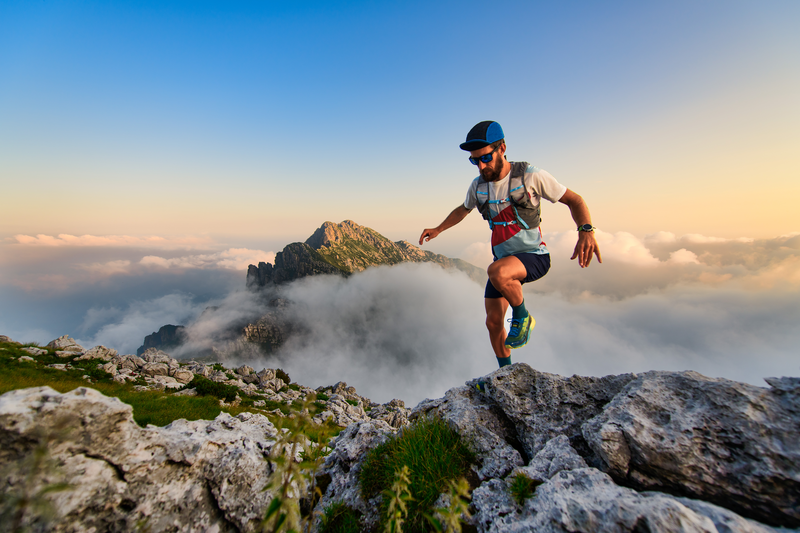
94% of researchers rate our articles as excellent or good
Learn more about the work of our research integrity team to safeguard the quality of each article we publish.
Find out more
REVIEW article
Front. Physiol. , 30 October 2020
Sec. Lipid and Fatty Acid Research
Volume 11 - 2020 | https://doi.org/10.3389/fphys.2020.578966
This article is part of the Research Topic New Insights on Implications of Adipocytokines in Obesity, Metabolic Dysfunction, Rheumatoid and Cardiovascular Diseases: Potential of Therapeutic Interventions View all 14 articles
Besides its role as an energy storage organ, adipose tissue can be viewed as a dynamic and complex endocrine organ, which produces and secretes several adipokines, including hormones, cytokines, extracellular matrix (ECM) proteins, and growth and vasoactive factors. A wide body of evidence showed that adipokines play a critical role in various biological and physiological functions, among which feeding modulation, inflammatory and immune function, glucose and lipid metabolism, and blood pressure control. The aim of this review is to summarize the effects of several adipokines, including leptin, diponectin, resistin, chemerin, lipocalin-2 (LCN2), vaspin, omentin, follistatin-like 1 (FSTL1), secreted protein acidic and rich in cysteine (SPARC), secreted frizzled-related protein 5 (SFRP5), C1q/TNF-related proteins (CTRPs), family with sequence similarity to 19 member A5 (FAM19A5), wingless-type inducible signaling pathway protein-1 (WISP1), progranulin (PGRN), nesfatin-1 (nesfatin), visfatin/PBEF/NAMPT, apelin, retinol binding protein 4 (RPB4), and plasminogen activator inhibitor-1 (PAI-1) in the regulation of insulin resistance and vascular function, as well as many aspects of inflammation and immunity and their potential role in managing obesity-associated diseases, including metabolic, osteoarticular, and cardiovascular diseases.
Adipose tissue is currently considered as an endocrine organ, a complex structure involved not only in fat storage but also in releasing several bioactive polypeptides, collectively named “adipokines” (Kershaw and Flier, 2004; Galic et al., 2010). These adipokines are able to modulate body weight, appetite, glucose homeostasis, inflammation, and blood pressure (Hida et al., 2005; Trujillo and Scherer, 2006; Rabe et al., 2008; Mancuso, 2016; Wang and Scherer, 2016; Weschenfelder et al., 2020). On the other hand, hormones produced in different organs, such as the gut and the cardiovascular system, are integrated in a complex network centered in adipose tissue (Kershaw and Flier, 2004; Galic et al., 2010).
Adipokines are released by either adipocytes (the most abundant being leptin and adiponectin) or preadipocytes, adipose tissue-infiltrated immune cells, or other cell types within adipose tissue. Moreover, adipocytes are also able to produce proinflammatory cytokines, particularly in the chronic subinflammatory state of obesity, and antinflammatory cytokines (Villarroya et al., 2018). In addition, the various immune cell types within adipose tissue contribute to this release. In healthy individuals, type 2 innate lymphoid cells and other Th2 immune cells promote adipose glucose homeostasis by enhancing insulin sensitivity in adipocytes and browning/brown adipose tissue (BAT) activation. On the other hand, pathogenic inflammation of white adipose tissue (WAT) leads to the activation of pro-inflammatory pathways in adipocytes and resident immune cells following obesity, enhancing the production of pro-inflammatory, and inhibiting release of antinflammatory cytokines and adipokines (Villarroya et al., 2018). Recently, it has also been demonstrated that local pro-inflammatory signaling in BAT could repress the thermogenic function thereby impairing diet-induced thermogenesis (Villarroya et al., 2018). All together, these adipose tissue derived factors could be critically involved in the development of insulin resistance, type 2 diabetes mellitus (T2DM), and cardiovascular disease associated with obesity, as reviewed by various authors (Antuna-Puente et al., 2008; Cao, 2014; Nakamura et al., 2014).
More recently, other adipokines such as chemerin, lipocalin-2 (LCN2), vaspin, and omentin-1 have been described as signal molecules involved in neuroendocrine-immune interactions (Carrión et al., 2019; Xie and Chen, 2019). Besides adipose tissue, these adipokines can be produced by synoviocytes, osteoclasts, osteoblasts, chondrocytes, and inflammatory cells which have migrated in the joint microenvironment, and show potent immunomodulatory activities in rheumatic disease, such as in osteoarthritis (OA) and rheumatoid arthritis (RA) (Carrión et al., 2019; Xie and Chen, 2019). In addition, novel adipokines such as follistatin-like 1 (FSTL1), secreted protein acidic and rich in cysteine (SPARC), secreted frizzled-related protein 5 (SFRP5), C1q/TNF-related proteins (CTRPs), as well as family with sequence similarity to 19 member A5 (FAM19A5), wingless-type inducible signaling pathway protein-1 (WISP1), progranulin (PGRN), nesfatin-1 (nesfatin), visfatin/PBEF/NAMPT, apelin, retinol binding protein 4 (RPB4), and plasminogen activator inhibitor-1 (PAI-1) have been recently identified in adipose tissue. However, the mechanisms underlying the molecular and physiological actions of these adipokines are not entirely clear, and further investigations are needed to develop novel therapeutic agents directed to these targets. In this review, we aimed to summarize the effects of adipokines in the regulation of insulin resistance and vascular function, as well as inflammation and immunity and their potential role in managing obesity-associated diseases, including metabolic, rheumatic, and cardiovascular disorders (Table 1).
Table 1. Summary of effects of known adipokines on obesity and metabolic, rheumatic, and cardiovascular diseases.
Leptin is a polypeptide hormone produced by adipocytes and is considered a pro-inflammatory adipokine involved in the “low-grade inflammatory state” described in overweight and with obesity patients (Lago et al., 2009). It is well known that leptin is able to decrease food intake and increase energy expenditure by binding to and activating the long form of a membrane receptor (LEPR-B), highly expressed in the hypothalamus and other brain regions. The expression of LEPR-B was also detected in the nucleus of the solitary tract of the hindbrain, including subpopulations expressing glucagon-like peptide 1 (GLP-1) and cholecystokinin (CCK) (Rosenbaum and Leibel, 1999; Ahima et al., 2000; Schwartz et al., 2000; Myers et al., 2009).
The binding of leptin to LEPR-B leads to activation of a number of signaling pathways, such as Janus kinase 2 (JAK2)/signal transducer and activator of transcription 3 (STAT3), insulin receptor substrate (IRS)-1, phosphatidylinositol 3-kinase (PI3K), SH2-containing protein tyrosine phosphatase 2 (SHP2)-mitogen-activated protein kinase (MAPK), and 5’-adenosine monophosphate-activated protein kinase (AMPK)/acetyl-CoA carboxylase, and other pathways (Morris and Rui, 2009; Dalamaga et al., 2013; Moon et al., 2013; Park and Ahima, 2015). In particular, the activation of JAK2/STAT3 signaling was found to be critically involved in modulatory effects of leptin on energy homeostasis (Bates et al., 2003; Dardeno et al., 2010). The role of leptin in obesity-induced adipose inflammation has been described by Deng et al. (2013). In this context, leptin was found to stimulate CD4 + TH1 IFNγ secretion to induce adipocyte MHCII expression.
Leptin is known to be mainly involved in response and defense against reductions of body fat that could lead to impairment of survival and reproductive fitness. Therefore, CNS actions of leptin are necessary for energy stores, as well as normal energy homeostasis, reproduction, and the like.
Therefore, improving or restoring central leptin sensitivity is an interesting target in pharmacological treatment of weight loss.
The association of leptin with amylin, a peptide hormone released with insulin by pancreatic beta cells which modulate glucose and energy homeostasis, showed enthusiastic results, predominantly mediated by decreased feeding and increased central leptin sensitivity in diet-induced obesity (Roth et al., 2008; Trevaskis et al., 2010). In addition to its role in feeding control and energy expenditure, leptin also upregulates pro-inflammatory cytokines, including tumor necrosis factor (TNF)-α and interleukin (IL)-6; these factors are associated with insulin resistance and T2DM (López-Jaramillo et al., 2014). In this context, pro-opiomelanocortin (POMC)-expressing neurons located into the hypothalamic arcuate nucleus (ARC) are critically involved in leptin’s effects on glucose homeostasis in obesity and insulin resistance states (Huo et al., 2009; Berglund et al., 2012). In particular, leptin was found to activate anorexigenic neurons co-expressing POMC and cocaine- and amphetamine-regulated transcript (CART), and inhibit orexigenic neurons co-expressing agouti-related peptide (AgRP) and neuropeptide Y (NPY). During fasting, a rapid reduction of leptin concentrations in serum was found. The fall in leptin stimulates the expression of AgRP and NPY and suppresses POMC and CART, thereby resulting in stimulated food intake and reduced energy expenditure (Ahima et al., 1999; Cowley et al., 2001). Leptin was also found to inhibit neurons that express melanin-concentrating hormone and orexins, in the lateral hypothalamus, resulting in inhibition of food intake (Abizaid et al., 2006; Robertson et al., 2008). Regarding its role on energy expenditure, leptin has been reported to act primarily in the hypothalamus. The result of this signaling is an increase in the sympathetic tone that promotes lipid mobilization in the WAT and thermogenesis in the BAT through enhanced uncoupling protein 1 (UCP1) expression (Scarpace et al., 1997; Pandit et al., 2017).
Several studies showed that circulating levels of leptin are highly related to percentage of body fat in humans suffering from obesity and normal body weight individuals; increased size of adipocytes due to accumulation of triglyceride induces an increase of leptin as an adaptive response signaling control of energy balance to the central nervous system (CNS) (Rosenbaum and Leibel, 1999; Ahima et al., 2000; Schwartz et al., 2000; Hafizuallah, 2006; Myers et al., 2009). A more recent study on Saudi women with high body mass index (BMI), waist circumference, and metabolic syndrome confirmed a significant correlation between leptin levels and obesity (Al-Amodi et al., 2018). The first clinical trial that studied the potential pharmacological effects of leptin on polygenic or simple obesity dates back to 1999 (Heymsfield et al., 1999). In this context, recombinant methionyl human leptin was injected subcutaneous daily at high dose (e.g., a 100-kg patient in the 0.3 mg/kg dose group was administered 30 mg of leptin in 6 ml in two divided doses). The study, although original, was little appreciated because of inconsistent results and not requiring that patients reduced their food intake. On the other hand, recombinant leptin treatment is widely established and approved to treat obesity causes by leptin deficiency. Farooqi et al. (2002) showed that daily subcutaneous injections of recombinant human leptin for up to 4 years induced beneficial effects on various phenotypic abnormalities, such as appetite and fat mass, in children suffering from congenital obesity due to genetic deficiency of the leptin gene.
Over the years, different clinical trials have studied the potential effect of recombinant leptin to mitigate weight regain after caloric restriction or weight loss (Rosenbaum et al., 2005). Zelissen et al. (2005) evaluated the effects on body weight of subcutaneous administration of 10 mg recombinant leptin twice daily for 12 weeks associated with a restricted 500 kcal diet. However, this study did not show any significant change in body weight between active and placebo groups. Actually, elevated leptin levels in individuals affected by obesity point to a state of resistance to this peptide which could be due defects in leptin receptor signaling (Levin et al., 2004; Munzberg et al., 2004). Different studies on abnormal adipokine profiles in people suffering from obesity and T2DM have resulted in ambiguous findings. In these patients, Liu W. et al. (2020) described significantly increased leptin and reduced adiponectin levels. In addition, although it has been hypothesized a direct effect of leptin on pancreatic β-cell function (Lee et al., 2011), acting as an insulin sensitizer, and improving metabolism in patients with leptin-deficiency, it does not increase insulin sensitivity in humans with obesity (Mittendorfer et al., 2011) and does not lead to activation of intracellular signaling pathways and/or improve diabetes and glycemic control in individuals suffering from obesity and T2DM (Moon et al., 2011). Specifically, a short-term (2 weeks) pilot study conducted on humans suffering from obesity with T2DM treated with recombinant human leptin (20–80 mg/day) did not show improved insulin action, although recombinant leptin resulted in three- to 150-fold increase in plasma concentrations of leptin (Mittendorfer et al., 2011). In addition, in patients affected by obesity with T2DM, metreleptin injection at a dose of 10 mg/kg/day for 16 weeks did not modify body weight or circulating inflammatory markers but decreased HbA(1c) marginally (Moon et al., 2011).
Further studies are needed to clarify the role of leptin treatment in personalized management of people suffering from T2DM.
Leptin exerts modulatory effects on both innate and adaptive immunity activating LEPR expressed on immune cell, chondrocytes, and osteoblasts (Gómez et al., 2011; Conde et al., 2012; Procaccini et al., 2012). Different studies have described the involvement of the leptin in several physiological and pathophysiological conditions, including inflammation, immunity, and rheumatic diseases (Conde et al., 2014; Scotece et al., 2014b; Tian et al., 2014). A wide body of evidence showed that leptin serum levels are significantly increased in RA, and related to disease course and activity (Lee et al., 2007; Yoshino et al., 2011; Cao et al., 2016; Lee and Bae, 2016). In addition, the synovial/serum leptin ratio was positively associated with erosions in patients with RA compared to non-erosive RA controls (Olama et al., 2012). In particular, leptin was found to induce expression of IL-6 and IL-8 in synovial fibroblasts in patients with RA, via activation of JAK2/STAT3, nuclear factor kappa-light-chain-enhancer of activated B cells (NF-κB), and AP-1 signaling pathways (Muraoka et al., 2013; Yang et al., 2013), representing a new therapeutic target in the treatment of RA. On the other hand, cardiovascular risk is also highly related to obesity and RA pathology. Accordingly, Batun-Garrido et al. (2018) showed that leptin and IL-6 levels are associated with cardiovascular risk in RA patients (Batun-Garrido et al., 2018).
Leptin antagonists have been proposed for the prevention of RA in genetically susceptible individuals (Fazeli et al., 2006). In particular, a monoclonal antibody (mAb) against the human leptin receptor has been designed that blocks human monocyte and T cell activation in tissue-specific manner (Fazeli et al., 2006).
There is growing evidence regarding the implication of leptin in the pathogenesis of CVD. Increased plasma leptin levels were observed in patients with essential hypertension (Beltowski, 2006; Momin et al., 2006; Katsiki et al., 2018). Leptin was also found to induce vasodilator effects in humans with coronary artery disease (Beltowski, 2006; Momin et al., 2006; Katsiki et al., 2018). However, administration of leptin did not modify blood pressure, probably because of concomitant stimulation of the sympathetic nervous system, natriuresis, and NO-dependent vasorelaxation (Beltowski, 2006). More recently, Simonds et al. (2014) reported that increased leptin levels in diet-induced obesity drive an increase in blood pressure in rodents, suggesting that pharmacological approaches based on the modulation of leptin effects on specific subpopulations of neurons could represent a potentially useful therapeutic strategy for obesity-associated hypertension and cardiovascular disease. In addition to its effects on blood pressure, leptin regulates cardiac contractile function, metabolism, cell size, and production of extracellular matrix (ECM) components in cardiomyocytes (Dong et al., 2006; Madani et al., 2006; Palanivel et al., 2006). In this context, Palanivel et al. (2006) reported the distinct effects of long- and short-term leptin treatment on glucose and fatty acid uptake and metabolism in HL-1 cardiomyocytes. These differences might lead to lipotoxic damage in heart failure (Palanivel et al., 2006). Moreover, leptin reduced myocardial reperfusion-induced death (Smith et al., 2006) and induced the elongation of myocardial cells and eccentric dilatation with compensation (Abe et al., 2007). In accordance with these results, it has been suggested an important role of leptin in the regulation of cardiomyocyte hypertrophy (Adiarto et al., 2007). As regards its effects on atherosclerosis, leptin was shown to play a critical role in the early phase of this disease. Leptin was able to initiate monocyte/macrophage recruitment into the vessel wall, increasing so the production of superoxides by mitochondria and expression of MCP-1 in aortic endothelial cells (Yamagishi et al., 2001). The inconsistent correlation of leptin levels with preclinical atherosclerosis may depend on the pathophysiological context of patients, medications, or other factors not yet identified. It can be said that therapeutic interventions targeting leptin might potentially prevent or reduce cardiovascular complications.
Adiponectin (also known as Acrp30, AdipoQ, GBP-28, and apM1) is a 244-amino acid protein se-creted by different cell types, such as adipocytes and endothelial cells (Scherer et al., 1995; Maeda et al., 1996; Achari and Jain, 2017). Different isoforms of adiponectin were described: globular, trimeric [low molecular weight (LMW)], hexameric [middle molecular weight (MMW)], and multimeric [high molecular weight (HMW)] adiponectin isoforms (Garaulet et al., 2007). The insulin-sensitizing and anti-inflammatory effects of adiponectin are mediated by two isoforms of adiponectin receptors (AdipoR1 and AdipoR2). In particular, AMPK phosphorylation and increased expression of peroxisome proliferator-activated receptor α (PPARα) through adiponectin receptor (AdipoR)1 and AdipoR2, respectively, are involved in mediating fatty acid metabolism and the insulin sensitizing actions of adiponectin in skeletal muscle (Kadowaki and Yamauchi, 2005; Ye and Scherer, 2013; Kim and Park, 2019). In this context, the binding of adiponectin with its receptors leads to the activation of various signaling pathways such as IRS-1/2, AMPK, and p38 MAPK. Activation of IRS-1/2 by adiponectin signaling is mainly involved in insulin-sensitizing effects of adiponectin in insulin responsive tissues. In addition, PI3K/Akt pathway plays a critical role in mediating metabolic actions of insulin, such as protein synthesis, lipogenesis, glucose uptake and utilization, glycogen synthesis, and reduced lipolysis and gluconeogenesis (Yamauchi et al., 2007).
In the liver, adiponectin activates glucose transporters and inhibits gluconeogenesis via AMPK, stimulating fatty acid oxidation and decreasing inflammation through the PPARα pathway (Wu et al., 2003). Adiponectin expression and release are strongly linked to healthy adipocyte differentiation, because they are modulated by PPARγ, and to insulin sensitivity. In fact, PPARγ was also found to regulate GLUT4 expression in adipocytes. In agreement, both expression and release of adiponectin are inhibited by inflammation and other stress phenomena occurring in obesity/T2DM (Astapova and Leff, 2012). Because of these positive actions, adiponectin has been widely investigated in human and animal models. In rodents, a significant increase of glucose uptake and fat oxidation in skeletal muscle, paralleled by reduced production of glucose in the liver and improved insulin sensitivity were found following administration of recombinant adiponectin (Berg et al., 2001; Fruebis et al., 2001; Yamauchi et al., 2001). Moreover, adiponectin was found to modulate feeding behavior and energy expenditure during fasting through central effects (Kubota et al., 2007). On the other hand, the central contribution of adiponectin is a rather marginal mechanism, being the main action in peripheral tissues.
It is well known the involvement of adiponectin in obesity-associated comorbidities, including T2DM and cardiovascular disease (Achari and Jain, 2017). Circulating levels of adiponectin are inversely associated with obesity, insulin sensitivity, T2DM, and metabolic syndrome (Arita et al., 1999; Hotta et al., 2000; Weyer et al., 2001; Mohan et al., 2005; Gilardini et al., 2006; Yamauchi et al., 2007; Wang et al., 2008).
Over the years, adiponectin has been mainly studied for its effects on metabolic syndrome, T2DM, and atherosclerosis. However, the opposing effects observed for some of its isorforms on effector cells of arthritis shifted the focus toward this disease (Neumann et al., 2011; Fatel et al., 2018).
In particular, HMW adiponectin was found to stimulate IL-6 secretion in human monocytes, while LMW adiponectin decreased IL-6 and stimulated secretion of IL-10 in LPS-activated monocytes (Neumeier et al., 2006). To evaluate the potential therapeutic effects of adiponectin in RA, Lee et al. (2018) generated various monoclonal antibodies (mAbs) specific to different adiponectin isoforms. Some clones of mAb, such as KH4-8, effectively inhibited expression of IL-6 and IL-8 in recombinant adiponectin-stimulated human osteoblasts, while other clones, KH7-33 and KH4-8, improved rheumatic symptoms in a mouse model of collagen-induced arthritis (CIA). Lee et al. (2018) found that antibodies against either MMW/HMW or MMW alone significantly ameliorated CIA in mice; therefore, a possible contribution of both adiponectin isoforms to progression of arthritis has been hypothesized. These results suggest that targeting adiponectin with mAb could be beneficial in inflammatory conditions. Previous studies showed a positive association between circulating adiponectin levels, disease activity, and radiographic disease progression (Neumann et al., 2011; Fatel et al., 2018). More recently, Liu R. et al. (2020) showed that intra-articular injection of adiponectin increased synovial inflammation by T follicular helper cells in a mouse model of CIA. Surprisingly, the correlation with disease activity has been found in some studies but not in others (Fatel et al., 2018), a discrepancy which still needs to be elucidated.
Different reports have described the protective activity of adiponectin in atherosclerosis and cardiovascular disease (Ouchi and Walsh, 2007; Villarreal-Molina and Antuna-Puente, 2012).
Adiponectin decreased inflammation in vascular endothelial cells, smooth muscle cell, cardiac myocytes, and macrophages predominantly by activating AMPK and cyclic AMP-protein kinase A (cAMP-PKA) (Nigro et al., 2013; Subedi and Park, 2013). The antinflammatory activity of adiponectin may be mediated by direct effects on inflammatory cells or through suppression of the NF-kB signaling pathway (Ouchi et al., 2003; Kobayashi et al., 2004; Shibata et al., 2004; Nigro et al., 2013). In addition, studies showed that overexpression of adiponectin protects from atherosclerotic plaque formation (Okamoto et al., 2008), whereas deficiency of adiponectin results in increased incidence of atherosclerosis (Nawrocki et al., 2010). In particular, in adiponectin knocked out mice, there have been observed inflammatory vascular lesions following mechanical injury, with neointimal thickening and stimulated proliferation of vascular smooth muscle cells that was shown to be attenuated by restoring physiological levels of adiponectin (Kadowaki and Yamauchi, 2005). The vasoprotective effects of adiponectin could be due to increased production of nitric oxide through activation of endothelial NO synthase (eNOS) via AMPK-dependent (Kobayashi et al., 2004; Ouchi et al., 2004) as well as cyclooxygenase 2-dependent mechanisms (Ohashi et al., 2009).
Recently, plasma concentrations of adiponectin have been investigated in patients with coronary artery disease and slow coronary flow. Low plasma levels of adiponectin were found in atherosclerotic coronary artery disease (Diah et al., 2019). A recent metanalysis showed that pravastatin, a 3-hydroxy-3-methylglutaryl coenzyme reductase inhibitor, widely used in the primary and secondary prevention of cardiovascular diseases, increases circulating adiponectin levels, which could be a mechanism underlying the beneficial effects of pravastatin in atherosclerosis (Shu and Chi, 2019).
However, some studies have suggested that the association between adiponectin concentrations and risk of vascular events is strictly dependent on ethnicity (Gardener et al., 2013). Therefore, it is necessary to obtain more data in diverse populations.
Resistin (or “resistance to insulin”) was discovered in mice in 2001 as an adipocyte-derived signaling molecule, and named for its role in inducing insulin resistance (Steppan et al., 2001a). Resistin belongs to a family of resistin-like molecules which include various members with distinct expression patterns and biological effects (Steppan et al., 2001b). Human resistin is expressed in adipose tissue, bone marrow, lung (Patel et al., 2003), placental tissue (Yura et al., 2003), pancreatic islet cells (Minn et al., 2003), and plasma (Yannakoulia et al., 2003). Inconsistent associations between serum resistin levels and body fat have been found with positive (Zhang et al., 2002), inverse (Way et al., 2001; Rajala et al., 2002; Steppan and Lazar, 2002) or no correlations (Fain et al., 2003), in rodents. Increased serum resistin levels were found in humans suffering from obesity respect to lean individuals (Schäffler et al., 2004; Vendrell et al., 2004). Moreover, elevated resistin levels were observed to induce insulin resistance in several rat and mouse models (Rajala et al., 2004; Rangwala et al., 2004; Satoh et al., 2014), suggesting an involvement of hyper-resistinemia in insulin resistance. However, the possible relationships between circulating resistin levels and insulin resistance in individuals affected by obesity with and without diabetes have not been clarified. Al-Harithy and Al-Ghamdi (2005) found a positive correlation between serum resistin levels and insulin resistance in women with T2DM and women suffering from obesity or overweight women whithout T2DM, while other studies failed to report associations between serum resistin levels and insulin resistance (Gerber et al., 2005; Bu et al., 2012). The discrepant results were probably due to small sample sizes, population differences, disease status, and use of non-standardized assays of resistin (Huang and Yang, 2016; Park H. K. et al., 2017). Recently, a metanalysis of 15 studies confirmed a positive correlation between circulating resistin levels and insulin resistance in individuals suffering from obesity and T2DM with hyperresistinemia, but not in patients with normal resistin levels. In this context, resistin has been hypothesized as a new therapeutic target for the prevention and treatment of diabetes mellitus (Su et al., 2019).
The role of resistin in regulating inflammatory pathways in the context of rheumatic diseases has been well verified. Resistin expression was detected in synovial fluid (SF) and serum of both RA and OA patients. In particular, resistin was shown to accumulate in the inflamed joints of RA patients and to induce the development of arthritis accompanied by leukocytic infiltration and hyperplasia of the synovium following injection into the joints of healthy mice. In addition, different reports suggest that resistin has pro-inflammatory properties (Bokarewa et al., 2005). Interestingly, Kassem et al. (2010) reported that serum resistin could be a good prognostic marker in RA. Thommesen et al. (2006) showed that resistin exerts stimulatory effects on osteoblast proliferation and its expression is increased during osteoclast differentiation, through protein kinase C (PKC) and PKA signaling mechanisms. In human articular chondrocytes, resistin treatment led to increased expression of various cytokines and chemokines, including TNF-α, IL-6, and IL-12 (Zhang Z. et al., 2010), along with different catabolic enzymes and markers of cartilage, including metalloproteinase (MMP)-1, MMP-2, and ADAMTS-4, while it decreased type II collagen alpha 1 and aggrecan (Fang et al., 2011).
Recently, resistin was also found to promote expression of MCP-1 in synovial fibroblasts from OA patients and monocyte migration (Chen et al., 2019), confirming potential therapeutic implications of this adipokine for patients affected by OA.
A positive correlation between serum resistin levels and hypertension was shown in humans (Takata et al., 2008; Zhang L. et al., 2010; Thomopoulos et al., 2011). In this context, the potential role of resistin as a risk factor for hypertension has also been confirmed in a recent meta-analysis (Zhang et al., 2017). Moreover, resistin has been suggested to play a modulatory role on angiogenesis, thrombosis, and vascular smooth muscle cell migration and proliferation, which contribute to pathogenesis and progression of atherosclerosis (Jamaluddin et al., 2013). In particular, human resistin was able to cause a significant increase in the expression of pro-inflammatory factors, such as MCP-1, endothelin-1, and MMPs (Manduteanu et al., 2010; Hsu et al., 2011). Human resistin was also found to increase the proliferation and migration of human endothelial cells and vascular smooth muscle cells and endothelial permeability, through oxidative stress and activation of p38 MAPK signaling pathways (Jamaluddin et al., 2013). Associations between serum resistin levels and development of coronary artery disease were also observed in humans. A number of studies found increased levels of resistin in angina pectoris patients undergoing coronary angiography (Lee and Kim, 2012). Elevated resistin levels were found to be predictive of coronary atherosclerosis, independently of CRP levels, in asymptomatic subjects (Park and Ahima, 2013). Resistin is also a predictor of major adverse cardiovascular events in patients with coronary artery disease, including cardiovascular death and myocardial infarction (MI; On et al., 2007; Kreçki et al., 2011). Additionally, various studies confirmed elevated levels of resistin in humans with acute coronary syndromes and its relationship with severe myocardial injury and poor prognosis (Lee and Kim, 2012). On the other hand, further studies are necessary to understand the specific signaling mechanisms involved in the effects of resistin in cardiovascular disease.
Chemerin is an inflammatory cell-derived chemokine structurally and evolutionary related to cathelicidin precursors (antibacterial peptides), cystatins (cysteine protease inhibitors), and kininogens (Wittamer et al., 2003). It is highly expressed in adipose tissue in vivo and showed to promote adipogenesis in a 3T3-L1 fibroblast preadipocyte model (Goralski et al., 2007). Different chemerin isoforms exist depending on protease mediated cleavage of the peptide sequence (Zabel et al., 2005; Yamaguchi et al., 2011; Zhao et al., 2018). Wittamer et al. (2004) showed that cleavage must be extremely specific in order to maintain the active chemerin S157 peptide as an agonist on its G-protein-coupled receptor encoded by the gene CMKLR1 (also known as ChemR23). ChemR23 is widely known to act through Gi and ERK1/2 (Wittamer et al., 2003); however, Kaur et al. (2010) demonstrated that chemerin-induced endothelial angiogenesis is mediated through ChemR23 and downstream activation of Akt (PKB), p38, and ERK1/2 (Kaur et al., 2010). The connection between chemerin and obesity indicates that it may contribute to the development of obesity. Elevated chemerin gene expression and its receptor were observed in adipose tissue of obese and type 2 diabetic gerbil Psammomys obesus with respect to lean, normoglycemic P. obesus (Bozaoglu et al., 2007). On the other hand, food restriction was accompanied by reduced gene expression of chemerin in WAT, in rats (Stelmanska et al., 2013). In addition, chemerin administration displayed modulatory effects on several adipokines such as leptin, adiponectin, and IL-6, which are involved in the modulation of metabolic and inflammatory responses (Goralski et al., 2007). In humans, the existence of a positive correlation was found between both local and circulating levels of chemerin, BMI, and obesity-related biomarkers. Moreover, chemerin has been hypothesized as a possible link between obesity and the development of T2DM (Ernst and Sinal, 2010). Gene expression of chemerin was increased in adipose tissue of patients suffering from obesity with T2DM (Bozaoglu et al., 2007). In mouse preadipocyte cell line 3T3-L1, chemerin has been suggested to stimulate insulin sensitivity in adipose tissue by enhancing insulin-stimulated uptake of glucose and IRS-1 tyrosine phosphorylation (Kalra et al., 1999; Seeger et al., 2008). In humans, no significant differences in plasma chemerin concentrations were found between T2DM patients and normal subjects. On the other hand, in subjects with normal glucose tolerance, circulating levels of chemerin are reported to be significantly associated with BMI, circulating triglycerides and blood pressure (Bozaoglu et al., 2007). These results indicate that chemerin plays a pivotal role in the modulation of energy balance, making it a promising pharmacological tool in obesity treatment.
Different studies described a positive correlation between systemic chemerin and CRP in various chronic inflammatory diseases, including rheumatic diseases (Buechler, 2014; Fatima et al., 2014; Mariani and Roncucci, 2015). In agreement, chemerin might have a pivotal role in the pathophysiology of RA (Buechler, 2014; Fatima et al., 2014; Mariani and Roncucci, 2015). Patients with rheumatic diseases express both chemerin and its receptor in chondrocytes and synovial fibroblasts (Berg et al., 2010; Kaneko et al., 2011; Yamaguchi et al., 2011). However, the biological effects of its different isoforms in rheumatic diseases are not clearly defined. Mouse chem156S, homologous to human chemerin isoform chem157S, showed antinflammatory activity in macrophage (Cash et al., 2008) and in LPS-induced acute lung injury model (Luangsay et al., 2009). Moreover, Zhao et al. (2018) described the isoform chem156F in SF samples from patients with rheumatic diseases. Chemerin has been hypothesized to stimulate synovial fibroblasts motility and leukocyte migration into the joint, as well as the expression of IL-6, MCP1, MMP-3, and Toll-like receptor 4 (TLR4) in synovial fibroblasts from RA patients (Conde et al., 2011; Kaneko et al., 2011; Eisinger et al., 2012). Chemerin could therefore play a critical role in innate and adaptative immunity by acting as a chemoattractant agent for natural killer cells, macrophages, and dendritic cells (Meder et al., 2003; Wittamer et al., 2003). Interestingly, tocilizumab, a humanized mAb directed to IL-6 receptor, decreased serum chemerin levels in RA patients (Fioravanti et al., 2019).
Besides its role in adipogenesis and energy metabolism (Bozaoglu et al., 2007; Goralski et al., 2007; Ernst et al., 2010; Roman et al., 2012), recent studies reported a key role for chemerin in the pathologenesis of cardiovascular diseases, including coronary heart disease (Kaur et al., 2018). Recently, Wu et al. (2020) reported increased epicardial fat volume, lower adiponectin expression and higher chemerin and vascular endothelial growth factor (VEGF) expression in patients with coronary artery disease compared to healthy individuals. Despite some limitations, the study reported that expression of adiponectin gradually decreased with the increase of epicardial fat volume, while the expression of chemerin and VEGF gradually increased with the increase of epicardial fat volume, displaying a positive correlation with coronary artery disease. In addition, a study conducted in 40 patients suffering from T2DM with cardiovascular disease showed that serum concentrations of chemerin, omentin-1, and visfatin correlated with the prognosis of cardiovascular complications. In particular, chemerin and visfatin (pro-inflammatory markers) levels were positively correlated with increased C-reactive protein (CRP), triglycerides, fasting blood glucose (FBG), serum cholesterol, and microalbuminuria, while omentin-1 (antinflammatory marker) levels were significantly reduced (Ahmed et al., 2020). On the other hand, chemerin stimulates the progression of atherosclerosis in ApoE–/– mice, confirming its crucial role in the pathologic process of cardiovascular diseases (Liu et al., 2019). In addition, atorvastatin and rosuvastatin were found to decrease chemerin levels in patients with acute MI (Tunçez et al., 2019). On the basis of these findings, it has been suggested a significant role of chemerin in human vascular health and disease.
Lipocalin 2 (LCN2), also known as neutrophil gelatinase-associated lipocalin (NGAL), is a glycoprotein recently identified as a cytokine derived from adipose tissue. It is able to bind small substances, including steroids and lipopolysaccharides (Flower, 1996; Chu et al., 1998). Expression of LCN2 was shown in a number of tissues such as uterus (Huang et al., 1999), immune cells (Borregaard and Cowland, 2006), liver, spleen, kidney, and bone marrow (Cowland and Borregaard, 1997; Aigner et al., 2007). Two receptors have been identified for LCN2: the solute carrier family 22 member 17 (SLC22A17 or 24p3R) binding mouse LCN2 (Devireddy et al., 2005) and the megalin/glycoprotein GP330, a low-density lipoprotein receptor involved in binding of human LCN2 protein (Hvidberg et al., 2005). Different studies reported its role in apoptosis (Devireddy et al., 2001), transport of fatty acids (Chu et al., 1998) and iron (Yang et al., 2002), regulation of inflammation (Cowland and Borregaard, 1997), and metabolism (Yan et al., 2007).
Despite LCN2 expression is altered in obesity (Sommer et al., 2009; Jang et al., 2012), its role in the pathogenesis of this disease is not entirely clear. Transcriptional activation of LCN2 gene in adipose tissue has been hypothesized to be related to inflammation and obesity (Garay-Rojas et al., 1996; Shen et al., 2006). Notably, a role for LCN2 in the adipose tissue remodeling has also been suggested (Garay-Rojas et al., 1996; Shen et al., 2006). On the other hand, Xiaoli Chen’s group demonstrated that molecular disruption of LCN2 in mice significantly exacerbates diet-induced obesity, dyslipidemia, fatty liver disease, and insulin resistance (Guo et al., 2010). In this context, LCN2 may be considered one of the mediators responsible for the low-level systemic inflammation observed in metabolic syndrome associated with obesity (Jang et al., 2012; Abella et al., 2015), representing a future therapeutic target.
In joint tissues, LCN2 expression is induced after stimulation with inflammatory factors and in response to mechanical loading (Gupta et al., 2007; Katano et al., 2009; Conde et al., 2011). Elevated LCN2 concentrations were found in SF of RA patients (Gupta et al., 2007; Katano et al., 2009; Staikos et al., 2013). LCN2 is also believed to play a role in degenerative articular diseases (Gómez et al., 2011), and it has been suggested as a biomarker of cartilage degradation in arthritic diseases (Wilson et al., 2008). In chondrocytes, IL-1β, LPS, certain adipokines (leptin and adiponectin), and dexamethasone were found able to modulate LCN2 expression (Conde et al., 2011). Moreover, it was also suggested to establish a feedback regulatory loop with nitric oxide (Gomez et al., 2013). Although some data suggest that LCN2 is involved in joint pathophysiology, further studies are needed to better clarify its role in clinical rheumatology.
Increasing evidences suggest the involvement of LCN2 in inflammatory processes in cardiovascular diseases (Sivalingam et al., 2017). In particular, LCN2 plays a crucial role in vascular remodeling and plaque instability in atherosclerosis (Hemdahl et al., 2006; Folkesson et al., 2007). LCN2 has been reported to regulate the enzymatic activity of MMP-9, acting as a key mediator of plaque instability in atherosclerosis (Cakirca and Turgut, 2018). In addition, elevated LCN2 concentrations have been found in heart failure, coronary heart disease, and stroke (Elneihoum et al., 1996; Damman et al., 2008; Yndestad et al., 2009; Zografos et al., 2009), providing biological plausibility for the potential role of LCN2 as a biomarker in cardiovascular disease. Despite a wide literature supporting a role of LCN2 in the pathophysiology of cardiovascular disease, to date there are insufficient data regarding the clinical usefulness of monitoring LCN2 concentrations in the treatment of cardiovascular diseases (Cruz et al., 2012).
Visceral adipose tissue-derived serpin (vaspin) is a 47-kDa protein, belonging to the serine protease inhibitor family (Hida et al., 2000). Human, rat, and mouse vaspin proteins are constituted by 395, 392, and 394 amino acids, respectively, and display a 40% homology with α1-antitrypsin (Hida et al., 2005). Increasing numbers of studies emphasize the role of vaspin in obesity and related metabolic disorders. Vaspin was found to be mainly expressed in visceral adipose tissue of Otsuka Long-Evans Tokushima fatty (OLETF) rats (Hida et al., 2000, 2005), an animal model of T2DM characterized by abdominal obesity, insulin resistance, hypertension, and dyslipidemia (Kawano et al., 1992), with higher plasma levels at age 30 weeks, concomitantly with peak of insulin concentrations. In humans, vaspin was also reported to be expressed in several tissues, such as visceral and subcutaneous adipose tissue and skeletal muscle (Klöting et al., 2006; Klöting et al., 2011; Nicholson et al., 2018, 2019). Interestingly, human vaspin mRNA expression in adipose tissue was found to be modulated in a fat depot-specific manner and to be related to obesity and insulin resistance (Klöting et al., 2006). In addition, vaspin was more expressed in subcutaneous adipose tissue and skeletal muscle of older patients with obesity compared to lean age-matched controls (Nicholson et al., 2019). A recent metanalysis of six studies aimed to evaluate the relationship between vaspin and obesity confirmed increased concentrations of vaspin in patients suffering from obesity and T2DM (Feng et al., 2014). Administration of recombinant human vaspin to obese mice fed a high-fat and high-sucrose diet was found to improve glucose tolerance and insulin sensitivity, reduce the gene expression of leptin, TNF-α, and resistin, and stimulate adiponectin gene expression (Hida et al., 2005), suggesting an insulin-sensitizing role for vaspin. Klöting et al. (2011) showed that central and peripheral vaspin administration to both obese db/db and lean C57BL/6 mice led to a reduction in food intake. More recently, Heiker et al. (2013) found that recombinant vaspin administration induced a similar increase in insulin sensitivity in both db/db and C57BL6 mice. The same authors proposed that the anorexigenic effects of vaspin could be related to inhibition of a protease that degrades a putative anti-orexigenic factor. Similarly, we have previously reported that administration of vaspin into the hypothalamic ARC of rats decreased food intake, probably due to reduction of NPY and increase of POMC mRNA levels (Brunetti et al., 2011). In humans, serum vaspin concentrations displayed a pre-prandial rise followed by a significant reduction in response to meals (Jeong et al., 2010). Moreover, vaspin serum concentrations have been reported to be significantly related to obesity and impaired insulin sensitivity. However, this correlation seemed to be abrogated in T2DM (Youn et al., 2008). It has been proposed that the effects of vaspin on glycemic control are mediated by kallikrein 7, a protease cleaving human insulin in vitro. Vaspin was found to inhibit kallikrein 7 through its serpin activity. Therefore, vaspin-induced antidiabetic effects could be related, at least in part, to a decreased degradation of circulating insulin (Heiker et al., 2013). In addition, vaspin has been suggested to be one of the effector molecules under the control of PPARγ, as confirmed by upregulated vaspin mRNA expression in rodents following pioglitazone treatment (Wada, 2008). Additional data in humans will facilitate the entire understanding of vaspin mechanism of action, making it possible to develop novel treatment strategies.
The potential role of vaspin in rheumatic diseases, including OA and RA, has not been fully evaluated. Vaspin was found to attenuate receptor activator of nuclear factor kappa-B ligand (RANKL)-induced osteoclast formation in murine macrophage cell line RAW264.7, decrease apoptosis of human osteoblasts, and regulate the osteogenic differentiation of pre-osteoblast cell line MC3T3-E1 (Kamio et al., 2013; Liu et al., 2016). Recent in vitro studies demonstrated that vaspin exerted protective effects against high fat diet-induced bone loss, and promoted osteoblastic differentiation through activation of the Smad-Runx2 signaling pathway (Wang et al., 2020). Conflicting reports were published on serum vaspin levels in rheumatic diseases. In particular, Bao et al. (2014) showed that vaspin serum concentrations were lower in OA patients respect to healthy subjects. On the other hand, higher serum vaspin levels were found in psoriatic arthritis and RA patients respect to healthy subjects (Ozgen et al., 2010; Colak et al., 2019). Importantly, Klaasen et al. (2012) showed that vaspin levels were increased following treatment with glucocorticoids in RA patients. In other works, no association was found between serum vaspin levels and serum CRP levels or leukocyte count in SF of RA patients (Senolt et al., 2010). In an opposite way, vaspin serum levels did not show statistically significant differences in juvenile idiopathic arthritis children with active joints compared to patients with no active joints and no association was found between plasma vaspin levels and presence or number of active joints (Cantarini et al., 2011).
Limited and inconsistent clinical data are available regarding the association between vaspin levels and cardiovascular disease. Serum vaspin levels were significantly decreased in patients with coronary artery disease respect to healthy controls (Kadoglou et al., 2011). Similarly, decreased serum vaspin concentrations were observed in patients suffering from coronary artery disease, with respect to age-matched normal subjects (Kobat et al., 2012). In contrast, a positive association of serum vaspin levels with carotid atherosclerosis was found, independently of insulin resistance, in the general population (Esaki et al., 2014). Recently, Hao et al. (2016) reported that concentrations of vaspin were associated with the presence of both coronary artery disease and T2DM. A more recent report showed that vaspin cannot be considered as a biochemical marker for diagnosis of coronary artery disease in the general population (Stančík et al., 2017). In contrast, recently vaspin has been found highly expressed in human macrophages and vascular smooth muscle cells in coronary atheromatous plaques (Sato et al., 2018). As for the potential mechanisms mediating the effects of vaspin in vascular endothelial cells, it has been hypothesized that this peptide might protect blood vessels through prevention of free fatty acid-induced apoptosis in human vascular endothelial cells via upregulation of the PI3K/Akt/eNOS signaling pathway (Jung et al., 2011; Zieger et al., 2018). Vaspin was found to inhibit ICAM-1 expression induced by TNF-α by preventing generation of reactive oxygen species and activating NF-κB/PKC theta within vascular smooth muscle cells (Phalitakul et al., 2011; Liu et al., 2014). More recently, vaspin was found to suppress migration and proliferation of vascular smooth muscle cells, through downregulating ERK1/2 and c-Jun N-terminal kinase (JNK) pathways, and increasing collagen production through upregulation of PI3K/Akt pathways (Sato et al., 2018). Unfortunately, further studies are needed in order to elucidate the role of vaspin as a biomarker and/or a therapeutic agent in atherosclerotic cardiovascular disease.
Omentin-1 and omentin-2 are fat depot specific secretory proteins and their gene expression was reported in visceral stromal vascular cells, but not fat cells (Yang et al., 2006; Rabe et al., 2008). In humans, omentin-1 and omentin-2 genes are located adjacent to each other in the 1q22–q23 chromosomal region, which is linked to T2DM in several populations (de Souza Batista et al., 2007). Omentin-1 is the most common isoform in human plasma (de Souza Batista et al., 2007). Expression of this isoform is predominantly found in human omental adipose tissue specifically in visceral but not subcutaneous tissue, slightly expressed in gut, lung, heart, and rarely reported in muscle and kidney (Fu et al., 2004). Omentin-1 showed antinflammatory properties and has been identified as a major visceral (omental) adipokine which plays important roles in glucose homeostasis, lipid metabolism, insulin resistance, and diabetes (Senthilkumar et al., 2018). Omentin increases insulin signal transduction through activation of Akt/PKB, modulating body fat distribution between visceral and subcutaneous fat depots (Yang et al., 2006).
However, an inverse correlation between serum levels of omentin-1 and obesity was reported (de Souza Batista et al., 2007). Omentin enhances the effect of insulin on glucose metabolism (de Souza Batista et al., 2007) and its circulating concentrations are elevated after weight loss (Moreno-Navarrete et al., 2010). Furthermore, omentin levels are positively correlated with adiponectin and high-density lipoprotein levels (de Souza Batista et al., 2007). Howewer, further studies are needed to define the physiological role of omentin in glucose metabolism, its target tissues, receptors, or relevant signal transduction pathways.
Although a positive relationship between excessive weight and increased risk of developing RA in autoantibody positive individuals has been suggested, probably by production of various bioactive peptides, including omentin, it is not entirely clear the role of this peptide in rheumatic diseases. In an explorative study, conducted by Maijer et al. (2015), it has been reported a positive association between serum omentin and increased inflammatory state in autoantibody positive subjects at risk of developing RA. On the other hand, a reduction of omentin-1 concentrations in SF omentin-1 levels was observed in chronic inflammatory RA with respects to OA patients (Senolt et al., 2010), and SF levels were negatively correlated with self-reported pain and physical disability in OA patients (Li Z. G. et al., 2012). An independent relationship was showed between omentin and MMP-3 levels in patients with mild but not severe RA (Robinson et al., 2017). To date, the role of omentin in rheumatic diseases is unclear.
It is well known that omentin-1 plays a pivotal role in vasodilatation, development of endothelial dysfunction, and arterial calcification (Tsuji et al., 2001; Yamawaki et al., 2010, 2011; Duan et al., 2011). Similarly to adiponectin, omentin-1 induces the activation of AMPK and endothelial nitric oxide synthase (Chen et al., 2003; Stejskal et al., 2003), playing a role in cellular energy homeostasis and vascular tone regulation. In addition, omentin-1 was found to be able to inhibit TNF-α production in vascular endothelial cells (Tan et al., 2010). In particular, omentin exerts a NO-mediated endothelium-dependent vasodilating effect in rat isolated vessels, in vitro (Yamawaki et al., 2010), probably related, at least in part, to a direct phosphorylation of endothelial nitric oxide synthase (Yamawaki et al., 2010). Omentin was also reported to induce revascularization and endothelial cell function through the stimulation of the Akt/eNOS signaling pathway (Maruyama et al., 2012). Antinflammatory effects of omentin, including suppression of inflammation induced by TNF-α and CRP in vascular endothelial cells, have been proposed to be involved in its vasoprotective effects, in humans (Tan et al., 2010; Yamawaki et al., 2011), and reduced concentrations of omentin in plasma have been proposed as a marker of endothelial dysfunction (Moreno-Navarrete et al., 2011). In addition, it has been observed that chronic omentin administration suppresses monocrotaline-induced pulmonary arterial hypertension in rats (Kazama et al., 2013). Moreover, our research group showed that subacute omentin-1 administration significantly decreased blood pressure and pulse pressure, in normotensive rats, probably through enhanced synthesis of NO in the vascular system, which, in turn, could be mediated by stimulated adiponectin and inhibited IL-6 gene expression in pericardial adipose tissue (Brunetti et al., 2014). Omentin-1 concentrations are inversely associated with markers of obesity, suggesting a possible role for omentin-1 in obesity related cardiovascular disease (de Souza Batista et al., 2007).
Follistatin-like 1 was first described in 1993 as TSC-36 (TGFB-stimulated clone 36) in a study designed to identify genes regulated by transforming growth factor beta (Shibanuma et al., 1993). Thereafter, it was reported as a preadipocyte/adipocyte-secreted protein with a possible implication in obesity and inflammation (Lehr et al., 2012; Fan et al., 2013). Overweight and mild obesity might be associated with increased FSTL1 levels; however, Horak et al. (2018) observed a significant decline in FSTL1 level in individuals suffering from severe obesity compared to non-obese subjects. The authors suggested that decreased FSTL1 concentrations observed in people with severe obesity result from a reduction of adipogenesis accompanied by a senescence of preadipocytes, increased adipocyte apoptosis, and epigenetic FSTL1 silencing (Horak et al., 2018). Furthermore, FSTL1 exerted pro-inflammatory effects in both 3T3-L1 adipocytes and RAW264.7 macrophages. In particular, recombinant FSTL1 increased mRNA levels of IL-6, TNF-α, and MCP-1, in a dose-dependent manner, and activated IKKβ-NFκB and JNK signaling pathways in both cell lines. In addition, the same authors demonstrated an impairment of insulin signaling induced by FSTL1, in 3T3-L1 adipocytes, as confirmed by the attenuation of phosphorylation of both Akt and IRS-1 following insulin treatment. These findings suggest a possible role for FSTL1 as a mediator of inflammatory response and insulin resistance in obesity (Fan et al., 2013).
Controversial data have been reported for FSTL1 with respect to its possible effects on inflammation in rheumatic diseases. In CIA model, FSTL1 promoted inflammation, while in anti-type II collagen antibody-induced arthritis (CAIA) model, it inhibited inflammation. Opposite results might due to differences in disease development in animal models or in the extensive post-transcriptional regulation of FSTL1 (Mattiotti et al., 2018). Interestingly, FSTL1 has been shown to trigger inflammatory responses in both immune and non-immune cells, inducing TNF-α, IL-1β, and IL-6 secretion, in in vitro studies (Miyamae et al., 2006). Moreover, recombinant FSTL1 stimulated secretion of IFN-γ by T cells (Clutter et al., 2009), and the pro-inflammatory actions of FSTL1 have been suggested to be mediated by activation of TLR4 signaling (Murakami et al., 2012). In vivo studies demonstrated a causative role of FSTL1 in inflammation (Miyamae et al., 2006; Clutter et al., 2009). Accordingly, adenovirus-mediated administration of FSTL1 to mice increased CIA (Miyamae et al., 2006), while its neutralization with specific antibody showed antinflammatory effects (Clutter et al., 2009).
In addition, significantly increased levels of FSTL1 in serum and its overexpression in synovial tissue were observed in patients with RA (Clutter et al., 2009; Li et al., 2011). However, FSTL1 overexpression could be a consequence of RA rather than its cause (Ehara et al., 2004).
Follistatin-like 1 has been reported to participate in modulating a number of biological processes, such as vascularization and immunomodulation and signaling pathways (Mattiotti et al., 2018). Its cardioprotective role has been intensively studied, though its mechanisms remain unclear. In multiple models of myocardial injury, the overexpression of FSTL1 has been demonstrated to act as a protective factor (Oshima et al., 2008). On the other hand, FSTL1 KO neonatal mice showed an overall enlargement of the heart (Sylva et al., 2011). In addition, decreased ischemic damage and improved cardiac performance after reperfusion were found following treatment with FSTL1, in preclinical model (Ogura et al., 2012). Intramuscular administration of an adenoviral vector expressing FSTL1 (Ad-FSTL1) induced a significant elevation of perfusion recovery and capillary vessel formation in the ischemic hind limbs of mice. In addition, Ad-FSTL1 enhanced migration and differentiation into vascular-like structures and suppressed apoptosis under conditions of serum deprivation, in cultured endothelial cells (Ouchi et al., 2008). The stimulatory effects of FSTL1 on endothelial cell function and revascularization following ischemic insult were associated with phosphorylation of Akt and stimulation of eNOS (Ouchi et al., 2008). A study of cardiac regeneration showed that FSTL1 induced various effects on cardiomyocyte proliferation and protection from apoptosis related to post-translational modification of the protein (Wei et al., 2015). However, patients with heart failure and acute coronary syndrome showed a significant increase of FSTL1 circulating concentrations (Widera et al., 2009; El-Armouche et al., 2011; Sylva et al., 2011; Tanaka et al., 2016). In this context, FSTL1 serum levels were correlated with mortality (Widera et al., 2009). Consequently, FSTL1 could have a negative prognostic value in these patients (El-Armouche et al., 2011; Widera et al., 2012).
Secreted protein acidic and rich in cysteine, also known as osteonectin or BM40, is a profibrotic protein that seems to have pleiotropic functions (Termine et al., 1981; Kos and Wilding, 2010). It exerts various functions, including modulation of the assembly and organization of ECM (Bornstein, 1995; Bradshaw, 2009), and regulation of a number of intracellular signaling pathways, in addition to affecting cell migration, proliferation, and differentiation (Chlenski and Cohn, 2010). In particular, it has been reported to bind various structural and soluble ECM proteins in a Ca2+-dependent manner, such as collagens (Sage et al., 1989; Sasaki et al., 1997; Chlenski et al., 2004; Kaufmann et al., 2004), vitronectin (Rosenblatt et al., 1997), fibrinogen fragments (Wang et al., 2006), thrombospondin-1 (Clezardin et al., 1988, 1991), VEGF (Kupprion et al., 1998), and platelet-derived growth factor (PDGF) (Raines et al., 1992). Although the functional role of this binding is not completely clear, SPARC has been proposed as an extracellular chaperone (Martinek et al., 2007). It was found that SPARC is secreted by adipocytes, inhibited adipogenesis, and promoted adipose tissue fibrosis (Kos et al., 2009; Lee et al., 2009; Kos and Wilding, 2010). In addition, studies suggested the involvement of SPARC in the pathogenesis of obesity and T2DM (Termine et al., 1981). Overexpression of SPARC modulates the expression levels of various pro-inflammatory cytokines, critically involved in insulin resistance, glucose and lipid metabolism during adipogenesis (Shen et al., 2014). Moreover, SPARC regulated thermogenesis in white and brown adipocytes, directly interacting with VEGF in adipocytes (Mukherjee et al., 2020). Recently, deletion of SPARC expression has been reported to cause diabetes mellitus in mice, demonstrating that SPARC deficient mice represent a reliable model for obesity and its metabolic complications, including diabetes mellitus (Atorrasagasti et al., 2019). On the other hand, clinical studies showed a link between increased SPARC levels and T2DM, diabetic retinopathy, and nephropathy (Munjal et al., 1994; Kanauchi et al., 2000; Taneda et al., 2003). A cross-sectional study on women with gestational diabetes mellitus showed higher SPARC and lower adiponectin levels as compared to normal glucose tolerance controls. Importantly, SPARC levels significantly correlated with inflammation and dyslipidemia, pointing to a potential involvement of SPARC in the pathophysiology of gestational diabetes mellitus (Xu et al., 2013). Approximately 20% of children with obesity exhibited the metabolically healthy obese (MHO) phenotype. A more favorable adipokine profile, characterized by decreased osteonectin, leptin, and RBP-4 and increased adiponectin levels was shown in MHO children with respect to metabolically unhealthy children suffering from obesity. In addition, higher levels of leptin, resistin, and RBP-4 paralleled by lower adiponectin, but similar osteonectin and FGF-21 levels, were reported in MHO children as compared to normal weight healthy controls. Therefore, a possible involvement of dysregulation of adipokines in the metabolic consequences of obesity in children can be suggested. It has been proposed that reduced concentrations of SPARC, paralleled by a healthy adipokine profile, might be used as an early marker of the MHO phenotype (Fu et al., 2018). Therefore, SPARC might represent a key player in the pathology of obesity and metabolic syndrome.
Secreted protein acidic and rich in cysteine could also be implicated in the pathogenesis of rheumatic diseases. Although widely expressed in mammalian tissues, the protein SPARC represents the major non-collagenous protein in bone (Bradshaw and Sage, 2001; Robey and Boskey, 2003). Transforming growth factor-1 β (TGF-1β) and IL-1β regulate the production of SPARC by chondrocytes at pre- and post-translational levels (Nakamura et al., 1996). In the absence of SPARC, mice develop progressive low turnover osteopenia. Trabecular bone volume is dramatically decreased, while cortical bone has compromised matrix quality (Delany et al., 2000; Boskey et al., 2003). SPARC-null mice showed decreased osteoblast and osteoclast, as well as reduced rate of bone formation. In addition, SPARC promotes osteoblastic commitment, differentiation, and survival, in vitro (Bradshaw et al., 2003; Delany et al., 2003; Robey and Boskey, 2003). Importantly, increased SPARC levels were reported in synovial fibroblasts from patients with RA or OA.
In the heart, SPARC is mainly expressed by fibroblasts and endothelial cells, and to a lesser extent by cardiac myocardial cells (Chen et al., 2004; Harris et al., 2011). In addition, it has been well documented that SPARC is also overexpressed in atherosclerotic vascular lesions (Myasoedova et al., 2018). SPARC secretion occurs upon myocardial injury and at sites of cardiac remodeling (hypertrophy and fibrosis). In this context, Schellings et al. (2009) showed a SPARC-induced improvement of cardiac function following MI via regulation of post-synthetic procollagen processing in mice. Recently, Deckx et al. (2019) showed a novel inotropic function of SPARC in the heart. In particular, extracellular SPARC was found to directly elevate cardiomyocyte contraction and cardiac function both in healthy myocardium and during cardiac dysfunction induced by coxsackie virus, in ex vivo and in vivo studies (Deckx et al., 2019). The authors suggested a possible therapeutic application of SPARC when myocyte contractile function is diminished such as in myocarditis-related cardiac injury. SPARC also increases collagen cross-linking via its cysteine-rich repeats, and acts as a cofactor for TGF-β receptor signaling, leading to increased cardiac fibrosis. SPARC affinity for collagen was reported to be increased after its proteolytic cleavage by several MMPs, such as MMP-2, -3, -7, and -13 (Maurer et al., 1997). SPARC also regulates the activity of multiple growth factors including fibroblast growth factor 2 (Chen and Frangogiannis, 2010), PDGF (Motamed et al., 2002), and TGF-β (Francki et al., 2004; Schellings et al., 2009), which are crucially involved in fibrosis. However, despite SPARC-induced positive effect in the acute phase of MI, detrimental effects in pressure overload have been suggested to be induced by the increased SPARC-mediated collagen cross-linking (Bradshaw et al., 2009) and aging (Bradshaw et al., 2010), as it causes left ventricular diastolic dysfunction. In this context, monocytes and macrophages play a pivotal role in tissue fibrosis. SPARC is also expressed by inflammatory cells derived from bone marrow, suggesting a role for SPARC produced by infiltrating leukocytes in inflammatory response and fibrosis in the heart (Bradshaw, 2016). Therefore, SPARC appears to play a critical role in cardiac function and could be considered a potential therapeutic target.
Secreted frizzled-related protein 5 is an antinflammatory adipokine secreted by WAT (Ouchi et al., 2010). It has been described as an endogenous blocker of WNT5A (wingless-type family member 5a signaling), an uncommon member of the WNT family proteins, involved in various cellular functions, such as inflammation, proliferation, differentiation, and migration (Kimura-Yoshida et al., 2005; Kim et al., 2010; Teo and Kahn, 2010; Neng et al., 2014). Although WNT5A was reported to be able to regulate cell proliferation and adipogenesis via MAPK, data on the relationships between WNT5A and SFRP5 in humans with obesity are limited and inconsistent (Hu W. et al., 2013; Lu et al., 2013; Miyoshi et al., 2014; Ji et al., 2017; Tang et al., 2018).
In 2012, Schulte and collaborators reported a significant increase of both WNT5A and SFRP5 concentrations in patients suffering from obesity compared with lean patients. In contrast, low plasma levels of SFRP5 have been detected in Chinese patients suffering from obesity (Hu Z. et al., 2013). Recently, Akoumianakis et al. (2019) found that circulating WNT5A concentrations were significantly higher in patients with obesity, paralleled by decreased plasma levels of SFRP5. Certainly, SFRP5 seems to play a critical role in obesity, as well as insulin resistance, and diabetes mellitus (Ouchi et al., 2010; Carstensen et al., 2013; Hu Z. et al., 2013; Cheng et al., 2015).
As regards to T2DM, it has been speculated that SFRP5 might prevent macrophage mediated inflammation of adipose tissue by antagonizing WNT5A protein with consequent improvement of insulin sensitivity. An important defensive function exerted by SFRP5 in the pathogenesis of T2DM has been described by Ouchi et al. (2010). In this context, mice deficient in SFRP5 (SFRP5−/−) fed a high-fat diet exhibited higher macrophage-mediated adipose tissue inflammation and insulin resistance respect to wild type mice due to uncontrolled activity of WNT5A. Conversely, in obese and diabetic mice, SFRP5 single dose administration enhanced metabolic function and reduced adipose tissue inflammation. The authors proposed that SFRP5 could neutralize the non-canonical activation of JNK implemented by WNT5a protein in macrophages and adipocytes through both paracrine and autocrine mechanisms (Ouchi et al., 2010).
Type 2 diabetes mellitus patients showed a decrease in plasma concentrations of SFRP5 which were unfavorably related to the evaluation of the homeostatic insulin resistance model, suggesting that SFRP5 could play a defensive action in the pathogenesis of T2DM (Hu Z. et al., 2013). By contrast, different studies have observed elevated SFRP5 plasma levels in T2DM patients (Lu et al., 2013; Canivell et al., 2015). Other studies are necessary to clarify the role of SFRP5 in obesity and metabolic syndrome.
Despite some data point to an involvement of WNT5A in the pathogenesis of RA, there is no clear evidence supporting a role of SFRP5 in rheumatic diseases. In particular, WNT5A enhanced gene expression of several cytokines that could be involved in RA progression (Blumenthal et al., 2006; Pereira et al., 2008; Halleskog et al., 2012; Rauner et al., 2012). In addition, higher WNT5A levels were detected in SF of patients with RA and OA (Sen et al., 2000) and its inhibition blocks the initiation of cultured synovial fibroblasts in patients with RA (Sen et al., 2001). As for SFRP5, it suppresses the inflammatory response in fibroblast-like synoviocytes of RA patients, inhibiting WNT signaling (Kwon et al., 2014).
In spite of WNT5A has been particularly investigated in the progression of atherosclerosis (Pashirzad et al., 2017; Tong et al., 2019), studies on the relationship between WNT5A and SFRP5 in cardiovascular diseases are limited. Raised expression of WNT5A was reported in murine and human atherosclerotic lesions (Christman et al., 2008; Malgor et al., 2014). Recently, it has been suggested that SFRP5 could restore WNT5A-induced endothelial dysfunction (Cho et al., 2018).
An antinflammatory and antiapoptotic action for SFRP5 was also detected in a mouse model of ischemia/reperfusion injury (Nakamura et al., 2016), supporting a protective role on myocardial damage. Treatment of cardiomyocytes with angiotensin II is used to stimulate SFRP5, brain natriuretic peptide, and TNF-α, which induce cardiac hypertrophy (Jin et al., 2015).
Recently, it has been investigated the relationship between serum level of SFRP5 and severity of coronary artery disease. The results highlighted that serum SFRP5 was decreased in unstable coronary artery disease patients as compared to stable coronary artery disease patients and control subjects. Moreover, serum SFRP5 levels were inversely related to the presence and complexity of coronary artery disease (Safoura and Gholam, 2018).
More recently, Du et al. (2019) showed that high serum SFRP5 levels measured during the acute phase of ST segment elevation MI (STEMI) were significantly linked to early-stage myocardial recovery following primary percutaneous coronary intervention, suggesting that SFRP5 might represent a possible therapeutic target in acute STEMI.
C1q/TNF-related proteins are a family of adipokines discovered in 2004 produced by different organs (Wong et al., 2004; Kopp et al., 2010b), and they have a structural similarity to adiponectin. CTRPs regulate energy homeostasis via adipoR1, adipoR2, and possibly other unknown receptors (Seldin et al., 2014). In particular, they modulate glucose and fat metabolism in peripheral tissues (Davis and Scherer, 2008; Wong et al., 2008; Peterson et al., 2010, 2012, 2014; Seldin et al., 2012, 2013; Wei et al., 2012, 2014; Petersen et al., 2017), food intake via a central mechanism (Byerly et al., 2013, 2014), inflammatory processes in adipose tissue (Enomoto et al., 2011), and adipocyte differentiation (Wei et al., 2013). On the other hand, much of the data regarding specific members are conflicting. Recently, preclinical and clinical studies have found that CTRP6 expression is upregulated in adipose tissue in both obesity and diabetic states (Lei et al., 2017). Stromal vascular cells and macrophages are among the main sources of CTRP6 (Kopp et al., 2010a). Increased expression of CTRP6 compromised glucose disposal of in peripheral tissues in mice. By contrast, deletion of the CTRP6 gene potentiated the effect of insulin and increased metabolism and energy expenditure in diet-induced obese mice (Lei et al., 2017). CTRP6 could regulate local inflammation and glucose metabolism by an action on macrophages and adipocytes. In particular, recombinant CTRP6 increased TNF-α expression and production in cultured macrophages, while decreased CTRP6 limited circulating inflammatory cytokines and pro-inflammatory macrophages in adipose tissue. In addition, CTRP6-overexpressing mice or CTRP6-treated adipocytes had decreased insulin-stimulated Akt phosphorylation and glucose uptake, while lack of CTRP6 improved Akt activation stimulated by insulin in adipose tissue. These effects suggest CTRP6 as a new metabolic/immune regulator linking obesity to inflammation and insulin resistance. Further complicating their role in obesity is that similar to adiponectin, CTRPs circulate in different isoforms that may contribute to their function in human diseases, but the current knowledge about the role of some CTRPs has not yet been fully elucidated.
In rheumatic disease, CTRP6 was found to regulate the activation of the complement system and its gene knock down worsens joint pathology in a mouse model of RA (Murayama et al., 2015). On the other hand, CTRP3 has also been recognized as a growth factor, promoting proliferation of chondrogenic precursors and chondrocytes (Maeda et al., 2006). In addition, it was reported that CTRP3 inhibits LPS-induced inflammatory cytokine production from human adipocytes, monocytes, and fibroblasts (Weigert et al., 2005; Kopp et al., 2010a; Hofmann et al., 2011), exerting an action in the development of CIA in mice (Murayama et al., 2014).
CTRP1 is primarily secreted by stromal vascular cells, including adipose tissue macrophages, preadipocytes, and endothelial cells (Innamorati et al., 2002). CTRPs display significant activities in myocardial protection and vasodilation (Schäffler and Buechler, 2012). In fact, in heart, as well as in other tissue such as placenta, muscle, kidneys, prostate, and ovaries, was detected CTRP1 mRNA expression (Wang et al., 2016). The first works on cardiovascular disease described it as a potent antithrombotic factor, able to prevent collagen-induced platelet aggregation (Lasser et al., 2006). Recent evidences demonstrate that increased CTRP1 serum levels are protective against acute ischemic injury in coronary artery disease (Yuasa et al., 2016). Additional studies suggested the involvement of CTRP3 in the pathogenesis of MI (Yi et al., 2012; Choi et al., 2014; Lin et al., 2014; Wu et al., 2015). Immediately after a MI, a significant reduction of CTRP3 mRNA in adipose tissue and circulating levels of CTRP3 protein occurs in mice (Yi et al., 2012; Wu et al., 2015). In particular, CTRP3 causes mitochondrial biogenesis in cardiac tissue and vascular relaxation (Zhang et al., 2016). Exogenous CTRP3 pretreatment (adenovirus-delivered or recombinant CTRP3) showed increased preservation and improved cardiac function in animal models of MI (Wurm et al., 2007; Wu et al., 2015). In addition, CTRP3 has been hypothesized to be involved in progressive remodeling after MI (Wurm et al., 2007; Wu et al., 2015). On the other hand, CTRP9 was also described as a novel vasodilating adipokine that may produce vasoprotective actions via adiponectin receptor 1/AMPK/eNOS signaling pathway (Zheng et al., 2011). Recently, the relationships between serum CTRP9 levels and adhesion molecules in patients with T2DM and coronary artery disease have been investigated. It was found an important correlation between serum CTRP9 levels and adhesion molecules in coronary artery disease and T2DM patients, similarly to serum TNF-α levels in patients with coronary artery disease. Elevated circulating levels of CTRP9 in T2DM and coronary artery disease patients suggest a compensatory response aiming to restore a functional balance to insulin resistance, the inflammatory environment, and endothelial dysfunction in these patients (Moradi et al., 2018).
Family with sequence similarity to 19 member A5, known as TAFA5 is part of the TAFA family, secreted proteins expressed in the brain (Tom Tang et al., 2004).
Recent reports showed that FAM19A5 is released by subcutaneous, brown, epididymal, and perirenal adipose tissues (Wang et al., 2018). Interestingly, FAM19A5 levels in human adipocytes were significantly downregulated by TNF-α-induced inflammation (Tourniaire et al., 2013) suggesting that pro-inflammatory cytokines produced during obesity may cause FAM19A5 downregulation. However, differential expression of FAM19A5 in lean humans and individuals suffering from obesity has not been reported yet, and FAM19A5 could be regarded either a biomarker or a potential drug target of obesity-related cardiovascular disease in humans (Zarzour et al., 2018). A recent experimental study conducted on 223 individuals revealed that serum FAM19A5 concentrations were significantly elevated in patients with T2DM compared with subjects without T2DM. The plasma concentrations of FAM19A5 were positively correlated with waist circumference, waist–hip ratio, alanine aminotransferase, fasting plasma glucose, glycated hemoglobin, and mean brachial-ankle pulse wave velocity. Therefore, FAM19A5 could represent a biomarker of cardio-metabolic disease (Lee et al., 2019).
Despite several reports have shown that FAM19A5 is involved in various biological functions (Paulsen et al., 2008; Shahapal et al., 2019), studies on rheumatic disease are limited. FAM19A5 strongly stimulated mouse bone marrow-derived macrophages, resulting in chemotactic migration and inhibition of RANKL-induced osteoclastogenesis. The suppressive effect on osteoclastogenesis induced by FAM19A5 was completely reversed by a formyl peptide receptor antagonist (FPR) 2 WRW4 or by deficiency of FPR2, pointing to an important role of FPR2 in the regulation of osteoclastogenesis (Park M. Y. et al., 2017). These results suggest that FAM19A5 and its target receptor FPR2 could negatively regulate osteoclastogenesis. However, further works are necessary to elucidate its role in rheumatic diseases.
It has been suggested that FAM19A5 might inhibit the proliferation and migration of smooth muscle cells (Wang et al., 2018). The authors also confirmed that FAM19A5 has been able to prevent post-injury neointima formation in rat carotid arteries and mouse femoral arteries in vivo (Wang et al., 2018). Given that FAM19A5 is expressed in human adipocytes and its mRNA expression and protein synthesis are downregulated by TNF-α (Tourniaire et al., 2013; Wang et al., 2018), it has been hypothesized that the production of FAM19A5 may be conditioned by cardio-metabolic disorders related to obesity in humans. Finally, Lee et al. (2019) suggested that FAM19A5 concentrations might reflect metabolic and cardiovascular status in humans. Further studies are needed to clarify its role in this disease.
Wingless-type inducible signaling pathway protein-1 (also known as CCN 4) is a component of the secreted protein associated with the ECM and a target gene of the WNT1 wingless pathway (Katoh and Katoh, 2007). WISP1 is released in various organs and tissues, such as brain, heart, pancreas, lung, intestine, kidney, ovaries, spleen, and displays anti-apoptotic effects through the PI3K and Akt pathways (Maiese et al., 2012). In addition, the signal transduction pathways of WISP1 are extensive, involving PI3K, PKB (Akt), mitogen MAPK, JNK, caspases, forkhead transcription factors, sirtuins, c-myc, glycogen synthase kinase-3β (GSK-3β), β-catenin, miRNAs, and the mechanistic target of rapamycin (mTOR) (Maiese, 2014). Murahovschi et al. (2015) showed that WISP1 is mainly found in visceral fat in patients with obesity and it is linked to markers of insulin resistance and inflammation in normal glucose tolerant patients. Murahovschi et al. (2015) showed that WISP1 is mainly found in visceral fat in patients with obesity and it is linked to markers of insulin resistance and inflammation in normal glucose tolerant patients. However, the specific function of WISP1 in the induction of insulin resistance is unclear. A study conducted on women with gestational diabetes and insulin resistance showed an increase in circulating WISP1 levels, which correlated with BMI and insulin resistance (Sahin Ersoy et al., 2016). More recently, it has been reported that WISP1 levels are increased in patients with obesity and are directly related to adiposity, regardless of glycemic status or insulin resistance; furthermore, they are highly correlated with increased plasma IL-8 (Barchetta et al., 2017). Ferrand et al. (2017) suggested that WISP1 could inhibit PPARγ activity. This evidence indicates that WISP1 acts as an unfavorable regulator of adipogenesis. On the other hand, a study published by Tacke et al. (2018) showed no differences in WISP1 levels among individuals with normal glucose tolerance, impaired glucose tolerance, and T2DM. However, a recent report suggested that WISP1 is strongly linked to the progression of insulin resistance, in particular higher circulating levels of WISP1 were found in patients suffering from obesity together with increased insulin resistance (Yaribeygi et al., 2019). Overall, WISP1 seems to play a critical role in many molecular pathways and cellular events; however, further studies are necessary to further deepen its involvement in obesity and metabolic disfunction.
Wingless-type inducible signaling pathway protein-1 plays a modulatory function on skeletal growth and bone repair (Yanagita et al., 2007). In particular, it modulates mesenchymal proliferation and osteoblastic differentiation and chondrogenic differentiation (French et al., 2004). Blom et al. (2009) have shown that the synthesis of WISP-1 was significantly intensified in the synovium and cartilage of mice with experimental OA, as well as in human OA cartilage and synovium. WISP1 regulates the expression of MMP and chondrocyte and macrophage aggrecanases with subsequent joint cartilage injury, in OA animal models (Blom et al., 2009). In addition, WISP-1 is involved in the regulation of osteogenesis through bone morphogenetic protein 2 (Ono et al., 2011). Considering its role in the control of cellular proliferation in the musculoskeletal system, WISP1 has been hypothesized as a potential therapeutic target in rheumatic diseases. On the other hand, Li H. H. et al. (2012) have linked WISP1 to the progression of inflammation, where recombinant WISP1 aggravates pro-inflammatory responses in LPS stimulated macrophages.
In this context, WISP1 is considered an important factor for the appearance of OA. In synovial fibroblasts of OA, WISP1 can stimulate the integrins αvβ5, PI3K, PKB (Akt), and NF-κB which drive upregulation of IL-6 production (Hou et al., 2013). In addition, WISP1 promoted chondrocyte hypertrophy through TGF-β signaling and activin-like kinase (ALK)1/Smad 1/5/8 pathway (van den Bosch et al., 2014). The detrimental effects of WISP1 in arthritic disease could be related to its role in promoting fibrosis (Berschneider et al., 2014; Jian et al., 2014).
In symptomatic humans suffering from OA with disease progression, higher WISP1 synovial expression was noted respect to non-progressing patients. In particular, WISP1 expression has been inversely related to the methylation levels of a positional CpG-dinucleotide (cg10191240), with damaged areas showing a significant hypomethylation for this CpG compared to preserved cartilage. Furthermore, it was noted that methylation levels were allele-dependent for a single intron nucleotide polymorphism near cg10191240. These results indicate that an increase in WISP1 is harmful to cartilage integrity in patients with OA (van den Bosch et al., 2019). The pathogenic role of WISP1 in OA has been also confirmed in Wisp1–/– mice (van den Bosch et al., 2017).
In regards to cardiovascular diseases, various studies confirmed WISP1 positive effects on vascular and cardiovascular repair (Price et al., 2004; Reddy et al., 2011; Liu et al., 2013). It has been observed that WISP1 was upregulated after MI in animal models, and recombinant WISP1 was able to promote cell survival and collagen secretion in isolated myocytes and fibroblasts (Colston et al., 2007). WISP1 might regulate cardiac endothelial signaling and contribute to beneficial effects of post-MI treatments, as described by Wright et al. (2018). On the other hand, WISP1 seems able to rescue cardiomyocytes from doxorubicin toxicity (Lahoti et al., 2012), and prevent cardiomyocyte cell death through PI3K, Akt, and survivin (Venkatesan et al., 2010).
Other adipokines, such as PRGN (also known as granulin/epithelin precursor), nesfatin-1 (nesfatin), visfatin/PBEF/NAMPT, apelin, RBP-4, and PAI-1 have been described as signal molecules involved in obesity and metabolic syndrome.
PROGRANULIN is a glycoprotein of approximately 75–80 kDa which is composed of seven granulin/epithelin repeats (granulins) (Wei et al., 2016). It can undergo enzymatic proteolysis into small homologous subunits. Both full-length protein and its constituent granulin peptides are biologically active with anti- and pro-inflammatory actions, respectively (Wei et al., 2016). PGRN is secreted by a broad spectrum of cells, including adipocytes, macrophages, and chondrocytes (Abella et al., 2017a). PGRN has been described as an adipokine with antinflammatory properties mediated by its competitive binding to TNF-α receptors (TNFR1 and TNFR2) (Abella et al., 2016, 2017b). Elevated PGRN expression in visceral adipose tissue and circulating PGRN concentrations are associated with visceral obesity, type 2 diabetes, and dyslipidemia (Youn et al., 2009; Qu et al., 2013; Shafaei et al., 2016). In particular, hyperprogranulinemia could be involved in the pathogenesis of obesity-associated insulin resistance, impairing insulin signaling and insulin-induced glucose uptake both in vitro and in vivo, whereas PGRN deficiency could protect from high fat diet-induced insulin resistance (Qu et al., 2013; Korolczuk and Bełtowski, 2017). In addition, different studies have demonstrated a link between hyperprogranulinemia, type 2 diabetes, and its renal, retinal, and microangiopathic complications (Xu et al., 2015; Albeltagy et al., 2019). Recent studies demonstrated that the loss of PGRN expression in vivo results in hypersusceptibility to collagen-induced arthritis, which can be reversed by the administration of recombinant PGRN (Tang et al., 2011). TNF-transgenic (TNF-Tg) mice with deletion of one or both alleles of PGRN exhibited significantly increased synovitis, pannus formation, destruction of the ankle joints, and loss of cartilage matrix (Tang et al., 2011). These data suggest that PGRN exerts its antinflammatory effects through inhibition of TNF/TNFR signaling in vivo. However, clinical studies have reported elevated PGRN levels in cartilage, SF, as well as serum from OA and RA patients (Cerezo et al., 2015; Abella et al., 2016), with elevated circulating PGRN levels significantly correlated to disease activity in RA patients (Yamamoto et al., 2014). Interestingly, the balance between PGRN and TNF seems to play a key role in RA progression, as the ratio PGRN/TNF is closely correlated with RA stages (Yamamoto et al., 2014). PGRN could be considered as a relatively new player in the pathogenesis of atherosclerosis. In this context, data suggest that PGRN might exert a role in the cross-talk of immunity and lipid turnover (Okura et al., 2010; de Jager and Pasterkamp, 2013). PGRN is also expressed by endothelial and T cells (Kawase et al., 2013; Abella et al., 2017a). In particular, PGRN improves tissue repair by active recruitment of leukocytes and increasing capillary perfusion after acute injury (Jian et al., 2012). It was found that PGRN mildly inhibits MCP-1-mediated monocyte migration, while it augments TNFα-mediated human aortic smooth muscle cell migration (Kojima et al., 2009). Additionally, PGRN inhibits TNFα-induced IL-8 release. It has been recently established that PGRN might have prognostic value in subclinical atherosclerosis (carotid artery intima media thickening), independent of other cardiovascular risk factors (Yoo et al., 2013).
NESFATIN-1 (nesfatin) is an N-terminal 82-amino-acid peptide nucleobindin-2-derived adipokine, involved in satiety induction and energy homeostasis. Nesfatin was first described as an anorexigenic molecule secreted by the hypothalamus (Oh et al., 2006), subcutaneous adipose tissue, gastric mucosa, pancreatic cells, and testes (Ayada et al., 2015). To date, its mechanism of action is not clarified. Central and peripheral nesfatin-1 injections inhibit food intake, in rodents (Cowley and Grove, 2006; Stengel and Taché, 2013), and a lack of nesfatin-1 in the brain leads to an increase of appetite and body fat and weight (Shimizu et al., 2009). Nesfatin-1-induced inhibition of feeding could be mediated through the inhibition of orexigenic neurons (García-Galiano et al., 2010; Stengel and Taché, 2010). In addition, nefastin-1 was found to regulate the activity of gastric distention-sensitive neurons and gastric motility via the melanocortin pathway in the central nucleus of amygdala (Wang et al., 2014). Various investigators suggested a possible role of nesfatin-1 in metabolic syndrome and T2DM (Gonzalez et al., 2011). Nesfatin-1 plays a role in obesity, hypertension, and diabetes through either the CNS or the peripheral sympathetic nervous system. The effects of nesfatin-1 on sympathetic nervous system activity could be a target for new anti-obesity drugs in leptin resistant people suffering from obesity (Masuo, 2014). In relation to rheumatic diseases, a study conducted by Kvlividze et al. (2019) demonstrated a positive association between blood serum nesfatin-1 levels and CRP in RA patients. Further studies showed a relationship between the concentration of nesfatin-1 and the severity of clinical manifestations of this disease, pointing to a pro-inflammatory effect of nesfatin-1 in the pathogenesis of RA. In addition, nesfatin-1 levels correlated with MMP-2 (Robinson et al., 2018). Nesfatin-1 has been detected in human and murine chondrocytes, where it was found to induce pro-inflammatory mediators such as COX-2, IL-8, IL-6, and CCL3 in chondrocytes from OA patients (Scotece et al., 2014a). Higher nesfatin-1 levels have also been detected in serum from OA patients compared to controls (Jiang et al., 2013). Moreover, serum and SF nesfatin-1 concentrations have been associated with radiographic severity of OA (Zhang et al., 2015), where its levels also correlated with CRP and IL-18 in serum and SF, respectively. In contrast to rheumatic desease, nesfatin-1 was described as an antinflammatory adipokine (Tang et al., 2012; Scotece et al., 2014a) which decrease cardiovascular risk (Dai et al., 2013; Ding et al., 2015). A few available studies show that this peptide affects energy metabolism of murine and human cardiomyocytes, by eliciting insulin-like effects. In rat heart, nesfatin-1 directly depresses contractility and relaxation via cGMP, PKG, and ERK1/2, and limits ischemia/reperfusion damage, displaying a post-conditioning protection. Nesfatin-1 actions are proposed to involve an unknown G-protein coupled receptor (Ramesh et al., 2017). However, in the rat heart, functional studies suggest an interaction with natriuretic peptide receptor-type A. These data open up novel perspectives to clarify not only the biological significance of nesfatin-1, but also its therapeutic potential in metabolic dependent cardiovascular diseases (Imbrogno et al., 2015). In addition, it has been observed that central and peripheral nesfatin-1 administration induces blood pressure elevation, probably mediated by α- and β-adrenergic receptors, respectively (Shimizu et al., 2009; Osaki and Shimizu, 2013). The authors speculated that nesfatin-1 might have a direct action on vascular smooth muscle, without involving the hypothalamic area (Shimizu et al., 2009; Osaki and Shimizu, 2013). However, recent reports suggest that nesfatin-1 may cause an increase in blood pressure in normotensive and hypotensive rats by increasing catecholamine, as well as vasopressin and renin levels (Aydin, 2013a,b). Finally, a recent study aimed to determine whether central cholinergic system may have a functional role in nesfatin-1-induced cardiovascular effect, in normotensive rats (Aydin et al., 2018).
A meta-analysis identified a significant increase of visfatin in patients diagnosed with overweight/obesity, T2DM, metabolic syndrome, and cardiovascular diseases (Chang et al., 2011). Additionally, it induces insulin resistance in many tissues and causes pancreatic beta cells dysfunction at later stages (Kumari and Yadav, 2018). These studies suggest that visfatin/PBEF/NAMPT could represent an important target to develop new drugs for metabolic and inflammatory diseases. In rheumatic diseases, visfatin/PBEF/NAMPT has been described as primarily a pro-inflammatory peptide (Meier et al., 2012; Neumann et al., 2016). In this context, visfatin/PBEF/NAMPT was found to be strongly related to various risk factors of both OA and OP, including obesity and diabetes mellitus. Interestingly, visfatin/PBEF/NAMPT has also been linked to several OA and OP pathologic features (Franco-Trepat et al., 2019). Visfatin/PBEF/NAMPT is a key factor for the progression of atherothrombotic disease and plaque destabilization by activating MMPs, promoting oxidation of lipoproteins by phospholipases, stimulating the release of acute phase proteins and pro-inflammatory cytokines (Anfossi et al., 2010). Moreover, visfatin/PBEF/NAMPT induces proliferation of vascular smooth muscle cells and fibroblasts, and plays a role in myocardial fibrosis and cardiac remodeling, in capillary tube neoformation mediated by the VEGF (Xiao et al., 2009).
APELIN has also been found in different tissues, such as brain, lung, kidney, bloodstream, and adipocytes (Lee et al., 2000; Kawamata et al., 2001; Boucher et al., 2005). Various studies confirmed that apelin plays a key role in energy metabolism and pathophysiology of obesity (Schinzari et al., 2017; Bertrand et al., 2018). Moreover, increased plasma apelin levels were found in individuals with obesity (Heinonen et al., 2005) as well as in those with type 2 diabetes (Li et al., 2006). A potential involvement of apelin in the pathogenesis of rheumatic diseases has been suggested, as confirmed by findings showing lower levels of this peptide in early state RA patients compared to healthy controls (Di Franco et al., 2012). More recently, apelin concentrations correlated positively with MMP-2 and inversely MMP-9, in RA patients (Gunter et al., 2017). Apelin was found to stimulate proliferation of chondrocytes and increase MMP-1, MMP-3, MMP-9, and IL-1β expression, in vitro. In agreement, increased expression of MMP-3, MMP-9, IL-1β, ADAMTS-4, and ADAMTS-5, as well as decreased level of collagen II were found following intra-articular injections of apelin, in rats. Proteoglycan in articular cartilage was also depleted following apelin treatment (Hu et al., 2010). A role for apelin in bone metabolism was also hypothesized, as confirmed by previous findings showing that apelin stimulated proliferation of osteoblasts through the APJ/PI3k/Akt pathway and exerted protective effects against apoptosis (Tang et al., 2007). On the other hand, increased apelin levels were found in both serum and SF of OA patients compared to healthy individuals (Hu et al., 2011). Both apelin and its receptor are expressed in vascular smooth muscle, endothelial, and myocardial cells (Maguire et al., 2009) and play key roles in regulation of cardiovascular functions (Yu et al., 2014). Also, plasma apelin concentrations are markedly reduced in patients with severe chronic heart failure (Chong et al., 2006). Apelin was also hypothesized to be critically involved in myocardial response to infarction and ischemia. In summary, clinical and experimental studies support a role for apelin system in cardiovascular diseases, making it an attractive therapeutic target.
A relevant number of studies suggested an important link between RBP4 and adiposity (Aeberli et al., 2007; Haider et al., 2007; Jia et al., 2007; Klöting et al., 2007), insulin resistance (Gavi et al., 2007; Klöting et al., 2007; Perseghin et al., 2007; Stefan et al., 2007; Möhlig et al., 2008), type 2 diabetes (Cho et al., 2006; Takebayashi et al., 2007), and the metabolic syndrome (Qi et al., 2007). The effects of RBP4 could be mediated by retinoic acid receptors (RARs), retinoic acid-X receptors (RXRs), or receptors such as Megalin/gp320. Moreover, cAMP-mediated pathways, PPARγ and PPARα were found to be deeply involved in the regulation of RBP4 gene expression in BAT (Rosell et al., 2012). However, its metabolic effects on glucose metabolism in humans remain uncertain (von Eynatten and Humpert, 2008). In addition, Wei et al. (2019) demonstrated that elevated RBP4 serum levels were associated with increased risk of insulin resistance in patients with early and untreated AR. In patients with obesity, RBP4 could play a pivotal role in atherosclerotic process; however, the link between RBP4 and lipid profile needs further extensive research. To date, it is difficult to determine if RBP4 could constitute a novel biomarker useful in CVD (Rychter et al., 2020). At the time of this review, there is insufficient data regarding the contribution to CVD and RA.
One other adipokine involved in obesity is PAI-1, a member of the serine protease inhibitor family. Various reports showed the complicated interaction between PAI-1, obesity, and metabolic disorders (Giltay et al., 1998). In particular, different studies demonstrated increased PAI-1 levels in subjects with obesity, metabolic syndrome, and T2DM (Vague et al., 1986; Landin et al., 1990; Juhan-Vague et al., 2003; Bilgili et al., 2008). Various reports described elevated PAI-1 levels in patients with systemic lupus erythematosus (SLE) and RA. In addition, individuals with SLE and RA showed a marked susceptibility in response to polymorphisms of PAI-1 (Angles-Cano et al., 1979; Glas-Greenwalt et al., 1984; Violi et al., 1990). However, more recently Bae and Lee (2020) demonstrated significantly increased circulating PAI-1 levels in patients with SLE but not in those with RA. On other hand, no association between PAI-1 4G/5G polymorphism and susceptibility to SLE and RA have been reported (Bae and Lee, 2020). In view of these results, it is clear that further studies are warranted to elucidate the direct contribution of PAI-1 in the pathogenesis of SLE and RA. Conversely, the connection between PAI-1 and cardiovascular events is well known. In particular, elevated plasma PAI-1 concentrations were found in both blood and coronary plaques of metabolic syndrome patients (Zorio et al., 2008). Similarly, elevated plasma PAI-1 levels have been associated with impaired fibrinolytic activity in stroke and CVD (Cesari et al., 2010; Jung et al., 2018).
Experimental and clinical evidence links adipokine actions with obesity, metabolic dysfunction, rheumatic, and cardiovascular diseases. Considering the pleiotropic actions of these molecules and their metabolic, immunomodulatory, and miscellaneous effects both locally and systemically, the study of their function in the pathogenesis of these diseases is very complicated. Discordant results have been observed for many adipokines, with both pro- and antinflammatory effects reported for the same molecule, evidencing that their biological actions could be strictly dependent on the inflammatory context and disease status. Further research is needed to define the contribution of the adipokine network in these disorders, with the aim of identifying the adipokines with potential diagnostic and/or prognostic significance in managing obesity-associated diseases, such as metabolic dysfunction, rheumatic, and cardiovascular diseases.
LR, GO, LB, and SL contributed to the design and wrote the manuscript in consultation with CF and AC. All authors critically reviewed the manuscript.
The authors declare that the research was conducted in the absence of any commercial or financial relationships that could be construed as a potential conflict of interest.
Abe, Y., Ono, K., Kawamura, T., Wada, H., Kita, T., Shimatsu, A., et al. (2007). Leptin induces elongation of cardiac myocytes and causes eccentric left ventricular dilatation with compensation. Am. J. Physiol. Heart Circ. Physiol. 292, H2387–H2396.
Abella, V., Pino, J., Scotece, M., Conde, J., Lago, F., Gonzalez-Gay, M. A., et al. (2017a). Progranulin as a biomarker and potential therapeutic agent. Drug Discov. Today 22, 1557–1564. doi: 10.1016/j.drudis.2017.06.006
Abella, V., Scotece, M., Conde, J., Gómez, R., Lois, A., Pino, J., et al. (2015). The potential of lipocalin-2/NGAL as biomarker for inflammatory and metabolic diseases. Biomarkers 20, 565–571. doi: 10.3109/1354750x.2015.1123354
Abella, V., Scotece, M., Conde, J., Lopez, V., Pirozzi, C., Pino, J., et al. (2016). The novel adipokine progranulin counteracts IL-1 and TLR4-driven inflammatory response in human and murine chondrocytes via TNFR1. Sci. Rep. 6:20356.
Abella, V., Scotece, M., Conde, J., Pino, J., Gonzalez-Gay, M. A., Gomez-Reino, J. J., et al. (2017b). Leptin in the interplay of inflammation, metabolism and immune system disorders. Nat. Rev. Rheumatol. 13, 100–109. doi: 10.1038/nrrheum.2016.209
Abizaid, A., Gao, Q., and Horvath, T. L. (2006). Thoughts for food: brain mechanisms and peripheral energy balance. Neuron 51, 691–702. doi: 10.1016/j.neuron.2006.08.025
Achari, A. E., and Jain, S. K. (2017). Adiponectin, a therapeutic target for obesity, diabetes, and endothelial dysfunction. Int. J. Mol. Sci. 18:1321. doi: 10.3390/ijms18061321
Adiarto, S., Emoto, N., Iwasa, N., and Yokoyama, M. (2007). Obesity-induced upregulation of myocardial endothelin-1 expression is mediated by leptin. Biochem. Biophys. Res. Commun. 353, 623–627. doi: 10.1016/j.bbrc.2006.12.066
Aeberli, I., Biebinger, R., Lehmann, R., L’allemand, D., Spinas, G. A., and Zimmermann, M. B. (2007). Serum retinol-binding protein 4 concentration and its ratio to serum retinol are associated with obesity and metabolic syndrome components in children. J. Clin. Endocrinol. Metab. 92, 4359–4365. doi: 10.1210/jc.2007-0468
Ahima, R. S., Kelly, J., Elmquist, J. K., and Flier, J. S. (1999). Distinct physiologic and neuronal responses to decreased leptin and mild hyperleptinemia. Endocrinology 140, 4923–4931. doi: 10.1210/endo.140.11.7105
Ahima, R. S., Saper, C. B., Flier, J. S., and Elmquist, J. K. (2000). Leptin regulation of neuroendocrine systems. Front. Neuroendocrinol. 21:263–307. doi: 10.1006/frne.2000.0197
Ahmed, H. H., Shousha, W. G., El-Mezayen, H. A., Emara, I. A., and Hassan, M. E. (2020). New biomarkers as prognostic factors for cardiovascular complications in type 2 diabetic patients. Indian J. Clin. Biochem. 35, 54–62. doi: 10.1007/s12291-018-0784-4
Aigner, F., Maier, H. T., Schwelberger, H. G., Wallnöfer, E. A., Amberger, A., Obrist, P., et al. (2007). Lipocalin-2 regulates the inflammatory response during ischemia and reperfusion of the transplanted heart. Am. J. Transplant. 7, 779–788. doi: 10.1111/j.1600-6143.2006.01723.x
Akoumianakis, I., Sanna, F., Margaritis, M., Badi, I., Akawi, N., Herdaman, L., et al. (2019). Adipose tissue-derived WNT5A regulates vascular redox signaling in obesity via USP17/RAC1-mediated activation of NADPH oxidases. Sci. Transl. Med. 11:eaav5055. doi: 10.1126/scitranslmed.aav5055
Al-Amodi, H. S., Abdelbasit, N. A., Fatani, S. H., Babakr, A. T., and Mukhtar, M. M. (2018). The effect of obesity and components of metabolic syndrome on leptin levels in Saudi women. Diabetes Metab. Syndr. 12, 357–364. doi: 10.1016/j.dsx.2017.12.030
Albeltagy, E. S., Hammour, A. E., and Albeltagy, S. A. (2019). Potential value of serum progranulin as a biomarker for the presence and severity of micro vascular complications among Egyptian patients with type 2 diabetes mellitus. J. Diabetes Metab. Disord. 18, 217–228. doi: 10.1007/s40200-019-00406-1
Al-Harithy, R. N., and Al-Ghamdi, S. (2005). Serum resistin, adiposity and insulin resistance in Saudi women with type 2 diabetes mellitus. Ann. Saudi Med. 25, 283–287. doi: 10.5144/0256-4947.2005.283
Anfossi, G., Russo, I., Doronzo, G., Pomero, A., and Trovati, M. (2010). Adipocytokines in atherothrombosis: focus on platelets and vascular smooth muscle cells. Mediators Inflamm. 2010:174341.
Angles-Cano, E., Sultan, Y., and Clauvel, J. P. (1979). Predisposing factors to thrombosis in systemic lupus erythematosus: possible relation to endothelial cell damage. J. Lab. Clin. Med. 94, 312–323.
Antuna-Puente, B., Feve, B., Fellahi, S., and Bastard, J. P. (2008). Adipokines: the missing link between insulin resistance and obesity. Diabetes Metab. 34, 2–11. doi: 10.1016/j.diabet.2007.09.004
Arita, Y., Kihara, S., Ouchi, N., Takahashi, M., Maeda, K., Miyagawa, J., et al. (1999). Paradoxical decrease of an adipose-specific protein, adiponectin, in obesity. Biochem. Biophys. Res. Commun. 425, 560–564. doi: 10.1016/j.bbrc.2012.08.024
Astapova, O., and Leff, T. (2012). Adiponectin and PPARγ: cooperative and interdependent actions of two key regulators of metabolism. Vitam. Horm. 90, 143–162.
Atorrasagasti, C., Onorato, A., Gimeno, M. L., Andreone, L., Garcia, M., Malvicini, M., et al. (2019). SPARC is required for the maintenance of glucose homeostasis and insulin secretion in mice. Clin. Sci. 133, 351–365. doi: 10.1042/cs20180714
Ayada, C., Toru, U., and Korkut, Y. (2015). Nesfatin-1 and its effects on different systems. Hippokratia 19, 4–10.
Aydin, B., Guvenc, G., Altinbas, B., Niaz, N., and Yalcin, M. (2018). Modulation of nesfatin-1-induced cardiovascular effects by the central cholinergic system. Neuropeptides 70, 9–15. doi: 10.1016/j.npep.2018.05.001
Aydin, S. (2013a). Multi-functional peptide hormone NUCB2/nesfatin-1. Endocrine 44, 312–325. doi: 10.1007/s12020-013-9923-0
Aydin, S. (2013b). Role of NUCB2/nesfatin-1 as a possible biomarker. Curr. Pharm. Des. 19, 6986–6992. doi: 10.2174/138161281939131127143422
Bae, S. C., and Lee, Y. H. (2020). Association between plasminogen activator inhibitor-1 (PAI-1) 4G/5G polymorphism and circulating PAI-1 level in systemic lupus erythematosus and rheumatoid arthritis. Z. Rheumatol. 79, 312–318. doi: 10.1007/s00393-019-00689-y
Bao, J. P., Jiang, L. F., Chen, W. P., Hu, P. F., and Wu, L. D. (2014). Expression of vaspin in the joint and the levels in the serum and synovial fluid of patients with osteoarthritis. Int. J. Clin. Exp. Med. 7, 3447–3453.
Barchetta, I., Cimini, A. F., Capoccia, D., De Gioannis, R., Porzia, A., Mainiero, F., et al. (2017). WISP1 Is a marker of systemic and adipose tissue inflammation in dysmetabolic subjects with or without type 2 diabetes. J. Endocr. Soc. 1, 660–670. doi: 10.1210/js.2017-00108
Bates, S. H., Stearns, W. H., Dundon, T. A., Schuber, M., Tso, A. W. K., Banks, A. S., et al. (2003). Stat3 signalling is required for leptin regulation of energy balance but not reproduction. Nature 421, 856–859. doi: 10.1038/nature01388
Batun-Garrido, J. A. J., Salas-Magana, M., and Juarez-Rojop, I. E. (2018). Association between leptin and IL-6 concentrations with cardiovascular risk in patients with rheumatoid arthritis. Clin. Rheumatol. 37, 631–637. doi: 10.1007/s10067-017-3897-x
Beltowski, J. (2006). Role of leptin in blood pressure regulation and arterial hypertension. J. Hypertens. 24, 789–801. doi: 10.1097/01.hjh.0000222743.06584.66
Berg, A. H., Combs, T. P., Du, X., Brownlee, M., and Scherer, P. E. (2001). The adipocyte-secreted protein Acrp30 enhances hepatic insulin action. Nat. Med. 7, 947–953. doi: 10.1038/90992
Berg, V., Sveinbjornsson, B., Bendiksen, S., Brox, J., Meknas, K., and Figenschau, Y. (2010). Human articular chondrocytes express ChemR23 and chemerin; ChemR23 promotes inflammatory signalling upon binding the ligand chemerin (21-157). Arthritis Res. Ther. 12:R228.
Berglund, E. D., Vianna, C. R. Jr., Donato, J., Kim, M. H., Chuang, J. C., Lee, C. E., et al. (2012). Direct leptin action on POMC neurons regulates glucose homeostasis and hepatic insulin sensitivity in mice. J. Clin. Invest. 122, 1000–1009. doi: 10.1172/jci59816
Berschneider, B., Ellwanger, D. C., Baarsma, H. A., Thiel, C., Shimbori, C., White, E. S., et al. (2014). miR-92a regulates TGF-β1-induced WISP1 expression in pulmonary fibrosis. Int. J. Biochem. Cell Biol. 53, 432–441. doi: 10.1016/j.biocel.2014.06.011
Bertrand, C., Pradère, J. P., Geoffre, N., Deleruyelle, S., Masri, B., Personnaz, J., et al. (2018). Chronic apelin treatment improves hepatic lipid metabolism in obese and insulin-resistant mice by an indirect mechanism. Endocrine 60, 112–121. doi: 10.1007/s12020-018-1536-1
Bilgili, S., Celebiler, A. C., Dogan, A., and Karaca, B. (2008). Inverse relationship between adiponectin and plasminogen activator inhibitor-1 in metabolic syndrome patients. Endoc. Regulat. 42, 63–68.
Blom, A. B., Brockbank, S. M., van Lent, P. L., van Beuningen, H. M., Geurts, J., Takahashi, N., et al. (2009). Involvement of the Wnt signaling pathway in experimental and human osteoarthritis: prominent role of Wnt-induced signaling protein 1. Arthritis Rheum. 60, 501–512. doi: 10.1002/art.24247
Blumenthal, A., Ehlers, S., Lauber, J., Buer, J., Lange, C., Goldmann, T., et al. (2006). The wingless homolog WNT5A and its receptor Frizzled-5 regulate inflammatory responses of human mononuclear cells induced by microbial stimulation. Blood 108, 965–973. doi: 10.1182/blood-2005-12-5046
Bokarewa, M., Nagaev, I., Dahlberg, L., Smith, U., and Tarkowski, A. (2005). Resistin, an adipokine with potent proinflammatory properties. J. Immunol. 174, 5789–5795. doi: 10.4049/jimmunol.174.9.5789
Bornstein, P. (1995). Diversity of function is inherent in matricellular proteins: an appraisal of thrombospondin 1. J. Cell. Biol. 130, 503–506. doi: 10.1083/jcb.130.3.503
Borregaard, N., and Cowland, J. B. (2006). Neutrophil gelatinase-associated lipocalin, a siderophore-binding eukaryotic protein. Biometals 19, 211–215. doi: 10.1007/s10534-005-3251-7
Boskey, A. L., Moore, D. J., Amling, M., Canalis, E., and Delany, A. M. (2003). Infrared analysis of the mineral and matrix in bones of osteonectin-null mice and their wild type controls. J. Bone Miner. Res. 18, 1005–1011. doi: 10.1359/jbmr.2003.18.6.1005
Boucher, J., Masri, B., Daviaud, D., Gesta, S., Guigne’, C., Mazzucotelli, A., et al. (2005). Apelin, a newly identified adipokine up-regulated by insulin and obesity. Endocrinology 146, 1764–1771. doi: 10.1210/en.2004-1427
Bozaoglu, K., Bolton, K., McMillan, J., Zimmet, P., Jowett, J., Collier, G., et al. (2007). Chemerin is a novel adipokine associated with obesity and metabolic syndrome. Endocrinology 148, 4687–4694. doi: 10.1210/en.2007-0175
Bradshaw, A. D. (2009). The role of SPARC in extracellular matrix assembly. J. Cell Commun. Signal. 3, 239–246. doi: 10.1007/s12079-009-0062-6
Bradshaw, A. D. (2016). The role of secreted protein acidic and rich in cysteine (SPARC) in cardiac repair and fibrosis: does expression of SPARC by macrophages influence outcomes? J. Mol. Cell. Cardiol. 93, 156–161. doi: 10.1016/j.yjmcc.2015.11.014
Bradshaw, A. D., Baicu, C. F., Rentz, T. J., Van Laer, A. O., Boggs, J., Lacy, J. M., et al. (2009). Pressure overload-induced alterations in fibrillar collagen content and myocardial diastolic function: role of secreted protein acidic and rich in cysteine (SPARC) in post-synthetic procollagen processing. Circulation 119, 269–280.
Bradshaw, A. D., Baicu, C. F., Rentz, T. J., Van Laer, A. O., Bonnema, D. D., and Zile, M. R. (2010). Age-dependent alterations in fibrillar collagen content and myocardial diastolic function: role of SPARC in post-synthetic procollagen processing. Am. J. Physiol. Heart Circ. Physiol. 298, H614– H622.
Bradshaw, A. D., Graves, D. C., Motamed, K., and Sage, E. H. (2003). SPARC-null mice exhibit increased adiposity without significant differences in overall body weight. Proc. Natl. Acad. Sci. U.S.A. 100, 6045–6050. doi: 10.1073/pnas.1030790100
Bradshaw, A. D., and Sage, E. H. (2001). SPARC, a matricellular protein that functions in cellular differentiation and tissue response to injury. J. Clin. Invest. 107, 1049–1054. doi: 10.1172/jci12939
Brunetti, L., Di Nisio, C., Recinella, L., Chiavaroli, A., Leone, S., Ferrante, C., et al. (2011). Effects of vaspin, chemerin and omentin-1 on feeding behavior and hypothalamic peptide gene expression in the rat. Peptides 32, 1866–1871. doi: 10.1016/j.peptides.2011.08.003
Brunetti, L., Leone, S., Orlando, G., Ferrante, C., Recinella, L., Chiavaroli, A., et al. (2014). Hypotensive effects of omentin-1 related to increased adiponectin and decreased interleukin-6 in intra-thoracic pericardial adipose tissue. Pharmacol. Rep. 66, 991–995. doi: 10.1016/j.pharep.2014.06.014
Bu, J., Feng, Q., Ran, J., Li, Q., Mei, G., and Zhang, Y. (2012). Visceral fat mass is always, but adipokines (adiponectin and resistin) are diversely associated with insulin resistance in Chinese type 2 diabetic and normoglycemic subjects. Diabetes Res. Clin. Pract. 96, 163–169. doi: 10.1016/j.diabres.2011.12.014
Buechler, C. (2014). Chemerin, a novel player in inflammatory bowel disease. Cell. Mol. Immunol. 11, 315–316. doi: 10.1038/cmi.2014.14
Byerly, M. S., Petersen, P. S., Ramamurthy, S., Seldin, M. M., Lei, X., Provost, E., et al. (2014). C1q/TNF-related protein 4 (CTRP4) is a unique secreted protein with two tandem C1q domains that functions in the hypothalamus to modulate food intake and body weight. J. Biol. Chem. 289, 4055–4069. doi: 10.1074/jbc.m113.506956
Byerly, M. S., Swanson, R., Wei, Z., Seldin, M. M., McCulloh, P. S., and Wong, G. W. (2013). A central role for C1q/TNF-related protein 13 (CTRP13) in modulating food intake and body weight. PLoS One 8:e62862. doi: 10.1371/journal.pone.0062862
Cakirca, G., and Turgut, F. H. (2018). Serum matrix metalloproteinase-9, tissue inhibitor of metalloproteinase-1 and matrix metalloproteinase-9/ neutrophil gelatinase-associated lipocalin complex levels in patients with early-stage diabetic nephropathy. Iran. J. Kidney Dis. 12, 299–304.
Canivell, S., Rebuffat, S., Ruano, E. G., Kostov, B., Sisò-Almirall, A., Novials, A., et al. (2015). Circulating SFRP5 levels are elevated in drug-näive recently diagnosed type 2 diabetic patients as compared with prediabetic subjects and controls. Diabetes Metab. Res. Rev. 31, 212–219. doi: 10.1002/dmrr.2599
Cantarini, L., Simonini, G., Fioravanti, A., Generoso, M., Bacarelli, M. R., Dini, E., et al. (2011). Circulating levels of the adipokines vaspin and omentin in patients with juvenile idiopathic arthritis, and relation to disease activity. Clin. Exp. Rheumatol. 29, 1044–1048.
Cao, H., Lin, J., Chen, W., Xu, G., and Sun, C. (2016). Baseline adiponectin and leptin levels in predicting an increased risk of disease activity in rheumatoid arthritis: a meta-analysis and systematic review. Autoimmunity 49, 547–553. doi: 10.1080/08916934.2016.1230847
Carrión, M., Frommer, K. W., Pérez-García, S., Müller-Ladner, U., Gomariz, R. P., and Neumann, E. (2019). The adipokine network in rheumatic joint diseases. Int. J. Mol. Sci. 20:4091. doi: 10.3390/ijms20174091
Carstensen, M., Herder, C., Kempf, K., Erlund, I., Martin, S., Koenig, W., et al. (2013). Sfrp5 correlates with insulin resistance and oxidative stress. Eur. J. Clin. Invest. 43, 350–357. doi: 10.1111/eci.12052
Cash, J. L., Hart, R., Russ, A., Dixon, J. P., Colledge, W. H., Doran, J., et al. (2008). Synthetic chemerin-derived peptides suppress inflammation through ChemR23. J. Exp. Med. 205, 767–775. doi: 10.1084/jem.20071601
Cerezo, L. A., Kuklova, M., Hulejova, H., Vernerova, Z., Kasprikova, N., Veigl, D., et al. (2015). Progranulin is associated with disease activity in patients with rheumatoid arthritis. Mediators Inflamm. 2015:740357.
Cesari, M., Pahor, M., and Incalzi, R. A. (2010). Plasminogen activator inhibitor-1 (PAI-1): a key factor linking fibrinolysis and age-related subclinical and clinical conditions. Cardiovasc. Ther. 28, 72–91.
Chang, Y. H., Chang, D. M., Lin, K. C., Shin, S. J., and Lee, Y. J. (2011). Visfatin in overweight/obesity, type 2 diabetes mellitus, insulin resistance, metabolic syndrome and cardiovascular diseases: a meta-analysis and systemic review. Diabetes Metab. Res. Rev. 27, 515–527. doi: 10.1002/dmrr.1201
Chen, H., Huang, X. N., Stewart, A. F., and Sepulveda, J. L. (2004). Gene expression changes associated with fibronectin-induced cardiac myocyte hypertrophy. Physiol. Genomics 18, 273–283. doi: 10.1152/physiolgenomics.00104.2004
Chen, H., Montagnani, M., Funahashi, T., Shimomura, I., and Quon, M. J. (2003). Adiponectin stimulates production of nitric oxide in vascular endothelial cells. J. Biol. Chem. 278, 45021–45026. doi: 10.1074/jbc.m307878200
Chen, W., and Frangogiannis, N. G. (2010). The role of inflammatory and fibrogenic pathways in heart failure associated with aging. Heart Fail. Rev. 15, 415–422. doi: 10.1007/s10741-010-9161-y
Chen, W. C., Wang, S. W., Lin, C. Y., Tsai, C. H., Fong, Y. C., Lin, T. Y., et al. (2019). Resistin enhances monocyte chemoattractant protein-1 production in human synovial fibroblasts and facilitates monocyte migration. Cell Physiol. Biochem. 52, 408–420. doi: 10.33594/000000029
Cheng, L., Zhang, D., and Chen, B. (2015). Declined plasma sfrp5 concentration in patients with type 2 diabetes and latent autoimmune diabetes in adults. Pak. J. Med. Sci. 31, 602–605.
Chlenski, A., and Cohn, S. L. (2010). Modulation of matrix remodeling by SPARC in neoplastic progression. Semin. Cell. Dev. Biol. 21, 55–65. doi: 10.1016/j.semcdb.2009.11.018
Chlenski, A., Liu, S., Baker, L. J., Yang, Q., Tian, Y., Salwen, H. R., et al. (2004). Neuroblastoma angiogenesis is inhibited with a folded synthetic molecule corresponding to the epidermal growth factor-like module of the follistatin domain of SPARC. Cancer Res. 64, 7420–7425. doi: 10.1158/0008-5472.can-04-2141
Cho, Y. K., Kang, Y. M., Lee, S. E., Lee, Y., Seol, S. M., Lee, W. J., et al. (2018). Effect of SFRP5 (Secreted Frizzled-Related Protein 5) on the WNT5A (Wingless-Type Family Member 5A)-induced endothelial dysfunction and its relevance with arterial stiffness in human subjects. Arterios. Thromb. Vasc. Biol. 38, 1358–1367. doi: 10.1161/atvbaha.117.310649
Cho, Y. M., Youn, B. S., Lee, H., Lee, N., Min, S. S., Kwark, S. H., et al. (2006). Plasma retinol-binding protein-4 concentrations are elevated in human subjects with impaired glucose tolerance and type 2 diabetes. Diabetes Care 29, 2457–2461. doi: 10.2337/dc06-0360
Choi, K. M., Hwang, S. Y., Hong, H. C., Choi, H. Y., Yoo, H. J., Youn, B. S., et al. (2014). Implications of C1q/TNF-related protein-3 (CTRP-3) and progranulin in patients with acute coronary syndrome and stable angina pectoris. Cardiovasc. Diabetol. 13:14. doi: 10.1186/1475-2840-13-14
Chong, K. S., Gardner, R. S., Morton, J. J., Ashley, E. A., and McDonagh, T. A. (2006). Plasma concentrations of the novel peptide apelin are decreased in patients with chronic heart failure. Eur. J. Heart Fail. 8, 355–360. doi: 10.1016/j.ejheart.2005.10.007
Christman, M. A., Goetz, D. J., Dickerson, E., McCall, K. D., Lewis, C. J., Benencia, F., et al. (2008). Wnt5a is expressed in murine and human atherosclerotic lesions. Am. J. Physiol. Heart Circ. Physiol. 294, H2864–H2870.
Chu, S. T., Lin, H. J., Huang, H. L., and Chen, Y. H. (1998). The hydrophobic pocket of 24p3 protein from mouse uterine luminal fluid: fatty acid and retinol binding activity and predicted structural similarity to lipocalins. J. Pept. Res. 52, 390–397. doi: 10.1111/j.1399-3011.1998.tb00663.x
Clezardin, P., Malaval, L., Ehrensperger, A. S., Delmas, P. D., Dechavanne, M., and Mcgregor, J. L. (1988). Complex formation of human thrombospondin with osteonectin. Eur. J. Biochem. 175, 275–284. doi: 10.1111/j.1432-1033.1988.tb14194.x
Clezardin, P., Malaval, L., Morel, M. C., Guichard, J., Lecompte, T., Trzeciak, M. C., et al. (1991). Osteonectin is an alpha-granule component involved with thrombospondin in platelet aggregation. J. Bone Miner. Res. 6, 1059–1070. doi: 10.1002/jbmr.5650061007
Clutter, S. D., Wilson, D. C., Marinov, A. D., and Hirsch, R. (2009). Follistatin-like protein 1 promotes arthritis by up-regulating IFN-gamma. J. Immunol. 182, 234–239. doi: 10.4049/jimmunol.182.1.234
Colak, S., Omma, A., Sandikci, S. C., Yucel, C., Omma, T., and Turhan, T. (2019). Vaspin, neutrophil gelatinase-associated lipocalin and apolipoprotein levels in patients with psoriatic arthritis. Bratisl. Lek. Listy 120, 65–69. doi: 10.4149/bll_2019_010
Colston, J. T., de la Rosa, S. D., Koehler, M., Gonzales, K., Mestril, R., Freeman, G. L., et al. (2007). Wnt-induced secreted protein-1 is a prohypertrophic and profibrotic growth factor. Am. J. Physiol. Heart Circ. Physiol. 293, H1839–H1846.
Conde, J., Gomez, R., Bianco, G., Scotece, M., Lear, P., Dieguez, C., et al. (2011). Expanding the adipokine network in cartilage: identification and regulation of novel factors in human and murine chondrocytes. Ann. Rheum. Dis. 70, 551–559. doi: 10.1136/ard.2010.132399
Conde, J., Scotece, M., Abella, V., Lopez, V., Pino, J., Gomez-Reino, J. J., et al. (2014). An update on leptin as immunomodulator. Expert Rev. Clin. Immunol. 10, 1165–1170. doi: 10.1586/1744666x.2014.942289
Conde, J., Scotece, M., Lopez, V., Gomez, R., Lago, F., Pino, J., et al. (2012). Adiponectin and leptin induce VCAM-1 expression in human and murine chondrocytes. PLoS One 7:e52533. doi: 10.1371/journal.pone.0052533
Cowland, J. B., and Borregaard, N. (1997). Molecular characterization and pattern of tissue expression of the gene for neutrophil gelatinase-associated lipocalin from humans. Genomics 45, 17–23. doi: 10.1006/geno.1997.4896
Cowley, M. A., and Grove, K. L. (2006). To be or NUCB2, is nesfatin the answer? Cell Metab. 4, 421–422. doi: 10.1016/j.cmet.2006.11.001
Cowley, M. A., Smart, J. L., Rubinstein, M., Gerdàn, M. G., Diano, S., Horvath, T. L., et al. (2001). Leptin activates anorexigenic pomc neurons through a neural network in the arcuate nucleus. Nature 411, 480–484. doi: 10.1038/35078085
Cruz, D. N., Gaiao, S., Maisel, A., Ronco, C., and Devarajan, P. (2012). Neutrophil gelatinase-associated lipocalin as a biomarker of cardiovascular disease: a systematic review. Clin. Chem. Lab. Med. 5, 1533–1545.
Dai, H., Li, X., He, T., Wang, Y., Wang, Z., Wang, S., et al. (2013). Decreased plasma nesfatin-1 levels in patients with acute myocardial infarction. Peptides 46, 167–171. doi: 10.1016/j.peptides.2013.06.006
Dalamaga, M., Chou, S. H., Shields, K., Papageorgiou, P., Polyzos, S. A., and Mantzoros, C. S. (2013). Leptin at the intersection of neuroendocrinology and metabolism: current evidence and therapeutic perspectives. Cell Metab. 18, 29–42. doi: 10.1016/j.cmet.2013.05.010
Damman, K., van Veldhuisen, D. J., Navis, G., Voors, A. A., and Hillege, H. L. (2008). Urinary neutrophil gelatinase associated lipocalin (NGAL), a marker of tubular damage, is increased in patients with chronic heart failure. Eur. J. Heart Fail. 10, 997–1000. doi: 10.1016/j.ejheart.2008.07.001
Dardeno, T. A., Chou, S. H., Moon, H. S., Chamberland, J. P., Fiorenza, C. G., and Mantzoros, C. (2010). Leptin in human physiology and therapeutics. Front. Neuroendocrinol. 31:377–393. doi: 10.1016/j.yfrne.2010.06.002
Davis, K. E., and Scherer, P. E. (2008). Adiponectin: no longer the lone soul in the fight against insulin resistance? Biochem. J. 416, e7–e9.
de Jager, S. C., and Pasterkamp, G. (2013). Crosstalk of lipids and inflammation in atherosclerosis: the PRO of PGRN? Cardiovasc. Res. 100, 4–6. doi: 10.1093/cvr/cvt199
de Souza Batista, C. M., Yang, R. Z., Lee, M. J., Glynn, N. M., Yu, D. Z., Pray, J., et al. (2007). Omentin plasma levels and gene expression are decreased in obesity. Diabetes 56, 1655–1661. doi: 10.2337/db06-1506
Deckx, S., Johnson, D. M., Rienks, M., Carai, P., Van Deel, E., Van der Velden, J., et al. (2019). Extracellular SPARC increases cardiomyocyte contraction during health and disease. PLoS One 14:e0209534. doi: 10.1371/journal.pone.0209534
Delany, A. M., Amling, M., Priemel, M., Howe, C., Baron, R., and Canalis, E. (2000). Osteopenia and decreased bone formation in osteonectin-null mice. J. Clin. Invest. 105, 915–923. doi: 10.1172/jci7039
Delany, A. M., Kalajzic, I., Bradshaw, A. D., Sage, E. H., and Canalis, E. (2003). Osteonectin-null mutation compromises osteoblast formation, maturation, and survival. Endocrinology 144, 2588–2596. doi: 10.1210/en.2002-221044
Deng, T., Lyon, C. J., Minze, L. J., Lin, J., Zou, J., Liu, J. Z., et al. (2013). Class II major histocompatibility complex plays an essential role in obesity-induced adipose inflammation. Cell Metab. 17, 411–422. doi: 10.1016/j.cmet.2013.02.009
Devireddy, L. R., Gazin, C., Zhu, X., and Green, M. R. (2005). A cell-surface receptor for lipocalin 24p3 selectively mediates apoptosis and iron uptake. Cell 123, 1293–1305. doi: 10.1016/j.cell.2005.10.027
Devireddy, L. R., Teodoro, J. G., Richard, F. A., and Green, M. R. (2001). Induction of apoptosis by a secreted lipocalin that is transcriptionally regulated by IL-3 deprivation. Science 293, 829–834. doi: 10.1126/science.1061075
Di Franco, M., Spinelli, F. R., Metere, A., Gerardi, M. C., Conti, V., Boccalini, F., et al. (2012). Serum levels of asymmetric dimethylarginine and apelin as potential markers of vascular endothelial dysfunction in early rheumatoid arthritis. Mediators Inflamm. 2012:347268.
Diah, M., Lelo, A., Lindarto, D., and Mukhtar, Z. (2019). Plasma concentrations of adiponectin in patients with coronary artery disease and coronary slow flow. Acta Med. Indones. 51, 290–295.
Ding, S., Qu, W., Dang, S., Xie, X., Xu, J., Wang, Y., et al. (2015). Serum nesfatin-1 is reduced in type 2 diabetes mellitus patients with peripheral arterial disease. Med. Sci. Monit. 21, 987–991. doi: 10.12659/msm.892611
Dong, F., Zhang, X., and Ren, J. (2006). Leptin regulates cardiomycoyte contractile function through endothelin-1 receptor–NADPH oxidase pathway. Hypertension 47, 222–229. doi: 10.1161/01.hyp.0000198555.51645.f1
Du, Y., Zhao, Y., Zhu, Y., Hu, C., Zhang, J., Ji, Q., et al. (2019). High serum secreted frizzled-related protein 5 levels associates with early improvement of cardiac function following ST-segment elevation myocardial infarction treated by primary percutaneous coronary intervention. J. Atheroscler. Thromb. 26, 868–878. doi: 10.5551/jat.47019
Duan, X. Y., Xie, P. L., Ma, Y. L., and Tang, S. Y. (2011). Omentin inhibits osteoblastic differentiation of calcifying vascular smooth muscle cells through the PI3K/Akt pathway. Amino Acids 41, 1223–1231. doi: 10.1007/s00726-010-0800-3
Ehara, Y., Sakurai, D., Tsuchiya, N., Nakano, K., Tanaka, Y., Yamaguchi, A., et al. (2004). Follistatin-related protein gene (FRP) is expressed in the synovial tissues of rheumatoid arthritis, but its polymorphisms are not associated with genetic susceptibility. Clin. Exp. Rheumatol. 22, 707–712.
Eisinger, K., Bauer, S., Schäffler, A., Walter, R., Neumann, E., Buechler, C., et al. (2012). Chemerin induces CCL2 and TLR4 in synovial fibroblasts of patients with rheumatoid arthritis and osteoarthritis. Exp. Mol. Pathol. 92, 90–96. doi: 10.1016/j.yexmp.2011.10.006
El-Armouche, A., Ouchi, N., Tanaka, K., Doros, G., Wittkopper, K., Schulze, T., et al. (2011). Follistatin-like 1 in chronic systolic heart failure: a marker of left ventricular remodeling. Circ. Heart Fail. 4, 621–627. doi: 10.1161/circheartfailure.110.960625
Elneihoum, A. M., Falke, P., Axelsson, L., Lundberg, E., Lindgarde, F., and Ohlsson, K. (1996). Leukocyte activation detected by increased plasma levels of inflammatory mediators in patients with ischemic cerebrovascular diseases. Stroke 27, 1734–1738. doi: 10.1161/01.str.27.10.1734
Enomoto, T., Ohashi, K., Shibata, R., Higuchi, A., Maruyama, S., Izumiya, Y., et al. (2011). Adipolin/C1qdc2/CTRP12 functions as an adipokine that improves glucose metabolism. J. Biol. Chem. 286, 34552–34558. doi: 10.1074/jbc.m111.277319
Ernst, M. C., Issa, M., Goralski, K. B., and Sinal, C. J. (2010). Chemerin exacerbates glucose intolerance in mouse models of obesity and diabetes. Endocrinology 151, 1998–2007. doi: 10.1210/en.2009-1098
Ernst, M. C., and Sinal, C. J. (2010). Chemerin: at the crossroads of inflammation and obesity. Trends Endocrinol. Metab. 21, 660–667. doi: 10.1016/j.tem.2010.08.001
Esaki, E., Adachi, H., Hirai, Y., Yamagishi, S., Kakuma, T., Enomoto, M., et al. (2014). Serum vaspin levels are positively associated with carotid atherosclerosis in a general population. Atherosclerosis 233, 248–252. doi: 10.1016/j.atherosclerosis.2013.12.040
Fain, J. N., Cheema, P. S., Bahouth, S. W., and Lloyd Hiler, M. (2003). Resistin release by human adipose tissue explants in primary culture. Biochem. Biophys. Res. Commun. 300, 674–678. doi: 10.1016/s0006-291x(02)02864-4
Fan, N., Sun, H., Wang, Y., Wang, Y., Zhang, L., Xia, Z., et al. (2013). Follistatin-like 1: a potential mediator of inflammation in obesity. Mediators Inflamm. 2013:752519.
Fang, W. Q., Zhang, Q., Peng, Y. B., Chen, M., Lin, X. P., Wu, J. H., et al. (2011). Resistin level is positively correlated with thrombotic complications in Southern Chinese metabolic syndrome patients. J. Endocrinol. Invest. 34, e36–e42.
Farooqi, I. S., Matarese, G., Lord, G. M., Keogh, J. M., Lawrence, E., Agwu, C., et al. (2002). Beneficial effects of leptin on obesity, T cell hyporesponsiveness, and neuroendocrine/metabolic dysfunction of human congenital leptin deficiency. J. Clin. Invest. 110, 1093–1103. doi: 10.1172/jci0215693
Fatel, E. C. S., Rosa, F. T., Simao, A. N. C., and Dichi, I. (2018). Adipokines in rheumatoid arthritis. Adv. Rheumatol. 58:25.
Fatima, S. S., Rehman, R., Baig, M., and Khan, T. A. (2014). New roles of the multidimensional adipokine: chemerin. Peptides 62, 15–20. doi: 10.1016/j.peptides.2014.09.019
Fazeli, M., Zarkesh-Esfahani, H., Wu, Z., Maamra, M., Bidlingmaier, M., Pockley, A. G., et al. (2006). Identification of a monoclonal antibody against the leptin receptor that acts as an antagonist and blocks human monocyte and T cell activation. J. Immunol. Methods 312, 190–200. doi: 10.1016/j.jim.2006.03.011
Feng, R., Li, Y., Wang, C., Luo, C., Liu, L., Chuo, F., et al. (2014). Higher vaspin levels in subjects with obesity and type 2 diabetes mellitus: a meta-analysis. Diabetes Res. Clin. Pract. 106, 88–94. doi: 10.1016/j.diabres.2014.07.026
Ferrand, N., Béreziat, V., Moldes, M., Zaoui, M., Larsen, A. K., and Sabbah, M. (2017). WISP1/CCN4 inhibits adipocyte differentiation through repression of PPARγ activity. Sci. Rep. 7:1749.
Fioravanti, A., Tenti, S., Bacarelli, M. R., Damiani, A., Li Gobbi, F., Bandinelli, F., et al. (2019). Tocilizumab modulates serum levels of adiponectin and chemerin in patients with rheumatoid arthritis: Potential cardiovascular protective role of IL-6 inhibition. Clin. Exp. Rheumatol. 37, 293–300.
Flower, D. R. (1996). The lipocalin protein family: structure and function. Biochem. J. 318, 1–14. doi: 10.1042/bj3180001
Folkesson, M., Kazi, M., Zhu, C., Silveira, A., Hemdahl, A. L., Hamsten, A., et al. (2007). Presence of NGAL/MMP-9 complexes in human abdominal aortic aneurysms. Thromb. Haemost. 98, 427–433. doi: 10.1160/th06-11-0638
Francki, A., McClure, T. D., Brekken, R. A., Motamed, K., Murri, C., Wang, T., et al. (2004). Sparc regulates TGF-beta1-dependent signaling in primary glomerular mesangial cells. J. Cell. Biochem. 91, 915–925. doi: 10.1002/jcb.20008
Franco-Trepat, E., Guillán-Fresco, M., Alonso-Pérez, A., Jorge-Mora, A., Francisco, V., Gualillo, O., et al. (2019). Visfatin connection: present and future in osteoarthritis and osteoporosis. J. Clin. Med. 8:1178. doi: 10.3390/jcm8081178
French, D. M., Kaul, R. J., D’Souza, A. L., Crowley, C. W., Bao, M., Frantz, G. D., et al. (2004). WISP-1 is an osteoblastic regulator expressed during skeletal development and fracture repair. Am. J. Pathol. 165, 855–867. doi: 10.1016/s0002-9440(10)63348-2
Fruebis, J., Tsao, T. S., Javorschi, S., Ebbets-Reed, D., Erickson, M. R., Yen, F. T., et al. (2001). Proteolytic cleavage productproduct of 30-kDa adipocyte complement-related protein increases fatty acid oxidation in muscle and causes weight loss in mice. Proc. Natl. Acad. Sci. U.S.A. 98, 2005–2010. doi: 10.1073/pnas.98.4.2005
Fu, J., Li, Y., Esangbedo, I., Li, G., Feng, D., Li, L., et al. (2018). Circulating osteonectin and adipokine profiles in relation to metabolically healthy obesity in chinese children: findings from BCAMS. Am. Heart Assoc. 7:e009169.
Fu, M., Gong, D. W., Damcott, C., Sabra, M., Yang, R., and Pollin, T. (2004). Systematic analysis of omentin 1 and omentin 2 on 1q23 as candidate genes for type 2 diabetes in the old order amish. Diabetes 53:A59.
Galic, S., Oakhill, J. S., and Steinberg, G. R. (2010). Adipose tissue as an endocrine organ. Mol. Cell. Endocrinol. 316, 129–139.
Garaulet, M., Hernandez-Morante, J. J., de Heredia, F. P., and Tebar, F. J. (2007). Adiponectin, the controversial hormone. Public Health Nutr. 10, 1145–1150. doi: 10.1017/s1368980007000638
Garay-Rojas, E., Harper, M., Hraba-Renevey, S., and Kress, M. (1996). An apparent autocrine mechanism amplifies the dexamethasone- and retinoic acid-induced expression of mouse lipocalin-encoding gene 24p3. Gene 170, 173–180. doi: 10.1016/0378-1119(95)00896-9
García-Galiano, D., Navarro, V. M., Gaytan, F., and Tena-Sempere, M. (2010). Expanding roles of NUCB2/nesfatin-1 in neuroendocrine regulation. J. Mol. Endocrinol. 45, 281–290. doi: 10.1677/jme-10-0059
Gardener, H., Crisby, M., Sjoberg, C., Hudson, B., Goldberg, R., Mendez, A. J., et al. (2013). Serum adiponectin in relation to race–ethnicity and vascular risk factors in the northern manhattan study. Metab. Syndr. Relat. Disord. 11, 46–55. doi: 10.1089/met.2012.0065
Gavi, S., Stuart, L. M., Kelly, P., Melendez, M. M., Mynarcik, D. C., Gelato, M. C., et al. (2007). Retinol-binding protein 4 is associated with insulin resistance and body fat distribution in nonobese subjects without type 2 diabetes. J. Clin. Endocrinol. Metab. 92, 1886–1890. doi: 10.1210/jc.2006-1815
Gerber, M., Boettner, A., Seidel, B., Lammert, A., Bar, J., Schuster, E., et al. (2005). Serum resistin levels of obese and lean children and adolescents: biochemical analysis and clinical relevance. J. Clin. Endocrinol. Metab. 90, 4503–4509. doi: 10.1210/jc.2005-0437
Gilardini, L., McTernan, P., Girola, A., da Silva, N. F., Alberti, L., Kumar, S., et al. (2006). Adiponectin is a candidate marker of metabolic syndrome in obese children and adolescents. Atherosclerosis 189, 401–407. doi: 10.1016/j.atherosclerosis.2005.12.021
Giltay, E. J., Elbers, J. M., Gooren, L. J., Emeis, J. J., Kooistra, T., Asscheman, H., et al. (1998). Visceral fat accumulation is an important determinant of PAI-1 levels in young, nonobese men and women: modulation by cross-sex hormone administration. Arterioscler. Thromb. Vasc. Biol. 18, 1716–1722. doi: 10.1161/01.atv.18.11.1716
Glas-Greenwalt, P., Kant, K. S., and Allen, C. (1984). Fibrinolysis in health and disease: severe abnormalities in systemic lupus erythematosus. J. Lab. Clin. Med. 104:962.
Gómez, R., Conde, J., Scotece, M., Gomez-Reino, J. J., Lago, F., and Gualillo, O. (2011). What’s new in our understanding of the role of adipokines in rheumatic diseases? Nat. Rev. Rheumatol. 7, 528–536. doi: 10.1038/nrrheum.2011.107
Gomez, R., Scotece, M., Conde, J., Lopez, V., Pino, J., Lago, F., et al. (2013). Nitric oxide boosts TLR-4 mediated lipocalin 2 expression in chondrocytes. J. Orthop. Res. 31, 1046–1052. doi: 10.1002/jor.22331
Gonzalez, R., Perry, R. L., Gao, X., Gaidhu, M. P., Tsushima, R. G., Ceddia, R. B., et al. (2011). Nutrient responsive nesfatin-1 regulates energy balance and induces glucose-stimulated insulin secretion in rats. Endocrinology 152, 3628–3637. doi: 10.1210/en.2010-1471
Goralski, K. B., McCarthy, T. C., Hanniman, E. A., Zabel, B. A., Butcher, E. C., Parlee, S. D., et al. (2007). Chemerin, a novel adipokine that regulates adipogenesis and adipocyte metabolism. J. Biol. Chem. 282, 28175–28188. doi: 10.1074/jbc.m700793200
Gunter, S., Solomon, A., Tsang, L., Woodiwiss, A. J., Robinson, C., Millen, A. M., et al. (2017). Apelin concentrations are associated with altered atherosclerotic plaque stability mediator levels and atherosclerosis in rheumatoid arthritis. Atherosclerosis 256, 75–81. doi: 10.1016/j.atherosclerosis.2016.11.024
Guo, H., Jin, D., Zhang, Y., Wright, W., Bazuine, M., Brockman, D. A., et al. (2010). Lipocalin-2 deficiency impairs thermogenesis and potentiates diet-induced insulin resistance in mice. Diabetes 59, 1376–1385. doi: 10.2337/db09-1735
Gupta, K., Shukla, M., Cowland, J. B., Malemud, C. J., and Haqqi, T. M. (2007). Neutrophil gelatinase-associated lipocalin is expressed in osteoarthritis and forms a complex with matrix metalloproteinase 9. Arthritis Rheum. 56, 3326–3335. doi: 10.1002/art.22879
Haider, D. G., Schindler, K., Prager, G., Bohdjalian, A. L., Wolzt, M., and Ludvik, B. (2007). Serum retinol-binding protein 4 is reduced after weight loss in morbidly obese subjects. J. Clin. Endocrinol. Metab. 92, 1168–1171. doi: 10.1210/jc.2006-1839
Halleskog, C., Dijksterhuis, J. P., Kilander, M. B., Becerril-Ortega, J., Villaescusa, J. C., Lindgren, E., et al. (2012). Heterotrimeric G protein-dependent WNT-5A signaling to ERK1/2 mediates distinct aspects of microglia proinflammatory transformation. J. Neuroinflammation. 9:111.
Hao, F., Zhang, H., Zhu, J., Kuang, H., Yu, Q., Bai, M., et al. (2016). Association between vaspin level and coronary artery disease in patients with type 2 diabetes. Diabetes Res. Clin. Pract. 113, 26–32. doi: 10.1016/j.diabres.2015.12.001
Harris, B. S., Zhang, Y., Card, L., Rivera, L. B., Brekken, R. A., and Bradshaw, A. D. (2011). SPARC regulates collagen interaction with cardiac fibroblast cell surfaces. Am. J. Physiol. Heart Circ. Physiol. 301, H841–H847.
Heiker, J. T., Klöting, N., Kovacs, P., Kuettner, E. B., Sträter, N., Schultz, S., et al. (2013). Vaspin inhibits kallikrein 7 by serpin mechanism. Cell Mol. Life. Sci. 70, 2569–2583. doi: 10.1007/s00018-013-1258-8
Heinonen, M. V., Purhonen, A. K., Miettinen, P., Pääkkönen, M., Pirinen, E., Alhava, E., et al. (2005). Apelin, orexin-A and leptin plasma levels in morbid obesity and effect of gastric banding. Regul. Pept. 130, 7–13. doi: 10.1016/j.regpep.2005.05.003
Hemdahl, A. L., Gabrielsen, A., Zhu, C., Eriksson, P., Hedin, U., Kastrup, J., et al. (2006). Expression of neutrophil gelatinase-associated lipocalin in atherosclerosis and myocardial infarction. Arterioscler. Thromb. Vasc. Biol. 26, 136–142. doi: 10.1161/01.atv.0000193567.88685.f4
Heymsfield, S. B., Greenberg, A. S., Fujioka, K., Dixon, R. M., Kushner, R., and Hunt, T. (1999). Recombinant leptin for weight loss in obese and lean adults: a randomized, controlled, dose-escalation trial. JAMA 282, 1568–1575. doi: 10.1001/jama.282.16.1568
Hida, K., Wada, J., Eguchi, J., Zhang, H., Baba, M., Seida, A., et al. (2005). Visceral adipose tissue derived serine protease inhibitor: a unique insulin-sensitizing adipocytokine in obesity. Proc. Natl. Acad. Sci. U.S.A. 102, 10610–10615. doi: 10.1073/pnas.0504703102
Hida, K., Wada, J., Zhang, H., Hiragushi, K., Tsuchiyama, Y., Shikata, K., et al. (2000). Identification of genes specifically expressed in the accumulated visceral adipose tissue of OLETF rats. J. Lipid Res. 41, 1615–1622.
Hofmann, C., Chen, N., Obermeier, F., Paul, G., Buchler, C., Kopp, A., et al. (2011). C1q/TNF-related protein-3 (CTRP-3) is secreted by visceral adipose tissue and exerts antiinflammatory and antifibrotic effects in primary human colonic fibroblasts. Inflamm. Bowel Dis. 17, 2462–2471. doi: 10.1002/ibd.21647
Horak, M., Kuruczova, D., Zlamal, F., Tomandl, J., and Bienertova-Vasku, J. (2018). Follistatin-like 1 is downregulated in morbidly and super obese Central-European population. Dis. Markers 2018:4140815.
Hotta, K., Funahashi, T., Arita, Y., Takahashi, M., Matsuda, M., and Okamoto, Y. (2000). Plasma concentrations of a novel, adipose-specific protein, adiponectin, in type 2 diabetic patients. Arterioscler. Thromb. Vasc. Biol. 2000, 1595–1599. doi: 10.1161/01.atv.20.6.1595
Hou, C. H., Tang, C. H., Hsu, C. J., Hou, S. M., and Liu, J. F. (2013). CCN4 induces IL-6 production through αvβ5 receptor, PI3K, Akt, and NF-κB singling pathway in human synovial fibroblasts. Arthritis Res. Ther. 15:R19.
Hsu, W. Y., Chao, Y. W., Tsai, Y. L., Lien, C. C., Chang, C. F., Deng, M. C., et al. (2011). Resistin induces monocyte-endothelial cell adhesion by increasing ICAM-1 and VCAM-1 expression in endothelial cells via p38MAPK-dependent pathway. J. Cell. Physiol. 226, 2181–2188. doi: 10.1002/jcp.22555
Hu, P. F., Chen, W. P., Tang, J. L., Bao, J. P., and Wu, L. D. (2010). Apelin plays a catabolic role on articular cartilage: in vivo and in vitro studies. Int. J. Mol. Med. 26, 357–363.
Hu, P. F., Tang, J. L., Chen, W. P., Bao, J. P., and Wu, L. D. (2011). Increased apelin serum levels and expression in human chondrocytes in osteoarthritic patients. Int. Orthop. 35, 1421–1426. doi: 10.1007/s00264-010-1100-y
Hu, W., Li, L., Yang, M., Luo, X., Ran, W., Liu, D., et al. (2013). Circulating Sfrp5 is a signature of obesity-related metabolic disorders and is regulated by glucose and liraglutide in humans. J. Clin. Endocrinol. Metab. 98, 290–298. doi: 10.1210/jc.2012-2466
Hu, Z., Deng, H., and Qu, H. (2013). Plasma SFRP5 levels are decreased in Chinese subjects with obesity and type 2 diabetes and negatively correlated with parameters of insulin resistance. Diabetes Res. Clin. Pract. 99, 391–395. doi: 10.1016/j.diabres.2012.11.026
Huang, H. L., Chu, S. T., and Chen, Y. H. (1999). Ovarian steroids regulate 24p3 expression in mouse uterus during the natural estrous cycle and the preimplantation period. J. Endocrinol. 162, 11–19. doi: 10.1677/joe.0.1620011
Huang, X., and Yang, Z. (2016). Resistin’s, obesity and insulin resistance: the continuing disconnect between rodents and humans. J. Endocrinol. Invest. 39, 607–615. doi: 10.1007/s40618-015-0408-2
Huo, L., Gamber, K., Greeley, S., Silva, J., Huntoon, N., Lemg, X. H., et al. (2009). Leptin-dependent control of glucose balance and locomotor activity by POMC neurons. Cell Metab. 9, 537–547. doi: 10.1016/j.cmet.2009.05.003
Hvidberg, V., Jacobsen, C., Strong, R. K., Cowland, J. B., Moestrup, S. K., Borregaard, N., et al. (2005). The endocytic receptor megalin binds the iron transporting neutrophil-gelatinase-associated lipocalin with high affinity and mediates its cellular uptake. FEBS Lett. 579, 773–777. doi: 10.1016/j.febslet.2004.12.031
Imbrogno, S., Angelone, T., and Cerra, M. C. (2015). Nesfatin-1 and the cardiovascular system: central and pheripheral actions and cardioprotection. Curr. Drug Targets 16, 877–883. doi: 10.2174/1389450116666150408101431
Innamorati, G., Whang, M. I., Molteni, R., Le Gouill, C., and Birnbaumer, M. (2002). GIP, a G-protein-coupled receptor interacting protein. Regul. Pept. 109, 173–179. doi: 10.1016/s0167-0115(02)00201-x
Jamaluddin, M. S., Yan, S., Lu, J., Liang, Z., Yao, Q., and Chen, C. (2013). Resistin increases monolayer permeability of human coronary artery endothelial cells. PLoS One 8:e84576. doi: 10.1371/journal.pone.0084576
Jang, Y., Lee, J. H., Wang, Y., and Sweeney, G. (2012). Emerging clinical and experimental evidence for the role of lipocalin-2 in metabolic syndrome. Clin. Exp. Pharmacol. Physiol. 39, 194–199. doi: 10.1111/j.1440-1681.2011.05557.x
Jeong, E., Youn, B. S., Kim, D. W., Kim, E. H., Park, J. W., Namkoong, C., et al. (2010). Circadian rhythm of serum vaspin in healthy male volunteers: relation to meals. J. Clin. Endocrinol. Metab. 95, 1869–1875. doi: 10.1210/jc.2009-1088
Ji, H., Li, H., Zhuang, J., Su, Y., Wen, J., Zhang, J., et al. (2017). High serum level of secreted frizzled-related protein 5 (sfrp5) is associated with future cardiovascular events. Cardiovasc. Ther. 2:115.
Jia, W., Wu, H., Bao, Y., Wang, C., Lu, J., Zhu, J., et al. (2007). Association of serum retinol-binding protein 4 and visceral adiposity in Chinese subjects with and without type 2 diabetes. J. Clin. Endocrinol. Metab. 92, 3224–3229. doi: 10.1210/jc.2007-0209
Jian, J., Konopka, J., and Liu, C. (2012). Insights into the role of progranulin in immunity, infection, and inflammation. J. Leukoc. Biol. 93, 199–208. doi: 10.1189/jlb.0812429
Jian, Y. C., Wang, J. J., Dong, S., Hu, J. W., Hu, L. J., Yang, G. M., et al. (2014). Wnt-induced secreted protein 1/CCN4 in liver fibrosis both in vitro and in vivo. Clin. Lab. 60, 29–35.
Jiang, L., Bao, J., Zhou, X., Xiong, Y., and Wu, L. (2013). Increased serum levels and chondrocyte expression of nesfatin-1 in patients with osteoarthritis and its relation with BMI, hsCRP, and IL-18. Mediators Inflamm. 2013:631251.
Jin, X., Guo, B., Yan, J., Yang, R., Chang, L., Wang, Y., et al. (2015). Angiotensin II increases secreted frizzled-related protein 5 (sFRP5) expression through AT1 receptor/Rho/ROCK1/JNK signaling in cardiomyocytes. Mol. Cell. Biochem. 408, 215–222. doi: 10.1007/s11010-015-2497-9
Juhan-Vague, I., Alessi, M. C., Mavri, A., and Morange, P. E. (2003). Plasminogen activator inhibitor-1, inflammation, obesity, insulin resistance and vascular risk. J. Thromb. Haemost. 1, 1575–1579. doi: 10.1046/j.1538-7836.2003.00279.x
Jung, C. H., Lee, W. J., Hwang, J. Y., Seol, S. M., Kim, Y. M., Lee, Y. L., et al. (2011). Vaspin protects vascular endothelial cells against free fatty acid-induced apoptosis through a phosphatidylinositol 3-kinase/Akt pathway. Biochem. Biophys. Res. Commun. 413, 264–269. doi: 10.1016/j.bbrc.2011.08.083
Jung, R. G., Motazedian, P., Ramirez, F. D., Simard, T., and Di Santo, P. (2018). Association between plasminogen activator inhibitor-1 and cardiovascular events: a systematic review and meta-analysis. Thromb. J. 16:12.
Kadoglou, N. P., Gkontopoulos, A., Kapelouzou, A., Fotiadis, G., Theofilogiannakos, E. K., Kottas, G., et al. (2011). Serum levels of vaspin and visfatin in patients with coronary artery disease-Kozani study. Clin. Chim. Acta 412, 48–52. doi: 10.1016/j.cca.2010.09.012
Kadowaki, T., and Yamauchi, T. (2005). Adiponectin and adiponectin receptors. Endocr. Rev. 26, 439–451. doi: 10.1210/er.2005-0005
Kalra, S. P., Dube, M. G., Pu, S., Xu, B., Horvath, T. L., and Kalra, P. S. (1999). Interacting appetite regulating pathways in the hypothalamic regulation of body weight. Endocr. Rev. 20, 68–100. doi: 10.1210/er.20.1.68
Kamio, N., Kawato, T., Tanabe, N., Kitami, S., Morita, T., Ochiai, K., et al. (2013). Vaspin attenuates RANKL-induced osteoclast formation in RAW264.7 cells. Connect Tissue Res. 54, 147–152. doi: 10.3109/03008207.2012.761978
Kanauchi, M., Nishioka, M., and Dohi, K. (2000). Secreted protein acidic and rich in cysteine (SPARC) in patients with diabetic nephropathy and tubulointerstitial injury. Diabetologia 43, 1076–1077. doi: 10.1007/s001250051493
Kaneko, K., Miyabe, Y., Takayasu, A., Fukuda, S., Miyabe, C., Ebisawa, M., et al. (2011). Chemerin activates fibroblast-like synoviocytes in patients with rheumatoid arthritis. Arthritis Res. Ther. 13:R158.
Kassem, E., Mahmoud, L., and Salah, W. (2010). Study of resistin and YKL-40 in rheumatoid arthritis. J. Am. Sci. 6, 1004–1012.
Katano, M., Okamoto, K., Arito, M., Kawakami, Y., Kurokawa, M. S., Suematsu, N., et al. (2009). Implication of granulocyte-macrophage colony-stimulating factor induced neutrophil gelatinase-associated lipocalin in pathogenesis of rheumatoid arthritis revealed by proteome analysis. Arthritis Res. Ther. 11:R3.
Katoh, M., and Katoh, M. (2007). WNT signaling pathway and stem cell signaling network. Clin. Cancer Res. 13, 4042–4045. doi: 10.1158/1078-0432.ccr-06-2316
Katsiki, N., Mikhailidis, D. P., and Banach, M. (2018). Leptin, cardiovascular diseases and type 2 diabetes mellitus. Acta Pharmacol. Sin. 39, 1176–1188. doi: 10.1038/aps.2018.40
Kaufmann, B., Muller, S., Hanisch, F. G., Hartmann, U., Paulsson, M., Maurer, P., et al. (2004). Structural variability of BM-40/SPARC/osteonectin glycosylation: implications for collagen affinity. Glycobiology 14, 609–619. doi: 10.1093/glycob/cwh063
Kaur, J., Adya, R., Tan, B. K., Chen, J., and Randeva, H. S. (2010). Identification of chemerin receptor (ChemR23) in human endothelial cells: chemerin-induced endothelial angiogenesis. Biochem. Biophys. Res. Commun. 391, 1762–1768. doi: 10.1016/j.bbrc.2009.12.150
Kaur, J., Mattu, H. S., Chatha, K., and Randeva, H. S. (2018). Chemerin in human cardiovascular disease. Vascul. Pharmacol. 110, 1–6. doi: 10.1016/j.vph.2018.06.018
Kawamata, Y., Habata, Y., Fukusumi, S., Hosoya, M., Fujii, R., Hinuma, S., et al. (2001). Molecular properties of apelin: tissue distribution and receptor binding. Biochim. Biophys. Acta 1538, 162–171. doi: 10.1016/s0167-4889(00)00143-9
Kawano, K., Hirashima, T., Mori, S., Saitoh, Y., Kurosumi, M., and Natori, T. (1992). Spontaneous long-term hyperglycemic rat with diabetic complications. Otsuka long-evans tokushima fatty (OLETF) strain. Diabetes 41, 1422–1428. doi: 10.2337/diabetes.41.11.1422
Kawase, R., Ohama, T., Matsuyama, A., Matsuwaki, T., Okada, T., Yamashita, T., et al. (2013). Deletion of progranulin exacerbates atherosclerosis in ApoE knockout mice. Cardiovasc. Res. 100, 125–133. doi: 10.1093/cvr/cvt178
Kazama, K., Okada, M., Hara, Y., and Yamawaki, H. (2013). A novel adipocytokine, omentin, inhibits agonist-induced increases of blood pressure rats. Vet. Med. Sci. 75, 1029–1034. doi: 10.1292/jvms.12-0537
Kershaw, E. E., and Flier, J. S. (2004). Adipose tissue as an endocrine organ. J. Clin. Endocrinol. Metab. 89, 2548–2556.
Kim, J., Kim, J., Kim, D. W., Ha, Y., Ihm, M. H., Kim, H., et al. (2010). Wnt5a induces endothelial inflammation via beta-catenin-independent signaling. J. Immunol. 185, 1274–1282. doi: 10.4049/jimmunol.1000181
Kim, Y., and Park, C. W. (2019). Mechanisms of adiponectin action: implication of adiponectin receptor agonism in diabetic kidney disease. Int. J. Mol. Sci. 20:1782. doi: 10.3390/ijms20071782
Kimura-Yoshida, C., Nakano, H., Okamura, D., Nakao, K., Yonemura, S., Belo, J. A., et al. (2005). Canonical Wnt signaling and its antagonist regulate anterior-posterior axis polarization by guiding cell migration in mouse visceral endoderm. Dev. Cell. 9, 639–650. doi: 10.1016/j.devcel.2005.09.011
Klaasen, R., Herenius, M. M., Wijbrandts, C. A., de Jager, W., van Tuyl, L. H., Nurmohamed, M. T., et al. (2012). Treatment-specific changes in circulating adipocytokines: a comparison between tumour necrosis factor blockade and glucocorticoid treatment for rheumatoid arthritis. Ann. Rheum. Dis. 71, 1510–1516. doi: 10.1136/annrheumdis-2011-200646
Klöting, N., Berndt, J., Kralisch, S., Kovacs, P., Fasshauer, M., Schön, M. R., et al. (2006). Vaspin gene expression in human adipose tissue: association with obesity and type 2 diabetes. Biochem. Biophys. Res. Commun. 339, 430–436. doi: 10.1016/j.bbrc.2005.11.039
Klöting, N., Graham, T. E., Berndt, J., Kralisch, S., Kovacs, P., Wason, C. J., et al. (2007). Serum retinol-binding protein is more highly expressed in visceral than in subcutaneous adipose tissue and is a marker of intra-abdominal fat mass. Cell Metab. 6, 79–87. doi: 10.1016/j.cmet.2007.06.002
Klöting, N., Kovacs, P., Kern, M., Heiker, J. T., Fasshauer, M., Schön, M. R., et al. (2011). Central vaspin administration acutely reduces food intake and has sustained blood glucose-lowering effects. Diabetologia 54, 1819–1823. doi: 10.1007/s00125-011-2137-1
Kobat, M. A., Celik, A., Balin, M., Altas, Y., Baydas, A., Bulut, M., et al. (2012). The investigation of serum vaspin level in atherosclerotic coronary artery disease. J. Clin. Med. Res. 4, 110–113.
Kobayashi, H., Ouchi, N., Kihara, S., Walsh, K., Kumada, M., Abe, Y., et al. (2004). Selective suppression of endothelial cell apoptosis by the high molecular weight form of adiponectin. Circ. Res. 94, e27–e31.
Kojima, Y., Ono, K., Inoue, K., Takagi, Y., Kikuta, K., Nishimura, M., et al. (2009). Progranulin expression in advanced human atherosclerotic plaque. Atherosclerosis 206, 102–108. doi: 10.1016/j.atherosclerosis.2009.02.017
Kopp, A., Bala, M., Buechler, C., Falk, W., Gross, P., Neumeier, M., et al. (2010a). C1q/TNF-related protein-3 represents a novel and endogenous lipopolysaccharide antagonist of the adipose tissue. Endocrinology 151, 5267–5278. doi: 10.1210/en.2010-0571
Kopp, A., Bala, M., Weigert, J., Buchler, C., Neumeier, M., Aslanidis, C., et al. (2010b). Effects of the new adiponectin paralogous protein CTRP-3 and of LPS on cytokine release from monocytes of patients with type 2 diabetes mellitus. Cytokine 2010, 51–57. doi: 10.1016/j.cyto.2009.10.001
Korolczuk, A., and Bełtowski, J. (2017). Progranulin, a new adipokine at the crossroads of metabolic syndrome, diabetes, dyslipidemia and hypertension. Curr. Pharm. Des. 23, 1533–1539. doi: 10.2174/1381612823666170124114524
Kos, K., and Wilding, J. P. (2010). SPARC: a key player in the pathologies associated with obesity and diabetes. Nat. Rev. Endocrinol. 6, 225–235. doi: 10.1038/nrendo.2010.18
Kos, K., Wong, S., Tan, B., Gummesson, A., Jernas, M., Franck, N., et al. (2009). Regulation of the fibrosis and angiogenesis promoter SPARC/osteonectin in human adipose tissue by weight change, leptin, insulin, and glucose. Diabetes 58, 1780–1788. doi: 10.2337/db09-0211
Kreçki, R., Krzemińska-Pakuła, M., Peruga, J. Z., Szcześniak, P., Lipiec, P., Wierzbowska-Drabik, K., et al. (2011). Elevated resistin opposed to adiponectin or angiogenin plasma levels as a strong, independent predictive factor for the occurrence of major adverse cardiac and cerebrovascular events in patients with stable multivessel coronary artery disease over 1-year follow-up. Med. Sci. Monit. 17, CR26–CR32.
Kubota, N., Yano, W., Kubota, T., Yamauchi, T., Itoh, S., Kumagai, H., et al. (2007). Adiponectin stimulates AMP-activated protein kinase in the hypothalamus and increases food intake. Cell Metab. 6, 55–68. doi: 10.1016/j.cmet.2007.06.003
Kumari, B., and Yadav, U. C. S. (2018). Adipokine visfatin’s role in pathogenesis of diabesity and related metabolic derangements. Curr. Mol. Med. 18, m116–m125.
Kupprion, C., Motamed, K., and Sage, E. H. (1998). SPARC (BM-40, osteonectin) inhibits the mitogenic effect of vascular endothelial growth factor on microvascular endothelial cells. J. Biol. Chem. 273, 29635–29640. doi: 10.1074/jbc.273.45.29635
Kvlividze, T. Z., Zavodovsky, B. V., Akhverdyan, Y. R., Polyakova, Y. V., Sivordova, L. E., Yakovlev, A. T., et al. (2019). Serum nesfatin-1 as a marker of systemic inflammation in rheumatoid arthritis. Klin. Lab. Diagn. 64, 53–56. doi: 10.18821/0869-2084-2019-64-1-53-56
Kwon, Y. J., Lee, S. W., Park, Y. B., Lee, S. K., and Park, M. C. (2014). Secreted frizzled-related protein 5 suppresses inflammatory response in rheumatoid arthritis fibroblast-like synoviocytes through down-regulation of c-Jun N-terminal kinase. Rheumatology 53, 1704–1711. doi: 10.1093/rheumatology/keu167
Lago, F., Gomez, R., Gomez-Reino, J. J., Dieguez, C., and Gualillo, O. (2009). Adipokines as novel modulators of lipid metabolism. Trends Biochem. Sci. 34, 500–510. doi: 10.1016/j.tibs.2009.06.008
Lahoti, T. S., Patel, D., Thekkemadom, V., Beckett, R., and Ray, S. D. (2012). Doxorubicin-induced in vivo nephrotoxicity involves oxidative stress-mediated multiple pro- and anti-apoptotic signaling pathways. Curr. Neurovasc. Res. 9, 282–295. doi: 10.2174/156720212803530636
Landin, K., Stigendal, L., Eriksson, E., Krotkiewski, M., Risberg, B., Tengborn, L., et al. (1990). Abdominal obesity is associated with an impaired fibrinolytic activity and elevated plasminogen activator inhibitor-1. Metabolism 39, 1044–1048. doi: 10.1016/0026-0495(90)90164-8
Lasser, G., Guchhait, P., Ellsworth, J. L., Sheppard, P., Lewis, K., Bishop, P., et al. (2006). C1qTNF-related protein-1 (CTRP-1): a vascular wallprotein that inhibits collagen-induced platelet aggregation by blocking VWF binding to collagen. Blood 107, 423–430. doi: 10.1182/blood-2005-04-1425
Lee, D. E., Kehlenbrink, S., Lee, H., Hawkins, M., and Yudkin, J. S. (2009). Getting the message across: mechanisms of physiological cross talk by adipose tissue. Am. J. Physiol. Endocrinol. Metab. 296, E1210–E1229.
Lee, D. K., Cheng, R., Nguyen, T., Fan, T., Kariyawasam, A. P., Liu, Y., et al. (2000). Characterization of apelin, the ligand for the APJ receptor. J. Neurochem. 74, 34–41. doi: 10.1046/j.1471-4159.2000.0740034.x
Lee, S. E., and Kim, H. S. (2012). Human resistin in cardiovascular disease. J. Smooth Muscle Res. 48, 27–35. doi: 10.1540/jsmr.48.27
Lee, S. W., Park, M. C., Park, Y. B., and Lee, S. K. (2007). Measurement of the serum leptin level could assist disease activity monitoring in rheumatoid arthritis. Rheumatol. Int. 27, 537–540. doi: 10.1007/s00296-006-0253-x
Lee, T. B., Hwang, H. J., Kim, J. A., Hwang, S. Y., Roh, E., Hong, S. H., et al. (2019). Association of serum FAM19A5 with metabolic and vascular risk facthors in uman subjects with or without type 2 diabetes. Diab. Vasc. Dis. Res. 16, 530–538. doi: 10.1177/1479164119860746
Lee, Y. A., Hahm, D. H., Kim, J. Y., Sur, B., Lee, H. M., and Ryu, C. J. (2018). Potential therapeutic antibodies targeting specific adiponectin isoforms in rheumatoid arthritis. Arthritis Res. 20:245.
Lee, Y. H., and Bae, S. C. (2016). Circulating leptin level in rheumatoid arthritis and its correlation with disease activity: a meta-analysis. Z. Rheumatol. 75, 1021–1027. doi: 10.1007/s00393-016-0050-1
Lee, Y. H., Magkos, F., Mantzoros, C. S., and Kang, E. S. (2011). Effects of leptin and adiponectin on pancreatic beta-cell function. Metabolism 60, 1664–1672. doi: 10.1016/j.metabol.2011.04.008
Lehr, S., Hartwig, S., Lamers, D., Famulla, S., Müller, S., Hanisch, F. G., et al. (2012). Identification and validation of novel adipokines released from primary human adipocytes. Mol. Cell. Proteomics. 11:M111.010504.
Lei, X., Seldin, M. M., Little, C. H., Choy, N., Klonisch, T., and Wong, G. W. (2017). C1q/TNF-related protein 6 (CTRP6) links obesity to adipose tissue inflammation and insulin resistance. J. Biol. Chem. 292, 14836–14850. doi: 10.1074/jbc.m116.766808
Levin, B. E., Dunn-Meynell, A. A., and Banks, W. A. (2004). Obesity-prone rats have normal blood-brain barrier transport but defective central leptin signaling before obesity onset. Am. J. Physiol. Regul. Integr. Comp. Physiol. 286, R143–R150.
Li, D., Wang, Y., Xu, N., Wei, Q., Wu, M., Li, X., et al. (2011). Follistatin-like protein 1 is elevated in systemic autoimmune diseases and correlated with disease activity in patients with rheumatoid arthritis. Arthritis Res. Ther. 13:R1.
Li, H. H., Li, Q., Liu, P., Liu, Y., Li, J., Wasserloos, K., et al. (2012). WNT1-inducible signaling pathway protein 1 contributes to ventilator-induced lung injury. Am. J. Respir. Cell. Mol. Biol. 47, 528–535. doi: 10.1165/rcmb.2012-0127oc
Li, L., Yang, G., Li, Q., Tang, Y., Yang, M., Yang, H., et al. (2006). Changes and relations of circulating visfatin, apelin, and resistin levels in normal, impaired glucose tolerance, and type 2 diabetic subjects. Exp. Clin. Endocrinol. Diabetes 114, 544–548. doi: 10.1055/s-2006-948309
Li, Z. G., Zhao, D. W., Xia, C. J., Wang, T. N., Liu, Y. P., Zhang, Y., et al. (2012). Decreased synovial fluid omentin-1concentrations reflect symptomatic severity in patients with knee osteoarthritis. Scand. J. Clin. Lab. Invest. 72, 623–628. doi: 10.3109/00365513.2012.726370
Lin, S., Ma, S., Lu, P., Cai, W., Chen, Y., and Sheng, J. (2014). Effect of CTRP3 on activation of adventitial fibroblasts induced by TGF-beta1 from rat aorta in vitro. Int. J. Clin. Exp. Pathol. 7, 2199–2208.
Liu, H., Dong, W., Lin, Z., Lu, J., Wan, H., Zhou, Z., et al. (2013). CCN4 regulates vascular smooth muscle cell migration and proliferation. Mol. Cells 36, 112–118. doi: 10.1007/s10059-013-0012-2
Liu, H., Xiong, W., Luo, Y., Chen, H., He, Y., Cao, Y., et al. (2019). Adipokine chemerin stimulates progression of atherosclerosis in ApoE–/– Mice. Biomed. Res. Int. 2019:7157865.
Liu, R., Zhao, P., Zhang, Q., Che, N., Xu, L., Qian, J., et al. (2020). Adiponectin promotes fibroblast-like synoviocytes producing IL-6 to enhance T follicular helper cells response in rheumatoid arthritis. Clin. Exp. Rheumatol. 38, 11–18.
Liu, S., Dong, Y., Wang, T., Zhao, S., Yang, K., Chen, X., et al. (2014). Vaspin inhibited proinflammatory cytokine induced activation of nuclear factor-kappa B and its downstream molecules in human endothelial EA.hy926 cells. Diabetes Res. Clin. Pract. 103, 482–488. doi: 10.1016/j.diabres.2013.12.002
Liu, W., Zhou, X., Li, Y., Zhang, S., Cai, X., Zhang, R., et al. (2020). Serum leptin, resistin, and adiponectin levels in obese and non-obese patients with newly diagnosed type 2 diabetes mellitus: a population-based study. Medicine 99:e19052. doi: 10.1097/md.0000000000019052
Liu, Y., Xu, F., Pei, H. X., Zhu, X., Lin, X., Song, C. Y., et al. (2016). Vaspin regulates the osteogenic differentiation of MC3T3-E1 through the PI3K-Akt/miR-34c loop. Sci. Rep. 6:25578.
López-Jaramillo, P., Gómez-Arbeláez, D., López-López, J., López-López, C., Martínez-Ortega, J., Gómez-Rodríguez, A., et al. (2014). The role of leptin/adiponectin ratio in metabolic syndrome and diabetes. Horm. Mol. Biol. Clin. Investig. 18, 37–45.
Lu, Y. C., Wang, C. P., Hsu, C. C., Chiu, C. A., Yu, T. H., Hung, W. C., et al. (2013). Circulating secreted frizzled-related protein 5 (Sfrp5) and wingless-type MMTV integration site family member 5a (Wnt5a) levels in patients with type 2 diabetes mellitus. Diabetes Metab. Res. Rev. 29, 551–556.
Luangsay, S., Wittamer, V., Bondue, B., De Henau, O., Rouger, L., Brait, M., et al. (2009). Mouse ChemR23 is expressed in dendritic cell subsets and macrophages, and mediates an anti-inflammatory activity of chemerin in a lung disease model. J. Immunol. 183, 6489–6499. doi: 10.4049/jimmunol.0901037
Madani, S., De Girolamo, S., Muñoz, D. M., Li, R. K., and Sweeney, G. (2006). Direct effects of leptin on size and extracellular matrix components of human pediatric ventricular myocytes. Cardiovasc. Res. 69, 716–725. doi: 10.1016/j.cardiores.2005.11.022
Maeda, K., Okubo, K., Shimomura, I., Funahashi, T., Matsuzawa, Y., and Matsubara, K. (1996). cDNA cloning and expression of a novel adipose specific collagen-like factor, apm1 (adiposemost abundant gene transcript 1). Biochem. Biophys. Res. Commun. 221, 286–289. doi: 10.1006/bbrc.1996.0587
Maeda, T., Jikko, A., Abe, M., Yokohama-Tamaki, T., Akiyama, H., Furukawa, S., et al. (2006). Cartducin, a paralog of Acrp30/adiponectin, is induced during chondrogenic differentiation and promotes proliferation of chondrogenic precursors and chondrocytes. J. Cell. Physiol. 206, 537–544. doi: 10.1002/jcp.20493
Maguire, J. J., Kleinz, M. J., Pitkin, S. L., and Davenport, A. P. (2009). [Pyr1]apelin-13 identified as the predominant apelin isoform in the human heart: vasoactive mechanisms and inotropic action in disease. Hypertension 54, 598–604. doi: 10.1161/hypertensionaha.109.134619
Maiese, K. (2014). WISP1: clinical insights for a proliferative and restorative member of the CCN family. Curr Neurovasc Res. 11, 378–389. doi: 10.2174/1567202611666140912115107
Maiese, K., Chong, Z. Z., Shang, Y. C., and Wang, S. (2012). Targeting disease through novel pathways of apoptosis and autophagy. Expert Opin. Ther. Targets 16, 1203–1214. doi: 10.1517/14728222.2012.719499
Maijer, K. I., Neumann, E., Muller-Ladner, U., Drop, D. A., Ramwadhdoebe, T. H., Choi, I. Y., et al. (2015). Serum vaspin levels are associated with the development of clinically manifest arthritis in autoantibody-positive individuals. PLoS One 10:e0144932. doi: 10.1371/journal.pone.0144932
Malgor, R., Bhatt, P. M., Connolly, B. A., Jacoby, D. L., Feldmann, K. J., Silver, M. J., et al. (2014). Wnt5a, TLR2 and TLR4 are elevated in advanced human atherosclerotic lesions. Inflamm. Res. 63, 277–285. doi: 10.1007/s00011-013-0697-x
Mancuso, P. (2016). The role of adipokines in chronic inflammation. Immuno Targets Ther. 5, 47–56. doi: 10.2147/itt.s73223
Manduteanu, I., Pirvulescu, M., Gan, A. M., Stan, D., Simion, V., Draomir, E., et al. (2010). Similar effects of resistin and high glucose on P-selectin and fractalkine expression and monocyte adhesion in human endothelial cells. Biochem. Biophys. Res. Commun. 391, 1443–1448. doi: 10.1016/j.bbrc.2009.12.089
Mariani, F., and Roncucci, L. (2015). Chemerin/chemR23 axis in inflammation onset and resolution. Inflamm. Res. 64, 85–95. doi: 10.1007/s00011-014-0792-7
Martinek, N., Shahab, J., Sodek, J., and Ringuette, M. (2007). Is SPARC an evolutionarily conserved collagen chaperone? J. Dent. Res. 86, 296–305. doi: 10.1177/154405910708600402
Maruyama, S., Shibata, R., Kikuchi, R., Izumiya, Y., Rokutanda, T., Araki, S., et al. (2012). Fat derived factor omentin stimulates endothelial cell function and ischemia induced revascularization via endothelial nitric oxide synthase-dependent mechanism. J. Biol. Chem. 287, 408–417. doi: 10.1074/jbc.m111.261818
Masuo, K. (2014). Nesfatin-1 could be a strong candidate obesity or diabetes medication, if blood pressure elevation can be controlled. Hypertens. Res. 37, 98–99. doi: 10.1038/hr.2013.152
Mattiotti, A., Prakash, S., Barnett, P., and van den Hoff Maurice, J. B. (2018). Follistatin-like 1 in development and human diseases. Cell. Mol. Life Sci. 75, 2339–2354. doi: 10.1007/s00018-018-2805-0
Maurer, P., Gohring, W., Sasaki, T., Mann, K., Timpl, R., and Nischt, R. (1997). Recombinant and tissue-derived mouse BM-40 bind to several collagen types and have increased affinities after proteolytic activation. Cell. Mol. Life Sci. 53, 478–484. doi: 10.1007/s000180050059
Meder, W., Wendland, M., Busmann, A., Kutzleb, C., Spodsberg, N., John, H., et al. (2003). Characterization of human circulating TIG2 as a ligand for the orphan receptor ChemR23. FEBS Lett. 555, 495–499. doi: 10.1016/s0014-5793(03)01312-7
Meier, F. M., Frommer, K. W., Peters, M. A., Brentano, F., Lefevre, S., Schroder, D., et al. (2012). Visfatin/pre-B-cell colony-enhancing factor (PBEF), a proinflammatory and cell motility-changing factor in rheumatoid arthritis. J. Biol. Chem. 287, 28378–28385. doi: 10.1074/jbc.m111.312884
Minn, A. H., Patterson, N. B., Pack, S., Hoffmann, S. C., Gavrilova, O., Vinson, C., et al. (2003). Resistin is expressed in pancreatic islets. Biochem. Biophys. Res. Commun. 310, 641–645. doi: 10.1016/j.bbrc.2003.09.061
Mittendorfer, B., Horowitz, J. F., DePaoli, A. M., McCamish, M. A., Patterson, B. W., and Klein, S. (2011). Recombinant human leptin treatment does not improve insulin action in obese subjects with type 2 diabetes. Diabetes 60, 1474–1477. doi: 10.2337/db10-1302
Miyamae, T., Marinov, A. D., Sowders, D., Wilson, D. C., Devlin, J., Boudreau, R., et al. (2006). Follistatin-like protein-1 is a novel proinflammatory molecule. J. Immunol. 177, 4758–4762. doi: 10.4049/jimmunol.177.7.4758
Miyoshi, T., Doi, M., Usui, S., Iwamoto, M., Kajiya, M., Takeda, K., et al. (2014). Low serum level of secreted frizzled-related protein 5, an anti-inflammatory adipokine, is associated with coronary artery disease. Atherosclerosis 233, 454–459. doi: 10.1016/j.atherosclerosis.2014.01.019
Mohan, V., Deepa, R., Pradeepa, R., Vimaleswaran, K. S., Mohan, A., Velmurugan, K., et al. (2005). Association of low adiponectin levels with the metabolic syndrome. the Chennai Urban Rural epidemiology study (CURES-4). Metabolism 54, 476–481. doi: 10.1016/j.metabol.2004.10.016
Möhlig, M., Weickert, M. O., Gnadamgahi, E., Arafat, A. M., Spranger, J., Pfeiffer, A. F. H., et al. (2008). Retinol-binding protein 4 is associated with insulin resistance, but appears unsuited for metabolic screening in women with polycystic ovary syndrome. Eur. J. Endocrinol. 158, 517–523. doi: 10.1530/eje-07-0833
Momin, A. U., Melikian, N., Shah, A. M., Grieve, D. J., Wheatcroft, S. B., John, L., et al. (2006). Leptin is an endothelial-independent vasodilator in humans with coronary artery disease: evidence for tissue specificity of leptin resistance. Eur. Heart J. 27, 2294–2299. doi: 10.1093/eurheartj/ehi831
Moon, H. S., Dalamaga, M., Kim, S. Y., Polyzos, S. A., Hamnvik, O. P., Magkos, F., et al. (2013). Leptin’s role in lipodystrophic and nonlipodystrophic insulin-resistant and diabetic individuals. Endocrine Rev. 34, 377–412. doi: 10.1210/er.2012-1053
Moon, H. S., Matarese, G., Brennan, A. M., Chamberland, J. P., Liu, X., Fiorenza, C. G., et al. (2011). Efficacy of metreleptin in obese patients with type 2 diabetes: cellular and molecular pathways underlying leptin tolerance. Diabetes 60, 1647–1656. doi: 10.2337/db10-1791
Moradi, N., Fadaei, R., Emamgholipour, S., Kazemian, E., Panahi, G., Vahedi, S., et al. (2018). Association of circulating CTRP9 with soluble adhesion molecules and inflammatory markers in patients with type 2 diabetes mellitus and coronary artery disease. PLoS One 13:e0192159. doi: 10.1371/journal.pone.0192159
Moreno-Navarrete, J. M., Catalán, V., Ortega, F., Gómez-Ambrosi, J., Ricart, W., Frühbeck, G., et al. (2010). Circulating omentin concentration increases after weight loss. Nutr. Metab. 7:27. doi: 10.1186/1743-7075-7-27
Moreno-Navarrete, J. M., Ortega, F., Castro, A., Sabater, M., Ricart, W., and Fernandez-Real, J. M. (2011). Circulating omentin as a novel biomarker of endothelial dysfunction. Obesity 19, 1552–1559. doi: 10.1038/oby.2010.351
Morris, D. L., and Rui, L. (2009). Recent advances in understanding leptin signaling and leptin resistance. Am. J. Physiol. Endocrinol. Metab. 297, E1247–E1259.
Motamed, K., Funk, S. E., Koyama, H., Ross, R., Raines, E. W., and Sage, E. H. (2002). Inhibition of PDGF-stimulated and matrix-mediated proliferation of human vascular smooth muscle cells by SPARC is independent of changes in cell shape or cyclin-dependent kinase inhibitors. J. Cell. Biochem. 84, 759–771. doi: 10.1002/jcb.10095
Mukherjee, S., Choi, M. J., Kim, S. W., and Yun, J. W. (2020). Secreted protein acidic and rich in cysteine (SPARC) regulates thermogenesis in white and brown adipocytes. Mol. Cell. Endocrinol. 506:110757. doi: 10.1016/j.mce.2020.110757
Munjal, I. D., McLean, N. V., Grant, M. B., and Blake, D. A. (1994). Differences in the synthesis of secreted proteins in human retinal endothelial cells of diabetic and nondiabetic origin. Curr. Eye Res. 13, 303–310. doi: 10.3109/02713689408995792
Munzberg, H., Flier, J. S., and Bjorbaek, C. (2004). Region-specific leptin resistance within the hypothalamus of diet-induced obese mice. Endocrinology 145, 4880–4889. doi: 10.1210/en.2004-0726
Murahovschi, V., Pivovarova, O., Ilkavets, I., Dimitrieva, R. M., Döcke, S., Keyhani-Nejad, F., et al. (2015). WISP1 is a novel adipokine linked to inflammation in obesity. Diabetes 64, 856–866. doi: 10.2337/db14-0444
Murakami, K., Tanaka, M., Usui, T., Kawabata, D., Shiomi, A., Iguchi-Hashimoto, M., et al. (2012). Follistatin-related protein/follistatin-like 1 evokes an innate immune response via CD14 and toll-like receptor 4. FEBS Lett. 586, 319–324. doi: 10.1016/j.febslet.2012.01.010
Muraoka, S., Kusunoki, N., Takahashi, H., Tsuchiya, K., and Kawai, S. (2013). Leptin stimulates interleukin-6 production via janus kinase 2/signal transducer and activator of transcription 3 in rheumatoid synovial fibroblasts. Clin. Exp. Rheumatol. 31, 589–595.
Murayama, M. A., Kakuta, S., Inoue, A., Umeda, N., Yonezawa, T., Maruhashi, T., et al. (2015). CTRP6 is an endogenous complement regulator that can effectively treat induced arthritis. Nat. Commun. 6:8483.
Murayama, M. A., Kakuta, S., Maruhashi, T., Shimizu, K., Seno, A., Kubo, S., et al. (2014). CTRP3 plays an important role in the development of collagen-induced arthritis in mice. Biochem. Biophys. Res. Commun. 443, 42–48. doi: 10.1016/j.bbrc.2013.11.040
Myasoedova, V. A., Chistiakov, D. A., Grechko, A. V., and Orekhov, A. N. (2018). Matrix metalloproteinases in pro-atherosclerotic arterial remodeling. J. Mol. Cell. Cardiol. 123, 159–167. doi: 10.1016/j.yjmcc.2018.08.026
Myers, M. G. Jr., Munzberg, H., Leinninger, G. M., and Leshan, R. L. (2009). The geometry of leptin action in the brain: more complicated than a simple ARC. Cell. Metab. 9, 117–123. doi: 10.1016/j.cmet.2008.12.001
Nakamura, K., Fuster, J. J., and Walsh, K. (2014). Adipokines: a link between obesity and cardiovascular disease. J Cardiol. 63, 250–259. doi: 10.1016/j.jjcc.2013.11.006
Nakamura, K., Sano, S., Fuster, J. J., Kikuchi, R., Shimizu, I., Ohshima, K., et al. (2016). Secreted frizzled-related protein 5 diminishes cardiac inflammation and protects the heart from ischemia/reperfusion injury. J. Biol. Chem. 291, 2566–2575. doi: 10.1074/jbc.m115.693937
Nakamura, S., Kamihagi, K., Satakeda, H., Katayama, M., Pan, H., Okamoto, H., et al. (1996). Enhancement of SPARC (osteonectin) synthesis in arthritic cartilage. Increased levels in synovial fluids from patients with rheumatoid arthritis and regulation by growth factors and cytokines in chondrocyte cultures. Arthritis Rheum. 39, 539–551. doi: 10.1002/art.1780390402
Nawrocki, A. R., Hofmann, S. M., Teupser, D., Basford, J. E., Durand, J. L., Jelicks, L. A., et al. (2010). Lack of association between adiponectin levels and atherosclerosis in mice. Arterioscler. Thromb. Vasc. Biol. 30, 1159–1165. doi: 10.1161/atvbaha.109.195826
Neng, Z., Li, Q., Zhigang, L., Qiong, G., Luoyan, Y., and Liao, D. (2014). Challenging role of Wnt5a and its signaling pathway in cancer metastasis (Review). Exp. Ther. Med. 8, 3–8. doi: 10.3892/etm.2014.1676
Neumann, E., Frommer, K. W., Vasile, M., and Muller-Ladner, U. (2011). Adipocytokines as driving forces in rheumatoid arthritis and related inflammatory diseases? Arthritis Rheum. 63, 1159–1169. doi: 10.1002/art.30291
Neumann, E., Junker, S., Schett, G., Frommer, K., and Muller-Ladner, U. (2016). Adipokines in bone disease. Nat. Rev. Rheumatol. 12, 296–302.
Neumeier, M., Weigert, J., Schäffler, A., Wehrwein, G., Muller-Ladner, U., Scholmerich, J., et al. (2006). Different effects of adiponectin isoforms in human monocytic cells. J. Leukoc Biol. 79, 803–808. doi: 10.1189/jlb.0905521
Nicholson, T., Church, C., Baker, D. J., and Jones, S. W. (2018). The role of adipokines in skeletal muscle inflammation and insulin sensitivity. J. Inflamm. 15:9.
Nicholson, T., Church, C., Tsintzas, K., Jones, R., Breen, L., Davis, E. T., et al. (2019). Vaspin promotes insulin sensitivity of elderly muscle and is upregulated in obesity. J. Endocrinol. 241, 31–43. doi: 10.1530/joe-18-0528
Nigro, E., Scudiero, O., Sarnataro, D., Mazzarella, G., Sofia, M., Bianco, A., et al. (2013). Adiponectin affects lung epithelial A549 cell viability counteracting TNFa and IL-1ß toxicity through AdipoR1. Int. J. Biochem. Cell Biol. 45, 1145–1153. doi: 10.1016/j.biocel.2013.03.003
Ogura, Y., Ouchi, N., Ohashi, K., Shibata, R., Kataoka, Y., Kambara, T., et al. (2012). Therapeutic impact of follistatin-like 1 on myocardial ischemic injury in preclinical models. Circulation 126, 1728–1738. doi: 10.1161/circulationaha.112.115089
Oh, I. S., Shimizu, H., Satoh, T., Okada, S., Adachi, S., Inoue, K., et al. (2006). Identification of nesfatin-1 as a satiety molecule in the hypothalamus. Nature 443, 709–712. doi: 10.1038/nature05162
Ohashi, K., Ouchi, N., Sato, K., Higuchi, A., Ishikawa, T. O., Herschman, H. R., et al. (2009). Adiponectin promotes revascularization of ischemic muscle through a cyclooxygenase 2-dependent mechanism. Mol. Cell. Biol. 29, 3487–3499. doi: 10.1128/mcb.00126-09
Okamoto, Y., Folco, E. J., Minami, M., Wara, A. K., Feinberg, M. W., Sukhova, G. K., et al. (2008). Adiponectin inhibits the production of CXC receptor 3 chemokine ligands in macrophages and reduces T-lymphocyte recruitment in atherogenesis. Circ. Res. 102, 218–225. doi: 10.1161/circresaha.107.164988
Okura, H., Yamashita, S., Ohama, T., Saga, A., Yamamoto-Kakuta, A., Hamada, Y., et al. (2010). HDL/ apolipoprotein A-I binds to macrophage-derived progranulin and suppresses its conversion into proinflammatory granulins. J. Atheroscler. Thromb. 17, 568–577. doi: 10.5551/jat.3921
Olama, S. M., Senna, M. K., and Elarman, M. (2012). Synovial/serum leptin ratio in rheumatoid arthritis: the association with activity and erosion. Rheumatol. Int. 32, 683–690. doi: 10.1007/s00296-010-1698-5
On, Y. K., Park, H. K., Hyon, M. S., and Jeon, E. S. (2007). Serum resistin as a biological marker for coronary artery disease and restenosis in type 2 diabetic patients. Circ. J. 71, 868–873. doi: 10.1253/circj.71.868
Ono, M., Inkson, C. A., Kilts, T. M., and Young, M. F. (2011). WISP-1/CCN4 regulates osteogenesis by enhancing BMP-2 activity. J. Bone Miner. Res. 26, 193–208. doi: 10.1002/jbmr.205
Osaki, A., and Shimizu, H. (2013). Peripheral administration of nesfatin-1 increases blood pressure in mice. Hypertens. Res. 37, 185–186. doi: 10.1038/hr.2013.122
Oshima, Y., Ouchi, N., Sato, K., Izumiya, Y., Pimentel, D. R., and Walsh, K. (2008). Follistatin-like 1 is an Akt-regulated cardioprotective factor that is secreted by the heart. Circulation 117, 3099–3108. doi: 10.1161/circulationaha.108.767673
Ouchi, N., Higuchi, A., Ohashi, K., Oshima, Y., Gokce, N., Shibata, R., et al. (2010). Sfrp5 is an anti-inflammatory adipokine that modulates metabolic dysfunction in obesity. Science 329, 454–457. doi: 10.1126/science.1188280
Ouchi, N., Kobayashi, H., Kihara, S., Kumada, M., Sato, K., Inoue, T., et al. (2004). Adiponectin stimulates angiogenesis by promoting cross-talk between amp-activated protein kinase and akt signaling in endothelial cells. J. Biol. Chem. 279, 1304–1309. doi: 10.1074/jbc.m310389200
Ouchi, N., Ohishi, M., Kihara, S., Funahashi, T., Nakamura, T., Nagaretani, H., et al. (2003). Association of hypoadiponectinemia with impaired vasoreactivity. Hypertension 42, 231–234. doi: 10.1161/01.hyp.0000083488.67550.b8
Ouchi, N., Oshima, Y., Ohashi, K., Higuchi, A., Ikegami, C., Izumiya, Y., et al. (2008). Follistatin-like 1, a secreted muscle protein, promotes endothelial cell function and revascularization in ischemic tissue through a nitric-oxide synthase-dependent mechanism. J. Biol. Chem. 283, 32802–32811. doi: 10.1074/jbc.m803440200
Ouchi, N., and Walsh, K. (2007). Adiponectin as an anti-inflammatory factor. Clin. Chim. Acta 380, 24–30. doi: 10.1016/j.cca.2007.01.026
Ozgen, M., Koca, S. S., Dagli, N., Balin, M., Ustundag, B., and Isik, A. (2010). Serum adiponectin and vaspin levels in rheumatoid arthritis. Arch. Med. Res. 41, 457–463. doi: 10.1016/j.arcmed.2010.08.012
Palanivel, R., Eguchi, M., Shuralyova, I., Coe, I., and Sweeney, G. (2006). Distinct effects of short- and longterm leptin treatment on glucose and fatty acid uptake and metabolism in HL-1 cardiomyocytes. Metabolism 55, 1067–1075. doi: 10.1016/j.metabol.2006.03.020
Pandit, R., Beerens, S., and Adan, R. A. H. (2017). Role of leptin in energy expenditure: the hypothalamic perspective. Am. J. Physiol. Regul. Integr. Comp. Physiol. 312, R938–R947.
Park, H. K., and Ahima, R. S. (2013). Resistin in rodents and humans. Diabetes Metab. J. 37, 404–414. doi: 10.4093/dmj.2013.37.6.404
Park, H. K., and Ahima, R. S. (2015). Physiology of leptin energy homeostasis, neuroendocrine function and metabolism. Metabolism 64, 24–34. doi: 10.1016/j.metabol.2014.08.004
Park, H. K., Kwak, M. K., Kim, H. J., and Ahima, R. S. (2017). Linking resistin, inflammation, and cardiometabolic diseases. Korean J. Intern. Med. 32, 239–247. doi: 10.3904/kjim.2016.229
Park, M. Y., Kim, H. S., Lee, M., Park, B., Lee, H. Y., Cho, E. B., et al. (2017). FAM19A5, a brain-specific chemokine, inhibits RANKL-induced osteoclast formation through formyl peptide receptor 2. Sci. Rep. 7:15575.
Pashirzad, M., Shafiee, M., Rahmani, F., Behnam-Rassouli, R., Hoseinkhani, F., Ryzhikov, M., et al. (2017). Role of Wnt5a in the pathogenesis of inflammatory diseases. J. Cell. Physiol. 232, 1611–1616. doi: 10.1002/jcp.25687
Patel, L., Buckels, A. C., Kinghorn, I. J., Murdock, R., Holbrook, J. D., Plumpton, C., et al. (2003). Resistin is expressed in human macrophages and directly regulated by PPARγ activators. Biochem. Biophys. Res. Commun. 300, 472–476. doi: 10.1016/s0006-291x(02)02841-3
Paulsen, S. J., Christensen, M. T., Vrang, N., and Larsen, L. K. (2008). The putative neuropeptide TAFA5 is expressed in the hypothalamic paraventricular nucleus and is regulated by dehydration. Brain Res. 1199, 1–9. doi: 10.1016/j.brainres.2007.12.074
Pereira, C., Schaer, D. J., Bachli, E. B., Kurrer, M. O., and Schoedon, G. (2008). Wnt5A/CaMKII signaling contributes to the inflammatory response of macrophages and is a target for the antiinflammatory action of activated protein C and interleukin-10. Arterioscler. Thromb. Vasc. Biol. 28, 504–510. doi: 10.1161/atvbaha.107.157438
Perseghin, G., Lattuada, G., De Cobelli, F., Esposito, A., Belloni, E., Canue, T., et al. (2007). Serum retinol-binding protein-4, leptin, and adiponectin concentrations are related to ectopic fat accumulation. J. Clin. Endocrinol. Metab. 92, 4883–4888. doi: 10.1210/jc.2007-0325
Petersen, P. S., Lei, X., Wolf, R. M., Rodriguez, S., Tan, S. Y., Little, H. C., et al. (2017). CTRP7 deletion attenuates obesity-linked glucose intolerance, adipose tissue inflammation, and hepatic stress. Am. J. Physiol. Endocrinol. Metab. 312, E309–E325.
Peterson, J. M., Aja, S., Wei, Z., and Wong, G. W. (2012). C1q/TNF-related protein-1 (CTRP1) enhances fatty acid oxidation via AMPK activation and ACC inhibition. J. Biol. Chem. 287, 1576–1587. doi: 10.1074/jbc.m111.278333
Peterson, J. M., Seldin, M. M., Tan, S. Y., and Wong, G. W. (2014). CTRP2 overexpression improves insulin and lipid tolerance in diet-induced obese mice. PLoS One 9:e88535. doi: 10.1371/journal.pone.0088535
Peterson, J. M., Wei, Z., and Wong, G. W. (2010). C1q/TNF-related protein-3 (CTRP3), a novel adipokine that regulates hepatic glucose output. J. Biol. Chem. 285, 39691–39701. doi: 10.1074/jbc.m110.180695
Phalitakul, S., Okada, M., Hara, Y., and Yamawaki, H. (2011). Vaspin prevents TNF-α-induced intracellular adhesion molecule-1 via inhibiting reactive oxygen species-dependent NF-κB and PKCθ activation in cultured rat vascular smooth muscle cells. Pharmacol. Res. 64, 493–500. doi: 10.1016/j.phrs.2011.06.001
Price, R. M., Tulsyan, N., Dermody, J. J., Schwalb, M., Soteropoulos, P., and Castronuovo, J. J. (2004). Gene expression after crush injury of human saphenous vein: using microarrays to define the transcriptional profile. J. Am. Coll. Surg. 199, 411–418. doi: 10.1016/j.jamcollsurg.2004.04.023
Procaccini, C., Jirillo, E., and Matarese, G. (2012). Leptin as an immunomodulator. Mol. Asp. Med. 33, 35–45. doi: 10.1016/j.mam.2011.10.012
Qi, Q., Yu, Z., Ye, X., Zhao, F., Huang, P., Hu, F. B., et al. (2007). Elevated retinol-binding protein 4 levels are associated with metabolic syndrome in Chinese people. J. Clin. Endocrinol. Metab. 92, 4827–4834. doi: 10.1210/jc.2007-1219
Qu, H., Deng, H., and Hu, Z. (2013). Plasma progranulin concentrations are increased in patients with type 2 diabetes and obesity and correlated with insulin resistance. Mediators Inflamm. 2013:360190.
Rabe, K., Lehrke, M., Parhofer, K. G., and Broedl, U. C. (2008). Adipokines and insulin resistance. Mol. Med. 14, 741–751.
Raines, E. W., Lane, T. F., Iruela-Arispe, M. L., Ross, R., and Sage, E. H. (1992). The extracellular glycoprotein SPARC interacts with platelet-derived growth factor (PDGF)-AB and -BB and inhibits the binding of PDGF to its receptors. Proc. Natl. Acad. Sci. U.S.A. 89, 1281–1285. doi: 10.1073/pnas.89.4.1281
Rajala, M. W., Lin, Y., Ranalletta, M., Yang, X. M., Qian, H., Gingerich, R., et al. (2002). Cell type-specific expression and coregulation of murine resistin and resistin-like molecule-α in adipose tissue. Mol. Endocrinol. 16, 1920–1930. doi: 10.1210/me.2002-0048
Rajala, M. W., Qi, Y., Patel, H. R., Takahashi, N., Banerjee, R., Pajvani, U. B., et al. (2004). Regulation of resistin expression and circulating levels in obesity, diabetes, and fasting. Diabetes 53, 1671–1679. doi: 10.2337/diabetes.53.7.1671
Ramesh, N., Gawli, K., Pasupulleti, V. K., and Unniappan, S. (2017). Metabolic and cardiovascular actions of nesfatin-1: implications in health and disease. Curr. Pharm. Des. 23, 1453–1464. doi: 10.2174/1381612823666170130154407
Rangwala, S. M., Rich, A. S., Rhoades, B., Shapiro, J. S., Obici, S., Rossetti, L., et al. (2004). Abnormal glucose homeostasis due to chronic hyperresistinemia. Diabetes 53, 1937–1941. doi: 10.2337/diabetes.53.8.1937
Rauner, M., Stein, N., Winzer, M., Goettsch, C., Zwerina, J., Schett, G., et al. (2012). WNT5A is induced by inflammatory mediators in bone marrow stromal cells and regulates cytokine and chemokine production. J. Bone Miner. Res. 27, 575–585. doi: 10.1002/jbmr.1488
Reddy, V. S., Valente, A. J., Delafontaine, P., and Chandrasekar, B. (2011). Interleukin-18/WNT1-inducible signaling pathway protein-1 signaling mediates human saphenous vein smooth muscle cell proliferation. J. Cell. Physiol. 226, 3303–3315. doi: 10.1002/jcp.22676
Robertson, S. A., Leinninger, G. M., and Myers, M. G. Jr. (2008). Molecular and neural mediators of leptin action. Physiol. Behav. 94, 637–642. doi: 10.1016/j.physbeh.2008.04.005
Robey, P. G., and Boskey, A. L. (2003). “Extracellular matrix and biomineraliztion of bone,” in Primer on the Metabolic Bone Diseases and Disorders of Mineral Metabolism, ed. M. J. Favus (Washington, DC: American Society for Bone and Mineral Research), 38–45.
Robinson, C., Tsang, L., Solomon, A., Woodiwiss, A. J., Gunter, S., Mer, M., et al. (2018). Nesfatin-1 and visfatin expression is associated with reduced atherosclerotic disease risk in patients with rheumatoid arthritis. Peptides 102, 31–37. doi: 10.1016/j.peptides.2018.02.002
Robinson, C., Tsang, L., Solomon, A., Woodiwiss, A. J., Gunter, S., Millen, A. M., et al. (2017). Omentin concentrations are independently associated with those of matrix metalloproteinase-3 in patients with mild but not severe rheumatoid arthritis. Rheumatol. Int. 37, 3–11. doi: 10.1007/s00296-016-3541-0
Roman, A. A., Parlee, D., and Sinal, C. J. (2012). Chemerin: a potential endocrine link between obesity and type 2 diabetes. Endocrine 42, 243–251. doi: 10.1007/s12020-012-9698-8
Rosell, M., Hondares, E., Iwamoto, S., Gonzalez, F. J., Wabitsch, M., Staels, B., et al. (2012). Peroxisome proliferator-activated receptors-α and -γ, and cAMP-mediated pathways, control retinol-binding protein-4 gene expression in brown adipose tissue. Endocrinology 153, 1162–1173. doi: 10.1210/en.2011-1367
Rosenbaum, M., Goldsmith, R., Bloomfield, D., Magnano, A., Weimer, L., and Heymsfield, S. (2005). Low-dose leptin reverses skeletal muscle, autonomic, and neuroendocrine adaptations to maintenance of reduced weight. J. Clin. Invest. 115, 3579–3586. doi: 10.1172/jci25977
Rosenbaum, M., and Leibel, R. L. (1999). The role of leptin in human physiology. N. Engl. J. Med. 341, 913–915. doi: 10.1056/nejm199909163411211
Rosenblatt, S., Bassuk, J. A., Alpers, C. E., Sage, E. H., Timpl, R., and Preissner, K. T. (1997). Differential modulation of cell adhesion by interaction between adhesive and counter-adhesive proteins: characterization of the binding of vitronectin to osteonectin (BM40, SPARC). Biochem. J. 324, 311–319. doi: 10.1042/bj3240311
Roth, J. D., Roland, B. L., Cole, R. L., Trevaskis, J. L., Weyer, C., Koda, J. E., et al. (2008). Leptin responsiveness restored by amylin agonism in diet-induced obesity: evidence from nonclinical and clinical studies. Proc. Natl. Acad. Sci. U.S.A. 105, 7257–7262. doi: 10.1073/pnas.0706473105
Rychter, A. M., Skrzypczak-Zielińska, M., Zielińska, A., Eder, P., Souto, E. B., Zawada, A., et al. (2020). Is the retinol-binding protein 4 a possible risk factor for cardiovascular diseases in obesity? Int. J. Mol. Sci. 21:E5229.
Safoura, A., and Gholam, B. (2018). Association of low serum level of secreted frizzled-related protein 5 (SFRP5) with the presence and severity of coronary artery disease. J. Basic Res. Med. Sci. 5, 35–40. doi: 10.29252/jbrms.5.4.35
Sage, H., Vernon, R. B., Funk, S. E., Everitt, E. A., and Angello, J. (1989). SPARC, a secreted protein associated with cellular proliferation, inhibits cell spreading in vitro and exhibits Ca+2-dependent binding to the extracellular matrix. J. Cell. Biol. 109, 341–356. doi: 10.1083/jcb.109.1.341
Sahin Ersoy, G., Altun Ensari, T., Subas, S., Giray, B., Simsek, E. E., and Cevik, O. (2016). WISP1 is a novel adipokine linked to metabolic parameters in gestational diabetes mellitus. J. Matern. Fetal. Neonatal. Med. 30, 942–946. doi: 10.1080/14767058.2016.1192118
Sasaki, T., Gohring, W., Mann, K., Maurer, P., Hohenester, E., Knäuper, V., et al. (1997). Limited cleavage of extracellular matrix protein BM-40 by matrix metalloproteinases increases its affinity for collagens. J. Biol. Chem. 272, 9237–9243. doi: 10.1074/jbc.272.14.9237
Sato, K., Shirai, R., Yamaguchi, M., Yamashita, T., Shibata, K., Okano, T., et al. (2018). Anti-atherogenic effects of vaspin on human aortic smooth muscle cell/macrophage responses and hyperlipidemic mouse plaque phenotype. Int. J. Mol. Sci. 19, E1732.
Satoh, H., Nguyen, M. T., Miles, P. D., Imamura, T., Usui, I., and Olefsky, J. M. (2014). Adenovirus-mediated chronic “hyper-resistinemia” leads to in vivo insulin resistance in normal rats. J. Clin. Invest. 114, 224–231. doi: 10.1172/jci20785
Scarpace, P. J., Matheny, M., Pollock, B. H., and Tümer, N. (1997). Leptin increases uncoupling protein expression and energy expenditure. Am. J. Physiol. 273(1 Pt 1), E226–E230.
Schäffler, A., Büchler, C., Müller-Ladner, U., Herfarth, H., Ehling, A., Paul, G., et al. (2004). Identification of variables influencing resistin serum levels in patients with type 1 and type 2 diabetes mellitus. Horm. Metab. Res. 36, 702–707. doi: 10.1055/s-2004-826015
Schäffler, A., and Buechler, C. (2012). CTRP family: linking immunity to metabolism. Trends Endocrinol. Metab. 23, 194–204. doi: 10.1016/j.tem.2011.12.003
Schellings, M. W., Vanhoutte, D., Swinnen, M., Cleutjens, J. P., Debets, J., van Leeuwen, R. E., et al. (2009). Absence of SPARC results in increased cardiac rupture and dysfunction after acute myocardial infarction. J. Exp. Med. 206, 113–123. doi: 10.1084/jem.20081244
Scherer, P. E., Williams, S., Fogliano, M., Baldini, G., and Lodish, H. F. (1995). A novel serum protein similar to C1q, produced exclusively in adipocytes. J. Biol. Chem. 270, 26746–26749. doi: 10.1074/jbc.270.45.26746
Schinzari, F., Veneziani, A., Mores, N., Barini, A., Di Daniele, N., Cardillo, C., et al. (2017). Beneficial effects of apelin on vascular function in patients with central obesity. Hypertension 69, 942–949. doi: 10.1161/hypertensionaha.116.08916
Schulte, D. M., Müller, N., Neumann, K., Oberhäuser, F., Faust, M., Güdelhöfer, H., et al. (2012). Pro-inflammatory wnt5a and anti-inflammatory sFRP5 are differentially regulated by nutritional factors in obese human subjects. PLoS One 7:e32437. doi: 10.1371/journal.pone.0032437
Schwartz, M. W., Woods, S. C., Porte, D. Jr., Seeley, R. J., and Baskin, D. G. (2000). Central nervous system control of food intake. Nature 404, 661–671. doi: 10.1038/35007534
Scotece, M., Conde, J., Abella, V., Lopez, V., Lago, F., Pino, J., et al. (2014a). NUCB2/nesfatin-1: a new adipokine expressed in human and murine chondrocytes with pro-inflammatory properties, an in vitro study. J. Orthop. Res. 32, 653–660. doi: 10.1002/jor.22585
Scotece, M., Conde, J., Vuolteenaho, K., Koskinen, A., Lopez, V., Gomez-Reino, J., et al. (2014b). Adipokines as drug targets in joint and bone disease. Drug Discov. Today 19, 241–258. doi: 10.1016/j.drudis.2013.07.012
Seeger, J., Ziegelmeier, M., Bachmann, A., Lössner, U., Kratzsch, J., Blüher, M., et al. (2008). Serum levels of the adipokine vaspin in relation to metabolic and renal parameters. J. Clin. Endocrinol. Metab. 93, 247–251. doi: 10.1210/jc.2007-1853
Seldin, M. M., Lei, X., Tan, S. Y., Stanson, K. P., Wei, Z., and Wong, G. W. (2013). Skeletal muscle-derived myonectin activates the mTOR pathway to suppress autophagy in liver. J. Biol. Chem. 288, 36073–36082. doi: 10.1074/jbc.m113.500736
Seldin, M. M., Peterson, J. M., Byerly, M. S., Wei, Z., and Wong, G. W. (2012). Myonectin (CTRP15), a novel myokine that links skeletal muscle to systemic lipid homeostasis. J. Biol. Chem. 287, 11968–11980. doi: 10.1074/jbc.m111.336834
Seldin, M. M., Tan, S. Y., and Wong, G. W. (2014). Metabolic function of the CTRP family of hormones. Rev. Endocr. Metab. Disord. 15, 111–123. doi: 10.1007/s11154-013-9255-7
Sen, M., Chamorro, M., Reifert, J., Corr, M., and Carson, D. A. (2001). Blockade of Wnt-5A/frizzled 5 signaling inhibits rheumatoid synoviocyte activation. Arthritis Rheum. 44, 772–781. doi: 10.1002/1529-0131(200104)44:4<772::aid-anr133>3.0.co;2-l
Sen, M., Lauterbach, K., El-Gabalawy, H., Firestein, G. S., Corr, M., and Carson, D. A. (2000). Expression and function of wingless and frizzled homologs in rheumatoid arthritis. Proc. Natl. Acad. Sci. U.S.A. 97, 2791–2796. doi: 10.1073/pnas.050574297
Senolt, L., Polanska, M., Filkova, M., Cerezo, L. A., Pavelka, K., Gay, S., et al. (2010). Vaspin and omentin: New adipokines differentially regulated at the site of inflammation in rheumatoid arthritis. Ann. Rheum. Dis. 69, 1410–1411. doi: 10.1136/ard.2009.119735
Senthilkumar, G. P., Anithalekshmi, M. S., Yasir, M., Parameswaran, S., Packirisamy, R. M., and Bobby, Z. (2018). Role of omentin 1 and IL-6 in type 2 diabetes mellitus patients with diabetic nephropathy. Diab. Metab. Syndrome 12, 23–26. doi: 10.1016/j.dsx.2017.08.005
Shafaei, A., Marjani, A., and Khoshnia, M. (2016). Serum progranulin levels in type 2 diabetic patients with metabolic syndrome. Rom. J. Intern. Med. 54, 211–216. doi: 10.1515/rjim-2016-0034
Shahapal, A., Cho, B. E., Yong, J. H., Jeong, I., Kwak, H., Lee, K. J., et al. (2019). FAM19A5 expression during embryogenesis and in the adult traumatic brain of FAM19A5-LacZ knock-in mice. Front. Neurosci. 13:917. doi: 10.3389/fnins.2019.00917
Shen, F., Hu, Z., Goswami, J., and Gaffen, S. L. (2006). Identification of common transcriptional regulatory elements in interleukin-17 target genes. J. Biol. Chem. 281, 24138–24148. doi: 10.1074/jbc.m604597200
Shen, Y., Zhao, Y., Yuan, L., Yi, W., Zhao, R., Yi, Q., et al. (2014). SPARC is over-expressed in adipose tissues of diet-induced obese rats and causes insulin resistance in 3T3-L1 adipocytes. Acta Histochem. 116, 158–166. doi: 10.1016/j.acthis.2013.06.004
Shibanuma, M., Mashimo, J., Mita, A., Kuroki, T., and Nose, K. (1993). Cloning from a mouse osteoblastic cell line of a set of transforming-growth-factor-beta 1-regulated genes, one of which seems to encode a follistatin-related polypeptide. Eur. J. Biochem. 217, 13–19. doi: 10.1111/j.1432-1033.1993.tb18212.x
Shibata, R., Ouchi, N., Kihara, S., Sato, K., Funahashi, T., and Walsh, K. (2004). Adiponectin stimulates angiogenesis in response to tissue ischemia through stimulation of AMP-activated protein kinase signaling. J. Biol. Chem. 279, 28670–28674. doi: 10.1074/jbc.m402558200
Shimizu, H., Oh-I, S., Okada, S., and Mori, M. (2009). Nesfatin-1: an overview and future clinical application. Endocr. J. 56, 537–543. doi: 10.1507/endocrj.k09e-117
Shu, X., and Chi, L. (2019). Effect of pravastatin treatment on circulating adiponectin: a meta-analysis of randomized controlled trials. Drug. Des. Devel. Ther. 13, 1633–1641. doi: 10.2147/dddt.s186992
Simonds, S. E., Pryor, J. T., Ravussin, E., Greenway, F. L., Dileone, R., Allen, A. M., et al. (2014). Leptin mediates the increase in blood pressure associated with obesity. Cell 159, 1404–1416. doi: 10.1016/j.cell.2014.10.058
Sivalingam, Z., Larsen, S. B., Grove, E. L., Hvas, A. M., Kristensen, S. D., and Magnusson, N. E. (2017). Neutrophil gelatinase-associated lipocalin as a risk marker in cardiovascular disease. Clin. Chem. Lab. Med. 56, 5–18.
Smith, C. C. T., Mocanu, M. M., Davidson, S. M., Wynne, A. M., Simpkin, J. C., and Yellon, D. M. (2006). Leptin, the obesity-associated hormone, exhibits direct cardioprotective effects. Br. J. Pharmacol. 149, 5–13. doi: 10.1038/sj.bjp.0706834
Sommer, G., Weise, S., Kralisch, S., Lossner, U., Bluher, M., Stumvoll, M., et al. (2009). Lipocalin-2 is induced by interleukin-1beta in murine adipocytes in vitro. J. Cell. Biochem. 106, 103–108. doi: 10.1002/jcb.21980
Staikos, C., Ververidis, A., Drosos, G., Manolopoulos, V. G., Verettas, D. A., and Tavridou, A. (2013). The association of adipokine levels in plasma and synovial fluid with the severity of knee osteoarthritis. Rheumatology 52, 1077–1083. doi: 10.1093/rheumatology/kes422
Stančík, M., Ságová, I., Kantorová, E., and Mokáň, M. (2017). The role of vaspin as a predictor of coronary angiography result in SCAD (stable coronary artery disease) patients. BMC Cardiovasc. Disord. 17:117. doi: 10.1186/s12872-017-0550-1
Stefan, N., Hennige, A. M., Staiger, H., Machann, J., Schick, F., Schleicher, E., et al. (2007). High circulating retinol-binding protein 4 is associated with elevated liver fat but not with total, subcutaneous, visceral, or intramyocellular fat in humans. Diabetes Care 30, 1173–1178. doi: 10.2337/dc06-2342
Stejskal, D., Ruzicka, V., Adamovska, S., Jurakova, R., Proskova, J., Jedelsky, L., et al. (2003). Adiponectin concentrations as a criterion of metabolic control in persons with type2 diabetes mellitus. Biomed. Pap. Med. Fac. Univ. Palacky Olomouc. Czech Repub. 147, 167–172. doi: 10.5507/bp.2003.023
Stelmanska, E., Sledzinski, T., Turyn, J., Presler, M., Korczynska, J., and Swierczynski, J. (2013). Chemerin gene expression is regulated by food restriction and food restriction refeeding in rat adipose tissue but not in liver. Regul. Pept. 181, 22–29. doi: 10.1016/j.regpep.2012.12.001
Stengel, A., and Taché, Y. (2010). Nesfatin-1-role as possible new potent regulator of food intake. Regul. Pept. 163, 18–23. doi: 10.1016/j.regpep.2010.05.002
Stengel, A., and Taché, Y. (2013). Role of brain NUCB2/nesfatin-1 in the regulation of food intake. Curr. Pharm. Des. 19, 6955–6959. doi: 10.2174/138161281939131127125735
Steppan, C. M., Bailey, S. T., Bhat, S., Brown, E. J., Banerjee, R. R., Wright, C. M., et al. (2001a). The hormone resistin links obesity to diabetes. Nature 409, 307–312. doi: 10.1038/35053000
Steppan, C. M., Brown, E. J., Wright, C. M., Bhat, S., Banerjee, R. R., Dai, C. Y., et al. (2001b). A family of tissue-specific resistin-like molecules. Proc. Natl. Acad. Sci. U.S.A. 98, 502–506. doi: 10.1073/pnas.98.2.502
Steppan, C. M., and Lazar, M. A. (2002). Resistin and obesity-associated insulin resistance. Trends Endocrinol. Metab. 13, 18–23. doi: 10.1016/s1043-2760(01)00522-7
Su, K. Z., Li, Y. R., Zhang, D., Yuan, J. H., Zhang, C. S., Liu, Y., et al. (2019). Relation of circulating resistin to insulin resistance in type 2 diabetes and obesity: a systematic review and meta-analysis. Front. Physiol. 10:1399. doi: 10.3389/fphys.2019.01399
Subedi, A., and Park, P. H. (2013). Autocrine and paracrine modulation of microRNA-155 expression by globular adiponectin in RAW 264.7 macrophages: involvement of MAPK/NF-κB pathway. Cytokine 64, 638–641. doi: 10.1016/j.cyto.2013.09.011
Sylva, M., Li, V. S., Buffing, A. A., van Es, J. H., van den Born, M., van der Velden, S., et al. (2011). The BMP antagonist follistatin-like 1 is required for skeletal and lung organogenesis. PLoS One 6:e22616. doi: 10.1371/journal.pone.0022616
Tacke, C., Aleksandrova, K., Rehfeldt, M., Murahovschi, V., Markova, M., Kemper, M., et al. (2018). Assessment of circulating Wnt1 inducible signalling pathway protein 1 (WISP-1)/CCN4 as a novel biomarker of obesity. J. Cell Commun. Signal. 12, 539–548. doi: 10.1007/s12079-017-0427-1
Takata, Y., Osawa, H., Kurata, M., Kurokawa, M., Yamauchi, J., Ochi, M., et al. (2008). Hyperresistinemia is associated with coexistence of hypertension and type 2 diabetes. Hypertension 51, 534–539. doi: 10.1161/hypertensionaha.107.103077
Takebayashi, K., Suetsugu, M., Wakabayashi, S., Aso, Y., and Inukai, T. (2007). Retinol binding protein-4 levels and clinical features of type 2 diabetes patients. J. Clin. Endocrinol. Metab. 92, 2712–2719. doi: 10.1210/jc.2006-1249
Tan, B. K., Adya, R., and Randeva, H. S. (2010). Omentin: a novel link between inflammation, diabesity, and cardiovascular disease. Trends Cardiovasc. Med. 20, 143–148. doi: 10.1016/j.tcm.2010.12.002
Tanaka, K., Valero-Munoz, M., Wilson, R. M., Essick, E. E., Fowler, C. T., Nakamura, K., et al. (2016). Follistatin like 1 regulates hypertrophy in heart failure with preserved ejection fraction. JACC Basic Transl. Sci. 1, 207–221. doi: 10.1016/j.jacbts.2016.04.002
Taneda, S., Pippin, J. W., Sage, E. H., Hudkins, K. L., Takeuchi, Y., Couser, W. G., et al. (2003). Amelioration of diabetic nephropathy in SPARC-null mice. J. Am. Soc. Nephrol. 14, 968–980. doi: 10.1097/01.asn.0000054498.83125.90
Tang, C. H., Fu, X. J., Xu, X. L., Wei, X. J., and Pan, H. S. (2012). The anti-inflammatory and anti-apoptotic effects of nesfatin-1 in the traumatic rat brain. Peptides 36, 39–45. doi: 10.1016/j.peptides.2012.04.014
Tang, Q., Chen, C., Zhang, Y., Dai, M., Jiang, Y., and Wang, H. (2018). Wnt5a regulates the cell proliferation and adipogenesis via MAPK-independent pathway in early stage of obesity. Cell. Biol. Int. 42, 63–74. doi: 10.1002/cbin.10862
Tang, S. Y., Xie, H., Yuan, L. Q., Luo, X. H., Huang, J., Cui, R. R., et al. (2007). Apelin stimulates proliferation and suppresses apoptosis of mouse osteoblastic cell line MC3T3-E1 via JNK and PI3-K/Akt signaling pathways. Peptides 28, 708–718. doi: 10.1016/j.peptides.2006.10.005
Tang, W., Lu, Y., Tian, Q. Y., Zhang, Y., Guo, F. J., Liu, G. Y., et al. (2011). The growth factor progranulin binds to TNF receptors and is therapeutic against inflammatory arthritis in mice. Science 332, 478–484. doi: 10.1126/science.1199214
Teo, J. L., and Kahn, M. (2010). The Wnt signaling pathway in cellular proliferation and differentiation: a tale of two coactivators. Adv. Drug Deliv. Rev. 62, 1149–1155. doi: 10.1016/j.addr.2010.09.012
Termine, J. D., Kleinman, H. K., Whitson, S. W., Conn, K. M., McGarvey, M. L., and Martin, G. R. (1981). Osteonectin, a bone-specific protein linking mineral to collagen. Cell 26, 99–105. doi: 10.1016/0092-8674(81)90037-4
Thommesen, L., Stunes, A. K., Monjo, M., Grøsvik, K., Tamburstuen, M. V., Kjøbli, E., et al. (2006). Expression and regulation of resistin in osteoblasts and osteoclasts indicate a role in bone metabolism. J. Cell. Biochem. 99, 824–834. doi: 10.1002/jcb.20915
Thomopoulos, C., Daskalaki, M., Papazachou, O., Rodolakis, N., Bratsas, A., Papadopoulos, D. P., et al. (2011). Association of resistin and adiponectin with different clinical blood pressure phenotypes. J. Hum. Hypertens. 25, 38–46. doi: 10.1038/jhh.2010.22
Tian, G., Liang, J. N., Wang, Z. Y., and Zhou, D. (2014). Emerging role of leptin in rheumatoid arthritis. Clin. Exp. Immunol. 177, 557–570. doi: 10.1111/cei.12372
Tom Tang, Y., Emtage, P., Funk, W. D., Hu, T., Arterburn, M., Park, E. E., et al. (2004). TAFA: a novel secreted family with conserved cysteine residues and restricted expression in the brain. Genomics 83, 727–734. doi: 10.1016/j.ygeno.2003.10.006
Tong, S., Ji, Q., Du, Y., Zhu, X., Zhu, C., and Zhou, Y. (2019). Sfrp5/Wnt pathway: a protective regulatory system in atherosclerotic cardiovascular disease. J. Interferon. Cytokine Res. 39, 472–482. doi: 10.1089/jir.2018.0154
Tourniaire, F., Romier-Crouzet, B., Lee, J. H., Marcotorchino, J., Gouranton, E., Salles, J., et al. (2013). Chemokine expression in inflamed adipose tissue is mainly mediated by NF-κB. PLoS One 8:e66515. doi: 10.1371/journal.pone.0066515
Trevaskis, J. L., Parkes, D. G., and Roth, J. D. (2010). Insights into amylin-leptin synergy. Trends Endocrinol. Metab. 21, 473–479. doi: 10.1016/j.tem.2010.03.006
Trujillo, M. E., and Scherer, P. E. (2006). Adipose tissue-derived factors: impact on health and disease. Endocr. Rev. 27, 762–778. doi: 10.1210/er.2006-0033
Tsuji, S., Uehori, J., Matsumoto, M., Suzuki, Y., Matsuhisa, A., Toyoshima, K., et al. (2001). Human intelectin is a novel soluble lectin that recognises galactofuranose in carbohydrate chains of bacterial cell wall. J. Biol. Chem. 276, 23456–23463. doi: 10.1074/jbc.m103162200
Tunçez, A., Altunkeser, B. B., Öztürk, B., Ateş, M. S., Tezcan, H., Aydoğan, C., et al. (2019). Comparative effects of atorvastatin 80 mg and rosuvastatin 40 mg on the levels of serum endocan, chemerin, and galectin-3 in patients with acute myocardial infarction. Anatol. J. Cardiol. 22, 240–249.
Vague, P., Juhan-Vague, I., Aillaud, M. F., Viard, R., Alessi, M. C., and Collen, D. (1986). Correlation between blood fibrinolytic activity, plasminogen activator inhibitor level, plasma insulin level, and relative body weight in normal and obese subjects. Metab. Clin. Exp. 35, 250–253. doi: 10.1016/0026-0495(86)90209-x
van den Bosch, M. H., Blom, A. B., Kram, V., Maeda, A., Sikka, S., Gabet, Y., et al. (2017). WISP1/CCN4 aggravates cartilage degeneration in experimental osteoarthritis. Osteoarthr. Cartilag. 25, 1900–1911. doi: 10.1016/j.joca.2017.07.012
van den Bosch, M. H., Blom, A. B., van Lent, P. L., van Beuningen, H. M., Blaney Davidson, E. N., van der Kraan, P. M., et al. (2014). Canonical Wnt signaling skews TGF-β signaling in chondrocytes towards signaling via ALK1 and Smad 1/5/8. Cell Signal. 26, 951–958. doi: 10.1016/j.cellsig.2014.01.021
van den Bosch, M. H. J., Ramos, Y. F. M., de Hollander, W., Bomer, N., Nelissen, R. G. H. H., Bovée, J. V. M. G., et al. (2019). Increased WISP1 expression in human osteoarthritic articular cartilage is epigenetically regulated and decreases cartilage matrix production. Rheumatology 58, 1065–1074. doi: 10.1093/rheumatology/key426
Vendrell, J., Broch, M., Vilarrasa, N., Molina, A., Gòmez, J. M., Gutičrrez, C., et al. (2004). Resistin, adiponectin, ghrelin, leptin, and proinflammatory cytokines: relationships in obesity. Obes. Res. 12, 962–971. doi: 10.1038/oby.2004.118
Venkatesan, B., Prabhu, S. D., Venkatachalam, K., Mummidi, S., Valente, A. J., Clark, R. A., et al. (2010). WNT1- inducible signaling pathway protein-1 activates diverse cell survival pathways and blocks doxorubicin-induced cardiomyocyte death. Cell. Signal. 22, 809–820. doi: 10.1016/j.cellsig.2010.01.005
Villarreal-Molina, M. T., and Antuna-Puente, B. (2012). Adiponectin: anti-inflammatory and cardioprotective effects. Biochimie 94, 2143–2149. doi: 10.1016/j.biochi.2012.06.030
Villarroya, F., Cereijo, R., Gavaldà-Navarro, A., Villarroya, J., and Giralt, M. (2018). Inflammation of brown/beige adipose tissues in obesity and metabolic disease. J. Intern. Med. 284, 492–504. doi: 10.1111/joim.12803
Violi, F., Ferro, D., and Valesini, G. (1990). Tissue plasminogen activator inhibitor in patients with systemic lupus erythematosus and thrombosis. Br. Med. J. 300, 1099–1102. doi: 10.1136/bmj.300.6732.1099
von Eynatten, M., and Humpert, P. M. (2008). Retinol-binding protein-4 in experimental and clinical metabolic disease. Expert Rev. Mol. Diagn. 8, 289–299. doi: 10.1586/14737159.8.3.289
Wada, J. (2008). Vaspin: a novel serpin with insulin-sensitizing effects. Expert Opin. Investig. Drugs 17, 327–333. doi: 10.1517/13543784.17.3.327
Wang, H., Chen, F., Li, J., Wang, Y., Jiang, C., Wang, Y., et al. (2020). Vaspin antagonizes high fat-induced bone loss in rats and promotes osteoblastic differentiation in primary rat osteoblasts through Smad-Runx2 signaling pathway. Nutr. Metab. 17:9.
Wang, H., Wang, R., Du, D., Li, F., and Li, Y. (2016). Serum levels of C1q/TNF-related protein-1 (CTRP1) are closely associated with coronary artery disease. BMC Cardiovasc. Disord. 16:92. doi: 10.1186/s12872-016-0266-7
Wang, H., Workman, G., Chen, S., Barker, T. H., Ratner, B. D., Sage, H., et al. (2006). Secreted protein acidic and rich in cysteine (SPARC/osteonectin/BM-40) binds to fibrinogen fragments D and E, but not to native fibrinogen. Matrix Biol. 25, 20–26. doi: 10.1016/j.matbio.2005.09.004
Wang, J., Li, H., Franco, O. H., Yu, Z., Liu, Y., and Lin, X. (2008). Adiponectin and metabolic syndrome in middle-aged and elderly Chinese. Obesity 16, 172–178. doi: 10.1038/oby.2007.42
Wang, Q., Guo, F., Sun, X., Gao, S., Li, Z., Gong, Y., et al. (2014). Effects of exogenous nesfatin-1 on gastric distention-sensitive neurons in the central nucleus of the amygdala and gastric motility in rats. Neurosci. Lett. 582, 65–67. doi: 10.1016/j.neulet.2014.09.003
Wang, Y., Chen, D., Zhang, Y., Wang, P., Zheng, C., Zhang, S., et al. (2018). Novel adipokine, FAM19A5, inhibits neointima formation after injury through sphingosine-1-phosphate receptor 2. Circulation 138, 48–63. doi: 10.1161/circulationaha.117.032398
Wang, Z. V., and Scherer, P. E. (2016). Adiponectin, the past two decades. J. Mol. Cell Biol. 8, 93–100. doi: 10.1093/jmcb/mjw011
Way, J. M., Gorgun, C. Z., Tong, Q., Uysal, K. T., Brown, K. K., Harrington, W. W., et al. (2001). Adipose tissue resistin expression is severely suppressed in obesity and stimulated by peroxisome proliferator-activated receptor γ agonists. J. Biol. Chem. 276, 25651–25653. doi: 10.1074/jbc.c100189200
Wei, J., Hettinghouse, A., and Liu, C. (2016). The role of progranulin in arthritis. Ann. N. Y. Acad. Sci. 1383, 5–20. doi: 10.1111/nyas.13191
Wei, K., Serpooshan, V., Hurtado, C., Diez-Cunado, M., Zhao, M., Maruyama, S., et al. (2015). Epicardial FSTL1 reconstitution regenerates the adult mammalian heart. Nature 525, 479–485. doi: 10.1038/nature15372
Wei, Y., Xia, N., Zhang, W., Huang, J., Ren, Z., Zhu, L., et al. (2019). Serum retinol-binding protein 4 is associated with insulin resistance in patients with early and untreated rheumatoid arthritis. Joint Bone Spine 86, 335–341. doi: 10.1016/j.jbspin.2018.07.002
Wei, Z., Lei, X., Petersen, P. S., Aja, S., and Wong, G. W. (2014). Targeted deletion of C1q/TNF-related protein 9 increases food intake, decreases insulin sensitivity, and promotes hepatic steatosis in mice. Am. J. Physiol. Endocrinol. Metab. 306, E779–E790.
Wei, Z., Peterson, J. M., Lei, X., Cebotaru, L., Wolfgang, M. J., Baldeviano, G. C., et al. (2012). C1q/TNF-related protein-12 (CTRP12), a novel adipokine that improves insulin sensitivity and glycemic control in mouse models of obesity and diabetes. J. Biol. Chem. 287, 10301–10315. doi: 10.1074/jbc.m111.303651
Wei, Z., Seldin, M. M., Natarajan, N., Djemal, D. C., Peterson, J. M., and Wong, G. W. (2013). C1q/tumor necrosis factor-related protein 11 (CTRP11), a novel adipose stroma-derived regulator of adipogenesis. J. Biol. Chem. 288, 10214–10229. doi: 10.1074/jbc.m113.458711
Weigert, J., Neumeier, M., Schaffler, A., Fleck, M., Scholmerich, J., Schutz, C., et al. (2005). The adiponectin paralog CORS-26 has anti-inflammatory properties and is produced by human monocytic cells. FEBS Lett. 579, 5565–5570. doi: 10.1016/j.febslet.2005.09.022
Weschenfelder, C., Schaan de Quadros, A., Lorenzon Dos Santos, J., Bueno Garofallo, S., and Marcadenti, A. (2020). Adipokines and adipose tissue-related metabolites, nuts and cardiovascular disease. Metabolites 10:32. doi: 10.3390/metabo10010032
Weyer, C., Funahashi, T., Tanaka, S., Hotta, K., Matsuzawa, Y., Pratley, R. E., et al. (2001). Hypoadiponectinemia in obesity and type 2 diabetes: close association with insulin resistance and hyperinsulinemia. J. Clin. Endocrinol. Metab. 86, 1930–1935. doi: 10.1210/jcem.86.5.7463
Widera, C., Giannitsis, E., Kempf, T., Korf-Klingebiel, M., Fiedler, B., Sharma, S., et al. (2012). Identification of follistatin-like 1 by expression cloning as an activator of the growth differentiation factor 15 gene and a prognostic biomarker in acute coronary syndrome. Clin. Chem. 58, 1233–1241. doi: 10.1373/clinchem.2012.182816
Widera, C., Horn-Wichmann, R., Kempf, T., Bethmann, K., Fiedler, B., Sharma, S., et al. (2009). Circulating concentrations of follistatin-like 1 in healthy individuals and patients with acute coronary syndrome as assessed by an immunoluminometric sandwich assay. Clin. Chem. 55, 1794–1800. doi: 10.1373/clinchem.2009.129411
Wilson, R., Belluoccio, D., Little, C. B., Fosang, A. J., and Bateman, J. F. (2008). Proteomic characterization of mouse cartilage degradation in vitro. Arthritis Rheum. 58, 3120–3131. doi: 10.1002/art.23789
Wittamer, V., Franssen, J. D., Vulcano, M., Mirjolet, J. F., Le Poul, E., Migeotte, I., et al. (2003). Specific recruitment of antigen-presenting cells by chemerin, a novel processed ligand from human inflammatory fluids. J. Exp. Med. 198, 977–985. doi: 10.1084/jem.20030382
Wittamer, V., Gregoire, F., Robberecht, P., Vassart, G., Communi, D., and Parmentier, M. (2004). The c-terminal nonapeptide of mature chemerin activates the chemerin receptor with low nanomolar potency. J. Biol. Chem. 279, 9956–9962. doi: 10.1074/jbc.m313016200
Wong, G. W., Krawczyk, S. A., Kitidis-Mitrokostas, C., Revett, T., Gimeno, R., and Lodish, H. F. (2008). Molecular, biochemical and functional characterizations of C1q/TNF family members: adipose-tissue-selective expression patterns, regulation by PPAR-gamma agonist, cysteine-mediated oligomerizations, combinatorial associations and metabolic functions. Biochem. J. 416, 161–177. doi: 10.1042/bj20081240
Wong, G. W., Wang, J., Hug, C., Tsao, T. S., and Lodish, H. F. (2004). A family of Acrp30/adiponectin structural and functional paralogs. Proc. Natl. Acad. Sci. U.S.A. 101, 10302–10307. doi: 10.1073/pnas.0403760101
Wright, L. H., Herr, D. J., Brown, S. S., Kasiganesan, H., and Menick, D. R. (2018). Angiokine Wisp-1 is increased in myocardial infarction and regulates cardiac endothelial signaling. JCI Insight 3:e95824.
Wu, D., Lei, H., Wang, J. Y., Zhang, C. L., Feng, H., Fu, F. Y., et al. (2015). CTRP3 attenuates post-infarct cardiac fibrosis by targeting Smad3 activation and inhibiting myofibroblast differentiation. J. Mol.Med. 93, 1311–1325. doi: 10.1007/s00109-015-1309-8
Wu, Q., Chen, Y., Chen, S., Wu, X., and Nong, W. (2020). Correlation between adiponectin, chemerin, vascular endothelial growth factor and epicardial fat volume in patients with coronary artery disease. Exp. Ther. Med. 19, 1095–1102.
Wu, X., Motoshima, H., Mahadev, K., Stalker, T. J., Scalia, R., and Goldstein, B. J. (2003). Involvement of AMP-activated protein kinase in glucose uptake stimulated by the globular domain of adiponectin in primary rat adipocytes. Diabetes 52, 1355–1363. doi: 10.2337/diabetes.52.6.1355
Wurm, S., Neumeier, M., Weigert, J., Schaffler, A., and Buechler, C. (2007). Plasma levels of leptin, omentin, collagenous repeat-containing sequence of 26-kDa protein (CORS-26) and adiponectin before and after oral glucose uptake in slim adults. Cardiovasc. Diabetol. 6:7.
Xiao, J., Xiao, Z. J., Liu, Z. G., Gong, H. Y., Yuan, Q., Wang, S., et al. (2009). Involvement of dimethylarginine dimethylaminohydrolase-2 in visfatin-enhanced angiogenic function of endothelial cells. Diabetes Metab. Res. Rev. 25, 242–249. doi: 10.1002/dmrr.939
Xie, C., and Chen, Q. (2019). Adipokines: new therapeutic target for osteoarthritis? Curr. Rheumatol. Rep. 21:71.
Xu, L., Ping, F., Yin, J., Xiao, X., Xiang, H., Ballantyne, C. M., et al. (2013). Elevated plasma SPARC levels are associated with insulin resistance, dyslipidemia, and inflammation in gestational diabetes mellitus. PLoS One 8:e81615. doi: 10.1371/journal.pone.0081615
Xu, L., Zhou, B., Li, H., Liu, J., Du, J., Zang, W., et al. (2015). Serum levels of progranulin are closely associated with microvascular complication in type 2 diabetes. Dis. Markers 2015:357279.
Yamagishi, S. I., Edelstein, D., Du, X. L., Kaneda, Y., Guzmàm, M., and Brownlee, M. (2001). Leptin induces mitochondrial superoxide production and monocyte chemoattractant protein-1 expression in aortic endothelial cells by increasing fatty acid oxidation via protein kinase A. J. Biol. Chem. 276, 25096–25100. doi: 10.1074/jbc.m007383200
Yamaguchi, Y., Du, X. Y., Zhao, L., Morser, J., and Leung, L. L. K. (2011). Proteolytic cleavage of chemerin protein is necessary for activation to the active form, Chem157S, which functions as a signaling molecule in glioblastoma. J. Biol. Chem. 286, 39510–39519. doi: 10.1074/jbc.m111.258921
Yamamoto, Y., Takemura, M., Serrero, G., Hayashi, J., Yue, B., Tsuboi, A., et al. (2014). Increased serum GP88 (Progranulin) concentrations in rheumatoid arthritis. Inflammation 37, 1806–1813. doi: 10.1007/s10753-014-9911-4
Yamauchi, T., Kamon, J., Waki, H., Terauchi, Y., Kubata, N., Hara, K., et al. (2001). The fat-derived hormone adiponectin reverses insulin resistance associated with both lipoatrophy and obesity. Nat. Med. 7, 941–946. doi: 10.1038/90984
Yamauchi, T., Nio, Y., Maki, T., Kobayashi, M., Takazawa, T., Iwabu, M., et al. (2007). Targeted disruption of adipor1 and adipor2 causes abrogation of adiponectin binding and metabolic actions. Nat. Med. 13, 332–339. doi: 10.1038/nm1557
Yamawaki, H., Kuramoto, J., Kameshima, S., Usui, T., Okada, M., and Hara, Y. (2011). Omentin a novel adipocytokine inhibits TNF-induced vascular inflammation in human endothelial cells. Biochem. Biophys. Res. Commun. 408, 339–343. doi: 10.1016/j.bbrc.2011.04.039
Yamawaki, H., Tsubaki, N., Mukohda, M., Okada, M., and Hara, Y. (2010). Omentin, a novel adipokine, induces vasodilatation in rat isolated blood vessels. Biochem. Biophys. Res. Commun. 393, 668–672. doi: 10.1016/j.bbrc.2010.02.053
Yan, Q. W., Yang, Q., Mody, N., Graham, T. E., Hsu, C. H., Xu, Z., et al. (2007). The adipokine lipocalin 2 is regulated by obesity and promotes insulin resistance. Diabetes 56, 2533–2540. doi: 10.2337/db07-0007
Yanagita, T., Kubota, S., Kawaki, H., Kawata, K., Komdo, S., Takano-Yamamoto, T., et al. (2007). Expression and physiological role of CCN4/Wnt-induced secreted protein 1 mRNA splicing variants in chondrocytes. FEBS J. 274, 1655–1665. doi: 10.1111/j.1742-4658.2007.05709.x
Yang, J., Goetz, D., Li, J. Y., Wang, W., Mori, K., Setlik, D., et al. (2002). An iron delivery pathway mediated by a lipocalin. Mol. Cell. 10, 1045–1056. doi: 10.1016/s1097-2765(02)00710-4
Yang, R. Z., Lee, M. J., Hu, H., Pray, J., Wu, H. B., Hansen, B. C., et al. (2006). Identification of omentin as a novel depot-specific adipokine in human adipose tissue: possible role in modulating insulin action. Am. J. Physiol. Endocrinol. Metab. 290, 1253–1261.
Yang, W. H., Liu, S. C., Tsai, C. H., Fong, Y. C., Wang, S. J., Chang, Y. S., et al. (2013). Leptin induces IL-6 expression through OBRl receptor signaling pathway in human synovial fibroblasts. PLoS One 8:e75551. doi: 10.1371/journal.pone.0075551
Yannakoulia, M., Yiannakouris, N., Bluher, S., Matalas, A. L., Klimis-Zacas, D., and Mantzoros, C. S. (2003). Body fat mass and macronutrient intake in relation to circulating soluble leptin receptor, free leptin index, adiponectin, and resistin concentrations in healthy humans. J. Clin. Endocrinol. Metab. 88, 1730–1736. doi: 10.1210/jc.2002-021604
Yaribeygi, H., Atkin, S. L., and Sahebkar, A. (2019). Wingless-type inducible signaling pathway protein-1 (WISP1) adipokine and glucose homeostasis. J. Cell. Physiol. 234, 16966–16970. doi: 10.1002/jcp.28412
Ye, R., and Scherer, P. E. (2013). Adiponectin, driver or passenger on the road to insulin sensitivity? Mol. Metab. 2, 133–141. doi: 10.1016/j.molmet.2013.04.001
Yi, W., Sun, Y., Yuan, Y., Lau, W. B., Zheng, Q., Wang, X., et al. (2012). C1q/tumor necrosis factor-related protein-3, a newly identified adipokine, is a novel antiapoptotic, proangiogenic, and cardioprotective molecule in the ischemic mouse heart. Circulation 125, 3159–3169. doi: 10.1161/circulationaha.112.099937
Yndestad, A., Landro, L., Ueland, T., Dahl, C. P., Flo, T. H., Vinge, L. E., et al. (2009). Increased systemic and myocardial expression of neutrophil gelatinase-associated lipocalin in clinical and experimental heart failure. Eur. Heart J. 30, 1229–1236. doi: 10.1093/eurheartj/ehp088
Yoo, H. J., Hwang, S. Y., Hong, H. C., Choi, H. Y., Yang, S. J., Choi, D. S., et al. (2013). Implication of progranulin and C1q/TNF-related protein-3 (CTRP3) on inflammation and atherosclerosis in subjects with or without metabolic syndrome. PLoS One 8:e55744. doi: 10.1371/journal.pone.0055744
Yoshino, T., Kusunoki, N., Tanaka, N., Kaneko, K., Kusunoki, Y., Endo, H., et al. (2011). Elevated serum levels of resistin, leptin, and adiponectin are associated with C-reactive protein and also other clinical conditions in rheumatoid arthritis. Intern. Med. 50, 269–275. doi: 10.2169/internalmedicine.50.4306
Youn, B. S., Bang, S. I., Klöting, N., Park, J. W., Lee, N., Oh, J. E., et al. (2009). Serum progranulin concentrations may be associated with macrophage infiltration into omental adipose tissue. Diabetes 58, 627–636. doi: 10.2337/db08-1147
Youn, B. S., Klöting, N., Kratzsch, J., Lee, N., Park, J. W., Song, E. S., et al. (2008). Serum vaspin concentrations in human obesity and type 2 diabetes. Diabetes 57, 372–377. doi: 10.2337/db07-1045
Yu, X. H., Tang, Z. B., Liu, L. J., Qian, H., Tang, S. L., Zhang, D. W., et al. (2014). Apelin and its receptor APJ in cardiovascular diseases. Clin. Chim. Acta 428, 1–8. doi: 10.1016/j.cca.2013.09.001
Yuasa, D., Ohash, K., Shibata, R., Mizutani, N., Kataoka, Y., Kabara, T., et al. (2016). C1q-TNF-related protein functions to protect against acute ischemic injury in the heart. FASEB J. 30, 1065–1075. doi: 10.1096/fj.15-279885
Yura, S., Sagawa, N., Itoh, H., Kakui, K., Nuamah, M. A., Korita, D., et al. (2003). Resistin is expressed in the human placenta. J. Clin. Endocrinol. Metab. 88, 1394–1397. doi: 10.1210/jc.2002-011926
Zabel, B. A., Allen, S. J., Kulig, P., Allen, J. A., Cichy, J., Handel, T. M., et al. (2005). Chemerin activation by serine proteases of the coagulation, fibrinolytic, and inflammatory cascades. J. Biol. Chem. 280, 34661–34666. doi: 10.1074/jbc.m504868200
Zarzour, A., Kim, H. W., and Weintraub, N. L. (2018). Understanding obesity-related cardiovascular disease: it’s all about balance. Circulation 138, 64–66. doi: 10.1161/circulationaha.118.034454
Zelissen, P. M., Stenlof, K., Lean, M. E., Fogteloo, J., Keulen, E. T., Wilding, J., et al. (2005). Effect of three treatment schedules of recombinant methionyl human leptin on body weight in obese adults: a randomized, placebo-controlled trial. Diabetes Obes. Metab. 7, 755–761. doi: 10.1111/j.1463-1326.2005.00468.x
Zhang, C. L., Feng, H., Li, L., Wang, J. Y., Wu, D., Hao, Y. T., et al. (2016). Globular CTRP3 promotes mitochondrial biogenesis in cardiomyocytes through AMPK/PGC-1alpha pathway. Biochim. Biophys Acta 1861, 3085–3094. doi: 10.1016/j.bbagen.2016.10.022
Zhang, J., Qin, Y., Zheng, X., Qiu, J., Gong, L., Mao, H., et al. (2002). The relationship between human serum resistin level and body fat content, plasma glucose as well as blood pressure. Zhonghua Yi Xue Za Zhi 82, 1609–1612.
Zhang, L., Curhan, G. C., and Forman, J. P. (2010). Plasma resistin levels associate with risk for hypertension among nondiabetic women. J. Am. Soc. Nephrol. 21, 1185–1191. doi: 10.1681/asn.2009101053
Zhang, Y., Li, Y., Yu, L., and Zhou, L. (2017). Association between serum resistin concentration and hypertension: a systematic review and meta-analysis. Oncotarget 8, 41529–41537. doi: 10.18632/oncotarget.17561
Zhang, Y., Shui, X., Lian, X., and Wang, G. (2015). Serum and synovial fluid nesfatin-1 concentration is associated with radiographic severity of knee osteoarthritis. Med. Sci. Monit. 21, 1078–1082. doi: 10.12659/msm.892875
Zhang, Z., Xing, X., Hensley, G., Chang, L. W., Liao, W., Abu-Amer, Y., et al. (2010). Resistin induces expression of proinflammatory cytokines and chemokines in human articular chondrocytes via transcription and messenger RNA stabilization. Arthritis Rheum. 62, 1993–2003.
Zhao, L., Yamaguchi, Y., Ge, X., Robinson, W. H., Morser, J., and Leung, L. L. K. (2018). Chemerin 156F, generated by chymase cleavage of prochemerin, is elevated in joint fluids of arthritis patients. Arthritis Res. Ther. 20:132.
Zheng, Q., Yuan, Y., Yi, W., Lau, W. B., Wang, Y., Wang, X., et al. (2011). C1q/TNF-related proteins, a family of novel adipokines, induce vascular relaxation through the adiponectin receptor-1/AMPK/eNOS/nitric oxide signaling pathway. Arterioscler. Thromb. Vasc. Biol. 31, 2616–2623. doi: 10.1161/atvbaha.111.231050
Zieger, K., Weiner, J., Krause, K., Schwarz, M., Kohn, M., Stumvoll, M., et al. (2018). Vaspin suppresses cytokine-induced inflammation in 3T3-L1 adipocytes via inhibition of NFκB pathway. Mol. Cell. Endocrinol. 460, 181–188. doi: 10.1016/j.mce.2017.07.022
Zografos, T., Haliassos, A., Korovesis, S., Giazitzoglou, E., Voridis, E., and Katritsis, D. (2009). Association of neutrophil gelatinase-associated lipocalin with the severity of coronary artery disease. Am. J. Cardiol. 104, 917–920. doi: 10.1016/j.amjcard.2009.05.023
Keywords: adipokines, obesity, type 2 diabetes mellitus, cardiovascular disorders, therapeutic targets, rheumatoid arthritis
Citation: Recinella L, Orlando G, Ferrante C, Chiavaroli A, Brunetti L and Leone S (2020) Adipokines: New Potential Therapeutic Target for Obesity and Metabolic, Rheumatic, and Cardiovascular Diseases. Front. Physiol. 11:578966. doi: 10.3389/fphys.2020.578966
Received: 01 July 2020; Accepted: 14 October 2020;
Published: 30 October 2020.
Edited by:
Osama Mohamed Ahmed, Beni-Suef University, EgyptReviewed by:
Rubén Cereijo, University of Barcelona, SpainCopyright © 2020 Recinella, Orlando, Ferrante, Chiavaroli, Brunetti and Leone. This is an open-access article distributed under the terms of the Creative Commons Attribution License (CC BY). The use, distribution or reproduction in other forums is permitted, provided the original author(s) and the copyright owner(s) are credited and that the original publication in this journal is cited, in accordance with accepted academic practice. No use, distribution or reproduction is permitted which does not comply with these terms.
*Correspondence: Luigi Brunetti, bHVpZ2kuYnJ1bmV0dGlAdW5pY2guaXQ=
Disclaimer: All claims expressed in this article are solely those of the authors and do not necessarily represent those of their affiliated organizations, or those of the publisher, the editors and the reviewers. Any product that may be evaluated in this article or claim that may be made by its manufacturer is not guaranteed or endorsed by the publisher.
Research integrity at Frontiers
Learn more about the work of our research integrity team to safeguard the quality of each article we publish.