhibited by AT1R knockdown, and M2 polarization was upregulated (Figures 4B–G). Transfection with the AT1R siRNA had almost the same effect as treatment with ARB on the consequences of treatment with Ang II, which provides more evidence that the pro-inflammatory effects of Ang II are mediated by AT1R (Figures 4H–J). Next, we asked whether there is a link between the regulated macrophage polarization and changes in YAP phosphorylation triggered by Ang II; or in other words, whether Ang II regulates macrophage polarization through phosphorylation of YAP. To test the effect of AT1R on YAP activity, we tested the expression of YAP-related genes CTGF and CYR61. As shown in Figure 5, Ang II can cause the down-regulation of CTGF and CYR61, while AT1R siRNA and Telmisartan treatment can alleviate this phenomenon.
Figure 4. The effect of AT1R siRNA transfection on macrophages was consistent with the effects of telmisartan treatment, further demonstrating that Ang II regulates macrophage polarization and adhesion activity by binding to AT1R. (A) AT1R siRNA knockdown efficiencies (n = 3, mean and S.D., t-test, &&P < 0.05 compared with siRNA NC;%%P < 0.05 compared with siRNA-165); (B) Flow cytometry analysis of CD68, CD86, and CD206 expression after 96 h co-culture in both groups. The data shown in the one-factor histogram for each group represents the cells in the red circle in the upper scatter plot. HAECs + THP-1 + Ang II (1 μM, 24 h) + siRNA NC group: CD68, 52.79%; CD86, 47.84%; CD206, 11.15%. HAECs + THP-1 + Ang II + AT1R siRNA group: CD68, 16.34%; CD86, 20.69%; CD206, 18.79%; (C–E) The proportion of CD68 +, CD86 +, and CD206 + cells, respectively (n = 3, mean and S.D., t-test, **P < 0.05 compared with the HAECs + THP-1 + siRNA NC group); (F) Fluorescence staining of adherent macrophages after AT1R siRNA transfection. All cells were labeled with DAPI (blue), and the number of adherent macrophages decreased significantly with AT1R siRNA transfection; (G) The ratio between the number of macrophages and the total number of cells (n = 3, mean and S.D., t-test, symbols are the same as in C,D,E); (H–J), The ratio between AT1R and GAPDH, p-YAP and YAP, or YAP and GAPDH expression was calculated based on the western blot results shown in Figure 3A (n = 3, mean and S.D., t-test, symbols are the same as in C–E).
Figure 5. Ang II can reduce the expression of CTGF and CYR61, while AT1R siRNA transfection and Telmisartan treatment can alleviate this effect. (A) The qRT-PCR results of CTGF in THP-1 from different group after 96 h co-culture; (B) The qRT-PCR results of CYR61 in THP-1 from different group after 96 h co-culture. (n = 3, mean and S.D., t-test, **P < 0.05 compared with the control group; ##P < 0.05 compared with the HAECs + THP-1 + Ang II group; ∧∧P < 0.05 compared with the HAECs + THP-1 + Ang II + DMSO group).
To determine whether YAP is a key mediator of Ang II regulation of macrophage polarization and adhesion, we knocked down YAP using an siRNA. To select the optimal siRNA for knocking down YAP expression, we tested the effect of different YAP siRNAs by RT-PCR. The RT-PCR results showed that the effect of YAP siRNA860 was the most stable (Figure 6A). Next, we established two groups: THP-1 cells + HAECs + Ang II + control siRNA and THP-1 cells + HAECs + Ang II + YAP siRNA. The cells were cultured as previously, after which THP-1 polarization and adhesion in each group were detected by flow cytometry (Figure 6D) and immunocytochemistry (Figure 6H), respectively. The results showed that siRNA knockdown of YAP increased THP-1 M1 polarization (Figure 6B–E) and increased the Ang II-induced macrophages adhesion rate (Figures 6F,G). Also, the ratio between the number of macrophages and total number of cells was higher after YAP knockdown (Figure 6I). Taken together, these findings indicate that Ang II regulates pro-inflammatory polarization and adhesion in macrophages by altering YAP expression.
Figure 6. Transfecting macrophages with a YAP siRNA further enhanced Ang II–induced macrophage M1 polarization and adhesion. (A) YAP siRNA efficiencies (n = 3, mean and S.D., t-test, &&P < 0.05 compared with siRNA NC;%%P < 0.05 compared with siRNA 860); (B,C) Western Blot of YAP after siRNA transfection *P < 0.05 compared with siRNA NC; (D) Flow cytometry analysis of CD68, CD86, and CD206 expression after 96 h co-culture in both groups. The data in the one-factor histogram represents the cells in the red circle in the upper scatter plot. HAECs + THP-1 + Ang II (1 μM, 24 h) + siRNA NC group: CD68, 41.94%; CD86, 45.73%; CD206, 9.72%. HAECs + THP-1 + Ang II + YAP siRNA group: CD68, 63.13%; CD86, 53.42%; CD206, 7.13%; (E–G) The proportion of CD68 +, CD86 +, and CD206 + cells, respectively (n = 3, mean and S.D., t-test, **P < 0.05 compared with the HAECs + THP-1 + siRNA NC group); (H) Fluorescence staining of adherent macrophages after YAP siRNA transfection. All cells were labeled with DAPI (blue), and the number of adherent macrophages was significantly decreased after transfection with the AT1R siRNA; (I) The ratio between the number of macrophages and the total number of cells (n = 3, mean and S.D., t-test, symbols are the same as in C,D,E).
The mice in each group received the aortic anatomy surgery after 48 days of feeding. Blood pressure levels of mice in groups 2 and 3 remained similar throughout the entire breeding process (relevant data is in the supplement). The incidence of AD in each group was 0/12 (group control), 10/12 (group 1), 5/12 (group 2), and 8/12 (group 3), respectively. Two mice in group 1, one in group 2 and group 3 each died during feeding time, and these mice were presented AD after aortic anatomy surgery. The incidence of AD in group 2 was the lowest, and that in group 3 was also lower than that in group 1. The aorta specimens of mice in each group are shown in (Figures 7-A, A), A HE staining was performed on the paraffin-embedded sections of the aortic specimens of each group (Figures 7-A,B). The black arrow was the ECs and the red arrow was the macrophages. From the staining results, it can be seen that compared with the control group, the morphology of aortic intima ECs in AD model mice was destroyed, macrophages infiltration occurred, aortic adventitia teared formed a false lumen, and a large amount of inflammation occurred around the false lumen. Compared with AD mice, after treatment with telmisartan, the morphology of mouse aortic intima ECs recovered, inflammatory cell infiltration decreased. And, some of the macrophages still infiltrated in the aorta of AD mice treated with nifedipine, the outer membrane structure scattered. Figures 7-A,C,D shows the difference in macrophage differentiation by aortic immunofluorescence in each group of mice. The double markers CD68 and CD86 represent M1 macrophages, and the double markers CD68 and CD206 represent M2 macrophages. Compared with the control group, the number of aortic macrophages in the AD model group increased, the macrophage was mainly M1 type, and the M2 type macrophages were less observed. Compared with the AD model group, after treatment with telmisartan, the proportion of M1 type macrophages in the infiltrated area decreased, and the proportion of M2 type macrophage increased. Nifedipine has the same trend of effect, but the effect is lighter than telmisartan (Figures 7-B, A,B).
Figure 7A. M1 macrophages infiltrate in the torn aortic wall in AD mice, and telmisartan treatment can increase M2 infiltration in the aortic wall. (A) Different groups of mouse aortic samples were presented. (B) HE staining of aortic tissue sections in each group. The first row is a magnified image of 100 times, and the second row is a magnified image of 400 times. The black arrow indicates the endothelial cells, and the red arrow indicates the macrophages. (C) 400-fold magnification of M1 macrophages (CD68 + /CD86 +) tissue fluorescent staining images. White arrow indicates M1 macrophages. (D) 400-fold magnification of M2 macrophages (CD68 + /CD206 +) tissue fluorescent staining images. White arrow indicates M2 macrophages.
Figure 7B. Quantification of tissue fluorescent staining images. (A) Quantification of M1 macrophages, (CD68 + /CD86 +) tissue fluorescent staining images. (B) Quantification of M2 macrophages (CD68 + /CD206 +) tissue fluorescent staining images. *P < 005 compared with control group; **P < 0.01 compared with control group; < 0.05 compared with AAD group.
Figures 8A–C shows the expression levels of serum ET-1, IL-6, and MMP 9 in each group of mice. The expression levels of ET-1, IL-6, and MMP9 in AD model mice were increased compared with control group. Compared with AAD model mice, the expression levels of serum ET-1, IL-6, and MMP 9 were decreased in mice treated with telmisartan, and also the expression levels of serum ET-1, IL-6, and MMP9 in mice after treatment with nifedipine were decreased. The telmisartan treatment group had lower levels of ET-1, IL-6, and MMP9 in the serum compared with the nifedipine group. The results of the experiment showed that telmisartan and nifedipine treatment can reduce the expression of serum ET-1, IL-6, and MMP 9, and the anti-inflammatory effect of telmisartan is better than that of nifedipine.
Figure 8. ELISA and Western Blot results of aortic tissue in each group of mice. (A) ET-1 ELISA results were shown. Both telmisartan and nifedipine treatments can effectively reduce ET-1 serum levels in the AD mice model. Telmisartan is slightly better than nifedipine. (B) IL-6 ELISA results were shown. Both telmisartan and nifedipine treatments can effectively reduce IL-6 serum levels in the AD mice model. Telmisartan is slightly better than nifedipine. (C) MMP 9 ELISA results were shown. Both telmisartan and nifedipine treatments can effectively reduce MMP 9 serum levels in the AD mice model. Telmisartan is slightly better than nifedipine. (D) Western Blot results of YAP and AT1R content in aortic tissue of each group were demonstrated. The contents of AT1R and p-YAP in the aortic tissue of AD group were significantly increased, and the total content of YAP was decreased. Telmisartan treatment can effectively alleviate this phenomenon, and nifedipine cannot affect the expression of AT1R and YAP. (n = 3, mean and S.D., t-test, *P < 0.01 compared with control group; **P < 0.05 compared with the control group; ∧∧P < 0.05 compared with the AD group; ##P < 0.05 compared with the AD + telmisartan group).
Figure 8D shows the Western Blot test results for each group of aortic tissues. The results showed that the YAP/p-YAP ratio of AD mice was decreased and the expression of AT1R protein was increased compared with the control mice. Compared with the AD model group mice, the YAP/p-YAP ratio and the AT1R protein expression were decreased in the mice treated with telmisartan. Compared with AAD model mice, treatment of AD mice with nifedipine had no significant effect on the expression of YAP, p-YAP, and AT1R proteins. From this result and the results of previous cell experiments, we can speculate that the ARB drug telmisartan can inhibit the expression of AT1R in the aortic tissue of the AAD model and reduce the phosphorylation level of YAP protein.
In this study, we showed that Ang II binding to AT1R activates YAP phosphorylation, upregulates M1 polarization, and promotes macrophage adhesion to ECs. Based on assessing biomarkers for inflammation, we found that Ang II can trigger endothelial cell inflammation by upregulating YAP phosphorylation. In addition, we showed that blocking AT1R can inhibit macrophage M1 polarization, reduce macrophage adhesion, and downregulate YAP phosphorylation. YAP knock down inhibited Ang II regulation of macrophages. Therefore, our findings confirm that Ang II binding to AT1R upregulates YAP phosphorylation, which promotes macrophage M1 polarization and adhesion, thus triggering the endothelial cell inflammatory response. At the same time, we found in animal experiments that serum inflammatory factors increased in AD mice, M1 macrophage infiltration in the aortic wall of the dissection, and the ratio of YAP/p-YAP in the tissue decreased. Telmisartan treatment can effectively alleviate the above phenomenon and effectively reduce the incidence of AD. This suggests that the AT1R-YAP pathway may play an important role in macrophage-guided intimal inflammation and contribute to AD formation. However, the specific signaling mechanism remains to be determined. Future work will explore whether the classic Hippo pathway is involved.
This study shows that Ang II can activate YAP phosphorylation by binding to AT1R, and that transfection with AT1R siRNA or treatment with the telmisartan can inhibit Ang II-induced YAP phosphorylation, which may trigger the intracellular localization of YAP from the nucleus to the cytoplasm (Wennmann et al., 2014). As known that G-protein-coupled receptor (GPCR) binding to the G protein subclass of receptors (Gαq/11) activates YAP (Yu et al., 2012), and AT1R can activates YAP in HEK293 cells (Wennmann et al., 2014), we expected AT1R to did the same regulation in macrophages. However, AT1R stimulation with Ang II resulted in a decrease in the YAP/p-YAP ratio in macrophages. Recent studies have shown that Ang II can increase endothelial cell YAP phosphorylation (Fu et al., 2019) and that angiotensin-converting enzyme 2 activation induces pulmonary artery cell apoptosis by up-regulating YAP phosphorylation, thereby attenuating pulmonary vascular remodeling (Yan et al., 2019). Furthermore, study results have shown that after silencing YAP in macrophages, M1 related markers IL-12B, IL-1β, and TNF-α were up-regulated, and the expression of M1 markers CD68 and CD86 were enhance. In contrast, overexpression of YAP in macrophages increased the mRNA levels of M2 related markers IL-10, TGF-β and VEGF-A, and the expression of M2 markers CD163 and CD204 was significantly increased (Zhang et al., 2021). Therefore, our findings correlate well with these recent studies, as they indicate that Ang II can increase YAP phosphorylation and regulate macrophage activity.
Ang II induced YAP phosphorylation in macrophages by binding to AT1R, which leads to the polarization of more M1 macrophages and adhesion of macrophages to endothelial cells, ultimately leading to enhanced inflammation and endothelial cell injury. Therefore, it is likely that Ang II causes damage to endothelial cells by regulating YAP in macrophages. This suggests that promoting YAP dephosphorylation in macrophages could help prevent artray intimal injury.
Macrophages can be induced to polarize to an M1 or M2 state in vitro, and M1 macrophage polarization can be induced by upregulating YAP phosphorylation. Our observation that the decrease in the YAP/p-YAP ratio induced by Ang II binding to AT1R induced THP-1 cells to differentiate into M1 cells is supported by previous research showing that hepatocytes ectopically expressing an artificially activated YAP protein induce macrophage M2 polarization (Guo et al., 2017; Huang et al., 2017). M1 macrophages are a type of pro-inflammatory cell, therefore, we concluded that Ang II binding to AT1R promotes inflammation by regulating YAP phosphorylation, which is consistent with the changes in inflammation-related indicators (IL-6, MMP-9, and ET-1) observed in this study. Our results are consistent with the notion that patients with AD often exhibit inflammation (del Porto et al., 2010).
In animal studies, this study found that ARB treatment did reduce the incidence of AD and was more effective than simply lowering blood pressure. This is also consistent with the results of previous research by Ju et al. (2013) etc. The incidence of AD increased by Ang II does not depend on the increase in blood pressure. In order to study the effect of AT1R on macrophages in AD model, this study did not choose to use Ang II for modeling, but instead used BAPN to make AD model. At the same time, in order to eliminate the effect of blood pressure reduction on AD, the nifedipine experimental group was set up, and the blood pressure of this group was basically consistent with the telmisartan group by regular tail pressure measurement. At the same time, we verified in the cell experiments that nifedipine has no effect on YAP in macrophages, and the data is shown in the supplement. The results in animal experiments are also consistent with the results in vitro cell experiments. ARB does reduce the infiltration of M1 macrophages in the vessel wall and increases the number of M2 macrophages in the vessel wall. This suggests that ARB attenuates the pro-inflammatory effects of macrophages in the vessel wall by blocking AT1R, and enhances repair function. In the Western Blot results, the ratio of YAP/p-YAP decreased in the aortic tear part, and this ratio was significantly higher in the telmisartan-treated group than in the AD group. These results are consistent with those in the cell experiment. This suggests that AT1R plays an important role in regulating macrophage polarization and affecting the development of AD, and is highly likely to produce effects through YAP. More in-depth in vivo mechanism research is needed in the future.
There were some limitations to this study. First, More in-depth research is needed in the future. In this study, Western Blot was used to detect mixed samples in the aortic samples, so the change of YAP in macrophages in the aortic tear parts was not accurately detected. Second, we were unable to assess the effects of YAP overexpression on macrophage polarization and adhesion, as we failed to successfully overexpress YAP. Due to the limitations of experimental conditions and financial constraints, we were not been able to complete this part of the study.
Taken together, the findings reported here provide a new foundation by studying the integrated roles that AT1R and YAP play in regulating macrophage polarization and adhesion as driving forces of vascular intimal injury. Hence, the role of YAP in macrophages will be an important focus for further study, as it could be an interesting target for pharmacological intervention in vascular intimal injury in the future.
AT1R induces YAP phosphorylation through binding to Ang II, and further promotes macrophages M1 polarization and adhesion to endothelial cells. ARB treatment effectively alleviates the above-mentioned pro-inflammatory phenomenon, reduces the incidence of AD in mice and affect macrophage polarization in mice aorta.
The raw data supporting the conclusions of this article will be made available by the authors, without undue reservation.
The animal study was reviewed and approved by the Ethics Committee of Chinese People’s Liberation Army General Hospital.
XW, LC, YH, GS, and SJ conducted the cell experiments. XW, AM, LC, and YH conducted the animal experiments. YG and JL did the data collection and analysis. XW and AM wrote this manuscript. HZ, WG, LC, YG, DR, JL, SJ, GS, and YH revised the manuscript critically. All authors participated in the design, interpretation of the studies and analysis of the data, review of the manuscript, and approved for the manuscript submission.
This study was supported by the Beijing Natural Science Foundation (7202190).
The authors declare that the research was conducted in the absence of any commercial or financial relationships that could be construed as a potential conflict of interest.
The Supplementary Material for this article can be found online at: https://www.frontiersin.org/articles/10.3389/fphys.2021.644903/full#supplementary-material
Chen, K., Varon, J., Wenker, O. C., Judge, D. K., Fromm, R. E. Jr., and Sternbach, G. L. (1997). Acute thoracic aortic dissection: the basics. J. Emerg. Med. 15, 859–867. doi: 10.1016/s0736-4679(97)00196-0
Daugherty, A., Manning, M. W., and Cassis, L. A. (2000). Angiotensin II promotes atherosclerotic lesions and aneurysms in apolipoprotein E-deficient mice. J. Clin. Invest. 105, 1605–1612. doi: 10.1172/jci7818
del Porto, F., Proietta, M., Tritapepe, L., Miraldi, F., Koverech, A., Cardelli, P., et al. (2010). Inflammation and immune response in acute aortic dissection. Ann. Med. 42, 622–629.
Ding, Y., Huang, L., Xian, X., Yuhanna, I. S., Wasser, C. R., Frotscher, M., et al. (2016). Loss of Reelin protects against atherosclerosis by reducing leukocyte-endothelial cell adhesion and lesion macrophage accumulation. Sci. Signal. 9:ra29. doi: 10.1126/scisignal.aad5578
Endlich, K., Kliewe, F., and Endlich, N. (2017). Stressed podocytes-mechanical forces, sensors, signaling and response. Pflugers Arch. 469, 937–949. doi: 10.1007/s00424-017-2025-8
Erbel, R., Aboyans, V., Boileau, C., Bossone, E., Bartolomeo, R. D., Eggebrecht, H., et al. (2014). 2014 ESC guidelines on the diagnosis and treatment of aortic diseases: document covering acute and chronic aortic diseases of the thoracic and abdominal aorta of the adult. The task force for the diagnosis and treatment of aortic diseases of the european society of cardiology (ESC). Eur. Heart J. 35, 2873–2926. doi: 10.1093/eurheartj/ehu281
Feng, X., Liu, P., Zhou, X., Li, M. T., Li, F. L., Wang, Z., et al. (2016). Thromboxane A2 activates YAP/TAZ protein to induce vascular smooth muscle cell proliferation and migration. J. Biol. Chem. 291, 18947–18958. doi: 10.1074/jbc.m116.739722
Fu, Y., Sun, S., Sun, H., Peng, J., Ma, X., Bao, L., et al. (2019). Scutellarin exerts protective effects against atherosclerosis in rats by regulating the Hippo-FOXO3A and PI3K/AKT signaling pathways. J. Cell. Physiol. 234, 18131–18145. doi: 10.1002/jcp.28446
Guo, X., Zhao, Y., Yan, H., Yang, Y., Shen, S., Dai, X., et al. (2017). Single tumor-initiating cells evade immune clearance by recruiting type II macrophages. Genes Dev. 31, 247–259. doi: 10.1101/gad.294348.116
Hiratzka, L. F., Bakris, G. L., Beckman, J. A., Bersin, R. M., Carr, V. F., and Casey, D. E. Jr., et al. (2010). 2010 ACCF/AHA/AATS/ACR/ASA/SCA/SCAI/SIR/STS/SVM guidelines for the diagnosis and management of patients with thoracic aortic disease. A report of the American college of cardiology foundation/American heart association task force on practice guidelines, American association for thoracic surgery, American college of radiology, American stroke association, society of cardiovascular anesthesiologists, society for cardiovascular angiography and interventions, society of interventional radiology, society of thoracic surgeons, and society for vascular medicine. J. Am. Coll. Cardiol. 55, e27–e129.
Huang, Y. J., Yang, C. K., Wei, P. L., Huynh, T. T., Whang-Peng, J., Meng, T. C., et al. (2017). Ovatodiolide suppresses. J. Hematol. Oncol. 10:60.
Ivanova, A. D., Tapilina, S. V., and Kuz’min, V. S. (2019). Role of muscarinic M1, M2, and M3 receptors in the regulation of electrical activity of myocardial tissue of caval veins during the early postnatal ontogeny. Bull. Exp. Biol. Med. 166, 421–425. doi: 10.1007/s10517-019-04364-9
Jia, X., Guo, W., Li, T. X., Guan, S., Yang, R. M., Liu, X. P., et al. (2013). The results of stent graft versus medication therapy for chronic type B dissection. J. Vasc. Surg. 57, 406–414. doi: 10.1016/j.jvs.2012.08.064
Ju, X., Ijaz, T., Sun, H., Ray, S., Lejeune, W., Lee, C., et al. (2013). Interleukin-6-signal transducer and activator of transcription-3 signaling mediates aortic dissections induced by angiotensin II via the T-helper lymphocyte 17-interleukin 17 axis in C57BL/6 mice. Arterioscler. Thromb Vasc. Biol. 33, 1612–1621. doi: 10.1161/atvbaha.112.301049
Kaji, S. (2018). Acute medical management of aortic dissection. Gen. Thorac. Cardiovasc. Surg. 67, 203–207.
Khayat, M., Cooper, K. J., Khaja, M. S., Gandhi, R., Bryce, Y. C., and Williams, D. M. (2018). Endovascular management of acute aortic dissection. Cardiovasc. Diagn. Ther. 8, S97–S107.
Kim, W., Khan, S. K., Liu, Y., Xu, R., Park, O., He, Y., et al. (2018). Hepatic Hippo signaling inhibits protumoural microenvironment to suppress hepatocellular carcinoma. Gut 67, 1692–1703. doi: 10.1136/gutjnl-2017-314061
Kochanek, K. D., Xu, J., Murphy, S. L., Minino, A. M., and Kung, H. C. (2011). Deaths: final data for 2009. Natl. Vital Stat. Rep. 60, 1–116.
Kurihara, T., Shimizu-Hirota, R., Shimoda, M., Adachi, T., Shimizu, H., Weiss, S. J., et al. (2012). Neutrophil-derived matrix metalloproteinase 9 triggers acute aortic dissection. Circulation 126, 3070–3080. doi: 10.1161/circulationaha.112.097097
Nienaber, C. A., and Clough, R. E. (2015). Management of acute aortic dissection. Lancet 385, 800–811.
Nurden, A. T. (2011). Platelets, inflammation and tissue regeneration. Thromb. Haemost. 105(Suppl. 1), S13–S33.
Ohno-Urabe, S., Aoki, H., Nishihara, M., Furusho, A., Hirakata, S., Nishida, N., et al. (2018). Role of macrophage Socs3 in the pathogenesis of aortic dissection. J. Am. Heart Assoc. 7:e007389.
Olsson, C., Thelin, S., Stahle, E., Ekbom, A., and Granath, F. (2006). Thoracic aortic aneurysm and dissection: increasing prevalence and improved outcomes reported in a nationwide population-based study of more than 14,000 cases from 1987 to 2002. Circulation 114, 2611–2618. doi: 10.1161/circulationaha.106.630400
Pacurari, M., Kafoury, R., Tchounwou, P. B., and Ndebele, K. (2014). The Renin-Angiotensin-aldosterone system in vascular inflammation and remodeling. Int. J. Inflam. 2014:689360.
Ren, A., Yan, G., You, B., and Sun, J. (2008). Down-regulation of mammalian sterile 20-like kinase 1 by heat shock protein 70 mediates cisplatin resistance in prostate cancer cells. Cancer Res. 68, 2266–2274. doi: 10.1158/0008-5472.can-07-6248
Schluter, K. D., and Wenzel, S. (2008). Angiotensin II: a hormone involved in and contributing to pro-hypertrophic cardiac networks and target of anti-hypertrophic cross-talks. Pharmacol. Ther. 119, 311–325. doi: 10.1016/j.pharmthera.2008.05.010
Valis, K., Prochazka, L., Boura, E., Chladova, J., Obsil, T., Rohlena, J., et al. (2011). Hippo/Mst1 stimulates transcription of the proapoptotic mediator NOXA in a FoxO1-dependent manner. Cancer Res. 71, 946–954. doi: 10.1158/0008-5472.can-10-2203
Wang, X., Zhang, H., Ge, Y., Liu, J., Rong, D., Cao, L., et al. (2020). Angiotensin type 1 receptor regulates yes-associated protein in vascular endothelial cells. Exp. Ther. Med. 19, 748–754.
Wennmann, D. O., Vollenbroker, B., Eckart, A. K., Bonse, J., Erdmann, F., Wolters, D. A., et al. (2014). The Hippo pathway is controlled by angiotensin II signaling and its reactivation induces apoptosis in podocytes. Cell Death Dis. 5:e1519. doi: 10.1038/cddis.2014.476
Yamamoto, S., Yancey, P. G., Zuo, Y., Ma, L. J., Kaseda, R., Fogo, A. B., et al. (2011). Macrophage polarization by angiotensin II-type 1 receptor aggravates renal injury-acceleration of atherosclerosis. Arterioscler. Thromb. Vasc. Biol. 31, 2856–2864. doi: 10.1161/atvbaha.111.237198
Yan, D., Li, G., Zhang, Y., and Liu, Y. (2019). Angiotensin-converting enzyme 2 activation suppresses pulmonary vascular remodeling by inducing apoptosis through the Hippo signaling pathway in rats with pulmonary arterial hypertension. Clin. Exp. Hypertens. 41, 589–598. doi: 10.1080/10641963.2019.1583247
Yu, F. X., Zhao, B., Panupinthu, N., Jewell, J. L., Lian, I., Wang, L. H., et al. (2012). Regulation of the Hippo-YAP pathway by G-protein-coupled receptor signaling. Cell 150, 780–791. doi: 10.1016/j.cell.2012.06.037
Keywords: macrophage, YAP, inflammatory, polarization, adhesion
Citation: Wang X, Zhang H, Ge Y, Cao L, He Y, Sun G, Jia S, Ma A, Liu J, Rong D and Guo W (2021) AT1R Regulates Macrophage Polarization Through YAP and Regulates Aortic Dissection Incidence. Front. Physiol. 12:644903. doi: 10.3389/fphys.2021.644903
Received: 22 December 2020; Accepted: 19 April 2021;
Published: 09 July 2021.
Edited by:
Vincenzo Lionetti, Sant’Anna School of Advanced Studies, ItalyReviewed by:
Dominic Del Re, Rutgers, The State University of New Jersey, United StatesCopyright © 2021 Wang, Zhang, Ge, Cao, He, Sun, Jia, Ma, Liu, Rong and Guo. This is an open-access article distributed under the terms of the Creative Commons Attribution License (CC BY). The use, distribution or reproduction in other forums is permitted, provided the original author(s) and the copyright owner(s) are credited and that the original publication in this journal is cited, in accordance with accepted academic practice. No use, distribution or reproduction is permitted which does not comply with these terms.
*Correspondence: Wei Guo, Z3Vvd2VpcGxhZ2hAc2luYS5jb20=
Disclaimer: All claims expressed in this article are solely those of the authors and do not necessarily represent those of their affiliated organizations, or those of the publisher, the editors and the reviewers. Any product that may be evaluated in this article or claim that may be made by its manufacturer is not guaranteed or endorsed by the publisher.
Research integrity at Frontiers
Learn more about the work of our research integrity team to safeguard the quality of each article we publish.