- 1Key Laboratory of Bio-Pesticide Innovation and Application, Engineering Research Centre of Biological Control, South China Agricultural University, Guangzhou, China
- 2Yongzhou Tobacco Company, Yongzhou, Hunan, China
- 3Department of Eco-Engineering, Guangdong Eco-Engineering Polytechnic, Guangzhou, China
- 4Independent Researcher, York, United Kingdom
Matrine, a naturally occurring heterocyclic compound, has been shown to enhance the pathogenicity of the entomopathogenic fungus Beauveria brongniartii against Spodoptera litura. In the current study, the biological impacts and synergism activities of these two agents on nutritional efficiency and antioxidant enzymes in S. litura were explored. Our results showed a high antifeedant activity of B. brongniartii and matrine on S. litura. The S. litura larvae were unable to pupate and emerge when treated with combinations of matrine and B. brongniartii. Following on, we measured the activities of five important antioxidant enzymes [superoxide dismutase (SOD), peroxidase (POD), catalase (CAT), acetylcholinesterase (AChE), and glutathione-S-transferase (GST)] when treated with B. brongniartii SB010 (1 × 109 spores/ml), matrine (0.5 mg/ml), and B. brongniartii SB010 (1 × 109 spores/ml) + matrine (0.5 mg/ml). The results indicated the detoxification activity of the five enzymes in the fat body and hemolymph of S. litura when facing a combined B. brongniartii and matrine challenge. The activities of the enzymes were significantly lower than that of the control group 7 days post-treatment, indicating the inhibitory effect of the two xenobiotics. Matrine had better inhibition effects than B. brongniartii in a majority of the trials. The improved detoxification activity of the five enzymes may be the internal mechanism of synergism of matrine on B. brongniartii.
Introduction
The tobacco cutworm, Spodoptera litura (Fabricius; Lepidoptera: Noctuidae), is a serious polyphagous herbivore, which has caused significant economic damage to cropping systems around the world. As a result, over the past decades large quantities of chemical pesticides have been applied for the control of S. litura. However, overuse of synthetic pesticides has resulted in reports of adverse effects, such as pesticide resistance and pest resurgence, and also the contribution to negative impacts on human health and other components of the environment (Cuthbertson and Murchie, 2005; Ishtiaq et al., 2012; Ahmad and Mehmood, 2015). Due to these reasons, finding alternate control methods including the use of natural control agents is continuing to play an important role in developing management strategies for S. litura (Revathi et al., 2014).
Entomogenous fungi are important agents for the biological control of S. litura (Chaudhari et al., 2016; Nguyen et al., 2017; Yang et al., 2019). As one of the most common insect pathogenic fungal species, fungi belonging to the genus Beauveria (Hypocreales: Cordycipitaceae) have shown high insecticidal activity against S. litura (Borja et al., 2018; Dhar et al., 2019; Yang et al., 2019). Among them, Beauveria brongniartii is one of the most effective pathogenic species and has been widely used in insect control (Fan et al., 2013; Liu et al., 2016). Previous studies have shown that B. brongniartii isolate SB010 has high pathogenic activity against different insect species (Huang, 2019; Ou, 2019).
Matrine, a naturally occurring heterocyclic compound, is isolated from the roots of Sophora flavescens (Kushen) and Sophora alopecuroides (Fabales: Fabaceae; Mao and Henderson, 2007; Zhang et al., 2012; Cheng et al., 2018). Since matrine was discovered, its biological activity has received worldwide attention. It has been shown that matrine has a wide range of biological properties including antitumor activity, antiviral activity, and antifungal activity (Li et al., 2010; Liu et al., 2014; Zanardi et al., 2015). As a well-known traditional medicine in China, matrine has also been shown to have broad spectrum insecticidal activity, as well as being relatively non-toxic to the environment (Huang and Xu, 2016; Ali et al., 2017). Based on the research of Wu et al. (2019), matrine could significantly increase the pathogenicity of B. brongniartii isolate SB010 and as such is considered as a potential control agent against S. litura.
Insects are known to cope with threats of both insecticide and pathogen through the action of different enzyme systems (Terriere, 1984; Roslavtseva et al., 1993; Serebrov et al., 2006; Dubovskiy et al., 2012). General esterases (ESTs), glutathione-S-transferase (GST), and multi-function oxidases (MFOs) are most commonly involved in insect defense (Li et al., 2007). MFOs involved in the P450 pathways are involved in detoxification of natural and synthetic xenobiotics (Feyereisen, 2005). GST plays a pivotal role in detoxification and cellular antioxidant defenses against oxidative stress by conjugating reduced glutathione to the electrophilic centers of natural and synthetic exogenous xenobiotics, including insecticides, allelochemicals, and endogenously activated compounds (Ortelli et al., 2003; Enayati et al., 2005; Lumjuan et al., 2005). ESTs play an important role in insect defense through catabolism of the esters of higher fatty acids that influence flight as well in the degradation of inert metabolic esters (Terriere, 1984; Roslavtseva et al., 1993) and various xenobiotics, including insecticides. Changes in the activities of these enzymes are reflected in insect resistance to insecticides as well as in degradation of toxic molecules produced during M. anisopliae infection and, therefore, play a key role in the protection of insects against pathogens (Terriere, 1984; Roslavtseva et al., 1993; Serebrov et al., 2006; Dubovskiy et al., 2012).
Several other insect enzymes [including antioxidant enzymes such as superoxide dismutase (SOD), catalase (CAT), and peroxidase (POD)] provide defenses against pathogens and insecticides (Felton and Summers, 1995). Previous studies showed that these enzymes can be quickly upregulated in response to xenobiotic threats and that increase in the activities of these enzymes are related to pesticide resistance and melanization in insects (Campa-Córdova et al., 2002; Müller et al., 2007; Gopalakrishnan et al., 2011; Wu et al., 2011). The phenol oxidase (PO) cascade protects insects from microbial infections through its involvement in the melanization of hemocytes attached to parasite surfaces (Cerenius and Söderhäll, 2004). Increased PO activity can improve immunity to xenobiotics and promote wound healing (Liu et al., 2009). Acetylcholinesterase (AChE) is a key enzyme catalyzing the hydrolysis of the neurotransmitter acetylcholine (Ach) in the nervous system of various organisms (Oehmichen and Besserer, 1982). AChE is affected by organophosphate and carbamate insecticides, botanical insecticides, and secondary fungal metabolites (Zibaee et al., 2009). Although previous experiments by Wu et al. (2019) showed that the combination of B. brongniartii and matrine had a synergistic effect; however, the specific antioxidant defense response in S. litura is not clear when facing a combined B. brongniartii and matrine challenge.
In this study, the biological impacts of B. brongniartii and matrine on nutritional efficiency and detoxification enzymes (GST, AChE, SOD, CAT, and POD) in S. litura were explored. Our results provide further information in support of the combined application of entomopathogenic fungi and natural chemicals in the development of S. litura control strategies.
Materials and Methods
Insects
Larvae of S. litura were reared on a semi-synthetic artificial diet prepared following the method of David et al. (1975) and kept under laboratory conditions of 26 ± 2°C, 65 ± 5% relative humidity (r.h.), and 16:8 h (light:dark) photoperiod at South China Agricultural University (SCAU), Guangzhou, China.
Chemicals and Fungal Preparations
Matrine (95% purity) was obtained from Guangdong New Scene Bioengineering Company, Yangjiang, China. Five different concentrations of matrine (0.05, 0.125, 0.25, 0.5, and 1.0 mg/ml) were prepared by dissolving matrine powder in methanol.
Beauveria brongniartii isolate SB010 was used in the trials. This isolate was obtained from soil samples taken from the experimental farm fields of SCAU and kept in the Key Laboratory of Biopesticides Innovation and Application of Guangdong Province, SCAU. A fungal spore suspension (1 × 109conidia/ml) was then prepared following the method of Ali et al. (2017).
Scheme of Experiments
The efficacy and nutrition trails were conducted by applying nine different individual or combined treatments of B. brongniartii and matrine as outlined in Table 1. In case of enzymatic studies, four different individual or combined treatments of B. brongniartii and matrine as shown in Table 2 were applied to study the variations in enzymatic studies.
Nutrition Analysis
Newly molted third instar larvae of S. litura from the same batch were used for the nutrition experiment. Individual or combined treatments of B. brongniartii and matrine as outlined in Table 1 were added during the molten state of artificial diet preparation. Artificial diet without the addition of fungal conidia or matrine designated as T1 in Table 1 served as control. The larvae (10 individuals) were individually placed in plastic cups (diameter: 3 cm and height: 4 cm) to feed on 1 g of pre-treated as well as control diet. The experimental setup was incubated at 25 ± 2°C, 80 ± 5% relative humidity, and 16:8 h (light:dark) photoperiod. The whole experimental setup was repeated thrice, thus each treatment was repeated 30 times. The larvae and artificial diet were weighed everyday by using an electronic balance (precision: 0.0001 g) to record the larvae weight gain and food weight loss until 21 days post application. The weight of the third instar larvae after 12 h of molting was used as the starting weight. The pupation rate and emergence rate were also recorded.
Nutritional indices were calculated according to Koul et al. (1997, 2004):
Relative growth rate (RGR) = (change in the larval dry weight per day/starting larval dry weight).
Relative consumption rate (RCR) = change in the larval diet dry weight per day/starting larval dry weight.
Efficiency of conversion of ingested food (ECI) = 100 × dry weight gain of the insect/dry weight of food consumed.
The number of successfully pupated S. litura larvae treated with individual or combined treatments of B. brongniartii and matrine was recorded after 30 days of treatment. The rate of pupation (%) was calculated by following equation.
Percent pupation = (Number of pupated larvae/Total number of larvae) × 100.
The number of successfully emerged adults from S. litura larvae treated with individual or combined treatments of B. brongniartii and matrine was recorded after 45 days of treatment. The rate of emergence (%) was calculated by following equation.
Percent adult emergence = (Number of adults/Total number of pupae) × 100.
Enzyme Analysis
Newly molted fourth instar larvae of S. litura were fed on a fresh artificial diet treated with a combined matrine and fungal conidia suspension as shown in Table 2. For control larvae, the worms were fed with artificial diet without the addition of fungal conidia or matrine. The treated larvae were placed in plastic cups followed by incubation at 26 ± 2°C and 65 ± 5% r.h. Following 3, 5, and 7 days, the samples (larvae) were collected and dissected to get access to their fat bodies and hemolymph. Each treatment consisted of three replicates and each replicate consisted of 10 larvae.
Fat body homogenates for enzyme assays were prepared by dissolving 0.6 g of fat bodies in 1.0 ml of specific buffers for each enzyme [0.05 mol/L Tris-HCL (pH 7.5) for GSTs assay; 0.02 mol/L PBS (pH 7.0) for AChE assay; 0.05 mol/L PBS (pH 7.8) for SOD assay; and 0.15 mol/L PBS (pH 7.0) for CAT assay; and 0.2 mol/L PBS (pH = 6.0) for POD assay] at 4°C followed by grinding with glass homogenizer on ice bath. The homogenates were centrifuged at 11,000 rpm for 10 min at 4°C, and the supernatant was taken as an enzyme source.
The hemolymph was sampled with a glass capillary through an incision in the cuticle and placed in cooled tubes to which 4 mg/ml phenylthiourea was added to prevent melanization. The hemolymph was centrifuged at 4°C for 10 min at 11,000 rpm, and the cell-free plasma fraction was used to determine the enzyme activity.
The GSTs were conducted using the procedures developed by Habig (1981). Incubation was carried out at 25°C for 5 min in 0.1 M Na-phosphate buffer (pH 6.5) containing 1 mM glutathione, 1 mM DNCB, and 20 μl of the sample. The reaction was initiated by adding DNCB solution in acetone. Concentration of 5-(2,4-dinitrophenyl) glutathione produced during the reaction was measured spectrophotometrically at the wavelength of 340 nm. One unit of enzyme will conjugate 10.0 nmol of CDNB with reduced glutathione per minute.
AChE activity was assayed by following Ellman et al. (1961) with some slight modifications. The reaction mixture contained 50 μl sample solution, 100 μl 45 μM 5-5-dithiobis-(2-nitrobenzoic acid), 100 μl acetylthiocholine iodide, and 90 μl sodium phosphate buffer. The change in absorbance at 405 nm was recorded for 40 min. One milliunit of AChE activity is the amount of enzyme that will generate 1.0 nmol of choline per minute.
SOD activity was measured in cell free extracts by nitro blue tetrazolium (NBT) reduction (Beauchamp and Fridovich, 1971). The assay mixture contained 0.2 ml 0.1M ethylene diamine tetraacetic acid (EDTA) containing 0.3 mM sodium cyanide (0.2 ml), 0.1 ml 1.5 mM nitroblue tetrazolium (NBT), 3 ml 0.067M potassium phosphate buffer, pH 7.8, and a series of samples ranging from 0.1 to 10 mg protein in different tubes. The tubes were placed in a light box providing uniform light intensity. The tubes were incubated for 5–8 min to achieve a standard temperature. At zero time, 0.05 ml 0.12 mM riboflavin was added and all the tubes were incubated for 12 min and absorbance was read at 560 nm after 1 min interval. One unit of SOD activity was defined as the amount of SOD required for inhibition of the reduction of NBT by 50% (A560) and was expressed as units per mg protein (U/mg protein).
CAT activity was assayed by the method described by Beers and Sizer (1952), in which the decomposition of H2O2 was analyzed spectrophotometrically at 240 nm. Reagent grade water (1.9 ml) and 0.059M hydrogen peroxide (1.0 ml) were pipetted into the cuvette. The cuvette was incubated in spectrophotometer for 4–5 min to achieve temperature equilibration and to establish blank rate if any. After 5 min, 0.1 ml of sample was added and change in absorbance at 240 nm was observed for 2–3 min. Change in absorbance per minute was calculated from the initial (45 s) linear portion of curve. One unit of CAT activity was defined as the amount of enzyme that decomposes 1 mmol H2O2/min at an initial H2O2 concentration of 30 mM at pH 7.0 and 25°C and was expressed as U/mg protein.
POD activity assay was performed by following Shannon et al. (1966). Briefly, 3.00 ml of reaction mixture having 2.1 ml H2O, 0.32 ml 14 mM potassium phosphate buffer, 0.16 ml 0.027% (v/v) hydrogen peroxide, and 0.32 ml 0.5% (w/v) pyrogallol was incubated 20°C for 10 min. The 0.1 ml of 14 mM potassium phosphate buffer and 0.1 ml of sample was added and followed by mixing through inversion. Change in absorbance was measured spectrophotometrically at the wavelength of 420 nm. Enzyme activity was expressed as U/mg protein.
The protein concentrations in the supernatants were determined by following the method of Bradford (1976) using bovine serum albumin as standard.
Data Analysis
Data were subjected to one-way ANOVA and mean values were compared using Tukey’s HSD test (p < 0.05). All data analysis was performed using SPSS 19.0 software.
Results
Effect on the Feeding and Food Utilization Efficiency of Spodoptera litura
After feeding the S. litura larvae on the treated diets, nutritional analysis results showed significant differences in RGRs of S. litura in response to individual or combined treatment of B. brongniartii and matrine after 7 days (F8,261 = 45.77; p < 0.01), 15 days (F8,261 = 38.04; p < 0.01), and 21 days (F8,261 = 54.89; p < 0.01). The results of the RGR are shown in Table 3. Individual RGR values of S. litura larvae in combined treatments of B. brongniartii and matrine were significantly lower than those observed for individual treatments of B. brongniartii or matrine at different time intervals. The lowest RGR value of 0.05 ± 0.004 g/(g/day) was observed for T9 at different time intervals. Highest RGR values were observed for T1 (control) having mean values of 16.17 ± 2.37, 19.26 ± 0.54, and 23.93 ± 0.12 g/(g/day) after 7, 14 and 21 days of treatment, respectively. The RGR values observed for different combined treatments of B. brongniartii and matrine (T6–T8) were statistically at par with each other after 7, 14 and 21 days of treatment.
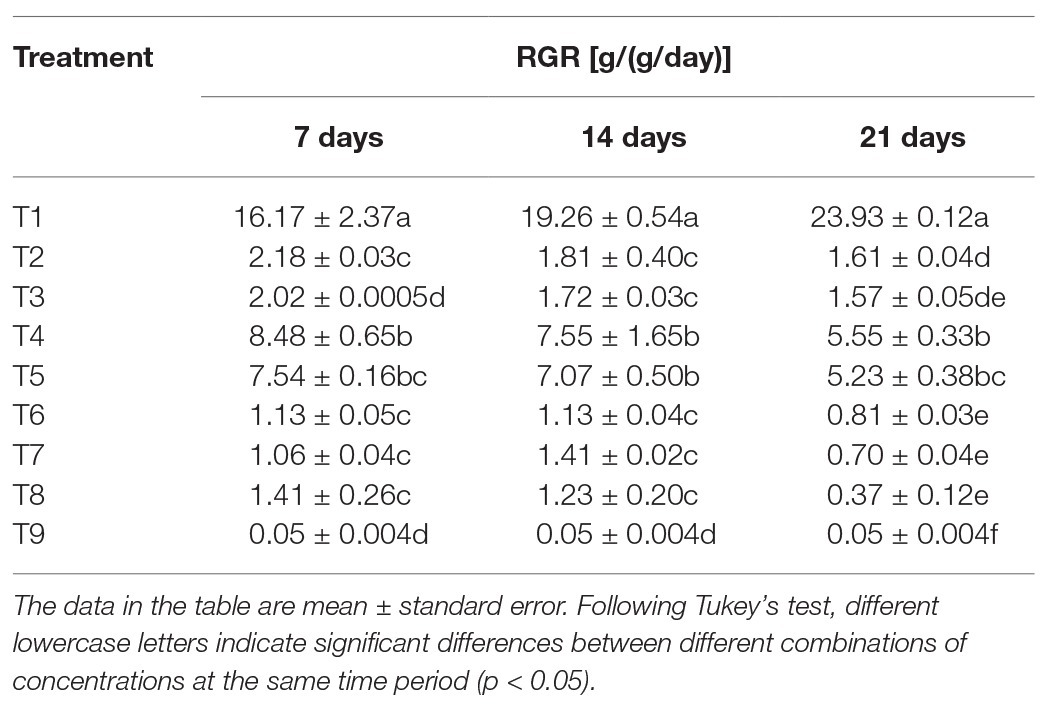
Table 3. Effect of Beauveria brongniartii and matrine on relative growth rate (RGR) of Spodoptera litura.
The analysis of RCR of S. litura in response to individual or combined treatment of B. brongniartii and matrine revealed significant differences after 7 days (F8,261 = 39.67; p < 0.01), 15 days (F8,261 = 41.23; p < 0.01), and 21 days (F8,261 = 69.51; p < 0.01) of application as shown in Table 4. Individual RCR values of S. litura larvae in combined treatments of B. brongniartii and matrine were significantly lower than those observed for individual treatments of B. brongniartii or matrine at different time intervals. The RCR values observed for different combined treatments of B. brongniartii and matrine (T7–T9) were statistically at par with each other after 7, 14, and 21 days of treatment. The lowest RCR values (0.82 ± 0.05, 1.00 ± 0.05, and 0.69 ± 0.05 g/(g/day) after 7, 14, and 21 days of treatment, respectively) were observed for T9 at different time intervals. Highest RCR values were observed for T1 (control) having mean values of 1.88 ± 0.02, 2.07 ± 0.05, and 2.29 ± 0.07 g/(g/day) after 7, 14, and 21 days of treatment, respectively. On the 7th day after treatment, the RCR value of S. litura in the T2 treatment was not significantly different from T1 (control). However, the other treatments were significantly lower than the control. Following 14 days after treatment, there was no significant difference among T1 (control), T2, and T3 treatments. However, the other treatments were significantly lower than T1 (control). On 21 days after treatment, the RCR values of treatment groups were significantly lower than the control group.
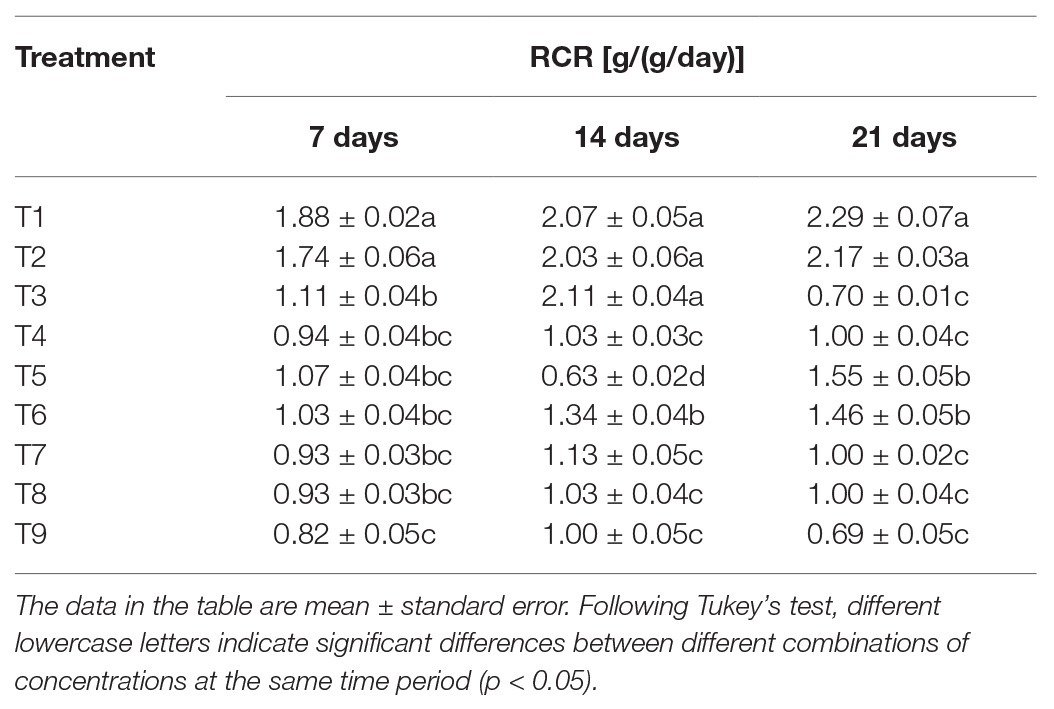
Table 4. Effect of Beauveria brongniartii and matrine on relative consumption rate (RCR) of Spodoptera litura.
The analysis of the ECI of S. litura in response to individual or combined treatment of B. brongniartii and matrine revealed significant differences after 7 days (F8,261 = 43.23; p < 0.01), 15 days (F8,261 = 45.89; p < 0.01), and 21 days (F8,261 = 63.31; p < 0.01) of application as shown in Table 5. Individual ECI values of S. litura larvae in combined treatments of B. brongniartii and matrine were significantly lower than those observed for individual treatments of B. brongniartii or matrine at different time intervals. The RCR values observed for different combined treatments of B. brongniartii and matrine (T6–T8) were statistically at par with each other after 14 and 21 days of treatment. The lowest ECI values (2.19 ± 0.01, 1.91 ± 0.01, and 1.23 ± 0.01% after 7, 14, and 21 days of treatment, respectively) were observed for T9. Highest ECI values were observed for T1 (control) having mean values of 65.47 ± 7.70, 34.02 ± 4.40, and 13.04 ± 0.61% after 7, 14, and 21 days of treatment, respectively. At three different time points, the ECI values of S. litura in the T4 treatment were not significantly different from that of the blank control (T1).
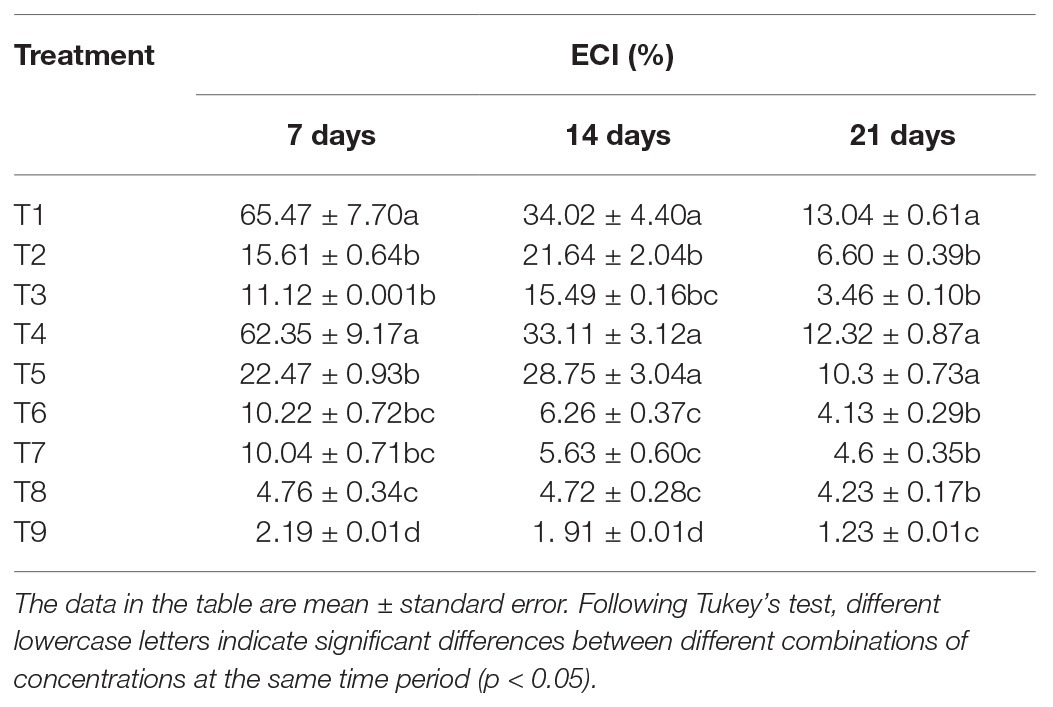
Table 5. Effects of Beauveria brongniartii and matrine on efficiency of conversion (ECI) of ingested food of Spodoptera litura.
Effects of Beauveria brongniartii and Matrine on Pupation and Emergence Rates in Spodoptera litura
Percent pupation of S. litura larvae treated with individual or combined treatment of B. brongniartii and matrine differed significant among different treatments after 30 days of application (F8,261 = 31.07; p < 0.01). The effects of B. brongniartii and matrine on the pupation rate of are shown in Figure 1. The pupation rates of S. litura treated with B. brongniartii SB010 and matrine were significantly lower than the control (T1). When feed with combinations of SB010 and matrine (0.5 and 1.0 mg/L), the pupation rates were zero, except those in T6.
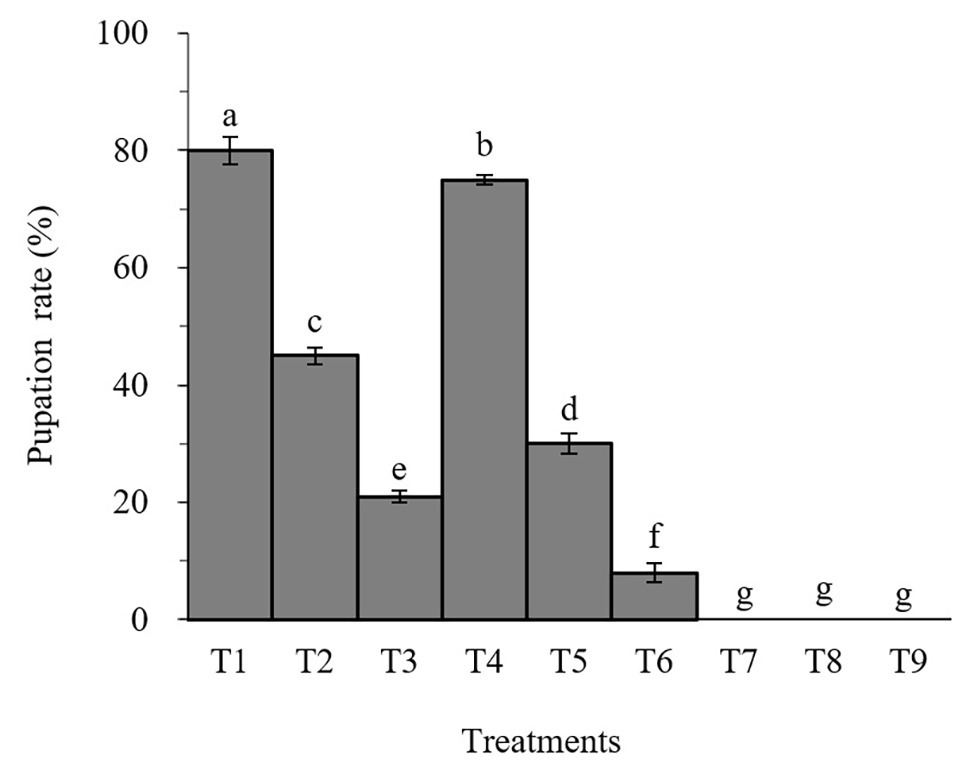
Figure 1. Effect of Beauveria brongniartii and matrine on the pupation rate of Spodoptera litura. Bars having different letters show significant differences between treatments. T1 = Control; T2 = Matrine 0.5 mg/L; T3 = Matrine 1.0 mg/L; T4 = B. brongniartii 1 × 108 conidia/ml; T5 = B. brongniartii 1 × 108 conidia/ml; T6 = B. brongniartii 1 × 108 conidia/ml + Matrine 0.5 mg/L; T7 = T6 = B. brongniartii 1 × 108 conidia/ml + Matrine 1.0 mg/L; T8 = B. brongniartii 1 × 109 conidia/ml + Matrine 0.5 mg/L; and T9 = B. brongniartii 1 × 109 conidia/ml + Matrine 1.0 mg/L.
Percent pupation of S. litura larvae treated with individual or combined treatment of B. brongniartii and matrine differed significant among different treatments after 45 days of application (F8,261 = 26.83; p < 0.01). As can be seen in Figure 2, the emergence rates of adult S. litura fed with B. brongniartii were significantly reduced compared to the control (T1). Also, the higher the concentration of fungal conidia and matrine, the higher the reduction in emergence rate. The results show that combined treatments of B. brongniartii and matrine can dramatically reduce the rate of emergence of S. litura to zero.
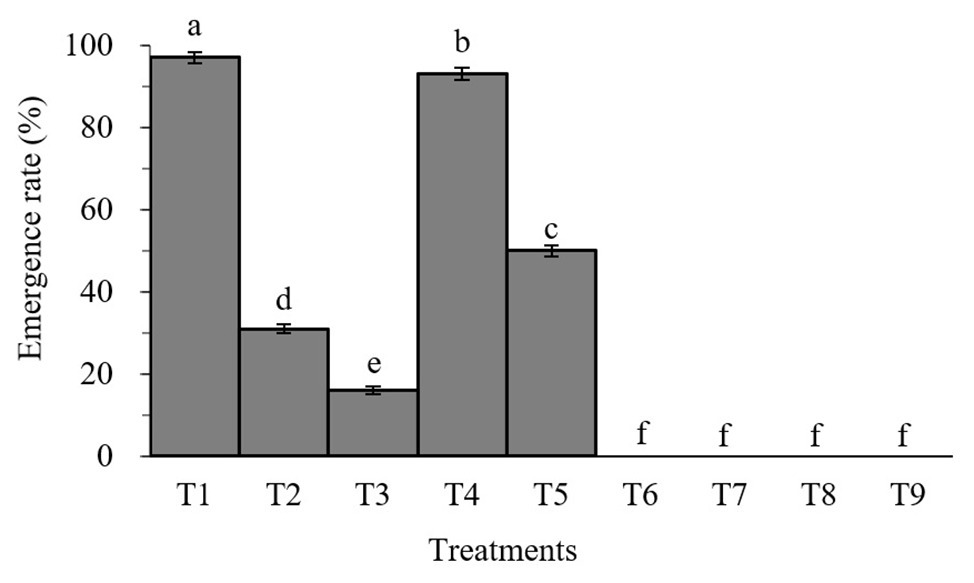
Figure 2. Effect of Beauveria brongniartii and matrine on the emergence rate of Spodoptera litura. Bars having different letters show significant differences between treatments. T1 = Control; T2 = Matrine 0.5 mg/L; T3 = Matrine 1.0 mg/L; T4 = B. brongniartii 1 × 108 conidia/ml; T5 = B. brongniartii 1 × 108 conidia/ml; T6 = B. brongniartii 1 × 108 conidia/ml + Matrine 0.5 mg/L; T7 = B. brongniartii 1 × 108 conidia/ml + Matrine 1.0 mg/L; T8 = B. brongniartii 1 × 109 conidia/ml + Matrine 0.5 mg/L; and T9 = B. brongniartii 1 × 109 conidia/ml + Matrine 1.0 mg/L.
Effects of Beauveria brongniartii and Matrine on the Enzyme Activities in Spodoptera litura
After exposure of the fourth instar larvae to the treated diet, GST activity was also affected both in the fat body and in the hemolymph (Figure 3). In the fat body, the highest activity was found on the 3rd day following treatment by matrine and B. brongniartii. The lowest activity was found on the 7th day after being treated by SB010 (Figure 3A). In the hemolymph (Figure 3B), GST activity showed a decreasing trend and was significantly lower than the control in all cases except on the 5th day when treated by B. brongniartii.
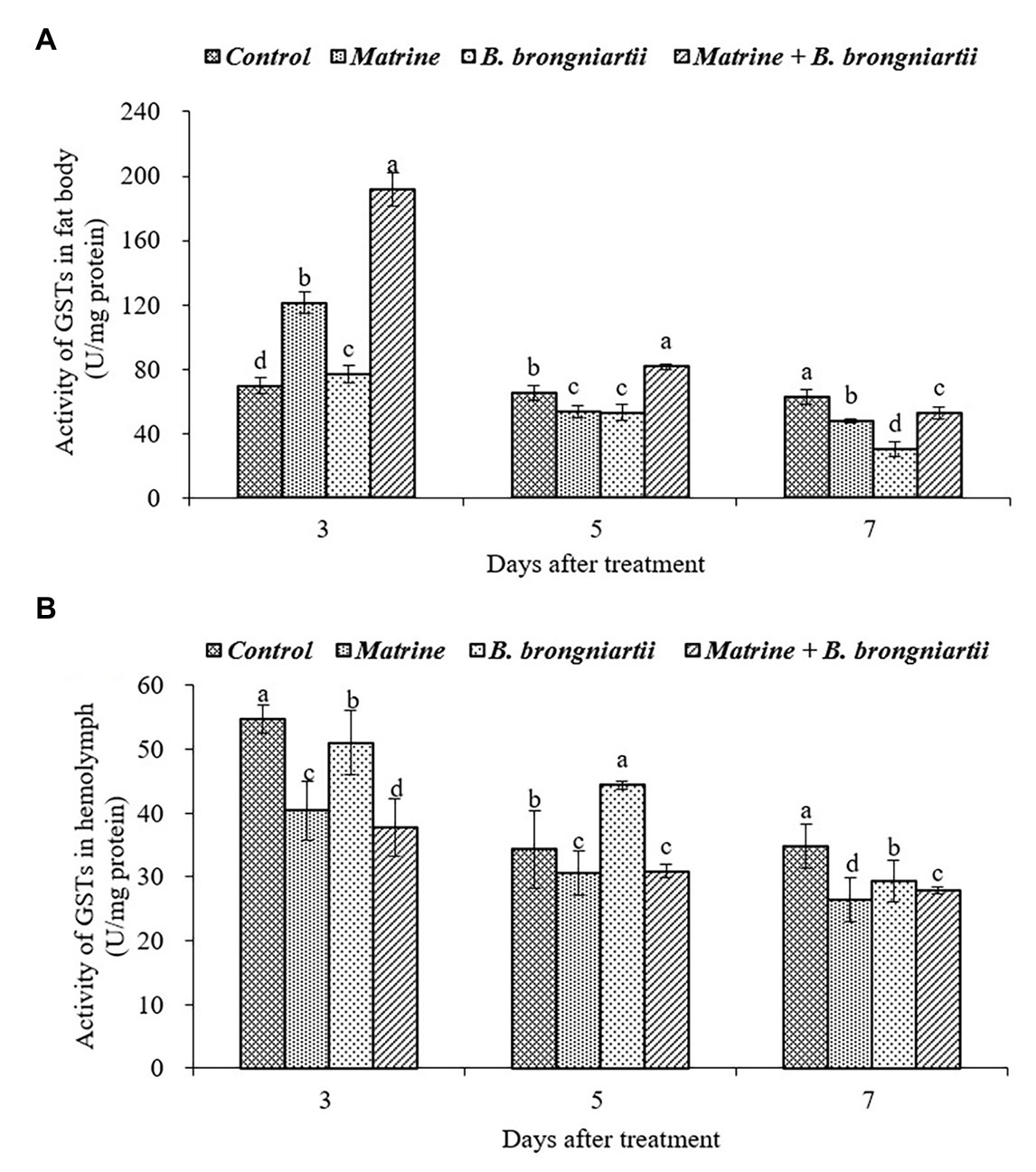
Figure 3. Changes in glutathione-S-transferase (GST) activity of Spodoptera litura following treatment with Beauveria brongniartii and matrine (A) in the fat body and (B) in hemolymph. Bars having different letters show significant differences between treatments at different time intervals.
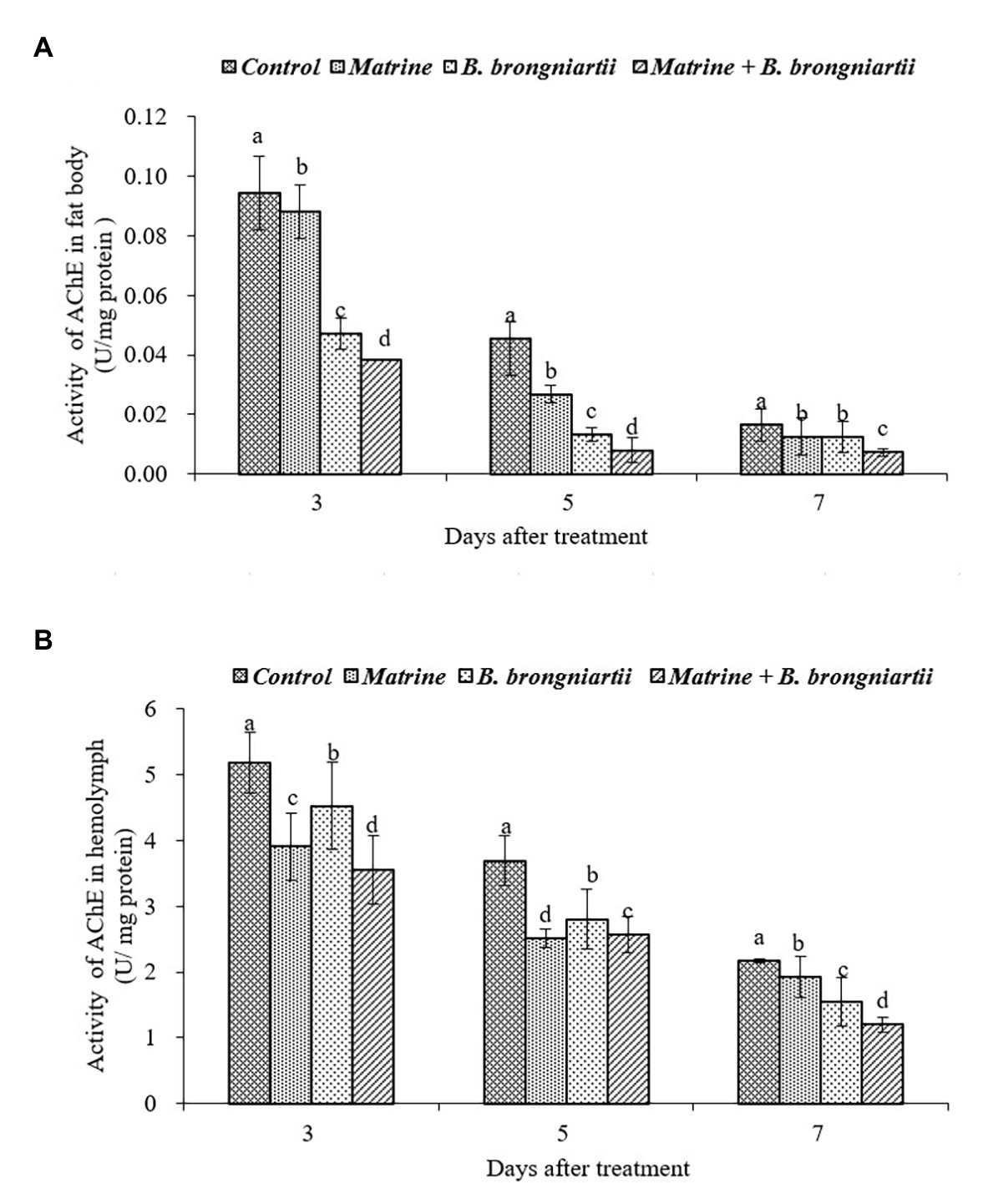
Figure 4. Changes in acetylcholinesterase (AChE) activity of Spodoptera litura after treatment with Beauveria brongniartii and matrine (A) in the fat body and (B) in hemolymph. Bars having different letters show significant differences between treatments at different time intervals.
The AChE activities in the fat body and in the hemolymph of S. litura showed the same trend (Figure 4). The results indicated that AChE activities decreased as time went by. The lowest activity in both the fat body and hemolymph was noted on the 7th day after feeding matrine and B. brongniartii. Activities of all treatments were significantly lower than the control.
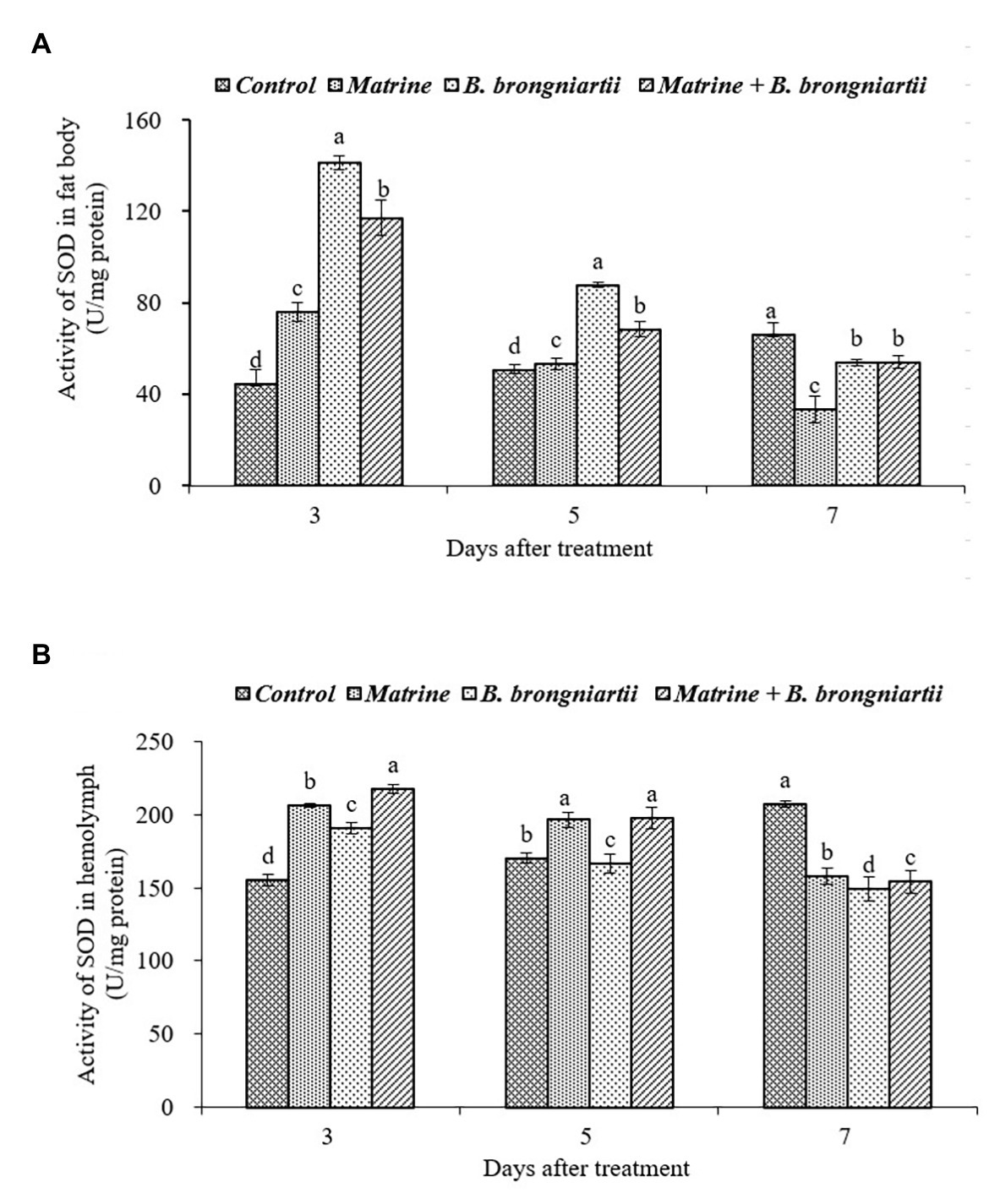
Figure 5. Changes in superoxide dismutase (SOD) activity of Spodoptera litura after treatment with Beauveria brongniartii and matrine (A) in the fat body and (B) in hemolymph. Bars having different letters show significant differences between treatments at different time intervals.
Following exposure of the fourth instar larvae of S. litura to treated diets with B. brongniartii and matrine, the enzyme activity of SOD differed significantly after 3, 5, and 7 days of feeding both in the fat body and in the hemolymph when compared to the control (Figure 5). The results showed that the activity of SOD following treatments was significantly different from that of the control. On days 3 and 5 after treatment, the activity of SOD in the fat body was significantly higher than that of the control (Figure 5A). The SOD activity then decreased significantly on day 7 compared to the control. The SOD activity in the hemolymph of the control showed an upward trend (Figure 5B). However, the values under different treatments decreased and showed a minimum activity 7 days post-treatment.
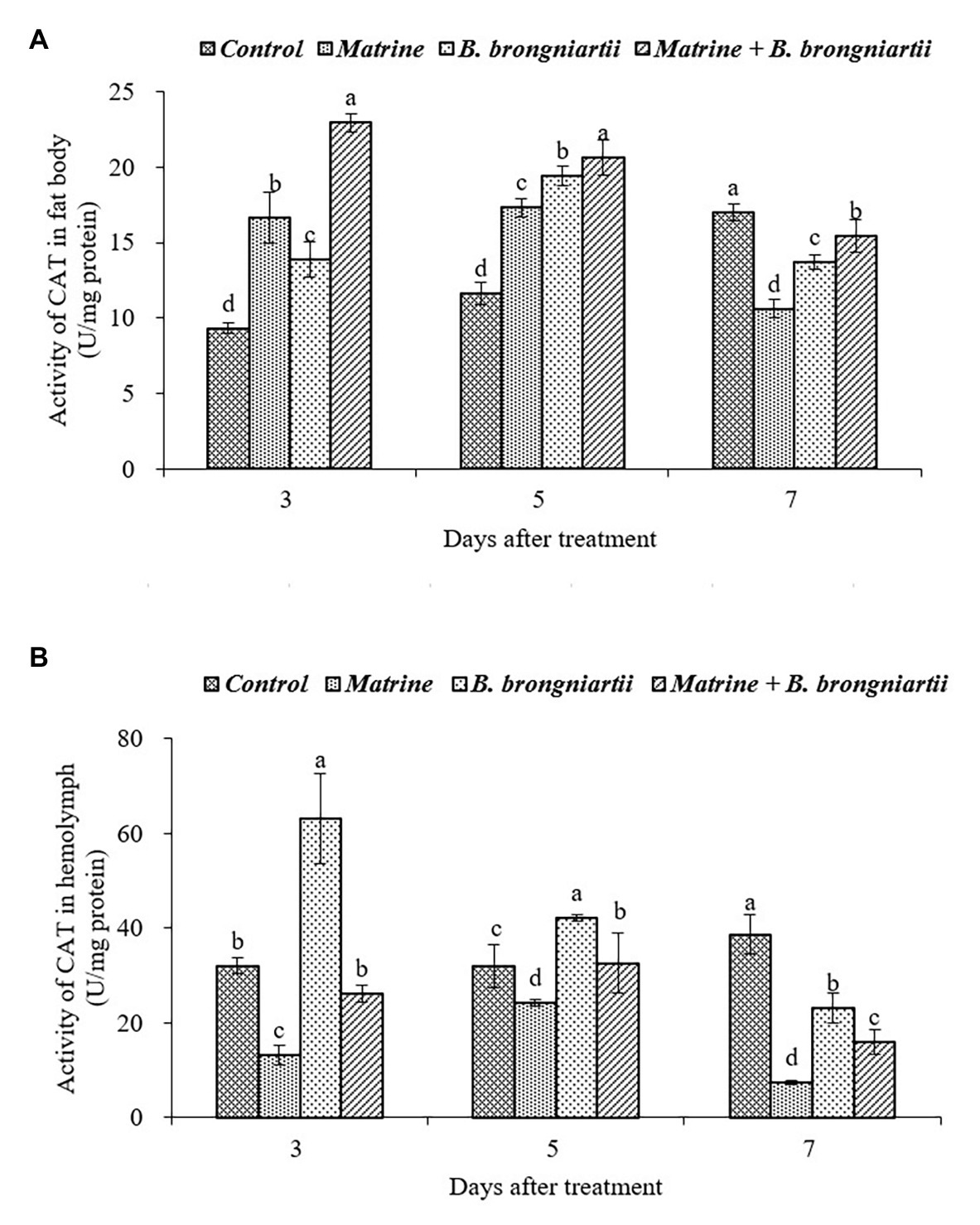
Figure 6. Changes in catalase (CAT) activity of Spodoptera litura following treatment with Beauveria brongniartii and matrine (A) in the fat body and (B) in hemolymph. Bars having different letters show significant differences between treatments at different time intervals.
As can be seen in Figure 6, feeding B. brongniartii and matrine could significantly affect the activity of CAT. On the 3rd and 5th days after treatment, CAT activity in the fat body showed an increasing trend and was significantly higher than the control after treatment by matrine, B. brongniartii and their combination. In comparison, the CAT activity decreased and was significantly lower in the control (Figure 6A). In the hemolymph (Figure 6B), only the CAT activity of S. litura feeding with matrine increased on the 3rd and 5th days following treatment. On the 7th day after treatment, CAT activity showed a decrease both in the fat body and in the hemolymph.
The exposure of the fourth instar larvae to the treated diet also resulted in varied values of POD both in the fat body and in the hemolymph (Figure 7). In the fat body, the highest activity was found on the 5th day after treatment by matrine and the lowest activity was noted on the 7th day after combined treatment by B. brongniartii and matrine. The values decreased gradually and became lowest in all treatments on the 7th day following treatment (Figure 7A). In the hemolymph, POD activity also showed a decreasing trend. On the 3rd and 5th days after treatment, POD activity in the hemolymph decreased when feed B. brongniartii and matrine separately, which was significantly lower than the combined treatment (Figure 7B).
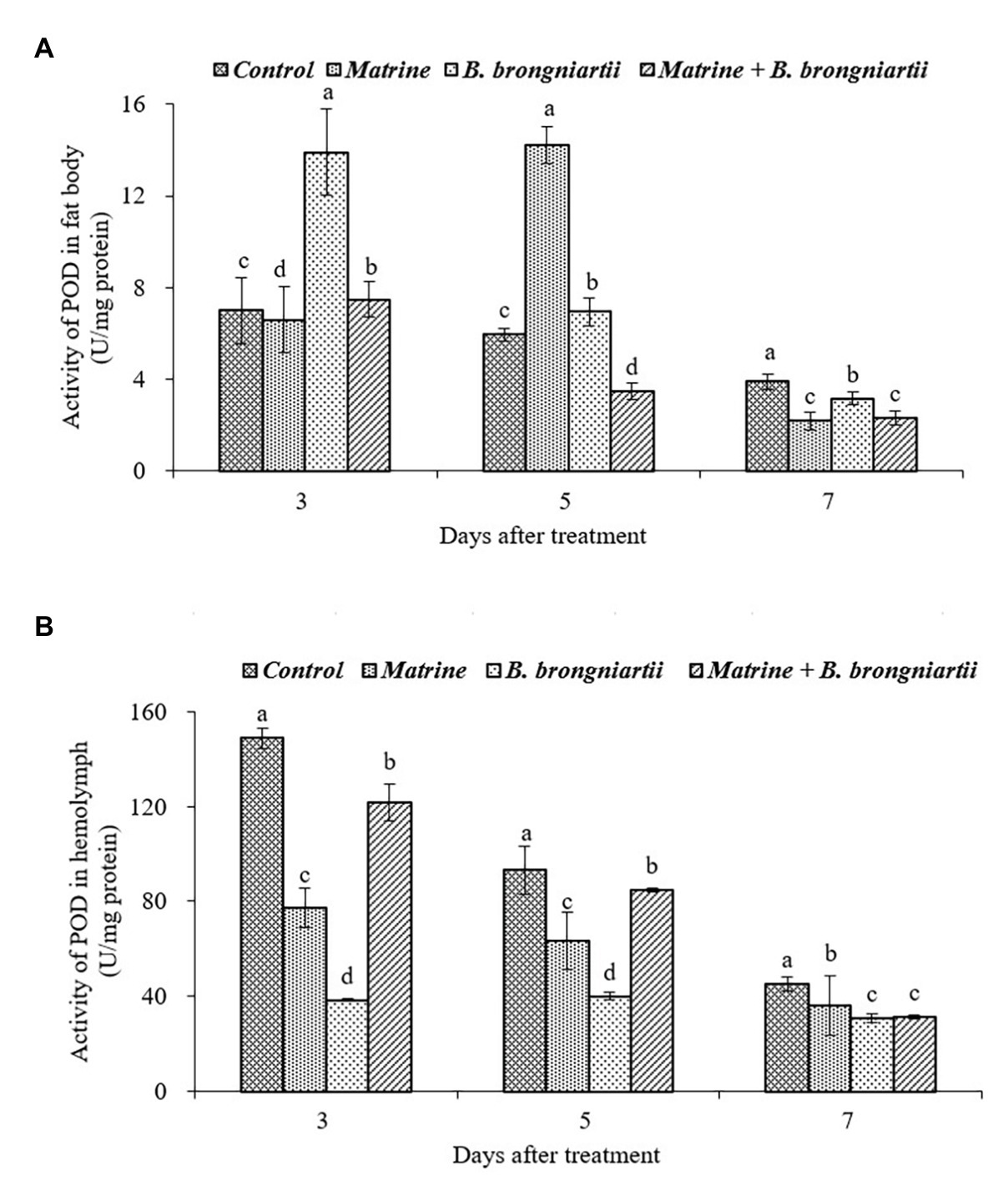
Figure 7. Changes in peroxidase (POD) activity of Spodoptera litura following treatment with Beauveria brongniartii and matrine (A) in the fat body and (B) in hemolymph. Bars having different letters show significant differences between treatments at different time intervals.
Discussion
Development of integrated control programs against S.litura involving B. brongniartii and chemical pesticides requires a clear understanding concerning the effects of chemicals on the physiology and pathogenicity of entomopathogenic fungi. Previous studies on B. brongniartii have only explained the pathogenic ability as well as lethal and sub lethal effects of this fungus against different insect pests (Fan et al., 2013; Goble et al., 2015; Mayerhofer et al., 2015; Soni et al., 2017). However, very few studies have elaborated the possible compatibility of B. brongniartii with natural chemicals (derived from plant or any other living organism) and other chemicals with novel mode of action (Sushil et al., 2018; Wu et al., 2019). Our previous findings revealed the synergistic interaction of B. brongniartii with matrine (plant derived chemical) against S. litura under laboratory as well as semi field conditions (Wu et al., 2019). This study reports the biological impacts of B. brongniartii and matrine on nutritional efficiency indices and detoxification enzymes (GST, AChE, SOD, CAT, and POD) in S. litura.
In the present study, the individual or joint applications of B. brongniartii and matrine caused reduction in nutritional efficiency indices (RGR, RCR, and ECI) of S. litura when compared with control although the nutritional efficiency indices (RGR, RCR, and ECI) observed for individual treatments of B. brongniartii and matrine were higher than those observed for joint applications of B. brongniartii and matrine. These results are in line with the findings of Rizwan-ul-Haq et al. (2009) who observed similar changes in RGR, RCR, and ECI of S. exigua in response to individual or joint treatments of ricinine (a botanical extract) and Bt (microbial pesticide). The reduction in RGR, RCR, and ECI of S. litura in response to B. brongniartii application alone or in combination with matrine can also be related to the degradation of insect gut peritrophic membrane by chitinase. Brandt et al. (1978) proposed that chitinases cause perforations in the membranes, thus facilitating entry of the pathogens into the tissues of susceptible insects. The addition of exogenous chitinase from Streptomyces griseus to the blood meal of the mosquito, Anopheles freeborni, prevented the peritrophic membrane from forming (Shahabuddin et al., 1993). Perforation of peritrophic membranes occurred in vivo after Spodoptera fifth instar larvae were fed on a diet containing recombinant ChiAII, a recombinant endochitinase encoded by Serratia marcescens (Regev et al., 1996).
Lower RGR, RCR, and ECI probably lead to a delay in larval growth, and the resulting formation of smaller pupa will have a direct relation to fecundity and longevity of the adult insect and even make them more susceptible to diseases and natural enemies (Khosravi et al., 2010). The rates of successful pupation as well as adult emergence of S. litura observed during this study were 0 when treated with B. brongniartii SB010 and 1.0 mg/ml matrine solution. Farooq and Freed (2016) studied the effect of a mixture of B. bassiana and six different insecticides, such as acetamiprid, methylamino avermectin, imidacloprid, and lufenuron, on the mortality of Musca domestica. Their results revealed that the lifespan, fecundity, egg hatching rate, percentage of pupation, pupal weight, and adult emergence rate of houseflies were significantly reduced, while the larval and pupal stages were prolonged.
GST is one of the main enzymes responsible for detoxification of insecticides or pathogens by insects (Claudianoc et al., 2006). Our findings showed an increase in GSTs activities during the initial 3 days following matrine treatment. This increase in enzyme activity in response shows the possible involvement of GSTs enzymes in the insecticide detoxification mechanism of S.litura (Jia et al., 2016). Luo and Zhang (2003) also observed similar fluctuation of GSTs in Plutella xylostella in response to matrine treatment. Although few reports are available on changes in GSTs activity following matrine treatment, the extent of changes can vary with change in the insect species targeted and the concentration of matrine used. In this study, we found that GSTs increased during initial periods following B. brongniartii treatment, which is similar to the findings of Ali et al. (2017) who also observed similar changes in GSTs activity of B. tabaci following Lecanicillium muscarium applications. They observed increases in activity of the said enzymes until 96 h post fungal application after which enzyme activities were restrained leading to metabolic imbalance and insect death. The treatment of S.litura with a combination of matrine and B. brongniartii resulted in a significant reduction of GSTs activities throughout the experimental period post 72 h of application. These results are in line with the findings of Ali et al. (2017) who have shown a similar reduction of enzyme activity in B. tabaci following joint application of matrine and L. muscarium. The changes in activities of detoxifying enzymes explained above can make the target pest more susceptible to fungal infection (Hall, 1963). The reduction in GSTs activities in response to joint application of matrine and B. brongniartii can be related to the sequence of chemical’s/pathogen’s action against B. tabaci. Insecticides normally act as a stressor increasing the susceptibility of a target pest to an entomopathogenic fungus (Furlong and Groden, 2001).
The enzyme AchE rapidly terminates nerve impulses by catalyzing the hydrolysis of the neurotransmitter, Ach at peripheral and central synapses of the insects’ nervous system (Bourne et al., 2016). AchE is also an important target site for insecticide action in the central nervous system of insects (Nathan et al., 2008). During this study, AchE activities of S. litura decreased throughout the experimental period following treatment with matrine and B. brongniartii alone or in combination. These results are in line with the findings of Ali et al. (2017) who have shown a similar reduction of AchE activities in B. tabaci following individual or joint application of matrine and L. muscarium. The decrease in AchE activities can be related to the mode of action of both agents. Matrine, an alkaloid extracted from S. flavescens is known to target insect Ach receptors which in turn effects AchE production (Liu et al., 2008). Luo et al. (1997) observed similar inhibition of AchE activity in turnip aphid (L. erysimi) following matrine application. The reduction in AchE activity of S. litura following B. brongniartii application is in line with the findings of Zibaee et al. (2009) who observed similar inhibition of AchE activity when Beauveria bassiana and its secondary metabolites were applied against sunn pest (Eurygaster integriceps). These changes in AchE activity of S. litura can be related to the production of a secondary metabolite named bassianolide by different species of Beauveria. Bassianolide can inhibit Ach receptors of insect muscles reducing the production of AchE (Xu et al., 2009).
Antioxidant enzymes (SOD, CAT, and POD) are also known as protective cellular system because SOD is known to increase the production of H2O2 from O2 through dismutation, while CAT and POD can catalyze H2O production from H2O2. These reactions can reduce the bio-membrane damage through reactive oxygen species (ROS; Ding et al., 2001). In our study, the activities of antioxidant enzymes (SOD, CAT, and POD) increased during the initial 3 days followed by a decrease when treated with B. brongniartii alone or in combination with matrine. The decreased activities of antioxidant enzymes (SOD, CAT, and POD) during the later experimental period can result in a reduced elimination of ROS which in turn can denature different biomolecules of the insect body. The denaturation of biomolecules can stop all the cellular processes, so leading to the death of the insect (Felton and Summers, 1995).
Concluding Remarks
In summary, our results showed a high antifeedant activity of B. brongniartii and matrine on S. litura. The results suggest that combined treatments of matrine and B. brongniartii cause significant reduction in pupation (%) and adult emergence (%) of S. litura. The enzymatic response of S. litura to combined matrine and B. brongniartii treatment displayed a reduction in GSTs, SOD, POD, and CAT after 3 days of infection. The changes in enzymatic activities suggest that the probability of B. brongniartii infection was increased by matrine and B. brongniartii which in turn enervated the insect defense against matrine. The chemical basis of this strong synergistic effect is possibly related to the disturbance of the Ach balance and changes in AchE activities of the S. litura as both matrine and B. brongniartii can target insect Ach receptors which in turn effects AchE production. Therefore, our results have revealed the complex biochemical processes involved in the synergistic action of matrine and B. brongniartii against S. litura. These findings can provide baseline information to explain the complex biochemical processes involved in the synergistic action of matrine and B. brongniartii against S. litura.
Data Availability Statement
The raw data supporting the conclusions of this article will be made available by the authors, without undue reservation.
Author Contributions
JhW and SA conceived and designed the research. CZ and XtY conducted the experiments and performed the data analysis. CZ, JyL, and SA wrote the manuscript. AGSC revised the manuscript. All authors read and approved the manuscript.
Funding
This research was funded by grants from the Science and Technology Program of Guangzhou, China (201807010019) and Key Area Research and Development Program of Guangdong Province (2018B020205003).
Conflict of Interest
The authors declare that the research was conducted in the absence of any commercial or financial relationships that could be construed as a potential conflict of interest.
Acknowledgments
We thank the handling editor and reviewers for their constructive comments and suggestions.
References
Ahmad, M., and Mehmood, R. (2015). Monitoring of resistance to new chemistry insecticides in Spodoptera litura (Lepidoptera, Noctuidae) in Pakistan. J. Econ. Entomol. 108, 1279–1288. doi: 10.1093/jee/tov085
Ali, S., Zhang, C., Wang, Z. Q., Wang, X. M., Wu, J. H., Cuthbertson, A. G. S., et al. (2017). Toxicological and biochemical basis of synergism between the entomopathogenic fungus Lecanicillium muscarium and the insecticide matrine against Bemisia tabaci (Gennadius). Sci. Rep. 7:46558. doi: 10.1038/srep46558
Beauchamp, C., and Fridovich, I. (1971). Superoxide dismutase: improved assays and an assay applicable to acrylamide gels. Anal. Biochem. 44, 276–287.
Beers, R. F., and Sizer, I. W. (1952). A spectrophotometric method for measuring the breakdown of hydrogen peroxide by catalase. J. Biol. Chem. 195, 133–140.
Borja, M. R., Franco, A. W. G., Leyva, E. R., Ortega, C. S., and Panduro, A. P. (2018). Interaction of Beauveria bassiana and Metarhizium anisopliae with chlorpyrifos ethyl and spinosid in Spodoptera frugiperda larvae. Pest Manag. Sci. 74, 2047–2052. doi: 10.1002/ps.4884
Bradford, M. M. (1976). A rapid and sensitive method for the quantitation of microgram quantities of protein utilizing the principle of proteindye binding. Anal. Biochem. 72, 248–254. doi: 10.1016/0003-2697(76)90527-3
Brandt, C. R., Adang, M. J., and Spence, K. D. (1978). The peritrophic membrane: ultrastructural analysis and function as a mechanical barrier to microbial infection in Orgyia pseudotsugata. J. Invertebr. Pathol. 32, 12–24.
Bourne, Y., Sharpless, K. B., Taylor, P., and Marchot, P. (2016). Steric and dynamic parameters influencing in situ cyclo additions to form triazole inhibitors with crystalline acetylcholinestrase. J. Amer. Chem. Soc. 138, 1611–1621. doi: 10.1021/jacs.5b11384
Campa-Córdova, A. I., Hernández-Saavedra, N. Y., and Ascencio, F. (2002). Superoxide dismutase as modulator of immune function in American white shrimp (Litopenaeus vannamei). Comp. Biochem. Physiol. C Pharmacol. Toxicol. Endocrinol. 133, 557–565. doi: 10.1016/s1532-0456(02)00125-4
Cerenius, L., and Söderhäll, K. (2004). The prophenoloxidase-activating system in invertebrates. Immunol. Rev. 198, 116–126. doi: 10.1111/j.0105-2896.2004.00116.x
Claudianoc, C., Ranson, H., Johnson, R. M., Biswas, S., Schuler, M. A., Berenbaum, M. R., et al. (2006). A deficit of detoxification enzymes: pesticide sensitivity and environmental response in the honeybee. Insect Mol. Biol. 15, 615–636. doi: 10.1111/j.1365-2583.2006.00672.x
Chaudhari, C. S., Chandele, A. G., Pokharkar, D. S., Dethe, M. D., and Firake, D. M. (2016). Pathogenicity of different isolates of entomopathogenic fungus, Nomuraea rileyi (Farlow) Samson against tobacco caterpillar, Spodoptera litura (Fabricius). Proc. Natl. Acad. Sci. India Sect. B Biol. Sci. 86, 1001–1007. doi: 10.1007/s40011-015-0537-6
Cheng, X., Ye, J., He, H., Liu, Z., Xu, C., Wu, B., et al. (2018). Synthesis, characterization and in vitro biological evaluation of two matrine derivatives. Sci. Rep. 8:15686. doi: 10.1038/s41598-018-33908-8
Cuthbertson, A. G. S., and Murchie, A. K. (2005). European red spider mite—an environmental consequence of persistent chemical pesticide application. Int. J. Environ. Sci. Technol. 2, 287–290. doi: 10.1007/BF03325888
David, W. A. L., Ellaby, S., and Taylor, G. (1975). Rearing Spodoptera exempta on semi-synthetic diets and on growing maize. Entomol. Exp. Appl. 19, 226–236. doi: 10.1111/j.1570-7458.1975.tb02374.x
Dhar, S., Jindal, V., Jaryal, M., and Gupta, V. K. (2019). Molecular characterization of new isolates of the entomopathogenic fungus Beauveria bassiana and their efficacy against the tobacco caterpillar, Spodoptera litura (Fabricius) (Lepidoptera: Noctuidae). Egypt. J. Biol. Pest Control 29:8. doi: 10.1186/s41938-019-0110-3
Ding, S. Y., Li, H. Y., Li, X. F., and Zhang, Z. Y. (2001). Effects of two kinds of transgenic poplar on protective enzymes system in the midgut of larvae of American white moth. J. For. Res. Jpn. 12, 119–122. doi: 10.1007/BF02867209
Dubovskiy, I. M., Slyamova, N. D., Kryukov, V. Y., Yaroslavtseva, O. N., Levchenko, M. V., Belgibaeva, A. B., et al. (2012). The activity of nonspecific esterases and glutathione-S-transferase in Locusta migratoria larvae infected with the fungus Metarhizium anisopliae (Ascomycota, Hypocreales). Entomol. Rev. 92, 27–31. doi: 10.1134/S0013873812010022
Ellman, G. L., Coutney, K. D., Andre, V., and Featherstone, R. M. (1961). A new and rapid calorimetric determination of acetylcholinestrase activity. Biochem. Pharmacol. 7, 88–95. doi: 10.1016/0006-2952(61)90145-9
Enayati, A. A., Ranson, H., and Hemingway, J. (2005). Insect glutathione transferases and insecticide resistance. Insect Mol. Biol. 14, 3–8. doi: 10.1111/j.1365-2583.2004.00529.x
Fan, J. H., Xie, Y. P., Xue, J. L., Zhang, Y. L., and Liu, R. (2013). The effect of Beauveria brongniartii and its secondary metabolites on the detoxification enzymes of the pine caterpillar Dendrolimus tabulaeformis. J. Insect Sci. 13, 1–13. doi: 10.1673/031.013.4401
Farooq, M., and Freed, S. (2016). Infectivity of housefly, Musca domestica (Diptera: Muscidae) to different entomopathogenic fungi. Braz. J. Microbiol. 47, 807–816. doi: 10.1016/j.bjm.2016.06.002
Felton, G. W., and Summers, C. B. (1995). Antioxidant systems in insects. Arch. Insect Biochem. 29, 187–197. doi: 10.1002/arch.940290208
Feyereisen, R. (2005). “Insect cytochrome P450 in comprehensive molecular insect science.” Vol. 4. eds. L. I. Gilbert, K. Iatrou, and S. S. Gill (Elsevier), 1–77.
Furlong, M. J., and Groden, E. (2001). Evaluation of synergistic interactions between the Colorado potato beetle (Coleoptera: Chrysomelidae) pathogen Beauveria bassiana and the insecticides, imidacloprid, and cyromazine. J. Econ. Entomol. 94, 344–356. doi: 10.1603/0022-0493-94.2.344
Goble, T. A., Conlong, D. E., and Hill, M. P. (2015). Virulence of Beauveria brongniartii and B. bassiana against Schizonycha affinis white grubs and adults (Coleoptera: Scarabaeidae). J. Appl. Entomol. 139, 134–145. doi: 10.1111/jen.12182
Gopalakrishnan, S., Chen, F. -Y., Thilagam, H., Qiao, K., Xu, W. -F., and Wang, K. -J. (2011). Modulation and interaction of immune-associated parameters with antioxidant in the immunocytes of crab Scylla paramamosain challenged with lipopolysaccharides. Evid.-Based Compl. Alt. 1, 1–8. doi: 10.1155/2011/824962
Habig, W. H. (1981). “Assays for differentiation of glutathione S-transferase” in Method in enzymology. ed. B. J. Willian (New York: Academic Press), 398–405.
Hall, I. M. (1963). “Microbial control” in Insect pathology an advanced treatise. ed. E. Steinhaus (New York: Academic Press), 477–511.
Huang, L. P. (2019). Identification of 29 Beauveria isolates and their virulence against Bemisia tabaci. M.Sc thesis. Guangzhou, China: South China Agricultural University (in Chinese).
Huang, J., and Xu, H. (2016). Matrine: bioactivities and structural modifications. Curr. Top. Med. Chem. 16, 3365–3378. doi: 10.2174/1568026616666160506131012
Ishtiaq, M., Saleem, M. A., and Razaq, M. (2012). Monitoring of resistance in Spodoptera exigua (Lepidoptera: Noctuidae) from four districts of the southern Punjab, Pakistan to four conventional and six new chemistry insecticides. Crop Prot. 33, 13–20. doi: 10.1016/j.cropro.2011.11.014
Jia, M., Cao, M., Li, Y., Tu, X., Wang, G., Nong, X., et al. (2016). Biochemical basis of synergism between pathogenic fungus Metarhizium anisopliae and insecticide chlorantraniliprole in Locusta migratoria (Meyen). Sci. Rep. 6:28424. doi: 10.1038/srep28424
Khosravi, R., Sendi, J. J., and Ghadamyari, M. (2010). Effect of Artemisia annua L. on deterrence and nutritional efficiency of lesser mulberry pyralid (Glyphodes pylolais Walker) (Lepidoptera: Pyralidae). J. Plant Prot. Res. 50, 423–428. doi: 10.2478/v10045-010-0071-8
Koul, O., Kaur, H., Goomber, S., and Wahab, S. (2004). Bio-efficacy and mode of action of rocaglamide from Aglaia elaeagnoidea (Syn. A. roxburghiana) against gram pod borer, Helicoverpa armigera (Hubner). J. Appl. Entomol. 128, 177–184. doi: 10.1111/j.1439-0418.2004.00829.x
Koul, O., Shankar, J. S., Mehta, N., Taneja, S. C., Tripathi, A. K., and Dhar, K. L. (1997). Bio-efficacy of crude extracts of Aglaia species (Meliaceae) and some active fractions against lepidopteran larvae. J. Appl. Entomol. 121, 245–248. doi: 10.1111/j.1439-0418.1997.tb01400.x
Li, S. G., Hau, R. M., Lin, H. F., Cao, H. Q., Hu, J., and Hu, P. (2010). Control effects of four biological pesticides and two chemical pesticides and their mixtures against mixed population of Nilaparvata lugens and Sogatella furcifera. Chin. Bull. Entomol. 47, 768–772.
Li, X., Schuler, M. A., and Berenbaum, M. R. (2007). Molecular mechanisms of metabolic resistance to synthetic and natural xenobiotics. Annu. Rev. Entomol. 52, 231–253. doi: 10.1146/annurev.ento.51.110104.151104
Liu, L. J., Alam, M. S., Hirata, K., Matsuda, K., and Ozoe, Y. (2008). Actions of quinolizidine alkaloids on Periplanta Americana nicotinic acetylcholine receptors. Pest Manag. Sci. 64, 1222–1228. doi: 10.1002/ps.1622
Liu, S., Niu, H., Xiao, T., Xue, C., Liu, Z., and Luo, W. (2009). Does phenoloxidase contributed to the resistance? Selection with butane-fipronil enhanced its activities from diamondback moths. Open Biochem. J. 3, 9–13. doi: 10.2174/1874091X00903010009
Liu, C. H., Wang, Y. W., Yu, H. C., Sun, Y. F., Hou, Y. M., Zhao, K. J., et al. (2016). Toxicity of Beauveria brongniartii to two cutworm species. Chin. J. Appl. Entomol. 53, 739–744.
Liu, Y., Xu, Y., Ji, W., Li, X., Sun, B., Gao, Q., et al. (2014). Anti-tumor activities of matrine and oxymatrine: literature review. Tumor Biol. 35, 5111–5119. doi: 10.1007/s13277-014-1680-z
Lumjuan, N., McCarroll, L., Prapanthadara, L., Hemingway, J., and Ranson, H. (2005). Elevated activity of an epsilon class glutathione transferase confers DDT resistance in the dengue vector, Aedes aegypti. Insect Biochem. Mol. Biol. 35, 861–871. doi: 10.1016/j.ibmb.2005.03.008
Luo, W. C., Li, Y. S., and Mu, L. Y. (1997). The toxicities of alkaloids from Sophora alopecuroides against turnip aphids and effects on several estereases. Acta Entomol. Sin. 40, 358–365 (in Chinese).
Luo, W. C., and Zhang, Q. (2003). The effects of Sophora alopecuroids alkaloids on metabolic esterases of the diamondback moth. Acta Entomol. Sin. 46, 122–125.
Mao, L. X., and Henderson, G. (2007). Antifeedant activity and acute and residual toxicity of alkaloids from Sophora flavescens (Leguminosae) against formosan subterranean termites (Isoptera: Rhinotermitidae). J. Econ. Entomol. 100, 866–870. doi: 10.1603/0022-0493(2007)100[866:aaaaar]2.0.co;2
Mayerhofer, J., Enkerli, J., Zelger, R., and Strasser, H. (2015). Biological control of the European cockchafer: persistence of Beauveria brongniartii after long term application in the euro region Tyrol. BioControl 60, 617–629. doi: 10.1007/s10526-015-9671-6
Müller, P., Donnelly, M. J., and Ranson, H. (2007). Transcription profiling of a recently colonised pyrethroid resistant Anopheles gambiae strain from Ghana. BMC Genomics 8:36. doi: 10.1186/1471-2164-8-36
Nathan, S. S., Choi, M. Y., Seoa, H. Y., Paik, C. H., Kalaivani, K., and Kim, J. D. (2008). Effect of azadirachtin on acetylcholinesterase (AChE) activity and histology of the brown planthopper Nilaparvata lugens (Stål). Ecotox. Environ. Safe. 70, 244–250. doi: 10.1016/j.ecoenv.2007.07.005
Nguyen, H. C., Tran, T. V., Ngyuen, Q. L., Nguyen, N. N., Nguyen, M. K., Nguyen, N. T. T., et al. (2017). Newly isolated Paecilomyces lilacinus and paecilomyces javanicus as novel biocontrol agents for Plutella xylostella and Spodoptera litura. Notu. Bot. Hort. Agrobot. Clujnapoca. 45, 280–286. doi: 10.15835/nbha45110726
Oehmichen, M., and Besserer, K. (1982). Forensic significance of acetylcholine esterase histochemistry in organophosphate intoxication. Int. J. Leg. Med. 89, 149–165. doi: 10.1007/BF01873797
Ortelli, F., Rossiter, L. C., Vontas, J., Ranson, H., and Hemingway, J. (2003). Heterologous expression of four glutathione transferase genes genetically linked to a major insecticide-resistance locus from the malaria vector Anopheles gambiae. Biochem. J. 373, 957–963. doi: 10.1042/BJ20030169
Ou, D. (2019). Toxicity evaluation of entomopathogenic fungi against Asian citrus psyllid. M.Sc thesis. Guangzhou, China: South China Agricultural University (in Chinese).
Regev, A., Keller, M., Strizhov, N., Sneh, B., Prudovsky, E., Chet, I., et al. (1996). Synergistic activity of a Bacillus thuringiensis delta-endotoxin and a bacterial endochitinase against Spodoptera littoralis larvae. Appl. Environ. Microbiol. 62, 3581–3586.
Revathi, K., Chandrasekaran, R., Thanigaivel, A., Kirubakaran, S. A., and Nathan, S. S. (2014). Biocontrol efficacy of protoplast fusants between Bacillus thuringiensis and Bacillus subtilis against Spodoptera litura Fabr. Arch. Phytopathol. Plant Prot. 47, 1365–1375. doi: 10.1080/03235408.2013.840999
Rizwan-ul-Haq, M., Hu, Q. B., Hu, M. Y., Lin, Q. S., and Wang, Q. L. (2009). Biological impacts of harmaline, ricinine and their combined effects with Bacillus thuringiensis on Spodoptera exigua (Lepidopter: Noctuidae). J. Pestic. Sci. 82, 327–334. doi: 10.1007/s10340-009-0257-x
Roslavtseva, S. A., Bakanova, E. I., and Eremina, O. Y. (1993). Esterases in arthropods and their role in the mechanisms of insect acaricide detoxification. Izv. Akad. Nauk Biol. 3, 368–375.
Serebrov, V. V., Gerber, O. N., Malyarchuk, A. A., Martemyanov, V. V., Aleksee, A. A., and Glupov, V. V. (2006). Effect of entomopathogenic fungi on detoxification enzyme activity in greater wax moth Galleria mellonella L. (Lepidoptera, Pyralidae) and role of detoxification enzymes in development of insect resistance to entomopathogenic fungi. Biol. Bull. 33, 581–586. doi: 10.1134/S1062359006060082
Shahabuddin, M., Toyoshima, T., Aikawa, M., and Kaslow, D. C. (1993). Transmission-blocking activity of a chitinase inhibitor and activation of malarial parasite chitinase by mosquito protease. Proc. Natl. Acad. Sci. U. S. A. 90, 4266–4270. doi: 10.1073/pnas.90.9.4266
Shannon, L. M., Kay, E., and Lew, J. Y. (1966). Peroxidase isozymes from horseradish roots. I. Isolation and physical properties. J. Biol. Chem. 241, 2166–2172.
Soni, S., Mehta, P. K., and Chandel, R. S. (2017). Susceptibility of white grub, Brahmina coriacea (Hope) infesting potato to local strains of Beauveria brongniartii (Saccardo) in Himachal Pradesh. J. Biol. Control 32, 41–47. doi: 10.18311/jbc/2018/16288
Sushil, S. N., Josh, D., Tripathi, G. M., Singh, M. R., Baitha, A., Rajak, D. C., et al. (2018). Exploring efficacious microbial bio-agents and insecticides against white grubs in sugarcane in indo-gangetic plains. Sugar Tech. 20, 552–557. doi: 10.1007/s12355-017-0556-0
Terriere, L. C. (1984). Induction of detoxication enzymes in insects. Annu. Rev. Entomol. 29, 71–88. doi: 10.1146/annurev.en.29.010184.000443
Wu, J. H., Yu, X., Wang, X. S., Tang, L. D., and Ali, S. (2019). Matrine enhances the pathogenicity of Beauveria brongniartii against Spodoptera litura (Lepidoptera: Noctuidae). Front. Microbiol. 10:1812. doi: 10.3389/fmicb.2019.01812
Wu, Q. J., Zhang, Y. J., Xu, B. Y., and Zhang, W. J. (2011). The defending enzymes in abamectin resistant Plutella xylostella. Chinese J. Appl. Entomol. 48, 291–295.
Xu, Y. Q., Orozco, R., Wijerante, E. M. K., Espinosa-Artiles, P., Lesile Gunatilaka, A. A., Stock, S. P., et al. (2009). Biosynthesis of the cyclooligomer depsipeptide bassianolide, an insecticidal virulence factor of Beauveria bassiana. Fungal Genet. Biol. 46, 353–364. doi: 10.1016/j.fgb.2009.03.001
Yang, H., Qin, C. S., Chen, Y. M., Zhang, G. Y., Dong, L. H., and Wan, S. Q. (2019). Persistence of Metarhizium (Hypocreales: Clavicipitaceae) and Beauveria bassiana (Hypocreales: Clavicipitaceae) in tobacco soils and potential as biocontrol agents of Spodoptera litura (Lepidoptera: Noctuidae). Environ. Entomol. 48, 147–155. doi: 10.1093/ee/nvy161
Zanardi, O. Z., Ribeiro, L. D. P., Ansante, T. F., Santos, M. S., Bordini, G. P., Yamamotoa, P. T., et al. (2015). Bioactivity of a matrine-based biopesticide against four pest species of agricultural importance. Crop Prot. 67, 160–167. doi: 10.1016/j.cropro.2014.10.010
Zhang, S. H., Zhang, Y., Zhuang, Y., and Han, Y. X. (2012). Matrine induces apoptosis in human acute myeloid leukemia cells via the mitochondrial pathway and Akt inactivation. PLoS One 7:e46853. doi: 10.1371/journal.pone.0046853
Zibaee, A., Bandani, A. R., and Tork, M. (2009). Effect of the entomopathogenic fungus, Beauveria bassiana, and its secondary metabolite on detoxifying enzyme activities and acetylcholinesterase (AChE) of the Sunn pest, Eurygaster integriceps (Heteroptera: Scutellaridae). Biocontrol Sci. Tech. 19, 485–498. doi: 10.1080/09583150902847127
Keywords: Spodoptera litura, matrine, Beauveria brongniartii, antioxidant enzymes, nutritional efficiency
Citation: Wu J, Li J, Zhang C, Yu X, Cuthbertson AGS and Ali S (2020) Biological Impact and Enzyme Activities of Spodoptera litura (Lepidoptera: Noctuidae) in Response to Synergistic Action of Matrine and Beauveria brongniartii . Front. Physiol. 11:584405. doi: 10.3389/fphys.2020.584405
Edited by:
Arash Zibaee, University of Guilan, IranReviewed by:
Ivan Dubovskiy, Novosibirsk State Agrarian University, RussiaOlga Yaroslavtseva, Institute of Systematics and Ecology of Animals (RAS), Russia
Copyright © 2020 Wu, Li, Zhang, Yu, Cuthbertson and Ali. This is an open-access article distributed under the terms of the Creative Commons Attribution License (CC BY). The use, distribution or reproduction in other forums is permitted, provided the original author(s) and the copyright owner(s) are credited and that the original publication in this journal is cited, in accordance with accepted academic practice. No use, distribution or reproduction is permitted which does not comply with these terms.
*Correspondence: Shaukat Ali, YWxpc2NhdUBzY2F1LmVkdS5jbg==