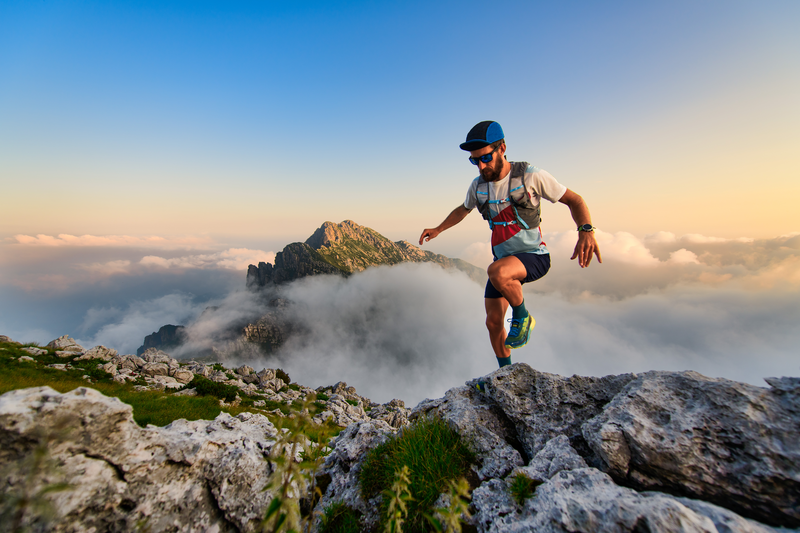
95% of researchers rate our articles as excellent or good
Learn more about the work of our research integrity team to safeguard the quality of each article we publish.
Find out more
ORIGINAL RESEARCH article
Front. Physiol. , 23 September 2020
Sec. Invertebrate Physiology
Volume 11 - 2020 | https://doi.org/10.3389/fphys.2020.572758
This article is part of the Research Topic No Frontiers for Tick-Borne Diseases View all 4 articles
Understanding the origin of ticks is essential for evaluating the risk of tick-borne disease introduction into new territories. However, when collecting engorged ticks from a host, it is virtually impossible to identify the geographical location where this tick was acquired. Recently, the elementome of tick exoskeleton was characterized by using scanning electron microscopy (SEM) and energy dispersive spectroscopy analysis (EDS). The objective of our preliminary proof-of-concept study was to evaluate the use of SEM-EDS for the analysis of tick exoskeleton elementome to gain insight into the tick geographic and host origin. For this preliminary analysis we used 10 samples of engorged ticks (larvae and nymphs of six species from three genera) collected from various resident hosts and locations. The elementome of the tick exoskeleton was characterized in dorsal and ventral parts with three scans on each part using an EDS 80 mm2 detector at 15 kV in a field emission scanning electron microscope. We used principal component analysis (PCA) (varimax rotation) to reduce the redundancy of data under the premise of losing information as little as possible. The PCA was used to test whether the different variables (tick species, stages, hosts, or geographic locations) differ in the composition of exoskeleton elementome (C, O, P, Cl, and Na). Analyses were carried out using SPSS. The PCA analysis explained a high percentage of variance using the first two factors, C and O (86.13%). The first PC (PC-1; 63.12%) was positively related to P, Cl, and Na, and negatively related to C. The second principal component (23.01%) was mainly positively related to C. In the space defined by the two extracted PC (PC-1 and PC-2), the elementome of tick samples was clearly associated with tick species, but not with developmental stages, hosts or geographic locations. A differentiated elementome pattern was observed within Romanian regions (CJ and TL) for the same tick species. The use of the SEM-EDS methodological approach provided additional information about the tick exoskeleton elementome with possible applications to the identification of tick origin host and location.
Understanding the geographical origin of ticks is essential for evaluating the risk of tick-bornedisease introduction to new territories. In general, the non-parasitic tick stages do not move too much, and the main territorial spread of ticks is by hosts (Ogden et al., 2013). Hence, the distance to which a tick is transported from its environmental origin where it has naturally detached (and eventually collected), highly depends on the movement and migration pattern of the hosts (de la Fuente et al., 2015; Abid et al., 2019). For long distance migrators, such as birds, dispersal can be significant, and the origin of ticks can be very distant. It has been hypothesized for instance that migratory birds, among others, could play a role in the dispersal and range extension of Ixodes scapularis in Canada (Ogden et al., 2008) and United States (Tonelli and Dearborn, 2019), of Ixodes ricinus in Europe (Sándor et al., 2014; Ciebiera et al., 2019), or Hyalomma spp. from northern Africa to Southern and Northern Europe (England et al., 2016), to name only a few. However, when collecting engorged ticks from a host, it is virtually impossible to identify the geographical location where this tick was acquired by the host and suppositions were made based on expected migration routes of birds or by modeling. Although birds carry mainly the immature stages of such ticks, some of them are spending a significant time feeding, which in case of two hosts ticks such as Hy. marginatum and Hy. rufipes can reach up to 26 days (Hoogstraal, 1979) and allow theoretically even long distance spreading.
A promising tool to track the origin and route of migration in birds, mammals, and insects is the measurement of stable isotopes in the tissues such as feathers, hair, or the chitinous integument, respectively (Hobson and Wassenaar, 2018). However, such methods are difficult to apply due to technological limitations in small organisms such as a larval tick. To overcome these limitations, the characterization of the chemical composition (or elementome) could be an alternative option.
In ticks, as in other terrestrial arthropods, the relative amount of chitin fibrils and protein matrix, protein composition, pH/water content of the matrix, composition of chemical elements, and cross-linking of the matrix protein affect the properties of the exoskeleton (Cribb et al., 2010; Flynn and Kaufman, 2015; Gallant et al., 2016; Gallant and Hochberg, 2017; Suppan et al., 2018). It has been shown that mechanical properties of tick cuticle change during tick feeding to support the increase in body size and mass during this process (Flynn and Kaufman, 2015).
Differences between tick species in the mechanical properties of tick cuticle have been reported (Kaufman and Flynn, 2018). Recently, the elementome of tick exoskeleton, cement and salivary glands were characterized using scanning electron microscopy (SEM) and energy dispersive spectroscopy analysis (EDS; de la Fuente et al., 2020; Villar et al., 2020). Additionally, elemental X-ray microanalysis was performed on ticks to show that hematin granules were derived from the blood meal (Agbede, 1986) and to show that salivary gland secretory granules have high calcium content commensurate with a role in secretory protein packaging (Mans et al., 2001).
The objective of our proof-of-concept study was to evaluate the use of SEM-EDS for the analysis of tick exoskeleton elementome to gain insight into the tick geographic location origin and relation to the host. Herein, the combined SEM-EDS approach was used for the analysis of the exoskeleton elementome in samples collected from different tick species, developmental stages, hosts and geographic locations (Supplementary Data S1, S2). The elementome was characterized in tick exoskeleton dorsal, ventral and fragment parts (Figure 1A). Finally, the PCA was used to test whether the different variables (tick species, stages, hosts, or geographic locations) differ in the composition of exoskeleton elementome. The preliminary results showed that the SEM-EDS methodological approach provides information about the tick exoskeleton elementome with putative implications in the tracking of tick origin.
Figure 1. SEM-EDS analysis of tick exoskeleton elementome. (A) Representative electron microscopy images used for SEM-EDS analysis of the elementome in tick exoskeleton dorsal, ventral and fragment parts. (B) Summary of the results obtained by SEM-EDS analysis of tick exoskeleton elementome. Chemical elements identified with higher levels in the different comparisons and analyses are shown close to each of the geographic locations, tick developmental stages and hosts. Only statistically significant differences are shown (Supplementary Data S1, S3).
For this study, 10 randomly selected samples of engorged ticks (larvae and nymphs of six species from three genera) collected from various resident hosts and locations were used (Table 1 and Supplementary Data S1). Ticks were identified based on morphological keys by Estrada-Peña et al. (2018). Ticks were preserved in absolute ethanol before individual examination.
Whole ticks were dehydrated in an incubator at 37°C for 24 h as a preparation for SEM photography. Specimens were mounted onto standard aluminum SEM stubs using conductive carbon adhesive tabs. Ticks were observed and photographed with a field emission scanning electron microscope (Zeiss GeminiSEM 500, Oberkochen, Germany) operating in high vacuum mode at an accelerating voltage of 2 kV and with no metallic coating. The elementome of the tick exoskeleton was characterized in dorsal and ventral parts (Figure 1A). Some tick specimens fractionated during preparation for analysis and were included as tick fragments (Figure 1A). The elementome was determined with three scans on each part using an EDS 80 mm2 detector at 15 kV (Oxford Instruments, Abingdon, United Kingdom). The relative abundance of chemical elements (percent of total atoms in the sample) were compared between groups by Student’s t-test with unequal variance and one-way ANOVA test1 when the number of spectra was higher than 5 (p < 0.05, n = 6–10 biological replicates) (Supplementary Data S1, S2).
The principal component analysis (PCA) is a dimension reduction technique for data analysis (Jolliffe and Cadima, 2016). Principal component analysis maps n-dimensional features to k-dimensional features (k ≤ n). The k-dimensional features are new orthogonal factors, called principal components (PC), which are reconstructed from the original n-dimensional features. The essence of the PCA is to reduce the redundancy of data under the premise of losing information as little as possible. In this study, we used PCA (varimax rotation) to analyze the chemical composition of the tick exoskeleton using chemical elements identified in all samples (C, O, P, Cl, and Na). The objective was to test whether the different tick species, stages, hosts or origin geographic locations differ in the exoskeleton elementome characterized with the PC. Analyses were carried out using SPSS (IBM SPSS Statistics for Windows, Version 25.0. IBM Corp. Armonk, NY; released 2017).
As in previous studies (Kaufman and Flynn, 2018; de la Fuente et al., 2020), the results of the analysis of tick exoskeleton elementome showed the presence with high relative abundance (>10 atomic %) of C, O, and N in tick samples (Supplementary Data S1, S2). Other chemical elements with low relative abundance (<10 atomic %) in tick samples included S, P, Cl, Na, K, Ca, Br, Si, Mo, and Mg (Supplementary Data S1, S2).
Despite the limited number of samples included in the analysis, matched-pair analyses showed some differences in the exoskeleton composition of some chemical elements between tick developmental stages (C, Cl, and K in I. ricinus larvae and nymphs; p < 0.05, Supplementary Data S1 reference 1 vs. 2) and hosts (O, Cl, Na in I. ricinus from Erinaceus roumanicus and Passer montanus; p < 0.05, Supplementary Data S1 reference 2 vs. 3) (Table 1 and Figure 1B). Other analyses by grouping all tick samples for comparison between different geographic locations and hosts showed differences between Romanian CJ and TL counties (O, P, Cl, Na, K; p < 0.005, Supplementary Data S1 reference 1,2 vs. 3,4,6) and between E. roumanicus and P. montanus hosts (O; p = 0.01, Supplementary Data S1 reference 1,2,4 vs. 3,5) (Table 1 and Figure 1B).
Some of these results may have functional implications. Our results for I. ricinus showed that Cl increased and C decreased in relative abundance in nymphs when compared to larvae (Figure 1B). Nymphs have a larger blood meal than larvae, which correlates with previous findings that Cl relative abundance increases with feeding in both salivary glands and cement while the main source for some of the elementome chemical elements such as C are tick and host derived proteins (de la Fuente et al., 2020). It has been demonstrated that the blood of mammals and birds living at higher altitudes show an increased O affinity which improves the O transport when compared between individuals of the same species but living at different elevations (Snyder, 1992). This could explain the difference between Cluj (484 m) and Tulcea (57 m). However, this variability can also be due to differences in metabolic activity or cross-linking mediated by O.
These preliminary analyses showed significant differences in the abundance of some exoskeleton chemical elements between tick developmental stages, hosts and geographic locations (Figure 1B). However, in most cases the matched-pair analyses were not conclusive due to multiple and/or unknown variables. Therefore, the only chemical elements present in samples from all geographic locations (C, O, P, Cl, and Na) were then used for PCA.
The PCA analysis explained a high percentage of variance using the first two factors, C and O (86.13%; Supplementary Data S3). The first PC (PC-1; 63.12%) was positively related to P, Cl, and Na, and negatively related to C (Table 2). The second principal component (23.01%; Supplementary Data S3) was mainly positively related to C (Table 2).
In the space defined by the two extracted PC (PC-1 and PC-2), the elementome of tick samples was clearly associated with tick species, but not with developmental stages, hosts or geographic locations (Figure 2). Nevertheless, as shown with matched-pair analyses (Figure 1B), within Romanian regions (CJ and TL), a clearly differentiated elementome pattern was observed (Figure 2).
Figure 2. Principal component analyses of tick exoskeleton elementome. Representation of the samples by tick species, developmental stage (L and N), host and geographic location in relation to the two axes extracted from the principal component analyses (PC-1 and PC-2) that are related with the chemical composition of the tick exoskeleton elementome. This plot allows to visually cluster the samples in relation to the chemical space defined by PC-1 and PC-2. Higher clustering along PC-1 suggests that samples are grouped by differences in P, Cl, Na, and O, and along PC-2 that are mainly grouped by C (see Table 2). The category “Undetermined” in Host analysis refers to ticks with unknown host origin (Data S1 and S3). L, larvae; N, nymphs.
The use of the SEM-EDS methodological approach provided additional information about the tick exoskeleton elementome and showed variations in the abundance of chemical elements. These variations were associated with tick developmental stages, hosts and geographic locations (Figure 1B). However, the PCA analysis showed that the exoskeleton elementome was associated with tick species but not with developmental stages, hosts or geographic locations. Although preliminary, these results together with previous reports (de la Fuente et al., 2020; Villar et al., 2020) support the use of SEM-EDS for the characterization of tick elementome in different organs and biomolecular complex structures such as cement and exoskeleton with possible applications to the identification of tick origin host and location.
Supported by this proof-of-concept study, future experiments should characterize tick exoskeleton elementome with a higher number of samples including laboratory-rated controls and combining both matched-pair and PCA analyses to get insight into tick origins.
All datasets presented in this study are included in the article/Supplementary Material.
Ethical review and approval was not required for the animal study because collection of ticks from vertebrates does not fall under the regulations of ethical permission, as all hosts were released and remained unharmed after the ticks were collected. The tick removal is not a painful procedure.
IP, PA, and EP performed the analysis and wrote the draft of the manuscript. AM collected the tick and developed the ideas of the work. JF coordinated the lab work. All authors contributed to the writing of the manuscript.
This research was partially supported by the Consejería de Educación, Cultura y Deportes, JCCM, Spain, project CCM17-PIC-036 (SBPLY/17/180501/000185). For AM, the samples were collected in the frame of the UEFISCDI project PCCDI 57/2018.
The authors declare that the research was conducted in the absence of any commercial or financial relationships that could be construed as a potential conflict of interest.
The reviewer BM declared a past co-authorship with one of the authors JF to the handling editor.
We thank all the collaborators who contributed to the collection of tick samples and provided the host photos used in Figure 1.
The Supplementary Material for this article can be found online at: https://www.frontiersin.org/articles/10.3389/fphys.2020.572758/full#supplementary-material
DATA S1 | Tick exoskeleton elementome analysis by SEM-EDS.
DATA S2 | Quantitative spectra of chemical elements of tick exoskeleton.
DATA S3 | Results of the Principal Component Analysis (PCA).
Abid, A., Khan, M. A., Zahid, H., Yaseen, P. M., Khan, M. Q., Nawab, J., et al. (2019). Seasonal dynamics, record of ticks infesting humans, wild and domestic animals and molecular phylogeny of Rhipicephalus microplus in Khyber Pakhtunkhwa Pakistan. Front. Physiol. 10:793. doi: 10.3389/fphys.2019.00793
Agbede, R. I. S. (1986). Scanning electron microscopy of digest cells in the midgut epithelium of Boophilus microplus. Exp. Appl. Acarol. 2, 329–335. doi: 10.1007/BF01193899
Ciebiera, O., Jerzak, L., Nowak-Chmura, M., and Bocheñski, M. (2019). Ticks (Acari: Ixodida) on birds (Aves) migrating through the Polish Baltic coast. Exp. Appl. Acarol. 77, 241–251. doi: 10.1007/s10493-019-00341-z
Cribb, B. W., Lin, C. L., Rintoul, L., Rasch, R., Hasenpusch, J., and Huang, H. (2010). Hardness in arthropod exoskeletons in the absence of transition metals. Acta Biomater. 6, 3152–3156. doi: 10.1016/j.actbio.2010.02.009
de la Fuente, J., Estrada-Peña, A., Cabezas-Cruz, A., and Brey, R. (2015). Flying ticks: anciently evolved associations that constitute a risk of infectious disease spread, Parasit. Vectors 8:538. doi: 10.1186/s13071-015-1154-1
de la Fuente, J., Lima-Barbero, J. F., Prado, E., Pacheco, I., Alberdi, P., and Villar, M. (2020). Anaplasma pathogen infection alters chemical composition of the exoskeleton of hard ticks (Acari:Ixodidae). Comput. Struct. Biotecnol. J. 18, 253–257. doi: 10.1016/j.csbj.2020.01.003
England, M. E., Phipps, P., Medlock, J. M., Atkinson, P. M., Atkinson, B., Hewson, R., et al. (2016). Hyalomma ticks on northward migrating birds in southern Spain: implications for the risk of entry of Crimean-Congo haemorrhagic fever virus to great britain. J. Vector Ecol. 41, 128–134. doi: 10.1111/jvec.12204
Estrada-Peña, A., Mihalca, A. D., and Petney, T. N. (2018). Ticks of Europe and North Africa: a guide to Species Identification, First ed. Berlin: Springer.
Flynn, P. C., and Kaufman, W. R. (2015). Mechanical properties of the cuticle of the tick Amblyomma hebraeum (Acari: Ixodidae). J. Exp. Biol. 218, 2806–2814. doi: 10.1242/jeb.123919
Gallant, J., and Hochberg, R. (2017). Elemental characterization of the exoskeleton in the whipscorpions Mastigoproctus giganteus and Typopeltis dalyi (Arachnida: Thelyphonida). Invertebr. Biol. 136, 345–359. doi: 10.1111/ivb.12187
Gallant, J. G., Hochberg, R., and Ada, E. (2016). Elemental characterization of the cuticle in the pseudoscorpion Halobisium occidentale. Invert. Biol. 135, 127–137. doi: 10.1111/ivb.12123
Hobson, K. A., and Wassenaar, L. I. (2018). Tracking Animal Migration with Stable Isotopes, Second ed. Cambridge, MA: Academic Press.
Hoogstraal, H. (1979). The epidemiology of tick- borne Crimean- Congo hemorrhagic fever in Asia. Europe and Africa. J. Med. Entomol. 15, 307–417. doi: 10.1093/jmedent/15.4.307
Jolliffe, I. T., and Cadima, J. (2016). Principal component analysis: a review and recent developments. Philos. Trans. A Math Phys. Eng. Sci. 374:20150202. doi: 10.1098/rsta.2015.0202
Kaufman, W. R., and Flynn, P. C. (2018). A comparison of the cuticular properties of the female ticks Ixodes pacificus and Amblyomma hebraeum (Acari: Ixodidae) throughout the feeding period. Exp. Appl. Acarol. 76, 365–380. doi: 10.1007/s10493-018-0306-3
Mans, B. J., Venter, J. D., Vrey, P. J., Louw, A. I., and Neitz, A. W. H. (2001). Identification of putative proteins involved in granule biogenesis of tick salivary glands. Electrophoresis 22, 1739–1746.
Ogden, N. H., Lindsay, L. R., Hanincová, K., Baker, I. K., Bigras-Poulin, M., Charron, D. F., et al. (2008). Role of migratory birds in introduction and range expansion of Ixodes scapularis ticks and of Borrelia burgdorferi and Anaplasma phagocytophilum in Canada. Appl. Environ. Microbiol. 74, 1780–1790. doi: 10.1128/AEM.01982-07
Ogden, N. H., Mechai, S., and Margos, G. (2013). Changing geographic ranges of ticks and tick-borne pathogens: drivers, mechanisms and consequences for pathogen diversity. Front. Cell. Infect. Microbiol. 3:46. doi: 10.3389/fcimb.2013.00046
Sándor, A. D., Mãrcuţan, D. I., D’Amico, G., Gherman, C. M., Dumitrache, M. O., and Mihalca, A. D. (2014). Do the ticks of birds at an important migratory hotspot reflect the seasonal dynamics of Ixodes ricinus at the migration initiation site? a case study in the danube delta. PLoS One. 9:e89378. doi: 10.1371/journal.pone.0089378
Snyder, G. K. (1992). Respiratory functions of avian and mammalian hemoglobins. Springer 13, 433–453. doi: 10.1007/978-3-642-76418-9_15
Suppan, J., Engel, B., Marchetti-Deschmann, M., and Nürnberger, S. (2018). Tick attachment cement - reviewing the mysteries of a biological skin plug system. Biol. Rev. Camb. Philos. Soc. 93, 1056–1076. doi: 10.1111/brv.12384
Tonelli, B. A., and Dearborn, D. C. (2019). An individual-based model for the dispersal of Ixodes scapularis by ovenbirds and wood thrushes during fall migration. Ticks Tick Borne Dis. 10, 1096–1104. doi: 10.1016/j.ttbdis.2019.05.012
Keywords: tick, exoskeleton, energy dispersive spectroscopy, elementomics, SEM
Citation: Pacheco I, Acevedo P, Prado E, Mihalca AD and de la Fuente J (2020) Targeting the Exoskeleton Elementome to Track Tick Geographic Origins. Front. Physiol. 11:572758. doi: 10.3389/fphys.2020.572758
Received: 22 June 2020; Accepted: 28 August 2020;
Published: 23 September 2020.
Edited by:
Zhijun Yu, Hebei Normal University, ChinaReviewed by:
Abid Ali, Abdul Wali Khan University Mardan, PakistanCopyright © 2020 Pacheco, Acevedo, Prado, Mihalca and de la Fuente. This is an open-access article distributed under the terms of the Creative Commons Attribution License (CC BY). The use, distribution or reproduction in other forums is permitted, provided the original author(s) and the copyright owner(s) are credited and that the original publication in this journal is cited, in accordance with accepted academic practice. No use, distribution or reproduction is permitted which does not comply with these terms.
*Correspondence: Andrei Daniel Mihalca, YW1paGFsY2FAdXNhbXZjbHVqLnJv; José de la Fuente, am9zZV9kZWxhZnVlbnRlQHlhaG9vLmNvbQ==
Disclaimer: All claims expressed in this article are solely those of the authors and do not necessarily represent those of their affiliated organizations, or those of the publisher, the editors and the reviewers. Any product that may be evaluated in this article or claim that may be made by its manufacturer is not guaranteed or endorsed by the publisher.
Research integrity at Frontiers
Learn more about the work of our research integrity team to safeguard the quality of each article we publish.