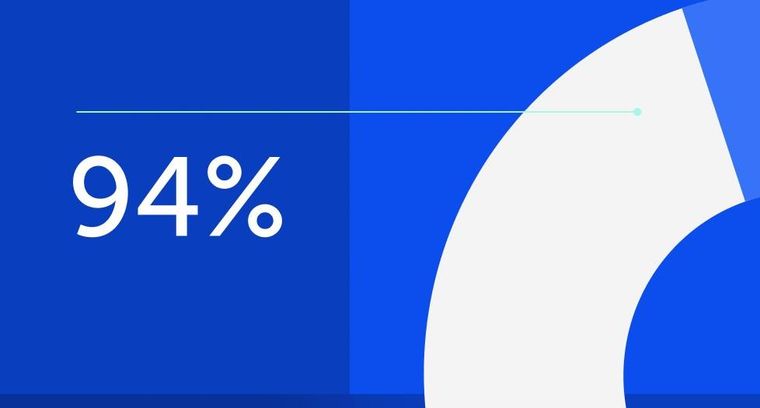
94% of researchers rate our articles as excellent or good
Learn more about the work of our research integrity team to safeguard the quality of each article we publish.
Find out more
REVIEW article
Front. Physiol., 22 February 2021
Sec. Integrative Physiology
Volume 12 - 2021 | https://doi.org/10.3389/fphys.2021.624052
This article is part of the Research TopicThe Tribute of Physiology for the Understanding of COVID-19 DiseaseView all 39 articles
Since December 2019, the coronavirus 2019 (COVID-19) pandemic has rapidly spread and overwhelmed healthcare systems worldwide, urging physicians to understand how to manage this novel infection. Early in the pandemic, more severe forms of COVID-19 have been observed in patients with cardiovascular comorbidities, who are often treated with renin-angiotensin aldosterone system (RAAS)-blockers, such as angiotensin-converting enzyme inhibitors (ACEIs) or angiotensin receptor blockers (ARBs), but whether these are indeed independent risk factors is unknown. The cellular receptor for the severe acute respiratory syndrome coronavirus 2 (SARS-CoV-2) is the membrane-bound angiotensin converting enzyme 2 (ACE2), as for SARS-CoV(-1). Experimental data suggest that expression of ACE2 may be increased by RAAS-blockers, raising concerns that these drugs may facilitate viral cell entry. On the other hand, ACE2 is a key counter-regulator of the RAAS, by degrading angiotensin II into angiotensin (1-7), and may thereby mediate beneficial effects in COVID-19. These considerations have raised concerns about the management of these drugs, and early comments shed vivid controversy among physicians. This review will describe the homeostatic balance between ACE-angiotensin II and ACE2-angiotensin (1-7) and summarize the pathophysiological rationale underlying the debated role of the RAAS and its modulators in the context of the pandemic. In addition, we will review available evidence investigating the impact of RAAS blockers on the course and prognosis of COVID-19 and discuss why retrospective observational studies should be interpreted with caution. These considerations highlight the importance of solid evidence-based data in order to guide physicians in the management of RAAS-interfering drugs in the general population as well as in patients with more or less severe forms of SARS-CoV-2 infection.
Since December 2019, the coronavirus disease 2019 (COVID-19) pandemic has rapidly spread and overwhelmed healthcare systems worldwide. Physicians and scientists urgently attempted to decipher the pathophysiology of the disease in order to define appropriate prevention of viral transmission and management of infected patients. The discovery that the viral receptor was a key enzyme of the renin-angiotensin-aldosterone system (RAAS), namely angiotensin-converting enzyme 2 (ACE2) (Chen Y et al., 2020; Hoffmann et al., 2020), shed light on the potential interactions between the novel coronavirus and the RAAS.
ACE2 is a mono-carboxypeptidase discovered by Donoghue et al. (2000), expressed in the epithelium of the respiratory tract, but also in the intestine, the central nervous system, the heart, the vessels (on endothelial cells), the kidney, and the testicle (Harmer et al., 2002; Hamming et al., 2004; Lee I. T. et al., 2020). In polarized epithelia of the lung, kidney, intestine, ACE2 is found at the apical membrane. In the lung, ACE2 is primarily found on a subset of epithelial cells (type II pneumocytes) facing the airspace, while the angiotensin-converting enzyme (ACE) is expressed on the endothelium, facing the blood. ACE2 had previously been shown to be the receptor for SARS-CoV, but the novel coronavirus has a higher affinity for ACE2 (Wrapp et al., 2020). ACE2 displays significant homology with ACE and is considered as a key counter-regulator of the RAAS, by degrading the octapeptide angiotensin (Ang) II into Ang (1-7) (Chappell, 2016). It also allows the transformation of Ang I into Ang (1-7), via the synthesis of an intermediate metabolite, Ang (1–9), although this pathway is quantitatively marginal. Ang (1-7) binds to the G protein-coupled Mas receptor and induces effects not only on the cardiovascular system, but in fact far beyond, generally “protective” because promoting vasodilation and limiting inflammation, fibrosis, coagulation, and capillary leakage (Kreutz et al., 2020). Biological effects of Ang (1-7) are indeed opposite to those induced by Ang II after binding to its type 1 (AT1) receptor (Figure 1). Importantly, angiotensin converting enzyme inhibitors (ACEIs) do not inhibit ACE2 (Tipnis et al., 2000). In times of emerging infectious threat, the interest toward what appears as a molecular cornerstone in the disease has grown rapidly.
Figure 1. ACE-angiotensin II/ACE2-angiotensin (1-7) homeostasis: general overview. Atg, angiotensinogen; Ang I, angiotensin I; Ang II, angiotensin II; Ang(1-9), angiotensin (1-9); Ang(1-7), angiotensin (1-7); ACEI, ACE inhibitor; ARB, Ang II receptor blocker; ACE, angiotensin converting enzyme; ACE, angiotensin converting enzyme 2; sACE, soluble ACE; sACE2, soluble ACE2; AT1-R, Ang II receptor type 1; AT2-R, Ang II receptor type 2; MAS, G protein-coupled MAS receptor; TMPRSS2, transmembrane protease serine 2; SARS-CoV-2, severe acute respiratory syndrome coronavirus 2; ADAM17, A disintegrin and metalloproteinase 17.
Since early in the pandemic, more severe forms of COVID-19 have been observed in patients with cardiovascular comorbidities such as hypertension, diabetes and coronary heart disease (Wang D. et al., 2020; Wu Y. et al., 2020; Zhou et al., 2020b). Several authors hypothesized that that the link between cardiovascular conditions and severity of the disease might be related to the frequent use of RAAS blockers in these patients. The underlying rationale came from experimental studies in rodents suggesting that RAAS blockers may be responsible for an increased expression of ACE2 (Ferrario et al., 2005a, b; Soler et al., 2009).
While concerns about the safety of these widely used drugs rapidly spread from the medical literature (Aronson and Ferner, 2020; Diaz, 2020; Esler and Esler, 2020; Fang et al., 2020) to general and social media, other authors suggested that modulating ACE-Ang II versus ACE2-Ang (1-7) homeostasis in favor of the latter, using RAAS blockers, might actually be beneficial in patients with COVID-19 (Hanff et al., 2020; Vaduganathan et al., 2020).
Consequently, the management of RAAS-blockers, especially ACEIs and angiotensin receptor blockers (ARBs) in patients at risk of infection or infected with severe acute respiratory syndrome coronavirus 2 (SARS-CoV-2), has been a matter of controversy (South et al., 2020). Many observational studies have attempted to elucidate whether these drugs increased the risk to become infected with COVID-19 and/or modified the course and prognosis of the disease in infected patients while several randomized clinical trials are ongoing to establish whether RAAS blockers should be maintained, discontinued, or even introduced de novo in patients with COVID-19.
This review aims to analyze existing data supporting each side of the debate and to describe the available clinical evidence in order to help clinicians understand the underlying pathophysiology and manage prescription of these drugs in the context of the COVID-19 pandemic. The closely related issue of mineralocorticoid receptor antagonists has been less widely debated although it would deserve a dedicated review in itself. The potential role of mineralocorticoid receptor antagonists is beyond the scope of the present review and is only briefly mentioned in this manuscript.
Early concerns regarding the potential deleterious role of RAAS blockers mainly relied on animal data suggesting that ACE2 expression or activity is increased by these drugs, thereby facilitating viral cell entry. However, as reviewed below, this observation is inconsistent in various animal models, is not established in humans, and most importantly, there are no data showing an increased expression of the transmembrane ACE2 protein in the lung. In addition, cardiovascular conditions themselves, independent of RAAS blockade treatment, may also, and probably to a larger extent, influence the expression of ACE2.
Since the discovery that the ACE2-Ang (1-7)-Mas receptor pathway was a key counter-regulatory system of the ACE-Ang II-AT1 receptor pathway through the past two decades, the role, tissue expression and regulation of ACE2 have been extensively studied and described (Santos et al., 2018), but remain incompletely understood, in part due to discrepant results. In particular, the influence of the pharmacological blockade of ACE and of the AT1 receptor on ACE2 is an unsettled issue.
As reviewed in Supplementary Tables 1A,B, animal studies investigating the effect of ACEIs and/or ARBs on the expression of ACE2 have relied on different animal species, disease models, and readouts (mRNA versus protein level, or enzyme activity). In the early publications warning against the use of RAAS blockers, a widely cited study was that by Ferrario et al. published in 2005. The authors studied the effects of a 12-day treatment of wild-type rats with an ACEI, an ARB, or their combination on expression and activity of the components of the RAAS, in plasma and cardiac tissue. Both losartan (an ARB) and lisinopril (an ACEI) increased the level of ACE2 mRNA in the heart compared to vehicle-treated animals. Losartan, but not lisinopril, also increased ACE2 activity in cardiac membranes [assessed from the rate of conversion of Ang II to Ang (1-7)] and cardiac tissue concentrations of Ang (1-7), while both drugs increased plasma levels of Ang (1-7) (Ferrario et al., 2005a). The same team found somewhat different results in the rat kidney, where lisinopril and losartan did not modify ACE2 gene expression but increased ACE2 enzyme activity in membranes of renal cortex, as well as plasma and urine levels of Ang (1-7) (Ferrario et al., 2005b).
The putative mechanisms underlying an upregulation of ACE2 in the presence of RAAS blockers are partially elucidated. Ang II has been shown to inhibit ACE2 expression through an AT1 receptor-mediated extracellular regulated (ERK)1/2 and p38 mitogen-activated protein (MAP) kinases, both in vitro in different cellular models and in vivo (Gallagher et al., 2006, 2008a,b; Koka et al., 2008). In addition, Ang II also down-regulates ACE2 at the post-transcriptional level. It has been shown that ACE2 and the AT1 receptor interact on the cell surface and that the binding of Ang II to its AT1 receptor leads the reduction of these AT1 receptor-ACE2 complexes, and to the internalization and ubiquitination of ACE2 (Deshotels et al., 2014). Therefore, the alleged underlying mechanism for an increased expression of ACE2 in the presence of RAAS blockers results from the alleviations of these AT1-receptor mediated inhibiting pathways. According to this hypothesis, both ACEIs (by inhibiting Ang II synthesis) and ARBs (by blocking the AT1-mediated effects of Ang II) are expected to upregulate ACE2, although ARBs interfere more directly with the involved pathway and are thereby potentially expected to have a stronger effect. In line with this, studies seem to have more consistently shown an up-regulation of ACE2 protein concentration in tissues of interest when animals are treated by ARBs (Ishiyama et al., 2004; Igase et al., 2005; Agata et al., 2006; Jessup et al., 2006; Takeda et al., 2007; Soler et al., 2009; Han et al., 2010; Sukumaran et al., 2011, 2012; Zhang et al., 2014), than in studies using ACEIs, which produced more discrepant results (Hamming et al., 2008; Burchill et al., 2012; Burrell et al., 2012; Yang et al., 2013; Zhang et al., 2014; Wang G. et al., 2016), as detailed in Supplementary Tables 1A,B.
However, the biological actions of ACEIS and ARBs on ACE2 in healthy animals remain unclear as not all studies have found similar results, even with each separate class of drug (Supplementary Table 1A). Although many studies have confirmed an increase in ACE2 expression, either at the mRNA and/or protein level, or an increased activity of the enzyme (Ocaranza et al., 2006; Soler et al., 2009; Li et al., 2015), others have shown no effect of RAAS blockers on ACE2 expression or activity (Han et al., 2010; Velkoska et al., 2010; Wösten-van Asperen et al., 2011; Wu C. et al., 2020).
More importantly, although of high relevance in the context of SARS-CoV-2 infection, data on the influence of RAAS blockers on the expression of ACE2 in the lungs are scarce. In ventilated rats with no other cause of lung injury, losartan did not influence ACE2 activity in bronchoalveolar lavage fluid (Wösten-van Asperen et al., 2011), while captopril was found to increase the expression of ACE2 in the lungs of control rats breathing spontaneously (Li et al., 2015). However, variations of ACE2 in lavage fluid may not reflect changes in tissue expression within the lung epithelium. In a recent publication, Wu C. et al. (2020) analyzed the effect of a 14-day infusion of enalapril or losartan on the expression on ACE2 in various tissues (ileum, kidney, heart, and lungs) of wild-type male C57BL/6J mice. Although plasma renin increased by over 100-fold with both drugs, confirming potent RAAS inhibition, neither enalapril nor losartan changed the abundance of ACE2 mRNA in any of the tissues, including the lungs. Likewise, in a recent publication, Wysocki et al. (2020) used kidney and lung lysates to examine the effect of captopril and telmisartan, administered for 2 weeks, on ACE2 expression in mice. Interestingly, in lysates of kidney cortex, ACE2 activity and protein did not differ significantly in captopril- or losartan-treated animals compared with vehicle-treated animals. However, in isolated kidney membranes, there was a profound decrease in kidney ACE2 protein. This decrease in membrane-bound ACE2 was associated with a significant increase in cytosolic ACE2 protein, interpreted as a possible internalization of the protein. In lung tissue, attempts to perform Western blots for ACE2 with either total lysates or isolated membranes did not yield a signal, in agreement with the low physiological expression of the enzyme in type 2 pneumocytes. Conversely, ACE2 activity was low but consistently detected and was not significantly modified by either captopril or telmisartan, both in lung total lysates and lung membranes. Overall, this study showed that ACE2 is not increased by RAAS blockers in these two organs (lung and kidney) that are potential key target sites for SARS-CoV-2 infection.
One potential explanation for these discrepant results is that ACE2 activity does not always correlate with mRNA and protein expression levels. Indeed, several authors have shown uncoupled variations between mRNA, the protein and its activity (Ferrario et al., 2005a, b; Wysocki et al., 2006; Hamming et al., 2008; Yang et al., 2013), revealing potential strong post-transcriptional and post-translational regulations of ACE2. Consequently, based on the readout, conclusions on the impact of RAAS-blockers on ACE2 vary markedly. In addition, as viral entry requires the transmembrane expression of ACE2 in the respiratory tract, any conclusions drawn from mRNA levels, or even from protein level or enzymatic activity of the circulating enzyme is highly speculative. As outlined by Wysocki et al. (2020), when assessing the relative quantities of ACE2 that can act as the SARS-CoV-2 receptor, what matters most is the abundance of full-length, membrane-bound ACE2, which was either not modified or decreased in their well-conducted study with appropriate experimental methodology. Furthermore, specific properties of each molecule within each drug-class may also explain discrepant results which may not be class-related. For instance, captopril is a member of a sulfhydryl class of ACEI that have direct antioxidant properties (Liu et al., 2006).
Other potential explanations for discrepant results between laboratories include the major experimental difficulties associated with the biochemical assessment of the various components of the RAAS, due to the poor specificity of antibodies and of synthetic substrates or inhibitors of ACE2, and to the poor availability of the most accurate assays of ACE2 activity such as high-performance liquid chromatography/mass spectrometry, as reviewed elsewhere (Chappell, 2016; Sparks et al., 2020).
Moreover, the observed effect of RAAS-blockers has been shown to differ between organs, unraveling the complexity of the communications between the local RAAS amongst different tissues. As detailed above, the same team, with similar methods and in the same animal model, found different effects of RAAS blockade in heart and kidney (Ferrario et al., 2005a, b). Effects may also vary within each organ. Burrell et al. (2012) showed that ramipril (an ACE inhibitor) restored ACE2 activity in the renal cortex, but not in the renal medulla after partial nephrectomy. In addition, many experimental studies used very high doses of RAAS blockers (Sriram and Insel, 2020), limiting their physiological relevance. Furthermore, duration (from a few days to several months) and route of administration of treatment are not homogeneous between studies, which may also explain discrepancies. This heterogeneity, combined with the lack of long-term effect evaluation of these drugs in animal models, further limits generalization to human settings. Finally, not only is the membrane bound form of ACE2 required for SARS-CoV-2 binding and infection, but other peptidases are also requisite on the host cells including furin and the transmembrane protease serine 2 (TMPRSS2), which further limits the interpretation of ACE2 expression as a direct explanation for facilitated viral entry (Hoffmann et al., 2020; Wrapp et al., 2020).
Another crucial difference between all experimental models is that although some of them specifically analyzed the impact of RAAS blockade on ACE2 in wild type animals or control conditions (Supplementary Table 1A), others used animal models of pathological conditions such as hypertension, heart failure, or organ injury, in order to analyze their effect in conditions for which they are commonly used in humans (Supplementary Tables 1A,B). However, these disease-models have complex and sometimes strong influences on ACE and ACE2 expressions and activities themselves. Indeed, animal models for diseases such as hypertension (Agata et al., 2006; Takeda et al., 2007; Yang et al., 2013) heart failure (Karram et al., 2005), or lung injury (Asperen et al., 2010; Wösten-van Asperen et al., 2011; Li et al., 2015) were associated with a decreased ACE2 protein expression in most observed tissues compared to wild type or control animals, when other models such as myocardial infarction mostly showed an increased ACE2 expression in the heart (Burrell et al., 2005; Ocaranza et al., 2006; Burchill et al., 2012). In the vast majority of these studies, RAAS blockers tended to restore a physiological level of ACE2 (Supplementary Tables 1A,B).
In summary, animal data on the expression of ACE2 in the presence of RAAS blockers yielded inconsistent results and it is highly uncertain that these treatments actually increase the expression of ACE2 in target organs of SARS-CoV-2. Interpretation of experimental data is all the more complex as (1) the effect differs depending on the animal model, (2) the effects of ACEIs, ARBs, or both combined, may differ, (3) the observed modifications of ACE2 expression vary depending on the tissue examined, (4) the effect on mRNA does not always correlate with the expression on the protein and/or its activity, (5) the level on soluble ACE2, often examined in models, does not reflect the level of transmembrane ACE2 (the only relevant one for viral cell entry), (6) biochemical evaluation of components of the RAAS is technically challenging, (7) other peptidases on the host cells are also requisite for viral entry, and (8) last and most importantly, these are only animals models, and these putative effects and mechanisms need to be confirmed in humans.
Of note, mineralocorticoid receptor antagonists may increase ACE2 expression to a larger extent than ACEIs and ARBs (Keidar et al., 2005; Kreutz et al., 2020).
In human, circulating ACE2 is found at very low levels in healthy subjects (Lew et al., 2008), is higher in men than in women (Soro-Paavonen et al., 2012; Ramchand et al., 2018, 2020), and increases with age (Rice et al., 2006). Plasma ACE2 has been shown to be increased in cardiovascular diseases such as heart failure (Epelman et al., 2009; Uri et al., 2016), coronary artery disease (Ortiz-Pérez et al., 2013; Ramchand et al., 2018), aortic stenosis (Ramchand et al., 2020), and atrial fibrillation (Walters et al., 2016). In addition, the level of plasma ACE2 is a biomarker of poor outcome in these conditions (Epelman et al., 2008, 2009; Ramchand et al., 2018; Ramchand and Burrell, 2020) as well as in the general population (Narula et al., 2020).
Human data on the influence of RAAS-blockers on ACE2 expression, independently of the effect of underlying diseases, is even sparser than animal data and summarized in Supplementary Table 2.
Although transmembrane ACE2 is the form of interest regarding viral entry into target cells, due to obvious difficulties to obtain tissue samples, the influence of RAAS blockers on protein expression of ACE2 in tissues of interest has rarely been studied in humans. In particular, human data on potential modifications of ACE2 expression in the lungs with RAAS-blockers are scarce. Very recently, using a panel of banked human tissue, Lee I. T. et al. (2020) reported that ACE2 robustly localizes within the motile cilia of airway epithelial cells. Interestingly, ACE2 expression was not increased in the nasal airway of ACEI or ARB users, and may even be slightly reduced in patients taking ACEIs. Three studies have described mRNA or protein expression of ACE2 in the kidney (Lely et al., 2004; Reich et al., 2008; Jiang et al., 2020). Neither ACE2 mRNA nor ACE2 protein expression were modified by the use of these drugs among patients with diabetes or membranous glomerulopathies (Lely et al., 2004). However, tubular ACE2 mRNA expression was found to be increased by ACEIs and ARBs in biopsies from patients with chronic allograft nephropathy or primary focal segmental glomerulosclerosis (Reich et al., 2008). In a recent study of more than 400 patients whose kidney transcriptomes were characterized by RNA-sequencing, no association between renal expression of ACE2 and RAAS-blockers was found. Interestingly, age was positively correlated with an increased mRNA expression in both kidney tissues and lung samples from the Genotype-Tissue Expression project (Jiang et al., 2020). In a study exploring the expression of ACE2 in the human intestine, ACEI users (n = 9) were shown to have increased ACE2 mRNA levels in duodenum biopsies (Vuille-dit-Bille et al., 2015) compared to non-users (n = 22), whereas ARBs were not associated with any modification in the expression of ACE2. Recently, in human myocardial samples, Stegbauer et al. (2020) showed that ACE2 protein level was increased in patients with severe aortic stenosis (but not in patients with severe mitral valve regurgitation) compared to control patients, and that ACEIs, but not ARBs, were associated with increased ACE2 expression (Stegbauer et al., 2020).
In most human studies, ACE2 expression was measured in plasma or urine samples (Supplementary Table 2). To our knowledge, only two studies reported an increased plasma ACE2 activity associated with RAAS blockers; Soro-Paavonen et al. (2012) showed an increase in plasma ACE2 activity among diabetic patients treated with ACEIs (a similar effect with ARBs was only observed in women), while Anguiano et al., reported that ARBs, but not ACEIs, increased plasma ACE2 activity in some subgroups of patients with chronic kidney disease (including those on dialysis). As reviewed in Supplementary Table 2, the reported effects of RAAS-blockers in all other studies were either an unmodified or decreased circulating ACE2. Indeed, several studies have examined the effect of RAAS blockers on plasma ACE2 in patients with heart failure, and consistently shown that the protein concentration and/or activity of the enzyme was either unchanged (Epelman et al., 2008; Uri et al., 2016; Chirinos et al., 2020), or decreased [in one cohort (Sama et al., 2020)], but never increased by these drugs. Similar results were found in chronic (Ramchand et al., 2018) or acute (Ortiz-Pérez et al., 2013) coronary artery disease, as well as in patients with aortic stenosis (Ramchand et al., 2020). A recent large study assessed the potential determinants of ACE2 levels in the general population within a subset of PURE (Prospective Urban Rural Epidemiology) participants. In 5216 subjects with hypertension, the authors found no associations between plasma ACE2 levels and ACEIs or ARBs (Narula et al., 2020).
Therefore, there is a large body of evidence suggesting that RAAS inhibitors do not upregulate plasma ACE2 in human. Of note, methodological considerations are again very important when interpreting studies reporting ACE2 activity, and may explain discrepancies. Indeed, as ACE can cleave the ACE2 fluorescent substrate commonly used to measure ACE2 activity, patients on ACEI may appear to have reduced ACE2 activities compared to controls when an ACE inhibitor is not included in the assay (Chappell, 2016).
Data obtained in urine samples are somewhat discrepant but overall do not support an increased ACE2 expression more than those obtained with plasma samples. Among diabetic patients, Liang et al. (2015) showed a decrease in urine ACE2 concentration with RAAS-blockers, when another study by Mariana et al. (2016) reported no effect of these drugs in patients with preserved renal function. Furuhashi et al. (2015) studied the effect of different anti-hypertensive medications among 100 hypertensive patients compared to 101 healthy controls. An increased urinary ACE2 protein concentration was found with the ARB olmesartan (received by 13 patients), but no significant change was observed with any other ARB (including losartan, telmisartan, valsartan, and candesartan) or with the ACEI enalapril. Of note, a distinct biological effect of olmesartan on ACE2 has not been reported elsewhere to our knowledge.
Very importantly, the level of expression of soluble ACE2 in plasma and urine may not reflect the tissue expression of transmembrane ACE2. Plasma ACE2 originates in part from shedding of the cell surface in tissues in which ACE2 is expressed, mainly in endothelial cells. ACE2 is cleaved from the cell membrane by ADAM17 (A disintegrin and metalloproteinase 17), and the regulation of this process is still poorly elucidated. In a recent study, Ramchand et al. (2020) analyzed plasma and myocardial expression of ACE2 in 22 patients with aortic stenosis and reported that higher circulating ACE2 levels were found in patients with reduced myocardial ACE2 gene expression, suggesting that increased levels of plasma ACE2 might actually reflect downregulation of the enzyme in tissues (Ramchand et al., 2020). Of note, a similar observation of opposite trends for myocardial and plasma activities of ACE2 was reported in dogs with heart failure (Larouche-Lebel et al., 2019). Regarding ACE2 expression in urine samples, ACE2 is unlikely to be physiologically filtered through the glomerulus due its size, so that urine ACE2 probably reflects its tubular expression (mainly in the apical membrane of the proximal tubule), after an ADAM17-mediated cleavage. Altogether, biological mechanisms underlying circulating ACE2 concentration/activity remain mostly hypothetical, as a modified ACE2 plasma concentration could reflect either a dysregulation of shedding or a modified tissue ACE2 expression.
Of note, even if an upregulation of circulating ACE2 was pharmacologically induced, this could actually be expected to be beneficial against SARS-CoV-2 viral infection by the binding of ACE2 to viral particles, preventing them to reach their target cells. This mechanism is the rationale for the therapeutic use of recombinant soluble ACE in the early phase of SARS-CoV-2 infection (Batlle et al., 2020; Monteil et al., 2020).
Overall, data regarding the expression of ACE2 in patients treated with RAAS blockers is scarce, is not in favor of an upregulation, and most importantly, there are no data showing an increased expression of the transmembrane ACE2 protein in the lung or upper respiratory tract (Kreutz et al., 2020), while an increase in the circulating form of the enzyme would not necessarily be deleterious and may even be protective. In addition, the binding of the viral spike protein to transmembrane ACE2 allows attachment of SARS-CoV-2 to the surface of target cells but is not the unique necessary step for viral cell entry. Penetration of the viral particle inside the cell requires the so-called priming of the spike protein by the cellular serine protease TMPRSS2, which then allows fusion of viral and cellular membranes (Hoffmann et al., 2020). In the murine study by Wu et al., mentioned above, the abundance of TMPRSS2 mRNA was not modified in any tissue after enalapril or losartan infusion.
Therefore, there is no solid experimental evidence supporting the concern that RAAS blockers may increase the expression of the transmembrane viral receptor and thereby facilitate its entry into the cell.
As explained above, whereas soluble ACE2 is expressed at very low levels in healthy subjects (Lew et al., 2008), it is markedly increased in cardiovascular disease. Therefore, although the impact of RAAS blockers on the expression of ACE2 remains highly speculative, the underlying conditions for which patients receive RAAS blockers on ACE2 may actually have a much more pronounced impact on ACE2 than these drugs. The reported increased risk of SARS-CoV-2 infection or severe disease in these conditions may indeed be mediated by modulations of ACE2, but these are more likely to be induced by the disease itself. The role of cardiovascular conditions as risk factors for COVID-19 deserves to be discussed and clarified.
The main reason for the initial concern regarding a potential deleterious role of RAAS blockers in COVID-19 arose from the early observation that more severe infections occurred in patients with cardiovascular comorbidities (Wu C. et al., 2020; Zhou et al., 2020b). An early and widely discussed risk factor was hypertension. In the first fairly large case-series published, describing patients in Wuhan (China), hypertension was highly prevalent in hospitalized-COVID-19 cases, and even more frequent among intensive care unit (ICU)-admitted patients (Wang D. et al., 2020). However, it was quickly noted that the vast majority of these results were not adjusted, even for age, whereas the prevalence of hypertension markedly increases with age (Forouzanfar et al., 2017; Wang et al., 2018). Indeed, although nearly all published studies reported an increased crude risk of mortality, ICU admission or severe disease among patients with hypertension, this association did not remain significant after adjusting for the main covariates, especially age and sex in the majority of the studies (Boulle et al., 2020; Bravi et al., 2020; Chen J. et al., 2020; Fried et al., 2020; Gupta et al., 2020; Iaccarino et al., 2020; Ioannou et al., 2020; Kim et al., 2020; Williamson et al., 2020; Yu et al., 2020).
However, a few large and properly adjusted studies still found a significant association between hypertension and mortality (Albitar et al., 2020; Berenguer et al., 2020; Cunningham et al., 2020; Giorgi Rossi et al., 2020; Hernández-Galdamez et al., 2020; Pan et al., 2020; Parra-Bracamonte et al., 2020; Reilev et al., 2020). A potential explanation for these partially discrepant results is that these studies included rather young patients, with a mean or median age below 50 years (Albitar et al., 2020; Cunningham et al., 2020; Hernández-Galdamez et al., 2020; Parra-Bracamonte et al., 2020). A study among very young hospitalized adults (18–34 years old) across the United States (USA) reported an adjusted odds ratio (OR) for death or mechanical ventilation of 2.36 among hypertensive adults (Cunningham et al., 2020). Accordingly, a large study conducted in the United Kingdom based on the National Health Service surveillance system which accounts for approximately 40% of the patients in the country evaluated the factors associated with COVID-19-related deaths compared to the general population (Williamson et al., 2020). The authors showed a strong and significant interaction between hypertension and age, hypertension being associated with a higher risk of mortality up to the age of 70 years, and a lower risk in older patients. Another potential explanation for discrepant results regarding hypertension is that obesity is very important confounder (Lighter et al., 2020; Mechanick et al., 2020; Simonnet et al., 2020) which was not always included in the models, especially as body mass index is a frequent missing data in databases.
Besides hypertension, diabetes mellitus, chronic kidney disease, and ischemic heart disease are other conditions frequently treated with RAAS blockers which were reported to be associated with poor outcome early in the pandemic.
Diabetes mellitus was independently associated with death in several studies. Petrilli et al. (2020) reported an adjusted hazard ratio (HR) of 1.24 (95% confidence interval [95% CI] 1.03; 1.5) for mortality among 5279 COVID-19 patients including 35% with diabetes in the United States. Similar results were reported in other occidental cohorts (Bravi et al., 2020; Giorgi Rossi et al., 2020; Iaccarino et al., 2020; Kim et al., 2020; Reilev et al., 2020; Sands et al., 2020; Williamson et al., 2020) and in the two Mexican case-series (Hernández-Galdamez et al., 2020; Parra-Bracamonte et al., 2020). In cohorts from China, results regarding diabetes have been more variable (Cen et al., 2020; Chen J. et al., 2020; Pan et al., 2020).
Chronic kidney disease was reported to be associated with an increased risk for mortality, independently of potential confounder, in multiple large-scaled studies across different regions worldwide (Berenguer et al., 2020; Boulle et al., 2020; Cariou et al., 2020; Chen J. et al., 2020; Fried et al., 2020; Hernández-Galdamez et al., 2020; Iaccarino et al., 2020; Ioannou et al., 2020; Kim et al., 2020; Mikami et al., 2020; Parra-Bracamonte et al., 2020; Perez-Guzman et al., 2020; Reilev et al., 2020; Williamson et al., 2020). Of note, a few other studies reported that the increased crude risk among chronic kidney disease patients was no-longer significant after adjusting for confounders (Cen et al., 2020; Chen L et al., 2020; Petrilli et al., 2020; van Gerwen et al., 2020). The proportion of patients with chronic kidney disease, and etiology of kidney injury varied markedly between all these studies, which may in part explain these differences. Interestingly, in a French multicenter study of 1,317 diabetic patients (CORONADO study), Cariou et al. (2020) reported adjusted odd ratios (ORs) of 2.14 for mortality [95% confidence interval (CI) 1.16; 3.94] for chronic kidney disease and 2.54 [95% CI 1.44; 4.50] for coronary artery disease.
Overall, in large-scale properly adjusted studies, diabetes mellitus and chronic kidney disease appear to be more frequently associated with mortality, and with higher adjusted risk, than hypertension (Chen J. et al., 2020; Williamson et al., 2020; Yu et al., 2020). Results regarding chronic heart disease are more discrepant (Giorgi Rossi et al., 2020; Ioannou et al., 2020; Kim et al., 2020; Parra-Bracamonte et al., 2020; Reilev et al., 2020), in part because most studies did not differentiate properly the underlying baseline cardiac comorbidities.
Results of studies cited in this paragraph which included more than 500 patients are summarized in Supplementary Table 3 which gives a large, although not exhaustive, overview of studies on the associations between cardio-metabolic comorbidities (in which RAAS blockers are frequently indicated and prescribed) and unadjusted and adjusted risk of adverse outcome in COVID-19.
While some authors were warning against the potential deleterious role of ACE2 and therefore of RAAS blockers, others have been claiming that restoring a disrupted ACE2-Ang1-7/ACE-Ang II homeostasis, for instance by using RAAS blockers, might actually be beneficial in COVID-19.
SARS-CoV-2, a betacoronavirus belonging to the 2B group, shares 70–80% genetic homology with SARS-CoV(-1) (Lu et al., 2020). When ACE2 was identified as the receptor for SARS-CoV in 2003 (3 years after its discovery), both in vitro (Li et al., 2003) and in vivo (Kuba et al., 2005), this led to investigate the role of ACE2 signaling in respiratory distress syndromes. In the lung, ACE2 is primarily expressed by type II alveolar epithelial cells, endothelial cells, and vascular smooth muscle cells (Wiener et al., 2007) and appears to play a crucial role in the pathophysiology of lung injury from various origins, even when not induced by SARS-CoV (Gu and Korteweg, 2007).
Early in the COVID-19 pandemic, in vitro studies have shown that SARS-CoV-2 and SARS-CoV shared the same receptor (Chen Y et al., 2020; Hoffmann et al., 2020). However, the affinity of SARS-CoV-2 for ACE2 was shown to be much higher than that of SARS-CoV (Chen Y et al., 2020; Wrapp et al., 2020). Shortly after, the pathogenicity of SARS-CoV-2 via ACE2 was confirmed in vivo in a murine model. Intranasally inoculated SARS-CoV-2 allowed viral replication accompanied by interstitial pneumonia and infiltration of inflammatory cells in the lung of transgenic mice bearing the human ACE2 gene, but not in wild-type mice (Bao et al., 2020).
The many clinical and biological resemblances between SARS-CoV (Lew, 2003; Lam et al., 2004) and SARS-CoV-2-related infections, and most importantly, their common receptor, have renewed interest in previous data generated in the years following the SARS outbreak in 2003.
As summarized in Table 1, the protective role of the ACE2-Ang (1-7)-Mas receptor pathway and, conversely, the deleterious role of the ACE-Ang II-AT1 receptor pathway, have been extensively demonstrated in multiple murine models of acute lung injury.
Table 1. Summary of the main experimental studies supporting a role of the ACE-Ang II/ACE2-Ang(1-7) homeostasis in lung injury.
In mouse models of severe lung injury induced by acid aspiration or sepsis, Imai et al., have shown that lung injury was worsened in ACE2−/− knock out mice compared to wild-type mice, and that recombinant human ACE2 protein partially rescued the phenotype. Conversely, the severity of acute lung injury was attenuated in ACE−/− knock out mice, in mice lacking the AT1 receptor, as well as in wild-type mice treated with the ARB losartan (Imai et al., 2005).
This crucial role of a disrupted ACE2-Ang (1-7)/ ACE-Ang II homeostasis balance has been confirmed by multiple other studies, in many other murine models of lung injury such as those induced by lipopolysaccharide (LPS) administration (Asperen et al., 2010; Wösten-van Asperen et al., 2011; Li et al., 2015; Ye and Liu, 2020), avian influenza A H5N1 (Yan et al., 2015), H7N9 (Yang et al., 2015), or respiratory syncytial virus (Gu et al., 2016) infections, seawater aspiration (Zhang et al., 2018), bleomycine administration (Wang L. et al., 2016), or hyperoxia (Fang et al., 2019). All these experimental models of acute lung injury have consistently shown a decreased lung expression of ACE2, and/or increased levels of plasma Ang II (Imai et al., 2005; Asperen et al., 2010; Wösten-van Asperen et al., 2011; Zou et al., 2014; Li et al., 2015; Yang et al., 2015; Gu et al., 2016; Fang et al., 2019), as well as a protective and deleterious roles of ACE2-Ang(1-7) and ACE-Ang II, respectively.
Regarding the specific pathogenicity of SARS-CoV, a series of experiments on lung injury induced by SARS-CoV or by the spike protein of the virus (Kuba et al., 2005) confirmed the crucial role of ACE2 as a receptor for the virus by showing that ACE2−/− knock out mice were markedly protected: viral replication as well as pulmonary lesions were strongly diminished compared with wild type mice. The authors also showed that upon SARS-CoV infection in wild-type mice, ACE2 protein expression in the lung was drastically reduced (Kuba et al., 2006; Gu and Korteweg, 2007). More specifically, binding of the spike protein of SARS-CoV to its receptor downregulated the latter both in vitro on cell lines and in vivo in the mouse. ACE2 downregulation disrupted the balance between ACE and ACE2 in the lung and increased levels of Ang-II, which then played a key role in lung injury. Downregulation of ACE2 induced by epithelial cell injury during acute respiratory distress syndrome might thereby be amplified by SARS-CoV-2 infection through endocytosis of ACE2 alongside viral particles.
In these models of lung injury, either induced by SARS-CoV or fromother origins, the ARB losartan (Imai et al., 2005; Kuba et al., 2005; Asperen et al., 2010; Wösten-van Asperen et al., 2011; Wong et al., 2012; Yan et al., 2015; Gu et al., 2016; Zhang et al., 2018; Ye and Liu, 2020) and to a lesser extent the ACEIs captopril (Li et al., 2015) or enalapril (Ye and Liu, 2020) have been shown to restore the ACE/ACE2 balance and to attenuate lung lesions and inflammation. Likewise, the administration of recombinant ACE2 (Imai et al., 2005; Zou et al., 2014; Gu et al., 2016; Wang L. et al., 2016), of ACE2 agonists (Fang et al., 2019), or of synthetic Ang (1-7) (Wösten-van Asperen et al., 2011; Zhang et al., 2018) have also been shown to attenuate lung injury. Interestingly, the ability of RAAS blockers to restore the ACE2-Ang (1-7)/ ACE-Ang II balance was not only demonstrated in models of lung injury but also in different models of cardiovascular disease (for instance in a pig model of cardiac arrest), as reviewed in Supplementary Tables 1A,B (Wang G. et al., 2016).
However, as outlined above for studies on pharmacologically induced modifications of ACE2 expression, it is very important while interpreting these studies to carefully consider the limitations of the biochemical assays to quantify the components of the RAAS (Chappell, 2016; Sparks et al., 2020; Chappell et al., 2021).
Importantly, although detailed mechanisms are beyond the scope of this review, the imbalance between the ACE-Ang II and ACE2-Ang (1-7) pathways also likely contributes to the endothelial dysfunction, to the inflammation and cytokine storm, and to the pro-thrombotic state observed in patients with severe forms of the disease. For instance, animal studies of LPS- or viral-induced lung injury have also shown that pharmacological blockade of the ACE-AngII-AT1 receptor pathway, or recombinant ACE2, two ways of restoring the ACE/ACE2 homeostasis, were associated with a decrease of cytokines such as interleukin-6 (IL-6) and tumor necrosis factor alpha (TNF-alpha) (Wong et al., 2012; Yan et al., 2015), and inhibited pivotal inflammatory mediators such as Toll-like receptor 4 (TLR4) (Ye and Liu, 2020), or NF-κB signaling pathways (Li et al., 2016; Fang et al., 2019). Reviews of these other crucial roles of ACE2 deregulation upon SARS-CoV-2 infection can be found elsewhere (Du et al., 2020; Pons et al., 2020; Zhang J. et al., 2020).
Overall, there are solid experimental data supporting a potential protective role of RAAS-blockers in SARS-CoV-2 pneumonia, through a restored ACE2/ACE balance. This is well-illustrated by the very large numbers of studies implemented early in the pandemic to examine whether ARBs such as losartan may be beneficial in patients with COVID-19, as detailed below.
In line with these solid experimental results, there are some data supporting a similar role for the RAAS, and more specifically protective and deleterious roles for the ACE2-Ang (1-7)-Mas receptor and ACE-Ang II-AT1 receptor pathways, respectively, in human pneumonia and acute respiratory distress syndrome.
Interestingly, a study from China showed increased levels of Ang II in patients with COVID-19 (Liu Y. et al., 2020). In pneumoniae of other origins, such as infections with respiratory syncytial virus in children (Gu et al., 2016), H5N1 (Zou et al., 2014) or H7N9 (Huang et al., 2014; Yang et al., 2015) in adults, small-scaled studies had previously shown increased levels of Ang II in the serum of infected patients, especially in the acute phase of the disease, and that Ang II levels may be associated with disease progression. Of note, in some of these studies (Huang et al., 2014; Zou et al., 2014), the range order of reported Ang II concentrations, including in control subjects (from 1,000 to 10,000 pg/mL, highly variable, and much higher than expected concentrations) questions the methodology used to measure Ang II (Chappell et al., 2021). Importantly, these results obtained in pneumoniae of various origins show that the fact that ACE2 is the viral receptor may amplify disruption of the ACE2/ACE balance, but is not required for this phenomenon.
About half of the variance in plasma ACE activity is explained by the insertion/deletion (I/D) polymorphisms of the enzyme, the D allele being associated with a higher activity. In a population of 96 patients with acute respiratory distress syndrome, the DD genotype frequency was found to be higher than in several control cohorts (Marshall et al., 2002). Similarly, in a cohort of 44 Vietnamese patients with SARS in 2003, the frequency of the D allele was significantly higher in the hypoxemic group than in the non-hypoxemic group (Itoyama et al., 2004).
However, data supporting a protective role of RAAS blockers in human pneumonia are a lot scarcer than in experimental models. In a meta-analysis, Caldeira et al. (2012) have shown that patients treated with ACEIs had a lower incidence of pneumonia (OR 0.66, 95% CI 0.55–0.80 in a total of 19 studies), although a similar effect was not observed for ARBs. In the case of SARS-CoV-2 and COVID-19, numerous observational studies that have examined the link between these treatments and severity of the disease are summarized in the third part of this review.
Very early in the pandemic, most scientific societies took position (Bavishi et al., 2020), all in favor of a continued use of both ACEIs and ARBs in patients taking these medication as part of their chronic treatment (Table 2). These recommendations were based on the theoretical considerations and experimental data detailed above, on the clear cardiovascular and renal benefits associated with these medications (Vaduganathan et al., 2020), and on the well-documented risk associated with the discontinuation of RAAS blockers — at least in some indications such as heart failure (Halliday et al., 2019). However, these recommendations were issued before any clinical evidence was available to directly support them, and since then, many studies, mostly observational, have been published to address this issue and are summarized below and in Supplementary Tables 4A,B. However, as recently reviewed (Cohen et al., 2020a), observational studies designed to analyze the effects of RAAS blockers in the COVID-19 pandemic suffer methodological flaws and need to be interpreted with great caution. Many clinical trials have been implemented to provide definite answers regarding the management of these drugs in the context of COVID-19 (Supplementary Table 5).
Table 2. Recommendations from scientific societies regarding the use of RAAS blockers in the COVID-19 pandemic.
Several studies have evaluated whether the chronic use of RAAS blockers was associated with an increased risk to contract a SARS-CoV-2 infection (Supplementary Table 4A). Mancia et al. (2020) conducted a population-based case-control study in the Lombardy region of Italy including a total of 6,272 patients with a confirmed SARS-CoV-2 infection matched with 30,759 beneficiaries of Regional Health Service as controls. Even though the use of ACEIs and ARBs was more frequent among patients than controls, this was driven by their higher prevalence of cardiovascular diseases. After adjustment, neither ACEIs (adjusted OR 0.96 and 95% CI 0.87–1.07) nor ARBs [0.95 (0.86–1.05)] were associated with COVID-19 or with a severe or fatal course of the disease. Similar conclusions were reported in two studies conducted in the United States. Mehta et al. (2020) analyzed data from 18,742 patients tested for COVID-19 in Ohio and Florida, of whom 2,285 were taking an ACEI or an ARB, and 1,735 had a positive test. No significant association was found between the use of RAAS blockers and test positivity (overlap propensity score–weighted OR, 0.97; 95%CI, 0.81–1.15) (Mehta et al., 2020). Likewise, in 12,594 patients tested for COVID-19 (of whom 5,894 were positive) in the state of New-York, Reynolds et al. (2020) reported that the likelihood of a positive test was not increased in users of ACEIs/ARBs compared to matched patients, in the total population as well as in the subgroup of hypertensive patients. In a case-control analysis of 571 patients with COVID-19 and 5,710 age- and sex-matched controls, all with hypertension, in Denmark, Fosbøl et al. (2020) reported that ACEI/ARB use was not significantly associated with a higher incidence of COVID-19 compared with other antihypertensive drugs [adjusted HR, 1.05 (95%CI, 0.80–1.36)]. In a case-control study conducted in South Korea using data from the Korean National Health Insurance System, 950 COVID-19 cases among 16,281 subjects with hypertension were retrospectively matched with 1,897 not infected controls, and multivariable-adjusted logistic regression demonstrated the absence of a significant association between exposure to RAAS-blockers and risk of COVID-19 (adjusted OR 1.161 [0.958–1.407)] (Son et al., 2020). Finally, in a large Israeli dataset of 14,520 individuals tested for SARS-CoV-2, of whom 1,317 were found positive, although ACEIs/ARBs were more frequent in positive cases than in negative cases, a multivariable logistic regression model found not significant association between the use of these medications and a positive result (adjusted OR = 1.19; 95% CI 0.96–1.47) (Chodick et al., 2020). A study conducted in Spain had a different design as it aimed to analyze the association between chronic use of RAAS blockers (compared to other antihypertensive drugs) and the risk of COVID-19 requiring hospital admission in 1,139 cases admitted with COVID-19 in seven hospitals in Madrid versus 11,390 matched controls admitted in 2018. Compared with users of other antihypertensive drugs, users of RAAS inhibitors had an adjusted OR for COVID-19 requiring admission to hospital of 0.94 (95% CI 0⋅77–1⋅15). Similar results were found for fatal cases and patients admitted to intensive care units (de Abajo et al., 2020).
Overall, the vast majority of observational studies, in different regions of the world, with different designs and adjustment procedures, consistently concluded that long-term treatment with RAAS-blockers was not associated with an increased adjusted risk of SARS-CoV-2 infection (Supplementary Table 4A).
Multiple observational studies had a different setting as they aimed at evaluating the association between chronic use of RAAS-blockers and outcome of the disease in patients with established COVID-19 infection and are listed in Supplementary Table 4B.
Most studies were conducted in hospitalized patients. Some had norestriction on hypertension and analyzed the association between RAASblockers and outcome in unselected inpatients with COVID-19 (Bean et al., 2020; Holt et al., 2020; Iaccarino et al., 2020; Lahens et al., 2020; Liabeuf et al., 2020; Shah et al., 2020). Except for onestudy which showed an increased risk of severe disease despite – potentially insufficient – adjustment (Liabeuf et al., 2020), and anotherstudy which found a negative association between RAAS blockerexposure and the composite of death or transfer to ICU(Bean et al., 2020), all other studies, and in particular all thosewhich analyzed mortality as an outcome, found no association betweenexposure and outcome after adjustment, although an increased risk was frequent in crude analyses. For instance, in an Italian cohort of 1,581 patients admitted for COVID-19 in 26 hospitals, Iaccarino et al. (2020) reported that neither ACEIs nor ARBS were associated with mortality after adjustment for confounders, although ACEIs were more frequently used in non-survivors. Similarly, in 531 African American patients hospitalized with COVID-19 in Georgia (United States), of whom 207 were on ACEIs/ARBs at baseline, after adjustment for covariates, there was no difference between users and non-users of these drugs in outcomes including mortality (Shah et al., 2020). Other studies restricted analyses to hypertensive patients (Gao et al., 2020; Pan et al., 2020; Tedeschi et al., 2020), or reported results in the subgroup of hypertensive patients (Andrea et al., 2020; Richardson et al., 2020; Trifirò et al., 2020). Similarly, the vast majority of these studies found no association between RAAS blockers and outcome, and in hypertensive patients this was true in unadjusted as well as adjusted analyses. Trifirò et al. (2020) analyzed the charts from 42,926 COVID-19 hospitalized patients by combining multiple Italian databases accounting for approximately a quarter of the Italian population. Almost 50% of the patients had at least one antihypertensive drug claim within 3 months prior to admission. Compared to calcium-channel blockers users, adjusted analyses showed no difference in the risk of death among ACEI (Hazard ratio (HR) 1.01, 95% CI [0.92; 1.12)] or ARB (HR 1.03, 95% CI [0.93; 1.14)] users (Trifirò et al., 2020).
A few studies included both inpatients and outpatients. Again, some had no restriction on hypertension (Fosbøl et al., 2020; Mehta et al., 2020) while others reported results in hypertensive patients (Bravi et al., 2020; Felice et al., 2020; Jung S.Y. et al., 2020; Reynolds et al., 2020; Son et al., 2020) or in patients with an indication for RAAS blockers (Giorgi Rossi et al., 2020). Overall, in most studies, findings were similar to those obtained in hospitalized patients, with no significant association between chronic RAAS blocker exposure and outcome of COVID-19 in adjusted analyses. In a nationwide population-based cohort study of 5,179 confirmed COVID-19 cases in South Korea, Jung et al., found that prior use of RAAS blockers was associated with an increased risk of in-hospital mortality in unadjusted analyses [OR 3.88, 95% CI (2.48; 6.05)], but this difference was ironed out when adjusted for age, sex, Charlson comorbidity index, immunosuppression and hospital type [adjusted OR: 0.88, 95% CI ([0.53; 1.44)]. In the subgroup of 1,157 hypertensive patients, there was no association between RAAS blockers and mortality, both in unadjusted [OR 0.74, 95% CI (0.43; 1.28)] and adjusted [0.71, 95% CI (0.40; 1.26)] analyses (Jung S.Y. et al., 2020). In a Danish retrospective cohort of 4,480 patients with COVID-19, of whom 895 were chronic ACEI/ARB users in a 6-month period prior to diagnosis, the unadjusted HR for mortality was 2.65 [95% CI (2.18; 3.23)] while the adjusted HR, after accounting for age and medical history, was 0.83 [95% CI (0.67; 1.03)] (Fosbøl et al., 2020). Reynolds et al. (2020) reported similar results among a cohort of 5,894 patients with COVID-19, of whom 2,573 with hypertension. After careful adjustment, no significant association was found between chronic-exposure to ACEIs/ARBs and severe illness, defined as a composite of intensive care admission, mechanical ventilation, or death, in all patients as well as in hypertensive patients (Reynolds et al., 2020).
Altogether, if some studies conducted in unselected population identified an association of RAAS blocker prescription with mortality in unadjusted analyses, once adjustment for age, sex and comorbidities was performed, chronic use of RAAS-blockers was not associated with worse outcome (in particular mortality) among patients with COVID-19, hospitalized or not. All these studies support the above-mentioned recommendations from scientific societies, not to discontinue these treatments despite the ongoing pandemic.
One randomized trial (CORONACION, NCT 04330300) had been designed to address this specific question in Ireland. The aim was to randomize patients with hypertension taking ACEIs/ARBs to either continue or switch to an alternative blood pressure medication and analyze COVID-19-related events. However, this trial has been interrupted due to a low incidence of COVID-19 in the study site.
Although potentially highly relevant, the specific role of mineralocorticoid receptor antagonists has been less studied, in part because these drugs are much less frequently prescribed than ACEIs and ARBs. In a recent very large observational study from a Swedish national registry, among 1,387,746 patients with a potential indication for RAAS blockers, 5.8% received a mineralocorticoid receptor antagonist. These medications were not associated with the risks of hospitalization for COVID-19 or mortality after adjustment for confounders (Savarese et al., 2020).
Most studies which analyzed “in-hospital” (and not chronic) exposure to treatment showed a strong protective effect associated with the use of RAAS blockers after adjustment for baseline comorbidities (Cannata et al., 2020; Chaudhri et al., 2020; Meng et al., 2020; Yang et al., 2020; Zhang P et al., 2020; Zhou et al., 2020a). For instance, in a retrospective analysis of 1,128 COVID-19 patients with hypertension admitted in 9 hospitals in the epicenter region of the pandemic in Hubei, China, authors compared patients based on their in-hospital anti-hypertensive regimen. They recorded 188 patients receiving ACEI/ARB during hospitalization and all-cause mortality at 28 days was significantly lower among them. This effect remained significant in a mixed-effect Cox model (using site as a random effect, after adjusting for age, gender, comorbidities, and in-hospital medications), with an adjusted hazard ratio of 0.42 [0.19–0.92] and in a propensity score-matched analysis (adjusted HR, 0.37; 95% CI, 0.15–0.89) (Zhang P et al., 2020).
However, as outlined in a letter by Cohen et al. (2020b) to warn the reader against interpretation of the study by Zhang P et al. (2020), as demonstrated in a dedicated study from our team (Lahens et al., 2020), and as recently discussed in a review on the methodology of observational studies focused on the issue of RAAS blockers and COVID-19 (Cohen et al., 2020a), this protective effect is majorly biased. Indeed, our study showed that cessation of a chronic RAAS blocker exposure upon hospital admission is frequent and occurs in those with the worst outcomes, and conversely for treatment continuation (Lahens et al., 2020). Therefore, treatment discontinuation is related directly or indirectly to disease severity and mortality. This induces a phenomenon of reverse causality whereby severity of the disease causes treatment cessation, and not the reverse. Conversely, the continued treatment arm is prone to immortal-time bias (Suissa, 2008). Overall, this generates a “healthy user-sick stopper bias” explaining that studies based on in-hospital exposure (instead of chronic exposure) find a spurious protective association between RAAS blockers and outcome in COVID-19 (Supplementary Table 4B).
Observational pharmaco-epidemiological studies are not suited to analyze the association between in-hospital exposure to RAAS blockers and outcome of COVID-19. The answer to this issue can only be provided by interventional randomized trials.
It is very important to note that most meta-analyses to date meant to analyze the association between RAAS-blockers and outcome have incorporated studies based on in-hospital exposure (Baral et al., 2020; Flacco et al., 2020; Greco et al., 2020; Grover and Oberoi, 2020; Guo X. et al., 2020; Mackey et al., 2020; Pranata et al., 2020; Zhang X. et al., 2020), so that their conclusions should be interpreted with great caution.
As of January 2021, optimal management of ACEIs/ARBs in patients with a COVID-19 infection remains uncertain. Several trials randomizing COVID-19 patients previously treated with RAAS blockers and admitted to hospital for treatment continuation or discontinuation (ACORES-2 in France, NCT04329195; ACEI-COVID in Austria, NCT04353596; RASCOVID-19 in Denmark, NCT04351581; RAASCOVID in Canada, NCT04508985; SWITCH-COVID in Brazil, NCT04493359) are currently recruiting (Supplementary Table 5), while results have been published for two trials.
The BRACE-CORONA study (NCT04364893) enrolled 659 patients hospitalized with a confirmed diagnosis of COVID-19 from 29 sites in Brazil, with chronic use of ACEIs/ARBs and randomly allocated to continuing or stopping these treatments for 30 days (Lopes et al., 2020). Results were presented at the European Society of Cardiology Congress in September 2020 and published very recently (Lopes et al., 2021). No difference was reported in the number of days alive and out of hospital at 30 days (primary outcome) between the suspending ACEIs/ARBs group and the continuing group. There was no difference in all-cause mortality at 30 days either (HR 0.97 (95% CI [0.38; 2.52)].
An international randomized trial (REPLACE COVID, NCT04338009) was published in January 2021: 152 patients hospitalized for COVID-19 and receiving an ACEI or an ARB before admission were randomly assigned to continuation or discontinuation of this treatment. No difference in the primary endpoint assessing severity of disease course (a global rank score across four hierarchical tiers incorporating time to death, duration of mechanical ventilation, time on renal replacement or vasopressor therapy, and multiorgan dysfunction during the hospitalization) was observed between patients who continued or discontinued RAAS blocker therapy (Cohen et al., 2021). The authors concluded that RAAS blockers can be safely continued in patients with COVID-19 requiring hospital admission.
An even larger number of trials are testing the hypothesis that RAAS blockers, and in particular ARBs, might be beneficial in patients with SARS-CoV-2 pneumonia. We have identified 21 such trials, listed in Supplementary Table 5. For instance, two trials sponsored by the University of Minnesota (United States) are testing the efficiency of losartan in patients with COVID-19 either requiring hospitalization (assessing the respiratory severity at day 7, NCT04312009) or not requiring hospitalization (assessing the rate of hospital admission within 15 days of randomization, NCT04311177). Other trials are focusing on elderly patients: two French trials are assessing the effectiveness of telmisartan in elderly hospitalized patients (COVID-Aging, coordinated in Strasbourg, evaluating the 2-week survival rate, NCT04359953) or in outpatients (COVERAGE, coordinated in Bordeaux, evaluating a composite of hospitalization or death at day 14, NCT04356495). Other ongoing trials worldwide are listed in Supplementary Table 5.
Whereas an increased tissue expression of ACE2, either due to underlying conditions or to pharmacological treatment, might potentially facilitate SARS-CoV-2 infection and/or more severe forms of the disease on the one hand, on the other hand a higher ACE2 activity could be beneficial in infected patients by attenuating lung injury and inflammation. Because of these opposite potential effects of ACE2 on the disease (Wang K. et al., 2020), the role of RAAS blockers, which may modulate ACE2 expression and activity, is controversial (Figure 2).
Figure 2. Summarizing illustration. Theoretical pros and cons in the debate on the use of RAAS blockers in the context of the COVID-19 pandemic, and available experimental and clinical evidence to date. Overall, there are no evidence-based data supporting the discontinuation of these medications in the general population, or in patients with COVID-19. In hospitalized patients, these drugs should be managed according to usual clinical practice, taking into account hemodynamics and kidney function.
Several large studies seem to have ruled out that chronic exposure to RAAS blockers may predispose patients to infection, while studies on the association between exposure to RAAS blockers and severity of the disease in patients with COVID-19 have yielded more discrepant results. However, these apparent discrepancies are largely explained by disparities in study design, exposure measurement, adjustment methodologies, and often small sample size. Properly designed studies are also reassuring and consistently found no significant association between chronic exposure to RAAS blockers and outcome in infected patients. Preliminary results from randomized clinical trials did not raise concern regarding the continued use of the treatments in hospitalized patients (Figure 2).
Overall, available data corroborate statements from scientific societies against the preventive discontinuation of RAAS blockers in the general population, while management of these medications in infected patients, especially in hospitalized patients, should be clarified by the results of ongoing studies.
Despite extensive worldwide research since the discovery of ACE2 in 2000, the SARS outbreak in 2003, and the COVID-19 pandemic since December 2019, many gaps in knowledge remain regarding the regulation of ACE2 and its implications in the pathogenesis of SARS-CoV-2. The discrepancies in the literature highlight the need for integrated translational research projects, from molecular biology to animal and human pathophysiology, especially as many potential therapeutic targets will be directly impacted by a better knowledge of ACE2, a double-edged sword against the virus.
EV-P conceived the concept of the manuscript, drew the figures, and revised the manuscript and tables. SBG wrote the first draft of the manuscript and tables. All authors contributed to the literature review, wrote iterative drafts of manuscript and tables, and approved the final version.
The authors declare that the research was conducted in the absence of any commercial or financial relationships that could be construed as a potential conflict of interest.
The Supplementary Material for this article can be found online at: https://www.frontiersin.org/articles/10.3389/fphys.2021.624052/full#supplementary-material
Supplementary Table 1 | Influence of pharmacological blockade of the renin-angiotensin system on the expression of ACE2: animal models.
Supplementary Table 2 | Influence of the pharmacological blockade of the renin-angiotensin system on the expression of ACE2: human studies.
Supplementary Table 3 | Representative selection of large-scaled studies on the association between cardiovascular or metabolic comorbidities and outcome in COVID-19.
Supplementary Table 4 | Observational studies evaluating the impact of ACEIs/ARBs on the risk of a positive COVID-19 test (A) and on the course of the disease in infected patients (B).
Supplementary Table 5 | Ongoing clinical trials (last update: January 25th 2021).
Agata, J., Ura, N., Yoshida, H., Shinshi, Y., Sasaki, H., Hyakkoku, M., et al. (2006). Olmesartan is an Angiotensin II receptor blocker with an inhibitory effect on angiotensin-converting enzyme. Hypertens. Res. 29, 865–874. doi: 10.1291/hypres.29.865
Albitar, O., Ballouze, R., Ooi, J. P., and Sheikh Ghadzi, S. M. (2020). Risk factors for mortality among COVID-19 patients. Diabetes Res. Clin. Pract. 166, 108293. doi: 10.1016/j.diabres.2020.108293
Andrea, C., Francesco, M., Antonio, N., Evgeny, F., Marzia, S., Fabio, C., et al. (2020). Renin-angiotensin-aldosterone system inhibitors and outcome in patients with SARS-CoV-2 pneumonia: a case series study. Hypertension 76, e10–e12. doi: 10.1161/HYPERTENSIONAHA.120.15312
Anguiano, L., Riera, M., Pascual, J., Valdivielso, J. M., Barrios, C., Betriu, A., et al. (2015). Circulating angiotensin-converting enzyme 2 activity in patients with chronic kidney disease without previous history of cardiovascular disease. Nephrol. Dial. Transplant. 30, 1176–1185. doi: 10.1093/ndt/gfv025
Aronson, J. K., and Ferner, R. E. (2020). Drugs and the renin-angiotensin system in covid-19. BMJ 369:m1313. doi: 10.1136/bmj.m1313
Asperen, R. M. W., Lutter, R., Specht, P. A. C., van Woensel, J. B., van der Loos, C. M., Florquin, S., et al. (2010). Ventilator-induced inflammatory response in lipopolysaccharide-exposed rat lung is mediated by angiotensin-converting enzyme. The Am. J. Pathol. 176, 2219–2227. doi: 10.2353/ajpath.2010.090565
Bao, L., Deng, W., Huang, B., Gao, H., Liu, J., Ren, L., et al. (2020). The pathogenicity of SARS-CoV-2 in hACE2 transgenic mice. Microbiology 583, 830–833. doi: 10.1101/2020.02.07.939389
Baral, R., White, M., and Vassiliou, V. S. (2020). Effect of renin-angiotensin-aldosterone system inhibitors in patients with COVID-19: a systematic review and meta-analysis of 28,872 patients. Curr. Atheroscler. Rep. 22:61. doi: 10.1007/s11883-020-00880-6
Batlle, D., Wysocki, J., and Satchell, K. (2020). Soluble angiotensin-converting enzyme 2: a potential approach for coronavirus infection therapy? Clin. Sci. 134, 543–545. doi: 10.1042/CS20200163
Bavishi, C., Maddox, T. M., and Messerli, F. H. (2020). Coronavirus Disease 2019 (COVID-19) infection and renin angiotensin system blockers. JAMA Cardiol. 5:745. doi: 10.1001/jamacardio.2020.1282
Bean, D. M., Kraljevic, Z., Searle, T., Bendayan, R., Kevin, O., Pickles, A., et al. (2020). Angiotensin-converting enzyme inhibitors and angiotensin II receptor blockers are not associated with severe COVID-19 infection in a multi-site UK acute hospital trust. Eur. J. Heart Fail. 22, 967–974. doi: 10.1002/ejhf.1924
Berenguer, J., Ryan, P., Rodríguez-Baño, J., Jarrín, I., Carratalà, J., Pachón, J., et al. (2020). Characteristics and predictors of death among 4035 consecutively hospitalized patients with COVID-19 in Spain. Clin. Microbiol. Infect. 26, 1525–1536. doi: 10.1016/j.cmi.2020.07.024
Boulle, A., Davies, M.-A., Hussey, H., Ismail, M., Morden, E., Vundle, Z., et al. (2020). Risk factors for COVID-19 death in a population cohort study from the Western Cape Province, South Africa. Clin. Infect. Dis. ciaa1198. doi: 10.1093/cid/ciaa1198
Bravi, F., Flacco, M. E., Carradori, T., Volta, C. A., Cosenza, G., De Togni, A., et al. (2020). Predictors of severe or lethal COVID-19, including angiotensin converting enzyme inhibitors and Angiotensin II receptor blockers, in a sample of infected Italian citizens. PLoS One 15:e0235248. doi: 10.1371/journal.pone.0235248
Burchill, L. J., Velkoska, E., Dean, R. G., Griggs, K., Patel, S. K., and Burrell, L. M. (2012). Combination renin–angiotensin system blockade and angiotensin-converting enzyme 2 in experimental myocardial infarction: implications for future therapeutic directions. Clin. Sci. 123, 649–658. doi: 10.1042/CS20120162
Burrell, L. M., Burchill, L., Dean, R. G., Griggs, K., Patel, S. K., and Velkoska, E. (2012). Chronic kidney disease: cardiac and renal angiotensin-converting enzyme (ACE) 2 expression in rats after subtotal nephrectomy and the effect of ACE inhibition: angiotensin-converting enzyme 2 and chronic kidney disease. Exp. Physiol. 97, 477–485. doi: 10.1113/expphysiol.2011.063156
Burrell, L. M., Risvanis, J., Kubota, E., Dean, R. G., MacDonald, P. S., Lu, S., et al. (2005). Myocardial infarction increases ACE2 expression in rat and humans. Eur. Heart J. 26, 369–375; discussion 322–324.
Caldeira, D., Alarcao, J., Vaz-Carneiro, A., and Costa, J. (2012). Risk of pneumonia associated with use of angiotensin converting enzyme inhibitors and angiotensin receptor blockers: systematic review and meta-analysis. BMJ 345:e4260. doi: 10.1136/bmj.e4260
Cannata, F., Chiarito, M., Reimers, B., Azzolini, E., Ferrante, G., My, I., et al. (2020). Continuation versus discontinuation of ACE inhibitors or angiotensin II receptor blockers in COVID-19: effects on blood pressure control and mortality. Eur. Heart J. Cardiovasc. Pharmacother. 6, 412–414. doi: 10.1093/ehjcvp/pvaa056
Cariou, B., Hadjadj, S., Wargny, M., Pichelin, M., Al-Salameh, A., Allix, I., et al. (2020). Phenotypic characteristics and prognosis of inpatients with COVID-19 and diabetes: the CORONADO study. Diabetologia 63, 1500–1515. doi: 10.1007/s00125-020-05180-x
Cen, Y., Chen, X., Shen, Y., Zhang, X.-H., Lei, Y., Xu, C., et al. (2020). Risk factors for disease progression in patients with mild to moderate coronavirus disease 2019—a multi-centre observational study. Clin. Microbiol. Infect. 26, 1242–1247. doi: 10.1016/j.cmi.2020.05.041
Chappell, M. C. (2016). Biochemical evaluation of the renin-angiotensin system: the good, bad, and absolute? Am. J. Physiol. Heart Circ. Physiol. 310, H137–H152. doi: 10.1152/ajpheart.00618.2015
Chappell, M. C., Pirro, N. T., South, A. M., and Gwathmey, T. M. (2021). Concerns on the specificity of commercial ELISAs for the measurement of Angiotensin-(1-7) and Angiotensin II in human plasma. Hypertension [Epub ahead of print]. doi: 10.1161/HYPERTENSIONAHA.120.16724
Chaudhri, I., Koraishy, F. M., Bolotova, O., Yoo, J., Marcos, L. A., Taub, E., et al. (2020). Outcomes associated with the use of renin-angiotensin-aldosterone system blockade in hospitalized patients with SARS-CoV-2 infection. Kidney 360, 801–809. doi: 10.34067/KID.0003792020
Chen, J., Bai, H., Liu, J., Chen, G., Liao, Q., Yang, J., et al. (2020). Distinct clinical characteristics and risk factors for mortality in female inpatients with Coronavirus Disease 2019 (COVID-19): a sex-stratified, large-scale cohort study in Wuhan, China. Clinical Infectious Diseases [Epub ahead of print]. doi: 10.1093/cid/ciaa920
Chen, L., Yu, J., He, W., Chen, L., Yuan, G., Dong, F., et al. (2020). Risk factors for death in 1859 subjects with COVID-19. Leukemia 34, 2173–2183. doi: 10.1038/s41375-020-0911-0
Chen, Y., Guo, Y., Pan, Y., and Zhao, Z. J. (2020). Structure analysis of the receptor binding of 2019-nCoV. Biochem. Biophys. Res. Commun. 525, 135–140. doi: 10.1016/j.bbrc.2020.02.071
Chirinos, J. A., Cohen, J. B., Zhao, L., Hanff, T., Sweitzer, N., Fang, J., et al. (2020). Clinical and proteomic correlates of Plasma ACE2 (Angiotensin-Converting Enzyme 2) in human heart failure. Hypertension 76, 1526–1536. doi: 10.1161/HYPERTENSIONAHA.120.15829
Chodick, G., Nutman, A., Yiekutiel, N., and Shalev, V. (2020). Angiotensin-converting enzyme inhibitors and angiotensin-receptor blockers are not associated with increased risk of SARS-CoV-2 infection. J. Travel Med. 27:taaa069. doi: 10.1093/jtm/taaa069
Cohen, J. B., D’Agostino-McGowan, L., Jensen, E. T., Rigdon, J., and South, A. M. (2020a). Evaluating sources of bias in observational studies of angiotensin-converting enzyme inhibitor/angiotensin II receptor blocker use during coronavirus disease 2019: beyond confounding. J. Hypertens. [Epub ahead of print]. doi: 10.1097/HJH.0000000000002706
Cohen, J. B., Hanff, T. C., South, A. M., Sparks, M. A., Hiremath, S., Bress, A. P., et al. (2020b). Response by cohen et al to letter regarding article, “association of inpatient use of angiotensin-converting enzyme inhibitors and Angiotensin II receptor blockers with mortality among patients with hypertension hospitalized with COVID-19”. Circ. Res. 126, e140–e141. doi: 10.1161/CIRCRESAHA.120.317205
Cohen, J. B., Hanff, T. C., William, P., Sweitzer, N., Rosado-Santander, N. R., Medina, C., et al. (2021). Continuation versus discontinuation of renin–angiotensin system inhibitors in patients admitted to hospital with COVID-19: a prospective, randomised, open-label trial. Lancet Respir. Med. [Epub ahead of print]. doi: 10.1016/S2213-2600(20)30558-0
Cunningham, J. W., Vaduganathan, M., Claggett, B. L., Jering, K. S., Bhatt, A. S., Rosenthal, N., et al. (2020). Clinical outcomes in young us adults hospitalized with COVID-19. JAMA Internal Medicine [Epub ahead of print]. doi: 10.1001/jamainternmed.2020.5313
de Abajo, F. J., Rodríguez-Martín, S., Lerma, V., Mejía-Abril, G., Aguilar, M., García-Luque, A., et al. (2020). Use of renin–angiotensin–aldosterone system inhibitors and risk of COVID-19 requiring admission to hospital: a case-population study. Lancet 395, 1705–1714. doi: 10.1016/S0140-6736(20)31030-8
Deshotels, M. R., Xia, H., Sriramula, S., Lazartigues, E., and Filipeanu, C. M. (2014). Angiotensin II mediates angiotensin converting enzyme type 2 internalization and degradation through an Angiotensin II Type I receptor–dependent mechanism. Hypertension 64, 1368–1375. doi: 10.1161/HYPERTENSIONAHA.114.03743
Diaz, J. H. (2020). Hypothesis: angiotensin-converting enzyme inhibitors and angiotensin receptor blockers may increase the risk of severe COVID-19. J. Travel Med. 27:taaa041. doi: 10.1093/jtm/taaa041
Donoghue, M., Hsieh, F., Baronas, E., Godbout, K., Gosselin, M., Stagliano, N., et al. (2000). A novel angiotensin-converting enzyme–related carboxypeptidase (ACE2) converts Angiotensin I to Angiotensin 1-9. Circ. Res. 87, E1–E9. doi: 10.1161/01.RES.87.5.e1
Du, F., Liu, B., and Zhang, S. (2020). COVID-19: the role of excessive cytokine release and potential ACE2 down-regulation in promoting hypercoagulable state associated with severe illness. J. Thromb. Thrombol. [Epub ahead of print]. doi: 10.1007/s11239-020-02224-2
Epelman, S., Shrestha, K., Troughton, R. W., Francis, G. S., Sen, S., Klein, A. L., et al. (2009). Soluble angiotensin-converting enzyme 2 in human heart failure: relation with myocardial function and clinical outcomes. J. Cardiac. Fail. 15, 565–571. doi: 10.1016/j.cardfail.2009.01.014
Epelman, S., Tang, W. H. W., Chen, S. Y., Van Lente, F., Francis, G. S., and Sen, S. (2008). Detection of soluble angiotensin-converting enzyme 2 in heart failure. J. Am. Coll. Cardiol. 52, 750–754. doi: 10.1016/j.jacc.2008.02.088
Esler, M., and Esler, D. (2020). Can angiotensin receptor-blocking drugs perhaps be harmful in the COVID-19 pandemic? J. Hypertens. 38, 781–782. doi: 10.1097/HJH.0000000000002450
Fang, L., Karakiulakis, G., and Roth, M. (2020). Are patients with hypertension and diabetes mellitus at increased risk for COVID-19 infection? Lancet Respir. Medicine 8, e21. doi: 10.1016/S2213-2600(20)30116-8
Fang, Y., Gao, F., and Liu, Z. (2019). Angiotensin-converting enzyme 2 attenuates inflammatory response and oxidative stress in hyperoxic lung injury by regulating NF-κB and Nrf2 pathways. QJM Int. J. Med. 112, 914–924. doi: 10.1093/qjmed/hcz206
Felice, C., Nardin, C., Di Tanna, G. L., Grossi, U., Bernardi, E., Scaldaferri, L., et al. (2020). Use of RAAS inhibitors and risk of clinical deterioration in COVID-19: results from an Italian cohort of 133 hypertensives. Am. J. Hypertens. 33, 944–948. doi: 10.1093/ajh/hpaa096
Ferrario, C. M., Jessup, J., Chappell, M. C., Averill, D. B., Brosnihan, K. B., Tallant, E. A., et al. (2005a). Effect of angiotensin-converting enzyme inhibition and angiotensin II receptor blockers on cardiac angiotensin-converting enzyme 2. Circulation 111, 2605–2610. doi: 10.1161/CIRCULATIONAHA.104.510461
Ferrario, C. M., Jessup, J., Gallagher, P. E., Averill, D. B., Brosnihan, K. B., Ann Tallant, E., et al. (2005b). Effects of renin-angiotensin system blockade on renal angiotensin-(1-7) forming enzymes and receptors. Kid. Int. 68, 2189–2196. doi: 10.1111/j.1523-1755.2005.00675.x
Flacco, M. E., Acuti Martellucci, C., Bravi, F., Parruti, G., Cappadona, R., Mascitelli, A., et al. (2020). Treatment with ACE inhibitors or ARBs and risk of severe/lethal COVID-19: a meta-analysis. Heart 106, 1519–1524. doi: 10.1136/heartjnl-2020-317336
Forouzanfar, M. H., Liu, P., Roth, G. A., Ng, M., Biryukov, S., Marczak, L., et al. (2017). Global burden of hypertension and systolic blood pressure of at least 110 to 115 mm Hg, 1990-2015. JAMA 317:165. doi: 10.1001/jama.2016.19043
Fosbøl, E. L., Butt, J. H., Østergaard, L., Andersson, C., Selmer, C., Kragholm, K., et al. (2020). Association of angiotensin-converting enzyme inhibitor or angiotensin receptor blocker use with COVID-19 diagnosis and mortality. JAMA 324:168. doi: 10.1001/jama.2020.11301
Fried, M. W., Crawford, J. M., Mospan, A. R., Watkins, S. E., Munoz Hernandez, B., Zink, R. C., et al. (2020). Patient characteristics and outcomes of 11,721 patients with COVID19 hospitalized across the United States. Clin. Infect. Dis. ciaa1268. doi: 10.1093/cid/ciaa1268
Furuhashi, M., Moniwa, N., Mita, T., Fuseya, T., Ishimura, S., Ohno, K., et al. (2015). Urinary angiotensin-converting enzyme 2 in hypertensive patients may be increased by olmesartan, an angiotensin II receptor blocker. Am. J. Hypertens. 28, 15–21. doi: 10.1093/ajh/hpu086
Gallagher, P. E., Chappell, M. C., Ferrario, C. M., and Tallant, E. A. (2006). Distinct roles for ANG II and ANG-(1–7) in the regulation of angiotensin-converting enzyme 2 in rat astrocytes. Am. J. Physiol. Cell Physiol. 290, C420–C426. doi: 10.1152/ajpcell.00409.2004
Gallagher, P. E., Ferrario, C. M., and Tallant, E. A. (2008a). Regulation of ACE2 in cardiac myocytes and fibroblasts. Am. J. Physiol. Heart Circ. Physiol. 295, H2373–H2379. doi: 10.1152/ajpheart.00426.2008
Gallagher, P. E., Ferrario, C. M., and Tallant, E. A. (2008b). MAP kinase/phosphatase pathway mediates the regulation of ACE2 by angiotensin peptides. Am. J. Physiol. Cell Physiol. 295, C1169–C1174. doi: 10.1152/ajpcell.00145.2008
Gao, C., Cai, Y., Zhang, K., Zhou, L., Zhang, Y., Zhang, X., et al. (2020). Association of hypertension and antihypertensive treatment with COVID-19 mortality: a retrospective observational study. Eur. Heart J. 41, 2058–2066. doi: 10.1093/eurheartj/ehaa433
Giorgi Rossi, P., Marino, M., Formisano, D., Venturelli, F., Vicentini, M., and Grilli, R. (2020). Characteristics and outcomes of a cohort of COVID-19 patients in the Province of Reggio Emilia, Italy. PLoS One 15:e0238281. doi: 10.1371/journal.pone.0238281
Greco, A., Buccheri, S., D’Arrigo, P., Calderone, D., Agnello, F., Monte, M., et al. (2020). Outcomes of renin–angiotensin–aldosterone system blockers in patients with COVID-19: a systematic review and meta-analysis. Eur. Heart J. Cardiovasc. Pharmacother. 6, 335–337. doi: 10.1093/ehjcvp/pvaa074
Grover, A., and Oberoi, M. (2020). A systematic review and meta-analysis to evaluate the clinical outcomes in COVID-19 patients on angiotensin-converting enzyme inhibitors or angiotensin receptor blockers. Eur. Heart J. Cardiovasc. Pharmacother. [Epub ahead of print]. doi: 10.1093/ehjcvp/pvaa064
Gu, H., Xie, Z., Li, T., Zhang, S., Lai, C., Zhu, P., et al. (2016). Angiotensin-converting enzyme 2 inhibits lung injury induced by respiratory syncytial virus. Sci. Rep. 6:19840. doi: 10.1038/srep19840
Gu, J., and Korteweg, C. (2007). Pathology and pathogenesis of severe acute respiratory syndrome. Am. J. Pathol. 170, 1136–1147. doi: 10.2353/ajpath.2007.061088
Guo, X., Zhu, Y., and Hong, Y. (2020). Decreased mortality of COVID-19 with renin-angiotensin-aldosterone system inhibitors therapy in patients with hypertension: a meta-analysis. Hypertension 76, e13–e14. doi: 10.1161/HYPERTENSIONAHA.120.15572
Gupta, S., Hayek, S. S., Wang, W., Chan, L., Mathews, K. S., Melamed, M. L., et al. (2020). Factors associated with death in critically ill patients with coronavirus disease 2019 in the US. JAMA Intern. Med. 180, 1–12. doi: 10.1001/jamainternmed.2020.3596
Halliday, B. P., Wassall, R., Lota, A. S., Khalique, Z., Gregson, J., Newsome, S., et al. (2019). Withdrawal of pharmacological treatment for heart failure in patients with recovered dilated cardiomyopathy (TRED-HF): an open-label, pilot, randomised trial. Lancet 393, 61–73. doi: 10.1016/S0140-6736(18)32484-X
Hamming, I., Timens, W., Bulthuis, M., Lely, A., Navis, G., and van Goor, H. (2004). Tissue distribution of ACE2 protein, the functional receptor for SARS coronavirus. A first step in understanding SARS pathogenesis. J. Pathol. 203, 631–637. doi: 10.1002/path.1570
Hamming, I., Van Goor, H., Turner, A. J., Rushworth, C. A., Michaud, A. A., Corvol, P., et al. (2008). Differential regulation of renal angiotensin-converting enzyme (ACE) and ACE2 during ACE inhibition and dietary sodium restriction in healthy rats: renal ACE and ACE2 during ACE inhibition and low salt. Exp. Physiol. 93, 631–638. doi: 10.1113/expphysiol.2007.041855
Han, S.-X., He, G.-M., Wang, T., Chen, L., Ning, Y.-Y., Luo, F., et al. (2010). Losartan attenuates chronic cigarette smoke exposure-induced pulmonary arterial hypertension in rats: possible involvement of angiotensin-converting enzyme-2. Toxicol. Appl. Pharmacol. 245, 100–107. doi: 10.1016/j.taap.2010.02.009
Hanff, T. C., Harhay, M. O., Brown, T. S., Cohen, J. B., and Mohareb, A. M. (2020). Is there an association between COVID-19 mortality and the renin-angiotensin system? A call for epidemiologic investigations. Clin. Infect. Dis. 71, 870–874. doi: 10.1093/cid/ciaa329
Harmer, D., Gilbert, M., Borman, R., and Clark, K. L. (2002). Quantitative mRNA expression profiling of ACE 2, a novel homologue of angiotensin converting enzyme. FEBS Lett. 532, 107–110. doi: 10.1016/S0014-5793(02)03640-2
Hernández-Galdamez, D. R., González-Block, M. Á, Romo-Dueñas, D. K., Lima-Morales, R., Hernández-Vicente, I. A., Lumbreras-Guzmán, M., et al. (2020). Increased risk of hospitalization and death in patients with COVID-19 and pre-existing noncommunicable diseases and modifiable risk factors in Mexico. Arch. Med. Res. 51, 683–689. doi: 10.1016/j.arcmed.2020.07.003
Hoffmann, M., Kleine-Weber, H., Schroeder, S., Krüger, N., Herrler, T., Erichsen, S., et al. (2020). SARS-CoV-2 cell entry depends on ACE2 and TMPRSS2 and is blocked by a clinically proven protease inhibitor. Cell 181, 271.e8–280.e8. doi: 10.1016/j.cell.2020.02.052
Holt, A., Mizrak, I., Lamberts, M., and Lav Madsen, P. (2020). Influence of inhibitors of the renin–angiotensin system on risk of acute respiratory distress syndrome in Danish hospitalized COVID-19 patients. J. Hypertens. [Epub ahead of print]. doi: 10.1097/HJH.0000000000002515
Huang, F., Guo, J., Zou, Z., Liu, J., Cao, B., Zhang, S., et al. (2014). Angiotensin II plasma levels are linked to disease severity and predict fatal outcomes in H7N9-infected patients. Nat. Commun. 5:3595. doi: 10.1038/ncomms4595
Iaccarino, G., Grassi, G., Borghi, C., Ferri, C., Salvetti, M., Volpe, M., et al. (2020). Age and multimorbidity predict death among COVID-19 patients: results of the SARS-RAS study of the Italian society of hypertension. Hypertension 76, 366–372. doi: 10.1161/HYPERTENSIONAHA.120.15324
Igase, M., Strawn, W. B., Gallagher, P. E., Geary, R. L., and Ferrario, C. M. (2005). Angiotensin II AT 1 receptors regulate ACE2 and angiotensin-(1–7) expression in the aorta of spontaneously hypertensive rats. Am. J. Physiol. Heart Circ. Physiol. 289, H1013–H1019. doi: 10.1152/ajpheart.00068.2005
Imai, Y., Kuba, K., Rao, S., Huan, Y., Guo, F., Guan, B., et al. (2005). Angiotensin-converting enzyme 2 protects from severe acute lung failure. Nature 436, 112–116. doi: 10.1038/nature03712
Ioannou, G. N., Locke, E., Green, P., Berry, K., O’Hare, A. M., Shah, J. A., et al. (2020). Risk factors for hospitalization, mechanical ventilation, or death among 10 131 US veterans with SARS-CoV-2 infection. JAMA Netw. Open 3:e2022310. doi: 10.1001/jamanetworkopen.2020.22310
Ishiyama, Y., Gallagher, P. E., Averill, D. B., Tallant, E. A., Brosnihan, K. B., and Ferrario, C. M. (2004). Upregulation of angiotensin-converting enzyme 2 after myocardial infarction by blockade of Angiotensin II receptors. Hypertension 43, 970–976. doi: 10.1161/01.HYP.0000124667.34652.1a
Itoyama, S., Keicho, N., Quy, T., Phi, N. C., Long, H. T., Ha, L. D., et al. (2004). ACE1 polymorphism and progression of SARS. Biochem. Biophys. Res. Commun. 323, 1124–1129. doi: 10.1016/j.bbrc.2004.08.208
Jessup, J. A., Gallagher, P. E., Averill, D. B., Brosnihan, K. B., Tallant, E. A., Chappell, M. C., et al. (2006). Effect of angiotensin II blockade on a new congenic model of hypertension derived from transgenic Ren-2 rats. Am. J. Physiol. Heart Circ. Physiol. 291, H2166–H2172. doi: 10.1152/ajpheart.00061.2006
Jiang, X., Eales, J. M., Scannali, D., Nazgiewicz, A., Prestes, P., Maier, M., et al. (2020). Hypertension and renin-angiotensin system blockers are not associated with expression of angiotensin converting enzyme 2 (ACE2) in the kidney. Cardiovasc. Med. 41, 4580–4588. doi: 10.1101/2020.05.19.20106781
Jung, S.-Y., Choi, J. C., You, S.-H., and Kim, W.-Y. (2020). Association of renin-angiotensin-aldosterone system inhibitors with Coronavirus Disease 2019 (COVID-19)- related outcomes in Korea: a nationwide population-based cohort study. Clin. Infect. Dis. 71, 2121–2128. doi: 10.1093/cid/ciaa624
Karram, T., Abbasi, A., Keidar, S., Golomb, E., Hochberg, I., Winaver, J., et al. (2005). Effects of spironolactone and eprosartan on cardiac remodeling and angiotensin-converting enzyme isoforms in rats with experimental heart failure. Am. J. Physiol. Heart Circ. Physiol. 289, H1351–H1358. doi: 10.1152/ajpheart.01186.2004
Keidar, S., Gamliel-Lazarovich, A., Kaplan, M., Pavlotzky, E., Hamoud, S., Hayek, T., et al. (2005). Mineralocorticoid receptor blocker increases angiotensin-converting enzyme 2 activity in congestive heart failure patients. Circ. Res. 97, 946–953. doi: 10.1161/01.RES.0000187500.24964.7A
Kim, L., Garg, S., O’Halloran, A., Whitaker, M., Pham, H., Anderson, E. J., et al. (2020). Risk factors for intensive care unit admission and in-hospital mortality among hospitalized adults identified through the U.S. Coronavirus Disease 2019 (COVID-19)-associated hospitalization surveillance network (COVID-NET). Clin. Infect. Dis. [Epub ahead of print]. doi: 10.1093/cid/ciaa1012
Koka V., Huang, X. R., Chung, A. C., Wang, W., Truong, L. D., and Lan, H. Y. (2008). Angiotensin II up-regulates angiotensin I-converting enzyme (ACE), but down-regulates ACE2 via the AT1-ERK/p38 MAP kinase pathway. Am. J. Pathol. 172, 1174–1183. doi: 10.2353/ajpath.2008.070762
Kreutz, R., Algharably, E. A. E.-H., Azizi, M., Dobrowolski, P., Guzik, T., Januszewicz, A., et al. (2020). Hypertension, the renin–angiotensin system, and the risk of lower respiratory tract infections and lung injury: implications for COVID-19. Cardiovasc. Res. 116, 1688–1699. doi: 10.1093/cvr/cvaa097
Kuba, K., Imai, Y., and Penninger, J. (2006). Angiotensin-converting enzyme 2 in lung diseases. Curr. Opin. Pharmacol. 6, 271–276. doi: 10.1016/j.coph.2006.03.001
Kuba, K., Imai, Y., Rao, S., Gao, H., Guo, F., Guan, B., et al. (2005). A crucial role of angiotensin converting enzyme 2 (ACE2) in SARS coronavirus–induced lung injury. Nat. Med. 11, 875–879. doi: 10.1038/nm1267
Lahens, A., Mullaert, J., Gressens, S., Gault, N., Flamant, M., Deconinck, L., et al. (2020). Association between renin–angiotensin–aldosterone system blockers and outcome in coronavirus disease 2019: analysing in-hospital exposure generates a biased seemingly protective effect of treatment. J. Hypertens. [Epub ahead of print]. doi: 10.1097/HJH.0000000000002658
Lam, C. W. K., Chan, M. H. M., and Wong, C. K. (2004). Severe acute respiratory syndrome: clinical and laboratory manifestations. Clin. Biochem. Rev. 25, 121–132.
Larouche-Lebel, É, Loughran, K. A., Oyama, M. A., Solter, P. F., Laughlin, D. S., Sánchez, M. D., et al. (2019). Plasma and tissue angiotensin-converting enzyme 2 activity and plasma equilibrium concentrations of angiotensin peptides in dogs with heart disease. J. Vet. Intern. Med. 33, 1571–1584. doi: 10.1111/jvim.15548
Lee, I. T., Nakayama, T., Wu, C.-T., Goltsev, Y., Jiang, S., Gall, P. A., et al. (2020). ACE2 localizes to the respiratory cilia and is not increased by ACE inhibitors or ARBs. Nat. Commun. 11:5453. doi: 10.1038/s41467-020-19145-6
Lely, A., Hamming, I., van Goor, H., and Navis, G. (2004). Renal ACE2 expression in human kidney disease. J. Pathol. 204, 587–593. doi: 10.1002/path.1670
Lew, R. A., Warner, F. J., Hanchapola, I., Yarski, M. A., Manohar, J., Burrell, L. M., et al. (2008). Angiotensin-converting enzyme 2 catalytic activity in human plasma is masked by an endogenous inhibitor: endogenous inhibitor masks plasma ACE2 activity. Exp. Physiol. 93, 685–693. doi: 10.1113/expphysiol.2007.040352
Lew, T. W. K. (2003). Acute respiratory distress syndrome in critically ill patients with severe acute respiratory syndrome. JAMA 290:374. doi: 10.1001/jama.290.3.374
Li, W., Moore, M. J., Vasilieva, N., Sui, J., Wong, S. K., Berne, M. A., et al. (2003). Angiotensin-converting enzyme 2 is a functional receptor for the SARS coronavirus. Nature 426, 450–454. doi: 10.1038/nature02145
Li, Y., Zeng, Z., Cao, Y., Liu, Y., Ping, F., Liang, M., et al. (2016). Angiotensin-converting enzyme 2 prevents lipopolysaccharide-induced rat acute lung injury via suppressing the ERK1/2 and NF-κB signaling pathways. Sci. Rep. 6:27911. doi: 10.1038/srep27911
Li, Y., Zeng, Z., Li, Y., Huang, W., Zhou, M., Zhang, X., et al. (2015). Angiotensin-converting enzyme inhibition attenuates lipopolysaccharide-induced lung injury by regulating the balance between angiotensin-converting enzyme and angiotensin-converting enzyme 2 and inhibiting mitogen-activated protein kinase activation. Shock 43, 395–404. doi: 10.1097/SHK.0000000000000302
Liabeuf, S., Moragny, J., Bennis, Y., Batteux, B., Brochot, E., Schmit, J. L., et al. (2020). Association between renin-angiotensin system inhibitors and COVID-19 complications. Eur. Heart J. Cardiovasc. Pharmacother. [Epub ahead of print]. doi: 10.1093/ehjcvp/pvaa062
Liang, Y., Deng, H., Bi, S., Cui, Z. A. L., Zheng, D., et al. (2015). Urinary angiotensin converting enzyme 2 increases in patients with Type 2 Diabetic Mellitus. Kid. Blood Press Res. 40, 101–110. doi: 10.1159/000368486
Lighter, J., Phillips, M., Hochman, S., Sterling, S., Johnson, D., Francois, F., et al. (2020). Obesity in patients younger than 60 years is a risk factor for COVID-19 Hospital admission. Clin. Infect. Dis. 71, 896–897. doi: 10.1093/cid/ciaa415
Liu, Y., Yang, Y., Zhang, C., Huang, F., Wang, F., Yuan, J., et al. (2020). Clinical and biochemical indexes from 2019-nCoV infected patients linked to viral loads and lung injury. Sci. China Life Sci. 63, 364–374. doi: 10.1007/s11427-020-1643-8
Liu, Y.-H., Liu, L.-Y., Wu, J.-X., Chen, S.-X., and Sun, Y.-X. (2006). Comparison of captopril and enalapril to study the role of the sulfhydryl-group in improvement of endothelial dysfunction with ACE inhibitors in high dieted methionine mice. J. Cardiovasc. Pharmacol. 47, 82–88. doi: 10.1097/01.fjc.0000195306.61719.64
Lopes, R. D., Macedo, A. V. S., de Barros e Silva, P. G. M., Moll-Bernardes, R. J., Feldman, A., D’Andréa Saba Arruda, G., et al. (2020). Continuing versus suspending angiotensin-converting enzyme inhibitors and angiotensin receptor blockers: impact on adverse outcomes in hospitalized patients with severe acute respiratory syndrome coronavirus 2 (SARS-CoV-2)–The BRACE CORONA Trial. Am. Heart J. 226, 49–59. doi: 10.1016/j.ahj.2020.05.002
Lopes, R. D., Macedo, A. V. S., de Barros E Silva, P. G. M., Moll-Bernardes, R. J., Dos Santos, T. M., Mazza, L., et al. (2021). Effect of Discontinuing vs Continuing Angiotensin-Converting Enzyme Inhibitors and Angiotensin II Receptor Blockers on Days Alive and Out of the Hospital in Patients Admitted With COVID-19: A Randomized Clinical Trial. JAMA. 19, 254–264. doi: 10.1001/jama.2020.25864
Lu, R., Zhao, X., Li, J., Niu, P., Yang, B., Wu, H., et al. (2020). Genomic characterisation and epidemiology of 2019 novel coronavirus: implications for virus origins and receptor binding. Lancet 395, 565–574. doi: 10.1016/S0140-6736(20)30251-8
Mackey, K., King, V. J., Gurley, S., Kiefer, M., Liederbauer, E., Vela, K., et al. (2020). Risks and impact of angiotensin-converting enzyme inhibitors or angiotensin-receptor blockers on SARS-CoV-2 infection in adults: a living systematic review. Ann. Internal Med. 173, 195–203. doi: 10.7326/M20-1515
Mancia, G., Rea, F., Ludergnani, M., Apolone, G., and Corrao, G. (2020). Renin–angiotensin–aldosterone system blockers and the risk of covid-19. N. Engl. J. Med. 382, 2431–2440. doi: 10.1056/NEJMoa2006923
Mariana, C. P., Ramona, P. A., Ioana, B. C., Diana, M., Claudia, R. C., Stefan, V. D., et al. (2016). Urinary angiotensin converting enzyme 2 is strongly related to urinary nephrin in type 2 diabetes patients. Int. Urol. Nephrol. 48, 1491–1497. doi: 10.1007/s11255-016-1334-8
Marshall, R. P., Webb, S., Bellingan, G. J., Montgomery, H. E., Chaudhari, B., McAnulty, R. J., et al. (2002). Angiotensin converting enzyme insertion/deletion polymorphism is associated with susceptibility and outcome in acute respiratory distress syndrome. Am. J. Respir. Crit. Care Med. 166, 646–650. doi: 10.1164/rccm.2108086
Mechanick, J. I., Rosenson, R. S., Pinney, S. P., Mancini, D. M., Narula, J., and Fuster, V. (2020). Coronavirus and cardiometabolic syndrome. J. Am. Coll. Cardiol. 76, 2024–2035. doi: 10.1016/j.jacc.2020.07.069
Mehta, N., Kalra, A., Nowacki, A. S., Anjewierden, S., Han, Z., Bhat, P., et al. (2020). Association of use of angiotensin-converting enzyme inhibitors and Angiotensin II receptor blockers with testing positive for coronavirus disease 2019 (COVID-19). JAMA Cardiol. 5:1020. doi: 10.1001/jamacardio.2020.1855
Meng, J., Xiao, G., Zhang, J., He, X., Ou, M., Bi, J., et al. (2020). Renin-angiotensin system inhibitors improve the clinical outcomes of COVID-19 patients with hypertension. Emerg. Microbes Infect. 9, 757–760. doi: 10.1080/22221751.2020.1746200
Mikami, T., Miyashita, H., Yamada, T., Harrington, M., Steinberg, D., Dunn, A., et al. (2020). Risk factors for mortality in patients with COVID-19 in New York City. J. Gen. Intern. Med. [Epub ahead of print]. doi: 10.1007/s11606-020-05983-z
Monteil, V., Kwon, H., Prado, P., Hagelkrüys, A., Wimmer, R. A., Stahl, M., et al. (2020). Inhibition of SARS-CoV-2 infections in engineered human tissues using clinical-grade soluble human ACE2. Cell 181, 905.e7–913.e7. doi: 10.1016/j.cell.2020.04.004
Narula, S., Yusuf, S., Chong, M., Ramasundarahettige, C., Rangarajan, S., Bangdiwala, S. I., et al. (2020). Plasma ACE2 and risk of death or cardiometabolic diseases: a case-cohort analysis. Lancet 396, 968–976. doi: 10.1016/S0140-6736(20)31964-4
Ocaranza, M. P., Godoy, I., Jalil, J. E., Varas, M., Collantes, P., Pinto, M., et al. (2006). Enalapril attenuates downregulation of angiotensin-converting enzyme 2 in the late phase of ventricular dysfunction in myocardial infarcted rat. Hypertension 48, 572–578. doi: 10.1161/01.HYP.0000237862.94083.45
Ortiz-Pérez, J. T., Riera, M., Bosch, X., De Caralt, T. M., Perea, R. J., Pascual, J., et al. (2013). Role of circulating angiotensin converting enzyme 2 in left ventricular remodeling following myocardial infarction: a prospective controlled study. PLoS One 8:e61695. doi: 10.1371/journal.pone.0061695
Pan, W., Zhang, J., Wang, M., Ye, J., Xu, Y., Shen, B., et al. (2020). Clinical features of COVID-19 in patients with essential hypertension and the impacts of renin-angiotensin-aldosterone system inhibitors on the prognosis of COVID-19 patients. Hypertension 76, 732–741. doi: 10.1161/HYPERTENSIONAHA.120.15289
Parra-Bracamonte, G. M., Lopez-Villalobos, N., and Parra-Bracamonte, F. E. (2020). Clinical characteristics and risk factors for mortality of patients with COVID-19 in a large data set from Mexico. Ann. Epidemiol. 52, 93.e2–98.e2. doi: 10.1016/j.annepidem.2020.08.005
Perez-Guzman, P. N., Daunt, A., Mukherjee, S., Crook, P., Forlano, R., Kont, M. D., et al. (2020). Clinical characteristics and predictors of outcomes of hospitalized patients with COVID-19 in a multi-ethnic London NHS Trust: a retrospective cohort study. Clin. Infect. Dis. [Epub ahead of print]. doi: 10.1093/cid/ciaa1091
Petrilli, C. M., Jones, S. A., Yang, J., Rajagopalan, H., O’Donnell, L., Chernyak, Y., et al. (2020). Factors associated with hospital admission and critical illness among 5279 people with coronavirus disease 2019 in New York City: prospective cohort study. BMJ 369:m1966. doi: 10.1136/bmj.m1966
Pons, S., Fodil, S., Azoulay, E., and Zafrani, L. (2020). The vascular endothelium: the cornerstone of organ dysfunction in severe SARS-CoV-2 infection. Crit. Care 24:353. doi: 10.1186/s13054-020-03062-7
Pranata, R., Permana, H., Huang, I., Lim, M. A., Soetedjo, N. N. M., Supriyadi, R., et al. (2020). The use of renin angiotensin system inhibitor on mortality in patients with coronavirus disease 2019 (COVID-19): a systematic review and meta-analysis. Diabetes Metab. Syndrome Clin. Res. Rev. 14, 983–990. doi: 10.1016/j.dsx.2020.06.047
Ramchand, J., and Burrell, L. M. (2020). Circulating ACE2: a novel biomarker of cardiovascular risk. Lancet 396, 937–939. doi: 10.1016/S0140-6736(20)32011-0
Ramchand, J., Patel, S. K., Kearney, L. G., Matalanis, G., Farouque, O., Srivastava, P. M., et al. (2020). Plasma ACE2 activity predicts mortality in aortic stenosis and is associated with severe myocardial fibrosis. JACC Cardiovasc. Imag. 13, 655–664. doi: 10.1016/j.jcmg.2019.09.005
Ramchand, J., Patel, S. K., Srivastava, P. M., Farouque, O., and Burrell, L. M. (2018). Elevated plasma angiotensin converting enzyme 2 activity is an independent predictor of major adverse cardiac events in patients with obstructive coronary artery disease. PLoS One 13:e0198144. doi: 10.1371/journal.pone.0198144
Reich, H. N., Oudit, G. Y., Penninger, J. M., Scholey, J. W., and Herzenberg, A. M. (2008). Decreased glomerular and tubular expression of ACE2 in patients with type 2 diabetes and kidney disease. Kid. Int. 74, 1610–1616. doi: 10.1038/ki.2008.497
Reilev, M., Kristensen, K. B., Pottegård, A., Lund, L. C., Hallas, J., Ernst, M. T., et al. (2020). Characteristics and predictors of hospitalization and death in the first 11 122 cases with a positive RT-PCR test for SARS-CoV-2 in Denmark: a nationwide cohort. Int. J. Epidemiol. 49, 1468–1481. doi: 10.1093/ije/dyaa140
Reynolds, H. R., Adhikari, S., Pulgarin, C., Troxel, A. B., Iturrate, E., Johnson, S. B., et al. (2020). Renin–angiotensin–aldosterone system inhibitors and risk of Covid-19. N. Engl. J. Med. 382, 2441–2448. doi: 10.1056/NEJMoa2008975
Rice, G. I., Jones, A. L., Grant, P. J., Carter, A. M., Turner, A. J., and Hooper, N. M. (2006). Circulating activities of angiotensin-converting enzyme, its homolog, angiotensin-converting enzyme 2, and neprilysin in a family study. Hypertension 48, 914–920. doi: 10.1161/01.HYP.0000244543.91937.79
Richardson, S., Hirsch, J. S., Narasimhan, M., Crawford, J. M., McGinn, T., Davidson, K. W., et al. (2020). Presenting characteristics, comorbidities, and outcomes among 5700 patients hospitalized with COVID-19 in the New York City Area. JAMA 323:2052. doi: 10.1001/jama.2020.6775
Sama, I. E., Ravera, A., Santema, B. T., van Goor, H., ter Maaten, J. M., Cleland, J. G. F., et al. (2020). Circulating plasma concentrations of angiotensin-converting enzyme 2 in men and women with heart failure and effects of renin–angiotensin–aldosterone inhibitors. Eur. Heart J. 41, 1810–1817. doi: 10.1093/eurheartj/ehaa373
Sands, K. E., Wenzel, R. P., McLean, L. E., Korwek, K. M., Roach, J. D., Miller, K. M., et al. (2020). Patient characteristics and admitting vital signs associated with coronavirus disease 2019 (COVID-19)-related mortality among patients admitted with noncritical illness. Infect. Control Hosp. Epidemiol. 1–7. doi: 10.1017/ice.2020.461
Santos, R. A. S., Sampaio, W. O., Alzamora, A. C., Motta-Santos, D., Alenina, N., Bader, M., et al. (2018). The ACE2/Angiotensin-(1–7)/MAS axis of the renin-angiotensin system: focus on angiotensin-(1–7). Physiol. Rev. 98, 505–553. doi: 10.1152/physrev.00023.2016
Savarese, G., Benson, L., Sundström, J., and Lund, L. H. (2020). Association between renin–angiotensin–aldosterone system inhibitor use and COVID-19 Hospitalization and death: a 1,4 million patient Nation-Wide registry analysis. Eur. J. Heart Fail. [Epub ahead of print]. doi: 10.1002/ejhf.2060
Shah, P., Owens, J., Franklin, J., Jani, Y., Kumar, A., and Doshi, R. (2020). Baseline use of angiotensin-converting enzyme inhibitor/AT1 blocker and outcomes in hospitalized coronavirus disease 2019 African-American patients. J. Hypertens. [Epub ahead of print]. doi: 10.1097/HJH.0000000000002584
Simonnet, A., Chetboun, M., Poissy, J., Raverdy, V., Noulette, J., Duhamel, A., et al. (2020). High prevalence of obesity in severe acute respiratory syndrome Coronavirus-2 (SARS-CoV-2) requiring invasive mechanical ventilation. Obesity 28, 1195–1199. doi: 10.1002/oby.22831
Soler, M. J., Ye, M., Wysocki, J., William, J., Lloveras, J., and Batlle, D. (2009). Localization of ACE2 in the renal vasculature: amplification by angiotensin II type 1 receptor blockade using telmisartan. Am. J. Physiol. Renal Physiol. 296, F398–F405. doi: 10.1152/ajprenal.90488.2008
Son, M., Seo, J., and Yang, S. (2020). Association between renin-angiotensin-aldosterone system inhibitors and COVID-19 infection in South Korea. Hypertension 76, 742–749. doi: 10.1161/HYPERTENSIONAHA.120.15464
Soro-Paavonen, A., Gordin, D., Forsblom, C., Rosengard-Barlund, M., Waden, J., Thorn, L., et al. (2012). Circulating ACE2 activity is increased in patients with type 1 diabetes and vascular complications. J. Hypertens. 30, 375–383. doi: 10.1097/HJH.0b013e32834f04b6
South, A. M., Tomlinson, L., Edmonston, D., Hiremath, S., and Sparks, M. A. (2020). Controversies of renin–angiotensin system inhibition during the COVID-19 pandemic. Nat. Rev. Nephrol. 16, 305–307. doi: 10.1038/s41581-020-0279-4
Sparks, M. A., South, A. M., Badley, A. D., Baker-Smith, C. M., Batlle, D., Bozkurt, B., et al. (2020). Severe acute respiratory syndrome Coronavirus 2, COVID-19, and the renin-angiotensin system: pressing needs and best research practices. Hypertension 76, 1350–1367. doi: 10.1161/HYPERTENSIONAHA.120.15948
Sriram, K., and Insel, P. A. (2020). Risks of ACE inhibitor and ARB usage in COVID-19: evaluating the evidence. Clin. Pharmacol. Ther. 108, 236–241. doi: 10.1002/cpt.1863
Stegbauer, J., Kraus, M., Nordmeyer, S., Kirchner, M., Ziehm, M., Dommisch, H., et al. (2020). Proteomic analysis reveals upregulation of ACE2 (Angiotensin-Converting Enzyme 2), the putative SARS-CoV-2 receptor in pressure–but not volume-overloaded human hearts. Hypertension 76, e41–e43. doi: 10.1161/HYPERTENSIONAHA.120.16261
Suissa, S. (2008). Immortal time bias in pharmacoepidemiology. Am. J. Epidemiol. 167, 492–499. doi: 10.1093/aje/kwm324
Sukumaran, V., Veeraveedu, P. T., Gurusamy, N., Yamaguchi, K., Lakshmanan, A. P., Ma, M., et al. (2011). Cardioprotective effects of telmisartan against heart failure in rats induced by experimental autoimmune myocarditis through the modulation of angiotensin-converting Enzyme-2/Angiotensin 1-7/ Mas receptor axis. Int. J. Biol. Sci. 7, 1077–1092. doi: 10.7150/ijbs.7.1077
Sukumaran, V., Veeraveedu, P. T., Lakshmanan, A. P., Gurusamy, N., Yamaguchi, K., Ma, M., et al. (2012). Olmesartan medoxomil treatment potently improves cardiac myosin-induced dilated cardiomyopathy via the modulation of ACE-2 and ANG 1–7 mas receptor. Free Radic. Res. 46, 850–860. doi: 10.3109/10715762.2012.684878
Takeda, Y., Zhu, A., Yoneda, T., Usukura, M., Takata, H., and Yamagishi, M. (2007). Effects of aldosterone and Angiotensin II receptor blockade on cardiac angiotensinogen and angiotensin-converting enzyme 2 expression in dahl salt-sensitive hypertensive rats. Am. J. Hypertens. 20, 1119–1124. doi: 10.1016/j.amjhyper.2007.05.008
Tedeschi, S., Giannella, M., Bartoletti, M., Trapani, F., Tadolini, M., Borghi, C., et al. (2020). Clinical impact of renin-angiotensin system inhibitors on in-hospital mortality of patients with hypertension hospitalized for Coronavirus Disease 2019. Clin. Infect. Dis. 71, 899–901. doi: 10.1093/cid/ciaa492
Tipnis, S. R., Hooper, N. M., Hyde, R., Karran, E., Christie, G., and Turner, A. J. (2000). A Human homolog of angiotensin-converting enzyme: cloning and functional expression as a captopril-insensitive carboxypeptidase. J. Biol. Chem. 275, 33238–33243. doi: 10.1074/jbc.M002615200
Trifirò, G., Massari, M., Da Cas, R., Menniti Ippolito, F., Sultana, J., Crisafulli, S., et al. (2020). Renin–angiotensin–aldosterone system inhibitors and risk of death in patients hospitalised with COVID-19: a retrospective italian cohort study of 43,000 patients. Drug Saf. 43, 1297–1308. doi: 10.1007/s40264-020-00994-5
Uri, K., Fagyas, M., Kertesz, A., Borbely, A., Jenei, C., Bene, O., et al. (2016). Circulating ACE2 activity correlates with cardiovascular disease development. J. Renin Angiotensin Aldosterone Syst. 17:1470320316668435. doi: 10.1177/1470320316668435
Vaduganathan, M., Vardeny, O., Michel, T., McMurray, J. J. V., Pfeffer, M. A., and Solomon, S. D. (2020). Renin–angiotensin–aldosterone system inhibitors in patients with Covid-19. N. Engl. J. Med. 382, 1653–1659. doi: 10.1056/NEJMsr2005760
van Gerwen, M., Alsen, M., Little, C., Barlow, J., Genden, E., Naymagon, L., et al. (2020). Risk factors and outcomes of COVID-19 in New York City; a retrospective cohort study. J. Med. Virol. 93, 907–915. doi: 10.1002/jmv.26337
Velkoska, E., Dean, R. G., Burchill, L., Levidiotis, V., and Burrell, L. M. (2010). Reduction in renal ACE2 expression in subtotal nephrectomy in rats is ameliorated with ACE inhibition. Clin. Sci. 118, 269–279. doi: 10.1042/CS20090318
Vuille-dit-Bille, R. N., Camargo, S. M., Emmenegger, L., Sasse, T., Kummer, E., Jando, J., et al. (2015). Human intestine luminal ACE2 and amino acid transporter expression increased by ACE-inhibitors. Amino Acids 47, 693–705. doi: 10.1007/s00726-014-1889-6
Walters, T. E., Kalman, J. M., Patel, S. K., Mearns, M., Velkoska, E., and Burrell, L. M. (2016). Angiotensin converting enzyme 2 activity and human atrial fibrillation: increased plasma angiotensin converting enzyme 2 activity is associated with atrial fibrillation and more advanced left atrial structural remodelling. Europace 19, 1280–1287. doi: 10.1093/europace/euw246
Wang, D., Hu, B., Hu, C., Zhu, F., Liu, X., Zhang, J., et al. (2020). Clinical characteristics of 138 hospitalized patients with 2019 novel coronavirus–infected pneumonia in Wuhan, China. JAMA 323:1061. doi: 10.1001/jama.2020.1585
Wang, K., Gheblawi, M., and Oudit, G. Y. (2020). Angiotensin converting enzyme 2: a double-edged sword. Circulation 142, 426–428. doi: 10.1161/CIRCULATIONAHA.120.047049
Wang, G., Zhang, Q., Yuan, W., Wu, J., and Li, C. (2016). Enalapril protects against myocardial ischemia/reperfusion injury in a swine model of cardiac arrest and resuscitation. Int. J. Mol. Med. 38, 1463–1473. doi: 10.3892/ijmm.2016.2737
Wang, L., Li, Y., Qin, H., Xing, D., Su, J., and Hu, Z. (2016). Crosstalk between ACE2 and PLGF regulates vascular permeability during acute lung injury. Am. J. Transl. Res. 8, 1246–1252.
Wang, Z., Chen, Z., Zhang, L., Wang, X., Hao, G., Zhang, Z., et al. (2018). Status of hypertension in China: results from the China hypertension survey, 2012–2015. Circulation 137, 2344–2356. doi: 10.1161/CIRCULATIONAHA.117.032380
Wiener, R. S., Cao, Y. X., Hinds, A., Ramirez, M. I., and Williams, M. C. (2007). Angiotensin converting enzyme 2 is primarily epithelial and is developmentally regulated in the mouse lung. J. Cell. Biochem. 101, 1278–1291. doi: 10.1002/jcb.21248
Williamson, E. J., Walker, A. J., Bhaskaran, K., Bacon, S., Bates, C., Morton, C. E., et al. (2020). Factors associated with COVID-19-related death using OpenSAFELY. Nature 584, 430–436. doi: 10.1038/s41586-020-2521-4
Wong, M. H., Chapin, O. C., and Johnson, M. D. (2012). LPS-stimulated cytokine production in Type I cells is modulated by the renin–angiotensin system. Am. J. Respir. Cell Mol. Biol. 46, 641–650. doi: 10.1165/rcmb.2011-0289OC
Wösten-van Asperen, R. M., Lutter, R., Specht, P. A., Moll, G. N., van Woensel, J. B., van der Loos, C. M., et al. (2011). Acute respiratory distress syndrome leads to reduced ratio of ACE/ACE2 activities and is prevented by angiotensin-(1-7) or an angiotensin II receptor antagonist: ARDS leads to reduced ratio of ACE/ACE2 activities. J. Pathol. 225, 618–627. doi: 10.1002/path.2987
Wrapp, D., Wang, N., Corbett, K. S., Goldsmith, J. A., Hsieh, C.-L., Abiona, O., et al. (2020). Cryo-EM structure of the 2019-nCoV spike in the prefusion conformation. Microbiology 367, 1260–1263. doi: 10.1101/2020.02.11.944462
Wu, C., Ye, D., Mullick, A. E., Li, Z., Danser, A. H. J., Daugherty, A., et al. (2020). Effects of Renin-Angiotensin Inhibition on ACE2 (Angiotensin-Converting Enzyme 2) and TMPRSS2 (Transmembrane Protease Serine 2) Expression: Insights Into COVID-19. Hypertension 76, e29–e30. doi: 10.1161/HYPERTENSIONAHA.120.15782
Wu, Y., Guo, W., Liu, H., Qi, B., Liang, K., Xu, H., et al. (2020). Clinical outcomes of 402 patients with COVID-2019 from a single center in Wuhan, China. J. Med. Virol. 92, 2751–2757. doi: 10.1002/jmv.26168
Wysocki, J., Lores, E., Ye, M., Soler, M. J., and Batlle, D. (2020). Kidney and lung ACE2 expression after an ACE inhibitor or an Ang II receptor blocker: implications for COVID-19. JASN 31, 1941–1943. doi: 10.1681/ASN.2020050667
Wysocki, J., Ye, M., Soler, M. J., Gurley, S. B., Xiao, H. D., Bernstein, K. E., et al. (2006). ACE and ACE2 activity in diabetic mice. Diabetes 55, 2132–2139. doi: 10.2337/db06-0033
Yan, Y., Liu, Q., Li, N., Du, J., Li, X., Li, C., et al. (2015). Angiotensin II receptor blocker as a novel therapy in acute lung injury induced by avian influenza A H5N1 virus infection in mouse. Sci. China Life Sci. 58, 208–211. doi: 10.1007/s11427-015-4814-7
Yang, G., Tan, Z., Zhou, L., Yang, M., Peng, L., Liu, J., et al. (2020). Effects of Angiotensin II receptor blockers and ACE (Angiotensin-Converting Enzyme) inhibitors on virus infection, inflammatory status, and clinical outcomes in patients with COVID-19 and hypertension: a single-center retrospective study. Hypertension 76, 51–58. doi: 10.1161/HYPERTENSIONAHA.120.15143
Yang, P., Gu, H., Zhao, Z., Wang, W., Cao, B., Lai, C., et al. (2015). Angiotensin-converting enzyme 2 (ACE2) mediates influenza H7N9 virus-induced acute lung injury. Sci. Rep. 4:7027. doi: 10.1038/srep07027
Yang, Z., Yu, X., Cheng, L., Miao, L., Li, H., Han, L., et al. (2013). Effects of enalapril on the expression of cardiac angiotensin-converting enzyme and angiotensin-converting enzyme 2 in spontaneously hypertensive rats. Arch. Cardiovasc. Dis. 106, 196–201. doi: 10.1016/j.acvd.2013.01.004
Ye, R., and Liu, Z. (2020). ACE2 exhibits protective effects against LPS-induced acute lung injury in mice by inhibiting the LPS-TLR4 pathway. Exp. Mol. Pathol. 113:104350. doi: 10.1016/j.yexmp.2019.104350
Yu, C., Lei, Q., Li, W., Wang, X., Liu, W., Fan, X., et al. (2020). Clinical characteristics, associated factors, and predicting COVID-19 mortality risk: a retrospective study in Wuhan, China. Am. J. Prev. Med. 59, 168–175. doi: 10.1016/j.amepre.2020.05.002
Zhang, J., Tecson, K. M., and McCullough, P. A. (2020). Endothelial dysfunction contributes to COVID-19-associated vascular inflammation and coagulopathy. Rev. Cardiovasc. Med. 21:315. doi: 10.31083/j.rcm.2020.03.126
Zhang, P., Zhu, L., Cai, J., Lei, F., Qin, J.-J., Xie, J., et al. (2020). Association of inpatient use of angiotensin-converting enzyme inhibitors and Angiotensin II receptor blockers with mortality among patients with hypertension hospitalized with COVID-19. Circ. Res. 126, 1671–1681. doi: 10.1161/CIRCRESAHA.120.317134
Zhang, X., Yu, J., Pan, L., and Jiang, H. (2020). ACEI/ARB use and risk of infection or severity or mortality of COVID-19: a systematic review and meta-analysis. Pharmacol. Res. 158:104927. doi: 10.1016/j.phrs.2020.104927
Zhang, M., Gao, Y., Zhao, W., Yu, G., and Jin, F. (2018). ACE-2/ANG1-7 ameliorates ER stress-induced apoptosis in seawater aspiration-induced acute lung injury. Am. J. Physiol. Lung Cell. Mol. Physiol. 315, L1015–L1027. doi: 10.1152/ajplung.00163.2018
Zhang, Y., Li, B., Wang, B., Zhang, J., Wu, J., and Morgan, T. (2014). Alteration of cardiac ACE2/Mas expression and cardiac remodelling in rats with aortic constriction. Chinese J. Physiol. 57, 335–342. doi: 10.4077/CJP.2014.BAD268
Zhou, F., Liu, Y.-M., Xie, J., Li, H., Lei, F., Yang, H., et al. (2020a). Comparative impacts of ACE (Angiotensin-Converting Enzyme) inhibitors versus Angiotensin II receptor blockers on the risk of COVID-19 mortality. Hypertension 76, e15–e17. doi: 10.1161/HYPERTENSIONAHA.120.15622
Zhou, F., Yu, T., Du, R., Fan, G., Liu, Y., Liu, Z., et al. (2020b). Clinical course and risk factors for mortality of adult inpatients with COVID-19 in Wuhan, China: a retrospective cohort study. Lancet 395, 1054–1062. doi: 10.1016/S0140-6736(20)30566-3
Keywords: SARS-CoV-2, COVID-19, renin-angiotensin-aldosterone system, RAAS blockers, angiotensin converting enzyme inhibitors, angiotensin receptor blockers
Citation: Gressens SB, Leftheriotis G, Dussaule J-C, Flamant M, Levy BI and Vidal-Petiot E (2021) Controversial Roles of the Renin Angiotensin System and Its Modulators During the COVID-19 Pandemic. Front. Physiol. 12:624052. doi: 10.3389/fphys.2021.624052
Received: 30 October 2020; Accepted: 12 January 2021;
Published: 22 February 2021.
Edited by:
Amy C. Arnold, Pennsylvania State University, United StatesReviewed by:
Mark Chappell, Wake Forest School of Medicine, United StatesCopyright © 2021 Gressens, Leftheriotis, Dussaule, Flamant, Levy and Vidal-Petiot. This is an open-access article distributed under the terms of the Creative Commons Attribution License (CC BY). The use, distribution or reproduction in other forums is permitted, provided the original author(s) and the copyright owner(s) are credited and that the original publication in this journal is cited, in accordance with accepted academic practice. No use, distribution or reproduction is permitted which does not comply with these terms.
*Correspondence: Emmanuelle Vidal-Petiot, ZW1tYW51ZWxsZS52aWRhbC1wZXRpb3RAYXBocC5mcg==
Disclaimer: All claims expressed in this article are solely those of the authors and do not necessarily represent those of their affiliated organizations, or those of the publisher, the editors and the reviewers. Any product that may be evaluated in this article or claim that may be made by its manufacturer is not guaranteed or endorsed by the publisher.
Research integrity at Frontiers
Learn more about the work of our research integrity team to safeguard the quality of each article we publish.