- 1State Key Laboratory for Biology of Plant Diseases and Insect Pests, Institute of Plant Protection, Chinese Academy of Agricultural Sciences, Beijing, China
- 2College of Plant Protection, Hunan Agricultural University, Changsha, China
- 3College of Agriculture, Ludong University, Yantai, China
Thermal adaptation plays a fundamental role in the expansion and distribution of insects, and heat shock proteins (Hsps) play important roles in the temperature adaptation of various organisms. To determine the roles of Hsp genes (Hsp70, Hsp21, and sHsp21) on the high temperature tolerance of Agasicles hygrophila, we obtained complete cDNA (complementary DNA) sequences for Hsp70, Hsp21, and sHsp21 by rapid amplification of cDNA ends (RACE), analyzed their expression profiles under different high temperature treatments by quantitative reverse transcription polymerase chain reaction (RT-qPCR), and performed functional verification by RNA interference (RNAi). The open reading frames of Hsp70, Hsp21, and sHsp21 were 1940, 543, and 567 bp, encoding 650, 180, and 188 amino acids, respectively. Their molecular weights (MWs) were 71.757, 20.879, and 21.510 kDa, and the isoelectric points were 5.63, 6.45, and 6.24, respectively. Phylogenetic tree analysis showed that the Hsp70, Hsp21, and sHsp21 genes of A. hygrophila were relatively conserved in evolution. The Hsp70 and Hsp21 genes in A. hygrophila were homologous to those in Leptinotarsa decemlineata (87 and 79% similarity, respectively), and the sHsp21 gene in A. hygrophila was homologous to that in Lissorhoptrus oryzophilus (74% similarity). The amino acid polypeptide chain had highly conserved sequences of DLGGGTFD, VLVGGSTR, and GPTIEEVD. The sequence of EEVD was the characteristic motif of cytoplasmic Hsp70, and the highly conserved sequences of MALFR and MSLLP were characteristic sequences of Hsp2 and sHsp21, respectively. Relative quantitative real time PCR showed that the three Hsps could be induced by 4-h treatment at high temperatures. Significant upregulation of these Hsps was observed when the temperature was further increased. The RNAi results showed that the injection of the three Hsps’ dsRNA could suppress the expression at the gene level significantly. Compared with the control group, high temperature heat shock reduced the fecundity of A. hygrophila significantly, and the fecundity decreased with the increase in temperature. Our results suggest that Hsp70, Hsp21, and sHsp21 might play key roles in high temperature adaptation of A. hygrophila and help improve our understanding of their mechanism of thermotolerance.
Introduction
Alligator weed Alternanthera philoxeroides (Mart) Griesb (Amaranthaceae) is an aquatic and clonal weedy species native to South America (Buckingham, 1996). It has been recognized as a prominent problematic weed worldwide and is listed as one of the 16 most serious invasive species in China (State Environmental Protection Administration of China, and Chinese Academy of Sciences, 2003). Alternanthera philoxeroides was firstly introduced into China in the 1930s as a forage crop (Wang et al., 2005), and presently, it occurs in most regions of southern China and has become one of the most noxious weeds in China. Some invasive plants reach high densities and cause economic or environmental harm or threaten human health (National Invasive Species Council, 2001). The infestation of A. philoxeroides creates mats that restrict traffic in navigable waterways and limit fishing, swimming, and other recreational uses of lakes, rivers, and streams (Spencer and Coulson, 1976). The infestation also destroys landscape and biological diversity and threatens ecological integrity of the environment (Vogt et al., 1992; Ma and Wang, 2005). The alligator weed is a well-adapted species and it can reproduce both sexually and asexually, although the seeds are often not viable (Julien et al., 1995).
Biological control is a powerful management method with the potential to control invasive weeds without having to resort to expensive and temporary mechanical or chemical methods (Ma et al., 2010). Insects have been used successfully as biological control agents to suppress alligator weed in Florida and other states of the southeastern United States (Gangstad, 1976). The alligator weed flea beetle Agasicles hygrophila Selman & Vogt was the first host-specific insect introduced into the United States to control alligator weed in 1964 (Zeiger, 1967; Spencer and Coulson, 1976). It was first introduced into China from Florida in the United States to control alligator weed in 1986, and has been successfully used in southern China (Wang, 1989; Ma et al., 2003).
Temperature affects the distribution and abundance of living organisms, and is particularly important for ectotherms such as insects (Yu et al., 2012). According to our field survey in Hunan province, the population of A. hygrophila has two obvious peaks from June to July and October to November, but it drops sharply from August to September, leading to inefficient control of alligator weed (Li et al., 2011). Studies have shown that heat shock proteins (Hsps) exist widely in animals and likely play important roles in their thermal adaptation and thermo-tolerance or thermo-protection (Feder and Hofmann, 1999; Hoffmann et al., 2003; Fangue et al., 2006; MacRae, 2010; Lü et al., 2014). Under heat shock stress, the heat shock transcription factor (HSF) drives the expression of a broad range of heat-responsive genes, including Hsp70 and Hsp90, which are also expressed during the development of insects under normal conditions (Westwood et al., 1991). Different classes of Hsps can play different roles in the thermotolerance of certain species (Goto et al., 1998; Rinehart et al., 2000, 2007; Goto and Kimura, 2004; Aruda et al., 2011). To date, the characteristics of Hsp genes and their mRNA expression profiles in A. hygrophila, as well as the relationship between Hsp gene expression and thermotolerance, remain unknown.
To reveal the potential function of different Hsp genes in the thermotolerance of A. hygrophila, we isolated complete cDNA (complementary DNA) sequences for Hsp70, Hsp21, and sHsp21 by rapid amplification of cDNA ends polymerase chain reaction (RACE-PCR), and examined the expression patterns of these Hsp genes under different high temperature treatments. We also analyzed the phylogenetic relationships of these three Hsps by comparing them with Hsps from other insects. In addition, we used an RNA interference technique to examine their roles in heat tolerance. The present results could improve our understanding of the mechanisms of thermotolerance in A. hygrophila at the molecular level.
Materials and Methods
Experimental Insects and Plants
Agasicles hygrophila adults were collected from a pond field covered with alligator weed in Changsha, Hunan province, China (28°11′49′′ N, 112°58′42′′ E) in June 2016 by the sweeping method and were maintained on alligator weed plants in the laboratory at the Chinese Academy of Agricultural Sciences, Beijing, China (BJ, CAAS) under controlled conditions, which were 26 ± 2°C, 75 ± 5% relative humidity (RH), and a 12:12 h light–dark regime. The flea beetles were cultivated for three generations to eliminate maternal effects before the experiments.
Alligator weeds were collected from a pond at the Institute of Plant Protection, Hunan Academy of Agricultural Sciences and planted in sterilized soil in plastic boxes (30 cm × 30 cm × 15 cm) with sterilized soil. Alligator weeds were planted in the soil in a greenhouse at Langfang Experimental Station, Chinese Academy of Agricultural Sciences (LF, CAAS), Hebei province and watered daily.
Temperature Processing and Sample Collection
Newly emerged A. hygrophila adult beetles, which were less than 12 h old after eclosing were used for the experiments. Ten females and 10 males were put together in cylindrical boxes of 8 cm in diameter and 12 cm in height. Each temperature was studied using 10 boxes of A. hygrophila adult beetles. These beetles were exposed to heat shock at 30, 36, or 39°C for 4 h from 10:00 to 14:00 in a constant temperature incubator (RPX-450, Colin, Beijing) daily. The temperatures used were based on the temperature ranges in summer in Changsha, Hunan province of China (Zhao, 2017). Five females and 5 males were collected from each temperature group every day for a week. Each sample with 10 beetles was put into a 1.5 ml microtube, frozen in liquid nitrogen immediately, and subsequently kept in the refrigerator (DW-86L628, Haier Special Electric Appliance, Co., Ltd., Tsingtao) at −80 ± 1°C. The beetles at 30°C were used as the control group, and the heat-shock test and sample collection were replicated three times for each temperature treatment.
RNA Extraction and cDNA Synthesis
Total RNA from the samples was extracted using the TRIzol (Trizol reagent 10296028, United States) method and the reverse transcription kit (TransScript One-Step gDNA Removal and cDNA Synthesis SuperMix, AT311-03, TransGen Biotech, China) was used to synthesize first-strand cDNA and remove genomic DNA. Total RNA was stored in a −80 ± 1°C refrigerator prior to subsequent experimentation.
Cloning the Full-Length cDNA of Hsps
Specific primers were designed according to the cDNA obtained in step 2.3; then, 5′/3′ rapid amplification of cDNA ends (RACE) was performed to obtain full-length cDNAs according to the manufacturer’s user manual (SMARTer® RACE 5′/3′, Cat. No(s). 634858, Clontech Laboratories, Inc., Takara, CA, United States) using gene-specific primers corresponding to GSP1 and GSP2 (Supplementary Table S1). To ensure that the 5′ and 3′ fragments were derived from the same gene, specific primer sets flanking the open reading frames (ORFs) were designed and used to amplify the full-length cDNAs.
Sequence Analysis of Hsp cDNA
The full-length cDNAs of Hsps were used as query sequences to search for other insect Hsps in GenBank by BLAST software available at the NCBI website1. Sequence alignment and identity analyses were carried out with DNAMAN and MEGA6 or vector NTI. ORF Finder2 was used to find the ORF of cDNA. The amino acid sequences and the MW of the proteins were calculated using DNASTAR.
Homologous Alignment and Phylogenetic Analysis
To evaluate the molecular evolutionary relationship of Hsps in A. hygrophila with those in other insects, phylogenetic trees were constructed based on their amino acids sequences by MEGA 6 version 5 using the Neighbor-Joining (NJ) method (Tamura et al., 2011).
Relative Quantitative Real Time PCR
The PCR reactions were performed in a 20 μl total reaction volume including 10 μl of 2 × TransStart® Tip Green qPCR SuperMix (TransGen Biotech, China), 0.4 μl of Passive Reference Dye II, 0.4 μl of each of the gene-specific primers, 1 μl of cDNA templates, and 7.8 μl of ddH2O. Real-time PCR was carried out with specific primers to determine the expression level of Hsp70, Hsp21, and sHsp21. β-actin was used as an internal reference gene. Reactions were carried out on the 7500 Real-Time PCR System (Applied Biosystems, Grand Island, NY, United States). The thermal cycler parameters were as follows: 94°C for 30 s followed by 40 cycles each at 94°C for 5 s, and 60°C for 34 s. At the end was the melting curve stage following the default parameters. A standard curve was derived from the serial dilutions to quantify the copy numbers of target mRNAs. The standard curves for Hsp70, Hsp21, sHsp21, and the β-actin had slopes of −3.214, −3.313, −3.325, and −3.415; correlation coefficients (R2) of 0.998, 0.994, 0.991, and 1.000; amplification efficiencies of 104.714, 100.361, 99.884, and 96.265; and Y-intercepts of 44.530, 44.201, 47.418, and 49.371, respectively. Each cDNA sample was assayed in triplicate. The data were analyzed based on the Cp method according to the mathematical model of Pfaffl (Fleige et al., 2006), simplified to 2–△△Ct as follows:
Synthesis of Double-Stranded RNA
Three Hsp transcription templates were produced from the total cDNA of A. hygrophila using gene-specific primers containing a T7 promoter sequence; the T7 promoter was as described by Ghanim et al. (2007). The promoter sequences were as follows: TAATACGACTCACTATAGGG. Amplification reactions were conducted in 36.5 μl ddH2O, 5 μl 10 × PCR buffer (10 μM), 1 μl dNTPs (2.5 mM), 1 μl Taq DNA polymerase (TransGen Biotech, China), 2 μl T7 reverse primer, 2 μl T7 forward primer, and 2.5 μl cDNA template in a final volume of 50 μl. The PCR reaction procedure was 94°C for 5 min, 35 cycles of 94°C for 30 s, 55°C for 30 s, and 72°C for 1 min, and a final extension step of 72°C for 10 min. Amplification of PCR products was confirmed by separation on 1% agarose gels and visualized by staining with GelRed under UV light. The PCR products were purified using an AxyPrepTM DNA Gel Extraction Kit (Axygen) according to the manufacturer’s instructions. The PCR products were stored at −80 ± 1°C prior to the synthesis of dsRNA.
dsRNA was synthesized using the MEGAscript® T7 Transcription Kit (Ambion, Austin, TX, United States), and 1 μg PCR product (plasmid) was used as the transcription template. It was analyzed by 1% agarose gel electrophoresis and quantified spectrophotometrically. The dsRNA was stored at −80 ± 1°C or used for subsequent experiments immediately.
Expression Analysis of Three Hsps After RNAi
Newly emerged adult female beetles (<12 h after eclosing) were collected for dsRNA injection utilizing a PLI-100 Pico-Injector (Harvard Apparatus, Holliston, MA, United States) with an MP-255 Micromanipulator (Sutter, Novato, CA, United States) under an Olympus stereomicroscope. The dsRNA solution (the final amount of dsRNA reached 1.0 μg) was injected into the penultimate 2–3 conjunctivum on the abdomen of A. hygrophila female adults. Each experiment was repeated at least three times. Each repeat included 100 female individuals. After injection, every 10 females and 10 freshly emerged adult males were put together in cylindrical boxes of 8 cm in diameter and 12 cm in height with fresh A. philoxeroides stems. These beetles were exposed to heat shock at 30, 36, or 39°C for 4 h in a constant temperature incubator (RPX-450, Colin, Beijing), and the climate chamber was maintained at 75 ± 5% RH with a 12:12 h light–dark photoperiod (Guo et al., 2011). Five females were collected and used for expression analysis of the three Hsp genes at each temperature every day for a week. The blank control was injected with dsRNAs of EGFP.
Effects of RNAi on Fecundity of A. hygrophila
To confirm the specificity of RNAi, dsRNA solution (the final amount of dsRNA reached 1.0 μg) for Hsp70, Hsp21, and sHsp21 was injected into insects in the penultimate abdomen of newly emerged adult female beetles. Each treatment included 15 female beetles. Based on the results of the preliminary experiment, we injected about 30 females for our experiment to ensure the survival of 15 females for later experimental observation after injection. One female and one freshly emerged adult male were put together on damp filter paper in a 9 cm diameter Petri dish with fresh A. philoxeroides stems and they were exposed to heat shock at 30, 36, or 39°C for 4 h in a constant temperature incubator (RPX-450, Colin, Beijing), and the climate chamber was maintained at 75 ± 5% RH with a 12:12 h light–dark photoperiod (Guo et al., 2011). Each pair of A. hygrophila adults was observed, and laid eggs were counted and collected every 24 h for 6 days. The eggs were continuously examined every day and hatching larvae were removed and counted after egg batches began to hatch until no eggs had hatched for one successive week. Each treatment contained 300 eggs, and three replicates were performed for each treatment.
Adult Survival of A. hygrophila After RNAi
Bowler and Terblanche (2008) observed that adults of different ages respond differently to temperature exposure; thus, newly emerged adult A. hygrophila females and males (<12 h after eclosing) were collected for dsRNA injection. At least 15 females and 15 males of each treatment were assayed. After injection, one female and one male were put together on damp filter paper in a 9 cm diameter Petri dish with fresh A. philoxeroides stems and heat shocked for 4 h at each of the three temperatures (30, 36, and 39°C) in a constant temperature incubator (RPX-450, Colin, Beijing) and the climate chamber was maintained at 75 ± 5% RH, with a 12:12 h light–dark photoperiod (Guo et al., 2011). The survival of female and male adults was recorded daily until death. The blank control was injected with dsEGFP.
Statistical Analysis
Statistical analyses were conducted using the SAS System for Windows V8. One-way analysis of variance (ANOVA; SAS Institute Inc., 1996, United States) was used to analyze the differences among treatments followed by a least significant difference (LSD) test for multiple comparisons. Experimental data were checked for normality and homoscedasticity, and if needed, were arcsine square-root or log-transformed before analysis. Differences among mean values were determined using a LSD test at P < 0.05.
Results
The full-length cDNAs of Hsp70, Hsp21, and sHsp21 in A. hygrophila were obtained by RT-PCR and RACE-PCR and submitted to NCBI (GenBank accession numbers: MN138034, MN163037, and MN163038, respectively). They contained 1940, 543, and 567 bp, with ORFs encoding 650, 180, and 188 amino acids, respectively (Supplementary Figures S1, S3, S5). Their MWs were 71.757, 20.879, and 21.510 kDa and the oretical isoelectric points were 5.63, 6.45, and 6.24, respectively.
Sequence Analysis of Hsp70
Referring to the DNAMAN multiple alignment, the sequence of Hsp70 in A. hygrophila was highly similar to the Hsp70 family amino acid sequence of the related insect species (Supplementary Figure S2).
Homology analysis revealed that the amino acid sequence derived from the Hsp70 gene of A. hygrophila is highly similar to that encoded by homologous genes of other insect species, among which the similarity to the potato beetle was the highest (Leptinotarsa decemlineata) (Figure 1). The amino acid sequences derived from Hsp70 were highly conserved among insects.
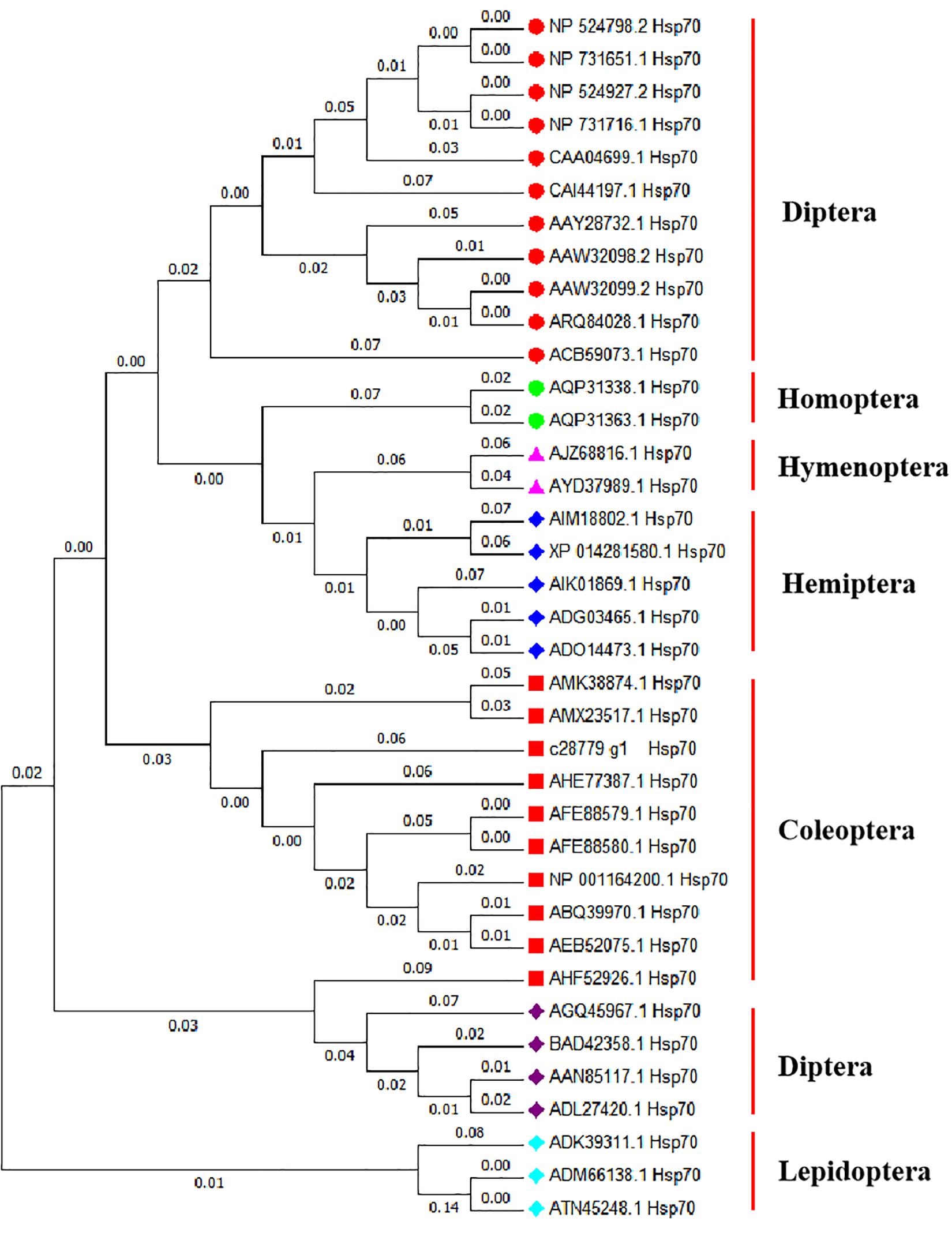
Figure 1. Evolutionary relationships of Hsp70 based on 37 amino acid sequences. The evolutionary history was inferred using the Neighbor-Joining method (Saitou and Nei, 1987; Zhang and Sun, 2008). The evolutionary distances were computed using the Poisson correction method (Zuckerkandl and Pauling, 1965) and are in the units of the number of amino acid substitutions per site. Evolutionary analyses were conducted in MEGA X (Kumar et al., 2018). Drosophila melanogaster hsp70Ab (NP 524798.2), Drosophila melanogaster hsp70Aa (NP 731651.1), Drosophila melanogaster hsp70Bb (NP 524927.2), Drosophila melanogaster hsp70Ba (NP 731716.1), Drosophila auraria hsp70 (CAA04699.1), Bactrocera oleae hsp70 (CAI44197.1), Delia antiqua hsp70 (AAY28732.1), Liriomyza huidobrensis hsp70 (AAW32098.2), Liriomyza sativae hsp70 (AAW32099.2), Liriomyza trifolii hsp70 (ARQ84028.1), Stratiomys singularior hsp70 (ACB59073.1), Laodelphax striatellus hsp70 (AQP31338.1), Nilaparvata lugens hsp70 (AQP31363.1), Cotesia chilonis hsp70 (AJZ68816.1), Trichogramma chilonis hsp70 (AYD37989.1), Bemisia tabaci hsp70 (ADG03465.1), Bemisia tabaci hsp70 (ADO14473.1), Orius sauteri hsp70 (AIK01869.1), Empoasca onukii hsp70 (AIM18802.1), Halyomorpha halys hsp70 (XP 014281580.1), Colaphellus bowringi hsp70 (AMK38874.1), Monochamus alternatus hsp70 (AMX23517.1), Lissorhoptrus oryzophilus hsp70 (AHE77387.1), Tenebrio molitor hsp70 (AFE88579.1), Tenebrio molitor hsp70 (AFE88580.1), Tribolium castaneum hsp70 (NP 001164200.1), Anatolica polita borealis hsp70 (ABQ39970.1), Microdera punctipennis hsp70 (AEB52075.1), Colaphellus bowringi hsp70 (AHF52926.1), Diamesa cinerella hsp70 (AGQ45967.1), Chironomus yoshimatsui hsp70 (BAD42358.1), Chironomus tentans hsp70 (AAN85117.1), Chironomus riparius hsp70 (ADL27420.1), Plutella xylostella hsp70 (ADK39311.1), Spodoptera litura hsp70 (ADM66138.1), Mythimna separata hsp70 (ATN45248.1).
Hsp70 sequences from Coleoptera were clustered in one branch, indicating that these Hsp70 sequences had relatively similar amino acid sequences. The Hsp70 sequence of A. hygrophila is most closely related to that of Leptinotarsa decemlineata (Figure 1).
Sequence Analysis of Hsp21
Referring to the DNAMAN multiple alignment, the sequence of Hsp21 in A. hygrophila was highly similar to the Hsp21 family amino acid sequence of the related insect species (Supplementary Figure S4). Homology and phylogenetic tree analysis revealed that the amino acid sequence derived from the Hsp21 gene of A. hygrophila is highly similar to that encoded by homologous genes of other insect species, among which the similarity to Lissorhoptrus oryzophilus was the highest (Figure 2).
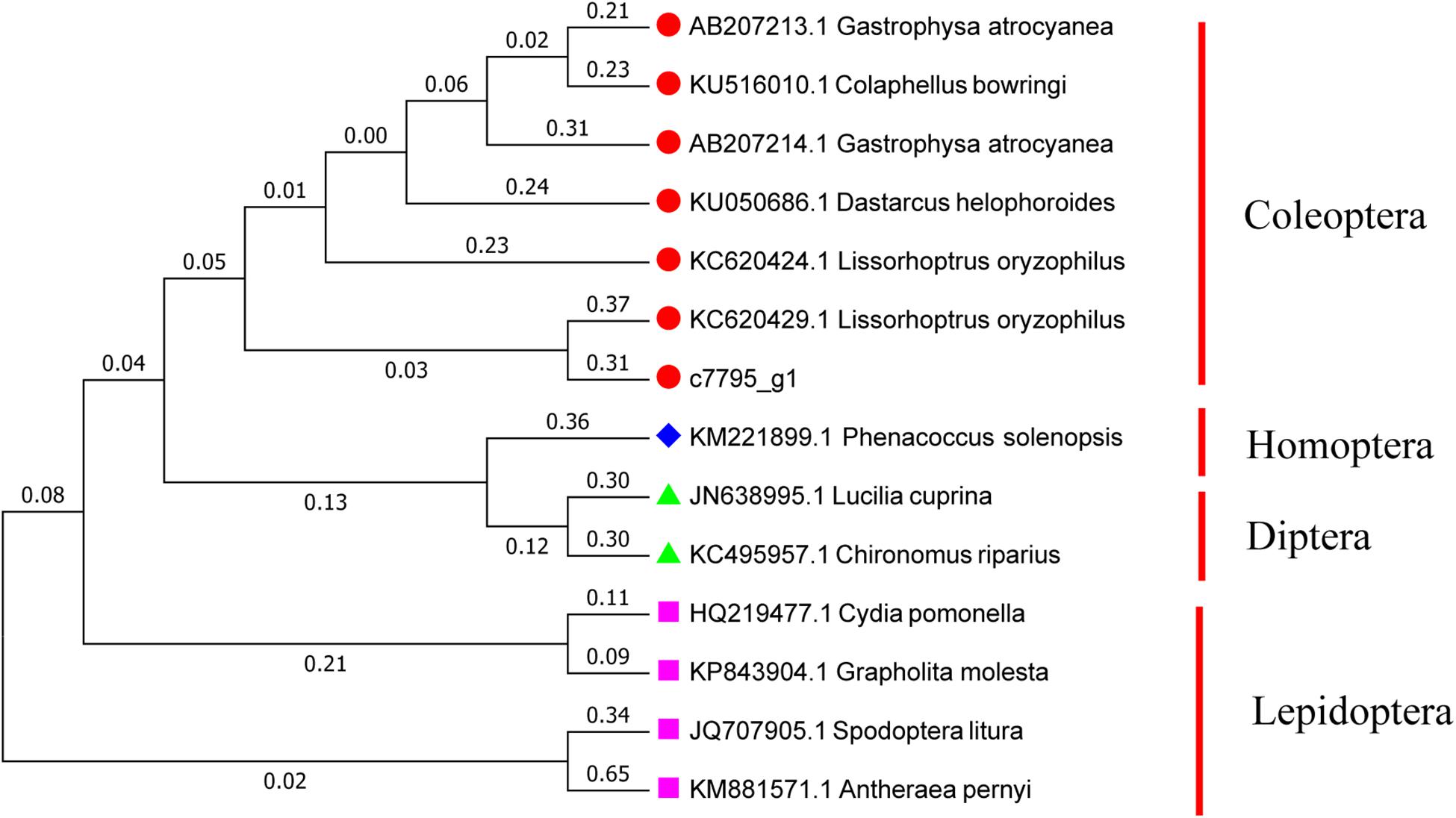
Figure 2. Gene system development of the Neighbor-Joining phylogenetic tree of Hsp21 in A. hygrophila. This tree is used to determine the relationships between different insects. The numbers above the branches indicate the percentages of bootstrap replicates in which each species is grouped together. The scale bar indicates the number of substitutions per site for each unit branch length. Colaphellus bowringi (AMK38872.1), Gastrophysa atrocyanea (BAD91164.1), Lissorhoptrus oryzophilus (AHE77375.1), Agasicles hygrophila (AHH25011.1), Dastarcus helophoroides (ANA11235.1), Harmonia axyridis (ART30112.1), Harmonia axyridis (ART30114.1), Gastrophysa atrocyanea (BAD91165.1), Lissorhoptrus oryzophilus (AHE77378.1), Lissorhoptrus oryzophilus (AHE77381.1), Galeruca daurica (ATY47553.1), Tribolium castaneum (XP_973344.1), Tribolium castaneum (XP_973378.1), Macrocentrus cingulum (ACF21815.1), Liriomyza huidobrensis (ABE57137.1), Musca domestica (AHK23446.1), Galleria mellonella (AXY94831.1), Spodoptera litura (ADK55523.1), Helicoverpa armigera (ATB54991.1), Bicyclus anynana (AFM73645.1), Eogystia hippophaecolus (AYA93247.1), Pteromalus puparum (ACO57620.1), Pteromalus puparum (AEM45800.1), Helicoverpa armigera (ATB54994.1), Bombyx mori (NP_001036985.1).
Sequence Analysis of sHsp21
Referring to the DNAMAN multiple alignment, the sequence of sHsp21 in A. hygrophila was highly similar to the sHsp21 family amino acid sequence of the related insect species (Supplementary Figure S6). Homology and phylogenetic tree analysis revealed that the amino acid sequence derived from the sHsp21 gene of A. hygrophila is highly similar to that encoded by homologous genes of other insect species, among which the similarity to Lissorhoptrus oryzophilus was the highest (Figure 3).
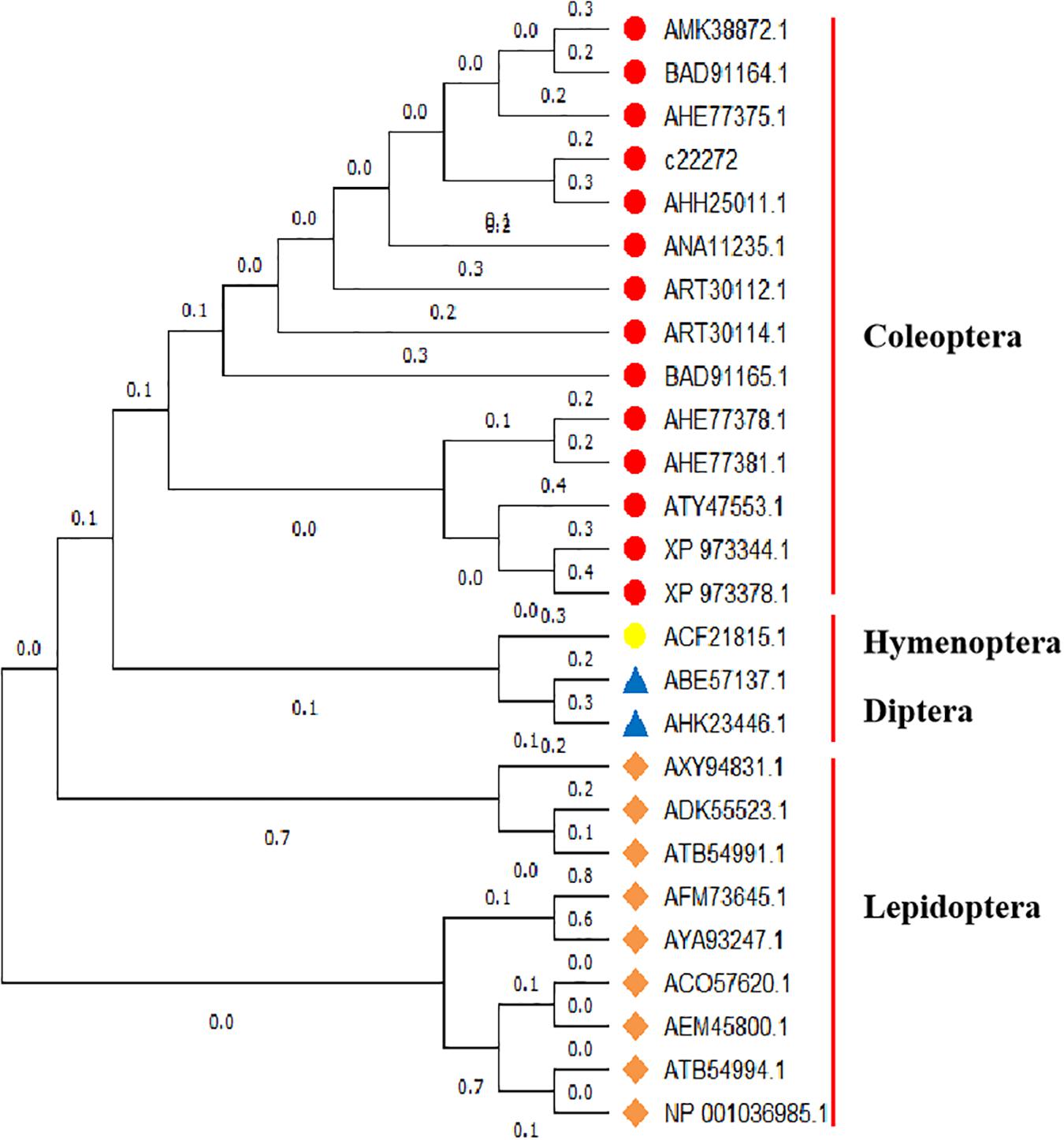
Figure 3. Gene system development of the Neighbor-Joining phylogenetic tree of sHsp21 in A. hygrophila. This analysis involved 28 amino acid sequences, and evolutionary analyses were conducted in MEGA X (Kumar et al., 2018). Lissorhoptrus oryzophilus (AHE77375.1), Colaphellus bowringi (AMK38872.1), Agasicles hygrophila (AHH25011.1), Harmonia axyridis (ART30114.1), Dastarcus helophoroides (ANA11235.1), Gastrophysa atrocyanea (BAD91164.1), Lissorhoptrus oryzophilus (AHE77378.1), Lissorhoptrus oryzophilus (AHE77381.1), Choristoneura fumiferana (ASQ43188.1), Galeruca daurica (ATY47553.1), Harmonia axyridis (ART30112.1), Tribolium castaneum (XP973344.1), Helicoverpa armigera (ATB54994.1), Chilo suppressalis (AGM90557.1), Chilo suppressalis (AGM90555.1), Liriomyza huidobrensis (ABE57137.1), Musca domestica (AHK23446.1), Pteromalus puparum (AEM45800.1), Gastrophysa atrocyanea (BAD91164.1), Fenneropenaeus chinensis (AEE81035.1), Spodoptera litura (ADK55523.1), Helicoverpa armigera (ATB54991.1), Galleria mellonella (AXY9483.1), Eogystia hippophaecolus (AYA93248.1), Eogystia hippophaecolus (AYA93247.1), Bicyclus anynana (AFM73645.1), Tribolium castaneum (XP973378.1), Lissorhoptrus oryzophilus (AHE77382.1), Gastrophysa atrocyanea (BAD91165.1).
Expression of Hsp70, Hsp21, and sHsp21 Under Thermal Stress
The expression levels of three Hsp mRNAs in A. hygrophila under standard (30°C) and high (36, 39°C) temperature treatments were determined by relative quantitative real time PCR. The expression of β-actin was used as an internal control. The results revealed that these three Hsps could be induced by 4 h treatment at high temperatures (Figure 4). Significant up-regulation of Hsps was observed when the temperature was further increased. At 39°C, the expression level of Hsp70 was significantly higher than that at 30°C (Figure 4A). The Hsp70 mRNA level was significantly higher at 36°C compared with that at 30°C (Figure 4A).
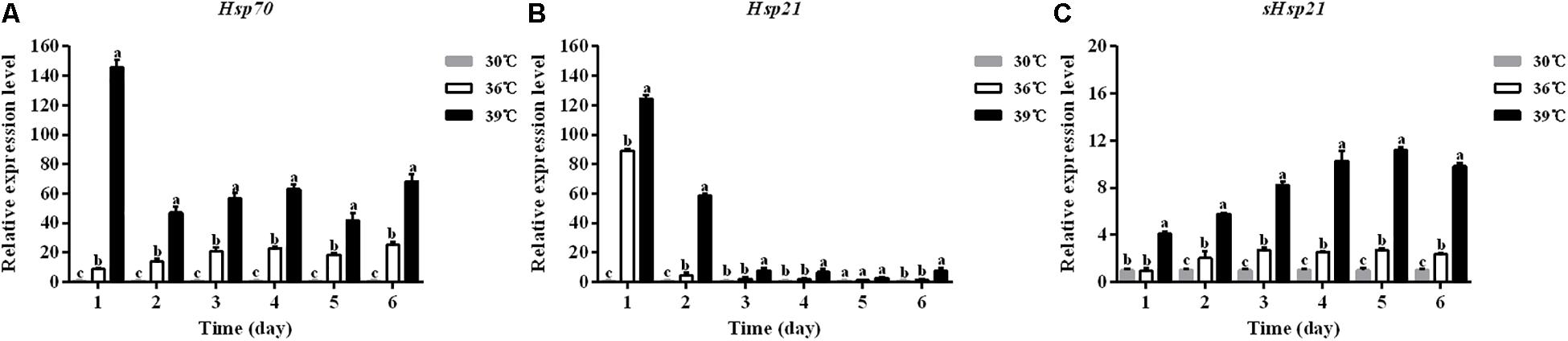
Figure 4. The mRNA expression profiles of three Hsp genes in A. hygrophila. The relative levels of Hsp mRNAs were examined at different temperatures (30, 36, and 39°C). Values are the means ± SD. The data were analyzed by one-way ANOVA followed by the least significant difference (LSD) test. Different letters indicate significant differences between means (P < 0.05). (A) Expression level of Hsp70, (B) the expression level of Hsp21, and (C) expression level of sHsp21.
The expression levels of Hsp21 in A. hygrophila were significantly higher at 39°C than at 30°C during the 6 days of measurement (Figure 4B). Two days after the daily 4 h heat shock treatment, the Hsp21 mRNA level was significantly higher at 36°C treatment compared with that at 30°C (Figure 4B). However, from days 3 to 6, there was no significant difference in the Hsp21 mRNA level between 36 and 30°C treatments (Figure 4B).
The expression levels of sHsp21 in A. hygrophila were significantly higher at 39°C than those at 30°C during the 6 days of measurement (Figure 4C). One day after the daily 4 h heat shock treatment, there was no significant difference in the sHsp21 mRNA level between 36 and 30°C treatments. However, from days 2 to 6, the sHsp21 mRNA level was significantly higher after 36°C treatment than that after 30°C treatment (Figure 4C).
Effects of dsRNA Injection on Expression of Three Hsps
Injection of dsHsp70, dsHsp21, and dssHsp21 into freshly emerged A. hygrophila adults could significantly inhibit the endogenous expression of Hsp mRNAs (Figure 5). From days 2 to 6 after the injection of dsHsp70, dsHsp21, and dssHsp21, the expression levels of these genes decreased significantly compared with the control group with the injection of dsEGFP (Figure 5). The expression levels of mRNAs were up-regulated significantly after heat shock at 36 and 39°C for 4 h every day, while the injection of dsRNAs suppressed the expression at the gene level (Figure 5).
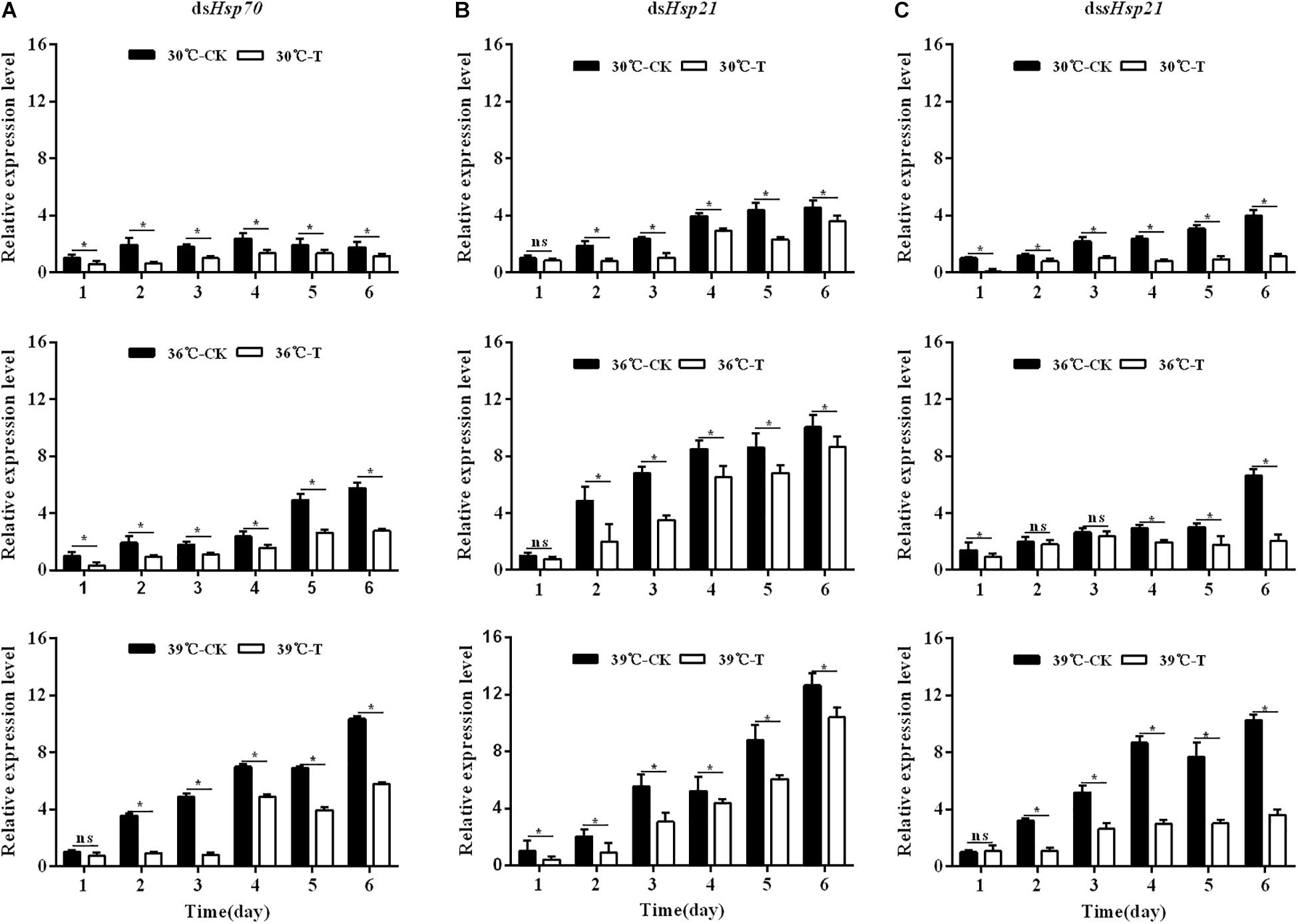
Figure 5. Relative expression levels of Hsp70, Hsp21, and sHsp21 after injection of dsRNA into freshly emerged A. hygrophila adults (A, Hsp70; B, Hsp21; C, sHsp21). Values are means ± SD. The data were analyzed by Student’s t-test. ∗P < 0.05. ns, not significant.
Effects of RNAi on A. hygrophila Fecundity
After the injection of dsHsp70, dsHsp21, or dssHsp21 into the freshly emerged A. hygrophila female abdomen, the fecundity of the female beetle was significantly lower than that of the control group with the injection of dsEGFP. The decrease was more dramatic with the increasing heat-shock treatment temperatures. At 30°C, there were no significant differences between the treatment and control groups. However, heat shock at 36 or 39°C led to a more significant decrease in the fecundity of A. hygrophila than treatment at 30°C (Figure 6). Figure 7 shows the differences between the three Hsp genes at different temperatures. At 30 and 39°C, the number of laid eggs for the dsshsp21 group was significantly lower than that of the dsHsp70 and dsHsp21 group. While the number of laid eggs for the dsHsp70 group was not significantly difference compared with the dsHsp21 group. At 36°C, there were no difference in laid eggs between three Hsp genes. Additionally, there were no difference in egg hating rate between three Hsp genes at the different temperatures (Figure 7). The silencing of these three Hsps not only significantly affected the reproduction of A. hygrophila but it also depressed the survival of A. hygrophila significantly (Figure 8).
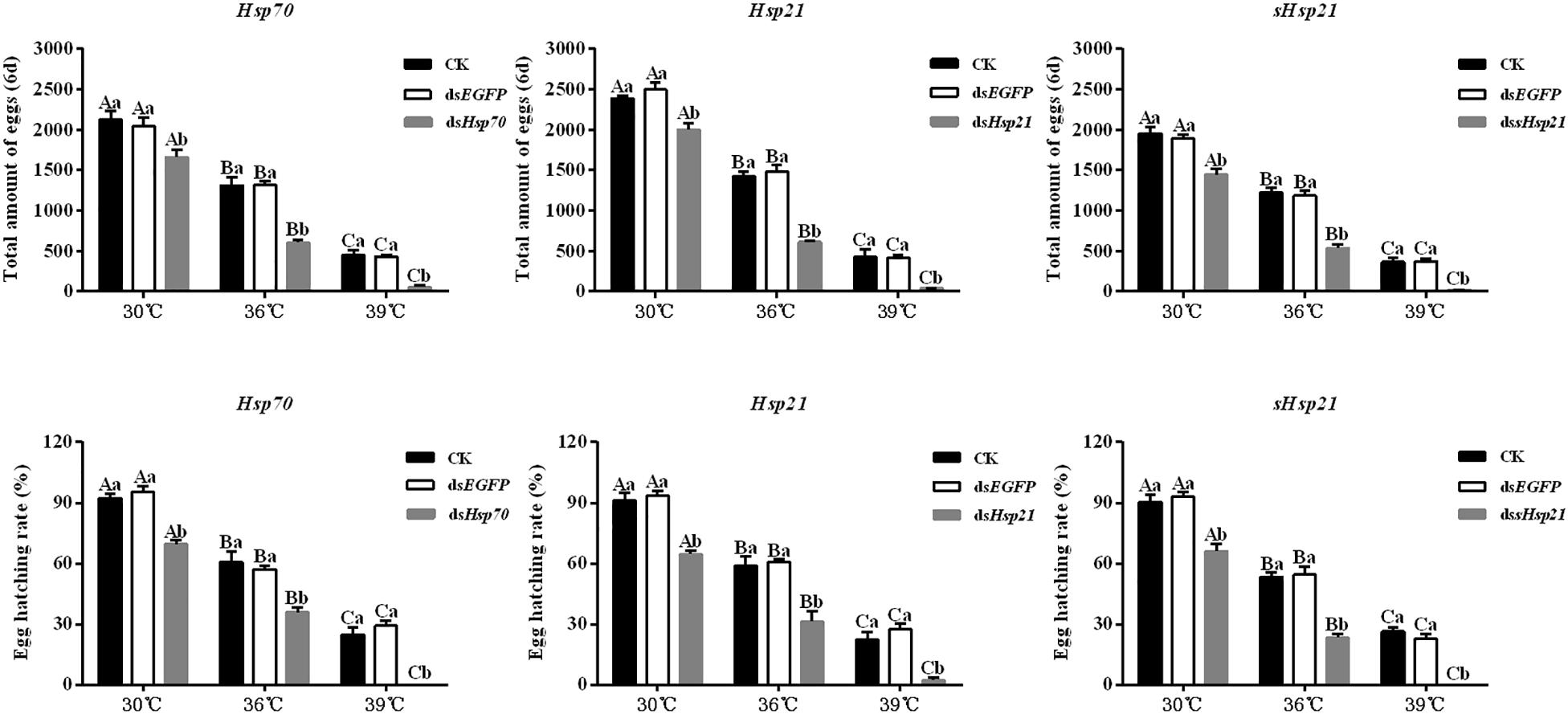
Figure 6. Fecundities and egg hatching rate of A. hygrophila after injection of dsHsp70, dsHsp21, and dssHsp21. Different amounts of dsEGFP were injected as the positive control. CK represents the no treatment as the negative control. Capital letters indicate the difference of the egg hatching rate and fecundity capacities of the A. hygrophila at the different temperatures. Lowercase letters refer to the differences in the egg hatching rate and fecundity capacities with different treatment of the same temperature. All values are shown as the mean ± SD. The data were analyzed by one-way ANOVA followed by the least significant difference (LSD) test. Different letters indicate significant differences between means (P < 0.05).
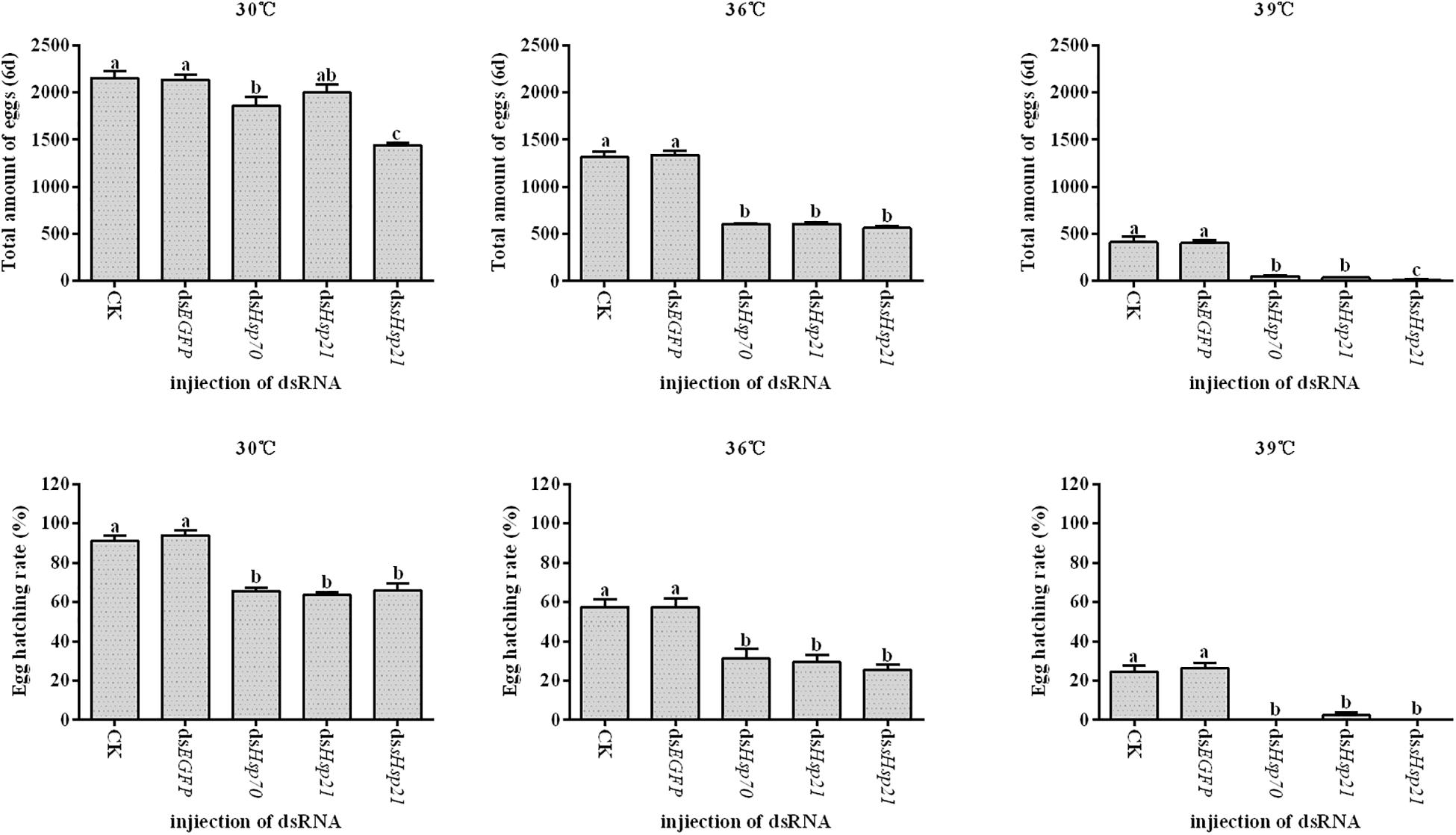
Figure 7. Fecundities and Egg hatch rate of A. hygrophila after injection of dsHsp70, dsHsp21, and dssHsp21. All values are shown as the mean ± SD. The data were analyzed by one-way ANOVA followed by the least significant difference (LSD) test. Different letters indicate significant differences between means (P < 0.05).
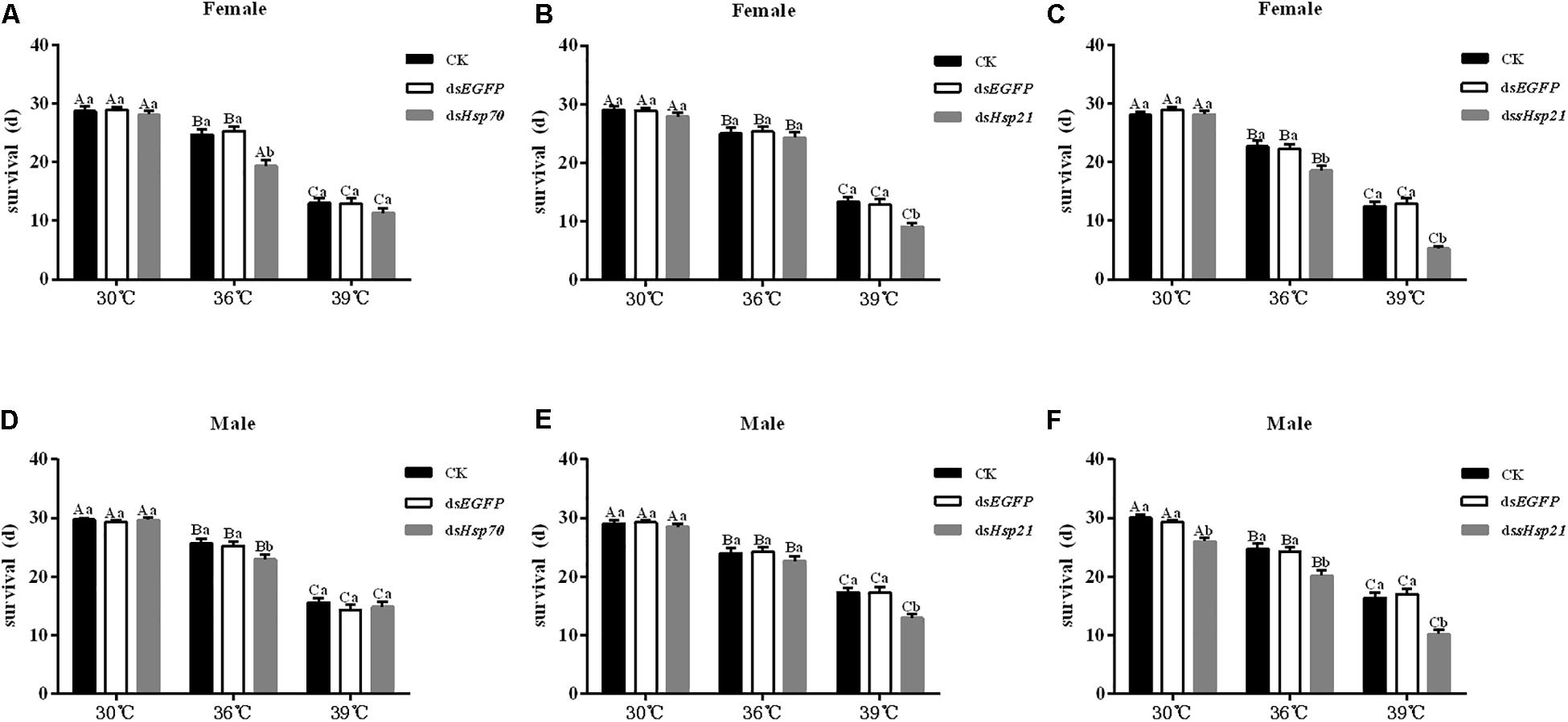
Figure 8. Adult survival results of A. hygrophila after injection of dsHsp70, dsHsp21, and dssHsp21. Different amounts of dsEGFP were injected the positive control. CK represents the no treatment as the negative control. Capital letters indicate the difference of the survival rates of the A. hygrophila at the different temperatures of 30, 36, and 39°C. All values are shown as the mean ± SE. The data were analyzed by one-way ANOVA followed by the least significant difference (LSD) test.
Discussion
Hsps are relatively conserved in evolution and play important roles in protecting living organisms from damage. Under physiological stress conditions, Hsps can maintain the normal structure of proteins and maintain the stability of the cytoskeleton (Tissières et al., 1974; Salvucci et al., 2000).
Studies have shown that Hsps, as important stress proteins, play a very important role in the stress resistance of organisms, and the Hsp gene may be one of the genes most closely related to temperature stress (Dahlgaard et al., 1998).
Previous studies on various Hsp genes have used cloning in insects, such as in Delia antiqua (Bin et al., 2006), Tribolium castaneum (Mahroof et al., 2005), Cotesia vestalis (Shi et al., 2013), and Microdera punctipennis (Ou et al., 2014). In this study, we cloned a full-length cDNA of 2053 bp encoding Hsp70 in A. hygrophila. The length of the ORF was 1940 bp, encoding 646 amino acids. The amino acid sequence of Hsp70 has a typical cytoplasmic characteristic motif EEVD (Demand et al., 1998; Vayssier et al., 1999). In addition, this sequence contains a GPTIEEVD sequence, which is included in most organisms, indicating that this regulatory sequence is highly conserved (Su et al., 2010). Moreover, based on the intermediate fragment, the 5′ RACE and 3′ RACE specific primers of Hsp21 and sHsp21 were designed, and the Hsp21 and sHsp21 sequences with lengths of 549 and 693 bp, respectively, were finally obtained. The ORFs of Hsp21 and sHsp21 were 538 and 567 bp, encoding 180 and 188 amino acids, respectively. Sequence analysis revealed that Hsp21 and sHsp21 were members of the small Hsp family including conserved domains such as the n-amino acid terminal domains, the -crystallin domains, and the c-amino acid terminal domains (Jong et al., 1998). Phylogenetic tree analysis showed that small MW Hsps of A. hygrophila and the small MW Hsps of other Coleoptera insects had high homology, so we speculate that they have a long independent evolutionary history.
In our current study, the construction of phylogenetic trees indicated that Hsp70, Hsp21, and sHsp21 genes are relatively conserved in evolution. Each tested Hsp gene in A. hygrophila could be clustered into one branch with other Coleoptera species of the same family, which could be well-distinguished from Hemiptera, Dictyoptera, Lepidoptera, Hymenoptera, and Diptera insects (Lü et al., 2014), indicating that Hsp70, Hsp21, or sHsp21 of Coleoptera insects have relatively similar amino acid sequences. At present, insect small Hsps are an infrequently studied part of the superfamily of Hsps, and the overall classification is not detailed enough. Specifically, the classification of sHsp21 of Coleoptera insects is limited. The research on small Hsps mainly focuses on a few model insects, such as Drosophila melanogaster (Tissières et al., 1974) and Bombyx mori (Li et al., 2009).
Our study showed that Hsp70, Hsp21, and sHsp21 genes in A. hygrophila showed similar expression patterns. The expression levels of these three Hsps increased with the increasing heat shock temperatures and were all higher than those in the control groups. These results are consistent with other previous studies showing significant up-regulation of Hsp gene expression levels when temperature was increased (Lü and Wan, 2011). It has been traditionally accepted that the expression levels of Hsps in the examined tissues changed obviously under high temperature treatments (Huang et al., 2007, 2009). However, in the study of Antheraea pernyi, the expression of sHsp21 was not obviously changed in fat bodies under high temperatures (Liu et al., 2013). Hsp genes are not only involved in thermal response but also may be related to reproduction in insects. For example, the expression of sHsp was also detected during male gametogenesis in Drosophilia melanogaster (Joanisse et al., 1998). Although the expression of Hsp70, Hsp21, and sHsp21 genes was detected in A. hygrophila after heat shock treatment in this study, determining whether these Hsp genes play a role in the reproduction of this beetle needs further study.
RNAi technology has been successfully applied to the studies of gene function in insects (Hannon, 2002; Ghanim et al., 2007). There are also successful examples in Coleoptera (Arakane et al., 2005; Shi et al., 2016). In our study, the target genes Hsp70, Hsp21, and sHsp21 were silenced with high efficiency in A. hygrophila, and the expression of these three Hsps was significantly down-regulated after RNA interference. Suppression of these three Hsps negatively affected fecundity and adult survival, causing significant declines in egg laying and egg hatch rates. Previous studies have demonstrated that heat shock protein genes affect insect reproduction and longevity. Xie et al. (2019) studied the functional of hsp18.3 gene in the Tribolium castaneum and found that after injection of dshsp18.3, one pair of the beetles can hardly spawn. When Tchsp18.3 was knocked down, the survival rate of adults and fecundity were significantly lower than the control group. Lapierre and Hansen (2012), Wiese et al. (2012), and Yanos et al. (2012) explored the lifespan of nematode Caenorhabditis elegans and found that the Hsp genes could be placed into longevity regulating pathway of C. elegans as well as improving the longevity of C. elegans. This phenomenon indicates that Hsp70, Hsp21, and sHsp21 play important roles both in the reproduction and in the thermo-tolerance of A. hygrophila. Additionally, previous studies also found that there was functional compensation between genes. Petko and Lindquist (1986) and Bossier et al. (1989) found yeast may possess (an)other, as yet unidentified, small Hsp(s), which could compensate for the lack of Hsp26 function. Zhang et al. (2019) found that there was strong functional compensation among these three AhVgs. In our study, whether these three Hsp genes have the functional compensation in regulating thermotolerance in A. hygrophila needs to be further investigated.
Previous studies of Hsps have shown that adult females have a greater tolerance than adult males to high temperature stress (Dahlgaard et al., 1998; Sørensen et al., 1999, 2005; Lansing et al., 2000; Andersen et al., 2006; Sarup et al., 2006; Lü and Wan, 2011). Drosophilia melanogaster (Dahlgaard et al., 1998; Lansing et al., 2000) females can tolerate high temperature stress better than males, and this is also the case for Drosophila buzzatii (Sørensen et al., 1999, 2005; Sarup et al., 2006) and Aedes aegypti (Andersen et al., 2006). In our study, whether adult females have a greater tolerance than adult males to high temperature stress needs to be further investigated. Knockdown of one of the three Hsp (Hsp70, Hsp21, and sHsp21) genes in A. hygrophila females reduced their reproduction significantly, and silencing of these Hsp genes also affected adult survival. Therefore, we speculated that Hsp70, Hsp21, and sHsp21 genes play important roles in the thermo-tolerance of A. hygrophila.
Conclusion
We obtained complete cDNA of three Hsps encoding the ORFs of A. hygrophila and analyzed the amino acid sequences and gene structures. The phylogenetic tree showed that Hsp70, Hsp21, and sHsp21 were relatively conservative in evolution, and their Hsps were highly homologous to those of Coleoptera insects. The expression of three Hsps genes was significantly up-regulated after heat shock. RNAi bioassays showed that three Hsps played important roles in A. hygrophila heat resistance and fecundity. The present results could improve our understanding of the mechanisms of thermotolerance in A. hygrophila at the molecular level.
Data Availability Statement
The datasets generated for this study can be found in the GenBank (accession numbers: MN138034 and MN163037–MN163038).
Author Contributions
JJ, JG, and ZZ conceived and designed the experiments. JJ and JG performed the experiments and wrote the manuscript. JJ, MZ, and ZZ analyzed the data. YW and FW provided the technical support.
Funding
This research was funded by the programs from the National Natural Science Foundation of China (Nos. 31272107 and 31572068) and the National Key Technology Research and Development Program of the Ministry of Science and Technology of China (No. 2015BAD201508B03).
Conflict of Interest
The authors declare that the research was conducted in the absence of any commercial or financial relationships that could be construed as a potential conflict of interest.
Acknowledgments
We would like to thank Editage (www.editage.cn) for English language editing.
Supplementary Material
The Supplementary Material for this article can be found online at: https://www.frontiersin.org/articles/10.3389/fphys.2019.01593/full#supplementary-material
Footnotes
References
Andersen, J. P., Schwartz, A., Gramsbergen, J. B., and Loeschcke, V. (2006). Dopamine levels in the mosquito Aedes aegypti during adult development, following blood feeding and in response to heat stress. J. Insect Physiol. 52, 1163–1170. doi: 10.1016/j.jinsphys.2006.08.004
Arakane, Y., Muthukrishnan, S., Kramer, K. J., Specht, C. A., Tomoyasu, Y., Lorenzen, M. D., et al. (2005). The Tribolium chitin synthase genes TcCHS1 and TcCHS2 are specialized for synthesis of epidermal cuticle and midgut peritrophic matrix. Insect Mol. Biol. 14, 453–463. doi: 10.1111/j.1365-2583.2005.00576.x
Aruda, A. M., Baumgartner, M. F., Reitzel, A. M., and Tarrant, A. M. (2011). Heat shock protein expression during stress and diapause in the marine copepod Calanus finmarchicus. J. Insect. Physiol. 57, 665–675. doi: 10.1016/j.jinsphys.2011.03.007
Bin, C., Takumi, K., Antónia, M., and Yukio, I. (2006). Cloning and characterization of the hsp70 gene, and its expression in response to diapauses and thermal stress in the onion maggot, Delia antiqua. J. Biochem. Mol. 39, 749–758. doi: 10.5483/BMBRep.2006.39.6.749
Bossier, P., Fitch, I. A., Boucherie, H., and Tuite, M. F. (1989). Structure and expression of a yeast gene encoding the small heat shock protein, Hsp26. Gene 78, 323–330. doi: 10.1016/0378-1119(89)90234-5
Bowler, K., and Terblanche, J. S. (2008). Insect thermal tolerance: what is the role of ontogeny, ageing and senescence? Biol. Rev. 83, 339–355. doi: 10.1111/j.1469-185X.2008.00046.x
Buckingham, G. R. (1996). Biological control of alligator weed, Alternanthera philoxeroides, the world’s first aquatic weed success story. Castanea 61, 232–243.
Dahlgaard, J., Loeschcke, V., Michalak, P., and Justesen, J. (1998). Induced thermotolerance and associated expression of the heat-shock protein hsp70 in adult Drosophila melanogaster. Funct. Ecol. 12, 786–793. doi: 10.1046/j.1365-2435.1998.00246.x
Demand, J., Lüders, J., and Hfeld, J. (1998). The carboxy-terminal domain of Hsc70 provides binding sites for a distinct set of chaperone cofactors. Mol. Cell. Biol. 18:2023. doi: 10.1128/mcb.18.4.2023
Fangue, N. A., Myriam, H., and Schulte, P. M. (2006). Intraspecific variation in thermal tolerance and heat shock protein gene expression in common killifish, Fundulus heteroclitus. J. Exp. Biol. 209, 2859–2872. doi: 10.1242/jeb.02260
Feder, M. E., and Hofmann, G. E. (1999). Heat-shock proteins, molecular chaperones, and the stress response: evolutionary and ecological physiology. Annu. Rev. Physiol. 61, 243–282. doi: 10.1146/annurev.physiol.61.1.243
Fleige, S., Walf, V., Huch, S., Prgomet, C., Sehm, J., and Pfaffl, M. W. (2006). Comparison of relative mRNA quantification models and the impact of RNA integrity in quantitative real-time RT-PCR. Biotechnol. Lett. 28, 1601–1613. doi: 10.1007/s10529-006-9127-2
Gangstad, O. E. (1976). Biological control operations on alligator weed. J. Aquat. Plant. Manage. 14, 50–53.
Ghanim, M., Kontsedalov, S., and Czosnek, H. (2007). Tissue-specific gene silencing by RNA interference in the whitefly Bemisia tabaci (Gennadius). Insect. Biochem. Mol. 37, 732–738. doi: 10.1016/j.ibmb.2007.04.006
Goto, S. G., and Kimura, M. T. (2004). Heat-shock-responsive genes are not involved in the adult diapause of Drosophila triauraria. Gene 326, 117–122. doi: 10.1016/j.gene.2003.10.017
Goto, S. G., Yoshida, K. M., and Kimura, M. T. (1998). Accumulation of hsp70 mRNA under environmental stresses in diapausing and nondiapausing adults of Drosophila triauraria. J. Iinsect. Physiol. 44, 1009–1015. doi: 10.1016/s0022-1910(97)00143-1
Guo, J. Y., Fu, J. W., Xian, X. Q., Ma, M. Y., and Wan, F. H. (2011). Performance of Agasicles hygrophila (Coleoptera: Chrysomelidae), a biological control agent of invasive alligator weed, at low non-freezing temperatures. Biol. Invasions. 14, 1597–1608. doi: 10.1007/s10530-010-9932-3
Hoffmann, A. A., Sørensen, J. G., and Loeschcke, V. (2003). Adaptation of Drosophila to temperature extremes: bringing together quantitative and molecular approaches. J. Therm. Biol. 28, 175–216. doi: 10.1016/s0306-4565(02)00057-8
Huang, L. H., Chen, B., and Kang, L. (2007). Impact of mild temperature hardening on thermotolerance, fecundity, and Hsp gene expression in Liriomyza huidobrensis. J. Insect Physiol. 53, 1199–1205. doi: 10.1016/j.jinsphys.2007.06.011
Huang, L. H., Wang, C. Z., and Kang, L. (2009). Cloning and expression of five heat shock protein genes in relation to cold hardening and development in the leafminer, Liriomyza sativa. J. Insect Physiol. 55, 279–285. doi: 10.1016/j.jinsphys.2008.12.004
Joanisse, D. R., Michaud, S., Inaguma, Y., and Tanguay, R. M. (1998). Small heat shock proteins of Drosophila: developmental expression and functions. J. Biosci. 23, 369–376. doi: 10.1007/bf02936130
Jong, W. W. D., Caspers, G. J., and Leunissen, J. A. M. (1998). Genealogy of the α -crystallin—small heat-shock protein superfamily. Int. J. Biol. Macromol. 22, 151–162. doi: 10.1016/S0141-8130(98)00013-0
Julien, M. H., Skarratt, B., and Maywald, G. F. (1995). Potential geographical distribution of alligator weed and its biological control by Agasicles hygrophila. J. Aquat. Plant. Manage. 33, 55–60.
Kumar, S., Stecher, G., Li, M., Knyaz, C., and Tamura, K. (2018). MEGA X: molecular evolutionary genetics analysis across computing platforms. Mol. Biol. Evol. 35, 1547–1549. doi: 10.1093/molbev/msy096
Lansing, E., Justesen, J., and Loeschcke, V. (2000). Variation in the expression of Hsp70, the major heat-shock protein, and thermotolerance in larval and adult selection lines of Drosophila melanogaster. J. Therm. Biol. 25, 443–450. doi: 10.1016/S0306-4565(00)00008-5
Lapierre, L. R., and Hansen, M. (2012). Lessons from C. elegans: signaling pathways for longevity. Trends Endocrinol. Metab. 23, 637–644. doi: 10.1016/j.tem.2012.07.007
Li, Y., Guo, J. Y., Zhu, X., Huang, S., and Wan, F. H. (2011). Effects of release density on the population dynamics of the biocontrol agent, Agasicles hygrophila (Coleoptera: Chrysomelidae). J. Biosaf. 20, 275–280.
Li, Z. W., Li, X., Yu, Q. Y., Xiang, Z. H., Kishino, H., and Zhang, Z. (2009). The small heat shock protein (sHSP) genes in the silkworm, Bombyx mori, and comparative analysis with other insect sHSP genes. BMC Evol. Biol. 9:215. doi: 10.1186/1471-2148-9-215
Liu, Q. N., Zhu, B. J., Dai, L. S., Fu, W. W., Lin, K. Z., and Liu, C. L. (2013). Overexpression of small heat shock protein 21 protects the Chinese oak silkworm Antheraea pernyi against thermal stress. J. Insect Physiol. 59, 848–854. doi: 10.1016/j.jinsphys.2013.06.001
Lü, Z. C., and Wan, F. H. (2011). Using double-stranded RNA to explore the role of heat shock protein genes in heat tolerance in Bemisia tabaci (Gennadius). J. Exp. Biol. 214, 764–769. doi: 10.1242/jeb.047415
Lü, Z. C., Wang, L. H., Zhang, G. F., Wan, F. H., Guo, J. Y., Yu, H., et al. (2014). Three heat shock protein genes from Bactrocera (Tetradacus) minax Enderlein: gene cloning, characterization, and association with diapause. Neotrop. Entomol. 43, 362–372. doi: 10.1007/s13744-014-0216-y
Ma, R. Y., and Wang, R. (2005). Invasive mechanism and biological control of alligator weed, Alternanthera philoxeroides (Amaranthaceae), in China. Chin. J. Appl. Environ. Biol. 11, 246–250. doi: 10.3321/j.issn:1006-687X.2005.02.028
Ma, R. Y., Wang, R., and Ding, J. Q. (2003). Classical biological control of exotic weeds. Acta Ecol. Sin. 12, 2677–2688.
Ma, R. Y., Zhang, P., Fan, R., Zhang, J., Lu, J., and Zhao, L. (2010). Performance of the biological control agent flea beetle Agasicles hygrophila (Coleoptera: Chrysomelidae), on two plant species Alternanthera philoxeroides (alligator weed) and A-sessilis (joyweed). Biol. Control 54, 9–13. doi: 10.1016/j.biocontrol.2010.02.012
MacRae, T. H. (2010). Gene expression, metabolic regulation and stress tolerance during diapause. Cell. Mol. Life Sci. 67, 2405–2424. doi: 10.1007/s00018-010-0311-0
Mahroof, R., Yan, Z. K., Neven, L., Subramanyam, B., and Bai, J. (2005). Expression patterns of three heat shock protein 70 genes among developmental stages of the red flour beetle, Tribolium castaneum (Coleoptera: Tenebrionidae). Comp. Biochem. Phys. A 141, 247–256. doi: 10.1016/j.cbpb.2005.05.044
National Invasive Species Council (2001). Meeting the Invasive Species Challenge: Management Plan. Washington, D.C: National Invasive Species Council.
Ou, J., Deng, H. M., Zheng, S. C., Huang, L. H., Feng, Q. L., and Liu, L. (2014). Transcriptomic analysis of developmental features of Bombyx mori wing disc during metamorphosis. BMC Genomics 15:820. doi: 10.1186/1471-2164-15-820
Petko, L., and Lindquist, S. (1986). Hsp26 is not required for growth at high temperatures, nor for thermotolerance, spore development, or germination. Cell 45, 885–894. doi: 10.1016/0092-8674(86)90563-5
Rinehart, J. P., Aiqing, L., Yocum, G. D., Robich, R. M., Hayward, S. A. L., and Denlinger, D. L. (2007). Up-regulation of heat shock proteins is essential for cold survival during insect diapause. Proc. Natl. Acad. Sci. U.S.A. 104, 11130–11137. doi: 10.1073/pnas.0703538104
Rinehart, J. P., Yocum, G. D., and Denlinger, D. L. (2000). Developmental upregulation of inducible hsp70 transcripts, but not the cognate form, during pupal diapause in the flesh fly, Sarcophaga crassipalpis. Insect. Biochem. Mol. 30, 515–521. doi: 10.1016/s0965-1748(00)00021-7
Saitou, N., and Nei, M. (1987). The neighbor-joining method: a new method for reconstructing phylogenetic trees. Mol. Biol. Evol. 4, 406–425. doi: 10.1093/oxfordjournals.molbev.a040454
Salvucci, M. E., Stecher, D. S., and Henneberry, T. J. (2000). Heat shock proteins in whiteflies, an insect that accumulates sorbitol in response to heat stress. J. Therm. Biol. 25, 363–371. doi: 10.1016/s0306-4565(99)00108-4
Sarup, P., Sorensen, J. G., Dimitrov, K., Barker, J. S., and Loeschcke, V. (2006). Climatic adaptation of Drosophila buzzatii populations in southeast Australia. Heredity 96, 479–486. doi: 10.1038/sj.hdy.6800828
Shi, J. F., Mu, L. L., Chen, X., Guo, W. C., and Li, G. Q. (2016). RNA interference of chitin synthase genes inhibits chitin biosynthesis and affects larval performance in Leptinotarsa decemlineata (Say). Int. J. Biol. Sci. 12, 1319–1331. doi: 10.7150/ijbs.14464
Shi, M., Wang, Y.-N., Zhu, N., and Chen, X.-X. (2013). Four heat shock protein genes of the Endoparasitoid Wasp, Cotesia vestalis, and their transcriptional profiles in relation to developmental stages and temperature. PLoS One 8:e59721. doi: 10.1371/journal.pone.0059721
Sørensen, J. G., Michalak, P., Justesen, J., and Loeschcke, V. (1999). Expression of the heat-shock protein Hsp70 in Drosophila buzzatii lines selected for thermal resistance. Hereditas 131, 155–164. doi: 10.1111/j.1601-5223.1999.00155.x
Sørensen, J. G., Norry, F. M., Scannapieco, A. C., and Loeschcke, V. (2005). Altitudinal variation for stress resistance traits and thermal adaptation in adult Drosophila buzzatii from the New World. J. Evol. Biol. 18, 829–837. doi: 10.1111/j.1420-9101.2004.00876.x
Spencer, N. R., and Coulson, J. R. (1976). The biological control of alligatorweed, Alternanthera philoxeroides, in the United States of America. Aquat. Bot. 2, 177–190. doi: 10.1016/0304-3770(76)90019-X
State Environmental Protection Administration of China, and Chinese Academy of Sciences (2003). The Announcement About the First List if Invasive Species in China. Beijing: Chinese Academy of Sciences.
Su, X. R., Du, L. L., Li, Y., Li, Y. R., Zhou, J., and Li, T. W. (2010). Cloning and expression of hsp70 gene of sipuncula Phascolosoma esculenta. Fish Shellfish Immunol. 28, 461–466. doi: 10.1016/j.fsi.2009.12.014
Tamura, K., Peterson, D., Peterson, N., Stecher, G., Nei, M., and Kumar, S. (2011). MEGA5: molecular evolutionary genetics analysis using maximum likelihood, evolutionary distance, and maximum parsimony methods. Mol. Biol. Evol. 28, 2731–2739. doi: 10.1093/molbev/msr121
Tissières, A., Mitchell, H. K., and Tracy, U. M. (1974). Protein synthesis in salivary glands of Drosophila melanogaster: relation to chromosome puffs. J. Mol. Biol. 84, 389–398. doi: 10.1016/0022-2836(74)90447-1
Vayssier, M., Le, G. F., Fabien, J. F., Philippe, H., Vallet, C., Ortega, P. G., et al. (1999). Cloning and analysis of a Trichinella britovi gene encoding a cytoplasmic heat shock protein of 72 kDa. Parasitology 119, 81–93. doi: 10.1017/s0031182099004461
Vogt, G. B., Quimby, P. C., and Kay, S. H. (1992). Effects of weather on the biological control of alligator weed in the lower Mississippi valley region, 1973-83. J. Radioanal. Nucl. Chem. 192, 265–274. doi: 10.1007/BF02041730
Wang, B. R., Li, W. G., and Wang, J. B. (2005). Genetic diversity of Alternanthera philoxeroides in China. Aquat. Bot. 81, 277–283. doi: 10.1016/j.aquabot.2005.01.004
Wang, R. (1989). “Biological control of weeds in China: a status report,” in Proceeding of the Seventh International Symposium on Biological Control of Weeds (Melbourne: CSIRO), 689–693.
Westwood, J. T., Clos, J., and Wu, C. (1991). Stress-induced oligomerization and chromosomal relocalization of heat-shock factor. Nature 353, 822–827. doi: 10.1038/353822a0
Wiese, M., Antebi, A., and Zheng, H. (2012). Regulation of neuronal APL-1 expression by cholesterol starvation. PLoS One 7:e32038. doi: 10.1371/journal.pone.0032038
Xie, J., Hu, X. X., Zhai, M. F., Yu, X. J., Song, X. W., Gao, S. S., et al. (2019). Characterization and functional analysis of hsp18.3 gene in the red flour beetle, Tribolium castaneum. Insect Sci. 26, 263–273. doi: 10.1111/1744-7917.12543
Yanos, M. E., Bennett, C. F., and Kaeberlein, M. (2012). Genome-wide RNAi longevity screens in Caenorhabditis elegans. Curr. Genomics 13, 508–518. doi: 10.2174/138920212803251391
Yu, H., Wan, F. H., and Guo, J. Y. (2012). cDNA cloning of heat shock protein genes and their expression in an indigenous cryptic species of the whitefly Bemisia tabaci complex from China. J. Agric. Sci. 11, 293–302. doi: 10.1016/s2095-3119(12)60013-6
Zeiger, C. F. (1967). Biological control of alligatorweed with Agasicles n. sp. in Florida. Hyacinth Control J. 6, 31–34.
Zhang, H., Wang, Y., Liu, Y. R., Zhao, M., Jin, J., Zhou, Z., et al. (2019). Identification and expression patterns of three vitellogenin genes and their roles in reproduction of the alligatorweed flea beetle Agasicles hygrophila (Coleoptera: Chrysomelidae). Front. Physiol. 10:368. doi: 10.3389/fphys.2019.00368
Zhang, W., and Sun, Z. (2008). Random local neighbor joining: a new method for reconstructing phylogenetic trees. Mol. Phylogenet. Evol. 47, 117–128. doi: 10.1016/j.ympev.2008.01.019
Zhao, M. T. (2017). Ecological performance and transcriptome analyses of the two geographic population of Agasicles hygrophila in response to heat stresses. CAAS 2, 13–25.
Keywords: Agasicles hygrophila, Alternanthera philoxeroides, heat shock protein (Hsp), RT-qPCR, RNAi, fecundity, survival
Citation: Jin J, Zhao M, Wang Y, Zhou Z, Wan F and Guo J (2020) Induced Thermotolerance and Expression of Three Key Hsp Genes (Hsp70, Hsp21, and sHsp21) and Their Roles in the High Temperature Tolerance of Agasicles hygrophila. Front. Physiol. 10:1593. doi: 10.3389/fphys.2019.01593
Received: 16 August 2019; Accepted: 19 December 2019;
Published: 14 January 2020.
Edited by:
Peng He, Guizhou University, ChinaReviewed by:
Guan-Heng Zhu, University of Kentucky, United StatesQi Fang, Zhejiang University, China
Copyright © 2020 Jin, Zhao, Wang, Zhou, Wan and Guo. This is an open-access article distributed under the terms of the Creative Commons Attribution License (CC BY). The use, distribution or reproduction in other forums is permitted, provided the original author(s) and the copyright owner(s) are credited and that the original publication in this journal is cited, in accordance with accepted academic practice. No use, distribution or reproduction is permitted which does not comply with these terms.
*Correspondence: Jianying Guo, guojianying@caas.cn