- 1Central Laboratory for Agricultural Climate, ARC, Giza, Egypt
- 2Department of Animal and Fish Production, Faculty of Agriculture (Saba Basha), Alexandria University, Alexandria, Egypt
- 3WorldFish, Africa Aquaculture Research and Training Center, Abbassa, Egypt
- 4National Institute of Oceanography and Fisheries (NIOF), Cairo, Egypt
The main objective of the present research was to investigate the impacts of commercial immunostimulants on growth, non-specific immune response, antioxidant enzymes, and intestinal morphometry of Nile tilapia, Oreochromis niloticus. Fish (100 ± 6.5 g) were randomly divided into five groups in triplicates (150 fish in each replicate), stocked in 20 m2 of aerated concrete ponds. The fish were fed on a control diet (30.0% crude protein) (control), and four experimental diets supplemented with Yeast Plus®, Digestarom®, and Biotronic® Top3 at 1 kg ton–1, and Sanolife PRO-F® at 0.5 kg ton–1. After the experimental period, the highest significant yield (kg m–3), mean final weight (g fish–1), average weight gain (g fish–1), and specific growth rate (% body weight day–1), were recorded in fish fed on the diet supplemented by Sanolife PRO-F, followed by Yeast Plus ponds. The lowest yield was recorded in the control group. However, the feed conversion ratio was significantly decreased with Sanolife PRO-F diet. Furthermore, the hematological analysis increased in the following ascending order: Sanolife PRO-F®; Yeast Plus®; Biotronic® Top3 and finally Digestarom® groups. The lowest concentrations of white blood cells, red blood cells, hematocrite, and hemoglobin (P ≤ 0.05) were observed in the control group. The levels of phagocytic activity and phagocytic index were significantly higher in fish fed with Sanolife PRO-F® group (P ≤ 0.05). Likewise, serum lysozyme activity was significantly highest in Sanolife PRO-F (0.63 and 0.68 U/mL, after 0.5 min and 3 min, respectively). Levels of total serum proteins, globulin, Immunoglobulin M, catalase, and super oxide dismutase were significantly higher in fish fed with Sanolife PRO-F® supplement. On the contrary, length measurement of the intestinal villus height/width, absorption area, crypt depth, and goblet cells, were significantly lower in the control group, whereas their highest values was observed in fish fed Sanolife PRO-F (P ≤ 0.05). Consequently, Sanolife PRO-F® is recommended at a level of 0.5 kg ton–1, to improve the growth performance, antioxidative capacity, and immune response of Nile tilapia.
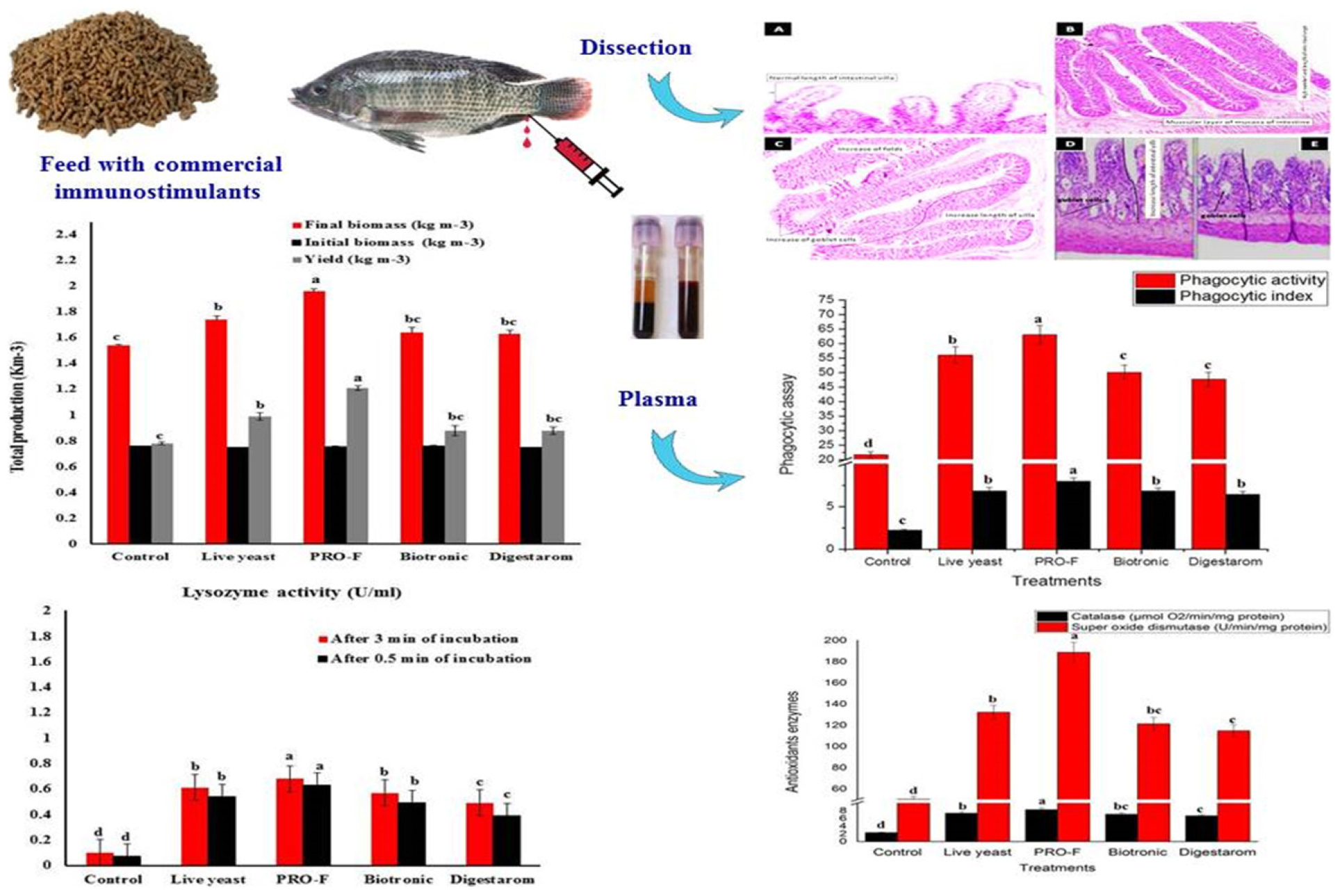
Graphical Abstract. The immunostimulatory effects of commercial feed additives on growth performance, non-specific immune response, antioxidants assay, and intestinal morphometry of Nile tilapia, O. niloticus.
Introduction
In fact, the sustainability of fish farming has been changed over the past years through intensive cultivation methods. Sustainable aquaculture development strategies are supported by assessing the effectiveness of commercial immunostimulants (prebiotics and probiotics as well as organic acids), to explore their effects for promoting growth, survival present, improving the non-specific immune response, antioxidants assay, and maintaining of intestine health (Newaj-Fyzul and Austin, 2015; Khalila et al., 2018; Ismail and Al-Hamdani, 2019).
Immunostimulant plays an essential role in increasing the growth performance of aquatic organisms via improving the efficiency of feed digestion and utilization (Ringø et al., 2012). Probiotics supplements are identified as living microorganisms from gram-negative or positive bacteria, fungi, and algae that can improve the nutritional content of the feed used to feed fish when added in appropriate quantities (Oliveira et al., 2017). Scientists used commercial probiotics, such as; Bacillus spp., Streptococcus, Lactococcus lactis, Pseudomonas fluorescens, Vibrio alginolyticus, Saccharomyces cerevisiae, Debrayomyces hansenii, Spirulina, to enhance growth performance and immune response over past years in juvenile Nile tilapia, Oreochromis niloticus (Abdel-Tawwab et al., 2008; Abdel-Tawwab and Ahmad, 2009; Abdel-Tawwab, 2012). They give many benefits, such as increasing the rate of nutritional conversion of feed, improving growth efficiency via improving the balance of gastrointestinal microbial communities, as well as increasing the creation of supplemental digestive enzymes and increasing immune system responses, against pathogenic bacteria by producing inhibitory compounds (Bozkurt et al., 2014; Amenyogbe et al., 2020). Besides, the beneficial effect of organic acids as a functional feed additive on digestion track, gut microbiota and morphology, gut pH, growth, nutrient utilization, feed palatability, and hepatopancreas pathophysiology for Nile tilapia, O. niloticus (Ng and Koh, 2017; Abd El-Naby et al., 2019; Abdel-Tawwab et al., 2020a). Elsabagh et al. (2018) and Amenyogbe et al. (2020) stated that the addition of commercial immunostimulants in aquatic-feeds is expected to increase substantially in the coming years due to changing global regulatory controls that attempt to provide more sustainable aquaculture via enhancing growth capacity, feeding efficiency, and immune responses as well as reducing the intestinal inflammation in Nile tilapia, O. niloticus.
Nile tilapia, O. niloticus, became one of the most important freshwater fish in Egypt and many other countries all over the world (El-Sayed, 2019; Allam et al., 2020). The present study attempts to link between the feed additives industry sector and the sustainability of applied aquaculture strategies and farm biosecurity by evaluating commercial immunostimulants (prebiotics and probiotics as well as organic acids) to find out which one is the most effective to apply and thus to improve the growth performance. Therefore, the current study was performed to explore the impacts of commercial immunostimulants (Yeast Plus®, Sanolife PRO-F®, Biotronic® Top3, and Digestarom®) on total production per cubic meter, growth efficiency, non-specific immune response, antioxidants assay, and maintenance of intestine health of Nile tilapia, O. niloticus.
Materials and Methods
Experimental Diets
The control diet consists of 30% crude protein and 4% crude lipid to meet the requirements of Nile tilapia. One of the four feed additives were added to 1 kg of the control diet, prepared according to the guides of the manufacturer, at levels of 0.5 g feed Sanolife PRO-F®, 1 g Yeast Plus®, 1 g Biotronic® Top3, and 1 g Digestarom®. All diets were prepared by mixing the feed ingredients with 100 ml of water thoroughly. After that, ingredients are placed in a pelletizer on die 4 mm diameter, and then dried in the oven at 60°C. Diets were kept in the refrigerator (4°C) until use. Ingredients and proximate chemical composition of the experimental diets presented in Table 1.
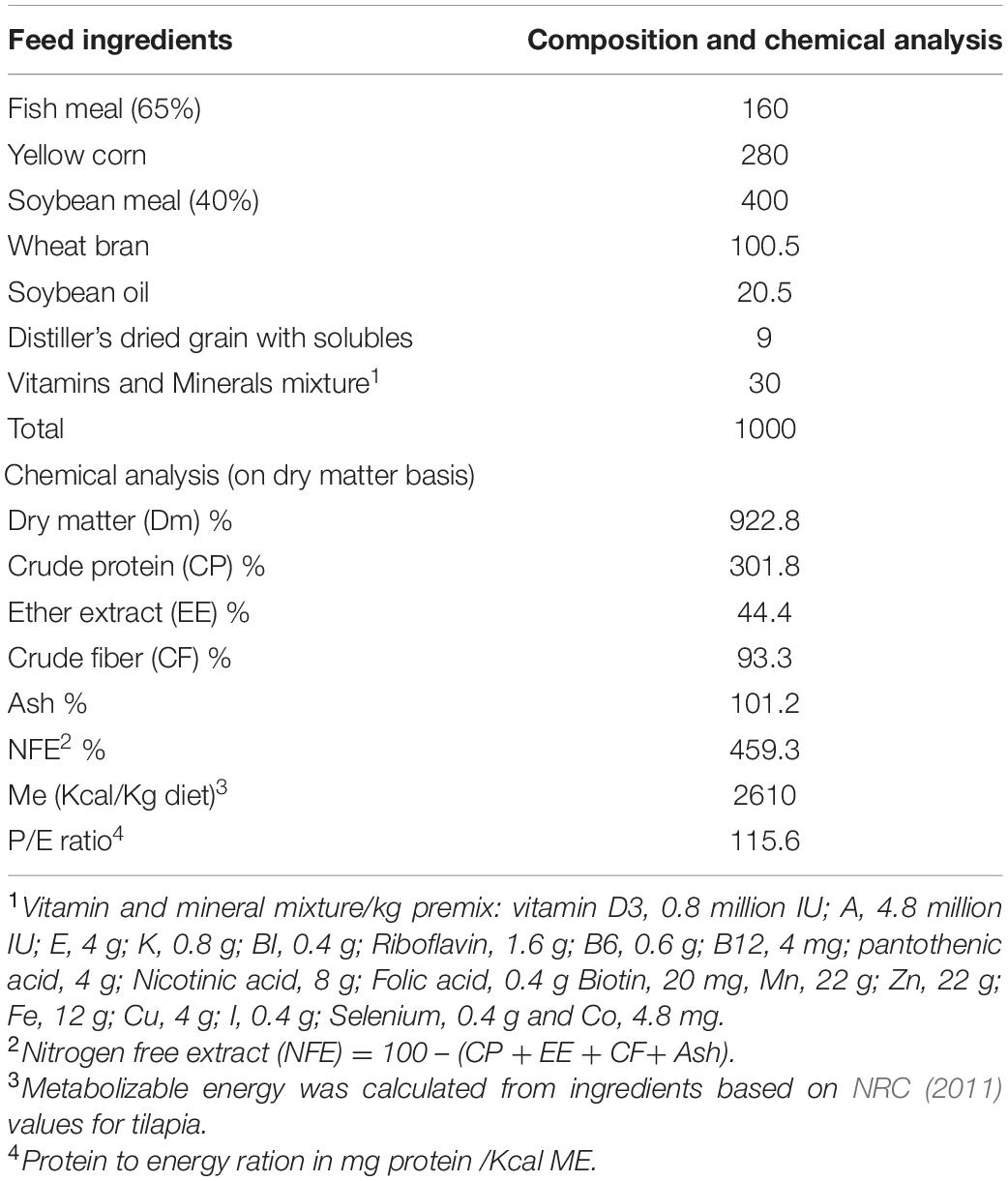
Table 1. Ingredients and proximate chemical composition of the experimental diet without supplementation (g kg–1 on dry matter basis).
Feed Supplements
Yeast Plus® produced by Angel Yeast Egypt Company Ltd., Egypt, which contains Saccharomyces cerevisiae (2.21 × 1012 CFU/g), Lactobacillus sp. (2 × 1012 CFU/g); the recommended dose is 1 kg ton–1. Sanolife PRO-F® is a blend of Bacillus strains, i.e., Bacillus subtilis (3.25 × 109 CFU/g), Bacillus licheniformis (3.50 × 109 CFU/g), Bacillus pumilus (3.25 × 109 CFU/g) produced by INVE Aquaculture, Belgium, with a total number 1.0 × 1010 CFU/g, the recommended dose is 0.5 kg ton–1. Biotronic® Top3 produced by Biomin GmbH, Austria, which contains 99 g formic acid (85%), 145 g ammonium formate (35%), 100 g acetic acid (99.5%), 50 g propionic acid (99.5%), 40 g fumaric acid (85%), and vermiculite up to 1 kg; the recommended dose is 1 kg ton–1. Digestarom® as digestive enzymes produced by Biomin, GmbH, Austria, that contained active components: 1-Carvon, Anethole, and 1-Menthol, used in the diet of aquatic animals, like shrimp and fish; the recommended dose is 1 kg ton–1.
Experimental Fish and Facilities
Healthy Nile tilapia fingerlings were obtained from the nursery earthen pond in a private fish farm in Kafr-El-Sheikh governorate. Fish were stocked in 20 m–3 concrete ponds (2.5 m width × 8 m length × 0.90 m height), fed on the control diet (30% crude protein), and acclimatized for 14 days prior to the experiment. After acclimation, fish (100 ± 6.5 g) were randomly distributed into fifteen aerated concrete ponds at a density of 50 fish pond–1. The experimental ponds were divided into five treatments with three triplicate groups for each. Ponds were siphoned and supplied with a running freshwater before the first feeding.
Fish in each pond were fed on 3% of the total live body weight of the fish, and were regulated properly according to Eurell et al. (1978). Every 2 weeks, the total fish consumption in each pond were collected, weighted and the total amount of feed consumed by the fish was adjusted accordingly. The daily amount of feed was administrated 6 days week–1, two equal meals per day, for 8 weeks on two occasions (9.00 am and 3.00 pm). Moreover, fish mortality was recorded, and thus the quantity of feed was readjusted accordingly.
Physico-Chemical Analyses of Water Samples
Water samples were taken from each experimental pond for quality evaluation during 8 weeks. The pH, temperature (°C), and dissolved oxygen (mg L–1) were measured using a multiparametric equipment HANNA (HI 9829). In the meantime, nitrogen compounds, such as unionized ammonia (NH3; mg L–1), nitrites (NO2; mg L–1), and nitrates (NO3; mg L–1) were analyzed and measured using a DREL spectrophotometer 2000 (HACH Company, Loveland, CO, United States) (Boyd, 1990).
Calculated Parameters
Growth performance was determined at the end of the experiment (8 weeks), including total biomass gain (TBG), weight gain (IAWG, g), feed conversion ratio (FCR), and fish survival (%) were calculated as follows:
Total biomass gain (yield; kg m–3) = [(Final biomass 58 – Initial biomass 0) / water volume (m3)];
Individual weight gain (IWG, g fish–1) = [(FW – IW0) / number of fish];
Specific growth rate (SGR; %/day) = 100 [Ln (FW) – Ln (IW0)] / days of the experiment;
Feed conversion ratio (FCR) = [Feed intake (g) / Weight gain (g)];
Fish survival (%) = 100 [(Initial Population – Final Population) / Initial Population].
Bleeding and Serum Samples Collection
At the end of the experiment, prior to weighing and blooding samples were taken, the fish were anesthetized using clove oil (5 mL L–1), five fish from each pond were anesthetized and blood samples were drawn from through the caudal vessels of the treated fish. Another portion of blood sample was drawn with a syringe containing anticoagulants (0.1 ml of sodium citrate solution) and used to determine white blood cells (WBC), red blood cells (RBC), hematocrit (Ht), and hemoglobin (Hb) as well as phagocytosis. The remaining blood sample was transferred to Eppendorf tubes, without anticoagulant, and centrifuged for 10 min at 2000 × g. Serum was collected by a micropipette, and then stored in Eppendorf tubes at −20°C until blood parameters, serum lysozyme activity, and different immune parameters as well as different biomarkers were analyzed.
Determination of Hematological Parameters
Total red blood cell (RBCs) and white blood cell (WBCs) count as well as the packed cell volume (PCV), were determined manually using a Neubauer’s haemocytometer with Hayem solution as diluent. WBC percentages were determined by counting 1500 and 200 cells, respectively. Hemoglobin concentration (Hb) was determined via using a hemoglobin reagent set (Zeist Chem Diagnostics), according to the cyanomethaemoglobin method of Drabkin (1945).
Determination of Phagocytic Activity and Index
Yeast strain: The department of poultry and fish diseases, Faculty of Veterinary Medicine, Alexandria University, was supplied Candida albicans strain that was used for phagocytic assay study according to Kawahara et al. (1991).
Phagocytic activity (PA) = phagocytic cells % containing yeast cells.
Phagocytic index (PI) = Yeast cells phagocytized numbers / Phagocytic cells numbers.
Determination of Immunological Parameters
Total proteins in fish serum as well as serum albumin were determined according to Doumas et al. (1981), using commercial kits produced by Pasteur, Lab of Chemroy. Serum globulin was determined by subtracting the total serum albumin from total serum protein according to Kumar et al. (2005). Total Immunoglobulin M assay was conducted in fish serum according to the previously mentioned procedure Milla et al. (2010).
Serum lysozyme activity was determined according to Ellis (1990), methodology using a Micrococcus lysodeikticus lysis. In brief, 0.02% of M. lysodeikticus was lyophilized at 0.05 mM, using phosphate buffer (pH 6.4) as a substrate, 10 μL of fish serum was added to 250 μL of bacterial suspension in duplicate wells of a U-bottom microtitre plate and absorbance reduction was determined at 480 nm, after 0.5 and 4.5 min of incubation at 23°C, using a microplate reader.
Determination of Antioxidants Enzymes in Serum
Catalase activity was determined according to Claiborne (1985) methodology, using a mixture of 19 mM hydrogen peroxide, 50 mM of potassium phosphate buffer, 10% PMS and 7.4 pH, under 25°C, while the absorption change was recorded at 240 nm. Super oxide dismutase activity was determined according to Payá et al. (1992) methodology, with some modifications Peixoto et al. (2006), using a detection molecule instead of cytochrome c, as nitrotetrazolium blue chloride (NBC), via mixture of (10 mM) hypoxanthine, (10 mM) NBT, and (100 mM, pH 7.0) potassium phosphate. The reaction was performed by control to inhibit 50% reduction of nitrotetrazolium blue chloride.
Intestinal Morphometric Analysis
The intestinal tract was collected from the fish of the experimental groups as well as the control group and rapidly placed in a 10% formalin buffer for 24 h. From each part, fifteen sections were subjected to histomorphometric analysis, in order to estimate the intestinal perimeter ratio (arbitrary units; AU) according to Dimitroglou et al. (2009). The villi length/width were determined, and the number of intestinal goblet cells was counted and the average number of goblet cells was determined according to Samanya and Yamauchi (2002).
Statistical Analysis
The data obtained were analyzed statistically via a fully randomized design by one-way ANOVA, using SPSS (Standard version 17.0; SPSS, Chicago, IL, United States). Tukey’s test was used as a post-hoc test to analyze the variations between mean values at p ≤ 0.05 levels (Dytham, 2011). Values were presented as the mean value ± SE of replicates.
Results
Physico-Chemical Parameters of Water Quality
The mean values of water quality parameters were 5.47 ± 0.31 mg L–1 for DO, 7.62 ± 0.27 for pH, 0.03 ± 0.001 mg L–1 for unionized ammonia, 0.31 ± 0.029 mg L–1 for nitrite, and 3.17 ± 0.37 mg L–1 for nitrate on day zero. Water quality parameters at the end of the experiment are shown in Table 2. The ponds were supplemented by Sanolife PRO-F® and Yeast Plus® in the feeds, and showed improvements in all water quality parameters compared to Biotronic®, Digestarom® and control ponds.
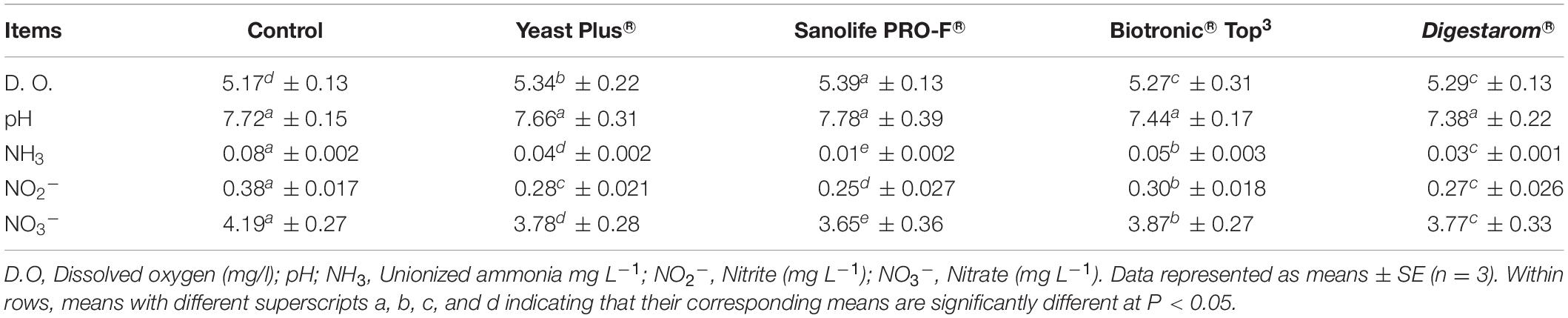
Table 2. Effect of commercial immunostimulants on water quality parameters in different groups of Nile tilapia, O. niloticus after 8 weeks.
Growth Performance and Feed Utilization Parameters
After 8 weeks of feeding trial, there were significant changes in fish growth indices and total production of Nile tilapia (P < 0.05; Figure 1 and Table 3). The highest significant yield (kg m–3), final average weight (g fish–1), IWG (g fish–1), and SGR (% body weight day–1) were recorded in fish fed on the diet supplemented by Sanolife PRO-F® followed by that received Yeast Plus®, while the lowest performance was observed on the control group. However, the feed conversion ratio was significantly decreased with the Sanolife PRO-F diet. There were no significant differences in the fish survival among all treatments.
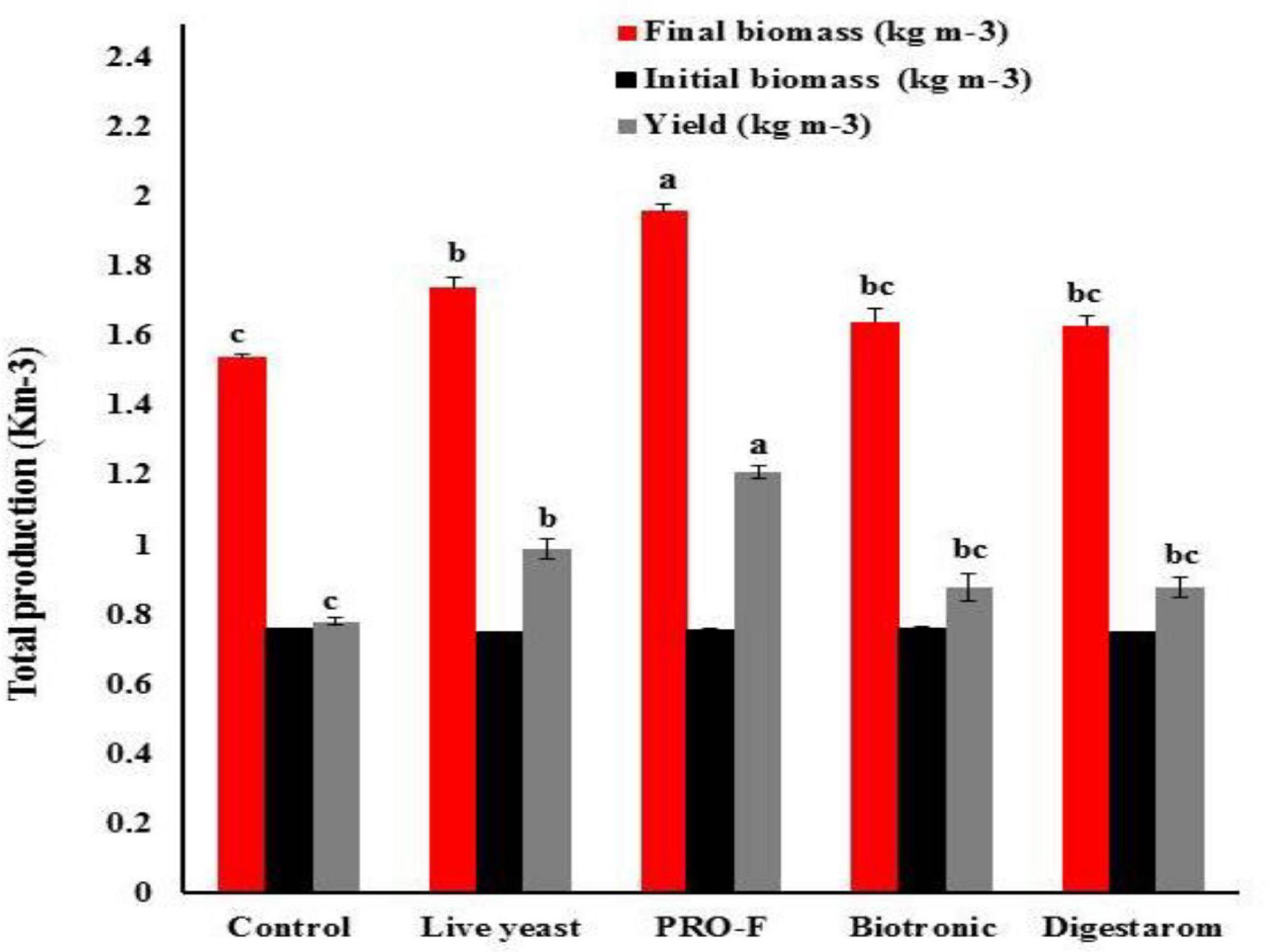
Figure 1. Total production of Nile tilapia, O. niloticus fed diets with commercial immunostimulants for 8 weeks. Bars superscripted by different letters within the same axis are significantly different (P < 0.05), (n = 3; means ± SE).
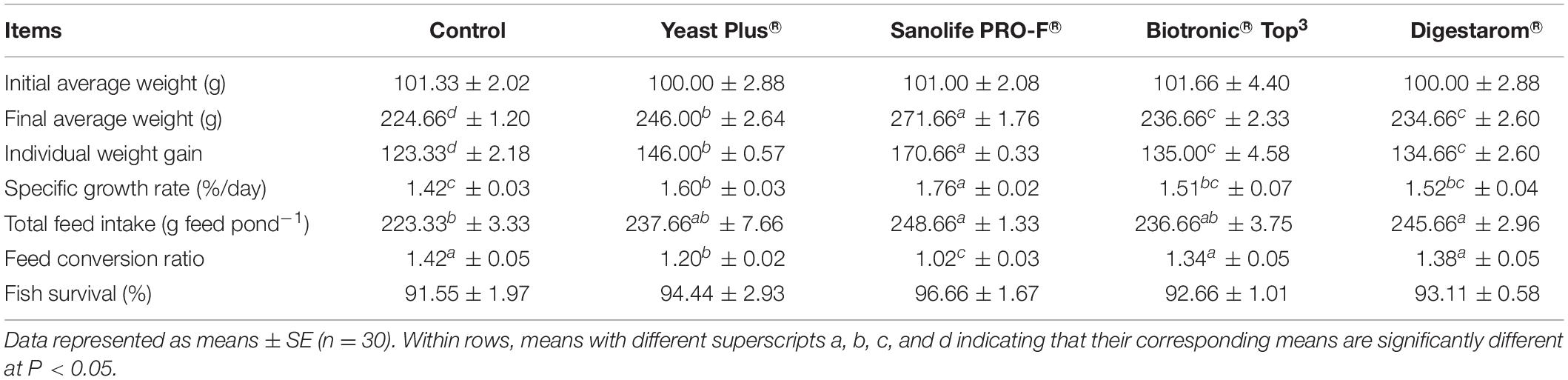
Table 3. Effect of commercial immunostimulants on growth performance of Nile tilapia, O. niloticus for 8 weeks.
Hematological Parameters
Values of RBCs, WBCs, Hb, and PCV of the experimental fish are shown in Table 4. These parameters significantly (P < 0.05) increased in the following ascending order: Sanolife PRO-F®; Yeast Plus®; Biotronic Top3 and finally Digestarom® groups. The lowest RBCs, WBCs, Hb, and PCV levels (p ≤ 0.05) were observed in the control diet.

Table 4. Changes in hematological parameters of Nile tilapia, O. niloticus fed on diets with commercial immunostimulants for 8 weeks.
Immune Parameters
It is observed that phagocytic activity as well as phagocytic index in experimental fish supplemented with Sanolife PRO-F® were significantly highest as their values were 63.00 ± 1.00% and 7.98 ± 0.03, respectively, compared to all other treatments (Figure 2), Additionally, serum lysozyme in fish supplemented with Sanolife PRO-F was significantly higher compared to all other treatments after 0.5 and 3 min of incubation in O. niloticus (Figure 3).
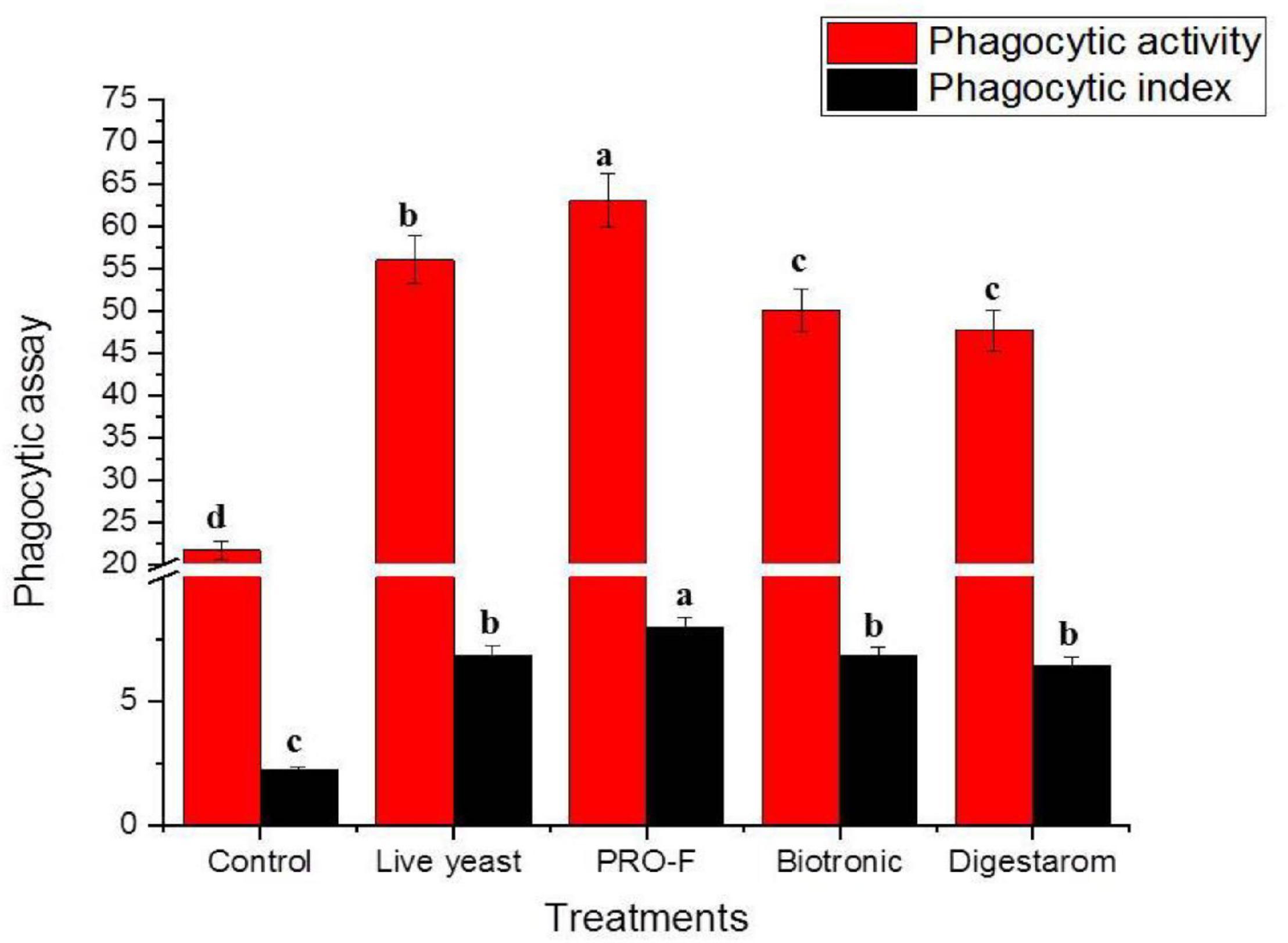
Figure 2. Phagocytic activity % and phagocytic index of Nile tilapia, O. niloticus, fed diets with commercial immunostimulants for 8 weeks. Bars superscripted by different letters within the same axis are significantly different (P < 0.05), (n = 15; means ± SE).
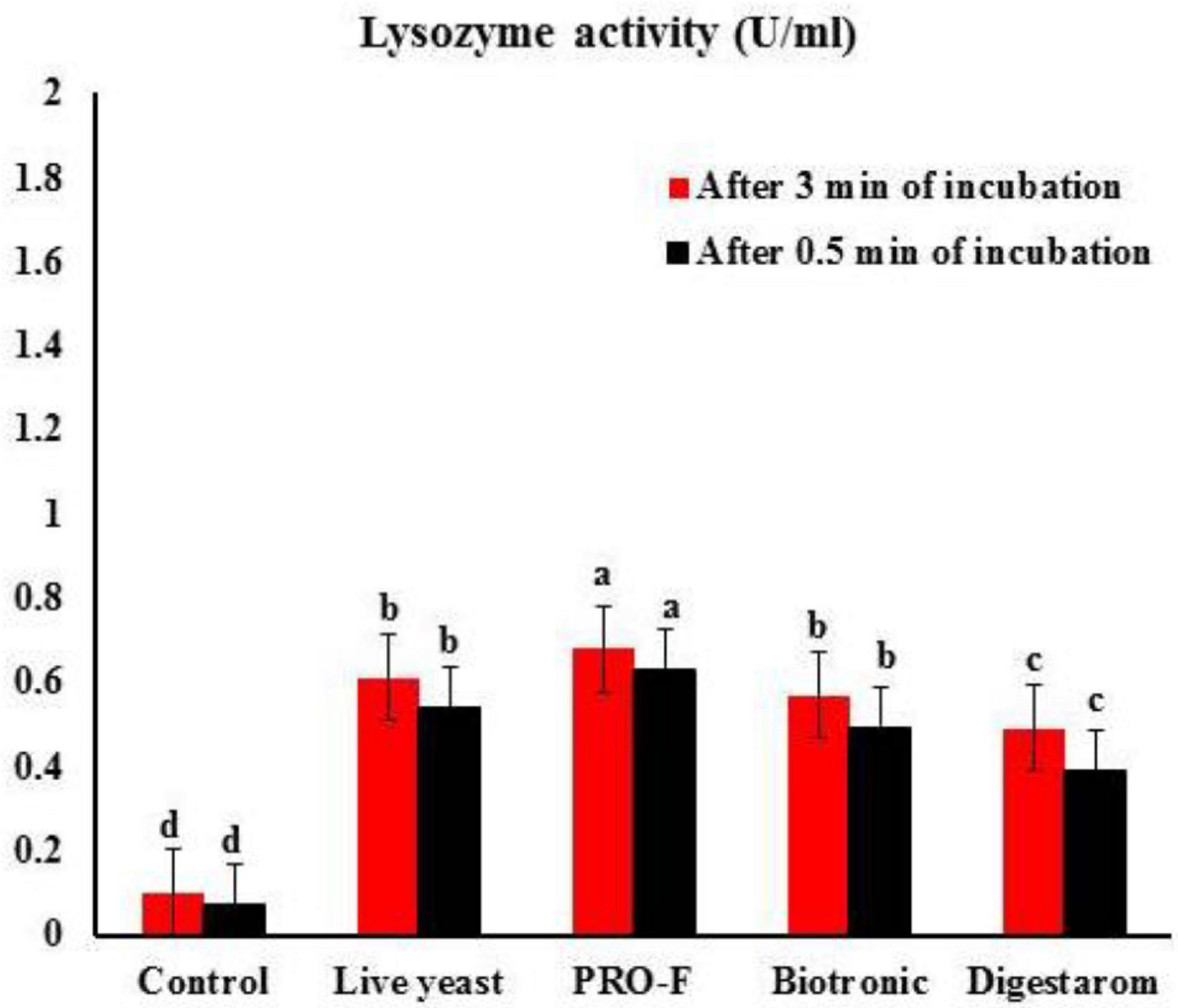
Figure 3. Lysozyme activity after 0.5 min and 3 min of incubation in Nile tilapia, O. niloticus fed diets with commercial immunostimulants for 8 weeks. Bars superscripted by different letters within the same axis are significantly different (P < 0.05), (n = 15; means ± SE).
It is worth noting that total protein, and globulin showed significant increased values in fish group fed diet supplemented by Sanolife PRO-F® (Table 5). In regard to the IgM levels in Nile tilapia, O. niloticus, the best values were obtained in the group supplemented with Sanolife PRO-F®, compared to the other fish groups (Figure 4).

Table 5. Serum total protein, albumin, globulin, and Alb./Glob. ratio in Nile tilapia, O. niloticus fed on diets with commercial immunostimulants for 8 weeks.
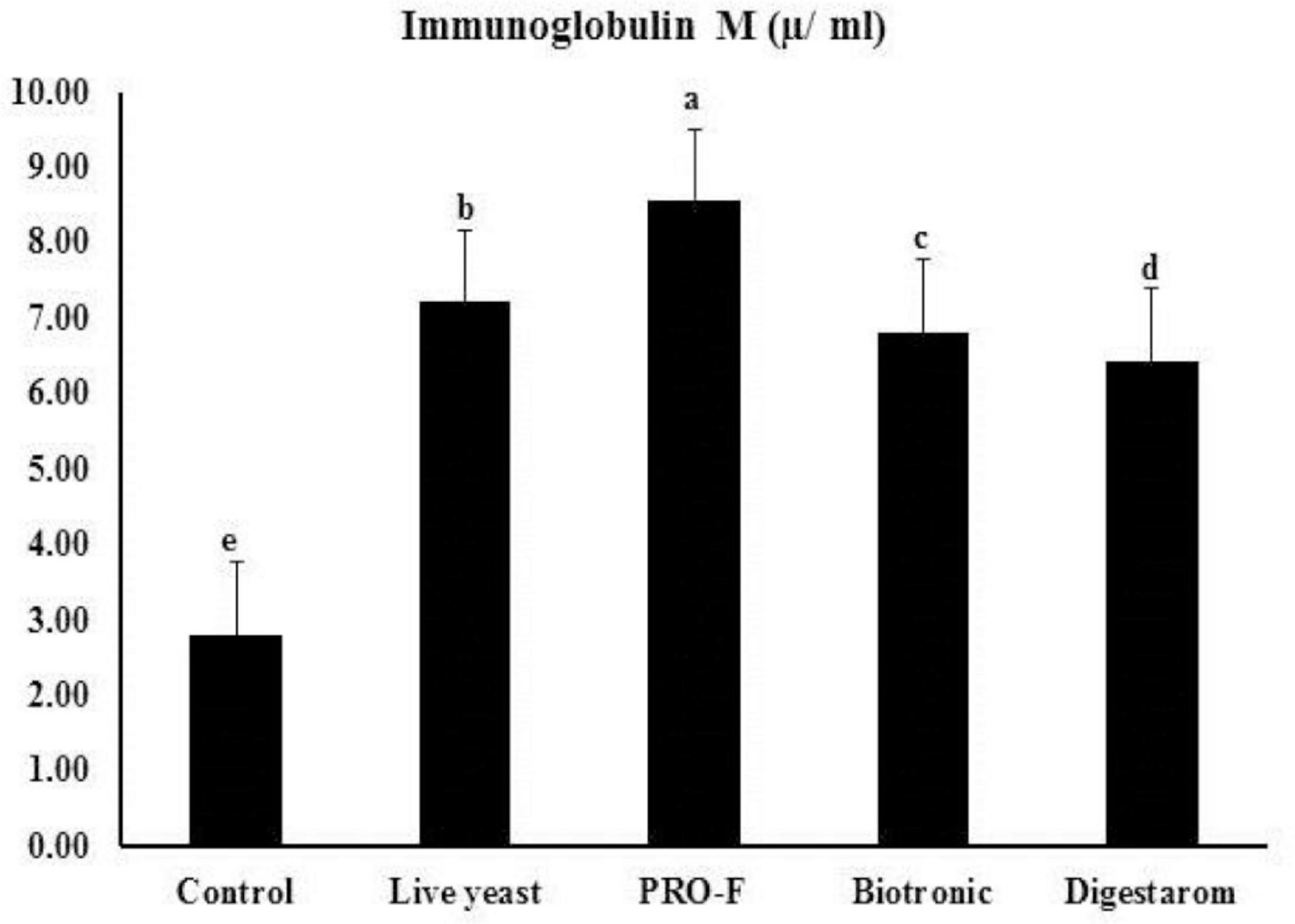
Figure 4. Immunoglobulin M (μ ml– 1) activity of Nile tilapia, O. niloticus fed diets with commercial immunostimulants for 8 weeks. Bars superscripted by different letters within the same axis are significantly different (P < 0.05), (n = 15; means ± SE).
Antioxidants Enzyme Assay
The best results for CAT and SOD levels were recorded in Nile tilapia, O. niloticus supplemented with Sanolife PRO-F (Figure 5). The lowest values were observed in the control fish group.
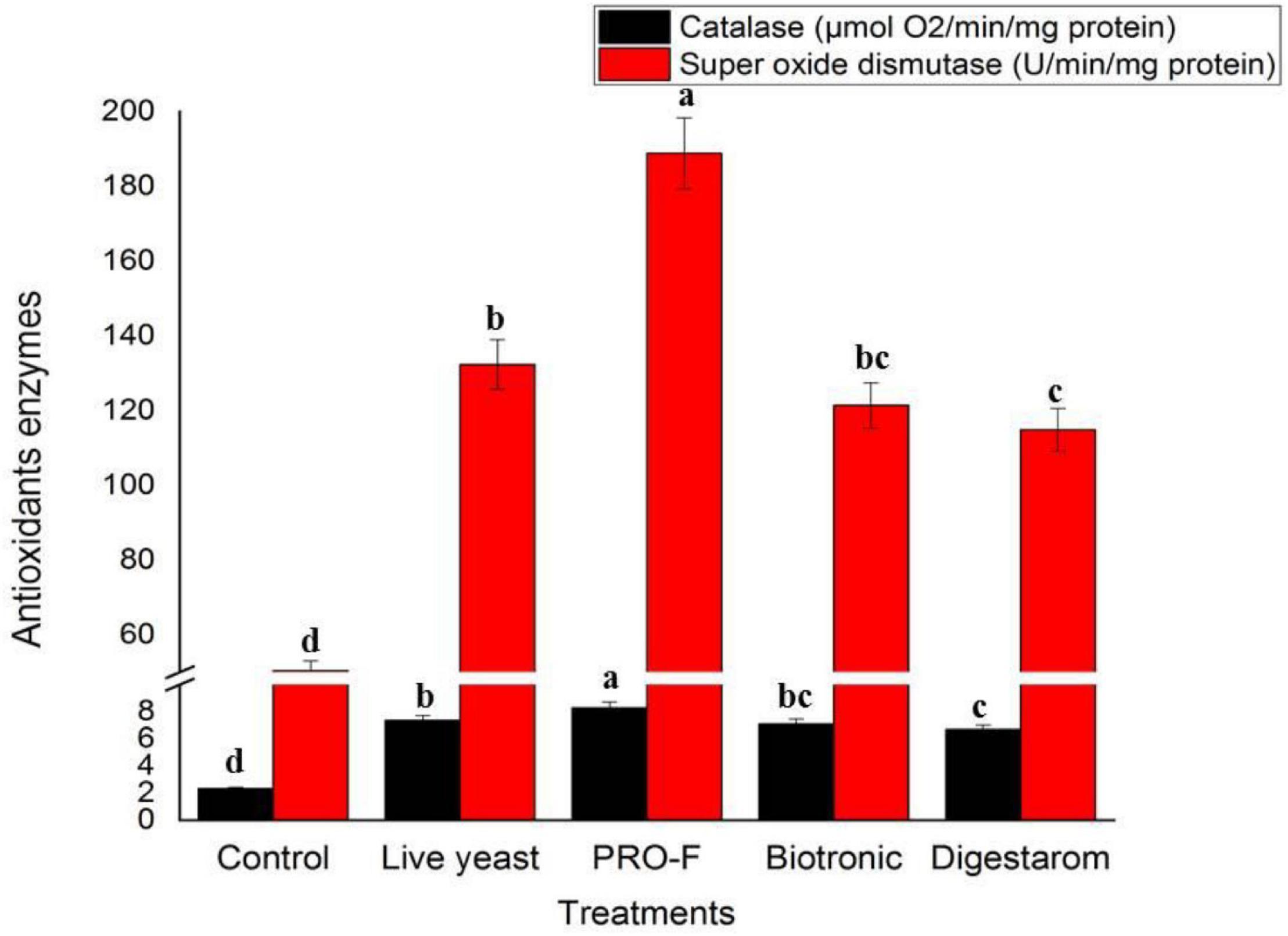
Figure 5. Activities of serum CAT and SOD of Nile tilapia, O. niloticus fed diets with commercial immunostimulants for 8 weeks. Bars superscripted by different letters within the same axis are significantly different (P < 0.05), (n = 15; means ± SE).
Morphometric Analysis of Intestinal Tract
The results of the morphological analysis of intestinal villi height/width, crypt depth as well as number of goblet cells are summarized in Table 6 and Figures 6A–E. The best intestinal villi height/width, crypt depth, and number of goblet cells were recorded in fish groups supplemented with Sanolife PRO-F®, Yeast Plus®, and Biotronic® Top3. The epithelium lining the intestine was simple columnar cells, which contain enterocytes, goblet cells and scattered ciliated cells. The length of the intestinal villi, in the anterior and terminal parts of the intestine, was significantly increased with the Sanolife PRO-F® and live Yeast® groups. The number of PAS-positive goblet cells was also significantly increased in the Sanolife PRO-F® and Yeast Plus® groups.

Table 6. Morphometric analysis of intestinal tract in Nile tilapia, O. niloticus fed diets with commercial immunostimulants for 8 weeks.
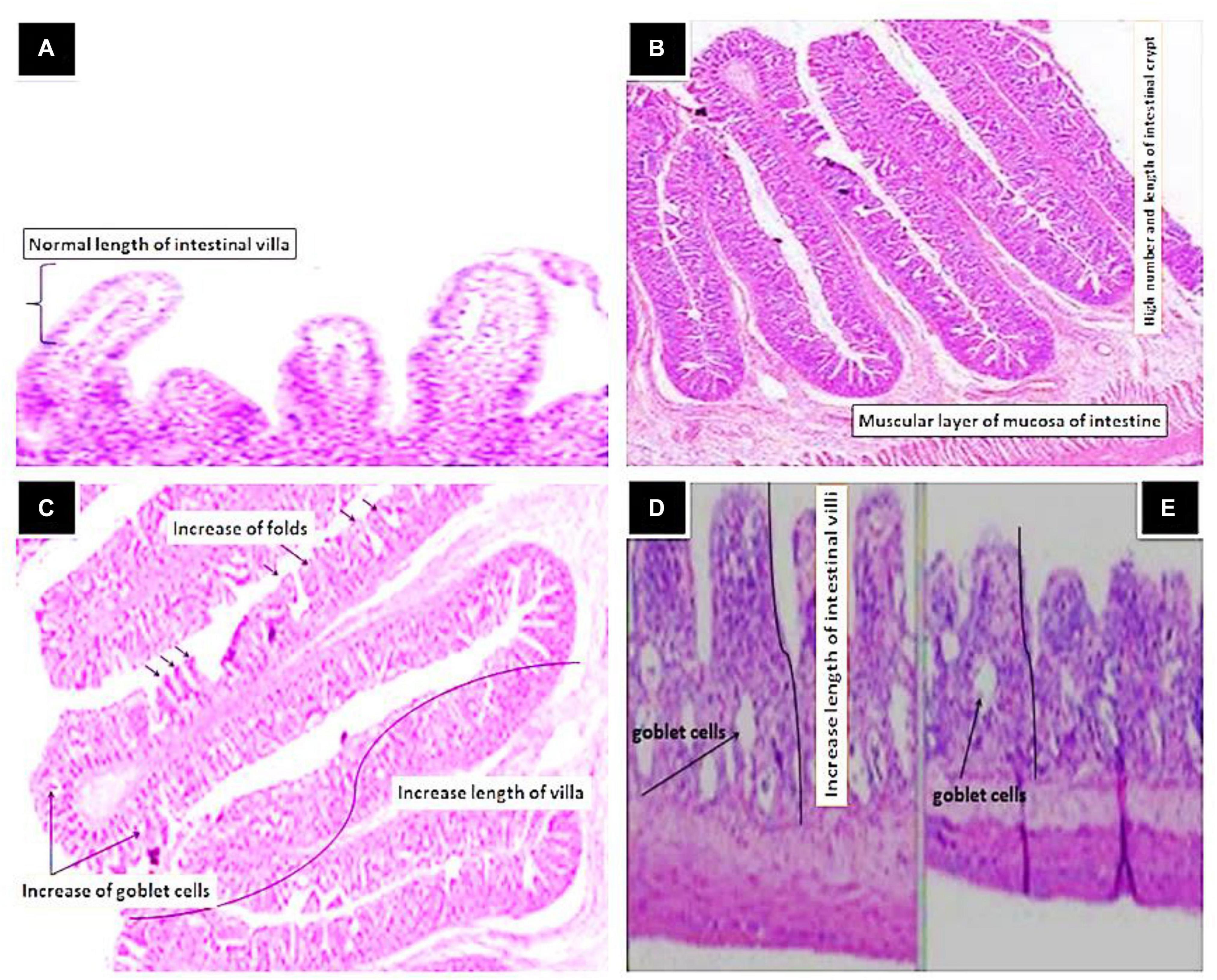
Figure 6. Histological section of Intestinal tract of Nile tilapia, O. niloticus fed Control (A) showing normal length of intestinal villi and crypt (H&E × 20), Yeast Plus® (B) showing in length of villi and mucosal folds and high number of intestinal crypt (Arrow), Sanolife PRO-F® (C) showing increase of the mucosal folds and high numbers of goblet cells as well as increase in length of intestinal villi and high number of intestinal crypt (Arrow), Biotronic® Top3 (D) and Digestarom® (E) showing slight activation of goblet cells in the lamina propria and slight increase length of intestine villi (Arrows) (H&E × 20).
Discussion
The current study evaluated the use of different immunostimulants as dietary supplementations for O. niloticus on growth performance, total production Kg m–3, and immune response.
Water Quality Assessments
In the current study, feed additives of PRO-F® and Yeast Plus® treatments showed significant improvements in all water quality parameters as compared with the control ponds. These findings may be due to certain probiotic mixtures of Bacillus sp., which generate groups of exogenous enzymes that broken away digested nutrients (Farzanfar, 2006; Liu L. et al., 2012; Tran et al., 2016). Furthermore, the use of healthy microbes (bacteria) and plankton in fish culture helps to improve water quality parameters, that provides a good environment for increasing rates of growth and maintaining fish health (El-Haroun et al., 2006; Gobi et al., 2018; Joffre and Verdegem, 2019; Abdel-Tawwab et al., 2020b). In addition, the microbiota excreted with fish feces contribute to improved positive bacterial species that strengthen the parameters of water quality (Verschuere et al., 2000; Balcázar et al., 2006; Bosma and Verdegem, 2011).
Growth Performance and Feed Utilization
According to the current study, the highest increase in growth performance and total production per m3 were recorded in fish feed on diets supplemented with PRO-F followed by live yeast. These findings show that both immunostimulants have a beneficial impact on growth efficiency rates and make aquaculture more sustainable (Froese, 2006; Sun et al., 2010; Yossa et al., 2018). The obtained results may be due to the increase of digestive enzymes that contribute to improved nutrition and enhanced intestinal absorption, thereby boosting fish growth (Abdel-Tawwab et al., 2008, 2020b; Abdel-Tawwab and Ahmad, 2009; Abdel-Tawwab, 2012).
Hematological Parameters
Blood measurements are indeed a crucial factor in determining nutritional suitability, concentrations and toxicity, as well as their impact on the circulatory system in aquatic animals (Hari et al., 2004; Dash et al., 2015). In the current study, all blood parameters; RBC, WBC, Hb, and PCV, were improved by feed additives of probiotics, prebiotics, and organic acids compared to the control diet without supplements. The hematological parameters were improved during experiment as they were affected by feed additives (Nayak et al., 2007; Ramesh et al., 2015). It is clearly evident that there is an increase in hemoglobin, and hematocrit in Nile tilapia, O. niloticus, which fed on Bacillus amyloliquefaciens (Reda and Selim, 2015), as well as increased white blood cell in Labeo rohita (Ham.) which subjected on Bacillus subtilis diets (Kumar et al., 2008).
Immune Parameters
Immunostimulants supplementation has been shown to improve fish immunity by stimulating cellular and muscular immune function (Kim et al., 2015; Kuebutornye et al., 2019). These results were confirmed through the immunological aspect of blood lysosome activity, total protein, globulin, immunoglobulin M, and antioxidative enzymes of O. niloticus. In general, phagocytic activity and index were improved when fish were fed on probiotics, prebiotics, and organic acids containing diets compared to the control fish, due to the antimicrobial compounds generated from the immunostimulants (Rahman et al., 2018), and to the improve of the natural complement, phagocytic activities, and serum peroxidase (Salinas et al., 2008; Ramesh et al., 2015). These results potentially indicate to the contribution of Bacillus and yeast-based probiotic administration in enhancing and promoting the phagocytic activity as well as the phagocytic index of Nile tilapia (Telli et al., 2014; Liu et al., 2017).
Lysozyme is a basic humoral immune defense factor typically developed in aquatic animals when it targets bacterial peptidoglycans, often Gram-positive bacteria, in the cell membrane, culminating in the stimulation of bacterial phagocytosis by phagocytic cells (Magnadottir et al., 2005; Selim and Reda, 2015). In addition, Zhou et al. (2010) found that the probiotics improved lysozyme activity in the probiotic group with a significant difference higher than in the control group. The present findings revealed a substantial improvement significantly in lysozyme. These findings align with Aly et al. (2008) and Liu C.H. et al. (2012) who discovered that fish fed on diets supplemented by Bacillus sp. was substantially higher lysozyme activity than those in fish fed a control diet for 4 weeks. Studies using yeast as a dietary additive to strengthen the innate immunity of Nile tilapia also support these findings (Abdel-Tawwab et al., 2008, 2020b; Abdel-Tawwab, 2012; Khalila et al., 2018).
Serum parameters are considered to become the main factor in evaluating the immune function and immune system response in fish species (Mulcahy, 1971; Van Hai, 2015). It is worth noted that total proteins, globulin, and immunoglobulin M levels showed increased values in the groups fed on diets with probiotics and prebiotics at the end of the experiment. Likewise, there is an improvement of globulin seen in probiotics dependent on Bacilli (Reda and Selim, 2015) and yeast–based diets that fed to Nile tilapia (Abdel-Tawwab et al., 2008, 2020a, 2020b; Abdel-Tawwab, 2012). Microorganisms used throughout the tilapia culture have been shown to be successful improving in the autoimmune reaction, including such B. subtilis, Saccharomyces cerevisiae, Lactobacillus acidophilus, and Bacillus amyloliquefaciens (Abdel-Tawwab et al., 2008; Selim and Reda, 2015; Abou-El-Atta et al., 2019; Kuebutornye et al., 2019).
Antioxidants Activity
The results revealed that CAT and SOD activity significantly increased in fish groups fed on diets supplemented with probiotics and prebiotics. Similarly, Guardiola et al. (2016) found that the antioxidant activity of Pediococcus acidilactici was observed to cause regulation of the CAT and SOD activities as feed supplements in aqua-feeds. These results may be due to functions by releasing stopped oxygen levels (ROS) free radicals, inducing oxidative stress which can interrupt and influence metabolic processes and thereby destroy cell groups. Each condition that raises the accumulation of ROS is considered oxidative stress, which can contribute to the disturbance and control of mitochondrial function and thereby damage cell components (Abdel-Tawwab and Wafeek, 2017; Mansour et al., 2017; Gobi et al., 2018).
Morphometric Analysis of Intestinal Tract
Gut morphology is a crucial factor affected by immunostimulant diets and regulates metabolic physiology, resulting in improved digestion and absorption of nutrients, resulting in improved growth efficiency in fish (Cerezuela et al., 2013; Hoseinifar et al., 2014; Adeshina et al., 2019; Sewaka et al., 2019). In the current research, the progress of intestinal morphometrics led to the increased digestion and absorption of nutrients after feeding on probiotics, prebiotics, and organic acids as dietary feed supplements. These indicate to a rise in the region of enterocyte absorption contributing to improved growth efficiency (Ferguson et al., 2010; Giatsis et al., 2016). The present study indicated that the best morphometry of the intestine, crypt depth, and goblet cell count, in current the experiment, were recorded in the fish groups fed on diets supplemented with PRO-F® and Yeast Plus®. The elevation of intestinal villus height/length relative to the control group showed a statistically significant improvement that could increase surface intestinal absorption, boost nutrient absorption, and consequently improve growth efficiency (Sun et al., 2010; Pirarat et al., 2011; Yossa et al., 2018; Khalil et al., 2019).
Conclusion
The current study found an improving effects on growth performance, nutrient utilization, hemato-biochemical, non-specific immune parameters, antioxidant enzyme, and the intestinal morphometric of Nile tilapia, O. niloticus fed on a diet supplemented with Sanolife PRO-F® or Yeast Plus® at 0.5 or 1.0 kg ton–1, respectively.
Data Availability Statement
The raw data supporting the conclusions of this article will be made available by the authors, without undue reservation.
Ethics Statement
The animal study was reviewed and approved by the (ALEXU-IACUC) Institutional Animal Care and Use Committee AU: 14/07/20/2/7. Written informed consent was obtained from the owners for the participation of their animals in this study.
Author Contributions
MK: data curation, methodology, software, visualization, writing, and original draft preparation. TS: investigation, monitoring, and supervision. EO: investigation, monitoring, and reviewing. AF: conceptualization and reviewing. AN: conceptualization, supervision, software, and validation. HK: conceptualization, data creation, writing- original draft preparation, and reviewing and editing. All authors contributed to the article and approved the submitted version.
Conflict of Interest
The authors declare that the research was conducted in the absence of any commercial or financial relationships that could be construed as a potential conflict of interest.
References
Abd El-Naby, A. S., Khattaby, A. E.-R. A., Samir, F., Awad, S. M., and Abdel-Tawwab, M. (2019). Stimulatory effect of dietary butyrate on growth, immune response, and resistance of Nile tilapia, Oreochromis niloticus against Aeromonas hydrophila infection. Animal Feed Sci. Technol. 254:114212. doi: 10.1016/j.anifeedsci.2019.114212
Abdel-Tawwab, M. (2012). Interactive effects of dietary protein and live bakery yeast, Saccharomyces cerevisiae on growth performance of Nile tilapia, Oreochromis niloticus (L.) fry and their challenge against Aeromonas hydrophila infection. Aquaculture Int. 20, 317–331. doi: 10.1007/s10499-011-9462-8
Abdel-Tawwab, M., Abdel-Rahman, A. M., and Ismael, N. E. (2008). Evaluation of commercial live bakers’ yeast, Saccharomyces cerevisiae as a growth and immunity promoter for Fry Nile tilapia, Oreochromis niloticus (L.) challenged in situ with Aeromonas hydrophila. Aquaculture 280, 185–189. doi: 10.1016/j.aquaculture.2008.03.055
Abdel-Tawwab, M., Adeshina, I., and Issa, Z. A. (2020a). Antioxidants and immune responses, resistance to Aspergilus flavus infection, and growth performance of Nile tilapia, Oreochromis niloticus, fed diets supplemented with yeast, Saccharomyces serevisiae. Animal Feed Sci. Technol. 263:114484. doi: 10.1016/j.anifeedsci.2020.114484
Abdel-Tawwab, M., Mounes, H. A., Shady, S. H., and Ahmed, K. M. (2020b). Effects of yucca, Yucca schidigera, extract and/or yeast, Saccharomyces cerevisiae, as water additives on growth, biochemical, and antioxidants/oxidant biomarkers of Nile tilapia, Oreochromis niloticus. Aquaculture 533:736122. doi: 10.1016/j.aquaculture.2020.736122
Abdel-Tawwab, M., and Wafeek, M. (2017). Fluctuations in water temperature affected waterborne cadmium toxicity: hematology, anaerobic glucose pathway, and oxidative stress status of Nile tilapia, Oreochromis niloticus (L.). Aquaculture 477, 106–111. doi: 10.1016/j.aquaculture.2017.05.007
Abdel-Tawwab, M., and Ahmad, M. H. (2009). Live spirulina (Arthrospira platensis) as a growth and immunity promoter for Nile tilapia, Oreochromis niloticus (L.), challenged with pathogenic Aeromonas hydrophila. Aquaculture Res. 40, 1037–1046. doi: 10.1111/j.1365-2109.2009.02195.x
Abou-El-Atta, M. E., Abdel-Tawwab, M., Abdel-Razek, N., and Abdelhakim, T. M. (2019). Effects of dietary probiotic Lactobacillus plantarum and whey protein concentrate on the productive parameters, immunity response and susceptibility of Nile tilapia, Oreochromis niloticus (L.), to Aeromonas sobria infection. Aquaculture Nutr. 25, 1367–1377. doi: 10.1111/anu.12957
Adeshina, I., Jenyo-Oni, A., Emikpe, B. O., Ajani, E. K., and Abdel-Tawwab, M. (2019). Stimulatory effect of dietary clove, Eugenia caryophyllata, bud extract on growth performance, nutrient utilization, antioxidant capacity, and tolerance of African catfish, Clarias gariepinus (B.), to Aeromonas hydrophila infection. J. World Aquaculture Soc. 50, 390–405. doi: 10.1111/jwas.12565
Allam, B. W., Khalil, H. S., Mansour, A. T., Srour, T. M., Omar, E. A., and Nour, A. A. M. (2020). Impact of substitution of fish meal by high protein distillers dried grains on growth performance, plasma protein and economic benefit of striped catfish (Pangasianodon hypophthalmus). Aquaculture 517:734792. doi: 10.1016/j.aquaculture.2019.734792
Aly, S. M., Abd-El-Rahman, A. M., John, G., and Mohamed, M. F. (2008). Characterization of some bacteria isolated from Oreochromis niloticus and their potential use as probiotics. Aquaculture 277, 1–6. doi: 10.1016/j.aquaculture.2008.02.021
Amenyogbe, E., Chen, G., Wang, Z., Huang, J., Huang, B., and Li, H. (2020). The exploitation of probiotics, prebiotics and synbiotics in aquaculture: present study, limitations and future directions.: a review. Aquaculture Int. 28, 1–25.
Balcázar, J. L., De Blas, I., Ruiz-Zarzuela, I., Cunningham, D., Vendrell, D., and Múzquiz, J. L. (2006). The role of probiotics in aquaculture. Vet. Microbiol. 114, 173–186.
Bosma, R. H., and Verdegem, M. C. (2011). Sustainable aquaculture in ponds: principles, practices and limits. Livestock Sci. 139, 58–68. doi: 10.1016/j.livsci.2011.03.017
Boyd, C. E. (1990). Water Quality in Ponds for Aquaculture. Birmingham, ALA: Birmingham Publishing Company.
Bozkurt, M., Aysul, N., Küçükyilmaz, K., Aypak, S., Ege, G., Catli, A., et al. (2014). Efficacy of in-feed preparations of an anticoccidial, multienzyme, prebiotic, probiotic, and herbal essential oil mixture in healthy and Eimeria spp.-infected broilers. Poultry Sci. 93, 389–399. doi: 10.3382/ps.2013-03368
Cerezuela, R., Fumanal, M., Tapia-Paniagua, S. T., Meseguer, J., and Moriñigo, M. Á, and Esteban, M. Á (2013). Changes in intestinal morphology and microbiota caused by dietary administration of inulin and Bacillus subtilis in gilthead sea bream (Sparus aurata L.) specimens. Fish Shellfish Immunol. 34, 1063–1070. doi: 10.1016/j.fsi.2013.01.015
Claiborne, A. (1985). “Catalase activity,” in Handbook of Methods for Oxygen Free Radical Research, ed. R. A. Greenwald (Boca Raton, FL: CRC Press).
Dash, G., Raman, R. P., Prasad, K. P., Makesh, M., Pradeep, M., and Sen, S. (2015). Evaluation of paraprobiotic applicability of Lactobacillus plantarum in improving the immune response and disease protection in giant freshwater prawn, Macrobrachium rosenbergii (de Man, 1879). Fish Shellfish Immunol. 43, 167–174. doi: 10.1016/j.fsi.2014.12.007
Dimitroglou, A., Merrifield, D., Moate, R., Davies, S., Spring, P., Sweetman, J., et al. (2009). Dietary mannan oligosaccharide supplementation modulates intestinal microbial ecology and improves gut morphology of rainbow trout, Oncorhynchus mykiss (Walbaum). J. Anim. Sci. 87, 3226–3234. doi: 10.2527/jas.2008-1428
Doumas, B. T., Bayse, D. D., Carter, R. J., Peters, T. Jr., and Schaffer, R. (1981). A candidate reference method for determination of total protein in serum. I. Development and validation. Clin. Chem. 27, 1642–1650. doi: 10.1093/clinchem/27.10.1642
Drabkin, D. (1945). Crystallographic and optical properties of human hemoglobin, A proposal for the standarization of hemoglobin. Am. J. Med. 209, 268–270.
Dytham, C. (2011). Choosing and Using Statistics: A Biologist’s Guide. Hoboken, NJ: John Wiley & Sons.
El-Haroun, E., Goda, A. S., and Kabir Chowdhury, M. (2006). Effect of dietary probiotic Biogen® supplementation as a growth promoter on growth performance and feed utilization of Nile tilapia Oreochromis niloticus (L.). Aquaculture Res. 37, 1473–1480. doi: 10.1111/j.1365-2109.2006.01584.x
Elsabagh, M., Mohamed, R., Moustafa, E. M., Hamza, A., Farrag, F., Decamp, O., et al. (2018). Assessing the impact of Bacillus strains mixture probiotic on water quality, growth performance, blood profile and intestinal morphology of Nile tilapia, Oreochromis niloticus. Aquaculture Nutr. 24, 1613–1622. doi: 10.1111/anu.12797
Eurell, T., Lewis, D., and Grumbles, L. (1978). Comparison of selected diagnostic tests for detection of motile Aeromonas septicemia in fish. Am. J. Vet. Res. 39, 1384–1386.
Farzanfar, A. (2006). The use of probiotics in shrimp aquaculture. FEMS Immunol. Med. Microbiol. 48, 149–158. doi: 10.1111/j.1574-695x.2006.00116.x
Ferguson, R., Merrifield, D. L., Harper, G. M., Rawling, M. D., Mustafa, S., Picchietti, S., et al. (2010). The effect of Pediococcus acidilactici on the gut microbiota and immune status of on-growing red tilapia (Oreochromis niloticus). J. Appl. Microbiol. 109, 851–862. doi: 10.1111/j.1365-2672.2010.04713.x
Froese, R. (2006). Cube law, condition factor and weight–length relationships: history, meta-analysis and recommendations. J. Appl. Ichthyol. 22, 241–253. doi: 10.1111/j.1439-0426.2006.00805.x
Giatsis, C., Sipkema, D., Ramiro-Garcia, J., Bacanu, G. M., Abernathy, J., Verreth, J., et al. (2016). Probiotic legacy effects on gut microbial assembly in tilapia larvae. Sci. Rep. 6:33965.
Gobi, N., Vaseeharan, B., Chen, J.-C., Rekha, R., Vijayakumar, S., Anjugam, M., et al. (2018). Dietary supplementation of probiotic Bacillus licheniformis Dahb1 improves growth performance, mucus and serum immune parameters, antioxidant enzyme activity as well as resistance against Aeromonas hydrophila in tilapia Oreochromis mossambicus. Fish Shellfish Immunol. 74, 501–508. doi: 10.1016/j.fsi.2017.12.066
Guardiola, F., Porcino, C., Cerezuela, R., Cuesta, A., Faggio, C., and Esteban, M. (2016). Impact of date palm fruits extracts and probiotic enriched diet on antioxidant status, innate immune response and immune-related gene expression of European seabass (Dicentrarchus labrax). Fish Shellfish Immunol. 52, 298–308. doi: 10.1016/j.fsi.2016.03.152
Hari, B., Kurup, B. M., Varghese, J. T., Schrama, J., and Verdegem, M. (2004). Effects of carbohydrate addition on production in extensive shrimp culture systems. Aquaculture 241, 179–194. doi: 10.1016/j.aquaculture.2004.07.002
Hoseinifar, S. H., Sharifian, M., Vesaghi, M. J., Khalili, M., and Esteban, M. Á (2014). The effects of dietary xylooligosaccharide on mucosal parameters, intestinal microbiota and morphology and growth performance of Caspian white fish (Rutilus frisii kutum) fry. Fish Shellfish Immunol. 39, 231–236. doi: 10.1016/j.fsi.2014.05.009
Ismail, R., and Al-Hamdani, A. (2019). Effect of probiotic (Poultrystar®) and heat stress on some blood parameters in common carp (Cyprinus carpio L.). Iraqi J. Vet. Sci. 33, 221–225. doi: 10.33899/ijvs.2019.125539.1061
Joffre, O., and Verdegem, M. (2019). Feeding both pond and fish: A pathway to ecological intensification of aquaculture systems. Infofish Int. 3, 55–58.
Kawahara, E., Ueda, T., and Nomura, S. (1991). In vitro phagocytic activity of white-spotted char blood cells after injection with Aeromonas salmonicida extracellular products. Fish Pathol. 26, 213–214. doi: 10.3147/jsfp.26.213
Khalil, H. S., Mansour, A. T., Goda, A. M. A., and Omar, E. A. (2019). Effect of selenium yeast supplementation on growth performance, feed utilization, lipid profile, liver and intestine histological changes, and economic benefit in meagre, Argyrosomus regius, fingerlings. Aquaculture 501, 135–143. doi: 10.1016/j.aquaculture.2018.11.018
Khalila, H., Fayed, W., Mansour, A., Srour, T., Omar, E., Darwish, S., et al. (2018). Dietary supplementation of spirulina, Arthrospira platensis, with plant protein sources and their effects on growth, feed utilization and histological changes in nile tilapia, Oreochromis niloticus. J. Aquac. Res. Dev. 9:2.
Kim, M.-S., Min, E., Kim, J.-H., Koo, J.-K., and Kang, J.-C. (2015). Growth performance and immunological and antioxidant status of Chinese shrimp, Fennerpenaeus chinensis reared in bio-floc culture system using probiotics. Fish Shellfish Immunol. 47, 141–146. doi: 10.1016/j.fsi.2015.08.027
Kuebutornye, F. K., Abarike, E. D., and Lu, Y. (2019). A review on the application of Bacillus as probiotics in aquaculture. Fish Shellfish Immunol. 87, 820–828. doi: 10.1016/j.fsi.2019.02.010
Kumar, R., Mukherjee, S., Ranjan, R., and Nayak, S. (2008). Enhanced innate immune parameters in Labeo rohita (Ham.) following oral administration of Bacillus subtilis. Fish Shellfish Immunol. 24, 168–172. doi: 10.1016/j.fsi.2007.10.008
Kumar, S., Sahu, N., Pal, A., Choudhury, D., Yengkokpam, S., and Mukherjee, S. (2005). Effect of dietary carbohydrate on haematology, respiratory burst activity and histological changes in L. rohita juveniles. Fish Shellfish Immunol. 19, 331–344. doi: 10.1016/j.fsi.2005.03.001
Liu, C.-H., Chiu, C.-H., Wang, S.-W., and Cheng, W. (2012). Dietary administration of the probiotic, Bacillus subtilis E20, enhances the growth, innate immune responses, and disease resistance of the grouper, Epinephelus coioides. Fish Shellfish Immunol. 33, 699–706. doi: 10.1016/j.fsi.2012.06.012
Liu, H., Wang, S., Cai, Y., Guo, X., Cao, Z., Zhang, Y., et al. (2017). Dietary administration of Bacillus subtilis HAINUP40 enhances growth, digestive enzyme activities, innate immune responses and disease resistance of tilapia, Oreochromis niloticus. Fish Shellfish Immunol. 60, 326–333. doi: 10.1016/j.fsi.2016.12.003
Liu, L., Wu, J., Zhang, J., Li, Z., Wang, C., Chen, M., et al. (2012). A compatibility assay of ursolic acid and foodborne microbial exopolysaccharides by antioxidant power and anti-proliferative properties in hepatocarcinoma cells. J. Food Agric. Environ. 10, 111–114.
Magnadottir, B., Lange, S., Gudmundsdottir, S., Bøgwald, J., and Dalmo, R. (2005). Ontogeny of humoral immune parameters in fish. Fish Shellfish Immunol. 19, 429–439. doi: 10.1016/j.fsi.2005.03.010
Mansour, A. T.-E., Goda, A. A., Omar, E. A., Khalil, H. S., and Esteban, M. Á (2017). Dietary supplementation of organic selenium improves growth, survival, antioxidant and immune status of meagre, Argyrosomus regius, juveniles. Fish Shellfish Immunol. 68, 516–524. doi: 10.1016/j.fsi.2017.07.060
Milla, S., Mathieu, C., Wang, N., Lambert, S., Nadzialek, S., Massart, S., et al. (2010). Spleen immune status is affected after acute handling stress but not regulated by cortisol in Eurasian perch, Perca fluviatilis. Fish Shellfish Immunol. 28, 931–941. doi: 10.1016/j.fsi.2010.02.012
Mulcahy, M. F. (1971). Serum protein changes associated with ulcerative dermal necrosis (UDN) in the trout Salmo trutta L. J. Fish Biol. 3, 199–201. doi: 10.1111/j.1095-8649.1971.tb03663.x
Nayak, S., Swain, P., and Mukherjee, S. (2007). Effect of dietary supplementation of probiotic and vitamin C on the immune response of Indian major carp, Labeo rohita (Ham.). Fish Shellfish Immunol. 23, 892–896. doi: 10.1016/j.fsi.2007.02.008
Newaj-Fyzul, A., and Austin, B. (2015). Probiotics, immunostimulants, plant products and oral vaccines, and their role as feed supplements in the control of bacterial fish diseases. J. Fish Dis. 38, 937–955. doi: 10.1111/jfd.12313
Ng, W. K., and Koh, C. B. (2017). The utilization and mode of action of organic acids in the feeds of cultured aquatic animals. Revi. Aquaculture 9, 342–368. doi: 10.1111/raq.12141
Oliveira, D., Vidal, L., Ares, G., Walter, E. H., Rosenthal, A., and Deliza, R. (2017). Sensory, microbiological and physicochemical screening of probiotic cultures for the development of non-fermented probiotic milk. LWT Food Sci. Technol. 79, 234–241. doi: 10.1016/j.lwt.2017.01.020
Payá, M., Halliwell, B., and Hoult, J. (1992). Interactions of a series of coumarins with reactive oxygen species: scavenging of superoxide, hypochlorous acid and hydroxyl radicals. Biochem. Pharmacol. 44, 205–214. doi: 10.1016/0006-2952(92)90002-z
Peixoto, F., Alves-Fernandes, D., Santos, D., and Fontaínhas-Fernandes, A. (2006). Toxicological effects of oxyfluorfen on oxidative stress enzymes in tilapia Oreochromis niloticus. Pesticide Biochem. Physiol. 85, 91–96. doi: 10.1016/j.pestbp.2005.10.007
Pirarat, N., Pinpimai, K., Endo, M., Katagiri, T., Ponpornpisit, A., Chansue, N., et al. (2011). Modulation of intestinal morphology and immunity in nile tilapia (Oreochromis niloticus) by Lactobacillus rhamnosus GG. Res. Vet. Sci. 91, e92–e97.
Rahman, A. N. A., Khalil, A. A., Abdallah, H., and ElHady, M. (2018). The effects of the dietary supplementation of Echinacea purpurea extract and/or vitamin C on the intestinal histomorphology, phagocytic activity, and gene expression of the Nile tilapia. Fish Shellfish Immunol. 82, 312–318. doi: 10.1016/j.fsi.2018.08.024
Ramesh, D., Vinothkanna, A., Rai, A. K., and Vignesh, V. S. (2015). Isolation of potential probiotic Bacillus spp. and assessment of their subcellular components to induce immune responses in Labeo rohita against Aeromonas hydrophila. Fish Shellfish Immunol. 45, 268–276. doi: 10.1016/j.fsi.2015.04.018
Reda, R. M., and Selim, K. M. (2015). Evaluation of Bacillus amyloliquefaciens on the growth performance, intestinal morphology, hematology and body composition of Nile tilapia, Oreochromis niloticus. Aquaculture Int. 23, 203–217. doi: 10.1007/s10499-014-9809-z
Ringø, E., Olsen, R. E., Vecino, J. G., Wadsworth, S., and Song, S. (2012). Use of immunostimulants and nucleotides in aquaculture: a review. J. Mar. Sci. Res. Dev. 2:104.
Salinas, I., Abelli, L., Bertoni, F., Picchietti, S., Roque, A., Furones, D., et al. (2008). Monospecies and multispecies probiotic formulations produce different systemic and local immunostimulatory effects in the gilthead seabream (Sparus aurata L.). Fish Shellfish Immunol. 25, 114–123. doi: 10.1016/j.fsi.2008.03.011
Samanya, M., and Yamauchi, K.-E. (2002). Histological alterations of intestinal villi in chickens fed dried Bacillus subtilis var. natto. Comp. Biochem. Physiol. A Mol. Integr. Physiol. 133, 95–104. doi: 10.1016/s1095-6433(02)00121-6
Selim, K. M., and Reda, R. M. (2015). Improvement of immunity and disease resistance in the Nile tilapia, Oreochromis niloticus, by dietary supplementation with Bacillus amyloliquefaciens. Fish Shellfish Immunol. 44, 496–503. doi: 10.1016/j.fsi.2015.03.004
Sewaka, M., Trullas, C., Chotiko, A., Rodkhum, C., Chansue, N., Boonanuntanasarn, S., et al. (2019). Efficacy of synbiotic Jerusalem artichoke and Lactobacillus rhamnosus GG-supplemented diets on growth performance, serum biochemical parameters, intestinal morphology, immune parameters and protection against Aeromonas veronii in juvenile red tilapia (Oreochromis spp.). Fish Shellfish Immunol. 86, 260–268. doi: 10.1016/j.fsi.2018.11.026
Sun, Y.-Z., Yang, H.-L., Ma, R.-L., and Lin, W.-Y. (2010). Probiotic applications of two dominant gut Bacillus strains with antagonistic activity improved the growth performance and immune responses of grouper Epinephelus coioides. Fish Shellfish Immunol. 29, 803–809. doi: 10.1016/j.fsi.2010.07.018
Telli, G. S., Ranzani-Paiva, M. J. T., de Carla Dias, D., Sussel, F. R., Ishikawa, C. M., and Tachibana, L. (2014). Dietary administration of Bacillus subtilis on hematology and non-specific immunity of Nile tilapia Oreochromis niloticus raised at different stocking densities. Fish Shellfish Immunol. 39, 305–311. doi: 10.1016/j.fsi.2014.05.025
Tran, N. H., Verdegem, M., and Joffre, O. (2016). The Nutritious Pond Porject at the International Fisheries Symposium 2016. Vietnam, VT: Phu Quoc.
Van Hai, N. (2015). Research findings from the use of probiotics in tilapia aquaculture: a review. Fish Shellfish Immunol. 45, 592–597. doi: 10.1016/j.fsi.2015.05.026
Verschuere, L., Rombaut, G., Sorgeloos, P., and Verstraete, W. (2000). Probiotic bacteria as biological control agents in aquaculture. Microbiol. Mol. Biol. Rev. 64, 655–671. doi: 10.1128/mmbr.64.4.655-671.2000
Yossa, R., Levesque, S., Groman, D. B., and Lora, J. H. (2018). Preliminary evaluation of purified lignin and hemicellulose as prebiotics candidates for Atlantic Salmon, Salmo salar L. J. Appl. Aquaculture 30, 256–271. doi: 10.1080/10454438.2018.1439795
Keywords: immunostimulants, phagocytic index, lysozyme, antioxidant enzyme, intestinal morphology
Citation: Kord MI, Srour TM, Omar EA, Farag AA, Nour AAM and Khalil HS (2021) The Immunostimulatory Effects of Commercial Feed Additives on Growth Performance, Non-specific Immune Response, Antioxidants Assay, and Intestinal Morphometry of Nile tilapia, Oreochromis niloticus. Front. Physiol. 12:627499. doi: 10.3389/fphys.2021.627499
Received: 09 November 2020; Accepted: 22 January 2021;
Published: 25 February 2021.
Edited by:
Jianmin Zhao, Yantai Institute of Coastal Zone Research, Chinese Academy of Sciences (CAS), ChinaReviewed by:
Yanjiao Zhang, Ocean University of China, ChinaMohsen Abdel-Tawwab, Agricultural Research Center, Egypt
Mahmoud A. O. Dawood, Kafrelsheikh University, Egypt
Copyright © 2021 Kord, Srour, Omar, Farag, Nour and Khalil. This is an open-access article distributed under the terms of the Creative Commons Attribution License (CC BY). The use, distribution or reproduction in other forums is permitted, provided the original author(s) and the copyright owner(s) are credited and that the original publication in this journal is cited, in accordance with accepted academic practice. No use, distribution or reproduction is permitted which does not comply with these terms.
*Correspondence: Hala Saber Khalil, aGFsYXNhYmVyMjAxMUB5YWhvby5jb20=; orcid.org/0000-0002-6236-6847