- 1Institute for Sustainable Plant Protection-CNR, Bari, Italy
- 2Section of Entomology and Zoology, Department of Soil, Plant and Food Sciences, University of Bari “A. Moro”, Bari, Italy
Understanding how entomopathogenic nematodes respond to temperature changes and have adapted to the local environment is crucial to improve their potential as biocontrol agents. In order to improve understanding of Heterorhabditis bacteriophora’s potential adaptability to future climate changes, full-length cDNA and the corresponding gene of heat shock protein 90 (Hsp90) were isolated and fully characterized. The reproductive potential of the Apulian strain of H. bacteriophora increased when the temperature rose from 23 to 30°C, but no reproduction was found at 12°C. Expression analyses revealed that Hb-hsp90-1 was differentially expressed in Infective Juveniles (IJs) and adults (hermaphrodites, females and males). Up-regulation of Hb-hsp90-1 was higher during the recovery process in Galleria mellonella larvae than adults, thus confirming the protective role of Hb-hsp90-1 in coping with the host environment. Silencing of Hb-hsp90-1 resulted in a significant reduction (76%) in the expression level. Silenced IJs took longer than untreated nematodes to infect G. mellonella, showing that Hb-hsp90-1 could be also involved in chemosensation. Furthermore, the number of adults and IJs recovered from G. mellonella infected with silenced nematodes and incubated at 30°C was higher than that obtained from G. mellonella infected with untreated nematodes. These data confirm the crucial role of Hb-hsp90-1 allowing acclimation to increased temperatures and modulation of the recovery process.
Introduction
Entomopathogenic nematodes (EPNs) of Steinernematidae and Heterorhabditidae families are obligate parasites of a wide range of insect pests (Lacey et al., 2015); they are used as biocontrol agents, mostly for soil-dwelling insects. Like most plant parasitic nematodes, EPNs infect host insects as infective juveniles (IJs), which are developmentally arrested third-stage larvae. IJs are long-lived, non-feeding, stress-resistant, and able to locate specific insects using chemosensory cues (Chaisson and Hallem, 2012; Rasmann et al., 2012). IJs carry symbiotic bacteria in their intestines: Photorhabdus spp. are associated with heterorhabditids and Xenorhabdus spp. with steinernematids. Inside a suitable host, IJs release these symbiotic bacteria into the insect’s hemocoel, killing the host in 24–48 h and thus providing nutrients for the nematodes (Lewis and Clarke, 2012).
Their pathogenicity in the field depends on several biotic and abiotic conditions, including temperature, humidity, soil type, application timing, and genetic traits (Grewal et al., 2006). Moreover, the effect of soil temperature on infectivity, survival and host-seeking behavior of EPNs is well known to vary among species and strains (Mukuka et al., 2010b; Lee et al., 2016). However, nematodes are also able to cope with the effects of harmful temperatures by using mechanisms such as heat shock response, developmental arrest and behavioral strategy (Mukuka et al., 2010a, b). Heat shock response is a highly conserved strategy among nematodes, but closely related species and strains of EPNs can differ greatly in their tolerance to higher temperatures (Mukuka et al., 2010a; Tarasco et al., 2015). This suggests that temperature tolerance can evolve rapidly. The way a species responds to heat stress affects not only its immediate tolerance level, but can also affect its long-term potential to increase tolerance. Hashmi et al. (1997) reported a correlation between the geographical origin of Heterorhabditis spp. and polymorphism of the hsp70A gene, highlighting that heat shock response to increasing temperature can affect the tolerance of Heterorhabditis strains.
Temperature is particularly crucial to organismal fitness and to behavior. To date very little is known about the heat shock genes involved in the response of Heterorhabditis bacteriophora to temperature change.
Hsp90s are involved in protein folding, protecting the proteome from misfolding and aggregation. All organisms except Archaea (Gupta, 1995; Chen et al., 2003) possess them, and they are highly conserved. Hsp90s are essential for viability under all conditions in eukaryotes, they are expressed in response to adverse environmental or chemical stresses and pathophysiological situations, and play an important role in cell survival (Pantzartzi et al., 2013). The Hsp90 gene family has undergone major duplication events leading to isoforms with cellular compartmentalization. In all vertebrates studied to date there are two known cytoplasmic isoforms which derived from duplication events. However, additional duplication events have occurred, thus increasing the number of total gene copies observed in vertebrates (Hoter et al., 2018). A total of 13 cytoplasmic genes have been identified in humans, 9 of which are pseudogenes, whereas the number of cytoplasmic gene copies in invertebrates is not uniform. To date, a single gene encoding for Hsp90 has been reported in nematodes and in Drosophila (Konstantopoulou and Scouras, 1998; Inoue et al., 2003; De Luca et al., 2009). Recently, two gene copies for hsp90 have been found in plant parasitic nematodes, differing each other in nucleotide sequence and intron–exon structure (Fanelli et al., 2018).
The present study reports on the isolation, gene organization and functional characterization of the Hb-hsp90-1 gene in H. bacteriophora. This research also investigated the ability of H. bacteriophora to adapt to future temperature changes in long-term experiments using decreased and increased temperatures and testing how the entomopathogenic nematode responds to increased temperature during the recovery process at the molecular level. Our functional characterization of the Hb-hsp90-1 gene demonstrated its evolutionary adaptive response to soil and insect environments, including an expanded gene family for hsp90 and expression profiles within increased temperature.
Materials and Methods
EPN Culture
Apulian EPN strain H. bacteriophora (LU1), kindly provided by Prof. Tarasco (Bari, Italy) was maintained under laboratory conditions using last instar-larvae of G. mellonella (L.) as the host (Woodring and Kaya, 1988). The infected G. mellonella cadavers were placed on White traps in order to obtain suspension of IJs (White, 1927). Harvested IJs were washed with distilled water three times, and stored at 15°C before use.
DNA and RNA Extraction
Total genomic DNA and total RNA were extracted from 2,000 frozen IJ stages which were ground in liquid nitrogen using a pestle and mortar. Total genomic DNA and RNA were extracted from the resulting powder using an AllPrep DNA/RNA kit (QIAGEN) for simultaneous purification of nucleic acids according to the manufacturer’s instructions.
Isolation of Hb-hsp90-1 Gene
Amplification of genomic DNA fragments was conducted on 50 ng of H. bacteriophora DNA with degenerate primers: U831 (5′-AAYAARACMAAGCCNTYTGGAC-3′) and L1110 (5′–TCRCARTTVTCCATGATRAAVAC–3′) (Skantar and Carta, 2004). PCR was performed with Taq DNA polymerase (Promega) as follows: initial denaturation at 94°C for 2 min, followed by 45 cycles of denaturation at 94°C for 20 s, annealing at 65°C for 5 s, at 60°C for 5 s, at 55°C for 5 s, 50°C for 5 s and extension at 68°C for 1 min with a final step at 68°C for 15 min. PCR product was extracted from the gel using the protocol provided by the manufacturer (NucleoSpin Gel and PCR Clean-up, Machery Nagel, Germany) and cloned into pGem T-easy vector System II (Promega). Plasmid inserts were sequenced and analyzed. The same procedure was used to amplify the partial Hb-hsp90 gene in individual nematodes (De Luca et al., 2004).
3′/5′ RACE
The 3′/5′ RACE of Hb-hsp90-1 was conducted on 1 μg of H. bacteriophora total RNA using SMART SMARTer RACE 5′/3′ Clontech, following the manufacturer’s protocol. The 3′ end of Hb-hsp90-1 was amplified using the gene specific primer EPNhsp3UTR1 (GACGACATCTCAAACGAGGA) and a long universal primer UPM (CTAATACGACTCAC TATAGGGCAAGCAGTGGTATCAACGCAGAGT).
PCR was performed as follows: 30 cycles at 94°C for 30 s, 64°C for 30 s, and 72°C for 90 s; and a final extension step at 72°C for 7 min. Using the short universal primer UPS (CTAATACGACTCACTATAGGGC) and either EPNhsp3UTR2 (GCCGTGAAGCACTTCTCCGT) or EPNhsp3UTR3 (GAAGGGCAGCTTGAGTTCCG) specific primers, nested PCR generated a band that was cloned and sequenced. The 5′ end of Hb-hsp90-1 was generated using the gene specific primer EPNhsp5UTR3 (CGGAACTCAAGCTGCCCTTC) and a long Universal Primer Mix UPM. PCR was performed as follows: 30 cycles at 94°C for 30 s, 62°C for 30 s, and 72°C for 2 min; and a final extension step at 72°C for 7 min. Using the short universal primer UPS and gene specific primer EPNhsp5UTR1 (TCCTCGTTTGAGATGTCGTC), nested PCR generated a band that was cloned and sequenced.
Sequences obtained with 3′/5′ RACE were used to draw primers 5′UTRhspHbFor (GCGGAACCAAGGATGTCTGACG) and 3′UTRhspHbrev (TGTGATCTCTGCGTCATCTCG), which were used to amplify the entire gene and to verify the continuity of the sequence on cDNA.
Expression Pattern Analysis of Hb-hsp90-1
Total RNA was extracted from 1000 IJs and 500 adults of H. bacteriophora using RNeasy Tissue Mini Kit following the manufacturer’s instructions (Qiagen), and then treated with RNase-free DNase I set (Qiagen) to eliminate any contaminating genomic DNA. First-strand cDNA was synthesized from 50 ng of total RNA using a QuantiTect Reverse transcription kit (Qiagen) following the manufacturer’s instructions.
The ΔΔCt method was used to calculate relative expression among life stages and a portion of the 18S rRNA gene was used as endogenous control to normalize the transcription levels.
Real-time PCR was performed in 20 μl volumes containing 4 ng of cDNA, 10 μl 2 x Fast Start SYBR Green master mix (Roche) and 8 pmol of each specific primer.
The gene-specific primers used were hspHbfor3 (GAGCCTCAGTCACATGCTAC)/3′UTRhspHbrev (TGTGAT CTCTGCGTCATCTCG). The thermal profile for real-time PCR was 10 min at 95°C followed by 40 cycles at 95°C for 30 s, at 58°C for 30 s, and 72°C for 40 s. Dissociation curve analysis of amplification products was performed at the end of each PCR to confirm that only one PCR product was amplified and detected: 1 min at 95°C, 30 s at 55°C and 30 s at 95°C. The real-time experiments were conducted on a Stratagene thermal cycler and fluorescent real-time PCR data were analyzed using MX3000P software.
Determination of Temperature Tolerance
Procedures described by Mukuka et al. (2010b), were followed in order to estimate the range of temperatures at which the Apulian H. bacteriophora (LU1) can infect last-instar larvae of G. mellonella. Twenty replicates of last-instar larvae of G. mellonella were used for each temperature (12, 23, and 30°C) and were infected with 600 IJs of H. bacteriophora (30 IJs for each replicate) and were placed in three separate incubators; 23°C was considered as the control temperature. Fifty adults (hermaphrodites, females and males) and 100 IJs which emerged from dissected G. mellonella cadavers, respectively, at five and nine days after infection, were collected, washed with distilled water, frozen in liquid nitrogen and stored at –80°C before use.
In parallel, 50 adult hermaphrodites and 100 IJs, which emerged from G. mellonella cadavers were placed separately in water suspension, for 6 h at 23, 12, and 30°C. After incubation in aqueous solution, the nematode samples were frozen in liquid nitrogen and stored at −80°C before use.
Total RNA and expression profiles of Hb-hsp90-1 were performed as described in section “3′/5′ RACE.”
Double Stranded RNA (dsRNA) Preparation for Hb-hsp90-1
Templates for dsRNA were made by PCR on cDNA generated from RNA extracted from the aqueous suspension of H. bacteriophora IJs using a RNeasy kit (QIAGEN) according to the manufacturer’s instructions.
Two separate PCR reactions were performed, in which a fragment of Hb-hsp-90-1 gene was amplified with a T7 promoter sequence incorporated at the 5′ end of either the sense or antisense strand.
The Hb-hsp-90-1 primer sequences used for the reactions were T7hspHbfor3 (TAATACGACTCACTATAGGGGAGCCT CAGTCACATGCTAC) and 3′UTRhspHbrev (TGTGATC TCTGCGTCATCTCG) in the first reaction, and hspHbfor3 (GAGCCTCAGTCACATGCTAC) and T73′UTRhspHbrev (TAATACGACTCACTATAGGGTGTGATCTCTGCGTCATCT CG) in the second reaction.
PCR products were cleaned using a PCR purification kit (QIAGEN) and 1 μg of each PCR product was used for in vitro transcription with a Megascript kit (Ambion) according to the manufacturer’s instructions. The RNA generated in the two reactions was annealed to generate dsRNA. DNA and single-stranded RNA were removed by nuclease digestion (Megascript kit; Ambion). dsRNA was purified using filter cartridges (Ambion) and eluted in 100 μl of elution solution (Ambion). The dsRNA was verified by 1% agarose gel electrophoresis and quantified using a NanoDrop spectrophotometer. Three hundred H. bacteriophora IJs were collected and soaked in 40 μl Hb-Hsp-90-1dsRNA solution (1 μg/μl) for 24, 48, and 72 h, while controls were incubated in elution buffer. Treated and control nematodes were cleaned three times with DEPC-treated water and total RNA was then extracted. qPCR was used to analyze transcript suppression after RNAi treatment. All experiments were performed three times.
A specific portion of 18S rRNA gene was used as the endogenous control. Real-time PCR was performed in 20 μl volumes containing 6 ng of cDNA, 10 μl of 2 × Fast Start SYBR Green master mix (Roche) and 8 pmol of each specific primer.
Gene-specific primers used to analyze Hb-hsp90-1 transcript suppression were hspHbfor (GGCTCAAGCATTACG TGATTCG) and qPCR hspHbrev (TGAGGCTCCTCCAGA GTGA). PCR was as follows: 10 min at 95°C followed by 40 cycles at 95°C for 30 s, 58°C for 30 s, and 72°C for 40 s. Dissociation curve analysis of amplification products was also performed.
RNAi by Soaking
Six hundred IJs were kept in 30 μl of Hb-hsp90-1 dsRNA (1 μg/μl)-treated nematodes and in 30 μl of soaking buffer-untreated nematodes for 48 h. Three G. mellonella larvae were transferred into one sterile 50 mm Petri dishes containing three Whatmann paper disks. Hundred treated and untreated IJs, were used to infect 3 G. mellonella in each Petri dish (approximately 30 IJs per G. melonella) at two different temperatures 23 and 30°C. The bioassay was repeated three times for each temperature and treatment.
Mortality was recorded after 24 and 48 h for each treatment and temperature. For each treatment, three dead larvae were dissected after 5 days and three dead larvae after 10 days and three dead larvae were left in White traps to recover the progeny. Adult, hermaphrodites and IJs were recovered and counted at 5 and 10 days.
Hb-hsp90-1 Silencing Effect on the Motility of IJs
The effect of Hb-hsp90-1 silencing on nematode motility was determined. Thirty treated and untreated nematodes H. bacteriophora IJs were placed in the center of a 90 mm plate containing 3 mm water agar (1% w/v) in a 30 μl aliquot of ds buffer. For each plate one G. mellonella larva was placed at a distance of 40 mm from the nematodes and three replicates were conducted for each treatment. Petri dishes were incubated at 23°C in darkness and checked after 4 h for IJs movement. After 4 h evaporation of the ds buffer allowed the IJs to begin movement over the agar surface. RNAi treated and untreated nematode were observed under microscope in the release point. After 24 h, untreated IJs were not present in the release point, whereas several treated IJs were still found in the release point. After 48 h all treated IJs were not found in the release point.
Data Analysis
Data were pooled and analyzed using a general linear model procedure (ANOVA) and significant differences between means were separated using Tukey’s HSD test (STATISTIX 9.0, 2008). All comparisons were made at 0.05 level of significance.
Results
Molecular Characterization of Hb-hsp90-1
A PCR reaction on H. bacteriophora genomic DNA using degenerate primers produced two fragments of 255 and 366 bp. A BLASTX search of these sequences confirmed that both corresponded to heat shock protein90. The 366 bp sequence differed from the 255bp fragment that it had two introns, which were 58 and 54 bp in length, respectively. No introns were present in the 255 bp fragment. Sequence analyses of six clones containing the 255 bp fragments revealed the presence of three different homologous sequences to hsp90 without introns.
The two bands of 255 and 366 bp were also amplified in individual specimens of H. bacteriophora. The 366 bp sequence was identical to that obtained on gDNA and was named Hb-hsp90-1, while three clones containing the 255 bp fragments were intronless and identical to those already obtained on the gDNA. The intronless isoforms were named Hb-hsp90-2, Hb-hsp90-3, Hb-hsp90-4 and Hb-hsp90-5. The intronless Hb-hsp90-2 showed a 100% identity with Hb-hsp90-1, while the remaining isoforms without introns showed a 75% similarity to each other (64/85 identities) and 91% positive (78/85) at the amino acid level. The intron-rich isoform Hb-hsp90-1 showed a 74–75% similarity to the other three intron less isoforms. Since all independent clones corresponding to the four partial Hb-hsp90 genes isolated in H. bacteriophora were correctly translated, they cannot be attributed to sequencing errors. All eight intron-rich clones sequenced showed six nucleotide substitutions some of these polymorphisms were present in more than one clone and they do not change the amino acid sequence. Pairwise comparisons between the different clones of intronless Hb-hsp90 isoforms revealed a 98–99% similarity within each group, while the Hb-hsp90-3 isoform showed a 74 and 75% similarity with Hb-hsp90-4 and Hb-hsp90-5, respectively. Pairwise comparison between Hb-hsp90-4 and Hb-hsp90-5 isoforms showed 89% similarity. These partial intronless isoforms showed 33–36 nucleotide differences compared with the corresponding region of the Hb-hsp90-1 gene. All intron losses in our data set are exact.
The 366 bp intron rich fragment was fully characterized and the complete Hb-hsp90-1 gene and the corresponding cDNA were determined.
3′/5′ RACE experiments on the 366 bp produced the full-length cDNA of the Hb-hsp90-1. The full-length Hb-hsp90-1 cDNA was 2318 bp long, containing an open reading frame of 2139 bp, a 42 bp 5′ UTR and a 137 bp 3′ UTR containing the polyadenylation signal (AATAAA).
Amplification of H. bacteriophora gDNA using a primer on 5′UTR region in combination with a primer used for a 5′ end RACE gave two products: a 1250 bp fragment, corresponding to the gene region encompassing exon and intron sequences, and a 950 bp intronless fragment, Hb-hsp90-2, identical to the Hb-hsp90-1 cDNA. Amplification of H. bacteriophora gDNA, using a primer on the 3′UTR region in combination with a primer used for a 3′end RACE, produced only one fragment that shows the presence of introns. Amplification of H. bacteriophora gDNA, using primers located in the UTR regions of the cDNA produced only one fragment of 2789 bp for Hb-hsp90-1. Thus, Hb-hsp90-2 can be designed as a retrogene showing 3–9 nucleotide differences from Hb-hsp90-1 cDNA and indicating a recent duplication. Phylogenetic analyses of all Hb-hsp90 sequences obtained clustered in three different groups with high support (Figure 1): Group I included all Hb-hsp90 sequences with introns and the retrogene, while Group II and III were all intronless sequences.
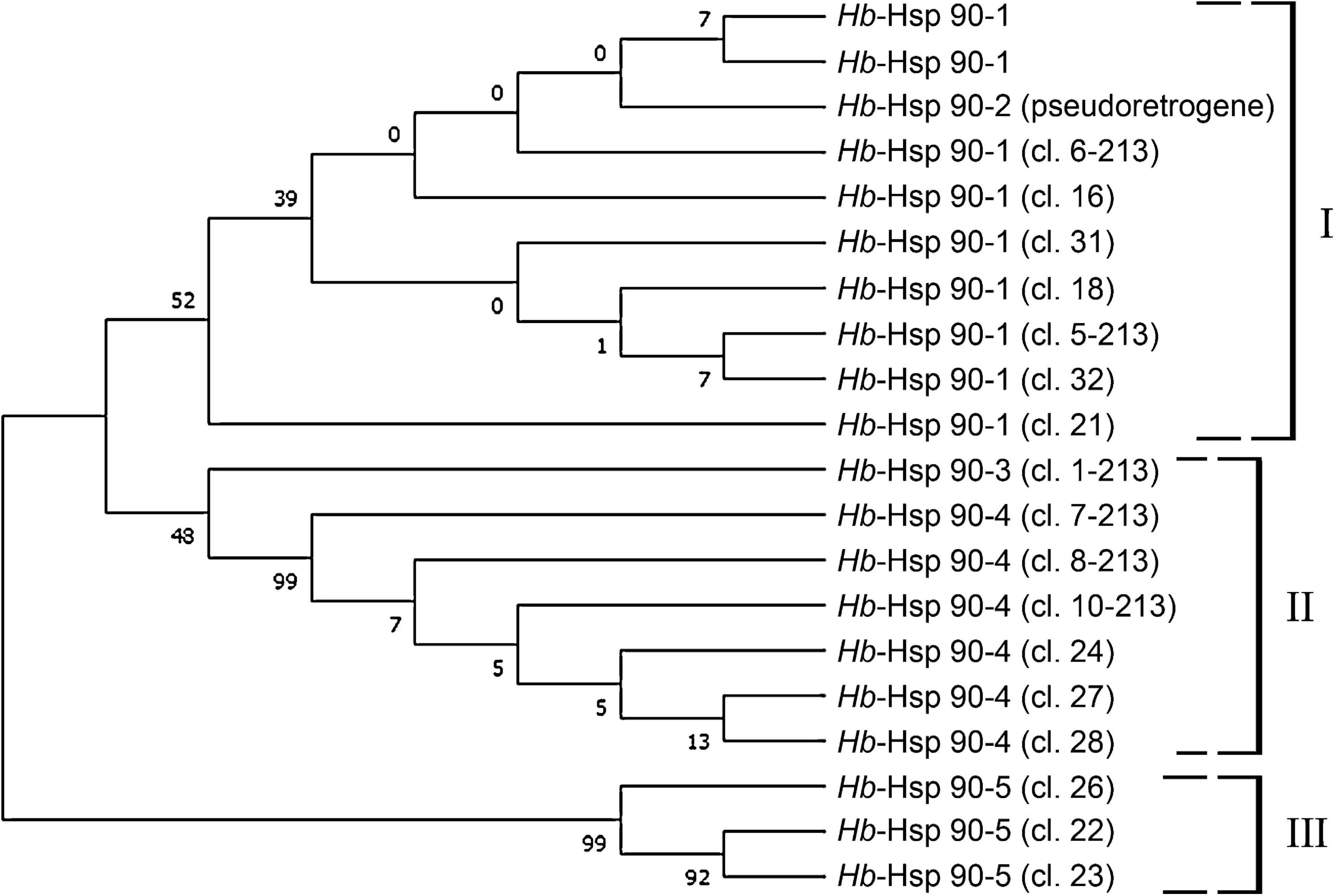
Figure 1. Phylogenetic analysis of the deduced amino acid sequences of Hsp90 isoforms from Heterorhabditis bacteriophora reveals the presence of distinct groupings and the duplication of the retropseudogene Hsp90-2 occurred relatively recently in Heterorhabditis bacteriophora.
The Hb-hsp90-1 ORF encoded a deduced protein of 712 amino acids showing the five typical features (Figure 2) common to all eukaryotic Hsp90 proteins, and the GxxGxG motif, consensus leucine zipper sequence, consensus LXXLL sequence and cytoplasmic Hsp90 sequence motif (MEEVD) are also present. The calculated molecular weight is 81,67 kDa and a theoretical pI is 4.87. A BLASTP search of the GenBank database using the Hb-Hsp90-1 amino acid sequence revealed that the best matches were to other nematodes including Ancylostoma ceylanicum (98% identical/96% similar/100% coverage), Ancylostoma duodenale (93%/96%/100%), Haemonchus contortus (93%/96%/100%), Angiostrongylus cantonensis (93%/96%/100% coverage), Necator americanus (93%/97%/100%), Caenorhabditis brenneri (89%/95%/100%), Caenorhabditis elegans (87%/94%/100%), Anisakis brevispiculata (84%/92%/100%), Brugia pahangi (84%/92%/100% coverage), Bursaphelenchus xylophilus (84%/92%/100%), Bursaphelenchus doui (83%/92%/100%), Steinernema carpocapsae (83%/92%/100%), Steinernema feltiae (81%/91%/100%), Ditylenchus destructor (81%/91%/100%), Heterodera glycines (80%/90%/100%), Meloidogyne incognita (79%/89%/100%), and Meloidogyne artiellia (78%/89%/100%). The newly obtained sequences were submitted to GenBank with the following accession numbers: LR890107–LR890114 for Hb-hsp90-1 sequences; LR890106 for mRNA Hb-hsp90-1; LR890105 for Hb-hsp90-2; LR890115–LR890117 for Hb-hsp90-3; LR890118–LR890120 for Hb-hsp90-3; LR890121–LR890123 for Hb-hsp90-5.
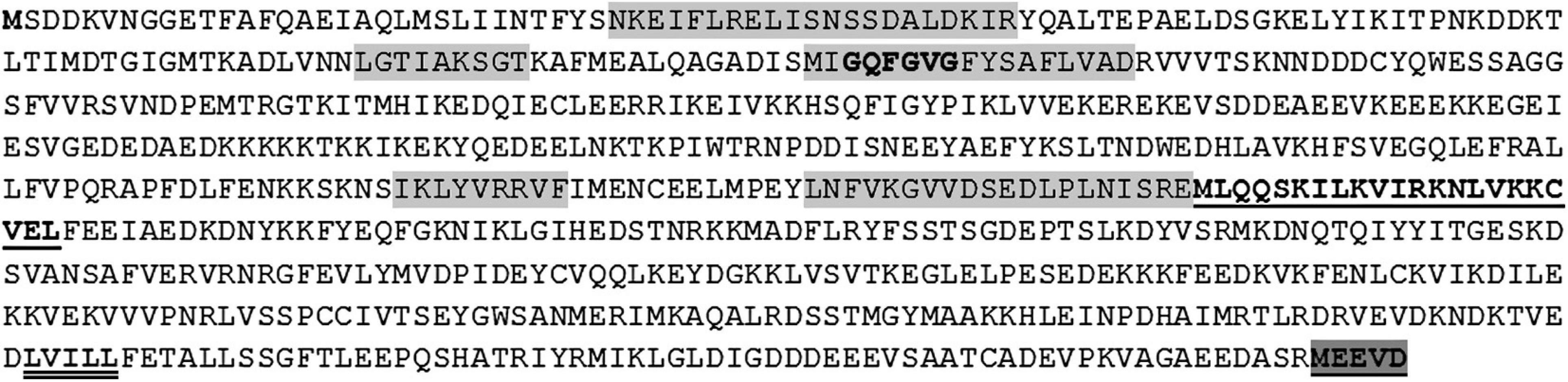
Figure 2. Deduced amino acid sequence of the Hb-Hsp90-1 of Heterorhabditis bacteriophora. The five Hsp90 signature sequences are shaded in light gray. GxxGxG motif is indicated in bold. The consensus leucine zipper sequence is double underlined. The consensus LxxLL sequence is indicated in bold underlined. The cytoplasmic Hsp90 sequence motif (MEEVD) is underlined and shaded in dark gray.
Gene Structure
The exon–intron boundaries of the genomic sequence of Hb-hsp90-1 gene were determined by aligning the genomic with the corresponding cDNA sequence which revealed 12 exons and 11 introns. The introns ranged in size from 54 to 70 bp and intron-exon junctions GT and AG were conserved.
Comparison of the complete hsp90 gene sequences from the free-living nematode C. elegans (Birnby et al., 2000), parasitic nematodes B. pahangi (Thompson et al., 2001) and M. artiellia (De Luca et al., 2009) and entomopathogenic nematode H. bacteriophora revealed that the number of introns is variable ranging from three introns in the free-living nematode to 9 in M. artiellia and 11 in H. bacteriophora and B. pahangi (Figure 3). Only the position of the last intron is conserved among the four nematodes. However, four more intron positions are conserved between Hb-hsp90-1 and Bp-hsp83 genes: the second, sixth, eighth, and ninth introns from H. bacteriophora are coincident with the third, sixth, seventh, and eighth in B. pahangi (Figure 3). Two more intron positions are conserved between Hb-hsp90-1 and Mt-hsp90 genes: the second and eighth introns from H. bacteriophora coincide with the second and eighth in M. artiellia (Figure 3). In addition Hb-hsp90-1 and Ce-hsp90 genes share other two intron positions: the first and fifth from H. bacteriophora coincide with the first and second in C. elegans (Figure 3).
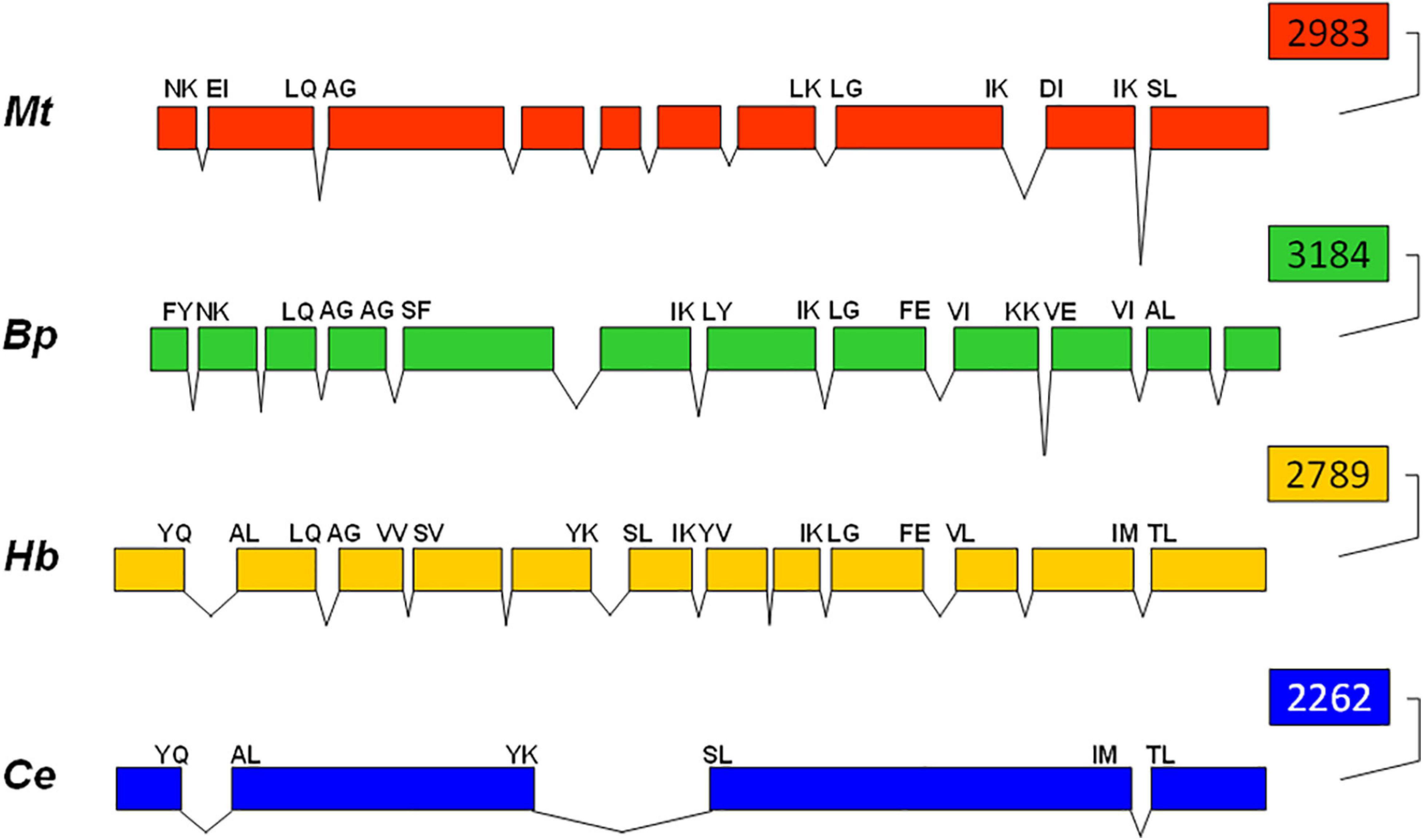
Figure 3. Comparison of intron-exon structure of Hb-hsp90-1 of Heterorhabditis bacteriophora compared with those of Meloidogyne artiellia, Brugia pahangi, and Caenorhabditis elegans. Exons are shown as boxes and introns as V-shaped lines. The amino acid at intron junctions indicated conserved positions between nematodes.
Expression Profiles of Hb-hsp90-1 in Adults and IJs During Parasitic Development
To evaluate the expression of Hb-hsp90-1 during invasion of G. mellonella host (Lepidoptera, Pyralidae), we isolated IJs and adults and determined their expression profiles. As shown in Figure 4, the Hb-hsp90-1 gene was constitutively expressed in the examined stages, but the Hb-hsp90-1 expression level was 10-fold higher in adults than in the IJs (Figure 4), thus suggesting that it plays a different role during different life stages.
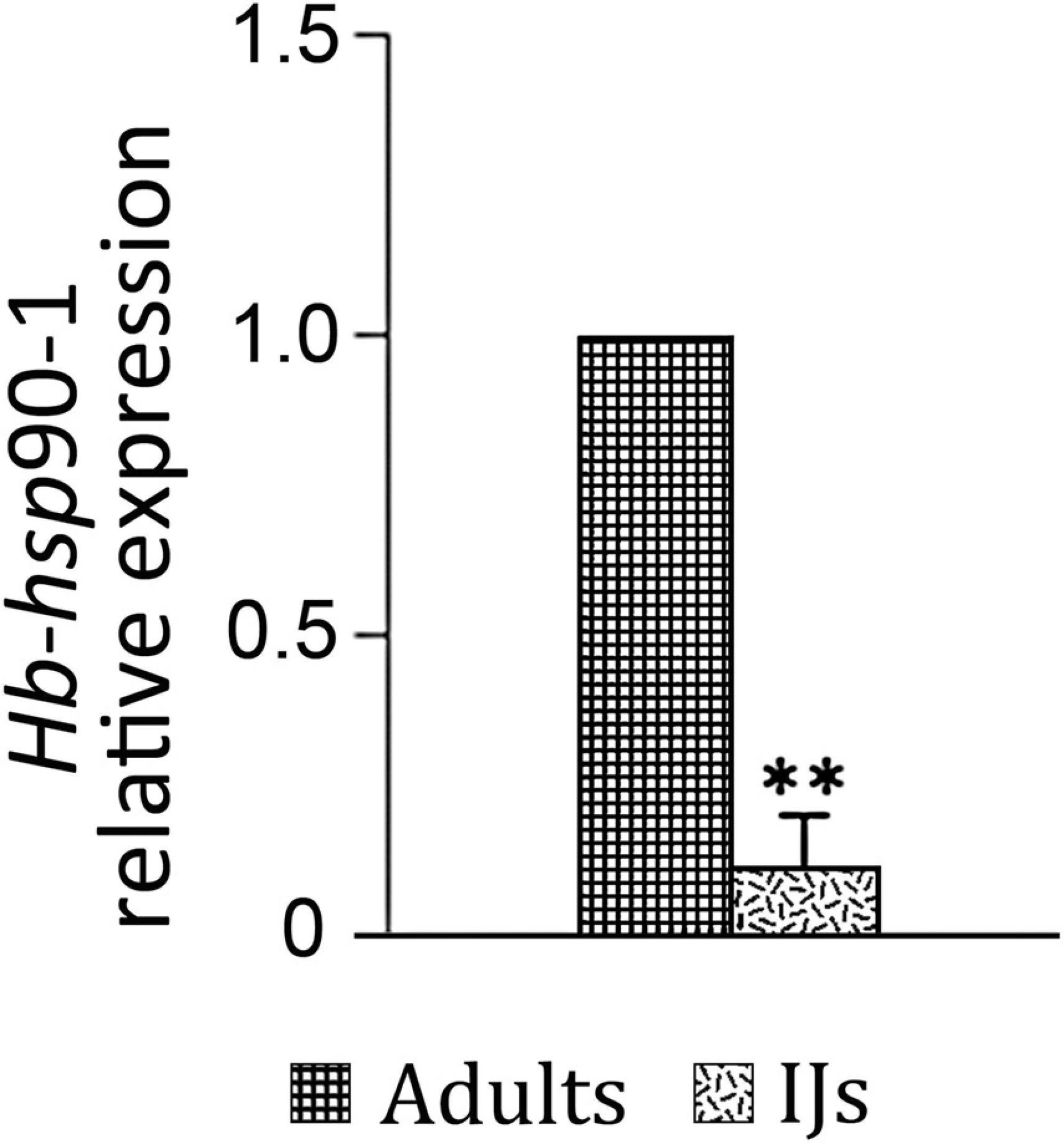
Figure 4. Expression of the Hb-hsp90-1 in Infective Juveniles (IJs) and adults. Bars indicate standard error of mean data (n = 3). (**P < 0.01).
Evaluation of the Effects of Lower and Higher Temperatures on Expression Profiles of Hb-hsp90-1 in Adults and IJs
In order to explore the effects of temperature levels on gene expression in H. bacteriophora, adults and IJs were incubated in aqueous suspension at 12 and 30°C, which are temperatures occurring in Apulia, respectively in winter and summer. Light microscope observations revealed that nematodes had promising levels of heat tolerance. Exposure of IJs to 12°C reduced their relative level of Hb-hsp90-1 transcript by 90% (p < 0.01) compared to the control at 23°C, while exposure to 30°C caused a 30% reduction (p < 0.05) (Figure 5A). Exposure of adults to 12°C reduced the relative level of Hb-hsp90-1 transcript by 45%, while exposure to 30°C caused a 62% reduction (p < 0.05) (Figure 5B). These data showed the lower and upper temperatures of 12 and 30°C, as initiating heat stress temperatures in H. bacteriophora.
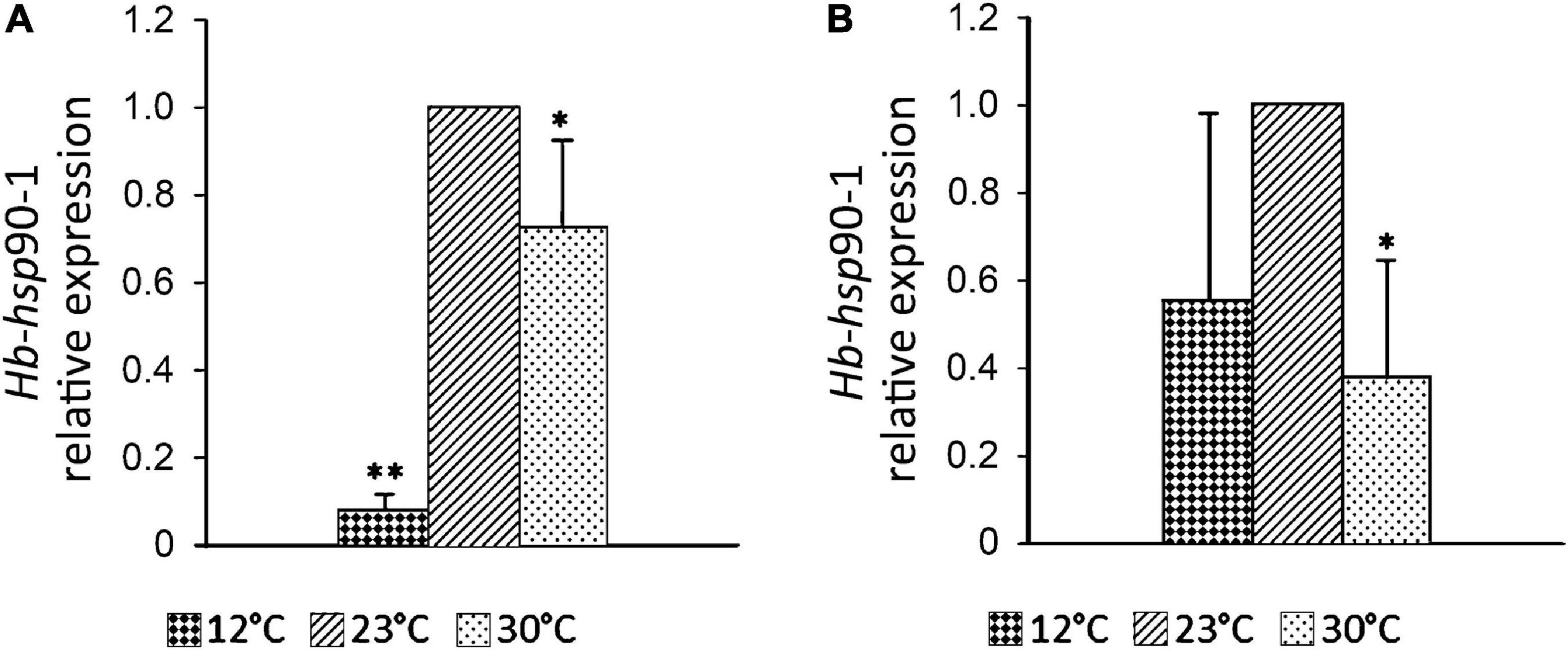
Figure 5. Expression profiles of the Hb-hsp90-1 of Heterorhabditis bacteriophora at 12°C, 23°C, and 30°C in IJs (A) and adults (B) in aqueous solution. Bars indicate standard error of mean data (n = 3). Significant differences (*P < 0.01; **P < 0.05) were found between control and treated nematodes.
Pathogenicity Analysis at the Lower and Higher Temperatures
Last-instar larvae of G. mellonella were infected with H. bacteriophora and then incubated at 12, 23, and 30°C for 10 days in order to determine the ability of IJs to be pathogenic. At 23°C, infected G. mellonella larvae died between the fourth and sixth day after infection; at 30°C, all infected G. mellonella larvae died after the fourth day after infection; at 12°C G. mellonella larvae survived or died of natural causes and no EPN reproduction was observed. At 23°C, adults and IJs were collected on the fifth and tenth day, respectively; at 30°C both adults and IJs were collected on the fifth and eighth day, respectively. Our results confirmed that IJ emergence from cadavers is related to temperature, with greater IJ emergence at 30°C than at 23°C. These results showed that the infectivity and reproductive potential of H. bacteriophora increased with increasing temperature (30°C), as observed by other authors (Tarasco et al., 2015; Raheel et al., 2017), whereas the low temperature caused the inactivity of the IJs in combination with their symbiotic bacteria.
Effect of Higher Temperature on Hb-hsp90-1 Expression During Parasitic Development
Infected last-instar larvae of G. mellonella were incubated at 23 and 30°C for 10 days to evaluate the expression of Hb-hsp90-1during invasion of G. mellonella and exposure to higher temperature. Adults and IJs were collected as described in the previous section and expression levels of Hb-hsp90-1 were measured.
The expression level of Hb-hsp90-1 at 30°C in IJs (Figure 6A) increased 8.84 times (p < 0.01) compared to the control at 23°C, but decreased by 80% (p < 0.01) in adults (Figure 6B). The up-regulation of Hb-hsp90-1 in IJs confirmed this gene’s important role for a normal lifespan and protection of protein folding at higher temperatures suggesting a continuous protective mechanism contributing to the tolerance of this strain. On the contrary, Hb-hsp90-1 expression in adults decreased in comparison with the control, suggesting that it plays a different role during their development.
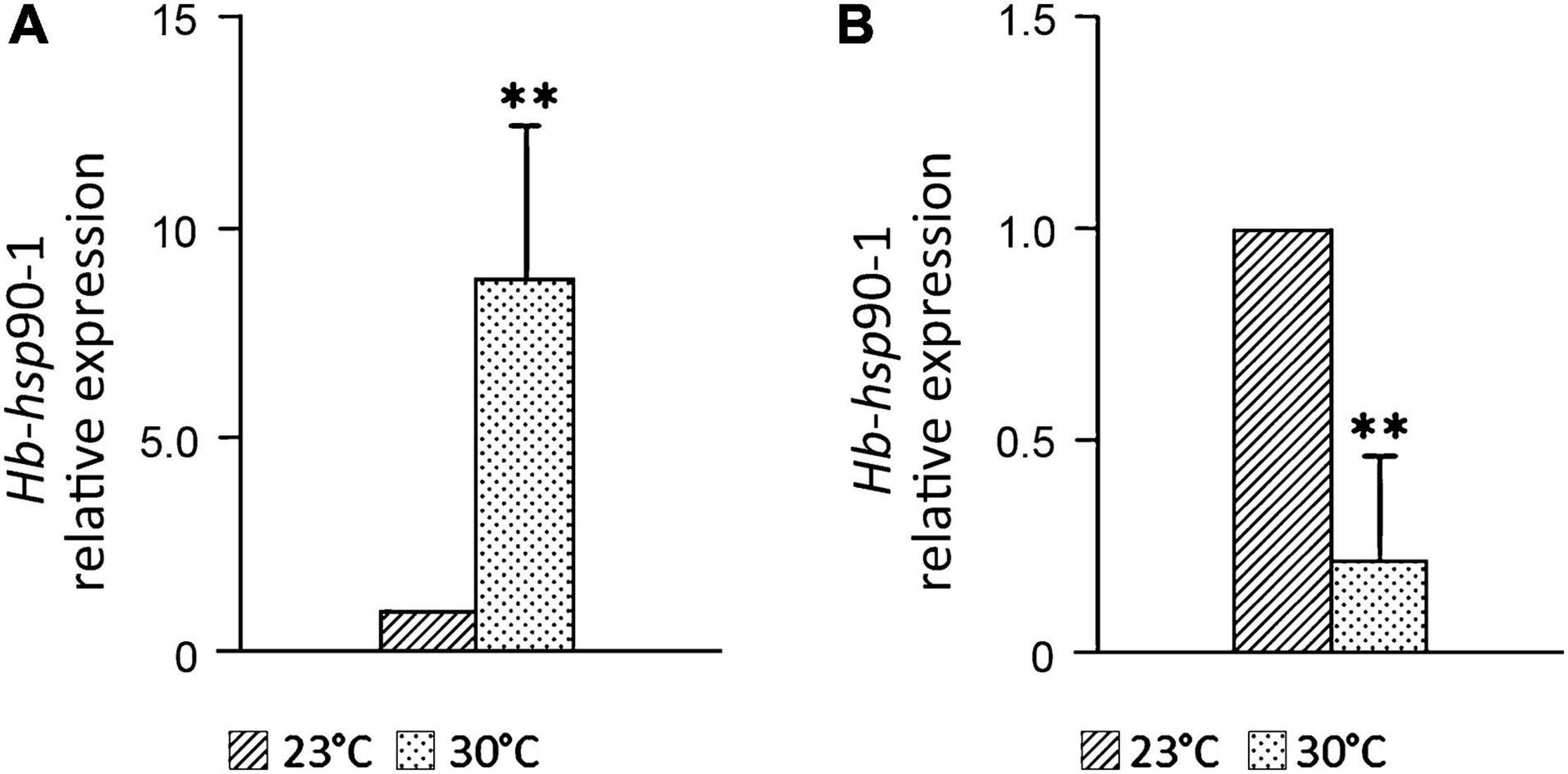
Figure 6. Expression profiles of the Hb-hsp90-1 of Heterorhabditis bacteriophora at 30°C in Infective Juveniles (IJ), (A) and adults (B) during parasitic development. Bars indicate standard error of mean data (n = 3). Significant differences (**P < 0.05) were found between temperature control and higher temperature.
Functional Analysis of dsRNA Hb-hsp90-1 Under Different Temperature Conditions
In order to assess the efficiency of the RNAi method, the expression levels of silenced Hb-hsp90-1 were determined in IJs and a consistent and statistically significant (P < 0.01) 76% reduction was observed between untreated and dsRNA-treated IJs after 48 h of soaking at 23°C (Figure 7). The effect of gene silencing on nematode phenotype was also assessed by light microscope, revealing motile inhibition of silenced Hb-hsp90-1 IJs (Figure 8). Untreated and silenced IJs were transferred in Petri dishes, containing a water agar layer, in the presence of unrestrained G. mellonella larvae (Figure 9B). After 4 h several untreated nematodes were visible at the release point, while after 24 h, no untreated nematodes were present at the release point. However, treated nematodes after 4 h, were at the release point (data not shown), and after 24 h several treated nematodes were still present at the release point (Figure 9B). After 48 h no silenced IJs were present at the release point (data not shown). These observations seem to suggest that the silencing of Hb-hsp90-1 impaired IJs thus reducing the ability to leave the release point and to locate G. mellonella compared to untreated IJs. Furthermore, this observation is consistent with the role of Hsp90 for normal lifespan and chemoreception in C. elegans (Birnby et al., 2000).
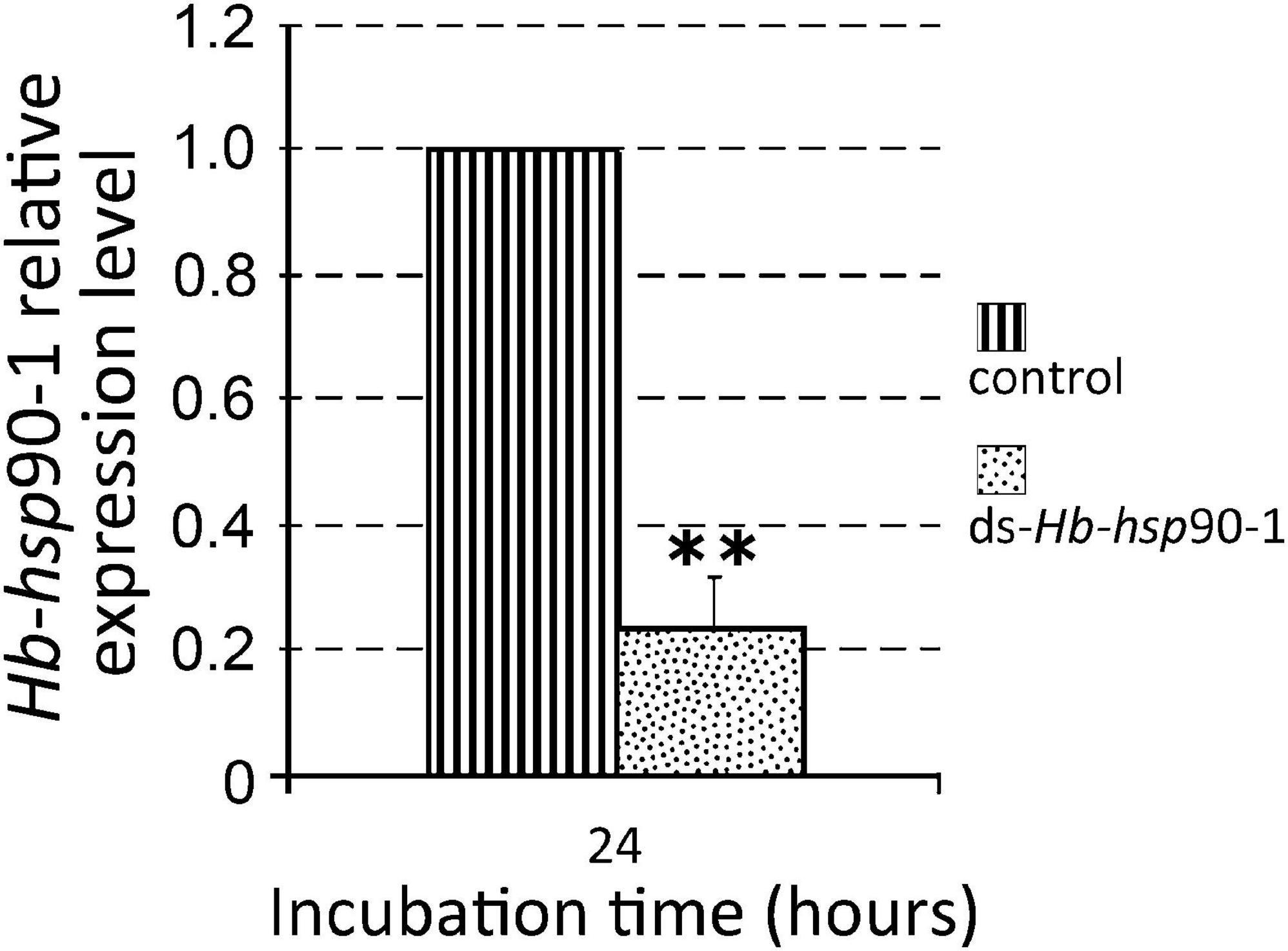
Figure 7. Expression of Hb-hsp90-1 in Infective Juveniles (IJs) of Heterorhabditis bacteriophora treated with dsRNA. Control consisted of untreated IJs for 24 h at 23°C. Significant differences (**P < 0.01) were found between treated and untreated IJs.
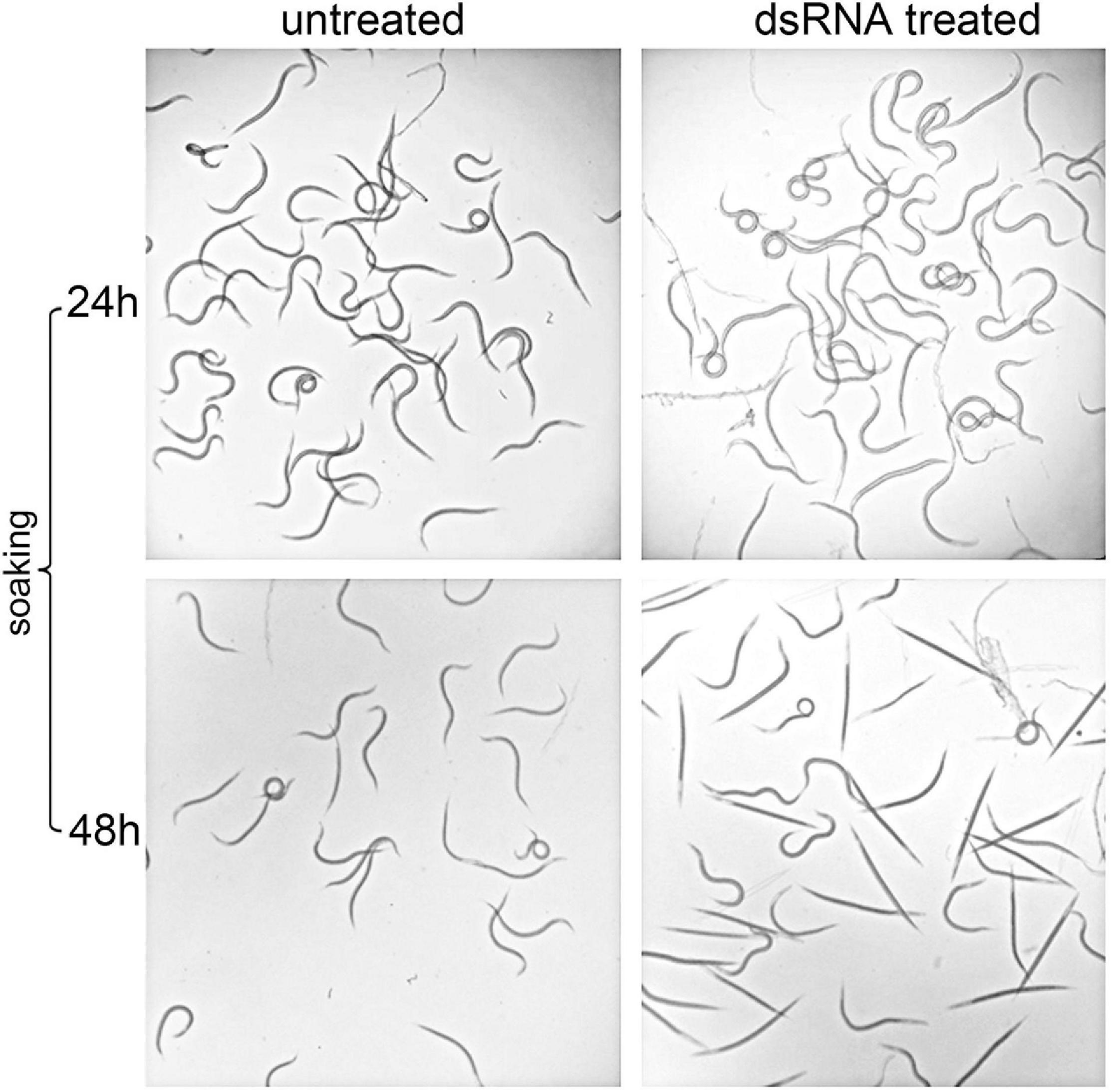
Figure 8. Light microscopy observations of RNAi mediated phenotypes of Infective Juveniles (IJs) of Heterorhabditis bacteriophora treated with dsRNA Hb-hsp90-1 after 24 and 48 h soaking at 23°C.
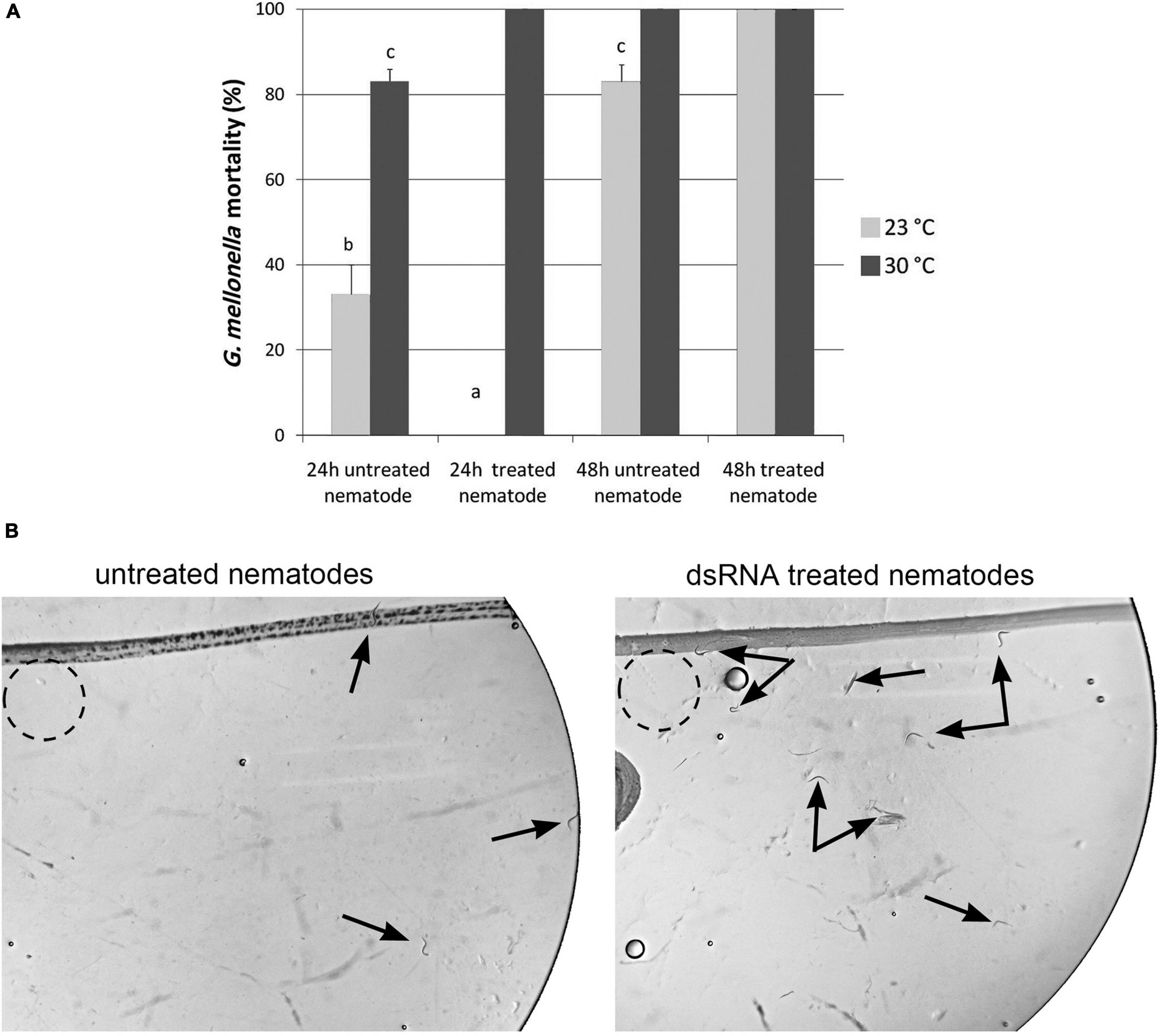
Figure 9. Mean percentage mortality of Galleria mellonella larvae after 24 and 48 h following exposure to dsRNA treated Infective Juveniles (IJs) and untreated Infective Juveniles at 23 and 30°C (A). Different letters above bars indicate statistical differences. Untreated and silenced Infective Juveniles (IJs) were transferred on Petri dishes, containing water agar layer, in presence of Galleria mellonella larvae to assess nematode attraction (B).
In order to further explore the role of Hb-hsp90-1, we investigated the infection and pathogenicity of silenced IJs in last-instar G. mellonella larvae at 23°C and 30°C.
After 24 h at 23°C and at 30°C, it was observed that untreated nematodes produced a G. mellonella mortality rate of 33 and 83%, respectively. After 24 h at 23 and 30°C, treated IJs produced no G. mellonella mortality at 23°C, while produced a larval mortality rate of 100% at 30°C (Figure 9A). After 48 h, treated and untreated nematodes caused high percentage of larvae mortality (>80%) at both 23 and 30°C (Figure 9A). These results clearly confirmed that the Hb-hsp90-1 gene is required for recovery process during normal growth and exposure to higher temperatures. Thus IJs are the thermotolerant stage of H. bacteriophora.
The untreated and dsRNA-treated G. mellonella were incubated at 23 and 30°C and dissected after 5 and 10 days in order to count adults and IJs. After 5 days at 23°C, the same number of adults were found in infected G. mellonella for both the untreated and treated nematodes, while after 10 days, no significant differences in numbers of adults and IJs were observed between untreated and treated nematodes (Figures 10, 11). After 5 days at 30°C, no significant differences were observed for adults and IJs between treated and untreated G. mellonella; but after 10 days at 30°C, adults and IJs were counted and no significant differences were observed between treated and untreated (Figures 10, 11). After 10 days at 23°C, no significant differences in numbers of adults and IJs, recovered using White traps, were observed between untreated and treated nematodes, but significantly higher than those recovered from dissected G. mellonella. After 10 days at 30°C, the numbers of adults and IJs in White traps were significantly higher (almost double) in G. mellonella infected with treated nematodes than those obtained with untreated nematodes (Figures 10, 11). These observations further confirmed that the Hb-hps90-1 gene plays a role in the recovery process, increasing the reproduction potential of H. bacteriophora, and in protecting it from higher temperature.
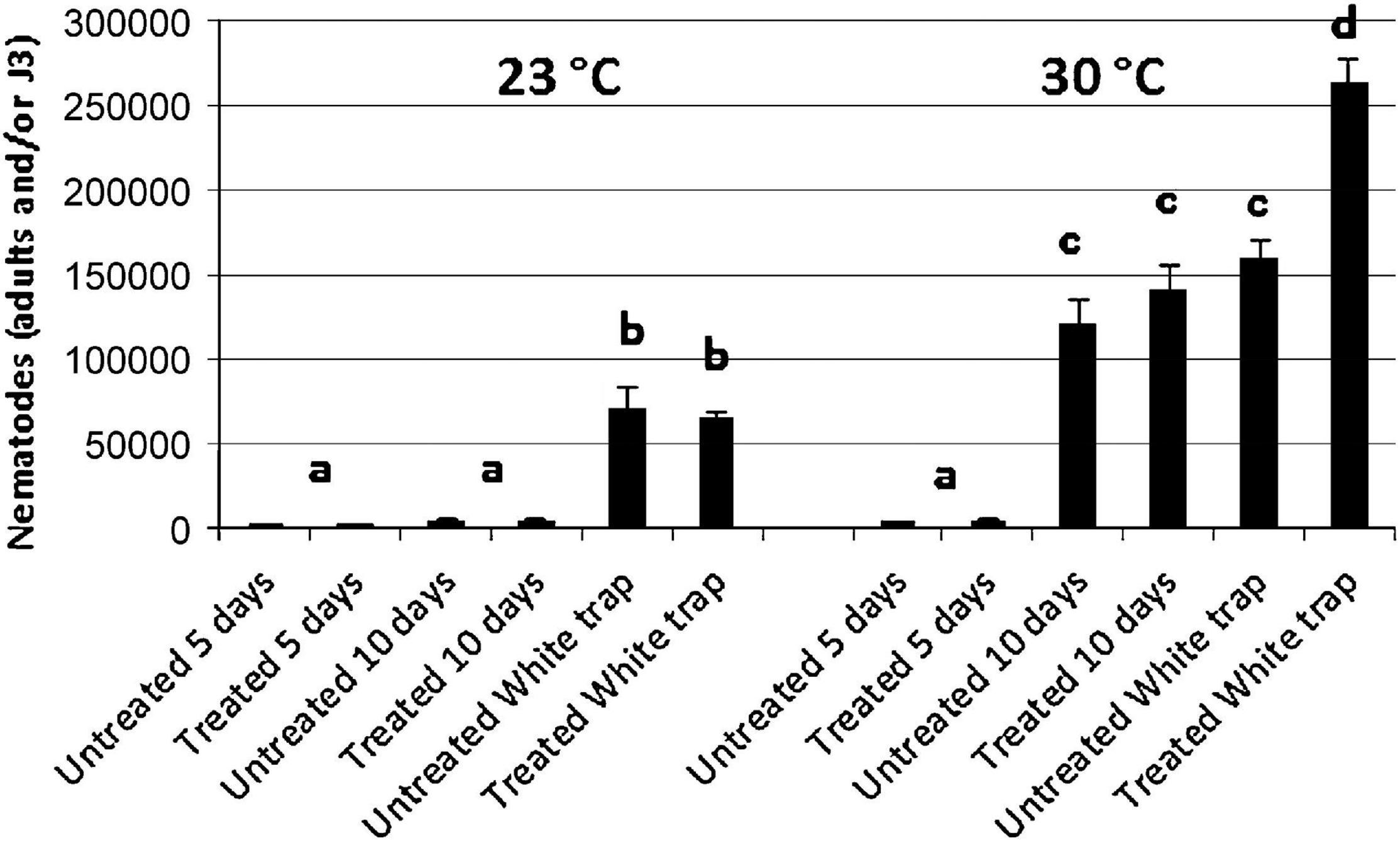
Figure 10. Number of adults and/or Infective Juveniles recovered from Galleria mellonella larvae after 5 and 10 days from infection with treated and untreated Infective Juveniles (IJs) at 23 and 30°C. Bars with the same letter have no significant differences (P < 0.05).
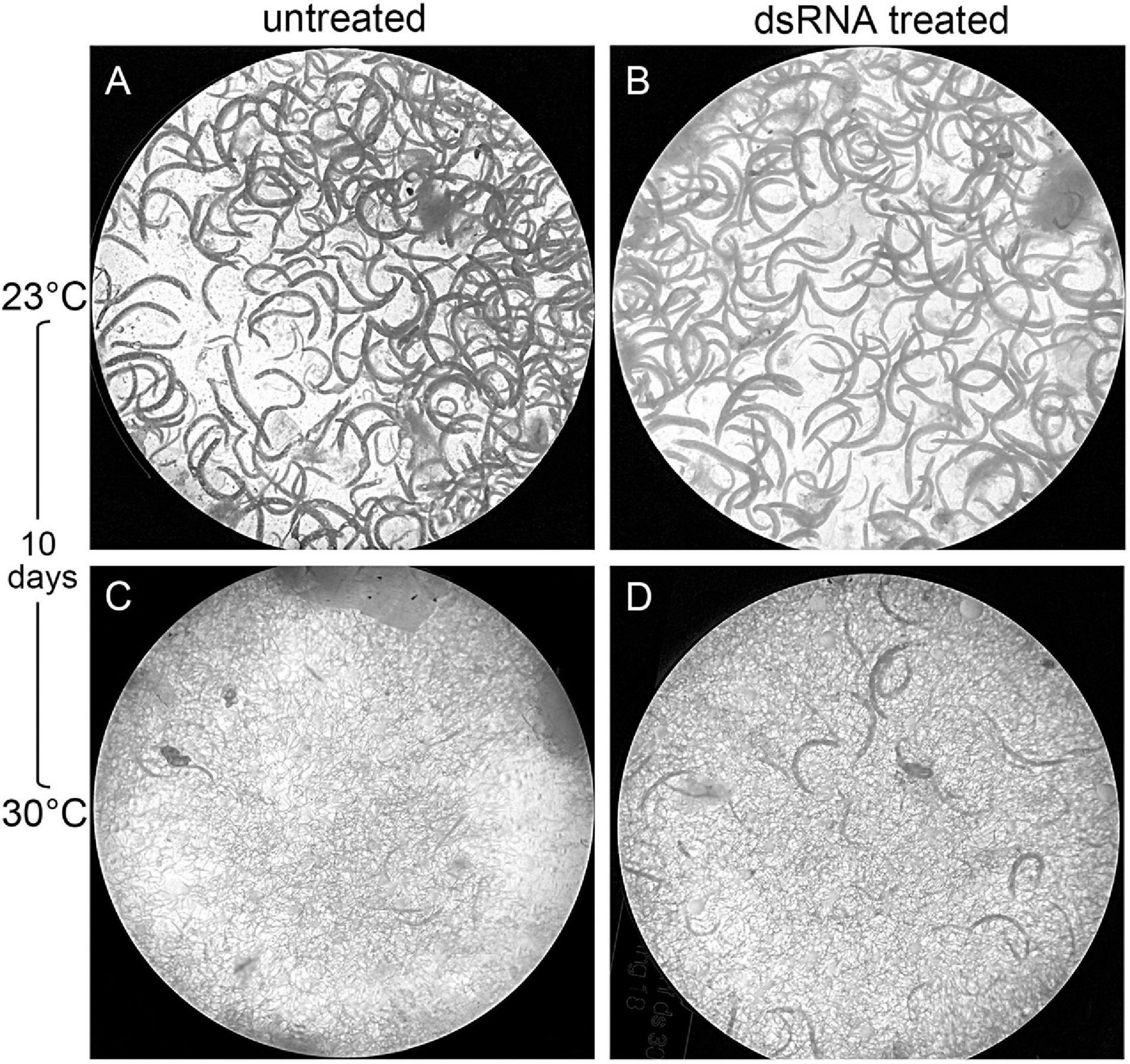
Figure 11. Effects of temperatures on the reproductive potential of treated and untreated Heterorhabditis bacteriophora inside Galleria mellonella larvae after 10 days infection. (A) untreated nematodes at 23°C; (B) dsRNA treated nematodes at 23°C; (C) untreated nematodes at 30°C; (D) dsRNA treated nematodes at 30°C.
Discussion
Soil is the natural habitat of EPNs and protects them from harmful environmental conditions. Their failure as efficient and effective biocontrol agents may be due to the interaction of different factors affecting their performance, such as ultraviolet radiation, extreme temperatures, and desiccation caused by low moisture levels (Shapiro-Ilan et al., 2006). The aim of this study was to elucidate at the molecular level the adaptability of Apulian H. bacteriophora, and also to investigate its development at different temperature levels in order to evaluate the effects of climate change on its host-seeking strategy and efficacy as a biocontrol agent. Since little is known about its molecular response to temperature changes the present study investigated the characterization and function of the Hb-hsp90-1 gene in H. bacteriophora and also examined its role in normal development and recovery process under different temperature conditions, i.e., no stress, low and high temperatures.
To date, little research has focused on the sequence structure and diversity of the hsp90 genes in Heterorhabditis and Steinernema spp. nematodes. The present study reports for the first time the characterization of five isoforms of Hb-hsp90 gene (named Hb-hsp90-1, Hb-hsp90-2, Hb-hsp90-3 to 5) in H. bacteriophora which differ in sequence diversity and in the presence/absence of introns. Sequence diversity and gene copy number variations (CNV) have been reported in plants, animals and in the nematode model C. elegans revealing that these mechanisms are important for genomic variation and usually affect gene families involved in adaptation to stressful or new environments (Maydan et al., 2010; Locke et al., 2015; Zmienko et al., 2016). It has been also reported by Farslow et al. (2015) that these mechanisms also occurred in C. elegans populations cultured in laboratory conditions. This finding provided evidence that these changes may be adaptative to laboratory conditions and then fixed. Thus the occurrence of different hsp90 DNA fragments in the Apulian H. bacteriophora, maintained in laboratory conditions, provide insights that these mechanism could be active in the EPN contributing to a de novo genetic novelty for hsp90 fragments and so the Apulian H. bacteriophora could be more prone to stressful and novel environments.
The intron-rich Hb-hsp90-1 isoform was fully characterized showing 99% identities with the annotated sequence (Hba_19207) of H. bacteriophora genome present in WormBase Parasite. The deduced protein determined in the present study is shorter than that reported in WormBase Parasite, showing the same length with other nematode Hsp90. Hb-Hsp90-1protein revealed the five highly conserved signature sequences and the conserved motif (MEEV) at the C-terminus (Figure 2). Sequence analyses revealed that Hb-hsp90-1 gene has eleven introns and 12 exons less than the Hba_19207 sequence showing twelve introns and 13 exons which predicted using bioinformatic programs. Introns are short, like those in the corresponding hsp-90 genes of other nematodes thus confirming that introns are under natural selection (Blumenthal and Spieth, 1996; De Luca et al., 2009; Carmel et al., 2012). A cross-species comparison demonstrated that genes involved in stress response, cell proliferation, differentiation and development have short introns and lower intron densities than genes with other functions (Jeffares et al., 2006). Moreover, organisms with short generation times, such as the free-living nematode C. elegans, have short introns and a significantly lower genome-wide intron density. This is because they are exposed to rapid environmental changes and so must rapidly respond to changing conditions, thus suggesting that introns may be detrimental to this process (Li et al., 2017). However, the intron density of Hb-hsp90-1 is identical to Bp-hsp90 of B. pahangi and Mt-hsp90 of M. artiellia and higher than C. elegans daf-21, which suggest that parasitic nematode’s introns might contribute to optimal gene expression, generate alternative spliced transcripts and increase the diversity in orthologous genes with identical function. The comparison of intron positions among the four nematode hsp90 homologs revealed three introns (Figure 3; 1, 5, and 11 introns) of Hb-hsp90-1 in conserved positions compared with C. elegans daf-21, and that only the last intron of Hb-hsp90-1 was in a conserved position in all four nematodes, suggesting that it is ancestral in nematodes (Rogozin et al., 2005; Henricson et al., 2010). Only two introns (2 and 8) of Hb-hsp90-1 were in conserved positions compared with the two parasitic nematodes M. artiellia and B. pahangi. Introns in conserved positions among orthologous genes are indicative of the functional importance of these introns within the coding region and suggest that they were already present in the equivalent positions of the corresponding ancestral gene. Thus, we can speculate that higher number of introns in Hb-hsp90-1 may affect the expression of the gene at several levels including stability and translation efficiency and adaptation to environmental changes. These findings seem to confirm that the number and the positions of introns in homologous hsp90 genes have changed during evolution providing a source of information about the evolutionary past (Carmel et al., 2007; Chorev and Carmel, 2012). The occurrence of the three intronless isoforms, Hb-hsp90-3 to 5 in the Italian strain of H. bacteriophora, also present in individual nematodes, suggests that they may be retropseudogenes or paralogous genes arising by gene duplication events with different sequence and gene structures leading to organismal evolution and acquisition of new functions (Katju and Lynch, 2003; Baskaran and Rödelsperger, 2015). A recent report documents two isoforms of the hsp90 gene in the plant parasitic nematode Pratylenchus penetrans, these differ in their nucleotide sequence and genomic structure (the presence of one or two introns) and are the result of independent duplication events (Pantzartzi et al., 2013; Fanelli et al., 2018). The occurrence of the Hb-hsp90-2 retrogene, which is 99% identical to Hb-hsp90-1 cDNA, is of great interest and emerged via reverse transcription of the corresponding mRNA, then randomly inserted into the genome. The partial duplication of Hb-hsp90-2 may be a non-functional gene fragment, and thus it can be speculated that this is an intronless intermediate, which then might accumulate new introns and new functions or may subsequently be deleted from the genome (Bai et al., 2007; Milanowski et al., 2016). Hb-hsp90-2 may be a young retropseudogene that is still recognizable because of its high sequence identity with Hb-hsp90-1. The in silico and phylogenetic analyses using all Hb-hsp90 sequences determined in this study show that all H. bacteriophora sequences group in different clusters, supporting the existence of different Hb-hsp90 isoforms (Figure 1) as shown in P. penetrans and other Eukaryotes. It has been reported in humans that the rate of retention of hsp90 duplicates is high because this gene family is involved in stress response (Pantzartzi et al., 2013). Our analyses support the existence of multiple genes in H. bacteriophora and the retention of duplicated genes could be correlated to different environmental stimuli upon their transfer from soil to insect hosts. Therefore, it will be necessary to prove whether these are expressed or defective copies of functional genes.
EPNs have adapted to develop and function within temperature ranges typical for their habitat, and so environmental characterization of EPNs can help in the selection of the strains most useful to control pests (Susurluk and Ehlers, 2008; Mukuka et al., 2010b; Shapiro-Ilan et al., 2014; Ulu and Susurluk, 2014). This process of heat acclimation increases thermotolerance and the duration of heat endurance (Treinin et al., 2003). Most H. bacteriophora strains have been isolated in sandy soil and near the sea, thus resulting a warm-adapted nematode. The current study demonstrates that the indigenous Apulian H. bacteriophora strain is well adapted to warm temperatures, since it can infect G. mellonella between 23 and 30°C. Furthermore, its reproduction was higher at 30°C, when it proved capable for killing its host more rapidly than at the control temperature. Conversely, the Apulian H. bacteriophora population was unable to reproduce at 12°C in G. mellonella indicating that this strain is not cold tolerant as IJs are already arrested stages adapted to withstand the unfavorable environmental conditions outside the host (De Luca et al., 2009). These results confirm the direct effects of the temperature on H. bacteriophora infectivity and pathogenicity as also observed by Castelletto et al. (2014).
Little is known about the molecular pathways or genes involved in the H. bacteriophora recovery process and signaling in response to temperature changes. Recent data showed that of heat shock proteins induced during the recovery process of H. bacteriophora IJs, Hb-hsp90-1 gene was up-regulated (Moshayov et al., 2013), confirming that IJs experience heat shock as part of their life cycle when they transfer from soil to host insects. It has been demonstrated in three plant parasitic nematodes, M. artiellia, P. vulnus, and B. xylophilus, and in the free-living nematode C. elegans, that the hsp-90 gene is involved in normal larval development, dauer formation regulation, chemosensation, proteostasis and response to temperature changes (De Luca et al., 2009; Wang et al., 2012; Fanelli et al., 2019). Chemotaxis in EPNs plays an important role in their host-seeking behavior, temperature changes can cause variability in host selection and can negatively affect EPN efficacy as biocontrol agents (Chaisson and Hallem, 2012; Lee et al., 2016). It has been reported that several heat shock proteins are induced in response to temperature changes enhancing tolerance of Heterorhabditis species to higher temperature (Mukuka et al., 2010a, b). Thus, we evaluated how Hb-hsp90-1 expression changed during normal growth and in response to temperature changes. Our results revealed that Hb-hsp90-1 is constitutively expressed in the studied developmental stages. Moreover, the level of Hb-hsp90-1 transcripts in adults is 10-fold higher than in IJs, thus confirming that H. bacteriophora IJs are arrested metabolic stages and the increase of Hb-hsp90-1 level during the recovery process reflected the transition from the arrested stages to the parasitic stages (Figure 4). In addition, the higher level of Hb-hsp90-1 in adults indicates that this gene is differentially regulated during normal growth. Exposure of H. bacteriophora IJs and adults in aqueous medium to different temperatures (12, 23, and 30°C) for 6 h, revealed that Hb-hsp90-1expression was down-regulated in both stages at 12 and 30°C, compared with 23°C, confirming the direct influence of temperature on these stages of H. bacteriophora (Figure 5). To validate the relationships between temperature increase, parasitic behavior and reproduction, transcript levels of Hb-hsp90-1 were determined in IJs and adults recovered from G. mellonella at 23 and 30°C after 10 days of incubation (Figure 6). Hb-hsp90-1 expression was up-regulated in IJs (Figure 6A), confirming that Hb-hsp90-1 is induced during the recovery process and thus involved in parasitic behavior within a restricted temperature range (23–30°C); an 80% reduction in the Hb-hsp90-1 level was actually observed in adults (Figure 6B). These results clearly demonstrate that IJs are the temperature tolerant stages of H. bacteriophora suggesting that Hb-hsp90-1 may act as chaperone for those molecules involved in temperature increase along with those involved in chemosensation and in interaction with G. mellonella hosts. On the contrary, adults reduced Hb-hsp90-1 expression to cope with adverse environmental conditions suggesting that adults are not thermotolerant stages. Thus, our results suggest that Hb-hsp90-1 could be also a part of the olfactory response in H. bacteriophora as found in other parasitic and free-living nematodes suggesting that this gene can optimize the adaptation of IJs to host life stages during seasonal temperature changes as also observed by others authors (Chaisson and Hallem, 2012; Lee et al., 2016).
To further confirm the function of Hb-hsp90-1 during normal lifespan and temperature increase, the gene was knocked down using RNAi as described in literature (Fanelli et al., 2005; Ciche and Sternberg, 2007; Lourenco-Tessutti et al., 2015) and the effect of gene silencing was followed in the IJs. Silenced IJs showed motile inhibition and behavioral aberration when observed using light microscopy (Figure 8), together with a 76% reduction in the Hb-hsp90-1 transcription level (Figure 7). The comparison of the behavior of treated and untreated IJs, laid at a specific release point within the agar plates in presence of G. mellonella larvae, revealed that both treated and untreated IJs were able to move within the release point, but whereas untreated IJs left the release point after 4 h, treated IJs were able to leave it after 24 h (Figure 9B). This finding prompted us to infer that the Hb-hsp90-1 silencing somehow impaired the motility in treated IJs. These results clearly demonstrated that the silencing effect is transient and not detectable after 24 h, highlighting the key role of Hb-hsp90-1 during the normal lifespan and it could be also involved in chemosensation in EPNs.
It was then tested whether temperature changes can alter the parasitic behavior of H. bacteriophora, using, untreated and silenced IJs, to infect G. mellonella cultured at 23 and 30°C. After 24 h, the mortality percentage of G. mellonella infected with untreated IJs at 23°C was lower than that of G. mellonella infected with untreated IJs at 30°C (Figure 9A). After 24 h, G. mellonella infected with silenced IJs showed no mortality at 23°C while those infected with silenced IJs at 30°C showed 100% mortality (Figure 9A). After 48 h, the percentage of larva mortality infected with the untreated IJs increased both at 23 and 30°C, but G. mellonella mortality was still higher at 30°C than at 23°C. After 48 h, the mortality percentage of G. mellonella infected with silenced IJs at both 23 and 30°C was higher (Figure 9A). These results show that RNAi on IJs of H. bacteriophora works well and it is effective at 23°C. Conversely, the dsRNA is no longer stable at 30°C and thus nematodes can reproduce more rapidly. All together these observations confirm that Hb-hsp90-1 is required for host location and the recovery process. It appears that a temperature of 30°C did not alter the recovery process of H. bacteriophora when infected G. mellonella larvae were cultured under constant temperature conditions. These observations clearly demonstrate that IJs are the thermotolerant stages of Apulian H. bacteriophora.
The dissection of G. mellonella infected with untreated and treated IJs to 23°C for 5 days revealed the same number of nematodes; after 10 days there were no significant differences between infected G. mellonella with untreated and treated IJs in the number of nematodes (Figures 10, 11). The dissection of G. mellonella infected with untreated and treated IJs to 30°C for 5 days revealed no significant differences in the number of nematodes between infected G. mellonella with untreated and treated IJs; after 10 days at 30°C no significant differences in the number of nematodes between infected G. mellonella with untreated and treated IJs (Figures 10, 11), but it was higher than that after 5 days. It is noteworthy that, after 10 days at 30°C, the numbers of adults and IJs in the White traps containing G. mellonella infected with silenced nematodes were significantly higher than those found in G. mellonella with untreated nematodes (Figures 10, 11). Thus, we can speculate that the continuous and higher expression of Hb-hsp90-1 can contribute to the survival of nematodes exposed to a higher temperature for a long time allowing H. bacteriophora IJs to acclimate, to modulate the recovery process and to enable them to target seasonal hosts or host life stages. In conclusion, our data demonstrate, for the first time, that Hb-hsp90-1 plays an important role in the recovery process for normal development and during temperature increases. Furthermore, these results seem to suggest that the expression level of Hb-hsp90-1 can contribute to the strong correlation between IJs lifespan and heat tolerance in H. bacteriophora, thus contributing to generate adapted and non-adapted strains. The occurrence of duplicated isoforms of Hb-hsp90-1 gene suggests that these may contribute to increasing the efficacy of the native strain of H. bacteriophora for insect control in the field. Finally, this study provides evidence that silencing by soaking can be used with success in H. bacteriophora, but after 24 h it is transient and unstable thus revealing that soaking technique can be used for identification of gene function and expression in EPNs.
Data Availability Statement
The data presented in the study are deposited in the GenBank repository, accession numbers: LR890107–LR890114 for Hb-hsp90-1 sequences; LR890106 for mRNA Hb-hsp90-1; LR890105 for Hb-hsp90-2; LR890115–LR890117 for Hb-hsp90-3; LR890118–LR890120 for Hb-hsp90-3; LR890121-LR890123 for Hb-hsp90-5.
Author Contributions
EF and FD conceived and designed the experiments and oversaw the manuscript preparation. EF performed the laboratory experiments and contributed to writing the results. AT counted nematodes and performed the microscope observations. ET performed the nematode cultures and stage separations. FD analyzed the data analysis and wrote the manuscript, which was read and approved by all authors.
Conflict of Interest
The authors declare that the research was conducted in the absence of any commercial or financial relationships that could be construed as a potential conflict of interest.
Acknowledgments
The authors thank Sarah Jane Christopher of the University of Bari (DISSPA) for her linguistic revision of the MS.
References
Bai, Y., Casola, C., Feschotte, C., and Betrán, E. (2007). Comparative genomics reveals a constant rate of origination and convergent acquisition of functional retrogenes in Drosophila. Genome Biol. 8:R11. doi: 10.1186/gb-2007-8-1-r11
Baskaran, P., and Rödelsperger, C. (2015). Microevolution of duplications and deletions and their impact on gene expression in the nematode Pristionchus pacificus. PLoS One 10:e0131136. doi: 10.1371/journal.pone.0131136
Birnby, D. A., Link, E. M., Vowels, J. J., Tian, H., Colacurcio, P. L., and Thomas, J. H. (2000). A transmembrane guanylyl cyclase (DAF-11) and Hsp90 (DAF-21) regulate a common set of chemosensory behaviors in Caenorhabditis elegans. Genetics 155, 85–104.
Blumenthal, T., and Spieth, J. (1996). Gene structure and organization in Caenorhabditis elegans. Curr. Opin. Genet. Dev. 6, 692–698. doi: 10.1016/s0959-437x(96)80022-0
Carmel, L., Csuros, M., and Koonin, E. V. (2012). Origin and evolution of spliceosomal introns. Biol. Direct 7, 11–39. doi: 10.1186/1745-6150-7-11
Carmel, L., Rogozin, I. B., Wolf, Y. I., and Koonin, E. V. (2007). Evolutionary conserved genes preferentially accumulate introns. Genome Res. 17, 1045–1050. doi: 10.1101/gr.5978207
Castelletto, M. L., Gang, S. S., Okubo, R. P., Tselikova, A., Nolan, T. J., Platzer, E. G., et al. (2014). Diverse host-seeking behaviors of skin-penetrating nematodes. PLoS Pathog. 10:e1004305. doi: 10.1371/journal.ppat.1004305
Chaisson, K. E., and Hallem, E. A. (2012). Chemosensory behaviors of parasites. Trends Parasitol. 28, 427–436. doi: 10.1016/j.pt.2012.07.004
Chen, S., Li, J., Han, X., and Moens, M. (2003). Effect of temperature on the pathogenicity of entomopathogenic nematodes (Steinernema and Heterorhabditis spp.) to Delia radicum. BioControl 48, 713–724.
Chorev, M., and Carmel, L. (2012). The function of introns. Front. Genet. 3, 1–15. doi: 10.3389/fgene.2012.00055
Ciche, T. A., and Sternberg, P. W. (2007). Postembryonic RNAi in Heterorhabditis bacteriophora: a nematode insect parasite and host for insect pathogenic symbionts. BMC Dev. Biol. 7, 101–112. doi: 10.1186/1471-213X-7-101
De Luca, F., Di Vito, M., Fanelli, E., Reyes, A., Greco, N., and De Giorgi, C. (2009). Characterization of the heat shock protein 90 gene in the plant parasitic nematode Meloidogyne artiellia and its expression as related to different developmental stages and temperature. Gene 440, 16–22. doi: 10.1016/j.gene.2009.03.020
De Luca, F., Fanelli, E., Di Vito, M., Reyes, A., and De Giorgi, C. (2004). Comparison of the sequences of the D3 expansion of the 26S ribosomal genes reveals different extent of heterogeneity in different populations and species of Pratylenchus from the Mediterranean region. Eur. J. Plant Pathol. 110, 949–957. doi: 10.1007/s10658-004-0813-4
Fanelli, E., Di Vito, M., Jones, J. T., and De Giorgi, C. (2005). Analysis of chitin syntase function in a plant parasitic nematode, Meloidogyne artiellia, using RNAi. Gene 349, 87–95. doi: 10.1016/j.gene.2004.11.045
Fanelli, E., Troccoli, A., and De Luca, F. (2019). Functional variation of two novel cellulases, Pv-eng-5 and Pv-eng-8, and the Heat Shock 90 Gene, Pv-hsp-90, in Pratylenchus vulnus and their expression in response to different temperature stress. Int. J. Mol. Sci. 20:107. doi: 10.3390/ijms20010107
Fanelli, E., Troccoli, A., Capriglia, F., Lucarelli, G., Vovlas, N., Greco, N., et al. (2018). Sequence variation in ribosomal DNA and in the nuclear hsp90 gene of Pratylenchus penetrans (Nematoda: Pratylenchidae) populations and phylogenetic analysis. Eur. J. Plant Pathol. 152, 355–365. doi: 10.1007/s10658-018-1480-1
Farslow, J. C., Lipinski, K. J., Packard, L. B., Edgley, M. L., Taylor, J., Flibotte, S., et al. (2015). Rapid increase in frequency of gene copy-number variants during experimental evolution in Caenorhabditis elegans. BMC Genomics 16, 1044–1061. doi: 10.1186/s12864-015-2253-2
Grewal, P. S., Bornstein-Forst, S., Burnell, A. M., Glazer, I., and Jagdale, G. B. (2006). Physiological, genetic, and molecular mechanisms of chemoreception, thermobiosis, and anhydrobiosis in entomopathogenic nematodes. Biol. Control 38, 54–65. doi: 10.1016/j.biocontrol.2005.09.004
Gupta, R. S. (1995). Phylogenetic analysis of the 90 kD heat shock family of protein sequences and an examination of the relationships among animals, plants and fungi species. Mol. Biol. Evol. 12, 1063–1073.
Hashmi, G., Hashmi, S., Selvan, S., Grewal, P., and Gaugler, R. (1997). Polymorphism in heat shock protein gene (hsp70) in entomopathogenic nematodes (Rhabditida). J. Thermal Biol. 22, 143–149. doi: 10.1016/S0306-4565(97)00005-3
Henricson, A., Forslund, K., and Sonnhammer, E. L. L. (2010). Orthology confers intron position conservation. BMC Genomics 11, 412–425. doi: 10.1186/1471-2164-11-412
Hoter, A., El-Sabban, M. E., and Naim, H. Y. (2018). The HSP90 family: Structure, regulation, function and implications in health and disease. Int. J. Molec. Sci. 19, 2560–2602. doi: 10.3390/ijms19092560
Inoue, T., Takamura, K., Yamae, H., Ise, N., Kawakami, M., Tabuse, Y., et al. (2003). Caenorhabditis elegans DAF-21 (HSP90) is characteristically and predominantly expressed in germline cells: Spatial and temporal analysis. Develop. Growth Differ. 45, 369–376. doi: 10.1046/j.1440-169x.2003.00706.x
Jeffares, D. C., Mourier, T., and Penny, D. (2006). The biology of intron gain and loss. Trends Genet. 22, 16–22. doi: 10.1016/j.tig.2005.10.006
Katju, V., and Lynch, M. (2003). The structure and early evolution of recently arisen gene duplicates in the Caenorhabditis elegans genome. Genetics 165, 1793–1803.
Konstantopoulou, I., and Scouras, Z. G. (1998). The Heat-Shock Gene hsp83 of Drosophila auraria. Genomic organization, nucleotide sequence, and long antiparallel coupled ORFs (LAC ORFs). J. Mol. Evol. 46, 334–343. doi: 10.1007/pl00006310
Lacey, L. A., Grzywacz, D., Shapiro-Ilan, D. I., Frutos, R., Brownbridge, M., and Goettel, M. S. (2015). Insect pathogens as biological control agents: Back to the future. J. Invertebr. Pathol. 132, 1–41. doi: 10.1016/j.jip.2015.07.009
Lee, J. H., Dillman, A. R., and Hallem, E. A. (2016). Temperature-dependent changes in the host-seeking behaviors of parasitic nematodes. BMC Biol. 14, 36–52. doi: 10.1186/s12915-016-0259-0
Lewis, E. E., and Clarke, D. J. (2012). “Nematode parasites and entomopathogens,” in Insect Pathology, eds F. E. Vega and H. K. Kaya (Amsterdam: Elsevier), 395–424. doi: 10.1016/b978-0-12-384984-7.00011-7
Li, Y. F., Xu, Y. H., and Ma, Z. W. (2017). Comparative analysis of the exon-intron structure in eukaryotic genomes. Yangtze Med. 1, 50–64. doi: 10.4236/ym.2017.11006
Locke, M. E. O., Milojevic, M., Eitutis, S. T., Patel, N., Wishart, A. E., Daley, M., et al. (2015). Genomic copy number variation in Mus musculus. BMC Genomics 16, 497–514. doi: 10.1186/s12864-015-1713-z
Lourenco-Tessutti, I. T., Souza, J. D. A. jr., Martins-de-Sa, D., Viana, A. B., Carneiro, R. M., Togawa, R. C., et al. (2015). Knock-down of heat-shock protein 90 and isocitratelyase gene expression reduced root-knot nematode reproduction. Phytopathology 105, 628–637. doi: 10.1094/PHYTO-09-14-0237-R
Maydan, J. S., Lorch, A., Edgley, M. L., Flibotte, S., and Moerman, D. G. (2010). Copy number variations in the genomes of twelve natural isolates of Caenorhabditis elegans. BMC Genomics 11, 62–73. doi: 10.1186/1471-2164-11-62
Milanowski, R., Gumińska, N., Karnkowska, A., Ishikawa, T., and Zakryś, B. (2016). Intermediate introns in nuclear genes of euglenids – are they a distinct type? BMC Evol. Biol. 16, 49–60. doi: 10.1186/s12862-016-0620-5
Moshayov, A., Koltai, H., and Glazer, I. (2013). Molecular characterization of the recovery process in the entomopathogenic nematode Heterorhabditis bacteriophora. Int. J. Parasitol. 43, 843–852. doi: 10.1016/j.ijpara.2013.05.009
Mukuka, J., Strauch, O., Hoppe, C., and Ehlers, R. (2010a). Improvement of heat and desiccation tolerance in Heterorhabditis bacteriophora through cross-breeding of tolerant strains and successive genetic selection. BioControl 55, 511–521. doi: 10.1007/s10526-010-9271-4
Mukuka, J., Strauch, O., Waeyenberge, L., Viaene, N., Moens, M., and Ehlers, R. (2010b). Heat tolerance among different strains of the entomopathogenic nematode Heterorhabditis bacteriophora. BioControl 55, 423–434. doi: 10.1007/s10526-009-9255-4
Pantzartzi, C. N., Drosopoulou, E., and Scouras, Z. G. (2013). Assessment and reconstruction of novel HSP90 genes: duplication, gains and losses in fungal and animal lineages. PLoS One 8:e73217. doi: 10.1371/journal.pone.0073217
Raheel, M., Javed, N., Khan, S. A., Aatif, H. M., and Ahmed, S. (2017). Effect of temperature on the reproductive potential of indigenous and exotic species of entomopathogenic nematodes inside Galleria mellonella L. larvae. Pak. J. Zool. 49, 395–397. doi: 10.17582/journal.pjz/2017.49.1.sc12
Rasmann, S., Helder, J., and van der Putten, W. H. (2012). Ecology and evolution of soil nematode chemotaxis. J. Chem. Ecol. 38, 615–628. doi: 10.1007/s10886-012-0118-6
Rogozin, I. B., Sverdlov, A. V., Babenko, V. N., and Koonin, E. V. (2005). Analysis of evolution of exon-intron structure of eukaryotic genes. Brief. Bioinf. 6, 118–134. doi: 10.1093/bib/6.2.118
Shapiro-Ilan, D. I., Gouge, D. H., Piggott, S. J., and Patterson Fife, J. (2006). Application technology and environmental considerations for use of entomopathogenic nematodes in biological control. Biol. Control. 38, 124–133. doi: 10.1016/j.biocontrol.2005.09.005
Shapiro-Ilan, D. I., Blackburn, D., Duncan, L., El-Borai, F. E., Koppenhofer, H., Tailliez, P., et al. (2014). Characterization of biocontrol traits in Heterorhabditis floridensis: a species with broad temperature tolerance. J. Nematol. 46, 336–345.
Skantar, A. M., and Carta, L. K. (2004). Molecular characterization and phylogenetic evaluation of the hsp90 gene from selected nematodes. J. Nematol. 36, 466–480.
Susurluk, A., and Ehlers, R.-U. (2008). First persistence of the entomopathogenic nematode Heterorhabditis bacteriophora in different crops. Biocontrol 53, 627–641. doi: 10.1007/s10526-007-9104-2
Tarasco, E., Clausi, M., Rappazzo, G., Panzavolta, T., Curto, G., Sorino, R., et al. (2015). Biodiversity of entomopathogenic nematodes in Italy. J. Helminthol. 89, 359–366.
Thompson, F. J., Cockroft, A. C., Wheatley, I., Britton, C., and Devaney, E. (2001). Heat shock and developmental expression of hsp83 in the filarial nematode Brugia pahangi. Eur. J. Biochem. 268, 5808–5815. doi: 10.1046/j.0014-2956.2001.02525.x
Treinin, M., Shliar, J., Jiang, H., Powell-Coffman, J. A., Bromberg, Z., and Horowitz, M. (2003). HIF-1 is required for heat acclimation in the nematode Caenorhabditis elegans. Physiol. Genomics 14, 17–24. doi: 10.1152/physiolgenomics.00179.2002
Ulu, T. C., and Susurluk, I. A. (2014). Heat and desiccation tolerances of Heterorhabditis bacteriophora strains and relationships between their tolerances and some bioecological characteristics. ISJ 11, 4–10.
Wang, F., Wang, Z., Li, D., and Chen, Q. (2012). Identification and characterization of a Bursaphelenchus xylophilus (Aphelenchida: Aphelenchoididae) thermotolerance-related gene: Bx-HSP90. Int. J. Mol. Sci. 13, 8819–8833. doi: 10.3390/ijms13078819
White, G. F. (1927). A method for obtaining infective nematode larvae from cultures. Science 66, 302–303. doi: 10.1126/science.66.1709.302-a
Woodring, J. L., and Kaya, H. K. (1988). Steinernematid and heterorhabditid nematodes: A handbook of biology and techniques. Fayetteville, AR: Southern Cooperative Series Bulletin.
Zmienko, A., Samelak-Czajka, A., Kozlowski, P., Szymanska, M., and Figlerowicz, M. (2016). Arabidopsis thaliana population analysis reveals high plasticity of the genomic region spanning MSH2, AT3G18530 and AT3G18535 genes and provides evidence for NAHR-driven recurrent CNV events occurring in this location. BMC Genomics 17, 893–908. doi: 10.1186/s12864-016-3221-1
Keywords: acclimation, EPN, gene duplication, heat shock protein, thermotolerance, silencing
Citation: Fanelli E, Troccoli A, Tarasco E and De Luca F (2021) Molecular Characterization and Functional Analysis of the Hb-hsp90-1 Gene in Relation to Temperature Changes in Heterorhabditis bacteriophora. Front. Physiol. 12:615653. doi: 10.3389/fphys.2021.615653
Received: 20 October 2020; Accepted: 26 January 2021;
Published: 23 February 2021.
Edited by:
Ioannis Eleftherianos, George Washington University, United StatesReviewed by:
Adler Ray Dillman, University of California, Riverside, United StatesItamar Glazer, Institute of Plant Sciences, Agricultural Research Organization, Volcani Center, Israel
Copyright © 2021 Fanelli, Troccoli, Tarasco and De Luca. This is an open-access article distributed under the terms of the Creative Commons Attribution License (CC BY). The use, distribution or reproduction in other forums is permitted, provided the original author(s) and the copyright owner(s) are credited and that the original publication in this journal is cited, in accordance with accepted academic practice. No use, distribution or reproduction is permitted which does not comply with these terms.
*Correspondence: Francesca De Luca, francesca.deluca@ipsp.cnr.it